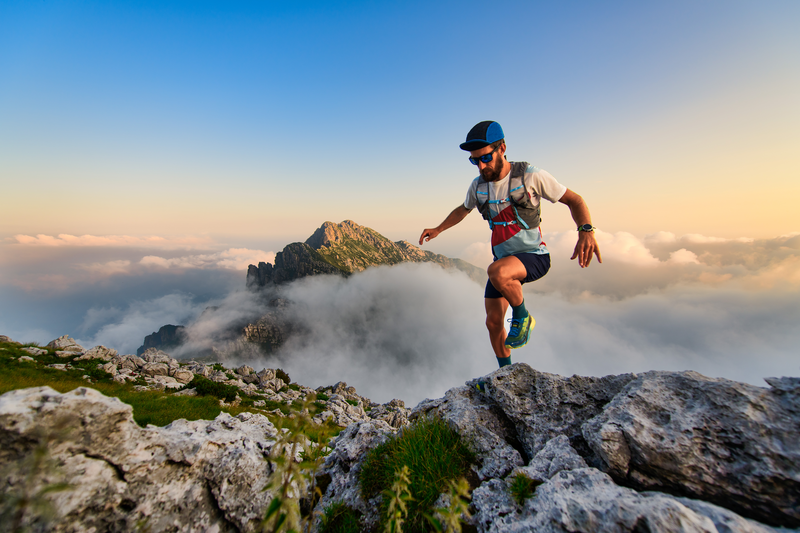
95% of researchers rate our articles as excellent or good
Learn more about the work of our research integrity team to safeguard the quality of each article we publish.
Find out more
ORIGINAL RESEARCH article
Front. Plant Sci. , 20 March 2023
Sec. Plant Pathogen Interactions
Volume 14 - 2023 | https://doi.org/10.3389/fpls.2023.1143171
This article is part of the Research Topic Advances in Integrated Disease Management (IDM) For Soil-Borne Plant Pathogens: Innovative Approaches and Underlying Action Mechanism at Molecular Level View all 13 articles
Root-knot nematode (RKN) is a major factor that limits the growth and productivity of important Cucumis crops, such as cucumber and melon, which lack RKN-resistance genes in their genome. Cucumis metuliferus is a wild Cucumis species that displays a high degree of RKN-resistance. WRKY transcription factors were involved in plant response to biotic stresses. However, little is known on the function of WRKY genes in response to RKN infection in Cucumis crops. In this study, Cucumis metuliferus 60 WRKY genes (CmWRKY) were identified in the C. metuliferus genome, and their conserved domains were classified into three main groups based on multiple sequence alignment and phylogenetic analysis. Synteny analysis indicated that the WRKY genes were highly conserved in Cucumis crops. Transcriptome data from of C. metuliferus roots inoculated with RKN revealed that 16 CmWRKY genes showed differential expression, of which 13 genes were upregulated and three genes were downregulated, indicating that these CmWRKY genes are important to C. metuliferus response to RKN infection. Two differentially expression CmWRKY genes (CmWRKY10 and CmWRKY28) were selected for further functional analysis. Both CmWRKY genes were localized in nucleus, indicating they may play roles in transcriptional regulation. This study provides a foundation for further research on the function of CmWRKY genes in RKN stress resistance and elucidation of the regulatory mechanism.
In plant, WRKY genes belong to one of large plant transcription factors family that is widely involved in various physiological processes (Goyal et al., 2022). WRKY proteins contain one or two highly conserved WRKY domains that contain approximately 60 amino acid residues. The WRKY domain is located in the C-terminal region and includes a highly conserved WRKYGQK motif followed by a zinc finger motif (Kazuhiko et al., 2005). According to the number of WRKY domains and the structure of the zinc finger motif, WRKY proteins were grouped into three groups (1, 2 and 3) (Zhang and Wang, 2005). Group 1 proteins contain an N-terminal and a C-terminal WRKY domains with C2H2 motif. Group 2 proteins contain one WRKY domain but can be classified into five subgroups (2a-2e) according to C2H2 zinc-finger motif structure (Grzechowiak et al., 2022). Group 3 proteins also have one WRKY domain, but the structure of zinc-finger is C2-H-C (Ning et al., 2017). With the release of many plant genomes, whole-genome identification of WRKY genes has been reported in many plant species, and the number of WRKY family members in a species varies from dozens to hundreds (He et al., 2016; Liu et al., 2017; Karkute et al., 2018; Li et al., 2018; Chen et al., 2020; Wei et al., 2022; Zhang et al., 2022; Pan et al., 2023; Ren et al., 2023).
WRKY transcription factors are extensively involved in plant development and growth, and abiotic and biotic stresses responses by binding to the conserved motif in the promoter of their target genes (Duan et al., 2007). One focus of research on WRKY genes is their roles in regulating plant defense against biotic stress (Wani et al., 2021). WRKY genes play important roles in the plant response to diverse pathogens, including bacterial pathogens (Kim et al., 2008; Vo et al., 2018), fungal pathogens (Zheng et al., 2006) and nematodes (Chinnapandi et al., 2017). A function of WRKY genes is to regulate PAMP (pathogen-associated molecular pattern) triggered immunity (PTI) and effector-triggered immunity (ETI). WRKY transcription factors play important roles in the pathways that can activate PTI and ETI (Eulgem, 2006; Jones and Dangl, 2006; Eulgem and Somssich, 2007). In addition, WRKY transcription factors can regulate plant hormone signaling and involved in biotic stress responses. Plant WRKY genes can response to various plant hormones including ethylene, jasmonate, salicylic acid (SA), gibberellin and abscisic acid (Dai et al., 1999; Zhang et al., 2009; Grunewald et al., 2012). WRKY genes can regulate plant biotic defense mechanisms through other pathways. For example, WRKY transcription factors are responsive to regulate biosynthesis of lignin, phosphate acquisition, and other secondary metabolites (Wang et al., 2010; Schluttenhofer and Yuan, 2015; Yang et al., 2016).
