- 1Tibet Plateau Walnut Industry Research Institute, College of Horticulture and Gardening, Yangtze University, Jingzhou, Hubei, China
- 2Hubei Academy of Foresty, Wuhan, China
- 3Department of Chemistry, Faculty of Science, University of Hradec Kralove, Hradec Kralove, Czechia
- 4ICAR-Central Citrus Research Institute, Nagpur, Maharashtra, India
- 5Botany and Microbiology Department, College of Science, King Saud University, Riyadh, Saudi Arabia
- 6Plant Production Department, College of Food and Agricultural Sciences, King Saud University, Riyadh, Saudi Arabia
Walnut (Juglans regia) is an important nut tree species in the world, whereas walnut trees often face inadequate phosphorus (P) levels of soil, negatively limiting its growth and yield. Arbuscular mycorrhizal fungi (AMF) can colonize walnut roots, but whether and how AMF promotes walnut growth, physiological activities, and P acquisition is unclear. The present study aimed to evaluate the effects of Diversispora spurca on plant growth, chlorophyll component concentrations, leaf gas exchange, sugar and P concentrations, and expression of purple acid phosphatase (PAP) and phosphate transporter (PT) genes in leaves of J. regia var. Liaohe 1 seedling under moderate (100 μmol/L P) and low P (1 μmol/L P) levels conditions. Three months after inoculation, the root mycorrhizal colonization rate and soil hyphal length were 45.6−53.2% and 18.7−39.9 cm/g soil, respectively, and low P treatment significantly increased both root mycorrhizal colonization rate and soil hyphal length. Low P levels inhibited plant growth (height, stem diameter, and total biomass) and leaf gas exchange (photosynthetic rate, transpiration rate and stomatal conductance), while AMF colonization significantly increased these variables at moderate and low P levels. Low P treatment limited the level of chlorophyll a, but AMF colonization did not significantly affect the level of chlorophyll components, independent on soil P levels. AMF colonization also increased leaf glucose at appropriate P levels and leaf fructose at low P levels than non-AMF treatment. AMF colonization significantly increased leaf P concentration by 21.0−26.2% than non-AMF colonization at low and moderate P levels. Low P treatment reduced the expression of leaf JrPAP10, JrPAP12, and JrPT3;2 in the inoculated plants, whereas AMF colonization up-regulated the expression of leaf JrPAP10, JrPAP12, and JrPT3;2 at moderate P levels, although AMF did not significantly alter the expression of JrPAPs and JrPTs at low P levels. It is concluded that AMF improved plant growth, leaf gas exchange, and P acquisition of walnut seedlings at different P levels, where mycorrhizal promotion of P acquisition was dominated by direct mycorrhizal involvement in P uptake at low P levels, while up-regulation of host PAPs and PTs expressions at moderate P levels.
Introduction
Phosphorus (P) is an important nutrient for plant growth and productivity. However, in the soil, P is immobilized in the form of aluminum/iron or calcium/magnesium phosphate (Pi), which prevents it from being acquired by plants. In addition, the utilization rate of Pi in agriculture is 15−20%, making crops often faced with P starvation (Malhotra et al., 2018). The use of a large amount of P fertilizers leads to the decline in soil physical and chemical properties, pollutes soil and aquatic environment, changes biodiversity, and triggering significant carbon emissions (Li et al., 2022). To deal with P starvation, plant roots absorb Pi directly from the soil by Pi transporters (PTs) or indirectly through their symbiotic arbuscular mycorrhizal fungi (AMF) (Wang et al., 2021). The inorganic Pi absorbed by the roots is loaded through the Casparian band in the xylem vessels and then transferred to the shoots via the protein, phophate 1 (Sandhu and Rouached, 2022).
Attempts have also been made to use some beneficial microorganisms to enhance plant growth under P starvation conditions, such as the AMF (Adeyemi et al., 2021). AMF is an ancient class of fungi that establish mycorrhizal symbiosis on about 80% of terrestrial plants, where AMF obtains their required sugars and fatty acids from the plant partners in exchange for the acquired P (Smith and Smith, 2011). In addition, mycorrhizal extraradical mycelium can extend beyond the Pi depletion zone in the rhizosphere to absorb Pi that cannot be obtained by plants, increase the area of root absorption area, release organic acids such as citrate and malate to dissolve organic Pi, and activate expressions of specific PT genes in both mycorrhizal roots and AMF to absorb and transfer Pi, thus enhancing plant P acquisition (Etesami et al., 2021). AMF also changes Pi absorption kinetic parameters and stimulates other microbial activities in the soil to jointly promote P absorption of host plants (Smith et al., 2015). In addition to PTs, purple acid phosphatase (PAP) genes are also involved in mycorrhizal enhancement of host P acquisition. In soybeans, overexpression of GmPAP33 resulted in increased plant P concentrations after AMF inoculation and also participated in arbuscule degradation (Li et al., 2019).
Walnut (Juglans regia L.) is an important nut tree species in the world, cultivated in Asia, North America, Europe and South America, of which China is the world’s largest walnut producer, with 7.8 million hm2 of walnut planted area and a yield of 4.806 million t in 2020 (Ma and Ning, 2021). However, the soil fertility of walnut orchards in China is relatively low, especially the Olsen-P level, resulting in low fruit yield and oil content (Zhang, 2014), which seriously limits the high yield and quality of walnut. In addition, in the United States, many walnut trees in Lake County, California also suffer from P starvation, mainly in the volcanic soil (Serr, 1960), resulting in small and thin leaves, thin and few branches, and purplish red in the petiole and leaf backside in severe cases. Some AMF populations have been found to inhabit the rhizosphere of walnuts and confer many important physiological contributions to the host walnut (Ma et al., 2021). Mao et al. (2022) observed that the AMF colonization rate of walnut roots in Yunnan, China ranged from 75.67% to 84.37%, along with the genus Acaulospora, Diversispora, Funneliformis, Glomus, Rhizophagus, Scuteiiospora, Sclerocystis, and Septoglomus recorded in the rhizosphere. And, further inoculation revealed that these native AMF species significantly increased leaf and root P content (Mao et al., 2022). In walnut seedlings inoculated with five AMF species, Huang et al. (2020) observed improved plant growth, coupled with the best effect on D. spurca. Mycorrhizal walnut seedlings recorded higher mineral element concentrations, including P than non-mycorrhizal seedlings, regardless of soil water regimes (Behrooz et al., 2019). This shows the potential value of AMF for P acquisition by walnut trees. However, it is not clear whether and how the efficient AMF strain D. spurca affects P acquisition, leaf gas exchange, and sugar accumulation in walnut plants under soil P-deficient and P-sufficient conditions.
The purpose of this study was to analyze the effects of an efficient AMF strain (D. spurca) on growth and leaf P concentrations, gas exchange, chlorophyll component concentrations, sugar accumulation, and PAP and PT gene expressions in walnut seedlings grown in 1 and 100 μmol/L P levels.
Materials and methods
Plant culture
The seeds of walnut variety Liaohe No. 1 were provided by the Walnut Technology Extension Center of Baokang (Hubei, China). The seeds were surface disinfected with 75% ethanol for 8 min, washed with distilled water, soaked in distilled water for a week, and then sown in autoclaved sands for the germination in an incubator at 28°C/20°C (day and night temperature) and 80% relative humidity. The seedlings with four leaves were transplanted into plastic pots (2.4 L) pre-filled with hydrochloric acid-washing sand to reduce the interference of P in the substrate.
Based on the results of Huang et al. (2020), we selected the D. spurca strain as the fungal material because it showed relatively good effects on improving walnut growth. The D. spurca strain was isolated from the rhizosphere of tomato in Shouguang (Shandong, China). After the morphological identification, the strain of D. spurca was trapped by white clover under potted conditions. After approximately 11 weeks, the D. spurca-colonized roots and potted substrates were collected as the mycorrhizal fungal inoculums and stored at 4°C after natural air-drying. Before use, the spore density was 15 spores/g. The inoculation of D. spurca was carried out at the time of transplanting. A total of 120 g of mycorrhizal fungal inoculums was applied to the designed pot as the inoculation treatment. The equal amount of autoclaved mycorrhizal inoculums was applied to the uninoculated pot as the uninoculation treatment, followed by 2 mL of filtered (25 μm) solution with equal amount of mycorrhizal inoculums added to maintain the consistency of the microbiota except for the target strain.
Seven days after the inoculation, P treatments were applied. P concentrations in the potted substrate were achieved by controlling the KH2PO4 level in Hoagland nutrient solutions (pH 7.0), where 1 μmol/L and 100 μmol/L KH2PO4 was defined as the low P and moderate P level (Li et al., 2010). To reduce the difference in K levels of nutrient solutions among treatments, additional KNO3 was added to the P-deficient treatment to ensure the consistent K level. The nutrient solution was used at an intensity of 150 mL per pot at the three-day intervals.
