- 1Department of Genetics and Microbiology, Faculty of Science, Charles University, Prague, Czechia
- 2Laboratory of Growth Regulators, Centre of the Region Haná for Biotechnological and Agricultural Research, Institute of Experimental Botany, Czech Academy of Sciences, v.v.i. and Palacký University, Olomouc, Czechia
Exogenously applied brassinosteroids (BRs) improve plant response to drought. However, many important aspects of this process, such as the potential differences caused by different developmental stages of analyzed organs at the beginning of drought, or by BR application before or during drought, remain still unexplored. The same applies for the response of different endogenous BRs belonging to the C27, C28-and C29- structural groups to drought and/or exogenous BRs. This study examines the physiological response of two different leaves (younger and older) of maize plants exposed to drought and treated with 24-epibrassinolide (epiBL), together with the contents of several C27, C28-and C29-BRs. Two timepoints of epiBL application (prior to and during drought) were utilized to ascertain how this could affect plant drought response and the contents of endogenous BRs. Marked differences in the contents of individual BRs between younger and older maize leaves were found: the younger leaves diverted their BR biosynthesis from C28-BRs to C29-BRs, probably at the very early biosynthetic steps, as the levels of C28-BR precursors were very low in these leaves, whereas C29-BR levels vere extremely high. Drought also apparently negatively affected contents of C28-BRs (particularly in the older leaves) and C29-BRs (particularly in the younger leaves) but not C27-BRs. The response of these two types of leaves to the combination of drought exposure and the application of exogenous epiBL differed in some aspects. The older leaves showed accelerated senescence under such conditions reflected in their reduced chlorophyll content and diminished efficiency of the primary photosynthetic processes. In contrast, the younger leaves of well-watered plants showed at first a reduction of proline levels in response to epiBL treatment, whereas in drought-stressed, epiBL pre-treated plants they were subsequently characterized by elevated amounts of proline. The contents of C29- and C27-BRs in plants treated with exogenous epiBL depended on the length of time between this treatment and the BR analysis regardless of plant water supply; they were more pronounced in plants subjected to the later epiBL treatment. The application of epiBL before or during drought did not result in any differences of plant response to this stressor.
1 Introduction
Drought is one of the most significant stressors affecting agricultural production worldwide; thus, it is crucial to alleviate its negative effect on plants. Various approaches to achieve this are possible. Aside from classical breeding and genetic engineering, the treatment of plants with chemical compounds such as antioxidants, phytohormones, polyethylene glycol, etc., has also been reportedly tried. One group of these compounds are brassinosteroids (BRs): steroid phytohormones showing a wide range of functions in the regulation of plant growth and development and significantly participating in plant defense against diverse environmental stressors (Ahammed et al., 2022).
More than 170 studies focused on BRs and drought stress have been published during the past three decades. Most of these studies were performed with plants treated with exogenous BRs, although mutants in genes associated with BR synthesis or signaling have also been utilized (for review see, e.g., Holá, 2019; Sidhu and Bali, 2022). However, the results of these studies are so variable that it is still impossible to draw a clear conclusion about the role of BRs in plant response to drought. To demonstrate this, let us randomly choose just two parameters strongly associated with plant drought response: i) catalase activity (CAT; an important enzymatic antioxidant) and ii) the proline content (important osmoprotectant as well as a non-enzymatic antioxidant). Chandrasekaran et al. (2017) and Sivakumar et al. (2017) reported a reduced CAT activity in drought-stressed BR-treated tomato plants compared to non-treated ones. Completely opposite results were published in some previous analyses performed with the same species (Behnamnia et al., 2009; Yuan et al., 2010). Similarly, in several studies made with various drought-stressed plant species, reduced levels of proline were observed after BR treatment (Li et al., 2012; Gursude et al., 2014; Ahmed et al., 2017; Hemmati et al., 2018). Other studies on this topic, where proline was also analyzed, reported its increased levels in drought-stressed BR-treated plants (e.g., Zhu et al., 2014; Shahana et al., 2015; Younesian et al., 2017; Lv et al., 2020).
The above-mentioned variability of the data on the relationship between BRs and plant drought response results from many factors such as the inter- or intra-species differences, the developmental stage of analyzed plants/organs, duration of the drought period, the method utilized for the drought simulation, the BR type and concentration, the method of plant treatment with BRs, timepoints of BR application, etc. (for more details see Holá, 2019). Unfortunately, studies that would purposely focus on the effects these possible sources of variability can have on plant responses to BRs are very rare. Thus, we decided to focus on two following aspects in order to ascertain how they could affect plant responses to BRs: i) to analyze the response of two different leaves (already developed and still developing ones at the beginning of drought period) of plants exposed to drought and treated with BRs, and ii) to compare two timepoints of BR application (prior to drought and during drought).
There is extremely little knowledge on the possible different response of leaves already developed before drought compared to leaves developing during this abiotic stress to the treatment with exogenous BRs. So far, only Gomes et al. (2013) examined some photosynthetic parameters in three different leaves of papaya stressed by drought and treated with BRs. They reported that BR application led to slightly more pronounced drought-induced degradation of chlorophyll (Chl) in the older leaves compared to the younger ones. On the other hand, Wang et al. (2015) compared the stomatal density, width and length in BR-treated drought-stressed young and mature leaves of grapevine plants and found that BR treatment significantly affected these parameters only in the young leaves. This seems to indicate that fully developed leaves could indeed respond to the combination of drought and BR treatment in a different manner than leaves that are still developing. However, these differences could depend on both plant species and/or the respective evaluated parameter.
Similarly, there is very little knowledge on the potentially different impact of BR treatment before or during drought. If we compare the results of studies dealing with BR application only before the stress period with studies where BRs were applied only during the stress period, we can perhaps discern slightly different responses of plants to these treatments. For example, Behnamnia et al. (2009) sprayed tomato plants before the onset of drought and found mostly increased activities of several antioxidant enzymes including CAT in their drought-stressed BR-treated plants. On the other hand, Sivakumar et al. (2017) treated plants of the same species with BRs during stress and in this case, the CAT activity decreased. However, due to the high variability of many different aspects of experimental setups in BR/drought studies, it is very complicated to ascertain whether there truly could be some significant differences between these two main potential timepoints of BR application. So far, only two groups of authors applied BRs at these timepoints in the same study in order to purposely compare the respective effects. Unfortunately, in both cases, a different type of BR application for each timepoint was used. Hashemi et al. (2015) compared the effect of seed priming before drought and leaf spraying during the stress period. They found almost no difference between these two approaches/timepoints, but each method required a different BR concentration for the maximum effect. In an earlier experiment by Farooq et al. (2009), no difference between the effect of seed soaking prior to drought and leaf spraying during the drought period was observed.
In addition to the two above-mentioned aims of our study, we further wanted to examine the effect of drought per se and in combination with the exogenous application of BRs on their endogenous levels in leaves (and roots). Our focus was again on the possible differences caused by the leaf age/development before or during the drought period. Several studies revealed that plant exposure to drought can cause changes in BR levels in pea (Jager et al., 2008), soybean (Janeczko et al., 2011), rice (Ding et al., 2014; Zhang et al., 2020; Zhang et al., 2022b), barley (Gruszka et al., 2016; Malaga et al., 2020), tobacco (Duan et al., 2017), foxtail millet (Tang et al., 2017), Chinese cabbage, white cabbage and kale (Pavlović et al., 2018), maize (Tůmová et al., 2018), Kentucky bluegrass (Chen et al., 2019) and tomato (Nie et al., 2019). However, different plant species showed a rather varied response in this respect and the majority of these studies analyzed either only the contents of total BRs or the contents of the two most biologically active BRs, i.e., castasterone (CS) and/or brassinolide (BL). No other member of big BR family was studied. Regarding the effects of the exogenous BR application on the endogenous BR levels, all studies dealing with the such topic have been made under non-stress conditions (with the exception of Efimova et al., 2014, who studied salinity stress) and focused again mostly on the total BR content (Mao et al., 2017; Fu et al., 2019; Nie et al., 2019; Setsungnern et al., 2019; Chen et al., 2021; Zhang et al., 2021; Liu et al., 2022). Beside CS and BL, some authors also determined the contents of some C27- and C29-BRs (Janeczko and Swaczynová, 2010; Janeczko et al., 2010; Janeczko et al., 2011; Filek et al., 2019; Tarkowská et al., 2020) and more detailed analyses including some BR biosynthetic precursors were performed by Bajguz et al. (2019) and Chmur and Bajguz (2021). However, all these studies were made with plants under non-stress conditions. To our knowledge, no data on the possible changes in the contents of various individual endogenous BRs or their precursors, induced by the treatment of plants with exogenously applied BRs under drought conditions, are available at this time.
Thus, the purpose of our study can be summarized into several main objectives. Firstly, we wanted to examine potential differences between the response of younger and older leaves to exogenous BR application, both in drought conditions and conditions of sufficient water supply (we hypothesized that the older leaves, as well as the leaves of stressed plants, should probably show a more pronounced response, because BRs generally seem to function particularly under suboptimal conditions). The second objective was comparison of the effect of BR application before or during drought period (our hypothesis here was that the application during drought could affect plants more strongly, because they were already stressed and BRs could thus immediately show their anti-stress effects). The third objective consisted in the evaluation of the general effect that plant treatment with 24-epiBL has on the portfolio/contents of various BRs belonging to three different structural groups, and the potential differences in this respects caused by the length of time between exogenous BR application and endogenous BR determination (we hypothesized that the effects of exogenous BRs will probably diminish with time and that plants treated with 24-epiBL, a representative of C28-BRs, could probably divert their BR biosynthesis into C29- or C27-BR biosynthetic pathways). Finally, we also wanted to examine whether the contents of individual BRs differ between younger and older leaves (and roots) and whether these contents change due to drought treatment (we expected a positive answer but could not predict the nature of these differences and/or changes).
2 Materials and methods
2.1 Plant material and cultivation conditions
The drought-sensitive maize (Zea mays L.) inbred line 2023 (Benešová et al., 2012) from the CEZEA Maize Breeding Station (Čejč, Czech Republic) was used for the experiments. Plants were grown in plastic pots (15 cm diameter, 23 cm height; 1 plant per pot) filled with the mix (10:1) of garden soil (Garden Compost, Agro CS, Czech Republic) and sand (Spielsand Sahara sand, WECO, Germany) at the greenhouse facility of the Faculty of Science, Charles Univerzity, Prague, the Czech Republic, 50°04’ N, 14°25’ E, 238 m above the sea level) under semi-controlled conditions during the spring season (April, May). The conditions in the greenhouse were: the average temperature of 25/20°C, the average relative air humidity of 60/80% day/night, natural irradiance, and watering of plants with tap water as necessary. Moderate drought stress was simulated by the cessation of watering starting at the day 35 after the date of sowing and maintained for two weeks.
The first measurements/samplings (Timepoint 1) were made from the 32 d-old plants (at this time all plants had three fully developed leaves). The second measurement/sampling point (Timepoint 2) was executed after additional 3 d when the drought simulation started. Timepoint 3 occurred after 7 d of drought and the last samplings/measurements (Timepoint 4) were at the end of the drought period. All morphological, physiological, and biochemical parameters were assessed during these four timepoints (with the exception of the determination of endogenous BR contents, which was done only at Timepoint 4). The volumetric soil water content was 24.1% at Timepoint 1, 22.6% at Timepoint 2, 22.5% for the normally-watered plants and 13.8% for stressed plants at Timepoint 3, and 21.8% for normally-watered plants and 9.3% for stressed plants at Timepoint 4.
Separate plants were used for i) plant morphology assessment (16 biologic replicates), ii) determination of the relative water content (RWC), photosynthetic pigments contents and Chl fluorescence measurements (8 biologic replicates); iii) the membrane damage index (MDI) (6 biologic replicates); iv) gas exchange measurements (6 biologic replicates); v) determination of the malondialdehyde (MDA) content (8-9 biologic replicates); vi) determination of the proline content (9 biologic replicates); and vii) determination of the BR contents (3-4 biologic replicates). In all cases, the 3rd (i.e., fully developed at the beginning of drought) or the 4th (i.e., developing during drought) leaves were used for the physiological/biochemical analyses. In addition, the BR contents were also determined in the roots.
2.2 BR treatments
The 10-6 M aqueous solution of 24-epibrassinolide (epiBL; (22R,23R,24R)-2α,3α,22,23-tetrahydroxy-24-methyl-7-oxa-7-homo-5α-cholestan-6-one; Sigma-Aldrich-Merck) used for treatments was prepared from the 10-4 M stock solution containing distilled water:96% ethanol (10:1; ethanol was used for the dissolution of epiBL and water was then gradually added with continuous stirring till the stock solution of fully dissolved epiBL was achieved) and contained also 0.05% of nonionic detergent Tween® 20. The corresponding control solution (C) had the same composition except epiBL. The concentration of epiBL was chosen based on our previous experiments (unpublished data). One group of plants was sprayed with the epiBL solution at Timepoint 1 (the BR1 variants, application prior to drought), another group of plants was sprayed at Timepoint 3 (the BR2 variants, application during drought). The respective samplings/measurements at these timepoints were always done before the epiBL treatment. In all cases, the whole aboveground part of plants was always sprayed (the amount of solution applied per plant was approximately 10 ml). Thus, at the end of the experiments (Timepoint 4), six experimental variants were available: three originated from plants that were well-watered during the whole experiment, and another three were from plants exposed to drought simulation.
2.3 Plant development and morphology
Plant development was monitored by counting the number of fully developed leaves in all plants of the respective variants throughout the whole experiment. The dry masses of the shoot (DMS) and roots (DMR) together with the plant height (measured from the surface of the soil in the pots to the youngest fully developed leaf node) were also assessed.
2.4 RWC and gas exchange
The RWC was evaluated by a standard method described in Čatský (1960). A small piece (approx. 3-4 cm2) was cut from the respective leaf and immediately weighed (FW). Then it was put into distilled water and left saturating for 5 hours. At that moment the saturated weight (SW) was obtained and the leaf piece was left to dry completely at 80°C and again weighed (DW). The RWC was calculated as (FW-DW)/(SW-DW).
The net transpiration rate (E), the stomatal conductance (gS) and the net photosynthetic rate (PN) were determined by gasometric measurements using the portable LCpro+ device (ADC BioScientific, Hoddesdon, UK) with the following conditions in the measuring chamber: the temperature 25°C, the ambient CO2 concentration 550 ± 50 μL L-1, the airflow rate 205 ± 30 μmol s-1, irradiance 650 μmol m-2 s-1 of photosynthetically active radiation. These measurements were performed between 9:00 and 12:00 AM, Central European Time, at the middle part of the respective leaves.
2.5 Photosynthetic pigments contents and Chl fluorescence (OJIP) analysis
Six small discs (0.6 cm2) were cut from the middle part of the leaf blade and incubated for 7 days in 5 mL of N,N-dimethylformamide at 4°C in the dark (the extracts were stirred several times during this period). After extraction, the absorbances at 480, 664, 647 and 710 nm were measured spectrophotometrically. The contents of Chl a, b and total carotenoids (Car) were evaluated using the formulae of Wellburn (1994).
Chl fluorescence was measured on the top side of dark-adapted (20 min) leaves with the portable fluorometer FluorPen FP100max (Photon System Instruments, Brno, Czech Republic) between 8:30 and 9:00 AM, Central European Time. The measurements were started with a saturating pulse (blue light, 455 nm, 3000 μmol m-2 s-1). After that the Chl fluorescence transient was recorded at a time scale from 10 µs to 2 ms, representing the so-called OJIP curve. Fluorescence values F0 (the initial fluorescence intensity recorded at 40 μs), FK (the fluorescence intensity at the K-step of the OJIP curve, 300 μs), FJ (the fluorescence intensity at the J-step of the OJIP curve, 2 ms), FI (the fluorescence intensity at the I-step of the OJIP curve, 30 ms), and FM=FP (the maximum fluorescence intensity) were used for the calculations of various parameters of the JIP test according to Strasser et al. (2000) and Stirbet and Govindjee (2011). These parameters can be utilized for the description of the performance of various steps of the photosynthetic electron transport chain (PETC; see Supplementary Table 1 for their definitions and formulae).
To obtain further information on the primary photosynthetic processes in various epiBL-treated/control or stressed/well-watered experimental variants, the normalizations of chlorophyll fluorescence transients leading to calculations of relative variable fluorescences WOI, WOJ and WOK were performed according to Yusuf et al. (2010). The positions/amplitudes of the WOI curves after the I-step can inform about the size of the pool of end electron acceptors in the PETC after Photosystem (PS) I (the lower positions reflect the lower size of this pool). WOJ and WOK serve for further calculations of so-called difference kinetics (ΔW), which are always based on comparisons of some treatment versus the respective control. In our case they were based either on the comparison of epiBL-treated (BR1, BR2) and control (C) plants subjected to the same watering conditions, or on the comparison of non-watered and well-watered plants subjected to the same type of treatment. The difference kinetics ΔWOJ and ΔWOK enabled us to visualize K- and L-bands of OJIP curves, respectively. If the respective K-band showed a negative amplitude, the state of the oxygen-evolving complex (OEC) of PSII was more active in the BR1/BR2 variants than in C plants (or, alternatively, in the non-watered plants than in the well-watered ones), if it showed a positive amplitude, the reverse was true. The position/amplitude of the L-band which yields information on the energetic connectivity among individual PSII units in the PETC of compared variants, can be interpreted in a similar way (Yusuf et al., 2010).