Previous studies have shown that WRKY genes play important roles in plant-cyst nematode interactions. Grunewald et al. (2008) reported that knock down WRKY23 expression can reduces infection by the cyst nematode (Grunewald et al., 2008). Yang et al. (2017) reported that overexpression of three WRKY transgenes increases resistance to the cyst nematode (Yang et al., 2017). Huang et al. (2022) conducted soybean RNA-seq transcriptome sequencing of soybean for compatible and incompatible reactions to cyst nematode and observed that interactions between cyst nematode infection and WRKY genes were activated (Huang et al., 2022). An increasing number of studies have shown that WRKY genes also are involved in plant-root knot nematode (RKN) interaction. The tomato WRKY gene SlWRKY45 is a RKN-responsive gene that enhances susceptibility to RKN (Chinnapandi et al., 2017). Tomato SlWRKY3 can activate defense-signaling pathways associated with lipids and hormone-mediated pathway and acts as a positive regulator for resistance to Meloidogyne javanica (Chinnapandi et al., 2019). Ribeiro et al. (2022) reported that protease inhibitors and nucleotide-binding site leucine-rich repeat WRKY genes are essential for resistance in cowpea (Ribeiro et al., 2022).
Cucumis genus contains two important crops: melon and cucumber. Infections by RKN species cause heavy losses of cucumber and melon. RKN-infections seriously restricts the production and quality of cultivated cucumber and melon, due to the genome lack RKN-resistance genes (Guan et al., 2014). Cucumis metuliferus is considered to be a semi-wild or wild relative of melon and cucumber (Weng, 2010). Although cucumber and melon are highly susceptible to RKN infection, several C. metuliferus accessions are highly resistant to RKN species, including Meloidogyne javanica and Meloidogyne incognita (Walters et al., 2006; Ling et al., 2021). However, few genes involved in RKN-resistance in C. metuliferus have been reported. Given the lack of RKN-resistance genes in the melon and cucumber genomes, the identification of genes associated with RKN resistance in their wild relative C. metuliferus is of great importance. In this study, we identified 60 CmWRKY genes and classified them into three groups. Comparative genome analysis indicated that WRKY genes are highly conserved in C. metuliferus, cucumber and melon. In addition, the expression profile of CmWRKY genes responsive at early stage of RKN infection was investigated. The results will provide a clue for further research on the WRKY genes that regulate RKN-resistance in Cucumis crops.
The genome data of C. metuliferus were downloaded from CuGenDB (Zheng et al., 2019). HMMER program was used to search candidate WRKY genes using the WRKY domains (PF03106.7) and the e-valve was set to 1e-10 (http://hmmer.org/). The SMART software (http://smart.embl.de/) was used to prove WRKY domains in the candidate genes.
The WRKY domains of CmWRKY proteins and seven selected Arabidopsis WRKY (AtWRKY) proteins were used to create multiple protein sequence alignments using ClustalW2 (https://www.ebi.ac.uk/Tools/msa/clustalw2/) with default settings. The conserved motifs of CmWRKY proteins were identified using MEME software (http://meme-suite.org/) with setting –nmotifs to 10. MEGA11 (Tamura et al., 2021) was used to construct phylogenetic trees for WRKY domains. Bootstrap analysis was performed with 1000 replications to evaluate support rate.
The locations of the CmWRKYs were extracted from the C. metuliferus genome gff3 annotation files and a custom Python script was used to display the distribution of CmWRKYs on the chromosomes. MCScanX was used to detect the duplication events of CmWRKYs. The syntenic analysis among C. metuliferus, cucumber and melon were constructed using Bioconda JCVI package (Tang et al., 2008). The WRKY genes of melon and cucumber were obtained from CuGenDB (Zheng et al., 2019).
The RKN-resistant C. metuliferus were planted in greenhouse with temperature of 25°C (day) and 18°C (night). Meloidogyne incognita was maintained on susceptible water spinach in a glasshouse at 22–26°C. J2 juveniles of Meloidogyne incognita were used as inoculate. J2 juveniles were resuspended in dH2O, and the concentration of Meloidogyne incognita used for inoculation was 1,000/mL.
For RNA-seq, Cm3 roots inoculated with Meloidogyne incognita were used as treatment and inoculated with dH2O were used as control. Two time points (3 day after inoculate (3DAI) and 7DAI) were selected to analysis CmWRKYs expression profiling at early stage of Meloidogyne incognita infection. Cm3 roots were used to RNA-seq (Hiseq 2500) and performed on three independent biological replicates for each time point. The RNA-seq data processing and DEGs analysis were carried out using salmon pipeline (Patro et al., 2017) and DESeq2 (Love et al., 2014). By comparing the treatment with control at the same time point, the genes were identified as DEGs with adjusted P<0.01 and log2FoldChange >1.
We performed real-time RT-PCR (Qrt-PCR) using cfx96 real time system (Bio-Rad, USA). The PCR program was set to initial denaturation at 94°C for 3 minutes followed by 35 cycles of denaturation at 94°C for 30 s, annealing at 55 or 60°C for 30 s and extension at 72°C for 30s. The RT-PCR experiments were carried out at least three biological replicates. Besides of 3DAI and 7DAI samples, other two time points (14 DAI and 28DAI) were added in qRT-PCR experiment, which were late stage of Mi infection. The analysis of relative gene expression data were carried out according to Ling et al (Walters et al., 2006).