All treated seedlings were placed in a greenhouse from May 4, 2020 to August 4, 2020, where environmental conditions were described in detail by Zou et al. (2021)
Experimental design
This experiment consisted of two factors: one was inoculation with (+A) and without (-A) D. spurca and the other was P treatments with 1 μmol/L (P1) and 100 μmol/L (P100) P. Each treatment was replicated eight times in 32 pots, with one walnut seedling planted in each pot in a randomized arrangement.
Determinations of variables
After three months of treatments, the plants were harvested. Plant height, stem diameter, and total biomass were measured directly before harvesting. At the same time, a portable Li-6400 photosynthetic apparatus (Li-Cor Inc., Lincoln, USA) was used to determine leaf gas exchange starting at 9:00 am on a sunny day before harvest. The soil attached to the roots was gently shaken off for hyphal length analysis, based on the method outlined by Bethlenfalvay and Ames (1987). A portion of root segments were cut, and root mycorrhizal staining was performed using trypan blue method described by Phillips and Hayman (1970). After microscopic observation, the root mycorrhizal colonization rate (%) was estimated as the percentage of the length of root segments colonized by AMF to the total length of root segments examined.
Eight plants from each treatment were divided equally into two parts, one of which was immediately frozen in liquid nitrogen and then stored at -80 °C for analysis of gene expressions. The other part was killed at 105 °C for 3 min after chlorophyll determination, then dried at 75 °C to constant weight, ground to powder, and passed through a 2 mm sieve for P concentration determination. The ICP Spectrometer (IRIS Advantage, Thermo, Waltham, USA) was used to analyze leaf P concentration. The concentration of glucose, fructose and sucrose in leaves was determined according to the colorimetric method described in detail by Wu et al. (2015b). The concentration of chlorophyll components was extracted with 80% acetone and determined using the method described by He et al. (2022).
The sequences of PAP genes (PAP10 and PAP12) and PT genes (PT3;1 and PT3;3) in Arabidopsis were obtained from the NCBI database (http://www.ncbi.nlm.nih.gov) and then compared with genome-wide of walnut (http://aegilops.wheat.ucdavis.edu/Walnut/data.php). The primer sequences (Supplementary Table S1) of JrPAP10, JrPAP12, JrPT3;1, and JrPT3;3 genes were designed using Primer5 premier 5.0 software and synthesized by Shanghai Bioengineering Co., Ltd. (Shanghai, China). Total RNA of leaf samples was extracted using an EASY spin Plus plant RNA kit (Aidlab). The reverse transcription of RNA was performed using the PrimeScript™ RT reagent kit with gDNA eraser kit (Takara). The 18S rRNA of walnut was used as the reference gene for qRT-PCR amplification. qRT-PCR was performed using the fluorescent dye method (2×AceQ® qPCR SYBR® Green Master Mix) with three biological replicates per treatment. The 2-ΔΔCt method (Livak and Schmittgen, 2001) was used to calculate the relative gene expression, in which the relative expression was normalized by the treatment with non-inoculation of D. spurca at P100 levels.
Statistical analysis
The data obtained from this experiment were presented using the means ± standard deviation. A two-factor (P treatments and AMF inoculations) analysis of variance was used for statistical analysis, with arcsine transformation preprocessed for percentages. Significant differences were compared at the 0.05 level using the Duncan’s new multiple range test. All statistical analyses were performed under SAS software.
Results
Changes in root AMF colonization and soil hyphal length
No mycorrhizal colonization was observed in roots of walnut seedlings inoculated without D. spurca, while mycorrhizal structures were visible in roots of walnut seedlings inoculated with D. spurca (Figure 1A), where the root mycorrhizal colonization rate ranged from 45.6% to 53.2% (Figure 1B) and soil hyphal length varied from 18.7 to 39.9 cm/g soil (Figure 1C), respectively. The P1 treatment significantly increased the root AMF colonization rate and soil hyphal length by 16.7% and 113.4%, respectively, compared with P100 treatment. P treatments and AMF inoculations significantly interacted with each other on soil hyphal length (Table 1).
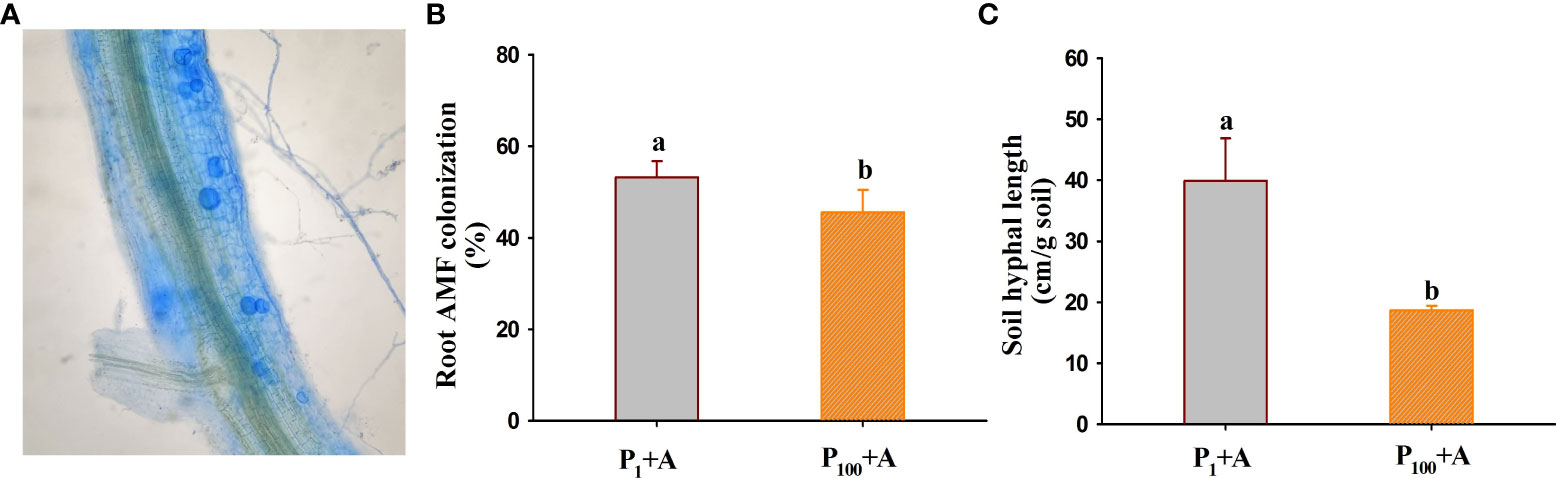
Figure 1 Root colonization (A) of Diversispora spurca and changes in root mycorrhizal colonization rate (B) and soil hyphal length (C) of walnut seedlings inoculated with D. spurca grown in 1 and 100 μmol/L phosphorus levels. Data (means ± SD, n = 4) followed by different letters above the bars indicate significant (P < 0.05) differences. P1+A, the walnut seedlings inoculated with D. spurca at 1 μmol/L phosphorus levels; P1-A, the walnut seedlings inoculated without D. spurca at 1 μmol/L phosphorus levels; P100+A, the walnut seedlings inoculated with D. spurca at 100 μmol/L phosphorus levels; P100-A, the walnut seedlings inoculated without D. spurca at 100 μmol/L phosphorus levels.
