- 1State Key Laboratory of Tree Genetics and Breeding, Key Laboratory of Tree Breeding of Zhejiang Province, Research Institute of Subtropical Forestry, Chinese Academy of Forestry, Hangzhou, Zhejiang, China
- 2Forestry Faculty, Nanjing Forestry University, Nanjing, Jiangsu, China
- 3College of Life Sciences, Fujian Agriculture and Forestry University, Fuzhou, China
- 4Institute of Virology and Biotechnology, Zhejiang Academy of Agricultural Sciences, Hangzhou, Zhejiang, China
A cadmium (Cd) tolerance protein (SpCTP3) involved in the Sedum plumbizincicola response to Cd stress was identified. However, the mechanism underlying the Cd detoxification and accumulation mediated by SpCTP3 in plants remains unclear. We compared wild-type (WT) and SpCTP3-overexpressing transgenic poplars in terms of Cd accumulation, physiological indices, and the expression profiles of transporter genes following with 100 μmol/L CdCl2. Compared with the WT, significantly more Cd accumulated in the above-ground and below-ground parts of the SpCTP3-overexpressing lines after 100 μmol/L CdCl2 treatment. The Cd flow rate was significantly higher in the transgenic roots than in the WT roots. The overexpression of SpCTP3 resulted in the subcellular redistribution of Cd, with decreased and increased Cd proportions in the cell wall and the soluble fraction, respectively, in the roots and leaves. Additionally, the accumulation of Cd increased the reactive oxygen species (ROS) content. The activities of three antioxidant enzymes (peroxidase, catalase, and superoxide dismutase) increased significantly in response to Cd stress. The observed increase in the titratable acid content in the cytoplasm might lead to the enhanced chelation of Cd. The genes encoding several transporters related to Cd2+ transport and detoxification were expressed at higher levels in the transgenic poplars than in the WT plants. Our results suggest that overexpressing SpCTP3 in transgenic poplar plants promotes Cd accumulation, modulates Cd distribution and ROS homeostasis, and decreases Cd toxicity via organic acids. In conclusion, genetically modifying plants to overexpress SpCTP3 may be a viable strategy for improving the phytoremediation of Cd-polluted soil.
1 Introduction
Rapid economic and technological developments have resulted in the release of a relatively large amount of heavy metal waste, which has led to increased environmental pollution. In China, more than 1.3 × 105 km2 of cultivated land has been polluted with cadmium (Cd) (MER and MLR, 2014; Jia et al., 2022). Soil degradation due to Cd contamination is becoming a major issue affecting food security and quality (Rehman et al., 2020). Heavy metal pollution has threatened the sustainable development of various industries (e.g., agriculture) (Yessica et al., 2020). Heavy metal pollution has attracted the attention of researchers in several disciplines (Li et al., 2018; Angulo-Bejarano et al., 2021). Cadmium is one of the most dangerous heavy metals because of its high toxicity and bioconcentration factor (Singh P et al., 2020; Cui et al., 2021) As a non-essential element in plants, Cd can disrupt plant metabolic activities, adversely affect photosynthesis, exacerbate the effects of nutrient deficiency as well as oxidative stress, and even inhibit cell proliferation and division (Rasafi et al., 2020; Shamelashvili et al., 2020; Yazdi, 2021). Cadmium stress can also inhibit plant growth in mild cases and cause plant death in severe cases (Yang et al., 2022). In heavily polluted areas, Cd can enter the human body through the food chain, with detrimental and irreversible effects on human health (Fu and Xi, 2020). Therefore, the remediation of Cd-contaminated soil is a major challenge that must be addressed (Wan et al., 2016; Han et al., 2020).
Phytoremediation has attracted widespread interest as an economically feasible and eco-friendly method for rescuing Cd-contaminated soils (Hamid et al., 2020; Raza et al., 2020). The Cd accumulation and detoxification capacities of plants are the main determinants of phytoremediation efficiency (Wang et al., 2021; Jia et al., 2022). The remediation of Cd-contaminated soil by hyperaccumulating plants is one of the most promising environmentally friendly remediation methods (Yaashikaa et al., 2022). It may be improved by increasing the absorption rate, transport, and accumulation of Cd in plants, which depends on the synergism between physiological and molecular mechanisms (Chai et al., 2020).
Elucidating the mechanisms regulating Cd tolerance and accumulation in plants is important for increasing the efficiency of the phytoremediation of Cd-polluted soil. Cadmium that is taken up by plants accumulates in the above-ground plant parts via the long-distance transport mediated by many transporters. Cd uptake, transport and distribution in plants depend on their metal transporters, such as zinc/iron-regulated transporter (ZRT/IRT-related ZRT-IRT-like proteins (ZIP)), heavy metal ATPase (HMA), Natural Resistance-Associated Macrophage Protein (NRAMP), and ATP-binding cassette (ABCC) (Zhang et al., 2013; Jiang et al., 2021; Yue et al., 2021). Cd in the external environment can be transported from the soil to the cell interior through the ZIP transporter on the plasma membrane (Viehweger, 2014). Then Cd2+ is transported to xylem through NRAMP, HMA, etc (He et al., 2015). The heterologous expression of NRAMP3 from the hyperaccumulator Sedum alfredii in Brassica juncea reportedly does not significantly affect Cd tolerance, but it significantly increases the Cd content in the above-ground transgenic plant parts, implying that SaNRAMP3 regulates the root-to-shoot translocation of Cd (Feng et al., 2018). HMA genes encode proteins that mediate the transport of metals from the roots to the shoots (Peng et al., 2017). In Brassica rapa, HMA3 regulates Cd accumulation and increases the root-to-shoot Cd translocation rate (Zhang et al., 2019). In addition, some of the cadmium that enters the cytoplasm is transported into the vesicles by the action of ABC transporter and metal tolerance protein 1 (MTP1) (Lin and Aarts, 2012; Park et al., 2012).
The Cd tolerance of plants depends on physiological detoxification-related processes (Li et al., 2022). After passing through the cytoplasmic membrane, Cd is chelated with ligands to inhibit reactions between Cd and other cellular compounds. Plants often increase the accumulation of titratable acid to cope with heavy metal stress. When Cu was sprayed on leaves of Hibiscus sabdariffa, the contents of anthocyanin and titratable acid were increased significantly (Patricio et al., 2018). In lettuce, humic acid can stimulate production of organic acids by improving dark fixation of carbon dioxide or prevent the harmful effects of Cd, thereby increases titratable acidity of leaves (Haghighi et al., 2013). In response to lead stress, 5-aminolevulinic acid minimizes the potential for lead ions to inhibit metabolism by increasing the expression of genes encoding enzymes associated with malate and citrate metabolism, leading to the chelation of lead ions (Singh R et al., 2020).
Under heavy metal stress conditions, reactive oxygen species (ROS) levels increase substantially in plants. Heavy metal-tolerant plants then produce relatively large amounts of ROS-scavenging antioxidant enzymes to protect against heavy metal-induced oxidative stress (Dvorak et al., 2020). Superoxide dismutase (SOD) was inhibited under high Cd treatment, and excess ROS also led to significantly higher levels of oxidative damage (Zhang et al., 2020). A novel Nicotiana tabacum Cd transporter gene NtNRAMP3 preventing chlorophyll degradation and reducing ROS accumulation under Cd stress conditions. Nevertheless, knockdown of the NtNRAMP3 gene could increase the activities of SOD, POD and CAT thereby improving the Cd tolerance of tobacco to a great degree (Jia et al., 2022). Furthermore, SaCu/Zn SOD expression in S. alfredii is induced by Cd stress; the overexpression of this gene increases the resistance of transgenic A. thaliana plants to oxidative stress (Li et al., 2017).
New plant genes that may increase the efficiency of Cd phytoremediation were recently identified. For example, in A. thaliana, overexpressing the IRON MAN (IMA) gene encoding a small peptide improves Cd tolerance, whereas silencing BRUTUS (BTS) expression can increase the efficiency of the phytoremediation of Cd-contaminated soil (Zhu et al., 2020; Meng et al., 2022). Therefore, the functions of some key Cd stress-related genes in plants may be exploited to alleviate Cd toxicity and improve the Cd tolerance of plants. However, the physiological and molecular mechanisms underlying the functions of these novel genes related to Cd tolerance have not been precisely characterized, which is necessary for the sequential identification of novel genes. We previously detected five new proteins related to Cd tolerance or accumulation in the hyperaccumulator Sedum plumbizincicola, of which the production of Cadmium Tolerance Protein 3 (SpCTP3) is strongly induced by Cd treatments (Liu et al., 2019). Moreover, the expression of SpCTP3, which belongs to the Nod19 superfamily, is up-regulated by stress. Populus × canescens (i.e., poplar species) is potentially useful for phytoremediations because of its fast growth rate and high biomass as well as the availability of an established genetic transformation system. The genetic transformation of this poplar species with Cd hyperaccumulation-related candidate genes from S. plumbizincicola may generate transgenic plants capable of accumulating high Cd levels, making them relevant for the phytoremediation of Cd-contaminated soil. Hence, the mechanisms controlling Cd accumulation, subcellular distribution, and chemical morphology in SpCTP3-overexpressing poplar trees under Cd stress conditions should be clarified. In this study, we examined the effects of SpCTP3 on Cd absorption, distribution, and detoxification to improve its utility for Cd phytoremediation. The data presented herein will provide researchers with the theoretical basis for applying SpCTP3 in the remediation of Cd-polluted lands by transgenic woody plants.
2 Materials and methods
2.1 Vector construction and plant transformation
The S. plumbizincicola SpCTP3 gene was cloned by a PCR amplification using the primers SpCTP3-F/R. The full-length SpCTP3 sequence comprises 1,383 base pairs. The amplified SpCTP3 was incorporated into the pBI121 vector via homologous recombination for the subsequent expression under the control of the CaMV 35S promoter. An Agrobacterium tumefaciens-mediated leaf disc transformation method was used to insert SpCTP3 into P. × canescens plants (Wen et al., 2022). Transgenic plants were identified by a genomic PCR amplification using the primers SpCTP3-F/R and the positive lines were further analyzed by quantitative real-time PCR (qRT-PCR). The relative expression levels were calculated according to the 2−ΔΔCt method (Jia et al., 2022). The TUBU (Beta tubulin) gene was selected as an internal control. Primer details are listed in Table S1. Four-week-old transgenic rooted poplar plants and the control group were grown for 4 weeks in Hoagland nutrient solution (i.e., hydroponic system). The nutrient solution was renewed every 3 days. For the heavy metal treatment experiments, plants were grown for 30 days in Hoagland nutrient solution supplemented with 100 μmol/L CdCl2. The growth chamber conditions were set as follows: 16-h day (25°C)/8-h night (18°C), 50%–60% relative humidity, and a photon flux density of 120 μmol/m2/s. Plants were photographed and their phenotypes were analyzed.
2.2 Determination of Cd elements
The Cd-treated lines were harvested separately and rinsed thoroughly with tap water. They were then soaked in 20 mmol/L EDTA for 20 min and washed three times with deionized water. The roots, stems, and leaves of each line were collected and dried at 70°C until they reached a constant weight. At least 0.2 g dried material was used to measure the Cd concentration. Briefly, the samples were ground to fine powder, and digested with a mixture consisting of HNO3:HClO4 (9:1, v/v) at 120–200°C in a microwave-accelerated reaction system (CEM, Matthews, NC, USA). The final volume was adjusted to 25 mL with distilled water. The Cd concentration was analyzed using the iCAP-7400 inductively coupled plasma optical emission spectroscopy (ICP-OES) system (Thermo Fisher Scientific, California, USA). The heavy metal translocation factor was calculated according to the following formula (Dineshkumar et al., 2019): heavy metal content in the aerial plant parts/heavy metal content in the roots.
2.3 Separation of subcellular components and analysis of the Cd content
Fresh leaves and roots (2.0 g) were homogenized using a precooled mortar and 20 mL precooled extraction solution [50 mmol/L Tris-HCl, 250 mmol/L sucrose, and 1.0 mmol/L C4H10O2S2 (pH 7.5)]. After centrifuging the samples at 1,250 × g for 15 min, the precipitate was collected as the cell wall component. The supernatant was centrifuged at 20,800 × g for 45 min to obtain the precipitate (organelles) and supernatant (soluble fraction). All steps were completed at 4°C. After the collected components were digested, their Cd concentrations were determined by ICP-OES.
