- 1Colegio de Ciencias Biológicas y Ambientales, Universidad San Francisco de Quito, Quito, Ecuador
- 2Instituto Biósfera, Universidad San Francisco de Quito, Quito, Ecuador
- 3Department of Biology, University of Nebraska Omaha, Omaha, NE, United States
The high-elevation peatlands of the páramos of the northern Andes constitute a diverse environment that harbors large numbers of species and several types of plant communities along altitudinal, latitudinal, and environmental gradients. However, little is known about the structure and functioning of these ecosystems, including peatland vegetation types and their relative contribution to the production and accumulation of peat soils. In this paper we characterized the structure of peatland plant communities of the humid páramos of northern Ecuador by describing the distribution of plant growth-forms and their aboveground biomass patterns. Along an elevation gradient of 640 m we sampled vegetation in 16 peatlands and aboveground biomass in four peatlands. Three distinct peatland vegetation types were identified: High elevation Cushion peatlands, dominated by Plantago rigida and Distichia muscoides, Sedge and rush peatlands dominated by Carex spp. and Juncus spp., and Herbaceous and shrubby peatlands, with a more heterogenous and structurally complex vegetation. In terms of aboveground biomass, we found an 8-fold reduction in the higher peatlands compared to the lower sites, suggesting that the steep elevational gradients characteristic of Andean environments might be crucial in structuring the physiognomy and composition of peatland vegetation, either through its effects on temperature and other environmental factors, or through its effects on the age and development of soils. Additional studies are needed to evaluate the potential effects of temperature, hydrology, micro-topography, geological setting, and land-use, which are likely to influence vegetation patters in these peatlands.
Introduction
The South American páramos are a striking biome of the northern Andes. Guarding the highest mountain peaks of Venezuela, Colombia, Ecuador and northern Perú, these complex and diverse ecosystems emerge above the tree-line covering vast areas shaped by a long history of glacial and, in some regions, volcanic activity (Balslev and Luteyn, 1992). The resulting topography is tortuous, crisscrossed by deep ravines, glacial terraces, and steep slopes where the strong elevation gradient (roughly between 3200 m and the lower limit of glaciers) drastically affects the nature of biotic communities (Hofstede et al., 2014). Páramo ecosystems have been widely recognized for their extremely high levels of biodiversity and endemism, but also as natural laboratories to understand biogeographical and evolutionary patterns that control the development and distribution of high-elevation plant species and their sensitivity to climate change (Madriñán et al., 2013; Anthelme et al., 2014; Sanchez-Baracaldo and Thomas, 2014). Specifically, the physiognomy and species composition of plant communities and the role of soils in regulating hydrological flows have been extensively studied with emphasis on the impacts of burning, grazing, expanding agriculture, afforestation and, more recently, climate change (Hofstede and Rossenaar, 1995; Suárez and Medina, 2001; Farley et al., 2004; Anthelme et al., 2021; Lasso et al., 2021; Thompson et al., 2021). However, most studies have only considered well-drained upland soils, thus overlooking a vast and complex system of páramo peatlands that, in some areas, can cover up to 25% of the páramo landscape (Hribljan et al., 2017) and potentially contribute substantially to the diversity and functioning of páramo ecosystem. Previous research on these peatlands of the northern Andes has been mostly focused on the patterns of carbon dynamics and storage (Cooper et al., 2015; Hribljan et al., 2016; Hribljan et al., 2017), the environmental factors that control the distribution of peat forming species, and the high rates of peat accumulation reported for these environments (Benavides et al., 2013; Benfield et al., 2021). However, little is known about the structure and functioning of these ecosystems, including critical information regarding the distribution of different peatland vegetation types and their relative contribution to the production and accumulation of peat soils. In this paper we offer a first attempt at understanding the composition, structure, and aboveground biomass patterns of high-elevation peatland communities along an elevation gradient in the páramos of northern Ecuador.
Research on the vegetation of páramo peatlands is limited to a few studies that emphasized the description of vegetation types, and patterns of carbon dynamics. These studies have reported large heterogeneity in plant community structure, mostly related to elevation, the chemical characteristics of ground water inputs into the peatlands and other environmental (e.g. water level) and anthropogenic disturbance gradients (Schmidt-Mumm and Vargas Ríos, 2012; Ruthsatz et al., 2020; Benavides et al., 2023). Deil et al. (2011) defined eleven plant associations for peatlands along the Andes, including two associations (Muhlenbergia fastigiata-Distichlis and Gentiana sedifolia-Carex) that coarsely coincide with some peatland plant communities of the northern Andes. In contrast, Schmidt-Mumm and Vargas Ríos (2012) recognized eighteen separate plant communities that occur across terrestrial-aquatic transition in the páramo of Chingaza in central Colombia.
Páramo peatlands have also been recognized for their high rates of peat-accumulation, which are among the highest for mountain peatlands worldwide (Chimner and Karberg, 2008; Benavides et al., 2013; Hribljan et al., 2016). The rapid peat-growth rates in these peatlands are attributed to highly productive vegetation communities and, in recent geological times, to increased mineral nutrients and warmer temperatures (Hribljan et al., 2016; Benfield et al., 2021; Suárez et al., 2021). Therefore, plant community structure and growth are significant factors in the functioning of these peatlands both as a source of organic matter and as a protective layer for the underlying peat soils.
In a previous study on north-eastern Ecuador’s páramo peatlands we offered a first evaluation of peatland types found at elevations above 3500 m (Hribljan et al., 2017). By using field surveys and a multi-date, multi-sensor technique, we were able to delineate three main types of páramo peatlands and discern their individual contribution to soil carbon storage at the landscape level. Despite the accuracy of the mapping technique that we used, questions remain about the precision of that classification at smaller scales, and about the structure and composition of those vegetation types. Moreover, additional information is needed to explore the environmental factors that might control the different peatland vegetation types found in this part of the northern Andes. This information is essential to future studies focusing on water regulation mechanisms and carbon accumulation patterns in these ecosystems, as well as conservation and restoration of an ecosystem that has been severely damaged by grazing, drainage, and land-use change across its distribution (Suárez et al., 2022a). From this perspective, the main objectives of this study are to i) contribute to the description of the composition and structure of peatland plant communities of the northern Ecuador’s humid páramos and ii) describe their aboveground biomass patterns. For this exploratory study we described the structure of the plant communities by characterizing the coverage of the main plant growth forms (sensu Ramsay and Oxley, 1997) because they might be more useful than individual species in terms of exploring the physiognomy and functional attributes of the peatlands (e.g. carbon storage). Ultimately our goal is to provide a basis for better management and future research in a critical component of the páramo ecosystems that has been largely overlooked and plays a significant role in the ecosystem services provided by this environment.
