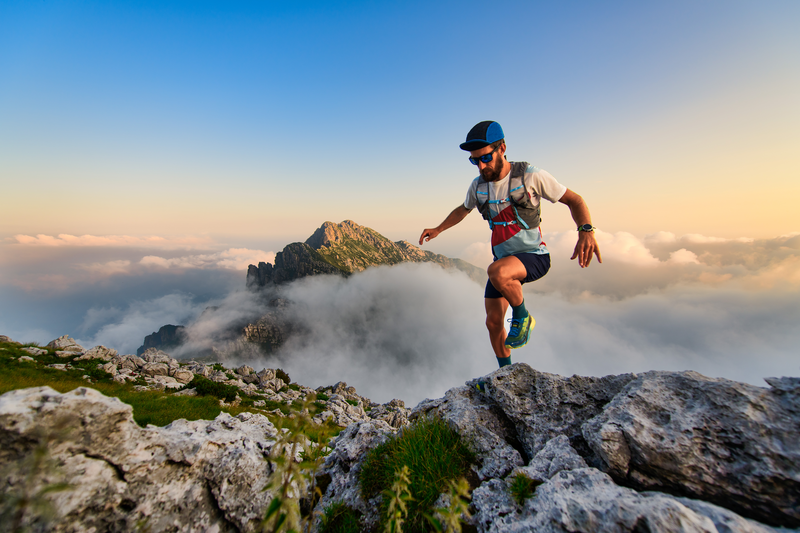
94% of researchers rate our articles as excellent or good
Learn more about the work of our research integrity team to safeguard the quality of each article we publish.
Find out more
ORIGINAL RESEARCH article
Front. Plant Sci. , 09 March 2023
Sec. Plant Breeding
Volume 14 - 2023 | https://doi.org/10.3389/fpls.2023.1126606
This article is part of the Research Topic From Classical Breeding to Modern Biotechnological Advancement in Horticultural Crops - Trait Improvement and Stress Resilience View all 12 articles
Cadmium (Cd) as a potentially toxic heavy metal that not only pollutes the environment but also interferes with plant growth. Nitric oxide (NO) regulates plant growth and development as well as abiotic stress response. However, the mechanism underpinning NO-induced adventitious root development under Cd stress remains unclear. In this study, cucumber (Cucumis sativus ‘Xinchun No. 4’) was used as the experimental material to investigate the effect of NO on the development of adventitious roots in cucumber under Cd stress. Our results revealed that, as compared to Cd stress, 10 μM SNP (a NO donor) could considerably increase the number and length of adventitious roots by 127.9% and 289.3%, respectively. Simultaneously, exogenous SNP significantly increased the level of endogenous NO in cucumber explants under Cd stress. Our results revealed that supplementation of Cd with SNP significantly increased endogenous NO content by 65.6% compared with Cd treatment at 48 h. Furthermore, our study indicated that SNP treatment could improve the antioxidant capacity of cucumber explants under Cd stress by up-regulating the gene expression level of antioxidant enzymes, as well as reducing the levels of malondialdehyde (MDA), hydrogen peroxide (H2O2) and superoxide anion () to alleviate oxidative damage and membrane lipid peroxidation. Application of NO resulted in a decrease of the , MDA, and H2O2 level by 39.6%, 31.4% and 60.8% as compared to Cd-alone treatment, respectively. Besides that, SNP treatment significantly increased the expression level of related genes involved in glycolysis processes and polyamine homeostasis. However, application of NO scavenger 2-(4-carboxy -2-phenyl)-4, 4, 5, 5-tetramethy limidazoline -1-oxyl -3-oxide (cPTIO) and the inhibitor tungstate significantly reversed the positive role of NO in promoting the adventitious root formation under Cd stress. These results suggest that exogenous NO can increase the level of endogenous NO, improve antioxidation ability, promote glycolysis pathway and polyamine homeostasis to enhance the occurrence of adventitious roots in cucumber under Cd stress. In summary, NO can effectively alleviate the damage of Cd stress and significantly promote the development of adventitious root of cucumber under Cd stress.
Cadmium (Cd), a widely spread heavy metal, is easily absorbed by plant roots, thus enters the food chain, and eventually poses a substantial threat to human health (Falco et al., 2005; Ahmad et al., 2016a; Ahmad et al., 2016b). It has been discovered that Cd, as a non-essential element for plant growth and development, disturbs nutrient and water uptake/transport (Rivelli et al., 2014; Hafsi et al., 2022). Moreover, Cd induces a number of stress responses in plants including ion balance changes (Kucerova et al., 2020; Hafsi et al., 2022), change in antioxidant enzymes activities (Guo et al., 2019), photosynthesis inhibition (Rizwan et al., 2018), and changes in the expression of related genes and proteins (Muhammad et al., 2019; Manara et al., 2020). Plant response to abiotic stress is usually accompanied by an increase in the level of reactive oxygen species (ROS) (Polle and Schützendübel, 2003; Kohli et al., 2019; Mansoor et al., 2022), and the increase in ROS content caused the destruction of cell structure and function (Panyuta et al., 2016; Kohli et al., 2019; Mansoor et al., 2022). Previous research have demonstrated that Cd stress could trigger the ROS generation, such as hydrogen peroxide (H2O2) and superoxide radical () accumulation in plants (Qi et al., 2021; Li et al., 2022). Some studies have also shown that excessive accumulation of ROS under Cd stress can trigger protein post-translational modification (Gzyl et al., 2015), enzyme inactivation and denaturation, DNA and RNA damage, resulting in cell damage and cell death (Singh et al., 2016). These series of reactions may aggravate the degree of lipid peroxidation (Heyno et al., 2008), disrupts metabolic activities and eventually affect plant growth and development (Yu et al., 2015; Anwar et al., 2021; El Rasafi et al., 2022). It has been demonstrated that plants have a series of antioxidant defense system to mitigate the oxidative damage caused by ROS (Gill and Tuteja, 2010). Antioxidant enzymes such as ascorbate peroxidase (APX), superoxide dismutase (SOD), catalase (CAT) or glutathione reductase (GR) have been demonstrated to regulate accumulation of ROS and protect plants from oxidative damage under Cd stress (Irfan et al., 2014; Guo et al., 2019). Therefore, the possibility of oxidative signal or oxidative damage depends on the balance between antioxidant enzyme activity and ROS level (Møller et al., 2007).
Nitric oxide (NO) has been implicated as an essential signaling molecule in plants. Numerous studies have discovered that NO plays an essential role in the regulation of plant growth and development including seed germination (Ren et al., 2020), root growth and development (Pagnussat et al., 2002; Sun et al., 2019; Liu et al., 2022a), pollen tube germination (Prado et al., 2004) and fruit senescence (Zuccarelli et al., 2021). The increasing evidence indicates that, NO function in plant stress response. As a multi-functional regulator, NO signaling is involved in a range of abiotic stress responses to mitigate oxidative damage caused by abiotic stress (Parankusam et al., 2017; Gao et al., 2022; Xia et al., 2022). For example, exogenous NO could stabilize the cell membranes in hulless barley under drought stress (Gan et al., 2015). Moreover, application of NO could upregulate the gene expression of antioxidative enzymes to enhance the antioxidant capacity under Cd stress (Chen et al., 2010).Thus, the protective roles of NO in alleviating oxidative injury have focused on regulating antioxidant systems, reducing the generation of ROS, mediating related gene expression, and maintaining protein stability, eventually enhancing plant stress tolerance (Terrón-Camero et al., 2019; Wei et al., 2020).
Cucumber is a member of the Cucurbitaceae family (Hashem et al., 2018). As one of the most popular vegetables, cucumber is shallow-rooted crop and is used to be an bioindicator species to assess toxicity of soils polluted by Cd (An et al., 2004). As mentioned above, NO plays an essential role in regulating plant growth and development. Moreover, it has been shown that NO is involved in the response to Cd stress. However, the mechanism underpinning NO-induced adventitious root development in cucumber under Cd stress remains unclear. The aim of this study was to investigate the role of NO in promoting the development of adventitious root in cucumber under Cd stress. Therefore, we conduct this experiment to test the effect of NO on root development, oxidative defence, glycolysis and polyamine metabolism in cucumber under Cd stress. The objective of this study was to provide evidence to elucidate the potential mechanism of NO signaling in responses to Cd stress in plants.