2.4 Extraction and quantitative analysis of Cd chemical forms
Cadmium in different chemical forms was extracted as described by Ouyang et al. (2019). Inorganic titratable acid cadmium (CdE) was extracted with 80% ethanol, water-soluble cadmium (CdW) was extracted with deionized water, Cd integrated with pectin and protein (CdNaCl) was extracted with 1 mol/L NaCl, insoluble CdHPO4, Cd3(PO4)2, and other Cd-phosphate complexes (CdHAC) were extracted with 2% HAC, oxalic acid bound cadmium (CdHCl) was extracted with 0.6 mol/L HCl, and the rest was residual cadmium (Cdr). Approximately 0.5 g samples were shaken for the 22-h extraction at 25°C. The proportion of the sample weight and extraction solution volume was adjusted to 1:10 (w/v) before a centrifugation at 5,000 × g for 10 min. The precipitate was resuspended twice with the same extraction solution, shaken at 25°C for 2 h, and then centrifuged at 5,000 × g for 10 min. Finally, the supernatants after three centrifugations were combined. Each pooled solution was then evaporated on an electric plate to a constant weight. After digesting the materials, the Cd concentrations of the three parts were determined by ICP-OES.
2.5 Measurement of the Cd2+ net flux
To determine the net Cd2+ flow rate in different lines treated with Cd stress, nine fine roots (approximately 1.5 mm diameter) were selected from each Cd-treated transgenic line and the WT control. The Cd2+ net flux was measured according to the noninvasive micro-test (NMT) technique (NMT100 Series; Younger, USA LLC, Amherst, MA, USA) as previously described (He et al., 2015; Chen et al., 2017). Each fine root was equilibrated in the measurement solution (0.05mM CdSO4, 0.25 mmol/L NaCl, 0.1 mmol/L Na2SO4, 0.05 mmol/L KCl, 0.15 mmol/L MES, pH6.0) for 30 min and then transferred to fresh measurement solution before determining the Cd2+ net flux. The root Cd2+ flow rate was analyzed by moving the electrode between two points (30 μm) on the root surface to measure the voltage difference perpendicular to the root surface.
2.6 Analysis of antioxidants and antioxidative enzyme activities
To analyze antioxidant enzyme activities and the contents of ROS (O2·− and H2O2), 1.0 g leaf and root samples were collected from the WT and transgenic plants and homogenized in 8 mL 50 mmol·L−1 sodium phosphate buffer (pH 7.8) using a prechilled mortar. The samples were then centrifuged at 10,000 × g for 15 min at 4°C. The supernatant was used to measure enzyme activities and the contents of ROS. The superoxide dismutase (SOD) activities were determined using NBT (Nitroblue tetrazolium) photoreduction method as absorbance 560 nm as described by Akbari et al. (2020). The peroxidase (POD) activity was assayed spectrophotometrically using hydrogen peroxide and guaiacol as extracts at 470 nm (Dai et al., 2020). Catalase (CAT) activity was tested in potassium phosphate buffer (pH 7.8) containing 3 mM H2O2 at 240 nm. The H2O2 content and the O2·− level were determined as described by Alexieva et al. (2001) and Jing et al. (2020). H2O2 content was determined using trichloroacetic acid (0.1% w/v) at 630 nm (Alexieva et al., 2001). For determination of O2·− level, supernatant was mixed with 10 mM of hydroxylamine hydrochloride and left for 20 min followed by the addition of sulfanilamide and naphthylamine. After 20 min of incubation at 25°C, absorbance was measured at 530 nm, and calculations were done using the standard curve of NaNO2 (Jing et al., 2020).
2.7 Determination of titratable acid contents
For titratable acid assay, 0.1 g sample of each treatment was added to water and then ground into a homogenate. The final volume was adjusted to 5 mL for the extraction of titratable acid. The titratable acid content was determined by a titration using sodium hydroxide (0.1 mol/L) and phenolphthalein (1%) as the pH indicator (Huo et al., 2009).
2.8 Analysis of transporter gene expression profiles
Total RNA was extracted from Cd-treated and WT poplar samples using the RNAprep Pure Plant Plus Kit (TIANGEN, Beijing), after which 800 ng total RNA in 20 μL was used to synthesize cDNA. The qRT-PCR analysis was performed using SYBR Green Premix Ex Taq II (TAKARA, Japan). The primer sequence refers to He et al. (2015) and Ma et al. (2014). Information regarding the qRT-PCR primers is provided in Table S1. The qRT-PCR reaction mixture was prepared in a volume of 20 μL, with 10 μL of SYBR green master mix, cDNA template (2 μL), ddH2O (6.8 μL), ROX Reference Dye 0.4μL and 0.4 μL of each of the primer. The qRT-PCR conditions were set as follows; 95°C for 30s, 40 cycles of 95°C for 5 s, and 60°C for 30 s, followed by melting of PCR products step: 95°C for 15 s, 60°C for 1 min, 95°C for 15 s. Each sample was analyzed using three technical replicates to ensure the results were reliable. Gene expression levels were calculated according to the 2−ΔΔCt method, with TUBU selected as the reference gene. Relative gene expression levels were visualized in a heatmap on the basis of Z-score transformed values.
2.9 Statistical analysis
For each experiment, at least three plants were sampled. Statistical analyses were performed using the SPSS software (version 18.0). The significance of the differences between the treated and control samples was analyzed via a one-way ANOVA followed by Duncan’s test (p< 0.05). Data are presented herein as the mean ± standard deviation (SD). The Kruskal Wallis test was used if the data did not meet the assumptions for ANOVA (equality of variances) according to Levene’s test. A principal components analysis (PCA) was performed and the results were plotted using the ggbiplot package of R (version 3.6.4).
3 Results
3.1 Development of poplar lines overexpressing SpCTP3
Genomic DNA was extracted from the WT and transgenic lines using the CTAB method. The transgenic lines were verified using SpCTP3-F/R primers (Table S1). Of the 13 putatively transformed lines, 11 were confirmed as transgenic (Figure S1). The PCR results showed that SpCTP3 was expressed in the transgenic poplar plants (Figure S1). Total RNA was extracted from some of the transgenic lines and reverse transcribed to cDNA for the qRT-PCR analysis. Three independent lines (OE-3, OE-6, and OE-7) with high SpCTP3 expression levels were selected for the subsequent analyses.
3.2 SpCTP3 has no effect on the biomass of the transgenic poplar under Cd treatment
There was no significant difference in the dry weights of the untreated WT and transgenic plants. After the Cd treatment, the dry weight of the above-ground parts of the transgenic and WT plants decreased by an average of 28.49% and 12.96% (relative to the control), respectively. The dry weight of the root system decreased slightly after the Cd treatment, but this decrease was not significant. Accordingly, SpCTP3 expression has no effect of the biomass of transgenic poplar plants exposed to Cd stress (Figure 1).
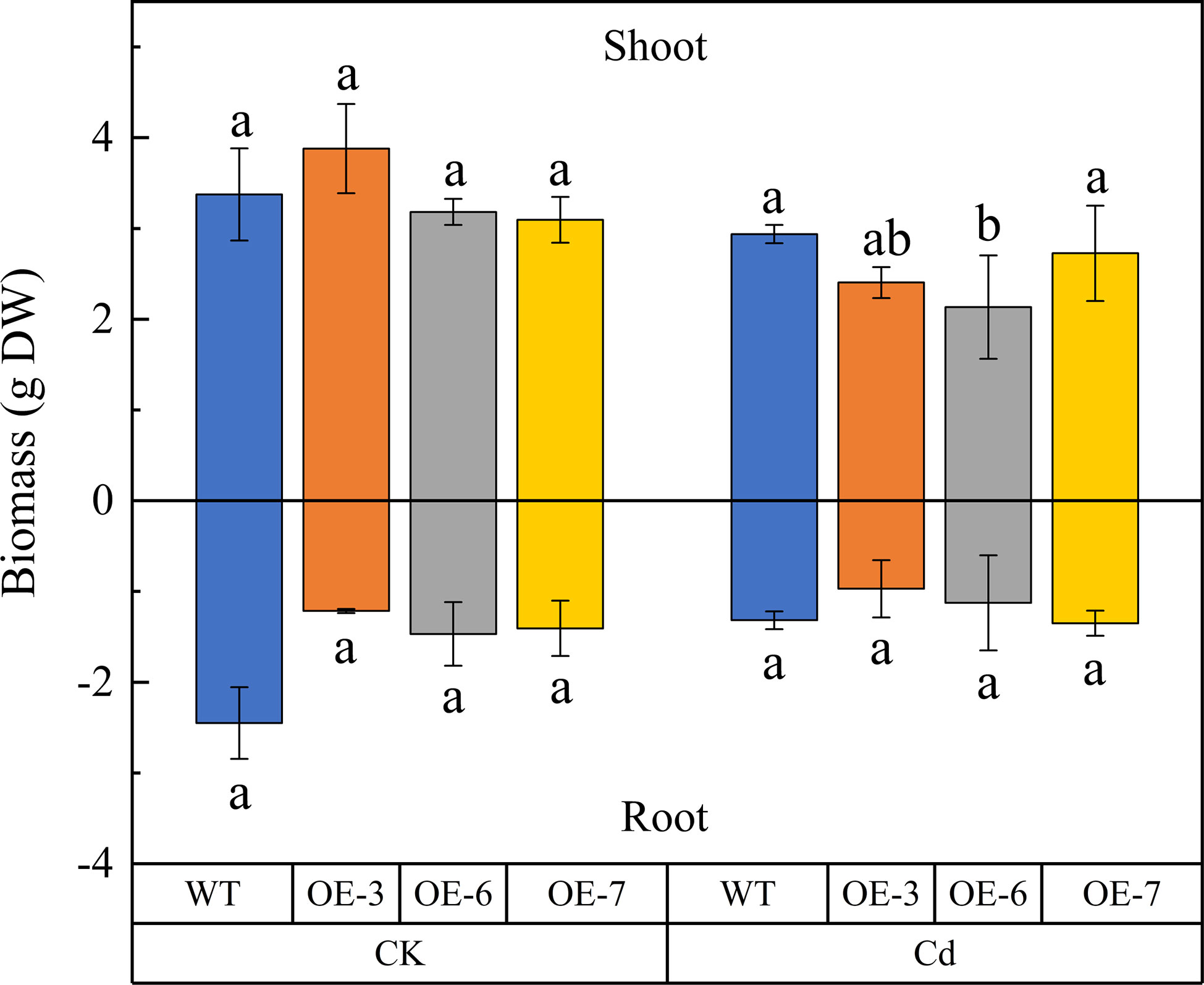
Figure 1 Effect of cadmium (Cd) stress on the shoot and root biomasses of SpCTP3-overexpressing transgenic and WT lines. Data are presented as the mean ± SD of three independent replicates. Different letters above bars indicate significant differences among treatments (p< 0.05; n = 3).
3.3 SpCTP3 overexpression increased the Cd accumulation in transgenic poplars
To further functionally characterize SpCTP3, we measured the Cd content of specific parts of the WT and transgenic plants treated with Cd for 30 days (Figure 2). Notably, the root and leaf Cd contents were higher for the SpCTP3-overexpressing transgenic lines than for the WT controls. In contrast, there were no obvious differences in the stem Cd contents. The distribution of Cd was in the order of roots, leaves, and stems. The leaf Cd content was 48.58%–82.49% higher for the transgenic lines than for the WT controls. Similarly, the translocation factor was higher for the transgenic lines than for the WT plants. These observations indicated that compared with the WT plants, the transgenic poplar plants were better able to transport Cd from the roots to the aerial parts.