The CmWRKY10 and CmWRKY28 CDS sequence were cloned into the super1300-GFP vector to generate super1300- CmWRKY10-GFP and super1300- CmWRKY28-GFP. These vectors were used to transform Agrobacterium tumefaciens GV3101. Nicotiana benthamiana were grown for 4-week-old and infiltrated with transformed A. tumefaciens GV3101 through in infiltration buffer (10 mM MgCl2, 10 mM MES, and 0.1 mM acetosyringone) to an OD600 of 0.5. Leaves were imaged 48h after inoculation; images were acquired by an inverted confocal microscope (Zeiss LSM700, Germany) at 488 nm for GFP.
To identify WRKY family genes in the C. metuliferus genome, WRKY genes of cucumber and melon were used to search against the C. metuliferus genome. The homologous proteins were identified as candidate CmWRKY genes using HMMER and SMART software. Finally, 60 CmWRKY genes in C. metuliferus genome were identified. These genes were predicted to contain WRKY domains based on search of the Pfam and SMART databases. All CmWRKY genes can be mapped to 12 chromosomes of C. metuliferus. Accordingly, we renamed the CmWRKY genes from CmWRKY1 to CmWRKY60 according to their location order from chromosome 1 to 12 (Figure 1A; Table S1). The distribution of CmWRKY genes on the chromosomes was not evenly, with chr06 containing the highest number of CmWRKY members (10), followed by chr07 (7) and chr08 (7), whereas chr05 contained only one CmWRKY gene. In order to conduct a comparative analysis among Cucumis genus, we mapped 60 cucumber WRKY genes (CsWRKY) and 58 melon WRKY genes (MeWRKY) to their chromosomes, respectively (Tables S2, S3, Figures S1, S2). It was noted that no WRKY gene cluster was detected in their genomes, indicating the lack of tandem replication events of WRKY genes loci in Cucumis species.
Figure 1 Mapping of the WRKY genes to C. metuliferus chromosomes (A) and alignment of amino acid sequences for selected CmWRKY and AtWRKY domain (B). (A) to simplify the presentation, we renamed the putative WRKY genes from CmWKRY1 to CmWRKY60 based on their order on the chromosomes. (B) alignment was performed using Clustal W. The suffix ‘N’ or ‘C’ indicates the N-terminal WRKY domain or the C-terminal WRKY domain, respectively. The conserved WRKY amino acid signature is highlighted in different colors, and gaps are marked with dots.
Multi-sequence alignment analysis of CmWRKY proteins was conducted using the WRKY domains. According to the group or subgroup information of Arabidopsis WRKY (AtWRKY), seven AtWRKY domains were randomly selected as group or subgroup representatives for the analysis. As shown in Figure 1B and Figure S3, the highly conserved amino acid sequence WRKYGQK was detected within 57 CmWRKY proteins, whereas the remaining three CmWRKY proteins (CmWRKY14, CmWRKY42, and CmWRKY55) had a WRKYGKK motif sequence. To understand the phylogenetic relationships of CmWRKY proteins, a phylogenetic tree for CmWRKY and AtWRKY WRKY domains was constructed. As shown in Figure 2, the CmWRKY genes were grouped into three large groups (Groups 1-3). Among the 60 CmWRKY proteins, 12 were assigned to group 1, 43 belonged to group 2, and five were assigned to group 3. The phylogenetic tree of the CmWRKY domains indicated that the group 2 CmWRKY proteins can be further grouped into five distinct subgroups (a-e). In addition, we also constructed a phylogenetic tree using WRKY gene sequences from C. metuliferus, cucumber (CsWRKY) and melon (MeWRKY). The number of WRKY gene family members in the three Cucumis crops genomes was almost identical, and the classification of the genes was similar, indicating that WRKY genes are highly conserved in these Cucumis crops (Figure S4).
Figure 2 Phylogenetic tree representing relationships among WRKY domains of Cucumis metuliferus and Arabidopsis thaliana. The amino acid sequences of the WRKY domains of all CmWRKY and AtWRKY proteins were aligned with Clustal W. The phylogenetic tree was constructed using the neighbor-joining method in MEGA. The red arcs indicate different groups (or subgroups) of WRKY domains. Colored circlesrepresent putative orthologs from C. metuliferus (green) and Arabidopsis (red).
To detect the CmWRKY gene structure variations, the intron and exon structure compositions of CmWRKY were analyzed. As shown in Figure 3, among the coding sequences of CmWRKY genes, 27 (approximately 45%) had 3 exons, 10 CmWRKY genes contained 4 exons, and one WRKY gene (CmWRKY18) had the highest number (8) of exons. The average exon number for per CmWRKY gene is 3.5, while the average number of CmWRKY gene in some groups were significantly higher than the overall average number, with 5.6 for group 2b, 4.9 for group 1 and 4.5 for group2a members.
Figure 3 The gene structure and conserved motifs of CmWRKY genes. Left panel: the phylogenetic tree of CmWRKY domain. Right panel: the gene structure and conserved motifs. The exons, UTR and introns are indicated by yellow boxes, black lines and blue boxs, respectively.The motifs are represented by different colored boxes from number 1-10.
