- Instituto de Biología Molecular y Celular de Plantas (Consejo Superior de Investigaciones Científicas-Universidad Politécnica de Valencia), Ciudad Politécnica de la Innovación, Valencia, Spain
The SUPERMAN (SUP) gene was described in Arabidopsis thaliana over 30 years ago. SUP was classified as a cadastral gene required to maintain the boundaries between reproductive organs, thus controlling stamen and carpel number in flowers. We summarize the information on the characterization of SUP orthologs in plant species other than Arabidopsis, focusing on the findings for the MtSUP, the ortholog in the legume Medicago truncatula. M. truncatula has been widely used as a model system to study the distinctive developmental traits of this family of plants, such as the existence of compound inflorescence and complex floral development. MtSUP participates in the complex genetic network controlling these developmental processes in legumes, sharing conserved functions with SUP. However, transcriptional divergence between SUP and MtSUP provided context-specific novel functions for a SUPERMAN ortholog in a legume species. MtSUP controls the number of flowers per inflorescence and the number of petals, stamens and carpels regulating the determinacy of ephemeral meristems that are unique in legumes. Results obtained in M. truncatula provided new insights to the knowledge of compound inflorescence and flower development in legumes. Since legumes are valuable crop species worldwide, with high nutritional value and important roles in sustainable agriculture and food security, new information on the genetic control of their compound inflorescence and floral development could be used for plant breeding.
Introduction
Most angiosperm flowers are organized in four concentric whorls: sepals (W1), petals (W2), stamens (W3) and carpel/s (W4), (Smyth et al., 1990). The number of floral organs and the placement of the organs within each whorl are genetically determined and MADS-box floral homeotic genes play a crucial role in the specification of floral organ identity (Bowman et al., 2012). Other classes of genes, the ones that determine the boundaries of different cell identities, are also crucial players during floral development (Yu and Huang, 2016). They were classified as “cadastral genes”, to which the Arabidopsis SUPERMAN (SUP) gene was assigned (Bowman et al., 1992; Sakai et al., 1995).
SUP is a transcriptional repressor, extensively studied in Arabidopsis thaliana, that encodes a plant-specific EPF-like protein with one Cys2-His2 zinc finger DNA binding domain and a C-terminus EAR-like (DLELRL) motif (Sakai et al., 1995; Hiratsu et al., 2002; Hiratsu et al., 2003; Hiratsu et al., 2004). The specific expression of SUP at the boundary between W3 and W4 (Sakai et al., 1995; Prunet et al., 2017) led to its classification as a cadastral gene specifying the stamens-carpel boundary. The supernumerary male organs at the expense of the female one of the sup-1 mutant (Figure 1A) (Bowman et al., 1992) was initially associated with the expansion of the MADS-box genes APETALA3 (AP3) and PISTILLATA (PI) expression closer to the centre of the floral meristem (Sakai et al., 1995; Prunet et al., 2017). Initially, models to explain the SUP function were based on a single allele: sup-1 (flo-10) (Bowman et al., 1992; Sakai et al., 1995; Prunet et al., 2017). However, the study of other sup alleles displaying phenotypes deviating from sup-1 (Figure 1A) has shed light on the SUP functions (Breuil-Broyer et al., 2016; Prunet et al., 2017; Xu et al., 2018). SUP is a gene controlling the stamens-carpel boundary setting and is linked to floral meristem termination (FMT) at the early stages of flower development.
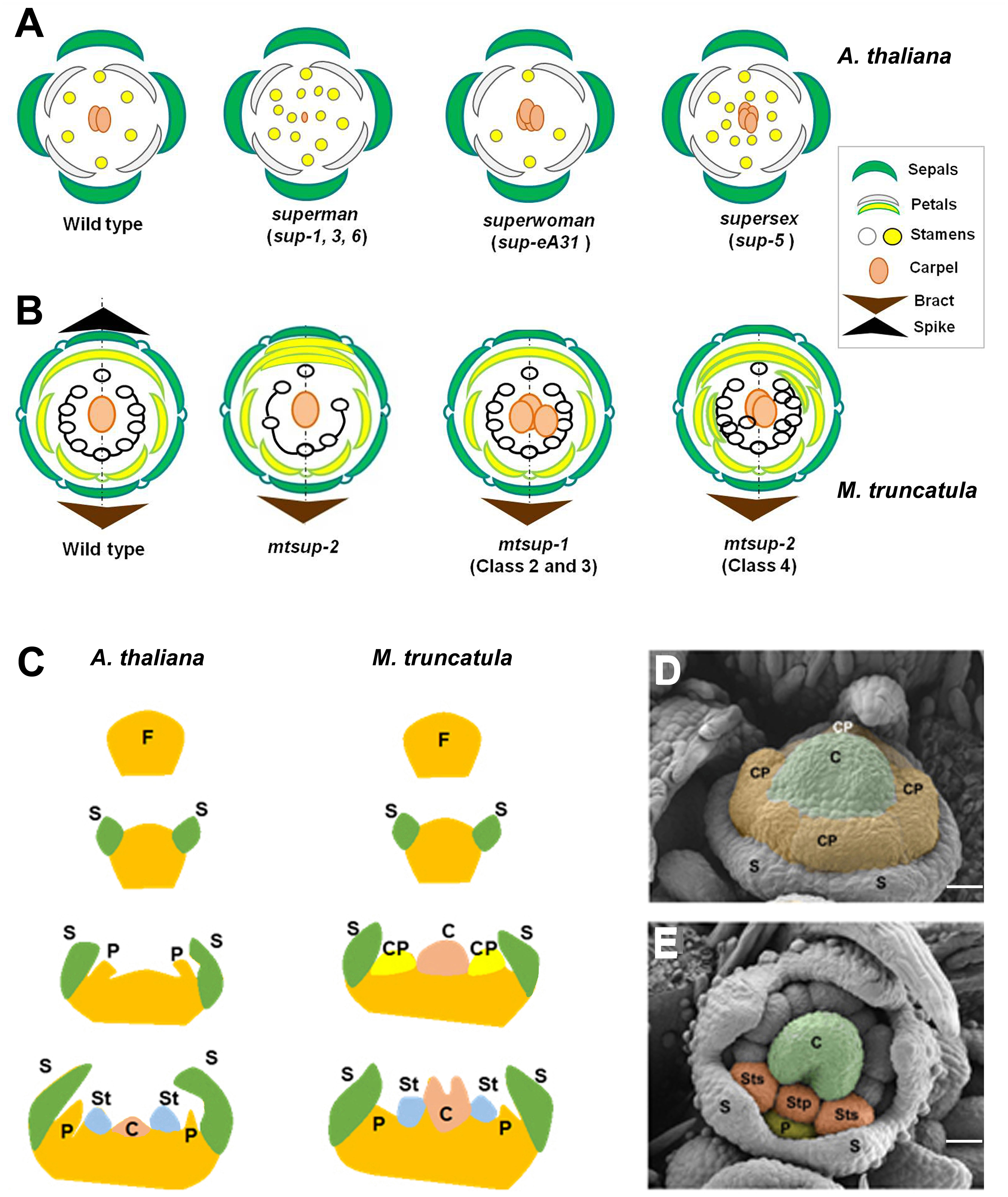
