- 1National Engineering Research Center for Modernization of Traditional Chinese Medicine - Hakka Medical Resources Branch, School of Pharmacy, Gannan Medical University, Ganzhou, China
- 2Animal Physiology and Neurobiology Section, Department of Biology, KU Leuven, Leuven, Belgium
- 3Department of Phytochemistry, Medicinal Plants and Drug Research Institute, Shahid Beheshti University, Tehran, Iran
- 4Leishmania Diagnostic & Drug Delivery Research Laboratory, University of Peshawar, Peshawar, Pakistan
- 5Fisheries and Marine Resource Technology Discipline, Khulna University, Khulna, Bangladesh
- 6Center of Environment Climate Change and Public Health, Utkal University, Vani Vihar, Bhubaneswar, Odisha, India
Chimonanthus grammatus is used as Hakka traditional herb to treat cold, flu, etc. So far, the phytochemistry and antimicrobial compounds have not been well investigated. In this study, the orbitrap-ion trap MS was used to characterize its metabolites, combined with a computer-assisted structure elucidation method, and the antimicrobial activities were assessed by a broth dilution method against 21 human pathogens, as well as the bioassay-guided purification work to clarify its main antimicrobial compounds. A total of 83 compounds were identified with their fragmentation patterns, including terpenoids, coumarins, flavonoids, organic acids, alkaloids, and others. The plant extracts can strongly inhibit the growth of three Gram-positive and four Gram-negative bacteria, and nine active compounds were bioassay-guided isolated, including homalomenol C, jasmonic acid, isofraxidin, quercitrin, stigmasta-7,22-diene-3β,5α,6α-triol, quercetin, 4-hydroxy-1,10-secocadin-5-ene-1,10-dione, kaempferol, and E-4-(4,8-dimethylnona-3,7-dienyl)furan-2(5H)-one. Among them, isofraxidin, kaempferol, and quercitrin showed significant activity against planktonic Staphylococcus aureus (IC50 = 13.51, 18.08 and 15.86 µg/ml). Moreover, their antibiofilm activities of S. aureus (BIC50 = 15.43, 17.31, 18.86 µg/ml; BEC50 = 45.86, ≥62.50, and 57.62 µg/ml) are higher than ciprofloxacin. The results demonstrated that the isolated antimicrobial compounds played the key role of this herb in combating microbes and provided benefits for its development and quality control, and the computer-assisted structure elucidation method was a powerful tool for chemical analysis, especially for distinguishing isomers with similar structures, which can be used for other complex samples.
1 Introduction
As a Hakka herb, Chimonanthus grammatus M.C. Liu (ChG) is from the Calycanthaceae family and only distributed in Ganzhou, Jiangxi Province, China. The local Hakka people have cultivated it for decades and utilized it as a traditional herb with effects on cold, flu, cough, and rheumatic arthritis (Jiang et al., 2015; Hu et al., 2022). There is a tradition to put the tender stem and leaves inside fishpond for protecting fish against infections (Liu et al., 2011), indicating the plant should have antimicrobial activities and related active compounds. Although ChG was documented as a new species in 1984, most local people treat it as other local Chimonanthus plants, such as C. praecox, C. salicifolius, or C. nitens, which have much longer history as herbal medicine (Flora of China Editorial Committee, 2008). Chimonanthus plants have been developed as several medicaments, such as ‘Shan la mei ke li’ (granules for wind-heat type common cold, fever, chills, sore throat, etc.), ‘La mei you’ (oil for skin ulcer, eczema, swelling pain, etc.).
Chimonanthus is only distributed in south China, and by now most research works have been done for these common species, from which more than one hundred compounds were isolated, including alkaloids, sesquiterpenoids, coumarins, flavonoids, organic acids, etc. (Xiao and Liu, 2003; Deng et al., 2004; Wang et al., 2011; Li and Zou, 2018; Shuaifeng and Zhengrong, 2018; Shu et al., 2019a; Guo et al., 2021), exhibiting antimicrobial, anti-tumor, anti-inflammatory, antioxidant, anti-lipids, hypoglycemia, anti-depression, liver protection, cough suppressant, anti-diarrhea, and other biological activities (Lingyun et al., 2012; Bingkun and Yaoming, 2003; Zhang et al., 2016; Zhang et al., 2017; Ye et al., 2020). Chimonanthus plants, like C. praecox, also showed some toxicity against rats, rabbits, cattle, or goats, with possible kidney damage, causing hematuria, which was reported as the reason for its alkaloids. However, rare studies have been done on ChG, such as genetic diversity and comparison studies (Jiang et al., 2012; Jiang et al., 2013; Jiang et al., 2015), the essential oil and its activity (Liu et al., 2011), and several isolated non-volatile compounds (Wang, 2012; Ling, 2014; Ling and Zou, 2014; Zhang et al., 2017; Li et al., 2020). By now, the chemical profiling of ChG and its antimicrobial compound basis have still not been clearly elucidated. Therefore, we focused on a comprehensive study investigating the chemical profile via UHPLC-HRMS-MS, antimicrobial activities against various human pathogens, and identification of the main antimicrobial compounds via bioassay-guided purification. In addition, for the active extracts and abundant compounds, we determined their IC50, MBC, and antibiofilm activities to help assess their medicinal potentials.
2 Materials and methods
2.1 Botanical sample preparation and reagents
Herbal materials were collected from Geyou forestry station (25°28’ N, 115°44’ E) and Maogongfa hill (25°29’ N, 115°45’ E), Anyuan County, Ganzhou, China. The plant was identified as Chimonanthus grammatus M.C. Liu according to the Flora of China and http://www.theplantlist.org. The voucher specimens (No.GZ1805) were stored in the Herbarium of Chinese Medicine at Gannan Medical University. The reagents included water, DMSO, formic acid, trifluoroacetic acid, acetonitrile, methanol, ethyl acetate, hexane, antibacterial controls (ciprofloxacin and chloramphenicol), antifungal control (miconazole), and antibiofilm control (sodium dodecyl sulfate). The stem and leaves, as well as the reagents, were prepared with the same methods and resources as previously described (Hu et al., 2021a). Resazurin salt was from Acros Organics (Lot# AC189900010, Geel, Belgium). A reference compound, quercetin was purchased from Sigma-Aldrich (Lot#SLBM7336V, Darmstadt, Germany).
2.2 Antimicrobial and antibiofilm testing
The crude extracts and separated fractions were assessed for their antimicrobial activities by a broth microdilution method in 96-well microtiter plates, and the IV (inhibition value %), IC50 (concentration inhibiting growth by 50%) calculations, and heat map analysis were performed as described before (Hu et al., 2021a). The 21 human pathogens for assessing the extracts included six Gram-positive (G+) bacteria [SE: Staphylococcus epidermidis (ATCC 1457), SA: Staphylococcus aureus (ATCC6538, Rosenbach), LI: Listeria innocua (LMG 11387), EF: Enterococcus faecalis (HC-1909-5), ML: Micrococcus luteus (DPMB 3) and BC: Bacillus cereus (LMG9610)], nine Gram-negative (G–) bacteria [PA: Pseudomonas aeruginosa (PAO1), EC: Escherichia coli (ATCC 47076), SS: Shigella sonnei (LMG 10473), SF: Shigella flexneri (LMG10472), EA: Enterobacter aerogenes (ATCC 13048), AB: Acinetobacter baumannii (RUH134), SLE: Salmonella enterica subsp. enterica (ATCC 13076), BD: Brevundimonas diminuta (from Prof. Rob Lavigne at KU Leuven) and AH: Aeromonas hydrophila (ATCC 7966)], and six fungi [CP: Candida parapsilosis (ATCC 22019), CA: C. albicans (SC 5314), CG: C. glabrata (ATCC 2001), CAU: C. auris (OS299), CU: C. utilis (IHEM 4005), and SC: Saccharomyces cerevisiae (ATCC 7754)]. The abundant pure compounds were also tested against a biofilm strain: Staphylococcus aureus (USA 300). The preformed biofilm and biofilm formation activities were tested for BIC50 (concentration inhibiting biofilm formation by 50%), and BEC50 (concentration required to eradicate 50% of a preformed biofilm) as described before (Kipanga et al., 2020).
2.3 Isolation and purification
The large-scale extracts were prepared by a sonication assistance method in methanol and ethyl acetate separately, then combined as the final ChG extract. The mixture of extraction was separated via a silica gel column in a similar way as the previous study (Hu et al., 2021a). In brief, 6.4 g of dried extracts (obtained from 200 g plant materials) was used for the elution by the mobile phases consisting of hexane (A), ethyl acetate (B), methanol (C), and 25% acetic acid in methanol (D) with a gradient from 95% A and 5% B to 100% D (5%-10% difference per 10 min, flow rate: 40 mL/min). Then 242 fractions were collected. The eight most active fractions were selected for HPLC separation and compound purification with semi-preparative C18 column using conditions summarized in Table 1.
2.4 UHPLC-HRMS-MS condition and data analysis
The MS samples were randomly sampled from the extract and redissolved in MeOH at 0.1 mg/mL, then 5 μl of 20-times dilution was injected for each MS detection. An Ultimate 3000 UHPLC system coupled to an ultra-high resolution Orbitrap Elite hybrid mass spectrometer was used. The equipment setting, data analysis, and heat map were done as described before (Hu et al., 2021a), from which small changes were made for the MS optimization like the previous study (Hu et al., 2021b), as follows: full scan mode with 100-1500 m/z mass range, MS/MS fragmentation for top 10 precursors (dd-MS2-TOP 10) with repetition in the range of 100-1500 m/z. The MS data were analyzed by the software packages, Xcalibur 4.2, Freestyle™ 1.5, ACD/MS Workbook Suite 2021 with MS Fragmenter, and ChromGenius.
3 Results and discussion
3.1 Chemical profiling of ChG
The MS data of ChG extract was automatically matched with a manually built database via ACD/MS Workbook Suite. The total ion chromatogram (TIC) of the plant extract was extracted by the matched ions and the results of extracted ion chromatogram (EIC) in positive ion mode were shown as an example in Figure 1. The identified compounds and their fragmentations were listed in Table 2. A total of 83 components were tentatively identified, including 21 terpenoids, 20 coumarins, 19 flavonoids, 15 organic acids, 4 alkaloids, 2 glycosides, 1 anthraquinone, and 1 steroid. Of note, 5,6,7-trimethoxycoumarin and kaempferol had the highest relative contents (23% and 19%, respectively) according to the peak area comparison, and the other main components with more than 2% contents are E-4-(4,8-dimethylnona-3,7-dienyl)furan-2(5H)-one, scopoletin, nicotiflorin, scoparone, quercitrin, quercetin, isofraxidin, fraxetin, luteolin, cucumin E, and melanoxetin. To date, this is the first report on the chemical profiling of ChG non-volatile compounds by mass spectrometry, as well as the fragmentation patterns of most compounds here.
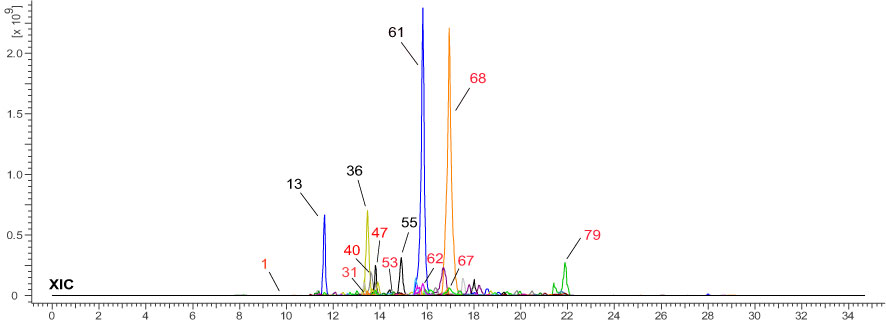
Figure 1 Total ion chromatogram of Chimonanthus grammatus, and the main components (marked with the same number as in Table 2).
