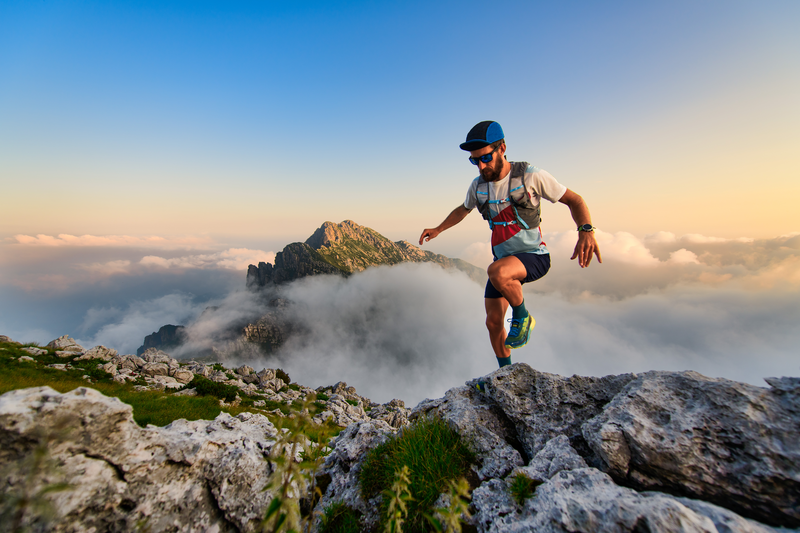
94% of researchers rate our articles as excellent or good
Learn more about the work of our research integrity team to safeguard the quality of each article we publish.
Find out more
ORIGINAL RESEARCH article
Front. Plant Sci. , 18 May 2023
Sec. Technical Advances in Plant Science
Volume 14 - 2023 | https://doi.org/10.3389/fpls.2023.1083177
This article is part of the Research Topic Advances in Breeding for Waterlogging Tolerance in Crops View all 6 articles
Direct-seeded rice (DSR) is a promising alternative to the traditional puddled rice system. It has become more popular among rice growers as a result of socioeconomic shifts and global climate change. Although DSR offers advantages, rice plants experience greater anaerobic stress at sowing from unpredicted rainfall. Rice is unique among cereals in its ability to germinate under anaerobiosis. The coleoptile of rice rapidly elongates above the water surface to obtain more oxygen and enhance vigorous seedling growth. A panel of 115 landraces and four check varieties were subjected to anaerobic stress with a water level of 10 cm for up to 15 days. The present study observed significant variation in anaerobic germination percentage (AGP) (10%–100%) and anaerobic vigor index (AVI) (150–4,433). Landraces Karuthakar, Poovan samba, Mattaikar, Edakkal, Manvilayan, and Varappu kudainchan were identified as genotypes tolerant to early water submergence. The shoot and root length of susceptible landraces were significantly lower than the tolerant landraces under hypoxia condition, implying that landraces with longer shoots and roots had a higher survival rate. The response index substantiated this. The results clearly show that tolerant and moderately tolerant landraces possessed higher mean values for root and shoot lengths than susceptible landraces. The landraces grouped under the long–bold category had superior AGP and AVI scores to other grain type groups. This raises the possibility that differences in kernel breadth, which is linked to grain type, could affect anaerobic germination potential. Molecular confirmation using gene-specific markers, viz., DFR, TTP_G4, RM478, RM208, and RM24161, for which the polymorphic information content (PIC) value ranged from 0.36 (RM478) to 0.68 (RM206) suggests that this diverse panel of landraces must be assessed further using advanced molecular tools to precisely clarify the genetic mechanism behind this phenomenon. The tolerant landraces thus identified may become donors in breeding programs. The introduction of these traits would contribute to the development of rice varieties tolerant to anaerobic stress, resulting in sustainable yields. This solution could promote the DSR system across the world.
Direct-seeded rice (DSR) is a cultivation method that has gained popularity across rice-growing regions in recent years. Contributing factors to this trend the include reduced costs of production, reduced water demand, reduced labor requirements, and early maturity. Particularly in Asia, vast areas once covered by puddled transplant systems have adopted DSR systems (Ghosal et al., 2020). However, sudden rain and consequent flooding immediately after sowing or irrigation with saline water often produces anaerobic stress in plants cultivated by the DSR system (Islam et al., 2022). The seeds undergo hypoxia/anoxia, which in turn decelerates germination and seedling establishment due to the failure of adequate shoot and root formation. This phenomenon frequently results in complete crop failure (Ismail et al., 2009; Miro et al., 2017; Lal et al., 2018). Anaerobic stress has been identified as a major barrier to the broader adoption of DSR. Anaerobic stress results in poor germination and inadequate crop establishment, and is caused by unlevelled fields, unpredicted heavy rains after sowing, and severe weed infestation. Therefore, the wider adoption of DSR requires the development of rice varieties possessing the ability to germinate well under anaerobic stress and tolerate flooding at the time of germination.
Rice (Oryza sativa L.) is a semiaquatic plant (in contrast to other cereals), with its potential to germinate under hypoxic conditions due to its α-amylase genes (Guglielminetti et al., 1995; Hwang et al., 1999). These genes enable the seedlings to cope with the low levels of oxygen and sugar starvation that occur during hypoxia. Complete flooding at the time of germination leads to anoxia and results in poor or no germination. Rapid shoot elongation is a mechanism to escape anoxia. It contributes to anaerobic germination tolerance (AGT). In this way, seedlings can reach the water surface and diffuse O2 to elongate the roots and shoots (Ismail et al., 2009; Kretzschmar et al., 2015). Adaptive mechanisms and some of the key traits associated with anaerobic stress tolerance include anaerobic germination percentage (AGP) (Septiningsih et al., 2013; Barik et al., 2019), anaerobic vigor index (AVI) (Barik et al., 2019), response index (RI) (Islam et al., 2022), rapid elongation of the coleoptile (Kretzschmar et al., 2015), and carbohydrate reserves (Ella et al., 2011; Ismail et al., 2012).
Quantitative trait loci (QTL) mapping studies have identified numerous QTLs that govern major and minor effects of AGT (Jiang et al., 2006; Angaji et al., 2010; Septiningsih et al., 2013; Ghosal et al., 2019; Ghosal et al., 2020). QTL qAG9-2 has been identified with 33.5% phenotypic variance on chromosome 9 (Angaji et al., 2010) and was fine mapped to the gene OsTPP7 (Kretzschmar et al., 2015). This QTL is responsible for starch mobilization and favors the elongation of the coleoptile under anaerobic stress. Other QTLs were also identified as controlling AGT, such as qAG7.1 with 31.7% phenotypic variance (Septiningsih et al., 2013) and qAG7 with 22.3% phenotypic variance (Baltazar et al., 2014). To transfer the target loci into superior breeding lines, DNA markers closely linked with the target gene or QTL should be effective. Previously, DNA markers for AGT, such as RM 24161 and TTP_G4 for AG1, RM 3475 for qAG1–2 (Angaji et al., 2010), DFR for qAG9–2 (Kretzschmar et al., 2015), RM 478 for AG2 (Kim et al., 2019), and RM 341 and RM 206 for AGT (Reddy et al., 2015), were developed and are being used in marker-assisted breeding programs (Kim et al., 2019).
