- 1Department of Environmental Sciences, Faculty of Natural Resources, Yazd University, Yazd, Iran
- 2Department of Soil Science, University of Tehran, Tehran, Iran
Dust causes adverse effects on the physiological and biochemical characteristics of plants and limits their use in the development of the green belt. Air Pollution Tolerance Index (APTI) is an important tool to screen out plants, based on their tolerance or sensitivity level to different air pollutants. The aim of this study was to investigate the effect of two plant growth-promoting bacterial strains (Zhihengliuella halotolerans SB and Bacillus pumilus HR) and their combination as a biological solution on APTI of three desert plant species of Seidlitzia rosmarinus, Haloxylon aphyllum and Nitraria schoberi under dust stress (0 and 1.5 g m-2 30 days-1). Dust caused a significant decrease of 21% and 19%, respectively, in the total chlorophyll of N. schoberi and S. rosmarinus, an 8% decrease in leaf relative water content, a 7% decrease in the APTI of N. schoberi, and a decrease of 26 and 17% in protein content of H. aphyllum and N. schoberi, respectively. However, Z. halotolerans SB increased the amount of total chlorophyll in H. aphyllum and S. rosmarinus by 236% and 21%, respectively, and the amount of ascorbic acid by 75% and 67% in H. aphyllum and N. schoberi, respectively. B. pumilus HR also increased the leaf relative water content in H. aphyllum and N. schoberi by 10% and 15%, respectively. The inoculation with B. pumilus HR, Z. halotolerans SB and the combination of these two isolates decreased the activity of peroxidase by 70%, 51%, and 36%, respectively, in N. schoberi, and 62%, 89%, and 25% in S. rosmarinus, respectively. These bacterial strains also increased the concentration of protein in all three desert plants. Under dust stress, H. aphyllum had a higher APTI than the other two species. Z. halotolerans SB, which had been isolated from S. rosmarinus, was more effective than B. pumilus HR in alleviating the effects of dust stress on this plant. Therefore, it was concluded that plant growth-promoting rhizobacteria can be effective at improving the mechanisms of plant tolerance to air pollution in the green belt.
1 Introduction
Dust is a collection of fine particles of natural or industrial origin that is considered as one of the most widespread air pollutants. In recent years, the presence of dust particles suspended in the air has become a major environmental problem (Abbasi et al., 2019). Although dust pollution has always been a natural phenomenon, its amount sometimes reaches an unacceptable level, affecting all aspects of human life. In recent years, human activities have intensified the phenomenon (Middleton, 2019).
Plants can naturally absorb fine dust particles from the atmosphere and act as an air cleaner filter. The development of green plants around the cities is one of the most appropriate biological methods to reduce air pollution in these areas (Alotaibi et al., 2020). Since urban green belts can largely prevent the entry of fine dust and pollution into cities, their creation has gained special importance (Hamraz et al., 2014). Nowadays the use of plants resistance to environmental stress, such as dust, in creating green spaces is very important and a priority. The importance of these plants can be understood by the fact that they not only purify the air by biofiltering pollutants through absorption, impingement and adsorption (Escobedo et al., 2008), but also have positive effects on the soil and water quality of the area, in addition to adding aesthetic value to it (Pradhan et al., 2016). Leaves are prominent receivers of air pollutants, but show variable behavior towards different types of air pollutants (Sahu and Sahu, 2015). A few plants show tolerance to a particular air pollutant, while others have high mortality rates and are therefore sensitive (Nadgórska–Socha et al., 2017). Tolerant species are suitable for planting, while sensitive species can be used as both bioindicators and biomonitors to provide quantitative and qualitative information, respectively, about the surrounding environment (Priyanka and Dibyendu, 2009; Trivedy and Goel, 2010; Kwon et al., 2020).
It has been reported that the gaseous and particulate pollutants in the atmosphere have direct effect on many morphological, physiological and biochemical characteristics of plants (Steubing et al., 1989) such as chlorophyll a and b, photosynthesis, the metabolism of proline, the activity of antioxidant enzymes such as catalase and peroxidase, leaf relative water content, ascorbic acid concentration, protein concentration, soluble sugars and pH (Najafi Zilaie et al., 2022a; Najafi Zilaie et al., 2022b; Zilaie et al., 2022). Although the tolerance of plants to these pollutants has been studied according to different aspects such as peroxidative activity (Xu et al., 2014), leaf conductance, glutathione concentration (Pasqualini et al., 2001; Hoque et al., 2007), membrane permeability (Farooq and Beg, 1980) and other biochemical parameters, contradictory results have been obtained when the parameters are studied individually even for the same species. Accordingly, Singh and Rao (1983) developed a formula through the aggregation of four biochemical parameters (relative water content, ascorbic acid, chlorophyll content, and leaf extract pH), known as Air Pollution Tolerance Index (APTI). The APTI has been praised by a wide range of researchers for its combination of different parameters that show more reliable results than any analysis based on a single parameter (Neel et al., 2018; Bellini and De Tullio, 2019; Manjunath and Reddy, 2019).
Plant growth-promoting rhizobacteria (PGPR) have been shown to improve the plant tolerance to environmental stresses by various mechanisms (Etesami and Maheshwari, 2018). These bacteria have multiple plant growth-promoting (PGP) traits under different stressful conditions (Etesami and Maheshwari, 2018; Etesami and Glick, 2020). In the study of Tiepo et al. (2018), the use of PGPR (Azospirillum brasilense and Bacillus sp.) improved the physiological traits of Cariniana estrellensis seedlings such as relative water content, water potential, intracellular CO2 concentration and electrolyte leakage under water deficit stress conditions. In another study, the inoculation of mesquite transplants with Azospirillum brasilense showed a positive effect on the morphological and physiological parameters of the plant (e.g., biomass, root system, and chlorophyll a and b) under drought stress conditions. Hajiabadi et al. (2021) also isolated two bacterial isolates of B. pumilus and Z. halotolerans, from the rhizosphere of Halostachys belangeriana and S. rosmarinus, respectively, and measured their PGP traits. The results of these researchers indicated that the mentioned bacterial isolates have several PGP traits such as the ability to solubilize phosphates, produce 1-aminocyclopropane-1-carboxylic acid (ACC) deaminase enzyme, auxin, and siderophore and fix N2. The inoculation of these two bacterial isolates also improved the tolerance of wheat to salinity and increased its growth and yield. The positive effect of B. pumilus (Ansari et al., 2019) and Z. halotolerans (Jha et al., 2012) on plant growth has also been reported in other studies. In the present study, the effect of single inoculation and co-inoculation of these two bacterial isolates Bacillus pumilus HR, isolated from Halostachys belangeriana, and Z. halotolerans SB, isolated from S. rosmarinus, on the APTI of three desert plant species of S. rosmarinus, H. aphyllum and N. schoberi was investigated under dust stress. The research questions of this study were: (i) Would these bacterial strains improve APTI of three desert plant species of S. rosmarinus, H. aphyllum and N. schoberi; (ii) Would the bacterial strain isolated from the rhizosphere of S. rosmarinus have a greater effect on the tolerance of this plant against pollution stress compared to the bacterial strain isolated from another desert plant; and (iii) Would the combination of these two bacterial strains have a greater effect on plant growth compared to their single inoculation. To our knowledge, this is the first study to investigate the effect of PGPR on APTI of plants. Therefore, the results of this study are promising and can be used for better management of planting desert plants of the green belt around cities in dry areas.
2 Materials and methods
2.1 Rhizobacterial strains and inoculum preparation
In this study, two rhizobacterial strains, B. pumilus HR (accession number: MW295357), previously isolated from the halophyte Halostachys belangeriana, and Z. halotolerans SB (accession number: MW295355), previously isolated from the halophyte S. rosmarinus, were used (Hajiabadi et al., 2021). These bacterial strains were characterized in our previous study (Hajiabadi et al., 2021). These strains were also able to colonize the roots of S. rosmarinus (Zilaie et al., 2022), H. aphyllum (Najafi Zilaie et al., 2022b), and N. schoberi (Najafi Zilaie et al., 2022a) under salinity and dust stress conditions. For preparing the bacterial inoculum, two bacterial strains were grown in a nutrient broth (NB) culture medium separately until they both reached the late exponential-phase (3 × 108 cells mL−1) at 28 ± 2°C. The bacterial cells were then washed twice and re-suspended in sterile distilled water. Since the two bacterial strains were supposed to be inoculated into these three plants together, we also showed that the two strains did not have any antagonistic effects on each other’s growth by an in vitro assay (Najafi Zilaie et al., 2022b).
2.2 Treatments and experimental design
To evaluate the effect of two strains HR and SB on APTI of three desert plant species (S. rosmarinus, H. aphyllum and N. schoberi) under dust stress, a plant growth assay was carried out in a completely randomized design with factorial arrangement (4 × 2 × 3) with three replications in a research greenhouse located at Department of Environmental Engineering, Yazd-University, Yazd, Iran. The experimental treatments included: (a) rhizobacterial strain factor at four levels: (i) non-inoculated seedlings (control), (ii) seedlings inoculated with B. pumilus HR, (iii) seedlings inoculated with Z. halotolerans SB, and (iv) seedlings co-inoculated with the HR and SB strains; (b) dust stress factor at 2 levels: 0 and 1.5 g m-2 30 days-1; and (c) plant species factor (S. rosmarinus, H. aphyllum and N. schoberi).