3.2 Computer-assisted structure elucidation by MS
UHPLC-HRMS-MS data was analyzed for chemical identification in several steps. Firstly, precursor ions were obtained by auto calculation in the ACD/MS Workbook Suite. Then, the ultra-high-resolution mass was searched in chemical databases, such as COCONUT (https://coconut.naturalproducts.net/), and a manually built database of Chimonanthus plants. The latter (custom) database (Supplementary Material 1) should provide the best chance for correct identifications, because these compounds were isolated and identified from Chimonanthus plants before. In all, 83 compounds were identified from the ChG extract, including 17 isomers of 39 compounds. To illustrate the details, taking [M+H]+ 303.0499 m/z and [M-H]- 301.0350 m/z precursor ions as an example, there were 4 peaks from the ChG extract with the same ions eluting at the retention times of 12.997, 13.330, 13.902, and 15.840 min (Figure 2-S1). The mass was compatible with the chemical formulas C15H10O7 or C15H11O7+. After searching the ultra-high-resolution mass in databases, 55 isomers were found (Figure 2-S2), of which only quercetin was reported in Chimonanthus plants before (Shu et al., 2019). Therefore, public databases were searched for fragmentation spectra corresponding to the experimental MS2 spectra(Figure 2-S3a). In addition, MS Fragmenter was used to predict the fragmentation patterns for the compounds without available spectra (Figure 2-S3b). Those four isomers have quite similar fragments in MS2 spectra, suggesting that their structures could come from the same chemical class. In this step, most of the 55 isomers were eliminated, and several possible ones were retained as candidates according to the fragmentation pattern predictions. The main fragmentation pathway of quercetin is shown in Figure 2-S3B, in which the most intense peaks were assigned by MS Fragmenter, such as 257 (C14H11O6+), 229 (C13H9O4+), 165 (C8H5O4+), 137 (C7H5O3+), 285 (C15H9O6+), 303 (C15H11O7+), 247 (C13H11O5+), 111 (C6H7O2+), 121 (C7H5O3+), 275 (C14H11O6+), 193 (C9H5O5+), etc. To confirm these predictions and also distinguish the isomers with similar structures, ChromGenius was used for retention time calculations (Figure 2-S4), then those four isomers were assigned as the final identifications.
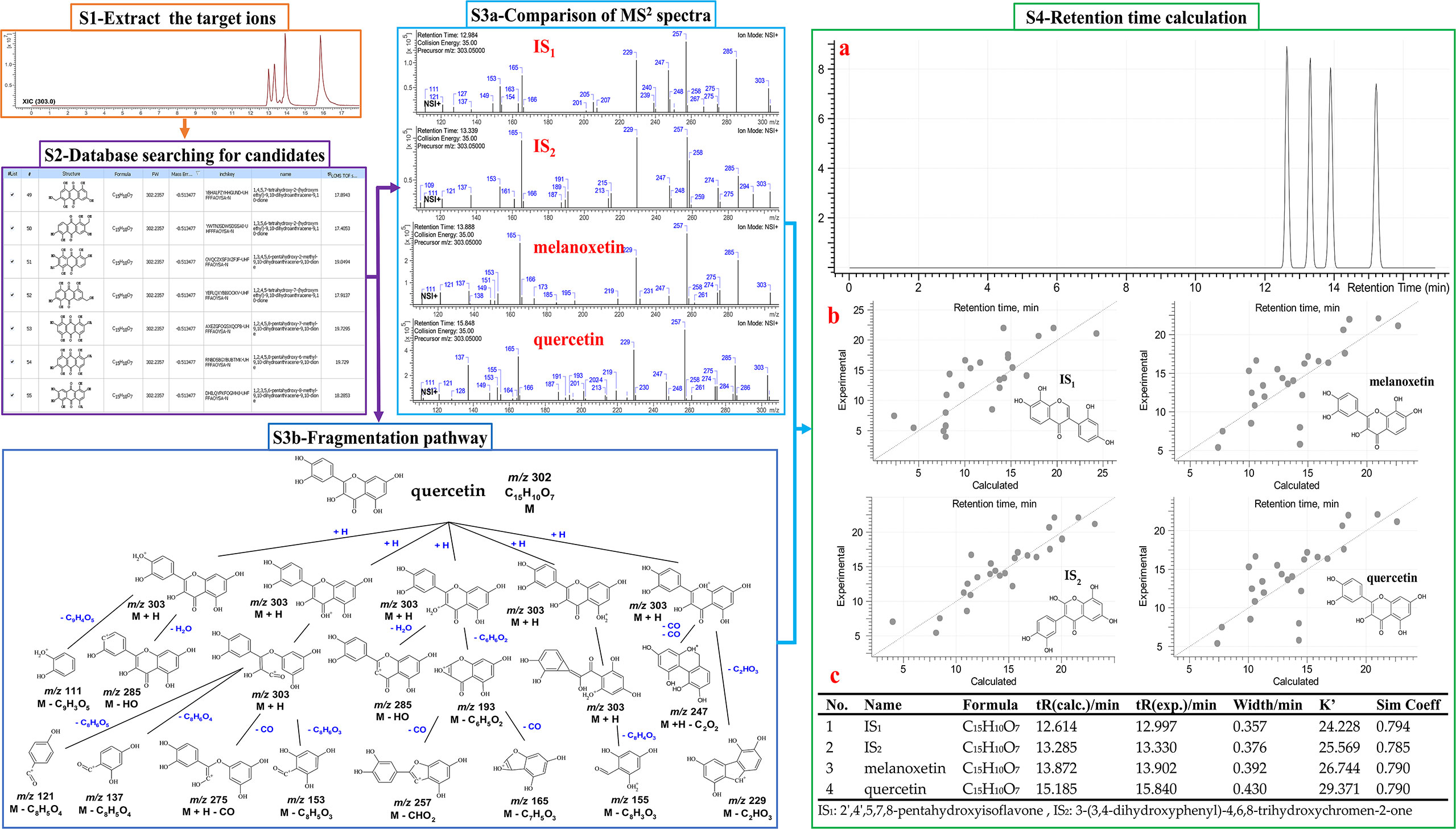
Figure 2 MS data analysis method. S1-4: identification steps 1-4. S1: the extracted ion chromatography XIC of 303.0499 m/z [C15H11O7] + in positive mode. S2: search with the exact mass in the COCONUT database. S3A: fragments of the four ions in MS2 spectra. S3B: the main fragmentation pathway of quercetin predicted by MS Fragmenter. S4: chromatographic retention time predictions (a), regression curves (b) of four isomers by ChromGenius, and the calculated parameters (c).
Based on those steps, the spectra of all four compounds can be interpreted and verified: 2’,4’,5,7,8-pentahydroxyisoflavone (IS1), 3-(3,4-dihydroxyphenyl)-2,6,8-trihydroxy-4H-chromen-4-one (IS2), melanoxetin, and quercetin. Those four compounds come from two core structures, isoflavone (IS1, IS2) and flavones (melanoxetin, quercetin) substituted with hydroxyl groups at different positions. This leads to different polarity notwithstanding chemical similarity (Willett et al., 1998), so they can be distinguished via ChromGenius for retention time prediction. The calculation of retention time was performed under the same UHPLC-HRMS-MS condition for all 55 isomers. The chromatography of the final four isomers was generated by the calculation with the regression equation: 0.03*tR2 + 1.936*tR - 6.696, and are listed in Figure 2-S4c, where these four components gave k’ values (retention time factor) and similarity coefficients (Sim Coeff) as 24.228, 25.569, 26.744, 29.371, and 0.794, 0.785, 0.790, 0.790, respectively. The k’ values measured the period of time that the sample component resided in a stationary phase relative to the time it resides in the mobile phase, and it was calculated as (tR – t0)/t0, in which tR and t0 implied the retention time and dead time, respectively. According to the definition in ChromGenius, Sim Coeff is applied by the structural similarity to the current, and the value which stands closer to 1 provides better results for the calculation. Hence, it was considered that the calculation of these four isomers in Figure 2-S4 exhibited sufficient reliability. Moreover, to check the mass identification and computer-assistant elucidation method (McEachran et al., 2018; Hu et al., 2021a), a reference compound (quercetin) was measured under the same condition, and the retention time and fragmentation pattern were used to confirm the predicted results.
Another 16 sets of isomers were similarly analyzed based on their calculated tR: isomers in positive mode of m/z 149.0597 (C9H8O2), 153.0546 (C8H8O3), 193.0495 (C10H8O4), 209.0444 (C10H8O5), 225.1849 (C14H24O2), 237.0757 (C12H12O5), 239.2006 (C15H26O2), 287.0550 (C15H10O6), 347.2230 (C22H26N4), 355.1024 (C16H18O9), 383.0761 (C20H14O8), 385.1129 (C17H20O10), 387.1074 (C20H18O8), 443.0973 (C22H18O10), 449.1078 (C21H21O11+ or C21H20O11), and 595.1657 (C27H31O15+ or C27H30O15). Although the MS with the computer-assisted method is excellent to distinguish most isomers, there are still 2 types of isomers not identified due to their quite similar structures which require NMR identification for the pure compounds, such as m/z 347.2230 (C22H26N4) and m/z 443.0973 (C22H18O10). For m/z 347.2230, there are two isomer peaks in the ChG extract, where the first peak corresponds to either (+)-calycanthine, (-)-calycanthine, or (-)iso-calycanthine, and the second peak is meso-chimonanthine or chimonanthine. The two peaks of m/z 443.0973 were identified as 4,4’-biisofraxidin (or 3,3’-biisofraxidin), and arteminorin A, respectively.
The fragmentation prediction and retention time calculation provided additional information to narrow the scope of chemical candidates and have been used by many researchers before(Pelander et al., 2009; Blaženović et al., 2018; McEachran et al., 2018; Tabudravu et al., 2019). However, the method could be doubted, especially for the reliability of the compound fragmentation patterns, which can be influenced by experimental factors, such as instrument type, dissociation technique, ionization mode, collision energy, etc. According to previous studies, the fragmentation mechanisms are highly reproducible even across instruments (Stein, 2012; Hufsky et al., 2014), and the variations were mainly from the intensity of the fragments. Hence, it is feasible to do the comparison based on the predicted fragments (Champarnaud and Hopley, 2011). In MS Fragmenter, the identification scores for comparing the experimental fragmentation with the predictions were ranked, but it is clear that if we only focus on the scores generated by software, the fragmentation predictions will not be reliable for chemical identification (Schymanski et al., 2009). Therefore, we also used ChromGenius to check the retention time of all the chemical candidates. Afterward, the final results were generated according to both of them.
3.3 Antimicrobial activities of C. grammatus extracts
Four solvents with different polarities (water, methanol, ethyl acetate, and hexane), were used to extract the stem and leaf powder of ChG. The dried extracts, redissolved in DMSO, were tested against 21 different microbes, and the antimicrobial activities were shown in Figure 3A. ChG extracts inhibited the growth of three G+ and four G– bacteria (IV>50%), and their IC50 values are lower than 1 mg/mL estimated from a two-fold dilution series. So far, other bioactivities of different ChG parts have also been reported: antioxidant activity (IC50 = 0.5-10 mg/mL by DPPH assay) of flower extracts due to the flavonoids (Zhang et al., 2017), antioxidant (IC50 = 12.145 mg/mL by DPPH assay) and antimicrobial activity of leaf essential oil (MIC = 2.25-4.5 mg/mL against E. coli, P. aeruginosa, S. aureus, S. typhi, S. dysenteriae, B. subtilis, B. thuringiensis, S. luteus, and A. aerogenes (Liu et al., 2011), AChE activity (IC50 = 8.153 and 4.812 mg/mL for petroleum ether and n-BuOH extract, respectively) and cytotoxicity of leaf extracts (0.5-1 mg/mL against A549 cells) (Ling, 2014). Here, the hexane extract of ChG showed activity against B. diminuta and M. luteus (IC50 = 933 and 957 μg/mL, respectively), and the EtOAc extract exhibited good inhibition against M. luteus (IC50 = 497 μg/mL). The MeOH extract had broad spectrum against five bacteria, including two G+ bacteria (Staphylococcus epidermidis and S. aureus, IC50 = 926 and 498 μg/mL, respectively), and three G– bacteria (Escherichia coli, S. flexneri and S. enterica subsp. enterica, IC50 = 863, 907 and 964 μg/mL, respectively), while the water extract only inhibited the growth of B. diminuta (IC50 = 935 μg/mL). The MeOH and EtOAc extracts showed broad spectrum with high inhibition values, thus, they were used to extract ChG for bioassay-guided purification to identify the bioactive compounds.