Cucumis sativus L. (‘Xinchun No. 4’) was used in this experiment. The sterilized cucumber seeds were pre-soaked in distilled water for 5 hours. The seeds were germinated on filter paper in petri dishes and then incubated in a climate box at 25°C with a 14 h photoperiod (200 μmols-1m-2). The experiment was repeated three times, with 10 seedlings per replicate.
Experiment 1: Sodium nitroprusside (SNP, purity≥98.5%, Solarbio, China) as a NO donor. Cucumber explants were placed in petri dishes containing distilled water or different concentrations of SNP (0, 1, 10, 100, 500 μM) under Cd stress for 5 days. The concentrations of NO was selected based on the results of our previous studies (Niu et al., 2017; Niu et al., 2019). These media were changed every day in order to keep the solution fresh.
Experiment 2: 200 μM 2-(4-carboxy-2-phenyl)-4, 4, 5, 5-tetramethylimidazoline -1-oxyl-3-oxide (c-PTIO, purity≥98%, Sigma, USA) as NO scavenger, 200 μM tungstate (Solarbio, China) as a NO inhibitor. The concentrations of CdCl2, NO scavenger or inhibitor were based on the results of a preliminary experiment.
The NO content was determined using the Greiss reagent method with minor modifications (Xuan et al., 2012). Cucumber explants were ground and mixed with 4 mL of 50 mM ice cold acetic acid buffer (containing 4% zinc diacetate). The mixture was centrifuged at 10000 g for 15 min at 4°C, and the supernatant was collected. Then, 0.1 g of charcoal was added. After vortex and filtration, the filtrate was mixed with 1mL Greiss reagent at room temperature for 30 min. Finally, the absorbance was assayed at 540 nm.
For measuring MDA, 0.2 g of samples were ground in ice bath and extracted with 5 mL trichloroacetic acid (TCA). The homogenate was transferred to a centrifuge tube and centrifuged at 4°C at 12000 g for 15 min. The supernatant was added to 0.5% TBA solution. The mixture is heated in a boiling water bath for 30 min and then centrifuged for 10 min. The absorbance of the supernatant was measured at 450 nm, 532 nm and 600 nm (Liu et al., 2022b). For estimating generation, the samples were homogenized with potassium phosphate buffer (pH 7.8) and centrifuged for 10 min. The supernatant was added to hydroxylamine hydrochloride and reacted at 25°C for 20 min. Finally, the absorbance was measured at 530 nm (Gong et al., 2014). Superoxide accumulation was also examined by nitroblue tetrazolium (NBT) staining, as described previously (Wang et al., 2019). H2O2 content in cucumber explants was determined as described by the method with minor modification (Liao et al., 2011). 0.5 g of cucumber explants were ground in liquid nitrogen and then homogenized in 3 mL ice-cold aceton. After centrifugation at 10000 g for 10 min at 4°C, the reaction mixture composed of 0.5 mL of the supernatant, 0.5 mL of trichloromethane (CHCl3), 1.5 mL carbon tetrachloride (CCl4) and 2.5 mL of distilled water. The mixture was then centrifuged at 1000 g for 1 min and the supernatant fractions were collected for H2O2 determination. In addition, H2O2 was detected with the DAB method with some modifications. Briefly, leaves were placed in the diaminobenzidine (DAB) staining solution. After then, the treated leaves were placed in 95% ethanol for 10 min. The reaction of DAB with H2O2 could produce the deep brown polymerization product (Yang et al., 2013).
In order to investigate the effect of NO on antioxidant system, glycolysis and polyamine pathway during adventitious rooting under Cd stress. The relative expression of genes encoding for antioxidant enzymes, glycolysis pathway and polyamine biosynthetic enzymes were measured. Cucumber explants were ground into powder with liquid nitrogen. Total RNA was extracted using the DP419 kit (TianGen, Beijing, China). Quantitative real-time PCR reactions were performed using SYBR Green SuperReal PreMix Plus kit (TianGen, Beijing, China) according to the cycling parameters: 95°C for 15 min; 95°C for 10 s and 60°C for 32 s, 40 cycles. qRT-PCR amplification primers are shown in Table 1. The relative expression of the gene was calculated by the 2 -ΔΔCT method.
Three independent replicates were set for each experiment. Means were separated by Duncan test at 0.05 probability level. Analysis of variance (ANOVA) was done. SPSS V. 13.0 was used for statistical analysis.
To understand the effect of exogenous NO on the development of adventitious root under Cd stress, we performed a dose-response experiment with NO. As shown in Figure 1, compared to CK treatment, CdCl2 treatment significantly reduced root number and root length by 66.3% and 81.7%, respectively. Moreover, the development of adventitious roots altered considerably with increasing concentrations of NO donor, SNP (1, 10, 100, 500 μM). As shown in Figure 1, the root number and root length of 10 μM NO treatment was significantly increased by 127.9% and 289.3%, respectively, as compared to CdCl2 treatments. However, a high concentration of NO (500 μM) obviously decreased the number and length of adventitious roots under Cd stress (Figures 1A, B). Therefore, exogenous NO displayed a concentration-dependent influence on adventitious rooting under Cd stress, and these results indicated that 10 μM NO significantly ameliorated the adverse effect of Cd stress on the development of adventitious roots.
Figure 1 Effect of NO on adventitious root formation under Cd stress. The primary roots were removed from 5-day-old seedlings. Explants were then incubated for 5 days with distilled water (CK) or 1 μM CdCl2, 1 μM CdCl2 +1 μM SNP, 1 μM CdCl2 + 10 μM SNP, 1 μM CdCl2 + 100 μM SNP, 1 μM CdCl2 + 500 μM SNP. Ten explants were used per replicate. The numbers (A) and root length (B) of adventitious roots were expressed as mean ± SE (n = 3). Photographs (C) were taken after five days of the treatments indicated. Bars with different letters are significantly different at P < 0.05 according to Duncan’s multiple range test.
To further investigate the key role of NO in affecting adventitious root formation in cucumber under Cd stress, NO scavengers or inhibitor was utilized in this experiment. As shown in Figure 2, NO treatment obviously induced the adventitious rooting under Cd stress. However, application of cPTIO or tungstate significantly inhibited the NO-promoted adventitious rooting under Cd stress. The number of adventitious roots which treated with NO scavenger or inhibitor decreased by 73% and 67.6%, respectively, when compared to CdCl2 + NO treatment (Figure 2A). Meanwhile, the adventitious root length of explants treated with cPTIO or tungstate reduced by 68.9% and 44.3%, respectively, as compared to that of NO treatment (Figure 2B). These results implied that NO might be responsible for promoting the formation of adventitious root under Cd stress.
Figure 2 Effect of cPTIO or tungstate on adventitious root formation under Cd stress. The primary roots were removed from 5-day-old seedlings. Explants were then incubated for 5 days with distilled water (CK) or 1 μM CdCl2, 1 μM CdCl2 +10 μM SNP, 1 μM CdCl2 + 10 μM SNP + 200 μM cPTIO, 1 μM CdCl2 + 10 μM SNP + 200 μM tungstate. Ten explants were used per replicate. The numbers (A) and root length (B) of adventitious roots were expressed as mean ± SE (n = 3). Photographs (C) were taken after five days of the treatments indicated. Bars with different letters are significantly different at P < 0.05 according to Duncan’s multiple range test.
In order to further validate the influence of NO on adventitious root production under Cd stress, endogenous NO level was detected during NO-induced adventitious rooting under Cd stress condition (Figure 3). The concentration of endogenous NO in CdCl2 treatment gradually decreased during the process of adventitious root development (Figure 3). However, the level of endogenous NO which treated with CdCl2+NO was considerably higher than those in Cd group, reaching a maximum at 48 h. As shown in Figure 3, at 48 h, exogenous NO treatment significantly enhanced endogenous NO level by 65.6% as compared to CdCl2. However, cPTIO and tungstate treatments obviously decreased endogenous NO level during adventitious root development, which was 65.3% and 55.5% lower than that of NO treatment, respectively (Figure 3).
