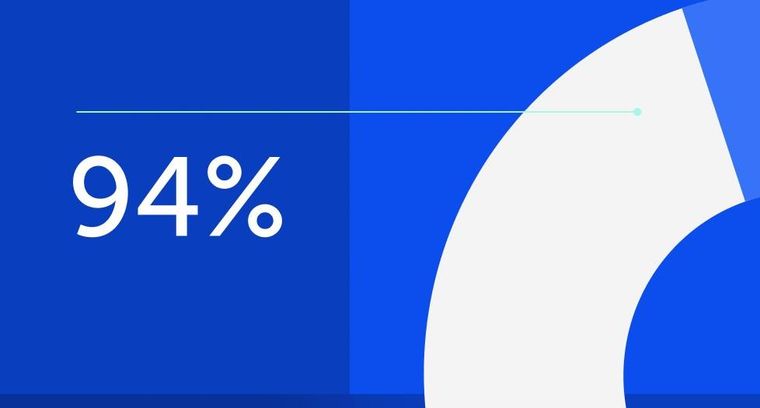
94% of researchers rate our articles as excellent or good
Learn more about the work of our research integrity team to safeguard the quality of each article we publish.
Find out more
ORIGINAL RESEARCH article
Front. Plant Sci., 22 February 2023
Sec. Plant Abiotic Stress
Volume 14 - 2023 | https://doi.org/10.3389/fpls.2023.1125529
This article is part of the Research TopicAbiotic Stress Mechanisms and Enhancement in Crops: Physiological and Biochemical ApproachesView all 15 articles
The present study investigates the efficiency of nitrogen (N) amendments in the management of ozone (O3) stress in two varieties (Kashi Sheetal and Kashi Harittima) of Indian bean (Dolichos lablab L.). Two O3 concentrations, ambient (44.9 ppb) and elevated (74.64 ppb) were used, and each O3 concentration has 3 nitrogen (N) dose treatments viz recommended (N1), 1.5 times recommended (N2), 2 times recommended (N3) and no nitrogen, which served as control (C). The experiment concluded Kashi Sheetal as O3 tolerant, as compared to Kashi Harittima. N amendments were effective in the partial amelioration of O3 stress, with N2 being the most effective nitrogen dose, at both ambient and elevated O3 concentrations. Kashi Sheetal has been determined to be O3 tolerant due to greater endogenous levels of H2O2 accumulation and enzymatic antioxidant contents with O3 exposure. The O3-sensitive variety, Kashi Harittima, responded more positively to N treatments, at both O3 concentrations. The positive effect of N amendments is attributed to the stimulated antioxidative enzyme activity, rather than the biophysical processes like stomatal conductance. Strengthened defense upon N amendments was attributed to the enhanced activities of APX and GR in Kashi Sheetal, while in Kashi Harittima, the two enzymes (APX and GR) were coupled by SOD and CAT as well, during the reproductive phase. Yield (weight of seeds plant-1) increments upon N (N2) amendments were higher in Kashi Harittima (O3 sensitive), as compared to Kashi Sheetal (O3 tolerant) at both ambient and elevated O3 concentration, due to higher antioxidant enzymatic response and greater rate of photosynthesis in the former.
Over the past few decades, speedy industrial growth and unrestrained urbanization in developing nations have greatly increased the concentration of primary and secondary pollutants in the atmosphere (Dhevagi et al., 2021). The concentration of phytotoxic secondary pollutant tropospheric ozone (O3) depends on the levels of certain primary air pollutants such as nitrogen oxides (NOx), and volatile organic compounds (VOCs). In recent years, tropospheric ozone has shown a severe impact on plants because of its high oxidative potential (Duque et al., 2021). Background O3 concentration in the troposphere has increased by 36% since pre-industrial times (Mukherjee and Hazra, 2022). Nearly one-quarter of the world is currently at risk of high O3 and it is predicted that the levels could rise to 20% by 2050 (Meehl et al., 2018). Because of chemical interactions between O3 precursors, industrial pollutants, and sunlight, surface ozone levels are increasing at a rate of 0.5 to 2.5 percent each year (Wang et al., 2022). Because it has a negative impact on people’s health, plants, and the ecosystem worldwide, the rising concentration of ground-level O3 has become a global issue (Mills et al., 2013). Even if strict adherence to the air quality regulations of 2000 is maintained, the O3-induced damage is projected to worsen, according to future emission scenario legislation taking emissions for the year 2030 (CLE-2030) (Van Dingenen et al., 2009). A number of modeling studies have depicted high tropospheric ozone concentration in the future thus making the study of the impact of elevated ozone on plants even more important.
The stomata are the primary entry point for ozone into plant leaves (Stella et al., 2013). Ozone quickly reacts with intracellular components and forms reactive oxygen species (ROS) such as hydrogen peroxide (H2O2), superoxide radicals (O2-), and hydroxyl radicals (OH·) inside the leaf (Zhang et al., 2017). These ROS enhance the antioxidant defense response downstream, which mitigates the fatal consequences of accumulating ROS. Contradictorily, when ROS generation surpasses the antioxidant efficiency, plants show ozone vulnerability in the form of foliar injury symptoms, alteration in metabolic processes, yield loss, and other ozone-related effects (Singh et al., 2014; Singh et al., 2018; Hayes et al., 2020). Excessive ROS levels within the cells brought on by O3 damage the membranes (lipid peroxidation), oxidize proteins, degrade RNA and DNA, enhance the production of secondary metabolites, degrade chlorophyll, and ultimately result in apoptosis (Choudhury et al., 2017). However, a vast variety of antioxidants and ROS-scavenging enzymes such as catalase (CAT), superoxide dismutase (SOD), glutathione reductase (GR), and ascorbate peroxidase (APX), produced by cells upon O3 exposure to attenuate the impacts of ROS in the cells (Picchi et al., 2017). In order to maintain steady development in plants and to aid in the damage repair caused by various stressors, nutrient supplements have frequently been utilized (Sahoo and Tiwari, 2021). Nitrogen (N) is the fourth most common element in living things and is theoretically an important component that supports plant development (Sahoo and Tiwari, 2021). Nitrogen amendment not only maintains greater protein levels it can also be used for repair processes and facilitates the remobilization of nutrients to reproductive parts, sustaining better production (Zeng et al., 2017). Nitrogen applications tend to improve the photosynthetic potential of the plant which can be commonly coupled with stomatal conductance (Zhang et al., 2018). The addition of nitrogen may partly counteract the detrimental effects of O3 on a few plants’ morphological characteristics (Yendrek et al., 2013). Plants can either strengthen their antioxidant defense against an O3-induced ROS surge, fix more carbon to use for O3-induced injury, or direct more biomass into reproductive organs to sustain greater yields in response to N amendments (Podda et al., 2019).