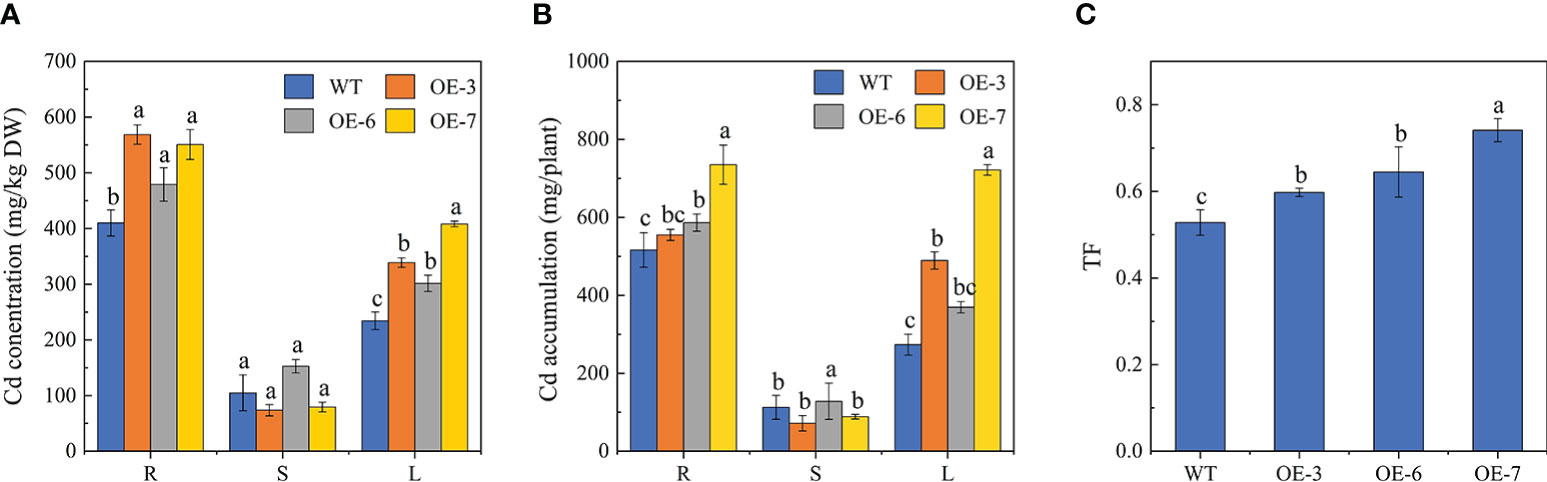
Figure 2 (A) Cd concentrations. (B) Cd accumulation. (C) Root-to-leaf Cd translocation factors (TFs).
3.4 Overexpression of SpCTP3 altered the distribution and chemical forms of Cd in transgenic plants
In this study, Cd2+ was differentially distributed in various subcellular components. Most of the Cd in the roots and leaves of the transgenic lines was stored in the soluble fraction, followed by the cell wall and organelles (Figure 3). In the WT plants, 36.12% and 56.77% of the Cd were present in the cell wall and soluble fraction, respectively; the rest of the Cd was detected in the organelle fraction. In the WT leaves, 44.47% and 47.52% of the Cd content was distributed in the cell wall and soluble fraction, respectively. The cell wall Cd content was lower for the transgenic lines than for the WT plants, whereas the opposite pattern was observed for the Cd content in the soluble fraction.
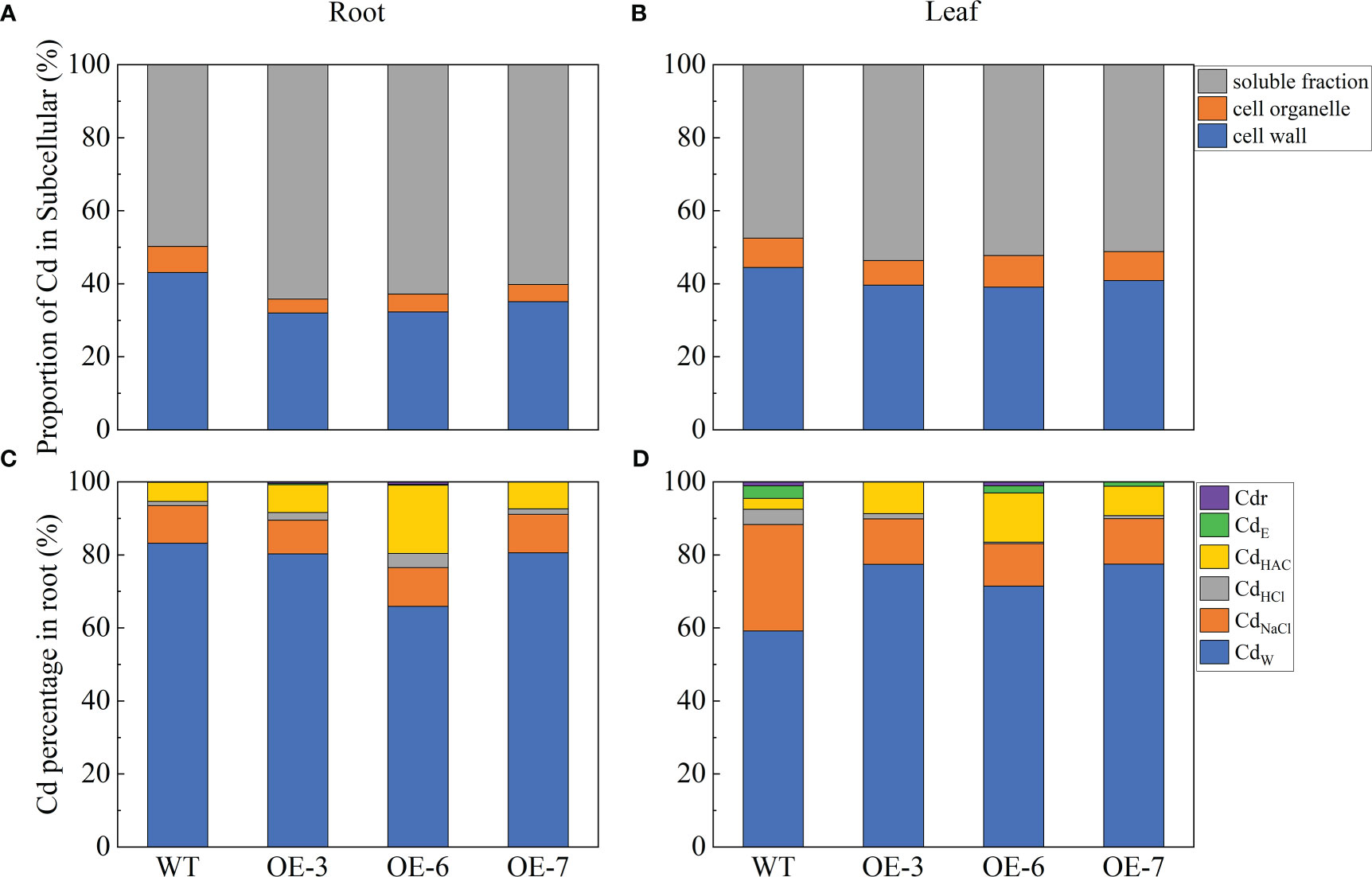
Figure 3 Cd subcellular distribution and Cd chemical forms in WT and SpCTP3-overexpressing poplar roots and leaves treated with 100 μmol·L−1 Cd for 30 days. (A) Cd subcellular distribution in the roots. (B) Cd subcellular distribution in the leaves. (C) Cd chemical forms in the roots. (D) Cd chemical forms in the leaves. CdE, inorganic Cd; CdW, water-soluble Cd; CdNaCl, pectate- and protein-integrated Cd; CdHAc, insoluble CdHPO4, Cd3(PO4)2, and other Cd-phosphate complexes; CdHCl, oxalic acid-bound Cd; Cdr, Cd in residues.
Cadmium absorbed by plants is present in cells in diverse forms and affects plant growth and development. We extracted various forms of Cd and determined the Cd contents using an ICP-OES system. The CdW content was highest in the roots and leaves of the transgenic and WT plants (Figure 3). The Cd in the WT roots was mostly detected as CdW (59.19%), followed by CdNaCl (29.11%) and then CdHCl, CdHAc, and CdE. The Cd in the transgenic roots was also mostly detected as CdW (average of 75.59%), although the CdHAc content was also relatively high. The Cd in the leaves of the transgenic and WT plants was predominantly in the CdE, CdNaCl, and CdHAc forms. Compared with the WT control, the Cd treatment of the transgenic plants decreased the CdW and CdNaCl contents, while increasing the CdHCl and CdHAc contents. Hence, the overexpression of SpCTP3 altered the Cd distribution and chemical forms in the transgenic plants.
3.5 Overexpression of SpCTP3 enhanced the net flux of Cd2+ in poplar roots
To clarify the changes in Cd uptake by the roots of transgenic and WT poplar plants exposed to Cd stress, we used the NMT technique to analyze the Cd2+ flow rate at 120 μm from the root tip after the Cd treatment. The Cd2+ flow rate was 72.927–163.48 pmol/cm2/s for the transgenic lines and 44.707–61.058 pmol/cm2/s for the WT plants (Figure 4). The net influx of Cd2+ was higher for the transgenic roots than for the WT roots.
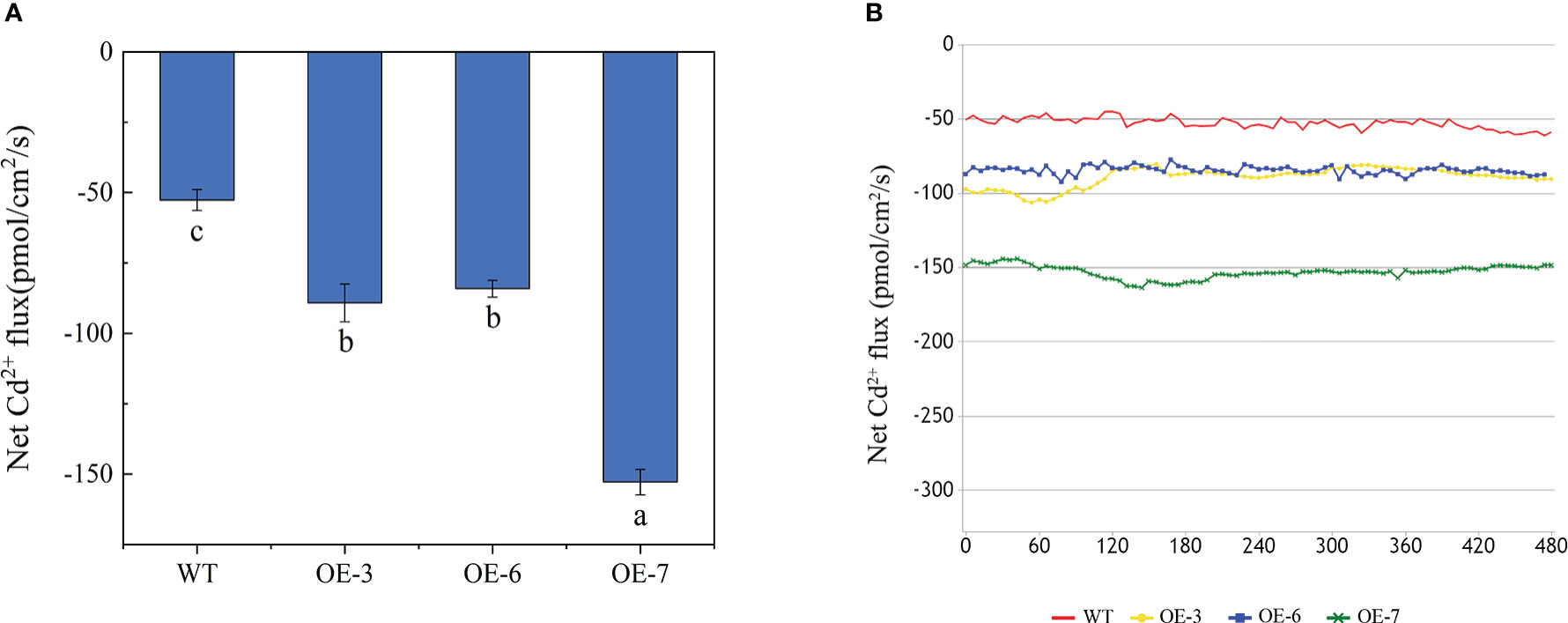
Figure 4 Effects of Cd treatments on the Cd2+ net flux in the roots of WT and transgenic lines. (A) Results of the statistical analysis of the steady-state Cd2+ net flux. (B) Time-course of the Cd2+ net flux at the root surface. Different letters on bars indicate significant differences in the Cd concentrations among lines (p< 0.05).
3.6 Overexpression of SpCTP3 increased the ROS content and affected antioxidant enzyme activities
An exposure to Cd stress results in different degrees of oxidative stress in plants. The and H2O2 concentrations were compared between the WT and transgenic poplar plants (Figure 5). For both the WT and transgenic samples, the Cd treatment induced a significant H2O2 and burst. The leaf content was 2-times higher in the transgenic plants than in the WT plants. In the roots, the H2O2 and contents increased in the transgenic plants by varying degrees, resulting in levels that were significantly higher than those in the WT plants. Additionally, H2O2 and accumulated more in the leaves than in the roots, although this phenomenon was significantly less pronounced in the WT plants than in the transgenic plants.