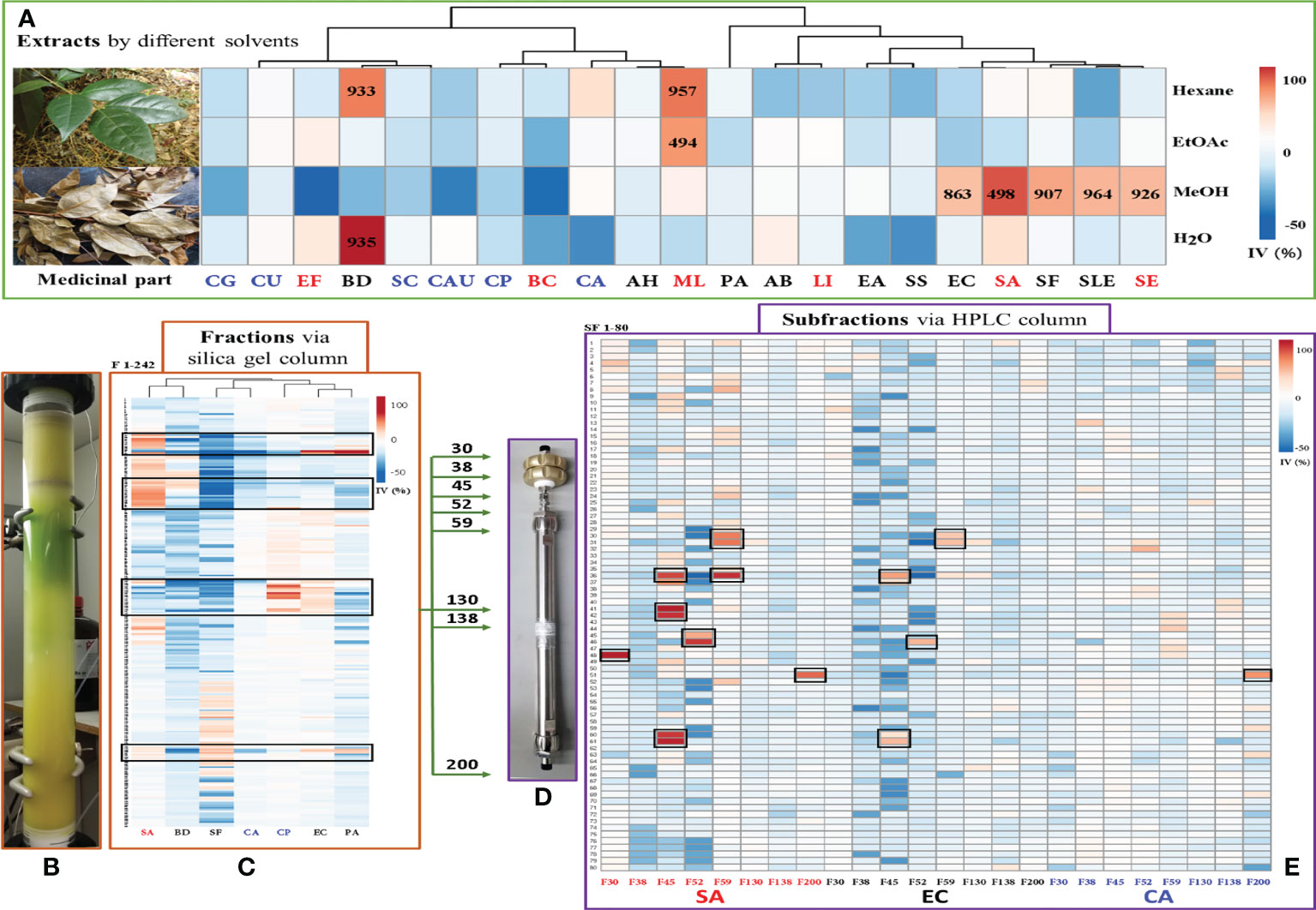
Figure 3 Bioassay-guided purification work of antimicrobial compounds in C grammatus. (A) Image of plant materials and the heat map of antimicrobial activities (IC50 in μg/mL) of different extracts; (B) Normal-phase (silica gel) column; (C) Heat map of antimicrobial activity of chromatographic fractions; (D) reversed-phase (C18) column; (E) Heat map of activity of subfractions against SA, EC, and CA, as representatives of G+, G– bacteria, and fungi. IV: inhibition value; The microbes’ abbreviations are six fungi, nine G– bacteria, and six G+ bacteria marked in blue, black, and red, respectively.
In Chimonanthus genus, there are five other species, including C. praecox, C. salicifolius, C. nitens, C. zhejiangensis, and C. campanulatus. Previous studies mainly concentrated on the first three species, which extracts had activity on antimicrobial, anti-inflammatory, anti-tumor, analgesic, antitussive, expectorant, antipyretic, etc. (Shu et al., 2019) By now, the antimicrobial activities have been reported for the extracts of C. nitens leaves, C. salicifolius leaves, C. zhejiangensis leaves, C. praecox seed, as well as the essential oil of C. praecox leaves, C. grammatus leaves, and the total flavonoids of C. salicifolius leaves. The methanol extract of C. praecox seed can inhibit several plant pathogens, such as Fusarium oxysporum, Sclerotinia sderotiorum, Alternaria solani, Setosphaeria turcica, and Bipolaris maydis (Jiwen et al., 2005). The water extract of C. nitens leaves was active against S. aureus, E. coli, P. aeruginosa, S. flexneri, Diplococcus pneumoniae, Hemolytic Streptococcus B, and Influenza Bacillus (Ping et al., 1996; JC et al., 2002). The ethyl acetate, dichloromethane, and n-hexane fractions from the methanol extract of C. salicifolius leaves were reported to inhibit S. aureus, E. coli, Bacillus subtilis, and P. aeruginosa (Yongxiang et al., 2017). The water and ethanol extracts of C. zhejiangensis can inhibit S. aureus, E. coli, P. aeruginosa, Klebsiella aerogenes, Salmonella typhi, K. pneumoniae, S. pneumoniae, Haemophilus influenzae (Dongqing et al., 2005). Moreover, the essential oil of C. praecox leaves inhibited S. aureus, B. subtilis, and Proteus species (Zhuxiang and Gongxi, 2010), and the essential oil of C. praecox root and fruits were active against S. aureus and C. albicans (Zhifen et al., 2019), while C. praecox flower esential oil inhibited S. epidermidis, E. coli, Bacillus coagulans, Sarcina luteu, S. aureus, B. subtilis, K. pneumoniae, Proteus vulgaris, S. epidermidis (Ying et al., 2010). The total flavonoids of C. salicifolius leaves exhibited activity against S. typhimurium, S. enteritidis, E. sakazakii, S. paratyphi B, S. castellani. E. coli and B. cereus (Huiping et al., 2018). Compared with other Chimonanthus plants, the different ChG extracts showed the antimicrobial activity against several pathogens in this study, supporting its potential as a medicinal herb.
3.4 Active compounds elucidated via bioassay-guided purification
A total of 6.4 g ChG extracts were obtained from the plant materials, then resolved on a normal-phase Silica gel column (Figure 3B) into 242 fractions. Several microorganisms were tested with each fraction, and the inhibition values of all 242 fractions were clustered in a heat map (Figure 3C). The eight most active fractions (F30, 38, 45, 52, 59, 130, 138, and 200) were selected for further purification. Afterward, around 2 mg of these fractions were injected into HPLC with a semipreparative reversed phase C18 column for isolating the active compounds. From each HPLC run, 80 subfractions were collected after optimizing separation conditions (see Table 1) and tested against selected microorganisms: S. aureus (Gram-positive), E. coli (Gram-negative), and C. albicans (fungus), which were amongst the most prevalent causes of bacterial and fungal infections in humans. The inhibition values of each subfraction were shown in Figure 3D, and their HPLC-DAD chromatographs in Figure 4A. Thus, 12, 6, and 1 active subfractions were obtained and assigned in the peaks of chromatographs. These peaks were collected and retested for their antimicrobial effects to confirm their activity, then they were analyzed by UHPLC-HRMS-MS for chemical identification.
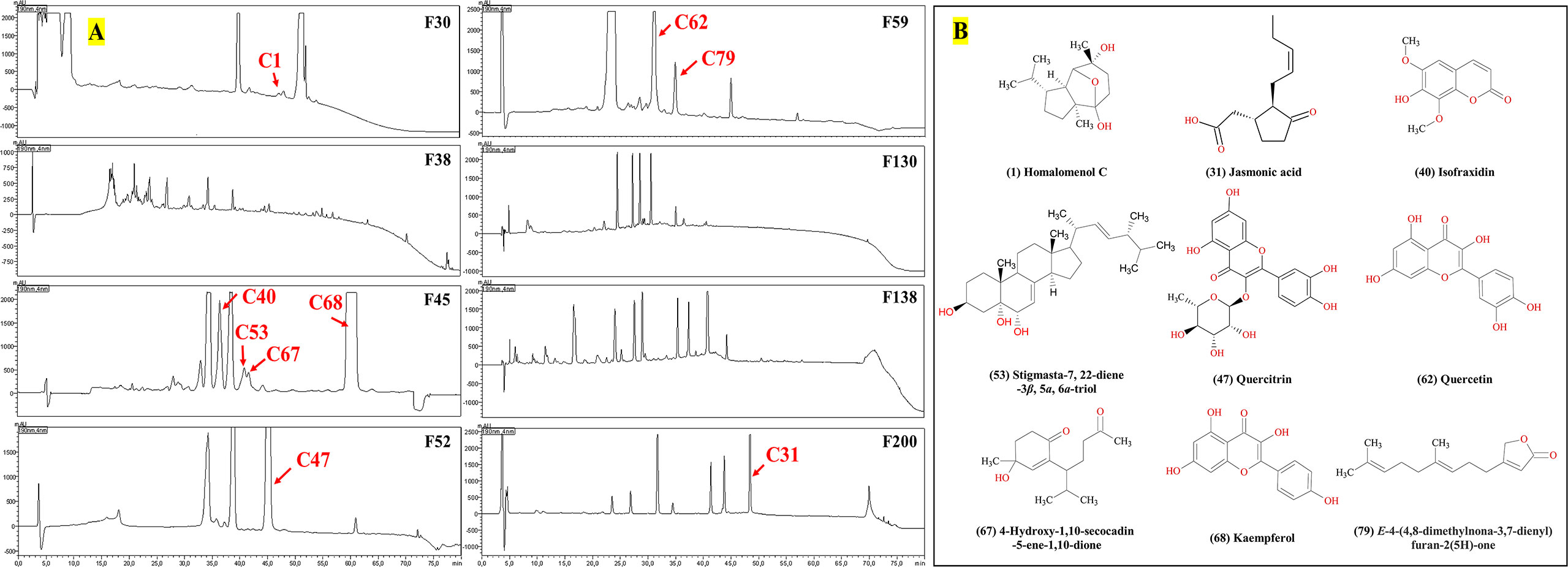
Figure 4 Antimicrobial compounds in the HPLC–DAD chromatograms (A) from C. grammatus, and their structures (B).
The active peaks were analyzed in both positive and negative ion mode by the mass spectrometer, showing that all 13 peaks corresponded to nine different compounds, homalomenol C (1), isofraxidin (40), 4-hydroxy-1,10-secocadin-5-ene-1,10-dione (67), stigmasta-7,22-diene-3β,5α,6α-triol (53), kaempferol (68), E-4-(4,8-dimethylnona-3,7-dienyl)furan-2(5H)-one (79), quercitrin (47), jasmonic acid (31), and quercetin (62) (for their identification, see 3.2). All these compounds were already isolated from Chimonanthus plants, which supports their identification. Their structures were shown in Figure 4B. According to the bioassay-guided purification, all nine compounds inhibited the growth of the Gram-positive bacterium, S. aureus, while compounds 40, 68, and 62 were also active against E. coli, and only compound 31 was active against C. albicans (Figures 3E, 4A).