Figure 3 Changes in the endogenous NO level during NO-induced adventitious root formation under Cd stress. The primary roots were removed from 5-day-old seedlings. Explants were then incubated for 72 h with distilled water (CK) or 1 μM CdCl2, 1 μM CdCl2 +10 μM SNP, 1 μM CdCl2 + 10 μM SNP + 200 μM cPTIO, 1 μM CdCl2 + 10 μM SNP + 200 μM tungstate. Bars with different letters are significantly different at P < 0.05 according to Duncan’s multiple range test.
The levels of MDA, H2O2 and in cucumber explants under Cd stress were measured in our experiment (Figure 4). As shown in Figure 4A, CdCl2 treatment significantly increased MDA content in cucumber explants which compared to that of control. However, exogenous NO significantly decreased MDA level under Cd stress. As compared to Cd stress, the MDA content which treated with NO treatment significantly decreased by 31.4% (Figure 4A). However, cPTIO or tungstate treatment significantly elevated the content of MDA compared to CdCl2 + NO treatment. Also, CdCl2 treatment significantly increased the content of in cucumber explants. Exogenous NO could reverse the increase in level which caused by Cd stress (Figures 4B, D). As shown in Figure 4B, application of NO obviously decreased the level by 39.6% when compared to CdCl2 treatment. However, the content of in cucumber explants which treated with cPTIO or tungstate was significantly higher than that of CdCl2 + NO treatment. Furthermore, the effect of NO treatment on H2O2 level followed the same pattern as the effect on level (Figures 4C, E). These results indicated that NO could obviously alleviate membrane lipid peroxidation and inhibit the accumulation of ROS, thus reducing oxidative damage and promoting the formation of adventitious root in cucumber under Cd stress.
Figure 4 Effect of NO on MDA content (A), content (B) and H2O2 content (C) during adventitious root development in cucumber under Cd stress. Explants were incubated for 48 h with distilled water (CK) or 1 μM CdCl2, 1 μM CdCl2 +10 μM SNP, 1 μM CdCl2 + 10 μM SNP + 200 μM cPTIO, 1 μM CdCl2 + 10 μM SNP + 200 μM tungstate. Photograph showing NBT (D) and DAB (E) staining after 48 h of treatments. Bars with different letters are significantly different at P < 0.05 according to Duncan’s multiple range test.
We further explored the effect of NO on the antioxidant system during adventitious root development under Cd stress. As shown in Figure 5, CdCl2 treatment has a significant effect on the expression level of antioxidant enzymes during the process of adventitious root formation. Compared to CK treatment, Cd treatment significantly decreased the expression level of ascorbate peroxidase (APX), Cu, Zn-superoxide dismutase (Cu, Zn-SOD), glutathione reductase (GR) and peroxidase (POD) (Figure 5). However, the expression of these genes in NO treatment was significantly higher than that of Cd stress alone (Figure 5). As shown in Figure 5A, exogenous NO significantly increased APX relative expression by 90.8% compared with Cd treatment alone. Meanwhile, Zn/Cu-SOD, CAT, GR and POD relative expression of CdCl2 + NO treatment was significantly higher 63.3%, 31.0%, 43.0% and 40.9% than those of Cd treatment, respectively (Figure 5). Nevertheless, NO scavengers or inhibitor obviously down-regulated the transcriptional levels of the antioxidant enzymes compared to those of NO treatment under Cd stress (Figure 5). Thus, these results might give an exploration of the positive role of NO in hindering ROS production by regulating the transcriptional levels of antioxidant enzymes.
Figure 5 Effect of NO on the expression level of APX (A), Zn/Cu-SOD (B), CAT (C), GR (D) and POD (E) in cucumber explants under Cd stress at 48 (h) Explants were incubated for 2 days with distilled water (CK) or 1 μM CdCl2, 1 μM CdCl2 +10 μM SNP, 1 μM CdCl2 + 10 μM SNP + 200 μM cPTIO, 1 μM CdCl2 + 10 μM SNP + 200 μM Tungstate. The values (means ± SE) are the average of three independent experiments. Bars with different letters are significantly different at P < 0.05 according to Duncan’s multiple range test.
We evaluated the effect of NO on glycolysis pathway during the development of adventitious root in cucumber under Cd stress. As shown in Figure 6, compared with CK treatment, CdCl2 treatment significantly down-regulated the gene expression levels of FK, PK and HK. However, compared to those of Cd treatment, exogenous NO significantly enhanced the expression level of glycolysis-related genes. As shown in Figures 6A, B, exogenous NO resulted in a 83.8% increase in PFK relative expression and a 87.1% increase in FK relative expression compared with Cd treatment alone, respectively. Moreover, Cd treatment decreased PK relative expression by 12.4% and caused a 52.7% decrease in HK relative expression compared with NO + Cd treatment, respectively (Figures 6C, D). On the contrary, NO scavengers or inhibitor obviously reversed the positive effect of NO on regulating the mRNA transcription level of these genes (Figure 6). Therefore, NO promoted adventitious rooting under Cd stress through regulating glycolysis-related gene expression.
Figure 6 Effect of NO on the expression level of PFK (A), FK (B), PK (C) and HK (D) in cucumber explants under Cd stress at 48 (h) Explants were incubated for 2 days with distilled water (CK) or 1 μM CdCl2, 1 μM CdCl2 +10 μM SNP, 1 μM CdCl2 + 10 μM SNP + 200 μM cPTIO, 1 μM CdCl2 + 10 μM SNP + 200 μM Tungstate. The values (means ± SE) are the average of three independent experiments. Bars with different letters are significantly different at P < 0.05 according to Duncan’s multiple range test.
Cd stress significantly decreased the expression level of arginine decarboxylase (ADC) and ornithine decarboxylase (ODC) in our experiment (Figures 7A, B). As shown in Figure 7, compared to CdCl2 treatment, the expression level of ADC and ODC in CdCl2 + NO treatment were significantly higher than those of CdCl2 treatment alone. Moreover, CdCl2 + NO treatment resulted in a 32.2% decrease in PAO relative expression compared with Cd treatment. However, NO scavenger or inhibitor treatment could reverse the effect of NO on the expression level of polyamine enzymes (Figure 7). These results imply that application of NO could regulate polyamine homeostasis during adventitious root development in response to Cd stress.
Figure 7 Effect of NO on the expression level of ADC (A), ODC (B) and PAO (C) in cucumber explants under Cd stress at 48 (h) Explants were incubated for 2 days with distilled water (CK) or 1 μM CdCl2, 1 μM CdCl2 +10 μM SNP, 1 μM CdCl2 + 10 μM SNP + 200 μM cPTIO, 1 μM CdCl2 + 10 μM SNP + 200 μM Tungstate. The values (means ± SE) are the average of three independent experiments. Bars with different letters are significantly different at P < 0.05 according to Duncan’s multiple range test.
Cadmium stress as an environmental factor has a significant impact on the growth and development of plants. It has been confirmed that Cd stress is an important limiting factor for plant growth and development, which inhibits the growth of plants to a certain extent (Azevedo et al., 2012; El Rasafi et al., 2022). As an important signal molecule in plants, NO is involved in a variety of abiotic stress response in plants. In this experiment, we demonstrated that NO could promote the development of adventitious roots of cucumber under Cd stress. In our study, the root number and root length of adventitious roots under Cd stress condition were significantly lower than those of the control (Figure 1). These results showed that Cd stress could significantly inhibit the process of adventitious root in cucumber explants. Previous studies found that Cd stress inhibited the adventitious root formation in plants (Li et al., 2019; Gong et al., 2022). However, suitable concentration of NO treatment significantly promoted adventitious root formation under Cd stress (Figure 1). Previous studies have shown that NO can resist abiotic stress through protecting cell membrane stability, up-regulating antioxidant enzyme activity and inducing resistance-related gene expression (Fan et al., 2015; Kaya et al., 2015). For example, it has been reported that NO could alleviate Cd toxicity through maintaining the growth regulation and nutritional status in cauliflower (Ma et al., 2022). Also, Zhao et al (2022) found that NO enhanced Cd resistance of Pleurotus eryngii through overcoming oxidative damage and regulating short-chain dehydrogenase/reductase famliy. Our results implied that suitable concentration of NO might alleviate the negative effect of Cd stress on the adventitious rooting of cucumber. Moreover, several studies indicated that NO could regulate the growth and development of plant roots, including root elongation, lateral root growth and adventitious root formation (Díaz et al., 2021; Wang et al., 2021; Liu Y.Y. et al., 2022). In our experiment, low concentration of NO significantly alleviated the inhibitory effect of Cd stress on the formation of adventitious root, while high concentration of NO obviously inhibited the occurrence of adventitious root under Cd stress (Figure 1). These results showed that NO had a concentration-dependent effect on adventitious root formation under Cd stress. In addition, NO scavengers or inhibitor dramatically reduced the root number and root length of cucumber explants (Figure 2), implying that NO plays a vital role in the development of adventitious roots under Cd stress.