Figure 1 Comparative floral development of Arabidopsis thaliana and Medicago truncatula. (A) Comparative schematic representation among superman (sup) mutant alleles in Arabidopsis regarding floral organ number. Wild type A. thaliana flower: 4 sepals, 4 petals, 6 stamens and two fused carpels. (B) Comparative schematic representation among superman (mtsup) mutant alleles in Medicago regarding floral organ number. Wild type M. truncatula flower: 5 sepals, 5 petals, 10 stamens, 9 fused (staminal tube) and one free, and a single carpel. In Arabidopsis, the ‘superman’ class of mutants harbors supernumerary stamens and reduced or absent carpel, resembling mtsup-2 showing additional petals at the expense of stamens. An increased number of carpels characterize the “superwoman” class. Similar phenotypes displayed the mtsup-1 (class 2 and 3) alleles, with two or three carpels in M. truncatula. The “supersex” class, to which sup-5 allele belong, produces more stamens and additional carpels. This phenotype is observed in mtsup-1 allele (class 4). Also, additional petals are produced by this allele. (C) Left. In A. thaliana organ differentiation is centripetal and sequential. First are differentiated the sepal primordia, then the petal primordia, the stamen primordia and finally the carpel primordium. Right. In M. truncatula, the four common primordia differentiate petals and stamens in W2 and W3 respectively. (D) Floral meristem of M. truncatula showing the early carpel primordium (C, green) in the centre, the four common primordia (CP, orange) and the sepal primordia (S). (E) Each common primordium differentiates petals (P, yellow) in W2 and antepetal (Stp) and antesepal (Sts) stamens in W3 (orange). VM, vegetative meristem; FM, floral meristem; I1, primary inflorescence meristem; I2, secondary inflorescence meristem; spk, spike; S, sepal primordium; CP, common primordium; P, petal primordium; St, stamen primordium; C, carpel primordium. Scale bars, 25 μm in (D, E) Adapted from Benlloch et al., 2003; Breuil-Broyer et al., 2016; Prunet et al., 2017 and Rodas et al., 2021.
It has been described that SUP is required for the correct timing to turn off WUSCHEL (WUS) from the floral meristem centre (FMC), thus controlling the floral meristem termination. WUS activity is required for the stem cell division at the floral meristem centre, a prolonged expression of WUS would lead to a delayed floral meristem termination, and more floral organs could be produced. However, SUP and WUS do not show an overlapping spatial expression and, in sup mutants, WUS expression is prolonged (Prunet et al., 2017; Xu et al., 2018). Moreover, SUP contributes to carpel medial region formation and the tissues derived from this region (Breuil-Broyer et al., 2016).
These studies provided new information to generate different models to explain SUP functions. One of the models proposes that SUP indirectly promotes floral meristem termination by repressing B-class genes. This model explains the different sup alleles phenotypes (Figure 1A), showing an indistinct male-female boundary and a sporadic carpel development (sup-1). By contrast, the increased number of stamens and carpels in the sup-5 mutant (Figure 1A) supports a second model that proposes that SUP controls the balance of cell proliferation and differentiation at W3 and W4 (Breuil-Broyer et al., 2016). To this regard, the effect of the overexpression of SUP-like genes supports the activity of SUP as a cell proliferation control gene (Nandi et al., 2000; Bereterbide et al., 2001; Hiratsu et al., 2002; Kazama et al., 2009; Nibau et al., 2011; Zhao et al., 2014). Recent studies demonstrated that SUP regulates both stem cell proliferation in the floral meristem and floral organogenesis through fine-tuning auxin biosynthesis (Xu et al., 2018). This mechanism might explain all sup mutant phenotypes (Figure 1A). Studies in SUP have shown its broad spectrum of action, highlighting how different are the floral phenotypes according to the type of mutation (Bowman et al, 2012; Breuil-Broyer et al, 2016).