To evaluate the structural diversification and conservation of CmWRKYs, the different motifs of all CmWRKY proteins were identified using MEME. As shown in Figure 3, motif1, motif2 and motif3 were the most conserved motifs, and motif1 is shared by all CmWRKYs, while motif9 is the rarest which is shared by only 5 members(CmWRKY2,10,18,29 and 57) of group 2b (Table S4). Each member of CmWRKYs family contains 3.6 motifs on average, while group 2b members contain the largest number of motifs (6), followed by group 1 CmWRKYs(4.4) and group 2e (4). It is noted that, in the same phylogenetic clade, the motifs of CmWRKY proteins exhibited similar compositions and distributions, suggesting that CmWRKY genes of the same clade may be genes with similar functions.
To further explore the expansion and evolution of CmWRKY genes, the gene duplication events within the CmWRKY gene family were analyzed by conducting a genome synteny analysis. Thirteen recent duplication events involving 23 CmWRKY genes were detected (Figure 4A). In contrast, tandem duplication events were not observed among CmWRKY genes. These results indicated that some CmWRKY genes were possibly derived from genome segmental duplication events and segmental duplication events play an important role in the evolution of CmWRKY genes.
Figure 4 (A) Schematic representations of inter-chromosomal relationships of CmWRKY genes. Gray lines indicate synteny blocks in the Cucumis metuliferus genome, duplicated WRKY gene pairs are connected with red lines. (B) Synteny analysis of WRKY genes among Cucumis metuliferus, cucumber and melon. Gray lines in the background indicate the collinear blocks within these three Cucumis crops, and the red lines highlight the syntenic WRKY gene pairs. Cm represents C. metuliferus.
To examine the potential evolutionary relationships among CmWRKY genes, CmWRKY, CsWRKY and MeWRKY genes were used to conduct comparative syntenic analysis. Fifty-three CmWRKY genes and 52 melon WRKY genes showed syntenic relationships with CsWRKY genes, respectively (Figure 4B). A total of 48 syntenic relationships were detected among C. metuliferus, cucumber and melon WRKY genes, suggesting that most WRKY genes in the three genomes were conserved. Combing syntenic and phylogenetic analyses, all WRKY genes in these three Cucumis genomes displayed orthologous or paralogous gene relationships. No species-specific WRKY genes were detected, indicating that no new WRKY genes had evolved among Cucumis crops after their divergence from Citrullus genus.
Transcriptome data from C. metuliferus roots inoculated with M. incognita at an early stage of infection (3 and 7 days after infection (DAI) were used to detect the expression profile of CmWRKY genes in response to RKN infection. A total of 16 CmWRKY genes displayed a differential expression pattern at corresponding time points (3 or 7DAI), of which 13 CmWRKY genes were significantly upregulated, and three CmWRKY (CmWRKY10, 52 and 59) genes were significantly downregulated (p<0.01) (Figure 5). The expression of these differentially expressed genes (DEGs) were further verified by real-time PCR (RT-PCR). The results of RT-PCR were basically consistent with the RNA-sequencing (RNA-seq) (Figure 6; Table S5). However, in some instances, the differential expression in the RNA-seq data was significantly higher than that observed by RT-PCR. For example, at 3 DAI, the RNA-seq data showed indicated that CmWRKY28 expression was 24 times higher compared with that of the control, whereas RT-PCR indicated that expression increased only eight times. It was noted that all DEGs did not show differential expression at 14 and 28 DAI, indicating that these WRKY DEGs only respond to RKN infection at an early stage of infection.
Figure 5 Expression pattern of CmWRKY genes in roots of Cucumis metuliferus in response to Meloidogyne incognita inoculation. The transcript levels of CmWRKY genes at 3 and 7DAI were investigated based on transcriptome data. The expression level of CmWRKY genes is shown as a heatmap using TPM value (log2). 3D-CK and 7D-CK represent the control of at 3DAI and 7DAI, respectively. The color scale shows increasing expression levels from red to blue.
Figure 6 Real-time PCR detection of the expression patterns of 16 CmWRKY genes in roots of Cucumis metuliferus response to Meloidogyne incognita inoculation. The relative expression (y-axis) was calculated in accordance with the description in the Methods. The time points 0d, 3d, 7d, 14d, and 28d (x-axis) indicate the time(days) after inoculation. The error bars were calculated based on three replicates. Stars indicate a significant differences compared with the control (0d) (*p<0.05, **p<0.01).
In this study, we selected the downregulated CmWRKY10 and the upregulated CmWRKY28 to investigate WRKY gene function in response to RKN infection (Figure 7A). CmWRKY10 and CmWRKY28 proteins were predicted to be localized nucleus, using protein PredicProtein software. To verify prediction for two CmWRKY proteins, the fusion GFP proteins vectors super1300:-CmWRKY10-GFP and super1300:-CmWRKY28-GFP were transiently expressed in N. benthamiana leaves. The signal from the green fluorescent protein (GFP) signal was observed with a laser-scanning confocal microscopy. The epidermal cells from leaves expressing the respective CmWRKY fusion protein were only detected in the nucleus. In contrast, super1300: GFP was detected in the cytoplasm and the nucleus (Figure 7B). These data suggested that the CmWRKY10 and CmWRKY28 proteins were nuclear localized proteins, which was consistent with the forementioned predictions. The two CmWRKY transcription factors may play roles in the response of C. metuliferus to RKN infection.
Figure 7 Subcellular localization analysis of CmWRKY10 and CmWRKY28 genes in tobacco leaf cells. (A) Schematic diagram of the control (super1300:GFP) and super1300:CmWRKYs-GFP fusion protein. (B) Transient expression of super1300: GFP and super1300:CmWRKYs-GFP in the tobacco leaf. A 48 h of after inoculation, green fluorescence signal was observed by laser confocal fluorescence microscopy.
