- 1Key Laboratory of Aquatic Organism Protection and Ecological Restoration in Cold Waters, Heilongjiang River Fisheries Research Institute, Chinese Academy of Fishery Sciences, Harbin, China
- 2Heilongjiang River Basin Fisheries Ecology Observation and Research Station of Heilongjiang Province, Harbin, China
- 3Jilin Chagan Lake National Nature Reserve Administration, Songyuan, China
Freshwater ecosystems are threatened by eutrophication, which causes persistent and harmful algal blooms. Filter-feeding bivalve mollusks and submerged macrophytes (SMs) alleviate the eutrophication effects by inhibiting phytoplankton biomass blooms. However, very little is known about whether and how the combined manipulation of filter-feeding bivalves and SMs control eutrophication and influence phytoplankton assemblages. Here, we performed a nutrient-enriched freshwater mesocosm experiment to assess the combined effects of the filter-feeding bivalve Cristaria plicata, a cockscomb pearl mussel, and the macrophyte Hydrilla verticillate on the biomass and composition of phytoplankton assemblages. We found that addition of C. plicata and H. verticillate decreased the water nutrient concentrations and suppressed overall phytoplankton biomass. Further, distinct differences in taxa between restoration and control treatments were observed and noticeably competitive exclusion of cyanobacteria in the restoration treatments occurred. An antagonistic interaction between filter-feeding bivalves and SMs was only detected for total cyanobacteria biomass demonstrating that a larger magnitude of SM restoration may override the effect of filter-feeding bivalves. Our results suggest that manipulation, through the addition of bivalves as grazers, associated with the restoration of SMs, is an efficient approach for reducing cyanobacterial blooms and alleviating eutrophication.
1. Introduction
Eutrophication of freshwater ecosystems, driven primarily by over enrichment of nitrogen (N) and phosphorus (P) (Carpenter et al., 1998), is a serious threat to water quality, biodiversity and other key ecosystem functions (Smith et al., 2006; Cook et al., 2018; Liu et al., 2021). Nutrient enrichment promotes the appearance and persistence of harmful algal blooms (Heisler et al., 2008; Conley et al., 2009) and the decline of submerged macrophytes (SMs) (Zhang et al., 2017), altering the food web structure (Fujibayashi et al., 2018; Briland et al., 2020). Occurrences of eutrophication are expected to increase with climate and land-use changes (Jeppesen et al., 2010; Bergström and Karlsson, 2019; Le Moal et al., 2019), inducing regime switches from a macrophyte‐dominated clear state to phytoplankton‐dominated turbid state (Jeppesen et al., 2007a). Considering that human activity is the primary cause of the eutrophication, it is crucial to reduce anthropogenic contributions to aquatic ecosystems and to find effective approaches to control cyanobacterial blooms that usually dominate eutrophic waterbodies.
The restoration of SMs is considered a crucial measure for the rehabilitation of shallow eutrophic lakes (Liu et al., 2020; Li et al., 2021a), as SMs display certain functional traits, that they use to stabilize the clear-water state (Puijalon et al., 2011; Su et al., 2019; Rao et al., 2020). For example, SMs could suppress algal growth by competing for light and nutrients (Lürling et al., 2006), producing algae-inhibiting allelochemicals to interfere the photosynthetic activities (Zhu et al., 2010) and change other physiological and biochemical processes (Zhu et al., 2021; He et al., 2023), and providing grazing zooplankton with a daytime refuge against fish predation (Burks et al., 2001). In addition, SMs can facilitate nutrient uptake from the water column and sediment (Sand-Jensen and Borum, 1991) and reduce sediment resuspension (Horppila and Nurminen, 2003). Earlier studies involving small-scale experiments (Barrow et al., 2019; Amorim and Moura, 2020) and natural aquatic ecosystems (Chao et al., 2022; Peng et al., 2022) have repeatedly reported that the restoration of SMs decreases the phytoplankton abundance and increases water clarity. Thus, usage of SMs is a prospective tool for the elimination of algal blooms (Jeppesen et al., 2007b). The submerged macrophyte restoration is, therefore, expected to prevent or mitigate the expansion of cyanobacterial blooms.
Another restoration technique to improve water quality is the biomanipulation of filter-feeding freshwater animals, such as mussels; however, its effectiveness remains debatable. For example, grazing studies involving filter-feeding mussels, such as zebra mussels and triangle sail mussels, in Europe and China demonstrated that they can efficiently consume pelagic algae and detritus (e.g. MacIsaac et al., 1992; Gao et al., 2017). Further, mussels, as grazers, can reduce or even prevent algal blooms (Gulati et al., 2008). Furthermore, Wu and Culver (1991) found abundant zebra mussels in Lake Erie, and noticed that filter-feeding Daphnia were able to reduce edible algal density and enhance water transparency. Interestingly, some mussels display food selectivity and avoid consuming cyanobacteria resulting in dominance of cyanobacteria over other forms (Hwang et al., 2004; Colvin et al., 2015). Contrary to Hwang et al. (2004) and Baker et al. (1998); Colvin et al. (2015) reported that the invasion of zebra mussels led to a decline in Microcystis biomass in the Hudson River.
Numerous combined technologies for controlling lake eutrophication have been developed, demonstrating that the combined effect of the two technologies was better than the technology alone. For example, the combination of large herbivorous zooplankton and submerged macrophytes proved to be more efficient at controlling the biomass of cyanobacteria (Amorim and Moura, 2020). In addition, the successful restoration of submersed macrophytes improved the water quality in a eutrophic lake after the removal of common carp (Knopik and Newman, 2018). Despite recent advances on biological restoration methods related to eutrophication, little is known about whether and how the combined manipulation of filter-feeding bivalves and SMs control eutrophication and influence phytoplankton assemblages. Given the complexities of climatically, thermally, ecologically, and hydrologically induced change in natural lakes (Richardson et al., 2019), mesocosm studies have been heralded as a useful means to investigate the effects of multiple factors under manipulated or controlled environmental conditions while supporting realistic levels of biocomplexity (Stewart et al., 2013; Fordham, 2015).