Compound inflorescence and floral development in legumes. Distinctive traits
In addition to the well-known capacity to fix nitrogen symbiotically, some distinctive features of legumes are the presence of compound leaves and inflorescences and a complex floral development (Ferrándiz et al., 1999; Singer et al., 1999; Benlloch et al., 2003). All these traits make them of interest for the study of unique developmental processes (Hofer and Ellis, 2014; Cañas and Beltrán, 2018).
Most legumes show complex raceme inflorescences with more than one branching. In the model legume Medicago truncatula the primary inflorescence meristem (I1) differentiates a secondary inflorescence meristem (I2). The existence of the I2 is linked to the compound inflorescence development and is a distinctive feature compared to Arabidopsis, which produces a unique inflorescence meristem (IM) before differentiating the floral meristem (FM) (Tucker, 2003; Benlloch et al., 2007). The I2 is a transient meristem, and its identity is given by a genetic function of VEGETATIVE1 (VEG1) in Pisum sativum and MtFRUITFULLc (MtFULc) in M. truncatula (Cheng et al., 2018). It has been proposed that this function was derived from the sub-functionalization of the AGL79 MADS-box gene clade within the AP1/SQUA/FUL family (Berbel et al., 2012). The perpetual activity of the I2 meristem will define the number of flowers per inflorescence and its termination as a residual vegetative organ (stub or spike) in the legume compound inflorescences (Benlloch et al., 2003; Benlloch et al., 2015). In the model legume M. truncatula, the identity of the I1 and FM, also involved in this developmental process, are specified by MtTERMINAL FLOWER1 (MtTFL1) and MtAPETALA1 (MtAP1) or MtPROLIFERATING INFLORESCENCE MERISTEM (MtPIM), respectively (Benlloch et al., 2006; Cheng et al., 2018). Their spatial and temporal expression and mutual repression control the compound inflorescence development in M. truncatula (Cheng et al., 2018).
The wild type flower of M. truncatula (Figure 1B) displays pentamerous floral organs per whorl: five sepals in W1, five petals in W2 (a keel petal formed by two fused petals, two wing petals and one standard or vexillum), 10 stamens in W3 (nine fused in a staminal tube and one free) and a single carpel in W4 (Benlloch et al., 2003; Cañas and Beltrán, 2018). In contrast to Arabidopsis, organ differentiation shows a high degree of spatial and temporal overlapping. Even more characteristic is the presence of common primordia (CP), ephemeral meristems from which petals and stamens will differentiate, and the early carpel differentiation (Ferrándiz et al., 1999; Tucker, 2003; Benlloch et al., 2003; Roque et al., 2018). The model species A. thaliana (Figure 1C left) shows a centripetal and sequential organ differentiation. First, the sepal primordia are differentiated, then the petal primordia, followed by the stamen primordia, and finally, the carpel primordium. In contrast, M. truncatula (Figure 1C right) shows unidirectional differentiation of the organ primordia with a high degree of overlapping. Unique differences are the presence of four common primordia and the early carpel primordium differentiation (Figures 1D, E). Despite the functional divergence of the duplicated floral homeotic MADS-box genes in M. truncatula, the specification of the floral organs is conserved in this model legume (Roque et al., 2018).
MtSUPERMAN: Conserved and new functions controlling compound inflorescence and floral development
The SUP gene has been widely studied in A. thaliana. However, there is scant information on the role of SUP orthologs in other plant species. The petunia PhSUP gene (Nakagawa et al., 2004) had been the only SUP ortholog functionally characterized on its own species until it was studied in the model legume M. truncatula (Rodas et al., 2021). Recently, the SMALL REPRODUCTIVE ORGANS (SRO) gene was described as the SUP ortholog in rice (Xu et al., 2022).
The results obtained from the functional characterization of MtSUP in Medicago (Rodas et al., 2021) uncovered new context-specific functions in a different plant species. This information may have changed not only the previously proposed idea of SUPERMAN as a boundary gene but also made MtSUP a key player of the complex regulatory network behind the compound inflorescence development, being an undescribed function for a SUP ortholog in eudicots. Nevertheless, there are also similarities between MtSUP and other SUP orthologs regarding flower development.
The floral phenotypes of mtsup mutants (Figure 1B) are different to Arabidopsis, Petunia and rice mutants in several respects. However, sup, phsup and mtsup mutants have in common the increase in the numbers of both stamens and carpels in their respective flowers. Thus, the early floral meristem function of SUP is conserved in these three species (Nakagawa et al., 2004; Rodas et al., 2021). However, the rice SUP ortholog controls the size of male and female organs but not their number (Xu et al., 2022).
MtSUP transcript is firstly detected in the I2 meristem and later in the FM (Figures 2A, B). The expression pattern of MtSUP during floral organogenesis (Rodas et al., 2021) showed that even before the carpel primordium is initiated MtSUP transcript is already detected in the floral meristem centre and later in the common primordia (Figure 2C). The proliferation of extra petals was a distinctive feature discovered for the mtsup mutants during floral development (Figure 1B). At the common primordia, the meristematic cells that will produce petals and stamens coexist, and a given number of meristematic cells will give place to the organ primordia (Bossinger and Smyth, 1996).