A significant portion of India’s protein needs is fulfilled by pulses, one of the country’s essential crops, which are grown on an area of 28.3 million hectares and produced approximately 25.7 million metric tonnes in the fiscal year 2020–21 (Statista, 2021). In Asia, where the mean ambient ozone concentration ranged between 35 and 75 ppb during the growing season of the crop, legumes had a substantial production loss of 10 to 66 percent (Emberson et al., 2009). Dolichos lablab L. is a versatile crop, several plant components may be consumed as food, animal feed, and green manure (Davari et al., 2018; Rana et al., 2021). It is a low-cost source of protein and micronutrients when compared to other legumes, highlighting the importance of its study in view of food security and nutrition in near future (Letting et al., 2021). These micronutrients, which play a significant role in the diets of resource-limited households in rural areas, include phosphorus, fiber, niacin, and thiamine (Letting et al., 2021). The most recent study shows how efficient lablab bean extracts are at preventing the spread of viral illnesses like influenza and SARS-CoV-2, which has been called a global pandemic (Liu et al., 2020). To the best of our knowledge, the sensitivity of Dolichos lablab L. varieties towards tropospheric ozone has not been defined. The present work is the first effort to characterize the sensitivity of two varieties of Dolichos lablab L. towards O3 stress. In addition, it also exemplifies the efficiency of nitrogen amendments in the management of O3 stress and the mechanistic approach adopted therein. Through our work, we hypothesize that the ameliorative effect of N amendments in O3-stressed plants is mostly credited to the antioxidant response rather than the biophysical parameters like stomatal conductance.
The area of study was the Botanical Garden at Banaras Hindu University, Varanasi. It is a sub-urban site located in the Indian subcontinent at 25014’ N latitude, 82003’ E longitude, and 76.19 m above sea level. The crop was grown during the winter season from November 2021 to March 2022. A subtropical humid climate prevails in the area, with distinctive summer, rainy, and winter seasons. The soil is sandy loam in texture (sand 45%, silt 28%, clay 27%) having an organic carbon content of 0.67%, pH 7.4, nitrogen content of 0.12%, and phosphorus content of 0.065%.
Two varieties of Indian bean (Dolichos lablab L.) namely, Kashi Sheetal and Kashi Harittima were used for the experiment. The seeds of the above varieties were procured from the Indian Institute of Vegetable Research, Varanasi (IIVR-IARI). Kashi Sheetal is a semi-pole type variant with low-temperature tolerance. It is very rich in protein and can give a yield of about 18-20 tonnes/hectare. The color of flowers of this variety is violet and the pods were green with a dark red lining at their edges. Kashi Harittima is reasonably resistant to Dolicho’s yellow mosaic virus and also exhibits tolerance against aphids and pod borers. It is a high-yielding variety with parchment-free green-colored pods and white flowers.
The study was carried out by installing custom-built open-top chambers (OTCs) in the experimental area under ambient field circumstances. The diameter of the OTCs was 1.5m and the height was 1.8m. OTCs were set up in the botanical garden and were categorized as ambient O3 OTCs and elevated O3 OTCs (ambient + 30 ppb) on the basis of O3 concentrations maintained in them. The experimental setup is explained in Figure 1. Each level of O3 treatment was further supplemented with 3 doses of nitrogen amendments viz recommended (AN1 and EN1), 1.5 times recommended (AN2 and EN2) and 2 times recommended (AN3 and EN3). For each O3 treatment, control was also maintained (AC and EC), wherein no N treatment was given (Figure 2). Each treatment was replicated thrice. The nitrogen fertilizer was applied to the soil and the treatment was given in two phases i.e, one in the vegetative phase and the other in the reproductive phase. In OTCs, seeds were hand sown in accordance with standard agricultural procedures. Ozone was produced by the ozone generator (A1G, Faraday, India) and then transported through a connecting tube to the OTCs designated for elevated O3. For ozone formation, the generator utilized high-frequency corona discharge technology. The release of ozone into the growth chamber was set at multiple points at the base of the OTCs. The experimental setup and design are displayed in Figure 1.
Continuous O3 monitoring was done throughout the experiment. The concentrations of elevated ozone were standardized once the OTCs were mounted in the field. Ozone generators were calibrated to create the necessary levels of elevated ozone (ambient + 30 ppb). Using an automated real-time O3 monitoring instrument (Model APOA 370, HORIBA Ltd., Japan), eight-hour O3 monitoring (9:00-17:00 h) was carried out during the growth period.
By using a histochemical analysis, the test plants’ flag leaves in the reproductive phase were studied in-situ localization of accumulated hydrogen peroxide (H2O2). For each cultivar, three randomly chosen leaf samples from different treatments were taken. The procedure given by Thordal-Christensen et al. (1997) was followed for studying the in-situ localization of H2O2 in leaves using 3, 3′-Diaminobenzidine (DAB).
Photosynthetic rate (A), stomatal conductance (gs), and internal CO2 concentration (Ci) were analyzed by using a portable photosynthetic instrument (CIRAS-3, PP SYSTEMS). All these parameters were measured for both ambient and elevated conditions. Three randomly chosen plants per plot had their fourth fully grown leaf from the top examined for physiological parameters. At 40 DAG and 60 DAG, measurements were made between 9:00 and 10:30 hours on cloud-free days. The instrument was calibrated utilizing a known source of CO2 set at 510 ppm and the photosynthetic active radiation (PAR) set at 1200 mmol m-2 s-1.