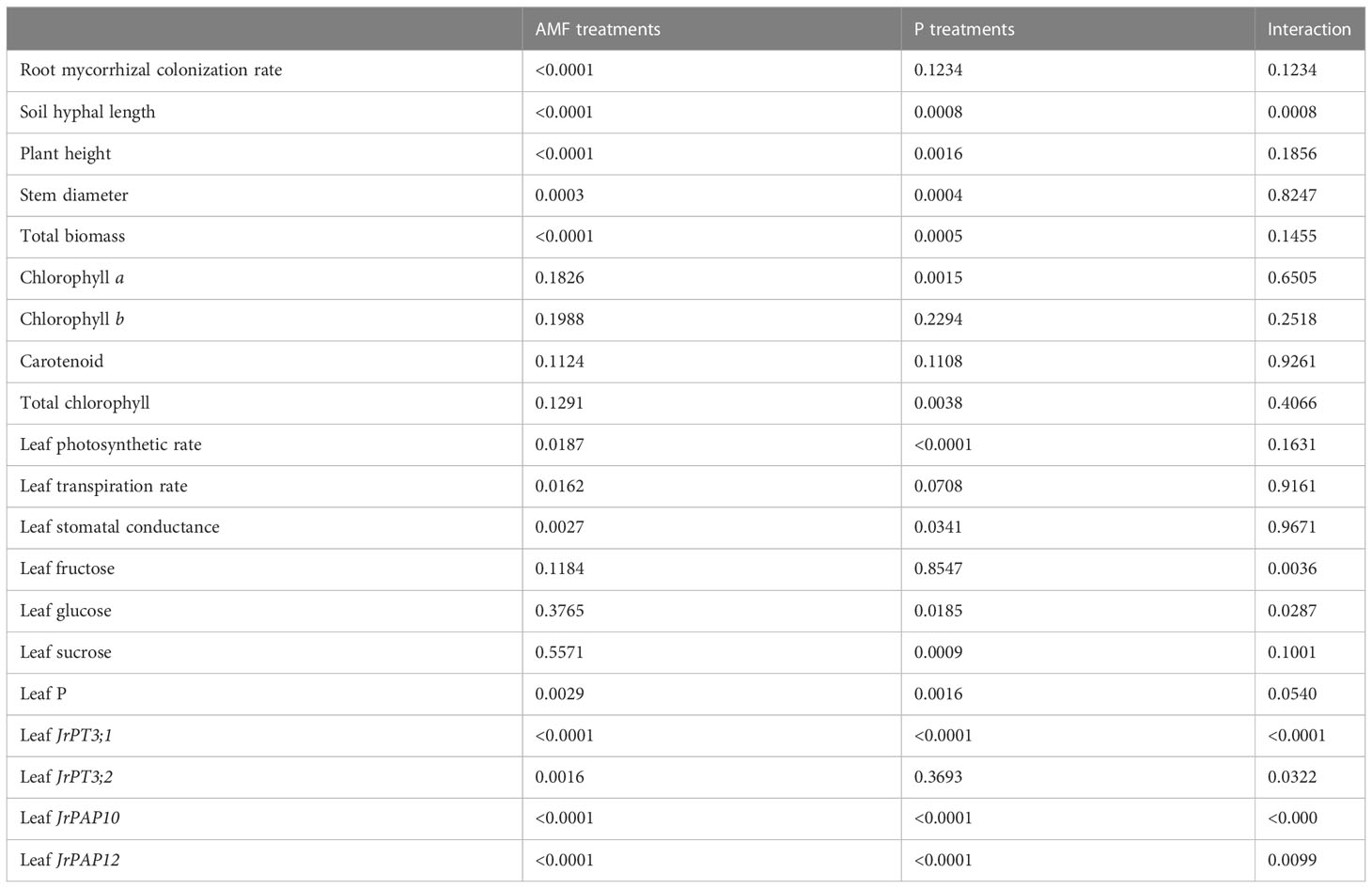
Table 1 Significance in interaction between arbuscular mycorrhizal fungi (AMF) treatments and phosphorus (P) treatments.
Plant growth responses
Walnut plants clearly responded to the P treatment as well as the D. spurca inoculation (Figure 2A). Both P treatments and AMF inoculations significantly altered plant growth variables, including plant height, stem diameter, and total biomass (Figures 2B–D). The P100 treatment significantly increased plant height, stem diameter, and total biomass by 6.0%, 14.1% and 32.9% in uninoculated plants and by 11.4%, 11.0% and 11.7% in inoculated plants, respectively, compared with the P1 treatment. On the other hand, inoculation with D. spurca also significantly increased plant height, stem diameter, and total biomass by 12.9%, 14.2%, and 51.5% under P1 conditions and by 18.7%, 11.2%, and 27.3% under P100 conditions, respectively, compared with the uninoculation control. There was not any significant interaction between AMF inoculation and P treatment on these growth variables (Table 1).
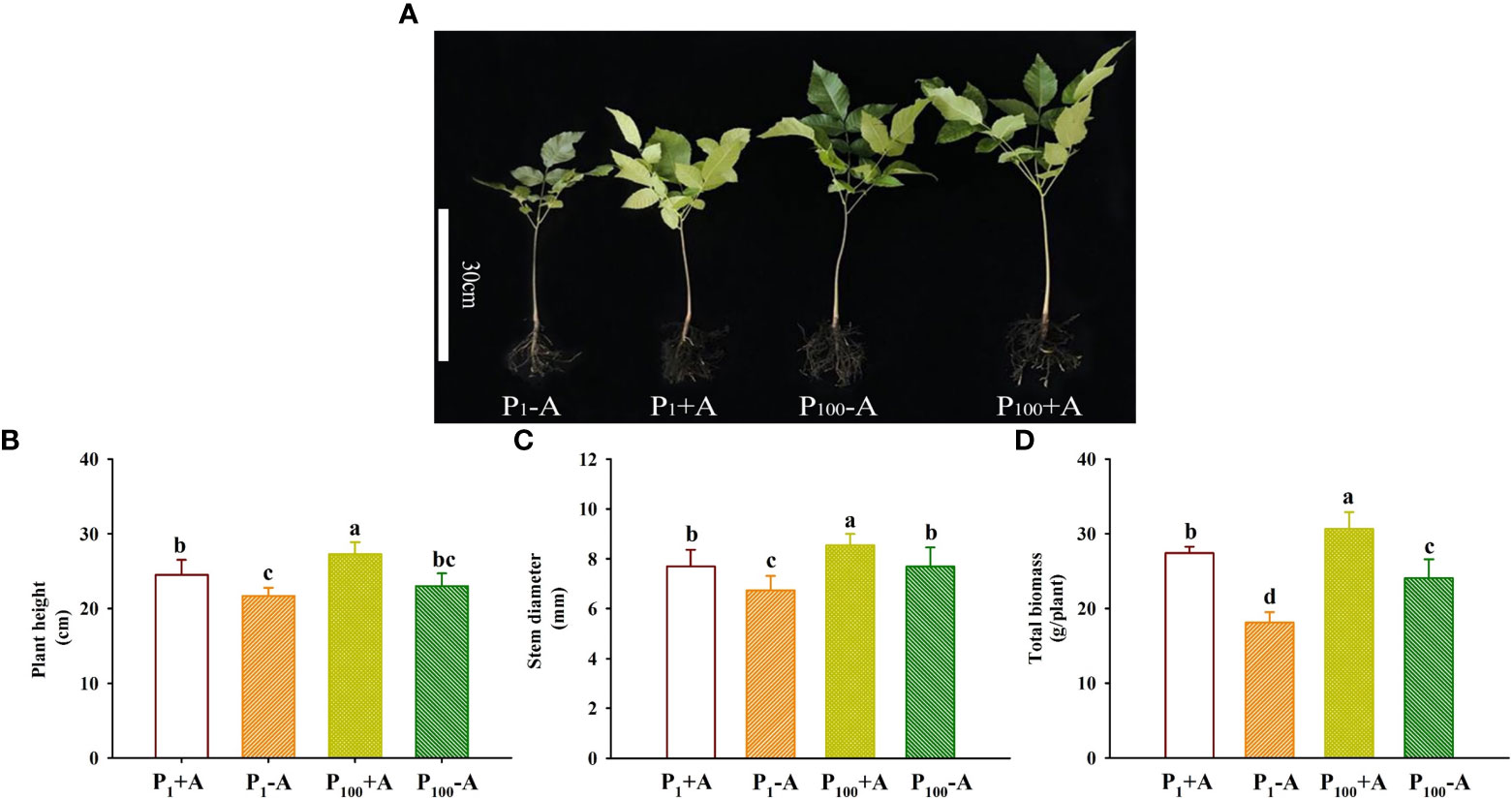
Figure 2 Changes in plant growth performance (A), plant height (B), stem diameter (C), and total biomass production (D) of walnut seedlings inoculated with Diversispora spurca grown in 1 and 100 μmol/L phosphorus levels. Data (means ± SD, n = 4) followed by different letters above the bars indicate significant (P < 0.05) differences. The abbreviations were shown in Figure 1.
Responses of leaf chlorophyll component concentrations
P100 treatment significantly promoted concentrations of chlorophyll a in leaves of uninoculated plants by 39.8% as well as concentrations of chlorophyll a and total chlorophyll in leaves of inoculated plants by 47.4% and 39.7%, respectively, compared to P1 treatment (Figure 3). On the other hand, although walnut seedlings inoculated with D. spurca maintained relatively higher concentrations of chlorophyll a, chlorophyll b, carotenoid, and total chlorophyll, the difference was not significant, independent of substrate P levels. No significant interaction appeared on leaf chlorophyll component concentrations (Table 1).
