- 1Department of Genetics and Plant Breeding, Agriculture University, Jodhpur, Rajasthan, India
- 2Global Wheat Program, International Maize and Wheat Improvement Center (CIMMYT), Mexico DF, Mexico
- 3Crop Improvement Division, ICAR–Vivekanand Parvatiya Krishi Anushandhan Sansthan, Almora, Uttarakhand, India
- 4Department of Plant Pathology, Bidhan Chandra Krishi Viswavidyalaya, Mohanpur, West Bengal, India
Spot blotch (SB) caused by Bipolaris sorokiniana (teleomorph Cochliobolus sativus) is one of the devastating diseases of wheat in the warm and humid growing areas around the world. B. sorokiniana can infect leaves, stem, roots, rachis and seeds, and is able to produce toxins like helminthosporol and sorokinianin. No wheat variety is immune to SB; hence, an integrated disease management strategy is indispensable in disease prone areas. A range of fungicides, especially the triazole group, have shown good effects in reducing the disease, and crop-rotation, tillage and early sowing are among the favorable cultural management methods. Resistance is mostly quantitative, being governed by QTLs with minor effects, mapped on all the wheat chromosomes. Only four QTLs with major effects have been designated as Sb1 through Sb4. Despite, marker assisted breeding for SB resistance in wheat is scarce. Better understanding of wheat genome assemblies, functional genomics and cloning of resistance genes will further accelerate breeding for SB resistance in wheat.
Introduction
Spot blotch (SB) caused by the hemibiotrophic fungus Bipolaris sorokiniana (teleomorph Cochliobolus sativus) syn. Drechslera sorokiniana, syn. Helminthosporium sativum is the most devastating disease of wheat grown in warm and humid areas. In Eastern Gangetic Plains (EGP) of India, Bangladesh and Nepal, B. sorokiniana appears in a complex with Pyrenophora tritici-repentis (Died.) Drechs. (anamorph Drechslera tritici-repentis (Died.) Shoemaker) responsible for tan spot (TS) and is commonly known as Helminthosporium leaf blight (HLB) (Duveiller et al., 2005). Occurrence of SB is more frequent in the humid and warmer wheat growing areas of South Asia (SA), Latin America and Africa (He et al., 2022) (Figure 1). Globally, the disease appears in approximately 25-million-hectare (mha) areas, out of which 10 mha areas are present in EGP. Besides, wheat grown under subtropical lowland of Bolivia, Brazil and Argentina in Latin America, Tanzania, rainfed areas of Zambia and Madagascar in Africa provides congenial environments for SB (van Ginkel and Rajaram, 1998). Under favorable conditions, the disease may cause yield loss of above 50% (Sharma and Duveiller, 2004), with an average yield loss of 15-20% in SA, and yield loss in the farmer’s field reported up to 16% in Nepal and 15% in Bangladesh (Villareal et al., 1995; Saari, 1998; Duveiller, 2002).
B. sorokiniana causes a typical symptom of light brown colored lesions of oval or oblong to elliptical shape on leaves, sheath, nodes or glume of its host plants. Size of the lesion gradually increases, then partial to whole leaf may become chlorotic, turning brown and drying up (Figure 2). The pathogen transmits through infected seeds, stubbles and soil, and secondary infection may take place through air. Other than SB, B. sorokiniana is also responsible for seedling blight, common root rot, head blight and black point in wheat (Al-Sadi, 2021). No positive association was found among spot blotch, root rot and black point in wheat, indicating that different mechanisms of host resistance exist in different plant parts (Conner, 1990). Cross infection between different species is rarely reported; one such example is isolates collected from wheat root were able to infect barley (Valjavec-Gratian and Steffenson, 1997). A number of articles have been published pertaining to pathogen biology, disease management, breeding and molecular aspects, including genome sequence of B. sorokiniana, gene/QTL mapping, marker-assisted selection (MAS) and genomic selection. The present work aims to summarize the most important findings, especially those reported in recent years, in the area of SB management in wheat.
Pathogen biology
The pathogen produces olive brown mycelia and light grayish colonies on potato dextrose agar (PDA) medium at early stage, which turns into black at later stage. Conidia are brown colored, elliptical, straight or curved multiple celled with 3-9 septa tapering at the ends, measuring 10-28 × 40-120 µm (Acharya et al., 2011). Variability under natural conditions among the B. sorokiniana isolates is sufficiently high (Sultana et al., 2018). Bipolaris sorokiniana is the asexual stage of the pathogen, which multiplies mostly through conidia. Its sexual stage has not been reported in natural conditions except in Zambia, due to a lack of sexual compatibility between the opposite mating (A, a) types. Under controlled conditions, sexual spores of B. sorokiniana were isolated from barley (Zhong and Steffenson, 2001) and recently from wheat in Bangladesh (Sultana et al., 2018).
The fungus can produce toxins like helminthosporol and hydrolytic enzymes that trigger pathogenesis. Prehelminthosporium is the most abundant and active compound produced by B. sorokiniana, which damages membrane permeability and affects mitochondrial oxidative phosphorylation and chloroplast photophosphorylation (Kumar et al., 2002). A necrotrophic effector gene ToxA interacts with the susceptibility gene Tsn1 in wheat to initiate disease development (McDonald et al., 2017). ToxA was initially identified in P. tritici-repentis and then in Parastagonospora nodorum, but molecular evidence indicated that the gene in the former was acquired from the latter through horizontal gene transfer (Friesen et al., 2006). ToxA was found in B. sorokiniana populations from Australia (McDonald et al., 2017), USA (Friesen et al., 2018), India (Navathe et al., 2019b) and Mexico (Wu et al., 2020), with various occurrence frequencies, from 10.2% in Mexico to 86.7% in USA.
Molecular markers may be useful to detect the pathogen on wheat plants before the appearance of visible symptoms, as well as on alternative hosts and volunteer plants. A sequence characterized amplified region (SCAR) marker SCARBS600 was developed to diagnose B. sorokiniana (Aggarwal et al., 2011). Alternatively, DNA sequence of ribosomal internal transcribe spacer (ITS), β-tubulin gene and translational elongation factor 1-α (EF-1α) can also be used to diagnose this pathogen, as did in a study to detect B. sorokiniana in volunteer plants in China (Sun et al., 2015). Using universal rice primers (URP), Aggarwal et al. (2010) successfully grouped 40 B. sorokiniana isolates from different geographical origins of India. High level of genetic diversity among the isolates from Brazil and Mexico was reported using UPR markers (Mann et al., 2014).
Draft genome sequences of eight virulent accessions of B. sorokiniana from India, Australia, USA and China are currently available (https://www.ncbi.nlm.nih.gov/data-hub/genome/?taxon=45130). A highly virulent isolate BS_112 (GenBank accession number KU201275) from India has a genome size of 35.64 Mb and 10,460 genes were predicted with an average gene length of 435-545 bp and gene density of 250-300 genes/Mb (Aggarwal et al., 2019). A phylogenetic analysis was carried out among 254 isolates of B. sorokiniana with global origin using gene sequences of ITS, TEF-1 and GAPDH, and the results indicated the presence of a broad and geographically undifferentiated global population (Sharma et al., 2022).
Biochemical and molecular events associated with SB infection
Bipolaris sorokiniana initially has a biotrophic phase represented by the epidermal invasion and fungal hyphal growth, followed by the necrotrophic phase in the mesophyll cells (Kumar et al., 2002). The germinating conidia penetrates the cuticle and epidermis of wheat plant with the help of an appressorium at its germinating tube, getting entered mostly through the anticlinal cell walls. The levels of sesquiterpene molecule ‘prehelminthosporol’ increases in the extracellular matrix of the cell at the site of apposition, helping pathogen to intrude further into the cell (Jansson and Akesson, 2003).
The first toxin compound known to confer virulence to B. sorokiniana was named ‘Victoxinin’ and the second one was ‘sorokinianin’. Another toxin isolated and characterized was ‘bipolaroxin’, which was again structurally a sesquiterpene and had a role in pathogenicity and host selectivity (Jahani, 2005). Helminthosporol was found to enhance the susceptibility of genotypes like Sonalika and CIANO T79. However, it is important to note that helminthosporol and its derivatives are not solely responsible for deciding the susceptibility of a genotype. A multitude of other factors like cell wall apposition, cuticle thickness, leaf anatomy, pathogen specificity, host defense responses etc., are also involved in the pathosystem, making both resistance/susceptibility of the host and virulence/avirulence of the pathogen (Ibeagha et al., 2005; Jahani et al., 2014).
For initial invasion, B. sorokiniana produces various cell wall degrading enzymes like glucosidase, cellulases, pectinases, xylanase. Endopolygalacturonase (EPG) loosens the cell wall by cleaving α-(1→4) linkages of the homogalacturonan, an important constituent of the middle lamella of the cell wall (Ridley et al., 2001; Janni et al., 2008). To protect the host cell from EPG, plant cell produces polygalacturonase inhibiting protein (PGIP), which elicits the defense response of a plant by accumulating oligogalacturonides (Ridley et al., 2001). PGIPs have a proven role against the fungal colonization in many dicot species as well as wheat plant against Bipolaris (Kemp et al., 2003).