For the enzymatic assay, 0.2 gm of fresh weight of leaf was taken and homogenized using liquid nitrogen. 5 ml of extracting buffer was used to homogenize the leaf tissues in order to extract antioxidant enzymes. The buffer was prepared using 1 M phosphate buffer of pH 7.0 of polyvinylpyrrolidone (PVP), phenyl methane sulfonyl fluoride (PMSF), Ethylenediaminetetraacetic acid (EDTA), and Triton-X-100 at 4°C. Antioxidant enzymes like superoxide dismutase (SOD), ascorbate peroxidase (APX), catalase (CAT), and glutathione reductase (GR) were estimated by the protocols (Fridovich, 1975; Nakano and Asada, 1981; Aebi, 1984; Anderson, 1996). The method developed by Heath and Packer (Heath and Packer, 1968) was used to estimate the malondialdehyde (MDA) content which represents lipid peroxidation. Leaf tissues (0.5 g) were extracted in 5% TCA, and 4 mL of 20% TCA containing 0.5% TBA was added. Following centrifugation, the sample mixture’s absorbance was measured at 532 and 600 nm.
In order to determine yield, five plants per OTC were harvested in the first week of March and quantified for yield attributes. The number of seeds plant-1, the weight of seeds plant-1, and the test weight per 1000 seeds were estimated for each treatment.
All the statistical tests were executed using SPSS software (SPSS Inc. version 25.0, IBM Corp, New York). The three-way ANOVA was performed to examine the significance of the age of sampling, nitrogen treatment, and varieties of beans. Using the Shapiro-Wilk test, the normality of each dataset was examined, and the distribution was determined to be normal in all instances since the P values were all over the threshold of significance (0.05). For both cultivars, the data was examined via principal component analysis (PCA). The correlation matrix and regression technique were used to perform the PCA.
During the growth period of the crop, the mean concentration of ambient O3 and elevated O3 was found to be 44.9 ppb and 74.64 ppb, respectively. The ambient O3 concentration ranged from 29 ppb to 66 ppb and the elevated O3 concentration ranged between 58 ppb to 97 ppb (Figure 3).
Histochemical detection of H2O2 in the leaves of both varieties of Dolichos lablab L. indicated the presence of H2O2 (Figure 4). The interaction of H2O2 with DAB under elevated O3 (EO3) as compared to ambient O3 (AO3) produced a stronger reddish-brown stain in the leaf tissues of plants of both varieties. As compared with Kashi Harittima, leaves of Kashi Sheetal contained higher concentrations of H2O2. Furthermore, as nitrogen amendment amounts are increased, the H2O2 content of both varieties of Indian bean decreased significantly.
Figure 4 Histochemical localization of hydrogen peroxide (H2O2) stained with DAB (brown color) in two varieties of Dolichos lablab L. exposed to AO3 and EO3 under different nitrogen fertilization levels.
Plants grown in elevated O3 conditions showed significantly higher levels of lipid peroxidation (LPO) in their leaves than plants grown in ambient O3 conditions in both varieties of Dolichos lablab L. (Figures 5, 6). All three types of nitrogen treatments (N1, N2, and N3) showed significantly decreased lipid peroxidation as compared to control in both Kashi Sheetal and Kashi Harittima. For both varieties, the degree of lipid peroxidation was higher during the reproductive period than during the vegetative phase (Figures 5, 6). In both the vegetative and reproductive stages, Kashi Harittima plants had increased lipid peroxidation than Kashi Sheetal plants. The differences in the level of lipid peroxidation between treatments N2 and N3 were non-significant for both phases of growth. The results of the four-way ANOVA show that LPO varied significantly due to two individual factors such as age and treatment. Significant variations in LPO were also observed due to interactions of age with variety, ozone, and treatment factors (Table 1).
Figure 5 Variations in antioxidative enzymes in both varieties of Dolichos lablab L grown under AO3 and EO3 in the vegetative phase. Values are mean ± SE. Level of significance between AO3 and EO3 treated plants based on t-test; ns; not significant; *, P ≤ 0.05; **, P ≤ 0.01; ***, P ≤ 0.001.
Figure 6 Variations in antioxidative enzymes in both varieties of Dolichos lablab L grown under AO3 and EO3 in the reproductive phase. Values are mean ± SE. Level of significance between AO3 and EO3 treated plants based on t-test; ns; not significant; *, P ≤ 0.05; **, P ≤ 0.01; ***, P ≤ 0.001.
Table 1 F-ratio and level of significance of selected biochemical and physiological characteristics of Dolichos lablab L.
Antioxidative enzyme tests revealed that plants grown under EO3 conditions had much higher levels of enzymatic activity than in AO3. In both growth phases, Kashi Sheetal plants had greater APX activity than Kashi Harittima plants (Figures 5, 6). At all ages and in both varieties of Indian bean, there was no significant difference in the activity of the APX enzyme between the N2 and N3 treatments as compared to the control. N1 and N2 treatments showed significantly higher activities of APX in comparison to control in both EO3 and AO3 conditions. APX activity was significantly higher in the reproductive phase in both the varieties at EO3 and AO3 conditions. Results of four-way ANOVA revealed that APX varied significantly due to all individual factors and their interactions except age*ozone*variety and age*ozone*treatment (Table 1). Similar results were also revealed for activities of other antioxidative enzymes like CAT, GR, and SOD where the activity of these enzymes increased in reproductive stages of growth (Figures 5, 6). N1, N2, and N3 treatments showed significantly higher values of enzymatic activity as compared to the control but there was no significant difference between N2 and N3 treatments. The activity of CAT, GR, and SOD enzymes also increased significantly in the EO3 conditions as compared to AO3 conditions at all ages and this accounts for both varieties of Dolichos lablab L. (Figures 5, 6). GR and SOD showed significant variations for all four individual factors and some of their interactions such as age*variety and age *treatment. CAT showed significant variations for all individual factors and their interactions except age and its interaction with ozone and variety (Table 1).