In this study, we designed a 32-day nutrient-enriched freshwater mesocosm experiment to explore the potential interactions between the filter-feeding bivalves and SMs and their impact on the biomass and composition of phytoplankton assemblages. We reasoned that categorizing cyanobacteria based on the adaptations to avoid predation (e.g. colonial and filamentous cyanobacteria) may lead to greater insights into the combined effects of filter-feeding bivalves and SMs on the restoration of eutrophic water bodies. We hypothesized that: (i) biomanipulation via addition of filter-feeding bivalves and restoration of submerged macrophyte, under nutrient enrichment, will likely affect phytoplankton assemblages and control the growth of cyanobacteria; (ii) the interactive effects are likely to be superior to either alone for controlling eutrophication.
2. Material and methods
2.1. Study site and experimental design
The outdoor mesocosm experiment was conducted between 25 June and 27 July 2021 in 16 cylindrical polyethylene mesocosms on land – at the Chagan Lake Observation and Research Station near Chagan Lake (45.25°N, 124.28°E). The mesocosms had a diameter of 1 m and a constant water depth of 1.2 m; they contained 0.2 m sediment and 780 L of unfiltered water collected from Chagan Lake (Figure 1). Chagan Lake is a shallow eutrophic freshwater lake (mean depth: 2.5 m) in a catchment area dominant by agricultural lands and grasslands and has relatively high allochthonous inputs of nutrients (especially nitrogen and phosphorus) through precipitation and surface run-off (Liu et al., 2019; Du et al., 2022). Nitrogen and phosphorus, as dissolved mixtures of sodium nitrate (NaNO3) and potassium dihydrogen phosphate (KH2PO4), respectively, were added daily to each mesocosm to equate to a nutrient load of 36 μg/L and 5 μg/L, which adhered to the Redfield ratio (Redfield, 1958). The walls of the mesocosms were scrubbed daily to prevent periphyton growth. During the experiment period, evaporation losses from the mesocosms were replaced with unfiltered lake water when not compensated for by rainfall.
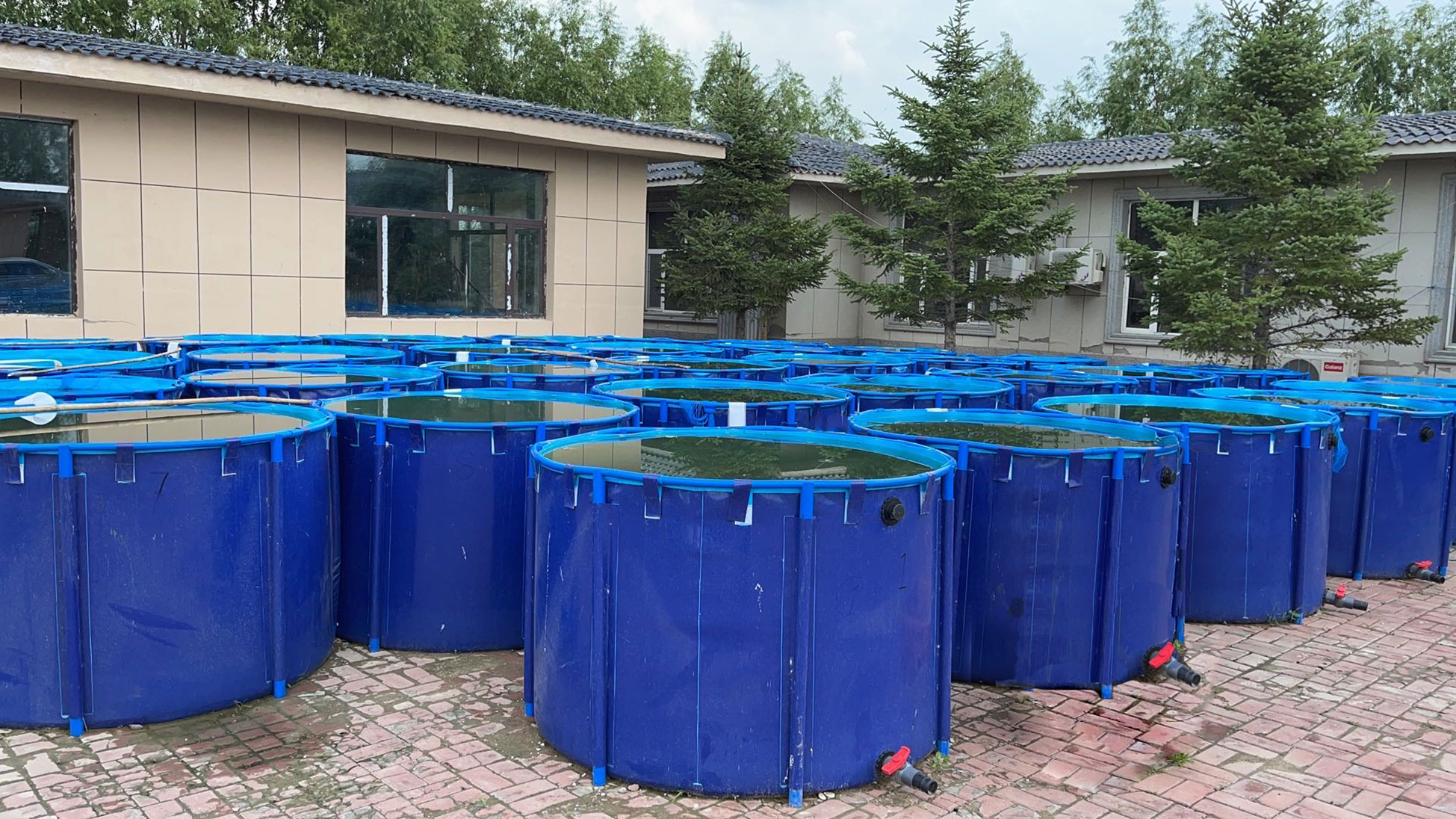
Figure 1 Experimental mesocosms used in our study at the Chagan Lake Observation and Research Station.
