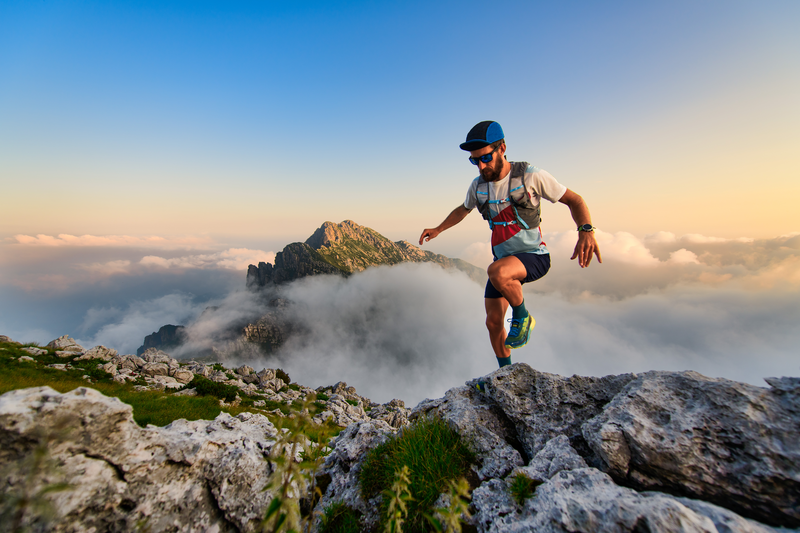
94% of researchers rate our articles as excellent or good
Learn more about the work of our research integrity team to safeguard the quality of each article we publish.
Find out more
REVIEW article
Front. Plant Sci. , 28 March 2023
Sec. Aquatic Photosynthetic Organisms
Volume 14 - 2023 | https://doi.org/10.3389/fpls.2023.1144326
This article is part of the Research Topic Algal Metabolism View all 8 articles
Natural antioxidants are more attractive than synthetic chemical oxidants because of their non-toxic and non-harmful properties. Microalgal bioactive components such as carotenoids, polysaccharides, and phenolic compounds are gaining popularity as very effective and long-lasting natural antioxidants. Few articles currently exist that analyze microalgae from a bibliometric and visualization point of view. This study used a bibliometric method based on the Web of Science Core Collection database to analyze antioxidant research on bioactive compounds in microalgae from 1996 to 2022. According to cluster analysis, the most studied areas are the effectiveness, the antioxidant mechanism, and use of bioactive substances in microalgae, such as carotene, astaxanthin, and tocopherols, in the fields of food, cosmetics, and medicine. Using keyword co-occurrence and keyword mutation analysis, future trends are predicted to improve extraction rates and stability by altering the environment of microalgae cultures or mixing extracts with chemicals such as nanoparticles for commercial and industrial applications. These findings can help researchers identify trends and resources to build impactful investigations and expand scientific frontiers.
Antioxidants are biological macromolecules that shield organisms or physical products from oxidative radicals (de Souza et al., 2019). The significance of antioxidants in reducing oxidative processes and the harmful effects of reactive oxygen species (ROS) is crucial in both food systems and the human body (Cakmakci et al., 2015). Since the 1970s, tert-butyl hydroquinone (TBHQ), butylated hydroxytoluene (BHT), EDTA, and other compounds have become some of the most widely utilized chemical antioxidants in the food business (Ngo et al., 2012), but this could cause liver damage and even cancer (Thannickal and Fanburg, 2000; Waris and Ahsan, 2006). Nowadays, the pursuit of health has made it a global trend to look for natural antioxidants that can replace chemical antioxidants and are non-toxic, noncarcinogenic, and biodegradable. In this context, microalgae present a promising alternative to meet the rising demand for sources of natural antioxidants. According to the worldwide algae market analysis report 2019–2025, the market for algae is predicted to increase by US$414.8 Thousand, with a compound growth rate of 6.6% (Uma et al., 2022).
Microalgae play a fundamental role in ecosystems (Guedes et al., 2011). Microalgal biomass and intracellular compounds have been studied and used as renewable natural resources for a wide spectrum of applications. However, microalgal biomass is typically utilized to produce biofuels or create powders and pills to strengthen immunity and prevent disease (Chacon-Lee and Gonzalez-Marino, 2010), making it challenging to include them directly in bulk meals (Kova et al., 2013). This is due to their color and odor. Additionally, studies on microalgal biomass have only included well-known species like Spirulina, Chlorella and Dunaliella (Gouveia et al., 2008). When exposed to light, carbon dioxide, and other external stressors, the abundance and variety of bioproducts they can produce, including high-quality proteins, long-chain polyunsaturated fatty acids, carotenoids, vitamins, minerals, and phenolics (Plaza et al., 2009). Microalgae-derived bioactive compounds have been investigated for their varied activities. Chronakis (Chronakis, 2001) researched Spirulina obturation proteins in 2001 and determined that microalgae proteins have superior gelling capabilities. Ursu et al. (Ursu et al., 2014) studied Chlorella proteins’ ability to emulsify in 2014. According to several studies, lutein and zeaxanthin are the significant pigments that cause the human macula to turn yellow and maintain proper visual function (Le and Xiao-Ming, 2010). In addition, various microalgal bioactive substances have specific antioxidant properties and may be a reliable source of natural antioxidants (Li et al., 2007). Such bioactive molecules serve as important foundations for biotechnological applications, particularly in the pharmaceutical, nutraceutical, and cosmetics sectors (Rani et al., 2021). For instance, Ayna (Ayna, 2020) researched the in vitro anticancer effects of beta-tocopherol, alpha-carotene, and ascorbic acid on PC-3 prostate cancer cells. These compounds play the role of oxidants in prostate cancer by reducing cell viability and increasing ROS production and lipid peroxidation (LPO). Carotenoids can be used as a natural antioxidant in anti-aging skin care products in the cosmetics industry, in addition to being incorporated as a natural pigment to color cosmetics (Foo et al., 2021).
The field of study on microalgal antioxidants is flourishing, and each year there are more research articles in this area. However, it is a challenge for researchers to locate research gaps and hotspots among the numerous relevant articles. Bibliometrics, which Alan Pritchard established in 1969 and uses mathematical and statistical methods to quantitatively analyze all sorts of knowledge vectors, is considered as a potent instrument to handle this challenge with the emergence of current information technology and statistics (Merigo et al., 2016). The most widely used bibliometric tools in use today are VOSviewer, Gephi, CiteSpace, and Bibexcel. Numerous publications about microalgae and bioproducts antioxidants have recently been published, including the following: management of oxidative stress by microalgae (Cirulis et al., 2013); microalgae under environmental stress as a source of antioxidants (Gauthier et al., 2020); astaxanthin in skin health, repair, and disease (Davinelli et al., 2018); microalgae integrated into innovative food with potential health benefits (Caporgno and Mathys, 2018). There aren’t many bibliometric studies on microalgae, nevertheless, and there haven’ t been any studies that illustrate the antioxidant qualities of the bioactive components of microalgae. Through the use of other visual analysis tools like CiteSpace and VOSviewer, this paper offers a quantitative and visual analysis of the literature about antioxidant research on highly valuable microalgal metabolites, as well as a detailed discussion of a number of hotspots and significant research topics to comprehend the knowledge structure, research hotspots, trends, and research frontiers (Wang et al., 2022).
Reliable sources must be retrieved in order to guarantee the accuracy of the knowledge mapping (Xia and Duan, 2022). The Web of Science (WOS) database was used as the data source in this article. The WOS database search selected “Web of Science Core Collection” and input in the search formula: “(TS=(Antioxida*) OR TS=(Anti-oxida*)) AND TS=(Microalgae)”. Any terms with the same root as the word are guaranteed to appear in the search result when a word is finished with an asterisk, thus, this strategy searched for papers that contain the word antioxidant/antioxidant and its derivatives in their title, abstract, or keywords. According to our search formula, we found that Hirayama, S. et al. (Hirayama et al., 1996) released the first publication on microalgae antioxidant activities study in 1996, which can be retrieved from WoSCC. Consequently, the search data’ s time frame was set from January 1996 to November 2022 (the search date was 24 November 2022). The final result was the extraction of 2093 publication records, each of which had the following information: author, title, source document, abstract, and cited references.