Significantly higher rates of photosynthesis were observed in Kashi Sheetal plants at both ages and in all types of N treatment as compared to Kashi Harittima plants. The plants of both varieties grown in EO3 conditions showed a considerable reduction in the rate of photosynthesis in comparison to plants grown in AO3 conditions. In the vegetative phase, the Kashi Sheetal showed an 11.2, 25.5, and 26.5% increase at AO3 and 8, 24.1, and 27.4% increase at EO3 for N1, N2, and N3 treatments, respectively (Table 2). There was a 12.3, 26.9, and 28% increase at AO3 and a 13, 28, and 30% increase at EO3 for N1, N2, and N3 treatments, respectively of the Kashi Harittima variety in the vegetative phase (Table 2). Similarly in the reproductive phase, the Kashi Sheetal showed a 14.7, 30.3, and 28.4% increase at AO3 and 14.8, 27.8, and 26.5% increase at EO3 for N1, N2, and N3 treatments, respectively (Table 3). There was an 11.5, 24.2, and 26.3% increase at AO3 and a 10.2, 22.7, and 25% increase at EO3 for N1, N2, and N3 treatments, respectively of the Kashi Harittima variety in the reproductive phase (Table 3). After analyzing the degree of percent changes between the N treatments and control in both varieties, the N2 treatment was the most effective treatment in increasing the rate of photosynthesis. According to the four-way ANOVA results, the rate of photosynthesis varied significantly for all individual factors, and some of their interactions such as age*variety, variety*treatment, and age*variety*treatment (Table 1). Similar results were also observed for stomatal gas conductance and internal CO2 concentration in both the varieties of Dolichos lablab under AO3 and EO3 conditions. For both parameters, the Kashi Sheetal variety showed better results in all types of N treatments as compared to Kashi Harittima. The values of stomatal gas conductance and internal CO2 concentration were significantly reduced in EO3 conditions in comparison to AO3 for both varieties at all ages. Stomatal gas conductance and internal CO2 concentration varied significantly for all four individual factors and the interactions of age with ozone, variety, and treatment (Table 1).
Table 2 Effect of N treatment (C, control; N1, recommended N dose; N2, 1.5-times recommended N dose and N3, 2-times recommended N dose) on physiological parameters of Dolichos lablab L. under AO3 at vegetative stage and reproductive stage.
Table 3 Effect of N treatment (C, control; N1, recommended N dose; N2, 1.5-times recommended N dose and N3, 2-times recommended N dose) on physiological parameters of Dolichos lablab L. under EO3 at vegetative stage and reproductive stage. Values are mean ± SE. a) Kashi Sheetal, b) Kashi Harittima.
There was a significant reduction in the weight of seeds plant-1 for both varieties under EO3 conditions as compared to AO3 conditions. For the Kashi Sheetal variety, there was a 20.7,34.5, and 35.4% increase at AO3 and 26.8, 35.8, and 36.8% increase at EO3 for N1, N2, and N3treatments, respectively in comparison to control (Table 4). There was a 22.4, 42.6, and 43.8% increase at AO3 and a 28.7, 40.4, and 41.2% increase at EO3 for N1, N2, and N3 treatments, respectively of the Kashi Harittima variety as compared to control (Table 4). There was a significant reduction in the test weight of 1000 seeds for both varieties under EO3 conditions as compared to AO3 conditions except for the control of both varieties. For the Kashi Sheetal variety, there was a 9.7, 16.1, and 17.3% increase at AO3 and 8.01, 12.4, and 12.9% increase at EO3 for N1, N2, and N3 treatments, respectively in comparison to control. There was a 14.4, 20.2, and 20.6% increase at AO3 and an 18.8, 28.4, and 28.9% increase at EO3 for N1, N2, and N3 treatments, respectively of the Kashi Harittima variety as compared to control (Table 4). The percent change of all treatments was higher for the Kashi Harittima variety in comparison to the Sheetal variety. Only the N2 and N3 treatments of the Harittima variety were significant for the number of seeds plant-1 as compared to the control. All the other values of Sheetal and Harittima varieties for the number of seeds plant-1 were found to be insignificant.
Table 4 Variations in the number of seeds plant-1, wt. of seeds plant-1, and test wt. per 1000 seeds of both varieties of Dolichos lablab L. grown under AO3 and EO3.
Sicard et al. (2017) predicted a rise in O3 concentration under all climate change scenarios and identified South Asia as one of the primary O3 hotspot regions. Intense irradiance, elevated temperature, and low moisture are the ideal circumstances for O3 generation in the Indo-Gangetic plains, where high episodes of O3 are common occurrences (Sarkar and Agrawal, 2010). In monitoring studies conducted at the current experimental site between 2002 - 2012, the concentration of O3 was clearly on the rise (Tiwari and Agrawal, 2018). The results of the present experiment evidently revealed the presence of high ozone episodes during the reproductive phase of both varieties of Dolichos lablab L. The increased O3 levels during the reproductive phase can be reasoned by the high temperature in the months of January, February, and March as compared to November and December months. High O3 concentrations have also been recorded in earlier experiments conducted at the current experimental location during the same time period (Singh et al., 2018; Yadav et al., 2019).
Out of the two varieties of Dolichos lablab L. exposed to ambient and elevated O3 concentrations, Kashi Sheetal had higher endogenously generated H2O2 in its control leaves as compared to Kashi Harittima, as evident through the histochemical assay of H2O2 localization (Figure 4). Related findings have reported that cultivars with higher levels of endogenous H2O2 are more tolerant than cultivars with lower levels of H2O2 (Caregnato et al., 2013; Yadav et al., 2019; Gupta and Tiwari, 2020). Caregnato et al. (2013) reported significantly higher H2O2 localization in the leaves of two varieties of Phaseolus vulgaris L. grown in EO3 conditions in comparison to AO3 conditions. The high H2O2 localization in the leaves is because of the excessive ROS generated due to severe oxidative stress which happens due to elevated O3 fumigation. With an increased dose of nitrogen treatments, there was a significant degree of reduction in the amount of H2O2 localization in the leaves of both varieties (Figure 4). It was reported that H2O2 was produced in the leaves of Cymopsis tetragonoloba L. Taub. (Cluster bean) was significantly decreased with increasing nitrogen amendment doses (Gupta and Tiwari, 2020). It is suggested that the surplus nitrogen available to the plant gets allocated to improve the antioxidative potential of the plants which results in improved scavenging of the H2O2 (Podda et al., 2019). It is noted that N2 treatment was found to be sufficient in the management of O3 stress under both O3 exposure conditions.