The experiment had a factorial design (2 x 2) to evaluate of the effects bivalves, macrophytes, and their interaction, on water nutrient concentrations and phytoplankton assemblages in mesocosms. Cristaria plicata was chosen as the filter-feeding bivalve in our mesocosms as it is an excellent cleaner of suspended particles (Yu et al., 2020), and Hydrilla verticillate, was used as the macrophyte owing to its allelopathic effects on phytoplankton (Gao et al., 2015) and nutrient removal capability (Li et al., 2021b). Three treatments (bivalve: C. plicata alone; macrophyte: H. verticillate alone; bivalve + macrophyte: C. plicata and H. verticillate together) and a control (both species absent), each one with four replicates, were randomly assigned to the mesocosms and all received a common nutrient loading over the entire experiment. The bivalve, macrophyte, and bivalve + macrophyte treatments have been proposed as strategies for mitigating eutrophication for many temperate shallow lakes, and consequently, served as the restoration treatments (Søndergaard et al., 2007; Zhang et al., 2014). We added two C. plicata with a biomass of 413.8 ± 13.6 standard error (S.E.) g/m2 to the bivalve treatment mesocosms. C. plicata were hung with string bags, 30 cm above the sediment surface. Individual H. verticillate samples were purchased from a commercial nursery. At the beginning of the experiment, the average stem length of H. verticillate was 34 ± 0.8 S.E. cm and they were bundled together in groups of five to eight and weighted down in the sediment to encourage root growth. The total wet weight of macrophytes within each mesocosm was 650 ± 10.3 S.E. g L-1.
2.2. Sample collection and analysis
Samples of water nutrients and chlorophyll a concentrations were collected at the beginning of the experiment (day 0) and on day 4, 8, 12, 16, 20, 24, 28, and 32. The water samples were collected with a tube sampler at two different depths (surface and 5 cm above the sediment), from which subsamples were taken for water nutrients and phytoplankton analysis. We determined concentrations of total phosphorus (TP), phosphate (PO4-P), total nitrogen (TN), ammonia nitrogen (NH4-N), nitrate nitrogen (NO3-N) and nitrite nitrogen (NO2-N) using standard methods (American Public Health Association, 1992). Chlorophyll a (as a proxy of total phytoplankton biomass) concentrations determined spectrophotometrically from matter retained on Whatman GF/C glass microfiber filters after cold ethanol extraction in darkness (Jespersen and Christoffersen, 1987).
To characterize the phytoplankton assemblage composition, we collected phytoplankton from all enclosures. Phytoplankton sampling was done at the beginning (day 0) and at the end of the experiment (day 32). A subsample of the mixed tube sample water was immediately fixed with Lugol’s solution. All samples were analyzed using a Sedgewick-Rafter counting chamber and an inverted microscope (RVL-100-G, ECHO, San Diego, California, USA). At least 500 natural units were enumerated and identified to the genus level (Hu and Wei, 2006). Cell volumes of each phytoplankton taxa were calculated after approximation to the nearest geometric standard solid (Hillebrand et al., 1999). The biomass estimates were calculated, assuming that the density of the organisms equals that of water (1 mm3 L-1 = 1 mg L-1) (Wetzel and Likens, 2000). As chlorophyll a (μg/L) measured using the spectrophotometric method and total phytoplankton biomass (mm3/L) estimated from microscope counts and measurements were positively correlated (R2 = 0.82, p < 0.001), we used the latter measurement to estimate the biomass of cyanobacteria genera. In the case of cyanobacteria, species were classified into colonies and filaments based on their life form.
2.3. Statistical analyses
Prior to analyses, water nutrient and chlorophyll a concentration data were natural logarithm-transformed to meet the assumptions of normality and homoscedasticity when necessary. Principal response curve (PRC) method was used to evaluate the time‐dependent influence of the bivalve (C. plicata), submerged macrophyte (H. verticillate) and their potential interactions on key water nutrient concentrations in response to nutrient enrichment. The PRC method is a special case of partial redundancy analysis (RDA) and requires repeated observations from multiple time periods in order to represent the deviation in the treatments from the controls over time (Van den Brink and Braak, 1999; Oksanen et al., 2020). The statistical significance of the PRC models was tested using the Monte Carlo permutation test (Van den Brink and Braak, 1999). The PRC analyses displayed an affinity for the different water nutrient (response) variables with the trajectory by giving each variable a quantitative score. In our study, higher scores of water nutrient variables in a restoration treatment group, resulted in more pronounced responses compared with the control treatment during the experiment (Van den Brink and Braak, 1999). Statistical differences among the control and restoration treatments at the beginning and the end of the experiment were compared using Kruskal-Wallis test. If a significant difference was found, post hoc comparisons among treatments were performed using Wilcoxon test.
Subsequently, we investigated the effects of bivalve addition (bivalve), macrophyte addition (macrophyte), and their interaction (bivalve + macrophyte) on phytoplankton biomass (chlorophyll a concentration). For chlorophyll a concentrations collected multiple times (i.e., on day 0, 4, 8, 12, 16, 20, 24, 28, and 32), we performed a two-way repeated measures ANOVA (RM-ANOVA) using a restricted maximum likelihood (REML) method. If there was a main effect of bivalve, macrophyte, or their interaction, we performed post-hoc analyses on the data under each treatment. If there was a significant (p < 0.05) interaction with time, we performed post-hoc analyses on the data within each sampling time.
The shifts in phytoplankton assemblage composition over time and across treatments were evaluated using a multivariate ordination technique: principal coordinate analyses (PCoA). The PCoA was performed using Hellinger-transformed species data (Legendre and Gallagher, 2001) and a Bray-Curtis dissimilarity matrix. The PCoA was paired with a permutational multivariate analysis of variance (PERMANOVA; Anderson, 2001; Oksanen et al., 2020) to test for statistically significant differences in phytoplankton assemblage composition in different treatments with an F-type test (999 permutations) using the same dissimilarity matrix (Bray-Curtis) and transformed species data.
The effect of the addition of filter-feeding bivalves and SMs on the biomass of the cyanobacteria genera was tested using a generalised linear mixed-effects model (GLMM; Bolker et al., 2009; Harrison et al., 2018) with a normal distribution. In separate analyses, dependent variable were (i) total biomass of cyanobacteria, (ii) biomass of filamentous cyanobacteria, and (iii) biomass colonial cyanobacteria. Models were fitted using bivalve, macrophyte and their interaction as fixed effects. All models included mesocosm identity as a random effect. We reported the GLMM marginal R2 (R2 m) that describes the variance explained by the fixed effects alone, and the conditional R2 (R2 C) that describes the variance explained by both fixed and random effects (Nakagawa and Schielzeth, 2013).
Statistical analyses were performed using R statistical (version 4.0.3) software (R Core Team, 2020). The PRC, PCoA and PERMANOVA were performed using the vegan package version 2.5-7 (Oksanen et al., 2020). The RM-ANOVA was performed using the ez package version 4.4-0 (Lawrence, 2016). We conducted GLMM using the glmmTMB package version 1.1.3 (Brooks et al., 2017). The MuMIn package version 1.46.0 (Bartoń, 2022) was used to generate the R2 value of each model.
