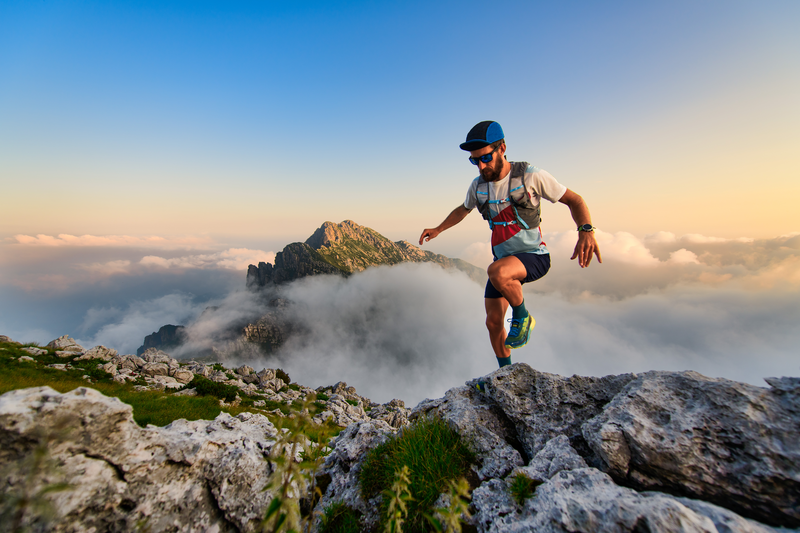
95% of researchers rate our articles as excellent or good
Learn more about the work of our research integrity team to safeguard the quality of each article we publish.
Find out more
ORIGINAL RESEARCH article
Front. Plant Sci. , 22 February 2023
Sec. Plant Nutrition
Volume 14 - 2023 | https://doi.org/10.3389/fpls.2023.1132414
This article is part of the Research Topic Plant Nutrient Use Efficiency in the Era of Climate Change View all 5 articles
Projected global climate change is a potential threat to nutrient utilization in agroecosystems. However, the combined effects of elevated [CO2] and canopy warming on plant nutrient concentrations and translocations are not well understood. Here we conducted an open-air field experiment to investigate the impact of factorial elevated [CO2] (up to 500 μmol mol-1) and canopy air warming (+2°C) on nutrient (N, P, and K) status during the wheat growing season in a winter wheat field. Compared to ambient conditions, soil nutrient status was generally unchanged under elevated [CO2] and canopy warming. In contrast, elevated [CO2] decreased K concentrations by 11.0% and 11.5% in plant shoot and root, respectively, but had no impact on N or P concentration. Canopy warming increased shoot N, P and K concentrations by 8.9%, 7.5% and 15.0%, but decreased root N, P, and K concentrations by 12.3%, 9.0% and 31.6%, respectively. Accordingly, canopy warming rather than elevated [CO2] increased respectively N, P and K transfer coefficients (defined as the ratio of nutrient concentrations in the shoot to root) by 22.2%, 27.9% and 84.3%, which illustrated that canopy warming played a more important role in nutrient translocation from belowground to aboveground than elevated [CO2]. These results suggested that the response of nutrient dynamics was more sensitive in plants than in soil under climate change.
Increasing atmospheric CO2 concentration ([CO2]) is a major driver of climate change. Carbon dioxide is projected to reach more than 500 ppm, which may raise global temperature by more than 2°C by the end of this century (IPCC, 2021). It has been widely reported that elevated [CO2] stimulates terrestrial plant growth through the [CO2] fertilization effect (Wang et al., 2019; Chen et al., 2022), and indirectly affects nutrient absorption and availability (Lam et al., 2012a; Wang et al., 2018a). It is generally accepted that crop yield was increased through enhancing leaf photosynthesis under elevated [CO2] (Zhang et al., 2013; Wang et al., 2020b), while warming reduced crop biomass due to increased phenological development and leaf respiration (Ruiz-Vera et al., 2013; Venugopalan et al., 2021). By contrast, the high isoflavone reductase-like gene expression mitigated the negative impacts of elevated temperature in wheat (Shokat et al., 2021). However, crop responses to factorial elevated [CO2] and warming have not been sufficiently addressed.