2.6 Membrane damage and proline content
The MDI was determined according to Sullivan (1972). Twelve small discs (0.6 cm2) were cut from the middle part of the leaf blade and incubated in distilled water for 24 h at 4°C. After adjustment to the room temperature, the electrical conductances of the samples were measured using the Gryf 158 conductometer (Gryf HB spol. s.r.o., Czech Republic). The samples were then boiled in a water bath for 15 min, again adjusted to room temperature and the conductances were measured again. The MDI was calculated as the ratio of the conductance values before and after boiling.
To determine the MDA content, a modified method by Hodges et al. (1999) was utilized. 0.2 g of leaf tissue were ground in liquid nitrogen and then homogenized in 80% ethanol and centrifuged at 14000× g and 4°C for 20 min. The supernatant was added separately to the thiobarbituric acid (TBE) reaction mixture (TBA+; 0.65% TBA, 20% trichloroacetic acid and 0.01% butylated hydroxytoluene) and the TBA- reaction mixture (20% trichloroacetic acid and 0.01% butylated hydroxytoluene). In the next step, the samples with TBA+/- reaction mixtures were temperated at 95°C for 30 min. After cooling to room temperature, the samples were centrifuged again (14000× g, 4°C, 20 min). The absorbance of the supernatant was measured at 440 nm, 532 nm and 600 nm. The MDA content was calculated according to Hodges et al. (1999).
The proline content was determined spectrophotometrically according to Bates et al. (1973). The frozen samples were homogenized in 3% aqueous sulfosalicylic acid and treated with acid-ninhydrin and acetic acid. This reaction mixture was boiled in a water bath for 30 min and then cooled on ice. 3 mL of toluene were added to the cooled samples. After phase stabilization (approximately 20 min at room temperature), the absorbance of the toluene phase was measured at 520 nm and the proline content was calculated based on its calibration curve.
2.7 BR contents
Contents of various BRs were determined after their extraction and purification using ultra-high performance liquid chromatography (UHPLC) followed by mass spectrometry ((+)ESI-MS/MS) analysis according to Tarkowská et al. (2016).
Frozen maize tissue samples of 50 mg FW were homogenized to a fine consistency using 2.8-mm zirconium oxide beads (Retsch GmbH & Co. KG, Haan, Germany) and a MM 400 vibration mill (Retsch GmbH & Co. KG, Haan, Germany) at a frequency of 27 Hz for 3 min. The samples were then extracted overnight with stirring at 4°C using a benchtop laboratory rotator Stuart SB3 (Bibby Scientific Ltd., Staffordshire, UK) after adding 1 mL ice-cold 60% acetonitrile and a mixture of stable isotope internal standards (OlChemIm Ltd., Olomouc, Czech Republic) including [2H3]BL, [2H3]CS, [2H3]24-epiBL, [2H3]24-epiCS, [2H3]28-norBL, [2H3]28-norCS, [2H3]TY, [2H3]campestanol, [2H3]campesterol, [2H3]6-deoxocathasterone, [2H3]6-deoxotyphasterol and [2H3]6-oxocampestanol. The samples were further centrifuged, purified on polyamide SPE columns (Supelco, Bellefonte, PA, USA) and then analyzed by UHPLC-MS/MS (Micromass, Manchester, UK). The data were analyzed using Masslynx 4.2 software (Waters, Milford, MA, USA) and the BR contents were finally quantified by the standard isotope-dilution method (Rittenberg and Foster, 1940).
2.8 Statistical analysis
The original data are shown in Supplementary File 1. Mean values and standard deviations (SD) were calculated for all parameters. The data were first subjected to Welch´s ANOVA. Where appropriate, the pairwise differences between experimental variants were analyzed using Welch’s t-tests, for multiple comparisons, Games-Howell post hoc tests were applied. The data from the 3rd and 4th leaves were statistically analyzed separately because it was not technically possible to determine BR contents (or some of the evaluated biochemical parameters) in all sample variants (3rd and 4th leaves, roots) together in one run and we did not want to introduce artificial differences to our data that could be actually caused by the different analytical runs.
3 Results
3.1 Timepoint 1
The data from Timepoint 1 are shown in Supplementary File 1; they were obtained only in order to characterize basal levels of plant performance prior to any epiBL treatment or stress induction. Thus, there is no point in their presentation here or in the subsequent discussion.
3.2 Timepoint 2 (no drought, early epiBL treatment)
The BR1 treatment significantly reduced levels of proline in the 4th leaves whereas in the 3rd leaves this treatment had no effect (Supplementary Table 2). Slight OEC inactivation and reduction of the energetic connectivity among PSII complexes (inferred from the positions of the ΔWOJ or ΔWOK curves above zero), as well as moderate reduction in the pool size of end electron acceptors in the PETC (inferred from the relative positions of the respective WOI curves) in the 3rd leaves of the epiBL-treated plants (but not in the 4th leaves), was suggested by the graphical analysis of Chl fluorescence curves (Figure 1, Supplementary Figure 1). Aside from this, plants of the BR1 and C variants did not significantly differ in any other parameter at this timepoint (Supplementary Table 2).
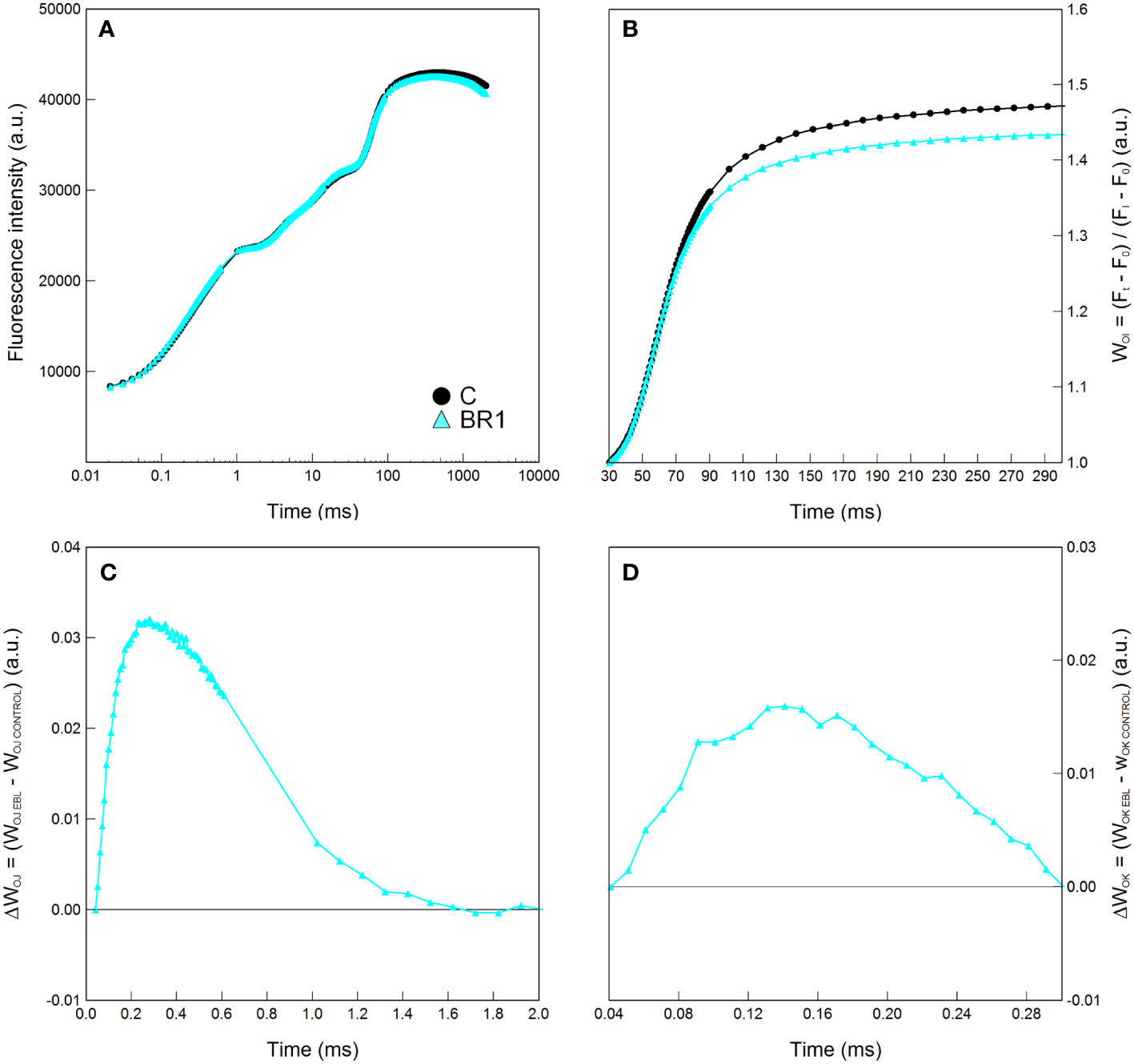
Figure 1 Graphical analysis of the OJIP chlorophyll fluorescence data measured in maize 3rd leaves at Timepoint 2. Plants were well-watered and either treated with 24-epibrassinolide (the BR1 variant) or with water (the C variant). The OJIP curve (A), the relative variable fluorescence WOI (B) and the difference kinetics ΔWOJ (C) and ΔWOK (D) are shown. Only the part between the I and P points of the OJIP curve is shown for the WOI. ΔWOJ reveals the K-band; ΔWOK reveals the L-band and were calculated from the comparison of BR1 and C variants; the latter are represented by the zero point of the respective y axes in graphs in panels (C, D). Mean values (n = 8) are shown. a.u., alternative units.
3.3 Timepoint 3 (drought, early epiBL treatment)
One week of drought stress significantly reduced the plant height in comparison to the well-watered plants, as well as the DMS of the epiBL-treated plants (Table 1). RWC, E, PN and gS values also significantly decreased due to drought (Table 1; for RWC, this decrease was statistically significant only in the 3rd leaves of plants treated with the control solution, for the gas exchange parameters, it was significant only in the 4th leaves of both epiBL-treated and control plants and in the 3rd leaves of the epiBL-treated plants). Similar drought-induced reduction was observed for the contents of photosynthetic pigments in the 3rd leaves; this was more pronounced in the control plants compared to the epiBL-treated ones (Table 1). One week of drought led also to an increase of the MDI values in the leaves of the control plants and the proline content in the leaves of both control and epiBL-treated plants, but not to any significant changes in the MDA content (Table 1). The efficiency of the PETC did not seem to be particularly affected by drought at this timepoint (Figure 2, Supplementary Figure 2); the exceptions being mostly the parameters related to the electron transport after PSII (φRE01, ψRE01, δRE01, RE01/RC, PITOTAL) in the 4th leaves of the epiBL-treated plants (Table 1).
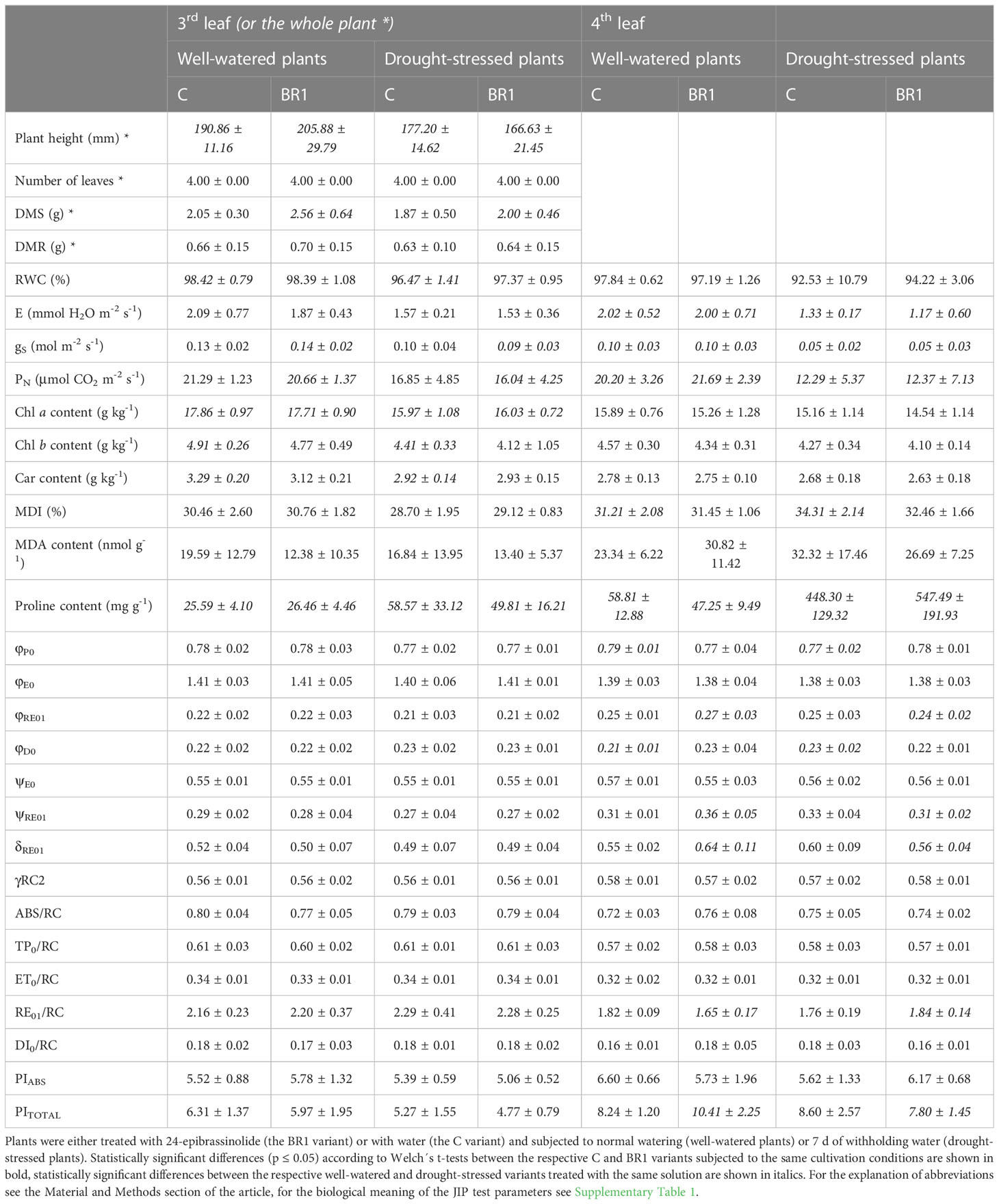
Table 1 Selected morphological, physiological and biochemical parameters measured at Timepoint 3 in maize leaves.