Epidemiology and host range
Generally, temperature between 16-32°C enables SB development (Acharya et al., 2011), and in Indian subcontinent, the disease predominantly spread when temperature exceeds 26°C as it favors heavy sporulation (Chaurasia et al., 2000). Teleomorph develops in a range of 16-24°C with the optimum temperature of 20°C and can survive up to seven months under natural conditions in Zambia; while the anamorph can survive sufficiently large range of temperature from 4-36°C (Duveiller and Sharma, 2009). High temperature and high relative humidity enhance disease severity, SB outbreak in Brazil occurred when the leaves remain wet for >18 hrs in a day with a mean temperature of >18°C (Reis, 1991). In EGP, leaf wetness period >12hrs due to rainfall or dew coinciding with high temperature and humidity are believed to favor the onset of infection (Duveiller et al., 2005). In addition, delayed sowing of wheat due to the rice-wheat cropping system causes yield loss due to terminal heat stress (Hasan et al., 2021; Narendra et al., 2021); besides, high residual soil moisture and increased duration of leaf wetness due to foggy weather can also increase the disease severity (Duveiller, 2004; Duveiller et al., 2005). The waterlogged condition due to flooding in the Ganges belt sharply declines the conidia viability, and B. sorokiniana conidia isolated from soil after August, the monsoon month with high rainfall, becomes non-pathogenic (Pandey et al., 2005). This in turn implies that seeds might be the main source of inoculum in EGP.
SB is a polycyclic disease with the initial sources of inoculum being contaminated seeds, infected soil, straw, volunteer plants and secondary hosts. B. sorokiniana has a large host range, and more than 65 graminaceous hosts have been identified in China (Chang and Wu, 1998). Among the cereals, hexaploid wheat and barley are most common hosts, along with durum and emmer wheat, triticale, oats, rice, rye, maize, pearl millet, foxtail millet and several grass species like Phalaris minor, Agropyron pectinatum, A. repens, Festuca spp. (Gupta et al., 2017). A list of plant species that harbors B. sorokiniana is given in Table 1. The three most common species, Setaria glauca, Echinochloa colonum and Pennisetum typhoids act as a natural harbor of B. sorokiniana in EGP (Pandey et al., 2005). In rice-wheat cropping system, rice plants may serve as a host for the pathogen (Acharya et al., 2011); but in eastern India the source of primary inoculum is still debatable, with infected seeds and weeds being the most probable inoculum sources (Neupane et al., 2010).
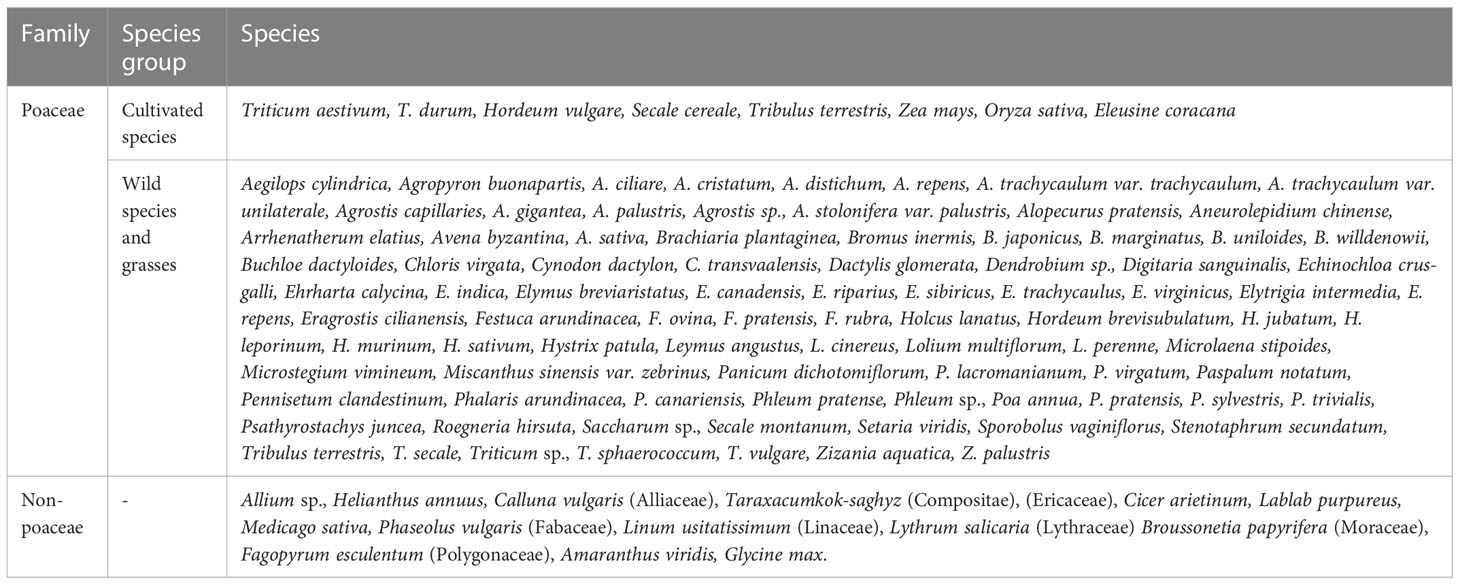
Table 1 Host species of B. sorokiniana (Modified from Manamgoda et al., 2014).
Disease management strategies
Management of SB through resistant varieties is the most economical and environment-friendly approach, which, however, is compromised by a lack of highly resistant varieties. Under this circumstance, cultivation of resistant varieties may be supplemented with other strategies like adjusting sowing time and fungicides application to reduce the SB severity in disease prone areas. Details of these strategies are described below.
Chemical control
Seed treatment is always useful to avoid the introduction of additional inoculum. Seed treatment with carboxin or thiram can effectively reduce the load of primary inoculum, especially for seeds with more than 20% infection rate (Mehta et al., 1992). However, seed treatment alone cannot guarantee low spot blotch infection in field (Singh et al., 2014) and foliar fungicidal application is often indispensable. Triazole fungicides like propiconazole, tebuconazole, flutriafol, iprodione, prochloraz, and triadimenol are effective in SB management, e.g., application of Opus (epoxiconazole) significantly reduced the disease severity and maintained it below 10% (Sharma and Duveiller, 2006). In addition, application of Carbendazim and Azoxystrobin has also shown efficacy in controlling the disease (Navathe et al., 2019a). Applying both seed treatment and foliar spray can further reduce the disease, especially when the latter is conducted twice, e.g., upon the appearance of initial infection symptom and 10-20 days later (Singh et al., 2014; Navathe et al., 2019a). Systemic fungicides are more effective than contact fungicides, but the recommended dose should be strictly followed to avoid the emergence of resistant pathotypes against fungicides.
Despite its effectiveness in SB management, fungicidal application increases the cost of cultivation and brings environmental hazardousness. An estimated cost of 153.5 million AUD including application cost is required for fungicides to control wheat diseases in Australia (Murray and Brennan, 2009). Besides, excessive use of systemic fungicides may lead to changes in pathogenic virulence and development of resistance against fungicides. Fungicide resistance has been reported for leaf blight related pathogens P. tritici-repentis causing tan spot (Sautua and Carmona, 2021). This is due to the directional selection on pathogen population for resistant pathotypes.
Poor nutrient management is reported to be associated with higher SB infection (Sharma and Duveiller, 2004). Appropriate nitrogen application reduces SB infection, and balanced application of nitrogen along with phosphorus and potassium can further reduce SB severity (Sharma et al., 2006a). Exogenous use of silicon significantly reduces SB severity by increasing the incubation period of the pathogen in wheat (Domiciano et al., 2010). Similarly, application of silver nanoparticles significantly reduced SB infection in wheat, with the induced lignin deposition in vascular bundles (Mishra et al., 2014).
Cultural practices
Crop rotation is an effective practice for minimizing the primary inoculum load of B. sorokiniana in wheat, and the rotation systems of wheat-rice, wheat-oat, wheat-sunflower, and wheat-soybean may be adopted instead of wheat monoculture. Crop rotation provides time to decompose the infected stubble in the field, which helps in improving soil health. Crop residue burning reduces inoculum load up to 90%; but is associated with environmental hazardousness. Alternatively, tillage can be adopted to minimize the load of primary inoculum from the infected stubble. But this may delay the sowing of wheat crop particularly in rice-wheat cropping system, exposing wheat to SB conducive conditions. Zero tillage, minimum tillage or use of happy seeder are alternatives to traditional tillage practices in rice-wheat cropping system (Acharya et al., 2011). Zero tillage facilitates the sowing 10-15 days earlier which helps in escaping the terminal heat stress and results in yield gain by 10-25% in EGP (Joshi et al., 2007c; McDonald et al., 2022). Early sowing is effective in reducing SB, subjected to selection of suitable variety for early sowing as 1) the genotypes must have capacity to tolerate high temperature at early crop growth stage; 2) proper management of foliar blight diseases; as sometimes higher leaf bight incidence was observed upon early sowing due to high residual moisture and humidity (Duveiller, 2004). Therefore, judicial selection of resistant variety is required to minimize the trade off in yield gain by early sowing and higher incidence of leaf blight. In a study, PBW 343, HUW 234 and HUW 468 were found suitable for growing under zero tillage practices (Joshi et al., 2007a).