Chinese agroecosystems are vulnerable to global climate change. Wheat is one of the most widely planted staple agricultural food crops (Nagai and Makino, 2009), but its yield could be severely affected by elevated [CO2] and global warming (Ray et al., 2015; Wang et al., 2019). Soil nutrient dynamics and plant nutrient absorption are critical factors determining the impact of elevated [CO2] and warming on food productivity (Wheeler and von Braun, 2013; Wang et al., 2020a; Wei et al., 2021). Previous reviews concluded that elevated [CO2] increased the average grain yield of C3 grasses (wheat, rice, and barley) by about 19% (Kimball, 2016). However, the negative impact of warming can negate the positive effect of elevated [CO2] on crop productivity (Ruiz-Vera et al., 2013; Wang et al., 2019). Whereas, Shokat et al. (2021) reported that high isoflavone reductase-like gene expression promoted grain yield under the combined treatment of elevated [CO2] and heat stress in wheat. Furthermore, the negative impact of warming played an overwhelming role in plant nutrient utilization in comparison with elevated [CO2] (Wang et al., 2019). To date, it remains unclear whether the impact of elevated [CO2] offsets the effect of canopy warming on nutrient transfer and soil nutrient status.
Nitrogen (N), phosphorus (P), and potassium (K) are recognized as the most limiting factors affecting the crop physiological function and production. Elevated [CO2] reduced nutrient concentrations owing to the dilution effect of [CO2] or inhibited investment in Rubisco and nitrate (Bloom et al., 2010; Ainsworth and Long, 2021), but increased nutrient accumulation in plants (Wang et al., 2018a). Furthermore, a large-scale meta-analytic study showed that the nutrient (N, P, K) declined in foliar and grain tissues under elevated [CO2] (Loladze, 2014). Meanwhile, warming accelerated leaf transpiration and more nutrients were acquired for aboveground biomass (Wang et al., 2018b). As a consequence, more nutrients are translocated from soil to plants (Viciedo et al., 2021). Nutrient availability was not only determined by soil properties, but also regulated by elevated [CO2] and global warming (Wang et al., 2016; Osanai et al., 2017). It is likely that changes in plant physiological metabolism can alter the translocation and accumulation of nutrients, which could finally affect soil nutrient dynamics under future climate changes (Calleja-Cabrera et al., 2020). Loladze (2002) reported that elevated [CO2] led to a global imbalance of essential elements in plants, and could intensify malnutrition in human populations under future climate conditions. Ma et al. (2007) showed that elevated [CO2] decreased the availability of N and P in a FACE (free-air [CO2] enrichment) system, but the response of nutrient availability to elevated [CO2] varied among nutrients and growth stages. Some studies reported that the availability of P and ammonium ( -N) increased under elevated [CO2] or combined with warming (Bhattacharyya et al., 2014), although elevated [CO2] and warming decreased soil nutrient status in terrestrial ecosystems (Jauregui et al., 2015). So far, the factorial combination of elevated [CO2] and canopy warming on belowground processes and ecosystem functioning remains elusive.
This study was a factorial elevated [CO2] (to 500 ppm) and warming (by 2°C) experiment conducted in an open-field system. We hypothesize that both elevated [CO2] and warming would increase nutrient requirement, and thus stimulate nutrient translocation from belowground to aboveground, finally decreasing soil nutrient availability. The findings of this study provide insights into fertilizer management in cropland and strategies for sustainable production under future climates.
The experimental site was located in Jiangsu Province, China (31°30′N, 120°33′E). The soil is formed on clayey lacustrine deposits as Gleyic Stagnic Anthrosol. Initial soil analysis was 19.2 g organic C kg-1, 1.3 g total N kg-1, 0.9 g total P kg-1, 15.0 g total K kg-1and pH of 7.0. The soil at the experimental site is classified as loam with sand of 33.8%, silt of 38.6%, and clay of 27.6%. The experimental site belongs to a humid subtropical climate with an average annual temperature of 16 °C and annual precipitation of 1100-1200 mm (Wang et al., 2018b).
The facility operation followed the procedures described by Wang et al. (2019). The treatments were randomly arranged in three blocks, with each block having four treatments (rings). The area of each ring is 50 m2. The four treatments included ambient condition (CK), elevated [CO2] (500 ppm, CE), canopy warming (+2°C, WA) by infrared heaters, and combined treatment of elevated [CO2] and canopy warming (CW). An interval of 28 m was set up between rings to avoid any potential contamination across treatments.