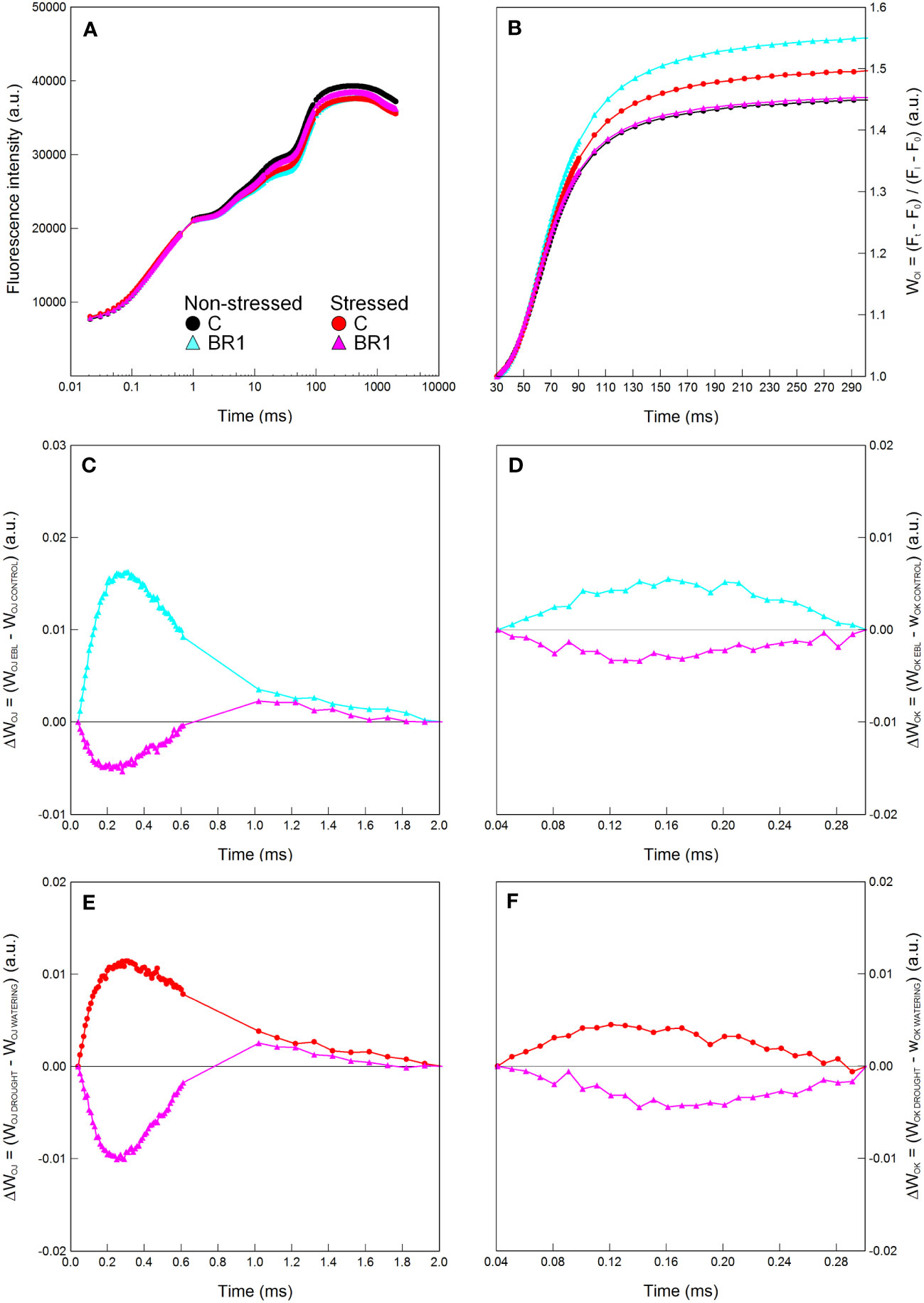
Figure 2 Graphical analysis of the OJIP chlorophyll fluorescence data measured in maize 4th leaves at Timepoint 3. Plants were either treated with 24-epibrassinolide (the BR1 variant) or with water (the C variant) and subjected to normal watering (non-stressed plants) or 7 d of withholding water (stressed plants). The OJIP curve (A), the relative variable fluorescence WOI (B) and the difference kinetics ΔWOJ (C, E) and ΔWOK (D, F) are shown. ΔWOJ and ΔWOK were calculated either from the comparison of the respective BR1 and C variants subjected to the same watering regime (C, D) or from the comparison of the respective non-stressed and stressed plants subjected to the same type of treatment (E, F). For other information see legend to Figure 1.
The early epiBL treatment had no statistically significant effect on the values of most parameters evaluated in plants subjected to either well-watered or drought conditions, with the exception of the DMS which was greater in the well-watered BR1 plants compared to the respective C variant. Additionally, their 4th leaves were again characterized by significantly lower contents of proline. They also showed higher values of the JIP test parameters related to the electron transport after PSII (Table 1) and had a greater size of the pool of end electron acceptors in the PETC (Figure 2); however, this did not apply to the 3rd leaves of these plants or to the drought-stressed epiBL-treated plants (Figure 2, Supplementary Figure 2). The inactivation of the OEC in the 4th leaves of the epiBL-treated well-watered plants appeared at this timepoint as well, although to a less extent than in the 3rd leaves at the Timepoint 2. However, in the drought-stressed plants, the epiBL treatment positively affected the OEC function (Figure 2). No particular effect of the early epiBL treatment on the energetic connectivity between PS II units was found at this timepoint (Figure 2).
3.4 Timepoint 4 (drought, early and late epiBL treatments)
Further drought-induced reduction of the values of all morphological parameters in comparison to the well-watered plants was observed after an additional week of drought simulation with the only exception for the DMR of the C variant (Figure 3). The same applied to the RWC and all gas exchange parameters; the reduction of the values of these parameters was more pronounced in the 3rd leaves than in the 4th leaves (Figures 4, 5). The contents of photosynthetic pigments and the efficiency of the PETC also significantly decreased due to drought. In this case, both leaves responded more-or-less similarly, although the most pronounced changes for the 3rd leaves were usually observed in the BR2 variants, whereas for the 4th leaves in the C variants (Figures 4–7, Supplementary Table 3). On the other hand, the exposure of plants to drought caused a significant increase in the dissipation of the excess excitation energy in the PETC (Supplementary Table 3) and the MDI values (similar in both leaves, Figures 4, 5). The same applied to the content of proline; the change of this parameter was particularly pronounced in the 4th leaves (Figures 4, 5). We found almost no statistically significant differences in the MDA content between drought-stressed and well-watered plants, probably due to the high biologic variability of the samples (Figures 4, 5).
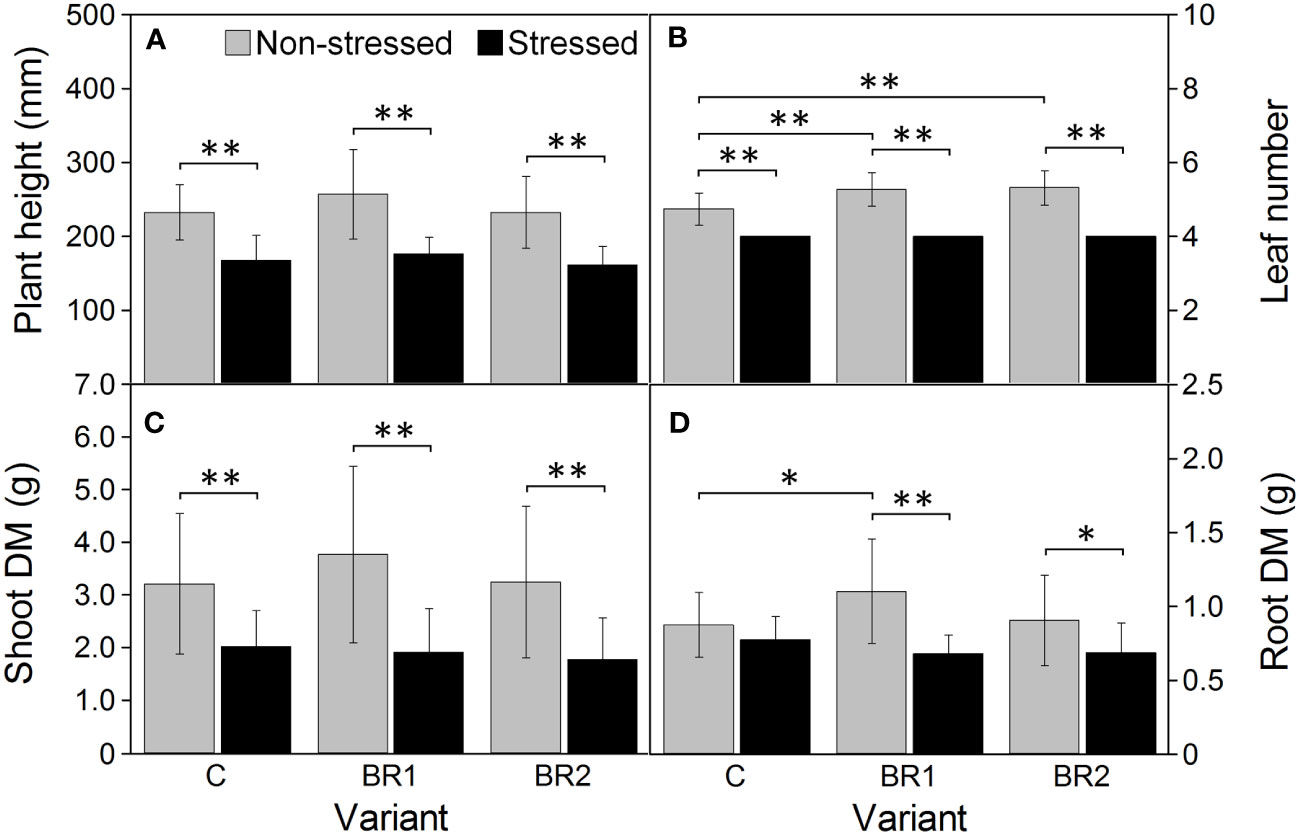
Figure 3 Plant height (A), the number of fully developed leaves (B), the dry mass of shoot (C) and the dry mass of roots (D) measured in maize at Timepoint 4. Plants were subjected to normal watering (non-stressed plants) or 14 d of withholding water (stressed plants) and treated with 24-epibrassinolide (the BR1 and BR2 variants) or with water (the C variant) either before the start of drought period (C, BR1) or during the drought period (BR2). Mean values ± SD are shown (n = 16). Asterisks indicate significant (p ≤ 0.05; *) or highly significant (p ≤ 0.01; **) differences between mean values according to Welch´s t-tests, resp. Games-Howell tests, made separately for each treatment (in case of the differences between non-stressed and stressed plants), resp. for each watering regime (in case of the differences between C, BR1 and BR2 variants).
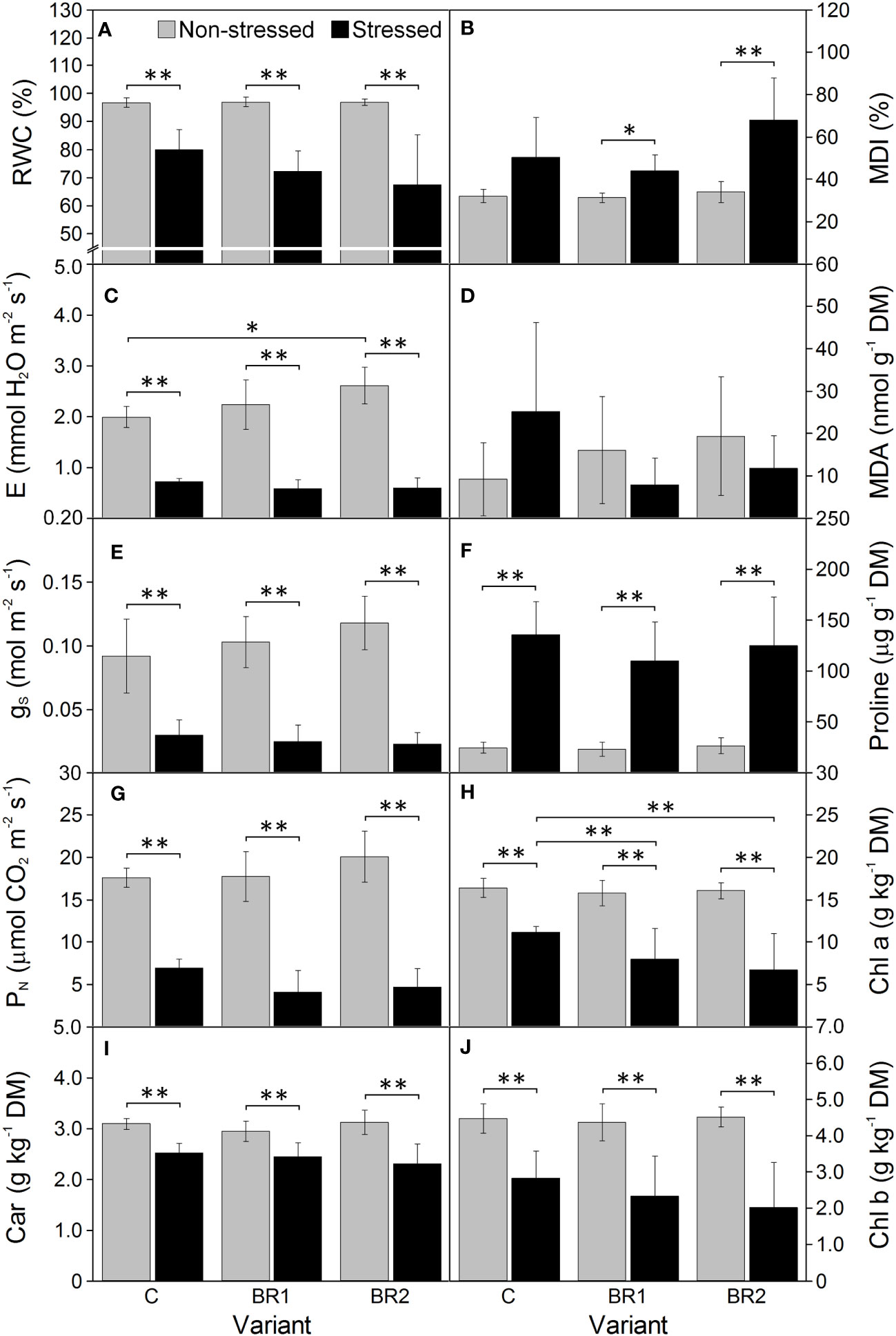
Figure 4 The relative water content (A), the membrane damage index (B), the transpiration rate (C), the malondialdehyde content (D), the stomatal conductance (E), the proline content (F), the net photosynthetic rate (G), the contents of chlorophylls a and b (H, J) and total carotenoids (I) measured in maize 3rd leaves at Timepoint 4. Plants were subjected to normal watering (non-stressed plants) or 14 d of withholding water (stressed plants) and treated with 24-epibrassinolide (the BR1 and BR2 variants) or with water (the C variant) either before the start of drought period (C, BR1) or during the drought period (BR2). Mean values ± SD are shown (n = 6 for gas exchange parameters, 8-9 for other parameters). For other information see legend to Figure 3. DM, dry matter. Asterisks indicate significant (p ≤ 0.05; *) or highly significant (p ≤ 0.01; **) differences between mean values according to Welch´s t-tests, resp.
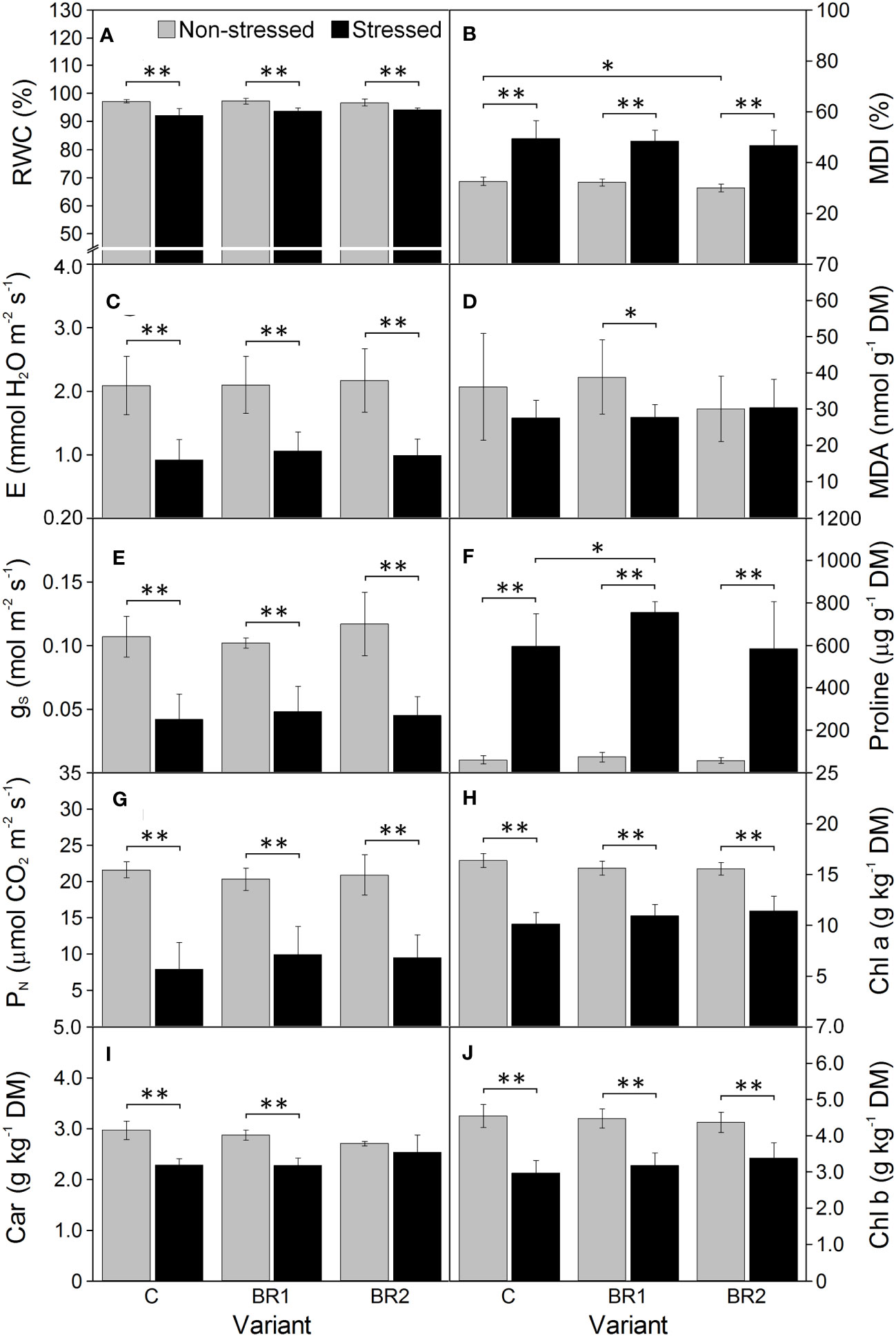
Figure 5 The relative water content (A), the membrane damage index (B), the transpiration rate (C), the malondialdehyde content (D), the stomatal conductance (E), the proline content (F), the net photosynthetic rate (G), the contents of chlorophylls a and b (H, J) and total carotenoids (I) measured in maize 4th leaves at Timepoint 4. Plants were subjected to normal watering (non-stressed plants) or 14 d of withholding water (stressed plants) and treated with 24-epibrassinolide (the BR1 and BR2 variants) or with water (the C variant) either before the start of drought period (C, BR1) or during the drought period (BR2). Mean values ± SD are shown (n = 6 for gas exchange parameters, 8-9 for other parameters). For other information see legend to Figure 3. DM, dry matter. Asterisks indicate significant (p ≤ 0.05; *) or highly significant (p ≤ 0.01; **) differences between mean values according to Welch´s t-tests, resp.