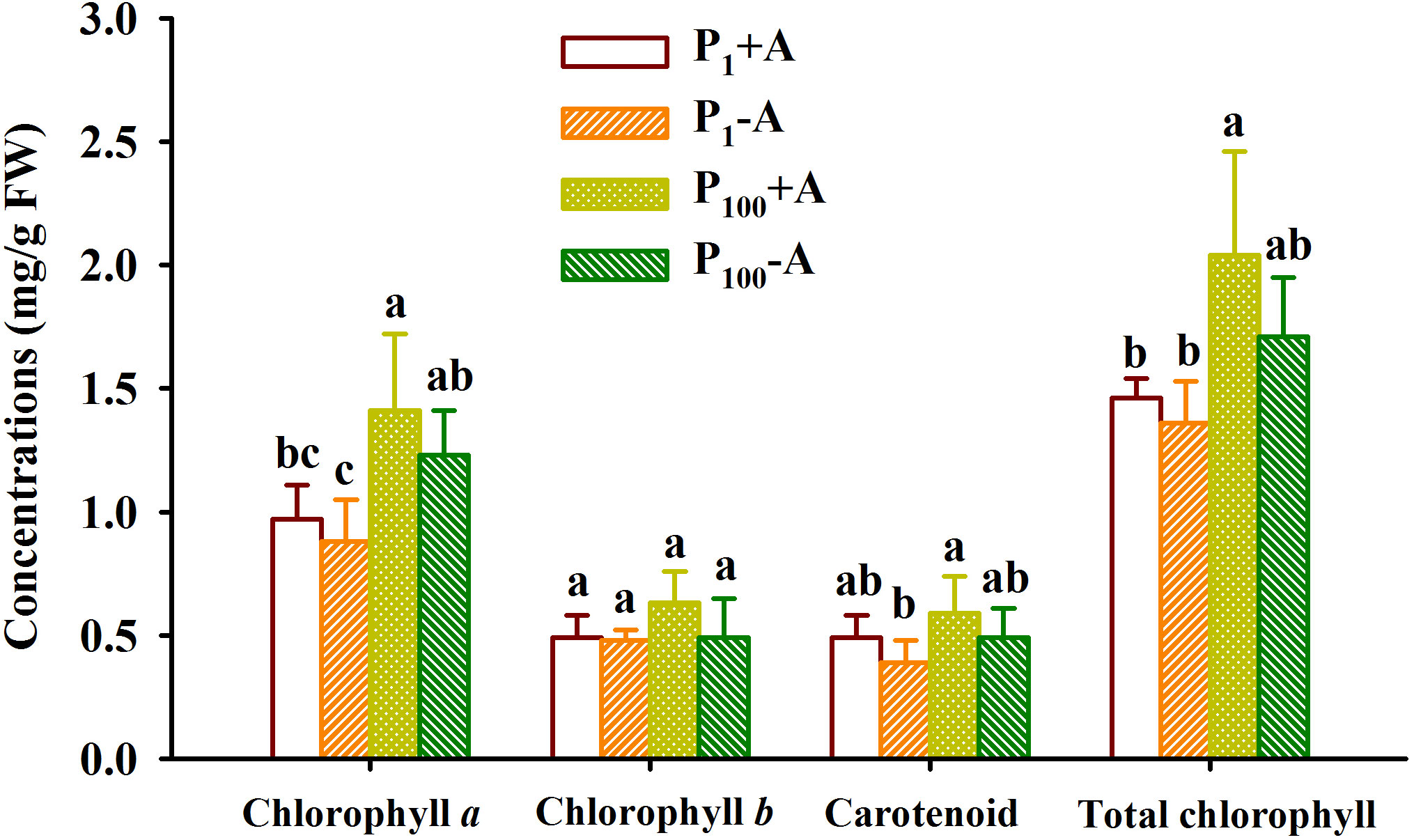
Figure 3 Changes in leaf chlorophyll component concentrations of walnut seedlings inoculated with Diversispora spurca grown in 1 and 100 μmol/L phosphorus levels. Data (means ± SD, n = 4) followed by different letters above the bars indicate significant (P < 0.05) differences. The abbreviations were shown in Figure 1.
Responses of leaf gas exchange
The application of P100 significantly increased leaf photosynthetic rate, transpiration rate and stomatal conductance by 14.0%, 18.2%, and 15.4% in inoculated plants and 34.6%, 26.3%, and 16.3% in uninoculated plants, respectively, compared with P1 conditions (Figures 4A–C). Similarly, D. spurca-inoculated plants showed significantly higher leaf photosynthetic rate, transpiration rate, and stomatal conductance, with increases of 23.4%, 35.1%, and 25.0% under P1 conditions, and 4.5%, 26.4%, and 24.0% under P100 conditions, respectively. No significant interaction appeared on leaf gas exchange variables (Table 1).
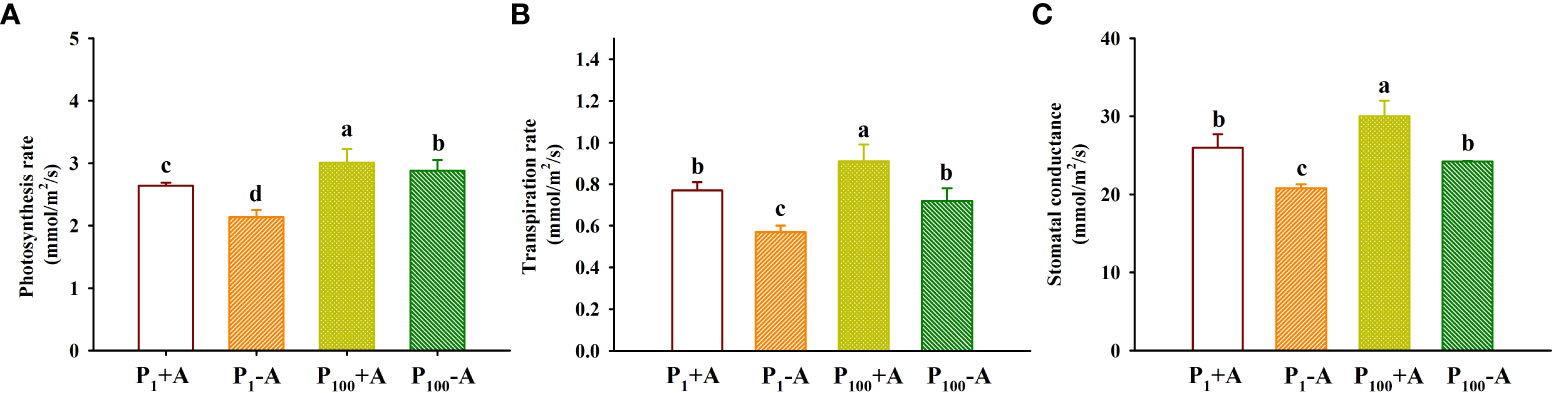
Figure 4 Changes in leaf photosynthesis rate (A), transpiration rate (B), and stomatal conductance (C) of walnut seedlings inoculated with Diversispora spurca grown in 1 and 100 μmol/L phosphorus levels. Data (means ± SD, n = 4) followed by different letters above the bars indicate significant (P < 0.05) differences. The abbreviations were shown in Figure 1.
Responses of leaf sugars
P100 treatment dramatically reduced leaf glucose concentration of uninoculated plants, while distinctly increased leaf glucose concentration of inoculated plants by 19.7%, along with the reduction of leaf fructose and sucrose by 38.5% and 29.4%, respectively, compared with P1 treatment (Figure 5). On the other hand, mycorrhizal plants recorded 33.6% significantly higher leaf fructose concentration under P1 conditions and 30.0% significantly higher leaf glucose concentration under P100 conditions, respectively. In addition, AMF inoculation and P treatment significantly interacted to affect leaf fructose and glucose concentrations (Table 1).