Several reports suggested that the endogenous NO accumulation has been implicated as being responsible for the development of adventitious root in plants (Kang et al., 2018; Altamura et al., 2023). Compared to CK treatment, Cd stress significantly decreased endogenous NO levels during adventitious root development (Figure 3), implying that Cd stress might lead to a significant reduction of adventitious root formation through inhibiting endogenous NO production. However, exogenous NO significantly increased the endogenous NO production during adventitious root formation under Cd stress (Figure 3). Our results are in agreement with previous data on the implication of NO generation during root growth and development under stress conditions. For example, Zhang et al. (2022) suggested that endogenous NO was required for melatonin to stimulate the lateral roots growth of cucumber seedlings under nitrate stress. Moreover, Li et al., (2019) reported that NO significantly elevated endogenous NO level during the adventitious root formation in mung bean hypocotyl under cadmium and osmotic stresses. These observations support the view that NO could promote the formation of adventitious root through enhancing the endogenous NO production under Cd stress.
Excessive ROS results in membrane lipid peroxidation and cell oxidation, causing serious damage to plants (Huang et al., 2019). Meanwhile, MDA level is considered to be an indicator of lipid peroxidation during response to various environmental stresses (Gaweł et al., 2004). Previous study has suggested that Cd treatment caused oxidative stress (He et al., 2014) by increasing the contents of H2O2 and MDA of rice seedlings. Moreover, under Cd stress, H2O2 and MDA in wheat plants significantly increased (Kaya et al., 2019). Similarly, in our study, the results showed that CdCl2 treatment significantly increased the ROS level and MDA content of cucumber explants during adventitious root formation (Figure 4), resulting in oxidative damage (Jaleel et al., 2007), eventually inhibiting adventitious root formation. However, exogenous NO significantly decreased the levels of MDA, H2O2 and in cucumber explants to further alleviate the oxidative damage and membrane lipid peroxidation of cucumber explants under Cd stress (Laspina et al., 2005; Jaleel et al., 2007). Previous studies have shown that NO plays a key role in alleviating oxidative stress under Cd stress (Panda et al., 2011; Kaya et al., 2020). For instance, exogenously applied NO significantly reduced oxidative stress and proline content of wheat seedlings under Cd stress (Kaya et al., 2020). Similar to our results, application of NO resulted in an obviously decrease of , H2O2 and MDA content to decrease the Cd stress of cauliflower (Ma et al., 2022). These results suggested that NO is involved in ameliorating oxidative impairment under Cd stress. However, the treatment of NO scavengers or inhibitor significantly reversed the positive effect of NO on alleviating the oxidative damage during adventitious root formation under Cd stress (Figure 4). These results indicated that NO could significantly reduce the degree of membrane lipid peroxidation and alleviate oxidative stress under Cd stress, thus promoting the occurrence of adventitious roots under Cd stress.
Overproduction of ROS caused oxidative damage, plants need to counteract the toxicity of ROS through a highly efficient antioxidative defense system (Dumanović et al., 2021). At present, plants have effective antioxidant defense mechanisms to alleviate the effects of oxidative stress in plants. Xu et al. (Xu et al., 2008) found that transgenic Arabidopsis plants structurally overexpressed peroxisome gene HvAPX1, which reduced ROS accumulation and significantly improved the tolerance of Arabidopsis plants to Cd stress. In addition, Cd stress mediates the transcriptional expression of APX, GR, Cu/ZnSOD and other related antioxidant enzyme genes in ryegrass, which effectively alleviates the oxidative damage (Luo et al., 2011). Our results showed that Cd stress significantly affected the expression level of antioxidant enzyme genes (Figure 5). Moreover, compared to Cd treatment, exogenous NO significantly up-regulated the gene expression levels of these antioxidant enzymes, indicating that NO could alleviate oxidative damage of cucumber explants through enhancing the antioxidant system as well as eliminating excess ROS and MDA (Mostofa et al., 2019; Terrón-Camero et al., 2019). It has been investigated that NO significantly increased the activities of antioxidant enzymes of wheat under Cd stress (Kaya et al., 2019). Moreover, NO have the ability to enhance the antioxidant activities in bamboo plants under Cd stress (Emamverdian et al., 2021). Our present investigation suggested that NO could obviously enhance the plant defense system during adventitious rooting in the response to Cd stress.
The glycolysis process is the basis for controlling carbohydrate metabolism, which also considered to be one of the key pathways for plant respiration (Plaxton, 1996; Sun et al., 2021). In addition, glycolysis has been demonstrated to be involved in the plant response to abiotic stress (Zhang et al., 2011a; Dong et al., 2020; Sun et al., 2021). For example, it has been reported that the increase of PFK and PK activity could enhance the tolerance to salt stress(Zhong et al., 2016). Moreover, the enhancement of the expression level of PFK, PK and PEPC of cucumber leaves allows to convert more carbohydrates and maintain the normal physiological metabolism of cucumber (Zhong et al., 2016). Previous study found that Cd stress caused changes in carbohydrate metabolism, glycolysis and pentose phosphate pathway-related enzymes in pea (Devi et al., 2007). Moreover, Shahid et al (2019) found that Cd treatment significantly inhibited the activities of FK, HK, PFK and PK in potato plants. In our study, we found that Cd stress significantly down-regulated the gene expression levels of key glycolysis enzymes during adventitious root formation (Figure 6), indicating that Cd stress may lead to the inhibition of glycolysis pathway and further affect respiratory pathway during the adventitious rooting. However, under Cd stress, NO treatment could significantly up-regulate the gene expression levels of PFK, PK, FK and HK (Figure 6). In agreement with the present study, NO could obviously elevate the activities of FK to improve the chilling tolerance of banana fruit (Wang et al., 2015). Similarly, Pandey et al (2019) found that NO treatment may up-regulated the expressional level of HK1-like, phosphofructokinase 6-like and PK which involved in glycolysis pathway during seed germination of chickpea. Furthermore, previous study suggested exogenous nitric oxide improved NaCl tolerance by enhancing glycolysis metabolism in barley seedlings (Ma et al., 2021). These results implied that NO plays an essential role in regulating glycolysis metabolism in plant. In the present study, NO may trigger glycolysis metabolic pathway through increasing the gene expression levels of key glycolysis enzymes to produce more energy and activate intermediate metabolism during the process of adventitious root formation under Cd stress, in order to enhance resistance to Cd stress (Shu et al., 2011).
Previous studies found that polyamines (PAs) play an essential role in plant growth and development, as well as response to biotic and abiotic stress (Wimalasekera et al., 2011; Rakesh et al., 2021). In plants, it has been reported that PAs could be produced by ornithine decarboxylase (ODC) or arginine decarboxylase (ADC) pathway, respectively (Groppa and Benavides, 2008). Meanwhile, polyamine oxidase (PAO) plays a major role in mediating PAs degradation in plant (Goyal and Asthir, 2010). In our study, the gene expression of ADC and ODC was significantly down-regulated in CdCl2 treatment, as compared to CK (Figures 7A, B). However, exogenous NO obviously enhanced the gene expression of ADC and ODC which compared with Cd stress, resulting in the accumulation of endogenous polyamine. In addition, removing endogenous NO further implied that NO is involved in PAs accumulation through increasing the expression of ADC and ODC (Figures 7A, B) under Cd stress. Previous studies indicated that PAs metabolism plays a vital role in abiotic stress responses (Gupta et al., 2013). Also, it has been reported that NO could obviously regulate the transcriptional level of polyamine metabolism genes of Medicago truncatula (Filippou et al., 2013). Moreover, exogenous NO resulted in cold tolerance by regulating the expression level of ADC and ODC of tea root (Wang et al., 2020). Furthermore, application of NO could significantly increase the expression of PA biosynthetic enzyme and lower the activity of PAO activity under salt stress (Tailor et al., 2019). In our study, Cd stress remarkably enhanced the expression of PAO while a significant decline in PAO expression was observed in NO treatment (Figure 7C) which may help maintaining PAs levels. These results suggested that NO might enhance abiotic stress tolerance through regulating PAs metabolim. Thus, during adventitious root development under Cd stress, exogenous NO might positively modulate PAs homeostasis through regulating polyamines - related genes expression for adapting to Cd stress condition.
