Corrigendum: Magnesium application improves the morphology, nutrients uptake, photosynthetic traits, and quality of tobacco (Nicotiana tabacum L.) under cold stress
- 1College of Resources and Environment/International Magnesium Institute, Fujian Agriculture and Forestry University, Fuzhou, China
- 2Institute of Tobacco Sciences, Fujian Provincial Tobacco Monopoly Bureau, Fuzhou, China
- 3Guangdong Provincial Key Laboratory of Crop Genetics and Improvement/Crops Research Institute, Guangdong Academy of Agricultural Sciences, Guangzhou, China
Cold stress is one of the major constraints limiting the productivity of many important crops, including tobacco (Nicotiana tabacum L.) production and quality worldwide. However, the role of magnesium (Mg) nutrition in plants has been frequently overlooked, especially under cold stress, and Mg deficiency adversely affects plant growth and development. Here, we evaluated the influence of Mg under cold stress on tobacco morphology, nutrient uptake, photosynthetic and quality attributes. The tobacco plants were grown under different levels of cold stress, i.e., 8°C, 12°C, 16°C, including with a controlled temperature of 25°C, and evaluated their effects with Mg (+Mg) and without Mg (–Mg) application. Cold stress resulted in reduced plant growth. However, the +Mg alleviated the cold stress and significantly increased the plant biomass on an average of 17.8% for shoot fresh weight, 20.9% for root fresh weight, 15.7% for shoot dry weight, and 15.5% for root dry weight. Similarly, the nutrients uptake also increased on average for shoot-N (28.7%), root-N (22.4%), shoot-P (46.9%), root-P (7.2%), shoot-K (5.4%), root-K (28.9%), shoot-Mg (191.4%), root-Mg (187.2%) under cold stress with +Mg compared to –Mg. Mg application significantly boosted the photosynthetic activity (Pn 24.6%) and increased the chlorophyll contents (Chl-a (18.8%), Chl-b (25%), carotenoids (22.2%)) in the leaves under cold stress in comparison with –Mg treatment. Meanwhile, Mg application also improved the quality of tobacco, including starch and sucrose contents, on an average of 18.3% and 20.8%, respectively, compared to –Mg. The principal component analysis revealed that tobacco performance was optimum under +Mg treatment at 16°C. This study confirms that Mg application alleviates cold stress and substantially improves tobacco morphological indices, nutrient absorption, photosynthetic traits, and quality attributes. In short, the current findings suggest that Mg application may alleviate cold stress and improve tobacco growth and quality.
Introduction
Tobacco (Nicotiana tabacum L.), belonging to the family Solanaceae, originated from tropical and subtropical America but is now widely cultivated in China (Zhao et al., 2009; Zhou et al., 2020). In southeast China, which has a subtropical monsoon climate and average annual precipitation of 1558.1 mm, magnesium deficiency often occurs in acidic soils due to low cation exchange capacity and easy leaching (Guo et al., 2016). Tobacco is sensitive to cold stress. However, extreme temperatures have occurred frequently in recent years, severely restricting crops grown naturally. In the main tobacco-producing areas of Fujian Province, China, we found that the distribution of exchangeable Mg in the soil was uneven. Some areas were severely deficient in magnesium (Figure 1A). Meanwhile, we found that the field temperature in the early stage of tobacco seedling transplanting was lower than the temperature required for the normal growth of tobacco seedlings (Figures 1B, C), which profoundly affects the growth of tobacco plants and their tobacco quality.
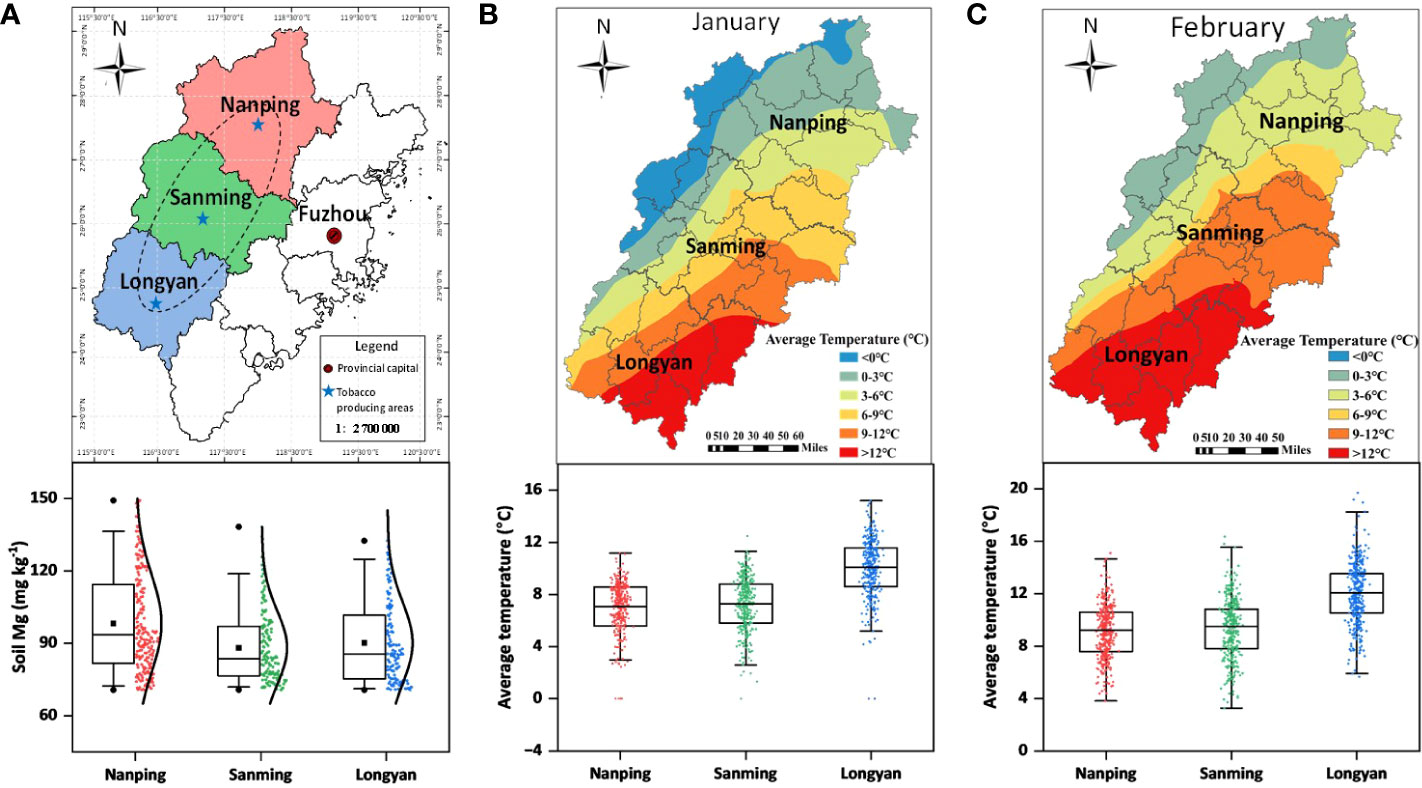
Figure 1 Map representing the geographic distribution of tobacco. (A) major flue-cured tobacco producing areas; (B, C) average temperature during the months of January and February.
Cold stress is a crucial environmental factor influencing the plants’ growth, yield, quality, and geographical distribution (Wang Y. et al., 2019). Tobacco (Nicotiana tabacum L.) is one of the most important economic crops in south China and often poses cold stress and hinders early sowing (Cui et al., 2014). Hence, tobacco growth is inhibited under cold stress and manifested by morphological traits, nutrient uptake, photosynthetic traits, and quality attributes (Li et al., 2021). For example, cold stress negatively affects the morphological traits such as shoot and root length, leaf area, stem diameter, etc., thus affecting the morphological formation of tobacco, and photosynthetic activity is also reduced and deteriorates the quality (Gechev et al., 2003; Xu et al., 2010). It has also been reported that warm-season crops are very sensitive to cold stress. For example, low temperature during the flowering time in soybean (Glycine max) decreases the number of pods and seeds per plant and results in low yield and poor quality (Yang et al., 2004). In cotton (Gossypium hirsutum L.), the cold stress for short time during later growth stage encounters the leaf spot lesions (Zhao et al., 2012). Similarly, when maize (Zea mays) encounters a cold injury during emergence to maturity, it results in poor germination rate and growth of leaves, inhibits the photosynthetic activity, slow filling rate and accumulation of dry matter, and finally low yield and poor quality (Prasad et al., 1994). Therefore, it is imperative to improve the cold tolerance of tobacco seedlings subjected to low temperature in the early spring.
So far, various studies have reported the adaptive responses of plants to different abiotic stresses. For instance, the application of ALA (5-aminolevulinic acid) increases the enzyme activity and nutrient content of cucumber seedlings and enhances the tolerance of cucumber seedlings to cold stress (Anwar et al., 2018). Glycine betaine and 24-epibrassinolide alleviate cold stress and improve the growth of peaches and peppers (Peng et al., 2019; Wang L. et al., 2019). The exogenous brassinolactone application effectively relieves the cold stress on the physiological metabolism of rice at the booting stage and improves yield (Wang S. et al., 2020). Salicylic acid (SA) alleviates chilling stress by improving the quality of fresh tobacco leaves, the enzyme activity of the antioxidant system, and the quality of tobacco leaves (He et al., 2020). Nevertheless, the role of Mg under cold stress to improve crop growth, yield, and quality has been neglected or not fully elucidated.
Magnesium (Mg) is an essential micronutrient for plant growth and development, as it is involved in various physiological and biochemical processes such as photosynthesis and enzymatic activities (Verbruggen and Hermans, 2013; Chen et al., 2018). Mg is considered a central atom of the chlorophyll molecule, and about 35% of total plant Mg is associated with chlorophyll and hence could play a key role in photosynthesis, energy metabolism, carbohydrate accumulation, and stress resistance (Cakmak and Kirkby, 2008; Farhat et al., 2016; Chen et al., 2018). Hence, Mg in plants directly affects yield and quality because of its diverse role in plant physiology. One of the most well-known physiological impacts of Mg shortage is its negative impact on plant photosynthetic activity and the transportation of photoassimilates to the sink organs (Geng et al., 2021). It has also been widely accepted that photosynthesis is very sensitive to cold stress, and the photosynthetic activity is significantly inhibited by the cold stress (Van Heerden and Kruger, 2000; Jin et al., 2011; Banerjee and Roychoudhury, 2019) and results in reduced synthesis and transportation of photoassimilates, and leads to poor crop yield and quality (Rude and Gruber, 2004; Wang Z. et al., 2020). Hence, understanding the effect of Mg under cold stress for improving tobacco plant growth is of great practical and theoretical significance for high yielding and quality. Therefore, in this present study, a controlled experiment was established to study the effect of Mg application under varying levels of cold stress on tobacco; i) morphological traits, ii) concentration of mineral nutrients in different plant organs, iii) photosynthetic traits and gas exchange parameters, iv) quality attributes.