All visual analyses in this study were performed using VOSviewer 1.6.18.0, CiteSpace 6.1.R3, and Gephi, respectively. VOSviewer is a free JAVA-based software developed in 2009 by VanEck and Waltman from the Centre for Science and Technology Studies (CWTS) at Leiden University in the Netherlands (van Eck and Waltman, 2010), and this paper primarily uses VOSviewer for keyword co-occurrence and density analysis. CiteSpace is a multifaceted, dynamic visual analysis software developed by Chen CM (Chen, 2004) in 2004, focusing on the analysis of the underlying knowledge contained in the scientific literature (Sun et al., 2019). CiteSpace is used in this work for subject, country/region, institution, co-author, highly cited literature, keyword clustering, and burstness analysis. The analysis of hotspots in this research area is made possible by the keyword co-occurrence and clustering methods. This analysis clarifies how the research on antioxidants in bioactive substances in microalgae has developed, gives a proper understanding of the research spectrum, and serves as a guide for the field’s future development.
CiteSpace is used to analyze co-occurrence or collaborative networks from 1996 to 2022, with a one-year time slice. The selection criteria were each article’s top 50 cited or widely used sections (Tao et al., 2022). CiteSpace employs the Cosine algorithm to determine the strength of the relationships between keywords during the clustering process and the log-likelihood ratio (LLR) method to name each cluster. The cosine algorithm (Equation 1) groups keywords based on similarity. And the latter (Equation 2) proposes nomenclature to identify hotspots for current research in a given field.
In Equation 1, i and j represent two subject terms, respectively, cij is the number of co-occurrences of i and j; fi denotes the number of occurrences of i; fj denotes the number of occurrences of j.
LLR is the log-likelihood ratio of wi for category Cj; p(Ci/Vij and are the density functions in categories Cj and , respectively.
The findings of this research are detailed in four sections that make up this section. Regarding annual publication volume, appropriate fields, countries, and institutions, Sections 3.1 and 3.2 give an overview of the current research and collaboration on antioxidants in microalgae and their byproducts. The highly cited papers are listed in Section 3.3, which also conducts a cluster analysis using the highly cited articles’ keywords. The research frontiers are analyzed using keywords in section 3.4.
The number of published papers is an essential indication of a better understanding of each stage of research development (Wang et al., 2022). The quantity of published literature that CiteSpace has counted is represented by a line graph. According to statistics of disclosure, investigations of antioxidant enzymes and substances found in microalgae began as early as 1996. The article is titled Evaluation of Active Oxygen Effect on Chlorella Vulgaris Photosynthesis by Hirayama, S in Free Radical Research. Examining the connection between O2 and an active oxygen scavenging mechanism in Chlorella Vulgaris is the primary objective of this study (Hirayama et al., 1996). People’s interest in this study has increased from 1996 to date, and the growth rate of cumulative publications grew steadily over time (Figure 1), following the equation y = 2.58645*exp(x/5.15) - 6.5987, where the year 0 is defined as 1996. The relevant research progress can be divided into three phases based on the general development pattern. The first phase, which lasted from 1996 to 2005, saw a relatively modest number of articles published annually that stabilized at around 10, with scientific activity still in its infancy. A total of 41 papers were issued during this 10-year period, representing 1.96% of the total. The number of papers published between 2006 and 2014 exhibited an upward trend, with a faster growth rate than between 1996 and 2005. A total of 292 papers were posted throughout nine years, accounting for 13.95% of the total papers, with 32 papers getting published on average per year. The number of antioxidation of high-value metabolites in microalgae research publications grew rapidly from 2015 to 2022. At this stage, known as the rapid development stage, 84.09% of all published material was accessible (Sun et al., 2019). 2015 to 2022 can be viewed as the boom stage, when an increasing number of academics began to study this topic.
Select subject categories, countries, and institutions from CiteSpace 6.1.R3 for analysis, and set the time slice as “1996-2022”. The year of each slice is 1, and the threshold value of g index is K=25. Table 1 collates the top ten relevant disciplines, countries and institutions in terms of the number of articles published on microalgal antioxidant research.
Each WoSCC article has been divided into one or more disciplinary groups. According to the number of publications in the 2093 linked articles, Table 1 presents the top ten subject groups. The subject category with the most publications was “Biotechnology and Applied Microbiology,” which had 529. Other popular subject categories were “Food Science and Technology,” “Environmental Sciences,” “Marine and Freshwater Biology,” “Biochemistry and Molecular Biology,” etc. Among the ten topic areas, “Biochemistry & Molecular Biology” had the highest centrality (0.46), which suggested that it is more significant and influential. Overall, research on microalgal antioxidants is often an interdisciplinary endeavor combining numerous fields.
Figure 2A shows that China has the largest node size indicating that China published the most publications, with 456 papers, followed by India, Spain, Brazil, Italy, and Portugal. Furthermore, the purple circle of the outer ring represents betweenness centrality, a measure of influence that reveals how closely nations, publications or journals are related to one another. A high betweenness centrality means that articles/authors from that country and region have a more significant influence on the field of study because it connects other articles/authors, and therefore more information and pathways pass through them. France (0.29) has the largest purple circle, indicating that French research in this field has been the most influential. China (0.2), Spain (0.19), Portugal (0.18), the United States (0.16), India (0.15), and Italy (0.15), Brazil (0.12), among others, also have high betweenness centrality. These countries have made a substantial contribution to the understanding of high-value metabolite antioxidation in microalgae. Finally, red citation rings indicate the research on the antioxidation of microalgae in a particular country has increased suddenly in a certain period (Chen et al., 2012).
Figure 2 Based on the information of microalgae antioxidant research from 1996 to 2022, including (A) a network map of countries/regions cooperation created by CiteSpace; (B) a string diagram created by Gephi of the network of countries/regions cooperating.
A national cooperation network (Figure 2B) was developed between 1996 and 2022 for this research to better understand the number of nations and areas that have contributed to the field of study on the antioxidation of high-value metabolites in microalgae. China and the United States, Spain and Portugal, South Korea and India, Egypt and Saudi Arabia, and France and Tunisia all have significant alliances, according to an examination of the national cooperation networks.
CiteSpace examined 2392 institutions that had written articles about microalgal antioxidants. Among the top 10 institutions by several publications, Univ Sao Paulo’ s research on this topic was earlier, having produced literature in 1998 (Miranda et al., 1998). The University of Porto has published 48 publications, ranking first. The Chinese Academy of Sciences (43) is in second place, followed by the Ocean Univ China (32). The fact that China has the majority of the top 10 institutions demonstrates how dominant Chinese research is in this field. The University of Porto has the highest value for intermediate centrality (0.19), indicating that it has a higher impact on the development of microalgal antioxidants. The collaboration links between the institutions are depicted in Figure 3, with a focus on Chinese Acad Sci and Ocean Univ China, which form a research core strength and work closely with several other institutions.
Table 2 shows the top 10 authors with the most cited research in this field from 1996 to 2022, using the total number of papers and the total number of citations worldwide as statistical indicators. Among the 8236 authors involved in microalgal antioxidant research, Ibanez, E. has the highest total number of citations (2849) and the most published papers (26 with an H-index of 21), whose main research interests are the extraction of functional components from different natural sources and the study of these components’ structure, function, and application. According to the total number of publications, other writers who have significantly advanced the study of microalgae antioxidants include Guedes, A.C., Chang, J.S., and Cifuentes, A.
The publications that receive the most citations are typically regarded as landmark pieces (Chen et al., 2012). Table 3 shows the top 10 studies on the antioxidation of highly valuable metabolites in microalgae. Among them, the most frequently referenced literature is “The promising future of microalgae: current status, challenges, and optimization of a sustainable and renewable industry for biofuels, feed, and other products” (Khan et al., 2018), in which the advantages of microalgae for the production of biofuels and various bioactive compounds are described. Furthermore, Koyande A.K. (Koyande et al., 2019) discussed numerous microalgal proteins as well as other bio-active substances that contribute to improved health. Analysis of several other highly cited literature shows that the antioxidant capacity of bioactive substances in microalgae, especially carotenoids, phenols, and polysaccharides, and their use in industrial, food, and pharmaceutical applications is a significant study area.
The document co-citation network can categorize the topics covered in this field and extract noun phrases from the titles, keywords or abstracts of articles that cite specific clusters. This study co-cited and clustered 2093 academic papers that studied microalgae antioxidants from 1996 to 2022. The clustering analysis was based on keyword clustering and used log-likelihood ratios (LLR) to provide clustering labels and visualizes the dynamics of the article clustering network using a “timeline view” and a “clustering view”. The labels for each of the 14 keyword clusters were extracted using the LLR technique (Figure 4). The weighted average silhouette s value was 0.8777, while the modality Q value was 0.7367. In general, the modality Q value had to be greater than 0.3 for the separation clustering structure to be significant, and the silhouette s value had to be greater than 0.5 for the clustering to be effective. The largest subnetwork had 912 nodes, representing 77% of the entire network. Specific feature information is presented in Table 4.