There are several economically important crops in the Cucumis genus, such as melon and cucumber. Owing to the lack of RKN-resistance genes in the cucumber and melon genomes, RKN seriously restricts the yield and quality of cultivated melon and cucumber (Weng, 2010). C. metuliferus is a wild relative of cucumber and melon that shows a high degree of resistance to RKN (Walters et al., 2006). The resistance of C. metuliferus to RKN is attained through inhibition of the development and growth of RKN at the J2 stage (Ling et al., 2021). However, the resistance mechanism of C. metuliferus remains unclear, and the genes involved in the interactions between C. metuliferus and RKN have not been identified. With publication of the C. metuliferus genome (Ling et al., 2017), it is feasible to identify important pathogen-resistance gene using the C. metuliferus genomic data. WRKY genes are important transcription factors family that are extensively involved in plant response to biological stress. In this study, we identified 60 CmWRKY genes in the C. metuliferus genomes. The number of CmWRKY genes is similar to that in the genomes of cucumber (60) and melon (58). It was noted that the member number of WRKY genes in C. metuliferus, cucumber, and melon is significantly lower than that of Arabidopsis (72) and rice (101) (Abdullah-Zawawi et al., 2021). No genome-wide duplication events were detected in the reported genomes of C. metuliferus, cucumber and melon. This can be attributed to lack of segmental duplication and tandem duplication events in the genomes of Cucumis crops genome (Ling et al., 2011; Chen et al., 2020). Genome duplication is a source of new genes that assume novel functions during evolution (Lawton-Rauh, 2003). Given the lack of genome duplication events, some new WRKY genes with new functions may be absent in Cucumis crops compared with other plants, such as Arabidopsis and rice. In the present study, we observed that WRKY genes were highly conserved in C. metuliferus, cucumber and melon. Most of the WRKY genes (48) displayed a syntenic relationship and no species-specific WRKY genes were detected in any of genomes. Taken together, these results indicated that the WRKY genes in Cucumis might retain the functions of the ancestral WRKY genes in plants.
Ling reported that NBS-LRR genes, which are an important pathogen-resistance genes, comprise more members (104) in C. metuliferus compared with those in cucumber (66) and melon (67) (Ling et al., 2017). Insertion/deletion (indel) events in the Cucumis genome have deleted many NBS-LRR genes in cucumber and melon. However, we did not detect indel events in the WRKY gene loci in the three genomes. Unlike NBS-LRR genes, WRKY genes not only function in disease and pathogen resistance, but also participate widely in various important physiological activities of plants. Thus, the deletion of WRKY genes may severely affect the physiology of plants. The number of WRKY gene members in C. metuliferus, cucumber and melon remained essentially unchanged.
Recent studies have revealed that WRKY genes are involved in plant-nematode interactions (Chinnapandi et al., 2019; Ribeiro et al., 2022). Most of this research has focused on tomato and soybean. The response of WRKY genes to nematode infection in cucurbitaceous crops has not been reported previously. A previous transcriptomic study of C. metuliferus roots infected by RKN has showed that plant hormone-related genes are involved in the response to RKN infection and that cytoskeleton-related genes are crucial regulators of C. metuliferus resistance to RKN (Ling et al., 2011). However, this study did not report on whether CmWRKY genes responded to nematode infection. In the present study, we analyzed the response of WRKY genes at an early stage of RKN infection, and identified 16 differentially expressed WRKY genes, of which 13 were upregulated and three were downregulated. The present expression analysis showed that CmWRKY genes are involved in the early response of the plant to nematode infection. We identified the orthologous genes of CmWRKY10 and CmWRKY28 in cucumber, which corresponds to Csav3_3g021980 and CsaV3_1G002180, respectively. The expression profiles of the two CsWRKY genes under RKN treatment were investigate, and no significantly differential expression can be detected for the two genes under RKN treatment in 3DAI and 7DAI (Figure S5). The results indicated that the differential expression of CmWRKY genes was related to nematode resistance. This is the first report on the response of WRKY genes to nematode in a cucurbitaceous crop. Therefore, this research enhances our understanding of WRKY gene regulation of plant resistance to RKN. However, the downstream target genes regulated by WRKY genes need to be identified to further clarify the anti-nematode function of WRKY genes.
Due to lack of root knot nematode (RKN) resistant genes in their genome, two important Cucumis crops melon and cucumber are suffered from serious RKN disease. Cucumis metuliferus is a wild Cucumis species, which displays a high level of RKN-resistance. WRKY transcription factors were involved in plant response to biotic stresses. In this study, we identified a total of 60 WRKY genes in Cucumis metuliferus genome. Syntonic analysis indicated that WRKY genes are highly conserved in Cucumis metuliferus, cucumber and melon. The expression patterns of CmWRKY genes under RKN stress treatment were also investigated, and 16 CmWRKY genes displayed differential expression at early stage of RKN infection were identified, in which two selected CmWRKY genes were located on nuclear by subcellular localization analysis. This is first report on WRKY genes expression profile under RKN stress in Cucumis crops. This study provided clues for further research on CmWRKY function in RKN-resist mechanism. Analysis of RKN-responding gene in RKN-resist Cucumis metuliferus can also provide clues to explore resistant genes in RKN-sensitive cucumber and melon.
The datasets presented in this study can be found in online repositories. The names of the repository/repositories and accession number(s) can be found below: https://ngdc.cncb.ac.cn/, PRJNA330972.