Materials and methods
Plant culture and cold stress
The experiment was conducted under controlled conditions from March to June 2020 at the Fujian Institute of Tobacco Agricultural Sciences, Fujian, China. The seeds of the tobacco cultivar “Cuibiyihao” (Nicotiana tabacum L. cv. CB-1) were surface sterilized with 75% ethanol and 30% hydrogen peroxide (1:1) for 5 min. The seeds were then rinsed several times with sterilized water before being vernalized at 4°C for 12h in the dark (Forner et al., 2015; Anwar et al., 2018). In short, first, the vernalized seeds were sown in plastic trays, and seedlings were raised for 2 months under greenhouse conditions. Second, sixty healthy tobacco seedlings were selected and transplanted to hydroponic pots for adaptation to a nutrient solution environment at 25°C for 2 weeks. Third, 36 tobacco seedlings with uniform size and growth were selected and randomly divided into three equal parts and transferred to four different growth chambers, each with 12 hydroponic pots, and set the temperature of 8°C, 12°C, 16°C and 25°C respectively, because of frequent severe changes in temperature since last few years in the growing season of tobacco (Figures 1B, C). The black hydroponic pot with a lid, 12 cm long, 8 cm wide, and 10 cm high, with a volume of about 1000 mL, was used for the test. The black color was mainly used for shading, and the black lid had holes in the middle for the growth of plants.
The hydroponic pots of each growth chamber were divided into two sets (6 hydroponic pots for each). One set was treated with magnesium addition (+Mg) and four different temperatures, i.e., 8°C, 12°C, 16°C and 25°C, and named as +Mg8, +Mg12, +Mg16 and +Mg25, respectively. The other set without magnesium (–Mg) and the abovementioned temperatures are –Mg8, –Mg12, –Mg16 and –Mg25, respectively. It is important to investigate the effect of Mg because the main tobacco-producing areas of Fujian Province have an uneven distribution of exchangeable Mg in the soil, and some areas are severely deficient in Mg (Figure 1A). So, hydroponic pots of +Mg were supplemented with a modified Hoagland’s nutrient solution containing magnesium (pH 6.5-7.0; 995μM KNO3, 931μM Ca(NO3)2·4H2O, 397μM MgSO4·7H2O and 282μM KH2PO4. Micronutrients: 22.5μM Fe(II)-EDTA, 46.2μM H3BO4, 14.3μM MnCl2·4H2O, 0.76μM ZnCl2, 0.32μM CuCl2·2H2O, 0.13Mμ Na2Mo4·2H2O). While, hydroponic pots of –Mg were supplemented with modified Hoagland’s nutrient solution without magnesium (pH 6.5-7.0, 995μM KNO3, 931μM Ca(NO3)2·4H2O, 147μM Na2SO4, and 282μM KH2PO4; micronutrients: 22.5μM Fe(II)-EDTA, 46.2μM H3BO4, 14.3μM MnCl2·4H2O, 0.76μM ZnCl2, 0.32μM CuCl2·2H2O, 0.13μM Na2Mo4·2H2O). The growth chambers were set to photosynthetically active radiation of 700 μmol·photon m-2·s-1, the relative humidity is 80%, 12 hours: 12 hours for light: dark cycle. The nutrient solution was replaced weekly. The experiment was harvested after eight weeks of temperature and Mg treatment.
Measurement of plant morphological traits
The tobacco plants were harvested and separated into shoots and roots. Shoot and root were weighed to obtain the fresh biomass of shoot fresh weight (SFW) and root fresh weight (RFW). To determine the shoot dry weight (SDW) and root dry weight (RDW), the plant material was oven-dried for 48h at 70°C (Zhang B. et al., 2020).
Analysis of mineral nutrients concentration in different plant organs
For different plant mineral analyses, plants’ roots and shoots were collected, oven-dried, ground, and passed through 60 mesh sieves. For nitrogen (N), phosphorous (P), and potassium (K) minerals analysis, 0.05 g of tobacco plant material of each root and shoot was digested in 5 mL of concentrated sulfuric acid (H2SO4) and 4.0 mL of hydrogen peroxide (H2O2) on digestion tubes. The digestion tubes were kept in the digestion block for approximately 2h, at a temperature of 200 ± 20°C until about 2ml of clear digestive fluid without granules was obtained. Then the deionized water was added to the digestive fluid and filtered using a 0.45-micron filter, and the extracts were stored at 4°C until nutrient determination (Huang et al., 2021). For magnesium (Mg) analysis, 0.1 g of tobacco plant material of each root and shoot was digested in a mixture of nitric acid (HNO3): perchloric acid (HClO4) in a ratio 5v:1v on the hot plate at 180°C until clear aliquot was obtained. The deionized water was added to the digestive fluid and filtered using a 0.45-micron filter, and the extracts were stored at 4°C for further analysis (Sharma et al., 2020). Total P and Mg in the samples were determined by an Inductively Coupled Plasma Optical Emission Spectrometer (ICP-OES). Total carbon (C) and total nitrogen (N) were measured by a Skalar Primacs SN100 (Netherlands, DUMAS). Total K was determined with a flame photometer.
Determination of chlorophyll and carotenoids contents
On the day of harvest, 0.1 g of fresh leaves of tobacco seeding were taken from each replication to measure leaf total chlorophyll and carotenoid contents using an ultraviolet and visible spectrophotometer (UV-VIS). The samples were put into 25 mL 80% acetone solution. They were then kept in darkness at room temperature overnight to allow for the extraction of the leaf pigments. The mixture of chlorophyll and 80% acetone solution was examined at 663 nm, 645 nm, and 470 nm to determine chlorophyll-a, chlorophyll-b, and carotenoids, respectively. The calculation method was as follows:
Determination of leaf gas exchange and fluorescence
Leaf gas exchange parameters, including net photosynthetic rate (Pn), stomatal conductance (Gs), and intercellular CO2 concentration (Ci), were measured for each plant with a CIRAS-3 portable photosynthesis system (PP System, Maryland USA). Measurements were made on an upper, third fully expanded leaf of an individual plant representing each monoculture tank, typically between 09:00 and 11:00 local time, and were measured once Pn was stable after 2 to 4 min and under the following conditions: 400 μmol·mol-1·CO2, 1000 μmol·m-2·s-1 photosynthetically active radiation, 25°C leaf temperature (Lee et al., 2011; Koch et al., 2019).
For fluorescence, tobacco seedlings were kept in the dark for 30 min, and one leaf was obtained from the same rosette of each plant sample. The three to four fully expanded leaves were selected for chlorophyll fluorescence yield detection to compare the different treatment leaves. Chlorophyll fluorescence measurements were obtained using a Plant Efficiency Analyzer (PEA, Hansatech, England). The following equations were used to calculate the Fv/Fm (photosystemII- PSII) (Lichtenthaler et al., 2005; Liu et al., 2018);
Where F0= initial fluorescence, Fv = variable fluorescence, Fm = maximum fluorescence, and Fv/Fm denotes the maximal quantum yield of PSII.
Statistical analyses
All the statistical analyses were performed using IBM-SPSS version 21.0 (IBM Corporation, New York, USA). Two-way analysis of variance (ANOVA) was performed to analyze the effect of Mg, temperature, and their interaction effect of tobacco morphological traits, minerals nutrients concentration in roots and shoots, photosynthetic traits, and quality parameters. All the measurements are presented as means with their standard errors (Mean ± SE). The statistically significant mean differences among various treatments were identified by using Duncan’s test at a significance level of P<0.05. The principal component analysis (PCA) was performed to evaluate the differences in biomass, minerals, nutrient content, photosynthetic traits, and quality parameters between the +Mg and –Mg under varying levels of low temperature (i.e., cold stress). The results were visualized with Origin Pro 2021 (Origin Lab Corporation, Northampton, USA).
Results
Effects of Mg on tobacco morphological traits under cold stress
Application of Mg significantly improved the tobacco morphological traits, including shoot fresh weight (SFW), root fresh weight (RFW), Shoot dry weight (SDW), and root dry weight (RDW) as compared to –Mg under cold stress (Figure 2).
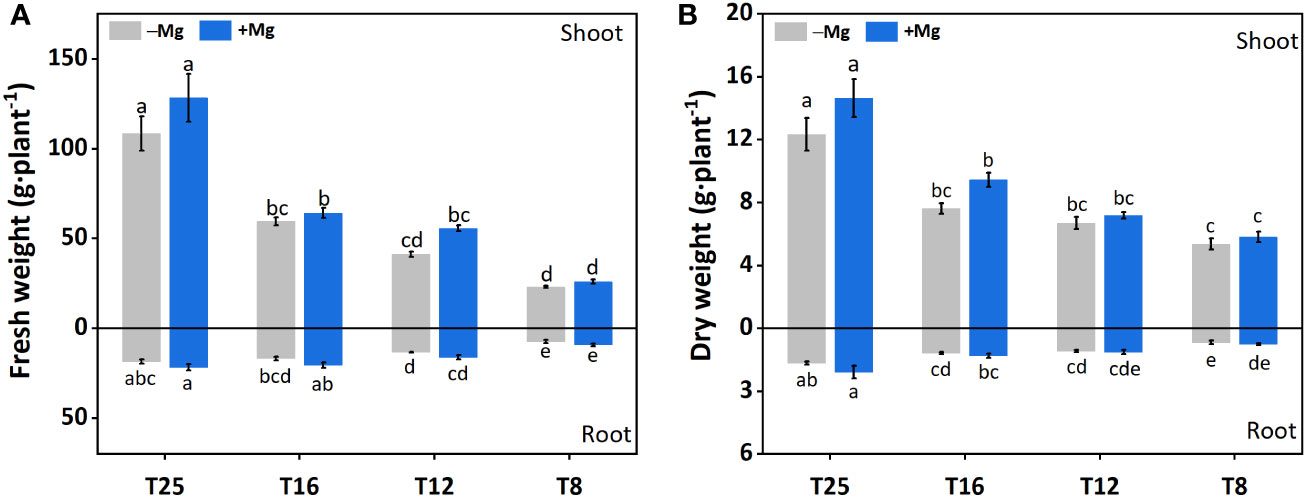
Figure 2 Effect of Mg application on tobacco morphological traits under different temperature. (A) root fresh weight (RFW) and shoot fresh weight (SFW); (B) root dry weight (RDW) and shoot dry weight (SDW). The different letters above the bars are indicating significant difference (Duncan P<0.05).