Genetic variation is the basis for any breeding program. It provides the breeder with pre-breeding material from which to choose (Palaniyappan et al., 2020). The landraces are called the ‘treasure of breeders’ as they harbor numerous varieties with diverse traits (i.e., in the case of this work, biotic and abiotic stress tolerance, nutrition, cooking, and quality traits). The genetic diversity of the rice germplasm, including the landraces, is relatively greater than other crop varieties. Being a primary center of origin for rice, India possesses more than 200,000 diverse rice varieties (Sathya, 2013). More than 10,000 popular indigenous rice accessions have been widely cultivated in India for millennia. Munagada (submerged) is a special landrace widely cultivated in the northern districts of Tamil Nadu, India. It can germinate in flood conditions and grow up to 3 feet in height (Stuart, 1895). The widespread cultivation of high-yield cultivars in a monoculture system has reduced the availability of most of the diverse indigenous rice landraces. Recent studies on anaerobic germination (AG) have identified several indigenous landraces with AGT such as MTU 1140 (Reddy et al., 2015); Bausaganthi, Patadhan, and Basantichudi (Barik et al., 2019); Barkhe Tauli, 498-2 A BR 8, Jagli Boro, ParaNellu, and Improved Blue Rose (Rauf et al., 2019); and Vellai kavuni, Varappu kudaichan, Norungan, and Karuppu kavuni (Mohanapriya et al., 2022). The application of intensive phenotyping protocols can validate the anaerobic germination tolerance of these genotypes. Breeders are encouraged to mine the existing diversity of rice varieties and identify novel potential donors for AGT.
The current study comprises the screening of a panel of 115 landraces along with four control varieties subjected to hypoxia to identify novel genotypes with AGT for further use in breeding programs. AGT-linked molecular markers have also been used in this study to screen the genotypes for allelic diversity associated with the targeted trait. We sought to identify traits associated with AG potential in the rice varieties, elucidate their physiological mechanisms, and document genetic and molecular variability so as to identify novel alleles.
A panel of 119 genotypes (Supplementary Table 1) containing 115 native landraces from south India and four control varieties, viz., ‘FR13 A’, ‘IR 42’, ‘CO 43’, and ‘CO 43 Sub1’, was assembled for this study. Seeds of the selected indigenous landraces were collected from farmers, and raised and purified during the Rabi season in 2020 at the Tamil Nadu Rice Research Institute (TRRI), Aduthurai, Tamil Nadu, India (10°99'85"N and 79°48'01"E). These uniform healthy seeds were screened for AGT. Morphological traits such as kernel length (KL), kernel breadth (KB), length-to-breadth ratio (LBR), and 100-seed weight (HSW) were recorded for the selected genotypes. Grains of the selected genotypes were classified into different grain types as per the literature (Ramaiah, 1969) (Supplementary Table 2).
The anaerobic germination tolerance experiment was conducted on 20 September 2021 and 4 October, 2022 on the 119 selected genotypes at the Tamil Nadu Rice Research Institute during Kharif. Uniformly sized and well-filled seeds were surface sterilized with 0.1% HgCl2 solution for 5 min followed by 10 min of thorough washing with distilled water. The sterilized seeds were allowed to germinate under laboratory conditions in Petri dishes using wet germination paper. Five pre-germinated seeds were selected from each genotype, and were sown 1.0 cm below the surface of the soil in fine clay soil-filled cups (measuring 10 × 8 cm) in a plastic tray (sized 60 × 30 × 40 cm) with three replicates. Cups were then immediately submerged in water 10 cm above the soil surface, where they were held for 15 days (Figure 1). A control batch in which the soil surface was suitably moistened instead of completely submerged was established. After 15 days of hypoxia, AGP was measured as the number of emerged seedlings. For each replicate, the characteristics associated with seedling vigor were recorded for five control and five experimental seedlings, comprising shoot length (SL), root length (RL), shoot-to-root ratio (SRR), number of leaves (NOL), number of roots (NOR), fresh weight (FW), and dry weight (DW). Anaerobic Vigor Index (AVI) was calculated using the formula given by Barik et al. (2019):
Figure 1 Anaerobic germination screening experiment (A). Landraces Poovan Samba (B), Karuthakar (C), Edakkal (D), ‘FR13 A’ (E), and ‘IR 42’ (F) compared under controlled and stressed environments.
The anaerobic response index (RI) was calculated using the formula proposed by Islam et al. (2022).
Total genomic DNA was isolated for all the genotypes using the CTAB (cetyltrimethylammonium bromide) method (Murray and Thompson, 1980). The quantity of the DNA was determined using a spectrophotometer based on an A260/A280 ratio. DNA was diluted with molecular-grade water before performing PCR and conducting electrophoretic analysis.
The allelic status of the AGT genotypes was examined using inserations and deletions (InDels) and simple sequence repeats (SSR) markers linked to AGT. The details of the markers used in this genotypic analysis are given in Table 1. PCR amplification was conducted in a Veriti master cycler with 10 µL of PCR reaction mixture containing 1 µL (25 ng/µL) of genomic DNA, 1 µL of SSR primer (2 mM), and 8 µL of the commercial PCR master mix (1×). Initial denaturation was at 94°C for 5 min, which was then followed by 35 cycles of denaturation at 94°C for 30 s, annealing at 55°C for 30 s, extension at 72°C for 1 min, and a final extension at 72°C for approximately 10 min. The amplified PCR products were then resolved in a 2.5% agarose gel and documented using the Syngene G: BOX F3 system. Amplified bands of each marker locus were scored according to the presence or absence of the bands in each of the studied rice genotypes. Genetic diversity parameters, such as the number of alleles and polymorphic information content (PIC), were calculated using the PowerMarker Ver3.25 program (Lu et al., 2005).
An analysis of variance (ANOVA) for traits associated with AG potential was conducted with R studio software (Racine, 2012) using the “agricolae” package (de Mendiburu and de Mendiburu, 2019). The Newman–Keuls test (Newman, 1939; Keuls, 1952) was used to compare the group means of the grain type and AGT, using the ‘agricolae’ package. Correlation between traits was estimated using the ‘corrplot’ package (Wei et al., 2017). Principal component analysis (PCA) was conducted using the ‘FactoMineR’ and ‘factoectra’ packages. Phenotypic clustering was performed using the Ward.D2 method with the help of Gower’s distance matrix (Murtagh and Legendre, 2014), whereas the significance of the cluster means was determined using the Newman–Keuls test. The Nei distance matrix was estimated and the Ward.D2 method of clustering was used to group the genotypes based on their molecular information. Based on per se performance, promising genotypes for AGT were identified.