The non–sterile soil (3 kg) used in plastic pots (40 cm × 25 cm) with drainage holes was air-dried at room temperature and passed through a 4–mm sieve. Soil properties are as follows: pH, 7.61; soil texture, sandy clay loam (28.2% clay, 12% silt, and 59.2% sand); Na, 2.15 meq kg−1; organic matter (OM), 1.8 g kg−1; , 48 meq kg−1; electrical conductivity (EC), 2.0 dS m−1; total nitrogen, 0.20 g kg−1; Ca, 1.8 meq kg−1; available phosphorus, 15.0 mg kg−1; NH4OAc–K, 368 mg kg−1; and calcium carbonate (CaCO3) equivalent, 32.5%. The properties of dust used are as follows: sand, 22.24%; clay, 21.83%; gravel, 2.01%; silt, 43.74%; calcium, 22.70%; magnesium, 2.42%; iron, 4.71%; sodium, 2.37%; aluminium, 4.08%; potassium, 0.79; zinc, 52.90 mg kg-1; cupper, 15.80 mg kg-1; cobalt, 12.70 mg kg-1; uranium, 1.96 mg kg-1; cadmium, 0.60 mg kg-1; nickel, 94.00 mg kg-1; vanadium, 73.40 mg kg-1; barium, 231.00 mg kg-1; chromium, 116 mg kg-1; and lead, 20.23 mg kg-1.
One-year seedlings of the same size of S. rosmarinus, H. aphyllum and N. schoberi were obtained from the nursery of Natural Resources and Watershed Management General Office of Yazd Province, Iran. The roots of these seedlings were immersed in each bacterial suspension (3 × 108 CFU mL−1) for two hours or in sterile water as un-inoculated control at room temperature (25°C). The S. rosmarinus, H. aphyllum and N. schoberi seedlings were also co-inoculated with both Z. halotolerans SB and B. pumilus HR by being immersed in equal volumes of the suspension of these two bacteria. After inoculation, the seedlings were singularly transferred to pots. Dust treatments were applied by dust simulator (Dustin-mizer Model 1212, https://www.amazon.com/Dustin-mizer-Model-1212-Includes-Deflector/dp/B002KIB680) 30 days after culturing these seedlings in the pots. The dust treatment was applied at 1.5 g m-2 30 days-1 once a week for 150 days according to a previous assay (Ahmadi Foroushani et al., 2021). After pouring the dust into the valve of the dust simulator, the content of dust (g m-2) was controlled by using a trap with dimensions of 1.6 × 2.3 m2. When applying dust, all the control plants were moved outside the greenhouse so that dust does not settle on them. The plants were grown in a glasshouse at 25 ± 2°C with a photoperiod of 16-h light and 8-h darkness and 60% relative humidity. At the end of the experiment period (150 days after planting in pots), APTI, peroxidase activity, and protein concentration were measured.
2.3 Measurements
2.3.1 APTI determination
Air pollution tolerance index (APTI) was determined according to the following formula (Zouari et al., 2018):
Where A, T, P, and R are ascorbic acid, total chlorophyll, pH of leaf extract and leaf relative water content, respectively.
2.3.2 Ascorbic acid determination
Ascorbic acid content of young and completely expanded leaves (one sample from each plant) was determined based on the spectrophotometric method according to a previous study (Mukherjee and Choudhuri, 1983). Briefly, the leaf sample (100 mg) was extracted with 10 mL of 1% metaphosphoric acid for 45 min at room temperature and filtered through Whatman No. 4 filter paper. The filtrate (1 mL) was then mixed with 9 mL of 2,6-dichlorophenolindophenol, and the absorbance was measured within 30 min at 520 nm against a blank. The content of ascorbic acid was calculated on the basis of the calibration curve of authentic L-ascorbic acid. The content of ascorbic acid was expressed in mg g−1 FW (fresh weight) of tissue.
2.3.3 Total chlorophyll determination
To extract total chlorophyll (chlorophyll a and chlorophyll b), 0.5 g fresh leaf samples (young and completely expanded leaves) were taken and homogenized with 10 mL of 80% acetone. The homogenized samples were centrifuged at 4,000 rpm for 10 min at 4°C. The supernatants were separated from the mixture and collected in a cuvette for further use. To measure chlorophyll a and chlorophyll b, the spectrophotometric method proposed by Lightenthaler (1987) was employed using a UV-VIS spectrophotometer (Varian Cary 50; Varian GmbH, Darmstadt, Germany) to measure absorbance of the extracts at 663.2 nm and 646.8 nm. The concentrations of the photosynthetic pigments present in the extracts were estimated using the following equation:
2.3.4 Leaf extract pH determination
Leaf extract pH was determined according to the protocol of Tak and Kakde (2017). For each treatment, four samples (0.5 g) of fresh leaves (young and completely expanded leaves) were crushed and homogenized in 50 mL deionized water, after which the mixture was centrifuged at 7,000×g for 10 min. The pH of the supernatant was measured using a digital pH meter (EYELA, Japan).
2.3.5 Relative water content determination
For determination of relative water content (RWC), each leaf (young and completely expanded leaves) was weighed on a digital scale (GF-300; A&D Company, Tokyo, Japan) with an accuracy of 0.001 g (FW) and then placed in Falcon tubes completely filled with distilled water. The tubes were left in the dark at 4°C for 24 h. After this period, the leaves were removed from water and placed on absorbent paper to remove excess water, and turgid weight (TW) was determined. Afterward, the leaves were dried at 70°C until stabilization, and dry weight was determined (DW). Leaf RWC was calculated using the following equation and expressed as a percentage (Ritchie et al., 1990):
2.3.6 Peroxidase activity determination
To measure the peroxidase (EC1.11.1.7) activity of young and completely expanded leaves, extraction from fresh leaves (0.5 g) was performed according to Mukherjee and Choudhuri (1983). The extract was frozen in liquid nitrogen and then ground in phosphate buffer (100 mM, pH 7.0). Homogenates were centrifuged at 4°C for 10 min under 15,000×g. The supernatant was kept at 4°C until used to measure the activity of peroxidase. The method of Hemeda and Klein (1990) was used to evaluate peroxidase activity. The rate of guaiacol oxidation in the presence of H2O2 recorded at 470 nm specifies the enzyme activity (U mg-1 protein).
2.3.7 Protein concentration determination
Protein concentration was estimated according to the method described by Bradford (1976). Young and completely expanded leaves (25 mg) were homogenized with 1 mL of TRIS-HCl (0.1 M) buffer and then 20 μL supernatant was mixed with 80 μL of distilled water. Then, 900 μL Bradford reagent was added and incubated for 2 min. The absorbance was measured at 595 nm in an UV–VIS spectrophotometer (Varian Cary 50; Varian GmbH, Darmstadt, Germany). The concentration of protein was determined using bovine serum albumin as standard. The concentration of protein was expressed as microgram bovine serum albumin (BSA) equivalent (μg BSA)/g leaves fresh weight.
2.4 Statistical analysis of data
After confirming the normality of the data by Kolmogorov–Smirnov test, experimental data were analyzed by three-way analysis of variance (ANOVA) using the SAS v.9.1 (SAS Institute Inc., Cary, NC) statistical analysis software. Post-hoc comparisons of means (mean ± SE, n = 3) using Tukey’s multiple-range test at p<0.05 were used to compare significant difference among treatments.
3 Results
3.1 Effect of strains on total chlorophyll of dust-stressed plant species
Dust treatment alone (bacteria free) decreased the amount of total chlorophyll by 18% and 22% in N. schoberi and S. rosmarinus, respectively (Figure 1A). In dust free plants, the combination of two isolates (HR and SB isolates) caused a significant increase of 256% and 27% in total chlorophyll of H. aphyllum and S. rosmarinus plants, respectively, while in N. schoberi plant, B. pumilus HR strain caused the highest increase (52%) compared to its control. Under dust conditions, HR isolate increased the total amount of chlorophyll in H. aphyllum plant (236%), while in N. schoberi and S. rosmarinus plants, Z. halotolerans SB strain increased the amount of total chlorophyll compared to its control (respectively, 71% and 44%). The results also indicated that the effect of bacterial inoculation on increasing total chlorophyll was higher in H. aphyllum plant than the other two species (Figure 1A).