3. Results
3.1. Treatment effects on physicochemical parameters
No significant differences were found for the physicochemical parameters among the treatments at the beginning of the experiment (Kruskal-Wallis test: P > 0.05; supplementary Table S1, Figure S1). At the end of the experiment, the biomass of C. plicata and the total wet weight of macrophyte increased to 450.2 ± 12.8 standard error (S.E.) g/m2 and 3257.6 ± 52.9 S.E. g L-1, respectively. During the experiment, nutrient concentrations in the water shifted in parallel in the restoration treatments (i.e., bivalve, macrophyte and bivalve + macrophyte treatments) relative to the control treatment, with the strongest treatment effects apparent in the bivalve + macrophyte treatment (Figure 2A). The principal response curves (PRC) revealed that 39.2% of the total variance present in water nutrient concentrations is explained by treatment (Monte Carlo, P < 0.001). Nitrogen and phosphorus loading led to increased nutrient concentrations in the control treatment, but the decline in the nitrogen to phosphorus ratio (N: P) of restoration treatments. Total phosphorus, total nitrogen, phosphate and nitrate nitrogen had high positive scores (Figure 2B), with the diagram indicating a decrease with the restoration treatment mesocosms. N: P, ammonia nitrogen and nitrite nitrogen had negative scores (Figure 2B), meaning treatment-related increases. At the end of the experiment, addition of filter-feeding bivalves and restoration of submerged macrophyte significantly decreased the concentrations of total phosphorus and total nitrogen (supplementary Table S1).
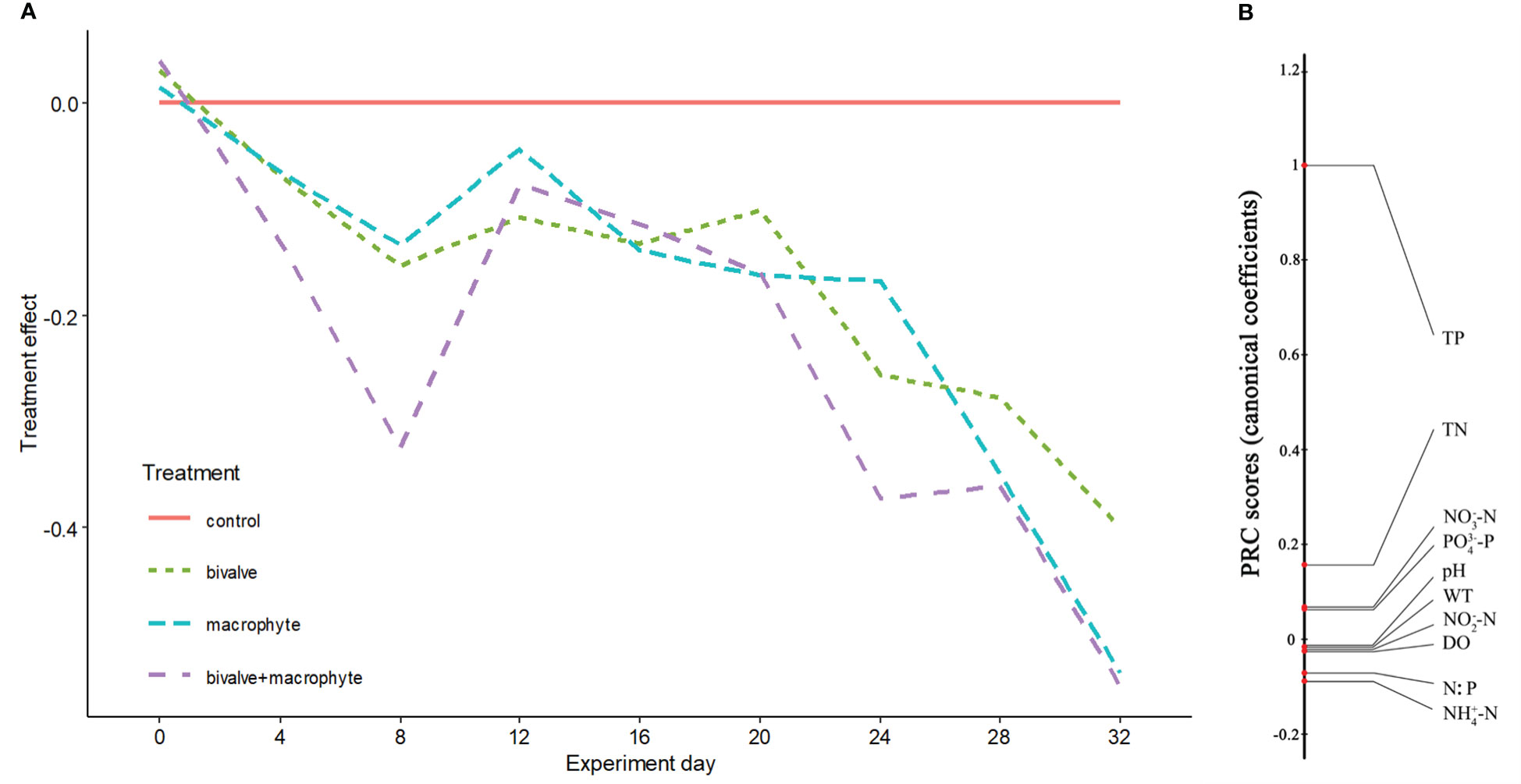
Figure 2 Principal response curves resulting from the analysis of water physicochemical variables. Panel (A) represents overall deviation from the control treatment mesocosms (control), for the other restoration treatment mesocosms (bivalve, macrophyte, and bivalve + macrophyte). This is expressed as a canonical coefficient of the first principal component axis (PC1), in comparison with the reference control mesocosms, represented by the zero line. Panel (B) shows canonical coefficients for the water physicochemical variables interpreted.