This study investigated variations in the physical characteristics and anaerobic germination potential of native south Indian landraces under normal and hypoxic conditions to assess the tolerance of these landraces to flooding at the time of germination. The variance analyses conducted on the anaerobic germination-associated traits of the 119 genotypes revealed highly significant genotypic variance within each individual year and in the pooled data over the years, except in the case of the fresh and dry weights of the seedlings (Table 2). A significant variation (p< 0.01) was found for AGP, ranging from 10% (Samba mosanam) to 100% (Karuthakar), and AVI ranged from 150 (Samba massanam) to 4,433 (Poovan samba) (Table 3). Shoot lengths of the germinating seedlings varied from 5.14 cm (Samba mosanam) to 35.76 cm (Poovan samba), whereas the root length varied from 1.69 cm (Salem samba) to 11.63 cm (Iravai pandi). The response index ranged from –1.92 (Thillainayagam) to 17.42 (Edakkal). The traits SRR (1.09–12.29), NOL (0.67–2.56) and NOR (2.40–11.41) showed considerable variation. Significant variations were found in morphological characteristics (KL, KB, and LBR) and test seed weight (p< 0.01) among the landraces studied. The KL, KB, LBR, and grain weight ranged from 4.00 (Kothamalli samba) to 9.70 mm (Chinnar), 1.60 (Jai Sri Ram) to 3.80 mm (Thondi), 1.67 (Kothamalli samba) to 3.95 (Chinna punchai), and 0.78 (Thulasi vasanai) to 3.65 g (Thondi), respectively, among the studied landraces.
Table 2 Estimated variance components for traits with anaerobic germination percentage of indigenous rice landraces evaluated in 2021 and 2022 at Tamil Nadu Rice Research Institute, Aduthurai, Tamil Nadu, India.
Table 3 Genetic variability parameters of studied traits of indigenous rice landraces germinating under anaerobic conditions.
The phenotypic coefficient of variation (PCV), genotypic coefficient of variation (GCV), heritability, and genetic advance (GA) were evaluated for the 119 rice genotypes. The PCV values ranged from 15.38% (LBR) to 88.15% (DW), whereas GCV ranged from 15.14% (LBR) to 71.06% (AVI). The PCV value was higher than the GCV value for all studied traits. This finding demonstrates the predominance of environmental interaction in determining the expression of these traits. AGT-associated traits, such as AGP, AVI, SL, and RL, had a high PCV coupled with strong GCV values. Broad-sense heritability varied widely from 18.23% to 98.85%. Traits associated with AG potential, such as AGP, AVI, RI, SL, and RL, along with seed morphological traits such as KL, KB, LBR, and HSW, had high heritability. Genetic advance as a percentage of means ranged from 12.87% (SRR) to 540.89% (RI). Traits such as AGP, AVI, SL, RL, RI, and grain weight displayed a high genetic advance as a percentage of mean (GAM) and high heritability.
Morphological characteristics of the grain, viz., KL, KB, LBR, and HSW, varied widely (Table 4). The studied landraces were grouped into five groups, viz., short–bold, short–slender, medium–slender, long–bold and long–slender (Ramaiah, 1969). Out of the total 119 genotypes, 10 were grouped under the short–bold type, whereas nine landraces were classified under the short–slender grain type. Thirteen landraces were grouped under the medium–slender type and 24 landraces were placed in the long–slender group. The majority, i.e., 63 landraces, were classified under the long–bold grain type.
Table 4 Pooled mean values of traits associated with anaerobic germination potential and grain morphology of native rice landraces.
Anaerobic germination percentage is a key trait to identify the genotypes that are tolerant to hypoxia under the DSR cultivation system. Samba masanam possessed the least AGP (10%), followed by Salem samba, Maranellu, and ‘IR 42’ (13.33%). Karuthakar displayed the maximum AGP (100%) after 15 days of hypoxia. On the basis of AGP, the landraces were divided into four categories, as proposed by Barik et al. (2019). Landraces displaying an AGP of ≥ 90% were categorized as “tolerant landraces”; 11 landraces were grouped under this category. With an AGP of 71%–89%, 36 landraces were grouped as “moderately tolerant landraces”. The 43 landraces with an AGP of 41%–70% were identified as “moderately susceptible landraces”. A total of 29 landraces had an AGP of ≤ 40% and were classified as “susceptible landraces”. Control varieties ‘FR13 A’, ‘CO 43’, and ‘CO 43 Sub1’ were found to possess an AGP of 41%–70% and were identified as moderately susceptible, whereas ‘IR 42’ was categorized as susceptible with an AGP of 13.34%. Under non-stressed, aerobic-controlled conditions, all 119 genotypes achieved 100% germination.
Shoot elongation under anaerobic stress is another important adaptive mechanism that helps emerging seedlings tolerate early-stage submergence. The response index (RI) is generally used to identify the genotypes with shoot elongation under stress compared with the control environment. RI values ranged from –11.92 (Thillainayagam) to 17.42 (Edakkal). Thirty-six landraces scored as susceptible or moderately susceptible had RI values less than zero. This finding implies a reduction of shoot length under hypoxia. In addition, AVI was calculated based on a seedling’s growth characteristics and AGP. Landraces Poovan samba (4,433), Karuthakar (4,206), Mattaikar (4,117), and Edakkal (4,046) had higher AVI values than the susceptible landraces Samba massanam (150), Salem samba (250), Maranellu (260), and ‘IR 42’ (279).
In the present study, 119 genotypes were grouped on the basis of the grain’s physical traits and traits associated with AG potential. Trait means were compared among different grain-type groups and AGT groups (see Table 5). Among the grain-type groups, the study compared the means of the selected traits among long–bold, long–slender, medium–slender and short–bold groups. The landraces from the short–slender group were also included in the short–bold group, because both short–slender and short–bold groups had so few accessions (i.e.,< 10). The mean AGP of the long–bold landraces was 67.72%, which was significantly higher than the mean AGP of the other grain-type groups (47.72%). The long–bold grain type group also achieved a significantly higher AVI and shoot length than the rest of the grain type groups. Conversely, the lowest AGP and AVI values were recorded for the short–bold grain-type group (Figure 2). No significant difference was found among the landraces of different grain-type groups in terms of root length, shoot-to-root ratio, number of leaves, number of roots, fresh weight, dry weight, or response index. However, the tolerant and susceptible categories differed significantly in terms of AGP, AVI, SL, RL, RI, HSW, KB, and LBR. The accessions in the tolerant and moderately tolerant categories had an AGP of more than 78.98% and an AVI of 2816, which were significantly higher than the susceptible categories. Furthermore, the accessions in the susceptible group had an AGP of 30.80% and an AVI of 801. The shoot length, root length, response index, and grain weight of the landraces in the tolerant and moderately tolerant groups were significantly different from those of the susceptible groups. Likewise, the kernel breadth and LBR values of the tolerant group were significantly different from the susceptible categories.
Table 5 Mean comparison of grain type and tolerance reaction of 119 rice landraces by anaerobic germination tolerance-associated trait.
Figure 2 Boxplot showing the variability of anaerobic germination tolerance -associated traits among different grain types. (A) Anaerobic germination percentage (%), (B) anaerobic vigor index, and (C) from left to right, response index, root length (cm) and shoot length (cm).