3.5 Antimicrobial and antibiofilm assessments against S. aureus
For the active compounds, only four of them can be collected for more than 1 mg in this study due to the method limitation, which we used to do the further activity tests, including 2.6 mg isofraxidin (40), 5.3 mg kaempferol (68), 2.8 mg quercitrin (47), 1.1 mg E-4-(4,8-dimethylnona-3,7-dienyl)furan-2(5H)-one (79). They were evaluated against two strains of S. aureus (ATCC6538 and USA300) for their potentials as antimicrobial reagents, including IC50 and MBC for antimicrobial activity, as well as BIC50 and BEC50 for anti-biofilm activity. For MBC, colony-forming units (CFU) were calculated to determine if the bactericidal effect was greater than 99%. All these tests were done by two-fold serial dilutions in DMSO with concentrations ranging from 0.12-250 µg/ml. The positive controls were ciprofloxacin and sodium dodecyl sulfate (SDS), which were diluted as 0.15-31.3 µg/ml and 0.02%-20%, respectively. The inhibition curves were analyzed by GraphPad and inferred parameters were listed in Table 3 and Figure 5.
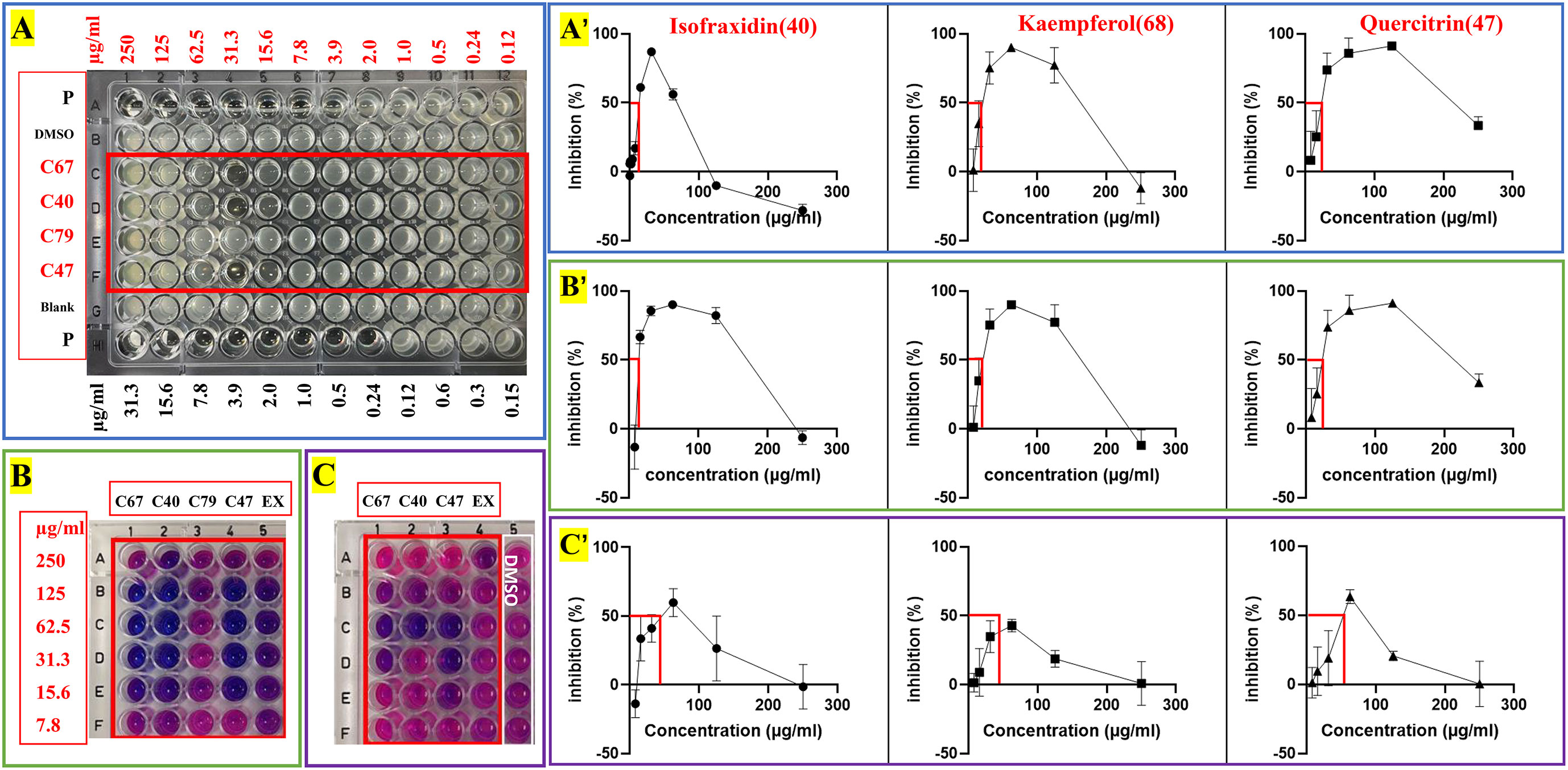
Figure 5 Antimicrobial and anti-biofilm activity against S. aureus of crude extracts and four pure compounds from C grammatus. (A) IC50 test; (B) biofilm formation test; (C) preformed biofilm test; P, Ciprofloxacin; EX, ChG extract (two-fold serial dilutions from 1000 µg/mL).
Here we noticed that higher concentrations (250, 125 µg/ml) of compounds 40, 68, and 79 showed lower inhibition values than the low concentrations (62.50, 31.30, 15.65 µg/ml) according to the OD and fluorescence measurements (Figures 5A–C). A similar phenomenon of kaempferol was reported against Bacillus cereus, which showed strong inhibition with ≥14mm inhibition zone in 10 μM (2.9 mg/mL) while 5 and 20 μM (1.4 and 5.7 mg/mL) had moderate activity with a 12-13.9 mm inhibition zone (Lee et al., 2011). The same was also observed for nalidixic acid against E.coli, where increasing the concentration after a certain point showed decreased bactericidal activity (Crumplin and Smith, 1975). Nalidixic acid at low concentrations can cause single-strand nicks in DNA, leading to DNA degradation and bacterial death, while higher concentrations can also inhibit RNA and protein synthesis, which prevents the synthesis of nucleases and reduce the DNA nicks because nucleases are needed to generate DNA nicks. The fact that a compound is bacteriostatic in high concentrations and bactericidal in low concentrations has important clinical implications since these compounds should preferably be used at a suitable low concentration, which may also provide other advantages like less adverse effects and less resistance development. Moreover, this interesting observation deserve further research, especially given its relevance for their mechanism of action.
In this study, IC50, BIC50, and BEC50 were calculated by the front part of each curve (Figures 5A–C), which means the low inhibition values of these high concentrations for each compound were not included in these calculations. Based on this, the isolated compounds, isofraxidin (40), kaempferol (68), and quercitrin (47) showed significant activity against planktonic S. aureus (IC50 = 13.5, 18.08, and 15.86 µg/ml), while E-4-(4,8-dimethylnona-3,7-dienyl)furan-2(5H)-one (79) exhibited slight activity (IC50 > 250 µg/ml). Although the positive control, ciprofloxacin, has roughly a 100-fold lower IC50 value (0.16 µg/ml), the compounds 40, 47, and 68 can nonetheless be considered as potential antimicrobial reagents. Indeed the well-known natural product, berberine, showed similar IC50 values (14.6 µg/ml) (Cernakova and Košťálová, 2008), and has been widely used as a herbal antibiotic for treating diarrhea and other gastrointestinal disorders for several decades (Gaba et al., 2021). On the other hand, they showed much higher antibiofilm activity (BIC50 = 15.43, 17.31, 18.86 µg/ml) than ciprofloxacin, especially for inhibiting biofilm formation. Also, these three compounds (BEC50 = 45.86, ≥62.50, and 57.62 µg/ml) can eradicate a preformed biofilm of S. aureus.
3.6 Discussion on the bioactivity of ChG active compounds
All nine active compounds were already reported in Chimonanthus species. Here, we list their published bioactivities (Table 4) and compare those with our results. Homalomenol C (1) was already reported to have antiplasmodial activity, cytotoxicity, and antifungal activities, while this is the first report about its activity against S. aureus. Jasmonic acid (31) is a fatty acid distributed in higher plants, bacteria, and fungi. In addition to being a famous plant hormone, it also has multiple bioactivities, including anticancer, antidepressant, anti-aggressive, antimicrobial, antioxidant, anti-inflammatory, anti-nociceptive, antiparasitic, etc. It was identified as an antifungal component against Pyricularia oryzae (syn. Magnaporthe oryzae) from wild rice. Isofraxidin (40) exerts cytotoxic, anti-fatigue, anti-stress, cholagogic, anti-inflammatory effects, protection against acute lung injury in mice, antimalarial, analgesic, sedative, hypnotic, anti-complement, antitussive, antioxidant, anti-diabetic, antilipidemic, antiviral activity, and antimicrobial activity against B. subtilis, S. aureus, E. coli, C. albicans, Aspergillus niger, and Ralstonia solanacearum. Our study adds antibiofilm activity against S. aureus. Quercitrin (47) is a component commonly found in various plants. So far, many bioactivities have been documented, including antiinflammation, antioxidative stress, antimicrobial, immunomodulation, analgesia, wound healing, vasodilation, etc. Increasing evidence has implied its antimicrobial effect against various microbes (viruses, in addition to over 22 bacteria, and 12 fungi), including S. aureus, E. coli, C. albicans, etc. Stigmasta-7,22-diene-3β,5α,6α-triol (53), and 4-hydroxy-1,10-secocadin-5-ene-1,10-dione (67) were active against S. aureus in this study; this activity of compound 67 was reported before, while the antimicrobial activity of compound 53 has not been published before. So far, no other bioactivity has been reported for them; this will require further research. Quercetin (62) is also a common compound represented in various plants, which was first described in 1936 and widely used due to its various actions, including antioxidant, anticancer, anti-inflammatory, hepato-protective, antiallergic, and antimicrobial activities. Its wide antimicrobial spectrum has been proven against over 32 bacteria and nine fungi. In addition, it also shows anti-biofilm activity against S. aureus. Kaempferol (68) is a very common compound that has been found in many herbal medicines and edible plants. It gained popularity due to its pharmacological properties, including antioxidant, anti-inflammatory, antibacterial, antiviral, antifungal, antiprotozoal, anticancer, hepatoprotective, neuroprotective, and cardioprotective activity, etc. As an antimicrobial, kaempferol is active against over 37 bacteria and nine fungi, including S. aureus, E. coli, C. albicans, etc. Kaempferol was also found active against biofilms, including S. aureus, C. parapsilosis, etc. E-4-(4,8-dimethylnona-3,7-dienyl)furan-2(5H)-one (79) has been isolated from Chimonanthus species and was reported to be active against B. subtilis, and E. coli, as well as diisobutyl phalate and 4-hydroxy-3-[(2E,6E)-3,7,11-trimethyl-2,6,10-dodecatrien-1-yl] benzoic acid, while scopoletin only showed activity against B. subtilis. (Ya et al., 2013). However, this study didn’t show its activity against E. coli during bioassay-guided separation, which could be the reason for the low content.
According to the previous study, there are many other compounds with antimicrobial activities isolated from Chimonanthus plants, including the alkaloids (calycanthine against plant pathogens, such as Alternaria brassicicola, Cladosporium fulvum and Botrytis cinerea; d-calycanthine and l-folicanthine against Exserohilum turcicum, Bipolaris maydis, Alternaria solani, Sclerotinia sderotiorum, and Fusarium oxysportium; etc.), the coumarins (scopoletin against M. lutus and E.coli; 5,6,7-trimethoxycoumarin, calycanthoside, and xeroboside against M. lutus; etc.) (Shu et al., 2019), the flavonoids, terpenoids, essential oil, etc. The chemical investigations in Chimonanthus revealed that these plants have high content of flavonoids (such as quercetin, kaempferol, rutin, hyperin, isoquercitrin, afzelin, etc.), coumarins and terpenoids [such as 21.43% 3-(4,8-dimethylnona-3,7-dienyl)-furan, 10.51% longifolenaldehyde, 11.85% (+)-2-bornanone, 5.69% Δ-cadinene of Chimonanthus nitens], while with low yield of bioactive alkaloids (Shu et al., 2019; He et al., 2022; Chen et al., 2023). The results of this study matched well with the chemical investigations of Chimonanthus plants, and the nine active compounds isolated from C. grammatus included three flavonoids (47, 62, 68), three terpenoids (1, 67, 79), one coumarin (40), steroid (53) and organic acid (31). These nine compounds have been reported in other Chimonanthus plants, such as homalomenol C from C. praecox fruit (Wang et al., 2011); Jasmonic acid from C. praecox flower bud (Zhang and Chen, 2012); isofraxidin from C. praecox flower bud, root, stem, C. salicifolius aerial parts and C. nitens leaf; quercitrin from C. praecox flower (Shu et al., 2019); stigmasta-7,22-diene-3β,5α,6α-triol from C. salicifolius aerial parts (Li et al., 2016); 4-hydroxy-1,10-secocadin-5-ene-1,10-dione from C. praecox rhizome (Y., 2013); quercetin from flower and leaf of C. praecox, C. salicifolius, C. nitens, C. zhejiangensis, and C. grammatus; kaempferol from flower, leaf in C. praecox, C. salicifolius, C. nitens, C. zhejiangensis, and C. grammatus; E-4-(4,8-dimethylnona-3,7-dienyl)furan-2(5H)-one from C. grammatus leaf (Wang, 2012; Shu et al., 2019).