Malondialdehyde is an intermediate product that is exclusively produced during the process of membrane lipid peroxidation, so measuring the MDA content gives an approximation of the degree of lipid peroxidation. Biochemical analysis of lipid peroxidation showed higher MDA content in the EO3 conditions in both varieties of Dolichos lablab L. as compared to AO3 at both developmental stages (Figures 5, 6). The findings suggest that greater O3 influx under EO3 conditions resulted in significant membrane damage in both kinds of Indian beans at both development phases. A 90.2% increase in MDA contents in Vigna mungo L. varieties was reported on exposure to EO3 conditions as compared to AO3 conditions (Dhevagi et al., 2021). Since lipid peroxidation is an important marker of O3 stress tolerance (Iglesias et al., 2006), Kashi Sheetal which showed a lesser degree of membrane lipid peroxidation under EO3 conditions as compared to Kashi Harittima, was considered to be more tolerant of the two experimental varieties. The level of MDA content in the leaves of both varieties of Dolichos lablab L. was significantly reduced upon nitrogen treatments at both the O3 concentrations. Similar findings were also reported by Pandey et al. (2018) in which MDA content in the leaves of Triticum aestivum L. was significantly decreased with increased nitrogen amendments. Podda et al. (2019) also found that nitrogen treatment had a beneficial impact on O3-exposed plants, seeing a substantial decrease in MDA and improved membrane stability after nitrogen treatment at both AO3 and EO3 conditions. It was observed that Kashi Sheetal produced a lesser amount of MDA in comparison to the Kashi Harittima, at all the nitrogen treatments during both developmental stages, indicating Kashi Harittima to be was less efficient in scavenging the O3-induced ROS leading to higher membrane disintegration than the Kashi Sheetal upon nitrogen treatments under both O3 exposure conditions. This observation points towards the higher sensitivity of the Kashi Harittima towards ozone stress. Furthermore, there was a negligible difference in the response between the N2 and N3 treatments for both varieties upon both O3 exposure conditions, which specifies the sufficiency of N2 treatment in alleviating O3 stress in the Indian bean. Pearson’s correlation coefficient test has shown a significant negative correlation of MDA contents with the enzymatic activities, suggesting a reduction in membrane lipid peroxidation of O3-stressed plants upon N amendments (Figure 7).
Figure 7 Pearson’s correlation coefficient for physiological and biochemical parameters of two varieties of Hyacinth bean at EO3.
The presence of an efficient antioxidant defense mechanism allows the higher plants to perceive and decipher ROS signals into necessary cellular responses (Yadav et al., 2019). In the current experiment, it was observed that the endogenous level of antioxidants was higher in Kashi Sheetal as compared to Kashi Harittima, at ambient O3 exposure, suggesting high tolerance of O3 stress in Kashi Sheetal. All the antioxidative enzymes showed higher activity under EO3 conditions as compared to AO3 conditions and this applies to both varieties at all developmental stages. Similar findings have also been reported by Pandey et al. (2018) in which the activities of antioxidative enzymes increased under EO3 conditions in two cultivars of Triticum aestivum L. It has been proved that the sustenance and regeneration of the antioxidative enzymatic pool indicate a strengthened defense response capable of O3 detoxification (Caregnato et al., 2013). In the present study, N amendments enhanced the antioxidant activities in both varieties, thereby proving its potential in the management of O3 injury. It was observed that the antioxidative system of O3 sensitive variety Kashi Harittima responded more positively at elevated O3, compared to ambient O3. At elevated O3, Kashi Harittima was capable of sustaining higher increments in the enzyme activities at both developmental stages, which evidently proves that N amendments were more favorable in encountering O3 stress in this variety, as compared to Kashi Sheetal. As per the PCA analysis, it was observed that Kashi Harittima, as the sensitive variety, demonstrated a stronger association of enzymatic anti-oxidants in component 1 as compared with the Kashi Sheetal (Figure 8). In the ascorbate-glutathione pathway, APX is the essential enzyme known for scavenging H2O2 by using ascorbic acid as an electron donor, whereas GR catalyzes glutathione reduction with ascorbate regeneration in plants (Ashraf, 2009). In the present experiment, N amendments intensified the role of APX and GR in inducing the plant’s defense system in both varieties. The higher APX and GR activity can be owed to the greater H2O2 level in EO3 conditions than in AO3, thereby ensuring the scavenging of the H2O2 in order to curtail O3 stress. Under stressful circumstances, Almeselmani et al. (2006) also observed an increment in APX and GR activity in the tolerant wheat cultivars HD77 and HD2817. The lower levels of H2O2 in Kashi Harittima, upon N amendments at elevated O3 treatment, can be explained by higher increments in CAT activity. Upon N amendments, whereas, Kashi Harittima was able to uphold the activities of SOD and CAT, in elevated O3-treated plants, Kashi Sheetal showed lesser increments in the response of these two enzymes, during the reproductive stage. It is to be noted that with a gradual increase in the dose of nitrogen treatments, there was a significant surge in the activities of the antioxidative enzymes in both varieties of Dolichos lablab L. at all growth stages. But the degree of increment of the activities of enzymes was insignificant between N2 and N3 treatments, suggesting the adequacy of N2 treatment in the management of O3 stress.
Figure 8 Principle Component Analysis (PCA) showed the association of considered parameters on two different components; i.e, component 1 and component 2. The parameters studied are APX, GR, SOD, CAT, LPO, Ci, gs, and (A) (a) Association of studied parameters in Kashi Harittima, (B) association of studied parameters in Kashi Sheetal.
O3 exposure is known to adversely affect the physiological parameters of plants, rate of photosynthesis, and stomatal conductance being directly affected (Singh et al., 2014; Tetteh et al., 2016; Dhevagi et al., 2021). This reduced photosynthetic rate caused by elevated ozone may be related to the degradation of the chloroplast structure, which prevents the production of chlorophyll under these conditions (Biswas and Jiang, 2011). In the present study, N supplementation resulted in the improvement of these two parameters in both the varieties of Dolichos lablab, at both O3 exposure conditions, further justifying the potential role of N amendments in depreciating O3 stress. This is further proved by significant correlation coefficient values of different physiological parameters of O3-exposed plants upon N amendments (Tables 2, 3). Increased gs upon N amendments have been reported in wheat (Wall et al., 2000), larch (Mao et al., 2014), cluster bean (Gupta and Tiwari, 2020), etc. A decrease in gs along with an increased A upon N amendments and O3 exposure in poplar has been reported by Zhang et al. (2018). A comparison of the physiological response of both varieties revealed that upon N supplementation, Kashi Harittima showed higher increments in A and gs, in comparison to Kashi Sheetal at all ages under both AO3 and EO3 conditions. Since the O3-induced depreciation of cellular performance largely depends upon O3 flux, which is directly controlled by stomatal movement (Yadav et al., 2019), increased stomatal conductance in O3-exposed plants of Indian bean in the present study, does not account for the reported improvement in the plant’s performance upon N amendments. It has been reported that the application of a higher N dose leads to an increased influx of O3, which may lead to the depletion of the antioxidant pool (Harmens et al., 2017; Marzuoli et al., 2018). Non-significant variations in gs due to O3 x variety and O3 x treatment interactions (Table 1) further prove that gs does not play any substantial role in the ameliorative effect of N amendments in the experimental plants. These observations lead to the conclusion that the upturn in the rate of photosynthesis upon N amendments in O3-exposed plants can be attributed to the enhanced activities of the enzymatic antioxidant pool, thus proving our hypothesis. Increased enzymatic antioxidants upon N implementation, ensure the scavenging of O3-induced oxidative entities, thus rendering protection to photosynthetic machinery in the chloroplasts.