Winter wheat (Triticum aestivum L.) of Yangmai No.14 was planted at a row spacing of 20 cm in November 2013 and harvested in May 2014. Based on local practice, the basal fertilizer in the form of urea (46% N) was applied at a rate of 187.5 kg ha-1, and top-dressed at a rate of 150 kg ha-1 at the elongation stage. The topdressing fertilizer was provided by a compound fertilizer (15 N: 15 P2O5: 15 K2O) at a rate of 375 kg ha-1 after the heading stage. The weed control and insecticide application were carried out according to local agronomic management. The wheat was cultivated under rain-fed conditions.
Plant and soil samples were taken at the elongation, heading, and ripening. Since climatic change treatment altered wheat development, soil and plant sampling was conducted based on the phenological stage. Wheat plants were randomly collected 1 m2 from each plot, plant sample was separated into shoot and root. Root samples were rinsed with water to get rid of the soil. Meanwhile, the shoot and root samples were rinsed and de-enzyme at 105°C for 0.5 h, and then oven-dried at 70°C for 48 h. All plant samples were ground to 0.25 mm. For soil samples, five soil cores (0-15 cm) were taken and then homogenized to form a mixed soil sample. After removing visible residues and stones, soil samples were passed through a 2 mm sieve and maintained at 4°C before analysis.
Soil available P was extracted with NaHCO3 and analyzed by a spectrophotometer (TU-1810, China). Soil available K concentrations were estimated using a flame photometer (FP6410, China) after extraction with 1 M ammonium acetate (NH4OAc). Soil -N and N concentrations were determined using a subsection flow analysis instrument (Skalar, Netherlands).
Plant shoot and root samples were pretreated with H2SO4-H2O2, and analyzed for N concentrations by the Kjeldahl digestion method. Phosphorus concentrations were determined by a spectrophotometer (TU-1810, China), and K concentrations by a flame photometer (FP6410, China).
Nutrient transfer coefficients were used to evaluate the impact of elevated [CO2] and canopy warming on the capacity of plant nutrient uptake (Wang et al., 2016). Nutrient transfer coefficients were estimated as:
general linear mixed model (GLM) was used to detect the [CO2], warming and growth stage (main factor), with blocks treated as a random variable. Accordingly, a three-way analysis of variance was used to test the differences between treatments and the growth stage. The probability level (P< 0.05) was considered to be statistically significant. Statistical analyses were conducted by SPSS v.22.0 (IBM Corp., Armonk, NY, USA).
When averaged across three growth stages, elevated [CO2] did not alter plant N or P concentrations (Figure 1), but reduced K concentrations by 11.0% (P = 0.001) in the shoot and 11.5% (P = 0.001) in the root (Figure 1 and Table 1). By contrast, canopy warming significantly increased shoot N, P, and K concentrations by 8.9% (P = 0.016), 7.5% (P = 0.054), and 15.0% (P< 0.001), but reduced root N and K concentrations by 12.3% (P< 0.01), and 31.6% (P< 0.001), respectively. However, plant N, P, and K concentrations varied among stages and reached the peak at the elongation stage. Significant interactions of [CO2] × warming and [CO2] × warming × stage were observed in the N and P concentrations: elevated [CO2] aggravated the positive effect of canopy warming on shoot N and P concentrations, especially at the elongation stage, but mitigated the adverse impact of canopy warming on root N and P concentrations at the heading stage.
Figure 1 Nitrogen (A), phosphorus (B), and potassium (C) Concentrations in plant shoots (upper) and roots (lower) under ambient condition (CK), elevated [CO2] alone (CE), canopy warming alone (WA) and combined treatment (CW). Different letters indicate significant differences between treatments in the same stage at P< 0.05.
Table 1 Summary of the GLMM analysis of nutrient concentrations in shoots and roots under simulated climate change conditions.
Elevated [CO2] and canopy warming had significant effects on nutrient transfer coefficients, and these effects varied with wheat growth stages (Table 2). When averaged across three growth stages, canopy warming significantly increased N, P, and K transfer coefficients by 22.2% (P = 0.001), 27.9% (P = 0.001), and 84.3% (P< 0.001), respectively. Elevated [CO2] enhanced P and K transfer coefficients by 27.9% (P = 0.058) and by 10.1% (P< 0.05), but did not affect the N transfer coefficient. A significant interaction of [CO2] × warming was detected for P (P< 0.001) and K (P< 0.01) transfer coefficients: the increases in P and K transfer coefficients by canopy warming were aggravated by elevated [CO2]. The impact of canopy warming appeared differently among stages as evidenced by significant warming × stage interaction.