Materials and methods
Study area
This study was conducted in the Chakana caldera, a volcanic system spanning roughly 50 km in the Eastern branch of Ecuador’s northern Andes with the last volcanic activity registered in the 1700s (Hall and Mothes, 2008). This area is characterized by carbon dense organic soils interbedded with volcanic ash and pumice layers (Hribljan et al., 2016). Glacial activity across the greater landscape created a complex and steep topography that contributes additional mineral inputs from side slope erosion. Our study sites straddle the Andean continental divide, at elevations between 3700 and 4340 m, and experience mean annual temperatures that range from 6°C at 3600 m, to 3.7°C at 4300 m (Suarez E., unpublished data). Precipitation at 3600 m can reach 1300 to 1700 mm yr-1, but clouds and mist coming from the Amazon basin supply a large amount of water that still needs to be quantified. The steep slopes of this volcanic complex are typically dominated by tussock grasses (e.g. Calamagrostis spp., Festuca spp.) interspersed with shrubs (e.g. Monticalia, Hypericum, Diplostephium spp., Valeriana spp.), and a rich layer of herbs and scandent vegetation including characteristic species of the genera Hypochaeris, Werneria, Senecio, and Phlegmariurus, among others. In the midst of these grasslands, glacial and volcanic activity carved a complex system of peatlands that, in this particular area, cover at least 24% of the landscape between 3500 and 4300 m (Hribljan et al., 2017).
For this study we analyzed vegetation patterns at 16 individual peatlands spanning diverse geographical settings and located between 3700 and 4340 m in the Cayambe-Coca National Park and its buffer zone (0° 19.417’S, 78° 12.180’W; 0° 14.370’S, 78° 7.929’W). In order to ensure a representative sample of the peatlands in this region, we selected sites that i) represented the elevation gradient of the study area, ii) showed little or no signs of recent human disturbance, and iii) encompassed all the general vegetation types that had been reported in the region (Hribljan et al., 2017). However, it must be noted that our selection of sites was largely biased towards peatlands that occurred within 5 km of roads. Peatlands which occurred alongside of a road and showed signs of potential hydrological impacts were not included. Additionally, four of those sites, exhibiting similar slope, aspect, and general topography within the same watershed and showing no signs of recent human disturbance, were selected to characterize altitudinal changes in aboveground biomass.
Plant community characterization
Percent cover and taxonomic identity of vascular and non-vascular plant species present in a set of eight randomly located plots (1x1 m) within each peatland were recorded. Vegetation data from plots were summarized by growth form (Ramsay and Oxley, 1997) and classified using hierarchical, polythetic, agglomerative cluster analysis using Sørensen distance measure and flexible beta linkages method with β= -0.25 using Vegan package in R 4.1.1 (Oksanen et al., 2022). None of the growth form categories proposed by Ramsay and Oxley (1997) were adequate to describe the stands of graminoid species which do not form tussocks and occur in more or less continuous stands or matts in poorly drained areas. To account for these species, we divided the tussock growth form into two groups: tussock graminoids (Calamagrostis spp., Festuca spp., and Cortaderia spp.) and non-tussock graminoids (e.g. Bromus lanatus, Agrostis spp., Carex spp., Eleocharis spp., Uncinia spp., and Juncus spp.). Indicator species analysis coupled with Monte Carlo Analysis provided a quantitative, objective criterion to prune the dendrogram and optimize the number of clusters (McCune et al., 2002; De Cáceres and Legendre, 2009). The cluster level with the smallest average p-value derived from the indicator species analysis was used as the optimal clustering level. Dominant growth forms for each group were determined using the Indicator Value Index (IndVal) component B while indicative growth forms were determined using IndVal component A (De Cáceres and Legendre, 2009).
In order to support the results of the cluster analysis, ordination of vegetation communities was also conducted on non-transformed data using non-metric Multidimensional Scaling (nMDS). The ordination solution was obtained in R® 4.1.1 using Bray–Curtis distance measure and the r-function metaMDS for constructing multiple runs, finding the best solution for each dimensionality with a stress less than 0.2.
Aboveground biomass
Plant biomass varies widely across species and environmental conditions, which means that gathering field-based ecosystem-scale estimates of aboveground biomass can be challenging. From this perspective, for this study we used plant growth forms (sensu Ramsay and Oxley, 1997) to estimate aboveground biomass. Plant growth forms are an attempt to group species that share architectural and functional characteristics which probably emerged among different species as a comparable response to significant environmental constraints. Our use of plant growth forms is based on the assumption that the variation in aboveground biomass will be lower within growth-form categories, than among individual species, or taxonomic groups.
Altitudinal changes in aboveground biomass were examined in four of the 16 peatlands located at 3920, 4125, 4240, and 4300 m. An additional measurement of plant cover in these four peatlands was conducted using a line intercept method (Floyd and Anderson, 1987; Carlsson et al., 2005; Rochefort et al., 2013) along 10 m long transects that were positioned in a stratified arrangement. This additional measurement of plant cover was intended to provide a more detailed measurement of the variability in plant community structure present at these sites. The number of transects measured in each site varied between 10 and 22, depending on the size of the peatland and the heterogeneity of plant cover. Along each transect we recorded the distance of the transect that intersected different growth forms and the dominant species of each one.
Aboveground biomass in these four peatlands was estimated by combining percent growth-form cover determined from the transect surveys with plant biomass estimates for each representative growth form found at each elevation. Polygons for each peatland were generated using a Garmin 60 CX GPS and uploaded into ArcGis® where we estimated peatland surface area using the assumption that all our sites were essentially flat. The average percent cover along the transects was used to calculate the proportion of each peatland’s area occupied by the eleven different plant growth forms. We then collected four to six samples of each growth form present in each site using a 30 x 30 cm PVC quadrat randomly positioned over representative individuals or patches of the most frequent species of each growth form, based on the data collected along the transects described above. For each sample, we clipped all aerial biomass within the quadrat down to where the first roots appeared on the stems. In the case of shrubs and tussock grasses, we excluded any aerial biomass that projected outside of the frame of the quadrat. Plant biomass samples were placed in bags, transported to the Laboratory of Aquatic Ecology at Universidad San Francisco de Quito, and dried at 65°C to a constant weight. The samples were then cut and homogenized, and replicate subsamples were reduced to ash in a muffle furnace (550°C for 5 hours). The ash content was used to calculate ash-free biomass of each sample. Finally, mean aboveground biomass of each peatland was calculated using the average biomass contributed by each growth form according to their proportional percentage of ground cover in each site.