Disease resistance
Growing resistant variety is the most effective method of managing crop disease. It is noteworthy that commercial varieties are moderately resistant to susceptible, and such varieties could be heavily infected under SB conducive environment. An early study by Sharma and Dubin (1996) showed increased resistance using multiline mixture resulted in reduction of area under disease progress curve (AUDPC) up to 57% and increased yield up to 8.6% than the component lines. Evaluation of wheat germplasm under different agro-climatic conditions has led to the identification of resistant genotypes. Genotypes SW 89-5193, SW 89-3060 and SW 89-5422 were resistant with 3.9, 2.6 and 3.5% reduction in grain weight, respectively, due to HLB, compared to 33% and 27.6% loss in susceptible cultivars BL 1135 and Sonalika, respectively (Sharma et al., 2004a). Sharma et al. (2004b) reported that SW 89-5422, Yangmai-6, Ning 8201, Chirya 7, Chirya 1 and CIGM90.455 were HLB resistant. These genotypes have been used in developing resistant lines. Furthermore, increasing the level of resistance in new varieties will be effective in managing SB in disease prone areas. Near immune line has been developed from the crosses between resistant genotypes (Kumar et al., 2019); yet further field evaluations are needed to confirm the stability of their resistance as well as their performance in other traits for possible release as varieties.
Genetics of disease resistance
Quantitative nature of SB resistance is predominantly reported in wheat (Singh et al., 2018; Bainsla et al., 2020; He et al., 2020), but reports are also available for major gene(s) governing SB resistance. Single dominant gene was postulated in the resistant genotypes Chirya 3 and MS#7 when they were crossed with common susceptible parent BL1473 (Neupane et al., 2007). Likewise, single dominant gene was reported in the resistant genotype DT 188, whereas digenic dominant resistance was reported in the lines E5895, HD 1927 and Motia (Adlakha et al., 1984). More genes were estimated in other resistant varieties, e.g., 2-3 genes in Gisuz, Cugap, Chirya 1 and Sabuf (Velazquez-Cruz, 1994), and three genes in Acc.8226, Mon/Ald and Suzhoe#8 (Joshi et al., 2004b). Populations in these two studies have already began to show a pattern similar to polygenic segregation, implying that most resistant sources are governed by multiple genes with minor effects, which increases the chances of deriving transgressive segregants in the progenies (Singh et al., 2018; He et al., 2020). The magnitude of heritability (h2) for SB resistance varied greatly in different studies, e.g., from 0.21 to 0.64 in Sharma et al. (2006b) and 0.85 to 0.89 in He et al. (2020), whereas most studies exhibited moderate to high heritability, providing a good opportunity to select resistant genotypes in breeding programs.
Detection of quantitative trait loci (QTL)
Quantitative disease resistance slows down the disease development by increasing the latency period, though, does not always show a clear-cut difference from qualitative resistance conferred by gene-for-gene interaction (Krattinger and Keller, 2016). Most of the QTLs for SB resistance were detected using bi-parental mapping population (Table 2), and four major QTLs have been designated as Sb1 through Sb4. Sb1 was mapped on chromosome arm 7DS, being co-located with Lr34 (Lillemo et al., 2013) that has been cloned and encodes an ABC (ATP binding cassette) transporter (Krattinger et al., 2009). Sb2 was detected on chromosome arm 5BL (Kumar et al., 2015), and proposed to be the same locus earlier mapped as QSb.bhu-5B in Yangmai 6 (Kumar et al., 2009). Later, Sb3 was identified in a winter wheat resistant line 621-7-1, being located on chromosome arm 3BS flanked by the markers Xbarc133 and Xbarc147 (Lu et al., 2016). Recently, Sb4 was detected and fine mapped on chromosome arm 4BL flanked by the markers YK12831 and YK12928 (Zhang et al., 2020). Besides, a necrosis insensitivity gene tsn1 was mapped on 5BL associated with ToxA insensitivity, and selection for genotypes with tsn1 may confer resistance against B. sorokiniana isolates with ToxA (Navathe et al., 2019b).
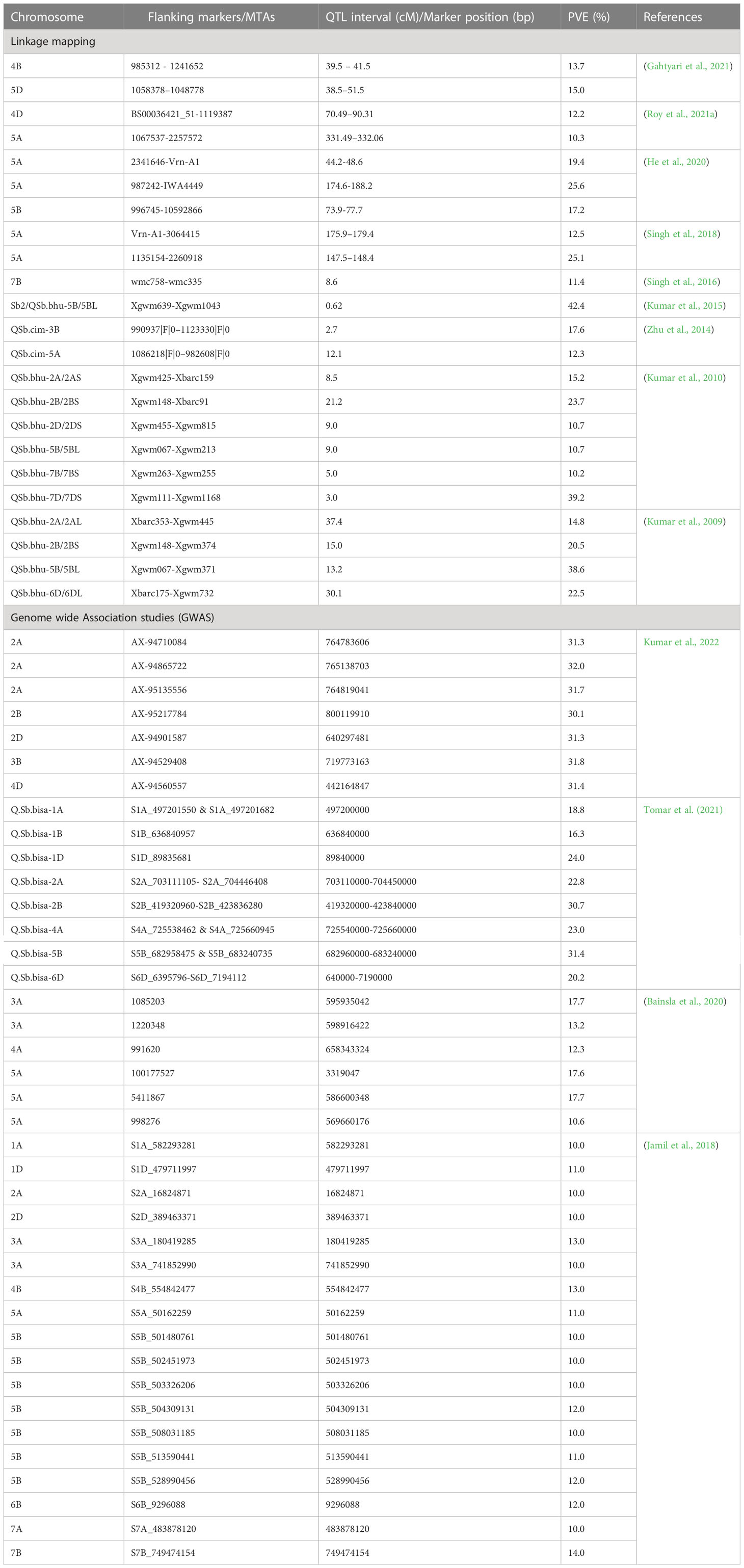
Table 2 QTLs/MTAs (PVE of ≥10%) mapped on different chromosomes for spot blotch resistance using linkage mapping and GWAS.
Genome wide association studies (GWAS) have been widely performed to detect SB resistance QTLs in wheat. In a study using 566 spring wheat landraces, Adhikari et al. (2012) reported four genomic regions on 1A, 3B, 7B and 7D that were associated with SB resistance. One of the markers, wPt-1159 on 3B, was also associated with resistance to powdery mildew, yellow rust and grain yield (Crossa et al., 2007), and thus could be more useful in breeding. A GWAS on 528 spring wheat landraces with global origin reported 11 significant markers on chromosomes 1B, 5A, 5B, 6B and 7B with phenotypic variation explained (PVE) ranging from 0.14 to 5.80% (Gurung et al., 2014). Recently, 25 significant marker-trait associations (MTA) were identified in a panel of 301 Afghan wheat lines, being located on chromosomes 1A, 1B, 1D, 2B, 2D, 3A, 3B, 4A, 5A, 5B, 6A, 7A, and 7D with PVE ranging from 2.0-17.7% (Bainsla et al., 2020). Major challenges are faced by wheat breeders due to most identified QTLs/MTAs are of minor effects and have no diagnostic markers. Till date, diagnostic markers are available only for Sb1 (Lr34) (Krattinger et al., 2009) and tsn1 (Faris et al., 2010). A list of QTLs/MTAs for SB resistance is shown in Table 2, and an integrated map indicating major genes, QTLs and MTAs on different wheat chromosomes is shown in Figure 3.