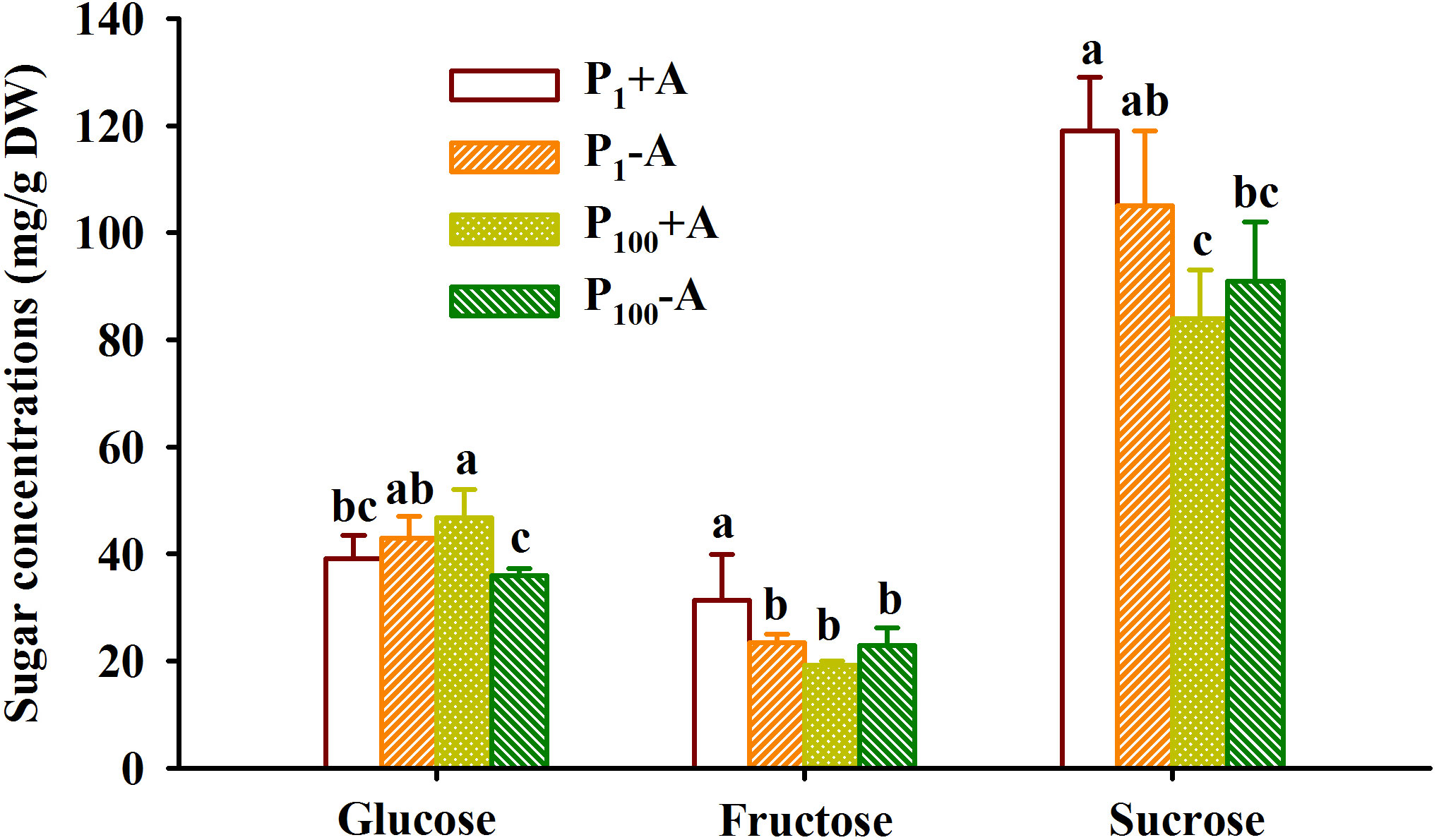
Figure 5 Changes in leaf fructose, glucose, and sucrose concentrations of walnut seedlings inoculated with Diversispora spurca grown in 1 and 100 μmol/L phosphorus levels. Data (means ± SD, n = 4) followed by different letters above the bars indicate significant (P < 0.05) differences. The abbreviations were shown in Figure 1.
Responses of leaf P concentration
P100 treatment dramatically increased leaf P concentration by 22.1% in uninoculated plants and 17.1% in inoculated plants, respectively, compared with P1 treatment (Figure 6). In addition, D. spurca inoculation significantly increased leaf P concentration by 26.2% and 21.0% under P1 and P100 conditions, respectively. No significant interaction was found on leaf P concentration (Table 1)
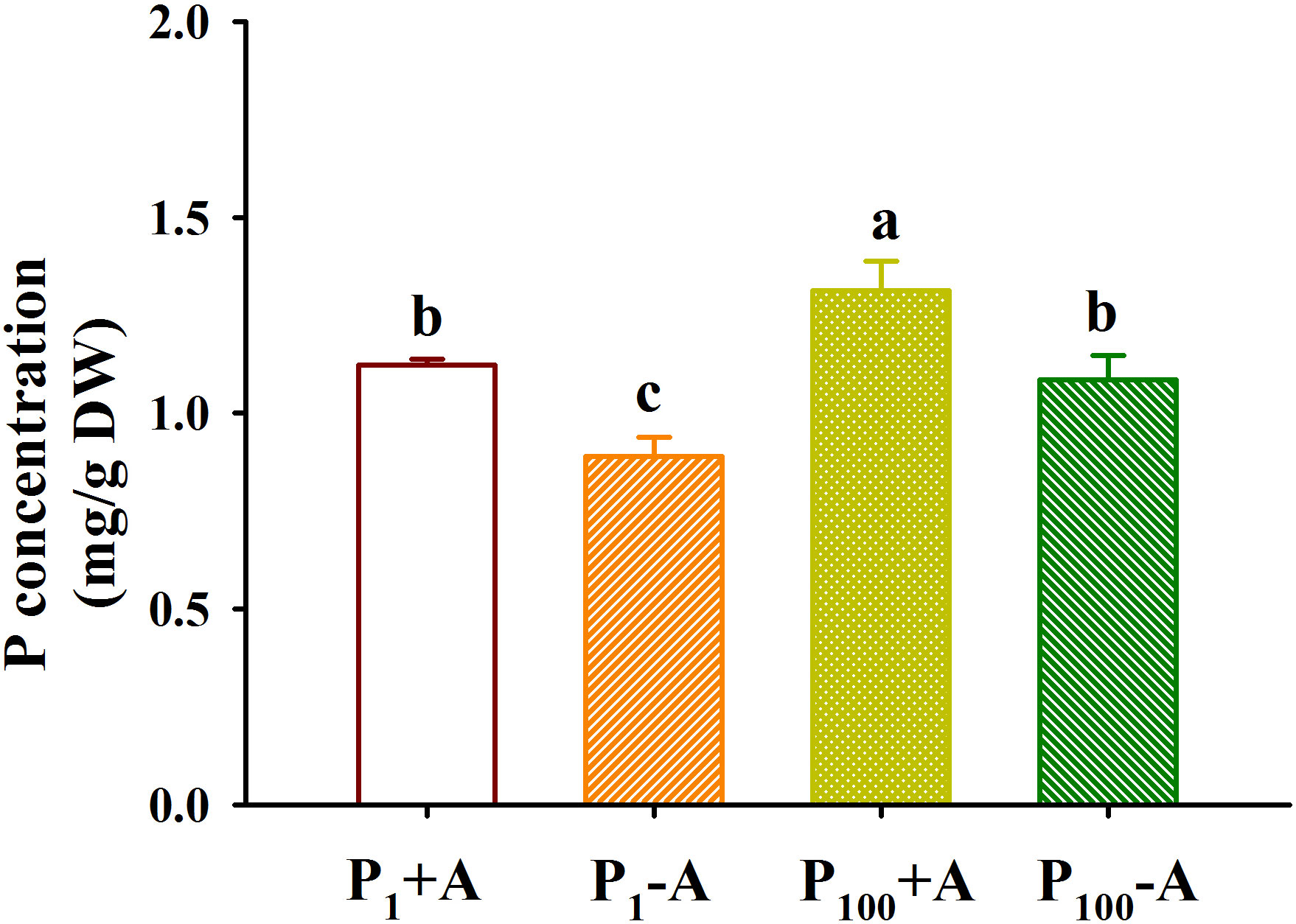
Figure 6 Changes in leaf phosphorus (P) concentrations of walnut seedlings inoculated with Diversispora spurca grown in 1 and 100 μmol/L P levels. Data (means ± SD, n = 4) followed by different letters above the bars indicate significant (P < 0.05) differences. The abbreviations were shown in Figure 1.
Responses of leaf PTs and PAPs expressions
P100 treatment dramatically up-regulated JrPAP10, JrPAP12, and JrPT3;2 expressions in mycorrhizal plants by 23.48-, 1.29-, and 3.79-fold and JrPT3;1 expression in non-mycorrhizal plants by 4.19-fold, along with a 0.77-fold down-regulated expression of JrPAP12 in non-mycorrhizal plants, compared with P1 treatment (Figure 7). Under P1 conditions, D. spurca inoculation did not alter expressions of JrPAP10, JrPAP12, JrPT3;1, and JrPT3;2. However, under P100 conditions, the fungal inoculation up-regulated JrPAP10, JrPAP12, and JrPT3;2 by 12.76-, 10.19-, and 6.59-fold, respectively, along with a 0.80-fold down-regulation of JrPT3;1 expression, compared with non-inoculation control. AMF inoculations and P treatments significantly interacted with each other to affect leaf JrPAP10, JrPAP12, JrPT3;1, and JrPT3;2 expression (Table 1).