Exogenous application of NO alleviated Cd damage and promoted the adventitious rooting in cucumber explants under Cd stress. Through further studies, our results suggested that NO could reduce oxidative damage and depress lipid peroxidation through improving the antioxidant capacity of cucumber during adventitious root formation in response to Cd stress. Additionally, NO alleviated the damage of Cd stress on the process of adventitious rooting through regulating glycolysis processes and polyamine homeostasis. Therefore, our study may provide new insights into the positive role of NO in promoting adventitious root development under Cd stress. Taken together, our study provided evidence that NO promoted the adventitious root development under Cd stress in cucumber explants through enhancing antioxidant capability, promoting glycolysis pathway and maintaining polyamine homeostasis. However, the regulatory mechanism underlying NO-induced adventitious root development under Cd stress is complex. Further research should focus on the molecular mechanism of NO-regulated rooting response under Cd stress.
The original contributions presented in the study are included in the article/Supplementary Material. Further inquiries can be directed to the corresponding author.
JY designed the experiments. LN and JY performed the experiments. LN, DW and QC performed data analysis. LN wrote the manuscript. YT, BZ and ZH edited the manuscript. All authors contributed to the article and approved the submitted version.
This work was supported by Doctoral Scientific Fund Project of Southwest University of Science and Technology (20zx7135) and Doctoral Scientific Fund Project of Southwest University of Science and Technology (20zx7132).
The authors declare that the research was conducted in the absence of any commercial or financial relationships that could be construed as a potential conflict of interest. The handling editor WL declared a past co-authorship with the author LN.
All claims expressed in this article are solely those of the authors and do not necessarily represent those of their affiliated organizations, or those of the publisher, the editors and the reviewers. Any product that may be evaluated in this article, or claim that may be made by its manufacturer, is not guaranteed or endorsed by the publisher.
Ahmad, P., Abd Allah, E. F., Hashem, A., Sarwat, M., Gucel, S. (2016a). Exogenous application of selenium mitigates cadmium toxicity in Brassica juncea l. (Czern & cross) by up-regulating antioxidative system and secondary metabolites. J. Plant Growth Regul. 35, 936–950. doi: 10.1007/s00344-016-9592-3
Ahmad, P., Abdel Latef, A. A., Abd Allah, E. F., Hashem, A., Sarwat, M., Anjum, N. A., et al. (2016b). Calcium and potassium supplementation enhanced growth, osmolyte secondary metabolite production, and enzymatic antioxidant machinery in cadmium-exposed chickpea (Cicer arietinum l.). Front. Plant Sci. 7. doi: 10.3389/fpls.2016.00513
Altamura, M. M., Piacentini, D., Rovere, F. D., Fattorini, L., Falasca, G., Betti, C. (2023). New paradigms in brassinosteroids, strigolactones, sphingolipids, and nitric oxide interaction in the control of lateral and adventitious root formation. Plants 12, 413. doi: 10.3390/plants12020413
An, Y. J., Kim, Y. M., Kwon, T. I., Jeong, S. W. (2004). Combined effect of copper, cadmium, and lead upon cucumis sativus growth and bioaccumulation. Sci. Total Environ. 326, 85–93. doi: 10.1016/j.scitotenv.2004.01.002
Anwar, S., Shafiq, F., Nisa, Z. U., Usman, U., Ashraf, M. Y., Ali, N. (2021). Effect of cadmium stress on seed germination, plant growth and hydrolyzing enzymes activities in mungbean seedlings. J. Seed Sci. 43, e202143042. doi: 10.1590/2317-1545v43256006
Azevedo, R. A., Gratão, P. L., Monteiro, C. C., Carvalho, R. F. (2012). What is new in the research on cadmium-induced stress in plants? Food Energy Secur. 1, 133–140. doi: 10.1002/fes3.10
Chen, F., Wang, F., Sun, H. Y., Cai, Y., Mao, W. H., Zhang, G. P., et al. (2010). Genotype-dependent effect of exogenous nitric oxide on cd-induced changes in antioxidative metabolism, ultrastructure, and photosynthetic performance in barley seedlings (Hordeum vulgare). J. Plant Growth Regul. 29, 394–408. doi: 10.1007/s00344-010-9151-2
Devi, R., Munjral, N., Gupta, A. K., Kaur, N. (2007). Cadmium induced changes in carbohydrate status and enzymes of carbohydrate metabolism, glycolysis and pentose phosphate pathway in pea. Environ. Exp. Bot. 61, 167–174. doi: 10.1016/j.envexpbot.2007.05.006
Díaz, A. S., da Cunha Cruz, Y., Duarte, V. P., de Castro, E. M., Magalhães, P. C., Pereira, F. J. (2021). The role of reactive oxygen species and nitric oxide in the formation of root cortical aerenchyma under cadmium contamination. Physiol. Plantarum 173, 2323–2333. doi: 10.1111/ppl.13582
Dong, W. K., Ma, X., Jiang, H. Y., Zhao, C. X., Ma, H. L. (2020). Physiological and transcriptome analysis of poa pratensis var. anceps cv. qinghai in response to cold stress. BMC Plant Biol. 20, 1–18. doi: 10.1186/s12870-020-02559-1
Dumanović, J., Nepovimova, E., Natić, M., Kuča, K., Jaćević, V. (2021). The significance of reactive oxygen species and antioxidant defense system in plants: A concise overview. Front. Plant Sci. 11. doi: 10.3389/fpls.2020.552969
El Rasafi, T., Oukarroum, A., Haddioui, A., Song, H., Kwon, E. E., Bolan, N., et al. (2022). Cadmium stress in plants: A critical review of the effects, mechanisms, and tolerance strategies. Crit. Rev. Env. Sci. Tec. 52, 675–726. doi: 10.1080/10643389.2020.1835435
Emamverdian, A., Ding, Y. L., Barker, J., Mokhberdoran, F., Ramakrishnan, M., Liu, G. H., et al. (2021). Nitric oxide ameliorates plant metal toxicity by increasing antioxidant capacity and reducing Pb and cd translocation. Antioxidants 10, 1981. doi: 10.3390/antiox10121981
Falco, G., Bocio, A., Llobet, J. M., Domingo, J. L. (2005). Health risks of dietary intake of environmental pollutants by elite sportsmen and sportswomen. Food Chem. Toxicol. 43, 1713–1721. doi: 10.1016/j.fct.2005.05.014
Fan, J. B., Chen, K., Amombo, E., Hu, Z. R., Chen, L., Fu, J. M. (2015). Physiological and molecular mechanism of nitric oxide (NO) involved in bermudagrass response to cold stress. PloS One 10, e0132991. doi: 10.1371/journal.pone.0132991
Filippou, P., Antoniou, C., Fotopoulos, V. (2013). The nitric oxide donor sodium nitroprusside regulates polyamine and proline metabolism in leaves of Medicago truncatula plants. Free Radical Bio. Med. 56, 172–183. doi: 10.1016/j.freeradbiomed.2012.09.037
Gan, L. P., Wu, X. L., Zhong, Y. (2015). Exogenously applied nitric oxide enhances the drought tolerance in hulless barley. Plant Prod. Sci. 18, 52–56. doi: 10.1626/pps.18.52
Gao, Z. Q., Zhang, J. Y., Zhang, J., Zhang, W. X., Zheng, L. L., Borjigin, T., et al. (2022). Nitric oxide alleviates salt-induced stress damage by regulating the ascorbate-glutathione cycle and Na+/K+ homeostasis in Nitraria tangutorum bobr. Plant Physiol. Bioch. 173, 46–58. doi: 10.1016/j.plaphy.2022.01.017
Gaweł, S., Wardas, M., Niedworok, E., Wardas, P. (2004). Malondialdehyde (MDA) as a lipid peroxidation marker. Wiadomosci Lekarskie 57, 453–455.