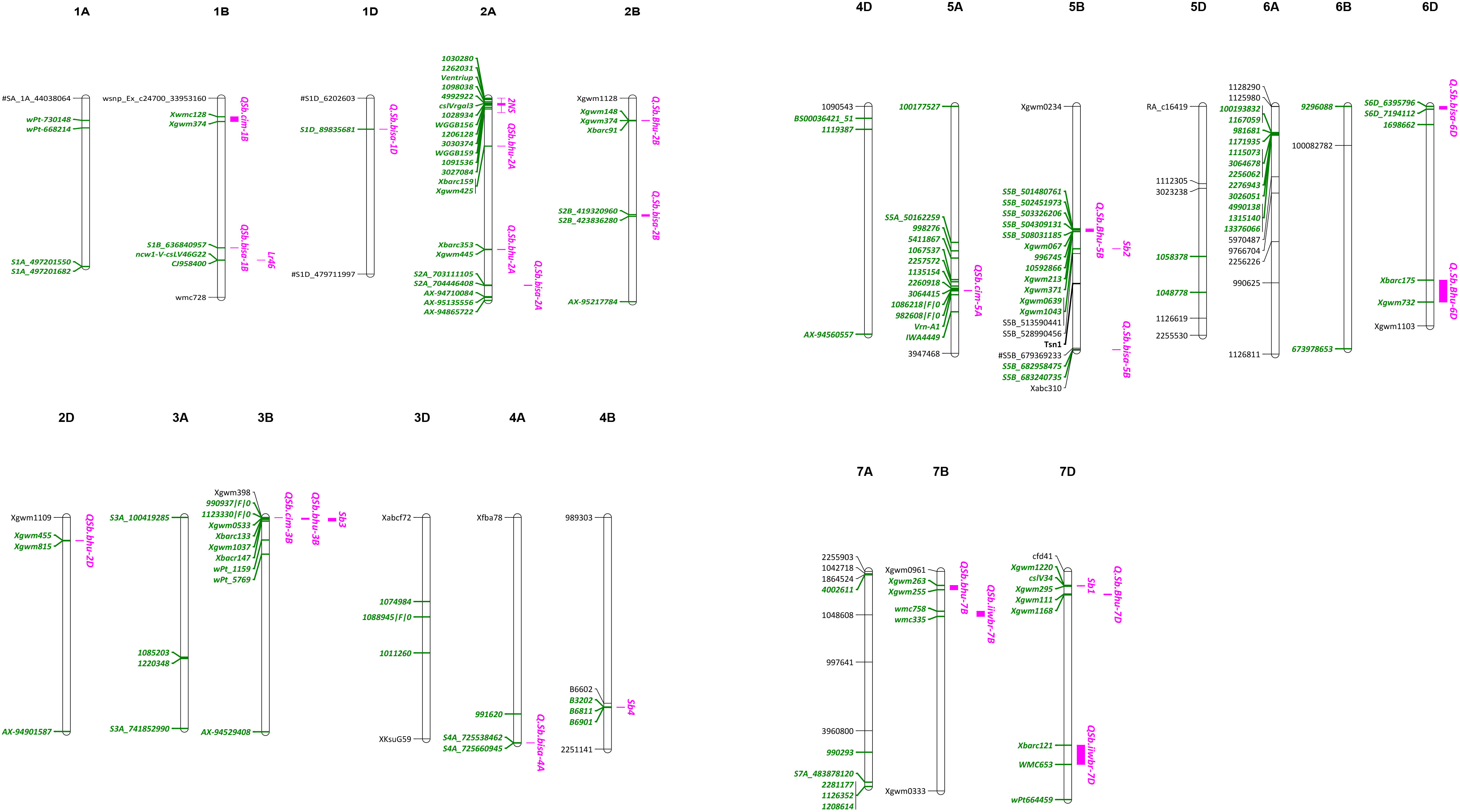
Figure 3 An integrated map showing genes and QTLs for SB resistance and their flanking markers (green color).
Traits associated with SB resistance
Association of SB resistance with plant height and heading date has been well established, with high stature and late maturity often associated with low SB severity (Singh et al., 2015; He et al., 2020). This is mostly due to disease escape mechanisms; though, the possibility of tight linkage of Rht and Vrn genes with SB resistance genes cannot be ruled out (Zhu et al., 2014; He et al., 2020). Nevertheless, such linkages can be broken, as early and short lines with good SB resistance have been identified in some studies (Joshi et al., 2002; Sharma and Duveiller, 2004; Joshi et al., 2007d). Such early lines are especially important for SA, where terminal heat stress and SB are major yield constraints.
Leaf orientation influences the plant micro-climate, particularly temperature and humidity, through regulating evapotranspiration, and germplasm with erect to semi-erect leaves often showed good SB resistance (Joshi and Chand, 2002). Leaf tip necrosis associated with SB resistance serves as a good morphological marker (Joshi et al., 2004a). Stay-green (SG) genotypes are photosynthetically more active under biotic and abiotic stress conditions, and a positive correlation of SG with SB resistance was reported (Joshi et al., 2007b).
Breeding for disease resistance
Sources of SB resistance
Green revolution has resulted in the development of cultivars of semi-dwarf, fertilizer responsive and wider adaptability, enabling the cultivation of wheat in non-traditional areas, including the humid and hot regions with severe SB epidemics. Significant genetic variation for SB resistance was observed among the genotypes evaluated in India, Nepal and Bangladesh (Sharma et al., 2004a; Joshi et al., 2007d). Large-scale SB screening programs were initiated in the late 1980s when the disease became a major threat to wheat production in SA (Duveiller and Sharma, 2012). Initially, resistant sources were identified from Latin American particularly Brazilian germplasm like BH 1146, CNT 1, Ocepar 7, as well as from China like Shanghai 1 to 8, Suzhoe 1 to 10, Wuhan 1 to 3, Ning 8201, Longmai 10 and Yangmai #6 (van Ginkel and Rajaram, 1998). Using such lines as resistant donors, promising genotypes were developed at CIMMYT-Mexico, which exhibited good resistance against SB when tested in Bolivia, Nepal, India and Bangladesh (Sharma et al., 2004b; Sharma and Duveiller, 2007). Recent large-scale germplasm screening activities involve a work on screening 19,460 accessions from Indian national gene bank under field conditions, and 868 accessions were found to be resistant to moderately resistant (Kumar et al., 2016). Further screening of unexplored germplasm from gene bank has identified near immune response in the genotypes EC664204, IC534306 and IC535188 (Kumar et al., 2022).
Wild relatives are a rich source of SB resistance. The 2NS chromosome segment transferred from Ae. ventricose has been associated with resistance against wheat blast (Singh et al., 2021; Roy et al., 2021b), rusts (Helguera et al., 2003), cereal cyst nematode (Jahier et al., 2001) and lodging (Singh et al., 2019), and recently it was also associated with SB resistance (Juliana et al., 2022b). Thinopyrum curvifolium (Mujeeb-Kazi et al., 1996; van Ginkel and Rajaram, 1998) and synthetic hexaploid wheat derived from crosses between T. turgidum and Aegilops tauschii (Mujeeb-Kazi et al., 2007) serve as additional resistant sources. Good examples are Chirya genotypes derived from wide hybridization and exhibited good SB resistance, like Chirya 1, Chirya 3 and Chirya 7 (Sharma et al., 2007; Joshi et al., 2007b). High proportion of SB resistance was reported among the synthetic hexaploids evaluated under controlled conditions (Lozano-Ramirez et al., 2022). However, genotypes with high level of field SB resistance are scarce, a major limitation in the progress of breeding program.
Development of resistant genotypes
Breeding for resistant genotypes through crossing programs were started in 1980s in CIMMYT, Mexico and still this center is playing an important role in global SB resistance breeding. Ever since 2009, a special nursery was formed as CSISA-SB (presently known as Helminthosporium Leaf Blight Screening Nursery, HLBSN), comprising high yielding SB resistant genotypes for testing over the different countries in SA, Africa and Latin America (Singh et al., 2015). Testing of the 4th CSISA-SB nursery at seven locations in Mexico, India and Bangladesh identified two stably resistant lines (CHUKUI#1 and VAYI#1) consistent over the locations (Singh et al., 2015).
The progress in achieving genetic gain for SB resistance is slow, due to reasons like quantitative inheritance, moderate heritability, strong genotype × environmental interaction, and high variability of the pathogen over time. Phenotypic selection is often associated with confounding traits, and molecular markers can be used to assist the pyramiding of resistance QTLs and selection for SB resistant genotypes (He et al., 2020). Superior genotypes have been developed from a marker assisted backcross program via transferring Qsb.bhu-2A and Qsb.bhu-5B from Chirya 3 and Qsb.bhu-2A from Ning 8201 into the genetic background of HUW 234 (Vasistha et al., 2015). Increased resistance up to near immunity could be obtained by stacking effective QTLs from multiple donors (Kumar et al., 2019). However, such QTL stacking could be compromised by QTL × QTL interaction, as demonstrated by Kumar et al. (2019) in a cross between “Yangmai#6” and “Chirya#3”, where QTLs on 6D and 7D have a masking effect on each other. This highlights the importance of understanding the mode of action (additive, dominant, or epistatic) of the QTLs to be utilized in breeding.
Genomic selection can improve the efficiency of breeding program by reducing phenotyping cost, time and increasing selection intensity and genetic gain. Studies on genomic selection for SB in wheat are limited, and a successful example was reported by Juliana et al. (2022a), where genomic selection showed significantly higher accuracy than the fixed effect model using few selected markers. However, there is a long way to go for genomic selection to completely replace phenotypic selection in wheat breeding.