Results
Vegetation community structure
Three distinct peatland vegetation communities were identified by the cluster analysis (Table 1) and by the nMDS (Figure 1):
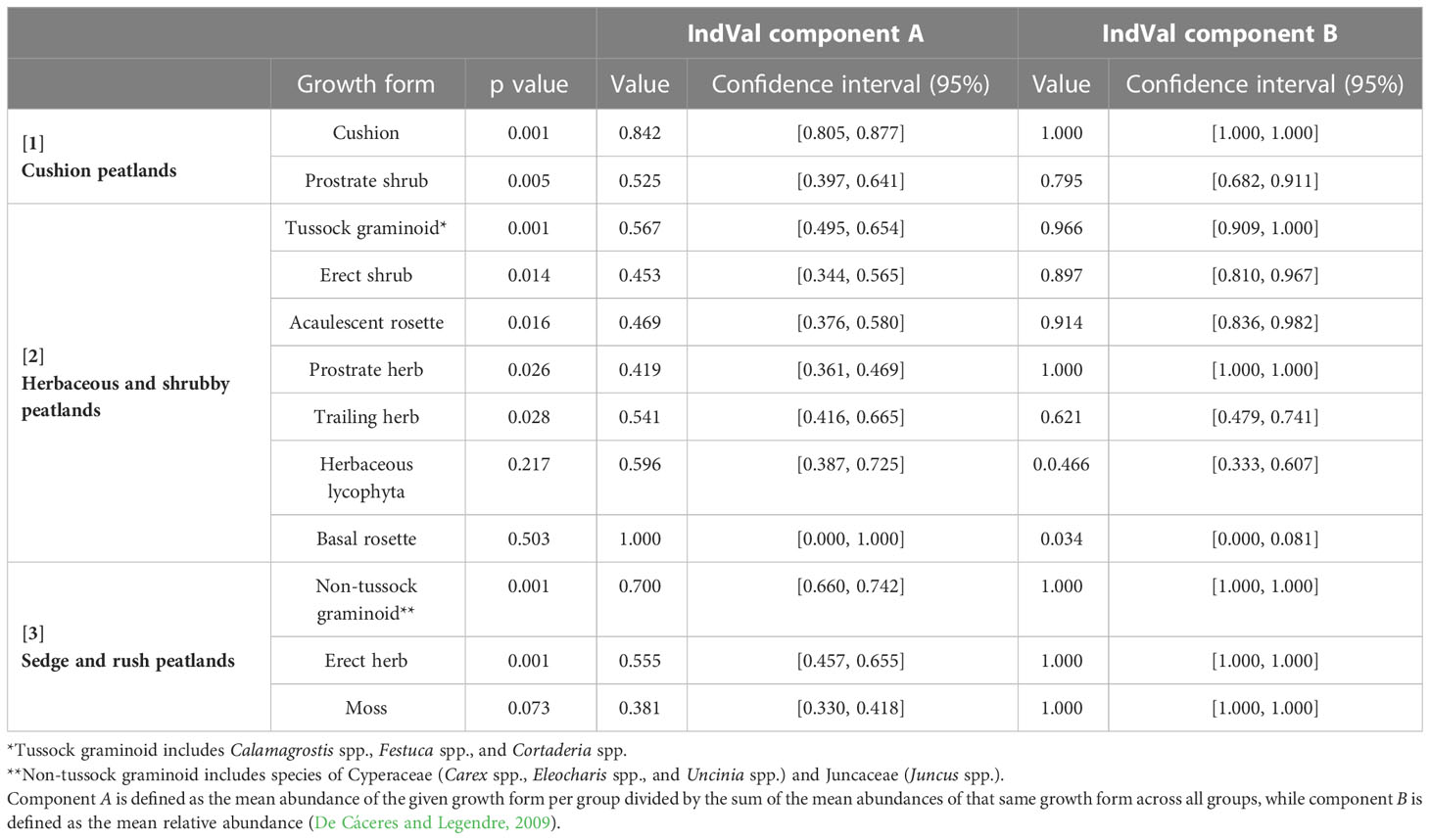
Table 1 Peatland growth form groups and accompanying group association (p value), indicator (IndVal component A) and dominance values (IndVal component B) based on data from 120 peatland plots within Cayambe-Coca National Park and its buffer zone.
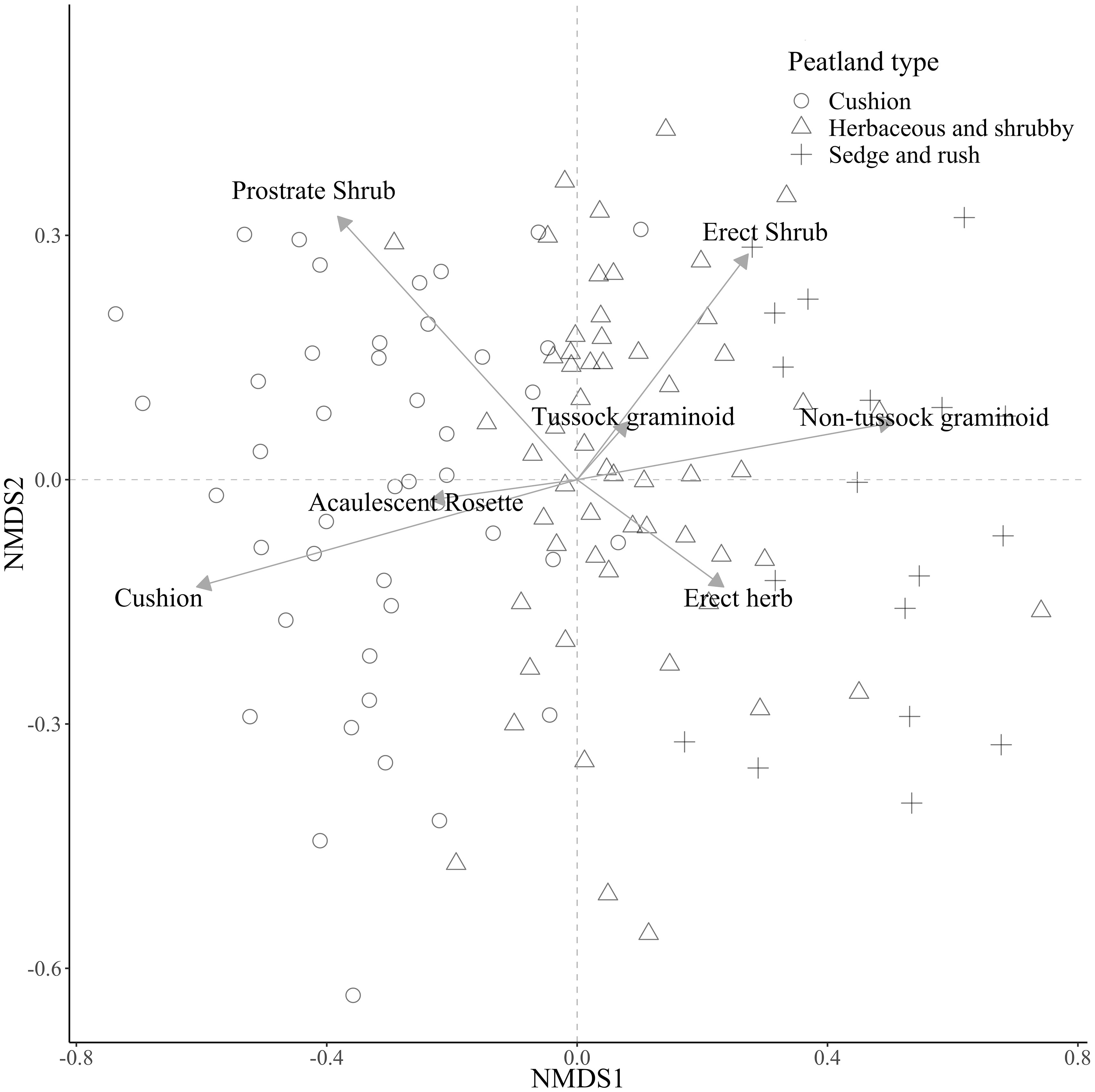
Figure 1 Results of a Non-metric Multidimensional Scaling (nMDS) performed on the vegetation cover of 128 1-m plots, collected at 16 peatlands in the páramos of northern Ecuador. Vegetation data from plots was summarized by growth-form following (Ramsay and Oxley, 1997). For details, please see text.
Group 1 (Cushion peatlands): dominated by cushion (Plantago rigida and Distichia muscoides) and prostrate shrub growth forms. The cushion growth form is indicative of this group (p= 0.001; IndVal A= 0.842).