Table 2 Nutrient transfer coefficients under ambient condition (CK), elevated [CO2] alone (CE), canopy warming alone (WA), and combined treatment (CW).
Elevated [CO2] and canopy warming did not alter soil nutrient status (Table 3), although elevated [CO2] slightly increased soil available P concentrations by 13.2% (P< 0.05). However, soil nutrient availability varied across stages with the peaking soil N concentrations occurring at the heading stage and the peaking P and K concentrations at the ripening stage. There was a significant interaction of [CO2] × warming × growing stages on soil K concentrations. A significant effect of elevated [CO2] × warming interaction was observed for soil N and available P concentrations: warming increased soil N and available P concentrations under ambient [CO2] but not under elevated [CO2].
Table 3 Soil nutrient status under ambient condition (CK), elevated [CO2] alone (CE), canopy warming alone (WA), and combined treatment (CW).
Elevated [CO2] alone decreased plant K concentrations, but the responses varied among growth stages (Table 1). The dilution effect in the K concentrations has been widely described in plants under elevated [CO2] (Han et al., 2015; Wang et al., 2019). Kanowski (2001) found that elevated [CO2] (790 ppm) reduced K concentrations in Flindersia. By contrast, elevated [CO2] did not alter the N and P concentrations for both shoot and root (Figure 1 and Table 1). This contrasts with other studies and indicates that elevated [CO2] is associated with the dilution of nutrient concentrations in wheat grain under sufficient fertilizer input (Lam et al., 2012b). Our previous study argued that elevated [CO2] did not affect plant nutrient concentrations under adequate fertilizer supply in the rice paddy field (Wang et al., 2018a). On the other hand, the reasons were ascribed to the levels of [CO2] elevation (500 ppm) in this study, which was much lower than in other studies (more than 550 ppm). Whereas, the P and K transfer coefficients were significantly increased by elevated [CO2], which was ascribed to an increase in nutrient demand by crop aboveground biomass (Wang et al., 2019). Indeed, we observed that elevated [CO2] significantly increased grain yield by 29.6% (Figure S1). Elevated [CO2] did not affect the N transfer coefficient, which is due to the inhibition of N assimilation or lower investment in Rubisco in the shoots of wheat (Bloom et al., 2010; Ainsworth and Long, 2021). These results indicated that the mechanisms for nutrient translocation from root to shoot varied with plant nutrient demands.
Canopy warming significantly increased nutrient concentrations in plant shoots (Figure 1 and Table 1). Warming-induced increase in plant N concentrations (29.8-32.7%) was also observed in a tallgrass prairie ecosystem (An et al., 2005). Trueman and Gonzalez-Meler (2005) have indicated that higher air temperature would increase the vapor pressure deficit of the canopy and leaf transpiration, thereby increasing nutrient translocation from root to shoot. Our previous study showed a significant increase in evapotranspiration under canopy warming conditions in this winter wheat field (Wang et al., 2018b). Moreover, we also found that canopy warming significantly reduced nutrient concentrations in roots and increased nutrient transfer coefficients (Tables 1, 2).
Our results observed that combined treatment of elevated [CO2] and canopy warming did not affect shoot nutrient concentrations (Figure 1). This is consistent with a previous study conducted by Cheng et al. (2010), who observed no significant change in rice N concentrations under concurrent elevated [CO2] (680 ppm) and high night temperature (+10°C). However, Jauregui et al. (2015) has shown that the interaction of elevated [CO2] (700 ppm) and temperature (+ 4°C) significantly decreased N and K concentrations, but did not affect the P concentrations in wheat leaf in a greenhouse study. In contrast, elevated [CO2] (550 ppm) and elevated air temperature (+ 2°C) significantly increased P uptake in rice in an open top chamber study (Bhattacharyya et al., 2014). The inconsistent results were attributed to differences in experimental designs and variations in crop cultivars, and the low statistical power of individual studies. Reich et al. (2016) found that temperature determined the response of plant N assimilation and mineral nutrient composition to elevated [CO2]. Similarly, our results found that canopy warming altered nutrient uptake response to elevated [CO2], with elevated [CO2] significantly increasing P and K transfer coefficients under canopy warming, but decreasing the P transfer coefficient under ambient temperature. Therefore, further studies are needed to reveal the mechanisms of plant nutrient assimilation under future concurrent elevated [CO2] and warming conditions.