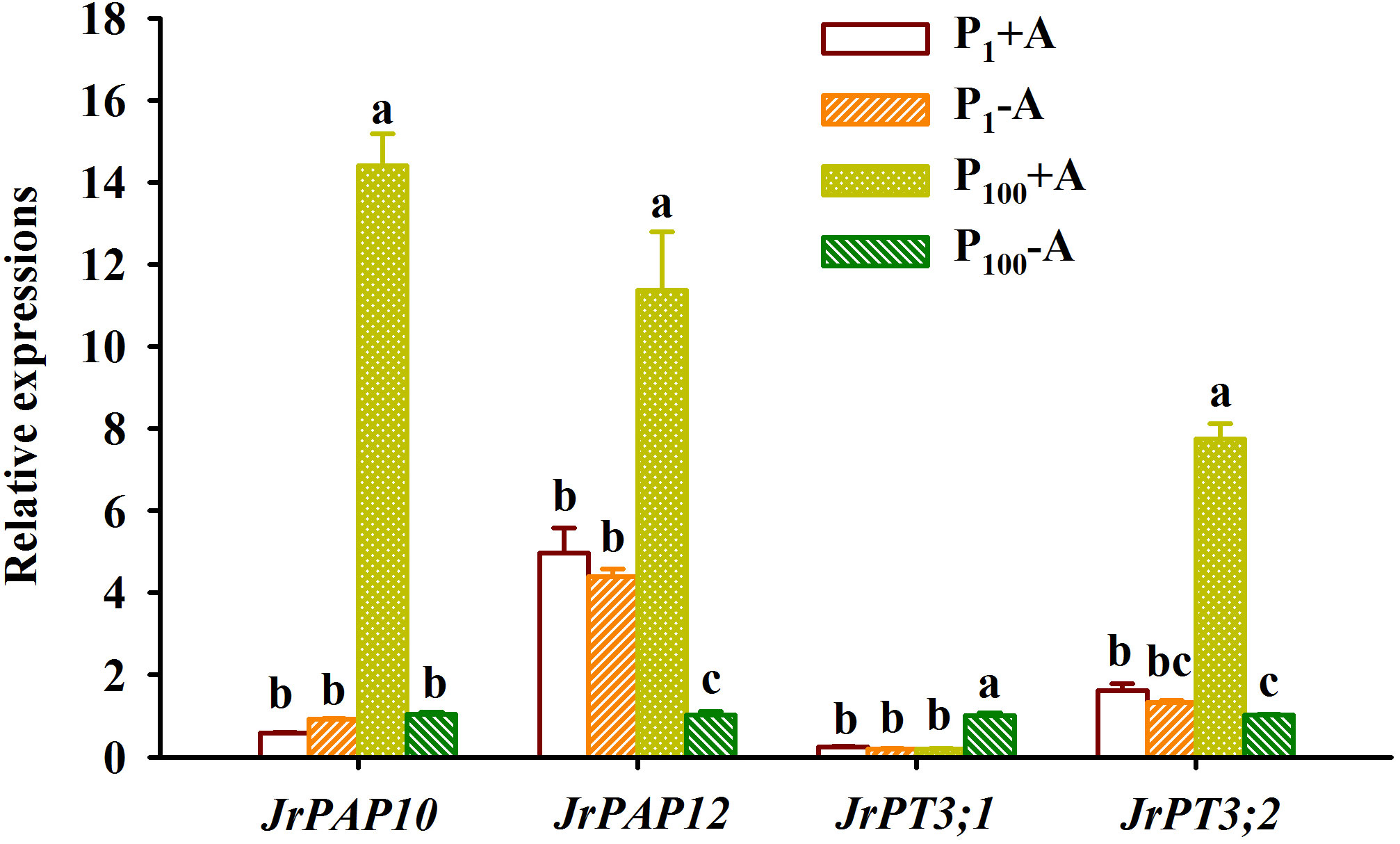
Figure 7 Changes in expressions of purple acid phosphatase (PAP) and phosphate transporter (PT) in leaves of walnut seedlings inoculated with Diversispora spurca grown in 1 and 100 μmol/L phosphorus levels. Data (means ± SD, n = 3) followed by different letters above the bars indicate significant (P < 0.05) differences. The abbreviations were shown in Figure 1.
Discussion
The results of this study showed that D. spurca was able to establish mycorrhizal symbiosis with the roots of walnut seedlings. Low P (P1) treatment dramatically stimulated root AMF colonization rate and soil hyphal length, compared with moderate P (P100) treatment. This is consistent with the results of Wu et al. (2015b) inoculating Funneliformis mosseae on trifoliate orange at 0.5 and 50 μmol/L P levels, but contrary to the results of Shao et al. (2021) inoculating Claroideoglomus etunicatum on tea plants at 0.5 and 50 μmol/L P levels. Usually, AMF colonization of roots is negatively correlated with substrate P levels, and thus low P levels in substrates stimulate hyphal growth and mycorrhizal formation (Wu et al., 2015a). Moreover, in the condition of substrate P deficiency, plant growth is more dependent on the mycorrhizal pathway to obtain P (Smith and Smith, 2012). In the present study, low P treatment significantly reduced plant height, stem diameter, and biomass production of walnut seedlings, but inoculation with D. spurca alleviated the inhibitory effects of low P stress on plant growth to varying degrees, and the promoting effect of mycorrhizae on total biomass was higher under P1 conditions than under P100 conditions, indicating a more prominent role of mycorrhizae under low P conditions. In addition to the growth improvement of walnut seedlings by D. spurca under P stress, similar results occurred under soil drought conditions (Ma et al., 2022), showing the important role of the D. spurca strain on plant growth of walnut seedlings.
In this study, inoculation with D. spurca did not significantly alter concentrations of chlorophyll a, chlorophyll b, carotenoid, and total chlorophyll, independent of substrate P levels. However, in maize, inoculation with Glomus mosseae significantly increased chlorophyll levels at 0.05 and 1 mmol/L P levels (Feng et al., 2002), which was associated with the promotion of host Mg and Fe acquisition by AMF (Zhang et al., 2018). This showed that the improvement of chlorophyll component concentrations by AMF is variable and may be dependent on AMF, host plants, and environmental conditions. Mycorrhiza-triggered changes in chlorophyll compositions were not significant in walnut seedlings, which in turn caused leaf glucose, fructose, and sucrose contents to be barely affected at low and moderate P levels, although mycorrhizal walnut plants showed higher fructose concentrations under P1 conditions as well as glucose concentrations at P100. AMF-induced sugar changes of walnut seedlings were associated with mycorrhizal roots forming a strong carbon pool and greater mycorrhizal roots consumed a large amount of sugar (Wu et al., 2015b; He et al., 2022). More experiments are required to verify the reason. However, D. spurca inoculation significantly elevated leaf photosynthetic rate, stomatal conductance, and transpiration rate, although low P treatment inhibited leaf gas exchange. Thus, under low P stress, AMF may accelerate leaf gas exchange in host plants by reducing stomatal resistance as well as increasing transpiration fluxes (Zhu et al., 2011). Another explanation is that AMF alters the levels of endogenous hormones, especially abscisic acid, and thus regulates leaf gas exchange (He et al., 2019).
The present study showed that D. spurca inoculation significantly increased leaf P levels of walnut seedlings, and the increase was higher at P1 levels than at P100 levels, showing the prominent effect of D. spurca on host P uptake at low P levels. This is because plant roots at P100 levels can take up sufficient P and therefore have relatively low dependence on arbuscular mycorrhizae, whereas at P1 levels, plant roots do not take up sufficient P and need to take up additional P to meet plant P demand with the help of AMF through its extraradical hyphal extension to areas inaccessible to the root (Song et al., 2001).
PAP is the largest class of acid phosphatases, secreting acid phosphatase to the cell wall or to the root surface and environment, mainly involved in the hydrolysis of multiple Pi esters in the rhizosphere or extra-plasmic space, thus facilitating plant P acquisition (Schenk et al., 2013; Wang et al., 2014). The present study showed that in D. spurca-inoculated walnut seedlings, P1 treatment significantly suppressed the expression of both JrPAP10 and JrPAP12, compared with P100 treatment, whereas in uninoculated plants, JrPAP12 expression was up-regulated. This suggested that mycorrhizal plants are distinct from non-mycorrhizal plants in terms of PAPs expression response in the face of P stress. This is due to the fact that mycorrhizal plants exhibited higher mycorrhizal colonization rate of roots and soil hyphal length under low P versus moderate P conditions, and they can better help the host to obtain P through reducing arbuscule degradation (Li et al., 2019), resulting in a reduced dependence on PAPs. D. spurca did not alter JrPAP10 and JrPAP12 expression under low P conditions. However, at P100 levels, D. spurca significantly up-regulated JrPAP10 and JrPAP12 expression. Combined with no significant changes in JrPT expressions by D. spurca at low P levels, this implies that at low P levels, mycorrhizae promote plant P acquisition mainly by increasing root mycorrhizal colonization rate and soil hyphal length, which in turn directly exploits the mycorrhizal pathway to promote host P acquisition (Smith and Smith, 2011; Smith et al., 2015). In Arabidopsis, it has been demonstrated that AtPAP10 is a secreted acid phosphatase and is an essential component of the adaptive response of plants to Pi limitation, but AtPAP12 is an intracellular acid phosphatase (Wang et al., 2014). Therefore, at P100 levels, D. spurca might promote the expression of intracellular acid phosphatase (JrPAP12) and secret acid phosphatase genes (JrPAP10). In addition, AtPAP10 on the root surface is controlled by ethylene only (Zhang et al., 2014), so whether mycorrhizal up-regulation of JrPAP10 is related to mycorrhizal triggering of ethylene synthesis remains to be further investigated.