Group 2 (Herbaceous and shrubby peatlands): dominated by tussock graminoids (Calamagrostis spp. and Cortaderia spp.), erect shrubs, prostrate herbs, and acaulescent rosettes, this group is not uniquely indicated by any of the 12 growth forms.
Group 3 (Sedge and rush peatlands): dominated by non-tussock graminoids (e.g. Carex sp. and Juncus sp.) and moss growth forms, the non-tussock graminoid growth form is indicative of this group (p= 0.001; IndVal A= 0.700).
The cushion peatland type tends to be found at higher elevations (>4100 m, Figure 2) and is characterized by high cover of cushion forming species (mean cover >40%, Indval B = 1.000) interspersed with a mix of other growth forms including moss, tussock graminoid, acaulescent rosettes, and prostrate shrubs. Sedge and rush peatlands dominate at lower elevations (<3950 m) and are predominantly covered by non-tussock graminoids (mean cover >30%, Indval B = 1.000) mixed with moss and herbs. Herbaceous and shrubby peatlands occur at intermediate elevations (3950 to 4050 m) and are characterized by heterogeneous plant communities with relatively equal cover of most growth forms. Moss represented the second most dominant growth form in all three peatland types (Figure 3).
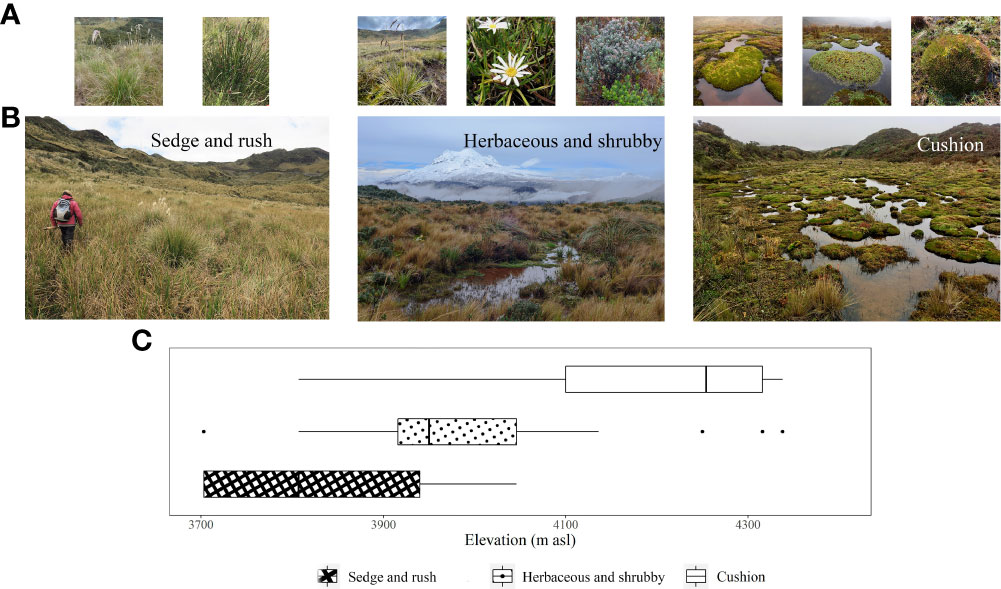
Figure 2 Examples and altitudinal distribution of the three main vegetation groups found in peatlands in the páramos of northern Ecuador. For each vegetation groups, the Figure depicts: (A) examples of characteristic species, (B) Representative images, and (C) Box-plot of the altitudinal distribution.
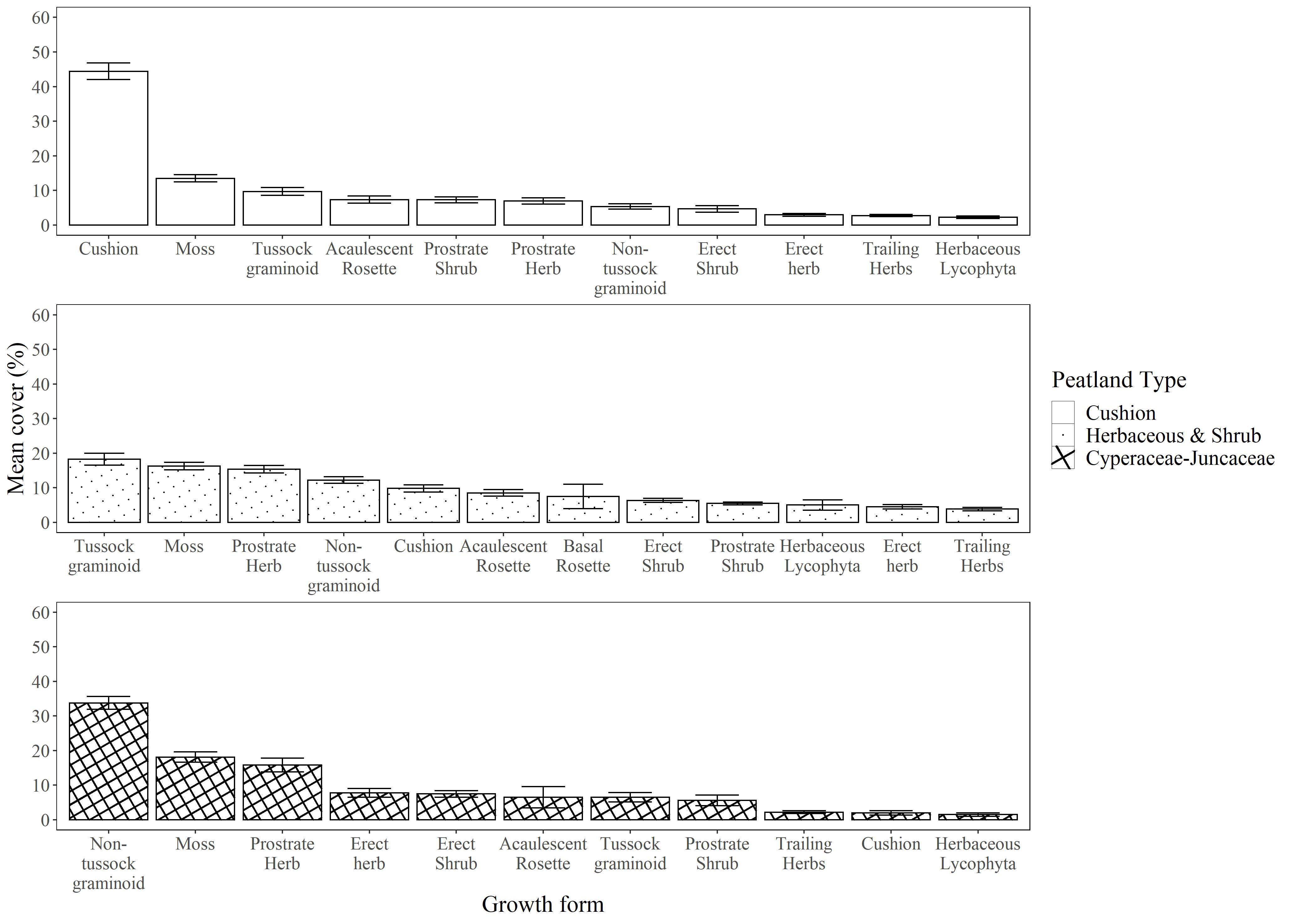
Figure 3 Dominance distribution of the three main vegetation groups found among 16 peatlands in the páramos of northern Ecuador. Dominance in each group was characterized through the mean percent ground coverage of growth-forms (sensu Ramsay and Oxley, 1997) derived from 128 1-m plots sampled at 16 individual peatlands.