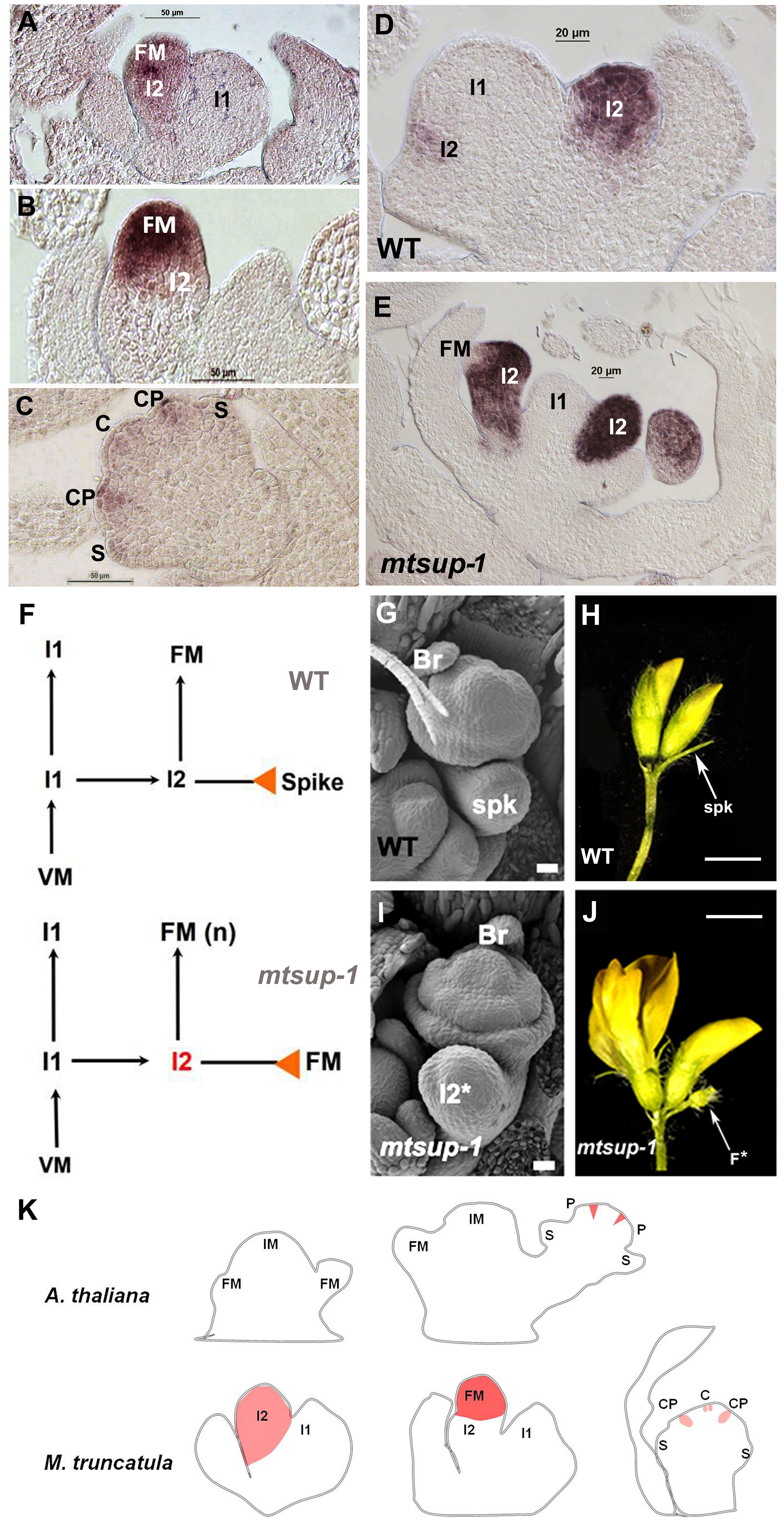
Figure 2 MtSUP controls compound inflorescence development in M. truncatula. (A) MtSUP transcript is firstly detected in the I2. (B) Later on MtSUP activity is detected in the FM. (C) During early floral development MtSUP expression is detected in the common primordia (CP). (D) MtFULc expression in the wild type (WT) flower. (E) MtFULc expression in the mtsup-1 mutant. MtFULc transcript occupies a wider area in MtSUP mutants compared to the WT. (F) Schematic representation of the compound inflorescence development in Medicago with the formation of an I2 and the terminal spike in the WT and a new FM instead the spike in the mtsup-1 mutant. (G) SEM image of a WT floral primordium with its respective bract and spike. (H) The WT of M. truncatula R108 produces one or two flowers per inflorescence and terminates in a spike. (I) In mtsup-1, the I2* (future spike) acquires floral identity. (J) In the mtsup-1 mutant the residual cells of the I2 terminate as a new flower (F*) instead a spike. (K) Comparative schematic representation of SUP and MtSUP expression patterns during inflorescence and flower development in Arabidopsis and Medicago. SUP and MtSUP are orthologs that have functionally diverged through changes in their gene transcription patterns. IM, inflorescence meristem; I1, primary inflorescence meristem; I2, secondary inflorescence meristem; FM, floral meristem; CP, common primordium; S, sepal primordium; P, petal primordium; C, carpel primordium; Br, bract; Spk, spike; F*, new flower. Scale bars, 20 μm in (G, I), and 2 mm in (H, J) Adapted from Rodas et al. (2021).
