- 1College of Resources and Environment, Northeast Agricultural University, Harbin, China
- 2College of Agriculture, Northeast Agricultural University, Harbin, China
- 3College of Engineering, Northeast Agricultural University, Harbin, China
Background: The nitrate regulates soybean nodulation and nitrogen fixation systemically, mainly in inhibiting nodule growth and reducing nodule nitrogenase activity, but the reason for its inhibition is still inconclusive.
Methods: The systemic effect of nitrate on nodule structure, function, and carbon distribution in soybean (Glycine max (L.) Merr.) was studied in a dual-root growth system, with both sides inoculated with rhizobia and only one side subjected to nitrate treatment for four days. The non-nodulating side was genetically devoid of the ability to form nodules. Nutrient solutions with nitrogen concentrations of 0, 100, and 200 mg L-1 were applied as KNO3 to the non-nodulating side, while the nodulating side received a nitrogen-free nutrient solution. Carbon partitioning in roots and nodules was monitored using 13C-labelled CO2. Other nodule responses were measured via the estimation of the nitrogenase activity and the microscopic observation of nodule ultrastructure.
Results: Elevated concentrations of nitrate applied on the non-nodulating side caused a decrease in the number of bacteroids, fusion of symbiosomes, enlargement of the peribacteroid spaces, and onset of degradation of poly-β-hydroxybutyrate granules, which is a form of carbon storage in bacteroids. These microscopic observations were associated with a strong decrease in the nitrogenase activity of nodules. Furthermore, our data demonstrate that the assimilated carbon is more likely to be allocated to the non-nodulating roots, as follows from the competition for carbon between the symbiotic and non-symbiotic sides of the dual-root system.
Conclusion: We propose that there is no carbon competition between roots and nodules when they are indirectly supplied with nitrate, and that the reduction of carbon fluxes to nodules and roots on the nodulating side is the mechanism by which the plant systemically suppresses nodulation under nitrogen-replete conditions.
1 Introduction
The effect of nitrate on N2 fixation in legumes is complex, and the underlying mechanism is not clear. Several studies have shown that the inhibitory effect of nitrate on nodule initiation and development in legumes depends on an interaction between nitrate and the autoregulation signal (Okamoto et al., 2009; Reid et al., 2011; Lin et al., 2018; Nishida et al., 2018). It is also thought that nitrate can accumulate in nodules, accelerating the senescence of the nodules (Cabeza et al., 2014). The subsequent metabolism of nitrite then produces nitric oxide, which easily combines with leghemoglobin to form nitrosyl leghemoglobin (LbNO), reducing the oxygen binding site of leghemoglobin and blocking the O2 supply (Becana et al., 1985; Minchin et al., 1989; Kanayama et al., 1990; Matamoros et al., 1999). Furthermore, the assimilation and reduction of nitrate in the plant require a sufficient energy source; thus, the proportion of carbohydrates available to nodules is reduced (Wasfi and Prioul, 1986; Fujikake et al., 2002).
Some studies have shown that supplying nitrates leads to carbon (C) competition between roots and nodules in legumes. Kouchi and Nakaji (1985) fed 13C to soybean (Glycine max (L.) Merr.) for 2.5 h and found that 13C was preferentially allocated to nodules compared to roots. In another study, a supply of 5 mM nitrate to soybean resulted in a 50% decrease in 14C radioactivity in nodules and a doubling of 14C radioactivity in roots (Fujikake et al., 2003b). Khan and Khan (1981) observed a similar phenomenon in pea (Pisum sativum L.). (Voisin et al. 2003a; Voisin et al. 2003b) concluded that nitrate inhibited nodule growth and reduced nodule dry weight, while 13C allocation was proportional to dry weight; thus, the proportion of 13C allocated to nodules was reduced.
Adequate C supply plays an important role in maintaining the stability of nodule structure. Supplying soybean roots with 5 mM nitrate under hydroponic conditions inhibited the expansion of infected cells in nodules (Fujikake et al., 2003b). When 50 mM nitrate was supplied to chickpea (Cicer arietinum L.) roots under sand culture conditions, infected cell nuclei disappeared, the bacteroids became sparse, and the nodule nitrogenase activity decreased (Sheokand et al., 1998). In studies of barrel medic (Medicago truncatula), trifolium (Trifolium subterraneum L.), lupin (Lupinus albus L. cv. Multolupa), and bean (Phaseolus vulgaris), nitrate supply reduced the number of infected cells and increased the number of uninfected cells in nodules; moreover, the bacteroids were irregularly shaped and decreased in number, the symbiosome membrane (SM) was destroyed, and the peribacteroid space (PBS) became larger (Dart and Mercer, 1965; De Lorenzo et al., 1990; Matamoros et al., 1999; Wang et al., 2020).
In previous studies on the relationships among nodule structure, C distribution and nitrate, nitrate was added directly to the roots, which failed to prevent the toxic effects caused by nitrate coming into direct contact with nodules. At the same time, experiments with split-rooted plants cannot avoid the effects of incomplete root systems caused by removal of the main root. We used grafting to prepare dual-root soybean plants with one side nodulating and another side non-nodulating to ensure the integrity of the root system on both sides. Meanwhile, we supply nitrate to the non-nodulating side, which excluded the toxic effects of nitrate on nodules. The hypothesis of this work is that roots and nodules compete for C during indirect nitrate exposure, which induces damage to nodule structure and down-regulation of N2 fixation. We measured the nodule nitrogenase activity and plant C distribution (13C labeling method), and observed the nodule structure. Our objective was to investigate the systemic effects of nitrate on nodule structure and assimilated C distribution by excluding the toxic effects of nitrate exposure and to provide new insights into the systemic inhibition of nodule N2 fixation by nitrate.
2 Materials and methods
This study was carried out in sand culture pots in 2019 at the Experimental Base of Northeast Agricultural University located in Xiangfang District, Harbin, Heilongjiang Province, China (geographical coordinates: 126°43′E, 45°44′N). Nodulating soybean (DongDa1 G. max L. cv.) and non-nodulating soybean (WDD01795, L8-4858 G., a mutant of normal nodular soybean Clark, obtained from the Academy of Agricultural Sciences in China, Beijing) were used. The non-nodulating soybeans do not nodulate throughout the growth period, even if inoculated with rhizobia. Two varieties of soybeans were seeded into fine-sand medium and cultured in an illuminated growth chamber at 30°C for approximately 3 days. Nodulating and non-nodulating soybean seedlings were grafted together as previously described (Zhang et al., 2020). One week after grafting, the shoot of nodulating soybean was retained, and the shoot of non-nodulating soybean was cut off from the grafting site. Each pot contained two root systems sharing one shoot (see Figure S1 for the detailed method for preparing dual-root soybean systems). At the VC stage (unfolded cotyledon stage), both root systems were inoculated with rhizobia as follows: field-grown soybean nodules that were frozen in the previous year were ground and added to the nutrient solution, with approximately 5 g of the ground nodule mass per liter. The inoculant was applied for five consecutive days. Before the VC stage, the plants were irrigated with distilled water once a day; from the VC stage to the V4 stage (fourth trifoliate leaf stage), the plants were irrigated with nutrient solution once a day, and from the V4 stage to the end of the experiment, the plants were irrigated with nutrient solution twice a day, in the morning and evening. The irrigation volume was 250 mL on each side of the root system. Nitrogen (N)-free nutrient solutions were prepared as previously described (Li et al., 2021), and the nutrient solution ingredients are listed in Table S2. The results of the previous study found that the effect of N concentration of 100 mg L-1 on nodulation was obvious (Lyu et al., 2019; Li et al., 2021). Therefore, in this experiment, KNO3 with N concentration of 100 mg L-1 and extreme N concentration (200 mg L-1) was selected to systemically analyze the effect of high nitrate concentrations on N2 fixation capacity of soybean nodules. While KNO3 was added to the non-nodulating side, K2SO4 was used to replace KNO3 on the nodulating side and in the control treatment to ensure an equal concentration of K+ in all the treatments. Stages were designated according to the description of Fehr et al. (1971).