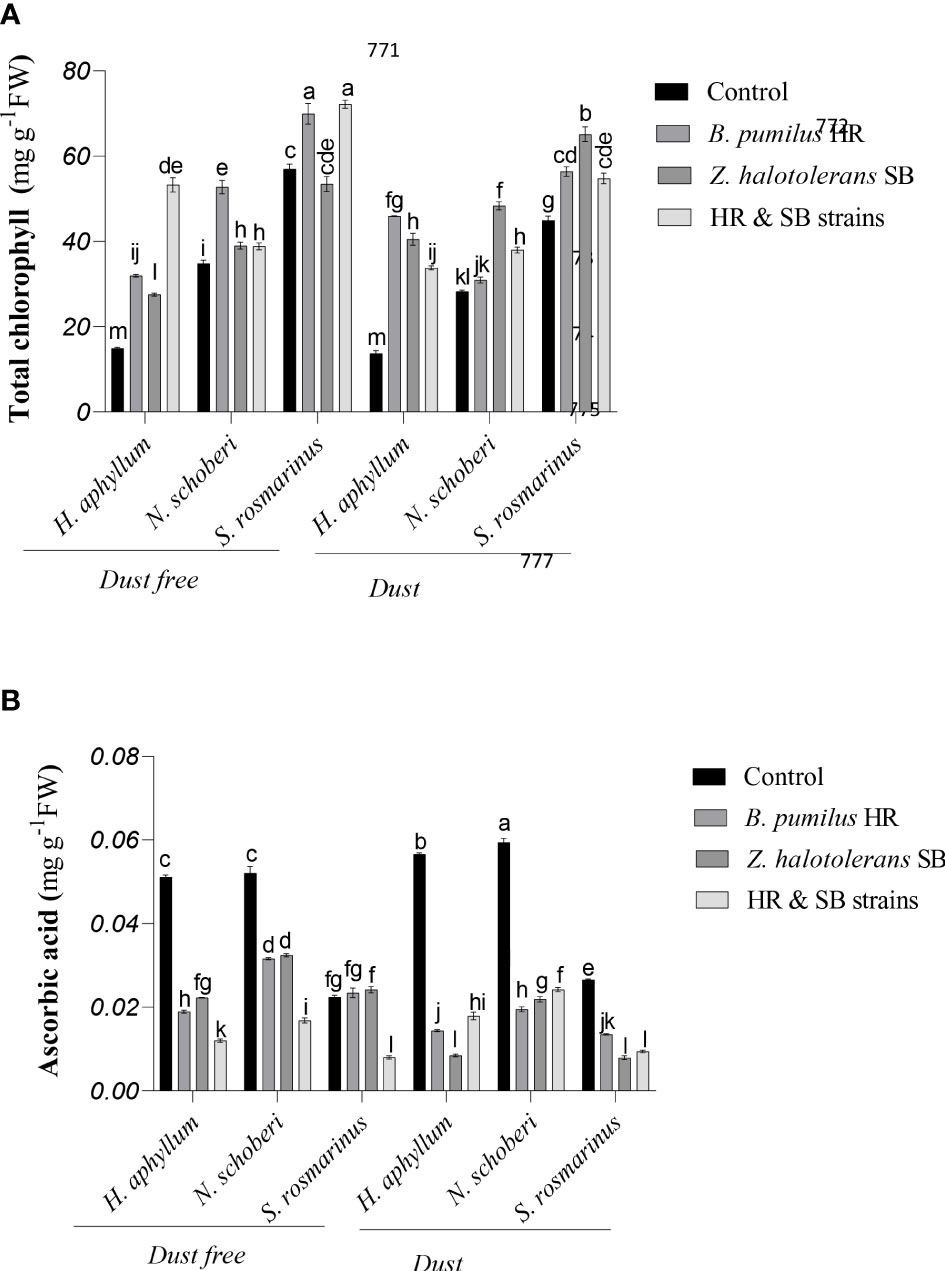
Figure 1 Triple interactions of treatments of bacterial strains, three desert plant species of S. rosmarinus, H. aphyllum and N. schoberi and dust on the content of total chlorophyll (A) and ascorbic acid (B) of the leaves of three plant species grown under greenhouse conditions for five months. Alphabets indicate significant differences among various treatments (Means ± SE, n = 3) according to Tukey’s multiple-range test at p<0.05.
3.2 Effect of strains on ascorbic acid of dust-stressed plant species
In H. aphyllum, N. schoberi and S. rosmarinus plants, the application of dust in the absence of bacteria caused a significant increase of 11%, 14% and 19% in ascorbic acid of these plants, respectively (Figure 1B). In dust free plants, the combination of two isolates significantly decreased ascorbic acid by 77%, 69% and 66%, respectively, in H. aphyllum, N. schoberi and S. rosmarinus compared to the control. Under dust conditions, SB isolate caused the highest decrease in ascorbic acid amount in H. aphyllum and S. rosmarinus plant (respectively, -85% and -70%), while in N. schoberi plants, HR strain caused the highest decrease in ascorbic acid amount compared to its control (-67%). The results also showed that the effect of bacterial inoculation on decreasing ascorbic acid was higher in H. aphyllum plant than the other two species (Figure 1B).
3.3 Effect of strains on leaf pH of dust-stressed plant species
In N. schoberi plant, the use of dust treatment in the absence of bacteria caused a 29% increase in leaf pH (Figure 2A). In dust free H. aphyllum plant, the inoculation with a combination of two isolates decreased the leaf pH by 19% compared to the control. Under dust conditions, in all three plants of H. aphyllum, N. schoberi and S. rosmarinus, SB isolate caused the greatest decrease in leaf pH compared to its control (-17%, -35% and -13%, respectively). The results also showed that the effect of bacterial inoculation on decreasing leaf pH was higher in N. schoberi plant than the other two species (Figure 2A).
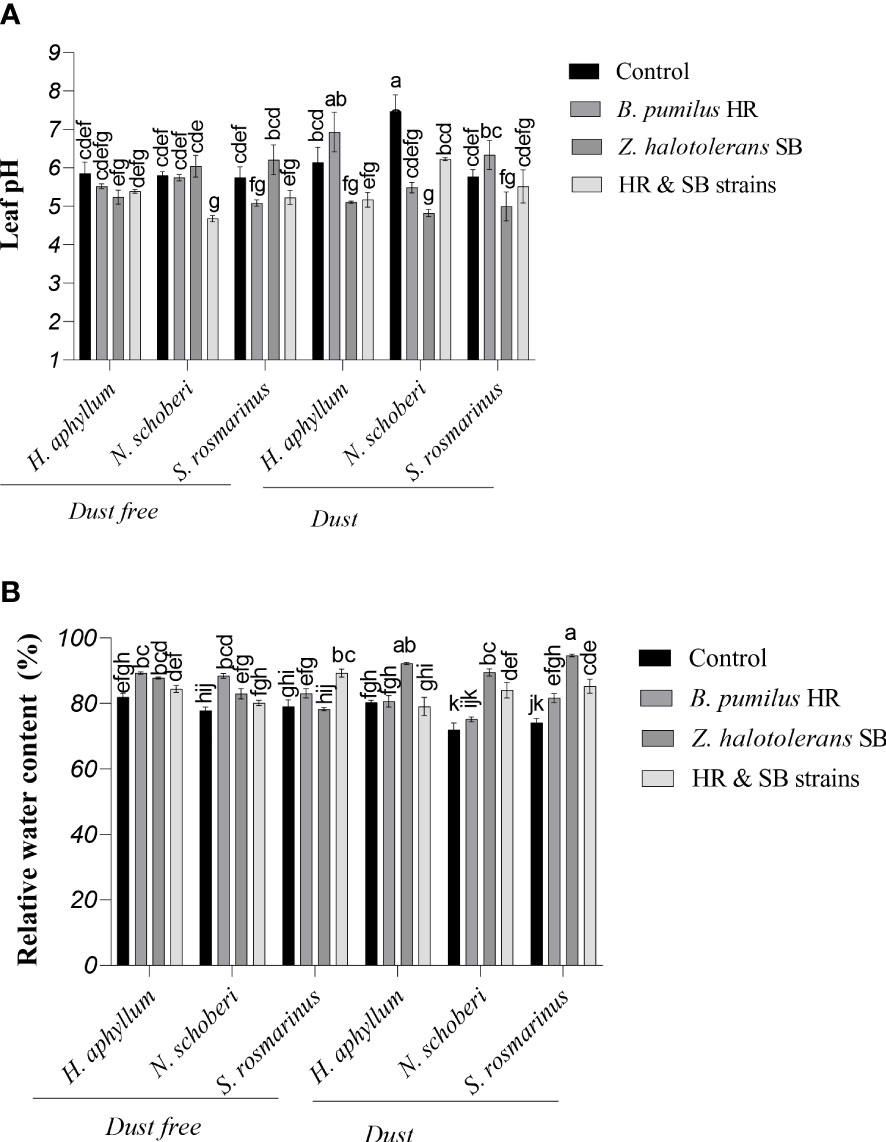
Figure 2 Triple interactions of treatments of bacterial strains, three desert plant species of S. rosmarinus, H. aphyllum and N. schoberi and dust on leaf pH (A) and relative water content (B) of the leaves of three plant species grown under greenhouse conditions for five months. Alphabets indicate significant differences among various treatments (Means ± SE, n = 3) according to Tukey’s multiple-range test at p<0.05.
3.4 Effect of strains on RWC of dust-stressed plant species
Under dust-free conditions, the inoculation with HR strain in H. aphyllum and N. schoberi plants increased the amount of relative water content (RWC) by 9% and 14% compared to the control, while the combination of two isolates in S. rosmarinus increased RWC by 12% compared to the control (Figure 2B). Under dust conditions in all three plants H. aphyllum, N. schoberi and S. rosmarinus, the inoculation with SB isolate caused a significant increase in RWC by 15%, 24% and 28%, respectively, compared to the control. The results also indicated that the effect of bacterial inoculation on increasing RWC was higher in S. rosmarinus plant than the other two species (Figure 2B).
3.5 Effect of strains on APTI of dust-stressed plant species
Under the dust free conditions, HR strain caused the most significant increase of APTI by 8% and 13%, respectively, in H. aphyllum and N. schoberi plants compared to control, while in S. rosmarinus plant, the co-inoculation of two isolates increased APTI by 11%. Under dust conditions, SB isolate caused the greatest increase in APTI in H. aphyllum, N. schoberi and S. rosmarinus plants compared to their controls (14%, 22% and 26%, respectively). The results also showed that the effect of bacterial inoculation on increasing APTI was higher in S. rosmarinus plant than the other two species (Figure 3).