The analysis of yield parameters showed a significant reduction in the EO3 conditions in comparison to AO3 conditions which accounts for both varieties of Dolichos lablab L. at all ages of growth (Table 4). An earlier study found that the yield of selected varieties of wheat (HUW 234 and 468, HD 3086 and 3118) and black gram (CO6 and VBN 1-8) were markedly reduced under EO3 as compared to AO3 (Yadav et al., 2019; Dhevagi et al., 2021). Both varieties of the Indian bean exhibited a significant increase in the weight of grains plant-1 upon all doses of N amendments (N1, N2, and N3). However, the difference in the magnitude of the increase in yield of N2 and N3 was inconsequential for both varieties indicating the sufficiency of N2 treatment in ameliorating oxidative conditions. Interestingly, the O3-sensitive Kashi Harittima variety showed a higher percent increment in the number/weight of seeds plant-1 upon N amendments as compared to Kashi Sheetal (Table 4). Higher enhancement in the antioxidative enzyme activity and greater increments in the rate of photosynthesis explain the higher yield improvements in Kashi Harittima. A previous experiment also reported similar findings in which an O3-sensitive wheat cultivar showed a higher percent increment in response to N amendments as compared to an O3-tolerant wheat cultivar under both AO3 and EO3 conditions (Pandey et al., 2018). The above results prove our first hypothesis that nitrogen treatments assist in strengthening the antioxidative enzyme pool for defense against oxidative stress conditions. In the present study, the analysis of H2O2 localization, antioxidative enzymes, physiological traits, and yield revealed that the Kashi Sheetal variety outperformed the Kashi Harittima variety in every aspect proving the second hypothesis that the differential response of the varieties to varied O3 conditions helps in determining their sensitivity to O3 stress. The more positive response of the O3-sensitive varieties upon N exposure provides a promising feature and can be used for promoting the farming of O3-sensitive varieties, thereby boosting agricultural production.
This experiment justifies the use of N amendments as an effective measure for the management of O3 injury. The higher endogenous levels of H2O2 accumulation and enzymatic antioxidant contents upon O3 exposure have established Kashi Sheetal to be O3 tolerant. The interaction between N amendments and O3 exposure had a more positive effect on O3-sensitive Kashi Harittima, as compared to Kashi Sheetal. Since the increased stomatal conductance upon N fertilization does not restrict the entry of O3 in plants, the higher photosynthetic rate, and subsequently yield were maintained by a stimulated enzymatic antioxidative response in plants at both O3 exposure conditions. The enzymatic response showed significant variations due to ozone and treatment and ozone and variety interactions, whereas the variations of stomatal conductance were insignificant, proving our theory. A more positive response of Kashi Harittima to N supplementation at both O3 exposure conditions can be associated with the sustenance of its higher enzymatic response at the reproductive stage as well, which was more prominent at the EO3 condition. The current study proved that N2 treatment (1.5 times recommended dose) was sufficient to partially ameliorate O3 stress and that larger nitrogen doses may not be more successful in doing so because they did not confer any further perks on the plant’s growth and development. However, more experiments are required to establish a dose-response relationship between N fertilization and O3 exposure doses.
The original contributions presented in the study are included in the article/supplementary material. Further inquiries can be directed to the corresponding author.
Conceptualization, writing-original draft, AS. Data curation, PM. Software, AM. Conceptualization, supervision, ST. All authors contributed to the article and approved the submitted version.
Council of Scientific and Industrial Research, India 09/013(0785)/2018-EMR-I
The authors would like to acknowledge the Head of the Department of Botany, Banaras Hindu University for providing the necessary facilities. The authors would like to thank the Council of Scientific and Industrial Research (CSIR)-HRDG, New Delhi, for providing fellowships.
The authors declare that the research was conducted in the absence of any commercial or financial relationships that could be construed as a potential conflict of interest.
All claims expressed in this article are solely those of the authors and do not necessarily represent those of their affiliated organizations, or those of the publisher, the editors and the reviewers. Any product that may be evaluated in this article, or claim that may be made by its manufacturer, is not guaranteed or endorsed by the publisher.
Aebi, H. (1984). Catalase in vitro. Methods enzymology 105, 121–126. doi: 10.1016/S0076-6879(84)05016-3
Almeselmani, M., Deshmukh, P. S., Sairam, R. K., Kushwaha, S. R., Singh, T. P. (2006). Protective role of antioxidant enzymes under high-temperature stress. Plant Sci. J. 171, 382–388. doi: 10.1016/j.plantsci.2006.04.009
Ashraf, M. (2009). Biotechnological approach of improving plant salt tolerance using antioxidants as markers. Biotechnol. Adv. 27, 84–93. doi: 10.1016/j.biotechadv.2008.09.003
Biswas, D. K., Jiang, G. M. (2011). Differential drought-induced modulation of ozone tolerance in winter wheat species. J. Exp. Bot. 62, 4153–4162. doi: 10.1093/jxb/err104
Caregnato, F. F., Bortolin, R. C., Junior, A. M. D., Moreira, J. C. F. (2013). Exposure to elevated ozone levels differentially affects the antioxidant capacity and the redox homeostasis of two subtropical Phaseolus vulgaris l. varieties. Chemosphere 93, 320–330. doi: 10.1016/j.chemosphere.2013.04.084
Choudhury, F. K., Rivero, R. M., Blumwald, E., Mittler, R. (2017). Reactive oxygen species, abiotic stress and stress combination. Plant J. 90, 856–867. doi: 10.1111/tpj.13299
Davari, S. A., Gokhale, N. B., Palsande, V. N., Kasture, M. C. (2018). Wal (Lablab purpureus l.): An unexploited potential food legumes. Int. J. Chem. Stud. 6, 946–949.