Figure 4 Map of the largest subnetwork of co-cited clustering of the antioxidant capacity of the bioactive substances in microalgae (Research literature from 1996 to 2022).
Table 4 Clustering information of the antioxidant capacity of the bioactive substances in microalgae.
Figure 5 shows a timeline view of the co-citation network divided into clusters. The clusters are arranged in descending order according to their size when viewed from the top. Cluster #0 studies bioactive compounds in microalgae and their relevance to health, while clusters #4 astaxanthin, #5 lipid, #7 hepatoprotective effects, #8 health-promoting effects, and #25 fucoxanthin are closely related to this cluster.
The research hotspots of antioxidation of high-value metabolites in microalgae mostly involve the following topics, as shown by the clustering findings and the content analysis of the literature in each cluster. (1) Reactive oxygen production, oxidative stress, and antioxidant mechanisms; (2) common assays used to assess the antioxidant activity of microalgae in vitro; (3) bioactive substances in different microalgae; (4) the potential use of microalgae in the food industry and in the medical, cosmetic, nutraceutical industries.
Reactive oxygen species (ROS), which include free radicals (•O2-, superoxide radicals; OH•, hydroxyl radical; HO2•, perhydroxy radical and RO•, alkoxy radicals) and non-free radicals (H2O2, hydrogen peroxide and 1O2, singlet oxygen) (Gill and Tuteja, 2010), are byproducts of cellular metabolism. There is convincing evidence that ROS has “two-sides” (Valko et al., 2006). At medium and low concentrations, ROS functions as a signaling factor in the physiological response of cells to hypoxia (Valko et al., 2007), and different biological systems use the mitogen-activated protein kinase (MAPK) pathway to send signals to the nucleus via redox reactions. However, numerous conditions can cause an organism to produce excessive amounts of ROS (Figure 6A), which can lead to physical damage by oxidizing proteins, damaging nucleic acids, and causing lipid peroxidation (Apel and Hirt, 2004).
Figure 6 (A) Sources of reactive oxygen species (ROS), created with https://biorender.com/ (accessed on 24 December 2022); (B) combined mechanisms of enzymatic and non-enzymatic antioxidants. SOD, Superoxide dismutase; CAT, Catalase; PrX, peroxiredoxin; APX, Ascorbate peroxidase; AsA, ascorbate; MDHA, monodehydroascorbate; MDHAR, monodehydroascorbate reductase; DHA, dehydroascorbate; DHAR, dehydroascorbate reductase; GSH, reduced glutathione; GSSG, oxidized glutathione; GR, glutathione reductase; GPX, Glutathione peroxidase.
Cells, however, have potent antioxidant defense mechanisms that can be separated into enzymatic systems (including SOD, APX, GPX, GST, and CAT) and non-enzymatic systems (such as ASH, GSH, alpha-tocopherol, carotenoids, and flavonoids) (Mittler et al., 2004). These systems cooperate in combating the negative consequences of ROS. The different enzymatic and non-enzymatic antioxidants that microalgae use to defend themselves against ROS damage are shown in Figure 6B. Microalgal bioactive components can serve as a reliable supply of antioxidants for scavenging ROS. For example, ascorbic acid (ASH, vitamin C), the most prevalent and potent water-soluble antioxidant among non-enzymatic antioxidants, is present in all plant tissues (Athar et al., 2008). Meanwhile, ASA and GSH are the two non-enzymatic antioxidants in higher plants in the most significant amounts, which play an essential role in scavenging ROS directly through the AsA-GSH cycle as electron donors (Hasanuzzaman et al., 2019). In microalgae, vitamins C and tocopherols (vitamins E) remove ROS by neutralizing free radicals with electrons, and glutathione acts as a cofactor for anti-oxidative stress detoxification enzymes and also directly scavenges hydroxyl radicals and singlet oxygen (Valko et al., 2007). In addition, according to studies, carotenoids are significant antioxidants in microalgae since they not only eliminate peroxyl and hydroxyl radicals but also act as sunscreen (Varela et al., 2015).
ABTS/TEAC/DPPH is the primary technique used to assess the antioxidant activity of microalgal extracts using in vitro chemical tests. It is important to remember that conclusions on the antioxidant activity should not be based on just one antioxidant assay but rather on a thorough assessment of the outcomes of various methods for measuring in vitro antioxidant activity (Alam et al., 2013). To this purpose, Table 5 provides several frequently used assay procedures with their principles, benefits, and insufficient to giving the investigator a thorough grasp of these in vitro assay techniques before implementing them.
The following is a discussion of some of the very valued bioactive compounds in microalgae.
The yellow and red colors that can be found in a variety of fruits and vegetables are caused by pigments that contain carotenoids (Stahl and Sies, 2003). There are two broad classifications of carotenoids: xanthophylls and carotenes. The difference between them is chemical: xanthophylls contain oxygen, while carotenes are hydrocarbons and do not contain oxygen. Carotenoids have antioxidant qualities that protect cells from reactive free radicals, prevent lipid oxidation, and possibly even promote the integrity of cell membranes (Gong and Bassi, 2016). The microalgae strains Dunaliella salina, Chlorella sp., Haematococcus pluvialis, and Spirulina sp. are ideal for producing carotenoids (Christaki et al., 2013). Naguib (Naguib, 2000) compared the antioxidant activity of various carotenoids and showed that astaxanthin (1.3) had the highest relative activity against peroxyl radicals in carotenoids, followed by 6-hydroxy-2,5,7,8-tetramethylchroman-2-carboxylic acid (1.0), α-tocopherol (0.9), lutein (0.4) and beta-carotene (0.2). This article focuses on β-carotene, astaxanthin and lutein.
The most biologically active red ketone carotenoid found in nature, astaxanthin (3,3’-dihydroxy-β-β-carotene 4,4’-dione), can be obtained from crustaceans, plants, bacteria, and fungi (Li et al., 2011). Among them, the Haematococcus Pluvialis is believed to be the best source of natural astaxanthin due to its astaxanthin content of up to 1-5% of cell dry weight (Wan et al., 2014). However, astaxanthin accumulation is constrained by the poor biomass productivity under normal circumstances. And under stressful conditions (high light or nutritional deficiency), fast-growing motile cells are typically used to promote astaxanthin production; however, biomass productivity is low (Hata et al., 2001). To induce astaxanthin accumulation under photoautotrophic circumstances, Zhang et al. (Zhang et al., 2016) first created the concept of heterotrophic cultures, followed by transferring the heterotrophic cells to a specific light/nutrient environment. The generation of astaxanthin was 4.2 times higher using this culture technique. Another important carotenoid pigment is β-carotene, which is mainly from Chlorella and Dunaliella. The microalga generates β-carotene in proportions equal to approximately 10–14% of its dry mass (Sathasivam et al., 2019). β-carotene content dramatically increased under photoheterotrophic circumstances: 67.5 mM acetate and 450 μM FeSO4 enriched growth conditions, and cellular β-carotene content rose to up to 70 pg cell-1 (Mojaat et al., 2008). Cations such as Fe2+ could increase -carotene production of β-carotene by causing oxidative stress. Due to recent investigations, zeaxanthin and the lutein are receiving more and more attention. Although it is well known that marigold resin contains antioxidants, certain microalgae have hundreds of times more lutein than marigold species (Fernandez-Sevilla et al., 2010). Numerous factors influence the lutein content of microalgae, including pH, which reduces the efficiency of CO2 and thus inhibits photosynthesis (Macias-Sanchez et al., 2010); high temperatures are beneficial for lutein accumulation (Fernandez-Sevilla et al., 2010), but too high temperatures are harmful. High radiation levels, the concentration of nitrogen in the medium, and the presence of oxidizing substances are partially responsible for the accumulation of lutein (Guedes et al., 2011).