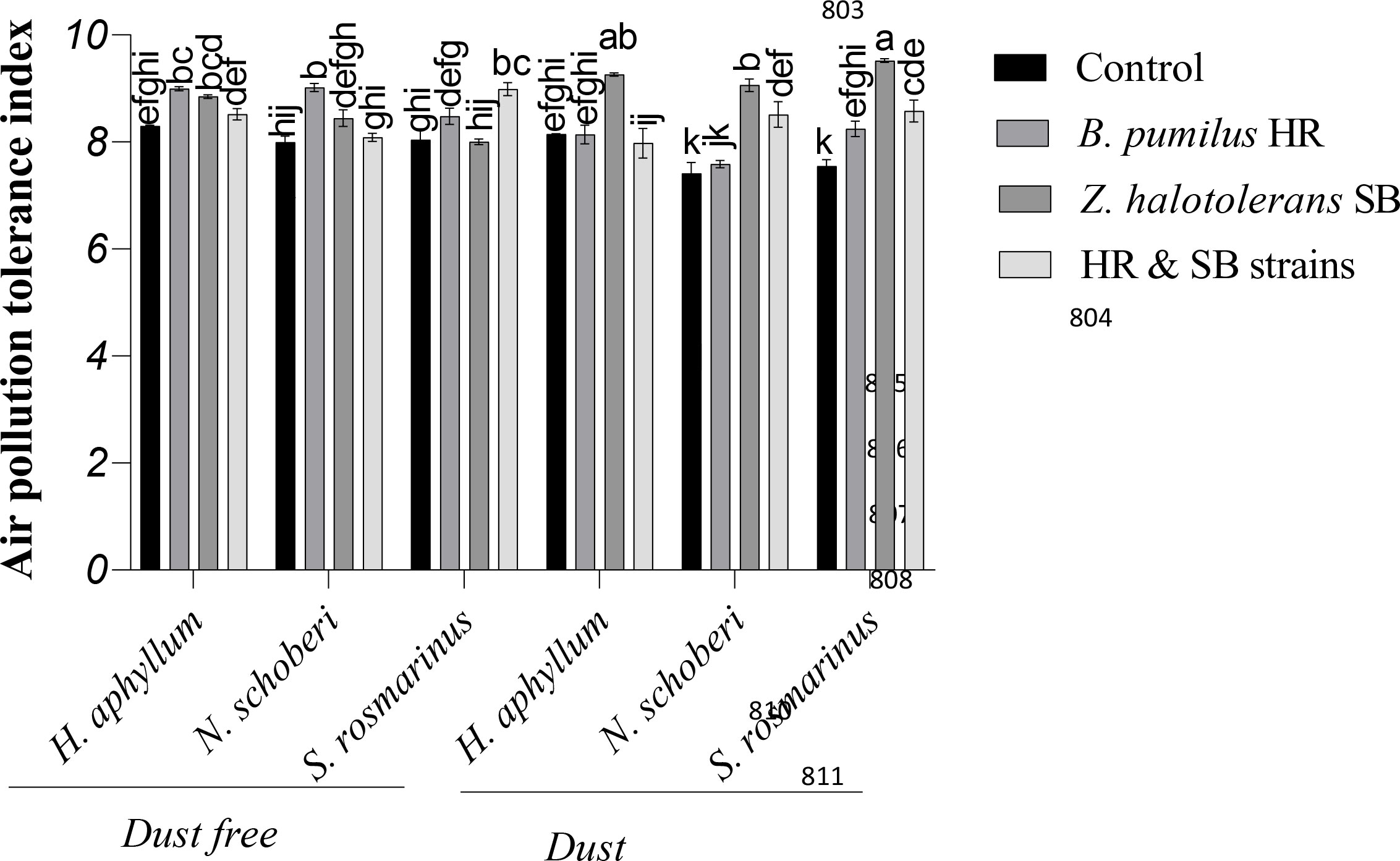
Figure 3 Triple interactions of treatments of bacterial strains, three desert plant species of S. rosmarinus, H. aphyllum and N. schoberi and dust on air pollution tolerance index (APTI) of three plant species grown under greenhouse conditions for five months. Alphabets indicate significant differences among various treatments (Means ± SE, n = 3) according to Tukey’s multiple-range test at p<0.05.
3.6 Effect of strains on peroxidase of dust-stressed plant species
In H. aphyllum, N. schoberi and S. rosmarinus plants, the application of dust treatment in the absence of bacteria caused a significant increase of 23%, 28% and 26% in peroxidase, respectively (Figure 4A). In dust free plants, the inoculation with HR isolate, SB isolate and the combination of these two isolates decreased the activity of peroxidase by 84%, 76% and 22% respectively, in H. aphyllum plant, by 64%, 60% and 39% in N. schoberi plant, and by 67%, 52% and 88% in S. rosmarinus, respectively, compared to the control. Also, under dust conditions, HR isolate, SB isolate and the combination of two isolates reduced peroxidase activity by 28%, 77% and 64% in H. aphyllum plant, by 51%, 48% and 36% in N. schoberi plant and by 90%, 43% and 25% in S. rosmarinus plant, respectively, compared to the control. The results also indicated that the effect of bacterial inoculation on increasing peroxidase activity was higher in S. rosmarinus plant than the other two species (Figure 4A).
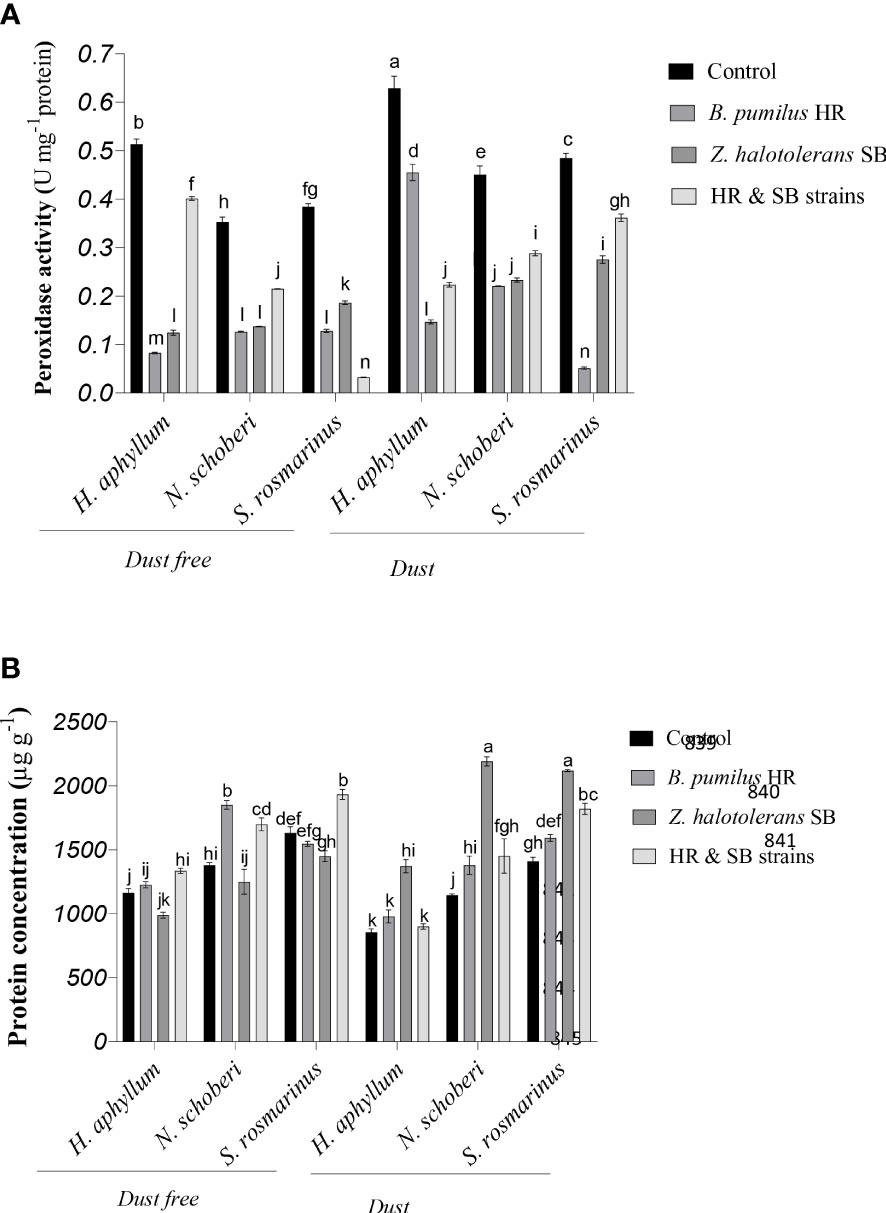
Figure 4 Triple interactions of treatments of bacterial strains, three desert plant species of S. rosmarinus, H. aphyllum and N. schoberi and dust on peroxidase activity (A) and protein concentration (B) of the leaves of three plant species grown under greenhouse conditions for five months. Alphabets indicate significant differences among various treatments (Means ± SE, n = 3) according to Tukey’s multiple-range test at p<0.05.