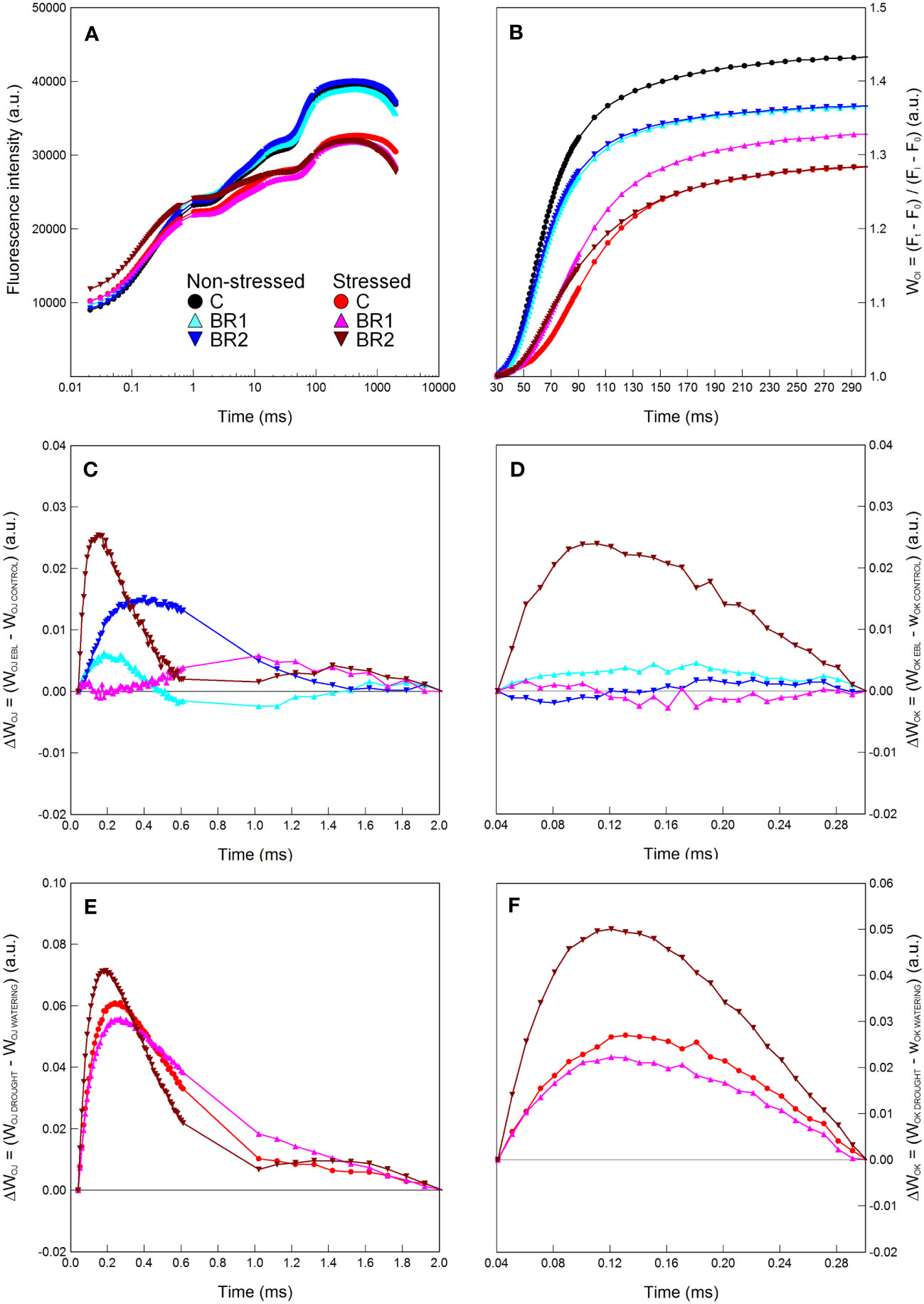
Figure 6 Graphical analysis of the OJIP chlorophyll fluorescence data measured in maize 3rd leaves at Timepoint 4. Plants were either treated with 24-epibrassinolide (the BR1 and BR2 variants) or with water (the C variant) either before the start of drought period (C, BR1) or during the drought period (BR2), and subjected to normal watering (non-stressed plants) or 14 d of withholding water (stressed plants). The OJIP curve (A), the relative variable fluorescence WOI (B) and the difference kinetics ΔWOJ (C, E) and ΔWOK (D, F) are shown. ΔWOJ and ΔWOK were calculated either from the comparison of the respective BR1/BR2 and C variants subjected to the same watering regime (C, D) or from the comparison of the respective non-stressed and stressed plants subjected to the same type of treatment (E, F). For other information see legend to Figure 1.
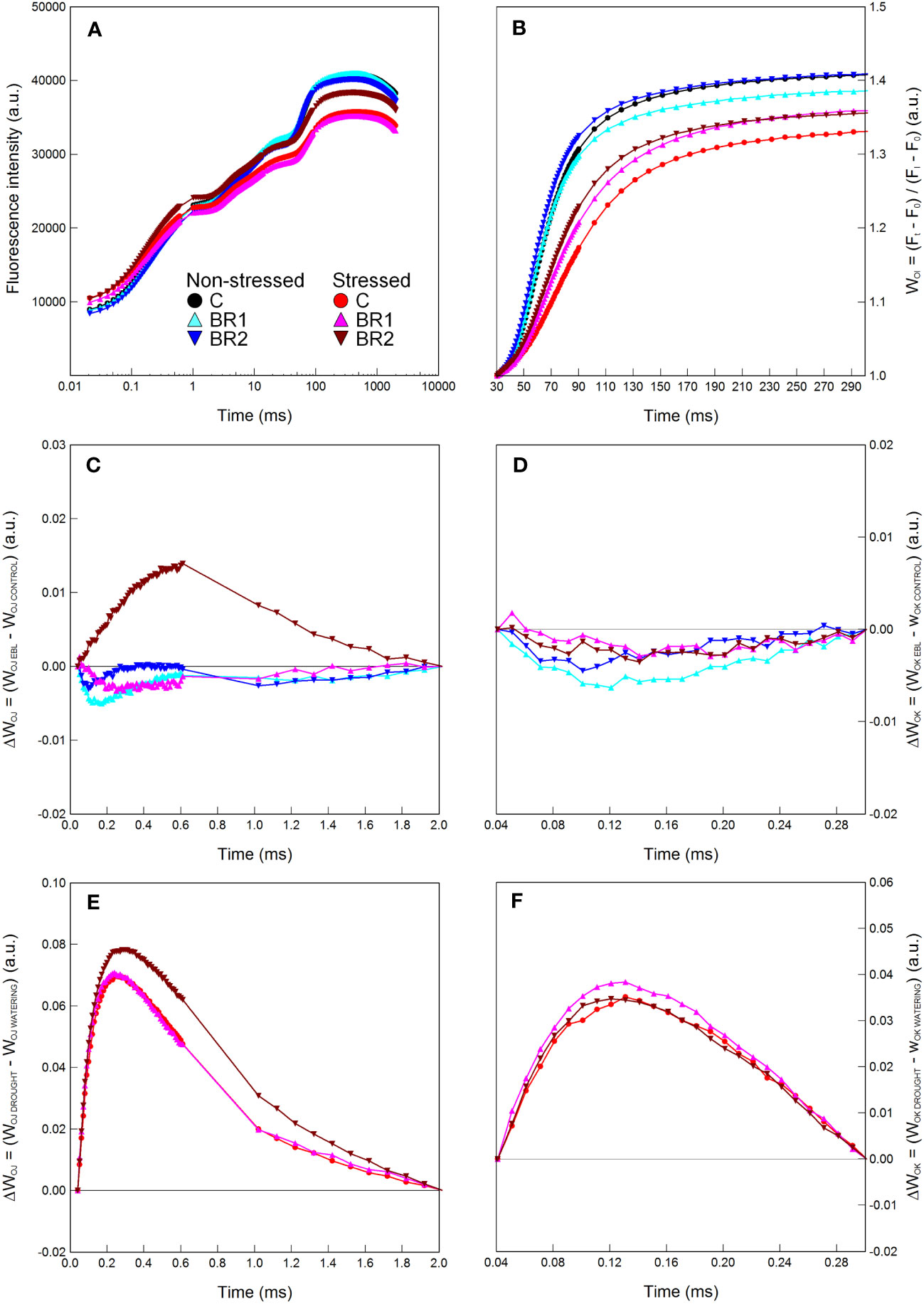
Figure 7 Graphical analysis of the OJIP chlorophyll fluorescence data measured in maize 4th leaves at Timepoint 4. Plants were either treated with 24-epibrassinolide (the BR1 and BR2 variants) or with water (the C variant) either before the start of drought period (C, BR1) or during the drought period (BR2), and subjected to normal watering (non-stressed plants) or 14 d of withholding water (stressed plants). The OJIP curve (A), the relative variable fluorescence WOI (B) and the difference kinetics ΔWOJ (C, E) and ΔWOK (D, F) are shown. ΔWOJ and ΔWOK were calculated either from the comparison of the respective BR1/BR2 and C variants subjected to the same watering regime (C, D) or from the comparison of the respective non-stressed and stressed plants subjected to the same type of treatment (E, F). For other information see legend to Figure 1.
The BR1 group of well-watered plants showed significantly higher DMR values in comparison to the C group and the number of fully developed leaves was also significantly higher in both epiBL-treated groups under these cultivation conditions (Figure 3). Regarding the DMS and the plant height, although we could find a slight trend of an epiBL-caused increase of the values of these parameters in the well-watered plants, due to the high biologic variability these differences were not statistically significant. Under drought conditions, neither BR1 nor BR2 variants significantly differed from their respective control in either morphological parameter (Figure 3).
There were almost no statistically significant differences in the RWC or the gas exchange parameters between our epiBL-treated and non-treated plants, although a trend of a slight increase of values of E, gS and PN from the C to the BR2 group could be seen in the 3rd leaves of the well-watered plants (Figure 4). Neither there were any significant differences between the epiBL-treated and non-treated variants in the contents of photosynthetic pigments. The exception from this was the Chl a content in the 3rd leaves of the drought-stressed plants, which was significantly lower in both BR1 and BR2 variants (Figure 4). The JIP test parameters describing the efficiency of the PETC also did not show any effect of the epiBL treatment either in the well-watered or in the drought-stressed plants (Supplementary Table 3). However, the late epiBL treatment showed a negative effect on the function of the OEC, as well as on the energetic connectivity among PSII complexes in the 3rd leaves of our drought-stressed plants (Figure 6); whereas in the 4th leaves or in the well-watered plants these effects were negligible (Figure 7). The size of the pool of end electron acceptors in the PETC was also slightly reduced after both epiBL treatments in the 3rd leaves of the well-watered plants, whereas for the drought-stressed plants, the BR1 variant showed a greater size of this pool compared to the C or BR2 variants (Figure 6). The differences between individual variants in this respect were less obvious in the 4th leaves (Figure 7).
Although the BR1 and BR2 variants exposed to drought showed a reduction of the MDA content in their 3rd leaves in comparison with the respective control, these differences were statistically non-significant due to the high biologic variability of samples (Figure 4). No significant differences were found between our epiBL-treated and non-treated plants in the MDI (the only exception being the difference between the BR2 and C variants in the 4th leaves of the well-watered plants; Figure 5). The proline content was significantly higher in the 4th leaves of the drought-stressed BR1 variant in comparison with the drought-stressed C variant (Figure 5).
Drought caused significant increase of the levels of BR biosynthetic precursors CR and CN in the 3rd leaves of the control plants while this increase was insignificant in root tissue (Figures 8, 9). In the 4th leaves, the levels of these compounds were much lower and no changes due to plant exposure to drought were observed (Figure 10). The levels of TY, a direct precursor of bioactive CS, were reduced at the end of the drought simulation period in leaves of the C and BR2 variants (and insignificantly also in roots; Figures 8–10). The levels of CS also showed a certain reduction, but statistically significant difference was found only for the 4th leaves of the C variant and roots of the BR2 variant, mostly due to the otherwise high variability of samples taken from the well-watered plants (Figures 8–10). A similar situation was observed for the content of a C29-analogue of CS, homoCS, with the exception that a significant difference from the C variant was found in the 3rd leaves (Figures 8–10). No statistically significant drought-induced changes in the norCS (C27-BR) levels were found, although there was a visible trend of the increase of the contents of this compound in the drought-stressed plants of C and BR2 variants compared to the well-watered ones (Figures 8–10). There were also no statistically significant differences in the BL content between the well-watered and the drought-stressed plants either in the 3rd leaves or in roots (Figures 8, 9). However, drought led to a significant reduction of the content of the most biologically active BR brassinolide in the 4th leaves of plants not treated with epiBL, i.e., BL (Figure 10). Maize was found to be capable for synthesizing 28-homodolichosterone (homoDS) whose levels showed also a reduction as a direct result of drought in both leaves and roots, resp. (Figures 8–10). The presence of epiBL and norBL was detected only in the 3rd leaves and (in much lower amounts) in roots (Figures 8, 9). The levels of these BRs did not significantly differ between well-watered and stressed plants.
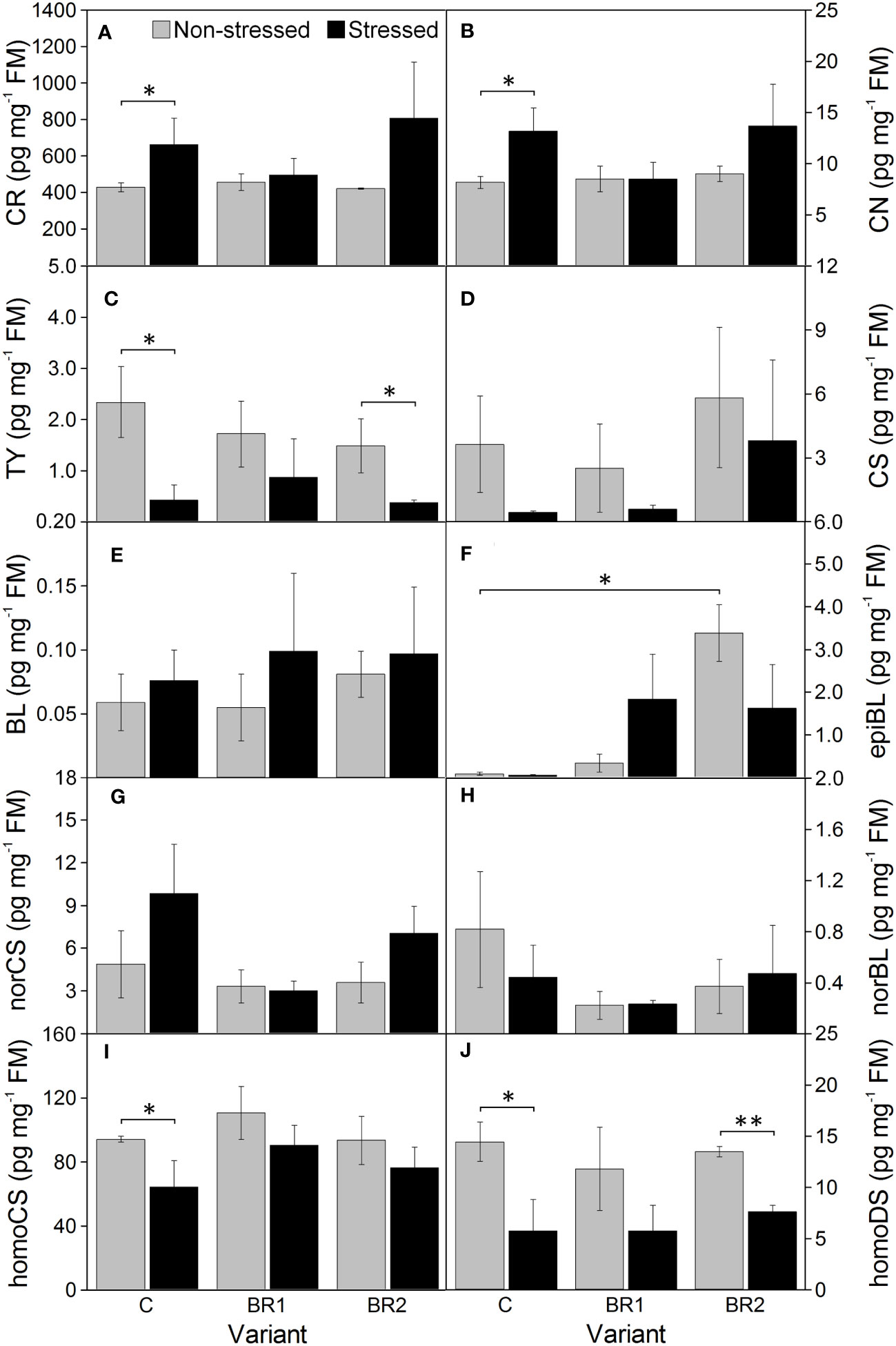
Figure 8 The contents of campesterol (A), campestanol (B), typhasterol (C), castasterone (D), brassinolide (E), 24-epibrassinolide (F), 28-norcastasterone (G), 28-norbrassinolide (H), 28-homocastasterone (I) and 28-homodolichosterone (J) measured in maize 3rd leaves at Timepoint 4. Plants were subjected to normal watering (non-stressed plants) or 14 d of withholding water (stressed plants) and treated with 24-epibrassinolide (the BR1 and BR2 variants) or with water (the C variant) either before the start of drought period (C, BR1) or during the drought period (BR2). Mean values ± SD are shown (n = 4). For other information see legend to Figure 3. FM, fresh matter. Asterisks indicate significant (p ≤ 0.05; *) or highly significant (p ≤ 0.01; **) differences between mean values according to Welch´s t-tests, resp.