3.2. Treatment effects on total phytoplankton
The two-way repeated measures ANOVA results revealed that bivalves (F1, 3 = 118.6, p < 0.001), macrophytes (F1, 3 = 39.7, p = 0.008), and their interactions (F1, 3 = 31.2, p = 0.011) had significant effects on phytoplankton biomass. We found a significant decline in chlorophyll a concentrations in the bivalve treatment after day 12 (p < 0.05), in addition, a significant decline in the bivalve + macrophyte treatment after day 8 (p < 0.05). Chlorophyll a concentrations markedly increased in the control treatment but decreased in the bivalve, and bivalve + macrophyte treatments (Figure 3). Chlorophyll a concentrations remained at a relatively stable level in macrophyte treatment (p > 0.05). At the end of the experiment, restoration mesocosms contained 63.7-91.8% less phytoplankton than those of the control treatment. Our analysis indicated a time-by-bivalve interaction (F1, 3 = 12.8, p = 0.037). After day 16, chlorophyll a concentrations were significantly lower in both bivalve and bivalve + macrophyte treatments than those in macrophyte treatment (Figure S2).
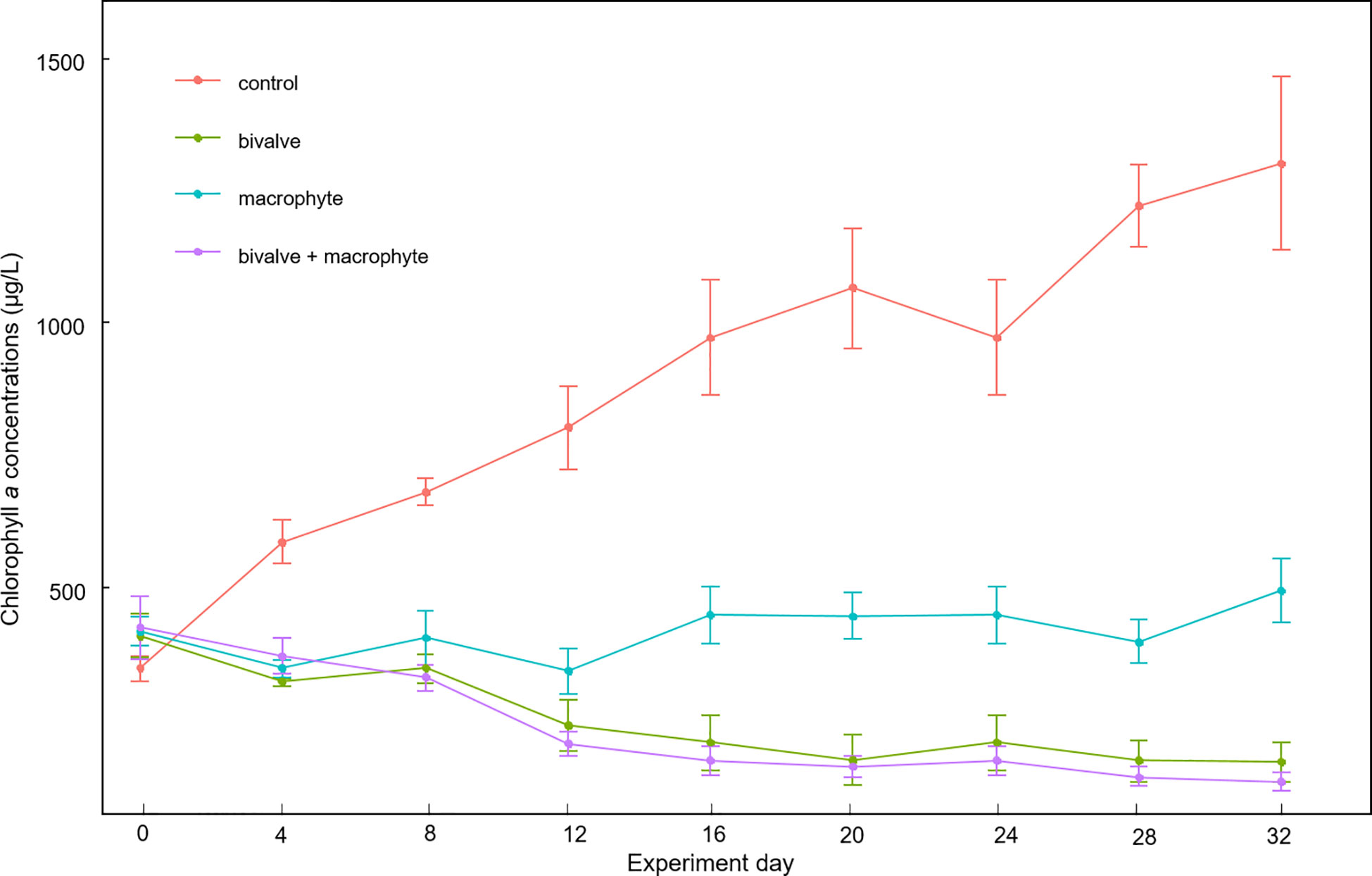
Figure 3 Mean values (± standard error) of temporal variations of chlorophyll a concentrations throughout the experiment for the different treatments.
We found a total of 68 phytoplankton genera throughout the experiment, with representatives from the following classes: Cyanophyceae (14), Bacillariophyceae (14), Chlorophyceae (34), Cryptophyceae (2) and Euglenophyceae (4) (supplementary Table S2). The principal coordinate analysis (PCoA) explained 44.36% of the species composition distribution through the first two axes (Figure 4). Initially (day 0), no treatments differed significantly in phytoplankton composition (P > 0.05; Table 1), and chlorophytes dominated the phytoplankton assemblage. By the end of the experiment (day 32), the phytoplankton compositions of the restoration treatments were significantly distinguishable from the controls (Figure 4). Addition of bivalves and/or macrophytes induced significant changes in phytoplankton assemblage structure (Table 1). Specifically, filamentous cyanobacteria, such as Anabaenopsis, Aphanizomenon, and Phormidium, and colonial cyanobacteria, such as Aphanocapsa, became abundant and dominant in the control treatment., while symmetrical desmids (e.g. Cosmarium, Micrasterias) tended to increase over time in the macrophyte treatment, and the large diatoms (e.g. Cymbella, Fragilaria, Thalassiosira) became dominant in the treatments with the addition of filter-feeding bivalves.