3.7 Effect of strains on protein of dust-stressed plant species
In H. aphyllum, N. schoberi and S. rosmarinus plants, the application of dust in the absence of bacteria significantly decreased protein concentration by 26%, 17%, and 14%, respectively (Figure 4B). In dust free plants, the inoculation of bacteria caused a significant increase in the concentration of protein, as the combination of two isolates increased protein concentration by 15% and 18% in H. aphyllum and S. rosmarinus, respectively, compared to the control. In N. schoberi plant, the inoculation with HR isolate and the combination of two isolates increased the concentration of protein by 34% and 23%, respectively, compared to the control. Also, under dust conditions, the inoculation with bacteria caused a significant increase in the concentration of protein, as in the inoculation of SB strain, the concentration of protein increased by 60%, 91% and 50%, respectively, in H. aphyllum, N. schoberi and S. rosmarinus plants compared to the control. The results also indicated that the effect of bacterial inoculation on increasing protein concentration was higher in N. schoberi plant than the other two species (Figure 4B).
4 Discussion
Dust causes adverse effects on the physiological and biochemical characteristics of plants (Sharifi et al., 2019; Meravi et al., 2021; Yaghmaei et al., 2022) and limits their use in the development of the green belt. In the present study, we also showed that dust could negatively affect the characteristics of three desert plant species of S. rosmarinus, H. aphyllum and N. schoberi. In the present research, with the application of dust, the amount of total chlorophyll in N. schoberi and S. rosmarinus species decreased significantly compared to the control, but this decrease was not significant in H. aphyllum species. An increase in dust concentration also induced a significant decrease in the content of total chlorophyll in four plant species (Fraxinus rotundifolia, Morus alba, Colias caucasica, and Melia azedarach) (Javanmard et al., 2019). A similar trend of reduced total chlorophyll in response to dust pollution has also been reported on H. aphyllum Bunge (Heydarnezhad, 2014; Najafi Zilaie et al., 2022b) and S. rosmarinus (Zilaie et al., 2022). Dust deposition on leaf surface may decrease the synthesis of chlorophyll because of shading effects (Sarma et al., 2017; Setsungnern et al., 2018). Another reason may be related to pigment degradation and inhibition of enzymes essential for biosynthesis of pigments due to incorporation of dust particles into leaf tissues (Lepeduš et al., 2003). In the present study, the application of bacterial strains increased the concentration of total chlorophyll in all three species. Under dust conditions, the effect of dust particle application on plant physiology is similar to the effects of drought stress (Rai, 2016). The increase in the amount of total chlorophyll in the plants inoculated with beneficial bacteria under dust conditions in the present study may be due to the ability of the bacterial strains to produce siderophore and auxin, as well as their ability to increase the absorption of nutrients involved in the structure of chlorophyll such as Mn and Mg (Zilaie et al., 2022; Najafi Zilaie et al., 2022a). Siderophores have a strong tendency to absorb iron (III), which is an essential nutrient for chlorophyll construction (Santoyo et al., 2019). The results of the present study also showed that the highest concentration of total chlorophyll was registered in H. aphyllum plant inoculated with Z. halotolerans; this can be due to the significant effect of two PGP traits of siderophore and auxin hormone in this bacterium (Hajiabadi et al., 2021).
Ascorbic acid in the leaves of plants has multiple functions through cell wall synthesis, cell division, photosynthetic carbon fixation, and acts as a strong reducing agent to protect plants against reactive oxygen species (ROS); thus it improves the ability of plants to withstand air pollution (Sahu et al., 2020). The level of tolerance in plants increases with increasing ascorbic acid content (Lima et al., 2000). The findings of the presented research also indicated that the amount of ascorbic acid in the leaves of three species increased significantly with the application of dust. This indicates that the three plant species respond to oxidative stress caused by dust with an increase in ascorbic acid content. These results are in line with previous research findings (Meerabai et al., 2012; Sahu et al., 2020). Leaf pH is an index of detoxification mechanism in plants for improving tolerance capacity against air pollution (Ninave et al., 2001). The leaf extract pH is known to impact the ascorbic acid synthesis of plants (Singare and Talpade, 2013). Increase in the leaf extract pH value has been reported to efficiently convert the hexose sugar to ascorbic acid and therefore a high pH is considered good for the tolerance of plants against air pollution (Shannigrahi et al., 2004; Enete and Ogbonna, 2012). The findings of the presented research also indicated that the leaf extract pH in N. schoberi species increased significantly with the application of dust. The penetration of chemical dust particles with an alkaline nature into the cell sap and their conversion to radicals cause an increase in leaf extract pH. High leaf pH also increases the efficiency of conversion from hexose to ascorbic acid (Singare and Talpade, 2013). In the current study, it seems likely that the penetration of alkaline dust particles (pH 7.6) into the leaf tissues, along with growing dust accumulation on the leaf surface, caused increased pH in the leaf extracts. In the present study, the bacterial strains significantly decreased the concentration of leaf ascorbic acid and pH of the leaf extract under dust stress, among which the SB strain had the most effect. Since plant oxidative stress is reduced by bacterial inoculation, the stress response leading to ascorbate production is less intense. Decreased ascorbic acid concentration in the plants inoculated with PGPB under dust stress has been reported in other studies (Walia et al., 2019; Najafi Zilaie et al., 2022b). These bacteria seemingly improved plant tolerance, but decreased plant tolerance responses.
Water content in plant leaves help maintain the physiological water balance in adverse environmental conditions (Yadav and Pandey, 2020). High relative water content of leaf favours transpiration rate and pollution resistance in plants (Jyothi and Jaya, 2010). The results of the presented research indicated that dust caused a significant decrease in RWC in N. schoberi species, but this decrease was not significant in H. aphyllum and S. rosmarinus species. This result is in line with earlier studies on other plants (Maletsika et al., 2015; Karami et al., 2017). The lowest relative water content among the investigated plant species was related to N. schoberi species, whose relative water content was equal to 71.95%. In a similar research, the application of dust treatment led to a decrease in the relative water content (Javanmard et al., 2019). Low RWC may be due to the effect of dust pollutant on leaf transpiration, since the crust formed by dust deposition on leaves can lead to blockage of the stomata and reduced transpiration (Zia-Khan et al., 2014; Masoud et al., 2019). Also, the reaction of dust particles with the cell membrane results in foliar injury and higher membrane permeability in dusted leaves, which may be another reason for low RWC value (Yaghmaei et al., 2022). Dust particles also cause loss of water and soluble nutrients in plants by increasing the permeability of plant cells and eventually causing premature aging of leaves (Meravi et al., 2021). Therefore, plants with a RWC less affected by stress were more resistant to dust (Taheri Analojeh et al., 2016). The results of the presented study showed that the inoculation of three plants with PGPR strains increased the relative water content in these plant species. The highest leaf RWC was observed in the plants inoculated with two strains (Z. halotolerans SB + B. pumilus HR) followed by the plants inoculated with B. pumilus HR strain compared to control crops under dust stress. One of the reasons for this increase in the plant species inoculated with bacterial strains could be the production of auxin and, thus, increase in the length and weight of roots compared to the control plant species under stress conditions (Etesami and Maheshwari, 2018; Najafi Zilaie et al., 2022b). These bacterial strains may also decrease the production of abscisic acid (Naz et al., 2009); in this way, they decrease the negative effects of stress on stomatal conductance, photosynthesis, and plant sensitivity to water deficiency (Etesami and Maheshwari, 2018). It seems that the plants inoculated with the bacterial strains have the ability to change the structure of the lateral root system and increase RWC (Bertrand et al., 2015; Shirmohammadi et al., 2020). Increased leaf RWC under stress in the plants inoculated with PGPR has also been reported in other studies (Tahir et al., 2017; Cedeño-García et al., 2018; Zilaie et al., 2022). In general, an increase in the number of lateral roots and root hairs causes addition of root surfaces available for nutrient and water uptake. Higher water and nutrient uptake by inoculated roots cause an improved water status of plants, which in turn could be the main factor enhancing plant growth (Etesami and Maheshwari, 2018).
The application of dust resulted in a significant decrease in APTI in N. schoberi species, but this decrease was not significant in H. aphyllum and S. rosmarinus species. The APTI indicates the plant’s ability to tolerate air pollution (Molnár et al., 2020). The reduction of APTI under dust conditions in the present research can be due to the effect of dust particles on the parameters of APTI. When the plant absorbs dust particles on the surface of its leaves, adverse effects occur at the physiological (change in pH and loss of relative water content) and biochemical (decrease in chlorophyll concentration) levels, which causes a decrease in APTI. APTI reduction under dust stress has been determined in other studies (Chaudhary and Rathore, 2019; Yadav and Pandey, 2020). The inoculation of these three plants with PGPR strains under dust stress increased APTI in the plants, as the inoculation with B. pumilus HR isolate had the greatest effect on increasing APTI in N. schoberi species. It can be assumed that the use of PGPR leads to the improvement of photosynthesis, and finally increasing the plant’s tolerance to dust stress by increasing the water use efficiency and relative water content as well as the concentration of chlorophyll (through the production of siderophore and auxin) and reducing pH. The results of the present study also showed that H. aphyllum species had a higher APTI (the least affected by stress) than the other two species. The plant species having high APTI are supposed to have greater defence against air pollution. This corroborates the earlier reports that the plants exposed to polluted environment tend to increase their tolerance ability with high APTI. Whereas the plants showing a decrease in APTI can be used as indicator (Prajapati and Tripathi, 2008; Jyothi and Jaya, 2010). The idea can also be used to identify the plants suited for plantation.