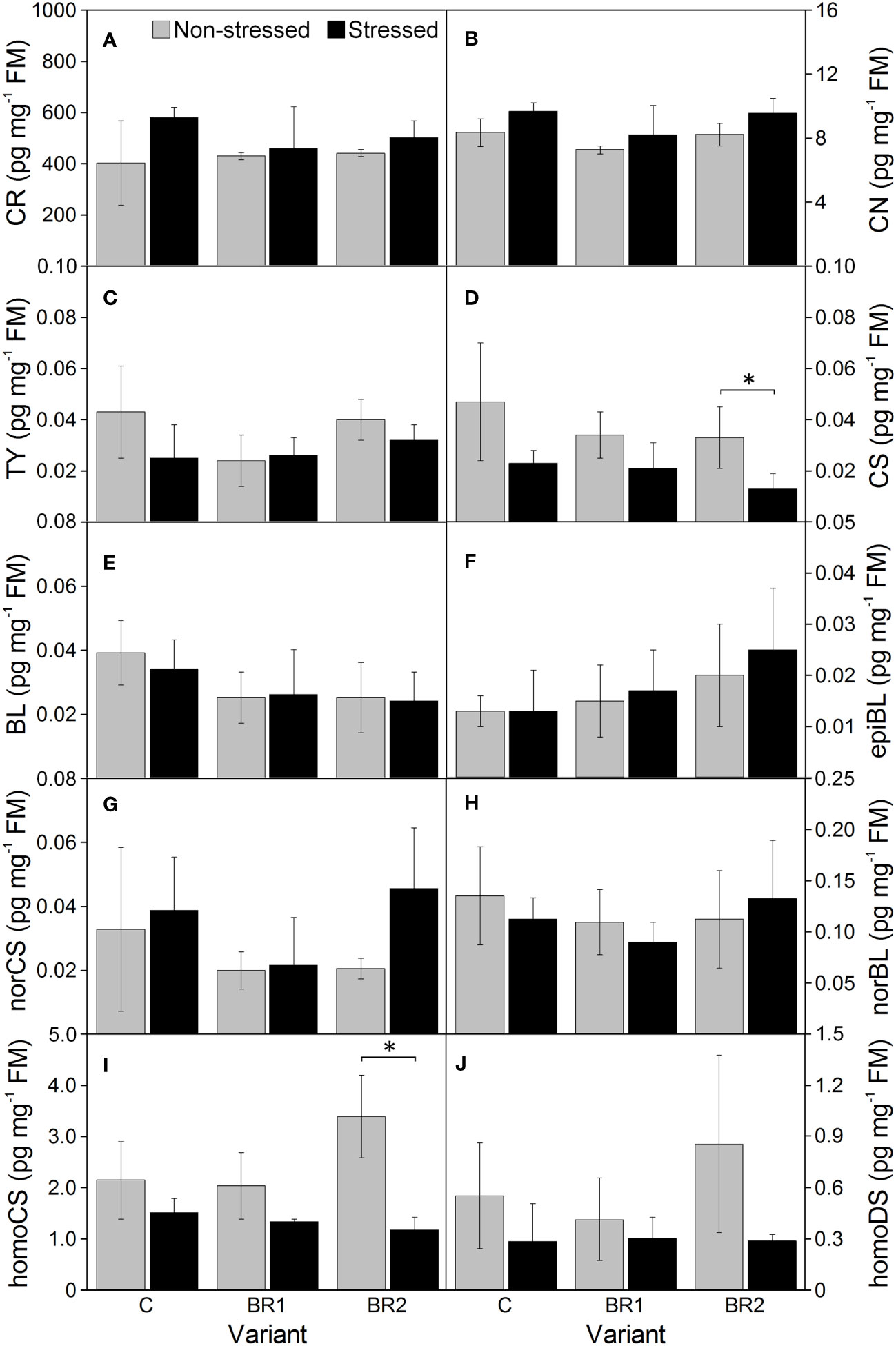
Figure 9 The contents of campesterol (A), campestanol (B), typhasterol (C), castasterone (D), brassinolide (E), 24-epibrassinolide (F), 28-norcastasterone (G), 28-norbrassinolide (H), 28-homocastasterone (I) and 28-homodolichosterone (J) measured in maize roots at Timepoint 4. Plants were subjected to normal watering (non-stressed plants) or 14 d of withholding water (stressed plants) and treated with 24-epibrassinolide (the BR1 and BR2 variants) or with water (the C variant) either before the start of drought period (C, BR1) or during the drought period (BR2). Mean values ± SD are shown (n = 4). For other information see legend to Figure 3. FM, fresh matter. Asterisks indicate significant (p ≤ 0.05; *) or highly significant (p ≤ 0.01; **) differences between mean values according to Welch´s t-tests, resp.
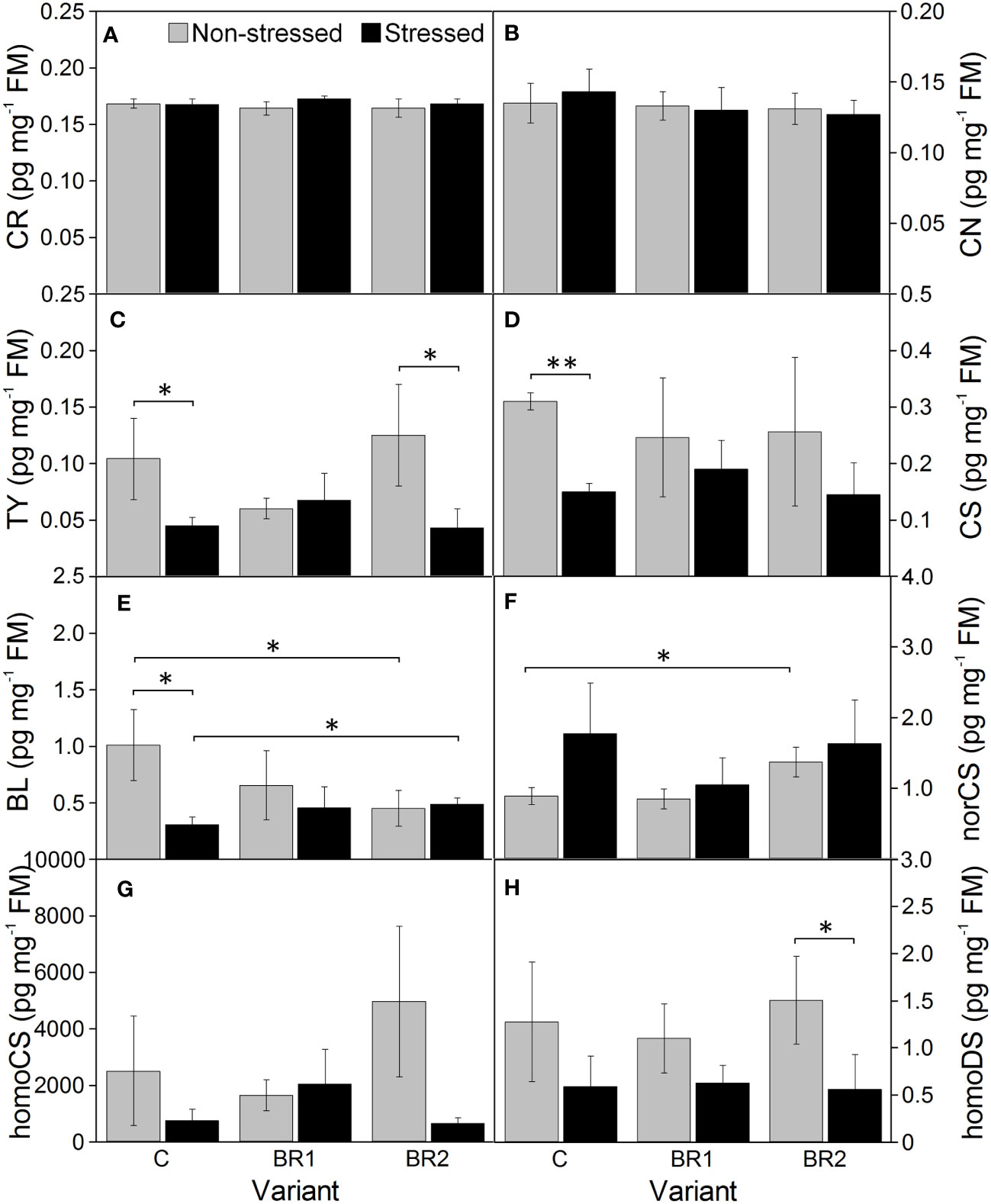
Figure 10 The contents of campesterol (A), campestanol (B), typhasterol (C), castasterone (D), brassinolide (E), 28-norcastasterone (F), 28-homocastasterone (G), and 28-homodolichosterone (H) measured in maize 4th leaves at Timepoint 4. Plants were subjected to normal watering (non-stressed plants) or 14 d of withholding water (stressed plants) and treated with 24-epibrassinolide (the BR1 and BR2 variants) or with water (the C variant) either before the start of drought period (C, BR1) or during the drought period (BR2). Mean values ± SD are shown (n = 4). For other information see legend to Figure 3. FM, fresh matter.
The exogenous epiBL treatment did not significantly affect the levels of endogenous BRs detected in leaves or roots of our experimental plants (Figures 8–10). The exception from this were BL levels in the 4th leaves of the BR2 variants, which significantly differed from the C variants regardless of the cultivation conditions (although with opposite trends; Figure 10). The 4th leaves of the well-watered BR2 plants had also higher levels of norCS (and, non-significantly, also of homoCS) compared to their controls (Figure 10). A statistically significant increase in the epiBL levels was observed in the 3rd leaves of the well-watered BR2 variant. There was also an increase in the stressed BR1 and BR2 variants. However, in this case, it was not statistically significant (Figure 8).
4 Discussion
BRs are generally considered to play many beneficial roles in plant development, physiology and biochemistry, particularly in plants subjected to various sub-optimum conditions. Their exogenous application was shown to improve plant response to both abiotic and biotic stress factors, such as high or low temperature, water deficit or water excess, high or low irradiance, salinity, nutrient deficiency or excess, exposure to heavy metals, herbicides, pesticides, viral or bacterial pathogens, fungi, etc. The number of studies dealing with this topic reaches many hundreds and as such, exceeds the possibilities of this paper to be thoroughly discussed. However, although many studies are currently available, various aspects of BR relationship to plant stress response still remain very poorly examined or not explored at all. Our experiments presented in this paper tried to add to our knowledge on some of these poorly understood topics. Thus, in the following paragraphs we will focus only on the five main aspects of our study, as stated in the list of our objectives at the end of the Introduction section. The reader interested in the general topic of BRs in relation to a particular type of plant stress, is referred to recent reviews and book chapters, e.g., (Ahammed et al., 2020; Ahammed et al., 2022), Ramirez and Poppenberger (2020); Basit et al. (2021); Li et al. (2021); Rehman et al. (2022); Sidhu and Bali (2022) and many others.
4.1 Effects of exogenous BR application on leaves of different age
One of the aims of our study was to analyze whether the exogenous application of BRs might differently mitigate the negative impact of drought on plants in two different leaves: those that were already fully developed at the beginning of stress and those that were still developing during stress period. Several authors (Mostajeran and Rahimi-Eichi, 2009; Gilgen and Feller, 2014; Budič et al., 2016) found that older leaves are more affected by drought than younger leaves. However, in relation to the BRs role in plant drought response, this aspect has not been previously examined. We exposed 5-weeks old maize plants to gradually induced moderate drought stress, which significantly reduced the values of the majority of the observed parameters associated with plant morphology and physiology. A trend of decreasing values after the exogenous epiBL application was found in the older, 3rd leaves, especially for the RWC and parameters characterizing photosynthesis. On the other hand, no such trend was observed for the younger 4th leaves for which we even registered a few signs of BR-induced improvement at the end of our experiments (efficiency of some parts of the PETC and the proline content).
The difference in observed responses of analyzed leaves to BRs application could be connected to BR-induced acceleration of leaf senescence. Several authors (e.g., Sağlam-Çağ, 2007; Bariş and Sağlam-Çağ, 2016; Fedina et al., 2017; Kim et al., 2019) showed that BRs promote leaf senescence (although the exact mechanism is still unknown and might be probably an indirect one; Jan et al., 2019). This is usually accompanied by an increase in membrane damage, reduced Chl contents and decreased photosynthetic efficiency. Thus, the older (3rd) leaves of our drought-stressed plants treated with epiBL could, particularly at Timepoint 4, already enter the beginning stage of the senescence (reflected in the more pronounced reduction of their Chl content and the PETC efficiency compared to the non-treated stressed plants). Even before the beginning of the drought simulation, the 3rd leaves of plants treated with epiBL already showed a worse efficiency of their PETC than the non-treated plants. On the other hand, the 4th leaves were younger so they have not entered the senescence phase even at Timepoint 4 and the BR treatment thus did not affect them negatively. Gomes et al. (2013) also found lower chlorophyll content as a sign of the beginning of senescence in the older drought-stressed papaya leaves after BR treatment but not in the younger ones (this did not apply to the non-stressed plants, similarly to our results). Interestingly, while the later study of these authors made with the same species (Gomes et al., 2018) confirmed that the development of younger leaves does not seem to be particularly accelerated by BRs, leaves that were already mature at the time of BR application showed slightly delayed senescence in BR-treated plants in this case. However, the respective plants were not subjected to any stressor so perhaps it might be the combination of stress and the BR treatment that particularly accelerates leaf senescence.
The expected mitigating effect of BRs on our drought-stressed plants was thus found only in their 4th leaves, but it was very mild and usually statistically non-significant. The reason for this could be that the 4th leaves of our plants did not particularly suffer from drought stress (their RWC values were still above 90% even after 2 weeks of drought simulation). The overwhelming majority of the authors that analyzed the BR/drought relationship by measuring various physiological and biochemical leaf parameters did not state the water status of leaves of their experimental plants at all and those that did usually worked with severely stressed leaves (RWC much below 70% or leaf water potential below -1.5 MPa) (Holá, 2019). It is thus highly probable that there was no opportunity for the epiBL treatment to show its potential as a drought-mitigating agens because the 4th leaves of our experimental plants simply did not need it.