2.1 Experimental treatments
The experiment included three treatments, designated N0, N100 and N200, between the VC and V4 stages. All the experimental materials were irrigated with a nutrient solution with N concentration of 14 mg L–1 KNO3 on both sides of the dual-root system. In the V4 stage, the N-free nutrient solution was added to both sides for N starvation over 10 days. After N starvation, in the R1 stage (42 days after grafting), the root systems subjected to the N100 and N200 treatments on the non-nodulating side were treated with a nutrient solution with a N concentration of 100 or 200 mg L-1 KNO3, and the nodulating sides of the dual-root systems were treated with the N-free nutrient solution. The N0 treatment was used as a control, and both sides were treated with the N-free nutrient solution. The experiment had a completely randomized design with three biological replications. After 4 days of treatment, the experimental materials of the three treatments were divided into two groups. One group was sampled for determination of nodule nitrogenase activity and light and electron microscopic observations, and the other group was subjected to a 13CO2 feeding.
13CO2 feeding was conducted following the method of Kouchi and Yoneyama (1984) with slight modifications. The assimilation chamber (1.2 m × 1.2 m × 1.0 m with acrylic sheets) was designed as a tightly closed system with a controllable CO2 generated device connected outside, and the air temperature and relative humidity were controlled at 27 ± 1°C and 60 ± 5%, respectively. The experiment was carried out under natural light on a sunny day. At the beginning of the feeding, 13CO2 was produced by adding 33.33 atom% Ba13CO3 and 1 mg L-1 HCl into the generated device. 13CO2 was imported into the assimilation chamber through the air inlet. The concentration of CO2 in the assimilation chamber was monitored continuously by an infrared C dioxide analyzer (Q-S151 Infrared CO2 Analyzer, Qubit Systems Inc., Canada), and the concentration of 13CO2 was maintained between 450 and 500 ppm (see Figure S3 for the 13CO2 assimilation device). Feeding was performed for 8 h with sampling.
2.2 Sampling and determination
Nodule nitrogenase activity was measured as follows: the nodulating sides of the roots were washed with distilled water, and the nodule nitrogenase activity was measured by the acetylene reduction method described by Xia et al. (2017).
Light microscopy was performed as follows: six nodules of similar size within 6 cm of the grafting site were randomly chosen and cut out together with an adjacent portion of the root. Then, they were fixed, sliced (nodules on top and the connected roots on the bottom, cutting perpendicular to the roots from top to bottom), dehydrated, and embedded as described by Feder and O'Brien (1968), observed under a light microscope (Nikon Eclipse E100) and photographed using an imaging system (Nikon DS-U3).
Transmission electron microscopy (TEM) was performed as follows: six nodules of similar size within 6 cm of the grafting site were randomly chosen and cut out together with an adjacent portion of the root. According to the method described by Goodchild and Bergersen (1966), the nodules were sliced and fixed in 2.5% glutaraldehyde in 0.1 mol L-1 phosphate buffer (pH 7.0), washed with the same buffer, postfixed in 1% osmic acid buffer for 2 h, dehydrated by an acetone series, and embedded with Epon812 resin. The samples were polymerized at 60°C, and other operations were performed at room temperature. Sections were prepared with an ULTRACUT-E ultrathin slicer, stained in uranyl acetate-lead citrate and examined using a Hitachi H_7650 electron microscope.
Sampling and measurements of assimilated 13CO2 were conducted as follows: at the end of the 13CO2 feeding, soybean plants with dual-root systems were cut at the grafting site. The plants were divided into shoots and underground parts; the underground parts were divided into root samples from the non-nodulated side and root and nodule samples from the nodulated side. All the samples were washed with distilled water to remove sand and blot-dried with filter paper. The samples were then oven-dried at 65°C. After that, the samples were converted to 13CO2 in an elemental analyzer, and the 13C isotope abundance in each organ was determined by isotope mass spectrometry (Thermo-Fisher Delta V Advantage IRMS).
2.3 Data analysis
Distribution of C in samples was calculated according to the following formula:
13C Accumulation=W×CT× (13Csample atom% - 13CN atom%)
where W is the dry matter weight, CT is the total C concentration, 13Csample is the 13C abundance in the sample, and 13CN is the natural 13C abundance.
IBM SPSS 21.0 (IBM Corp., Armonk, NY, USA), Origin 9.0 and Microsoft Excel were used to analyze the data and generate graphs. All data were tested for normality before one-way analysis of variance (ANOVA), and Duncan’s multiple range test was run for mean comparisons at a significance level of p<0.05.
3 Results
3.1 Effects of nitrate on nitrogenase activity in soybean nodules
The acetylene reduction activity in μmol of ethylene formed per plant per hour (ARA) and specific nitrogenase activity per gram dry weight of nodules per hour (SNA) were affected by the nitrate supply on the non-nodulating side in the same trend (Figure 1). The ARA and SNA in both the N100 and N200 treatments were more than 80% lower than those in the N0 treatment, and the ARA and SNA values between the N100 and N200 treatments were similar. This result indicated that the supply of nitrate on the non-nodulating side reduced nitrogenase activity on the nodulating side of dual-root soybean plants. In the experiment, when nitrate was supplied to the non-nodulating side, N-free nutrient solution was supplied to the nodulating side, the nodules were not exposed to nitrate, indicating that the nodule N2 fixation activity was systemically regulated by a certain mechanism in soybean plants.