Polysaccharides are heteropolymers comprising the major sugars galactose, xylose, and glucose joined together by glycosidic linkages (Chanda et al., 2019). Marine macro- and microalgae can produce a wide range of polysaccharides, either internally or externally, the majority of which are sulphated (sPS) (Raposo et al., 2015). Sulphate polysaccharides synthesized and released by marine microalgae have the ability to stop the accumulation of free radicals and reactive chemicals, according to Raposo et al. (Raposo et al., 2013). Subsequently, Tannin-spitz T et al. (Tannin-Spitz et al., 2005) corroborated this assertion and measured the ability of crude polysaccharide solutions to oxidize linoleic acid to estimate the antioxidant activity of polysaccharide solutions. The sulphated polysaccharide was shown to protect algae from ROS by scavenging free radicals and transferring them from cells to the culture medium. Likewise, the antioxidant activity is highly correlated with the content of sulfates. The heterogeneity and structural differences in the extracellular sulphate polysaccharides synthesized by different marine microalgae make research challenging. Contrary to seaweed, microalgae can be cultivated in controlled environments, improving the stability of their chemical and structural makeup (Raposo et al., 2013). Thus, microalgae polysaccharides may be essential for biobased materials in the life sciences (e.g., medical devices, pharmaceutics, food, and cosmetics).
Tocopherols, often known as vitamin E, are lipid-soluble compounds produced by photosynthesis, which act as antioxidants through single electron transfer (SET) and hydrogen atom transfer (HAT) (Herrera and Barbas, 2001). Research has shown that tocopherols can be extracted, purified, or concentrated from vegetable oils and other higher plant substances, and tocopherol-containing microalgae include Spirulina, Dunaliella tertiolecta, and Chlorella sp. The most active and extensively researched of the four isomers is α-tocopherol (Ogbonna, 2009). Tocopherols function as membrane stabilizers by preventing oxidative damage to vesicle-like structures (Powls and Redfearn, 1967), which play a role in the prevention of light-induced instances in the skin and eyes (Cahoon et al., 2003). The liver is one of the primary targets for ROS illness, and it has been demonstrated that phenolic substances, such as isoflavones and flavonoids, have a protective impact on the organ (Sanchez-Salgado et al., 2019). In addition, α-tocopherol may help Alzheimer’s patients feel better (Sano et al., 1997).
For tissue integrity and health benefits, polyunsaturated fatty acids (PUFAs), namely EPA and DHA, are crucial. Omega-3 and omega-6 fatty acids are necessary for the human body, but the body cannot manufacture them. The ability of numerous microalgal species (e.g. Porphyridium cruentum, Arthrospira platensis, Odontella, and Isochrysis galbana) to synthesize these critical fatty acids have been investigated (Khan et al., 2018). PUFAs are converted to triacylglycerols (TAG) or polar lipids (i.e., phospholipids, glycolipids) by the esterification of glycerol with PUFAs. These lipids offer an ideal structure to control the fluidity and function of membranes. Furthermore, the creation of additional metabolic products with high added value, such as β-carotene and astaxanthin, can be integrated with the production of lipids (Bellou et al., 2014).
Microalgae biomass should undergo an extraction procedure before use to make it a more valuable source of antioxidants (Cotas et al., 2020). Typical extraction techniques include ultrasounds methods, supercritical fluid extraction, percolation, and microwave methods. Microalgae extracts typically contain a variety of bioactive ingredients (Michalak et al., 2022). There is a trend toward using microalgae and their bioactive natural compounds in various fields, including the pharmaceutical, biomedical, cosmetic, and food industries. This research focuses on the application of the antioxidant capacity of microalgal bioactive chemicals to diverse sectors (Table 6).
The benefits of microalgae as a new functional and healthy element are becoming more well known. Patients at risk of macular degeneration can avoid the danger by giving them lutein in the form of a nutritional supplement (Fernandez-Sevilla et al., 2010). As most of the sPS in microalgae have strong antioxidant properties, they may protect human health from ROS that cause cancer, diabetes, some inflammatory and neurodegenerative diseases, and other ageing-related diseases such as Alzheimer’ s disease and cardiovascular disease (Raposo et al., 2015). Long-chain poly unsaturated fatty acids (LC-PUFA), such as docosahexanoic acid (C22:6) and eicosapentaenoic acid (C20:5), are good for cardiovascular health (Harris et al., 2008). Studies conducted in vivo and in vitro have demonstrated that astaxanthin works well to stop the oxidation of low-density lipoprotein (LDL), which is utilized for safeguarding the body against various ailments (Riccioni et al., 2011). Several studies have recently demonstrated that the addition of astaxanthin to the human diet can reduce oxidative stress and further improve the immune system in patients with cardiovascular disease (Park et al., 2010). Consumption of astaxanthin erythrocytes may prevent oxidative damage in heavy smokers by inhibiting lipid peroxidation (Kim et al., 2011).
In 1952, the Algae Mass-Culture Symposium proposed using microalgae for biochemical and dietary purposes (Caporgno and Mathys, 2018). Even though some beneficial microalgae cannot currently be used directly in food, several bioactive chemicals derived from these sources have been authorized for use in human or animal diets. The antioxidant functional components of the microalgal active ingredients have also received attention due to their potential for use as preservatives and antioxidants in the food industry to lengthen the shelf life of foods (Lourenco et al., 2019) as well as their ability to be added to foods to improve human and animal nutrition (Carocho et al., 2018). For instance, when farmed marine animals eat feed containing astaxanthin, their health, and nutritional quality also advance in addition to their appearance. In addition, roughly ten different microalgae or microalgae extracts can be used as food or components in Europe (Coulombier et al., 2021). Protein is currently the primary source of protein for food and feed, and algae may account for up to 18% of protein sources by mid-century. It was suggested several decades ago to add Dunaliella as a protein supplement to white wheat bread (Finney et al., 1984). Furthermore, a recent development in the food sector is the blend of inorganic nanoparticles (NP) and natural antioxidant chemicals. Due to the more robust antibacterial, antioxidant, UV barrier, oxygen removal, and low environmental impact properties of these compounds, their inclusion in biological and biodegradable matrices such as polysaccharides and proteins emphasizes the potential application of active food packaging (Vieira et al., 2022). However, due to a lack of technology and economies of scale in their production and processing, employing microalgae and their bioactive compounds as food alternatives presents hurdles (Caporgno and Mathys, 2018).
Algae are naturally exposed to oxidative stress and have developed several effective protective systems against ROS and free radicals, producing compounds that can be used in cosmetics to combat the harmful effects of UV radiation (Ariede et al., 2017). Chlorella vulgaris and Spirulina obtusifolia contain chlorophyll and carotenoids, due to their antioxidant capabilities, these compounds may help prevent oil oxidation in formulations, particularly in emulsions with many oily phases (Gouveia et al., 2008). Additionally, red microalgae extracts are used in skin care, sun protection, hair care, emollients, regeneration care products, and anti-aging lotions (Borowitzka, 2013). Furthermore, microalgae and their bioactive substances have been reported to be added mainly to cosmetic formulations as thickeners and water-binding agents (El Gamal, 2010) in addition to being an antioxidant, such as specific components of the microalgae extract react with various proteins on the skin to form a protective gel on the skin’s surface, preventing moisture loss (Yarkent et al., 2020).
It should be noted that thorough scientific trials are required for natural antioxidants before microalgae and its bioactive components can be used in food, medicine, or cosmetics. To effectively utilize natural antioxidants, clarifying their structural properties and removing any undesirable components is necessary (Rani et al., 2021).
As descriptive terms, keywords can be employed to analyze the historical development of a subject area (Yu et al., 2017). In this section, we examine the content by examining the keyword distribution. It will display the keywords co-occurrence network map, the keywords density visualization map, and the keywords with the strongest citation burst view.
The program VOSviewer built a network of co-occurring author keywords on antioxidants in microalgal bioactives. A total of 4750 authors’ keywords were used, and the top 50 most frequently occurring were selected to create Figure 7. The size of the nodes and words in Figure 7A corresponds to the nodes’ weights. The distance between two nodes indicates how closely they are connected. Generally, a lesser distance indicates a closer bond. A line between two keywords demonstrates that they have occurred together, and the more co-occurrences there are, the thicker the line gets (Gu et al., 2017). Keywords that appear more frequently are “microalgae,” “carotenoids,” “astaxanthin,” “bioactive compound,” and “oxidative stress”. Additionally, a density map of the top 50 keywords used by the writers was made using VOSviewer, with redder and brighter colors denoting greater amounts of research. Figure 7B shows that in addition to the more well-known keywords, “polysaccharides,” “lutein” and “antioxidant enzymes” also show up in the plots and might be of particular interest for further study.
Figure 7 (A) Top 50 keywords most prominently displayed in VOSviewer; (B) visualization of keyword density in microalgal antioxidation study.