Gill, S. S., Tuteja, N. (2010). Reactive oxygen species and antioxidant machinery in abiotic stress tolerance in crop plants. Plant Physiol. Bioch. 48 (12), 909–930. doi: 10.1016/j.plaphy.2014.08.004
Gong, B., Miao, L., Kong, W. J., Bai, J. G., Wang, X. F., Wei, M., et al. (2014). Nitric oxide, as a downstream signal, plays vital role in auxin induced cucumber tolerance to sodic alkaline stress. Plant Physiol. Bioch. 83, 258–266. doi: 10.1016/j.plaphy.2014.08.004
Gong, W. T., Niu, L. J., Wang, C. L., Wei, L. J., Pan, Y., Liao, W. B. (2022). Hydrogen peroxide is involved in salicylic acid-induced adventitious rooting in cucumber under cadmium stress. J. Plant Biol. 65, 43–52. doi: 10.1007/s12374-021-09332-3
Goyal, M., Asthir, B. (2010). Polyamine catabolism influences antioxidative defense mechanism in shoots and roots of five wheat genotypes under high temperature stress. Plant Growth Regul. 60, 13–25. doi: 10.1007/s10725-009-9414-8
Groppa, M. D., Benavides, M. P. (2008). Polyamines and abiotic stress: recent advances. Amino Acids 34, 35–45. doi: 10.1007/s00726-007-0501-8
Guo, J. J., Qin, S. Y., Rengel, Z., Gao, W., Nie, Z. J., Liu, H. G., et al. (2019). Cadmium stress increases antioxidant enzyme activities and decreases endogenous hormone concentrations more in cd-tolerant than cd-sensitive wheat varieties. Ecotox. Environ. safe. 172, 380–387. doi: 10.1016/j.ecoenv.2019.01.069
Gupta, K., Dey, A., Gupta, B. (2013). Plant polyamines in abiotic stress responses. Acta Physiol. Plant 35, 2015–2036. doi: 10.1007/s11738-013-1239-4
Gzyl, J., Chmielowska-Bąk, J., Przymusiński, R., Gwóźdź, E. A. (2015). Cadmium affects microtubule organization and post-translational modifications of tubulin in seedlings of soybean (Glycine max l.). Front. Plant Sci. 6. doi: 10.3389/fpls.2015.00937
Hafsi, C., Collado-Arenal, A. M., Wang, H., Sanz-Fernández, M., Sahrawy, M., Shabala, S., et al. (2022). The role of NADPH oxidases in regulating leaf gas exchange and ion homeostasis in arabidopsis plants under cadmium stress. J. Hazard. Mater. 429, 128217. doi: 10.1016/j.jhazmat.2022.128217
Hashem, A., Alqarawi, A. A., Radhakrishnan, R., Al-Arjani, A. B. F., Aldehaish, H. A., Egamberdieva, D., et al. (2018). Arbuscular mycorrhizal fungi regulate the oxidative system, hormones and ionic equilibrium to trigger salt stress tolerance in Cucumis sativus l. Saudi J. Biol. Sci. 25, 1102–1114. doi: 10.1016/j.sjbs.2018.03.009
He, J. Y., Ren, Y. F., Chen, X. L., Chen, H., et al. (2014). Protective roles of nitric oxide on seed germination and seedling growth of rice (Oryza sativa L.) under cadmium stress. Ecotox. Environ. Safe. 108, 114–119. doi: 10.1016/j.ecoenv.2014.05.021
Heyno, E., Klose, C., Krieger-Liszkay, A. (2008). Origin of cadmium-induced reactive oxygen species production: mitochondrial electron transfer versus plasma membrane NADPH oxidase. New Phytol. 179, 687–699. doi: 10.1111/j.1469-8137.2008.02512.x
Huang, H. L., Ullah, F., Zhou, D. X., Yi, M., Zhao, Y. (2019). Mechanisms of ROS regulation of plant development and stress responses. Front. Plant Sci. 10. doi: 10.3389/fpls.2019.00800
Irfan, M., Ahmad, A., Hayat, S. (2014). Effect of cadmium on the growth and antioxidant enzymes in two varieties of brassica juncea. Saudi J. Biol. Sci. 21, 125–131. doi: 10.1016/j.sjbs.2013.08.001
Jaleel, C. A., Manivannan, P., Sankar, B., Kishorekumar, A., Gopi, R., Somasundaram, R., et al. (2007). Water deficit stress mitigation by calcium chloride in catharanthus roseus: Effects on oxidative stress, proline metabolism and indole alkaloid accumulation. Colloids Surfaces B: Biointerfaces 60, 110–116. doi: 10.1016/j.colsurfb.2007.06.006
Kang, W., Wang, L. Y., Li, R., Zhang, C. C., Wu, L. Y., Li, H. L., et al. (2018). Endogenous nitric oxide and hydrogen peroxide detection in indole-3-butyric acid-induced adventitious root formation in Camellia sinensis. J. Integr. Agric. 17, 2273–2280. doi: 10.1016/S2095-3119(18)62059-3
Kaya, C., Ashraf, M., Alyemeni, M. N., Ahmad, P. (2020). Responses of nitric oxide and hydrogen sulfide in regulating oxidative defence system in wheat plants grown under cadmium stress. Physiol. Plantarum 168, 345–360. doi: 10.1111/ppl.13012
Kaya, C., Ashraf, M., Sönmez, O., Tuna, A. L., Aydemir, S. (2015). Exogenously applied nitric oxide confers tolerance to salinity-induced oxidativestress in two maize (Zea mays l.) cultivars differing in salinity tolerance. Turk. J. Agric. For. 39, 909–919. doi: 10.3906/tar-1411-26
Kaya, C., Okant, M., Ugurlar, F., Alyemeni, M. N., Ashraf, M., Ahmad, P. (2019). Melatonin-mediated nitric oxide improves tolerance to cadmium toxicity by reducing oxidative stress in wheat plants. Chemosphere 225, 627–638. doi: 10.1016/j.chemosphere.2019.03.026
Kohli, S. K., Khanna, K., Bhardwaj, R., Abd Allah, E. F., Ahmad, P., Corpas, F. J. (2019). Assessment of subcellular ROS and NO metabolism in higher plants: multifunctional signaling molecules. Antioxidants 8, 641. doi: 10.3390/antiox8120641
Kucerova, D., Labancova, E., Vivodova, Z., Kollarova, K. (2020). The modulation of ion homeostasis by silicon in cadmium treated poplar callus cells. Environ. Sci. pollut. R. 27, 2857–2867. doi: 10.1007/s11356-019-07054-1
Laspina, N. V., Groppa, M. D., Tomaro, M. L., Benavides, M. P. (2005). Nitric oxide protects sunflower leaves against cd-induced oxidative stress. Plant Sci. 169, 323–330. doi: 10.1016/j.plantsci.2005.02.007
Li, B. B., Fu, Y. S., Li, X. X., Yin, H. N., Xi, Z. M. (2022). Brassinosteroids alleviate cadmium phytotoxicity by minimizing oxidative stress in grape seedlings: Toward regulating the ascorbate-glutathione cycle. Sci. Hortic. 299, 111002. doi: 10.1016/j.scienta.2022.111002
Li, S. W., Li, Y., Leng, Y., Zeng, X. Y., Ma, Y. H. (2019). Nitric oxide donor improves adventitious rooting in mung bean hypocotyl cuttings exposed to cadmium and osmotic stresses. Environ. Exp. Bot. 164, 114–123. doi: 10.1016/j.envexpbot.2019.05.004
Liao, W. B., Huang, G. B., Yu, J. H., Zhang, M. L., Shi, X. L. (2011). Nitric oxide and hydrogen peroxide are involved in indole-3-butyric acid-induced adventitious root development in marigold. J. Hortic. Sci. Biotech. 86, 159–165. doi: 10.1080/14620316.2011.11512742
Liu, H. W., Wang, C. L., Li, C. X., Zhao, Z. X., Wei, L. J., Liu, Z. Y., et al. (2022a). Nitric oxide is involved in hydrogen sulfide-induced adventitious rooting in tomato (Solanum lycopersicum). Funct. Plant Biol. 49, 245–258. doi: 10.1071/FP21288