For SFW, the increasing trend was recorded with the increase in temperature, and a significant difference was found between different treatments. Increase in SFW under +Mg8, +Mg12, +Mg16 and +Mg25 was 12.9%, 35.0%, 7.7% and 18.2% compared to –Mg8, –Mg12, –Mg16 and –Mg25 treatments, respectively (Figure 2A). Similarly, RFW under +Mg treatment was significantly increased compared with –Mg treatments, while no significant difference was found at 8°C (Figure 2A). SDW was significantly increased under +Mg25 compared to –Mg25, while non-significant differences were recorded at 8°C and 12°C (Figure 2B). For RDW of the tobacco plant, significant differences were found among different treatments, and the maximum RDW was recorded at –Mg25 (1.65 g·plant-1) and +Mg25 (2.07 g·plant-1) (Figure 2B). The phenotypic differences for +Mg and –Mg were evident under different low temperatures. The results of two-way ANOVA showed the significant main effects of Mg and temperature and their interaction effect for RFW, while RFW showed significant effects only for Mg and temperature. In the case of SDW and RDW, Mg had no significant effect, while temperature had a significant effect (Table 1).
Effect of Mg on nutrients uptake under cold stress
Application of Mg under varying levels of temperature altered the nutrients uptake (Figure 3). For example, carbon (C) contents of the shoot were significantly higher under +Mg treatment compared with –Mg at all temperatures, and the highest C content (6221.42 mg) was recorded at +Mg25 (Figure 3A). The increase in shoot C content under +Mg was 8.5%, 2.9%, 43,2% and 19.3% compared with –Mg at 8°C, 12°C, 16°C, and 25°C, respectively. In contrast, root C contents were non-significant at 8°C and 12°C, while significantly higher (849.07 mg) only at 25°C (Figure 3A). The results of Two-way ANOVA showed that shoot and root C contents were significantly affected by the main effects of Mg, and temperature, while for interaction effect significant results found for shoot C, and non-significant for root C (Table 2).
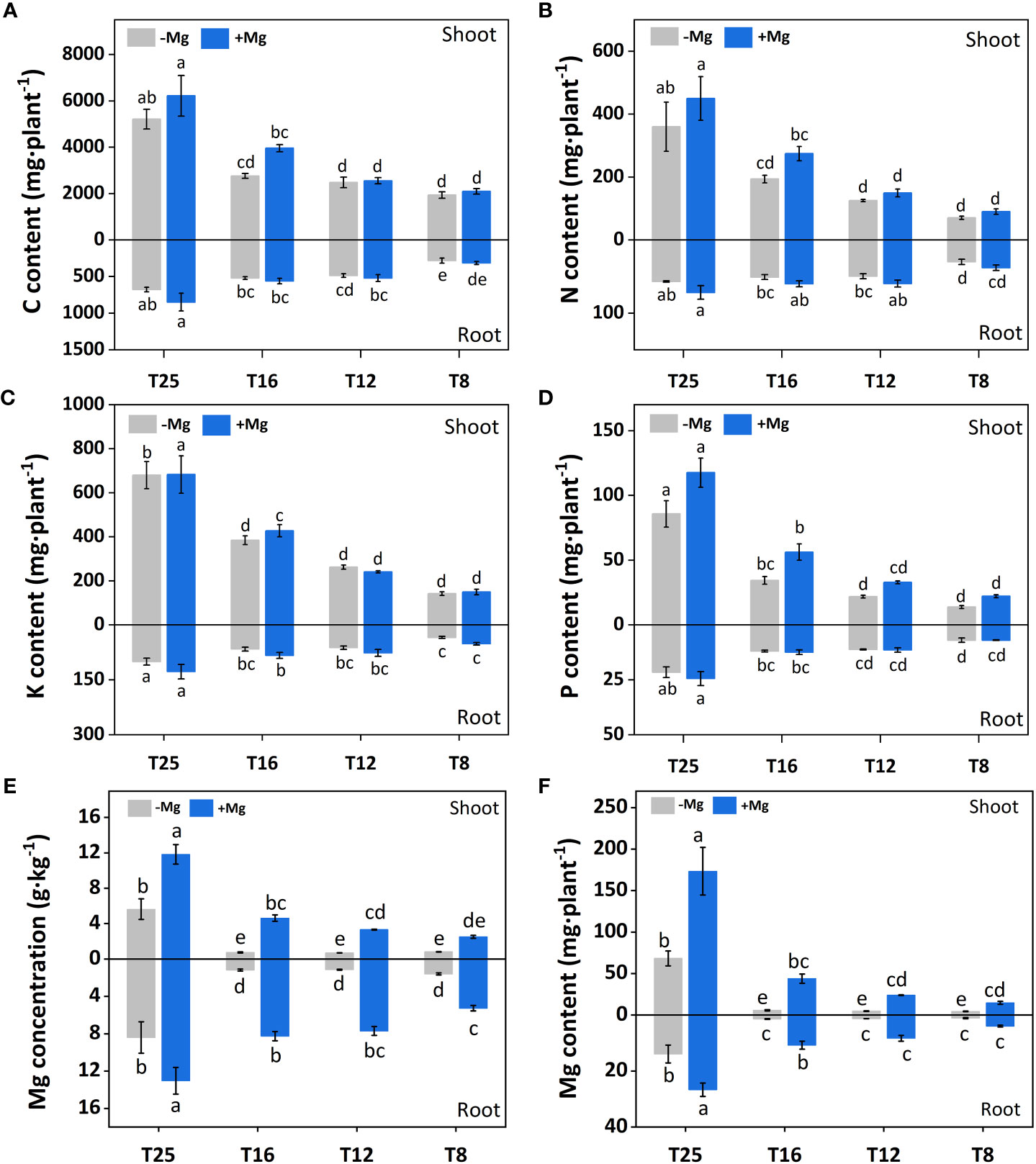
Figure 3 Effect of Mg application on concentration of minerals nutrients under different temperature in tobacco plant. (A) shoot-C content and root-C content; (B) shoot-N content and root-N content; (C) shoot-K content and root-K content; (D) shoot-P content and root-P content; (E) shoot-Mg concentration and root-Mg concentration; (F) shoot-Mg content and root-Mg content. The different letters above the bars are indicating significant difference (Duncan P<0.05).
Similarly, for nitrogen (N), a significant difference was observed for aboveground plant parts (i.e., shoot), and shoot N contents under +Mg treatment were significantly higher than –Mg at all temperatures and showed maximum N contents at 25°C (Figure 3B). For root, N contents were significantly higher at +Mg25 compared with other treatments (Figure 3B). Mg, temperature, and their interaction effect had a significant effect on shoot N content, while for root N, only temperature showed the significant effect (Table 2).
The potassium (K) contents of the shoot were not significantly affected among +Mg and –Mg treatments at 8°C, 12°C, 16°C, and 25°C temperature, while temperature had a significant effect. However, it significantly increased with increasing temperature. Maximum shoot K content (683.2 mg) was found at +Mg25 (Figure 3C). The root K content was significantly increased with increasing temperature and higher under +Mg application compared with –Mg under all temperatures, and maximum root K content was recorded under +Mg25 (Figure 3C). The Mg and temperature had significant effect on root K content. In contrast, the interaction effect for both root and shoot was non-significant (Table 2).
The +Mg significantly increased the shoot phosphorous (P) contents at all four temperature levels compared with –Mg. Shoot P contents significantly increased with increasing temperature, and maximum shoot contents (117.5 mg) were observed at +Mg25 (Figure 3D). Hence, Mg and temperature significantly affected shoot P. In contrast, Mg supplementation did not significantly affect P content in tobacco roots. While the increasing temperature also increased the root P contents, and the highest root P contents were recorded at +Mg25 (24.4 mg) (Figure 3D). Hence, the temperature had a significant effect on the root P content of tobacco plants (Table 2).
Mg treatment had a significant effect on the Mg contents of the tobacco plant. For instance, shoot Mg contents were significantly higher under +Mg treatment than –Mg under all temperature levels. The maximum shoot Mg was found under +Mg treatment at 25°C (Figure 3E). Similar results were recorded for root Mg contents (Figure 3F). Shoot and root Mg contents were maximum at +Mg25 about 173.34 mg·plant-1 and 26.68 mg·plant-1, respectively. The increase rates were 91.9% and 88.5%, respectively, compared with –Mg8. Thus, shoot and root Mg contents were significantly affected by Mg, temperature, and their interaction effect (Table 2).
Effects of Mg on gas exchange parameters under cold stress
The gas exchange parameters, including stomatal conductance (Gs), intercellular carbon dioxide concentration (Ci), transpiration rate (Tr), and photosynthetic rate (Pn), were significantly affected by the Mg application under cold stress. The Gs for both +Mg and –Mg decreased with the decrease in temperature level. The Gs under +Mg tobacco plants increased 36.9%, 62.0%, 9.0% and 29.2% compared to –Mg treatment at 8°C, 12°C, 16°C and 25°C, respectively. The highest value of Gs was recorded at 25°C, i.e., 420.67 mmol·m-2·s-1 under +Mg and 325.33 mmol·m-2·s-1 for –Mg treatment (Figure 4A). For Ci, there was an opposite result, the highest value was found under –Mg treatment about 359.00 μmol·mmol-1 at 25°C (Figure 4B). Whereas, the highest value of Tr and Pn was found under +Mg treatment about 6.72 mmol H2O·m-2·s-1 and 18.27 mmol·m-2·s-1, respectively, at 25°C (Figures 4C, D). Results of two-way ANOVA showed that Mg and temperature significantly affected Gs, Ci, and Pn (Table 3).