The observed change in proline levels in the response to epiBL treatment was another interesting phenomenon found only in the 4th leaves of our plants. We found that the epiBL application prior to any drought simulation results in significantly reduced proline contents in these leaves, but only in non-stressed plants. Furthemore, this effect did not persist with time: it was observed only during Timepoints 2 and 3, while at Timepoint 4 it faded away and was replaced by a positive effect of epiBL pre-treatment on proline levels in the drought-stressed plants. Proline in drought-stressed plants generally functions as an osmoprotectant and its increased accumulation also results in plant protection against oxidative stress (Ghosh et al., 2022). The majority of authors who analyzed the proline content in leaves of drought-stressed plants treated with exogenous BRs (or plants overproducing BRs due to transgenic modification of some BR biosynthetic gene) reported a positive effect of such treatment on this parameter. However, such effects were usually observed only in stressed plants, not in well-watered (control) ones, where BR treatment either did not have any significant effect or even resulted in reduced proline levels (e.g., Zhang et al., 2008; Anjum et al., 2011; Li and Feng, 2011; Talaat et al., 2015; Ahmed et al., 2017; Duan et al., 2017; Khamsuk et al., 2018; Kaya et al., 2019). Although Fu et al. (2019) reported that exogenous epiBL pre-treatment significantly elevates the expression of two key proline biosynthetic genes, P5CS and P5CR (as well as the activities of the respective enzymes and the proline content) in Elymus nutans plants, this applied only for plants subjected to cold stress but not for their non-stressed plants. On the other hand, Ábrahám et al. (2003) showed that treatment of non-stressed Arabidopsis plants with exogenous epiBL inhibits the expression of a P5CS gene and thus actually leads to reduced proline levels. It is possible that something similar occurred in our experimental plants. Exogenous BR treatment per se certainly does not seem to specifically induce proline biosynthesis (it can even act antagonistically) and it is only in combination with drought (or some other stress factor) that BR application can result in elevated proline levels and thus better osmoprotective and antioxidant parameters of BR-treated plants.
The reason that the effects of epiBL application took place only in the younger leaves of our plants is currently unknown. Whether it could be somehow associated with the general differences between younger and more mature (or senescing) leaves in proline levels (which can be different in various plant species, e.g., Cechin et al., 2006; Xue et al., 2008; Sperdouli and Moustakas, 2015), and whether it could be somehow associated with differences in the contents of specific brassinosteroids between these two types of leaves (see below) remains to be seen. The detailed examination of the expression of various genes associated with proline metabolism and/or transport together with a thorough analysis of the activities of the respective proteins in BR-treated, drought-stressed (or non-stressed) plants at different timepoints after the onset of drought and/or BR application, and in leaves in different developmental stage would be certainly worthwhile and while such analysis was outside the scope of our experiments presented in this paper, it is something we would like to focus on in the future.
4.2 Effects of exogenous BR application before or during drought
The second aspect we examined in our work was the role of a time when BRs were applied to our experimental plants. We used the epiBL application before (BR1 variants) and during (BR2 variants) drought simulation but we found almost no difference in the BR effects on plant physiology and morphology between these two timepoints. The earlier application slightly improved the plant morphology of our non-stressed plants (perhaps because epiBL had more time to act on plant growth and development), whereas the later application resulted in slightly higher gas exchange (particularly in their 3rd leaves). However, these differences were mostly negligible and certainly did not apply to the drought-stressed plants. There is very little knowledge on the difference between BR applications before drought and during drought. In two studies available on this topic, the authors used a different type of application and BR concentration for each timepoint, which makes a subsequent comparison inappropriate (Farooq et al., 2009; Hashemi et al., 2015). However, even in these cases, the authors did not find any difference between the effect of BR pre-treatment and post-treatment on their drought-stressed plants. The only marked difference between our drought-stressed BR1 and BR2 variants was that there was a clearly evident more negative effect of epiBL applied during the stress period on the performance of the OEC and the excitonic connectivity among individual PSII complexes observed in their 3rd leaves but not in the 4th ones. The primary photosynthetic processes are always more affected by BR treatment in more intensively stressed leaves and the OEC seems to be particularly sensitive to any change in BR homeostasis (Holá, 2022). However, all things considered, it certainly does not seem that the time of exogenous BR application is a particulary important factor that influences the response of drought-stressed plants to these phytohormones.
4.3 Effects of exogenous BR application on endogenous C27, C28-and C29- BRs
Our selection of these two separate timepoints for the BR application also meant that the endogenous BR contents were determined in the leaves and roots of our plants at either 17 d (BR1 variants, i.e., plants treated at Timepoint 1) or 7 d (BR2 variants, treated at Timepoint 3) after this application, respectively. Although in most cases the differences in the contents of individual BRs and/or their precursors between BR1/BR2 variants and the non-treated plants were not statistically significant, there was a clearly pronounced trend of a higher increase of the amounts of C29-BRs (homoCS, homoDS) in the 4th leaves and roots of the non-stressed BR2 variant. For norCS, which is a representative of C27-BRs, this increase in the 4th leaves was even statistically significant, whereas the content of BL (a representative of C28-BRs) significantly increased under stress conditions (particularly after the later BR application) but showed an opposite trend in our well-watered plants. These observed trends suggest that the changes in the levels of endogenous BRs caused by the exogenous BR application probably diminish with time (e.g. due to metabolization – see Schneider et al., 1994; Kolbe et al., 1995) and that the shorter period between the BR treatment and the analysis of endogenous BR contents is better for them to be detectable. This is supported also by our observation that the contents of epiBL in the 3rd leaves of our plants significantly increased in the order of C < BR1 < BR2, following the decreasing length of the period between epiBL spraying and BR content determination. We think that the detected epiBL levels in our BR1 and BR2 variants could be residues after the respective epiBL treatments, which persisted in a tissue of interest to the last sampling point. A similar situation was described for another monocot (wheat) plant by Janeczko and Swaczynová (2010). The absence of epiBL in the 4th leaves could be explained either by the fact that these leaves were yet rather small during the spraying and thus offered less area for BR entry (the BR1 variant), or by the possibility that these leaves could use a completely different mechanism how to deal with exogenously applied BRs. For instance, Nishikawa et al. (1995) showed that while hypocotyls and roots of cucumber are not much able to metabolize epiBL, petioles and leaves do have this ability. A similar situation was observed by the same research team in intact wheat seedlings. Thus, there might be some differences in the metabolization of particular exogenous substance in various plant parts/organs in this respect.
The effect of the exogenous BR application on the endogenous BR contents depends not only on when but also on how BRs are applied, as well as on plant species and BR concentration. We utilized foliage spraying as it is the most frequently employed method in BR/drought studies. However, the majority of information available on the possible changes in endogenous BR contents caused by the exogenous BR application comes from studies where BRs were either added directly into the growth medium and plants received them through roots, or by soaking of seeds in BR solutions (Supplementary File 2). Only Janeczko and Swaczynová (2010) sprayed wheat plants in the V2 stage with epiBL solutions and they did not observe any changes in the CS or BL contents in the 3rd leaves of their plants 11 d after spraying. Janeczko et al. (2011) also used direct injection of epiBL into the 1st and 2nd leaves of barley. Using this approach, they did not found any significant effect on endogenous CS or BL contents in the 7th leaf after 20 d. Notably, both CS and BL belong to the C28-BR group and none of these authors analyzed C27- or C29-BRs in their plants at that time. In later studies, some authors that applied BRs directly into the growth medium or by seed soaking also observed an increase in the levels of C27- or C29-BRs but not C28-BRs (Filek et al., 2019; Tarkowská et al., 2020) while others found that such type of application can elevate the contents of C28-BRs as well (depending on the concentration and/or plant species and analyzed plant organ) (Bajguz et al., 2019; Chmur and Bajguz, 2021). It seems that while the application of BRs into growth medium, particularly in hydroponic cultures, can evidently affect their contents in leaves/shoots (either by enabling their direct transport through a plant as suggested by Janeczko and Swaczynová, 2010, or, more probably, by an indirect effect on the regulation of BR biosynthesis), the direct treatment of leaves/shoots (as in our case) seems to have a more negligible effect on the endogenous BR levels. This is probably due to the fact that BRs applied in this manner are not transported over long distances (Symons and Reid, 2004; Symons et al., 2008) and their effect is only temporary and diminishes with time.
4.4 Effects of analyzed organ and leaf age on endogenous C27, C28-and C29-BRs
The biosynthetic precursors of C29-BRs (as well as the respective end products in monocots) are frequently found to be present in much higher amounts compared to C28-BRs in various plant species that have been examined to this date (Supplementary File 2). This is in good agreement with our findings: both the 3rd and 4th leaves and roots of our plants contained more homoCS and homoDL than BRs belonging to the C28 group. When the levels of BRs and/or their precursors in leaves and roots of our experimental plants were compared, we also found a good agreement between our data and the majority of data published previously by authors who simultaneously analyzed endogenous BR levels in shoots/leaves and roots of various plant species (Supplementary File 2). The contents of CS and BL are consistently reported to be higher in the aboveground vegetative parts of plants than in roots and our results supports this observation as well. The contents of TY were found by some authors to be also higher in leaves than in roots (Jiang et al., 2012, in rice, Pavlović et al., 2019, in Chinese cabbage, Lu et al., 2021 in sunflower), which also corresponds to our observations and to the results of earlier reports describing the lowest levels of BRs in root tissue (Bancos et al., 2002; Shimada et al., 2003; Symons and Reid, 2004). In contrast, there are some other authors who reported either similar levels of TY in shoots and roots or even TY elevation in roots (Supplementary File 2). Altogether it seems that the differences in the TY content between shoots/leaves and roots might depend on plant species or some other experimental aspects. A similar discrepancy of data can be observed also for CR and CN: while we found comparable amounts of these C28-BR precursors in the 3rd leaves and roots of our maize plants (much lower amounts were detected in the 4th leaves) and Nakamura et al. (2006) reported higher levels of CR and CN in rice shoots than in roots, the opposite situation was found by Shimada et al. (2003) and Kim et al. (2006) for Arabidopsis. Regarding C27- and C29-BRs, our data were again in good agreement with the findings of Yokota et al. (2001) and Luo et al. (2018), who reported lower levels of the end products of the respective biosynthetic pathways (or even the complete absence of these BRs) in roots of tomato and rice as compared to shoots.
The situation with the BR contents in the 3rd and the 4th leaf is more interesting than the simple comparison of BR quantity in leaves and roots. Actually, we think that this is probably our most interesting finding presented in this study. The aspect of the comparison of a profile of BRs belonging to various structural groups in younger and more mature leaves has not been much dealt with. When searching the available literature, we found only two studies discussing the content and portfolio of C27- to C29-BRs in the leaves of different ages. Zhang et al. (2022a) reported a slightly higher content of CS (a C28-BR) for older leaves compared with younger leaves of their tea (Camellia sinensis) plants. This is in agreement with our own findings, although in our case, the difference between leaves was of a much higher order of magnitude. On the other hand, the amount of norCS (a C27-BR, which is synthesized mostly from 6-oxocholestanol with cholesterol and cholestanol as earlier precursors (Bajguz et al., 2020) was lower in the 4th (younger) leaves of our plants, whereas Zhang et al. (2022a) did not report any differences. The second study published on this topic is that of Parada et al. (2022) using a grapevine (Vitis vinifera) as a model plant. In their case, CS and homoDS (C28- and C29-BRs, resp.) were detected in neither young nor mature leaves, epiCS was present in the young leaves only and the level of norTE and homoCS (C27- and C29-BRs, resp.) was higher in the mature leaves compared to younger ones. This is inconsistent with our results where the levels of homoCS are about an order of magnitude higher in our younger leaves (4th) than in the older leaves (3rd).
Our above-mentioned data lead strongly to the suggestion that the younger, 4th leaves of maize probably for some reason divert their BR biosynthesis from C28-BRs (TY, CS, BL, epiBL) to C29-BRs like homoCS. The biosynthesis of C27-, C28- and C29-BRs is interconnected at various levels. CS can be synthesized from many C27- to C29-BRs precursors including norCS, homoCS and homoDS (Bajguz et al., 2020). However, we think that the divergence of BR biosynthesis in our younger leaves probably occurs at the very early biosynthetic steps, prior to the beginning of the late C-22 oxidation pathway, because the 4th leaves appeared to be a very poor source of CR and CN. Thus, the BR biosynthesis in the younger maize leaves (at least in this inbred line) is probably strongly redirected from the CR (and/or cholesterol) branches to the parallel sitosterol branch of the BR biosynthetic pathway (Bajguz et al., 2020; Wei and Li, 2020). This might explain the highly elevated level of homoCS then. With respect to the levels of the homoDS, one can only speculate that within the sitosterol branch the biosynthesis is not further diverted via 22S-hydroxyisofucosterol, which is proposed to be a precursor of homoDS (Bajguz et al., 2020).
4.5 Effects of drought on endogenous C27, C28-and C29-BRs
The final part of our experiment to be discussed is the effect of drought per se on the levels of individual BRs and their precursors. We found that drought slightly enhanced amounts of CR and CN and reduced amounts of TY, homoCS and homoDS in the leaves (and to a less extent in roots) of our experimental plants. The reduction of homoCS levels was particularly pronounced in the 4th leaves. Drought also led to reduced levels of CS in the 3rd leaves (in the 4th leaves there were mostly no changes or even an increase), while for BL the situation was usually the opposite. Regarding C27-BRs, exposure of plants to drought was associated with generally higher amounts of norCS but mostly no changes of the norBL content. The observed changes in the levels of individual BRs are generally consistent with the results of our previous study made with the same inbred line of maize (Tůmová et al., 2018). Similarly to that study, we also did not detect the presence of either DS, DL, homoBL, homoDL or norTE. The unchanged levels of CS in our 4th leaves are also in agreement with previous studies on drought-stressed young pea leaves (Jager et al., 2008), tobacco leaves (Duan et al., 2017) or rice shoots (Ding et al., 2014). Additionally, in the last study, BL was detected in well-watered plants but not in the drought-stressed ones.
As stated above, CR and CN are precursors in the late C-22 oxidation pathway leading to the biosynthesis of the C28-BRs (Bajguz et al., 2020). Their slightly increased accumulation in our drought-stressed plants together with the reduced levels of the products of the subsequent early C-6 oxidation pathway (TY, CS) could indicate at least partial inhibition of this BR biosynthetic pathway after plant exposure to drought. However, CS can be also synthesized from homoCS and homoDS (Bajguz et al., 2020) and the reduced levels of these two BRs in our plants suggest the association of drought exposure not only with the inhibition of the C28-BRs biosynthetic pathway but also with the reduced biosynthesis of C29-BRs. Alternatively, CS can be also synthesized from norCS (Bajguz et al., 2020), and here the observed reduction of CS amounts in our 3rd leaves together with the accumulation of norCS could perhaps mean that the C27-biosynthesis per se is not inhibited by drought in maize but that the conversion of norCS to CS is. Thus, we suggest that drought inhibits C28-BRs and C29-BRs biosynthetic pathways whereas C27-BRs biosynthesis is not particularly affected. Further, the inhibition of the biosynthesis of C28-BRs applies particularly for the older leaves whereas the inhibition of C29-BR biosynthesis is more pronounced in the younger ones (which can be associated with the above-mentioned preferences for one or the other biosynthetic pathway in these two types of leaves).
It is worth emphasizing that this endogenous BR response to drought in maize might not be a universal response and does not have to apply to other plant species (or even cultivars of the same species). Even closely related species can show very different responses of their endogenous BR contents to drought as demonstrated by Pavlović et al. (2018) who analyzed three species of Brassica under control and drought conditions. They found a reduction of TY amounts, an elevation of CS amounts, and an even more significant elevation of BL amounts in their stressed Chinese cabbage plants whereas there were no changes in the other two species except a mild reduction of BL levels in kale and a mild increase of TY levels in white cabbage (Pavlović et al., 2018). Unfortunately, scientists who studied endogenous BR contents in plants under drought conditions usually analyzed only several (or only one) C28-BRs and no C27- or C29-BRs (Supplementary File 2). Only Gruszka et al. (2016) found increased CS levels and mostly unchanged homoBL levels in barley exposed to the insufficient water supply as compared to the well-watered plants. On the other hand, Malaga et al. (2020) reported mostly no changes for CS but a significant increase of homoCS amounts in leaves of some drought-sensitive barley cultivars but not in cultivars with moderate or high drought tolerance. Our previous study with maize showed intraspecific differences in drought-induced changes of endogenous BR levels as well: the drought-tolerant inbred line had been analyzed together with the drought-sensitive one and differed in the contents of some individual BRs (particularly TY, norCS, norBL) both under normal and drought conditions (Tůmová et al., 2018). Thus, to better understand the relationship between drought and biosynthesis/metabolism of various BRs, more detailed analyses of more plant species, more cultivars of the same species, made under mild, moderate, or severe drought conditions, and including plants/leaves of different developmental stages are clearly very much needed.