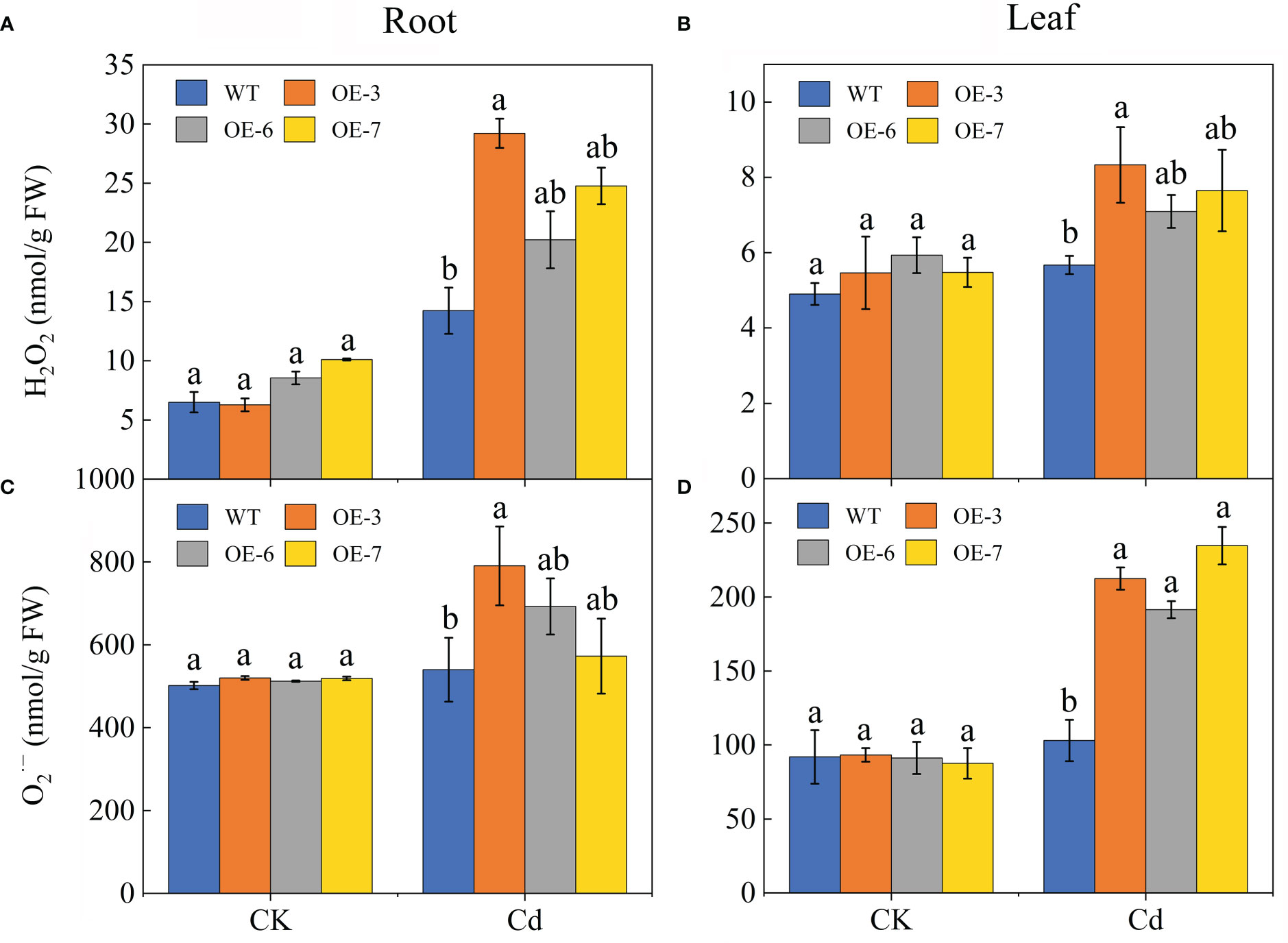
Figure 5 Effect of Cd stress on the ROS contents of the WT and SpCTP3-overexpressing transgenic poplar roots and leaves. (A) Root H2O2 concentrations. (B) Leaf H2O2 concentrations. (C) Root concentrations. (D) Leaf concentrations. Data are presented as the mean ± SD of three independent replicates. Different letters above bars indicate significant differences among treatments (p< 0.05).
Cadmium significantly affected the CAT, SOD, and POD activities (Figure 6). The CAT activity levels were significantly higher in the transgenic lines than in the WT plants after the Cd treatment. Compared with the WT control, the CAT activity was respectively 1.37-fold and 2.98-fold higher in the roots and leaves of the transgenic lines by Cd stress. Furthermore, there was no significant difference in the SOD activities of the Cd-treated transgenic and WT leaves, but the root SOD activities were slightly lower in the transgenic lines than in the WT control following the Cd treatment. In addition, the POD activity in the leaves was slightly higher in the transgenic lines than in the WT plants, but there was no significant difference in the root POD activity.
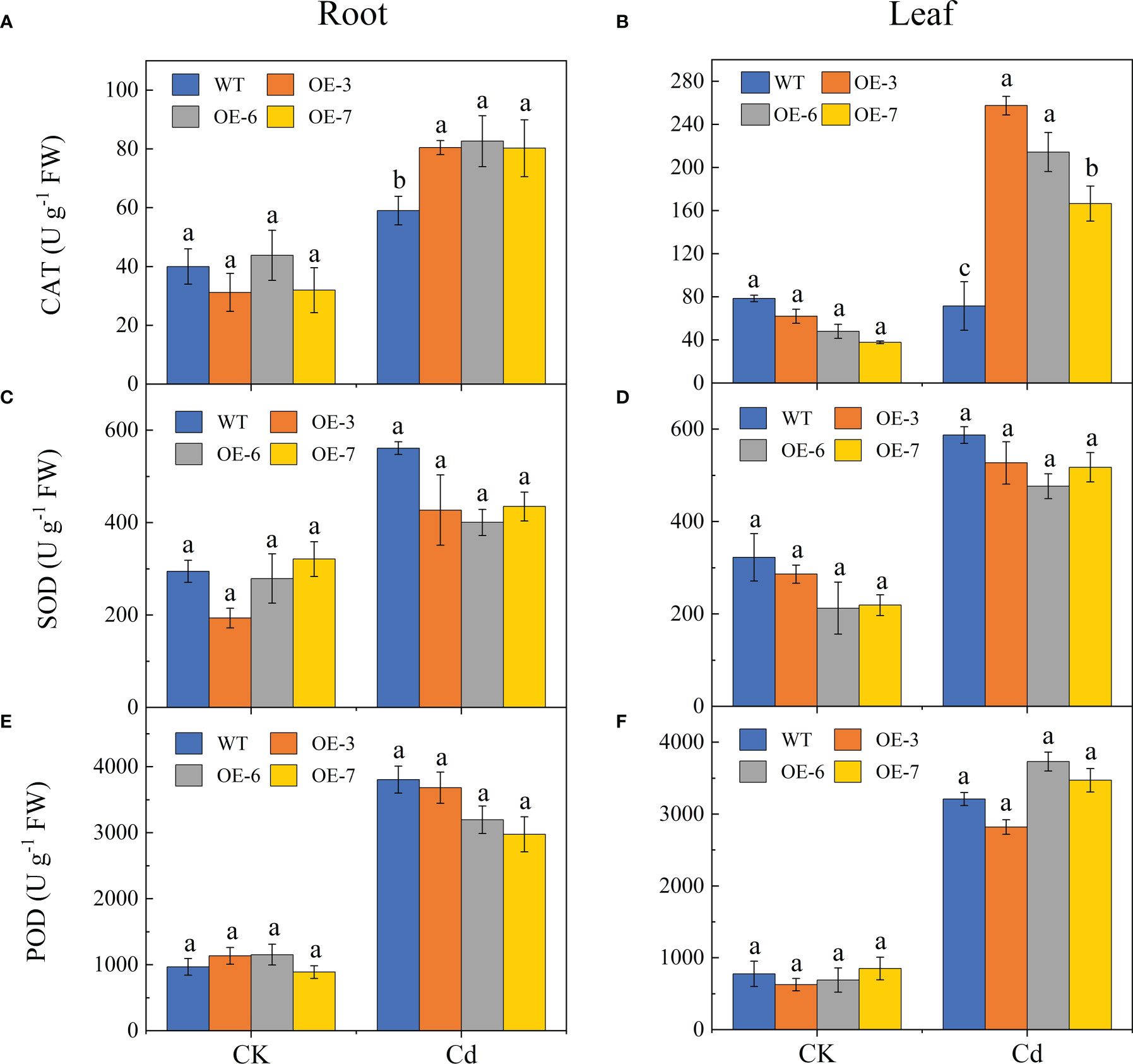
Figure 6 Effect of Cd stress on the antioxidant enzyme activities of the WT and SpCTP3-overexpressing poplar roots and leaves. (A) Root CAT activities. (B) Leaf CAT activities. (C) Root SOD activities. (D) Leaf SOD activities. (E) Root POD activities. (F) Leaf POD activities. Data are presented as the mean ± SD of three independent replicates. Different letters above bars indicate significant differences among treatments (p< 0.05).
3.7 Overexpression of SpCTP3 increased the titratable acid content of Cd-treated transgenic lines
Titratable acids are often considered to be important for the resistance of plants to heavy metal pollution because they contribute to the chelation of metal elements and convert metal ions to less toxic or non-toxic chelated states. Titratable acids were extracted from the SpCTP3-overexpressing and WT plants and the total organic acid content was determined via acid-base titration (Figure 7). The Cd treatment induced a substantial change in the titratable acid content. More specifically, the titratable acid content in the transgenic roots and leaves increased significantly, peaking at 20.76 μmol·g−1 in the roots (i.e., 31.87% higher than the titratable acid content of the control roots) and 30.81 μmol·g−1 in the leaves. Thus, the overexpression of SpCTP3 increased the titratable acid content of transgenic lines under Cd stress conditions.
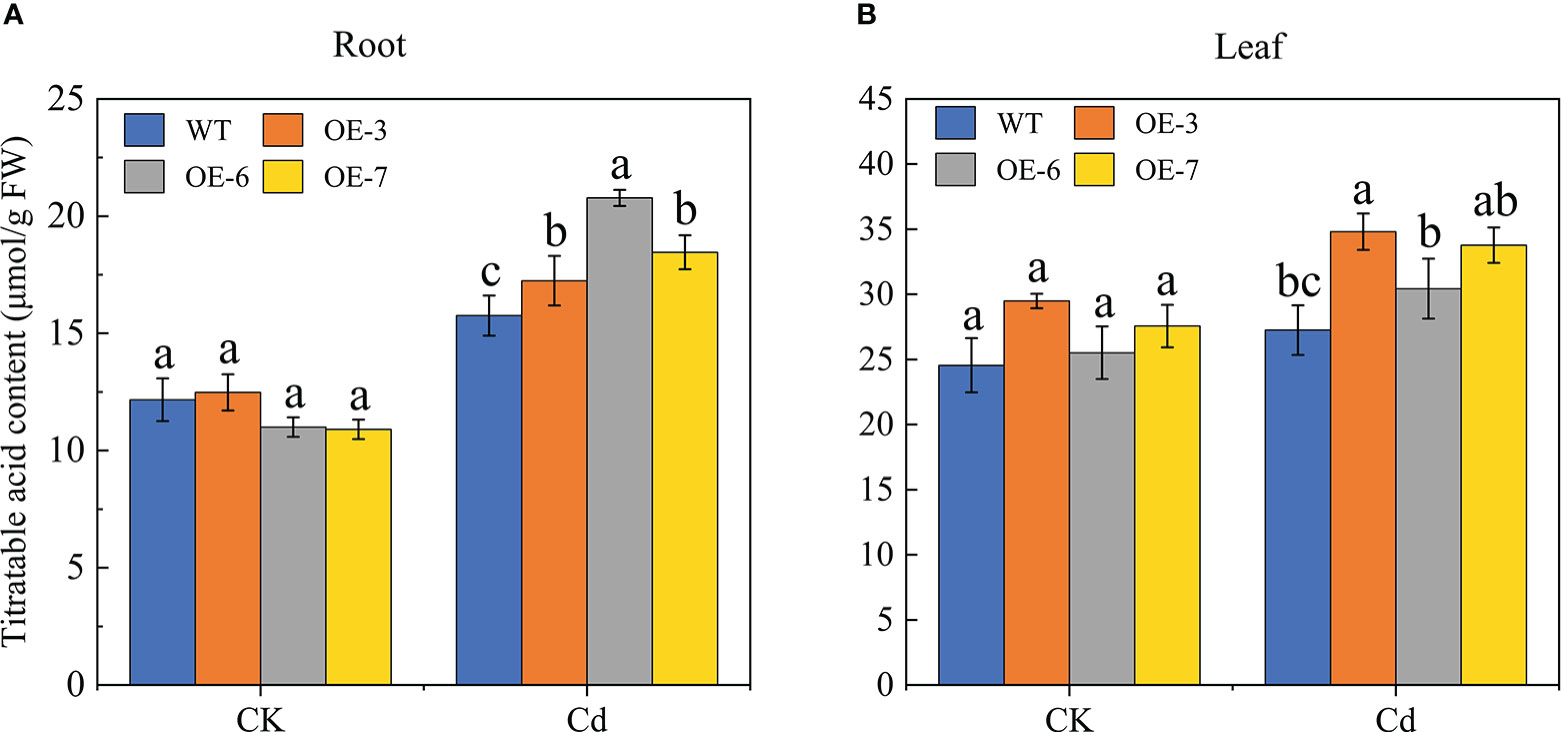
Figure 7 Titratable acid contents of the WT and SpCTP3-overexpressing transgenic poplar roots and leaves treated with 100 μmol·L−1 Cd for 30 days. (A) Root titratable acid contents. (B) Leaf titratable acid contents. Data are presented as the mean ± SD of three independent replicates. Different letters above bars indicate significant differences among treatments (p< 0.05).