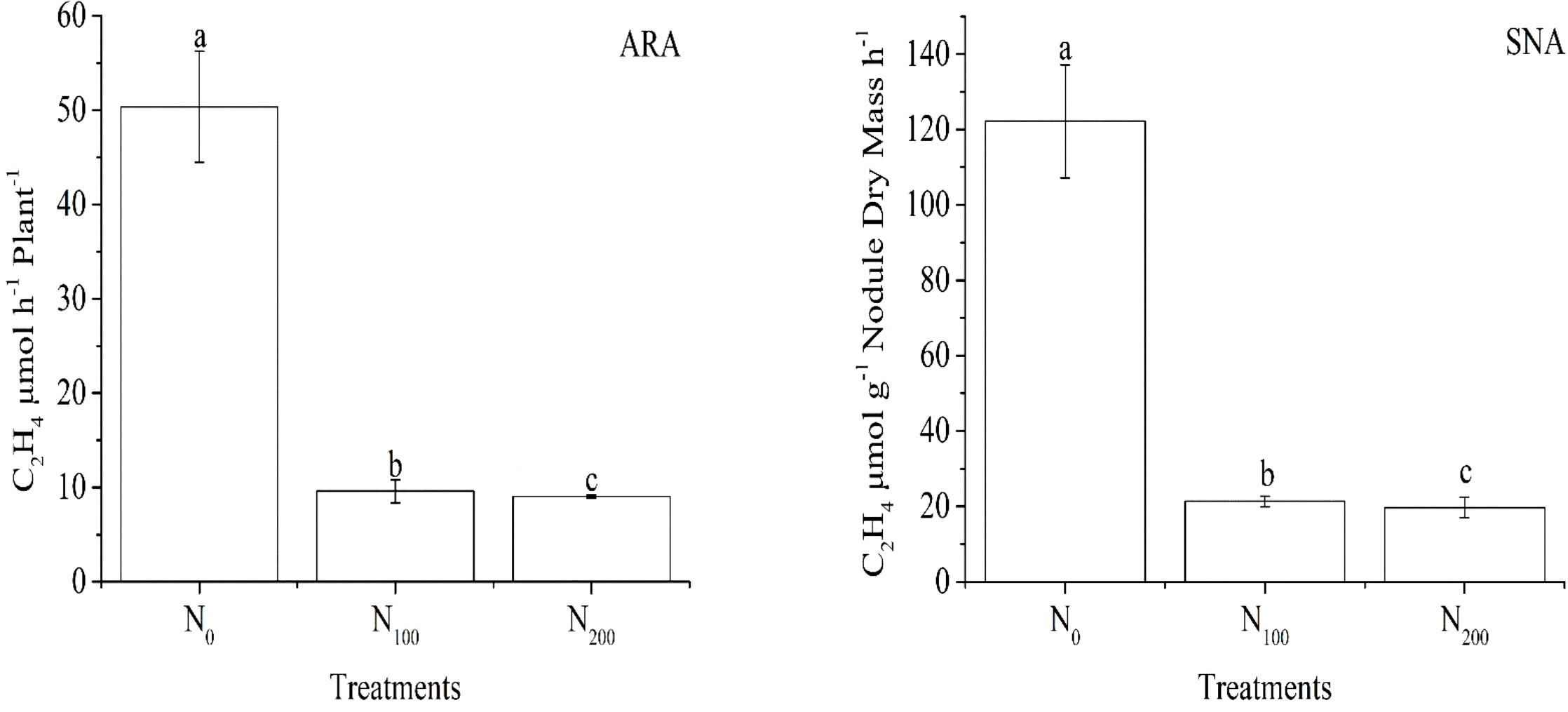
Figure 1 Acetylene reduction activity in μmol of ethylene formed per plant per hour (ARA) and specific nitrogenase activity per gram dry weight of nodules per hour (SNA) on the nodulating side of the dual-root soybean plants. The N0, N100, and N200 treatments represented the supply of KNO3 nutrient solution with N concentrations of 0, 100, and 200 mg·L-1 to the non-nodulating side, respectively, with N-free nutrient solution supplied to the nodulating side. Error bars are the standard error of three replicates. Different lowercase letters indicate a significant difference between the treatments at the 5% level.
3.2 Effects of nitrate on the anatomy of soybean nodules
3.2.1 Microstructure of soybean nodules
Supplying nitrate to the non-nodulating side resulted in changes in the nodule microstructure on the nodulating side (Figure 2). In the central part of the nodule, the infected cells were filled with bacteroids, and there were more infected cells than uninfected cells in the N0 treatment (Figure 2Acen). In the N100 treatment, a large number of vacuoles were produced in addition to bacteroids in the infected cells (Figure 2Bcen). In the N200 treatments, the vacuoles were enlarged compared with those in the N100 treatment; the number of infected cells was significantly lower and the number of uninfected cells was higher than those in the N0 and N100 treatments (Figure 2Ccen). The results indicated that, as the nitrate supply increased on the non-nodulating side, the number of infected cells in the central part of the nodules on the nodulating side decreased, the number of uninfected cells increased, and the vacuoles in the infected cells became enlarged.
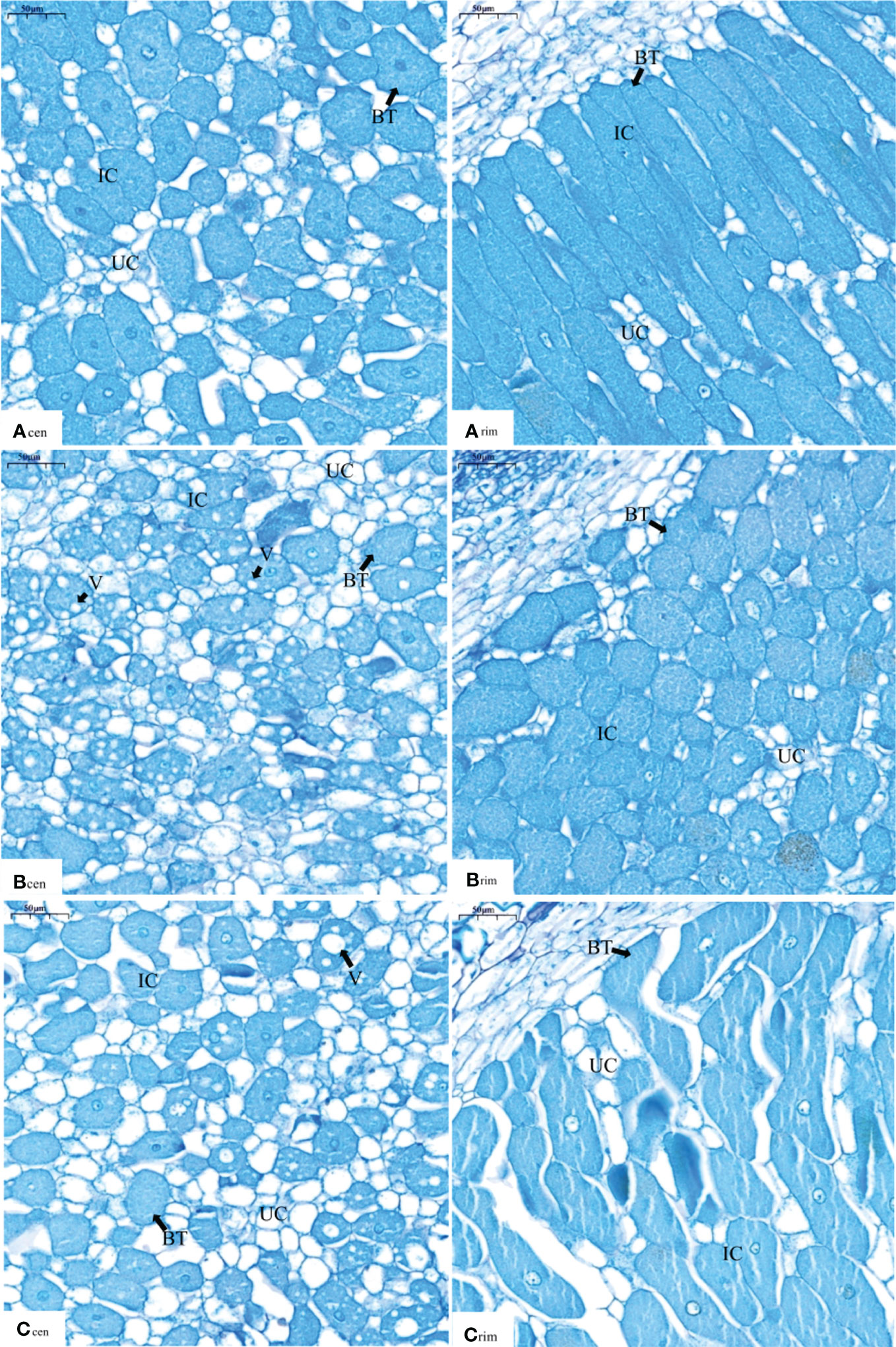
Figure 2 Light micrographs of nodules of dual-root soybean plants (×200). Acen, Bcen and Ccen are light micrographs of the central part of the nodules under the N0, N100, and N200 treatments, respectively. Arim, Brim, and Crim are light micrographs in the edge part of nodules close to the roots under the N0, N100, and N200 treatments, respectively. IC represents infected cells, UC represents uninfected cells, BT represents bacteroids, and V represents vacuoles.