As a role already described for SUP, MtSUP might control cell proliferation in the common primordia. In other words, MtSUP is involved in the determinacy of the common primordia as prolonged maintenance of these meristematic cells can give rise to extra organs (Bowman et al., 1989; Bossinger and Smyth, 1996). The supernumerary petals, stamens and carpels in mtsup mutants (Figure 1B) might also be explained by a delayed floral meristem termination linked to MtWUS persistence, as also occurs for WUS in sup mutants of A. thaliana (Prunet et al., 2017; Xu et al., 2018). MtWUS expression is not detected in the wild type after the floral apex flattens (Rodas et al., 2021). This is consistent with the early carpel initiation in legumes (Ferrándiz et al., 1999), as floral meristem termination happens when the pool of stem cells of the floral meristem centre is set to a female fate (Prunet et al., 2009). In mtsup-1, the expression of MtWUS is prolonged, thus the pool of stem cells remains undifferentiated during more time at the floral meristem centre. Contrary to SUP, which is not expressed in the floral meristem centre and plays a non-cell-autonomous function there (Prunet et al., 2017). MtSUP and MtWUS expression overlaps, both spatially and temporally at the I2 and the floral meristem centre, which would allow them to interact physically (Rodas et al., 2021). MtSUP has a novel function in the common primordia determinacy and seems to conserve its cell antiproliferative role in this unique feature of legumes.
A proper carpel primordium formation requires a correct floral meristem termination (Sakai et al., 2000; Prunet et al., 2017). MtSUP is expressed in the carpel marginal tissue that will develop the parietal placenta. It agrees with the defects in the marginal derived tissues of the gynoecium in mtsup mutants. Defects in placenta morphogenesis were also observed in the Petunia phsup mutants (Nakagawa et al., 2004), and the strong sup-5 mutant (Figure 1A) of A. thaliana (Gaiser et al., 1995). Therefore, the SUP orthologs PhSUP and MtSUP are required for proper floral meristem termination and the correct development of the carpel marginal tissues (Nakagawa et al., 2004; Rodas et al., 2021). Common aberrancies in the development of the placenta impacted ovule development in mtsup mutants, reducing fertility. Similar phenotypes were reported for Arabidopsis sup-5 (Gaiser et al., 1995) and Petunia phsup mutants (Nakagawa et al., 2004). Thus, the late floral function of SUP controlling ovule development is conserved in these three species (Gaiser et al., 1995; Nakagawa et al., 2004; Breuil-Broyer et al., 2016; Rodas et al., 2021).
Unlike Arabidopsis, MtSUP was first detected in the whole I2 (Figures 2A, B), similar to the expression of the I2 identity gene MtFULc (Figures 2D, E). This expression matched with the multiflowered phenotype in mtsup mutants, assigning MtSUP a determinant role in controlling the maturation rate of the I2. This novel function has not been described for any SUP-like gene. In M. truncatula cv.R108, the I2 derived from the I1 divides to produce one or two floral meristems (Benlloch et al., 2003). The I2 is a transient state between the vegetative and the reproductive tissue that remains immature until the floral identity acquisition (Prusinkiewicz et al., 2007). After producing a floral meristem, the remaining cells of the I2 enter senescence and produce the spike (Tucker, 1989; Benlloch et al., 2007). In mtsup mutants, the I2 gives rise to more floral meristems than the wild type because the residual cells of the I2 terminate as a floral meristem instead of a spike (Figures 2F–J). The I2 determinacy could also be linked to the gradual turn-off of MtWUS in the I2. As MtWUS prolongs its expression, the I2 could extend its activity in mtsup mutants, or MtSUP could influence the I2 activity by controlling cell proliferation. MtFULc transcript occupies a broader area in mtsup mutants compared to the wild type (Figures 2D, E), and there might be more cells expressing MtFULc. According to the expression analysis, MtSUP also seems to restrict MtPIM expression to the floral meristem and this restriction could be considered another way to control the determinacy of the I2. In mtsup mutants, MtPIM invades the expression domain of MtFULc in the I2 and the remnant cells that lose their vegetative nature and acquire floral identity (Rodas et al., 2021).
SUPERMAN was classified as a “cadastral gene” after studying the sup-1 (flo10) allele (Bowman et al., 1992). However, the results obtained in Medicago might support that the conserved ancestral function of SUP-like genes is the control of cell proliferation rather than a cadastral function. MtSUP does not have a typical boundary expression pattern since it is expressed in the whole I2 and floral meristem. This transcript localization correlates with the floral and inflorescence phenotypes of mtsup-1 mutant. Thus, the model that proposes the balance of cell proliferation explains mtsup mutants better than the model that considers that SUP is related to the repression of B-class MADS-box genes to the floral meristem centre. Indeed, MtPI expression (Roque et al., 2018) in mtsup-1 expands towards the W1 instead of expanding to the floral meristem centre (Rodas et al., 2021). In both flower and inflorescence development, there is no need to invoke a boundary function to explain mtsup mutants. Certainly, the phenotypic consequences of sup mutations in Arabidopsis are correlated to an over-proliferation of cells at W3 and the floral stem cells at the floral meristem centre (Prunet et al., 2009; Prunet et al., 2017; Xu et al., 2018). In addition, in P. hybrida the specific expression of PhSUP in the stamen primordia and the excessive proliferation of cells at the connective tissue in phsup1 anthers suggest that the control of cell division and growth is the function of this SUP ortholog (Nakagawa et al., 2004). Similar conclusions were reached with the SUP ortholog in rice. The authors stated that SRO is not a cadastral gene based on its expression pattern and the mechanisms through which SRO regulates reproductive organ development (Xu et al., 2018).
From an evolutionary point of view, SUP and MtSUP are orthologs that have functionally diverged through changes in their gene transcription patterns while keeping some common functions (Figure 2K). Such changes can occur through transposition, rearrangement, duplication or point mutations in the regulatory regions (Carroll, 2005), which are frequent after whole-genome duplications (WGD) events, a common phenomenon in the evolution of angiosperms (Cui et al., 2006). The WGD event that pre-dated the speciation of legumes ~50–60 million years ago had an essential role in structuring the M. truncatula genome and in the success of papilionoid legumes (Cannon et al., 2006). However, these rounds of polyploidization have contributed mainly to the gradual decline in the conserved synteny between species, as is the case for Arabidopsis and M. truncatula (Young et al., 2011). An example is the absence of collinearity in the flanking regions of MtSUP and SUP in their respective genomes (Rodas et al., 2021).