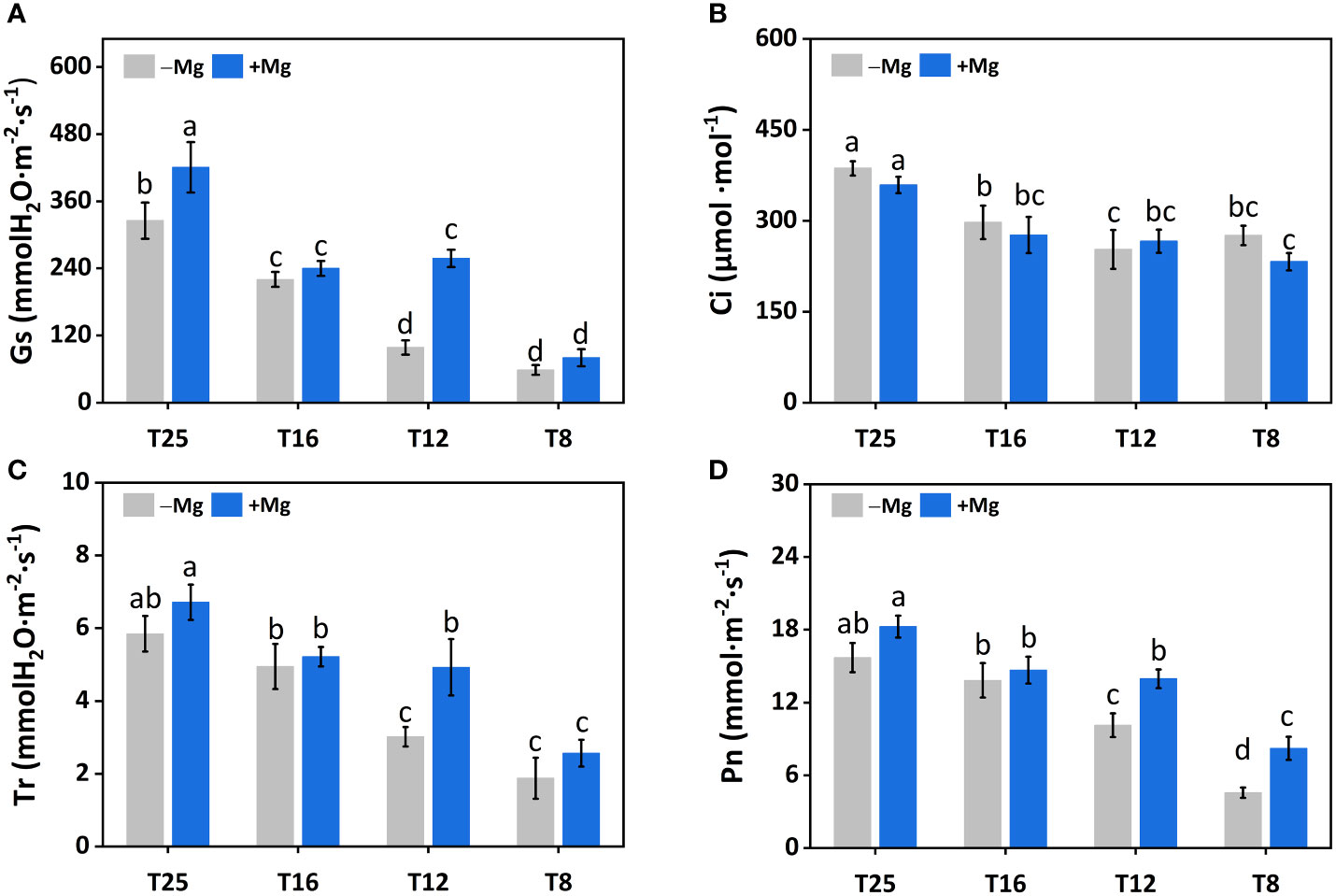
Figure 4 Effects of Mg application on gas exchange parameters under different temperature in tobacco plant. (A) Stomatal conductance (Gs); (B) intercellular CO2 (Ci); (C) Transpiration rate (Tr); (D) photosynthetic rate (Pn). The different letters above the bars are indicating significant difference (Duncan P<0.05).
The Mg application had a significant effect on chlorophyll and carotenoid contents. Chlorophyll-a (Chl-a) content was significantly affected by Mg at 12°C, 16°C and 25°C, and maximum Chl-a content was recorded at +Mg25 (Figure 5A). A similar trend was observed for chlorophyll-b (Chl-b) (Figure 5B) and total chlorophyll contents (Chl-a+b) (Figure 5C). The carotenoid content (Cart) was increased under +Mg about 17.6%, 25.0%, 17.6% and 20.8% compared to –Mg at 8°C, 12°C, 16°C and 25°C, respectively (Figure 5D). Moreover, Chl-a, Chl-b, Chl-a+b and Cart contents were significantly affected by Mg, temperature, and their interaction effect (Table 4).
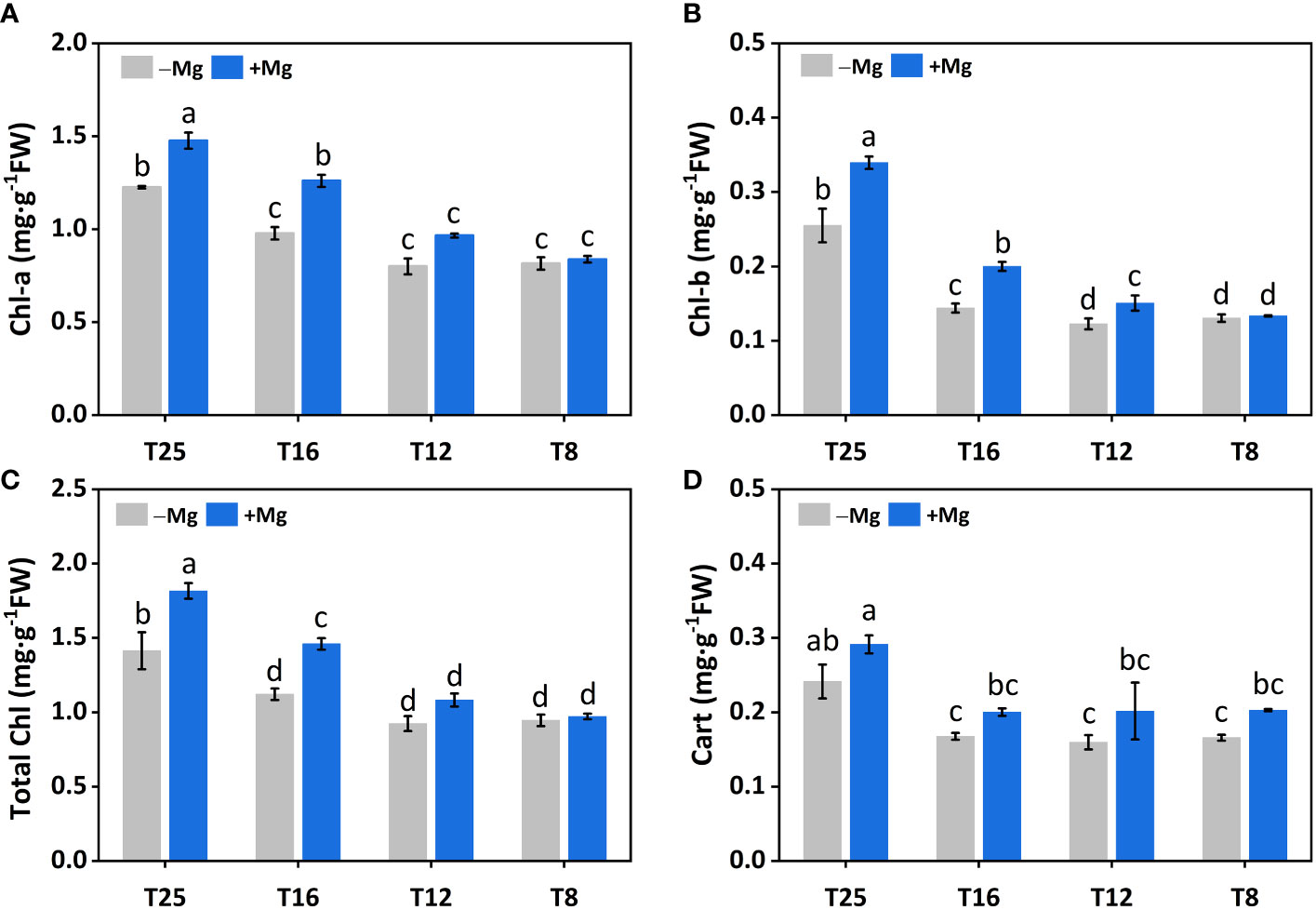
Figure 5 Effect of Mg application on chlorophyll of tobacco under different temperature. (A) chlorophyll-a (Chl-a); (B) chlorophyll-b (Chl-b); (C) total chlorophyll (Total Chl) (D) carotenoid (Cart). The different letters above the bars are indicating significant difference (Duncan P<0.05).
Effects of Mg on carbohydrate under cold stress
Mg supplementation had a significant effect in increasing the sucrose in tobacco plants. Similarly, the starch content increased with increasing temperature, and the maximum value was observed at 25°C 24.7% and 30.6% for both +Mg and –Mg treatment, respectively. It was increased by 7.7%, 7.5%, 33.8% and 24.1% under +Mg treatment compared to –Mg at 8°C, 12°C, 16°C and 25°C, respectively (Figure 6A). Hence, the sucrose contents reached a maximum about 17.3% and 16.9% at 25°C for +Mg and –Mg treatment, respectively. It was decreased by 40.2%, 8.5%, 32.1% and 2.5% under +Mg treatment compared to –Mg at 8°C, 12°C, 16°C and 25°C, respectively (Figure 6B). Results of two-way ANOVA have been shown that sucrose and starch were significantly affected by Mg and temperature (Table 5).
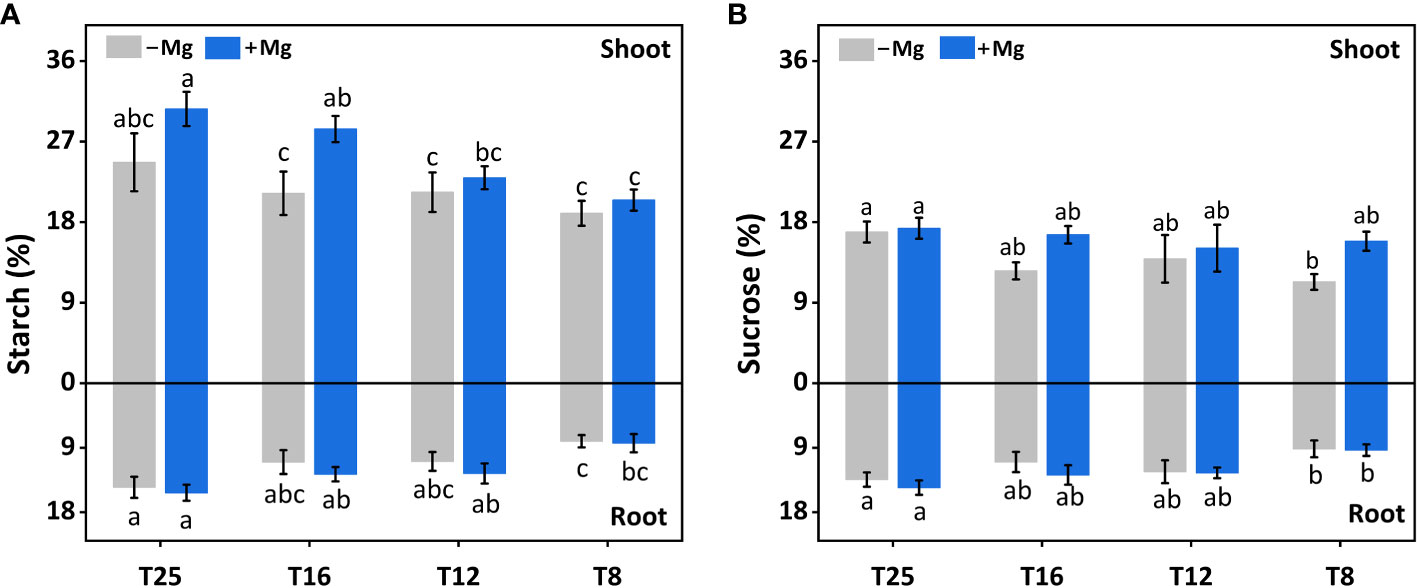
Figure 6 Effect of Mg application on carbohydrate of tobacco plant under different temperature. (A) shoot starch content and root starch content; (B) shoot sucrose content and root sucrose content. The different letters above the bars are indicating significant difference (Duncan P<0.05).