3.8 Expression of the genes involved in Cd2+ transport and detoxification
On the basis of the physiological data (Figure 8), differences in the Cd uptake by the roots and the subsequent translocation to the shoots may be a possible reason for diversity in the Cd accumulation between the WT and transgenic poplar plants. Therefore, we focused on the transcriptional regulation of some transporter genes involved in Cd2+ transport and detoxification. The expression levels of all 12 genes related to Cd transport and detoxification were differentially up-regulated in the roots after the Cd treatment (Figure 8). In the roots, the up-regulated expression of ZIP2 (encoding zinc-regulated transporter 2), ZIP6.2, and IRT (encoding an iron-regulated transporter-related protein) was greater in the transgenic lines than in the WT control. Among the leaves, the most up-regulated genes were IRT, YSL2 (encoding a yellow stripe-like protein), and CAX (encoding a cation exchanger). Moreover, the Cd treatment significantly up-regulated the expression of HMA4 (encoding heavy metal ATPase 4) only in the transgenic roots. Similar expression trends were observed for other genes in the leaves and roots, in which NAS (encoding a nicotianamine synthase), MTP1 (encoding metal tolerance protein 1), and Nramp1.3 (encoding natural resistance-associated macrophage protein 1.3) expression levels increased significantly in the Cd-treated transgenic lines.
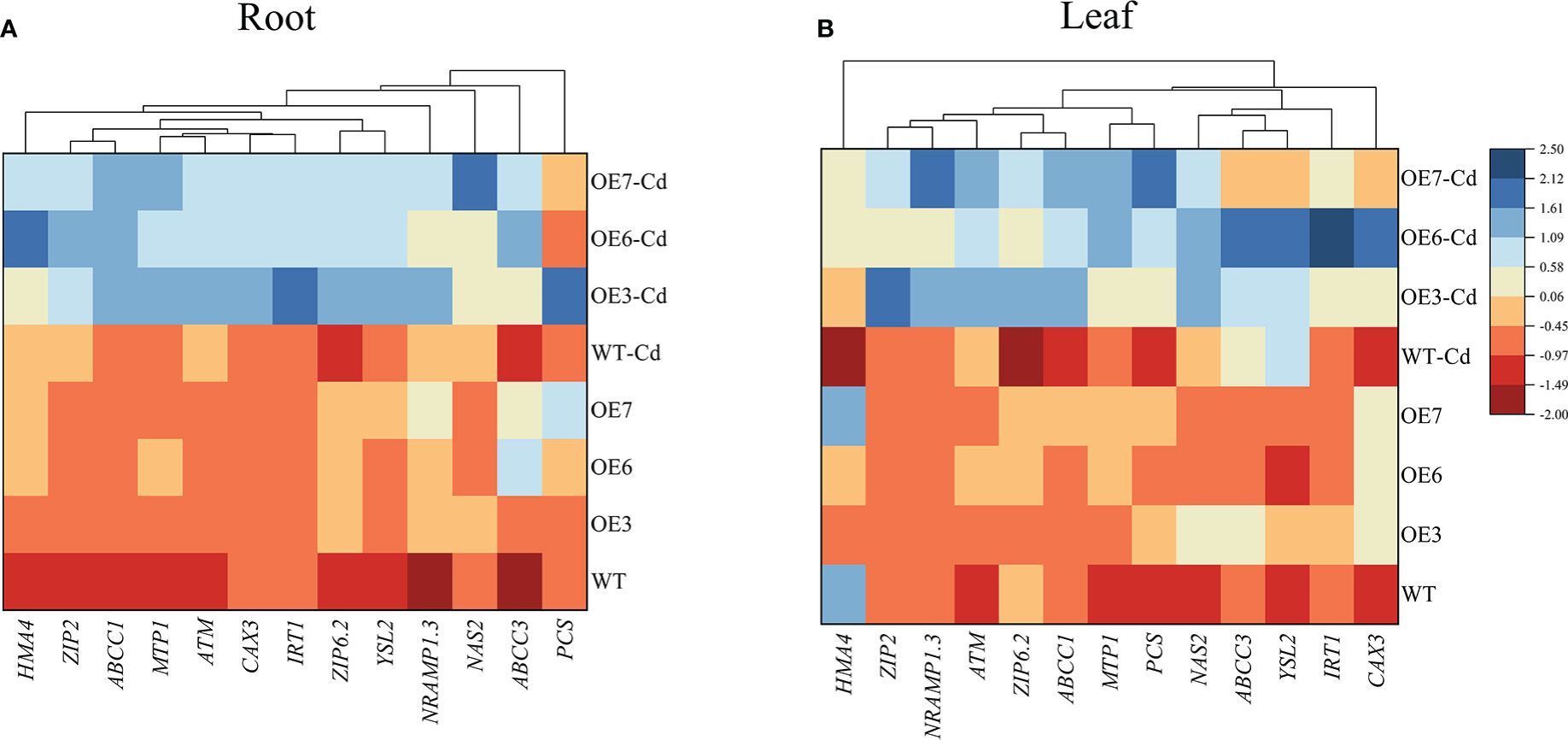
Figure 8 Expression levels of key transporter genes in the Cd-treated roots and leaves of WT and transgenic poplar lines treated with 0 or 100 μmol·L−1 Cd2+ for 30 days. (A) Root gene expression levels. (B) Leaf gene expression levels. Three biological replicates were analyzed. Red and blue respectively indicate negative and positive Z-scores for the gene expression levels.
3.9 Principal component analysis of physiological responses
To determine the response patterns of the WT and transgenic poplar plants to Cd2+, a PCA was conducted using the physiological data related to the exposure to Cd2+ (e.g., Cd concentration as well as ROS, antioxidant, and titratable acid contents) (Figure 9). The PCA results indicated that 88.11% of the total variance could be explained by the effects of the Cd stress on the WT and SpCTP3-overexpressing transgenic poplar plants, with principal components 1 and 2 respectively explaining 74.68% and 13.43% of the Cd-induced variance. There was a significant difference between the SpCTP3-overexpressing transgenic and WT poplar plants under Cd stress conditions.
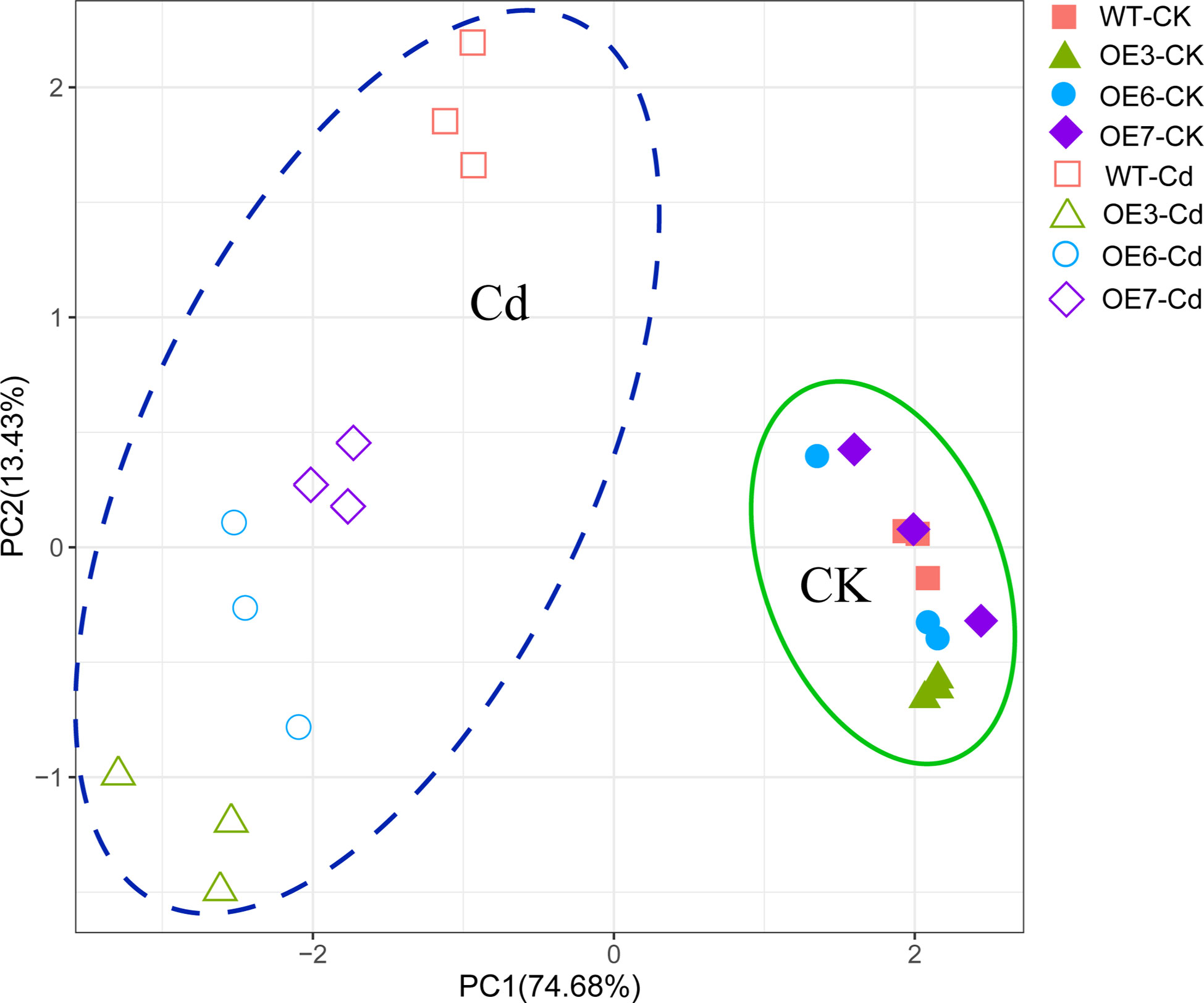
Figure 9 Principal component analysis plots of the Cd concentrations and ROS, antioxidant, and titratable acid contents in the roots and leaves of WT and transgenic plants.
4 Discussion
4.1 SpCTP3 overexpression enhanced Cd accumulation by regulating subcellular components and Cd chemical forms
The soluble fraction of cells consists mainly of vesicles, which contain many proteins, sugars, and organic acids that bind to heavy metals to decrease their effects and limit the amount of heavy metals entering organelles, thereby protecting cells from Cd stress (Li et al., 2014; Ouyang et al., 2019). For example, in Capsicum annuum roots, the Cd content in the soluble fraction can be as high as 77%–87% (Xin and Huang, 2014). Additionally, Cd is mainly distributed in the soluble fraction of the leaves and roots of Cd-tolerant radish varieties (Xin et al., 2017). We obtained similar results. In the WT control, the soluble fraction had the highest Cd content (56.77%), followed by the cell wall. In the transgenic lines, the rank-order for the Cd content was as follows: soluble fraction > cell wall > organelle (Figure 3). This trend was also detected in the leaves. Plants absorb Cd and store most of it in the soluble fraction, followed by the cell wall. However, plant responses to Cd stress may vary among species and stress levels. The transgenic poplar plants had a higher Cd capacity than the WT controls. The accumulation of Cd in the cell wall and soluble fraction can effectively decrease Cd toxicity in plants. In the current study, the Cd content decreased in the cell walls of the transgenic roots and leaves, whereas it increased in the soluble fraction. Hence, in the SpCTP3-overexpressing transgenic poplar plants, Cd ions were separated and fixed in the cytoplasm in the form of relatively stable metal chelates/complexes. Compared with the WT plants, the overexpression of SpCTP3 in the transgenic plants increased the Cd content of the roots and leaves, while also altering the subcellular distribution of Cd by increasing and decreasing the Cd proportions in the soluble fraction and cell wall, respectively. These findings reflect the importance of SpCTP3 for minimizing the toxic effects of Cd in plants.