The results of the present study also showed that with the application of dust, the peroxidase activity of these plants increased. The highest and the lowest activity of leaf peroxidase enzyme were related to the non-inoculated H. aphyllum under dust stress (0.63 U mg-1 protein) and B. pumilus HR-inoculated H. aphyllum (0.12 U mg-1 protein), respectively. The closing of the stomata by dust particles in the plant tissue is the fastest reaction of plants in response to the presence of dust, which disrupts the photosynthesis process, increases ROS and creates oxidative stress in the plants. The increase in the active forms of oxygen under stress increases the activity of some antioxidant enzymes such as peroxidase, which ultimately leads to the plant’s tolerance to environmental stresses (Ghanem et al., 2021). In the study of Najafi Zilaie et al. (2022b), the activity of peroxidase enzyme also increased in plants under dust stress. In the present study, the inoculation with PGPR strains decreased the peroxidase enzyme activity in these plants, among which B. pumilus HR isolate had the greatest effect on reducing the peroxidase activity of leaves. It can be supposed that PGPR reduced the concentration of ROS and free radicals by creating suitable conditions for the plant growth, and as a result, the activity of the crop’s antioxidant defense systems decreased. PGPR participate in reducing stress by increasing water absorption through producing auxin and enhancing root volume, which justifies the reduction in the production of antioxidants (Etesami and Maheshwari, 2018).
In this study, the amount of protein decreased under dust stress. It seems that the decrease in the photosynthetic efficiency of plants under dust stress has caused a decrease in the accumulation of photosynthetic materials, which has also led to a decrease in protein concentration (Setsungnern et al., 2018). In addition, due to the closing of the stomata, the production of free radicals (Thompson et al., 1984) may have played a role in damaging the protein structure. According to Treesubsuntorn et al. (2021), a decrease in protein production occurs under dust stress. In the present study, the inoculation of plants with bacterial isolates under dust stress increased the protein concentration in the plants, as the highest increase in the concentration of protein (67.83 µg g-1) among the studied species was observed in the N. schoberi species inoculated with the combination of two bacteria isolates. Preventing the destruction of protein or stimulating their synthesis with the support of biological fixation of N2 is one of the strategies of bacterial strains to withstand the stress of the crop, which ultimately causes an increase in the concentration of protein in the plant (Nawaz et al., 2020). Previously, nitrogen fixation by the same genera of studied bacteria has been reported (Emami et al., 2019). An increase in protein production under stressful conditions following the treatment of plant with PGPR has also been reported in other studies (Liu et al., 2019; Pan et al., 2019).
H. aphyllum species had the highest APTI compared to the other two species, and bacterial strains increased this index in the studied species. As mentioned before, one of the reasons for increasing RWC in plants inoculated with PGPR can be the production of growth phytohormones and the development of the root system to improve the absorption and efficiency of water use under stress conditions (Baldan et al., 2015). The plants inoculated with PGPR have the ability to alter the structure of the lateral root system and increase water absorption. It is known that PGPR decrease the negative effects of stress on plants by producing abscisic acid and thereby reduce the plant’s sensitivity to water shortage to some extent (Etesami and Beattie, 2017). The increase of water inside the plant tissue (as one of the determining parameters of APTI) increases the APTI in the plant. The results of the present research also indicated that bacterial strains especially SB strain caused a further increase in the relative water content in H. aphyllum species.
5 Conclusions
The results of the research indicated that the physiological parameters of three important desert plants (S. rosmarinus, H. aphyllum and N. schoberi) were negatively affected by dust stress. However, PGPR (Z. halotolerans SB and B. pumilus HR) could attenuate the adverse effects of the dust stress on these plants. Bacterial strain SB isolated from S. rosmarinus had a greater effect on alleviating the negative impacts of dust on most of the indicators measured in this plant than B. pumilus HR. However, B. pumilus HR performed better on H. aphyllum and N. schoberi, which explains the importance of bacteria-plant interaction. The results also showed that H. aphyllum species had a higher air pollution tolerance index (APTI) than the other two species. It was also concluded that the application of PGPR can improve the tolerance of these plants to air pollution (APTI) by improving the effective parameters. Therefore, in order to increase the tolerance of green belt plants to air pollution, the use of PGPR should also be considered as an effective factor in such studies.
Data availability statement
The original contributions presented in the study are included in the article/supplementary materials. Further inquiries can be directed to the corresponding author.
Author contributions
All authors contributed to the article and approved the submitted version.
Acknowledgments
For providing the necessary facilities for this study, we thank University of Tehran and Yazd University.
Conflict of interest
The authors declare that the research was conducted in the absence of any commercial or financial relationships that could be construed as a potential conflict of interest.
Publisher’s note
All claims expressed in this article are solely those of the authors and do not necessarily represent those of their affiliated organizations, or those of the publisher, the editors and the reviewers. Any product that may be evaluated in this article, or claim that may be made by its manufacturer, is not guaranteed or endorsed by the publisher.
References
Abbasi, S., Keshavarzi, B., Moore, F., Turner, A., Kelly, F. J., Dominguez, A. O., et al. (2019). Distribution and potential health impacts of microplastics and microrubbers in air and street dusts from asaluyeh county, Iran. Environ. pollut. 244, 153–164. doi: 10.1016/j.envpol.2018.10.039
Ahmadi Foroushani, M., Opp, C., Groll, M. (2021). Investigation of eolian dust deposition rates in different climate zones of southwestern Iran. Atmosphere 12, 229. doi: 10.3390/atmos12020229
Alotaibi, M. D., Alharbi, B. H., Al-Shamsi, M. A., Alshahrani, T. S., Al-Namazi, A. A., Alharbi, S. F., et al. (2020). Assessing the response of five tree species to air pollution in Riyadh city, Saudi Arabia, for potential green belt application. Environ. Sci. pollut. Res. 27, 29156–29170. doi: 10.1007/s11356-020-09226-w
Ansari, M., Shekari, F., Mohammadi, M. H., Juhos, K., Végvári, G., Biró, B. (2019). Salt-tolerant plant growth-promoting bacteria enhanced salinity tolerance of salt-tolerant alfalfa (Medicago sativa l.) cultivars at high salinity. Acta Physiol. Plantarum 41, 1–13. doi: 10.1007/s11738-019-2988-5
Baldan, E., Nigris, S., Romualdi, C., D’Alessandro, S., Clocchiatti, A., Zottini, M., et al. (2015). Beneficial bacteria isolated from grapevine inner tissues shape arabidopsis thaliana roots. PloS One 10, e0140252. doi: 10.1371/journal.pone.0140252
Bellini, E., De Tullio, M. C. (2019). Ascorbic acid and ozone: Novel perspectives to explain an elusive relationship. Plants 8, 122. doi: 10.3390/plants8050122
Bertrand, M., Barot, S., Blouin, M., Whalen, J., de Oliveira, T., Roger-Estrade, J. (2015). Earthworm services for cropping systems. a review. Agron. Sustain. Dev. 35, 553–567. doi: 10.1007/s13593-014-0269-7
Bradford, M. M. (1976). A rapid and sensitive method for the quantitation of microgram quantities of protein utilizing the principle of protein-dye binding. Analyt. Biochem. 72, 248–254. doi: 10.1016/0003-2697(76)90527-3
Cedeño-García, G. A., Gerding, M., Moraga, G., Inostroza, L., Fischer, S., Sepúlveda-Caamaño, M., et al. (2018). Plant growth promoting rhizobacteria with ACC deaminase activity isolated from Mediterranean dryland areas in Chile: Effects on early nodulation in alfalfa. Chilean J. Agric. Res. 78, 360–369. doi: 10.4067/S0718-58392018000300360
Chaudhary, I. J., Rathore, D. (2019). Dust pollution: Its removal and effect on foliage physiology of urban trees. Sustain. Cities Soc. 51, 101696. doi: 10.1016/j.scs.2019.101696
Emami, T., Mirzaeiheydari, M., Maleki, A., Bazgir, M. (2019). Effect of native growth promoting bacteria and commercial biofertilizers on growth and yield of wheat (Triticum aestivum) and barley (Hordeum vulgare) under salinity stress conditions. Cell. Mol. Biol. 65, 22–27. doi: 10.14715/cmb/2019.65.6.5
Enete, I. C., Ogbonna, C. E. (2012). Evaluation of air pollution tolerance index (APTI) of some selected ornamental shrubs in enugu city, Nigeria. J. Environ. Sci. Toxicol. Food Technol. 1, 22–25. doi: 10.9790/2402-0122225
Escobedo, F. J., Wagner, J. E., Nowak, D. J., de la Maza, C. L., Rodriguez, M., Crane, D. E. (2008). Analyzing the cost effectiveness of Santiago, chile’s policy of using urban forests to improve air quality. J. Environ. Manage. 86, 148–157. doi: 10.1016/j.jenvman.2006.11.029
Etesami, H., Beattie, G. A. (2017). Plant-microbe interactions in adaptation of agricultural crops to abiotic stress conditions, probiotics and plant health. Springer pp, 163–200. doi: 10.1007/978-981-10-3473-2_7
Etesami, H., Glick, B. R. (2020). Halotolerant plant growth–promoting bacteria: Prospects for alleviating salinity stress in plants. Environ. Exp. Bot. 178, 104124. doi: 10.1016/j.envexpbot.2020.104124
Etesami, H., Maheshwari, D. K. (2018). Use of plant growth promoting rhizobacteria (PGPRs) with multiple plant growth promoting traits in stress agriculture: Action mechanisms and future prospects. Ecotoxicol. Environ. Saf. 156, 225–246. doi: 10.1016/j.ecoenv.2018.03.013
Farooq, M., Beg, M. U. (1980). Effect of aqueous sulphur dioxide on the membrane permeability of common Indian tree leaves. New Bot. 7, 213–217.