Biotechnological approaches
Gene silencing through RNAi is a powerful tool for controlling insects, nematodes, viruses, fungal diseases like powdery mildew, and rusts (Qi et al., 2019). Utilization of RNAi in functional genomic analysis in B. sorokiniana was reported by Leng et al. (2011), and it can be further explored in SB pathogenesis and resistance breeding in wheat.
Liu et al. (2012) developed a new mapping strategy combining bulk segregant analysis and RNA-Seq called ‘BSR-Seq’, where transcripts are sequenced from extreme bulks, being a potential technique for marker discovery in large polyploid genome like wheat. Using BSR-Seq, five SB-resistance associated transcripts were identified on 5B and 3B chromosomes and their potential role in SB resistance were inferred (Saxesena et al., 2022).
Transgenic lines expressing foreign genes proved to be a potential approach to control insect and diseases in several crop plants. Examples include the heteroexpression of PvPGIP2 (Janni et al., 2008) and overexpression of TaPIMP1 and TaPIMP2 (Zhang et al., 2012; Wei et al., 2017) in transgenic wheat lines that enhanced the resistance against B. sorokiniana. However, government regulations on transgenic development are major concerns for researchers.
Additional techniques like genome editing (Zhang et al., 2017) and Eco-Tilling (Ajaz et al., 2021) have been increasingly utilized in wheat, having great potential to contribute to SB resistance breeding in near future.
Conclusion
Spot blotch is a disease of concern in warmer wheat growing areas of South Asia, Latin America and Africa. Most of the commercially grown cultivars are moderately resistant to susceptible and are subjected to significant yield losses under conducive climatic conditions. An integrated disease management strategy involving cultural practices, chemical control, resistant cultivars, etc., is needed to combat the disease. In addition, modern biotechnology brings new tools for the rapid and efficient development of resistant cultivars in wheat.
Author contributions
CR, XH, and PS conceptualized the manuscript. CR drafted the first version. NG and SM collected information and improved the first draft. XH and PS edited and approved the submitted version. All authors contributed to the article and approved the submitted version.
Funding
Funding received from Indian Council of Agricultural Research, India and One CGIAR Plant Health Initiative is acknowledged.
Conflict of interest
The authors declare that the research was conducted in the absence of any commercial or financial relationships that could be construed as a potential conflict of interest.
The reviewer SN declared a past collaboration with author PS to the handling editor at the time of review.
Publisher’s note
All claims expressed in this article are solely those of the authors and do not necessarily represent those of their affiliated organizations, or those of the publisher, the editors and the reviewers. Any product that may be evaluated in this article, or claim that may be made by its manufacturer, is not guaranteed or endorsed by the publisher.
References
Acharya, K., Dutta, A. K., Pradhan, P. (2011). Bipolaris sorokiniana (Sacc.) shoem.: The most destructive wheat fungal pathogen in the warmer areas. Aust. J. Crop Sci. 5, 1064–1071.
Adhikari, T. B., Gurung, S., Hansen, J. M., Jackson, E. W., Bonman, J. M. (2012). Association mapping of quantitative trait loci in spring wheat landraces conferring resistance to bacterial leaf streak and spot blotch. Plant Genome 5, 1–16. doi: 10.3835/plantgenome2011.12.0032
Adlakha, K., Wilcoxson, R., Raychaudhuri, S. (1984). Resistance of wheat to leaf spot caused by. Bipolaris sorokiniana. Plant Dis. 68, 320–321. doi: 10.1094/PD-69-320
Aggarwal, R., Gupta, S., Banerjee, S. A., Singh, V. B. (2011). Development of a SCAR marker for detection of Bipolaris sorokiniana causing spot blotch of wheat. Can. J. Microbiol. 57, 934–942. doi: 10.1139/w11-089
Aggarwal, R., Sharma, S., Singh, K., Gurjar, M. S., Saharan, M. S., Gupta, S., et al. (2019). First draft genome sequence of wheat spot blotch pathogen Bipolaris sorokiniana BS_112 from India, obtained using hybrid assembly. Microbiol. 8, e00308-19. doi: 10.1128/MRA.00308-19
Aggarwal, R., Singh, V. B., Shukla, R., Gurjar, M. S., Gupta, S., Sharma, T. R. (2010). URP-based DNA fingerprinting of Bipolaris sorokiniana isolates causing spot blotch of wheat. J. Phytopathol. 158, 210–216. doi: 10.1111/j.1439-0434.2009.01603.x
Ajaz, S., Benbow, H. R., Christodoulou, T., Uauy, C., Doohan, F. M. (2021). Evaluation of the susceptibility of modern, wild, ancestral, and mutational wheat lines to septoria tritici blotch disease. Plant Pathol. 70, 1123–1137. doi: 10.1111/ppa.13369
Al-Sadi, A. M. (2021). Bipolaris sorokiniana-induced black point, common root rot, and spot blotch diseases of wheat: A review. Front. Cell Infect. Microbiol. 11, 584899. doi: 10.3389/fcimb.2021.584899
Bainsla, N. K., Phuke, R. M., He, X., Gupta, V., Bishnoi, S. K., Sharma, R. K., et al. (2020). Genome-wide association study for spot blotch resistance in afghan wheat germplasm. Plant Pathol. 69, 1161–1171. doi: 10.1111/ppa.13191
Chang, N., Wu, Y. (1998). “Incidence and current management of Spot blotch of wheat in China,” in Helminthosporium blights of wheat: Spot blotch and tan spot. Eds. Duveiller, E., Dubin, H. J., Reeves, J., McNab, A. (El Batan, Mexico: CIMMYT), 119–125.
Chaurasia, S., Chand, R., Joshi, A. K. (2000). Relative dominance of Alternaria triticina pras. et prab. and Bipolaris sorokiniana (Sacc.) shoemaker in different growth stages of wheat (T. aestivum). J. Plant Dis. Protect. 107, 176–181.
Conner, R. (1990). Interrelationship of cultivar reactions to common root rot, black point, and spot blotch in spring wheat. Plant Dis. 74, 224–227. doi: 10.1094/PD-74-0224
Crossa, J., Burgueno, J., Dreisigacker, S., Vargas, M., Herrera-Foessel, S. A., Lillemo, M., et al. (2007). Association analysis of historical bread wheat germplasm using additive genetic covariance of relatives and population structure. Genetics 177, 1889–1913. doi: 10.1534/genetics.107.078659
Domiciano, G. P., Rodrigues, F. A., Vale, F. X. R., Filha, M. S. X., Moreira, W. R., Andrade, C. C. L., et al. (2010). Wheat resistance to spot blotch potentiated by silicon. J. Phytopathol. 158, 334–343. doi: 10.1111/j.1439-0434.2009.01623.x
Duveiller, E. (2002). “Helminthosporium blights of wheat: challenges and strategies for a better disease control,” in Advance of wheat breeding in China: Proceedings of the first national wheat breeding conference (Jinan, Shandong, People Republic of China: China Science and Technology Press), 57–66.
Duveiller, E. (2004). Controlling foliar blights of wheat in the rice-wheat systems of Asia. Plant Dis. 88, 552–556. doi: 10.1094/PDIS.2004.88.5.552
Duveiller, E., Kandel, Y. R., Sharma, R. C., Shrestha, S. M. (2005). Epidemiology of foliar blights (spot blotch and tan spot) of wheat in the plains bordering the Himalayas. Phytopathology 95, 248–256. doi: 10.1094/PHYTO-95-0248
Duveiller, E. M., Sharma, R. C. (2009). Genetic improvement and crop management strategies to minimize yield losses in warm non-traditional wheat growing areas due to spot blotch pathogen Cochliobolus sativus. J. Phytopathol. 157, 521–534. doi: 10.1111/j.1439-0434.2008.01534.x
Duveiller, E., Sharma, R. C. (2012). “Wheat resistance to spot blotch or foliar blight,” in Disease resistance in wheat. Ed. Sharma, I. (Cambridge, MA: CABI, Wallingford, Oxfordshire, UK), 120–135.