The functional study of SUP orthologous genes in other legume species (alfalfa, common bean) or plants with complex inflorescence (i.e. tomato, mustard) could help to understand SUP-like genes implications in the development of higher order meristems (i.e. I2). Alternatively, they could show the emergence of new functions for transcription factors when they are expressed in species with different architectures. Recently, the SINGLE FLOWER (SFL) gene, a MYB transcription factor expressed in the I2, was shown to perform a similar role to MtSUP in chickpea (Caballo et al., 2022). Multiflowered pea and chickpea mutants have been reported for three decades (Murfet, 1985; Singer et al., 1999; Gaur and Gour, 2002; Srinivasan et al., 2006; Devi et al., 2018). However, the correlation between the multiflowered mutants and the genes responsible requires further studies. The genes involved in the specification and determinacy of inflorescence meristems could be used as bioengineering tools to optimize inflorescence traits (Wang et al., 2021). In line with this, SUP-like genes in other crops should be studied to determine their possible roles in inflorescence development.
Author contributions
ER, AR and LC wrote the manuscript and RH, CG-M and JB contributed with valuable comments during the manuscript writing. All authors contributed to the article and approved the submitted version.
Funding
This work was supported by a grant from the Spanish Ministry of Science and Innovation (PID2019-106060RB-I00). AR acknowledges a Santiago Grisolía fellowship (GRISOLIA 2017/168) from the Generalitat Valenciana.
Acknowledgments
We want to thank John Wiley and Sons and The Plant Journal by the license (5444160987798) to reproduce some figure panels previously published in Rodas et al. (2021). We acknowledge the support of the publication fee by the CSIC Open Access Publication Support Initiative through its Unit of Information Resources for Research (URICI).
Conflict of interest
The authors declare that the research was conducted in the absence of any commercial or financial relationships that could be construed as a potential conflict of interest.
Publisher’s note
All claims expressed in this article are solely those of the authors and do not necessarily represent those of their affiliated organizations, or those of the publisher, the editors and the reviewers. Any product that may be evaluated in this article, or claim that may be made by its manufacturer, is not guaranteed or endorsed by the publisher.
References
Benlloch, R., Berbel, A., Ali, L., Gohari, G., Millán, T., Madueño, F. (2015). Genetic control of inflorescence architecture in legumes. Front. Plant Sci. 6, 1–14. doi: 10.3389/fpls.2015.00543
Benlloch, R., Berbel, A., Serrano-Mislata, A., Madueño, F. (2007). Floral initiation and inflorescence architecture: A comparative view. Ann. Bot. 100, 659–676. doi: 10.1093/aob/mcm146
Benlloch, R., D’Erfurth, I., Ferrandiz, C., Cosson, V., Beltrán, J. P., Cañas, L. A., et al. (2006). Isolation of mtpim proves Tnt1 a useful reverse genetics tool in Medicago truncatula and uncovers new aspects of AP1-like functions in legumes. Plant Physiol. 142, 972–983. doi: 10.1104/pp.106.083543
Benlloch, R., Navarro, C., Beltrán, J. P., Cañas, L. A. (2003). Floral development of the model legume Medicago truncatula: Ontogeny studies as a tool to better characterize homeotic mutations. Sexual Plant Reprod. 15, 231–241. doi: 10.1007/s00497-002-0157-1
Berbel, A., Ferrándiz, C., Hecht, V., Dalmais, M., Lund, O. S., Sussmilch, F. C., et al. (2012). VEGETATIVE1 is essential for development of the compound inflorescence in pea. Nat. Commun. 3, 797. doi: 10.1038/ncomms1801
Bereterbide, A., Hernould, M., Castera, S., Mouras, A. (2001). Inhibition of cell proliferation, cell expansion and differentiation by the arabidopsis SUPERMAN gene in transgenic tobacco plants. Planta 214, 22–29. doi: 10.1007/s004250100584
Bossinger, G., Smyth, D. R. (1996). Initiation patterns of flower and floral organ development in Arabidopsis thaliana. Development 122, 1093–1102. doi: 10.1242/dev.122.4.1093
Bowman, J. L., Sakai, H., Jack, T., Weigel, D., Mayer, U., Meyerowitz, E. M. (1992). SUPERMAN, a regulator of floral homeotic genes in arabidopsis. Development 114, 599–615. doi: 10.1242/dev.114.3.599
Bowman, J. L., Smyth, D. R., Meyerowitz, E. M. (1989). Genes directing flower development in arabidopsis. Plant Cell 1, 37–52. doi: 10.1105/tpc.1.1.37
Bowman, J. L., Smyth, D. R., Meyerowitz, E. M. (2012). The ABC model of flower development: then and now. Development 139 (22), 4095–4098. doi: 10.1242/dev.083972
Breuil-Broyer, S., Trehin, C., Morel, P., Boltz, V., Sun, B., Chambrier, P., et al. (2016). Analysis of the arabidopsis superman allelic series and the interactions with other genes demonstrate developmental robustness and joint specification of male-female boundary, flower meristem termination and carpel compartmentalization. Ann. Bot. 117, 905–923. doi: 10.1093/aob/mcw023
Caballo, C., Berbel, A., Ortega, R., Gil, J., Millán, T., Rubio, J., et al. (2022). The SINGLE FLOWER (SFL) gene encodes a MYB transcription factor that regulates the number of flowers produced by the inflorescence of chickpea. New Phytol. 234, 827–836. doi: 10.1111/nph.18019
Cañas, L. A., Beltrán, J. P. (2018). “Chapter 2. model legumes: functional genomics tools in medicago truncatula,” in Functional genomics in medicago truncatula. methods and protocols. methods in molecular biology series n° 1822. Eds. Cañas, L. A., Beltrán, JoséP. (New York: Springer Nature, Humana Press), 11–38, ISBN: ISBN: 978-1-4939-8632-3.