Liu, Y. Y., Wei, L. J., Feng, L., Zhang, M. L., Hu, D. L., Tie, J. Z., et al. (2022b). Hydrogen sulfide promotes adventitious root development in cucumber under salt stress by enhancing antioxidant ability. Plants 11, 935. doi: 10.3390/plants11070935
Luo, H. J., Li, H. Y., Zhang, X. Z., Fu, J. M. (2011). Antioxidant responses and gene expression in perennial ryegrass (Lolium perenne l.) under cadmium stress. Ecotoxicology 20, 770–778. doi: 10.1007/s10646-011-0628-y
Møller, I. M., Jensen, P. E., Hansson, A. (2007). Oxidative modifications to cellular components in plants. Annu. Rev. Plant Biol. 58, 459–481. doi: 10.1146/annurev.arplant.58.032806.103946
Ma, J., Saleem, M. H., Alsafran, M., Jabri, H. A., Mehwish, Rizwan, M., et al. (2022). Response of cauliflower (Brassica oleracea l.) to nitric oxide application under cadmium stress. Ecotox. Environ. Safe. 243, 113969. doi: 10.1016/j.ecoenv.2022.113969
Ma, Y., Yao, Y., Wang, Q. E., Gu, Z. X., Wang, P., Han, Y. B., et al. (2021). Mechanism of nitric oxide enhancing NaCl tolerance of barley seedlings based on physiol-biochemical analysis and LC-MS metabolomics. Environ. Exp. Bot. 189, 104533. doi: 10.1016/j.envexpbot.2021.104533
Manara, A., Fasani, E., Molesini, B., DalCorso, G., Pennisi, F., Pandolfini, T., et al. (2020). The tomato metallocarboxypeptidase inhibitor I, which interacts with a heavy metal-associated isoprenylated protein, is implicated in plant response to cadmium. Molecules 25, 700. doi: 10.3390/molecules25030700
Mansoor, S., Wani, O. A., Lone, J. K., Manhas, S., Kour, N., Alam, P., et al. (2022). Reactive oxygen species in plants: from source to sink. Antioxidants 11, 225. doi: 10.3390/antiox11020225
Mostofa, M. G., Rahman, M. M., Ansary, M. M. U., Fujita, M., Tran, L. S. P. (2019). Interactive effects of salicylic acid and nitric oxide in enhancing rice tolerance to cadmium stress. Int. J. Mol. Sci. 20, 5798. doi: 10.3390/ijms20225798
Muhammad, T., Zhang, J., Ma, Y., Li, Y., Zhang, F., Zhang, Y., et al. (2019). Overexpression of a mitogen-activated protein kinase SlMAPK3 positively regulates tomato tolerance to cadmium and drought stress. Molecules 24, 556. doi: 10.3390/molecules24030556
Niu, L. J., Yu, J. H., Liao, W. B., Xie, J. M., Yu, J., Lv, J., et al. (2019). Proteomic investigation of s-nitrosylated proteins during NO-induced adventitious rooting of cucumber. Int. J. Mol. Sci. 20, 5363. doi: 10.3390/ijms20215363
Niu, L. J., Yu, J. H., Liao, W. B., Yu, J. H., Zhang, M. L., Dawuda, M. M. (2017). Calcium and calmodulin are involved in nitric oxide-induced adventitious rooting of cucumber under simulated osmotic stress. Front. Plant Sci. 8. doi: 10.3389/fpls.2017.01684
Pagnussat, G. C., Simontacchi, M., Puntarulo, S., Lamattina, L. (2002). Nitric oxide is required for root organogenesis. Plant Physiol. 129, 954–956. doi: 10.1104/pp.004036
Panda, P., Nath, S., Chanu, T. T., Sharma, G. D., Panda, S. K. (2011). Cadmium stress-induced oxidative stress and role of nitric oxide in rice (Oryza sativa l.). Acta Physiol. Plant 33, 1737–1747. doi: 10.1007/s11738-011-0710-3
Pandey, S., Kumari, A., Shree, M., Kumar, V., Singh, P., Bharadwaj, C., et al. (2019). Nitric oxide accelerates germination via the regulation of respiration in chickpea. J. Exp. Bot. 70, 4539–4555. doi: 10.1093/jxb/erz185
Panyuta, O., Belava, V., Fomaidi, S., Kalinichenko, O., Volkogon, M., Taran, N. (2016). The effect of pre-sowing seed treatment with metal nanoparticles on the formation of the defensive reaction of wheat seedlings infected with the eyespot causal agent. Nanoscale Res. Lett. 11, 1–5. doi: 10.1186/s11671-016-1305-0
Parankusam, S., Adimulam, S. S., Bhatnagar-Mathur, P., Sharma, K. K. (2017). Nitric oxide (NO) in plant heat stress tolerance: current knowledge and perspectives. Front. Plant Sci. 8. doi: 10.3389/fpls.2017.01582
Plaxton, W. C. (1996). The organization and regulation of plant glycolysis. Annu. Rev. Plant Biol. 47, 185–214. doi: 10.1146/annurev.arplant.47.1.185
Polle, A., Schützendübel, A. (2003). “Heavy metal signalling in plants: linking cellular and organismic responses,” in Plant responses to abiotic stress. Eds. Hirt, H., Shinozaki, K. (Berlin, Heidelberg: Springer Press), 187–215. doi: 10.1007/978-3-540-39402-0_8
Prado, A. M., Porterfield, D. M., Feijó, J. A. (2004). Nitric oxide is involved in growth regulation and re-orientation of pollen tubes. Development 131, 2707–2714. doi: 10.1242/dev.01153
Qi, W. Y., Li, Q., Chen, H., Liu, J., Xing, S. F., Xu, M., et al. (2021). Selenium nanoparticles ameliorate Brassica napus l. cadmium toxicity by inhibiting the respiratory burst and scavenging reactive oxygen species. J. Hazard. Mater. 417, 125900. doi: 10.1016/j.jhazmat.2021.125900
Rakesh, B., Sudheer, W. N., Nagella, P. (2021). Role of polyamines in plant tissue culture: An overview. Plant Cell Tiss. Org. 145, 487–506. doi: 10.1007/s11240-021-02029-y
Ren, Y. F., Wang, W., He, J. Y., Zhang, L. Y., Wei, Y. J., Yang, M. (2020). Nitric oxide alleviates salt stress in seed germination and early seedling growth of pakchoi (Brassica chinensis l.) by enhancing physiological and biochemical parameters. Ecotox. Environ. Safe. 187, 109785. doi: 10.1016/j.ecoenv.2019.109785
Rivelli, A. R., Puschenreiter, M., De Maria, S. (2014). Assessment of cadmium uptake and nutrient content in sunflower plants grown under cd stress. Plant Soil Environ. 60, 80–86. doi: 10.17221/520/2013-pse
Rizwan, M., Ali, S., Abbas, T., Adrees, M., Zia-ur-Rehman, M., Ibrahim, M., et al. (2018). Residual effects of biochar on growth, photosynthesis and cadmium uptake in rice (Oryza sativa l.) under cd stress with different water conditions.J. Environ.Manage. 206, 676–683. doi: 10.1016/j.jenvman.2017.10.035
Shahid, M. A., Balal, R. M., Khan, N., Zotarelli, L., Liu, G. D., Sarkhosh, A., et al. (2019). Selenium impedes cadmium and arsenic toxicity in potato by modulating carbohydrate and nitrogen metabolism. Ecotox. Environ. Safe. 180, 588–599. doi: 10.1016/j.ecoenv.2019.05.037
Shu, L. B., Lou, Q. J., Ma, C. F., Ding, W., Zhou, J., Wu, J. H., et al. (2011). Genetic, proteomic and metabolic analysis of the regulation of energy storage in rice seedlings in response to drought. Proteomics 11, 4122–4138. doi: 10.1002/pmic.201000485
Singh, S., Singh, A., Bashri, G., Prasad, S. M. (2016). Impact of cd stress on cellular functioning and its amelioration by phytohormones: an overview on regulatory network. Plant Growth Regul. 80, 253–263. doi: 10.1007/s10725-016-0170-2
Sun, H. W., Feng, F., Liu, J., Zhao, Q. Z. (2019). Nitric oxide affects rice root growth by regulating auxin transport under nitrate supply. Front. Plant Sci. 9. doi: 10.3389/fpls.2019.01123