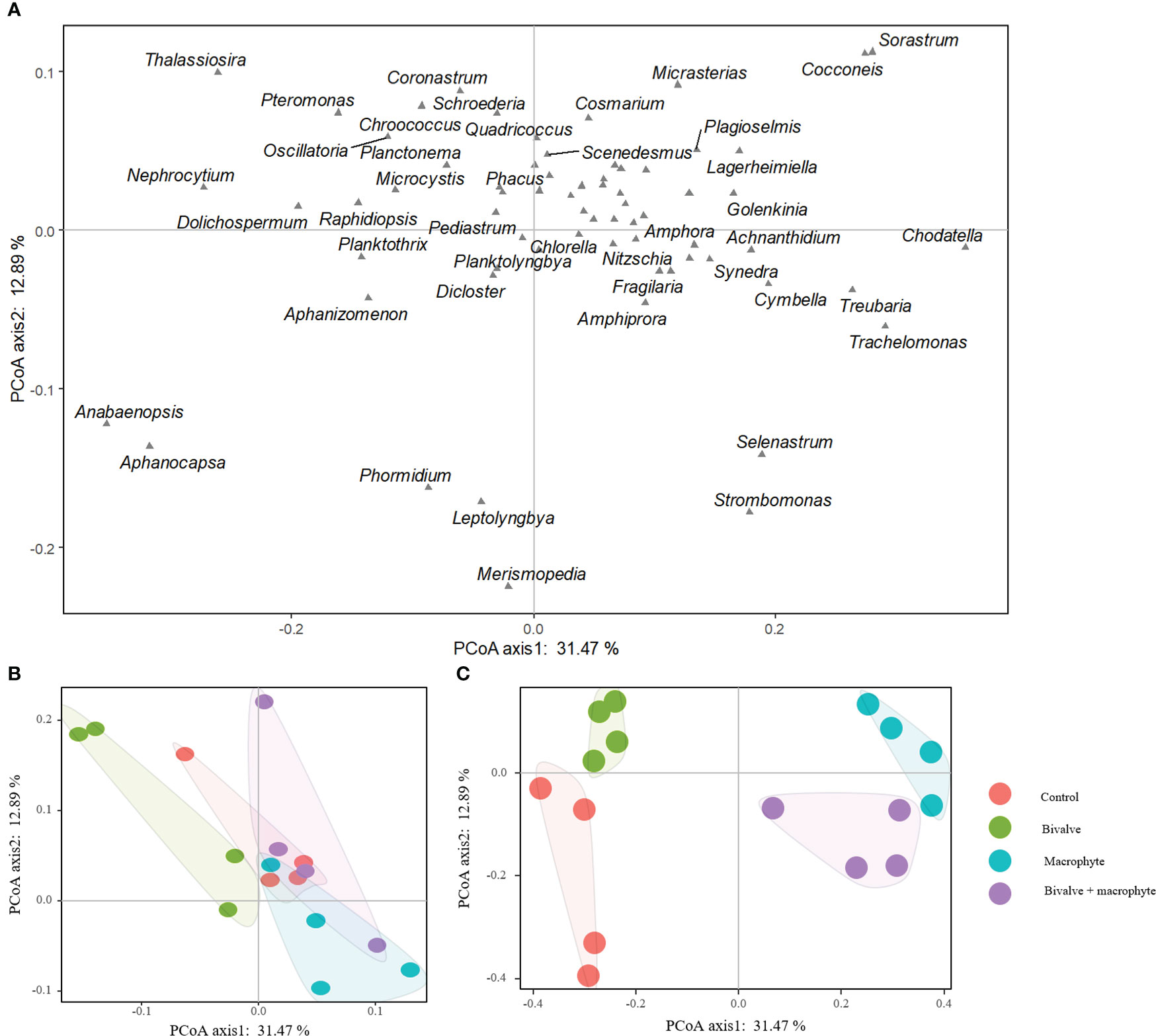
Figure 4 Two-dimensional ordination plots resulting from the principal coordinate analysis on the phytoplankton assemblage composition (A) comparing the control and restoration treatments on day 0 (B) and day 32 (C).
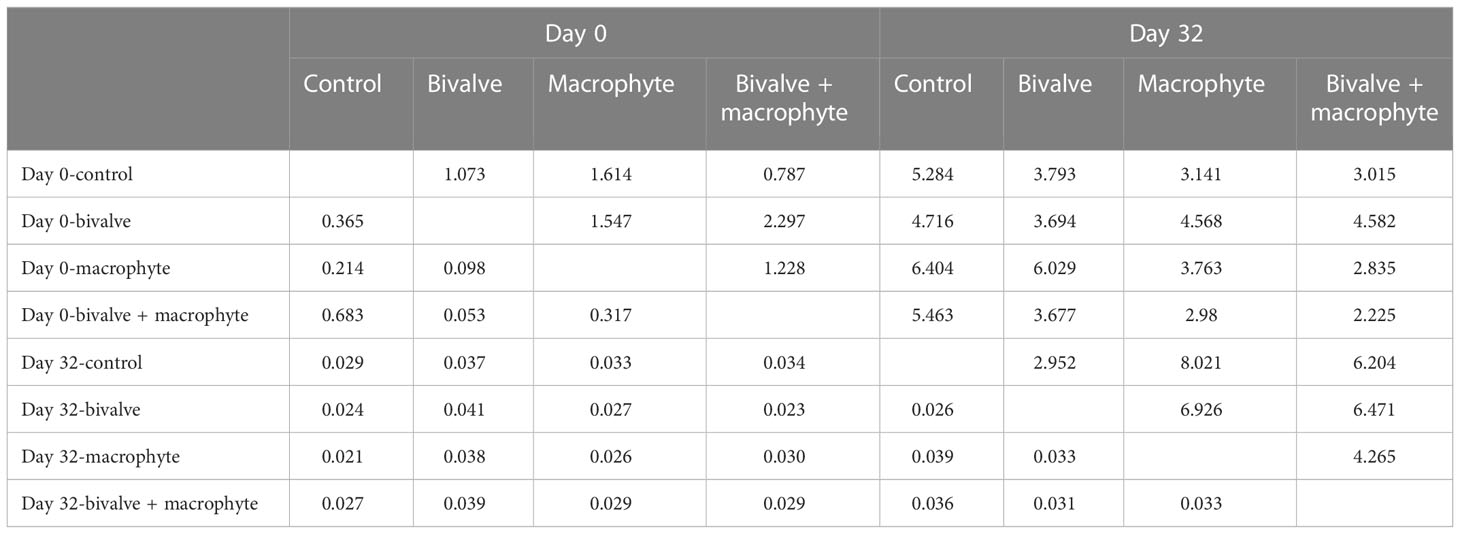
Table 1 Pseudo F-statistics (above diagonal) and p values (below diagonal) for pairwise PERMANOVA tests between the control and restoration treatments on two sampling days (day 0 and day 32). .