However, many natural compounds were not very clear for their bioactivities, such as antimicrobial activities. Hence, it is worth doing the biotest even if they are known. The bioassay-guided purification work normally obtains the main active compounds from plant extracts, as well as their activities, which can be quite useful to know the chemical basis of medicinal herb’s bioactivities. Although these nine compounds isolated here are known, it is still important to figure out all of them from C. grammatus via bioassay-guided purification. These active compounds can be used for quality control when developing the plant as medicine. Furthermore, we report for the first time the activity of compounds 1, 53, and 79 against S. aureus, and the antibiofilm activity of compounds 40 and 79. The BIC50 and BEC50 of compounds 40, 47, and 68 were measured for the first time via a resazurin-based viability staining method for microbial biofilms. According to the MS semiquantitative analysis, compounds 68 (19.3% content), 79 (6.1%), 47 (4.0%), 62 (3.7%), and 40 (3.1%) dominated in the ChG extract, which are the main roles in the antimicrobial activities of ChG, according to the bioassay in this study. Kaempferol (68) most likely accounts for much of the antimicrobial activity of the ChG extracts, but its oral bioavailability is limited and its pharmacokinetics are unfavorable (rapid elimination and metabolism) (Barve et al., 2009; Zabela et al., 2016). Bioactive analogs or certain formulations may circumvent these limitations (Ren et al., 2019), and topical use may be possible (Özay et al., 2019). Its safety permits use in food products as a preservative (Martinengo et al., 2021). In this study, some minor compounds also showed antimicrobial activity, suggesting that it is worth pursuing even those by bioassay-guided purification. To summarize, all nine compounds could contribute to the antimicrobial activity of ChG extracts and their potential additive, synergistic or antagonistic effects remain to be established.
4 Conclusions
We showed activity of different C. grammatus extracts against SA (IC50 = 498 μg/mL for methanol extract), ML (IC50 = 957 μg/mL for hexane extract, 494 μg/mL for EtOAc extract), BD (IC50 = 933 μg/mL for hexane extract, 935 μg/mL for water extract), EC (IC50 = 863 μg/mL for methanol extract), SF (IC50 = 907 μg/mL for methanol extract), SLE (IC50 = 964 μg/mL for methanol extract), and SE (IC50 = 926 μg/mL for methanol extract). These different extracts are active against different microorganisms, which may suggest that the plant contains different antimicrobial compounds, presumably with different mechanisms of action. Via bioassay-guided purification work, nine active compounds were identified, and all are active against S. aureus, meanwhile compounds 40, 47, 62, and 68 are active against E. coli, and only compound 1 is active against C. albicans in this study. Activity against biofilm formation and preformed biofilms of S. aureus strains was found for compound 40 (IC50 = 13.51, MBC≥250, BIC50 = 15.43, BEC50 = 45.86 µg/mL), 47 (IC50 = 15.86, MBC≥250, BIC50 = 18.86, BEC50 = 57.62 µg/mL) and 68 (IC50 = 18.1, MBC≥250, BIC50 = 17.31, BEC50≥62.50 µg/mL), but not for compound 79 (IC50>250, MBC>250, BIC50>250, BEC50>250 µg/mL). In addition, the MS identification approach used in this study demonstrated that computer-assisted structure elucidation with UHPLC-HRMS-MS can work well for compound identifications, especially for distinguishing isomers with similar structures. Combined with chromatographic separation, it can be used for the phytochemical analysis of herbal medicine and other complex samples. The antimicrobial compounds reported here provide the chemical basis of C. grammatus bioactivity and support the scientific development of its quality control and therapeutic use.
Data availability statement
The original contributions presented in the study are included in the article/Supplementary Material. Further inquiries can be directed to the corresponding authors.
Ethics statement
The procedure and experimental research were approved by the Ethics and Research Committee of Gannan Medical University (approval number: 2016413). All the methods, including for experimental or field studies, and the collection of plant material, comply with relevant guidelines and regulations.
Author contributions
Conceptualization, HbH; methodology, HbH and VT; software, HbH; validation, HbH and VT; formal analysis, HbH; investigation, HbH, BH, and HaH; data curation, HbH; writing—original draft preparation, HbH and VT; writing—review and editing, HbH, MY, AK, AKG, SKP, HaH, and WL; visualization, HbH; supervision, WL; project administration, WL and HbH; funding acquisition, WL, HbH, and HaH. All authors contributed to the article and approved the submitted version.
Funding
This work was funded by the Scientific Research Key Project of the Jiangxi Education Department (No. GJJ211501), International Cooperation and Exchange of the National Natural Science Foundation of China (No. 82111530101) - Fonds Wetenschappelijk Onderzoek of Belgium (No. VS06321N). HH1 is grateful for the support by China Scholarship Council (Grant Nos. 202008360249). Walter Luyten largely funded himself.
Conflict of interest
The authors declare that the research was conducted in the absence of any commercial or financial relationships that could be construed as a potential conflict of interest.
Publisher’s note
All claims expressed in this article are solely those of the authors and do not necessarily represent those of their affiliated organizations, or those of the publisher, the editors and the reviewers. Any product that may be evaluated in this article, or claim that may be made by its manufacturer, is not guaranteed or endorsed by the publisher.
Supplementary material
The Supplementary Material for this article can be found online at: https://www.frontiersin.org/articles/10.3389/fpls.2023.1138913/full#supplementary-material
References
Abdel-Raouf, N., Ibraheem, I. B. M., Abdel-Tawab, S., Naser, Y. A. G. (2011). Antimicrobial and antihyperlipidemic activity of isolated quercentin from Anabaena aequalisi. J. Phycol. 47, 955–962. doi: 10.1111/J.1529-8817.2011.01020.X
Acharyya, P., Sarma, A. (2014). Antimicrobial activity of compounds isolated from Artemisia caruifolia. Asian J. Biochem. Pharm. Res. 4, 60–65. Available at: https://saepub.com/acc.php?journal_name=AJBPR&volume=4&issue=4.
Ajibesin, K. K., Essien, E. E., Adesanya, S. A. (2011). Antibacterial constituents of the leaves of Dacryodes edulis. Afr. J. Pharm. Pharmacol. 5, 1782–1786. doi: 10.5897/AJPP11.371
Alvarez, M. A., Debattista, N. B., Pappano, N. B. (2008). Antimicrobial activity and synergism of some substituted flavonoids. Folia Microbiol. 2008 531 53, 23–28. doi: 10.1007/S12223-008-0003-4
Arima, H., Ashida, H., Danno, G. (2002). Rutin-enhanced antibacterial activities of flavonoids against Bacillus cereus and Salmonella enteritidis. Biosci. Biotechnol. Biochem. 66, 1009–1014. doi: 10.1271/BBB.66.1009
Aye, S. S., Hnin Aye, H., Ngwe, D. H., Myint, S. H. (2018). Comparative studies of antibacterial activity and phytochemical constituents of some Myanmar indigenous medicinal plants. J. Myanmar. Acad. Arts Sci. 16, 25–42. Available at: http://maas.edu.mm/Research/Admin/pdf/3drsan2aye.pdf.
Barve, A., Chen, C., Hebbar, V., Desiderio, J., Saw, C. L. L., Kong, A. N. (2009). Metabolism, oral bioavailability and pharmacokinetics of chemopreventive kaempferol in rats. Biopharm. Drug Dispos. 30, 356. doi: 10.1002/BDD.677
Bingkun, X., Yaoming, L. (2003). Advances study on taxonomy, Chemical Constituents and pharmacological effects of Chimonanthus. Res. Pract. Chinese Med. 2, 59–61. doi: 10.3969/j.issn.1673-6427.2003.02.032
Blaženović, I., Kind, T., Ji, J., Fiehn, O. (2018). Software tools and approaches for compound identification of LC-MS/MS data in metabolomics. Metab. 8, 31. doi: 10.3390/METABO8020031
Cai, L., Wu, C. D. (1996). Compounds from syzygium aromaticum possessing growth inhibitory activity against oral pathogens. J. Nat. Prod. 59, 987–990. doi: 10.1021/NP960451Q
Calderon-Montano, J. M., Burgos-Moron, E., Perez-Guerrero, C., Lopez-Lazaro, M. (2011). A review on the dietary flavonoid kaempferol. Mini Rev. Med. Chem. 11, 298–344. doi: 10.2174/138955711795305335
Cernakova, M., Košťálová, D. (2008). Antimicrobial activity of berberine–a constituent of Mahonia aquifolium. Folia Microbiol. 47 (4), 375–378. doi: 10.1007/BF02818693
Champarnaud, E., Hopley, C. (2011). Evaluation of the comparability of spectra generated using a tuning point protocol on twelve electrospray ionisation tandem-in-space mass spectrometers. Rapid Commun. Mass Spectrom. 25, 1001–1007. doi: 10.1002/RCM.4940
Chen, L., Li, X., Gu, Q. (2023). Chimonanthus salicifolius extract alleviates DSS-induced colitis and regulates gut microbiota in mice. Food Sci. Nutr. 00, 1–12. doi: 10.1002/FSN3.3282
Chen, J., Li, G., Sun, C., Peng, F., Yu, L., Chen, Y., et al. (2022). Chemistry, pharmacokinetics, pharmacological activities, and toxicity of quercitrin. Phyther. Res. 36, 1545–1575. doi: 10.1002/PTR.7397
Crumplin, G. C., Smith, J. T. (1975). Nalidixic acid: an antibacterial paradox. Antimicrob. Agents Chemother. 8, 251. doi: 10.1128/AAC.8.3.251
Cubukcu, B., Bray, D. H., Warhurst, D. C., Mericli, A. H., Ozhatay, N., Sariyar, G. (1990). In vitro antimalarial activity of crude extracts and compounds from Artemisia abrotanum L. Phyther. Res. 4, 203–204. doi: 10.1002/ptr.2650040510
del Valle, P., García-Armesto, M. R., de Arriaga, D., González-Donquiles, C., Rodríquez-Fernández, P., Rúa, J. (2016). Antimicrobial activity of kaempferol and resveratrol in binary combinations with parabens or propyl gallate against Enterococcus faecalis. Food Control 61, 213–220. doi: 10.1016/J.FOODCONT.2015.10.001
Deng, C., Song, G., Hu, Y. (2004). Rapid determination of volatile compounds emitted from Chimonanthus praecox flowers by HS-SPME-GC-MS. Z. fur Naturforsch. - Sect. C J. Biosci. 59, 636–640. doi: 10.1515/ZNC-2004-9-1005
Deng, S. P., Zhang, J. Y., Ma, Z. W., Wen, S., Tan, S., Cai, J. Y. (2021). Facile synthesis of long-term stable silver nanoparticles by kaempferol and their enhanced antibacterial activity against escherichia coli and Staphylococcus aureus. J. Inorg. Organomet. Polym. Mater. 31, 2766–2778. doi: 10.1007/S10904-020-01874-2
Dong, J., Ruan, J., Xu, N., Yang, Y., Ai, X. (2016). In vitro synergistic effects of fisetin and norfloxacin against aquatic isolates of Serratia marcescens. FEMS Microbiol. Lett. 363, 220. doi: 10.1093/FEMSLE/FNV220
Dongqing, C., Hao, F., Peilei, P., Meiai, L., Yitao, C. (2005). Experimental study on bacteriostasis of Chimonanthus zhejiangensis M.C.Liu. J. Pract. Tradit. Chin. Med. 21, 314. doi: 10.3969/j.issn.1004-2814.2005.05.073
Elansary, H. O., Szopa, A., Klimek-Szczykutowicz, M., Ekiert, H., Barakat, A. A., Al-Mana, F. A. (2020). Antiproliferative, antimicrobial, and antifungal activities of polyphenol extracts from Ferocactus species. Process. 8, 138. doi: 10.3390/PR8020138
Flora of China Editorial Committee (2008) Flora of China (English version,volumn 7) (Beijing, China: Missouri Botanical Garden Press). Available at: http://www.efloras.org/florataxon.aspx?flora_id=2&taxon_id=242312429 (Accessed 17, April, 2023).