The above results indicated that the photosynthetic system of tobacco plant had changed to some extent. With the increase of temperature, the chlorophyll of tobacco plants increased and the carbohydrate produced by the photosynthetic system also increased. In order to further clarify the relationship between chlorophyll (Chl-a, Chl-b, Chl-a+b), gas exchange parameters (Ci, Gs, Tr), carbohydrate (sucrose, starch reducing sugar) and photosynthetic rate (Pn), correlation analysis was conducted between chlorophyll (Chl-a, Chl-b, Chl-a+b), gas exchange parameters (Ci, Gs, Tr) carbohydrate (sucrose, starch reducing sugar) and photosynthetic rate (Pn). As shown in Figure 7, photosynthetic rate (Pn) was positively related to Ci, Gs, Tr, Chl-a, Chl-b, Chl-a+b, sucrose, starch and reducing, respectively.
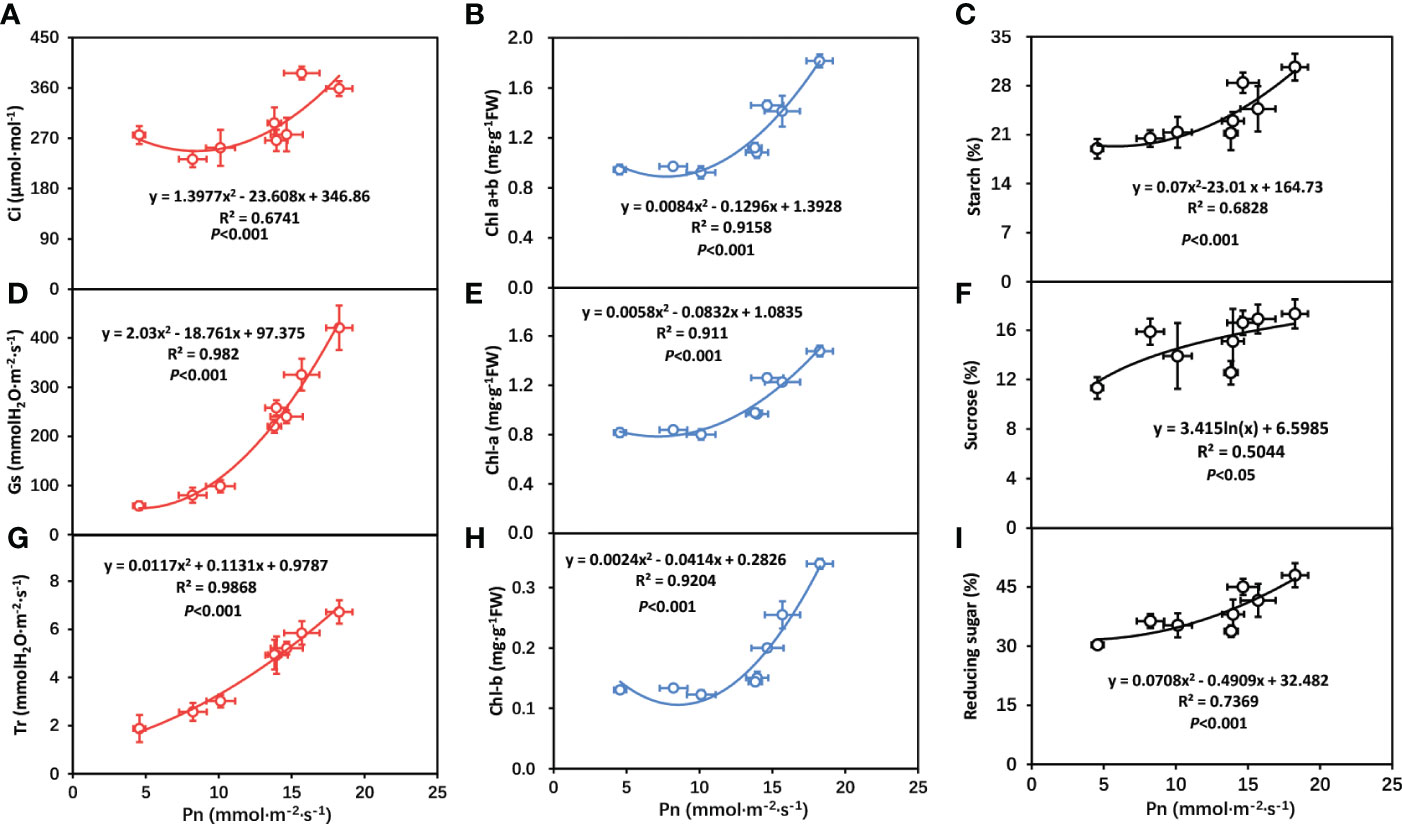
Figure 7 Correlation analysis of photosynthetic rate with gas exchange parameters in tobacco plants. (A) correlation between intercellular carbon dioxide concentration (Ci) and net photosynthetic rate (Pn); (B) correlation between total chlorophyll contents (Chl-a+b) and net photosynthetic rate (Pn); (C) correlation between starch and net photosynthetic rate (Pn); (D) correlation between stomatal conductance (Gs) and net photosynthetic rate (Pn); (E) correlation between chlorophyll-a contents (Chl-a) and net photosynthetic rate (Pn); (F) correlation between sucrose and net photosynthetic rate (Pn); (G) correlation between transpiration rate (Tr) and net photosynthetic rate (Pn); (H) correlation between chlorophyll-b contents (Chl-b) and net photosynthetic rate (Pn); (I) correlation between reducing sugar and net photosynthetic rate (Pn). The statistically significant mean differences among various treatments were identified by using Duncan’s test at a significance level of P<0.05.
JIP - test
The JIP-test parameters were used to analyze further tobacco’s photosynthetic behavior in various treatments (Figure 8). It was found that, under 25°C, the +Mg treatment did not significantly affect the photosynthetic behavior of the tobacco, but cold stress significantly affected JIP-test parameters (Figure 8A). With decreasing temperature, the TRO/RC, ETO/RC, ABS/CSm, TRO/CSm, ETO/CSm, PIabs, DFabs indices were significantly reduced with a decrease in temperature (Figures 8B, C). Cold stress significantly decreased the PI (abs), and PSII functional activity index based on standardized ABS absorption, and the lower the cold stress, the greater the decline (Figure 8D). Mg application significantly inhibited the decrease in PI (abs). Cold stress significantly decreased the DF (abs), and PSII driving force based on standardized ABS absorption.
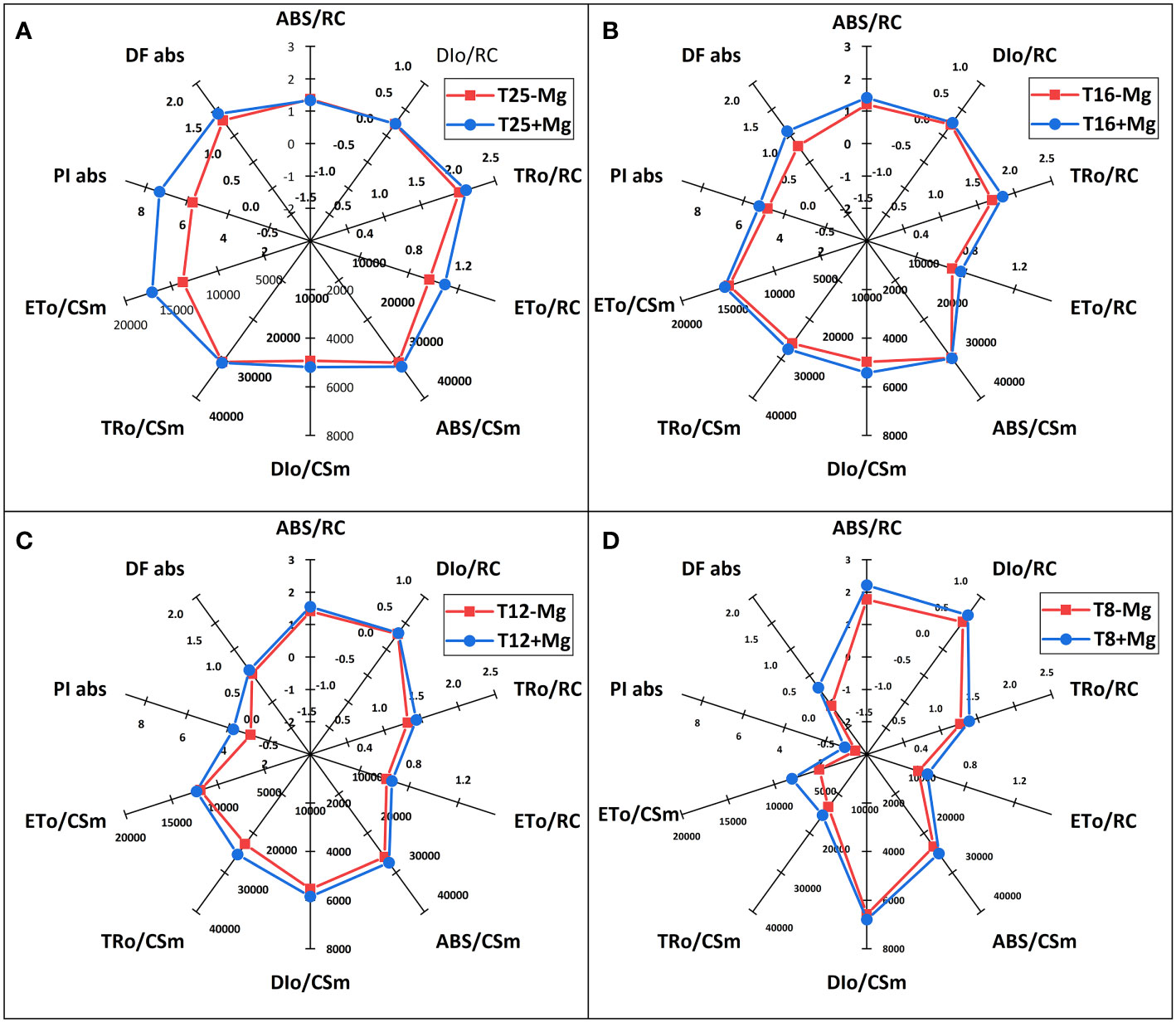
Figure 8 The JIP-test parameters in tobacco leaves under different temperature and Mg treatments. (A) 25°C; (B) 16°C; (C) 12°C; (D) 8°C.