5 Conclusions
The results of our experiments presented in this paper offered several interesting answers to the five main objectives of our study. Firstly, when determining the response of these two types of leaves to the combination of drought exposure and the application of exogenous epiBL, we found that the response of leaves of different ages differs: the older leaves can show accelerated senescence under such conditions reflected in their reduced chlorophyll content and diminished efficiency of the primary photosynthetic processes, whereas the younger leaves are characterized by interesting changes of proline levels in response to epiBL treatment (this deserves a further, more detailed exploration). Secondly, the exogenous application of epiBL before or during drought did not result in a different response of plants to this stress factor (which disproved our original hypothesis on this topic). Thirdly, we showed that the contents of C27- and C29-BRs in plants treated with exogenous epiBL depended on the length of time between this treatment and the BR analysis (which confirmed our original hypothesis) Finally, we demonstrated marked differences in the contents of individual BRs between younger and older maize leaves, which is, in our opinion, the most interesting result of our study. We propose that the younger maize leaves divert their BR biosynthesis from C28-BRs to C29-BRs, probably at the very early biosynthetic steps (prior to the beginning of the late C-22 oxidation pathway). Drought also apparently negatively affected biosynthetic pathways of C28-BRs (particularly in the older leaves) and C29-BRs (particularly in the younger leaves) but not C27-BRs in our maize plants.
Data availability statement
The original contributions presented in the study are included in the article/Supplementary Material. Further inquiries can be directed to the corresponding author.
Author contributions
HM and DH conceived the project, DT performed endogenous brassinosteroids measurements, HM and PČ carried out other biochemical analyses, MK and OR performed in situ measurements and samplings. HM, DH and DT drafted the manuscript and all authors reviewed and approved the manuscript. All authors contributed to the article and approved the submitted version.
Funding
The research was supported by the Charles University Grant Agency (grant no. 364721), the Charles University research funding projects SVV 260568 and 260679 and the Ministry of Education, Youth and Sports of the Czech Republic (European Regional Development Fund-Project ‘Centre for Experimental Plant Biology’ no. CZ.02.1.01/0.0/0.0/16_019/0000738).
Acknowledgments
We sincerely thank Magdalena Vlčková for her technical help with endogenous brassinosteroids measurements.
Conflict of interest
The authors declare that the research was conducted in the absence of any commercial or financial relationships that could be construed as a potential conflict of interest.
Publisher’s note
All claims expressed in this article are solely those of the authors and do not necessarily represent those of their affiliated organizations, or those of the publisher, the editors and the reviewers. Any product that may be evaluated in this article, or claim that may be made by its manufacturer, is not guaranteed or endorsed by the publisher.
Supplementary material
The Supplementary Material for this article can be found online at: https://www.frontiersin.org/articles/10.3389/fpls.2023.1139162/full#supplementary-material
Supplementary File 1 | The original data on the brassinosteroid contents, plant morphology and various physiological and biochemical characteristics measured at four different timepoints in the 3rd and 4th leaves (or roots) of maize subjected to normal watering or withholding water and treated with 24-epibrassinolide (the BR1 and BR2 variants) or with water (the C variant) either before the start of drought period (C, BR1) or during the drought period (BR2).
Supplementary File 2 | Heat maps showing the approximate contents of various brassinosteroids (BRs) and their precursors in the non-reproductive parts/organs of various plant species as reported from previously published studies. Only studies dealing with i) the effect of exogenous BR application on their endogenous contents; ii) the analysis of at least some BRs from both C28-, C29- and/or C27-groups at the same time; iii) the analysis of BR contents in both shoots/leaves and roots at the same time, or iv) the analysis of BR contents in plants subjected to drought are included here.
References
Ábrahám, E., Rigó, G., Székely, G., Nagy, R., Koncz, C., Szabadosz, L. (2003). Light-dependent induction of proline biosynthesis by abscisic acid and salt stress is inhibited by brassinosteroid in Arabidopsis. Plant Mol. Biol. 51, 363–372. doi: 10.1023/a:1022043000516
Ahammed, G. J., Li, X., Liu, A., Chen, S. (2020). Brassinosteroids in plant tolerance to abiotic stress. J. Plant Growth Regul. 39, 1451–1464. doi: 10.1007/s00344-020-10098-0
Ahammed, G. J., Sharma, A., Yu, J. (2022). Brassinosteroids in plant developmental biology and stress tolerance (Cambridge: Academic Press). doi: 10.1016/C2016-0-04006-8
Ahmed, A. H. H., Darwish, E., Alobaidy, M. G. (2017). Impact of putrescine and 24-epibrassinolide on growth, yield and chemical constituents of cotton (Gossypium barbadense l.) plant grown under drought stress conditions. Asian J. Plant Sci. 16, 9–23. doi: 10.3923/ajps.2017.9.23
Anjum, S. A., Wang, L. C., Farooq, M., Hussain, M., Xue, L. L., Zou, C. M. (2011). Brassinolide application improves the drought tolerance in maize through modulation of enzymatic antioxidants and leaf gas exchange. J. Agron. Crop Sci. 197, 177–185. doi: 10.1111/j.1439-037X.2010.00459.x
Bajguz, A., Chmur, M., Gruszka, D. (2020). Comprehensive overview of the brassinosteroid biosynthesis pathways: substrates, products, inhibitors, and connections. Front. Plant Sci. 11. doi: 10.3389/fpls.2020.01034
Bajguz, A., Orczyk, W., Gołębiewska, A., Chmur, M., Piotrowska-Niczyporuk, A. (2019). Occurrence of brassinosteroids and influence of 24-epibrassinolide with brassinazole on their content in the leaves and roots of Hordeum vulgare l. cv. golden promise. Planta. 249, 123–137. doi: 10.1007/s00425-018-03081-3
Bancos, S., Nomura, T., Sato, T., Molnar, G., Bishop, G. J., Koncz, C., et al. (2002). Regulation of transcript levels of the Arabidopsis cytochrome P450 genes involved in brassinosteroid biosynthesis. Plant Physiol. 130, 504–513. doi: 10.1104/pp.005439
Bariş, Ç.Ç., Sağlam-Çağ, S. (2016). The effects of brassinosteroids on sequential leaf senescence occurring in Glycine max l. Int. J. Biotechnol. Res. 6, 7–16.
Basit, F., Liu, J., An, J., Chen., M., He, C., Zhu, X., et al. (2021). Brassinosteroids as a multidimensional regulator of plant physiological and molecular responses under various environmental stresses. Environ. Sci. pollut. Res. Int. 28, 44768–44779. doi: 10.1007/s11356-021-15087-8
Bates, L. S., Waldren, R. P., Teare, I. D. (1973). Rapid determination of free proline for water-stress studies. Plant Soil. 39, 205–207. doi: 10.1007/BF00018060
Behnamnia, M., Kalantari, K., Ziaie, J. (2009). The effects of brassinosteroid on the induction of biochemical changes in Lycopersicon esculentum under drought stress. Turk. J. Bot. 33, 417–428. doi: 10.3906/bot-0806-12
Benešová, M., Holá, D., Fischer, L., Jedelský, P. L., Hnilička, F., Wilhelmová, N., et al. (2012). The physiology and proteomics of drought tolerance in maize: early stomatal closure as a cause of lower tolerance to short-term dehydration? PloS One 7, e38017. doi: 10.1371/journal.pone.0038017
Budič, M., Cigić, B., Šoštarič, M., Sabotič, J., Meglič, V., Kos, J., et al. (2016). The response of aminopeptidases of Phaseolus vulgaris to drought depends on the developmental stage of the leaves. Plant Physiol. Biochem. 109, 326–336. doi: 10.1016/j.plaphy.2016.10.007
Čatský, J. (1960). Determination of water deficit in disks cut out from leaf blades. Biol. Plant 2, 76–78.
Cechin, I., Rossi, S. C., Oliveira, V. C., Fumis, T. F. (2006). Photosynthetic tresponses and proline content of mature and young sunflower leaves under water deficit. Photosynthetica. 44, 144–461. doi: 10.1007/s11099-005-0171-2
Chandrasekaran, P., Sivakumar, R., Nandhitha, G., Vishnuveni, M., Boominathan, P., Senthilkumar, M. (2017). Impact of PPFM and PGRs on seed germination, stress tolerant index and catalase activity in tomato (Solanum lycopersicum l) under drought. Int. J. Curr. Microbiol. App. Sci. 6, 540–549. doi: 10.20546/ijcmas.2017.606.064
Chen, Y., Chen, Y., Shi, Z., Jin, Y., Sun, H., Xie, F., et al. (2019). Biosynthesis and signal transduction of ABA, JA, and BRs in response to drought stress of Kentucky bluegrass. Int. J. Mol. Sci. 20, 1289. doi: 10.3390/ijms20061289
Chen, Y., Chen, H., Xiang, J., Zhang, Y., Wang, Z., Zhu, D., et al. (2021). Rice spikelet formation inhibition caused by decreased sugar utilization under high temperature is associated with brassinolide decomposition. Environ. Exp. Bot. 190, 104585. doi: 10.1016/j.envexpbot.2021.104585
Chmur, M., Bajguz, A. (2021). Brassinolide enhances the level of brassinosteroids, protein, pigments, and monosaccharides in Wolffia arrhiza treated with brassinazole. Plants. 10, 1311. doi: 10.3390/plants10071311
Ding, J., Wu, J. H., Liu, J. F., Yuan, B. F., Feng, Y. Q. (2014). Improved methodology for assaying brassinosteroids in plant tissues using magnetic hydrophilic material for both extraction and derivatization. Plant Methods 10, 39. doi: 10.1186/1746-4811-10-39
Duan, F., Ding, J., Lee, D., Lu, X., Feng, Y., Song, W. (2017). Overexpression of SoCYP85A1, a spinach cytochrome p450 gene in transgenic tobacco enhances root development and drought stress tolerance. Front. Plant Sci. 8. doi: 10.3389/fpls.2017.01909
Efimova, M. V., Savchuk, A. L., Hasan, J. A. K., Litvinovskaya, R. P., Khripach, V. A., Kholodova, V. P., et al. (2014). Physiological mechanisms of enhancing salt tolerance of oilseed rape plants with brassinosteroids. Russ. J. Plant Physiol. 61, 733–743. doi: 10.1134/S1021443714060053
Farooq, M., Wahid, A., Basra, S. M. A., Din, I. U. (2009). Improving water relations and gas exchange with brassinosteroids in rice under drought stress. J. Agron. Crop Sci. 195, 262–269. doi: 10.1111/j.1439-037X.2009.00368.x
Fedina, E., Yarin, A., Mukhitova, F., Blufard, A., Chechetkin, I. (2017). Brassinosteroid-induced changes of lipid composition in leaves of Pisum sativum l. during senescence. Steroids. 117, 25–28. doi: 10.1016/j.steroids.2016.10.009
Filek, M., Sieprawska, A., Kościelniak, J., Oklestkova, J., Jurczyk, B., Telk, A., et al. (2019). The role of chloroplasts in the oxidative stress that is induced by zearalenone in wheat plants–the functions of 24-epibrassinolide and selenium in the protective mechanisms. Plant Physiol. Biochem. 137, 84–92. doi: 10.1016/j.plaphy.2019.01.030
Fu, J., Sun, P., Luo, Y., Zhou, H., Gao, J., Zhao, D., et al. (2019). Brassinosteroids enhance cold tolerance in Elymus nutans via mediating redox homeostasis and proline biosynthesis. Environ. Exp. Bot. 167, 103831. doi: 10.1016/j.envexpbot.2019.103831
Ghosh, U. K., Islam, M. N., Siddiqui, M. N., Cao, X., Khan, M. A. R. (2022). Proline, a multifaceted signalling molecule in plant responses to abiotic stress: understanding the physiological mechanisms. Plant Biol. 24, 227–239. doi: 10.1111/plb.13363
Gilgen, A. K., Feller, U. (2014). Effects of drought and subsequent rewatering on Rumex obtusifolius leaves of different ages: reversible and irreversible damages. J. Plant Interact. 9, 75–81. doi: 10.1080/17429145.2013.765043
Gomes, M. M. A., Pinheiro, D. T., Bressan-Smith, R., Campostrini, E. (2018). Exogenous brassinosteroid application delays senescence and promotes hyponasty in Carica papaya l. leaves. Theor. Exp. Plant Physiol. 30, 193–201. doi: 10.1007/s40626-018-0114-5
Gomes, M. M. A., Torres Netto, A., Campostrini, E., Bressan-Smith, R., Zullo, M. A. T., Ferraz, T. M., et al. (2013). Brassinosteroid analogue affects the senescence in two papaya genotypes submitted to drought stress. Theor. Exp. Plant Physiol. 25, 186–195.
Gruszka, D., Janeczko, A., Dziurka, M., Pociecha, E., Oklešťková, J., Szarejko, I. (2016). Barley brassinosteroid mutants provide an insight into phytohormonal homeostasis in plant reaction to drought stress. Front. Plant Sci. 7. doi: 10.3389/fpls.2016.01824
Gursude, A., Mandavia, C. K., Mandavia, M. K., Raval, L., Bangar, S. (2014). Influence of brassinosteroids and gibberellic acid on biochemical parameters of chickpea (Cicer arietinum l.) under water stress. Ind. J. Agric. Biochem. 27, 227–230.
Hashemi, N. B., Sadeghipour, O., Asl, A. R. (2015). The study effect of brassinosteroid application on yield and yield components of cowpea (Vigna unguiculata) under water stress conditions. Int. J. Biol. Pharm. Allied Sci. 4, 593–605.
Hemmati, K., Ebadi, A., Khomari, S., Sedghi, M. (2018). Influence of ascorbic acid and 24-epibrassinolide on physiological characteristics of pot marigold under water-stress condition. J. Plant Interact. 13, 364–372. doi: 10.1080/17429145.2018.1483033
Hodges, D. M., DeLong, J. M., Forney, C. F., Prange, R. K. (1999). Improving the thiobarbituric acid-reactive-substances assay for estimating lipid peroxidation in plant tissues containing anthocyanin and other interfering compounds. Planta. 207, 604–611. doi: 10.1007/s004250050524
Holá, D. (2019). “Role of brassinosteroids in the plant response to drought: do we know anything for certain?,” in Brassinosteroids: plant growth and development. Eds. Hayat, S., Yusuf, M., Bhardwaj, R., Bajguz, A. (Singapore: Springer), 101–168. doi: 10.1007/978-981-13-6058-9_5
Holá, D. (2022). “Brassinosteroids and primary photosynthetic processes,” in Brassinosteroids in plant developmental biology and stress tolerance. Eds. Ahammed, G. J., Sharma, A., Yu, J. (Cambridge: Academic Press), 59–104. doi: 10.1016/B978-0-12-813227-2.00015-1
Jager, C. E., Symons, G. M., Ross, J. J., Reid, J. B. (2008). Do brassinosteroids mediate the water stress response? Physiol. Plant 133, 417–425. doi: 10.1111/j.1399-3054.2008.01057.x
Jan, S., Abbas, N., Ashraf, M., Ahmad, P. (2019). Roles of potential plant hormones and transcription factors in controlling leaf senescence and drought tolerance. Protoplasma. 256, 313–329. doi: 10.1007/s00709-018-1310-5
Janeczko, A., Biesaga-Kościelniak, J., Oklešt'ková, J., Filek, M., Dziurka, M., Szarek-Łukaszewska, G., et al. (2010). Role of 24-epibrassinolide in wheat production: physiological effects and uptake. J. Agron. Crop Sci. 196 (4), 311–321. doi: 10.1111/j.1439-037X.2009.00413.x
Janeczko, A., Biesaga-Koscielniak, J., Dziurka, M., Oklešťková, J., Kocurek, M., Szarek-Lukaszewska, G., et al. (2011). Response of polish cultivars of soybean (Glycine max (L.) merr.) to brassinosteroid application;. Acta Sci. Pol. Agric. 10, 33–50.