Despite large differences in plant community structure, mean species richness was similar between the three peatland groups, with 19 ± 2.7 species at Sedge and rush peatlands, and 22.6 ± 2.04 and 23 ± 3.6 species at Cushion and Herbaceous and shrubby peatlands, respectively.
Aboveground biomass
Of the four peatlands analyzed for aboveground biomass, two fall within the Herbaceous and shrubby peatland category, and two within the cushion peatland category (Figure 2). Aboveground biomass decreased with increasing elevation (Table 2). Specifically, aboveground biomass at 3920 m (mean ± SE, 27 ± 17.6 Mg ha-1) was at least eight times greater than the biomass at higher elevations (1.7 ± 0.7 to 3.3 ± 1.0 Mg ha-1), representing an 85% decrease in biomass over 380 m of elevation. The relative contribution of different growth forms to total aboveground biomass also differed between elevations. Tussock graminoids accounted for 70 to 86% of the aboveground biomass in the lower sites (3900 m and 4125 m), 25% at 4240 m, and were not present at 4300 m. This sharp decline is due predominantly to the disappearance of two common tussock-forming species found in the lower sites: Cortaderia nitida and, to a lesser extent, Cortaderia sericantha. These tussock graminoids are replaced by lower stature vegetation—predominately cushion plants—in higher elevation sites (Table 2). Cushion plant contribution to aboveground biomass was negligible at 3920 and 4125 m but became a dominant functional group at the higher sites, contributing between 55 and 60% of the total peatland aboveground biomass.
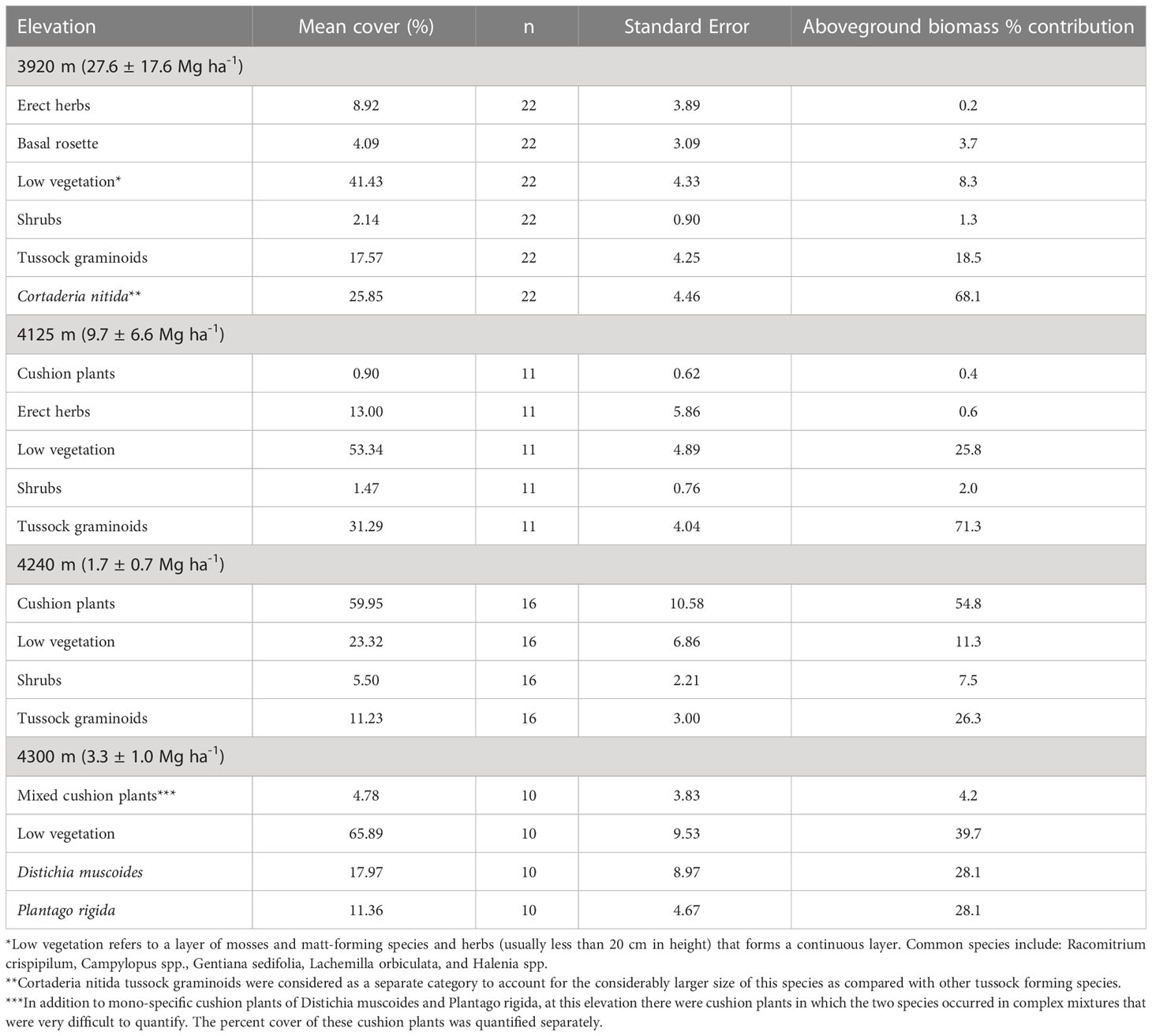
Table 2 Mean growth form percent cover and aboveground biomass contribution in four peatlands located along an altitudinal gradient in the Northeastern Andes of Ecuador.
Discussion
The peatland vegetation types that we found in this study are similar to those identified through a large-scale mapping effort conducted in the same region (Hribljan et al., 2017), with one specific distinction. While Hribljan et al. (2017) used the category “grass peatlands”, here we suggest a more general descriptor (Herbaceous and shrubby) to reflect the more complex and heterogeneous nature of the plant communities within this category. The other two categories (Cushion, Sedge and Rush peatlands) are similar to those described before, and also coincide with vegetation types described for high-elevation peatlands in the Colombian páramo (Benavides et al., 2023).
Vegetation structure across our sites is heterogeneous and seems strongly related to the steep altitudinal gradient of this region. At the lower portion of this gradient (< 3950 m) two types of peatlands were found: “Sedge and rush peatlands”, typically dominated by species of the genera Carex, Eleocharis and Juncus, and “Herbaceous and shrubby peatlands”, with a structurally diverse plant community in which tussock graminoids, mosses, herbs, acaulescent rosettes, basal rosettes, and erect shrubs form a complex matrix without clear dominance of any functional group. As these vegetation types can be found at the same elevations and, sometimes, even within the same site, their different structure probably reflects the influence of other environmental factors such as water table level, or water chemistry. Although in this study we did not measure these factors at all our sites, water table regimes might partially explain these differences, as high and constant water-table levels tend to favor the dominance of species such as Carex, Eleocharis and Juncus, which are known for their capacity to endure prolonged flooding (Ewing, 1996; Hough-Snee et al., 2015). This observation is also supported by a previous study in the same area which reported that a Herbaceous and shrubby peatland, and sedge dominated peatland, separated by less than 200 m, had mean water table levels of 2.8 ± 0.43 cm and -7.6 ± 0.73 cm, respectively, implying that the sedge dominated site was almost permanently flooded and had nearly 10 cm of difference in mean water table level in relation to the Herbaceous and shrubby peatland (Suárez et al., 2022b).