Simultaneously, photosynthesis’s energy transfer in the electron transport chain was analyzed. As the results showed, after cold stress, the light energy absorbed (ABS/RC) per active reaction center (RC) and the heat dissipation (DIo/RC) were increased, suggesting that after partial RC deactivation caused by cold stress, the energy dissipation efficiency of the remaining active RC improved, which increased the burden of active RC, to resist cold environmental stress. However, +Mg treatment significantly alleviates this procedure. Additionally, cold stress decreased the excitation energy used to reduce QA (TRo/RC) and the electron transfer capacity (ETo/RC) of a single RC in tobacco, while +Mg treatment significantly alleviated the decline of ETo/RC, causing more energy to enter the electron transfer, which was conducive to maintaining the normal operation of the PSΠ linear electron transfer and increasing the tobacco’s resistance to adversity, especially cold stress.
The phenomenological energy fluxes per the exciting cross sections (CS) of the grapevine leaves during the growing season are presented in Figure 9. 25°C measurements, the leaves were characterized by relatively high energy fluxes, which significantly decreased at 8°C. The rapid decrease of absorbed energy (ABS/RC) and electron transport per cross-section (ET/CS) was observed between 8-25°C (Figure 9). Then, the photosynthetic apparatus was deactivated gradually. The dissipated energy (DI/CS) did not change significantly at different temperatures but gradually increased after magnesium was added. The presented results indicate that the change of temperature (chilling stress) is the main factor of photosynthetic damage in tobacco leaves, and adding magnesium can improve the resistance to photosynthetic damage to some extent.
Principal component analysis
The principal component analysis (PCA) clustered the input variables of temperature and Mg into different groups (Figure 10). The first two components accounted for 82.1% (68.3% for PC1 and 13.8% for PC2) of the total variation for morphological traits (SFW, RFW, SDW, RDW), nutrients contents (shoot C (S.C), root C (R.C), shoot N (S.N), root N (R.N), shoot K (S.K), root K (R.K), shoot P (S.P), root P (R.P), shoot Mg (S.Mg), root Mg (R.Mg)), photosynthetic traits (Gs, Ci, Pn, Chl-a, Chl-b, Chl-a+b, Cart, ABS/RC, DIO/RC, TRO/RC, ETO/RC, ABS/CSm, DIO/CSm, TRO/CSm, ETO/CSm, PIabs, DFabs), and quality attributes (sucrose and starch), and overall these showed the best performance in under +Mg at 25°C.
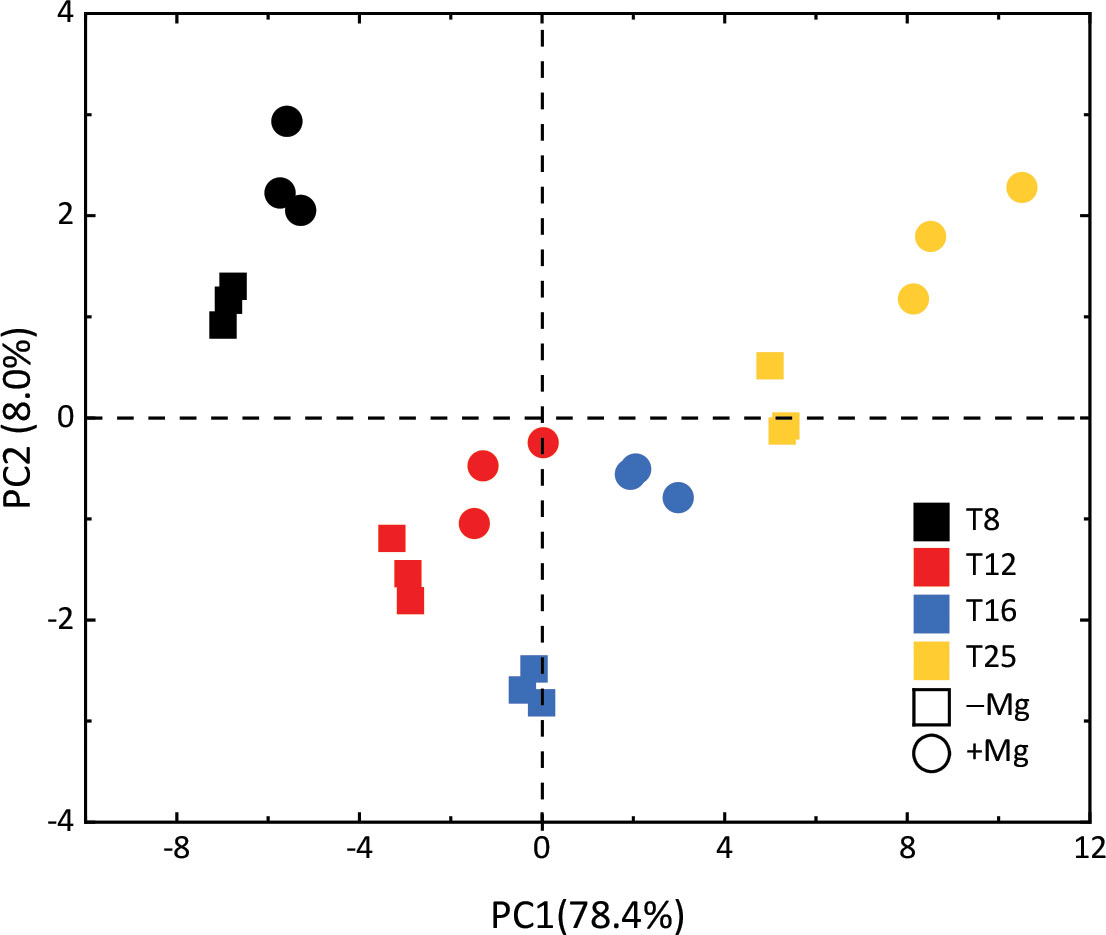
Figure 10 The principal component analysis (PCA) clustered the input variables of temperature and Mg into different groups.
Discussion
Tobacco is extremely sensitive to low temperatures, and cold stress is one of the critical abiotic stresses that significantly impact tobacco seedling growth and development (Yan et al., 2002; Zeng and Liu, 2006). Unpredictable lowering of temperature is an alarming threat to sustainable tobacco production and quality. The detrimental impacts of this low temperature can be alleviated by applying Mg, which is otherwise critical for plants to stay green under various stresses. For various reasons, the potential role of Mg under cold stress for improving tobacco growth, yield, and quality remain largely unknown. Considering the importance of Mg in improving chlorophyll synthesis and crop yield and quality (Tränkner et al., 2016), it is a matter of great interest to investigate its effects under varying levels of cold stress in tobacco.
Tobacco seedlings subjected to cold under stress under +Mg resulted in improved morphological traits in terms of SFW, RFW, and SDW (Figure 2). These findings are consistent with the previous findings of Mengutay et al. (2013) who found that an adequate supply of Mg significantly increased the shoot dry weight of wheat. This significant increase in biomass (i.e., SFW, RFW, SDW, and RDW) could be attributed to an increase in leaf area (Weraduwage et al., 2015) that provides higher light interception for chlorophyll synthesis (Weraduwage et al., 2015) and results in increased plant biomass. The improved root length could explain the other possible reason because Mg application significantly increases the root length that forages the soil nutrients extensively, improves the nutrient uptake, and increases plant biomass (Huang et al., 2021). The lowest plant biomass was recorded at 8°C compared to 25°C, and consistent with earlier studies that cold stress of 5°C significantly decreased the tobacco biomass (Wang Y. et al., 2019) because of the yellowing of leaves and inhibited photosynthetic activity. Hence, Mg supplementation can effectively alleviate this effect and promote the tobacco growth under cold stress, and may contribute to increase in biomass of tobacco plant.
The current study also showed the interesting results of higher root and shoot nutrients contents under +Mg treatment compared with –Mg for cold stress (Figure 3), suggesting the relevance of the cause-effect relationship between temperature and nutrients contents, and this effect was enhanced in the presence of Mg, and line with previous findings (Soniya et al., 2018; Dietrich et al., 2020; Perazzoli et al., 2020; Geng et al., 2021). The nutrient uptake takes place primarily through root interception (Onwuka and Mang, 2018), and the addition of Mg has a positive effect on root length, and as a result, nutrient uptake is increased (Huang et al., 2021). Moreover, it has been found that temperature and nutrient uptake had a positive relationship, and these findings were supported by the previous studies where the authors demonstrated that increasing temperature could significantly promote N, P, K, and Mg uptake (Ropokis et al., 2019; Fan et al., 2020; Vu et al., 2020). Temperature is also an important environmental factor for plant growth and development; hence, the optimum temperature may accelerate the biochemical reactions for better plant growth (Zhang et al., 2019; MacAlister et al., 2020).
Changes in photosynthetic characteristics in response to stress have received much attention in recent years. Hence, in this study, we also characterize the photosynthetic traits. We found higher chlorophyll contents, including Chl-a, Chl-b, and Cart under +Mg, and the previous finding also showed a positive correlation between chlorophyll contents and Mg (Peng et al., 2019). We found chlorophyll contents increased with increasing temperature and maximum at 25°C, suggesting a positive correlation between them, and similar results have been reported in wheat and rice (Kumar Tewari and Charan Tripathy, 1998; Zhao et al., 2020). The increase in PSII efficiency was associated with increased intracellular CO2 (Ci) because Mg application generally increases CO2 fixation, transportation, and distribution of photoassimilates that contribute to improved plant growth (Jia et al., 2021). This is consistent with our findings that gas exchange parameters, including gs, Ci, and Pn, were significantly increased under +Mg treatment and decreased under –Mg (Figure 5). Similar findings have been reported in broad beans (Hariadi and Shabala, 2004), indicating that Mg deficient leaves showed a decrease in Pn and vice versa. It has also been cited that Mg deficiency changes the physiological functioning of the PSII of sugar beet by decreasing the chlorophyll contents (Hermans et al., 2004). It has also been observed by other researchers that Mg deficiency results in a negative effect on photosynthesis electron transport capacity (Yang et al., 2012; Ye et al., 2019). These findings suggested that a decrease in Pn is associated with a decrease in gs and Ci.