In this study, a simple correlation analysis was conducted between the seedlings’ morphological traits and the early-stage submergence tolerance traits, such as AGP and AVI, among the genotypes under study. The AGP and AVI (r = 0.96***, p< 0.001) values were found to be significantly and positively associated with RI, shoot and root length, number of leaves and roots, grain breadth, and HSW. However, a positive and non-significant association was observed with grain length (Figure 3). Shoot length had a strong positive association with root length (r = 0.72***, p< 0.001) under hypoxic conditions. A significant negative correlation was observed between grain length–breadth ratio and AGP (r = –0.21*, p< 0.05) and AVI (r = –0.20*, p< 0.05). Furthermore, LBR exhibited a strong negative association with kernel breadth (r = –0.52***, p< 0.001) and grain weight, and a positive significant correlation with grain length. A highly significant association under anaerobic treatment was found between RI and the following traits: SRR, shoot length, and number of leaves. The association among shoot length, root length, SRR, and number of roots was significant and positive. Similarly, both grain length and breadth were positively correlated with grain weight.
Figure 3 Correlogram showing association between grain morphology traits and traits associated with anaerobic germination potential. AGP, anaerobic germination percentage; SL, shoot length; RL, root length; SRR, shoot-to-root ratio; NOL, number of leaves; NOR, number of roots; FW, fresh weight; DW, dry weight; AVI, anaerobic vigor index; RI, response index; KL, kernel length; KB, kernel breadth; LBR, length-to-breadth ratio; HSW, hundred-seed weight.
The phenotypic data for 14 morphological traits under hypoxia was used to conduct the principal component analysis to assess the contribution of the individual traits to total variation and to analyze the genetic variations among the native landraces. The first five principal components (PCs) with an eigenvalue of > 1 accounted for 79.15% of the total variation (Supplementary Figure 1). Among them, PC1, with an eigenvalue of 4.69, accounted for 33.53% of the variation followed by PC2, which accounted for 13.82% of the variation (Table 6). In PC1, AVI exhibited the highest positive value with a loading of 0.892, followed by shoot length (0.850), AGP (0.819), root length (0.664), and RI (0.497). Traits associated with AG potential, such as AVI, AGP, RI, shoot and root length, and grain weight, were key traits contributing to the total variation exhibited by PC1. In PC2, both fresh and dry weight contributed to the high variation. KL, KB, and HSW for PC3 and SRR for PC4, accounted for 0.503% and 10.24% of the total variation, respectively. Variations contributed by PC5 (9.06%) were driven by LBR. The results of the loading and biplot analysis revealed that traits such as AGP, AVI, RI, shoot length, root length, HSW, and grain breadth were crucial traits that contributed to the total variability of the landraces, whereas the rest of the traits contributed minimally toward the phenotypic variability (Figure 4).
Table 6 Principal components of traits associated with anaerobic germination potential for rice landraces studied under anaerobic stress.
Figure 4 Biplot principal component analysis of native rice landraces for principal component 1 vs. principal component 2. The biplot depicts the distribution patterns of indigenous accessions into four different quadrants based on the traits associated with anaerobic germination tolerance and clearly separating tolerant genotypes from susceptible genotypes. Color gradients represent the contribution of variables toward the phenotypic variance of the corresponding principal component. Supplementary Figure 2 shows the exact position of each genotype on the biplot.
Biplot analysis between PC1 and PC2 explains the distribution and nature of the diversity based on the variables as well as genotypes (Figure 4 and Supplementary Figure 2). The landraces considered in this study were efficiently divided into four quarters by the biplot of first two PCA components. These in turn divided most of the AG-resistant and susceptible (IR42) landraces into distinct quadrants. Highly tolerant landraces, such as Karuthakar, Edakkal, and Varappu kudainchan, were clustered within the first quadrant (top left), whereas Poovan samba, Mattaikar, Mandamaranellu, and Manvilayan were grouped within the fourth quadrant (bottom left). Furthermore, cultivars ‘IR42’, ‘FR13 A’, ‘CO 43’, and ‘CO 43 Sub1’ were placed in the second quadrant (top right). The first and the fourth quadrants together comprised all of the tolerant and moderately tolerant landraces, except Karnel (second quadrant) and Chittimutiyalu (third quadrant), with high AGP, AVI, RI, and shoot and root length values along with an LBR ratio of > 2.5. The second and third quadrants encompassed the majority of the susceptible landraces, including ‘IR 42’. Landraces such as Samba Massanam (10%), Maranellu (13.33%), and Salem samba (13.33%) were highly susceptible and belonged to the most divergent cluster in the second quadrant, which distinguishes them from the rest of the landraces.
The Ward.D2 cluster analysis method was applied to the indigenous rice landraces based on the traits associated with AG potential to group the landraces into six distinct clusters (Figure 5). The number of landraces in each group varied between four in clusters I and IV to 35 in cluster III (Table 7). Cluster IV comprised four tolerant landraces viz., Karuthakar, Poovan samba, Mattaikar and Edakkal, with the highest mean values for AGP (97.50%), AVI (4,200.28), SL (33.36 cm), RL (9.53 cm), RI (12.82), NOL (2.20), and NOR (6.95) compared with other clusters. Cluster VI, included 19 moderately tolerant landraces and seven tolerant landraces (≥ 90% AGP), such as Manvilayan, Mandamaranellu, Varappu kudainchan, Varisuriyan, Mohini samba, Katta samba, and Kaan, possessed a high mean value for SRR (4.29). The majority of landraces with high values for AGP-associated traits were in clusters IV and VI. These landraces possessed high values for AGP (> 73.33%), AVI (2,834–4,433), shoot length (25.11–35.76 cm), and RI. Cluster V had 26 landraces, 17 of which were identified as moderately tolerant (> 71% of AGP) and nine as moderately susceptible. Cluster III had 35 genotypes, forming the largest cluster, with an AGP of 16.67%–56.67%, and including ‘FR13 A’, ‘CO 43’, and ‘CO 43 Sub1’. Cluster II included 24 landraces, whereas susceptible control variety ‘IR 42’ clustered with other susceptible landraces Maranellu, Salem samba, and Samba masanam in cluster I. The mean values of each cluster significantly differed for all traits, except kernel length, kernel breadth, and grain weight. On average, the phenotypic within-cluster distance was found to be highest in cluster IV, followed by clusters I and II; cluster V had the lowest mean distance (Supplementary Table 3). The highest average distance was observed between clusters V and VI, followed by clusters II and VI; the lowest distance was observed between clusters II and III.
Figure 5 Dendrogram showing similarity index for the 119 native landraces based on traits associated with anaerobic germination potential. Colored branches indicate clusters I to VI (from left to right).
Table 7 Mean comparison of anaerobic germination tolerance-associated traits among the 119 indigenous rice landraces grouped in five clusters.
Thirteen alleles ranging from two to four alleles per locus were detected with the four gene-specific markers linked to AG tolerance (Figure 6). The average number of alleles per locus was 3.25. The PIC value for each marker locus enabled us to understand the polymorphism level among the landraces; PIC values ranged from 0.359 to 0.681, with an average of 0.496 per locus (Table 8). Marker RM 24161 was monomorphic among the studied landraces.
Figure 6 Representation of gel images of gene-linked markers. (A) DFR, (B) TTP_G4, (C) RM 206, (D) RM 478, and (E) RM 24161.
We conducted a single-factor ANOVA-based single-marker analysis to validate genetic associations among four polymorphic markers and the traits associated with AG potential. Statistically, five significant genetic associations were observed for four traits (SRR, KB, LBR, and HSW) (Table 9). These five significant marker–trait associations (MTAs) accounted for 6.28%–19.46% of the total observed phenotypic variance (R2). SRR showed a significant association (p< 0.05) with RM478 (R2 = 6.28%). Similarly, KB and LBR showed a highly significant association (p< 0.01) with RM206. HSW had a significant genetic association with two polymorphic markers (RM478 and RM206).