JL, JZ and ZM conceived the project, XP and YH contributed to WRKY gene family analysis, RL and QY contributed to functional experiment, YY, XL and BX contributed to experiment design. All authors contributed to the article and approved the submitted version.
This research is funded by Inner Mongolia Science and Technology Plan Project(2020GG0110), Central Public-interest Scientific Institution Basal Research Fund (No.IVF-BRF2022013), Key Laboratory of Biology and Genetic Improvement of Horticultural Crops, Ministry of Agriculture, P.R. China (CARS-25), the Science and Technology Innovation Program of the Chinese Academy of Agricultural Sciences (CAAS-ASTIP-2017-IVF).
The authors thank Robert McKenzie, PhD for editing this manuscript, and we also thank three reviewers for their effort to help us improving our manuscript.
The authors declare that the research was conducted in the absence of any commercial or financial relationships that could be construed as a potential conflict of interest.
All claims expressed in this article are solely those of the authors and do not necessarily represent those of their affiliated organizations, or those of the publisher, the editors and the reviewers. Any product that may be evaluated in this article, or claim that may be made by its manufacturer, is not guaranteed or endorsed by the publisher.
The Supplementary Material for this article can be found online at: https://www.frontiersin.org/articles/10.3389/fpls.2023.1143171/full#supplementary-material
Abdullah-Zawawi, M. R., Ahmad-Nizammuddin, N. F., Govender, N., Harun, S., Mohd-Assaad, N., Mohamed-Hussein, Z. A. (2021). Comparative genome-wide analysis of WRKY, MADS-box and MYB transcription factor families in arabidopsis and rice. Sci. Rep. 11 (1), 19678. doi: 10.1038/s41598-021-99206-y
Chen, C., Chen, X., Han, J., Lu, W., Ren, Z. (2020). Genome-wide analysis of the WRKY gene family in the cucumber genome and tran scriptome-wide identification of WRKY transcription factors that respond to biotic and abiotic stresses. BMC Plant Biol. 20, 1–19. doi: 10.1186/s12870-020-02625-8
Chinnapandi, B., Bucki, P., Braun, M. S. (2017). SlWRKY45, nematode-responsive tomato WRKY gene, enhances susceptibility to the root knot nematode; M. javanica infection. Plant Signal Behav. 12 (12), e1356530. doi: 10.1080/15592324.2017.1356530
Chinnapandi, B., Bucki, P., Fitoussi, N., Kolomiets, M., Borrego, E., Braun, M. S. (2019). Tomato SlWRKY3 acts as a positive regulator for resistance against the root-knot nematode meloidogyne javanica by activating lipids and hormone-mediated defense-signaling pathways. Plant Signal Behav. 14 (6), 1601951. doi: 10.1080/15592324
Dai, N., Schaffer, A., Petreikov, M., Shahak, Y., Giller, Y., Ratner, K., et al. (1999). Overexpression of arabidopsis hexokinase in tomato plants inhibits growth, reduces photosynthesis, and induces rapid senescence. Plant Cell 11, 1253–1266. doi: 10.1105/tpc.11.7.1253
Duan, M. R., Nan, J., Liang, Y. H., Mao, P., Lu, L., Li, L., et al. (2007). DNA Binding mechanism revealed by high resolution crystal structure of Arabidopsis thaliana WRKY1 protein. Nucleic Acids Res. 35, 1145–1154. doi: 10.1093/nar/gkm001
Eulgem, T. (2006). Dissecting the WRKY web of plant defense regulators. PloS Pathog. 2, e126. doi: 10.1371/journal.ppat.0020126
Eulgem, T., Somssich, I. E. (2007). Networks of WRKY transcription factors in defense signaling. Curr. Opin. Plant Biol. 10, 366–371. doi: 10.1016/j.pbi.2007.04.020
Goyal, P., Devi, R., Verma, B., Hussain, S., Arora, P., Tabassum, R., et al. (2022). WRKY transcription factors: Evolution, regulation, and functional diversity in plants. Protoplasma. 260 (2), 331–348. doi: 10.1007/s00709-022-01794-7
Grunewald, W., Karimi, M., Wieczorek, K., Van, de., Cappelle, E., Wischnitzki, E., Grundler, F., et al. (2008). A role for AtWRKY23 in feeding site establishment of plant-parasitic nematodes. Plant Physiol. 148 (1), 358–368. doi: 10.1104/pp.108.119131
Grunewald, W., Smet, I. D., Lewis, D. R., Löfke, C., Jansen, L., Goeminne, G., et al. (2012). Transcription factor WRKY23 assists auxin distribution patterns during arabidopsis root development through local control on avonol biosynthesis. Proc. Natl. Acad. Sci. U.S.A. 109, 1554–1559. doi: 10.1073/pnas.1121134109
Grzechowiak, M., Ruszkowska, A., Sliwiak, J., Urbanowicz, A., Jaskolski, M., Ruszkowski, M. (2022). New aspects of DNA recognition by group II WRKY transcription factor revealed by structural and functional study of AtWRKY18 DNA binding domain. Int. J. Biol. Macromol. 213, 589–601. doi: 10.1016/j.ijbiomac.2022.05.186
Guan, W., Zhao, X., Dickson, D. W., Mendes, M. L., Thies, J. (2014). Root knot nematode resistance, yield, and fruit quality of specialty melons grafted onto cucumis metulifer. HortScience 49, 1046–1051. doi: 10.21273/HORTSCI.49.8.1046