As mentioned above, elevated [CO2] and canopy warming significantly altered nutrient uptake. However, opposite to our hypothesis, elevated [CO2] or canopy warming did not affect soil nutrient status (Table 3). Previous studies reported that elevated [CO2] did not change soil N or P availability in paddy fields (Ma et al., 2007; Cheng et al., 2016), although it is generally accepted that elevated [CO2] increased nutrient demand through increasing plant biomass (Lam et al., 2012b; Wang et al., 2018a). However, a recent study demonstrated that [CO2] fertilization increased N and P availability in a P-limited forest ecosystem (Hasegawa et al., 2016). The present study was not constrained by nutrients due to the frequent fertilizer applications, which suggests that soil nutrient availability can be replenished by fertilizer input in an intensively managed agricultural ecosystem under future climate scenarios.
Our results showed that canopy warming increased soil N and available P concentrations under ambient conditions (Table 3). Warming greatly affects soil microbial and enzyme activity, which stimulates soil nutrient availability (Liu et al., 2015; Osanai et al., 2015; Wang et al., 2016). Warming increased nutrient mineralization, which leads to the stimulation of nutrient availability and increases nutrient assimilation by plants (Zuccarini et al., 2020; Iversen et al., 2022). In contrast, canopy warming did not significantly alter soil nutrient availability under elevated [CO2] (Table 3). The acceleration of soil nutrient availability is counteracted by the plant demand and soil moisture, and was even reduced under warming. Elevated temperature decreased soil moisture, resulting in a limitation in soil nutrient mineralization under dry conditions (Borken and Matzner, 2009; Wang et al., 2016). Our results demonstrated that both canopy warming alone and combined with elevated [CO2] generally increased nutrient transfer coefficients (Figure 1 and Table 2). Therefore, the long-term climatic change probably increases soil nutrient consumption, which has a negative impact on food production. However, the responses of soil nutrient status to future concurrent elevated [CO2] and canopy warming are complicated, which warrants further studies for developing adaptation strategies to future climate change.
Our results demonstrated that simulated climate change has a significant influence on nutrient concentrations and transfer coefficients. Canopy warming rather than elevated [CO2] increased N, P, and K concentrations in plant shoots, but reduced these concentrations in plant roots. Canopy warming significantly increased nutrient transfer coefficients. A similar trend was observed for nutrient transfer coefficients under elevated [CO2], but to less extent than canopy warming. This study demonstrated an increase in nutrient consumption under climate change in winter wheat. Our findings provide major implications for plant and soil nutrient management as affected by future climate change.
The original contributions presented in the study are included in the article/Supplementary material. Further inquiries can be directed to the corresponding authors.
JW performed the laboratory work, analyzed the data and drafted the manuscript. XS revised and improved the draft. SL improved the draft. GP and LL contributed ideas to the study and carried out the experimental design. All authors contributed to the article and approved the submitted version.
The experimental facility was supported by the National Natural Science Foundation of China (No. 32271679, 31901165) and the Natural Science Foundation of Fujian Province (No. 2020J01186). We are grateful to the Special Fund for Agro-scientific Research in the Public Interest (No. 200903003). The authors declare no conflict of interest.
The authors declare that the research was conducted in the absence of any commercial or financial relationships that could be construed as a potential conflict of interest.
All claims expressed in this article are solely those of the authors and do not necessarily represent those of their affiliated organizations, or those of the publisher, the editors and the reviewers. Any product that may be evaluated in this article, or claim that may be made by its manufacturer, is not guaranteed or endorsed by the publisher.