Under heavy metal stress conditions, multiple mechanisms may be activated in plants to prevent the excessive accumulation of metals in the cytosol and limit the toxicity of the metals. If heavy metals enter the soluble part of the cell, the internal heavy metal tolerance mechanism is activated, resulting in the production of metal chelators, including organic acids, amino acids, and binding proteins (Hall, 2002). Organic acids are an extremely important class of metal ligands that are crucial for the absorption, transport, storage, and detoxification of heavy metals (Long et al., 2003). The proportion of the Cd content in the cell wall of the roots and leaves of the transgenic poplar plants decreased, which was in contrast to the increase in the proportion of the Cd content in the soluble fraction, implying that more Cd ions in cytoplasm need to be immobilized in the form of stable metal chelates/complexes in the transgenic poplar plants than in the WT plants. Accordingly, poplar plants can avoid the harmful effects of Cd stress by decreasing the free Cd concentration in the cytoplasm. In response to the 30-day Cd treatment in this study, the titratable acid content was significantly higher in the transgenic poplar plants than in the WT plants. This indicates that SpCTP3 is critical for ameliorating the toxicity of Cd in plants.
The chemical form of Cd reflects the degree of migration and the toxicity of Cd (Kudo et al., 2021). Highly mobile inorganic and organic Cd compounds are usually more toxic than CdHAc, CdHCl, and CdNaCl (Wu et al., 2016). In our study, CdW was the dominant form in all transgenic lines. Additionally, the CdW content was lower in the roots of the transgenic lines than in the WT roots. The CdW form of Cd is converted to CdHAc and CdHCl. However, CdHAc is associated with Cd uptake, transport, and detoxification (Qiu et al., 2011). It is also indicative of the adaptive response of plants to an exposure to stress. CdW extracted as free inorganic Cd, and these free Cd2+ are not only easily localized in the organelles, but it is also transported to the above-ground tissues. Our analysis of leaves showed that CdW was more abundant in the transgenic lines than in the WT plants, indicating that the transgenic lines had considerably more free Cd2+ (Xin et al., 2017). The Cd content as well as the translocation factor were higher in the transgenic lines than in the WT plants, which is consistent with the results of a recent study (Lai et al., 2021). In the WT control, the proportion of CdNaCl increased, indicative of the increase in the binding between Cd and pectin or protein. Therefore, the leaf cell wall Cd content was higher for the WT plants than for the transgenic lines. Both CdHAc and CdHCl are mainly present in the cell wall and vesicles (Jiang et al., 2007). In the current study, CdHAc and CdHCl contents were higher in the transgenic lines than in the WT plants, which corresponds to the subcellular distribution of Cd. In summary, the conversion of Cd to CdHAc, CdHCl, and CdNaCl is an important mechanism related to the Cd uptake, translocation, and detoxification in plants.
4.2 SpCTP3 induced oxidation reaction under cadmium treatment
Plants take up heavy metals from the soil primarily through their roots. Therefore, determining the Cd uptake and efflux from the roots is important for measuring plant Cd accumulation. Noninvasive micro-test techniques can measure real-time ion transport changes in living plant and animal tissues, cells, and internal/external environments. Our NMT analysis revealed that the Cd in-flow rate was significantly higher for the transgenic roots than for the WT roots. The overexpression of SpCTP3 promoted the influx of Cd in root cells and the accumulation of Cd, indicating that the roots of the transgenic lines had a greater Cd uptake capacity than the WT roots, which is consistent with the findings of an earlier study by Zhang et al. (2021).
In our study, SpCTP3 was induced to be expressed by the Cd treatment and Cd accumulation was increased in the transgenic lines. Then ROS levels were significantly elevated, which induced the up-regulated expression of SpCTP3, thereby promoting ROS detoxification. This is one of the reasons for the increased antioxidant enzyme activities in the SpCTP3-overexpressing transgenic lines. In addition, Cd induced the accumulation of ROS in the roots and leaves, ultimately resulting in oxidative stress, which is in accordance with the findings of recent studies involving other plant materials (Bari et al., 2019; Zhang et al., 2020a). In the current study, Cd stress induced the excessive accumulation of in the roots and leaves. Moreover, the H2O2 content increased as the treatment duration increased. To protect against excessive ROS, plants employ a complex array of antioxidant defense mechanisms to prevent or mitigate the oxidative damage caused by abiotic stresses (Shafi et al., 2022). Because they are signaling compounds, the accumulation of H2O2 and may increase the activities of CAT, SOD, POD, and other enzymes during plant responses to Cd stress (Wrzaczek et al., 2013). Superoxide dismutase activity is positively correlated with the intracellular ROS level within a certain range (Zhou et al., 2021). Under stress conditions, an increase in the ROS content increases the activities of SOD and other antioxidant enzymes (Gupta et al., 2017). In addition, SOD converts superoxide radicals into less toxic substances in reactions that produce H2O2 (Fujita and Hasanuzzaman, 2022). This may explain the observed accumulation of H2O2 in the transgenic poplar lines. Subsequently, CAT and POD are activated as H2O2 scavengers to maintain plant redox homeostasis (Tadjouri et al., 2022). In our study, the SpCTP3-overexpressing transgenic lines had significantly elevated CAT and POD activities after the Cd treatment, and the CAT activities were significantly higher in the Cd-treated transgenic lines than in the WT plants (Figure 6). Similarly, Cd stress reportedly causes the SOD, POD, and CAT activities in the above-ground parts of rice plants to increase to some extent to limit the toxic effects of Cd (Afzal et al., 2018). An exposure to Cd stress can significantly increase SOD, CAT, APX, and POD activities in marigold (Calendula calypso) by 150%, 79%, 97%, and 60%, respectively, leading to the conversion of H2O2 to non-toxic oxygen and water (Farooq et al., 2020).
4.3 SpCTP3 overexpression enhanced Cd transport by up-regulating the expression of transporter genes
The physiological process leading to the accumulation of Cd in above-ground plant parts is divided into the following three stages: root cell absorption; ectoplastic pathway-, symplast pathway-, and apoplast pathway-mediated transport; and above-ground accumulation (Uraguchi et al., 2009). Cadmium is transported to the above-ground tissues via numerous transporter proteins. In plants, the NRAMP proteins form one of the heavy metal transporter families in monocotyledons and dicotyledons (Ishida and Corcino, 2022). Their functions have been demonstrated in rice (Chang et al., 2020), poplar (Chen et al., 2019), and other plants (Li et al., 2021).
The ability of roots to absorb Cd is the decisive factor influencing the accumulation of Cd in plant shoots (Chaney, 2015). The overexpression of the S. alfredii SaNRAMP1H gene significantly increases the Cd contents of tobacco plants (above-ground and below-ground parts), implying that SaNRAMP1H promotes Cd uptake and accumulation (Zhang et al., 2020). The ZIP family proteins also function as heavy metal transporters. A previous study showed that Cd2+ can enter plant root cells through the ZIP transporter, which regulates Cd uptake, sequestration, and translocation (Ma et al., 2014). In addition, the overexpression of the Vicia sativa VsIRT1 gene in A. thaliana enhances the transport and accumulation of Cd (Zhang et al., 2020b). In poplar, NRAMP1.3, ZIP2, and ZIP6.2 encode plasma membrane proteins that control the entry of Cd2+ into root cells. In the current study, the Cd treatment up-regulated the NRAMP1, ZIP2, and ZIP6.2 expression levels and increased the cytoplasmic Cd content in the transgenic lines; the Cd content was higher in the transgenic lines than in the WT plants (Figure 8).
Cytosolic Cd2+ may bind to phytochelatins (PCs), which are synthesized from glutathione in a reaction catalyzed by PC synthase (PCS) (Liu et al., 2021). The generated PC–Cd complex can be transported to vesicles via ABC transporters (e.g., ABCC1 and ABCC2) (Feng et al., 2020). Cytoplasmic Cd2+ may also be transported into vesicles via MTP1, which is located in the vesicle membrane (Singh et al., 2021). In poplar, PCS, ABCC1, and MTP1 are differentially expressed in response to Cd2+. In the present study, PCS, ABCC1, and MTP1 expression levels increased 3.7-fold, 7.4-fold, and 16.4-fold, respectively, in the Cd-treated transgenic poplar plants. Earlier research confirmed HMA4 is localized to the plasma membrane, wherein it helps export divalent cations (e.g., Cd2+/Zn2+) from the cytoplasm and facilitates their transport into the xylem (He et al., 2015; Wang et al., 2020). A recent study indicated HMA4 is responsible for the transport of Cd2+ through the central cylinder and xylem (Wiyono et al., 2021). In the root system, HMA4 expression increased significantly in the transgenic lines exposed to Cd stress, which increased the transport of Cd from the roots to the above-ground parts. Moreover, HMA4 expression was up-regulated in the roots, but not in the leaves. These results suggest that the substantial accumulation of Cd in the transgenic lines may have been due to enhanced heavy metal transport.
5 Conclusion
Transgenic poplar plants overexpressing SpCTP3 accumulated more Cd in the above-ground tissues than the WT plants, likely because of changes to Cd distribution and chemical forms. The transgenic poplar lines also had a higher net influx of Cd2+ into the roots than the WT controls. Consistent with this observation, the expression levels of transporter genes (e.g., ABCC1, MTP1, and ZIP6.2) involved in Cd2+ transport and detoxification, were higher in the transgenic roots and leaves than in the WT roots and leaves after the Cd2+ treatment. Moreover, in response to the exposure to Cd stress, the , H2O2, and titratable acid concentrations in the roots and leaves were higher for the transgenic lines than for the WT plants. These findings indicate that the overexpression of SpCTP3 promoted the accumulation of Cd by increasing the organic acid content, up-regulating the expression of key transporter genes, and regulating oxidative homeostasis. The results of this study provide a theoretical basis for improving plant characteristics via SpCTP3 expression to increase the utility of poplar plants for remediating Cd-contaminated soil.
Data availability statement
The original contributions presented in the study are included in the article/Supplementary Material. Further inquiries can be directed to the corresponding authors.
Author contributions
XH, SL and RZ designed the experiments. SL performed the experiments and conducted the data. SL and XH wrote the manuscript. XH contributed to manuscript revision. MY, XL, WQ, JX and HL analyzed the data. All authors contributed to the article and approved the submitted version.
Funding
This work was supported by National Key Research and Development Program of China (No 2021YFD2200201) and National Nonprofit Institute Research Grant of CAF (No. RISFZ-2021-01). The authors declared no competing financial interests.
Conflict of interest
The authors declare that the research was conducted in the absence of any commercial or financial relationships that could be construed as a potential conflict of interest.
Publisher’s note
All claims expressed in this article are solely those of the authors and do not necessarily represent those of their affiliated organizations, or those of the publisher, the editors and the reviewers. Any product that may be evaluated in this article, or claim that may be made by its manufacturer, is not guaranteed or endorsed by the publisher.