In the edge part of the nodules close to the root, most of the infected cells were rod-shaped, the bacteroids in the infected cells were densely packed with bacteroids, and there was basically no gap between the infected cells (Figure 2Arim). In the N100 treatment, the infected cells were filled with bacteroids somewhat less densely and were mostly spherical in shape compared with those in the control N0 (Figure 2Brim). In the N200 treatment, most of the infected cells had irregular shape different from the shape of cells in the control N0 and the N100 treatment, while the number of uninfected cells appeared to be similar to the numbers observed in the control N0 and the N100 treatment (Figure 2Crim). At the same time, the vertical cracks through the infected cells and the large spaces between the cells apparent in Figure 2Crim are likely to be due to mechanical damage to the nodule section, namely due to a stretching force that pulled the cells apart from each other in the horizontal direction. We think that this observation is artifactual because the contours resemble the drift of continents and clearly show which cell was mechanically separated from which other cells in the image. The results indicated that the structure of the infected cells close to the root changed as the concentration of nitrate supplied to the non-nodulating side increased.
3.2.2 Ultrastructure of soybean nodules
TEM was used to further observe the nodule structure, and it was found that the nitrate supply on the non-nodulating side had a great influence on the symbiotic structure of the infected cells on the nodulating side (Figure 3). In the infected cells in the N0 treatment, multiple bacteroids were wrapped with an SM to form a symbiosome (SB), and the SBs were positioned compactly (Figure 3A1). The SMs were clearly visible, the bacteroids in the SBs were dense, and the PBS between the bacteroids was very small. Most of the bacteroids were filled with large PHB granules, which appeared white and bright under TEM (Figure 3A2). Compared with the N0 treatment, some SBs were fused in the N100 treatment, and an incompletely degraded SM was observed in the fused SBs (Figure 3B1). The PBS became larger, the number of bacteroids decreased, the PHB granules in the bacteroids became smaller than those in the N0 treatment, and some of the PHB granules became translucent (Figure 3B2). In the N200 treatment, the number of PHB granules and the number of bacteroids were comparable with the N100 treatment. However, more PHB was present in the translucent form, and the PBS became larger. The SMs were less clearly outlined possibly indicating the onset of degradation, while the SBs were still well-distinguishable from the cytoplasm (Figure 3C1, C2). These results indicated that the supply of nitrate on the non-nodulating side led to a change in the symbiotic structure of the nodule-infected cells.
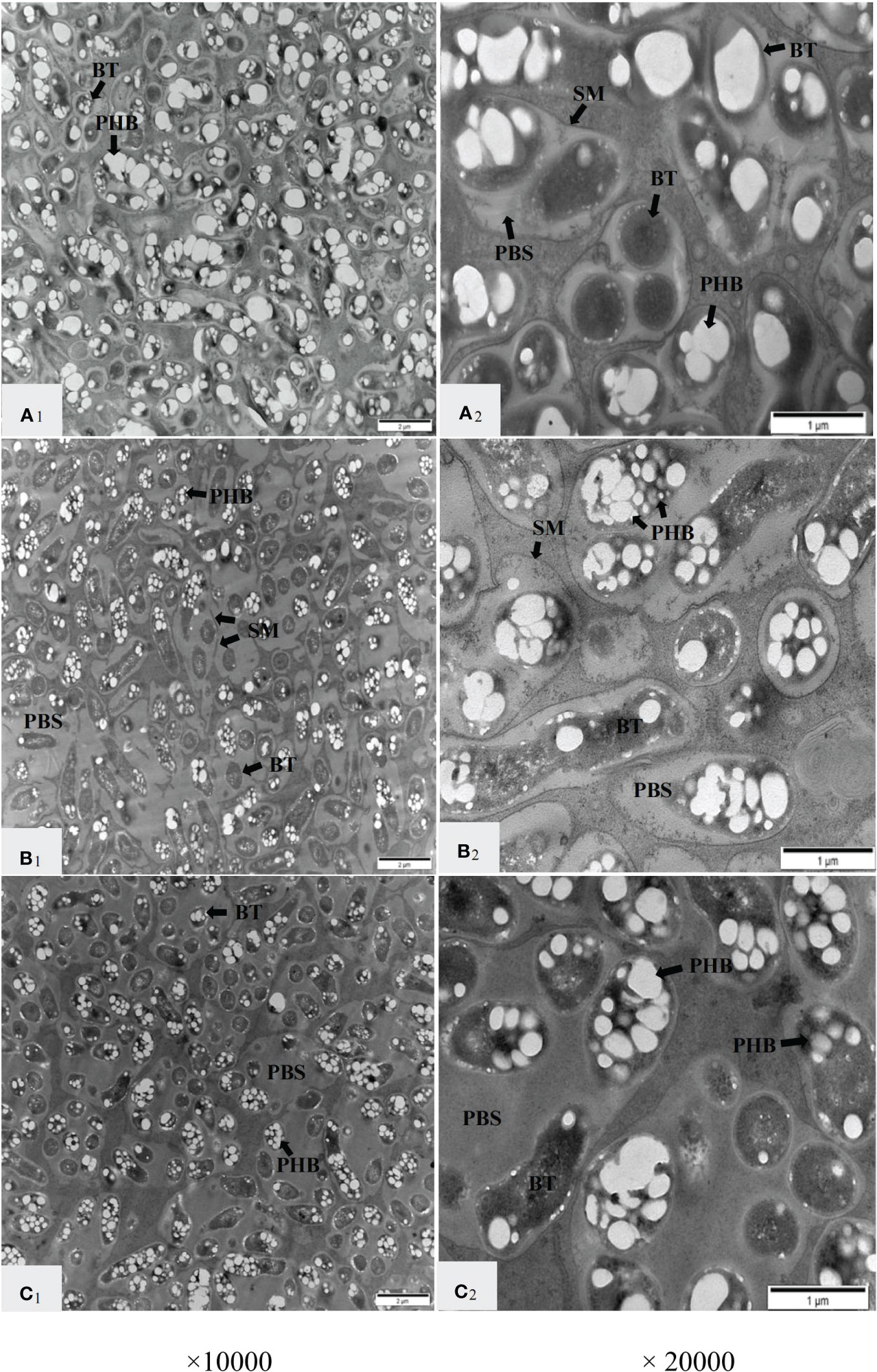
Figure 3 Transmission electron micrographs of infected cells in nodules on the nodulating side of dual-root soybean plants. A1 and A2 represent the N0 treatment; B1 and B2 represent the N100 treatment; C1 and C2 represent the N200 treatment. BT, bacteroids; SM, symbiosome membrane; PBS, peribacteroid space; PHB, polyhydroxybutyrate granules.
3.3 Effects of nitrate on assimilated C distribution in soybean plants
Dual-root soybean plants were fed with 13CO2 for 8 h after 4 days of nitrate supply on the non-nodulating side in the R1 stage, and we measured the dry weight of each organ (Table 1). The dry weights of the shoots in the N100 and N200 treatments were 9.6% and 17.8% higher than those in the N0 treatment, respectively (P<0.05). The dry weight of non-nodulating roots (Rootnon) in both the N100 and N200 treatments was 40.0% higher than that in the N0 treatment (P<0.05). The dry weight of roots on the nodulating side (Rootn) and nodules was not significantly affected by changes in the concentration of nitrate supplied on the non-nodulating side. These results indicated that the short-term supply of high nitrate concentrations to the non-nodulating side promoted the growth of the roots on the non-nodulating side and of the shoots but had a weaker effect on the growth of roots and nodules on the nodulating side.