Sucrose and starch are known as important tobacco quality indicators. It has been reported that temperature also efficiently induces sucrose and starch biosynthesis (Zhang B. et al., 2020). Plant Mg status has a pronounced impact on the transport and utilization of photosynthates, thereby significantly influencing the carbohydrate partitioning between source and sink organs (Cakmak and Kirkby, 2008). In this study, we found that sucrose and starch contents significantly improved under +Mg treatment and in line with the previous findings (Liu et al., 2008; Gerendás and Führs, 2013; Senbayram et al., 2015). It could be due to improved photosynthesis activity because various studies have elucidated that Mg has a significant role in photosynthesis by increasing chlorophyll contents (Jiang et al., 2015; Jia et al., 2021). We found that increasing temperature (25°C) increased the sugar contents compared to 8°C. The field experiments also showed a positive correlation between the temperature and sugar contents because the temperature is the control factor of enzymes in the biochemical process. Hence, with the increase in temperature, the enzymatic reaction increases, and the accumulation of carbohydrates also accelerates and results in improved sucrose and starch (Abidi et al., 2015; Sohag et al., 2020; Vosnjak et al., 2021).
The comprehensive analysis of PCA also showed the variation in morphological, the concentration of mineral nutrients, photosynthetic traits, and quality parameters for +Mg and –Mg treatments under cold stress (Figure 9). Similar results have also been reported that variation in morphological traits and mineral nutrients and photosynthetic traits can be attributed to the application of Mg (Ye et al., 2019; Jia et al., 2021).
Conclusion
Mg is an essential nutrient for plant growth and development, as it is involved in various physiological and biochemical processes such as photosynthesis and enzymatic activities. However, the role of Mg in improving plant growth and development under cold stress has not been fully elucidated. This study confirmed that the addition of Mg improved the photosynthetic system and nutrient uptake of tobacco plants under cold stress. The addition of Mg increased the chlorophyll content and photosynthetic rate, and increased the carbohydrate to improve the tolerance of tobacco plants. These results confirmed that magnesium application had significant protective effect on cold stress of tobacco. Further investigation is needed in tobacco fields under various cold stress scenarios by considering the Mg application to improve the tobacco yield and quality.
Data availability statement
The original contributions presented in the study are included in the article. Further inquiries can be directed to the corresponding authors.
Author contributions
JL: Experimental set-up and parameters determination, data analysis, writing-original draft, software, visualization. MM: writing and reviewing. AS, QG and YW: Parameters determination, data curation and software. HZ: Writing-reviewing. WL: Investigation, conceptualization, writing-reviewing. CZ: Conceptualization, data curation, writing-reviewing and editing. All the authors contributed to the article and approved the submitted version.
Funding
This research was funded by the Research Foundation of Fujian Provincial Tobacco Monopoly Bureau (2019350000240009), the Research Foundation of Guangdong Provincial Engineering & Technology Research Center for Tobacco Breeding and Comprehensive Utilization (202002), the Open Research Foundation of International Magnesium Institute (IMI2018-09) and the Science and Technology Innovation Foundation of FAFU (CXZX2020076A).
Conflict of interest
The authors declare that the research was conducted in the absence of any commercial or financial relationships that could be construed as a potential conflict of interest.
Publisher’s note
All claims expressed in this article are solely those of the authors and do not necessarily represent those of their affiliated organizations, or those of the publisher, the editors and the reviewers. Any product that may be evaluated in this article, or claim that may be made by its manufacturer, is not guaranteed or endorsed by the publisher.
References
Abidi, W., Cantín, C. M., Jiménez, S., Giménez, R., Moreno, MÁ, Gogorcena, Y. (2015). Influence of antioxidant compounds, total sugars and genetic background on the chilling injury susceptibility of a non-melting peach (Prunus persica (L.) batsch) progeny. J. Sci. Food Agric. 95, 351–358. doi: 10.1002/jsfa.6727
Anwar, A., Yan, Y., Liu, Y., Li, Y., Yu, X. (2018). 5-aminolevulinic acid improves nutrient uptake and endogenous hormone accumulation, enhancing low-temperature stress tolerance in cucumbers. Int. J. Mol. Sci. 19, 3379. doi: 10.3390/ijms19113379
Banerjee, A., Roychoudhury, A. (2019). Cold stress and photosynthesis. Photosynthesis productivity Environ. Stress, 27–37. doi: 10.1002/9781119501800.ch2
Cakmak, I., Kirkby, E. A. (2008). Role of magnesium in carbon partitioning and alleviating photooxidative damage. Physiologia plantarum 133, 692–704. doi: 10.1111/j.1399-3054.2007.01042.x
Chen, Z. C., Peng, W. T., Li, J., Liao, H. (2018). Functional dissection and transport mechanism of magnesium in plants. Semin. Cell Dev. Biol. 74, 142–152. doi: 10.1016/j.semcdb.2017.08.005
Cui, C., Wang, L., Zhou, Q., Tan, Z., Qu, C., Zhang, Z. (2014). Expression profiling of genes related to photosynthesis and antioxidant capacity in flue-cured tobacco seedlings subjected to chilling stress. Acta Ecol. Sin. 34, 6076–6089. doi: 10.5846/stxb201310242565
Dietrich, C. C., Rahaman, M. A., Robles-Aguilar, A. A., Latif, S., Intani, K., Müller, J., et al. (2020). Nutrient loaded biochar doubled biomass production in juvenile maize plants (Zea mays L.). Agronomy 10, 567. doi: 10.3390/agronomy10040567
Fan, X., Xu, D., Wang, D., Wang, Y., Zhang, X., Ye, N. (2020). Nutrient uptake and transporter gene expression of ammonium, nitrate, and phosphorus in ulva linza: adaption to variable concentrations and temperatures. J. Appl. Phycology 32, 1311–1322. doi: 10.1007/s10811-020-02050-2
Farhat, N., Elkhouni, A., Zorrig, W., Smaoui, A., Abdelly, C., Rabhi, M. (2016). Effects of magnesium deficiency on photosynthesis and carbohydrate partitioning. Acta physiologiae plantarum 38, 145. doi: 10.1007/s11738-016-2165-z
Forner, A., Vilana, R., Bianchi, L., Rodríguez-Lope, C., Reig, M., García-Criado, MÁ, et al. (2015). Lack of arterial hypervascularity at contrast-enhanced ultrasound should not define the priority for diagnostic work-up of nodules< 2 cm. J. Hepatol. 62, 150–155. doi: 10.1016/j.jhep.2014.08.028
Gechev, T., Willekens, H., Van Montagu, M., Inzé, D., Van Camp, W., Toneva, V., et al. (2003). Different responses of tobacco antioxidant enzymes to light and chilling stress. J. Plant Physiol. 160, 509–515. doi: 10.1078/0176-1617-00753
Geng, G., Cakmak, I., Ren, T., Lu, Z., Lu, J. (2021). Effect of magnesium fertilization on seed yield, seed quality, carbon assimilation and nutrient uptake of rapeseed plants. Field Crops Res. 264, 1082. doi: 10.1016/j.fcr.2021.108082
Gerendás, J., Führs, H. (2013). The significance of magnesium for crop quality. Plant Soil 368, 101–128. doi: 10.1007/s11104-012-1555-2
Guo, W., Nazim, H., Liang, Z., Yang, D. (2016). Magnesium deficiency in plants: An urgent problem. Crop J. 4, 83–91. doi: 10.1016/j.cj.2015.11.003
Hariadi, Y., Shabala, S. (2004). Screening broad beans (Vicia faba) for magnesium deficiency. II. photosynthetic performance and leaf bioelectrical responses. Funct. Plant Biol. 31, 539–549. doi: 10.1071/FP03202
He, X., Liu, T., Ren, K., Chen, J., Zhao, G., Hu, B., et al. (2020). Salicylic acid effects on flue-cured tobacco quality and curing characteristics during harvesting and curing in cold-stressed fields. Front. Plant Sci. 11. doi: 10.3389/fpls.2020.580597
Hermans, C., Johnson, G. N., Strasser, R. J., Verbruggen, N. (2004). Physiological characterisation of magnesium deficiency in sugar beet: acclimation to low magnesium differentially affects photosystems I and II. Planta 220, 344–355. doi: 10.1007/s00425-004-1340-4
Huang, X., Muneer, M. A., Li, J., Hou, W., Ma, C., Jiao, J., et al. (2021). Integrated nutrient management significantly improves pomelo (Citrus grandis) root growth and nutrients uptake under acidic soil of southern China. Agronomy 11, 1231. doi: 10.3390/agronomy11061231
Jiang, C., Zu, C., Shen, J., Shao, F., Li, T. (2015). Effects of selenium on the growth and photosynthetic characteristics of flue-cured tobacco (Nicotiana tabacum l.). Acta Societatis Botanicorum Poloniae 84, 71–77. doi: 10.5586/asbp.2015.006
Jia, Y., Xu, H., Wang, Y., Ye, X., Lai, N., Huang, Z., et al. (2021). Differences in morphological and physiological features of citrus seedlings are related to mg transport from the parent to branch organs. BMC Plant Biol. 21, 1–16. doi: 10.1186/s12870-021-03028-z
Jin, Y., Zhang, C., Yang, H., Yang, Y., Huang, C., Tian, Y., et al. (2011). Proteomic analysis of cold stress responses in tobacco seedlings. Afr. J. Biotechnol. 10, 18991–19004. doi: 10.5897/AJB11.900
Koch, M., Busse, M., Naumann, M., Jákli, B., Smit, I., Cakmak, I., et al. (2019). Differential effects of varied potassium and magnesium nutrition on production and partitioning of photoassimilates in potato plants. Physiologia plantarum 166, 921–935. doi: 10.1111/ppl.12846
Kumar Tewari, A., Charan Tripathy, B. (1998). Temperature-stress-induced impairment of chlorophyll biosynthetic reactions in cucumber and wheat. Plant Physiol. 117, 851–858. doi: 10.1104/pp.117.3.851
Lee, T. D., Barrott, S. H., Reich, P. B. (2011). Photosynthetic responses of 13 grassland species across 11 years of free-air CO2 enrichment is modest, consistent and independent of n supply. Global Change Biol. 17, 2893–2904. doi: 10.1111/j.1365-2486.2011.02435.x