Supplementary material
The Supplementary Material for this article can be found online at: https://www.frontiersin.org/articles/10.3389/fpls.2023.1111789/full#supplementary-material
References
Afzal, J., Hu, C., Imtiaz, M., Elyamine, A. M., Rana, M. S., Imran, M., et al. (2018). Cadmium tolerance in rice cultivars associated with antioxidant enzymes activities and Fe/Zn concentrations. Int. J. Environ. Sci. Technol. 16, 4241–4252. doi: 10.1007/s13762-018-2018-y
Akbari, M., Katam, R., Husain, R., Farajpour, M., Mahna, N. (2020). Sodium chloride induced stress responses of antioxidative activities in leaves and roots of pistachio rootstock. Biomolecules 10, 189. doi: 10.3390/biom10020189
Alexieva, V., Sergiev, I., Mapelli, S., Karanov, E. (2001). The effect of drought and ultraviolet radiation on growth and stress markers in pea and wheat. Plant Cell Environ. 24, 1337–1344. doi: 10.1046/j.1365-3040.2001.00778.x
Angulo-Bejarano, P. I., Puente-Rivera, J., Cruz-Ortega, R. (2021). Metal and metalloid toxicity in plants: An overview on molecular aspects. Plants (Basel) 10, 635. doi: 10.3390/plants10040635
Bari, M. A., Akther, M. S., Reza, M. A., Kabir, A. H. (2019). Cadmium tolerance is associated with the root-driven coordination of cadmium sequestration, iron regulation, and ROS scavenging in rice. Plant Physiol. Biochem. 136, 22–33. doi: 10.1016/j.plaphy.2019.01.007
Chai, H., Guo, J., Zhong, Y., Hsu, C. C., Zou, C., Wang, P., et al. (2020). The plasma-membrane polyamine transporter PUT3 is regulated by the Na +/H + antiporter SOS1 and protein kinase SOS2. New Phytol. 226, 785–797. doi: 10.1111/nph.16407
Chaney, R. L. (2015). How does contamination of rice soils with cd and zn cause high incidence of human cd disease in subsistence rice farmers. Curr. pollut. Rep. 1, 13–22. doi: 10.1007/s40726-015-0002-4
Chang, J., Huang, S., Yamaji, N., Zhang, W., Ma, J., Zhao, F., et al. (2020). OsNRAMP1 transporter contributes to cadmium and manganese uptake in rice. Plant Cell Environ. 43, 13843. doi: 10.1111/pce.13843
Chen, S., Han, X., Fang, J., Lu, Z., Qiu, W. (2017). Sedum alfredii SaNramp6 metal transporter contributes to cadmium accumulation in transgenic Arabidopsis thaliana. Sci. Rep. Uk 7, 13318. doi: 10.1038/s41598-017-13463-4
Chen, H., Wang, Y., Yang, H., Zeng, Q., Liu, Y. (2019). NRAMP1 promotes iron uptake at the late stage of iron deficiency in poplars. Tree Physiol. 39, 1235–1250. doi: 10.1093/treephys/tpz055
Cui, X., Mao, P., Sun, S., Huang, R., Fan, Y., Li, Y., et al. (2021). Phytoremediation of cadmium contaminated soils by amaranthus hypochondriacus l.: The effects of soil properties highlighting cation exchange capacity. Chemosphere 283, 131067. doi: 10.1016/j.chemosphere.2021.131067
Dai, L., Li, J., Harmens, H., Zheng, X., Zhang, C. (2020). Melatonin enhances drought resistance by regulating leaf stomatal behaviour, root growth and catalase activity in two contrasting rapeseed (Brassica napus l.) genotypes. Plant Physiol. Biochem. 149, 86–95. doi: 10.1016/j.plaphy.2020.01.039
Dineshkumar, M., Sivalingam, A., Thirumarimurugan, M. (2019). Phytoremediation potential of bacopa monnieri in the removal of heavy metals. J. Environ. Biol. 40, 753–757. doi: 10.22438/jeb/40/4(SI)/JEB_11
Dvorak, P., Krasylenko, Y., Zeiner, A., Samaj, J., Takac, T. (2020). Signaling toward reactive oxygen species-scavenging enzymes in plants. Front. Plant Sci. 11. doi: 10.3389/fpls.2020.618835
Farooq, A., Nadeem, M., Abbas, G., Shabbir, A., Khalid, M. S., Javeed, H. M. R., et al. (2020). Cadmium partitioning, physiological and oxidative stress responses in marigold (Calendula calypso) grown on contaminated soil: Implications for phytoremediation. Bull. Environ. Contam Toxicol. 105, 270–276. doi: 10.1007/s00128-020-02934-6
Feng, T., He, X., Zhuo, R., Qiao, G., Han, X., Qiu, W., et al. (2020). Identification and functional characterization of ABCC transporters for cd tolerance and accumulation in Sedum alfredii hance. Sci. Rep. 10, 20928. doi: 10.1038/s41598-020-78018-6
Feng, Y., Wu, Y., Zhang, J., Meng, Q., Wang, Q., Ma, L., et al. (2018). Ectopic expression of SaNRAMP3 from Sedum alfredii enhanced cadmium root-to-shoot transport in Brassica juncea. Ecotoxicol Environ. Saf. 156, 279–286. doi: 10.1016/j.ecoenv.2018.03.031
Fujita, M., Hasanuzzaman, M. (2022). Approaches to enhancing antioxidant defense in plants. Antioxidants 11, 925. doi: 10.3390/antiox11050925
Fu, Z., Xi, S. (2020). The effects of heavy metals on human metabolism. Toxicol. Mech. Methods 30, 167–176. doi: 10.1080/15376516.2019.1701594
Gupta, D. K., Pena, L. B., Romero Puertas, M. C., Hernández, A., Inouhe, M., Sandalio, L. M., et al. (2017). NADPH oxidases differentially regulate ROS metabolism and nutrient uptake under cadmium toxicity. Plant Cell Environ. 40, 509–526. doi: 10.1111/pce.12711
Haghighi, M., Kafi, M., Khoshgoftarmanesh, A. (2013). Effect of humic acid application on cadmium accumulation by lettuce leaves. J. Plant Nutr. 36 (10), 1521–1532. doi: 10.1080/01904167.2013.799182
Hall, J. L. (2002). Cellular mechanisms for heavy metal detoxification and tolerance. J. Exp. Bot. 53, 1–11. doi: 10.1093/jexbot/53.366.1
Hamid, Y., Tang, L., Hussain, B., Usman, M., Lin, Q., Rashid, M. S., et al. (2020). Organic soil additives for the remediation of cadmium contaminated soils and their impact on the soil-plant system: A review. Sci. Total Environ. 707, 136121. doi: 10.1016/j.scitotenv.2019.136121
Han, X., Zhang, Y., Yu, M., Zhang, J., Xu, D., Lu, Z., et al. (2020). Transporters and ascorbate-glutathione metabolism for differential cadmium accumulation and tolerance in two contrasting willow genotypes. Tree Physiol. 40, 1126–1142. doi: 10.1093/treephys/tpaa029
He, J., Li, H., Ma, C., Zhang, Y., Polle, A., Rennenberg, H., et al. (2015). Overexpression of bacterial gamma-glutamylcysteine synthetase mediates changes in cadmium influx, allocation and detoxification in poplar. New Phytol. 205, 240–254. doi: 10.1111/nph.13013
Huo, Y., Hu, H., Peng, S., Chen, Q. (2009). Contents and changes of organic acid in sand pears from different germplasm resources. Scientia Agricultura Sin. 42 (01), 216–223. doi: 10.3864/j.issn.0578-1752.2009.01.027
Ishida, J. K., Corcino, D. (2022). Role of natural resistance-associated macrophage proteins in metal ion transport in plants. Cation Transporters Plants chapter 18, 337–356. doi: 10.1016/B978-0-323-85790-1.00003-8
Jiang, Y., Han, J., Xue, W., Wang, J. (2021). Overexpression of SmZIP plays important roles in cd accumulation and translocation, subcellular distribution, and chemical forms in transgenic tobacco under cd stress. Ecotoxicol Environ. Saf. 214, 112097. doi: 10.1016/j.ecoenv.2021.112097
Jiang, H., Yang, J., Zhang, J. (2007). Effects of external phosphorus on the cell ultrastructure and the chlorophyll content of maize under cadmium and zinc stress. Environ. pollut. 147, 750–756. doi: 10.1016/j.envpol.2006.09.006
Jia, H., Yin, Z., Xuan, D., Lian, W., Han, D., Zhu, Z., et al. (2022). Mutation of NtNRAMP3 improves cadmium tolerance and its accumulation in tobacco leaves by regulating the subcellular distribution of cadmium. J. Hazard Mater 432, 128701. doi: 10.1016/j.jhazmat.2022.128701
Jing, X., Zhou, M., Nie, X., Zhang, L., Chen, K. (2020). OsGSTU6 contributes to cadmium stress tolerance in rice by involving in intracellular ROS homeostasis. J. Plant Growth Regul. 2021, 945–61. doi: 10.1007/s00344-020-10148-7
Kudo, H., Inoue, C., Sugawara, K. (2021). Effects of growth stage and cd chemical form on cd and zn accumulation in Arabidopsis halleri ssp. gemmifera. Int. J. Environ. Res. Public Health 18, 4214. doi: 10.3390/ijerph18084214
Lai, J., Liu, Z., Li, C., Luo, X. (2021). Analysis of accumulation and phytotoxicity mechanism of uranium and cadmium in two sweet potato cultivars. J. Hazard Mater 409, 124997. doi: 10.1016/j.jhazmat.2020.124997
Li, C., Dang, F., Cang, L., Zhou, C., Zhou, D. (2014). Integration of metal chemical forms and subcellular partitioning to understand metal toxicity in two lettuce (Lactuca sativa l.) cultivars. Plant Soil 384, 201–212. doi: 10.1007/s11104-014-2194-6
Li, J., Duan, Y., Han, Z., Shang, X., Zhang, K., Zou, Z., et al. (2021). Genome-wide identification and expression analysis of the NRAMP family genes in tea plant (Camellia sinensis). Plants (Basel) 10, 1055. doi: 10.3390/plants10061055
Li, Z., Han, X., Song, X., Zhang, Y., Jiang, J., Han, Q., et al. (2017). Overexpressing the Sedum alfredii Cu/Zn superoxide dismutase increased resistance to oxidative stress in transgenic Arabidopsis. Front. Plant Sci. 8. doi: 10.3389/fpls.2017.01010
Li, X., Li, B., Zheng, Y., Luo, L., Qin, X., Yang, Y., et al. (2022). Physiological and rhizospheric response characteristics to cadmium of a newly identified cadmium accumulator Coreopsis grandiflora hogg. (Asteraceae). Ecotoxicol Environ. Saf. 241, 113739. doi: 10.1016/j.ecoenv.2022.113739
Lin, Y., Aarts, M. (2012). The molecular mechanism of zinc and cadmium stress response in plants. Cell Mol. Life Sci. 69, 3187–3206. doi: 10.1007/s00018-012-1089-z
Liu, M., He, X., Feng, T., Zhuo, R., Qiu, W., Han, X., et al. (2019). cDNA library for mining functional genes in sedum alfredii hance related to cadmium tolerance and characterization of the roles of a novel SaCTP2 gene in enhancing cadmium hyperaccumulation. Environ. Sci. Technol. 53, 10926–10940. doi: 10.1021/acs.est.9b03237
Liu, J., Zhang, J., Kim, S. H., Lee, H. S., Marinoia, E., Song, W., et al. (2021). Characterization of Brassica rapa metallothionein and phytochelatin synthase genes potentially involved in heavy metal detoxification. PloS One 16, e252899. doi: 10.1371/journal.pone.0252899
Li, C., Yang, X., Xu, Y., Li, L., Wang, Y. (2018). Cadmium detoxification induced by salt stress improves cadmium tolerance of multi-stress-tolerant Pichia kudriavzevii. Environ. pollut. 242, 845–854. doi: 10.1016/j.envpol.2018.07.058
Long, X., Yang, X., Zheng-Qian, Y. (2003). Metal chelators in hyperaccumulator and their application in phytoremedi- ation. Plant Physiol. Commun. 39, 71–77. doi: 10.1021/js980090u
Ma, Y., He, J., Ma, C., Luo, J., Li, H., Liu, T., et al. (2014). Ectomycorrhizas with Paxillus involutus enhance cadmium uptake and tolerance in Populus x canescens. Plant Cell Environ. 37, 627–642. doi: 10.1111/pce.12183
Meng, X., Li, W., Shen, R., Lan, P. (2022). Ectopic expression of IMA small peptide genes confers tolerance to cadmium stress in Arabidopsis through activating the iron deficiency response. J. Hazard Mater 422, 126913. doi: 10.1016/j.jhazmat.2021.126913
MEP, MLR (2014). Nationwide soil pollution survey report (Ministry of Environment Protection and Ministry of Land Resources of the People’ s Republic of China).
Ouyang, J., Li, B., Xue, W., Jiang, Y., Li, C., Shang, X., et al. (2019). Cadmium uptake and accumulation, subcellular distribution and chemical forms in young seedlings of Salix babylonica l. Fresenius Environ. Bull. 28, 3637–3648.