Nitrate supply on the non-nodulating side had little effect on the 13C abundance of the shoots but significantly increased the 13C abundance of non-nodulating lateral roots and significantly decreased the 13C abundance of nodulating lateral roots and nodules (Table 2). The 13C abundance of nodules in the N100 and N200 treatments was 8.3% and 16.7% lower than that in the N0 treatment, respectively (P<0.05). The 13C abundance of Rootn in the N100 and N200 treatments was 7.7% and 15.4% lower than that in the N0 treatment, respectively (P<0.05). The abundance of C in the N100 and N200 treatments was 20.0% and 35.0% higher than that in the N0 treatment, respectively (P<0.05).
Based on the dry weights (Table 1), 13C abundance (Table 2) and total C concentration (Table S4) of dual-root soybean plants, the total 13C accumulation and the ratio of 13C accumulation in each organ to the 13C accumulation in the whole plant during 13CO2 feeding (13Cap) were calculated (Table 3). There was no significant difference in 13C accumulation in whole soybean plants (Planttotal), shoots and underground parts (Roottotal) among the three treatments. The 13C accumulation in Rootn and nodules decreased significantly with increasing nitrate supply concentration on the non-nodulating side, the 13C accumulation in the N200 treatment was 38.9% (P<0.05) and 30.0% (P<0.05) lower than that in the N0 treatment, respectively. The 13C accumulation in Rootnon increased significantly, and the 13C accumulation in the N100 and N200 treatments was 105.9% (P<0.05) and 129.4% (P<0.05) higher than that in the N0 treatment, respectively.
With increasing nitrate supply on the non-nodulating side, 13Cap values of the shoots increased, and in the N200 treatment they were 3.2% higher than that in the N0 treatment (P<0.05). However, Roottotal 13Cap decreased with increasing nitrate supply on the non-nodulating side, and the 13Cap in the N200 treatment was 24.1% lower than that in the N0 treatment (P<0.05). 13Cap values in nodules decreased with increasing nitrate supply concentration on the non-nodulating side, and the value for the N200 treatment was significantly lower than that for the N0 treatment. 13Cap values in Rootn samples were consistent with those of nodules, except that the values for both the N100 and N200 treatments were significantly lower than those for the N0 treatment. 13Cap values in Rootnon samples increased with increasing nitrate supply concentration, and the difference between the N100 and N200 treatments was not significant. However, the values for both treatments were significantly higher than the value for the N0 control. These results indicated that the increase in the nitrate supply concentration on the non-nodulating side significantly increased the amount and proportion of 13C accumulated in the roots on the non-nodulating side and decreased the amount and proportion of 13C accumulated in the roots and nodules on the nodulating side.
Comparing 13Cap in each organ of dual-root soybeans, the Shoot/Roottotal value of the N200 treatment was 38.2% (P<0.05) and 20.7% (P<0.05) higher than the values of the N0 and N100 treatments, respectively (Table 4). The values of (Rootn+Nodule)/Rootnon, Nodule/Rootnon, and Rootn/Rootnon all decreased with increasing nitrate supply on the non-nodulating side. There was no effect on Nodule/Rootn values when nitrate was supplied on the non-nodulating side. These results indicated that the assimilated 13C distributed to whole soybean roots decreased as the concentration of supplied nitrate on the non-nodulating side increased, the assimilated 13C distributed to Rootnon increased, and the assimilated 13C allocated to nodules and Rootn decreased. However, the proportion of assimilated 13C distributed to nodules and Rootn was not affected by the change in nitrate concentration on the non-nodulating side. In summary, fluxes of C to nodules and roots on the nodulating side were suppressed when the concentration of supplied nitrate on the non-nodulating side increased.
4 Discussion
4.1 Relationship between the ultrastructure of soybean nodules and the nitrate supply
Nitrate can be absorbed and transported from one side to the other in dual-root soybeans and inhibits nodule nitrogenase activity systemically (Xia et al., 2017; Lyu et al., 2019; Lyu et al., 2020; Li et al., 2021; Lyu et al., 2022). Infected cells are the main site of N2 fixation in nodules. Changes in the characteristics of the infected cells directly affect the N2 fixation capacity of nodules (Finan et al., 1983; Domigan et al., 1988). After supplying nitrate, bacteroids in the infected cells of Chamaecrista fasciculata nodules became sparse (Naisbitt and Sprent, 1993), newly formed bacteroids lysed and degraded, and viability of mature bacteroids impaired in nodules of barrel medic (Truchet and Dazzo, 1982; Lambert et al., 2020). In this study, when nitrate was supplied to the non-nodulating side for four days, no effect on nodule growth was observed (Table 1). However, the ARA and SNA values of the N100 and N200 treatments decreased by more than 80% compared with those of the N0 treatment (Figure 1). Meanwhile, with the increase in nitrate supply concentration, the number of infected cells decreased and the number of bacteroids also decreased. The structure of infected cells in the edge part of the nodule close to the roots changed from elongated to spherical and eventually lost regularity in shape at N200. Furthermore, numerous vacuoles appeared in the infected cells and became progressively larger (Figure 2). When no exogenous N was applied, and N2 fixation by nodules was the only source of this macronutrient, no vacuoles in the infected cells of soybean nodules were observed (Gordon et al., 1992). Under environmental stresses (e.g., oxygen limitation or salt stress), large vacuoles were formed around the host cell nucleus of infected cells in senescing soybean nodules. This vacuolization process was accompanied by the lysis of bacteroids (James et al., 1991; James et al., 1993). This is why we indicated that systemic effects of indirect nitrate supply on nodule nitrogenase activity were associated with the changes in nodule structure.
The SM encloses the bacteroids and controls the metabolic fluxes between the plant cytosols and the bacteroids, protecting bacteroids from the attack by host proteases (Streeter, 1991; Day et al., 1995; Udvardi and Day, 1997; Brear et al., 2013; Liu et al., 2018). Meanwhile, iron must cross the SM before being supplied to bacteroids. Iron is used to synthesize ferro proteins necessary for N2 fixation, and the oxygen-carrying protein leghemoglobin (Lb) is abundant in nodules (Udvardi and Day, 1997; Brear et al., 2013). In bacteroids, N2 fixation is regulated by oxygen (Batut and Boistard, 1994; Fischer, 1994). When the oxygen concentration is not in the correct range, the rhizobia released into the cytoplasm of plant cells can not differentiate into N2-fixing bacteria (Brewin, 1989). In this study, the SM was still visibly intact under the N100 treatment. However, its less clear outline under the N200 treatment may reflect the decomposition of the SM (Figure 3). Newcomb et al. (1977) found that reduced N2 fixation capacity in ineffective pea nodules was associated with the absence of the SM. The dismantling of the SM is associated with nodule senescence (Fargeix et al., 2004). Thus, changes of the SM structure in this experiment may block iron transport, affect oxygen supply, and cause premature senescence of nodules. A dedicated study with the SM-specific markers is necessary to clarify the fate of the SM in the course of a systemic response to elevated nitrate concentrations.
The PBS became larger with increasing nitrate supply on the non-nodulating side (Figure 3). The PBS stores some ions and metabolic substances (Bergersen and Appleby, 1981). Nitrate supply resulted in the enlarged PBS and decreased nodule nitrogenase activity in lupin nodules (De Lorenzo et al., 1990). Salt stress also resulted in the enlarged PBS and decreased nodule nitrogenase activity in fava bean (Vicia faba L.) (Trinchant et al., 1998). Although the biological significance of this enlargement of the PBS remains to be elucidated, our findings indicate that the elevated nitrate supply expands the PBS and leads to a decrease in the number of bacteroids. This decrease is accompanied by the fusion of SBs into larger structures, which may explain the expansion of the PBS. Because these ultrastructural rearrangements were associated with the strong suppression of the nitrogenase activity in nodules, we conclude that the microscopic observations caused by treatments N100 and N200 reflect the progress of N-induced premature nodule senescence.