Gaba, S., Saini, A., Singh, G., Monga, V. (2021). An insight into the medicinal attributes of berberine derivatives: A review. Bioorg. Med. Chem. 38, 116143. doi: 10.1016/J.BMC.2021.116143
Gao, L., Tang, Z., Li, T., Wang, J. (2021). Combination of kaempferol and azithromycin attenuates Staphylococcus aureus-induced osteomyelitis via anti-biofilm effects and by inhibiting the phosphorylation of ERK1/2 and SAPK. Pathog. Dis. 79, ftab048. doi: 10.1093/FEMSPD/FTAB048
Gehrke, I. T. S., Neto, A. T., Pedroso, M., Mostardeiro, C. P., Da Cruz, I. B. M., Silva, U. F., et al. (2013). Antimicrobial activity of Schinus lentiscifolius (Anacardiaceae). J. Ethnopharmacol. 148, 486–491. doi: 10.1016/J.JEP.2013.04.043
Gómez-Florit, M., Monjo, M., Ramis, J. M. (2014). Identification of quercitrin as a potential therapeutic agent for periodontal applications. J. Periodontol. 85, 966–974. doi: 10.1902/JOP.2014.130438
Guo, N., Shu, Q.b., Zhang, P.y., Fu, J.j., Wan, Y., Wang, X.m., et al. (2021). Six new cadinane-type sesquiterpenoids from the leaves of Chimonanthus nitens Oliv. Fitoterapia 154, 105019. doi: 10.1016/J.FITOTE.2021.105019
Hanmin, W. (2019). Study on the chemical constituents and activities of Saruma henryi Oliv. Available at: https://kns.cnki.net/kcms2/article/abstract?v=3uoqIhG8C475KOm_zrgu4lQARvep2SAkOsSuGHvNoCRcTRpJSuXuqdUR-qdnfmP5Ya2rdtQ0LJXWlB1QffOQdBpKQI0e6gCG&uniplatform=NZKPT.
Hasan, S., Singh, K., Danisuddin, M., Verma, P. K., Khan, A. U. (2014). Inhibition of major virulence pathways of Streptococcus mutans by quercitrin and deoxynojirimycin: A synergistic approach of infection control. PLoS One 9, e91736. doi: 10.1371/JOURNAL.PONE.0091736
He, J., Zhang, Y., Ouyang, K., Chen, L., Meng, W., Zhang, Y., et al. (2022). Extraction, chemical composition, and protective effect of essential oil from Chimonanthus nitens Oliv. leaves on dextran sodium sulfate-induced colitis in mice. Oxid. Med. Cell. Longev. 2022, 9701938. doi: 10.1155/2022/9701938
Henchiri, H., Bodo, B., Deville, A., Dubost, L., Zourgui, L., Raies, A., et al. (2009). Sesquiterpenoids from Teucrium ramosissimum. Phytochemistry 70, 1435–1441. doi: 10.1016/J.PHYTOCHEM.2009.08.012
Hu, H., Hu, C., Peng, J., Ghosh, A. K., Khan, A., Sun, D., et al. (2021a). Bioassay-guided interpretation of antimicrobial compounds in kumu, a TCM preparation from Picrasma quassioides’ stem via UHPLC-Orbitrap-Ion trap mass spectrometry combined with fragmentation and retention time calculation. Front. Pharmacol. 12. doi: 10.3389/FPHAR.2021.761751
Hu, H., Lee-Fong, Y., Peng, J., Hu, B., Li, J., Li, Y., et al. (2021b). Comparative research of chemical profiling in different parts of Fissistigma oldhamii by ultra-High-Performance liquid chromatography coupled with hybrid quadrupole-orbitrap mass spectrometry. Molecules 26, 960. doi: 10.3390/molecules26040960
Hu, Y. M., Liu, C., Cheng, K. W., Sung, H. H. Y., Williams, L. D., Yang, Z. L., et al. (2008). Sesquiterpenoids from Homalomena occulta affect osteoblast proliferation, differentiation and mineralization in vitro. Phytochemistry 69, 2367–2373. doi: 10.1016/J.PHYTOCHEM.2008.05.023
Hu, H., Yang, Y., Aissa, A., Tekin, V., Li, J., Panda, S. K., et al. (2022). Ethnobotanical study of hakka traditional medicine in ganzhou, China and their antibacterial, antifungal, and cytotoxic assessments. BMC Complement. Med. Ther. 22, 244. doi: 10.1186/S12906-022-03712-Z
Huang, W., Wen, Z., Wang, M., Xu, B., Zhou, B., Li, X. (2020). Anticomplement and antitussive activities of major compound extracted from Chimonanthus nitens Oliv. leaf. Biomed. Chromatogr. 34, e4736. doi: 10.1002/BMC.4736
Hufsky, F., Scheubert, K., Böcker, S. (2014). Computational mass spectrometry for small-molecule fragmentation. TrAC Trends Anal. Chem. 53, 41–48. doi: 10.1016/J.TRAC.2013.09.008
Huiping, W., Jianzhong, X., Weimin, L., Jiana, J. (2018). Optimization of extraction process of total flavonoids from Chimonanthus salicifolius S.Y.H by HPLC combined with response surface methodology and its antibacterial activity. Acta Agric. ZHEJIANGENSIS 30, 298–306. doi: 10.3969/j.issn.1004-1524.2018.02.17
Jaisinghani, R. N. (2017). Antibacterial properties of quercetin. Microbiol. Res. 8, 6877. doi: 10.4081/MR.2017.6877
Jang, J. Y., Shin, H., Lim, J. W., Ahn, J. H., Jo, Y. H., Lee, K. Y., et al. (2018). Comparison of antibacterial activity and phenolic constituents of bark, lignum, leaves and fruit of Rhus verniciflua. PLoS One 13, e0200257. doi: 10.1371/JOURNAL.PONE.0200257
JC, D., XZ, W., G, D. (2002). Experimental study on disinfection and bacteriostasis of Chimonanthus nitens Oliv. J. Jiangxi Univ Tradit Chin. Med. 33, 35. doi: 10.3969/j.issn.0411-9584.2002.06.031
Jiang, Y., Li, Y., Lu, S., Liu, Y., Peng, J., Zhu, D. (2012). Genetic diversity of Chimonanthus grammatus populations determined with inter-simple sequence repeats (ISSR) analysis: Implications for conservation. J. Med. Plants Res. 6, 1272–1278. doi: 10.5897/JMPR11.1337
Jiang, Y. M., Liu, Y. X., Zhang, Z.B., Lu, S. B., Peng, J. S., Xie, J., et al. (2013). New microsatellite DNA primers for Chimonanthus grammatus peculiarly distributed in southern China. Adv. Mater. Res. 726–731, 4509–4511. doi: 10.4028/WWW.SCIENTIFIC.NET/AMR.726-731.4509
Jiang, Y.-M., Wang, J., Liu, Y.-X., Zhang, Z.-B., Cao, G., Lu, S., et al. (2015). Genetic patterns investigation of wild Chimonanthus grammatus M.C.Liu by using SSR markers. Acta Ecol. Sin. 35, 203–209. doi: 10.1016/J.CHNAES.2015.07.007
Jiwen, Z., Chunping, Y. A. N. G., Zhao, P., Wenjun, W. (2005). Antibiotic constituents in Chimonanthus praecox seeds. Acta Bot. Boreali-occidentalia Sin. 25, 2068–2071. doi: 10.3321/j.issn:1000-4025.2005.10.023
Kang, S., Li, T. (2016). Study on sedative and hypnotic effects of isofraxidin. Zhongyiyao Xuebao 44, 30–32. doi: 10.3969/j.issn.1002-2392.2016.06.010
Kataoka, M., Hirata, K., Kunikata, T., Ushio, S., Iwaki, K., Ohashi, K., et al. (2001). Antibacterial action of tryptanthrin and kaempferol, isolated from the indigo plant (Polygonum tinctorium lour.), against Helicobacter pylori-infected Mongolian gerbils. J. Gastroenterol. 36 (1), 5–9. doi: 10.1007/S005350170147
Kipanga, P. N., Liu, M., Panda, S. K., Mai, A. H., Veryser, C., Van Puyvelde, L., et al. (2020). Biofilm inhibiting properties of compounds from the leaves of Warburgia ugandensis sprague subsp ugandensis against Candida and staphylococcal biofilms. J. Ethnopharmacol. 248, 112352. doi: 10.1016/J.JEP.2019.112352
Kuete, V., Alibert-Franco, S., Eyong, K. O., Ngameni, B., Folefoc, G. N., Nguemeving, J. R., et al. (2011). Antibacterial activity of some natural products against bacteria expressing a multidrug-resistant phenotype. Int. J. Antimicrob. Agents 37, 156–161. doi: 10.1016/J.IJANTIMICAG.2010.10.020
Lan, C., Juan, S., Wannian, Y., Lingzhi, F., Yingying, C., Lan, C., et al. (2020). Study on the antibiofilm activity of kaempferol in Candida albicans. J. Pharm. Pract. Serv. 413–417, 430. doi: 10.12206/J.ISSN.1006-0111.202004050
Lee, K. H., Kong, H. J., Cho, Y. L., Gue Joo, C., Kwon, S. S., Hwang, J. S., et al. (2012). Anti-microbial and anti-wrinkle effect of kaempferol and kaempferol rhamnosides isolated from Hibiscus cannabinus L. Korean J. Med. Crop Sci. 20, 454–460. doi: 10.7783/KJMCS.2012.20.6.454
Lee, K. A., Moon, S. H., Kim, K. T., Nah, S. Y., Paik, H. D. (2011). Antimicrobial effect of kaempferol on psychrotrophic Bacillus cereus strains outbreakable in dairy products. Food Sci. Anim. Resour. 31, 311–315. doi: 10.5851/KOSFA.2011.31.2.311
Lee, S. Y., So, Y. J., Shin, M. S., Cho, J. Y., Lee, J. (2014). Antibacterial effects of afzelin isolated from Cornus macrophylla on Pseudomonas aeruginosa, a leading cause of illness in immunocompromised individuals. Mol. 19, 3173–3180. doi: 10.3390/MOLECULES19033173
Li, D., Jiang, Y. Y., Jin, Z. M., Li, H. Y., Xie, H. J., Wu, B., et al. (2016). Isolation and absolute configurations of diastereomers of 8α-hydroxy-T-muurolol and (1α,6β,7β)-cadinane-4-en-8α,10α-diol from Chimonanthus salicifolius. Phytochemistry 122, 294–300. doi: 10.1016/J.PHYTOCHEM.2016.01.005
Li, Y., Sun, K., Liang, L., Wang, M., Sun, Z. (2020). A new monoterpene-flavanone conjugate from the aerial parts of Chimonanthus grammatus. Chem. Nat. Compd. 56, 811–813. doi: 10.1007/S10600-020-03158-0
Li, Q., Wang, L., Liu, S., Jinziyuan, J., Inc, B., Xu, J., et al. (2021). Inhibition of Salmonella enterica serovar typhimurium type III secretion system and infection using small molecule quercitrin. Res. Sq. doi: 10.21203/RS.3.RS-1129712/V1
Li, S., Zou, Z. (2018). Advance in studies on Flavinoids, Coumarins and pharmacological activity of Chinonanthus. Chin. Tradit. Herb. Drugs 49, 3425–3431. doi: 10.7501/j.issn.0253-2670.2018.14.032
Lim, Y.-H., Kim, I.-H., Seo, J.-J. (2007). In vitro activity of kaempferol isolated from the Impatiens balsamina alone and in combination with erythromycin or clindamycin against Propionibacterium acnes. J. Microbiol. 45, 473–477. Available at: https://koreascience.kr/article/JAKO200735822306674.pdf.