Park, J., Song, W., Ko, D., Eom, Y., Lee, Y. (2012). The phytochelatin transporters AtABCC1 and AtABCC2 mediate tolerance to cadmium and mercury. Plant J. 69, 278–288. doi: 10.1111/j.1365-313X.2011.04789.x
Patricio, A. B., María del, C. R. G., Santos, M. E. P., Montao, Y. A. R. (2018). Effect of foliar copper application on yield and anthocyanin concentration in hibiscus sabdariffa calyces. Rev. Fac Cienc Agrar 50 (2), 65–75.
Peng, J., Wang, Y., Ding, G., Ma, H., Zhang, Y., Gong, J., et al. (2017). A pivotal role of cell wall in cadmium accumulation in the crassulaceae hyperaccumulator Sedum plumbizincicola. Mol. Plant 10, 4. doi: 10.1016/j.molp.2016.12.007
Qiu, Q., Wang, Y., Yang, Z., Yuan, J. (2011). Effects of phosphorus supplied in soil on subcellular distribution and chemical forms of cadmium in two Chinese flowering cabbage (Brassica parachinensis l.) cultivars differing in cadmium accumulation. Food Chem. Toxicol. 49, 2260–2267. doi: 10.1016/j.fct.2011.06.024
Rasafi, T. E., Oukarroum, A., Haddioui, A., Song, H., Rinklebe, J. (2020). Cadmium stress in plants: A critical review of the effects, mechanisms, and tolerance strategies. Crit. Rev. Environ. Sci. Technol. doi: 10.1080/10643389.2020.1835435
Raza, A., Habib, M., Kakavand, S. N., Zahid, Z., Zahra, N., Sharif, R., et al. (2020). Phytoremediation of cadmium: Physiological, biochemical, and molecular mechanisms. Biol. (Basel) 9, 177. doi: 10.3390/biology9070177
Rehman, M. Z. U., Zafar, M., Waris, A. A., Rizwan, M., Shafaqat Ali, M. S., Sabir, M., et al. (2020). Residual effects of frequently available organic amendments on cadmium bioavailability and accumulation in wheat - ScienceDirect. Chemosphere 244, 125548. doi: 10.1016/j.chemosphere.2019.125548
Shafi, A., Hassan, F., Khanday, F. A. (2022). “Reactive oxygen and nitrogen species: Oxidative damage and antioxidative defense mechanism in plants under abiotic stress,” (Apple Academic Press), 71–99.
Shamelashvili, K., Svenlana, O., Shatorna, V. (2020). The toxic effect of cadmium on a living organism and its detoxification by zinc ions. Modern Sci. — Moderní Věda 3, 150–157.
Shi, W., Li, J., Kan, D., Yu, W., Chen, X. (2022). Sulfur metabolism, organic acid accumulation and phytohormone regulation are crucial physiological processes modulating the different tolerance to pb stress of two contrasting poplars. Tree Physiol. 9), 9. doi: 10.1093/treephys/tpac033
Singh, R., Kesavan, A. K., Landi, M., Kaur, S., Thakur, S., Zheng, B., et al. (2020). 5-aminolevulinic acid regulates Krebs cycle, antioxidative system and gene expression in Brassica juncea l. J. Biotechnol. 323, 283–292. doi: 10.1016/j.jbiotec.2020.09.004
Singh, R., Misra, A. N., Sharma, P. (2021). Differential responses of thiol metabolism and genes involved in arsenic detoxification in tolerant and sensitive genotypes of bioenergy crop Ricinus communis. Protoplasma 258, 391–401. doi: 10.1007/s00709-020-01577-y
Singh, P., Singh, I., Shah, K. (2020). Alterations in antioxidative machinery and growth parameters upon application of nitric oxide donor that reduces detrimental effects of cadmium in rice seedlings with increasing days of growth - ScienceDirect. South Afr. J. Bot. 131, 283–294. doi: 10.1016/j.sajb.2020.02.022
Tadjouri, H., Medjedded, H., Nemmiche, S., Chadli, R., Moulay, M. (2022). Stress response induced by cadmium in soybeans (Glycine max l.) and health risk assessment. Plant Physiol. Rep., 1–8. doi: 10.1007/s40502-022-00663-y
Uraguchi, S., Mori, S., Kuramata, M., Kawasaki, A., Arao, T., Ishikawa, S, et al. (2009). Root-to-shoot cd translocation via the xylem is the major process determining shoot and grain cadmium accumulation in rice. J. Exp. Bot. 60, 2677–2688. doi: 10.1093/jxb/erp119
Viehweger, K. (2014). How plants cope with heavy metals. Bot. Stud. 55, 35. doi: 10.1186/1999-3110-55-35
Wang, J., Chen, X., Chu, S., You, Y., Zhou, P. (2021). Comparative cytology combined with transcriptomic and metabolomic analyses of Solanum nigrum l. J. Hazardous Materials 423, 127168. doi: 10.1016/j.jhazmat.2021.127168
Wang, H., Chen, W., Sinumvayabo, N., Li, Y., Zhang, Y. (2020). Phosphorus deficiency induces root proliferation and cd absorption but inhibits cd tolerance and cd translocation in roots of Populus × euramericana. Ecotoxicol Environ. Saf. 204, 111148. doi: 10.1016/j.ecoenv.2020.111148
Wan, X., Lei, M., Chen, T. (2016). Cost-benefit calculation of phytoremediation technology for heavy-metal-contaminated soil. Sci. Total Environ. 563-564, 796–802. doi: 10.1016/j.scitotenv.2015.12.080
Wen, S., Ge, X., Wang, R., Yang, H., Bai, Y., Guo, Y., et al. (2022). An efficient agrobacterium-mediated transformation method for hybrid poplar 84K (Populus alba× P. glandulosa) using calli as explants. Int. J. Mol. Sci. 23, 2216. doi: 10.3390/ijms23042216
Wiyono, C., Inoue, C., Chien, M. F. (2021). HMA4 and IRT3 as indicators accounting for different responses to cd and zn by hyperaccumulator Arabidopsis halleri ssp. gemmifera. Plant Stress 2, 100042. doi: 10.1016/j.stress.2021.100042
Wrzaczek, M., Brosché, M., Kangasj Rvi, J. (2013). ROS signaling loops - production, perception, regulation. Curr. Opin. Plant Biol. 16, 575–582. doi: 10.1016/j.pbi.2013.07.002
Wu, H., Wang, J., Li, B., Ouyang, J., Wang, J., Shi, Q., et al. (2016). Salix matsudana koidz tolerance mechanisms to cadmium: Uptake and accumulation, subcellular distribution, and chemical forms. Polish J. Environ. Stud. 25, 1739–1747. doi: 10.15244/pjoes/62715
Xin, J., Huang, B. (2014). Subcellular distribution and chemical forms of cadmium in two hot pepper cultivars differing in cadmium accumulation. J. Agric. Food Chem. 62, 508–515. doi: 10.1021/jf4044524
Xin, J., Zhao, X., Tan, Q., Sun, X., Hu, C. (2017). Comparison of cadmium absorption, translocation, subcellular distribution and chemical forms between two radish cultivars (Raphanus sativus l.). Ecotoxicol Environ. Saf. 145, 258–265. doi: 10.1016/j.ecoenv.2017.07.042
Yaashikaa, P. R., Kumar, P. S., Jeevanantham, S., Saravanan, R. (2022). A review on bioremediation approach for heavy metal detoxification and accumulation in plants. Environ. pollut. 301, 119035. doi: 10.1016/j.envpol.2022.119035
Yang, L., Li, N., Kang, Y., Liu, J., Wang, Y., Sun, H., et al. (2022). Selenium alleviates toxicity in Amaranthus hypochondriacus by modulating the synthesis of thiol compounds and the subcellular distribution of cadmium. Chemosphere 291, 133108. doi: 10.1016/j.chemosphere.2021.133108
Yazdi, M. E. T. Y. (2021). Bio-indicators in cadmium toxicity: Role of HSP27 and HSP70. Environ. Sci. pollut. Res. Int. 28, 26359–26379. doi: 10.1007/s11356-021-13687-y
Yessica, E. Z., Martinez-Nava, G., Reyes-Hinojosa, D., Mendoza-Soto, L., Fernandez-Torres, J., López-Reyes, A., et al. (2020). Impact of cadmium toxicity on cartilage loss in a 3D in vitro model. Environ. Toxicol. Pharmacol. 74, 103307. doi: 10.1016/j.etap.2019.103307
Yue, X., Song, J., Fang, B., Wang, L., Zou, J., Su, N., et al. (2021). BcNRAMP1 promotes the absorption of cadmium and manganese in Arabidopsis. Chemosphere 283, 131113. doi: 10.1016/j.chemosphere.2021.131113
Zhang, Y., Chao, J., Li, X., Zhang, C., Khan, R., Du, S., et al. (2021). Comparative transcriptome combined with biochemical and physiological analyses provide new insights toward cadmium accumulation with two contrasting Nicotiana species. Physiol. Plant 173, 369–383. doi: 10.1111/ppl.13431
Zhang, H., Li, X., Xu, Z., Wang, Y., Teng, Z., An, M., et al. (2020). Toxic effects of heavy metals Pb and cd on mulberry (Morus alba l.) seedling leaves: Photosynthetic function and reactive oxygen species (ROS) metabolism responses. Ecotoxicol Environ. Saf. 195, 110469. doi: 10.1016/j.ecoenv.2020.110469
Zhang, X., Li, X., Zhang, X., Li, X., Tang, L., Guo, Y., et al. (2020b). The root iron transporter 1 governs cadmium uptake in Vicia sativa roots. J. Hazard Mater 398, 122873. doi: 10.1016/j.jhazmat.2020.122873
Zhang, L., Wu, J., Tang, Z., Huang, X., Wang, X., Wang, X., et al. (2019). Variation in the BrHMA3 coding region controls natural variation in cadmium accumulation in Brassica rapa vegetables. J. Exp. Bot. 70, 5865–5878. doi: 10.1093/jxb/erz310
Zhang, X., Yang, Z., Li, Z., Zhang, F., Hao, L. (2020a). Effects of drought stress on physiology and antioxidative activity in two varieties of Cynanchum thesioides. Braz. J. Bot. 43, 1–10. doi: 10.1007/s40415-019-00573-8
Zhang, K., Yuan, J., Kong, W., Yang, Z. (2013). Genotype variations in cadmium and lead accumulations of leafy lettuce (Lactuca sativa l.) and screening for pollution-safe cultivars for food safety. Environ. Sci-Proc Imp 15 (6), 1245–1255. doi: 10.1039/C3EM00158J
Zhang, J., Zhang, M., Song, H., Zhao, J., Yang, X. (2020). A novel plasma membrane-based NRAMP transporter contributes to cd and zn hyperaccumulation in Sedum alfredii hance. Environ. Exp. Bot. 176, 104121. doi: 10.1016/j.envexpbot.2020.104121
Zhou, Y., Wang, Y., Inyang, A. I. (2021). Ecophysiological differences between five mangrove seedlings under heavy metal stress. Mar. pollut. Bull. 172, 112900. doi: 10.1016/j.marpolbul.2021.112900
Keywords: cadmium tolerance protein 3, Sedum plumbizincicola, cadmium stress, subcellular distribution, transgenic poplars
Citation: Li S, Zhuo R, Yu M, Lin X, Xu J, Qiu W, Li H and Han X (2023) A novel gene SpCTP3 from the hyperaccumulator Sedum plumbizincicola redistributes cadmium and increases its accumulation in transgenic Populus × canescens. Front. Plant Sci. 14:1111789. doi: 10.3389/fpls.2023.1111789
Received: 30 November 2022; Accepted: 25 January 2023;
Published: 08 February 2023.
Edited by:
Diaa Abd El Moneim, Arish University, EgyptReviewed by:
Jagna Chmielowska-Bąk, Adam Mickiewicz University, PolandEwa Joanna Hanus-Fajerska, University of Agriculture in Krakow, Poland
Copyright © 2023 Li, Zhuo, Yu, Lin, Xu, Qiu, Li and Han. This is an open-access article distributed under the terms of the Creative Commons Attribution License (CC BY). The use, distribution or reproduction in other forums is permitted, provided the original author(s) and the copyright owner(s) are credited and that the original publication in this journal is cited, in accordance with accepted academic practice. No use, distribution or reproduction is permitted which does not comply with these terms.
*Correspondence: Haiying Li, lihaiying_2004@163.com; Xiaojiao Han, hanxiaojiao1004@163.com
†These authors have contributed equally to this work