3.3. Treatment effects on cyanobacteria
By the end of the experiment, the total biomass of cyanobacteria was explained by a positive interaction between bivalve and macrophyte addition. Bivalve and macrophyte addition, as single restoration approaches, resulted in statistically significantly lower cyanobacteria biomass than in the control mesocosms (Figure S3). However, in combination, the effects of bivalves and macrophytes partly counterbalanced each other, resulting in a weak antagonistic interaction, where the total biomass of cyanobacteria was higher than the linearly combined (additive) effects of bivalve and macrophyte additions as single restoration techniques. Decreases in filamentous cyanobacteria and colonial cyanobacteria in response to the addition of bivalves and macrophytes as single restoration approaches were similar (Table 2). Filamentous cyanobacteria were more sensitive to macrophytes than colonial cyanobacteria, that is, filamentous cyanobacteria biomass decreased more in response to the addition of macrophytes, as single restoration techniques, than the addition of bivalves, while colonial cyanobacteria were more sensitive to bivalves.

Table 2 Summary (coefficients and SE) of a generalized linear mixed-effects model to explain variations in cyanobacteria taxa biomass as a function of bivalve (presence and absence) and macrophyte (presence and absence).
4. Discussion
The development of SMs is considered as an important restoration strategy in eutrophic shallow lakes. Often restoration experiments do not capture the intricacies related to increased nutrient loading and the amount of filter-feeding animals, instead they primarily focus on assessing the effects of SMs (e.g. Bakker et al., 2013). The use of an experimental mesocosm approach is important for investigating the complexity observed in the field and to gain a mechanistic understanding about the single and interactive effects of multiple restoration measures (Amorim and Moura, 2020; Zhang et al., 2021; Boucher-Carrier et al., 2022). During the loading experiments we noticed that restoration of eutrophic waterbodies by manipulation, i.e., addition of filter-feeding bivalves and SMs, had a marked influence on water quality and algal biomass and community composition.
Our results of TP and TN reduction in the restoration treatments demonstrated that the addition of the filter-feeding bivalve C. plicata and the recovery of submerged macrophyte can significantly alleviate eutrophication. Although some species of rooted SMs are sensitive to relatively high nutrients and consequently get suppressed under eutrophic conditions (Søndergaard et al., 2010), in our study, the use of H. verticillata reduced the nutrient levels. As a rooted submerged macrophyte, H. verticillata can obtain nutrients from sediments via root uptake and from the water column via foliar uptake (Barko, 1982), thus acting as a major nutrient sink. Moreover, filter-feeding bivalves transfer nutrients (especially P) from the water column to the bottom, through excretion as well as biodeposition of faeces and pseudofaeces (Vaughn and Hoellein, 2018).
As expected, changes in nutrient concentrations and stoichiometry, which were influenced by filter-feeding bivalves and SMs, may have altered the phytoplankton assemblage composition during the mesocosm experiment. In agreement, a previous study observed suppressed Cyanobacterial taxa in lakes under P limitation (Havens et al., 2003). At the end of the experiment, the relatively high TN: TP ratios in the macrophyte (average of 37: 1) and the bivalve + macrophyte (average of 42: 1) treatments likely led to the competitive exclusion of cyanobacteria. These results concur with a previous study by Smith (1983) who noticed suppressed cyanobacterial blooms when the TN: TP ratio exceeded 29 to 1. Algae that are incapable of nitrogen fixation are reported to dominate under P-limited conditions (Schindler, 1977; Amano et al., 2010).
High aquatic N: P ratios in lakes are reported in agricultural regions (Arbuckle and Downing, 2001), suggesting that P is the principal production-limiting nutrient. In our study, relatively high TN: TP ratios (≈29) at the beginning indicated a phosphorus-limitation situation. During the loading experiments addition of N and P close to the Redfield ratio (N: P of 16: 1), relieves nutrient limitation, and promotes a much higher phytoplankton biomass development. Phytoplankton biomass showed a gradual increase under nutrient enrichment (the control treatment). Previous studies have reported a linear relationship between chlorophyll a concentration and TP for the lower nutrient ranges (TP < 5–100µgL-1) and asymptotic behavior at higher ranges (TP >100 µgL-1) (Canfield et al., 1984; Phillips et al., 2008; Borics et al., 2013). Noticeably, at the end of the experiment, TP concentration exceeded 100 µgL-1, we noticed an increase in phytoplankton biomass with nutrient enrichment, suggesting nutrients control phytoplankton biomass in nutrient‐rich waters (Richardson et al., 2019).
Even in a nutrient-enrichment scenario, as expected, responses to the addition of filter-feeding bivalves (Cristaria plicata) alone contributed to the decline in cyanobacterial and total phytoplankton biomass. The overall decline in cyanobacterial and phytoplankton biomass in these mesocosm can be explained by the direct grazing impacts of the filter-feeding bivalves (Gulati et al., 2008). The direct effects of grazing by C. plicata led to statistically significant decrease in filamentous or colonial taxa such as the genera Dolichospermum (formerly Anabaena), Microcystis, and Planktothrix (Bastviken et al., 1998; Dionisio Pires et al., 2005) which usually form harmful algal blooms. These results are in line with previous studies indicating that cyanobacteria are directly grazed by filter-feeding bivalves (Hwang et al., 2004; Gao et al., 2017), and support the possibility that addition of filter-feeding bivalves will alleviate algal blooms that are associated with eutrophication. Further, several studies have also shown that filter-feeding bivalves are selective feeders and the filtering rate can vary depending on food particle size and bivalve species. Some functional traits of cyanobacteria, including cell size, life form (e.g. single celled, colonial, or filamentous), nutritional deficiency, and toxin production, can prevent them from being grazed by mussels (White and Sarnelle, 2014; Boegehold et al., 2019). For example, Corbicula fluminea selectively filtered particles in the range of 0.2–2 µm (Rong et al., 2021), Dreissena Polymorpha preferred food particles from 5 to 40 μm (Sprung & Rose, 1988), and Venerupis corrugatus filtered out particles of 5 to 13 μm (Stenton-Dozey and Brown, 1992). Although we did not consider selective grazing effects of C. plicata on phytoplankton the composition of phytoplankton assemblages significantly differed between the control and the bivalve treatments at the end of the experiment. This result indicates that once nutrient limitation is alleviated, selective grazing would likely be the main factor affecting the structure of the phytoplankton assemblage. Additionally, the phytoplankton assemblages in the filter-feeding bivalve addition treatments appear to have adaptive responses to selective grazing pressure by C. plicata, as larger diatoms (e.g. Cymbella, Fragilaria, Thalassiosira) dominated the phytoplankton assemblages.