Ghanem, A.-M.F.M., Mohamed, E., Kasem, A. M. M. A., El-Ghamery, A. A. (2021). Differential salt tolerance strategies in three halophytes from the same ecological habitat: Augmentation of antioxidant enzymes and compounds. Plants 10, 1100. doi: 10.3390/plants10061100
Hajiabadi, A. A., Arani, A. M., Ghasemi, S., Rad, M. H., Etesami, H., Manshadi, S. S., et al. (2021). Mining the rhizosphere of halophytic rangeland plants for halotolerant bacteria to improve growth and yield of salinity-stressed wheat. Plant Physiol. Biochem. 163, 139–153. doi: 10.1016/j.plaphy.2021.03.059
Hamraz, H., Sadeghi-Niaraki, A., Omati, M., Noori, N. (2014). GIS-based air pollution monitoring using static stations and mobile sensor in Tehran/Iran. Int. J. Sci. Res. Environ. Sci. 2, 435. doi: 10.12983/ijsres-2014-p0435-0448
Hemeda, H. M., Klein, B. P. (1990). Effects of naturally occurring antioxidants on peroxidase activity of vegetable extracts. J. Food Sci. 55, 184–185. doi: 10.1111/j.1365-2621.1990.tb06048.x
Heydarnezhad, S. (2014). Impact of aeolian dust accumulation on some biochemical parameters in black saxaul (Haloxylon aphyllum bunge) leaves: a case study for the aran-bidgol region, Iran. Int. J. For. Soil Erosion (IJFSE) 4, 11–15.
Hoque, M. A., Banu, M. N. A., Okuma, E., Amako, K., Nakamura, Y., Shimoishi, Y., et al. (2007). Exogenous proline and glycinebetaine increase NaCl-induced ascorbate–glutathione cycle enzyme activities, and proline improves salt tolerance more than glycinebetaine in tobacco bright yellow-2 suspension-cultured cells. J. Plant Physiol. 164, 1457–1468. doi: 10.1016/j.jplph.2006.10.004
Javanmard, Z., Kouchaksaraei Tabari, M., Bahrami, H., Hosseini, S. M., Sanavi, S., Struve, D. (2019). Dust collection potential and air pollution tolerance indices in some young plant species in arid regions of Iran. iForest Biogeosci. For. 12, 558–564. doi: 10.3832/ifor3063-012
Jha, B., Gontia, I., Hartmann, A. (2012). The roots of the halophyte salicornia brachiata are a source of new halotolerant diazotrophic bacteria with plant growth-promoting potential. Plant Soil 356, 265–277. doi: 10.1007/s11104-011-0877-9
Jyothi, S. J., Jaya, D. S. (2010). Evaluation of air pollution tolerance index of selected plant species along roadsides in thiruvananthapuram, kerala. J. Environ. Biol. 31, 379–386.
Karami, L., Ghaderi, N., Javadi, T. (2017). Morphological and physiological responses of grapevine (Vitis vinifera l.) to drought stress and dust pollution. Folia Hortic. 29, 231. doi: 10.1515/fhort-2017-0021
Kwon, K.-J., Urrintuya, O., Kim, S.-Y., Yang, J.-C., Sung, J.-W., Park, B.-J. (2020). Removal potential of particulate matter of 12 woody plant species for landscape planting. J. People Plants Environ. 23, 647–654. doi: 10.11628/ksppe.2020.23.6.647
Lepeduš, H., Cesar, V., Suver, M. (2003). The annual changes of chloroplast pigments content in current-and previous-year needles of Norway spruce (Picea abies l. karst.) exposed to cement dust pollution. Acta Botanica Croatica 62, 27–35.
Lightenthaler, H. K. (1987). Chlorophylls and carotenoids: pigments of photosynthetic biomembranes. Methods Enzymol. 148, 350–382. doi: 10.1016/0076-6879(87)48036-1
Lima, J. S., Fernandes, E. B., Fawcett, W. N. (2000). Mangifera indica and phaseolus vulgaris in the bioindication of air pollution in bahia, Brazil. Ecotoxicol. Environ. Saf. 46, 275–278. doi: 10.1006/eesa.1999.1894
Liu, J., Tang, L., Gao, H., Zhang, M., Guo, C. (2019). Enhancement of alfalfa yield and quality by plant growth-promoting rhizobacteria under saline-alkali conditions. J. Sci. Food Agric. 99, 281–289. doi: 10.1002/jsfa.9185
Maletsika, P. A., Nanos, G. D., Stavroulakis, G. G. (2015). Peach leaf responses to soil and cement dust pollution. Environ. Sci. pollut. Res. 22, 15952–15960. doi: 10.1007/s11356-015-4821-z
Manjunath, B. T., Reddy, J. (2019). Comparative evaluation of air pollution tolerance of plants from polluted and non-polluted regions of bengaluru. J. Appl. Biol. Biotechnol. 7, 6–8. doi: 10.7324/JABB.2019.70312
Masoud, T., Bahrami, H., Hosseini, S. M., Modarres Sanavi, S. A. (2019). Effects of dust on morpho-physiological responses of fraxinus rotundifolia mill. Seedling Iranian J. For. 11, 309–323.
Meerabai, G., Ramana, V. C., Rasheed, M. (2012). Effect of industrial pollutants on physiology of cajanus cajan (L.)-fabaceae. Int. J. Environ. Sci. 2, 1889. doi: 10.6088/ijes.00202030072
Meravi, N., Singh, P. K., Prajapati, S. K. (2021). Seasonal variation of dust deposition on plant leaves and its impact on various photochemical yields of plants. Environ. Challenges 4, 100166. doi: 10.1016/j.envc.2021.100166
Middleton, N. (2019). Variability and trends in dust storm frequency on decadal timescales: Climatic drivers and human impacts. Geosciences 9, 261. doi: 10.3390/geosciences9060261
Molnár, V.É., Simon, E., Tóthmérész, B., Ninsawat, S., Szabó, S. (2020). Air pollution induced vegetation stress–the air pollution tolerance index as a quick tool for city health evaluation. Ecol. Indic. 113, 106234. doi: 10.1016/j.ecolind.2020.106234
Mukherjee, S. P., Choudhuri, M. A. (1983). Implications of water stress-induced changes in the levels of endogenous ascorbic acid and hydrogen peroxide in vigna seedlings. Physiol. Plantarum 58, 166–170. doi: 10.1111/j.1399-3054.1983.tb04162.x
Nadgórska–Socha, A., Kandziora-Ciupa, M., Trzęsicki, M., Barczyk, G. (2017). Air pollution tolerance index and heavy metal bioaccumulation in selected plant species from urban biotopes. Chemosphere 183, 471–482. doi: 10.1016/j.chemosphere.2017.05.128
Najafi Zilaie, M., Mosleh Arani, A., Etesami, H., Dinarvand, M. (2022a). Halotolerant rhizobacteria enhance the tolerance of the desert halophyte Nitraria schoberi to salinity and dust pollution by improving its physiological and nutritional status. Appl. Soil Ecol. 179, 104578. doi: 10.1016/j.apsoil.2022.104578
Najafi Zilaie, M., Mosleh Arani, A., Etesami, H., Dinarvand, M. (2022b). Improved salinity and dust stress tolerance in the desert halophyte Haloxylon aphyllum by halotolerant plant growth-promoting rhizobacteria. Front. Plant Sci. 13, 2625. doi: 10.3389/fpls.2022.948260
Nawaz, A., Shahbaz, M., Imran, A., Marghoob, M. U., Imtiaz, M., Mubeen, F. (2020). Potential of salt tolerant PGPR in growth and yield augmentation of wheat (Triticum aestivum l.) under saline conditions. Front. Microbiol. 11, 2019. doi: 10.3389/fmicb.2020.02019
Naz, I., Bano, A., Ul-Hassan, T. (2009). Isolation of phytohormones producing plant growth promoting rhizobacteria from weeds growing in khewra salt range, Pakistan and their implication in providing salt tolerance to glycine max l. Afr. J. Biotechnol. 8, 5762–5766.
Neel, R., Milan, Y., Singh, U. N. (2018). Air pollution tolerance index of various plant species growing in industrial area of orai district jalaun (UP) India. Flora Fauna (Jhansi) 24, 45–49. doi: 10.33451/florafauna.v24i1pp45-49
Ninave, S. Y., Chaudhari, P. R., Gajghate, D. G., Tarar, J. L. (2001). Foliar biochemical features of plants as indicators of air pollution. Bull. Environ. Contamination Toxicol. 67, 133–140. doi: 10.1007/s001280101
Pan, J., Peng, F., Xue, X., You, Q., Zhang, W., Wang, T., et al. (2019). The growth promotion of two salt-tolerant plant groups with PGPR inoculation: a meta-analysis. Sustainability 11, 378. doi: 10.3390/su11020378
Pasqualini, S., Batini, P., Ederli, L., Porceddu, A., Piccioni, C., De Marchis, F., et al. (2001). Effects of short-term ozone fumigation on tobacco plants: response of the scavenging system and expression of the glutathione reductase. Plant. Cell Environ. 24, 245–252. doi: 10.1111/j.1365-3040.2001.00671.x
Pradhan, A. A., Pattanayak, S. K., Bhadra, A. K., Ekka, K. (2016). Air pollution tolerance index of three species along national high way–6 between ainthapali to remed, sambalpur district, Western odisha, India. Biolife 4, 111–120.