At higher elevations, shrubs and grasses become less frequent and cushion plants -especially Distichia muscoides and Plantago rigida, dominate the community structure, resulting in a physiognomy which closely resembles that of some of the “Distichia peatlands” previously described for the Colombian páramo, and for the jalca and puna ecozones of Central and southern Perú, Bolivia, and northern Argentina (Maldonado-Fonken, 2014; Ruthsatz et al., 2020; Domic et al., 2021; Benavides et al., 2023), whereas, our low elevation sites roughly coincide with the structure of the “peatlands with mosses and shrubs” described from lower jalca sites (3719-3890 m) and from the bofedales of the Central Andes (Navarro and Maldonado, 2002; Maldonado-Fonken, 2014). Similarities in plant community structure are also apparent when comparing our sites with the general descriptions provided by Deil et al. (2011) and Benavides et al. (2023). For example, our higher sites (> 4200 m) exhibit the same matrix species (D. muscoides, P. rigida) of the hard cushion mires of the Andean belt (Squeo et al., 2006; Deil et al., 2011). These similarities in plant community structure across a gradient that spans at least 11 degrees of latitude suggest that elevation is an important driver of the distribution and functioning of plant communities in high-elevation Andean peatlands across a wide range of environmental conditions. The elevation pattern emphasized here does not exclude possible effects of water chemistry and other environmental factors on plant community structure. For example, semi aquatic environments are often considered as azonal, which means that water saturation patterns might be the driving factors of their structure and functioning (Cooper et al., 2010). From this perspective, further research is needed to explore the relative influence and potential interactions between elevation, water-table patterns, and water chemistry across different scales in these Andean peatlands.
The classification of peatland types suggested by our analysis should not be taken as a definitive and exhaustive description of unique peatland categories in the páramo of Ecuador. In very large peatlands, for example, more than one vegetation type could be present, with Sedge and rush communities dominating areas with higher water table, and Herbaceous and shrubby vegetation in the transition towards the upland páramo. Moreover, our results are strictly limited to the northern Ecuadorian Andes, a region developed on young volcanic soils. Observations in peatlands developed on different geological and climatological settings of the northern and southern Andes suggest that additional types exist including peatlands dominated by thick carpets of Sphagnum mosses, and other which are covered by dense stands of shrubs (Loricaria spp., Hypericum spp., and Linochilus spp.). Additional studies are needed across the region to confirm and complement the physiognomic groups that we have described here for the northern Ecuadorian Andes.
As expected for high-elevation peatlands, our results show that our sites are characterized by low carbon storage in the aboveground biomass pool, especially when compared with the large storage capacities (> 2000 Mg ha-1) that have been reported for the peatlands in this region (Hribljan et al., 2017). The values that we estimated at our study sites (3 to 27 Mg ha-1) are very similar to typical values reported for temperate non-forested (1.36 to 23 Mg ha-1) and tropical high-elevation peatlands (8 to 27 Mg ha-1) (Dyck and Shay, 1999; Murphy et al., 2009; Murphy and Moore, 2010; Zerbe et al., 2013). Moreover, despite exhibiting very different plant community structure, aboveground biomass values at our peatland sites were also within the range of values (7 to 30 Mg ha-1) previously reported for upland páramo ecosystems (Cardozo and Schnetter, 1976; Beekman and Verweij, 1987; Lutz and Vader, 1987; Ramsay and Oxley, 2001). However, the large biomass differences that we found across vegetation types along the altitudinal gradient, raise questions about the role of different plant functional groups or species in terms of forming an protecting the thick layers of peat that characterize these sites.
Together with the large changes in plant community structure and composition across the altitudinal gradient, our results also showed an 8-fold reduction in aboveground biomass in the higher peatlands compared to the lower sites (Table 2). This pattern could be explained by at least two non-mutually exclusive alternatives. On the one hand, this drastic decline in plant biomass occurs through an altitudinal gradient of only 640 m, suggesting that aboveground carbon storage in high elevation peatlands could be finely controlled by the changes in functional structure of plant communities, as driven by lower temperatures at higher elevations. This suggests that even slight increases in temperature, far smaller than those forecasted by climate change models for the Tropical Andes (Urrutia and Vuille, 2009), could impose profound changes in the structure and function of high-elevation peatlands in the northern Andes. At the same time, our research indicates that cushion plant cover decreases at lower elevation, underscoring the potentially drastic effect warming temperatures could have on high-elevation cushion peatlands. On the other hand, the decrease of aboveground biomass with increasing elevation could be related to the age of the peatlands that we studied along this gradient. As all our peatland sites formed after the retreat of glaciers roughly 10,000 years ago, sites at lower elevations are probably older than sites at the higher reaches of the mountain. In this scenario, the contrasting life-growth composition and aboveground biomass patterns that we found along the elevation gradient could be rather explained by the influence that time could have on nutrient concentration and other chemical characteristics of soils at higher elevations. In fact, Bosnian et al. (1993) suggested that cushion plants could be favored by low nutrient concentrations and low conductivity that are common in the poorly developed soils at higher elevations. The relative importance of these hypotheses cannot be evaluated with the limited geographical scope of this study, suggesting new avenues for further study of the history and vegetation dynamics of these peatlands.
Our study offers a first attempt at characterizing plant communities in high-elevation peatlands in the northern Ecuadorian Andes, with an emphasis on understanding the effects of elevation on the distribution, structure, and above ground biomass patterns of high-elevation peatland plant communities. Our data suggest that the steep elevational gradients characteristic of Andean environments, are crucial in the local structuring of the physiognomy and composition of peatland vegetation. However, the potential effects of geological setting, time of development, hydrology, micro-topography, and land-use, which are likely to influence vegetation patters in these peatlands, were outside the scope of this paper and warrant future research (Benavides et al., 2013; Urbina and Benavides, 2015). Similarly, additional studies are needed to understand the functional roles of different vegetation types on the development of the large soil carbon reservoirs present in these ecosystems.
Data availability statement
The original contributions presented in the study are included in the article/supplementary material. Further inquiries can be directed to the corresponding author.
Author contributions
ES: Project conceptualization, field work, data analysis, and writing of first draft of the manuscript. JH: analysis and writing and manuscript review. SC: field work, soil sample processing, vegetation sampling, and manuscript review. KH, VT, JZ: field work. RJ and LD: analysis, writing and review of the manuscript. All authors contributed to the article and approved the submitted version.
Funding
This project was funded by the PREPA, Chancellor, and Collaboration Grant programs of Universidad San Francisco de Quito (USFQ). Additional support was provided by the US-AID funded Sustainable Wetlands Adaptation and Mitigation Program (SWAMP) and US Forest Service through agreements 20-IJ-11242306-008 and 21-CS-11242306-030.