Sun, L. Y., Song, F. B., Zhu, X. C., Liu, S. Q., Liu, F. L., Wang, Y. J., et al. (2021). Nano-ZnO alleviates drought stress via modulating the plant water use and carbohydrate metabolism in maize. Arch. Agron. Soil Sci. 67, 245–259. doi: 10.1080/03650340.2020.1723003
Tailor, A., Tandon, R., Bhatla, S. C. (2019). Nitric oxide modulates polyamine homeostasis in sunflower seedling cotyledons under salt stress. Plant Signal. Behav. 14, 1667730. doi: 10.1080/15592324.2019.1667730
Terrón-Camero, L. C., Pelaez-Vico, M. A., Del-Val, C., Sandalio, L. M., Romero-Puertas, M. C. (2019). Role of nitric oxide in plant responses to heavy metal stress: exogenous application versus endogenous production. J. Exp. Bot. 70, 4477–4488. doi: 10.1093/jxb/erz184
Wang, B., Bian, B. T., Wang, C. L., Li, C. X., Fang, H., Zhang, J., et al. (2019). Hydrogen gas promotes the adventitious rooting in cucumber under cadmium stress. Plas One 14, e0212639. doi: 10.1371/journal.pone.0212639
Wang, Y. H., Xiong, F., Nong, S. H., Liao, J. R., Xing, A. Q., Shen, Q., et al. (2020). Effects of nitric oxide on the GABA, polyamines, and proline in tea (Camellia sinensis) roots under cold stress. Sci. Rep. 10, 12240. doi: 10.1038/s41598-020-69253-y
Wang, Y. S., Luo, Z. S., Khan, Z. U., Mao, L. C., Ying, T. J. (2015). Effect of nitric oxide on energy metabolism in postharvest banana fruit in response to chilling stress. Postharvest Biol. Tec. 108, 21–27. doi: 10.1016/j.postharvbio.2015.05.007
Wang, Y. Q., Lv, P. X., Kong, L. S., Shen, W. B., He, Q. J. (2021). Nanomaterial-mediated sustainable hydrogen supply induces lateral root formation via nitrate reductase-dependent nitric oxide. Chem. Eng. J. 405, 126905. doi: 10.1016/j.cej.2020.126905
Wei, L. J., Zhang, J., Wang, C. L., Liao, W. B. (2020). Recent progress in the knowledge on the alleviating effect of nitric oxide on heavy metal stress in plants. Plant Physiol. Bioch. 147, 161–171. doi: 10.1016/j.plaphy.2019.12.021
Wimalasekera, R., Tebartz, F., Scherer, G. F. E. (2011). Polyamines, polyamine oxidases and nitric oxide in development, abiotic and biotic stresses. Plant Sci. 181, 593–603. doi: 10.1016/j.plantsci.2011.04.002
Xia, H., Liu, X. L., Wang, Y. M., Lin, Z. Y., Deng, H. H., Wang, J., et al. (2022). 24-Epibrassinolide and nitric oxide combined to improve the drought tolerance in kiwifruit seedlings by proline pathway and nitrogen metabolism. Sci. Hortic 297, 110929. doi: 10.1016/j.scienta.2022.110929
Xu, P. L., Guo, Y. K., Bai, J. G., Shang, L., Wang, X. J. (2008). Effects of long-term chilling on ultrastructure and antioxidant activity in leaves of two cucumber cultivars under low light. Physiol. Plantarum 132, 467–478. doi: 10.1111/j.1399-3054.2007.01036.x
Xuan, W., Xu, S., Li, M. Y., Han, B., Zhang, B., Zhang, J., et al. (2012). Nitric oxide is involved in hemin-induced cucumber adventitious rooting process. J. Plant Physiol. 169, 1032–1039. doi: 10.1016/j.jplph.2012.02.021
Yang, W., Zhu, C. H., Ma, X. L., Li, G. J., Gan, L. J., Ng, D., et al. (2013). Hydrogen peroxide is a second messenger in the salicylic acid-triggered adventitious rooting process in mung bean seedlings. PloS One 8, e84580. doi: 10.1371/journal.pone.0084580
Yu, C. L., Sun, C. D., Shen, C. J., Wang, S. K., Liu, F., Liu, Y., et al. (2015). The auxin transporter, OsAUX 1, is involved in primary root and root hair elongation and in Cd stress responses in rice (Oryza sativa L.). Plant J 83, 818–830. doi: 10.1111/tpj.12929
Zhang, J. T., Zhang, Y., Du, Y. Y., Chen, S. Y., Tang, H. R. (2011a). Dynamic metabonomic responses of tobacco (Nicotiana tabacum) plants to salt stress. J. Proteome Res. 10, 1904–1914. doi: 10.1021/pr101140n
Zhang, Y. T., Liu, A. L., Hao, Y. W., Su, W., Sun, G. W., Song, S. W., et al. (2022). Nitric oxide is essential for melatonin to enhance nitrate tolerance of cucumber seedlings. Molecules 27, 5806. doi: 10.3390/molecules27185806
Zhao, C. S., Bao, Z. J., Feng, H. Y., Chen, L. C., Li, Q. (2022). Nitric oxide enhances resistance of pleurotus eryngii to cadmium stress by alleviating oxidative damage and regulating of short-chain dehydrogenase/reductase family. Environ. Sci. pollut. R. 29, 53036–53049. doi: 10.1007/s11356-022-19613-0
Zhong, M., Yuan, Y. H., Shu, S., Sun, J., Guo, S. R., Yuan, R. N., et al. (2016). Effects of exogenous putrescine on glycolysis and Krebs cycle metabolism in cucumber leaves subjected to salt stress. Plant Growth Regul. 79, 319–330. doi: 10.1007/s10725-015-0136-9
Zuccarelli, R., Rodríguez-Ruiz, M., Lopes-Oliveira, P. J., Pascoal, G. B., Andrade, S. C. S., Furlan, C. M., et al. (2021). Multifaceted roles of nitric oxide in tomato fruit ripening: NO-induced metabolic rewiring and consequences for fruit quality traits. J. Exp. Bot. 72, 941–958. doi: 10.1093/jxb/eraa526
Keywords: nitric oxide, cadmium, rooting response, antioxidants, glycolysis, polyamine pathway
Citation: Niu L, Tang Y, Zhu B, Huang Z, Wang D, Chen Q and Yu J (2023) Nitric oxide promotes adventitious root formation in cucumber under cadmium stress through improving antioxidant system, regulating glycolysis pathway and polyamine homeostasis. Front. Plant Sci. 14:1126606. doi: 10.3389/fpls.2023.1126606
Received: 18 December 2022; Accepted: 22 February 2023;
Published: 09 March 2023.
Edited by:
Weibiao Liao, Gansu Agricultural University, ChinaReviewed by:
Sajid Ali, Bahauddin Zakariya University, PakistanCopyright © 2023 Niu, Tang, Zhu, Huang, Wang, Chen and Yu. This is an open-access article distributed under the terms of the Creative Commons Attribution License (CC BY). The use, distribution or reproduction in other forums is permitted, provided the original author(s) and the copyright owner(s) are credited and that the original publication in this journal is cited, in accordance with accepted academic practice. No use, distribution or reproduction is permitted which does not comply with these terms.
*Correspondence: Jian Yu, eWppYW4wNTE1QDE2My5jb20=
Disclaimer: All claims expressed in this article are solely those of the authors and do not necessarily represent those of their affiliated organizations, or those of the publisher, the editors and the reviewers. Any product that may be evaluated in this article or claim that may be made by its manufacturer is not guaranteed or endorsed by the publisher.
Research integrity at Frontiers
Learn more about the work of our research integrity team to safeguard the quality of each article we publish.