The Supplementary Material for this article can be found online at: https://www.frontiersin.org/articles/10.3389/fpls.2023.1132414/full#supplementary-material
Ainsworth, E. A., Long, S. P. (2021). 30 years of free-air carbon dioxide enrichment (FACE): What have we learned about future crop productivity and its potential for adaptation? Global Change Biol. 27, 27–49. doi: 10.1111/gcb.15375
An, Y., Wan, S., Zhou, X., Subedar, A. A., Wallace, L. L., Luo, Y. (2005). Plant nitrogen concentration, use efficiency, and contents in a tallgrass prairie ecosystem under experimental warming. Global Change Biol. 11, 1733–1744. doi: 10.1111/j.1365-2486.2005.01030.x
Bhattacharyya, P., Roy, K. S., Dash, P. K., Neogi, S., Shahid, M., Nayak, A. K., et al. (2014). Effect of elevated carbon dioxide and temperature on phosphorus uptake in tropical flooded rice (Oryza sativa l.). Eur. J. Agron. 53, 28–37. doi: 10.1016/j.eja.2013.10.008
Bloom, A. J., Burger, M., Asensio, J. S. R., Cousins, A. B. (2010). Carbon dioxide enrichment inhibits nitrate assimilation in wheat and arabidopsis. Science 328, 899–903. doi: 10.1126/science.1186440
Borken, W., Matzner, E. (2009). Reappraisal of drying and wetting effects on c and n mineralization and fluxes in soils. Global Change Biol. 15, 808–824. doi: 10.1111/j.1365-2486.2008.01681.x
Calleja-Cabrera, J., Boter, M., Oñate-Sánchez, L., Pernas, M. (2020). Root growth adaptation to climate change in crops. Front. Plant Sci. 11, 544. doi: 10.3389/fpls.2020.00544
Chen, C., Riley, W. J., Prentice, I. C., Keenan, T. F. (2022). CO2 fertilization of terrestrial photosynthesis inferred from site to global scales. Proc. Natl. Acad. Sci. 119, e2115627119. doi: 10.1073/pnas.2115627119
Cheng, W., Sakai, H., Yagi, K., Hasegawa, T. (2010). Combined effects of elevated [CO2] and high night temperature on carbon assimilation, nitrogen absorption, and the allocations of c and n by rice (Oryza sativa l.). Agric. For. Meteorol. 150, 1174–1181. doi: 10.1016/j.agrformet.2010.05.001
Cheng, Y., Zhang, J., Zhu, J., Liu, G., Zhu, C., Wang, S. (2016). Ten years of elevated atmospheric CO2 doesn't alter soil nitrogen availability in a rice paddy. Soil Biol. Biochem. 98, 99–108. doi: 10.1016/j.soilbio.2016.04.003
Han, X., Hao, X., Lam, S. K., Wang, H., Li, Y., Wheeler, T., et al. (2015). Yield and nitrogen accumulation and partitioning in winter wheat under elevated CO2: A 3-year free-air CO2 enrichment experiment. Agricul. Ecosyst. Environ. 209, 132–137. doi: 10.1016/j.agee.2015.04.007
Hasegawa, S., Macdonald, C. A., Power, S. A. (2016). Elevated carbon dioxide increases soil nitrogen and phosphorus availability in a phosphorus-limited eucalyptus woodland. Global Change Biol. 22, 1628–1643. doi: 10.1111/gcb.13147
IPCC (2021). “Climate change 2021: The physical science basis,” in Contribution of working group I to the sixth assessment report of the intergovernmental panel on climate change. Eds. Masson-Delmotte, V., Zhai, P., Pirani, A., Connors, S. L., Péan, C., Berger, S., Caud, N., Chen, Y., Goldfarb, L., Gomis, M. I., Huang, M., Leitzell, K., Lonnoy, E., Matthews, J. B. R., Maycock, T. K., Waterfield, T., Yelekçi, O., Yu, R., Zhou, X. X. X. B. (Cambridge University Press. In Press).
Iversen, C. M., Latimer, J., Brice, D. J., Childs, J., Vander Stel, H. M., Defrenne, C. E., et al. (2022). Whole-ecosystem warming increases plant-available nitrogen and phosphorus in an ombrotrophic bog. Ecosystems, 26, 86–113. doi: 10.1007/s10021-022-00744-x
Jauregui, I., Aroca, R., Garnica, M., Zamarreño, ÁM, García-Mina, J. M., Serret, M. D., et al. (2015). Nitrogen assimilation and transpiration: Key processes conditioning responsiveness of wheat to elevated [CO2] and temperature. Physiol. Plantarum 155, 338–354. doi: 10.1111/ppl.12345
Kanowski, J. (2001). Effects of elevated CO2 on the foliar chemistry of seedlings of two rainforest trees from north-east Australia: Implications for folivorous marsupials. Austral Ecol. 26, 165–172. doi: 10.1046/j.1442-9993.2001.01103.x