4.2 Relationship between the distribution of assimilated C and the nitrate supply
When nitrate is directly added to roots, the C distribution between roots and nodules in soybean plants changes (Streeter, 1980; Kouchi et al., 1986; Fujikake et al., 2003b; Ishikawa et al., 2018), thus reducing the proportion of C transported to nodules and increasing the proportion of C transported to roots (Fujikake et al., 2003a). Sulieman et al. (2014) also observed similar phenomena in barrel medic. However, this nitrate supply method cannot exclude the toxic effect of nitrate on nodules (Becana et al., 1985; Wasfi and Prioul, 1986; Giannakis et al., 1988; Cabeza et al., 2014). This direct effect of nitrate on nodule growth must result in unequal C partitioning between roots and nodules. In this study, dual-root soybean plants with a single nodulating side were used to supply nitrate to the non-nodulating side, and the nodules on the nodulating side were not exposed to nitrate. With the increase in nitrate concentration, the proportion of assimilated C transported to non-nodulating lateral roots increased significantly, and the proportion of assimilated C transported to nodulating lateral roots and nodules decreased significantly. Moreover, the distribution ratio of assimilated C between nodules and roots on the nodulating side was not influenced by the nitrate concentration supplied on the non-nodulating side (Table 4). Previousely, Singleton and Kessel (1987) considered that NH4NO3 promoted root growth on the N supply side of split-root soybean, reported an increase in the proportion of C distributed to this side as a response to nitrate. We used two varieties of soybeans, nodulating and non-nodulating, for grafting. Due to the difference in varieties, the dry weight of roots on the non-nodulating side was smaller than that on the nodulating side. However, it still showed that more C was transported to the non-nodulating side after nitrate supply. These results indicated that the non-nodulating roots obtained more C than the nodulating side. Roots on the non-nodulating side competed for C with roots and nodules on the nodulating side. Nodulating lateral roots and nodules did not compete for C due to reduced C supply on this side.
PHB is the main C source in the process of bacteroid reproduction and N fixation and can be mobilized to support N fixation when the C supply is insufficient (Wong and Evans, 1971; Bergersen et al., 1991; Trainer and Charles, 2006). The amount of PHB in C. fasciculata nodules decreased with increasing nitrate supply concentration (Naisbitt and Sprent, 1993). In this study, the PHB granules became smaller and the hue of PHB granules in TEM images changed from bright to opaque in some bacteroids as the nitrate supply on the non-nodulating side increased (Figure 3). It is possible that this observation indicates the onset of PHB degradation to meet the C demand of bacteroids (Bergersen et al., 1991). We interpret this phenomenon as a consequence of the systemic blockage of C flow from the shoot to the nodulated side, which leads to insufficient C supply to nodules. Under the condition of C deficit caused by this systemic response, bacteroids probably intensify the utilization of deposited PHB. However, this mobilization of stored C does not compensate for the reduction in C influx via the nodule vasculature, which is manifested in the strongly reduced nitrogenase activity.
5 Conclusions
In a dual-root soybean system, where only one side has the ability to develop nodules, nitrate supply to the non-nodulating side systemically suppressed nodule nitrogenase activity, which was related to the change in nodule ultrastructure. Moreover, the two root systems competed for assimilated C from the shoots, and there is no C competition between roots and nodules without direct exposure to nitrate. Although the non-nodulating roots received consistently less photosynthetic C in the control N0 and in all the treatments (N100 and N200) compared with the nodulating side, with the increase in the concentration of applied nitrate, the symbiotic side received progressively less C with the following trend: N0 > N100 >N200. However, for the non-symbiotic side, the trend was the opposite: N0 < N100< N200.
Data availability statement
The original contributions presented in the study are included in the article/Supplementary Material. Further inquiries can be directed to the corresponding author.
Author contributions
All authors participated in the conception and the design of the study. SL and ZG designed the research. XL and CY conducted the research. FX, HoL and XW contributed in the experiment sampling. CM and SZ provided financial support. ZL revised the language. SL analyzed the data and wrote the manuscript. CW and HL revised the manuscript. All authors contributed to the article and approved the submitted version.
Funding
This work was supported by the National Key Research and Development Programme, Integration and Demonstration of High Quality, Simple and Efficient Cultivation Techniques for Soybean: [Grant Number 2020YFD1000903]; Heilongjiang Provincial Postdoctoral Science Foundation [NO. LBH-Z22074].
Acknowledgments
We thank Dr. Dong for providing the nodulating soybean seeds (Dongda 1). We thank the Academy of Agricultural Sciences for providing the non-nodulating soybean seeds (WDD01795, L8-4858).
Conflict of interest
The authors declare that the research was conducted in the absence of any commercial or financial relationships that could be construed as a potential conflict of interest.
Publisher’s note
All claims expressed in this article are solely those of the authors and do not necessarily represent those of their affiliated organizations, or those of the publisher, the editors and the reviewers. Any product that may be evaluated in this article, or claim that may be made by its manufacturer, is not guaranteed or endorsed by the publisher.
Supplementary material
The Supplementary Material for this article can be found online at: https://www.frontiersin.org/articles/10.3389/fpls.2023.1101074/full#supplementary-material
References
Batut, J., Boistard, P. (1994). Oxygen control in rhizobium. Antonie Van Leeuwenhoek 66, 129–150. doi: 10.1007/bf00871636
Becana, M., Aparicio-Tejo, P. M., Sánchez-Díaz, M. (1985). Nitrate and nitrite reduction by alfalfa root nodules: Accumulation of nitrite in Rhizobium melioti bacteroids and senescence of nodules. Physiologia Plantarum 64, 353–358. doi: 10.1111/j.1399-3054.1985.tb03352.x
Bergersen, F. J., Appleby, C. A. (1981). Leghaemoglobin within bacteroid-enclosing membrane envelopes from soybean root nodules. Planta 152, 534–543. doi: 10.1007/bf00380824
Bergersen, F. J., Peoples, M. B., Turner, G. L. (1991). A role for poly-β-hydroxybutyrate in bacteroids of soybean root nodules. Proc. R. Soc. B: Biol. Sci. 245, 59–64. doi: 10.1098/rspb.1991.0088
Brear, E. M., Day, D. A., Smith, P. M. C. (2013). Iron: an essential micronutrient for the legume-rhizobium symbiosis. Front. Plant Sci. 4. doi: 10.3389/fpls.2013.00359
Brewin, N. (1989). “The role of the plant plasma membrane in symbiosis,” in The plant plasma membrane, ed. Larsson, C., Moller, I. M. (Berlin, Heidelberg published: Springer-Verlag), 351–375.
Cabeza, R., Koester, B., Liese, R., Lingner, A., Baumgarten, V., Dirks, J., et al. (2014). An RNA sequencing transcriptome analysis reveals novel insights into molecular aspects of the nitrate impact on the nodule activity of Medicago truncatula. Plant Physiol. 164, 400–411. doi: 10.1104/pp.113.228312
Dart, P. J., Mercer, F. V. (1965). The influence of ammonium nitrate on the fine structure of nodules of Medicago tribuloides desr. and Trifolium subterraneum l. Archiv für Mikrobiologie 51, 233–257. doi: 10.1007/bf00408141
Day, D. A., Whitehead, L., Hendriks, J. H. M., Tyerman, S. D. (1995). “Nitrogen and carbon exchange across symbiotic membranes from soybean nodules,” in Nitrogen fixation: Fundamentals and applications, ed. Tikhonovich, I. A. (The Netherlands, Kluwer Academic Publishers), vol. 27. , 557–564.