Prajapati, S. K., Tripathi, B. D. (2008). Anticipated performance index of some tree species considered for green belt development in and around an urban area: A case study of varanasi city, India. J. Environ. Manage. 88, 1343–1349. doi: 10.1016/j.jenvman.2007.07.002
Priyanka, C., Dibyendu, B. (2009). Biomonitoring of air quality in the industrial town of asansol using the air pollution tolerance index approach. Res. J. Chem. Environ. 13, 46–51.
Rai, P. K. (2016). Impacts of particulate matter pollution on plants: Implications for environmental biomonitoring. Ecotoxicol. Environ. Saf. 129, 120–136. doi: 10.1016/j.ecoenv.2016.03.012
Ritchie, S. W., Nguyen, H. T., Holaday, A. S. (1990). Leaf water content and gas-exchange parameters of two wheat genotypes differing in drought resistance. Crop Sci. 30, 105–111. doi: 10.2135/cropsci1990.0011183X003000010025x
Sahu, C., Basti, S., Sahu, S. K. (2020). Air pollution tolerance index (APTI) and expected performance index (EPI) of trees in sambalpur town of India. SN Appl. Sci. 2, 1–14. doi: 10.1007/s42452-020-3120-6
Sahu, C., Sahu, S. K. (2015). Air pollution tolerance index (APTI), anticipated performance index (API), carbon sequestration and dust collection potential of Indian tree species–a review. Int. J. Emerg. Res. Manag. Technol. 4, 37–40.
Santoyo, G., Sánchez-Yáñez, J. M., de los Santos-Villalobos, S. (2019). Methods for detecting biocontrol and plant growth-promoting traits in rhizobacteria, methods in rhizosphere biology research. Springer pp, 133–149. doi: 10.1007/978-981-13-5767-1_8
Sarma, B., Chanda, S. K., Bhuyan, M. (2017). Impact of dust accumulation on three roadside plants and their adaptive responses at national highway 37, Assam, India. Trop. Plant Res. 4, 161–167. doi: 10.22271/tpr.2017.v4.i1.023
Setsungnern, A., Treesubsuntorn, C., Thiravetyan, P. (2018). Chlorophytum comosum–bacteria interactions for airborne benzene remediation: Effect of native endophytic enterobacter sp. EN2 inoculation and blue-red LED light. Plant Physiol. Biochem. 130, 181–191. doi: 10.1016/j.plaphy.2018.06.042
Shannigrahi, A. S., Fukushima, T., Sharma, R. C. (2004). Anticipated air pollution tolerance of some plant species considered for green belt development in and around an industrial/urban area in India: an overview. Int. J. Environ. Stud. 61, 125–137. doi: 10.1080/0020723032000163137
Sharifi, Z., Saeidi, M., Nosrati, E., Heidary, H. (2019). The effect of dust particles on grain yield and some of the physiological and biochemical characteristics of wheat in West of Iran. J. Plant Prod. (Agronom. Breed. Horticult.) 42, 149–164. doi: 10.22055/ppd.2019.21856.1470
Shirmohammadi, E., Alikhani, H. A., Pourbabaei, A. A., Etesami, H. (2020). Improved phosphorus (P) uptake and yield of rainfed wheat fed with p fertilizer by drought-tolerant phosphate-solubilizing fluorescent pseudomonads strains: a field study in drylands. J. Soil Sci. Plant Nutr. 20, 2195–2211. doi: 10.1007/s42729-020-00287-x
Singare, P. U., Talpade, M. S. (2013). Physiological responses of some plant species as a bio-indicator of roadside automobile pollution stress using the air pollution tolerance index approach. Int. J. Plant Res. 3, 9–16. doi: 10.5923/j.plant.20130302.01
Singh, S. K., Rao, D. N. (1983). Evaluation of plants for their tolerance to air pollution. Symposium on Air Pollution Control. 1983, 218–224.
Steubing, L., Fangmeier, A., Both, R., Frankenfeld, M. (1989). Effects of SO2, NO2, and O3 on population development and morphological and physiological parameters of native herb layer species in a beech forest. Environ. pollut. 58, 281–302. doi: 10.1016/0269-7491(89)90140-1
Taheri Analojeh, A., Azimzadeh, H.-R., Mosleh Arani, A., Sodaiezadeh, H. (2016). Investigating and comparing short period impact of dust on physiological characteristics of three species of pinus eldarica, cupressus sempervirens, and Ligustrum ovalifolium. Arabian J. Geosci. 9, 1–12. doi: 10.1007/s12517-015-2241-5
Tahir, H. A. S., Gu, Q., Wu, H., Raza, W., Hanif, A., Wu, L., et al. (2017). Plant growth promotion by volatile organic compounds produced by Bacillus subtilis SYST2. Front. Microbiol. 8, 171. doi: 10.3389/fmicb.2017.00171
Tak, A. A., Kakde, U. B. (2017). Assessment of air pollution tolerance index of plants: a comparative study. Int. J. Pharm. Pharm. Sci. 9, 83–89. doi: 10.22159/ijpps.2017v9i7.18447
Thompson, J. R., Mueller, P. W., Flückiger, W., Rutter, A. J. (1984). The effect of dust on photosynthesis and its significance for roadside plants. Environ. pollut. Ser. A. Ecol. Biol. 34, 171–190. doi: 10.1016/0143-1471(84)90056-4
Tiepo, A. N., Hertel, M. F., Rocha, S. S., Calzavara, A. K., De Oliveira, A. L. M., Pimenta, J. A., et al. (2018). Enhanced drought tolerance in seedlings of Neotropical tree species inoculated with plant growth-promoting bacteria. Plant Physiol. Biochem. 130, 277–288. doi: 10.1016/j.plaphy.2018.07.021
Treesubsuntorn, C., Setiawan, G. D., Permana, B. H., Citra, Y., Krobthong, S., Yingchutrakul, Y., et al. (2021). Particulate matter and volatile organic compound phytoremediation by perennial plants: Affecting factors and plant stress response. Sci. Total Environ. 794, 148779. doi: 10.1016/j.scitotenv.2021.148779
Trivedy, R. K., Goel, P. K. (2010). An introduction to air pollution (Telangana, INDIA: ABD Publishers).
Walia, K., Aggarwal, R., Bhardwaj, S. (2019). Leaf dust accumulation and its relationship with biochemical parameters of different plant species growing along national highway-22, India. Int. J. Chem. Stud. 7, 1386–1390.
Xu, J., Yang, J., Duan, X., Jiang, Y., Zhang, P. (2014). Increased expression of native cytosolic Cu/Zn superoxide dismutase and ascorbate peroxidase improves tolerance to oxidative and chilling stresses in cassava (Manihot esculenta crantz). BMC Plant Biol. 14, 1–14. doi: 10.1186/s12870-014-0208-4
Yadav, R., Pandey, P. (2020). Assessment of air pollution tolerance index (APTI) and anticipated performance index (API) of roadside plants for the development of greenbelt in urban area of bathinda city, punjab, India. Bull. Environ. Contamination Toxicol. 105, 906–914. doi: 10.1007/s00128-020-03027-0
Yaghmaei, L., Jafari, R., Soltani, S., Eshghizadeh, H. R., Jahanbazy, H. (2022). Interaction effects of dust and water deficit stresses on growth and physiology of Persian oak (Quercus brantii lindl.). J. Sustain. For. 41, 134–158. doi: 10.1080/10549811.2020.1845742
Zia-Khan, S., Spreer, W., Pengnian, Y., Zhao, X., Othmanli, H., He, X., et al. (2014). Effect of dust deposition on stomatal conductance and leaf temperature of cotton in northwest China. Water 7, 116–131. doi: 10.3390/w7010116
Zilaie, M. N., Arani, A. M., Etesami, H., Dinarvand, M., Dolati, A. (2022). Halotolerant plant growth-promoting rhizobacteria-mediated alleviation of salinity and dust stress and improvement of forage yield in the desert halophyte seidlitzia rosmarinus. Environ. Exp. Bot. 201, 104952. doi: 10.1016/j.envexpbot.2022.104952
Keywords: Bacillus pumilus, Haloxylon aphyllum, Nitraria schoberi, plant growth-promoting rhizobacteria, Seidlitzia Rosmarinus, Zhihengliuella halotolerans
Citation: Najafi Zilaie M, Mosleh Arani A and Etesami H (2023) The importance of plant growth-promoting rhizobacteria to increase air pollution tolerance index (APTI) in the plants of green belt to control dust hazards. Front. Plant Sci. 14:1098368. doi: 10.3389/fpls.2023.1098368
Received: 14 November 2022; Accepted: 27 February 2023;
Published: 10 March 2023.
Edited by:
Mohamed Lazali, University of Khemis Miliana, AlgeriaReviewed by:
Antonio Cellini, University of Bologna, ItalyHayssam M. Ali, King Saud University, Saudi Arabia
Copyright © 2023 Najafi Zilaie, Mosleh Arani and Etesami. This is an open-access article distributed under the terms of the Creative Commons Attribution License (CC BY). The use, distribution or reproduction in other forums is permitted, provided the original author(s) and the copyright owner(s) are credited and that the original publication in this journal is cited, in accordance with accepted academic practice. No use, distribution or reproduction is permitted which does not comply with these terms.
*Correspondence: Hassan Etesami, hassanetesami@ut.ac.ir; Asghar Mosleh Arani, amosleh@yazd.ac.ir