From 1996 to 2022, the keywords “microalgae metabolites antioxidation research” appeared to have a high citation burst (Figure 8). The blue lines represent this period. The starting and ending years of the burst duration are indicated by a red line segment that represents the time period during which a subject category was discovered to have a burst (Chen et al., 2014). The citation explosion started in 1998 for beta-carotene, with the highest explosion intensity (10.04) and the longest explosion time, cited for 17 years from 1998 to 2015. Astaxanthin, the term that came after the epidemic in 2002, was mentioned to have a 15-year duration and a 6.16 burst strength. Superoxide dismutase and antioxidant enzyme citation bursts persisted for more than ten years. It is worth noting that gene expression, seaweed, stability, salt stress, and light have emerged as a new field of study in recent years, while accumulation, lutein, Haematococcus pluvialis, fraction, lipid peroxidation, in vitro have been a research focus from 2009 to 2016.
Fucoxanthin, polysaccharides, salt stress, and light exposure are predicted to be the topics of future research based on keyword analysis.
Fucoxanthin, one of the carotenoids, as a natural pigment that belongs to the category of xanthophylls, is present exclusively in algae. Due to its numerous biological and health-promoting qualities, including its anti-diabetic, anti-obesity, anti-cancer, and antioxidant characteristics, fucoxanthin has received much attention lately (Muthuirulappan and Francis, 2013). The amount of fucoxanthin in microalgae has been shown to grow at light densities between 10 and 100 µmol/m2/s; however, the effects of varying light, nutrition, salinity, temperature, and carbon dioxide on fucoxanthin levels vary (Khaw et al., 2022). Therefore, investigations on temperature and salinity are required to achieve the ideal balance between fucoxanthin and biomass production while swiftly putting fucoxanthin into commercial production. For example, studies have been done to increase the stability and bioavailability of extracts such as astaxanthin by using nanoencapsulation methods. In the future, combining fucoxanthin with nanotechnology is possible, but only if it’s essential to prove that such effects are being exerted before usage and not toxic ones that are dangerous to people.
“Polysaccharide” also appears and shows some intensity in the keyword density graph. Microalgae polysaccharides can prolong the shelf life of vegetables and fruits and are mostly employed in food science and technology for health products, food safety, food coating preservation, food additives, and other purposes. Combining inorganic nanoparticles (NPs) with natural antioxidant compounds is a trend in the food industry that emphasizes the potential uses for active food packaging.
The objective data analysis was the foundation for the conclusions, but certain restrictions exist. First, we used the relatively homogeneous original English language literature from the WoSCC database. In addition, the use of other databases and publications in other languages at the same time may improve the rigorism. Second, the primary focus of this article is on the antioxidant properties of bioactive substances, but additional research on the functions and mechanisms of some essential antioxidant enzymes is warranted; one such enzyme is SOD, which serves as the body’ s first line of defense against ROS mediation and is crucial in treating diseases linked to oxidative stress. Third, carotenoids play a significant role in microalgae, which has been revealed by the bibliometric examination of the antioxidant capacity of bioactive compounds in microalgae. The varieties of microalgae species that produce lutein and carotene, as well as their use, should be examined more thoroughly in subsequent research. Finally, a change in scientific interest in antioxidant chemicals has been noticed, with a steady shift from the research of antioxidant vitamins and minerals to the study of antioxidant phytochemicals. From choosing the most suited microalgal strain to growth techniques and downstream processes, several sequential actions must be taken to produce bioproducts from microalgal biomass (e.g., harvesting, pretreatment, extraction, and purification). Although these treatments can improve the number of bioactive compounds in microalgae, they also impede the growth of microalgal biomass, somewhat restricting the commercial and industrial use of microalgae. The future trend will be to identify appropriate culture settings to increase extraction rates and, at the same time, to identify hydroxyl groups or proteins that can bind to the bioactive components of microalgae and increase the stability of those components through combinations.
This paper uses a bibliometric approach to evaluate trends and hotspots related to bioactive substances and antioxidant properties of microalgae. Between 1996 and 2022, 2093 original articles and reviews were retrieved. The field was found to have published few papers until 2006, gradually increasing through 2014 and then development in a period of rapid growth, with the most productive period currently occurring in 2021. Indicating that this field is an area of ongoing research interest, we speculate that antioxidant research on the bioactivity of microalgae will remain a hot research topic in the future.
Regarding national research, the number of global publications is unevenly distributed between countries, with only nine countries publishing more than 100 articles over 27 years. It is interesting to note that France, in tenth place in terms of several publications, surpassed China in terms of the number of cited times, ranking first. This might result from early research and tight relationships between France and other nations. As a result, international interaction and collaboration should be enhanced, and transnational and cross-team collaboration is key to accelerating research development. Regarding the results of the institutional analysis, the University of Porto and the Chinese Academy of Sciences show a substantial contribution in this field, with a high-citation burst from CSIC and the University of So Paulo. And finally, according to the keyword analysis, the most explored topic is the characterization of bioactive substances, and extraction and application are the focus of future research.
Overall, by systematically summarizing the literature in this field, this study demonstrates the dynamic development process and structural relationships of knowledge in related disciplines in data visualization and explores some hotspot areas. It is recommended to maintain cooperative exchanges between countries, institutions in the field of bioactive antioxidant function of microalgae substances, to focus on scientific hotspots and to promote the academic development of the area jointly.
NY: Methodology, Software, Validation, Investigation, Writing - Original Draft. QZ: Methodology, Software, Validation, Investigation. CJ: Formal analysis, Investigation, Resources. SW: Investigation, Resources. RC&LY: Data Curation, Visualization. BL: Writing - Review & Editing. XL: Supervision, Project administration. RZ: Supervision, Project administration. ZZ: Conceptualization, Resources, Writing - Review & Editing, Funding acquisition.All authors contributed to the article and approved the submitted version.
This research was funded by Zhejiang Institute of Strategy Research for China Engineering Science and Technology Development and the Basic Public Welfare Research Project of Zhejiang Province (LGN22C190010).
The authors declare that the research was conducted in the absence of any commercial or financial relationships that could be construed as a potential conflict of interest.
All claims expressed in this article are solely those of the authors and do not necessarily represent those of their affiliated organizations, or those of the publisher, the editors and the reviewers. Any product that may be evaluated in this article, or claim that may be made by its manufacturer, is not guaranteed or endorsed by the publisher.
Alam, M. N., Bristi, N. J., Rafiquzzaman, M. (2013). Review on in vivo and in vitro methods evaluation of antioxidant activity. Saudi Pharm. J. 21 (2), 143–152. doi: 10.1016/j.jsps.2012.05.002
Apel, K., Hirt, H. (2004). Reactive oxygen species: Metabolism, oxidative stress, and signal transduction. Annu. Rev. Plant Biol. 55, 373–399. doi: 10.1146/annurev.arplant.55.031903.141701
Ariede, M. B., Candido, T. M., Jacome, A. L. M., Velasco, M. V. R., de Carvalho, J. C. M., Baby, A. R. (2017). Cosmetic attributes of algae - a review. Algal Research-Biomass Biofuels Bioproducts 25, 483–487. doi: 10.1016/j.algal.2017.05.019
Athar, H. U. R., Khan, A., Ashraf, M. (2008). Exogenously applied ascorbic acid alleviates salt-induced oxidative stress in wheat. Environ. Exp. Bot. 63 (1-3), 224–231. doi: 10.1016/j.envexpbot.2007.10.018
Ayna, A. (2020). Apoptotic effects of beta-carotene, alpha-tocopherol and ascorbic acid on PC-3 prostate cancer cells. Hacettepe J. Biol. Chem. 48 (3), 211–218. doi: 10.15671/hjbc.519212
Badarinath, A. V., Rao, K. M., Chetty, C. S., Ramkanth, S., Rajan, T., Gnanaprakash, K. (2010). A review on In-vitro antioxidant methods: Comparisions, correlations and considerations. Int. J. Pharmtech Res. 2 (2), 974–4304.