Cannon, S. B., Sterckc, L., Rombautsc, S., Sato, S., Cheung, F., Gouzy, J., et al. (2006). Legume genome evolution viewed through the Medicago truncatula and Lotus japonicus genomes. Proc. Natl. Acad. Sciences U.S.A. 103, 14959–14964. doi: 10.1073/pnas.0603228103
Carroll, S. B. (2005). Evolution at two levels: On genes and form. PloS Biol. 3, 1159–1166. doi: 10.1371/journal.pbio.0030245
Cheng, X., Li, G., Tang, Y., Wen, J. (2018). Dissection of genetic regulation of compound inflorescence development in Medicago truncatula. Development 145 (3), dev158766. doi: 10.1242/dev.158766
Cui, L., Wall, P. K., Leebens-Mack, J. H., Lindsay, B. G., Soltis, D. E., Doyle, J. J., et al. (2006). Widespread genome duplications throughout the history of flowering plants. Genome Res. 16, 738–749. doi: 10.1101/gr.4825606
Devi, J., Mishra, G. P., Sanwal, S. K., Dubey, R. K., Singh, P. M., Singh, B. (2018). Development and characterization of penta-flowering and triple-flowering genotypes in garden pea (Pisum sativum l. var. hortense). PloS One 13, 1–15. doi: 10.1371/journal.pone.0201235
Ferrándiz, C., Navarro, C., Gómez, M. D., Cañas, L. A., Beltrán, J. P. (1999). Flower development in Pisum sativum: From the war of the whorls to the battle of the common primordia. Dev. Genet. 25, 280–290. doi: 10.1002/(SICI)1520-6408(1999)25:3<280::AID-DVG10>3.0.CO;2-3
Gaiser, J. C., Robinson-Beers, K., Gasser, C. S. (1995). The arabidopsis SUPERMAN gene mediates asymmetric growth of the outer integument of ovules. Plant Cell 7, 333–345. doi: 10.2307/3869855
Gaur, P. M., Gour, V. K. (2002). A gene producing one to nine flowers per flowering node in chickpea. Euphytica 128, 231–235. doi: 10.1023/A:1020845815319
Hiratsu, K., Matsui, K., Koyama, T., Ohme-Takagi, M. (2003). Dominant repression of target genes by chimeric repressors that include the EAR motif, a repression domain, in arabidopsis. Plant J. 34, 733–739. doi: 10.1046/j.1365-313X.2003.01759.x
Hiratsu, K., Mitsuda, N., Matsui, K., Ohme-Takagi, M. (2004). Identification of the minimal repression domain of SUPERMAN shows that the DLELRL hexapeptide is both necessary and sufficient for repression of transcription in arabidopsis. Biochem. Biophys. Res. Commun. 321, 172–178. doi: 10.1016/j.bbrc.2004.06.115
Hiratsu, K., Ohta, M., Matsui, K., Ohme-Takagi, M. (2002). The SUPERMAN protein is an active repressor whose carboxy-terminal repression domain is required for the development of normal flowers. FEBS Lett. 514, 351–354. doi: 10.1016/S0014-5793(02)02435-3
Hofer, J. M. I., Ellis, N. T. H. (2014). Developmental specialisations in the legume family. Curr. Opin. Plant Biol. 17, 153–158. doi: 10.1016/j.pbi.2013.11.014
Kazama, Y., Fujiwara, M. T., Koizumi, A., Nishihara, K., Kifune, E., Abe, T., et al. (2009). A SUPERMAN-like gene is exclusively expressed in female flowers of the dioecious plant silene latifolia. Plant Cell Physiol. 50, 1127–1141. doi: 10.1093/pcp/pcp064
Murfet, I. C. (1985). The influence of genes ar and n on senescence in Pisum sativum l. Ann. Bot. 55 (5), 675–683. doi: 10.1093/oxfordjournals.aob.a086946
Nakagawa, H., Ferrario, S., Angenent, G. C., Kobayashi, A., Takatsuji, H. (2004). The petunia ortholog of arabidopsis SUPERMAN plays a distinct role in floral organ morphogenesis. Plant Cell 16, 920–932. doi: 10.1105/tpc.018838
Nandi, A. K., Kushalappa, K., Prasad, K., Vijayraghavan, U. (2000). A conserved function for arabidopsis SUPERMAN in regulating floral-whorl cell proliferation in rice, a monocotyledonous plant. Curr. Biol. 10 (4), 215–218. doi: 10.1016/S0960-9822(00)00341-9
Nibau, C., Di Stilio, V. S., Wu, H. M., Cheung, A. Y. (2011). Arabidopsis and tobacco SUPERMAN regulate hormone signalling and mediate cell proliferation and differentiation. J. Exp. Bot. 62, 949–961. doi: 10.1093/jxb/erq325
Prunet, N., Morel, P., Negrutiu, I., Trehin, C. (2009). Time to stop: Flower meristem termination. Plant Physiol. 150, 1764–1772. doi: 10.1104/pp.109.141812
Prunet, N., Yang, W., Das, P., Meyerowitz, E. M., Jack, T. P. (2017). SUPERMAN prevents class b gene expression and promotes stem cell termination in the fourth whorl of Arabidopsis thaliana flowers. Proc. Natl. Acad. Sciences U.S.A. 114, 7166–7171. doi: 10.1073/pnas.1705977114
Prusinkiewicz, P., Erasmus, Y., Lane, B., Harder, L. D., Coen, E. (2007). Evolution and development of inflorescence architectures. Sci. (80-. ) 316, 1452–1456. doi: 10.1126/science.1140429
Rodas, A. L., Roque, E., Hamza, R., Gómez-Mena, C., Minguet, E. G., Wen, J., et al. (2021). MtSUPERMAN plays a key role in compound inflorescence and flower development in Medicago truncatula. Plant J. 105 (3), 816–830. doi: 10.1111/tpj.15075
Roque, E., Gómez-Mena, C., Ferrándiz, C., Beltrán, J. P., Cañas, L. A. (2018). “Chapter 18. functional genomics and genetic control of floral and fruit development in medicago truncatula: an overview,” in Functional genomics in medicago truncatula. methods and protocols. methods in molecular biology series n° 1822. Eds. Cañas, L. A., Beltrán, JoséP. (New York: Springer Nature, Humana Press), 273–290, ISBN: ISBN: 978-1-4939-8632-3.