Acknowledgments
The authors thank the Laboratory of Aquatic Ecology at USFQ for logistical support and the Ministry of the Environment of Ecuador for issuing the research permits (N.-04-2014-IC-FLO-DPAP-MA, MAAE-ARSFC-2020-1057 and MAATE-ARSFC-2022-2286) for our work at Cayambe-Coca National Park.
Conflict of interest
The authors declare that the research was conducted in the absence of any commercial or financial relationships that could be construed as a potential conflict of interest.
Publisher’s note
All claims expressed in this article are solely those of the authors and do not necessarily represent those of their affiliated organizations, or those of the publisher, the editors and the reviewers. Any product that may be evaluated in this article, or claim that may be made by its manufacturer, is not guaranteed or endorsed by the publisher.
References
Anthelme, F., Cauvy-Fraunié, S., Francou, B., Cáceres, B., Dangles, O. (2021). Living at the edge: increasing stress for plants 2–13 years after the retreat of a tropical glacier. Front. Ecol. Evol. 9. doi: 10.3389/fevo.2021.584872
Anthelme, F., Jacobsen, D., Macek, P., Meneses, R. I., Moret, P., Beck, S., et al. (2014). Biodiversity patterns and continental insularity in the tropical high Andes. Arctic. Antarctic. Alpine. Res. 46, 811–828. doi: 10.1657/1938-4246-46.4.811
Balslev, H., Luteyn, J. L. (1992). Páramo: an Andean ecosystem under human influence (London, New York: Academic Press).
Beekman, A., Verweij, P. (1987). Structure and nutrient status of a paramo bunchgrass vegetation in relation to soil and climate (Amsterdam: M. Science Thesis, Hugo de Vries Laboratory, University of Amsterdam).
Benavides, J. C., Vitt, D. H., Cooper, D. J. (2023). The high-elevation peatlands of the northern Andes, Colombia. Plants 12. doi: 10.3390/plants12040955
Benavides, J. C., Vitt, D. H., Wieder, R. K. (2013). The influence of climate change on recent peat accumulation patterns of distichia muscoides cushion bogs in the high-elevation tropical Andes of Colombia. J. Geophys. Res.: Biogeosci. 118, 1627–1635. doi: 10.1002/2013JG002419
Benfield, A. J., Yu, Z., Benavides, J. C. (2021). Environmental controls over Holocene carbon accumulation in distichia muscoides-dominated peatlands in the eastern Andes of Colombia. Quat. Sci. Rev. 251, 106687. doi: 10.1016/j.quascirev.2020.106687
Bosnian, A. F., van der Molen, P. C., Young, R., Cleef, A. M. (1993). Ecology of a paramo cushion mire. J. Veg. Sci. 4, 633–640. doi: 10.2307/3236128
Cardozo, H., Schnetter, M. L. (1976). Estudios ecológicos en el páramo de Cruz Verde, colombia. III. la biomasa de tres asociaciones vegetales y la productividad de calamagrostis effusa (HBK) steud. y paepalanthus columbiensis ruhl. en comparación con la concentración de clorofila. Caldasia 11, 69–83.
Carlsson, A. L. M., Bergfur, J., Milberg, P. (2005). Comparison of data from two vegetation monitoring methods in semi-natural grasslands. Environ. Monit. Assess. 100, 235–248. doi: 10.1007/s10661-005-6510-8
Chimner, R., Karberg, J. (2008). Long-term carbon accumulation in two tropical mountain peatlands, Andes mountains, Ecuador. Mires. Peat. 3.
Cooper, D. J., Kaczynski, K., Slayback, D., Yager, K. (2015). Growth and organic carbon production in peatlands dominated by distichia muscoides, Bolivia, south America. Arctic. Antarctic. Alpine. Res. 47, 505–510. doi: 10.1657/AAAR0014-060
Cooper, D. J., Wolf, E. C., Colson, C., Vering, W., Granda, A., Meyer, M. (2010). Alpine peatlands of the Andes, cajamarca, Peru. Arctic. Antarctic. Alpine. Res. 42, 19–33. doi: 10.1657/1938-4246-42.1.19
De Cáceres, M., Legendre, P. (2009). Associations between species and groups of sites: indices and statistical inference. Ecology 90, 3566–3574. doi: 10.1890/08-1823.1
Deil, U., Alvarez, M., Bauer, E.-M., Ramirez, C. (2011). The vegetation of seasonal wetlands in extratropical and orotropical south America. Phytocoenologia 41, 1–34. doi: 10.1127/0340-269x/2011/0041-0491
Domic, A. I., Capriles, J. M., Meneses, R. I., Pacheco, P. (2021). Plant community assembly is predicted by an environmental gradient in high-altitude wetlands in the semiarid western Bolivian Andes. Mires. Peat. 27, 1–12. doi: 10.19189/MaP.2019.JSP.StA.1916
Dyck, B. S., Shay, J. M. (1999). Biomass and carbon pool of two bogs in the experimental lakes area, northwestern Ontario. Can. J. Bot. 77, 291–304. doi: 10.1139/b98-215
Ewing, K. (1996). Tolerance of four wetland plant species to flooding and sediment deposition. Environ. Exp. Bot. 36, 131–146. doi: 10.1016/0098-8472(96)01000-3
Farley, K., Kelly, E., Hofstede, R. (2004). Soil organic carbon and water retention after conversion of grasslands to pine plantation in the Ecuadorian Andes. Ecosystems 7, 729–739. doi: 10.1007/s10021-004-0047-5
Floyd, D. A., Anderson, J. E. (1987). A comparison of three methods for estimating plant cover. J. Ecol. 75, 221–228. doi: 10.2307/2260547
Hall, M. L., Mothes, P. A. (2008). Volcanic impediments in the progressive development of pre-Columbian civilizations in the Ecuadorian Andes. J. Volcanol. Geothermal. Res. 176, 344–355. doi: 10.1016/j.jvolgeores.2008.01.039
Hofstede, R. G. M., Calles, J., López, V., Torres, F., Ulloa, J., Vásquez, A., et al. (2014). Los Páramos andinos ¿Qué sabemos? estado de conocimiento sobre el impacto del cambio climático en el ecosistema páramo (Quito: UICN).
Hofstede, R. G. M., Rossenaar, A. G. A. (1995). Biomass of grazed, burned, and undisturbed paramo grasslands, colombia. II. root mass and aboveground:belowground ratio. Arctic. Alpine. Res. 27, 13–18. doi: 10.2307/1552063
Hough-Snee, N., Nackley, L. L., Kim, S.-H., Ewing, K. (2015). Does plant performance under stress explain divergent life history strategies? the effects of flooding and nutrient stress on two wetland sedges. Aquat. Bot. 120, 151–159. doi: 10.1016/j.aquabot.2014.03.001
Hribljan, J., Suarez, E., Bourgeau-Chavez, L., Endres, S., Lilleskov, E. A., Chimbolema, S., et al. (2017). Multidate, multisensor remote sensing reveals high density of carbon-rich mountain peatlands in the páramo of Ecuador. Global Change Biol. 23, 5412–5425. doi: 10.1111/gcb.13807
Hribljan, J., Suárez, E., Heckman, K. A., Lilleskov, E. A., Chimner, R. A. (2016). Peatland carbon stocks and accumulation rates in the Ecuadorian páramo. Wetlands. Ecol. Manage. 24, 113–127. doi: 10.1007/s11273-016-9482-2
Lasso, E., Matheus-Arbelaez, P., Gallery, R. E., Garzon-Lopez, C., Cruz, M., Leon-Garcia, I. V., et al. (2021). Homeostatic response to three years of experimental warming suggests high intrinsic natural resistance in the paramos to warming in the short term. Front. Ecol. Evol. 9. doi: 10.3389/fevo.2021.615006
Lutz, R., Vader, P. (1987). Biomass, productivity and nutrient status in a Colombian bunchgrass paramo (Amsterdam: M. Science Thesis, Hugo de Vries Laboratory, University of Amsterdam).