Lichtenthaler, H., Buschmann, C., Knapp, M. (2005). How to correctly determine the different chlorophyll fluorescence parameters and the chlorophyll fluorescence decrease ratio RFd of leaves with the PAM fluorometer. Photosynthetica 43, 379–393. doi: 10.1007/s11099-005-0062-6
Li, Y., Ren, K., Hu, M., He, X., Gu, K., Hu, B., et al. (2021). Cold stress in the harvest period: effects on tobacco leaf quality and curing characteristics. BMC Plant Biol. 21, 1–15. doi: 10.1186/s12870-021-02895-w
Liu, H., Chen, X., Chen, R., Song, S., Sun, G. (2008). Effects of magnesium deficiency on growth and photosynthesis of flowering Chinese cabbage. Acta Hortic. 767, 169–175. doi: 10.17660/ActaHortic.2008.767.16
Liu, H., Xia, Y., Fan, H., Xu, Q., Du, S., Fang, Z., et al. (2018). Effect of imidazolium-based ionic liquids with varying carbon chain lengths on Arabidopsis thaliana: response of growth and photosynthetic fluorescence parameters. J. hazardous materials 358, 327–336. doi: 10.1016/j.jhazmat.2018.06.046
MacAlister, D., Muasya, A. M., Crespo, O., Ogola, J. B., Maseko, S. T., Valentine, A. J., et al. (2020). Effect of temperature on plant growth and stress tolerant traits in rooibos in the Western cape, south Africa. Scientia Hortic. 263, 109137. doi: 10.1016/j.scienta.2019.109137
Mengutay, M., Ceylan, Y., Kutman, U., Cakmak, I. (2013). Adequate magnesium nutrition mitigates adverse effects of heat stress on maize and wheat. Plant Soil 368, 57–72. doi: 10.1007/s11104-013-1761-6
Onwuka, B., Mang, B. (2018). Effects of soil temperature on some soil properties and plant growth. Advance Plants Agric. Res. 8, 34. doi: 10.15406/apar.2018.08.00288
Peng, Y. Y., Liao, L. L., Liu, S., Nie, M. M., Li, J., Zhang, L. D., et al. (2019). Magnesium deficiency triggers SGR-mediated chlorophyll degradation for magnesium remobilization. Plant Physiol. 181, 262–275. doi: 10.1104/pp.19.00610
Perazzoli, B. E., Pauletti, V., Quartieri, M., Toselli, M., Gotz, L. F. (2020). Changes in leaf nutrient content and quality of pear fruits by biofertilizer application in northeastern Italy. Rev. Bras. Fruticultura 42, e-530. doi: 10.1590/0100-29452020530
Prasad, T. K., Anderson, M. D., Martin, B. A., Stewart, C. R. (1994). Evidence for chilling-induced oxidative stress in maize seedlings and a regulatory role for hydrogen peroxide. Plant Cell 6, 65–74. doi: 10.2307/3869675
Ropokis, A., Ntatsi, G., Kittas, C., Katsoulas, N., Savvas, D. (2019). Effects of temperature and grafting on yield, nutrient uptake, and water use efficiency of a hydroponic sweet pepper crop. Agronomy 9, 110. doi: 10.3390/agronomy9020110
Rude, R. K., Gruber, H. E. (2004). Magnesium deficiency and osteoporosis: animal and human observations. J. Nutr. Biochem. 15, 710–716. doi: 10.1016/j.jnutbio.2004.08.001
Senbayram, M., Gransee, A., Wahle, V., Thiel, H. (2015). Role of magnesium fertilisers in agriculture: plant-soil continuum. Crop Pasture Sci. 66, 1219–1229. doi: 10.1071/CP15104
Sharma, N., Singh, V., Lee, Y., Kumar, S., Rai, P., Pathak, A., et al. (2020). Analysis of mineral elements in medicinal plant samples using LIBS and ICP-OES. Atomic Spectroscopy 41, 234–241. doi: 10.46770/AS.2020.06.003
Sohag, A. A. M., Tahjib-Ul-Arif, M., Afrin, S., Khan, M. K., Hannan, M. A., Skalicky, M., et al. (2020). Insights into nitric oxide-mediated water balance, antioxidant defence and mineral homeostasis in rice (Oryza sativa L.) under chilling stress. Nitric. Oxide 100, 7–16. doi: 10.1016/j.niox.2020.04.001
Soniya, V., Bhindhu, P., Sureshkumar, P. (2018). Critical level of magnesium for cowpea [Vigna unguiculata (L.) walp.] in ultisols of kerala. Legume Research-An Int. J. 1, 5. doi: 10.18805/LR-4267
Tränkner, M., Jákli, B., Tavakol, E., Geilfus, C. M., Cakmak, I., Dittert, K., et al. (2016). Magnesium deficiency decreases biomass water-use efficiency and increases leaf water-use efficiency and oxidative stress in barley plants. Plant Soil 406, 409–423. doi: 10.1007/s11104-016-2886-1
Van Heerden, P., Kruger, G. (2000). Photosynthetic limitation in soybean during cold stress. South Afr. J. Sci. 96, 201–206.
Verbruggen, N., Hermans, C. (2013). Physiological and molecular responses to magnesium nutritional imbalance in plants. Plant Soil 368, 87–99. doi: 10.1007/s11104-013-1589-0
Vosnjak, M., Sircelj, H., Hudina, M., Usenik, V. (2021). Response of chloroplast pigments, sugars and phenolics of sweet cherry leaves to chilling. Sci. Rep. 11, 1–13. doi: 10.1038/s41598-021-86732-y
Vu, D. H., Stuerz, S., Asch, F. (2020). Nutrient uptake and assimilation under varying day and night root zone temperatures in lowland rice. J. Plant Nutr. Soil Sci. 183, 602–614. doi: 10.1002/jpln.201900522
Wang, Z., Hassan, M. U., Nadeem, F., Wu, L., Zhang, F., Li, X. (2020). Magnesium fertilization improves crop yield in most production systems: A meta-analysis. Front. Plant Sci. 10, 1727. doi: 10.3389/fpls.2019.01727
Wang, L., Shan, T., Xie, B., Ling, C., Shao, S., Jin, P., et al. (2019). Glycine betaine reduces chilling injury in peach fruit by enhancing phenolic and sugar metabolisms. Food Chem. 272, 530–538. doi: 10.1016/j.foodchem.2018.08.085
Wang, Y., Wang, G., Zheng, Y., Zheng, Y., Li, S., Shao, J., et al. (2019). Polyamines are involved in chilling tolerance in tobacco (Nicotiana tabacum) seedlings. Plant Growth Regul. 89, 153–166. doi: 10.1007/s10725-019-00521-2
Wang, S., Zhao, H., Zhao, L., Gu, C., Na, Y., Xie, B., et al. (2020). Application of brassinolide alleviates cold stress at the booting stage of rice. J. Integr. Agric. 19, 975–987. doi: 10.1016/S2095-3119(19)62639-0
Weraduwage, S. M., Chen, J., Anozie, F. C., Morales, A., Weise, S. E., Sharkey, T. D. (2015). The relationship between leaf area growth and biomass accumulation in Arabidopsis thaliana. Front. Plant Sci. 6, 167. doi: 10.3389/fpls.2015.00167
Xu, S., Li, Y., Jin, H., Guan, Y., Zheng, Y., Zhu, S. (2010). Responses of antioxidant enzymes to chilling stress in tobacco seedlings. Agric. Sci. China 9, 1594–1601. doi: 10.1016/S1671-2927(09)60256-X
Yang, Y., Wang, X., Wang, S. (2004). Effect of osmoregulation on the plasma membrane redox activity in chilling injury sensitive soybean seeds. J. Plant Physiol. Mol. Biol. 30, 589–594.
Yang, G., Yang, L., Jiang, H., Li, Y., Wang, P., Chen, L. (2012). Physiological impacts of magnesium-deficiency in citrus seedlings: photosynthesis, antioxidant system and carbohydrates. Trees 26, 1237–1250. doi: 10.1007/s00468-012-0699-2
Yan, Z., Li, F., Yao, Z., Feng, Y., Wu, Y. (2002). Effects of PEG and chilling stress on some physiological characters of tobacco seedlings. Acta Agriculturae Universitatis Jilinensis 24, 14–19.
Ye, X., Chen, X. F., Deng, C. L., Yang, L. T., Lai, N. W., Guo, J. X., et al. (2019). Magnesium-deficiency effects on pigments, photosynthesis and photosynthetic electron transport of leaves, and nutrients of leaf blades and veins in Citrus sinensis seedlings. Plants 8, 389. doi: 10.3390/plants8100389
Zeng, S., Liu, F. (2006). A study on effects of low temperature at 5° c on transgenic tobacco with SOD or POD over-expressed. Guihaia 26, 488–491.
Zhang, B., Cakmak, I., Feng, J., Yu, C., Chen, X., Xie, D., et al. (2020). Magnesium deficiency reduced the yield and seed germination in wax gourd by affecting the carbohydrate translocation. Front. Plant Sci. 11, 797. doi: 10.3389/fpls.2020.00797
Zhang, P., Grutters, B., Van Leeuwen, C. H., Xu, J., Petruzzella, A., van den Berg, R. F., et al. (2019). Effects of rising temperature on the growth, stoichiometry, and palatability of aquatic plants. Front. Plant Sci. 9, 1947. doi: 10.3389/fpls.2018.01947
Zhao, Y., Han, Q., Ding, C., Huang, Y., Liao, J., Chen, T., et al. (2020). Effect of low temperature on chlorophyll biosynthesis and chloroplast biogenesis of rice seedlings during greening. Int. J. Mol. Sci. 21, 1390. doi: 10.3390/ijms21041390
Zhao, J., Li, S., Jiang, T., Liu, Z., Zhang, W., Jian, G., et al. (2012). Chilling stress-the key predisposing factor for causing alternaria alternata infection and leading to cotton (Gossypium hirsutum l.) leaf senescence. PloS One 7, 36126. doi: 10.1371/journal.pone.0036126
Zhao, L., Liu, F., Xu, W., Di, C., Zhou, S., Xue, Y., et al. (2009). Increased expression of OsSPX1 enhances cold subfreezing tolerance in tobacco and Arabidopsis thaliana. Plant Biotechnol. J. 7, 550–561. doi: 10.1111/j.1467-7652.2009.00423.x
Keywords: low temperature, magnesium, growth, nutrients uptake, quality, photosynthesis, tobacco
Citation: Li J, Muneer MA, Sun A, Guo Q, Wang Y, Huang Z, Li W and Zheng C (2023) Magnesium application improves the morphology, nutrients uptake, photosynthetic traits, and quality of tobacco (Nicotiana tabacum L.) under cold stress. Front. Plant Sci. 14:1078128. doi: 10.3389/fpls.2023.1078128
Received: 24 October 2022; Accepted: 24 January 2023;
Published: 06 February 2023.
Edited by:
Hannetz Roschzttardtz, Pontificia Universidad Católica de Chile, ChileReviewed by:
Abdallah Oukarroum, Mohammed VI Polytechnic University, MorrocoAhmed Fathy Yousef, University of Al-Azhar, Egypt
Copyright © 2023 Li, Muneer, Sun, Guo, Wang, Huang, Li and Zheng. This is an open-access article distributed under the terms of the Creative Commons Attribution License (CC BY). The use, distribution or reproduction in other forums is permitted, provided the original author(s) and the copyright owner(s) are credited and that the original publication in this journal is cited, in accordance with accepted academic practice. No use, distribution or reproduction is permitted which does not comply with these terms.
*Correspondence: Chaoyuan Zheng, zhengcy@cau.edu.cn; Wenqing Li, li-wqfjyc@163.com
†These authors have contributed equally to this work