Batista, A. P., Niccolai, A., Fradinho, P., Fragoso, S., Bursic, I., Rodolfi, L., et al. (2017). Microalgae biomass as an alternative ingredient in cookies: Sensory, physical and chemical properties, antioxidant activity and in vitro digestibility. Algal Research-Biomass Biofuels Bioproducts 26, 161–171. doi: 10.1016/j.algal.2017.07.017
Bellou, S., Baeshen, M. N., Elazzazy, A. M., Aggeli, D., Sayegh, F., Aggelis, G. (2014). Microalgal lipids biochemistry and biotechnological perspectives. Biotechnol. Adv. 32 (8), 1476–1493. doi: 10.1016/j.biotechadv.2014.10.003
Borowitzka, M. A. (2013). High-value products from microalgae-their development and commercialisation. J. Appl. Phycol 25 (3), 743–756. doi: 10.1007/s10811-013-9983-9
Cahoon, E. B., Hall, S. E., Ripp, K. G., Ganzke, T. S., Hitz, W. D., Coughlan, S. J. (2003). Metabolic redesign of vitamin e biosynthesis in plants for tocotrienol production and increased antioxidant content. Nat. Biotechnol. 21 (9), 1082–1087. doi: 10.1038/nbt853
Cakmakci, S., Topdas, E. F., Kalin, P., Han, H., Sekerci, P., Kose, L. P., et al. (2015). Antioxidant capacity and functionality of oleaster (Elaeagnus angustifolia l.) flour and crust in a new kind of fruity ice cream. Int. J. Food Sci. Technol. 50 (2), 472–481. doi: 10.1111/ijfs.12637
Caporgno, M. P., Mathys, A. (2018). Trends in microalgae incorporation into innovative food products with potential health benefits. Front. Nutr. 5. doi: 10.3389/fnut.2018.00058
Carocho, M., Morales, P., Ferreira, I. (2018). Antioxidants: Reviewing the chemistry, food applications, legislation and role as preservatives. Trends Food Sci. Technol. 71, 107–120. doi: 10.1016/j.tifs.2017.11.008
Chacon-Lee, T. L., Gonzalez-Marino, G. E. (2010). Microalgae for “Healthy” foods-possibilities and challenges. Compr. Rev. Food Sci. Food Saf. 9 (6), 655–675. doi: 10.1111/j.1541-4337.2010.00132.x
Chanda, M. J., Merghoub, N., Arroussi, H. E. (2019). Microalgae polysaccharides: the new sustainable bioactive products for the development of plant bio-stimulants? World J. Microbiol. Biotechnol. 35 (11), 177–. doi: 10.1007/s11274-019-2745-3
Chen, C. M. (2004). Searching for intellectual turning points: Progressive knowledge domain visualization. Proc. Natl. Acad. Sci. United States America 101, 5303–5310. doi: 10.1073/pnas.0307513100
Chen, C., Dubin, R., Kim, M. C. (2014). Emerging trends and new developments in regenerative medicine: a scientometric update, (2000 – 2014). Expert Opin. Biol. Ther. 14 (9), 1295. doi: 10.1517/14712598.2014.920813
Chen, C. M., Hu, Z. G., Liu, S. B., Tseng, H. (2012). Emerging trends in regenerative medicine: a scientometric analysis in CiteSpace. Expert Opin. Biol. Ther. 12 (5), 593–608. doi: 10.1517/14712598.2012.674507
Christaki, E., Bonos, E., Giannenas, I., Florou-Paneri, P. (2013). Functional properties of carotenoids originating from algae. J. Sci. Food Agricult. 93 (1), 5–11. doi: 10.1002/jsfa.5902
Chronakis, I. S. (2001). Gelation of edible blue-green algae protein isolate (Spirulina platensis strain pacifica): Thermal transitions, rheological properties, and molecular forces involved. J. Agric. Food Chem. 49 (2), 888–898. doi: 10.1021/jf0005059
Cirulis, J. T., Scott, J. A., Ross, G. M. (2013). Management of oxidative stress by microalgae. Can. J. Physiol. Pharmacol. 91 (1), 15–21. doi: 10.1139/cjpp-2012-0249
Cotas, J., Leandro, A., Monteiro, P., Pacheco, D., Figueirinha, A., Goncalves, A. M. M., et al. (2020). Seaweed phenolics: From extraction to applications. Mar. Drugs 18 (8), 47. doi: 10.3390/md18080384
Coulombier, N., Jauffrais, T., Lebouvier, N. (2021). Antioxidant compounds from microalgae: A review. Mar. Drugs 19 (10), 549. doi: 10.3390/md19100549
Davinelli, S., Nielsen, M. E., Scapagnini, G. (2018). Astaxanthin in skin health, repair, and disease: A comprehensive review. Nutrients 10 (4), 12. doi: 10.3390/nu10040522
de Souza, M. P., Hoeltz, M., Gressler, P. D., Benitez, L. B., Schneider, R. C. S. (2019). Potential of microalgal bioproducts: General perspectives and main challenges. Waste Biomass Valorization 10 (8), 2139–2156. doi: 10.1007/s12649-018-0253-6
El Gamal, A. A. (2010). Biological importance of marine algae. Saudi Pharm. journal: SPJ: Off. Publ. Saudi Pharm. Soc. 18 (1), 1–25. doi: 10.1016/j.jsps.2009.12.001
Fernandez-Sevilla, J. M., Fernandez, F. G. A., Grima, E. M. (2010). Biotechnological production of lutein and its applications. Appl. Microbiol. Biotechnol. 86 (1), 27–40. doi: 10.1007/s00253-009-2420-y
Finney, K. F., Pomeranz, Y., Bruinsma, B. L. (1984). Use of algae dunaliella as a protein supplement in bread. Cereal Chem. 61 (5), 402–406.
Foo, S. C., Khoo, K. S., Ooi, C. W., Show, P. L., Khong, N. M. H., Yusoff, F. M. (2021). Meeting sustainable development goals: Alternative extraction processes for fucoxanthin in algae. Front. Bioengineering Biotechnol. 8. doi: 10.3389/fbioe.2020.546067
Gauthier, M. R., Senhorinho, G. N. A., Scott, J. A. (2020). Microalgae under environmental stress as a source of antioxidants. Algal Research-Biomass Biofuels Bioproducts 52, 10. doi: 10.1016/j.algal.2020.102104
Gill, S. S., Tuteja, N. (2010). Reactive oxygen species and antioxidant machinery in abiotic stress tolerance in crop plants. Plant Physiol. Biochem. 48 (12), 909–930. doi: 10.1016/j.plaphy.2010.08.016
Gong, M. Y., Bassi, A. (2016). Carotenoids from microalgae: A review of recent developments. Biotechnol. Adv. 34 (8), 1396–1412. doi: 10.1016/j.biotechadv.2016.10.005
Gouveia, L., Batista, A. P., Sousa, I., Raymundo, A., Bandarra, N. M. (2008). “Microalgae in novel food products,” in Food chemistry research developments. Ed. Papadopoulos, K. N. (Hauppauge NY, USA: Nova SciencePublishers, Inc.), 1–37.
Gu, D., Li, J., Li, X., Liang, C. (2017). Visualizing the knowledge structure and evolution of big data research in healthcare informatics. Int. J. Med. Informatics. 98, 22–32. doi: 10.1016/j.ijmedinf.2016.11.006
Guedes, A. C., Amaro, H. M., Malcata, F. X. (2011). Microalgae as sources of carotenoids. Mar. Drugs 9 (4), 625–644. doi: 10.3390/md9040625
Harris, W. S., Kris-Etherton, P. M., Harris, K. A. (2008). Intakes of long-chain omega-3 fatty acid associated with reduced risk for death from coronary heart disease in healthy adults. Curr. Atheroscl. Rep. 10 (6), 503–509. doi: 10.1007/s11883-008-0078-z
Hasanuzzaman, M., Bhuyan, M., Anee, T. I., Parvin, K., Nahar, K., Al Mahmud, J., et al. (2019). Regulation of ascorbate-glutathione pathway in mitigating oxidative damage in plants under abiotic stress. Antioxidants 8 (9). doi: 10.3390/antiox8090384
Hata, N., Ogbonna, J. C., Hasegawa, Y., Taroda, H., Tanaka, H. (2001). Production of astaxanthin by haematococcus pluvialis in a sequential heterotrophic-photoautotrophic culture. J. Appl. Phycol 13 (5), 395–402. doi: 10.1023/a:1011921329568
Herrera, E., Barbas, C. (2001). Vitamin e: action, metabolism and perspectives. J. Physiol. Biochem. 57 (2), 43–56. doi: 10.1007/BF03179812
Herrero, M., Ibáñez, E., Cifuentes, A., Reglero, G., Santoyo, S. (2006). Dunaliella salina microalga pressurized liquid extracts as potential antimicrobials. J. Food Prot. 69 (10), 2471–2477. doi: 10.4315/0362-028x-69.10.2471
Hirayama, S., Ueda, R., Sugata, K. (1996). Evaluation of active oxygen effect on photosynthesis of chlorella vulgaris. Free Radical Res. 25 (3), 247–254. doi: 10.3109/10715769609149050
Khan, M. I., Jin, H. S., Kim, J. D. (2018). The promising future of microalgae: current status, challenges, and optimization of a sustainable and renewable industry for biofuels, feed, and other products. Microbial Cell Factories 17 (1), 36. doi: 10.1186/s12934-018-0879-x
Khaw, Y. S., Yusoff, F. M., Tan, H. T., Mazli, N., Nazarudin, M. F., Shaharuddin, N. A., et al. (2022). Fucoxanthin production of microalgae under different culture factors: A systematic review. Marine Drugs 20 (10), 25. doi: 10.3390/md20100592
Kim, J. H., Chang, M. J., Choi, H. D., Youn, Y. K., Kim, J. T., Oh, J. M., et al. (2011). Protective effects of haematococcus astaxanthin on oxidative stress in healthy smokers. J. Medicinal Food 14 (11), 1469–1475. doi: 10.1089/jmf.2011.1626
Klejdus, B., Kopecky, J., Benesová, L., Vacek, J. (2009). Solid-phase/supercritical-fluid extraction for liquid chromatography of phenolic compounds in freshwater microalgae and selected cyanobacterial species. J. Chromatogr. A 1216 (5), 763–771. doi: 10.1016/j.chroma.2008.11.096
Kova, D. J., Simeunovi, J. B., Babi, O. B., Mian, A., Milovanovi, I. L. (2013). Algae in food and feed. Food Feed Res. 40 (1), 21–31.