Sakai, H., Krizek, B. A., Jacobsen, S. E., Meyerowitz, E. M. (2000). Regulation of SUP expression identifies multiple regulators involved in arabidopsis floral meristem development. Plant Cell 12, 1607–1618. doi: 10.1105/tpc.12.9.1607
Sakai, H., Medrano, L. J., Meyerowitz, E. M. (1995). Role of SUPERMAN in maintaining arabidopsis floral whorl boundaries. Nature 378, 199–203. doi: 10.1038/378199a0
Singer, S., Sollinger, J., Maki, S., Fishbach, J., Short, B., Reinke, C., et al. (1999). Inflorescence architecture: A developmental genetics approach. Botanical Rev. 65, 385–410. doi: 10.1007/BF02857756
Smyth, D. R., Bowman, J. L., Meyerowitz, E. M. (1990). Early flower development in arabidopsis. Plant Cell 2 (8), 75–67. doi: 10.1105/tpc.2.8.755
Srinivasan, S., Gaur, P. M., Chaturvedi, S. K., Rao, B. V. (2006). Allelic relationships of genes controlling number of flowers per axis in chickpea. Euphytica 152, 331–337. doi: 10.1007/s10681-006-9219-z
Tucker, S. C. (1989). Overlapping organ initation and common primordia in flowers of Pisum sativum (Leguminosae : Papilionoideae). Am. J. Bot. 76, 714–729. doi: 10.1002/j.1537-2197.1989.tb11366.x
Tucker, S. C. (2003). Update on floral development floral development in legumes. Plant Physiol. 131, 911–926. doi: 10.1104/pp.102.017459
Wang, C., Yang, X., Li, G. (2021). Molecular insights into inflorescence meristem specification for yield potential in cereal crops. Int. J. Mol. Sci. 22 (7), 3508. doi: 10.3390/ijms22073508
Xu, Y., Prunet, N., Gan, E.-S., Wang, Y., Stewart, D., Wellmer, F., et al. (2018). SUPERMAN regulates floral whorl boundaries through control of auxin biosynthesis. EMBO J. 37, e97499. doi: 10.15252/embj.201797499
Xu, W., Zhu, W., Yang, L., Liang, W., Li, H., Yang, L., et al. (2022). SMALL REPRODUCTIVE ORGANS, a SUPERMAN-like transcription factor, regulates stamen and pistil growth in rice. New Phytol. 233, 1701–1718. doi: 10.1111/nph.17849
Young, N. D., Debellé, F., Oldroyd, G. E. D., Cannon, S. B., Udvardi, M. K., et al. (2011). The medicago genome provides insight into the evolution of rhizobial symbioses. Nature 480, 520–524.
Yu, H., Huang, T. (2016). Molecular mechanisms of floral boundary formation in arabidopsis. Int. J. Mol. Sci. 17 (3), 317. doi: 10.3390/ijms17030317
Keywords: SUPERMAN, legumes, Medicago truncatula, MtSUP, compound inflorescence, flower development, flower number
Citation: Rodas AL, Roque E, Hamza R, Gómez-Mena C, Beltrán JP and Cañas LA (2023) SUPERMAN strikes again in legumes. Front. Plant Sci. 14:1120342. doi: 10.3389/fpls.2023.1120342
Received: 09 December 2022; Accepted: 16 January 2023;
Published: 30 January 2023.
Edited by:
Jose C. Jimenez-Lopez, Department of Biochemistry, Cell & Molecular Biology of Plants, Spanish National Research Council (CSIC), SpainReviewed by:
Hokuto Nakayama, The University of Tokyo, JapanCopyright © 2023 Rodas, Roque, Hamza, Gómez-Mena, Beltrán and Cañas. This is an open-access article distributed under the terms of the Creative Commons Attribution License (CC BY). The use, distribution or reproduction in other forums is permitted, provided the original author(s) and the copyright owner(s) are credited and that the original publication in this journal is cited, in accordance with accepted academic practice. No use, distribution or reproduction is permitted which does not comply with these terms.
*Correspondence: Edelín Roque, ZWRyb3F1ZUBpYm1jcC51cHYuZXM=; Luis A. Cañas, bGNhbmFzQGlibWNwLnVwdi5lcw==