PTs are an important membrane protein found in the mitochondrial, plasma and plastid membranes of plants, controlling the uptake and translocation of Pi in plants (Nakamori et al., 2002). AMF usually induces the expression of host high-affinity PT genes to enhance plant uptake of P (Luo et al., 2019). The results of this study showed that low P treatment inhibited the expression of JrPT3;1 in uninoculated plants as well as the expression of JrPT3;2 in inoculated plants, suggesting that JrPT3;1 and JrPT3;2 are not genes up-regulated for expression at low P levels. Huang et al. (2022) also found that low P repressed the expression of PT1;1 in leaves of Camellia oleifera seedlings. However, walnut plants colonized by D. spurca did not show significant changes in JrPT3;1 and JrPT3;2 in leaves at low P levels, while at moderate P levels, JrPT3;1 was repressed and JrPT3;2 was up-regulated. This suggests that AMF only up-regulated the expression of JrPT3;2 at appropriate P levels. In addition, mycorrhizal regulation of host PTs expression may be closely related to host root-hair status, where substrate low P levels increase root-hair density (Wang et al., 2006; Zhang et al., 2019; Shao et al., 2021), thereby promoting host P acquisition by both root-hairs and mycorrhizal pathways. Shao et al. (2021) also found that Claroideoglomus etunicatum up-regulated the expression of CsPT1 but suppressed the expression of CsPT4 in leaves of tea plants under low and appropriate P conditions. Cao et al. (2022) also found that PTs expressions were dependent on the AMF species and plant tissues. It concludes that AMF-up-regulated PT expressions depend on AMF species, plant tissues, substrate P levels, and PT genes. On the other hand, at moderate P levels, mycorrhizal enhancement of plant P acquisition is related to the up-regulation of host PAPs and PTs expression. More work needs to be done around the expression of more PTs genes in leaves and roots under mycorrhization conditions. Mycorrhizae can specifically induce the expression of certain high and low affinity PT genes (Rausch et al., 2001). In the present study, JrPT3;2 may be a candidate PT gene specifically induced by AMF at moderate P levels, but functions of JrPT3;2 in arbuscule-containing cortical cells of roots remains to be investigated.
Conclusion
This study showed that low P treatment significantly inhibited plant growth, leaf gas exchange, chlorophyll component concentrations, and P concentration of walnut plants, but D. spurca significantly promoted plant growth and leaf gas exchange as well as P acquisition at low P levels. Although AMF did not induce the expression of JrPAP10, JrPAP12, JrPT3;1, and JrPT3;2 at low P levels, the promotion of P acquisition by AMF at low P levels may be related to the increase of root AMF colonization and soil hyphal length. However, mycorrhizal promotion of host P acquisition at moderate P levels was associated with AMF-increased expression of JrPT3;2, JrPAP10, and JrPAP12. This suggests that AMF has different strategies to promote host P acquisition at different P levels. More studies need to be carried out on expressions of whole family members of PTs and PAPs in leaves and roots of walnut seedlings under mycorrhization conditions.
Data availability statement
The original contributions presented in the study are included in the article/Supplementary Material. Further inquiries can be directed to the corresponding author.
Author contributions
Y-NZ, Y-JX, and Q-SW designed the experiment. G-MH and R-CL prepared the materials for the experiment. G-MH and R-CL analyzed the data. Y-NZ and R-CL wrote the manuscript. AS, KK, AH, EFA, and Q-SW revised the manuscript. All authors contributed to the article and approved the submitted version.
Funding
This work was supported by the Hubei Province ‘14th Five-Year’ Major Science and Technology Aid Tibet project (SCXX-XZCG-22016) and the Hubei Forestry Science and Technology Support Key Project ([2022]LYKJ10). The authors would like to extend their sincere appreciation to the Researchers Supporting Project Number (RSP2023R356), King Saud University, Riyadh, Saudi Arabia.
Acknowledgments
The authors would like to extend their sincere appreciation to the Researchers Supporting Project Number (RSP2023R356), King Saud University, Riyadh, Saudi Arabia.
Conflict of interest
The authors declare that the research was conducted in the absence of any commercial or financial relationships that could be construed as a potential conflict of interest.
Publisher’s note
All claims expressed in this article are solely those of the authors and do not necessarily represent those of their affiliated organizations, or those of the publisher, the editors and the reviewers. Any product that may be evaluated in this article, or claim that may be made by its manufacturer, is not guaranteed or endorsed by the publisher.
Supplementary material
The Supplementary Material for this article can be found online at: https://www.frontiersin.org/articles/10.3389/fpls.2023.1140467/full#supplementary-material
References
Adeyemi, N. O., Atayese, M. O., Sakariyawo, O. S., Azeez, J. O., Olubode, A. A., Ridwan, M., et al. (2021). Influence of different arbuscular mycorrhizal fungi isolates in enhancing growth, phosphorus uptake and grain yield of soybean in a phosphorus deficient soil under field conditions. Commun. Soil Sci. Plant Analy. 52, 1171–1183. doi: 10.1080/00103624.2021.1879117
Behrooz, A., Vahdati, K., Rejali, F., Lotfi, M., Sarikhani, S., Leslie, C. (2019). Arbuscular mycorrhiza and plant growth-promoting bacteria alleviate drought stress in walnut. HortScience 54, 1087–1092. doi: 10.21273/HORTSCI13961-19
Bethlenfalvay, G. J., Ames, R. N. (1987). Comparison of two methods for quantifying extraradical mycelium of vesicular-arbuscular mycorrhizal fungi. Soil Sci. Soc Am. J. 51, 834–837. doi: 10.2136/sssaj1987.03615995005100030049x
Cao, M. A., Liu, R. C., Xiao, Z. Y., Hashem, A., Abd Allah, E. F., Alsayed, M. F., et al. (2022). Symbiotic fungi alter the acquisition of phosphorus in Camellia oleifera through regulating root architecture, plant phosphate transporter gene expressions and soil phosphatase activities. J. Fungi 8, 800. doi: 10.3390/jof8080800
Etesami, H., Jeong, B. R., Glick, B. R. (2021). Contribution of arbuscular mycorrhizal fungi, phosphate–solubilizing bacteria, and silicon to p uptake by plant. Front. Plant Sci. 12, 699618. doi: 10.3389/fpls.2021.699618
Feng, G., Zhang, F., Li, X., Tian, C., Tang, C., Rengel, Z. (2002). Improved tolerance of maize plants to salt stress by arbuscular mycorrhiza is related to higher accumulation of soluble sugars in roots. Mycorrhiza 12, 185–190. doi: 10.1007/s00572-002-0170-0
He, J. D., Li, J. L., Wu, Q. S. (2019). Effects of Rhizoglomus intraradices on plant growth and root endogenous hormones of trifoliate orange under salt stress. J. Anim. Plant Sci. 29, 245–250.
He, W. X., Wu, Q. S., Hashem, A., Abd Allah, E. F., Muthuramalingam, P., Al-Arjani, A.-B. F., et al. (2022). Effects of symbiotic fungi on sugars and soil fertility and structure-mediated changes in plant growth of Vicia villosa. Agriculture 12, 1523. doi: 10.3390/agriculture12101523
Huang, Y. X., Lin, Y. L., Zhang, L. P., Wu, F., Yang, Y., Tan, M. X. (2022). Effects of AM fungi and inorganic phosphorus on phosphorus uptake and growth soil phosphorus fraction of Camellia oleifera seedlings. For. Res. 35, 33–41. doi: 10.13275/j.cnki.lykxyj.2022.005.004
Huang, G. M., Zou, Y. N., Wu, Q. S., Xu, Y. J., Kuča, K. (2020). Mycorrhizal roles in plant growth, gas exchange, root morphology, and nutrient uptake of walnuts. Plant Soil Environ. 66, 295–302. doi: 10.17221/240/2020-PSE
Li, Y. F., Jin, S. H., Ye, Z. Q., Huang, J. Q., Jiang, P. K. (2010). Root morphology and physiological characteristics in carya cathayensis seedlings with low phosphorus stress. J. Zhejiang A.&F. Univ, 27, 239–245.