De Lorenzo, C., Lucas, M. M., Vivo, A., Felipe, M. (1990). Effect of nitrate on peroxisome ultrastructure and catalase activity in nodules of Lupinus albus l. cv. multolupa. J. Exp. Bot. 41, 1573–1578. doi: 10.1093/jxb/41.12.1573
Domigan, N. M., Farnden, K. F., Robertson, J. G., Monk, B. C. (1988). Characterization of the peribacteroid membrane ATPase of lupin root nodules. Arch. Biochem. Biophysics 264, 564–573. doi: 10.1016/0003-9861(88)90322-0
Fargeix, C., Gindro, K., Widmer, F. (2004). Soybean (Glycine max. l.) and bacteroid glyoxylate cycle activities during nodular senescence. J. Plant Physiol. 161, 183–190. doi: 10.1078/0176-1617-01094
Feder, N., O'Brien, T. (1968). Plant microtechnique: Some principles and new methods. Am. J. Bot. 55, 123–142. doi: 10.1002/j.1537-2197.1968.tb06952.x
Fehr, W. R., Caviness, C. E., Burmood, D. T., Pennington, J. S. (1971). Stage of development descriptions for soybeans, Glycine max (L.) Merrill1. Crop Sci. 11, 929–931. doi: 10.2135/cropsci1971.0011183X001100060051x
Finan, T. M., Wood, J. M., Jordan, D. C. (1983). Symbiotic properties of C4-dicarboxylic acid transport mutants of Rhizobium leguminosarum. J. Bacteriology 154, 1403–1413. doi: 10.1128/jb.154.3.1403-1413.1983
Fischer, H. M. (1994). Genetic regulation of nitrogen fixation in rhizobia. Microbiological Rev. 58, 352–386. doi: 10.1128/mr.58.3.352-386.1994
Fujikake, H., Tamura, Y., Ohtake, N., Sueyoshi, K., Ohyama, T. (2003a). Photoassimilate partitioning in hypernodulation mutant of soybean (Glycine max (L.) merr.) NOD1-3 and its parent williams in relation to nitrate inhibition of nodule growth. Soil Sci. Plant Nutr. 49, 583–590. doi: 10.1080/00380768.2003.10410048
Fujikake, H., Yamazaki, A., Ohtake, N., Sueyoshi, K., Matsubayashi, Y., Ito, M., et al. (2003b). Quick and reversible inhibition of soybean root nodule growth by nitrate involves a decrease in sucrose supply to nodules. J. Exp. Bot. 54, 1379–1388. doi: 10.1093/jxb/erg147
Fujikake, H., Yashima, H., Sato, T., Norikuni, O., Sueyoshi, K., Ohyama, T. (2002). Rapid and reversible nitrate inhibition of nodule growth and N2 fixation activity in soybean (Glycine max (L.) merr.). Soil Sci. Plant Nutr. 48, 211–217. doi: 10.1080/00380768.2002.10409193
Giannakis, C., Nicholas, D. J., Wallace, W. (1988). Utilization of nitrate by bacteroids of Bradyrhizobium japonicum in the soybean root nodule. Planta 174, 51–58. doi: 10.1007/bf00394873
Goodchild, D. J., Bergersen, F. J. (1966). Electron microscopy of the infection and subsequent development of soybean nodule cells. J. Bacteriology 92, 204–213. doi: 10.1128/jb.92.1.204-213.1966
Gordon, A. J., Thomas, B. J., Reynolds, P. H. S. (1992). Localization of sucrose synthase in soybean root nodules. New Phytol. 122, 35–44. doi: 10.1111/j.1469-8137.1992.tb00050.x
Ishikawa, S., Ono, Y., Ohtake, N., Sueyoshi, K., Tanabata, S., Ohyama, T. (2018). Transcriptome and metabolome analyses reveal that nitrate strongly promotes nitrogen and carbon metabolism in soybean roots, but tends to repress it in nodules. Plants-Basel 7, 32. doi: 10.3390/plants7020032
James, E. K., Sprent, J. I., Hay, G. T., Minchin, F. R. (1993). The effect of irradiance on the recovery of soybean nodules from sodium chloride-induced senescence. J. Exp. Bot. 44, 997–1005. doi: 10.1093/jxb/44.6.997
James, E. K., Sprent, J. I., Minchin, F. R., Brewin, N. J. (1991). Intercellular location of glycoprotein in soybean nodules: effect of altered rhizosphere oxygen concentration. Plant Cell Environ. 14, 467–476. doi: 10.1111/j.1365-3040.1991.tb01516.x
Kanayama, Y., Watanabe, I., Yamamoto, Y. (1990). Inhibition of nitrogen fixation in soybean plants supplied with nitrate i. nitrite accumulation and formation of nitrosylleghemoglobin in nodules. Plant Cell Physiol. 31, 341–346. doi: 10.1093/oxfordjournals.pcp.a077913
Khan, A. A., Khan, A. A. (1981). Effects of nitrate nitrogen on growth, nodulation and distribution of 14C-labelled photosynthates in cowpea. Plant Soil 63, 141–147. doi: 10.1007/BF02374593
Kouchi, H., Akao, S., Yoneyama, T. (1986). Respiratory utilization of 13C-labelled photosynthate in nodulated root systems of soybean plants. J. Exp. Bot. 37, 985–993. doi: 10.1093/jxb/37.7.985
Kouchi, H., Nakaji, K. (1985). Utilization and metabolism of photoassimilated 13C in soybean roots and nodules. Soil Sci. Plant Nutr. 31, 323–334. doi: 10.1080/00380768.1985.10557440
Kouchi, H., Yoneyama, T. (1984). Dynamics of carbon photosynthetically assimilated in nodulated soya bean plants under steady-state conditions 1. development and application of 13CO2 assimilation system at a constant 13C abundance. Ann. Bot. 53, 875–882. doi: 10.1093/oxfordjournals.aob.a086757
Lambert, I., Pervent, M., Le Queré, A., Clément, G., Tauzin, M., Severac, D., et al. (2020). Responses of mature symbiotic nodules to the whole-plant systemic nitrogen signaling. J. Exp. Bot. 71, 5039–5052. doi: 10.1093/jxb/eraa221
Lin, J. S., Li, X., Luo, Z., Mysore, K. S., Wen, J., Xie, F. (2018). NIN interacts with NLPs to mediate nitrate inhibition of nodulation in Medicago truncatula. Nat. Plants 4, 942–952. doi: 10.1038/s41477-018-0261-3
Liu, A., Contador, C. A., Fan, K., Lam, H. M. (2018). Interaction and regulation of carbon, nitrogen, and phosphorus metabolisms in root nodules of legumes. Front. Plant Sci. 9. doi: 10.3389/fpls.2018.01860
Li, S., Xiao, F., Yang, D., Lyu, X., Ma, C., Dong, S., et al. (2021). Nitrate transport and distribution in soybean plants with dual-root systems. Front. Plant Sci. 12. doi: 10.3389/fpls.2021.661054
Lyu, X., Li, M., Li, X., Li, S., Yan, C., Ma, C., et al. (2020). Assessing the systematic effects of the concentration of nitrogen supplied to dual-root systems of soybean plants on nodulation and nitrogen fixation. Agronomy 10, 763. doi: 10.3390/agronomy10060763
Lyu, X., Sun, C., Lin, T., Wang, X., Li, S., Zhao, S., et al. (2022). Systemic regulation of soybean nodulation and nitrogen fixation by nitrogen via isoflavones. Front. Plant Sci. 13. doi: 10.3389/fpls.2022.968496
Lyu, X., Xia, X., Wang, C., Ma, C., Dong, S., Gong, Z. (2019). Effects of changes in applied nitrogen concentrations on nodulation, nitrogen fixation and nitrogen accumulation during the soybean growth period. Soil Sci. Plant Nutr. 65, 1–11. doi: 10.1080/00380768.2019.1667213