Kimball, B. A. (2016). Crop responses to elevated CO2 and interactions with H2O, n, and temperature. Curr. Opin. Plant Biol. 31, 36–43. doi: 10.1016/j.pbi.2016.03.006
Lam, S. K., Chen, D., Norton, R., Armstrong, R. (2012a). The effect of elevated atmospheric carbon dioxide concentration on the contribution of residual legume and fertilizer nitrogen to a subsequent wheat crop. Plant Soil 364, 81–91. doi: 10.1007/s11104-012-1314-4
Lam, S. K., Han, X., Lin, E., Norton, R., Chen, D. (2012b). Does elevated atmospheric carbon dioxide concentration increase wheat nitrogen demand and recovery of nitrogen applied at stem elongation? Agricul. Ecosyst. Environ. 155, 142–146. doi: 10.1016/j.agee.2012.04.012
Liu, Y., Zhou, H., Wang, J., Liu, X., Cheng, K., Li, L., et al. (2015). Short-term response of nitrifier communities and potential nitrification activity to elevated CO2 and temperature interaction in a Chinese paddy field. Appl. Soil Ecol. 96, 88–98. doi: 10.1016/j.apsoil.2015.06.006
Loladze, I. (2002). Rising atmospheric CO2 and human nutrition: toward globally imbalanced plant stoichiometry? Trends Ecol. Evol. 17, 457–461. doi: 10.1016/S0169-5347(02)02587-9
Loladze, I. (2014). Hidden shift of the ionome of plants exposed to elevated CO2 depletes minerals at the base of human nutrition. Elife 3, e02245. doi: 10.7554/eLife.02245
Ma, H.-L., Zhu, J.-G., Liu, G., Xie, Z.-B., Wang, Y.-L., Yang, L.-X., et al. (2007). Availability of soil nitrogen and phosphorus in a typical rice–wheat rotation system under elevated atmospheric [CO2]. Field Crops Res. 100, 44–51. doi: 10.1016/j.fcr.2006.05.005
Nagai, T., Makino, A. (2009). Differences between rice and wheat in temperature responses of photosynthesis and plant growth. Plant Cell Physiol. 50, 744–755. doi: 10.1093/pcp/pcp029
Osanai, Y., Janes, J. K., Newton, P. C. D., Hovenden, M. J. (2015). Warming and elevated CO2 combine to increase microbial mineralisation of soil organic matter. Soil Biol. Biochem. 85, 110–118. doi: 10.1016/j.soilbio.2015.02.032
Osanai, Y., Tissue, D. T., Bange, M. P., Anderson, I. C., Braunack, M. V., Singh, B. K. (2017). Plant-soil interactions and nutrient availability determine the impact of elevated CO2 and temperature on cotton productivity. Plant Soil 410, 87–102. doi: 10.1007/s11104-016-2981-3
Ray, D. K., Gerber, J. S., MacDonald, G. K., West, P. C. (2015). Climate variation explains a third of global crop yield variability. Nat. Commun. 6, 5989. doi: 10.1038/ncomms6989
Reich, M., van den Meerakker, A. N., Parmar, S., Hawkesford, M. J., De Kok, L. J. (2016). Temperature determines size and direction of effects of elevated CO2 and nitrogen form on yield quantity and quality of Chinese cabbage. Plant Biol. 18, 63–75. doi: 10.1111/plb.12396
Ruiz-Vera, U. M., Siebers, M., Gray, S. B., Drag, D. W., Rosenthal, D. M., Kimball, B. A., et al. (2013). Global warming can negate the expected CO2 stimulation in photosynthesis and productivity for soybean grown in the Midwestern united states. Plant Physiol. 162, 410–423. doi: 10.1104/pp.112.211938
Shokat, S., Novak, O., Siroka, J., Singh, S., Gill, K. S., Roitsch, T., et al. (2021). Elevated CO2 modulates the effect of heat stress responses in triticum aestivum by differential expression of isoflavone reductase-like (IRL) gene. J. Exp. Bot. 72 (21), 7594–7609. doi: 10.1093/jxb/erab247
Trueman, R. J., Gonzalez-Meler, M. A. (2005). Accelerated belowground c cycling in a managed agriforest ecosystem exposed to elevated carbon dioxide concentrations. Global Change Biol. 11, 1258–1271. doi: 10.1111/j.1365-2486.2005.00984.x
Venugopalan, V. K., Nath, R., Sengupta, K., Nalia, A., Banerjee, S., Chandran, M. A. S., et al. (2021). The response of lentil (Lens culinaris medik.) to soil moisture and heat stress under different dates of sowing and foliar application of micronutrients. Front. Plant Sci. 12. doi: 10.3389/fpls.2021.679469
Viciedo, D. O., de Mello Prado, R., Martinez, C. A., Habermann, E., Branco, R. B. F., de Cássia Piccolo, M., et al. (2021). Water stress and warming impact nutrient use efficiency of Mombasa grass (Megathyrsus maximus) in tropical conditions. J. Agron. Crop Sci. 207, 128–138. doi: 10.1111/jac.12452