Li, X. R., Sun, J., Albinsky, D., Zarrabian, D., Hull, R., Lee, T., et al. (2022). Nutrient regulation of lipochitooligosaccharide recognition in plants via NSP1 and NSP2. Nat. Commun. 13, 6421. doi: 10.1038/s41467-022-33908-3
Li, C. C., Zhou, J., Wang, X. R., Liao, H. (2019). A purple acid phosphatase, GmPAP33, participates in arbuscule degeneration during arbuscular mycorrhizal symbiosis in soybean. Plant Cell Environ. 42, 2015–2027. doi: 10.1111/pce.13530
Livak, K. J., Schmittgen, T. D. (2001). Analysis of relative gene expression data using real-time quantitative PCR and the 2-ΔΔCt. Methods 25, 402–408. doi: 10.1006/meth.2001.1262
Luo, Y. Y., Hao, X. J., Zhang, K. Y. (2019). Effect of inoculation of AM fungi on different phosphorus morphology of discarded soil in coal mining area. Southwest China J. Agric. Sci. 32, 381–388. doi: 10.16213/j.cnki.scjas.2019.2.026
Ma, T., Ning, D. L. (2021). Analysis of international competitiveness of China walnut industry. For. Sci. Technol. 64 (1), 3–7. doi: 10.13456/j.cnki.lykt.2019.12.26.0002
Ma, W. Y., Qin, Q. Y., Zou, Y. N., Kuča, K., Giri, B., Wu, Q. S., et al. (2022). Arbuscular mycorrhiza induces low oxidative burst in drought-stressed walnut through activating antioxidant defense systems and heat shock transcription factor expression. Front. Plant Sci. 13, 1089420. doi: 10.3389/fpls.2022.1089420
Ma, W. Y., Wu, Q. S., Xu, Y. J., Kuča, K. (2021). Exploring mycorrhizal fungi in walnut with a focus on physiological roles. Not. Bot. Horti Agrobo. 49, 12363. doi: 10.15835/nbha49212363
Malhotra, H., Vandana, Sharma, S., Pandey, R. (2018). “Phosphorus nutrition: Plant growth in response to deficiency and excess,” in Plant nutrients and abiotic stress tolerance. Eds. Hasanuzzaman, M., Fujita, M., Oku, H., Nahar, K., Hawrylak-Nowak, B. (Singapore: Springer), 171–190. doi: 10.1007/978-981-10-9044-8_7
Mao, J. H., Li, R. B., Jing, Y. B., Ning, D. L., Li, Y. P., Chen, H. Y. (2022). Arbuscular mycorrhizal fungi associated with walnut trees and their effect on seedling growth. J. For. Environ. 42, 71–80. doi: 10.13324/j.cnki.jfcf.2022.01.009
Nakamori, K., Takabatake, R., Umehara, Y., Kouchi, H., Izui, K., Hata, S. (2002). Cloning, functional expression, and mutational analysis of a cDNA for Lotus japonicus mitochondrial phosphate transporter. Plant Cell Physiol. 43, 1250–1253. doi: 10.1093/pcp/pcf141
Phillips, J. M., Hayman, D. S. (1970). Improved procedures for clearing roots and staining parasitic and vesicular-arbuscular mycorrhizal fungi for rapid assessment of infection. Trans. Br. Mycol. Soc 55, 158–161. doi: 10.1016/S0007-1536(70)80110-3
Rausch, C., Daram, P., Brunner, S., Jansa, J., Laloi, M., Leggewie, G., et al. (2001). A phosphate transporter expressed in arbuscule-containing cells in potato. Nature 414, 462–465. doi: 10.1038/35106601
Sandhu, J., Rouached, H. (2022). All roads lead to PHO1. Nat. Plants 8, 986–987. doi: 10.1038/s41477-022-01242-7
Schenk, G., Mitić, N., Hanson, G. R., Comba, P. (2013). Purple acid phosphatase: a journey into the function and mechanism of a colorful enzyme. Coordin. Chem. Rev. 257, 473–482. doi: 10.1016/j.ccr.2012.03.020
Serr, E. F. (1960). Walnut orchards on volcanic soils deficient in phosphorus. Calif. Agric. 14, 6–7.
Shao, Y. D., Hu, X. C., Wu, Q. S., Yang, T. Y., Srivastava, A. K., Zhang, D. J., et al. (2021). Mycorrhizas promote p acquisition of tea plants through changes in root morphology and p transporter gene expression. South Afr. J. Bot. 137, 455–462. doi: 10.1016/j.sajb.2020.11.028
Smith, S. E., Anderson, I. C., Smith, F. A. (2015). Mycorrhizal associations and phosphorus acquisition: from cells to ecosystems. Annu. Plant Rev. 48, 409–440. doi: 10.1002/9781118958841.ch14
Smith, S. E., Smith, F. A. (2011). Roles of arbuscular mycorrhizas in plant nutrition and growth: New paradigms from cellular to ecosystem scales. Annu. Rev. Plant Biol. 62, 227–250. doi: 10.1146/annurev-arplant-042110-103846
Smith, S. E., Smith, F. A. (2012). Fresh perspectives on the roles of arbuscular mycorrhizal fungi in plant nutrition and growth. Mycologia 104, 1–13. doi: 10.3852/11-229
Song, Y. C., Li, X. L., Feng, G. (2001). Effect of VAM fungi on phosphatase activity in maize rhizosphere. Chin. J. Appl. Ecol. 12, 593–596.
Wang, Y., Chen, Y. F., Wu, W. H. (2021). Potassium and phosphorus transport and signaling in plants. J. Integr. Plant Biol. 63, 34–52. doi: 10.1111/jipb.13053
Wang, L., Lu, S., Zhang, Y., Li, Z., Du, X., Liu, D. (2014). Comparative genetic analysis of arabidopsis purple acid phosphatases AtPAP10, AtPAP12, and AtPAP26 provides new insights into their roles in plant adaptation to phosphate deprivation. J. Integr. Plant Biol. 56, 299–314. doi: 10.1111/jipb.12184
Wang, Q. M., Peng, W. X., Lu, B. J., Pei, D. (2006). Histological study of in vitro adventitious roots of Juglans regia. Acta Bot. Boreal.-Occident. Sin. 26, 719–724.
Wu, Q. S., Li, Y., Zou, Y. N., He, X. H. (2015a). Arbuscular mycorrhiza mediates glomalin-related soil protein production and soil enzyme activities in the rhizosphere of trifoliate orange grown under different p levels. Mycorrhiza 25, 121–130. doi: 10.1007/s00572-014-0594-3
Wu, Q. S., Srivastava, A. K., Li, Y. (2015b). Effect of mycorrhizal symbiosis on growth behavior and carbohdyrate metabolism of trifoliate orange under different substrate p levels. J. Plant Growth Regul. 34, 495–508. doi: 10.1007/s00344-015-9485-x
Zhang, C. P. (2014). “Influence of nitrogen or phosphorous on water metabolism of juglans regia seedlings,” in Dissertation for the doctoral degree (Chinese Academy of Forestry, Beijing, China).
Zhang, T., Hu, Y., Zhang, K., Tian, C., Guo, J. (2018). Arbuscular mycorrhizal fungi improve plant growth of ricinus communis by altering photosynthetic properties and increasing pigments under drought and salt stress. Indust. Crops Prod. 117, 13–19. doi: 10.1016/j.indcrop.2018.02.087
Zhang, Y. J., Sun, F., Fettke, J., Schottler, M. A., Ramsden, L., Fernie, A. R., et al. (2014). Heterologous expression of AtPAP2 in transgenic potato influences carbon metabolism and tuber development. FEBS Lett. 588, 3726–3731. doi: 10.1016/j.febslet.2014.08.019
Zhang, F., Wang, P., Zou, Y. N., Wu, Q. S., Kuča, K. (2019). Effects of mycorrhizal fungi on root-hair growth and hormone levels of taproot and lateral roots in trifoliate orange under drought stress. Arch. Agron. Soil Sci. 65, 1316–1330. doi: 10.1080/03650340.2018.1563780
Zhu, X. C., Song, F. B., Liu, S. Q., Liu, T. D. (2011). Effects of arbuscular mycorrhizal fungus on photosynthesis and water status of maize under high temperature stress. Plant Soil 346, 189–199. doi: 10.1007/s11104-011-0809-8
Keywords: mycorrhiza, P deficit, phosphate transporter, purple acid phosphatase, walnut
Citation: Zou Y-N, Xu Y-J, Liu R-C, Huang G-M, Kuča K, Srivastava AK, Hashem A, Abd_Allah EF and Wu Q-S (2023) Two different strategies of Diversispora spurca-inoculated walnut seedlings to improve leaf P acquisition at low and moderate P levels. Front. Plant Sci. 14:1140467. doi: 10.3389/fpls.2023.1140467
Received: 09 January 2023; Accepted: 01 February 2023;
Published: 23 February 2023.
Edited by:
Muhammad Ahsan Altaf, Hainan University, ChinaReviewed by:
Karthikeyan Adhimoolam, Jeju National University, Republic of KoreaLihui Lyu, Northwest A&F University, China
Copyright © 2023 Zou, Xu, Liu, Huang, Kuča, Srivastava, Hashem, Abd_Allah and Wu. This is an open-access article distributed under the terms of the Creative Commons Attribution License (CC BY). The use, distribution or reproduction in other forums is permitted, provided the original author(s) and the copyright owner(s) are credited and that the original publication in this journal is cited, in accordance with accepted academic practice. No use, distribution or reproduction is permitted which does not comply with these terms.
*Correspondence: Qiang-Sheng Wu, d3VxaWFuZ3NoQDE2My5jb20=
†These authors share first authorship