He, Y., Mao, S., Gao, Y., Zhu, L., Wu, D., Cui, Y., et al. (2016). Genome-wide identification and expression analysis of WRKY transcription factors under multiple stresses in Brassica napus. PloS One 11, e0157558. doi: 10.1371/journal.pone.0157558
Huang, M., Jiang, Y., Qin, R., Jiang, D., Chang, D., Tian, Z., et al. (2022). Full-length transcriptional analysis of the same soybean genotype with compatible and incompatible reactions to heterodera glycines reveals nematode infection activating plant defense response. Front.Plant Sci. 13. doi: 10.3389/fpls.2022.866322
Jones, J. D., Dangl, J. L. (2006). The plant immune system. Nature 444, 323–329. doi: 10.1038/nature05286
Karkute, S. G., Gujjar, R. S., Rai, A., Akhtar, M., Singh, M., Singh, B. (2018). Genome wide expression analysis of WRKY genes in tomato (Solanum lycopersicum) under drought stress. Plant Gene 13, 8–17. doi: 10.1016/j.plgene.2017.11.002
Kazuhiko, Y., Takanori, K., Makoto, I., Masaru, T., Tomoko, Y., Takashi, Y., et al. (2005). Solution structure of an arabidopsis WRKY DNA binding domain. Plant Cell. 17 (3), 944–956. doi: 10.1105/tpc.104.026435
Kim, K. C., Lai, Z., Fan, B., Chen, Z. (2008). Arabidopsis WRKY38 and WRKY62 transcription factors interact with histone deacetylase 19 in basal defense. Plant Cell. 20 (9), 2357–2371. doi: 10.1105/tpc.107.055566
Lawton-Rauh, A. (2003). Evolutionary dynamics of duplicated genes in plants. Mol. Phylogenet Evol. 29 (3), 396–409. doi: 10.1016/j.ympev
Li, Y. M., Zhu, L., Zhu, H. Y., Song, P. Y., Guo, L. Q., Yang, L. M. (2018). Genome-wide analysis of the WRKY family genes and their responses to cold stress in watermelon. Czech J. Genet. Plant Breed 54, 168–176. doi: 10.17221/72/2017-CJGPB
Ling, J., Jiang, W., Zhang, Y., Yu, H., Mao, Z., Gu, X., et al. (2011). Genome-wide analysis of WRKY gene family in cucumis sativus. BMC Genomics 12, 471. doi: 10.1186/1471-2164-12-471
Ling, J., Mao, Z., Zhai, M., Zeng, F., Yang, Y., Xie, B. (2017). Transcriptome profiling of cucumis metuliferus infected by meloidogyne incognita provides new insights into putative defense regulatory network in cucurbitaceae. Sci. Rep. 7 (1), 3544. doi: 10.1038/s41598-017-03563-6
Ling, J., Xie, X., Gu, X., Zhao, J., Ping, X., Li, Y., et al. (2021). High-quality chromosome-level genomes of cucumis metuliferus and cucumis melo provide insight into cucumis genome evolution. Plant J. 107 (1), 136–148. doi: 10.1111/tpj.15279
Liu, Q. N., Liu, Y., Xin, Z. Z., Zhang, D. Z., Ge, B. M., Yang, R. P., et al. (2017). Genome-wide identifica tion and characterization of the WRKY gene family in potato (Solanum tuberosum). Biochem. Syst. Ecol. 71, 212–218. doi: 10.1016/j.bse.2017.02.010
Love, M. I., Huber, W., Anders, S. (2014). Moderated estimation of fold change and dispersion for RNA-seq data with DESeq2. Genome Biol. 15 (12), 550. doi: 10.1186/s13059-014-0550-8
Ning, P., Liu, C., Kang, J., Lv, J. (2017). Genome-wide analysis of WRKY transcription factors in wheat (Triticum aestivum l.) and differential expression under water deficit condition. PeerJ 4;5, e3232. doi: 10.7717/peerj.3232
Pan, H., Chen, Y., Zhao, J., Huang, J., Shu, N., Deng, H., et al. (2023). In-depth analysis of large-scale screening of WRKY members based on genome-wide identification. Front. Genet. 13. doi: 10.3389/fgene.2022.1104968
Patro, R., Duggal, G., Love, M. I., Irizarry, R. A., Kingsford, C. (2017). Salmon provides fast and bias-aware quantification of transcript expression. Nat. Methods 14 (4), 417–419. doi: 10.1038/nmeth.4197
Ren, L., Wan, W., Yin, D., Deng, X., Ma, Z., Gao, T., et al. (2023). Genome-wide analysis of WRKY transcription factor genes in toona sinensis: An insight into evolutionary characteristics and terpene synthesis. Front. Plant Sci. 13. doi: 10.3389/fpls.2022.1063850
Ribeiro, D. G., Mota, A. P. Z., Santos, I. R., Arraes, F., Grynberg, P., Fontes, W., et al. (2022). NBS-LRR-WRKY genes and protease inhibitors (PIs) seem essential for cowpea resistance to root-knot nematode. J. Proteomics. 261, 104575. doi: 10.1016/j.jprot
Schluttenhofer, C., Yuan, L. (2015). Regulation of specialized metabolism by WRKY transcription factors. Plant Physiol. 167, 295–306. doi: 10.1104/pp.114.251769
Tamura, K., Stecher, G., Kumar, S. (2021). MEGA11: Molecular evolutionary genetics analysis version 11. Mol. Biol. Evol. 38 (7), 3022–3027. doi: 10.1093/molbev/msab120
Tang, H., Bowers, J. E., Wang, X., Ming, R., Alam, M., Paterson, A. H. (2008). Synteny and collinearity in plant genomes. Science 320 (5875), 486–488. doi: 10.1126/science.1153917
Vo, K. T., Kim, C. Y., Hoang, T. V., Lee, S. K., Shirsekar, G., Seo, Y. S., et al. (2018). OsWRKY67 plays a positive role in basal and XA21-mediated resistance in rice. Front. Plant Sci. 11; 8. doi: 10.3389/fpls.2017.02220
Walters, S. A., Wehner, T. C., Daykin, M. E., Baker, R. B. (2006). Penetration rates of rootknot nematodes into cucumis sativus and c.metuliferus roots and subsequent histological changes. Nematropica 36, 231–242.