The 119 genotypes considered for the investigation were grouped into four clusters ranging from 16 landraces (cluster II) to 49 landraces (cluster IV) (Supplementary Figure 3). Cluster I included 33 landraces, whereas cluster III included 21. Cluster II included the majority of susceptible landraces, including ‘IR 42’ and ‘FR 13A’. Karuthakar and Poovan samba, in cluster III, formed a distinct sub-cluster. Cluster IV displayed a higher mean distance (3.73). Inter-cluster distance was highest between clusters I and IV, followed by the clusters I and III (Table 10).
Rice is widely consumed across the globe, accounting for 43% of overall food grain production in globe and 46% of cereal production in India (Mohanapriya et al., 2022). Rice productivity is heavily affected by abiotic stressors; thus, climate change and global warming present a risk to this important food source. Flooding is a serious problem that can cause complete crop failure. Within one decade, the flood-affected landmass in India increased from 19 to 40 Mha, i.e., from 12% to 25% of the total cultivable landmass (Kuanar et al., 2017). Deep-water, rain-fed lowland ecosystems constitute approximately 50 million hectares of land worldwide and account for one-third of global rice production areas (Oladosu et al., 2020). Thus, screening rice cultivars for AGT identifies early-stage flood-tolerant genotypes and their genetic potential. In this study, a diverse panel of rice landraces originating in southern Indian were collected and were conserved at TRRI, Aduthurai, Tamil Nadu, India. From the genotypes, 119 were studied for AGT under hypoxic conditions to identify promising cultivars for AGT improvement.
The statistical analyses of this work revealed a significant and wide range of variation among all the traits associated with anaerobic germination potential, except the fresh and dry weights of the seedlings. However, morphological traits of the grain, such as KL, KB, LBR, and HSW, which are governed by additive genes, showed high heritability (> 95%), suggesting that selection would be effective based on these traits. Furthermore, Vikram et al. (2016) and Barik et al. (2019) reported heritability > 60% for grain physical traits among indigenous rice landraces. AGT-associated traits were found to be highly heritable, except for SRR and NOL, which were grouped under low heritability. This analytic output suggests that these traits are complex in nature and are highly influenced by the environment. Earlier studies also found that traits associated with anaerobic germination potential had moderate-to-high heritability (Darko Asante et al., 2021). Of the 14 traits studied, 10 had an h2 > 0.60, indicating that phenotypic selection is effective for these traits under anaerobic stress. Traits such as RI, AVI, AGP, SL, RL, and HSW had a high genetic advance as a percentage of mean (GAM) coupled with high heritability. High heritability plus high genetic advance would be the best genotype selection indicators (Afrin et al., 2017). Thus, these traits should be given highest priority in improving anaerobic germination potential during crop breeding programs.
It is crucial to select rice genotypes with high seedling vigor for germination under anaerobic conditions in a DSR system. Among the 119 genotypes studied, the landraces Karuthakar, Poovan samba, Mattaikar, Edakkal, Manvilayan, Mandamaranellu, Varappu kudainchan, Varisurian, Katta samba, Kaan, and Mohini samba were found to have > 90% AGP, and are regarded as AGT landraces. Angaji et al. (2010); Barik et al. (2019), and Darko Asante et al. (2021) also studied rice accessions for AG tolerance. They reported that genotypes with > 90% AGP are AGT genotypes. The current investigation revealed considerable variations in AVI values (150–4,433) among the genotypes, which were greater than previous studies that reported ranges of 81–1,720 (Barik et al., 2019) and 0–870 (Mohanapriya et al., 2022) for AVI values. High AGP coupled with maximum AVI are the best indicators for AGT in rice (Mohanapriya et al., 2022). Indigenous landraces such as Karuthakar, Poovan samba, Mattaikar, and Edakkal were found to possess high AGP and AVI values.
This study observed significant differences between the control and experimental groups in terms of growth characteristics under hypoxic conditions. Susceptible landraces exhibited a significant reduction in both shoot and root length under hypoxia when compared with the tolerant landraces (Figure 7). Similar results were also obtained for the fresh and dry weights of the seedlings. Tolerant landraces possessed significantly higher shoot lengths under stress than the control genotypes. Shoot elongation is the result of a switch from high energy-demanding cell division to low energy-demanding cell growth (Atwell et al., 1982). During this phenomenon multiple processes occur, including the synthesis of cell walls and the uptake of solutes, which are less energy-demanding than protein synthesis. This might be due to the production of ethylene, which in turn triggers rapid shoot elongation in the germinating seeds in order to escape the hypoxia condition (Ismail et al., 2009; Darko Asante et al., 2021). The elongating coleoptiles behave as snorkels; they come into direct contact with air and facilitate gas exchange, enabling the embryos to develop (Mondal et al., 2020). It was also noticed in this study that the landraces with longer shoots had higher AGP values. This condition is primarily due to the rapid elongation of the shoots under submergence; rapid shoot elongation has been identified as an indicator of AGT (Ismail et al., 2009; Barik et al., 2019; Darko Asante et al., 2021). The high mean values for response index expressed by the tolerant and moderately tolerant groups further substantiate the importance of shoot elongation among AGT groups. Susceptible landraces exhibited reduced root length under hypoxic conditions, whereas tolerant and moderately tolerant (AGP > 70%) landraces possessed significantly higher root length under hypoxia than the controls. Edakkal, Karuthakar, and Varappu kudainchan minimized root length reduction and expressed higher shoot length. AGP and AVI when compared among the other tolerant genotypes which were expressing root elongation under hypoxia. Genotypes ‘FR13 A’ and ‘CO 43’ with the Sub1 locus recorded lower shoot length and RI than the AGT landraces. This finding infers that the Sub1 gene introgressed genotypes that were unable to cope with submergence during the germination process, despite being tolerant to vegetative-stage submergence. Sub1 locus-conferring genotypes undergo a quiescent strategy (Fukao et al., 2006) that conserves carbohydrates (Ella et al., 2003; Sarkar and Bhattacharjee, 2011; Darko Asante et al., 2021). These genotypes resume growth by activating and using ethylene production rather than shoot elongation after submergence subsides. Although the Sub1 locus is not crucial for anoxic rice growth, it provides an insight into why submergence-stimulated elongation occurs in environments with oxygen (Magneschi and Perata, 2009). Thus, it is evident that the mechanisms of tolerance to submergence at the germination and vegetative stages differ.
Figure 7 Boxplot depicting the shoot and root length of different tolerance group genotypes under controlled and hypoxia conditions. (A) Shoot length and (B) root length. The same letters on boxplots are not significant at p ≥ 0.05 while different letters are significant at p ≤ 0.05 based on the Newman–Keuls test.