Madriñán, S., Cortés, A., Richardson, J. (2013). Páramo is the world’s fastest evolving and coolest biodiversity hotspot. Front. Genet. 4. doi: 10.3389/fgene.2013.00192
Maldonado-Fonken, M. S. (2014). An introduction to the bofedales of the Peruvian high Andes. Mires. Peat. 15, 1–13.
McCune, B., Grace, J. B., Urban, D. L. (2002). Analysis of ecological communities (Gleneden Beach: MjM software design Gleneden Beach, OR).
Murphy, M., McKinley, A., Moore, T. (2009). Variations in above-and below-ground vascular plant biomass and water table on a temperate ombrotrophic peatland. Botany 87, 845–853. doi: 10.1139/B09-052
Murphy, M. T., Moore, T. R. (2010). Linking root production to aboveground plant characteristics and water table in a temperate bog. Plant Soil 336, 219–231. doi: 10.1007/s11104-010-0468-1
Navarro, G., Maldonado, M. (2002). Geografía ecológica de Bolivia: vegatación y ambientes acuáticos (Cochabamba, Bolivia: Centro de Ecología Simón I. Patiño).
Oksanen, J., Simpson, G. L., Blanchet, F. G., Kindt, R., Legendre, P., Minchin, P. R., et al. (2022) Vegan: community ecology package. Available at: https://CRAN.R-project.org/package=vegan.
Ramsay, P. M., Oxley, E. R. B. (1997). The growth form composition of plant communities in the ecuadorian páramos. Plant Ecol. 131, 173–192. doi: 10.1023/A:1009796224479
Ramsay, P. M., Oxley, E. B. (2001). An assessment of aboveground net primary productivity in Andean grasslands of central Ecuador. Mt. Res. Dev. 21, 161–167. doi: 10.1659/0276-4741(2001)021[0161:AAOANP]2.0.CO;2
Rochefort, L., Isselin-Nondedeu, F., Boudreau, S., Poulin, M. (2013). Comparing survey methods for monitoring vegetation change through time in a restored peatland. Wetlands. Ecol. Manage. 21, 71–85. doi: 10.1007/s11273-012-9280-4
Ruthsatz, B., Backes, B., Schittek, K. (2020). The vegetation of cushion peatlands in the argentine andes and changes in their floristic composition across a latitudinal gradient from 39°s to 22°s. Phytocoenologia 50, 249–278. doi: 10.1127/phyto/2020/0374
Sanchez-Baracaldo, P., Thomas, G. H. (2014). Adaptation and convergent evolution within the jamesonia-eriosorus complex in high-elevation biodiverse Andean hotspots. PloS One 9, e110618. doi: 10.1371/journal.pone.0110618
Schmidt-Mumm, U., Vargas Ríos, O. (2012). Plant communities in the terrestrial-aquatic transition zone in the paramo of chingaza, Colombia. Rev. Biol. Trop. 60, 35—64.
Squeo, F. A., Warner, B. G., Aravena, R., Espinoza, D. (2006). Bofedales: high altitude peatlands of the central Andes. Rev. Chil. Hist. Nat. 79, 245–255. doi: 10.4067/S0716-078X2006000200010
Suárez, E., Chimbolema, S., Chimner, R. A., Lilleskov, E. A. (2021). Root biomass and production by two cushion plant species of tropical high-elevation peatlands in the Andean páramo. Mires Peat 27, 1–9. doi: 10.19189/MaP.2020.OMB.StA.2131
Suárez, E., Chimbolema, S., Jaramillo, R., Zurita-Arthos, L., Arellano, P., Chimner, R. A., et al. (2022a). Challenges and opportunities for restoration of high-elevation Andean peatlands in Ecuador. Mitig. Adapt. Strateg. Glob. Change 27, 30. doi: 10.1007/s11027-022-10006-9
Suárez, E., Chimbolema, S., Jaramillo, R., Hribljan, J. A., Chimner, R. A., Lilleskov, E. A. (2022b). “Las turberas de páramo en el Ecuador,” in Turberas de páramo en el Ecuador: notas sobre ecología, conservación, y restauración de un ecosistema estratégico (Quito, Ecuador: Universidad San Francisco de Quito), 11–32.
Suárez, E., Medina, G. (2001). Vegetation structure and soil properties in Ecuadorian páramo grasslands with different histories of burning and grazing. Arctic. Antarctic. Alpine. Res. 33, 158–164. doi: 10.1080/15230430.2001.12003418
Thompson, J. B., Zurita-Arthos, L., Müller, F., Chimbolema, S., Suárez, E. (2021). Land use change in the Ecuadorian páramo: the impact of expanding agriculture on soil carbon storage. Null 53, 48–59. doi: 10.1080/15230430.2021.1873055
Urbina, J. C., Benavides, J. C. (2015). Simulated small scale disturbances increase decomposition rates and facilitates invasive species encroachment in a high elevation tropical Andean peatland. Biotropica 47, 143–151. doi: 10.1111/btp.12191
Urrutia, R., Vuille, M. (2009). Climate change projections for the tropical Andes using a regional climate model: temperature and precipitation simulations for the end of the 21st century. J. Geophys. Res.: Atmospheres. 114. doi: 10.1029/2008JD011021
Keywords: plant communities, aboveground biomass, Ecuador, Páramo, peatlands, elevation gradient
Citation: Suárez E, Hribljan JA, Chimbolema S, Harvey K, Triana V, Zurita JE, Jaramillo R and Doskocil LG (2023) Vegetation structure and aboveground biomass of Páramo peatlands along a high-elevation gradient in the northern Ecuadorian Andes. Front. Plant Sci. 14:1102340. doi: 10.3389/fpls.2023.1102340
Received: 18 November 2022; Accepted: 18 April 2023;
Published: 08 May 2023.
Edited by:
Barbara Vento, CONICET Mendoza, ArgentinaReviewed by:
Fabien Anthelme, Institut de Recherche pour le Développement, Marseille, FranceManuel Paneque, University of Chile, Chile
Katya Romoleroux, Pontificia Universidad Católica del Ecuador, Ecuador
Copyright © 2023 Suárez, Hribljan, Chimbolema, Harvey, Triana, Zurita, Jaramillo and Doskocil. This is an open-access article distributed under the terms of the Creative Commons Attribution License (CC BY). The use, distribution or reproduction in other forums is permitted, provided the original author(s) and the copyright owner(s) are credited and that the original publication in this journal is cited, in accordance with accepted academic practice. No use, distribution or reproduction is permitted which does not comply with these terms.
*Correspondence: Esteban Suárez, ZXN1YXJlekB1c2ZxLmVkdS5lYw==