Wang, W., Cai, C., He, J., Gu, J., Zhu, G., Zhang, W., et al. (2020b). Yield, dry matter distribution and photosynthetic characteristics of rice under elevated CO2 and increased temperature conditions. Field Crops Res. 248, 107605. doi: 10.1016/j.fcr.2019.107605
Wang, J., Hasegawa, T., Li, L., Lam, S. K., Zhang, X., Liu, X., et al. (2019). Changes in grain protein and amino acids composition of wheat and rice under short-term increased [CO2] and temperature of canopy air in a paddy from East China. New Phytol. 222, 726–734. doi: 10.1111/nph.15661
Wang, J., Li, L., Lam, S. K., Liu, X., Pan, G. (2020a). Responses of wheat and rice grain mineral quality to elevated carbon dioxide and canopy warming. Field Crops Res. 249, 107753. doi: 10.1016/j.fcr.2020.107753
Wang, J., Li, L., Lam, S. K., Zhang, X., Liu, X., Pan, G. (2018a). Changes in nutrient uptake and utilization by rice under simulated climate change conditions: A 2-year experiment in a paddy field. Agric. For. Meteorol. 250–251, 202–208. doi: 10.1016/j.agrformet.2017.12.25
Wang, J., Liu, X., Cheng, K., Zhang, X., Li, L., Pan, G. (2018b). Winter wheat water requirement and utilization efficiency under simulated climate change conditions: A penman-monteith model evaluation. Agric. Water Manage. 197, 100–109. doi: 10.1016/j.agwat.2017.11.015
Wang, J., Zhang, X., Li, L., Cheng, K., Zheng, J., Zheng, J., et al. (2016). Changes in micronutrient availability and plant uptake under simulated climate change in winter wheat field. J. Soils Sediments 16, 2666–2675. doi: 10.1007/s11368-016-1464-8
Wei, L., Wang, W., Zhu, J., Wang, Z., Wang, J., Li, C., et al. (2021). Responses of rice qualitative characteristics to elevated carbon dioxide and higher temperature: implications for global nutrition. J. Sci. Food Agric. 101, 3854–3861. doi: 10.1002/jsfa.11021
Wheeler, T., von Braun, J. (2013). Climate change impacts on global food security. Science 341, 508–513. doi: 10.1126/science.1239402
Zhang, G., Sakai, H., Tokida, T., Usui, Y., Zhu, C., Nakamura, H., et al. (2013). The effects of free-air CO2 enrichment (FACE) on carbon and nitrogen accumulation in grains of rice (Oryza sativa l.). J. Exp. Bot. 64, 3179–3188. doi: 10.1093/jxb/ert154
Keywords: climate change, free-air CO2 enrichment (FACE), global warming, nutrient dynamic, winter wheat, Southeast China
Citation: Wang J, Li L, Lam SK, Shi X and Pan G (2023) Changes in plant nutrient status following combined elevated [CO2] and canopy warming in winter wheat. Front. Plant Sci. 14:1132414. doi: 10.3389/fpls.2023.1132414
Received: 27 December 2022; Accepted: 08 February 2023;
Published: 22 February 2023.
Edited by:
Asif Naeem, Nuclear Institute for Agriculture and Biology, PakistanReviewed by:
Sajid Shokat, Nuclear Institute for Agriculture and Biology (NIAB), PakistanCopyright © 2023 Wang, Li, Lam, Shi and Pan. This is an open-access article distributed under the terms of the Creative Commons Attribution License (CC BY). The use, distribution or reproduction in other forums is permitted, provided the original author(s) and the copyright owner(s) are credited and that the original publication in this journal is cited, in accordance with accepted academic practice. No use, distribution or reproduction is permitted which does not comply with these terms.
*Correspondence: Jianqing Wang, amlhbnFpbmd3YW5nQGFsaXl1bi5jb20=; Xiuzhen Shi, c2h4emg4N0Bob3RtYWlsLmNvbQ==
Disclaimer: All claims expressed in this article are solely those of the authors and do not necessarily represent those of their affiliated organizations, or those of the publisher, the editors and the reviewers. Any product that may be evaluated in this article or claim that may be made by its manufacturer is not guaranteed or endorsed by the publisher.
Research integrity at Frontiers
Learn more about the work of our research integrity team to safeguard the quality of each article we publish.