The per se performance of indigenous landraces according to grain-type group and the tolerance group demonstrated differences in traits associated with anaerobic germination potential. Among the grain-type groups, the landraces from the long–bold category exhibited superior AGP and AVI compared with the other grain types. Similarly, tolerant and moderately tolerant landraces largely belonged to the bold grain type and had higher KB, LBR, and grain weight than susceptible landraces. This raises the possibility that differences in kernel breadth, linked to grain type, can affect anaerobic germination potential. Reports in the literature indicate that accessions with round-medium grain shape and with low apparent amylose content express higher shoot length and survival rates (Biselli et al., 2014; Nghi et al., 2019).
The highest association was observed between AGP and AVI, followed by shoot length with AVI and shoot length with AGP. Under anaerobic conditions, longer shoot length is associated with higher survival rates. Barik et al. (2019) and Darko Asante et al. (2021) reported a direct relationship between shoot length and survival percentage. Furthermore, due to a strong and positive correlation between these attributes, the enhancement of one trait improves the other. Among seed morphological traits, KB was positively associated with AGP and AVI, whereas LBR was negatively associated with AGP. Thus, an anaerobic vigor index would be a reliable selection tool for improving the anaerobic germination potential of rice genotypes.
Principal component analysis measures the contribution of each component to total variance (Sinha and Mishra, 2013). This measure can be used to identify traits with a significant impact on phenotypic variability (Ray et al., 2013). The current study revealed that the most discriminatory traits were AVI, AGP, shoot length, RI, KB, and grain weight. Earlier studies also reported traits such as AGP, AVI, and shoot length (Miro et al., 2017; Barik et al., 2019; Sudeepthi et al., 2020; Darko Asante et al., 2021), and fresh and dry weight (Barik et al., 2019; Sudeepthi et al., 2020) to be discriminating traits. Future landrace selection should be based on these traits to attain greater genetic gain in AGT breeding programs.
In this study, landraces were clustered on the basis of traits associated with anaerobic germination potential. The 119 rice genotypes were clustered into six different clusters containing between four (clusters I and IV) and 44 (cluster V) landraces each. Of the 47 tolerant landraces (> 70% AGP) identified, 30 (63.8%) were grouped in clusters I and VI. The landraces in clusters I and VI all exhibit long–bold grain types, except Uppu milagai, which exhibits medium–slender grains. This implies the possibility of a direct relationship between grain type and AGT. The clustering of indigenous landraces revealed that susceptible and tolerant landraces alike formed different distinct clusters. Our study discovered no duplicate genotypes, suggesting that landraces possess a high degree of difference in anaerobic germination potential (Barik et al., 2019). Hybridization would be effective if genotypes are selected from diverse clusters. Tolerant landraces studied here are good candidates for breeding programs attempting to develop rice varieties with AGT. These findings can also be exploited to generate novel recombinants for anaerobic germination potential; these in turn can reveal underlying genetic mechanisms and map the novel QTLs associated with AGT traits.
The two InDel and three SSR markers used in this study were selected based on earlier reports that investigated AGT (Kretzschmar et al., 2015; Reddy et al., 2015; Kim et al., 2019). Clustering based on molecular information grouped the studied landraces into four clusters, with all clusters comprising susceptible to tolerant landraces. MTAs offer clues regarding the presence of trait-linked QTLs/genes in diverse genetic backgrounds. In this research, single-marker analysis indicated significant (p< 0.05) associations for HSW with RM478 and RM206. Conversely, only the single marker RM206 could be significantly associated with KB and LBR. Higher phenotypic variance values of significant markers indicate that they control a considerable amount of genetic variation in grain dimensions and could be reliable genetic markers for the further improvement of rice grain shape. For AGT-associated traits, only SRR had a significant association with RM478 when compared with other traits. In marker-assisted breeding programs, a strong MTA is preferred over a weak MTA to effectively exploit that particular marker for trait improvement. Hence, further analysis using a diverse set of polymorphic markers dispersed over the entire genome or next-generation sequencing would help to identify strong MTAs for AGT-associated traits that could be exploited in future breeding programs to develop an AGT varieties.
In this study, promising trait-specific landraces were identified for traits associated with anaerobic germination potential and were compared with grain type. The genotypes that were identified as having anaerobic germination potential registered significantly higher values for the corresponding traits than the overall mean. Among the studied landraces, Karuthakar, Poovan samba, Mattaikar, Manvilayan, Edakkal, and Varappu kudainchan are promising candidates for traits associated with anaerobic germination potential. Salient agronomic features of these AGT landraces are reported in Supplementary Table 4. Early seedling vigor analysis outcomes suggest that Karuthakar, Poovan samba, and Mattaikar possess significant sheath and seedling elongation characteristics (Akshaya et al., 2020). These accessions can be used as parental lines that can be developed and released as AG-tolerant cultivars in breeding programs. Most tolerant accessions identified as having traits associated with anaerobic germination potential belonged to the long–bold grain-type group.
The development and use of AG-tolerant rice varieties has drawn considerable interest recently; such varieties will promote food supply and rice production stability during climate change and global warming. The outcomes of this study provide the possibility of improving rice cultivars with AGT, as these germplasms provide wider genetic variations for traits associated with anaerobic germination potential. Modern breeding tools based on sequencing approaches and genomic selection will enable crop breeders to target desirable traits in chromosomes, and identify and elucidate the genetic mechanisms at play. Genome-wide association studies would be the ideal way to study such a diverse panel of landraces. They also provide the possibility of discovering superior AG-tolerant alleles or novel genes, which may offer tolerance to early submergence during germination. This trait can reduce crop loss occurring after persistent flooding in flood-prone areas during monsoons. The current study identified novel sources of AGT in landraces Karuthakar, Poovan samba, Mattaikkar, Edakkal, and Manvilayan, which can be used as donors in future breeding programs. This may in turn pave the way for understanding the genetic mechanisms that underlie anaerobic germination tolerance.
The original contributions presented in the study are included in the article/Supplementary Material. Further inquiries can be directed to the corresponding authors.
All authors listed have made a substantial, direct, and intellectual contribution to the work, and approved it for publication.
The authors acknowledge the Department of Science and Technology (DST)-sponsored scheme (EMR/2016/003147) and author AS sincerely acknowledges the Council of Scientific and Industrial Research (CSIR), New Delhi, for CSIR-Junior Research Fellow (JRF) (File No: 09/641(0174)/2019-EMR-I) fellowship support.
This work was performed at the Tamil Nadu Rice Research Institute, Aduthurai, Tamil Nadu Agricultural University, Tamil Nadu, India.
The authors declare that the research was conducted in the absence of any commercial or financial relationships that could be construed as a potential conflict of interest.
All claims expressed in this article are solely those of the authors and do not necessarily represent those of their affiliated organizations, or those of the publisher, the editors and the reviewers. Any product that may be evaluated in this article, or claim that may be made by its manufacturer, is not guaranteed or endorsed by the publisher.