Wang, H., Avci, U., Nakashima, J., Hahn, M. G., Chen, F., Dixon, R. A. (2010). Mutation of WRKY transcription factors initiates pith secondary wall formation and increases stem biomass in dicotyledonous plants. Proc. Natl. Acad. Sci. U.S.A. 107, 22338–22343. doi: 10.1073/pnas.1016436107
Wani, S. H., Anand, S., Singh, B., Bohra, A., Joshi, R. (2021). WRKY transcription factors and plant defense responses: latest discoveries and future prospects. Plant Cell Rep. 40 (7), 1071–1085. doi: 10.1007/s00299-021-02691-8
Wei, Y. L., Jin, J. P., Liang, D., Gao, J., Li, J., Xie, Q., et al. (2022). Genome-wide identification of cymbidium sinense WRKY gene family and the importance of its group III members in response to abiotic stress. Front. Plant Sci. 13. doi: 10.3389/fpls.2022.969010
Weng, Y. (2010). Genetic diversity among cucumis metuliferus populations revealed by cucumber microsatellites. HortScience 45, 214–219. doi: 10.21273/HORTSCI.45.2.214
Yang, L., Zhao, X., Yang, F., Fan, D., Jiang, Y., Luo, K. (2016). PtrWRKY19, a novel WRKY transcription factor, contributes to the regulation of pith secondary wall formation in populus trichocarpa. Sci. Rep. 6, 18643. doi: 10.1038/srep18643
Yang, Y., Zhou, Y., Chi, Y., Fan, B., Chen, Z. (2017). Characterization of soybean WRKY gene family and identification of soybean WRKY genes that promote resistance to soybean cyst nematode. Sci. Rep. 7 (1), 17804. doi: 10.1038/s41598-017-18235-8
Zhang, Z. L., Shin, M., Zou, X., Huang, J., Ho, T. H., Shen, Q. J. (2009). A negative regulator encoded by a rice WRKY gene represses both abscisic acid and gibberellins signaling in aleurone cells. Plant Mol. Biol. 70 (1–2), 139–151. doi: 10.1007/s11103-009-9463-4
Zhang, Y., Wang, L. (2005). The WRKY transcription factor superfamily: its origin in eukaryotes and expansion in plants. BMC Evol. Biol. 5, 1–12. doi: 10.1186/1471-2148-5-1
Zhang, C., Wang, W., Wang, D., Hu, S., Zhang, Q., Wang, Z., et al. (2022). Genome-wide identification and characterization of the WRKY gene family in Scutellaria baicalensis georgi under diverse abiotic stress. Int. J. Mol. Sci. 23 (8), 4225. doi: 10.3390/ijms23084225
Zheng, Z., Qamar, S. A., Chen, Z., Mengiste, T. (2006). Arabidopsis WRKY33 transcription factor is required for resistance to necro trophic fungal pathogens. Plant J. 48 (4), 592–605. doi: 10.1111/j.1365-313X.2006.02901.x
Keywords: WRKY family, root knot nematode, cucumis crops, expression patterns, transcriptional regulation
Citation: Ling J, Liu R, Hao Y, Li Y, Ping X, Yang Q, Yang Y, Lu X, Xie B, Zhao J and Mao Z (2023) Comprehensive analysis of the WRKY gene family in Cucumis metuliferus and their expression profile in response to an early stage of root knot nematode infection. Front. Plant Sci. 14:1143171. doi: 10.3389/fpls.2023.1143171
Received: 12 January 2023; Accepted: 06 March 2023;
Published: 20 March 2023.
Edited by:
Musharaf Ahmad, University of Agriculture, Peshawar, PakistanReviewed by:
Dong-Liang Huang, Guangxi Academy of Agricultural Sciences, ChinaCopyright © 2023 Ling, Liu, Hao, Li, Ping, Yang, Yang, Lu, Xie, Zhao and Mao. This is an open-access article distributed under the terms of the Creative Commons Attribution License (CC BY). The use, distribution or reproduction in other forums is permitted, provided the original author(s) and the copyright owner(s) are credited and that the original publication in this journal is cited, in accordance with accepted academic practice. No use, distribution or reproduction is permitted which does not comply with these terms.
*Correspondence: Zhenchuan Mao, bWFvemhlbmNodWFuQGNhYXMuY24=; Jianlong Zhao, emhhb2ppYW5sb25nY2Fhc0AxNjMuY29t
†These authors have contributed equally to this work
Disclaimer: All claims expressed in this article are solely those of the authors and do not necessarily represent those of their affiliated organizations, or those of the publisher, the editors and the reviewers. Any product that may be evaluated in this article or claim that may be made by its manufacturer is not guaranteed or endorsed by the publisher.
Research integrity at Frontiers
Learn more about the work of our research integrity team to safeguard the quality of each article we publish.