Faris, J. D., Zhang, Z., Lu, H., Lu, S., Reddy, L., Cloutier, S., et al. (2010). A unique wheat disease resistance-like gene governs effector-triggered susceptibility to necrotrophic pathogens. Proc. Natl. Acad. Sci. U. S. A. 107, 13544–13549. doi: 10.1073/pnas.1004090107
Friesen, T. L., Holmes, D. J., Bowden, R. L., Faris, J. D. (2018). ToxA is present in the U.S. Bipolaris sorokiniana population and is a significant virulence factor on wheat harboring Tsn1. Plant Dis. 102, 2446–2452. doi: 10.1094/PDIS-03-18-0521-RE
Friesen, T. L., Stukenbrock, E. H., Liu, Z., Meinhardt, S., Ling, H., Faris, J. D., et al. (2006). Emergence of a new disease as a result of interspecific virulence gene transfer. Nat. Genet. 38, 953–956. doi: 10.1038/ng1839
Gahtyari, N. C., Roy, C., He, X., Roy, K. K., Reza, M. M. A., Hakim, M. A., et al. (2021). Identification of QTLs for spot blotch resistance in two bi-parental mapping populations of wheat. Plants 10, 973. doi: 10.3390/plants10050973
Gupta, P. K., Chand, R., Vasistha, N. K., Pandey, S. P., Kumar, U., Mishra, V. K., et al. (2017). Spot blotch disease of wheat: the current status of research on genetics and breeding. Plant Pathol. 67, 508–531. doi: 10.1111/ppa.12781
Gurung, S., Mamidi, S., Bonman, J. M., Xiong, M., Brown-Guedira, G., Adhikari, T. B. (2014). Genome-wide association study reveals novel quantitative trait loci associated with resistance to multiple leaf spot diseases of spring wheat. PloS One 9, e108179. doi: 10.1371/journal.pone.0108179
Hasan, U. W., Roy, C., Chattopadhyay, T., Ranjan, R. D., De, N. (2021). Effects of heat and drought stress on yield and physiological traits in wheat (Triticum aestivum l.). J. Crop Weed. 17 (1), 203–210. doi: 10.22271/09746315.2021.v17.i1.1424
He, X., Dreisigacker, S., Sansaloni, C., Duveiller, E., Singh, R. P., Singh, P. K. (2020). QTL mapping for spot blotch resistance in two bi-parental mapping populations of bread wheat. Phytopathology 110, 1980–1987. doi: 10.1094/PHYTO-05-20-0197-R
He, X., Gahtyari, N. C., Roy, C., Dababat, A. A., Brar, G. S., Singh, P. K. (2022). “Globally important non-rust diseases of wheat,” in Wheat improvement. Eds. Reynolds, M. P., Braun, H. J. (Cham: Springer), 143–158. doi: 10.1007/978-3-030-90673-3_9
Helguera, M., Khan, I. A., Kolmer, J., Lijavetzky, D., Zhong-qi, L., Dubcovsky, J. (2003). PCR assays for the Lr37-Yr17-Sr38 cluster of rust resistance genes and their use to develop isogenic hard red spring wheat lines. Crop Sci. 43, 1839–1847. doi: 10.2135/cropsci2003.1839
Ibeagha, A. E., Hückelhoven, R., Schäfer, P., Singh, D. P., Kogel, K. H. (2005). Model wheat genotypes as tools to uncover effective defense mechanisms against the hemibiotrophic fungus Bipolaris sorokiniana. Phytopathology 95, 528–532. doi: 10.1094/PHYTO-95-0528
Jahani, M. (2005). Characterization of toxin from bipolaris sorokiniana and development of molecular marker for identification of the pathogen (New Delhi: Indian Agricultural Research Institute). dissertation/Ph D thesis.
Jahani, M., Aggarwal, R., Gupta, S., Sharma, S., Dureja, P. (2014). Purification and characterization of a novel toxin from Bipolaris sorokiniana, causing spot blotch of wheat and analysis of variability in the pathogen. Cereal Res. Commun. 42, 252–261. doi: 10.1556/CRC.2013.0053
Jahier, A., Tanguy, D., Rivoal, K., Bariana, H. S. (2001). The Aegilops ventricosa segment on chromosome 2AS of the wheat cultivar ‘VPM1’ carries the cereal cyst nematode resistance gene Cre5. Plant Breed. 120, 125–128. doi: 10.1046/j.1439-0523.2001.00585.x
Jamil, M., Ali, A., Gul, A., Ghafoor, A., Ibrahim, A. M. H., Mujeeb-Kazi, A. (2018). Genome-wide association studies for spot blotch (Cochliobolus sativus) resistance in bread wheat using genotyping-by-sequencing. Phytopathology 108, 1307–1314.
Janni, M., Sella, L., Favaron, F., Blechl, A. E., De Lorenzo, G., D’Ovidio, R. (2008). The expression of a bean PGIP in transgenic wheat confers increased resistance to the fungal pathogen Bipolaris sorokiniana. Mol. Plant Microbe In. 21, 171–177. doi: 10.1094/MPMI-21-2-0171
Jansson, H. B., Akesson, H. (2003). Extracellular matrix, esterase and the phytotoxin prehelminthosporol in infection of barley leaves by Bipolaris sorokiniana. Eur. J. Plant Pathol. 109, 599–605. doi: 10.1023/A:1024773531256
Joshi, A. K., Chand, R. (2002). Variation and inheritance of leaf angle, and its association with spot blotch (Bipolaris sorokiniana) severity in wheat (Triticum aestivum). Euphytica 124, 283–291. doi: 10.1023/A:1015773404694
Joshi, A. K., Chand, R., Arun, B. (2002). Relationship of plant height and days to maturity with resistance to spot blotch in wheat. Euphytica 123, 221–228. doi: 10.1023/A:1014922416058
Joshi, A. K., Chand, R., Arun, B., Singh, R. P., Ortiz, R. (2007a). Breeding crops for reduced-tillage managementin the intensive, rice–wheat systems of south Asia. Euphytica 153, 135–151. doi: 10.1007/s10681-006-9249-6
Joshi, A. K., Chand, R., Kumar, S., Singh, R. P. (2004a). Leaf tip necrosis: A phenotypic marker associated with resistance to spot blotch disease in wheat. Crop Sci. 44, 792–796. doi: 10.2135/cropsci2004.7920
Joshi, A. K., Kumar, S., Chand, R., Ortiz-Ferrara, G. (2004b). Inheritance of resistance to spot blotch caused by Bipolaris sorokiniana in spring wheat. Plant Breed. 123, 213–219. doi: 10.1111/j.1439-0523.2004.00954.x
Joshi, A. K., Kumari, M., Singh, V. P., Reddy, C. M., Kumar, S., Rane, J., et al. (2007b). Stay green trait: Variation, inheritance and its association with spot blotch resistance in spring wheat (Triticum aestivum l.). Euphytica 153, 59–71. doi: 10.1007/s10681-006-9235-z
Joshi, A. K., Mishra, B., Chatrath, R., Ferrara, G. O., Singh, R. P. (2007c). Wheat improvement in India: present status, emerging challenges and future prospects. Euphytica 157, 431–446. doi: 10.1007/s10681-007-9385-7
Joshi, A. K., Ortiz-Ferrara, G., Crossa, J., Singh, G., Sharma, R. C., Chand, R., et al. (2007d). Combining superior agronomic performance and terminal heat tolerance with resistance to spot blotch (Bipolaris sorokiniana) of wheat in the warm humid gangetic plains of south Asia. Field Crop Res. 103, 53–61. doi: 10.1016/j.fcr.2007.04.010
Juliana, P., He, X., Poland, J., Roy, K. K., Malaker, P. K., Mishra, V. K., et al. (2022a). Genomic selection for spot blotch in bread wheat breeding panels, full−sibs and half−sibs and index−based selection for spot blotch, heading and plant height. Theor. Appl. Genet. 135, 1965–1983. doi: 10.1007/s00122-022-04087-y
Juliana, P., He, X., Poland, J., Shrestha, S., Joshi, A. K., Huerta-Espino, J., et al. (2022b). Genome-wide association mapping indicates quantitative genetic control of spot blotch resistance in bread wheat and the favorable effects of some spot blotch loci on grain yield. Front. Plant Sci. 13. doi: 10.3389/fpls.2022.835095
Kemp, G., Bergmann, C. W., Clay, R., van der Westhuizen, A., Pretorius, Z. A. (2003). Isolation of a polygalacturonase-inhibiting protein (PGIP) from wheat. Mol. Plant Microbe Interact. 16, 955–961. doi: 10.1094/MPMI.2003.16.11.955
Krattinger, S. G., Keller, B. (2016). Molecular genetics and evolution of disease resistance in cereals. New Phytol. 212, 320e332. doi: 10.1111/nph.14097
Krattinger, S. G., Lagudah, E. S., Spielmeyer, W., Singh, R. P., Huerta-Espino, J., McFadden, H., et al. (2009). A putative ABC transporter confers durable resistance to multiple fungal pathogens in wheat. Science 323, 1360–1363. doi: 10.1126/science.1166453
Kumar, S., Archak, S., Tyagi, R. K., Kumar, J., Vk, V., Jacob, S. R., et al. (2016). Evaluation of 19,460 wheat accessions conserved in the Indian national genebank to identify new sources of resistance to rust and spot blotch diseases. PloS One 11, e0167702. doi: 10.1371/journal.pone.0167702