The Supplementary Material for this article can be found online at: https://www.frontiersin.org/articles/10.3389/fpls.2023.1083177/full#supplementary-material
Afrin, W., Hossain, M. A., Islam, M. M. (2017). Evaluation of yield and yield contributing traits for submergence tolerance of rice. Progress. Agric. 28 (1), 7–11. doi: 10.3329/pa.v28i1.32851
Akshaya, M., Thirumurugan, T., Chitra, S., Nithila, S., Jeyaprakash, P. (2020). Genetic variability in rice (Oryza sativa l.) landraces for seedling vigour traits. Electronic J. Plant Breed. 11 (01), 91–96. doi: 10.37992/2020.1101.016
Angaji, S.A., Septiningsih, E. M., Mackill, D. J., Ismail, A. M. (2010). QTLs associated with tolerance of flooding during germination in rice (Oryza sativa l.). Euphytica 172 (2), 159–168. doi: 10.1007/s10681-009-0014-5
Atwell, B. J., Waters, I., Greenway, H. (1982). The effect of oxygen and turbulence on elongation of coleoptiles of submergence-tolerant and-intolerant rice cultivars. J. Exp. Bot. 33 (5), 1030–1044. doi: 10.1093/jxb/33.5.1030
Baltazar, M. D., Ignacio, J. C. I., Thomson, M. J., Ismail, A. M., Mendioro, M. S., Septiningsih, E. M. (2014). QTL mapping for tolerance of anaerobic germination from IR64 and the aus landrace nanhi using SNP genotyping. Euphytica 197 (2), 251–260. doi: 10.1007/s10681-014-1064-x
Barik, J., Kumar, V., Lenka, S. K., Panda, D. (2019). Genetic potentiality of lowland indigenous indica rice (Oryza sativa l.) landraces to anaerobic germination potential. Plant Physiol. Rep. 24 (2), 249–261. doi: 10.1007/s40502-019-00441-3
Biselli, C., Cavalluzzo, D., Perrini, R., Gianinetti, A., Bagnaresi, P., Urso, S., et al. (2014). Improvement of marker-based predictability of apparent amylose content in japonica rice through GBSSI allele mining. Rice 7 (1), 1–18. doi: 10.1186/1939-8433-7-1
Darko Asante, M., Ipinyomi, S. O., Ayodeji, A., Adjah, K. L., Aculey, P., Bam, R. K., et al. (2021). Genetic variability for and tolerance to anaerobic germination in rice (Oryza sativa l.). J. Crop Improv. 35 (6), 832–847. doi: 10.1080/15427528.2021.1884631
Ella, E. S., Dionisio-Sese, M. L., Ismail, A. M. (2011). Seed pre-treatment in rice reduces damage, enhances carbohydrate mobilization and improves emergence and seedling establishment under flooded conditions. AoB Plants 30 (7), 813–819. doi: 10.1093/aobpla/plr007
Ella, E. S., Kawano, N., Yamauchi, Y., Tanaka, K., Ismail, A. M. (2003). Blocking ethylene perception enhances flooding tolerance in rice seedlings. Funct. Plant Biol. 30 (7), 813–819. doi: 10.1071/FP03049
Fukao, T., Xu, K., Ronald, P. C., Bailey-Serres, J. (2006). A variable cluster of ethylene response factor–like genes regulates metabolic and developmental acclimation responses to submergence in rice. Plant Cell 18 (8), 2021–2034. doi: 10.1105/tpc.106.043000
Ghosal, S., Casal, C., Quilloy, F. A., Septiningsih, E. M., Mendioro, M. S., Dixit, S. (2019). Deciphering genetics underlying stable anaerobic germination in rice: phenotyping, QTL identification, and interaction analysis. Rice 12 (1), 1–15. doi: 10.1186/s12284-019-0305-y
Ghosal, S., Quilloy, F. A., Casal, C., Septiningsih, E. M., Mendioro, M. S., Dixit, S. (2020). Trait-based mapping to identify the genetic factors underlying anaerobic germination of rice: phenotyping, GXE, and QTL mapping. BMC Genet. 21 (1), 1–13. doi: 10.1186/s12863-020-0808-y
Guglielminetti, L., Yamaguchi, J., Perata, P., Alpi, A. (1995). Amylolytic activities in cereal seeds under aerobic and anaerobic conditions. Plant Physiol. 109 (3), 1069–1076. doi: 10.1104/pp.109.3.1069
Hwang, Y.-S., Thomas, B. R., Rodriguez, R. L. (1999). Differential expression of rice α-amylase genes during seedling development under anoxia. Plant Mol. Biol. 40 (6), 911–920. doi: 10.1023/A:1006241811136
Islam, M. R., Naveed, S. A., Zhang, Y., Li, Z., Zhao, X., Fiaz, S., et al. (2022). Identification of candidate genes for salinity and anaerobic tolerance at the germination stage in rice by genome-wide association analyses. Front. Genet. 8. doi: 10.3389/fgene.2022.822516
Ismail, A. M., Ella, E. S., Vergara, G. V., Mackill, D. J. (2009). Mechanisms associated with tolerance to flooding during germination and early seedling growth in rice (Oryza sativa). Ann. Bot. 103 (2), 197–209. doi: 10.1093/aob/mcn211
Ismail, A. M., Johnson, D. E., Ella, E. S., Vergara, G. V., Baltazar, A. M. (2012). Adaptation to flooding during emergence and seedling growth in rice and weeds, and implications for crop establishment. AoB Plants 2012. doi: 10.1093/aobpla/pls019
Jiang, L., Liu, S., Hou, M., Tang, J., Chen, L., Zhai, H., et al. (2006). Analysis of QTLs for seed low temperature germinability and anoxia germinability in rice (Oryza sativa l.). Field Crops Res. 98 (1), 68–75. doi: 10.1016/j.fcr.2005.12.015
Keuls, M. (1952). The use of the studentized range” in connection with an analysis of variance. Euphytica 1, 112–122.
Kim, S.-M., Kim, C.-S., Jeong, J.-U., Reinke, R. F., Jeong, J.-M. (2019). Marker-assisted breeding for improvement of anaerobic germination in japonica rice (Oryza sativa). Plant Breed. 138 (6), 810–819. doi: 10.1111/pbr.12719
Kretzschmar, T., Pelayo, M. A. F., Trijatmiko, K. R., Gabunada, L. F. M., Alam, R., Jimenez, R., et al. (2015). A trehalose-6-phosphate phosphatase enhances anaerobic germination tolerance in rice. Nat. Plants 1 (9), 1–5. doi: 10.1038/nplants.2015.124
Kuanar, S. R., Ray, A., Sethi, S. K., Chattopadhyay, K., Sarkar, R. K. (2017). Physiological basis of stagnant flooding tolerance in rice. Rice Sci. 24 (2), 73–84. doi: 10.1016/j.rsci.2016.08.008
Lal, B., Gautam, P., Nayak, A. K., Raja, R., Shahid, M., Tripathi, R., et al. (2018). Agronomic manipulations can enhance the productivity of anaerobic tolerant rice sown in flooded soils in rainfed areas. Field Crops Res. 220, 105–116. doi: 10.1016/j.fcr.2016.08.026
Lu, H., Redus, M. A., Coburn, J. R., Rutger, J.N., McCouch, S. R., Tai, T. H. (2005). Population structure and breeding patterns of 145 US rice cultivars based on SSR marker analysis. Crop Sci. 45 (1), 66–76. doi: 10.2135/cropsci2005.0066
Magneschi, L., Perata, P. (2009). Rice germination and seedling growth in the absence of oxygen. Ann. Bot. 103 (2), 181–196. doi: 10.1093/aob/mcn121
Miro, B., Longkumer, T., Entila, F. D., Kohli, A., Ismail, A. M. (2017). Rice seed germination underwater: morpho-physiological responses and the bases of differential expression of alcoholic fermentation enzymes. Front. Plant Sci. 8, 1857. doi: 10.3389/fpls.2017.01857
Mohanapriya, G., Kavitha, S., Manonmani, S. (2022). Identification of anaerobic germination tolerant landraces and validation of molecular marker in rice (Oryza sativa l.). Electronic J. Plant Breed. 13 (3), 873–881. doi: 10.37992/2022.1303.110
Mondal, S., Khan, M.I. R., Entila, F., Dixit, S., Panna Ali, M., Pittendrigh, B., et al. (2020). Responses of AG1 and AG2 QTL introgression lines and seed pre-treatment on growth and physiological processes during anaerobic germination of rice under flooding. Sci. Rep. 10 (1), 1–15. doi: 10.1038/s41598-020-67240-x
Murray, M. G., Thompson, W. F. (1980). Rapid isolation of high molecular weight plant DNA. Nucleic Acids Res. 8 (19), 4321–4326. doi: 10.1093/nar/8.19.4321
Murtagh, F., Legendre, P. (2014). Ward’s hierarchical agglomerative clustering method: which algorithms implement ward’s criterion? J. Classif. 31 (3), 274–295. doi: 10.1007/s00357-014-9161-z
Newman, D. (1939). The distribution of range in samples from a normal population, expressed in terms of an independent estimate of standard deviation. Biometrika 31 (1/2), 20–30.