Dhevagi, P., Ramya, A., Priyatharshini, S., Poornima, R. (2021). Effect of elevated tropospheric ozone on vigna mungo l. varieties. Ozone: Sci. Engineering. 44, 1–21.
Duque, L., Poelman, E. H., Steffan-Dewenter, I. (2021). Effects of ozone stress on flowering phenology, plant-pollinator interactions and plant reproductive success. Environ. pollut. 272, 115953. doi: 10.1016/j.envpol.2020.115953
Emberson, L. D., Büker, P., Ashmore, M. R., Mills, G., Jackson, L. S., Agrawal, M., et al. (2009). A comparison of north American and Asian exposure-response data for ozone effects on crop yields. Atmospheric Environ. 43, 1945–1953. doi: 10.1016/j.atmosenv.2009.01.005
Fridovich, I. (1975). Superoxide dismutases. Annu. Rev. Biochem. 44, 147–159. doi: 10.1146/annurev.bi.44.070175.001051
Gupta, G. S., Tiwari, S. (2020). Role of antioxidant pool in management of ozone stress through soil nitrogen amendments in two cultivars of a tropical legume. Funct. Plant Biol. 48, 371–385. doi: 10.1071/FP20159
Harmens, H., Hayes, F., Sharps, K., Mills, G., Calatayud, V. (2017). Leaf traits and photosynthetic responses of betula pendula saplings to a range of ground-level ozone concentrations at a range of nitrogen loads. J. Plant Physiol. 211, 42–52. doi: 10.1016/j.jplph.2017.01.002
Hayes, F., Sharps, K., Harmens, H., Roberts, I., Mills, G. (2020). Tropospheric ozone pollution reduces the yield of African crops. J. Agron. Crop Sci. 206, 214–228. doi: 10.1111/jac.12376
Heath, R. L., Packer, L. (1968). Photoperoxidation in isolated chloroplasts: I. kinetics and stoichiometry of fatty acid peroxidation. Arch. Biochem. biophysics 125, 189–198. doi: 10.1016/0003-9861(68)90654-1
Iglesias, D. J., Calatayud, Á., Barreno, E., Primo-Millo, E., Talon, M. (2006). Responses of citrus plants to ozone: leaf biochemistry, antioxidant mechanisms, and lipid peroxidation. Plant Physiol. Biochem. 44, 125–131. doi: 10.1016/j.plaphy.2006.03.007
Letting, F. K., Venkataramana, P. B., Ndakidemi, P. A. (2021). Breeding potential of lablab [Lablab purpureus (L.) sweet]: a review on characterization and bruchid studies towards improved production and utilization in Africa. Genet. Resour. Crop Evol. 68, 3081–3101. doi: 10.1007/s10722-021-01271-9
Liu, Y. M., Shahed-Al-Mahmud, M., Chen, X., Chen, T. H., Liao, K. S., Lo, J. M., et al. (2020). Carbohydrate-binding protein from the edible lablab beans effectively blocks the infections of influenza viruses and SARS-CoV-2. Cell Rep. 32, 108016. doi: 10.1016/j.celrep.2020.108016
Mao, Q., Watanabe, M., Makoto, K., Kita, K., Koike, T. (2014). High nitrogen deposition may enhance growth of a new hybrid larch F1 growing at two phosphorus levels. Landscape Ecol. Eng. 10, 1–8. doi: 10.1007/s11355-012-0207-2
Marzuoli, R., Monga, R., Finco, A., Chiesa, M., Gerosa, G. (2018). Increased nitrogen wet deposition triggers negative effects of ozone on the biomass production of Carpinus betulus l. young trees. Environ. Exp. Bot. 152, 128–136. doi: 10.1016/j.envexpbot.2017.10.017
Meehl, G. A., Tebaldi, C., Tilmes, S., Lamarque, J. F., Bates, S., Pendergrass, A., et al. (2018). Future heat waves and surface ozone. Environ. Res. Lett. 13 (6), 064004. doi: 10.1088/1748-9326/aabcdc
Mills, G., Wagg, S., Harmens, H. (2013). Ozone pollution: impacts on ecosystem services and biodiversity. Centre Ecol. Hydrology.
Mukherjee, A., Hazra, S. (2022). “Impact of elevated CO2 and O3 on field crops and adaptive strategies through agro-technology,” in Response of field crops to abiotic stress (CRC Press), 177–190.
Nakano, Y., Asada, K. (1981). Hydrogen peroxide is scavenged by ascorbate-specific peroxidase in spinach chloroplasts. Plant Cell Physiol. 22, 867–880.
Pandey, A. K., Ghosh, A., Agrawal, M., Agrawal, S. B. (2018). Effect of elevated ozone and varying levels of soil nitrogen in two wheat (Triticum aestivum l.) cultivars: Growth, gas exchange, antioxidant status, grain yield and quality. Ecotoxicology Environ. Saf. 158, 59–68. doi: 10.1016/j.ecoenv.2018.04.014
Picchi, V., Monga, R., Marzuoli, R., Gerosa, G., Faoro, F. (2017). The ozone-like syndrome in durum wheat (Triticum durum desf.): Mechanisms underlying the different symptomatic responses of two sensitive cultivars. Plant Physiol. Biochem. 112, 261–269. doi: 10.1016/j.plaphy.2017.01.011
Podda, A., Pisuttu, C., Hoshika, Y., Pellegrini, E., Carrari, E., Lorenzini, G., et al. (2019). Can nutrient fertilization mitigate the effects of ozone exposure on an ozone-sensitive poplar clone? Sci. Total Environ. 657, 340–350. doi: 10.1016/j.scitotenv.2018.11.459
Rana, R., Sayem, A. S. M., Sabuz, A. A., Rahman, M., Hos-Sain, A. (2021). Effect of lablab bean (Lablab purpureus l.) seed flour on the physicochemical and sensory properties of biscuits. Int. J. Food Sci. Agric. 5, 52–57. doi: 10.26855/ijfsa.2021.03.008
Sahoo, A., Tiwari, S. (2021). Role of soil nitrogen amendments in management of ozone stress in plants: a study of the mechanistic approach. J. Emerging Technol. Innovative Res. 8, 739–755.