Koyande, A. K., Chew, K. W., Rambabu, K., Tao, Y., Chu, D. T., Show, P. L. (2019). Microalgae: A potential alternative to health supplementation for humans. Food Sci. Hum. Wellness 8 (1), 16–24. doi: 10.1016/j.fshw.2019.03.001
Le, M., Xiao-Ming, L. (2010). Effects of lutein and zeaxanthin on aspects of eye health. J. Sci. Food Agric. 90 (1), 2–12. doi: 10.1002/jsfa.3785
Li, H. B., Cheng, K. W., Wong, C. C., Fan, K. W., Feng, C., Yue, J. (2007). Evaluation of antioxidant capacity and total phenolic content of different fractions of selected microalgae. Food Chem. 102 (3), 771–776. doi: 10.1016/j.foodchem.2006.06.022
Li, J., Zhu, D. L., Niu, J. F., Shen, S. D., Wang, G. C. (2011). An economic assessment of astaxanthin production by large scale cultivation of haematococcus pluvialis. Biotechnol. Adv. 29 (6), 568–574. doi: 10.1016/j.biotechadv.2011.04.001
Lourenco, S. C., Moldao-Martins, M., Alves, V. D. (2019). Antioxidants of natural plant origins: From sources to food industry applications. Molecules 24 (22), 25. doi: 10.3390/molecules24224132
Macias-Sanchez, M. D., Fernandez-Sevilla, J. M., Fernandez, F. G. A., Garcia, M. C. C., Grima, E. M. (2010). Supercritical fluid extraction of carotenoids from scenedesmus almeriensis. Food Chem. 123 (3), 928–935. doi: 10.1016/j.foodchem.2010.04.076
Madyastha, V. (2000). C-phycocyanin: A potent peroxyl radical scavenger in vivo and in vitro. Biochem. Biophys. Res. Commun. 275 (1), 20–25. doi: 10.1006/bbrc.2000.3270
Matos, J., Cardoso, C., Bandarra, N. M., Afonso, C. (2017). Microalgae as healthy ingredients for functional food: a review. Food Funct. 8 (8), 2672–2685. doi: 10.1039/c7fo00409e
Merigo, J. M., Cancino, C., Coronado, F., Urbano, D. (2016). Academic research in innovation: A country analysis. Scientometrics 108, 559–593. doi: 10.1007/s11192-016-1984-4
Michalak, I., Tiwari, R., Dhawan, M., Alagawany, M., Farag, M. R., Sharun, K., et al. (2022). Antioxidant effects of seaweeds and their active compounds on animal health and production - a review. Veterinary Q. 42 (1), 48–67. doi: 10.1080/01652176.2022.2061744
Miranda, M. S., Cintra, R. G., Barros, S. B. M., Mancini, J. (1998). Antioxidant activity of the microalga spirulina maxima. Braz. J. Med. Biol. Res. 31 (8), 1075–1079. doi: 10.1590/s0100-879x1998000800007
Mittler, R., Vanderauwera, S., Gollery, M., Van Breusegem, F. (2004). Reactive oxygen gene network of plants. Trends Plant Sci. 9 (10), 490–498. doi: 10.1016/j.tplants.2004.08.009
Mojaat, M., Pruvost, J., Foucault, A., Legrand, J. (2008). Effect of organic carbon sources and Fe2+ ions on growth and beta-carotene accumulation by dunaliella salina. Biochem. Eng. J. 39 (1), 177–184. doi: 10.1016/j.bej.2007.09.009
Muthuirulappan, S., Francis, S. P. (2013). Anti-cancer mechanism and possibility of nano-suspension formulation for a marine algae product fucoxanthin. Asian Pacific J. Cancer Prev. 14 (4), 2213–2216. doi: 10.7314/apjcp.2013.14.4.2213
Naguib, Y. M. A. (2000). Antioxidant activities of astaxanthin and related carotenoids. J. Agric. Food Chem. 48 (4), 1150–1154. doi: 10.1021/jf991106k
Ngo, D. H., Vo, T. S., Ngo, D. N., Wijesekara, I., Kim, S. K. (2012). Biological activities and potential health benefits of bioactive peptides derived from marine organisms. Int. J. Biol. Macromolecules 51 (4), 378–383. doi: 10.1016/j.ijbiomac.2012.06.001
Obon, J. M., Castellar, M. R., Cascales, J. A., Fernandez-Lopez, J. A. (2005). Assessment of the TEAC method for determining the antioxidant capacity of synthetic red food colorants. Food Res. Int. 38 (8-9), 843–845. doi: 10.1016/j.foodres.2005.01.010
Ogbonna, J. C. (2009). Microbiological production of tocopherols: current state and prospects. Appl. Microbiol. Biotechnol. 84 (2), 217–225. doi: 10.1007/s00253-009-2104-7
Park, J. S., Chyun, J. H., Kim, Y. K., Line, L. L., Chew, B. P. (2010). Astaxanthin decreased oxidative stress and inflammation and enhanced immune response in humans. Nutr. Metab. 7, 10. doi: 10.1186/1743-7075-7-18
Plaza, M., Herrero, M., Cifuentes, A. A., Ibáez, E. (2009). Innovative natural functional ingredients from microalgae. J. Agric. Food Chem. 57 (16), 7159–7170. doi: 10.1021/jf901070g
Powls, R., Redfearn, E. R. (1967). The tocopherols of the blue-green algae. Biochem. J. 104 (2), 24C–26C. doi: 10.1042/bj1040024C
Rani, A., Saini, K. C., Bast, F., Mehariya, S., Bhatia, S. K., Lavecchia, R., et al. (2021). Microorganisms: A potential source of bioactive molecules for antioxidant applications. Molecules 26 (4), 34. doi: 10.3390/molecules26041142
Raposo, M. F. D., de Morais, R., de Morais, A. (2013). Bioactivity and applications of sulphated polysaccharides from marine microalgae. Mar. Drugs 11 (1), 233–252. doi: 10.3390/md11010233
Raposo, M. F. D., de Morais, A. M. B., de Morais, R. (2015). Marine polysaccharides from algae with potential biomedical applications. Mar. Drugs 13 (5), 2967–3028. doi: 10.3390/md13052967
Riccioni, G., D’Orazio, N., Franceschelli, S., Speranza, L. (2011). Marine carotenoids and cardiovascular risk markers. Mar. Drugs 9 (7), 1166–1175. doi: 10.3390/md9071166
Romay, C., Gonzalez, R., Ledon, N., Remirez, D., Rimbau, V. (2003). C-phycocyanin: A biliprotein with antioxidant, anti-inflammatory and neuroprotective effects. Curr. Protein Pept. Science. 4 (3), 207–216. doi: 10.2174/1389203033487216
Safafar, H., van Wagenen, J., Moller, P., Jacobsen, C. (2015). Carotenoids, phenolic compounds and tocopherols contribute to the antioxidative properties of some microalgae species grown on industrial wastewater. Mar. Drugs 13 (12), 7339–7356. doi: 10.3390/md13127069
Sanchez-Salgado, J. C., Estrada-Soto, S., Garcia-Jimenez, S., Montes, S., Gomez-Zamudio, J., Villalobos-Molina, R. (2019). Analysis of flavonoids bioactivity for cholestatic liver disease: Systematic literature search and experimental approaches. Biomolecules 9 (3), 13. doi: 10.3390/biom9030102
Sano, M., Ernesto, C., Thomas, R. G., Klauber, M. R., Schafer, K., Grundman, M., et al. (1997). A controlled trial of selegiline, alpha-tocopherol, or both as treatment for alzheimer’s disease. New Engl. J. Med. 336 (17), 1216–1222. doi: 10.1056/NEJM199704243361704
Sathasivam, R., Radhakrishnan, R., Hashem, A., Abd Allah, E. F. (2019). Microalgae metabolites: A rich source for food and medicine. Saudi J. Biol. Sci. 26 (4), 709–722. doi: 10.1016/j.sjbs.2017.11.003