Janeczko, A., Swaczynová, J. (2010). Endogenous brassinosteroids in wheat treated with 24-epibrassinolide. Biol. Plant 54, 477–482. doi: 10.1007/s10535-010-0084-1
Jiang, Y., Bao, L., Jeong, S. Y., Kim, S. K., Xu, C., Li, X., et al. (2012). XIAO is involved in the control of organ size by contributing to the regulation of signaling and homeostasis of brassinosteroids and cell cycling in rice. Plant J. 70, 398–408. doi: 10.1111/j.1365-313X.2011.04877.x
Kaya, C., Ashraf, M., Wijaya, L., Ahmad, P. (2019). The putative role of endogenous nitric oxide in brassinosteroid-induced antioxidant defence system in pepper (Capsicum annuum l.) plants under water stress. Plant Physiol. Biochem. 143, 119–128. doi: 10.1016/j.plaphy.2019.08.024
Khamsuk, O., Sonjaroon, W., Suwanwong, S., Jutamanee, K., Suksamrarn, A. (2018). Effects of 24-epibrassinolide and the synthetic brassinosteroid mimic on chili pepper under drought. Acta Physiol. Plant 40, 106. doi: 10.1007/s11738-018-2682-z
Kim, H. B., Kwon, M., Ryu, H., Fujioka, S., Takatsuto, S., Yoshida, S., et al. (2006). The regulation of DWARF4 expression is likely a critical mechanism in maintaining the homeostasis of bioactive brassinosteroids in Arabidopsis. Plant Physiol. 140, 548–557. doi: 10.1104/pp.105.067918
Kim, Y., Park, S. U., Pham, G., Jeong, Y. S., Kim, S. H. (2019). ATBS1-INTERACTING FACTOR 2 negatively regulates dark-and brassinosteroid-induced leaf senescence through interactions with INDUCER OF CBF EXPRESSION 1. J. Exp. Bot. 71, 1475–1490. doi: 10.1093/jxb/erz533
Kolbe, A., Schneider, B., Porzel, A., Schmidt, J., Adam, G. (1995). Acyl-conjugated metabolites of brassinosteroids in cell suspension cultures of Ornithopus sativus. Phytochemistry. 38, 633–636. doi: 10.1016/0031-9422(94)00742-C
Li, K. R., Feng, C. H. (2011). Effects of brassinolide on drought resistance of Xanthoceras sorbifolia seedlings under water stress. Acta Physiol. Plant 33, 1293–1300. doi: 10.1007/s11738-010-0661-0
Li, Y. H., Liu, Y. J., Xu, X. L., Jin, M., An, L. Z., Zhang, H. (2012). Effect of 24-epibrassinolide on drought stress-induced changes in Chorispora bungeana. Biol. Plant 56, 192–196. doi: 10.1007/s10535-012-0041-2
Li, S., Zheng, H., Lin, L., Wang, F., Sui, N. (2021). Roles of brassinosteroids in plant growth and abiotic stress response. Plant Growth Regul. 93, 29–38. doi: 10.1007/s10725-020-00672-7
Liu, C., Feng, B., Zhou, Y., Liu, C., Gong, X. (2022). Exogenous brassinosteroids increases tolerance to shading by altering stress responses in mung bean (Vigna radiata l.). Photosynth. Res. 151, 279–294. doi: 10.1007/s11120-021-00887-3
Lu, H., Wang, Z., Xu, C., Li, L., Yang, C. (2021). Multiomics analysis provides insights into alkali stress tolerance of sunflower (Helianthus annuus l.). Plant Physiol. Biochem. 166, 66–77. doi: 10.1016/j.plaphy.2021.05.032
Luo, X. T., Cai, B. D., Yu, L., Ding, J., Feng, Y. Q. (2018). Sensitive determination of brassinosteroids by solid phase boronate affinity labeling coupled with liquid chromatography-tandem mass spectrometry. J. Chromatogr. A. 1546, 10–17. doi: 10.1016/j.chroma.2018.02.058
Lv, J., Zong, X. F., Shakeel Ahmad, A., Wu, X., Wu, C., Li, Y. P., et al. (2020). Alteration in morpho-physiological attributes of Leymus chinensis (Trin.) tzvelev by exogenous application of brassinolide under varying levels of drought stress. Chil. J. Agric. Res. 80, 61–71. doi: 10.4067/S0718-58392020000100061
Malaga, S., Janeczko, A., Janowiak, F., Waligórski, P., Oklestkova, J., Dubas, E., et al. (2020). Involvement of homocastasterone, salicylic and abscisic acids in the regulation of drought and freezing tolerance in doubled haploid lines of winter barley. Plant Growth Regul. 90, 173–188. doi: 10.1007/s10725-019-00544-9
Mao, J., Zhang, D., Li, K., Liu, Z., Liu, X., Song, C., et al. (2017). Effect of exogenous brassinolide (BR) application on the morphology, hormone status, and gene expression of developing lateral roots in Malus hupehensis. Plant Growth Regul. 82, 391–401. doi: 10.1007/s10725-017-0264-5
Mostajeran, A., Rahimi-Eichi, V. (2009). Effects of drought stress on growth and yield of rice (Oryza sativa l.) cultivars and accumulation of proline and soluble sugars in sheath and blades of their different ages leaves. Agric. Environ. Sci. 5, 264–272.
Nakamura, A., Fujioka, S., Sunohara, H., Kamiya, N., Hong, Z., Inukai, Y., et al. (2006). The role of OsBRI1 and its homologous genes, OsBRL1 and OsBRL3, in rice. Plant Physiol. 140, 580–590. doi: 10.1104/pp.105.072330
Nie, S., Huang, S., Wang, S., Mao, Y., Liu, J., Ma, R., et al. (2019). Enhanced brassinosteroid signaling intensity via SlBRI1 overexpression negatively regulates drought resistance in a manner opposite of that via exogenous BR application in tomato. Plant Physiol. Biochem. 138, 36–47. doi: 10.1016/j.plaphy.2019.02.014
Nishikawa, N., Shida, A., Toyama, S. (1995). Metabolism of 14C-labeled epibrassinolide in intact seedlings of cucumber and wheat. J. Plant Res. 108, 65–69. doi: 10.1007/BF02344307
Parada, F., Oklestkova, J., Arce-Johnson, P. (2022). Characterization of endogenous levels of brassinosteroids and related genes in grapevines. Int. J. Mol. Sci. 23, 1827. doi: 10.3390/ijms23031827
Pavlović, I., Mlinarić, S., Tarkowská, D., Oklestkova, J., Novák, O., Lepeduš, H., et al. (2019). Early brassica crops responses to salinity stress: a comparative analysis between Chinese cabbage, white cabbage, and kale. Front. Plant Sci. 10. doi: 10.3389/fpls.2019.00450
Pavlović, I., Petřík, I., Tarkowská, D., Lepeduš, H., Vujčić Bok, V., Radić Brkanac, S., et al. (2018). Correlations between phytohormones and drought tolerance in selected brassica crops: Chinese cabbage, white cabbage and kale. Int. J. Mol. Sci. 19, 2866. doi: 10.3390/ijms19102866
Ramirez, V. E., Poppenberger, B. (2020). Modes of brassinosteroid activity in cold stress tolerance. Front. Plant Sci. 11. doi: 10.3389/fpls.2020.583666
Rehman, A., Shahzad, B., Haider, F. U., Ullah, A., Khan, I. (2022). “Brassinosteroids in plant response to high temperature stress,” in Brassinosteroids in plant developmental biology and stress tolerance. Eds. Ahammed, G. J., Sharma, A., Yu, J. (Cambridge: Academic Press), 173–187. doi: 10.1016/B978-0-12-813227-2.00014-X
Rittenberg, D., Foster, G. L. (1940). A new procedure for quantitative analysis by isotope dilution, with application to the determination of amino acids and fatty acids. J. Biol. Chem. 133, 737–744.
Sağlam-Çağ, S. (2007). The effect of epibrassinolide on senescence in wheat leaves. Biotechnol. Biotechnol. Equip. 21, 63–65. doi: 10.1080/13102818.2007.10817415
Schneider, B., Kolbe, A., Porzel, A., Adam, G. (1994). A metabolite of 24-epi-brassinolide in cell suspension cultures of Lycopersicon esculentum. Phytochemistry. 36, 319–321. doi: 10.1016/S0031-9422(00)97068-7
Setsungnern, A., Treesubsuntorn, C., Thiravetyan, P. (2019). Exogenous 24-epibrassinolide enhanced benzene detoxification in Chlorophytum comosum via overexpression and conjugation by glutathione. Sci. Total. Environ. 662, 805–815. doi: 10.1016/j.scitotenv.2019.01.258
Shahana, T., Rao, P. A., Ram, S. S., Sujatha, E. (2015). Mitigation of drought stress by 24-epibrassinolide and 28-homobrassinolide in pigeon pea seedlings. Int. J. Mult. Curr. Res. 3, 905–911.
Shimada, Y., Goda, H., Nakamura, A., Takatsuto, S., Fujioka, S., Yoshida, S. (2003). Organ-specific expression of brassinosteroid biosynthetic genes and distribution of endogenous brassinosteroids in Arabidopsis. Plant Physiol. 131, 287–297. doi: 10.1104/pp.013029
Sidhu, G. P. S., Bali, A. S. (2022). “Plant responses to drought stress: role of brassinosteroids,” in Brassinosteroids in plant developmental biology and stress tolerance. Eds. Ahammed, G. J., Sharma, A., Yu, J. (Cambridge: Academic Press), 201–216. doi: 10.1016/B978-0-12-813227-2.00012-6
Sivakumar, R., Nandhitha, G. K., Chandrasekaran, P., Boominathan, P., Senthilkumar, M. (2017). Impact of pink pigmented facultative methylotroph and PGRs on water status, photosynthesis, proline and NR activity in tomato under drought. Int. J. Curr. Microbiol. App. Sci. 6, 1640–1651. doi: 10.20546/ijcmas.2017.606.192
Sperdouli, I., Moustakas, M. (2015). Differential blockage of photosynthetic electron flow in young and mature leaves of Arabidopsis thaliana by exogenous proline. Photosynthetica. 53, 471–477. doi: 10.1007/s11099-015-0116-3
Stirbet, A., Govindjee (2011). On the relation between the kautsky effect (chlorophyll a fluorescence induction) and photosystem II: basics and applications of the OJIP fluorescence transient. J. Photochem. Photobiol. B. Biol. 104, 236–257. doi: 10.1016/j.jphotobiol.2010.12.010
Strasser, R. J., Srivastava, A., Tsimilli-Michael, M. (2000). “The fluorescence transient as a tool to characterize and screen photosynthetic samples,” in Probing photosynthesis: mechanism, regulation and adaptation. Eds. Yunus, M., Pathre, U., Mohanty, P. (London: Taylor and Francis), 445–483.
Sullivan, C. Y. (1972). “Mechanisms of heat and drought resistance in grain sorghum and methods of measurement,” in Sorghum in seventies. Eds. Ganga, N., Rao, P., House, L. R. (New Delhi: Oxford & IBH Pub. Co), 247–264.
Symons, G. M., Reid, J. B. (2004). Brassinosteroids do not undergo long-distance transport in pea. implications for the regulation of endogenous brassinosteroid levels. Plant Physiol. 135, 2196–2206. doi: 10.1104/pp.104.043034
Symons, G. M., Ross, J. J., Jager, C. E., Reid, J. B. (2008). Brassinosteroid transport. J. Exp. Bot. 59, 17–24. doi: 10.1093/jxb/erm098
Talaat, N. B., Shawky, B. T., Ibrahim, A. S. (2015). Alleviation of drought-induced oxidative stress in maize (Zea mays l.) plants by dual application of 24-epibrassinolide and spermine. Environ. Exp. Bot. 113, 47–58. doi: 10.1016/j.envexpbot.2015.01.006
Tang, S., Li, L., Wang, Y., Chen, Q., Zhang, W., Jia, G., et al. (2017). Genotype-specific physiological and transcriptomic responses to drought stress in Setaria italica (an emerging model for Panicoideae grasses). Sci. Rep. 7, 10009. doi: 10.1038/s41598-017-08854-6
Tarkowská, D., Krampolová, E., Strnad, M. (2020). Plant triterpenoid crosstalk: the interaction of brassinosteroids and phytoecdysteroids in Lepidium sativum. Plants. 9, 1325. doi: 10.3390/plants9101325
Tarkowská, D., Novák, O., Oklestkova, J., Strnad, M. (2016). The determination of 22 natural brassinosteroids in a minute sample of plant tissue by UHPLC–ESI–MS/MS. Anal. Bioanal. Chem. 408, 6799–6812. doi: 10.1007/s00216-016-9807-2
Tůmová, L., Tarkowská, D., Řehořová, K., Marková, H., Kočová, M., Rothová, O., et al. (2018). Drought-tolerant and drought-sensitive genotypes of maize (Zea mays l.) differ in contents of endogenous brassinosteroids and their drought-induced changes. PloS One 13, e0197870. doi: 10.1371/journal.pone.0197870
Wang, Z., Zheng, P., Meng, J., Xi, Z. (2015). Effect of exogenous 24-epibrassinolide on chlorophyll fluorescence, leaf surface morphology and cellular ultrastructure of grape seedlings (Vitis vinifera l.) under water stress. Acta Physiol. Plant 37, 1729. doi: 10.1007/s11738-014-1729-z
Wei, Z., Li, J. (2020). Regulation of brassinosteroid homeostasis in higher plants. Front. Plant Sci. 11. doi: 10.3389/fpls.2020.583622
Wellburn, A. R. (1994). The spectral determination of chlorophylls a and b, as well as total carotenoids, using various solvents with spectrophotometers of different resolution. J. Plant Physiol. 144, 307–313. doi: 10.1016/S0176-1617(11)81192-2
Xue, X., Liu, A., Hua, X. (2008). Proline accumulation and transcriptional regulation of proline biothesynthesis and degradation in Brassica napus. BMB Rep. 42, 28–34. doi: 10.5483/bmbrep.2009.42.1.028
Yokota, T., Sato, T., Takeuchi, Y., Nomura, T., Uno, K., Watanabe, T., et al. (2001). Roots and shoots of tomato produce 6-deoxo-28-norcathasterone, 6-deoxo-28-nortyphasterol and 6-deoxo-28-norcastasterone, possible precursors of 28-norcastasterone. Phytochemistry. 58, 233–238. doi: 10.1016/S0031-9422(01)00237-0
Younesian, A., Norouzi, H. A., Gholipoor, M., Soltani, A. (2017). Consequences of ultrasonic waves radiation and 24-epi-brassinolid foliar application for reduction of water deficit stress on qualitative properties of red beans (Akhtar). J. Res. Ecol. 5, 686–699.
Yuan, G. F., Jia, C. G., Li, Z., Sun, B., Zhang, L. P., Liu, N., et al. (2010). Effect of brassinosteroids on drought resistance and abscisic acid concentration in tomato under water stress. Sci. Hortic. 126, 103–108. doi: 10.1016/j.scienta.2010.06.014
Yusuf, M. A., Kumar, D., Rajwanshi, R. J., Strasser, R., Tsimilli-Michael, M., Govindjee, K. (2010). Overexpression of γ-tocopherol methyl transferase gene in transgenic Brassica juncea plants alleviates abiotic stress: physiological and chlorophyll a fluorescence measurements. Biochem. Biophys. Acta 177, 1428–1438. doi: 10.1016/j.bbabio.2010.02.002
Zhang, W., Huang, H., Zhou, Y., Zhu, K., Wu, Y., Xu, Y., et al. (2022b). Brassinosteroids mediate moderate soil-drying to alleviate spikelet degeneration under high temperature during meiosis of rice. Plant Cell Environ. 4, 1340–1362. doi: 10.1111/pce.14436
Zhang, W., Sheng, J., Fu, L., Xu, Y., Xiong, F., Wu, Y., et al. (2020). Brassinosteroids mediate the effect of soil-drying during meiosis on spikelet degeneration in rice. Environ. Exp. Bot. 169, 103887. doi: 10.1016/j.envexpbot.2019.103887
Zhang, H., Yang, D., Wang, P., Zhang, X., Ding, Z., Zhao, L. (2022a). Feedback inhibition might dominate the accumulation pattern of BR in the new shoots of tea plants (Camellia sinensis). Front. Genet. 12. doi: 10.3389/fgene.2021.809608
Zhang, M., Zhai, Z., Tian, X., Duan, L., Li, Z. (2008). Brassinolide alleviated the adverse effect of water deficits on photosynthesis and the antioxidant of soybean (Glycine max l.). Plant Growth Regul. 56, 257–264. doi: 10.1007/s10725-008-9305-4
Zhang, J., Zhang, Y., Khan, R., Wu, X., Zhou, L., Xu, N., et al. (2021). Exogenous application of brassinosteroids regulates tobacco leaf size and expansion via modulation of endogenous hormones content and gene expression. Physiol. Mol. Biol. Plants 27, 847–760. doi: 10.1007/s12298-021-00971-x
Keywords: brassinosteroids, drought, endogenous content, exogenous application, leaf age, OJIP analysis, proline
Citation: Marková H, Tarkowská D, Čečetka P, Kočová M, Rothová O and Holá D (2023) Contents of endogenous brassinosteroids and the response to drought and/or exogenously applied 24-epibrassinolide in two different maize leaves. Front. Plant Sci. 14:1139162. doi: 10.3389/fpls.2023.1139162
Received: 06 January 2023; Accepted: 12 May 2023;
Published: 02 June 2023.
Edited by:
László Szabados, Biological Research Centre, Hungarian Academy of Sciences (MTA), HungaryReviewed by:
Hamid Manzoor, Bahauddin Zakariya University, PakistanAndrzej Bajguz, University of Białystok, Poland
Copyright © 2023 Marková, Tarkowská, Čečetka, Kočová, Rothová and Holá. This is an open-access article distributed under the terms of the Creative Commons Attribution License (CC BY). The use, distribution or reproduction in other forums is permitted, provided the original author(s) and the copyright owner(s) are credited and that the original publication in this journal is cited, in accordance with accepted academic practice. No use, distribution or reproduction is permitted which does not comply with these terms.
*Correspondence: Dana Holá, danahola@natur.cuni.cz