Sarkar, A., Agrawal, S. B. (2010). Elevated ozone and two modern wheat cultivars: an assessment of dose dependent sensitivity with respect to growth, reproductive and yield parameters. Environ. Exp. Bot. 69, 328–337. doi: 10.1016/j.envexpbot.2010.04.016
Sicard, P., Anav, A., De Marco, A., Paoletti, E. (2017). Projected global ground-level ozone impacts on vegetation under different emission and climate scenarios. Atmospheric Chem. Phys. 17, 12177–12196. doi: 10.5194/acp-17-12177-2017
Singh, A. A., Agrawal, S. B., Shahi, J. P., Agrawal, M. (2014). Assessment of growth and yield losses in two Zea mays l. cultivars (quality protein maize and non-quality protein maize) under projected levels of ozone. Environ. Sci. pollut. Res. 21, 2628–2641. doi: 10.1007/s11356-013-2188-6
Singh, A. A., Fatima, A., Mishra, A. K., Chaudhary, N., Mukherjee, A., Agrawal, M., et al. (2018). Assessment of ozone toxicity among 14 Indian wheat cultivars under field conditions: growth and productivity. Environ. Monit. Assess. 190, 1–14. doi: 10.1007/s10661-018-6563-0
Stella, P., Personne, E., Lamaud, E., Loubet, B., Trebs, I., Cellier, P. (2013). Assessment of the total, stomatal, cuticular, and soil 2-year ozone budgets of an agricultural field with winter wheat and maize crops. J. Geophys. Res. Biogeosci. 118, 1120–1132. doi: 10.1002/jgrg.20094
Tetteh, R., Yamaguchi, M., Izuta, T. (2016). Effect of ambient levels of ozone on photosynthetic components and radical scavenging system in leaves of African cowpea varieties. Afr. Crop Sci. J. 24, 127–142. doi: 10.4314/acsj.v24i2.2
Thordal-Christensen, H., Zhang, Z., Wei, Y., Collinge, D. B. (1997). Subcellular localization of H2O2 in plants. H2O2 accumulation in papillae and hypersensitive response during the barley–powdery mildew interaction. Plant J. 11, 1187–1194. doi: 10.1046/j.1365-313X.1997.11061187.x
Tiwari, S., Agrawal, M. (2018). Tropospheric ozone and its impacts on crop plants Vol. 1007 (Springer International Publishing), 978–973.
Van Dingenen, R., Dentener, F. J., Raes, F., Krol, M. C., Emberson, L., Cofala, J. (2009). The global impact of ozone on agricultural crop yields under current and future air quality legislation. Atmos. Environ. 43, 604–618. doi: 10.1016/j.atmosenv.2008.10.033
Wall, G. W., Adam, N. R., Brooks, T. J., Kimball, B. A., Pinter, P. J., LaMorte, R. L., et al. (2000). Acclimation response of spring wheat in a free-air CO2 enrichment (FACE) atmosphere with variable soil nitrogen regimes. 2. net assimilation and stomatal conductance of leaves. Photosynthesis Res. 66, 79–95. doi: 10.1023/A:1010646225929
Wang, C., Lin, J., Niu, Y., Wang, W., Wen, J., Lv, L., et al. (2022). Impact of ozone exposure on heart rate variability and stress hormones: A randomized-crossover study. J. Hazardous Materials 421, 126750. doi: 10.1016/j.jhazmat.2021.126750
Yadav, D. S., Rai, R., Mishra, A. K., Chaudhary, N., Mukherjee, A., Agrawal, S. B., et al. (2019). ROS production and its detoxification in early and late sown cultivars of wheat under future O3 concentration. Sci. Total Environ. 659, 200–210. doi: 10.1016/j.scitotenv.2018.12.352
Yendrek, C. R., Leisner, C. P., Ainsworth, E. A. (2013). Chronic ozone exacerbates the reduction in photosynthesis and acceleration of senescence caused by limited n availability in Nicotiana sylvestris. Global Change Biol. 19, 3155–3166. doi: 10.1111/gcb.12237
Zeng, J., Sheng, H., Liu, Y., Wang, Y., Wang, Y., Kang, H., et al. (2017). High nitrogen supply induces physiological responsiveness to long photoperiod in barley. Front. Plant Sci. 8, 569. doi: 10.3389/fpls.2017.00569
Zhang, L., Hoshika, Y., Carrari, E., Cotrozzi, L., Pellegrini, E., Paoletti, E. (2018). Effects of nitrogen and phosphorus imbalance on photosynthetic traits of poplar Oxford clone under ozone pollution. J. Plant Res. 131, 915–924. doi: 10.1007/s10265-018-1071-4
Keywords: nitrogen amendments, ozone, antioxidants, stomatal conductance, dolichos
Citation: Sahoo A, Madheshiya P, Mishra AK and Tiwari S (2023) Combating ozone stress through N fertilization: A case study of Indian bean (Dolichos lablab L.). Front. Plant Sci. 14:1125529. doi: 10.3389/fpls.2023.1125529
Received: 16 December 2022; Accepted: 07 February 2023;
Published: 22 February 2023.
Edited by:
Hirofumi Saneoka, Hiroshima University, JapanReviewed by:
Amit Kumar Mishra, Mizoram University, IndiaCopyright © 2023 Sahoo, Madheshiya, Mishra and Tiwari. This is an open-access article distributed under the terms of the Creative Commons Attribution License (CC BY). The use, distribution or reproduction in other forums is permitted, provided the original author(s) and the copyright owner(s) are credited and that the original publication in this journal is cited, in accordance with accepted academic practice. No use, distribution or reproduction is permitted which does not comply with these terms.
*Correspondence: Supriya Tiwari, c3Vwcml5YWJodUBnbWFpbC5jb20=
Disclaimer: All claims expressed in this article are solely those of the authors and do not necessarily represent those of their affiliated organizations, or those of the publisher, the editors and the reviewers. Any product that may be evaluated in this article or claim that may be made by its manufacturer is not guaranteed or endorsed by the publisher.
Research integrity at Frontiers
Learn more about the work of our research integrity team to safeguard the quality of each article we publish.