Matamoros, M., Baird, L., Escuredo, K., Dalton, D., Minchin, F., Iturbe-Ormaetxe, I., et al. (1999). Stress-induced legume root nodule senescence. physiological, biochemical, and structural alterations. Plant Physiol. 121, 97–112. doi: 10.1104/pp.121.1.97
Minchin, F. R., Becana, M., Sprent, J. I. (1989). Short-term inhibition of legume N2 fixation by nitrate : II. nitrate effects on nodule oxygen diffusion. Planta 180, 46–52. doi: 10.1007/bf02411409
Naisbitt, T., Sprent, J. I. (1993). The long-term effects of nitrate on the growth and nodule structure of the caesalpinioid herbaceous legume chamaecrista fasciculata michaux. J. Exp. Bot. 44, 829–836. doi: 10.1093/jxb/44.4.829
Newcomb, W., Syono, K., Torrey, J. G. (1977). Development of an ineffective pea root nodule: Morphogenesis, fine structure, and cytokinin biosynthesis. Can. J. Bot. 55, 1891–1907. doi: 10.1139/b77-217
Nishida, H., Tanaka, S., Handa, Y., Ito, M., Sakamoto, Y., Matsunaga, S., et al. (2018). A NIN-LIKE PROTEIN mediates nitrate-induced control of root nodule symbiosis in Lotus japonicus. Nat. Commun. 9, 499. doi: 10.1038/s41467-018-02831-x
Okamoto, S., Ohnishi, E., Sato, S., Takahashi, H., Nakazono, M., Tabata, S., et al. (2009). Nod factor/nitrate-induced CLE genes that drive HAR1-mediated systemic regulation of nodulation. Plant Cell Physiol. 50, 67–77. doi: 10.1093/pcp/pcn194
Reid, D., Ferguson, B., Gresshoff, P. (2011). Inoculation- and nitrate-induced CLE peptides of soybean control NARK-dependent nodule formation. Mol. Plant-Microbe Interact. 24, 606–618. doi: 10.1094/MPMI-09-10-0207
Sheokand, S., Babber, S., Swaraj, K. (1998). Nodule structure and functioning in Cicer arietinum as affected by nitrate. Biol. Plantarum 41, 435–443. doi: 10.1023/A:1001810813047
Singleton, P. W., Kessel, C. V. (1987). Effect of localized nitrogen availability to soybean half-root systems on photosynthate partitioning to roots and nodules. Plant Physiol. 83, 552–556. doi: 10.1104/pp.83.3.552
Streeter, J. G. (1980). Carbohydrates in soybean nodules: II. distribution of compounds in seedlings during the onset of nitrogen fixation. Plant Physiol. 66, 471–476. doi: 10.1104/pp.66.3.471
Streeter, J. G. (1991). “Transport and metabolism of carbon and nitrogen in legume nodules,” in Advances in botanical research, ed. Callow, J. A. (Academic Press Inc. published), vol. 18, 129–187.
Sulieman, S., Schulze, J., Tran, L. S. (2014). N-feedback regulation is synchronized with nodule carbon alteration in Medicago truncatula under excessive nitrate or low phosphorus conditions. J. Plant Physiol. 171, 407–410. doi: 10.1016/j.jplph.2013.12.006
Trainer, M. A., Charles, T. C. (2006). The role of PHB metabolism in the symbiosis of rhizobia with legumes. Appl. Microbiol. Biotechnol. 71, 377–386. doi: 10.1007/s00253-006-0354-1
Trinchant, J. C., Yang, Y. S., Rigaud, J. (1998). Proline accumulation inside symbiosomes of faba bean nodules under salt stress. Physiologia Plantarum 104, 38–49. doi: 10.1034/j.1399-3054.1998.1040106.x
Truchet, G. L., Dazzo, F. B. (1982). Morphogenesis of lucerne root nodules incited by Rhizobium meliloti in the presence of combined nitrogen. Planta 154, 352–360. doi: 10.1007/bf00393915
Udvardi, M. K., Day, D. A. (1997). Metabolite transport across symbiotic membranes of legume nodules. Annu. Rev. Plant Physiol. Plant Mol. Biol. 48, 493–523. doi: 10.1146/annurev.arplant.48.1.493
Voisin, A. S., Salon, C., Jeudy, C., Warembourg, F. R. (2003a). Root and nodule growth in Pisum sativum l. @ in relation to photosynthesis: Analysis using 13C-labelling. Ann. Bot. 92, 557–563. doi: 10.1093/aob/mcg174
Voisin, A. S., Salon, C., Jeudy, C., Warembourg, F. R. (2003b). Seasonal patterns of 13C partitioning between shoots and nodulated roots of N2- or nitrate-fed Pisum sativum l. Ann. Bot. 91, 539–546. doi: 10.1093/aob/mcg055
Wang, Q., Huang, Y., Ren, Z., Zhang, X., Ren, J., Su, J., et al. (2020). Transfer cells mediate nitrate uptake to control root nodule symbiosis. Nat. Plants 6, 800–808. doi: 10.1038/s41477-020-0683-6
Wasfi, M., Prioul, J. L. (1986). A comparison of inhibition of French-bean and soybean nitrogen fixation by nitrate, 1% oxygen or direct assimilate deprivation. Physiologia Plantarum 66, 481–490. doi: 10.1111/j.1399-3054.1986.tb05955.x
Wong, P. P., Evans, H. J. (1971). Poly-β-hydroxybutyrate utilization by soybean (Glycine max merr.) nodules and assessment of its role in maintenance of nitrogenase activity. Plant Physiol. 47, 750–755. doi: 10.1104/pp.47.6.750
Xia, X., Ma, C., Dong, S., Xu, Y., Gong, Z. (2017). Effects of nitrogen concentrations on nodulation and nitrogenase activity in dual root systems of soybean plants. Soil Sci. Plant Nutr. 63, 470–482. doi: 10.1080/00380768.2017.1370960
Keywords: nitrate, dual-root soybean, nodule structure, symbiosome, carbon distribution
Citation: Li S, Wu C, Liu H, Lyu X, Xiao F, Zhao S, Ma C, Yan C, Liu Z, Li H, Wang X and Gong Z (2023) Systemic regulation of nodule structure and assimilated carbon distribution by nitrate in soybean. Front. Plant Sci. 14:1101074. doi: 10.3389/fpls.2023.1101074
Received: 17 November 2022; Accepted: 13 January 2023;
Published: 06 February 2023.
Edited by:
Fernando Carlos Gómez-Merino, Colegio de Postgraduados (COLPOS), MexicoReviewed by:
Ricardo A. Cabeza, University of Talca, ChileIgor Kryvoruchko, United Arab Emirates University, United Arab Emirates
Jean-jacques Drevon, DEVA Domaine Experimental du Val d Ainan, France
Copyright © 2023 Li, Wu, Liu, Lyu, Xiao, Zhao, Ma, Yan, Liu, Li, Wang and Gong. This is an open-access article distributed under the terms of the Creative Commons Attribution License (CC BY). The use, distribution or reproduction in other forums is permitted, provided the original author(s) and the copyright owner(s) are credited and that the original publication in this journal is cited, in accordance with accepted academic practice. No use, distribution or reproduction is permitted which does not comply with these terms.
*Correspondence: Zhenping Gong, Z3pweXgyMDA0QDE2My5jb20=