Kumar, U., Joshi, A. K., Kumar, S., Chand, R., Röder, M. S. (2009). Mapping of resistance to spot blotch disease caused by Bipolaris sorokiniana in spring wheat. Theor. Appl. Genet. 118, 783–792. doi: 10.1007/s00122-008-0938-5
Kumar, U., Joshi, A. K., Kumar, S., Chand, R., Röder, M. S. (2010). Quantitative trait loci for resistance to spot blotch caused by Bipolaris sorokiniana in wheat (T. aestivum l.) lines ‘Ning 8201’ and ‘Chirya 3’. Mol. Breed. 26, 477–491. doi: 10.1007/s11032-009-9388-2
Kumar, U., Kumar, S., Prasad, R., Röder, M. S., Kumar, S., Chand, R., et al. (2019). Genetic gain on resistance to spot blotch of wheat by developing lines with near immunity. Crop Breed. Genet. Genom. 1, e190017. doi: 10.20900/cbgg20190017
Kumar, S., Pradhan, A. K., Kumar, U., Dhillon, G. S., Kaur, S., Budhlakoti, N., et al. (2022). Validation of novel spot blotch disease resistance alleles identified in unexplored wheat (Triticum aestivum l.) germplasm lines through KASP markers. BMC Plant Biol. 22, 618. doi: 10.1186/s12870-022-04013-w
Kumar, S., Röder, M. S., Tripathi, S. B., Kumar, S., Chand, R., Joshi, A. K., et al. (2015). Mendelization and fine mapping of a bread wheat spot blotch disease resistance QTL. Mol. Breed. 35, 218. doi: 10.1007/s11032-015-0411-5
Kumar, J., Schäfer, P., Hückelhoven, R., Langen, G., Baltruschat, H., Stein, E., et al. (2002). Bipolaris sorokiniana, a cereal pathogen of global concern: cytological and molecular approaches towards better control. Mol. Plant Pathol. 3, 185–195. doi: 10.1046/j.1364-3703.2002.00120.x
Leng, Y., Wu, C., Liu, Z., Friesen, T. L., Rasmussen, J. B., Zhong, S. (2011). RNA-Mediated gene silencing in the cereal fungal pathogen Cochliobolus sativus. Mol. Plant Pathol. 12, 289–298. doi: 10.1111/j.1364-3703.2010.00666.x
Lillemo, M., Joshi, A. K., Prasad, R., Chand, R., Singh, R. P. (2013). QTL for spot blotch resistance in bread wheat line Saar co-locate to the biotrophic disease resistance loci Lr34 and Lr46. Theor. Appl. Genet. 126, 711–719. doi: 10.1007/s00122-012-2012-6
Liu, S., Yeh, C. T., Tang, H. M., Nettleton, D., Schnable, P. S. (2012). Gene mapping via bulked segregant RNA-seq (BSR-seq). PloS One 7, e36406. doi: 10.1371/JOURNAL.PONE.0036406
Lozano-Ramirez, N., Dreisigacker, S., Sansaloni, C. P., He, X., Sandoval-Islas, J. S., Pérez-Rodríguez, P., et al. (2022). Genome-wide association study for spot blotch resistance in synthetic hexaploid wheat. Genes 13, 1387. doi: 10.3390/genes13081387
Lu, P., Liang, Y., Li, D., Wang, Z., Li, W., Wang, G., et al. (2016). Fine genetic mapping of spot blotch resistance gene Sb3 in wheat (Triticum aestivum). Theor. Appl. Genet. 129, 577–589. doi: 10.1007/s00122-015-2649-z
Manamgoda, D. S., Rossman, A. Y., Castlebury, L. A., Crous, P. W., Madrid, H., Chukeatirote, E., et al. (2014). The genus bipolaris. Stud. Mycol. 79, 221–288. doi: 10.1016/j.simyco.2014.10.002
Mann, M. B., Minotto, E., Feltrin, T., Milagre, L. P., Spadari, C., van der Sand, S. T. (2014). Genetic diversity among monoconidial and polyconidial isolates of Bipolaris sorokiniana. Curr. Microbiol. 69, 874–879. doi: 10.1007/s00284-014-0667-8
McDonald, M. C., Ahren, D., Simpfendorfer, S., Milgate, A., Solomon, P. S. (2017). The discovery of the virulence gene ToxA in the wheat and barley pathogen Bipolaris sorokiniana. Mol. Plant Pathol. 19, 432–439. doi: 10.1111/mpp.12535
McDonald, A. J., Singh, B., Keil, A., Srivastava, A., Craufurd, P., Kishore, A., et al. (2022). Time management governs climate resilience and productivity in the coupled rice–wheat cropping systems of eastern India. Nat. Food. 3, 542–551. doi: 10.1038/s43016-022-00549-0
Mehta, Y., Riede, C., Campos, L., Kohli, M. (1992). Integrated management of major wheat diseases in Brazil: an example for the southern cone region of Latin America. Crop Prot. 11, 517–524. doi: 10.1016/0261-2194(92)90168-5
Mishra, S., Singh, B. R., Singh, A., Keswani, C., Naqvi, A. H., Singh, H. B. (2014). Biofabricated silver nanoparticles act as a strong fungicide against Bipolaris sorokiniana causing spot blotch disease in wheat. PloS One 9, e97881. doi: 10.1371/journal.pone.0097881
Mujeeb-Kazi, A., Gul, A., Ahmad, I., Farooq, M., Rizwan, S., Bux, H., et al. (2007). Aegilops tauschii, as a spot blotch (Cochliobolus sativus) resistance source for bread wheat improvement. Pak. J. Bot. 39, 1207–1216.
Mujeeb-Kazi, A., Villareal, R., Gilchrist, L., Rajaram, S. (1996). Registration of five wheat germplasm lines resistant to helminthosporium leaf blight. Crop Sci. 36, 216–217. doi: 10.2135/cropsci1996.0011183X003600010054x
Murray, G. M., Brennan, J. P. (2009). The current and potential costs from diseases of wheat in Australia Vol. 69 (Barton: Grain Research and Development Corporation, Australia).
Narendra, M. C., Roy, C., Kumar, S., Virk, P., De, N. (2021). Effect of terminal heat stress on physiological traits, grain zinc and iron content in wheat (Triticum aestivum l.). Czech J. Genet. Plant Breed. 57, 43–50. doi: 10.17221/63/2020-CJGPB
Navathe, S., Chand, R., Mishra, V. K., Pandey, S. P., Kumar, U., Joshi, A. K. (2019a). Management of spot blotch and heat stress in spring wheat through azoxystrobin-mediated redox balance. Agr. Res. 9 (2), 169–178. doi: 10.1007/s40003-019-00417-7
Navathe, S., Yadav, P. S., Chand, R., Mishra, V. K., Vasistha, N. K., Meher, P. K., et al. (2019b). ToxA-Tsn1 interaction for spot blotch susceptibility in Indian wheat: An example of inverse gene-for-gene relationship. Plant Dis. 104, 71–81. doi: 10.1094/PDIS-05-19-1066-RE
Neupane, R. B., Sharma, R. C., Duveiller, E., Ortiz-Ferrara, G., Ojha, B. R., Rosyara, U. R., et al. (2007). Major gene controls of field resistance to spot blotch in wheat genotypes ‘Milan/Shanghai 7’ and ‘Chirya.3’. Plant Dis. 91, 692–697. doi: 10.1094/PDIS-91-6-0692
Neupane, A. C., Sharma, R. C., Duveiller, E., Shrestha, S. M. (2010). Sources of Cochliobolus sativus inoculum causing spot blotch under warm wheat growing conditions in south Asia. Cereal Res. Commun. 38 (4), 541–549. doi: 10.1556/CRC.38.2010.4.11
Pandey, S. P., Kumar, S., Kumar, U., Chand, R., Joshi, A. K. (2005). Sources of inoculum and reappearance of spot blotch of wheat in rice-wheat cropping systems in eastern India. Eur. J. Plant Pathol. 111, 47–55. doi: 10.1007/s10658-004-2404-9
Qi, T., Guo, J., Peng, H., Liu, P., Kang, Z., Guo, J. (2019). Host-induced gene silencing: A powerful strategy to control diseases of wheat and barley. Int. J. Mol. Sci. 20, 206. doi: 10.3390/ijms20010206
Reis, E. M. (1991). Integrated disease management: the changing concept of controlling head blight and spot blotch. In: Saunders, DA, Hettel, GP eds. Wheat in Heat Stressed Environments: Irrigated, Dry Areas and Rice Wheat Systems Mexico, DF: CIMMYT, 165–170.
Ridley, B. L., O’Neill, M. A., Mohnen, D. (2001). Pectins: Structure, biosynthesis, and oligogalacturonide-related signaling. Phytochemistry 57, 929–967. doi: 10.1016/S0031-9422(01)00113-3
Roy, C., Gahtyari, N. C., He, X., Mishra, V. K., Chand, R., Joshi, A. K., et al. (2021a). Dissecting quantitative trait loci for spot blotch resistance in south Asia using two wheat recombinant inbred line populations. Front. Plant Sci. 12, 641324. doi: 10.3389/fpls.2021.641324
Roy, C., Juliana, P., Kabir, M. R., Roy, K. K., Gahtyari, N. C., Marza, F., et al. (2021b). New genotypes and genomic regions for resistance to wheat blast in south Asian germplasm. Plants 10, 2693. doi: 10.3390/plants10122693
Saari, E. (1998). Leaf blight disease and associated soil-borne fungal pathogens of wheat in South and South East Asia. In: Duveiller, E., Dubin, H. J., Reeves, J., McNab, A. eds. Helminthosporium Blights of wheat: spot blotch and tan spot Mexico, DF: CIMMYT, 37–51.