Nghi, K. N., Tondelli, A., Valè, G., Tagliani, A., Marè, C., Perata, P., et al. (2019). Dissection of coleoptile elongation in japonica rice under submergence through integrated genome-wide association mapping and transcriptional analyses. Plant Cell Environ. 42 (6), 1832–1846. doi: 10.1111/pce.13540
Oladosu, Y., Rafii, M. Y., Arolu, F., Chukwu, S. C., Muhammad, I., Kareem, I., et al. (2020). Submergence tolerance in rice: review of mechanism, breeding and, future prospects. Sustainability 12 (4), 1632. doi: 10.3390/su12041632
Palaniyappan, S., Arunachalam, P., Banumathy, S., Mini, M. L., Muthuramu, S. (2020). Genetic divergence and clustering studies in advanced breeding lines of rice (Oryza sativa l.). Electronic J. Plant Breed. 11 (02), 499–504. doi: 10.37992/2020.1102.084
Racine, J. S. (2012). RSTUDIO: a platform-independent IDE for R and sweave. J Appl. Econ. 27 (1), 167–172.
Ramaiah, K. (1969). Grain classification. in rice research in India Vol. 629 (New delhi: ICAR Publication).
Rauf, M., Choi, Y.-M., Lee, S., Lee, M.-C., Oh, S., Hyun, D. Y. (2019). Evaluation of anaerobic germinability in various rice subpopulations: identifying genotypes suitable for direct-seeded rice cultivation. Euphytica 215 (2), 1–15. doi: 10.1007/s10681-019-2341-5
Ray, A., Deb, D., Ray, R., Chattopadhayay, B. (2013). Phenotypic characters of rice landraces reveal independent lineages of short-grain aromatic indica rice. AOB Plants 5. doi: 10.1093/aobpla/plt032
Reddy, V. A., Girija Rani, M., Satyanarayana, P. V., Suryanarayana, Y., Chamundeswari, N., Ravikumar, B. N. V. S. R., et al. (2015). Physiological and molecular response of rice genotypes for different types of flooding. Curr. Biotica 8 (4), 345–350.
Sarkar, R. K., Bhattacharjee, B. (2011). Rice genotypes with SUB1 QTL differ in submergence tolerance, elongation ability during submergence and re-generation growth at re-emergence. Rice 5 (1), 1–11. doi: 10.1007/s12284-011-9065-z
Sathya, A. (2013). Are the Indian rice landraces a heritage of biodiversity to reminisce their past or to reinvent for future. Asian Agrihist 17, 221–2325.
Septiningsih, E. M., Ignacio, J. C. I., Sendon, P., Sanchez, D. L., Ismail, A. M., Mackill, D. J. (2013). QTL mapping and confirmation for tolerance of anaerobic conditions during germination derived from the rice landrace ma-zhan red. Theor. Appl. Genet. 126 (5), 1357–1366. doi: 10.1007/s00122-013-2057-1
Sinha, A. K., Mishra, P. K. (2013). Morphology based multivariate analysis of phenotypic diversity of landraces of rice (Oryza sativa l.) of bankura district of West bengal. J. Crop Weed 9 (2), 115–121.
Sudeepthi, K., Srinivas, T., Ravi Kumar, BNVSR, Jyothula, D. P. B., Nafeez Umar, S. K. (2020). Principal component analysis for anaerobic germination traits in rice (Oryza sativa l.). IJCS 8 (1), 1977–1982. doi: 10.22271/chemi.2020.v8.i1ac.8555
Vikram, P., Kadam, S., Singh, B. P., Pal, J. K., Singh, S., Singh, O. N., et al. (2016). Genetic diversity analysis reveals importance of green revolution gene (Sd1 locus) for drought tolerance in rice. Agric. Res. 5 (1), 1‐12.
Keywords: anaerobic germination tolerance, genetic diversity, grain type, rapid shoot elongation, response index
Citation: Shanmugam A, Manivelan K, Deepika K, Nithishkumar G, Blessy V, Monihasri RB, Nivetha D, Roshini A, Sathya P, Pushpa R, Manimaran R, Subrahmaniyan K, Sassikumar D and Suresh R (2023) Unraveling the genetic potential of native rice (Oryza sativa L.) landraces for tolerance to early-stage submergence. Front. Plant Sci. 14:1083177. doi: 10.3389/fpls.2023.1083177
Received: 28 October 2022; Accepted: 04 April 2023;
Published: 18 May 2023.
Edited by:
Vidyasagar Sathuvalli, Oregon State University, United StatesReviewed by:
Sarla Neelamraju, Indian Institute of Rice Research (ICAR), IndiaCopyright © 2023 Shanmugam, Manivelan, Deepika, Nithishkumar, Blessy, Monihasri, Nivetha, Roshini, Sathya, Pushpa, Manimaran, Subrahmaniyan, Sassikumar and Suresh. This is an open-access article distributed under the terms of the Creative Commons Attribution License (CC BY). The use, distribution or reproduction in other forums is permitted, provided the original author(s) and the copyright owner(s) are credited and that the original publication in this journal is cited, in accordance with accepted academic practice. No use, distribution or reproduction is permitted which does not comply with these terms.
*Correspondence: Ramalingam Suresh, c3VyZXNoLnJAdG5hdS5hYy5pbg==; Datchinamoorthy Sassikumar, ZHNhc3Npa3VtYXJAZ21haWwuY29t
Disclaimer: All claims expressed in this article are solely those of the authors and do not necessarily represent those of their affiliated organizations, or those of the publisher, the editors and the reviewers. Any product that may be evaluated in this article or claim that may be made by its manufacturer is not guaranteed or endorsed by the publisher.
Research integrity at Frontiers
Learn more about the work of our research integrity team to safeguard the quality of each article we publish.