Shah, M. R., Liang, Y., Cheng, J. J., Daroch, M. (2016). Astaxanthin-producing green microalga Haematococcus pluvialis: From single cell to high value commercial products. Front. Plant Sci. 7. doi: 10.3389/fpls.2016.00531
Stahl, W., Sies, H. (2003). Antioxidant activity of carotenoids. Mol. Aspects Med. 24 (6), 345–351. doi: 10.1016/s0098-2997(03)00030-x
Sun, L. Q., Wang, C., Shi, Q. J., Ma, C. H. (2009). Preparation of different molecular weight polysaccharides from porphyridium cruentum and their antioxidant activities. Int. J. Biol. Macromolecules 45 (1), 42–47. doi: 10.1016/j.ijbiomac.2009.03.013
Sun, Y. Q., Wu, S. M., Gong, G. Y. (2019). Trends of research on polycyclic aromatic hydrocarbons in food: A 20-year perspective from 1997 to 2017. Trends Food Sci. Technol. 83, 86–98. doi: 10.1016/j.tifs.2018.11.015
Tannin-Spitz, T., Bergman, M., van-Moppes, D., Grossman, S., Arad, S. (2005). Antioxidant activity of the polysaccharide of the red microalga porphyridium sp. J. Appl. Phycol 17 (3), 215–222. doi: 10.1007/s10811-005-0679-7
Tao, S. Y., Yang, D. S., Zhang, L. X., Yu, L. T., Wang, Z. H., Li, L. L., et al. (2022). Knowledge domain and emerging trends in diabetic cardiomyopathy: A scientometric review based on CiteSpace analysis. Front. Cardiovasc. Med. 9. doi: 10.3389/fcvm.2022.891428
Thannickal, V. J., Fanburg, B. L. (2000). Reactive oxygen species in cell signaling. Am. J. Physiol. Lung Cell. Mol. Physiol. 279 (6), L1005–L1028. doi: 10.1152/ajplung.2000.279.6.L1005
Uma, V. S., Usmani, Z., Sharma, M., Diwan, D., Sharma, M., Guo, M., et al. (2022). Valorisation of algal biomass to value-added metabolites: emerging trends and opportunities. Phytochem. Rev. 2022 (2), 1–26. doi: 10.1007/s11101-022-09805-4
Ursu, A. V., Marcati, A., Sayd, T., Sante-Lhoutellier, V., Djelveh, G., Michaud, P. (2014). Extraction, fractionation and functional properties of proteins from the microalgae chlorella vulgaris. Bioresource Technol. 157, 134–139. doi: 10.1016/j.biortech.2014.01.071
Valko, M., Leibfritz, D., Moncol, J., Cronin, M. T. D., Mazur, M., Telser, J. (2007). Free radicals and antioxidants in normal physiological functions and human disease. Int. J. Biochem. Cell Biol. 39 (1), 44–84. doi: 10.1016/j.biocel.2006.07.001
Valko, M., Rhodes, C. J., Moncol, J., Izakovic, M., Mazur, M. (2006). Free radicals, metals and antioxidants in oxidative stress-induced cancer. Chemico-Biol Interact. 160 (1), 1–40. doi: 10.1016/j.cbi.2005.12.009
van Eck, N. J., Waltman, L. (2010). Software survey: VOSviewer, a computer program for bibliometric mapping. Scientometrics 84 (2), 523–538. doi: 10.1007/s11192-009-0146-3
Varela, J. C., Pereira, H., Vila, M., Leon, R. (2015). Production of carotenoids by microalgae: achievements and challenges. Photosynthesis Res. 125 (3), 423–436. doi: 10.1007/s11120-015-0149-2
Vieira, I. R. S., de Carvalho, A., Conte-Junior, C. A. (2022). Recent advances in biobased and biodegradable polymer nanocomposites, nanoparticles, and natural antioxidants for antibacterial and antioxidant food packaging applications. Compr. Rev. Food Sci. Food Saf. 21 (4), 3673–3716. doi: 10.1111/1541-4337.12990
Vismara, R., Vestri, S., Kusmic, C., Barsanti, L., Gualtieri, P. (2003). Natural vitamin e enrichment of artemia salina fed freshwater and marine microalgae. J. Appl. Phycol 15 (1), 75–80. doi: 10.1023/A:1022942705496
Wan, M. X., Hou, D. M., Li, Y. G., Fan, J. H., Huang, J. K., Liang, S. T., et al. (2014). The effective photoinduction of haematococcus pluvialis for accumulating astaxanthin with attached cultivation. Bioresource Technol. 163, 26–32. doi: 10.1016/j.biortech.2014.04.017
Wang, X., Hong, Y., Gu, Z., Cheng, L., Li, Z., Li, C., et al. (2022). Themes, trends, and knowledge structure in 30 years of starch research in food science and technology: a visualization review. Staürke = 74 (5-6), e2100274. doi: 10.1002/star.202100274
Waris, G., Ahsan, H. (2006). Reactive oxygen species: Role in the development of cancer and various chronic conditions. J. Carcinogenesis 5 (14), 14. doi: 10.1186/1477-3163-5-14
Wells, M. L., Potin, P., Craigie, J. S., Raven, J. A., Merchant, S. S., Helliwell, K. E., et al. (2017). Algae as nutritional and functional food sources: revisiting our understanding. J. Appl. Phycol 29 (2), 949–982. doi: 10.1007/s10811-016-0974-5
Xia, S., Duan, J. (2022). Mapping knowledge domains on managerial overconfidence. Heliyon 8 (12), e11823. doi: 10.1016/j.heliyon.2022.e11823
Yarkent, C., Gurlek, C., Oncel, S. S. (2020). Potential of microalgal compounds in trending natural cosmetics: A review. Sustain. Chem. Pharm. 17, 11. doi: 10.1016/j.scp.2020.100304
Yu, D. J., Xu, Z. S., Pedrycz, W., Wang, W. R. (2017). Information sciences 1968-2016: A retrospective analysis with text mining and bibliometric. Inf. Sci. 418, 619–634. doi: 10.1016/j.ins.2017.08.031
Keywords: microalgae, antioxidants, bioactive substances, bibliometrics, citespace
Citation: Yang N, Zhang Q, Chen J, Wu S, Chen R, Yao L, Li B, Liu X, Zhang R and Zhang Z (2023) Study on bioactive compounds of microalgae as antioxidants in a bibliometric analysis and visualization perspective. Front. Plant Sci. 14:1144326. doi: 10.3389/fpls.2023.1144326
Received: 14 January 2023; Accepted: 09 March 2023;
Published: 28 March 2023.
Edited by:
Ben Lucker, Prosel Biosciences, United StatesReviewed by:
Ali Parsaeimehr, Delaware State University, United StatesCopyright © 2023 Yang, Zhang, Chen, Wu, Chen, Yao, Li, Liu, Zhang and Zhang. This is an open-access article distributed under the terms of the Creative Commons Attribution License (CC BY). The use, distribution or reproduction in other forums is permitted, provided the original author(s) and the copyright owner(s) are credited and that the original publication in this journal is cited, in accordance with accepted academic practice. No use, distribution or reproduction is permitted which does not comply with these terms.
*Correspondence: Rongqing Zhang, cnF6aGFuZ0BtYWlsLnRzaW5naHVhLmVkdS5jbg==; Zhen Zhang, emhhbmd6aGVuQHRzaW5naHVhLXpqLmVkdS5jbg==
Disclaimer: All claims expressed in this article are solely those of the authors and do not necessarily represent those of their affiliated organizations, or those of the publisher, the editors and the reviewers. Any product that may be evaluated in this article or claim that may be made by its manufacturer is not guaranteed or endorsed by the publisher.
Research integrity at Frontiers
Learn more about the work of our research integrity team to safeguard the quality of each article we publish.