Lin, R. D., Chin, Y. P., Lee, M. H. (2005). Antimicrobial activity of antibiotics in combination with natural flavonoids against clinical extended-spectrum β-lactamase (ESBL)-producing Klebsiella pneumoniae. Phyther. Res. 19, 612–617. doi: 10.1002/PTR.1695
Lin, S., Li, H., Tao, Y., Liu, J., Yuan, W., Chen, Y., et al. (2020). In vitro and in vivo evaluation of membrane-active flavone amphiphiles: Semisynthetic kaempferol-derived antimicrobials against drug-resistant gram-positive bacteria. J. Med. Chem. 63, 5797–5815. doi: 10.1021/ACS.JMEDCHEM.0C00053
Ling, Q. (2014). Studies on chemical constituents and pharmacological activities of Chimonanthus grammatus leaves. Jiangxi Normal University, 1–54. Available at: https://kns.cnki.net/kcms2/article/abstract?v=3uoqIhG8C475KOm_zrgu4lQARvep2SAkVNKPvpjdBoadmPoNwLRuZ6teIYtu1RiUzznIKBRPjK2zikuoQLWpco1HMAyRSD4&uniplatform=NZKPT.
Ling, Q., Zou, Z. R. (2014). The study on chemical constituents and acetylcholinesterase inhibitory activity of petroleum ether extracts from leaves of Chimonanthus grammatus. J. Jiangxi Norm Univ (Nat Sci) 38, 196–200. doi: 10.3969/j.issn.1000-5862.2014.02.019
Lingyun, W., Zhibin, Z., Zhengrong, Z., Du, Z. (2012). Advances of Studies on Chemical Composition and Pharmacological Activity of Chimonanthus Lindl. Lishizhen Med. Mater. Medica Res. 12, 1981–1983. doi: 10.3969/j.issn.1008-0805.2012.12.072
Liu, F., Liu, C., Liu, W., Ding, Z., Ma, H., Seeram, N. P., et al. (2017). New sesquiterpenoids from Eugenia jambolana seeds and their anti-microbial activities. J. Agric. Food Chem. 65, 10214–10222. doi: 10.1021/ACS.JAFC.7B04066
Liu, L., Mu, Q., Li, W., Xing, W., Zhang, H., Fan, T., et al. (2015). Isofraxidin protects mice from LPS challenge by inhibiting pro-inflammatory cytokines and alleviating histopathological changes. Immunobiology 220, 406–413. doi: 10.1016/J.IMBIO.2014.10.007
Liu, Y., Yan, R., Lu, S., Zhang, Z., Zou, Z., Zhu, D. (2011). Chemical composition and antibacterial activity of the essential oil from leaves of Chimonanthus grammatus. Zhongguo Zhong Yao Za Zhi 36, 3149–3154. doi: 10.4268/cjcmm20112219
Lu, W., Cui, Y., Zhang, L. (2022). Isofraxidin exerts anti-diabetic, antilipidemic, and antioxidant effects and protects renal tissues via inhibition of NF-ĸB in streptozotocin-induced diabetic rats. Mol. Cell. Toxicol. 1–11. doi: 10.1007/S13273-021-00204-Y
Lu, Y. H., Zhang, Z., Shi, G. X., Meng, J. C., Tan, R. X. (2002). A new antifungal flavonol glycoside from Hypericum perforatum. J. Integr. Plant Biol. 44, 743–745. doi: 10.3321/j.issn:1672-9072.2002.06.019
Luan, N. Q., Thanh, P. T. Y., Dung, T. N., Ba, H.V. (2019). Inhibitory ability of extracts and compounds from leaves of Cassia grandis L.F to Propionibacterium acnes. AGU Int. J. Sci. 7, 1–11. Available at: https://apps.agu.edu.vn/qlkh//storage/app/agu/1575014577-01-ngo-quoc-luanpdf.pdf.
Martinengo, P., Arunachalam, K., Shi, C. (2021). Polyphenolic antibacterials for food preservation: Review, challenges, and current applications. Foods 10, 2469. doi: 10.3390/FOODS10102469
Martini, S., D’Addario, C., Colacevich, A., Focardi, S., Borghini, F., Santucci, A., et al. (2009). Antimicrobial activity against Helicobacter pylori strains and antioxidant properties of blackberry leaves (Rubus ulmifolius) and isolated compounds. Int. J. Antimicrob. Agents 34, 50–59. doi: 10.1016/J.IJANTIMICAG.2009.01.010
McEachran, A. D., Mansouri, K., Newton, S. R., Beverly, B. E. J., Sobus, J. R., Williams, A. J. (2018). A comparison of three liquid chromatography (LC) retention time prediction models. Talanta 182, 371–379. doi: 10.1016/j.talanta.2018.01.022
Memariani, H., Memariani, M., Ghasemian, A. (2019). An overview on anti-biofilm properties of quercetin against bacterial pathogens. World J. Microbiol. Biotechnol. 35, 1–16. doi: 10.1007/S11274-019-2719-5
Neto, G. C., Kono, Y., Hyakutake, H., Watanabe, M., Suzuki, Y., Sakurai, A. (1991). Isolation and identification of (-)-Jasmonic acid from wild rice, Oryza qfficinalis, as an antifungal substance. Agric. Biol. Chem. 55, 3097–3098. doi: 10.1271/BBB1961.55.3097
Niu, X., Wang, Y., Li, W., Mu, Q., Li, H., Yao, H., et al. (2015). Protective effects of isofraxidin against lipopolysaccharide-induced acute lung injury in mice. Int. Immunopharmacol. 24, 432–439. doi: 10.1016/j.intimp.2014.12.041
Niu, X., Xing, W., Li, W., Fan, T., Hu, H., Li, Y. (2012). Isofraxidin exhibited anti-inflammatory effects in vivo and inhibited TNF-α production in LPS-induced mouse peritoneal macrophages in vitro via the MAPK pathway. Int. Immunopharmacol. 14, 164–171. doi: 10.1016/j.intimp.2012.06.022
Okuyama, E., Hasegawa, T., Matsushita, T., Fujimoto, H., Ishibashi, M., Yamazaki, M. (2001). Analgesic components of saposhnikovia root (Saposhnikovia divaricata). Chem. Pharm. Bull. (Tokyo). 49, 154–160. doi: 10.1248/CPB.49.154
Otsuka, N., Liu, M. H., Shiota, S., Ogawa, W., Kuroda, T., Hatano, T., et al. (2008). Anti-methicillin resistant Staphylococcus aureus (MRSA) compounds isolated from Laurus nobilis. Biol. Pharm. Bull. 31, 1794–1797. doi: 10.1248/BPB.31.1794
Özay, Y., Güzel, S., Yumrutaş, Ö., Pehlivanoğlu, B., Erdoğdu, İ.H., Yildirim, Z., et al. (2019). Wound healing effect of kaempferol in diabetic and nondiabetic rats. J. Surg. Res. 233, 284–296. doi: 10.1016/J.JSS.2018.08.009
Özkütük, A. S. (2022). Antimicrobial effects of carnosic acid, kaempferol and luteolin on biogenic amine production by spoilage and food-borne pathogenic bacteria. Food Biosci. 46, 101588. doi: 10.1016/J.FBIO.2022.101588
Patel, R. V., Mistry, B. M., Shinde, S. K., Syed, R., Singh, V., Shin, H. S. (2018). Therapeutic potential of quercetin as a cardiovascular agent. Eur. J. Med. Chem. 155, 889–904. doi: 10.1016/J.EJMECH.2018.06.053
Pelander, A., Tyrkkö, E., Ojanperä, I. (2009). In silico methods for predicting metabolism and mass fragmentation applied to quetiapine in liquid chromatography/time-of-flight mass spectrometry urine drug screening. Rapid Commun. Mass Spectrom. 23, 506–514. doi: 10.1002/rcm.3901
Ping, L., Ming, H., Changchun, T., Yongjun, W., Qiwang, H., Wei, H. (1996). Experimental study on bacteriostatic, anti-inflammatory and antipyretic effect of shanlamei ganmao tea. Chin. J. Tradit Med. Sci. Technol. 3, 19–21. Available at: https://kns.cnki.net/kcms2/article/abstract?v=3uoqIhG8C44YLTlOAiTRKjkpgKvIT9NkZNmQNo4kSVo64EosRRLERxtsgVb3wO1-W2Zw9AuP8a-DiaA9ZAO6XISFdf79bn81&uniplatform=NZKPT.