Sautua, F. J., Carmona, M. A. (2021). Detection and characterization of QoI resistance in Pyrenophora tritici-repentis populations causing tan spot of wheat in Argentina. Plant Pathol. 70 (9), 2125–2136. doi: 10.1111/ppa.13436
Saxesena, R. R., Mishra, V. K., Chand, R., Kumar, U., Chowdhury, A. K., Bhati., J., et al. (2022). SNP discovery using BSR-seq approach for spot blotch resistance in wheat (Triticum aestivum l.), an essential crop for food security. Front. Genet. 13. doi: 10.3389/fgene.2022.859676
Sharma, R. C., Dubin, H. J. (1996). Effect of wheat cultivar mixtures on spot blotch (Bipolaris sorokiniana) and grain yield. Field Crop Res. 48, 95–101. doi: 10.1016/S0378-4290(96)01031-3
Sharma, R. C., Duveiller, E. (2004). Effect of helminthosporium leaf blight on performance of timely and late-seeded wheat under optimal and stressed levels of soil fertility and moisture. Field Crop Res. 89, 205–218. doi: 10.1016/j.fcr.2004.02.002
Sharma, R. C., Duveiller, E. (2006). Spot blotch continues to cause substantial grain yield reductions under resource-limited farming conditions. J. Phytopathol. 154, 482–488. doi: 10.1111/j.1439-0434.2006.01134.x
Sharma, R. C., Duveiller, E. (2007). Advancement toward new spot blotch resistant wheats in south Asia. Crop Sci. 47, 961–968. doi: 10.2135/cropsci2006.03.0201
Sharma, R. C., Duveiller, E., Ahmed, F., Arun, B., Bhandari, D., Bhatta, M. R., et al. (2004b). Helminthosporium leaf blight resistance and agronomic performance of wheat genotypes across warm regions of south Asia. Plant Breed. 123, 520–524. doi: 10.1111/j.1439-0523.2004.01006.x
Sharma, R., Duveiller, E., Gyawali, S., Shrestha, S., Chaudhary, N., Bhatta, M. (2004a). Resistance to helminthosporium leaf blight and agronomic performance of spring wheat genotypes of diverse origins. Euphytica 139, 33–44. doi: 10.1007/s10681-004-2292-2
Sharma, R. C., Duveiller, E., Ortiz-Ferrara, G. (2007). Progress and challenge towards reducing wheat spot blotch threat in the Eastern gangetic plains of south Asia: Is climate change already taking its toll? Field Crop Res. 103, 109–118. doi: 10.1016/j.fcr.2007.05.004
Sharma, P., Duveiller, E., Sharma, R. C. (2006a). Effect of mineral nutrients on spot blotch severity in wheat, and associated increases in grain yield. Field Crop Res. 95, 426–430. doi: 10.1016/j.fcr.2005.04.015
Sharma, P., Mishra, S., Singroha, G., Kumar, R. S., Singh, S. K., Singh, G. P. (2022). Phylogeographic diversity analysis of Bipolaris sorokiniana (Sacc.) shoemaker causing spot blotch disease in wheat and barley. Genes 13, 2206. doi: 10.3390/genes13122206
Sharma, R. C., Pandey-Chhetri, B., Duveiller, E. (2006b). Heritability estimates of spot blotch resistance and its association with other traits in spring wheat crosses. Euphytica 147, 317–327. doi: 10.1007/s10681-005-9018-y
Singh, P. K., Gahtyari, N. C., Roy, C., Roy, K. K., He, X., Tembo, B., et al. (2021). Wheat blast: A disease spreading by intercontinental jumps and its management strategies. Front. Plant Sci. 12. doi: 10.3389/fpls.2021.710707
Singh, P. K., He, X., Sansaloni, C. P., Juliana, P., Dreisigacker, S., Duveiller, E., et al. (2018). Resistance to spot blotch in two mapping populations of common wheat is controlled by multiple QTL of minor effects. Int. J. Mol. Sci. 19, 4054. doi: 10.3390/ijms19124054
Singh, D. P., Kumar, A., Solanki, I. S., Singh, S. P., Verma, J., Mahapatra, S., et al. (2014). Management of spot blotch of wheat caused by Bipolaris sorokiniana in wheat using fungicides. Indian Phytopath. 67 (3), 308–310.
Singh, V., Singh, G., Chaudhury, A., Ojha, A., Tyagi, B. S., Chowdhary, A. K., et al. (2016). Phenotyping at hot spots and tagging of QTLs conferring spot blotch resistance in bread wheat. Mol. Bio Rep. 43, 1293–1303. doi: 10.1007/s11033-016-4066-z
Singh, D., Wang, X., Kumar, U., Gao, L., Noor, M., Imtiaz, M., et al. (2019). High-throughput phenotyping enabled genetic dissection of crop lodging in wheat. Front. Plant Sci. 10, 394. doi: 10.3389/fpls.2019.00394
Singh, P. K., Zhang, Y., He, X., Singh, R. P., Chand, R., Mishra, V. K., et al. (2015). Development and characterization of the 4th CSISA-spot blotch nursery of bread wheat. Eur. J. Plant Pathol. 143, 595–605. doi: 10.1007/s10658-015-0712-x
Sultana, S, Adhikary, SK, Islam, MM, Rahman, SMM. (2018). Evaluation of pathogenic variability based on leaf blotch disease development components of Bipolaris sorokiniana in Triticum aestivum and agroclimatic origin. Plant Pathol J. 34, 93–103.
Sun, X. F., Zhang, D. X., Gong, G. S., Qi, X. B., Ye, K. H., Zhou, Y., et al. (2015). Spot blotch on volunteer wheat plants in sichuan, China. J. Plant Path. 97, 173–176.
Tomar, V., Singh, D., Dhillon, G. S., Singh, R. P., Poland, J., Joshi, A. K., et al. (2021). New QTLs for spot blotch disease resistance in wheat (Triticum aestivum l.) using genome-wide association mapping. Front. Genet. 11. doi: 10.3389/fgene.2020.613217
Valjavec-Gratian, M., Steffenson, B. J. (1997). Pathotypes of Cochliobolus sativus in barley in north Dakota. Plant Dis. 81, 1275–1278. doi: 10.1094/PDIS.1997.81.11.1275
van Ginkel, Rajaram, S. (1998). “Breeding for resistance to spot blotch in wheat: Global perspective,” in Helminthosporium blight of wheat: Spot blotch and tan spot. Eds. Duveiller, E., Dubin, H. J., Reeves, J., McNab, A. (Mexico: DF: CIMMYT), 376.
Vasistha, N. K., Balasubramaniam, A., Mishra, V. K., Chand, R., Srinivasa, J., Yadav, P. S., et al. (2015). Enhancing spot blotch resistance in wheat by marker-aided backcross breeding. Euphytica 207, 119–133. doi: 10.1007/s10681-015-1548-3
Velazquez-Cruz, C. (1994). Genetica de la resistencia a bipolaris sorokiniana en trigos harineros (Mexico: Colegio de Post-Graduados, Montecillo). dissertation/MSc Thesis.
Villareal, R. L., Mujeeb-Kazi, A., Gilchrist, L. I., Del Toro, E. (1995). Yield loss to spot blotch in spring bread wheat in warm nontraditional wheat production areas. Plant Dis. 79, 893–897. doi: 10.1094/PD-79-0893
Wei, X., Shan, T., Hong, Y., Xu, H., Liu, X., Zhang, Z. (2017). TaPIMP2, a pathogen-induced MYB protein in wheat, contributes to host resistance to common root rot caused by Bipolaris sorokiniana. Sci. Rep. 7, 1754. doi: 10.1038/s41598-017-01918-7
Wu, L., He, X., Lozano, N., Zhang, X., Singh, P. K. (2020). ToxA, a significant virulence factor involved in wheat spot blotch disease, exists in the Mexican population of Bipolaris sorokiniana. Trop. Plant Path. 46, 201–206. doi: 10.1007/s40858-020-00391-4
Zhang, Y., Bai, Y., Wu, G., Zou, S., Chen, Y., Gao, C., et al. (2017). Simultaneous modification of three homoeologs of TaEDR1 by genome editing enhances powdery mildew resistance in wheat. Plant J. 91, 714–724. doi: 10.1111/tpj.13599
Zhang, P., Guo, G., Wu, Q., Chen, Y., Xie, J., Lu, P., et al. (2020). Identification and fine mapping of spot blotch (Bipolaris sorokiniana) resistance gene Sb4 in wheat. Theor. Appl. Genet. 133, 2451–2459. doi: 10.1007/s00122-020-03610-3
Zhang, Z., Liu, X., Wang, X., Zhou, M., Zhou, X., Ye, X., et al. (2012). An R2R3 MYB transcription factor in wheat, TaPIMP1, mediates host resistance to Bipolaris sorokiniana and drought stresses through regulation of defense and stress-related genes. New Phytol. 196, 1155–1170. doi: 10.1111/j.1469-8137.2012.04353.x
Zhong, S., Steffenson, B. J. (2001). Virulence and molecular diversity in Cochliobolus sativus. Phytopathol. 91, 469–476. doi: 10.1094/PHYTO.2001.91.5.469
Keywords: Bipolaris sorokiniana, disease management, resistance breeding, spot blotch, wheat
Citation: Roy C, He X, Gahtyari NC, Mahapatra S and Singh PK (2023) Managing spot blotch disease in wheat: Conventional to molecular aspects. Front. Plant Sci. 14:1098648. doi: 10.3389/fpls.2023.1098648
Received: 15 November 2022; Accepted: 30 January 2023;
Published: 21 February 2023.
Edited by:
Kostya Kanyuka, National Institute of Agricultural Botany (NIAB), United KingdomReviewed by:
Amira M. I. Mourad, Assiut University, EgyptSudhir Navathe, Agharkar Research Institute, India
Xuening Wei, Institute of Crop Sciences (CAAS), China
Copyright © 2023 Roy, He, Gahtyari, Mahapatra and Singh. This is an open-access article distributed under the terms of the Creative Commons Attribution License (CC BY). The use, distribution or reproduction in other forums is permitted, provided the original author(s) and the copyright owner(s) are credited and that the original publication in this journal is cited, in accordance with accepted academic practice. No use, distribution or reproduction is permitted which does not comply with these terms.
*Correspondence: Pawan K. Singh, pk.singh@cgiar.org