Pirbalouti, A. G., Sajjadi, S. E., Parang, K. (2014). A review (Research and patents) on jasmonic acid and its derivatives. Arch. Pharm. (Weinheim). 347, 229–239. doi: 10.1002/ARDP.201300287
Punia Bangar, S., Chaudhary, V., Sharma, N., Bansal, V., Ozogul, F., Lorenzo, J. M., et al. (2022). Kaempferol: A flavonoid with wider biological activities and its applications. Crit. Rev. Food Sci. Nutr. 25, 1–25. doi: 10.1080/10408398.2022.2067121
Ramos, F. A., Takaishi, Y., Shirotori, M., Kawaguchi, Y., Tsuchiya, K., Shibata, H., et al. (2006). Antibacterial and antioxidant activities of quercetin oxidation products from yellow onion (Allium cepa) skin. J. Agric. Food Chem. 54, 3551–3557. doi: 10.1021/JF060251C
Rauf, A., Imran, M., Khan, I. A., ur-Rehman, M., Gilani, S. A., Mehmood, Z., et al. (2018). Anticancer potential of quercetin: A comprehensive review. Phyther. Res. 32, 2109–2130. doi: 10.1002/PTR.6155
Ren, J., Lu, Y., Qian, Y., Chen, B., Wu, T., Ji, G. (2019). Recent progress regarding kaempferol for the treatment of various diseases. Exp. Ther. Med. 18, 2759–2776. doi: 10.3892/ETM.2019.7886
Rocha, M. F. G., Sales, J. A., da Rocha, M. G., Galdino, L. M., de Aguiar, L., Pereira-Neto, W., et al. (2019). Antifungal effects of the flavonoids kaempferol and quercetin: a possible alternative for the control of fungal biofilms. Biofouling 35, 320–328. doi: 10.1080/08927014.2019.1604948
Rofeal, M., El-Malek, F. A., Qi, X. (2021). In vitro assessment of green polyhydroxybutyrate/chitosan blend loaded with kaempferol nanocrystals as a potential dressing for infected wounds. Nanotechnology 32, 375102. doi: 10.1088/1361-6528/ABF7EE
Salazar-Aranda, R., Granados-Guzmán, G., Pérez-Meseguer, J., González, G. M., De Torres, N. W. (2015). Activity of polyphenolic compounds against Candida glabrata. Mol. 20, 17903–17912. doi: 10.3390/MOLECULES201017903
Sati, P., Dhyani, P., Bhatt, I. D., Pandey, A. (2019). Ginkgo biloba flavonoid glycosides in antimicrobial perspective with reference to extraction method. J. Tradit. Complement. Med. 9, 15–23. doi: 10.1016/J.JTCME.2017.10.003
Schymanski, E. L., Meringer, M., Brack, W. (2009). Matching structures to mass spectra using fragmentation patterns: Are the results as good as they look? Anal. Chem. 81, 3608–3617. doi: 10.1021/AC802715E
Shen, P., Wang, H. G., Li, M. M., Ma, Q. Y., Zhou, C. W., Pan, F., et al. (2017). Isofraxidin inhibited proliferation and induced apoptosis via blockage of Akt pathway in human colorectal cancer cells. Biomed. Pharmacother. 92, 78–85. doi: 10.1016/J.BIOPHA.2017.05.065
Shu, Y., Liu, Y., Li, L., Feng, J., Lou, B., Zhou, X., et al. (2011). Antibacterial activity of quercetin on oral infectious pathogens. Afr. J. Microbiol. Res. 5, 5358–5361. doi: 10.5897/AJMR11.849
Shu, R. G., Wan, Y. L., Wang, X. M. (2019). Non-volatile constituents and pharmacology of Chimonanthus: A review. Chin. J. Nat. Med. 17, 161–186. doi: 10.1016/S1875-5364(19)30020-2
Shuaifeng, L., Zhengrong, Z. (2018). Advance in studies on flavinoids, coumarins and pharmacological activity of Chimonanthus. Chinese Tradit. Herb. Drugs. 49, 3425–3431. doi: 10.7501/j.issn.0253-2670.2018.14.032
Siriwong, S., Teethaisong, Y., Thumanu, K., Dunkhunthod, B., Eumkeb, G. (2016). The synergy and mode of action of quercetin plus amoxicillin against amoxicillin-resistant Staphylococcus epidermidis. BMC Pharmacol. Toxicol. 17, 1–14. doi: 10.1186/S40360-016-0083-8
Stein, S. (2012). Mass spectral reference libraries: An ever-expanding resource for chemical identification. Anal. Chem. 84, 7274–7282. doi: 10.1021/AC301205Z
Sun, H., Lv, H., Zhang, Y., Wang, X., Bi, K., Cao, H. (2007). A rapid and sensitive UPLC-ESI MS method for analysis of isofraxidin, a natural antistress compound, and its metabolites in rat plasma. J. Sep. Sci. 30, 3202–3206. doi: 10.1002/JSSC.200700251
Tabudravu, J. N., Pellissier, L., Smith, A. J., Subko, K., Autréau, C., Feussner, K., et al. (2019). LC-HRMS-Database screening metrics for rapid prioritization of samples to accelerate the discovery of structurally new natural products. J. Nat. Prod. 82, 211–220. doi: 10.1021/ACS.JNATPROD.8B00575
Tatsimo, S. J. N., Tamokou, J. D. D., Havyarimana, L., Csupor, D., Forgo, P., Hohmann, J., et al. (2012). Antimicrobial and antioxidant activity of kaempferol rhamnoside derivatives from Bryophyllum pinnatum. BMC Res. Notes 5, 1–6. doi: 10.1186/1756-0500-5-158
Tatsimo, S. J. N., Tamokou, J.-D., Tsague, V. T., Lamshoft, M., Sarkar, P., Bag, P. K., et al. (2017). Antibacterial-guided isolation of constituents from Senna alata leaves with a particular reference against multi-Drug-Resistant Vibrio cholerae and Shigella flexneri. Int. J. Biol. Chem. Sci. 11, 46–53. doi: 10.4314/ijbcs.v11i1.4
Thuy, L., Nguyen, K., Nhu, H., Hoang, T., Do, T. T., Van, T., et al. (2022). Sesquiterpenoids from the rhizomes of Homalomena pendula and their anti-inflammatory activities. Nat. Prod. Res. 25, 1–9. doi: 10.1080/14786419.2022.2056182
Ulanowska, K., Majchrzyk, A., Moskot, M., Jakóbkiewicz-Banecka, J., Węgrzyn, G. (2007). Assessment of antibacterial effects of flavonoids by estimation of generation times in liquid bacterial cultures. Biol. 2007 622 62, 132–135. doi: 10.2478/S11756-007-0042-3
Wang, L. Y. (2012). Preliminary study on chemical compositions and their bioactive in leaves of Chimonanthus grammatus. Jiangxi Normal University, 1–43. https://kns.cnki.net/kcms2/article/abstract?v=3uoqIhG8C475KOm_zrgu4lQARvep2SAkVR3-_UaYGQCi3Eil_xtLb0pl9zKRpXC66j5fUkVusEsehLg0oFy78PREI5VTdUWo&uniplatform=NZKPT.
Wang, W. X., Cao, L., Xiong, J., Xia, G., Hu, J. F. (2011). Constituents from Chimonanthus praecox (wintersweet). Phytochem. Lett. 3, 271–274. doi: 10.1016/J.PHYTOL.2011.04.012
Wang, Z., Jiang, S., Guo, X., Wang, H., Liu, X. (2015). Process optimization of extraction conditions for isofaxidin from Eleutherococcus senticosus and its antioxidant and antitumor activities. Nat. Prod. Res. Dev. 27, 1092–1098. doi: 10.16333/j.1001-6880.2015.06.028
Wang, Y. T., Yan, W., Chen, Q. L., Huang, W. Y., Yang, Z., Li, X., et al. (2017). Inhibition viral RNP and anti-inflammatory activity of coumarins against influenza virus. Biomed. Pharmacother. 87, 583–588. doi: 10.1016/J.BIOPHA.2016.12.117
Wang, S., Yao, J., Zhou, B., Yang, J., Chaudry, M. T., Wang, M., et al. (2018). Bacteriostatic effect of quercetin as an antibiotic alternative In vivo and its antibacterial mechanism In vitro. J. Food Prot. 81, 68–78. doi: 10.4315/0362-028X.JFP-17-214
Willett, P., Barnard, J. M., Downs, G. M. (1998). Chemical similarity searching. J. Chem. Inf. Comput. Sci. 38, 983–996. doi: 10.1021/ci9800211
Xiao, B., Liu, Y. (2003). Advances study on taxonomy, chemical constituents and pharmacological effects of Chimonanthus. Res. Pract. Chin. Med. 2, 59–61. doi: 10.3969/j.issn.1673-6427.2003.02.032
Xu, D., Hu, M. J., Wang, Y. Q., Cui, Y. L. (2019). Antioxidant activities of quercetin and its complexes for medicinal application. Mol. 24, 1123. doi: 10.3390/MOLECULES24061123
Y., Z. (2013). Studies on the screening of active fractions and chemical constituents of guizhou “Miao” medicine tiekuaizi. Guiyang College of Traditional Chinese Medicine, 1–48. Available at: https://kns.cnki.net/kcms2/article/abstract?v=3uoqIhG8C475KOm_zrgu4lQARvep2SAk9z9MrcM-rOU4mSkGl_LWf-rCw3Z7IPgX4jDipl1nFHDyXz8htNdRK6-JpeymweHs&uniplatform=NZKPT.
Ya, W., Lingyun, W., Zhengrong, Z., Zhibin, Z., Riming, Y., Du, Z. (2013). Chemical composition and antimicrobial activity of Chimonanthus grammatus leave. Food Sci. 34, 42–45. doi: 10.7506/spkx1002-6630-201319010
Yamazaki, T., Tokiwa, T. (2010). Isofraxidin, a coumarin component from Acanthopanax senticosus, inhibits matrix metalloproteinase-7 expression and cell invasion of human hepatoma cells. Biol. Pharm. Bull. 33, 1716–1722. doi: 10.1248/BPB.33.1716
Yang, M. L., Chen, J. J., Wei, H. B., Gao, K. (2016b). Cytotoxic sesquiterpenoids from Senecio densiserratus. Phytochem. Lett. 16, 236–240. doi: 10.1016/J.PHYTOL.2016.04.017
Yang, J. L., Dao, T. T., Hien, T. T., Zhao, Y. M., Shi, Y. P. (2019). Further sesquiterpenoids from the rhizomes of Homalomena occulta and their anti-inflammatory activity. Bioorg. Med. Chem. Lett. 29, 1162–1167. doi: 10.1016/J.BMCL.2019.03.031
Yang, L., Ding, W., Xu, Y., Wu, D., Li, S., Chen, J., et al. (2016a). New insights into the antibacterial activity of hydroxycoumarins against Ralstonia solanacearum. Mol. 21, 468. doi: 10.3390/MOLECULES21040468
Ye, X., An, Q., Chen, S., Liu, X., Wang, N., Li, X., et al. (2020). The structural characteristics, antioxidant and hepatoprotection activities of polysaccharides from Chimonanthus nitens Oliv. leaves. Int. J. Biol. Macromol. 156, 1520–1529. doi: 10.1016/J.IJBIOMAC.2019.11.200
Yongxiang, W., Xiang, W., Haitao, J., Kangjun, D., Chenglong, Z., Changyu, H. (2017). Study on total polyphenol content and antioxidant, antimicrobial activities of different polarity fractions of Chimonanthus salicifolicus Hu leaves. Food Mach. 33, 150–154. doi: 10.13652/j.issn.1003-5788.2017.08.033
Ying, Z., Yuan, Z., Zhezhi, W. (2010). Chemical composition and biological acitivities of essential oil from flower of Chimonanthus praecox (L.)Link. Lishizhen Med. Mater. Med. Res. 21, 622–625. doi: 10.3969/j.issn.1008-0805.2010.03.054
Zabela, V., Sampath, C., Oufir, M., Moradi-Afrapoli, F., Butterweck, V., Hamburger, M. (2016). Pharmacokinetics of dietary kaempferol and its metabolite 4-hydroxyphenylacetic acid in rats. Fitoterapia 115, 189–197. doi: 10.1016/J.FITOTE.2016.10.008
Zeng, Y., Nikitkova, A., Abdelsalam, H., Li, J., Xiao, J. (2019). Activity of quercetin and kaemferol against Streptococcus mutans biofilm. Arch. Oral. Biol. 98, 9–16. doi: 10.1016/J.ARCHORALBIO.2018.11.005
Zhang, W., Chen, Z. (2012). Polymer monolith microextraction coupled with HPLC for determination of jasmonates in wintersweet flowers. Analytical Letters. 46, 74–86. doi: 10.1080/00032719.2012.704535
Zhang, H., Feng, Q., Gong, J., Ma, J. (2018). Anticancer effects of isofraxidin against A549 human lung cancer cells via the EGFR signaling pathway. Mol. Med. Rep. 18, 407–414. doi: 10.3892/MMR.2018.8950
Zhang, X., Xu, M., Zhang, J., Wu, L., Liu, J., Si, J. (2017). Identification and evaluation of antioxidant components in the flowers of five Chimonanthus species. Ind. Crops Prod. 102, 164–172. doi: 10.1016/J.INDCROP.2017.03.014
Zhang, S., Zhang, H., Chen, L., Xia, W., Zhang, W. (2016). Phytochemical profiles and antioxidant activities of different varieties of Chimonanthus praecox. Ind. Crops Prod. 85, 11–21. doi: 10.1016/J.INDCROP.2016.02.009
Zhifen, W., Ni, Z., Xiaopan, M., Jin, Y., Yan, L., Weidong, P., et al. (2019). Chemical composition and in vitro antibacterial and antifungal activity of essential oils from different parts of Chimonanthus praecox (Linn.) link. Chin. J. Mod. Appl. Pharm. 36, 901–905. doi: 10.13748/j.cnki.issn1007-7693.2019.08.001
Keywords: Chimonanthus, Chimonanthus grammatus, MS Fragmenter, ChromGenius, isofraxidin, kaempferol, quercitrin
Citation: Hu H, Tekin V, Hu B, Yaghoobi M, Khan A, Ghosh AK, Panda SK, Huang H and Luyten W (2023) Metabolic profiling of Chimonanthus grammatus via UHPLC-HRMS-MS with computer-assisted structure elucidation and its antimicrobial activity. Front. Plant Sci. 14:1138913. doi: 10.3389/fpls.2023.1138913
Received: 06 January 2023; Accepted: 27 March 2023;
Published: 09 May 2023.
Edited by:
Chunnian He, Chinese Academy of Medical Sciences and Peking Union Medical College, ChinaReviewed by:
Min Xu, Kunming University of Science and Technology, ChinaShihong Luo, Shenyang Agricultural University, China
Dongfeng Yang, Zhejiang Sci-Tech University, China
Copyright © 2023 Hu, Tekin, Hu, Yaghoobi, Khan, Ghosh, Panda, Huang and Luyten. This is an open-access article distributed under the terms of the Creative Commons Attribution License (CC BY). The use, distribution or reproduction in other forums is permitted, provided the original author(s) and the copyright owner(s) are credited and that the original publication in this journal is cited, in accordance with accepted academic practice. No use, distribution or reproduction is permitted which does not comply with these terms.
*Correspondence: Haibo Hu, aGhiMjAxN0BnbXUuZWR1LmNu; Walter Luyten, d2FsdGVyLmx1eXRlbkBrdWxldXZlbi5iZQ==