SMs can also suppress algal growth via allelopathic controls and nutrient competition (van Donk and van de Bund, 2002; Mohamed, 2017; Zhu et al., 2021). However, in our experiments, at the end of the experiment a slight, but not significant increase in overall phytoplankton assemblage biomass (chlorophyll a) was noticed in the Macrophyte treatment. The phytoplankton blooms occurred when SMs were absent over the course of the nutrient loading experiment (the control treatment). While phytoplankton biomass initially decreased from day 0 to day 4, it then began to slightly increase until day 32, suggesting that SMs did cause a reduction in phytoplankton biomass. The submerged macrophyte H. verticillate, has been reported to produce and release allelochemicals that has inhibitory effects on Chlorella cell membrane (Zhang et al., 2012) and cyanobacteria (Wang et al., 2006; Gao et al., 2011). Over 32 days, SMs suppressed overall cyanobacteria biomass (Table 2), concurring with findings from other experimental studies (Lürling et al., 2006; Barrow et al., 2019; Amorim and Moura, 2020). Further, factors such as light and nutrient competition likely interacted with allelopathic controls in the mesocosms and led to the competitive exclusion of cyanobacteria.
Although unexpected, we found that filter-feeding bivalves in combination with SMs reduced the biomass of cyanobacteria, and noticeably the effect size of this interaction was less than the sum of their individual effects (i.e., an antagonistic interaction). This result is in line with the widely observed antagonistic interactions in freshwater ecosystems (Jackson et al., 2016; Segurado et al., 2018; He et al., 2021), such as nutrient-pesticide effects on benthic invertebrate richness (Chará-Serna et al., 2019), and fish-shrimp effects on zooplankton biomass (He et al., 2021). The mechanism for the antagonistic interactions is largely unknown, however, a possible explanation could be the asymmetry of mean effect size. In our study, the larger magnitude of the submerged macrophyte restoration may have overridden the effect of stock filter-feeding bivalves (Table 2), thereby negating its contribution to their net impact on the overall biomass of cyanobacteria (Sala et al., 2000; Barrow et al., 2019). An antagonism between filter-feeding bivalves and SMs was only detected for total cyanobacteria. For filamentous or colonial taxa, there was no significant interactive effects; rather filamentous cyanobacteria were more sensitive to macrophytes and colonial cyanobacteria to bivalves. The differential sensitivity of cyanobacterial taxa to different biomanipulation approaches has been noticed previously (Gazulha et al., 2012; Amorim and Moura, 2020), the reason being that cyanobacteria are a diverse and morphologically complex group of prokaryotes with different key ecological traits thus eliciting disparate responses (Mantzouki et al., 2016; Rangel et al., 2020).
5. Conclusions
Our first hypothesis that manipulation via addition of filter-feeding bivalves and restoration of submerged macrophyte will likely affect phytoplankton assemblages was confirmed, as this manipulation efficiently decreased water nutrient concentrations and the overall phytoplankton biomass. Since, phytoplankton were dominated by symmetrical desmids (e.g., Cosmarium, Micrasterias) and the large diatoms (e.g., Cymbella, Fragilaria, Thalassiosira) in the restoration treatments, with the competitive exclusion of cyanobacteria, our results also supported that manipulation could control the growth of cyanobacteria. Contrary to the second hypothesis, an antagonism between filter-feeding bivalves and SMs was detected but only for total cyanobacteria, demonstrating that the larger magnitude of the submerged macrophyte restoration may override the effect of stock filter-feeding bivalves. However, we should also noticed that the addition of bivalves combined with SMs was more efficient at decreasing nutrient concentrations than the isolated addition of bivalves, and at controlling total algal biomass than the isolated restoration of SMs. Overall, our results suggest that manipulation, through introduction of the stock of bivalves as grazers, associated with the restoration of SMs, is an efficient approach for reducing cyanobacterial blooms and alleviating eutrophication.
Data availability statement
The original contributions presented in the study are included in the article/Supplementary Material. Further inquiries can be directed to the corresponding author.
Author contributions
XD: Conceptualization, Methodology, Investigation, Formal analysis, Writing – original draft, Writing – review & editing, Funding acquisition. DS: Conceptualization, Investigation, Formal analysis, Writing – original draft, Writing – review & editing. HW: Investigation. JY: Resources. HL: Writing – original draft, Investigation. TH: Funding acquisition. All authors contributed to the article and approved the submitted version.
Funding
This work was financially supported by the National Key Research and Development Program of China (2019YFD0900602 and 2019YFD0900605), National Natural Science Foundation of China (No. 31802298) and the Finance Special Fund of Ministry of Agriculture and Rural Affairs (Fisheries Resources and Environment Survey in the Key Water Areas of Northeast China).
Conflict of interest
The authors declare that the research was conducted in the absence of any commercial or financial relationships that could be construed as a potential conflict of interest.
Publisher’s note
All claims expressed in this article are solely those of the authors and do not necessarily represent those of their affiliated organizations, or those of the publisher, the editors and the reviewers. Any product that may be evaluated in this article, or claim that may be made by its manufacturer, is not guaranteed or endorsed by the publisher.
Supplementary material
The Supplementary Material for this article can be found online at: https://www.frontiersin.org/articles/10.3389/fpls.2023.1069593/full#supplementary-material
References
Amano, Y., Sakai, Y., Sekiya, T., Takeya, K., Taki, K., Machida, M. (2010). Effect of phosphorus fluctuation caused by river water dilution in eutrophic lake on competition between blue-green alga Microcystis aeruginosa and diatom Cyclotella sp. J. Environ. Sci. 22 (11), 1666–1673. doi: 10.1016/S1001-0742(09)60304-1
Amorim, C. A., Moura, A. N. (2020). Effects of the manipulation of submerged macrophytes, large zooplankton, and nutrients on a cyanobacterial bloom: A mesocosm study in a tropical shallow reservoir. Environ. pollut. 265, 114997. doi: 10.1016/j.envpol.2020.114997
Anderson, M. J. (2001). A new method for non-parametric multivariate analysis of variance. Austral Ecol. 26 (1), 32–46. doi: 10.1111/j.1442-9993.2001.01070.pp.x
American Public Health Association. (1992). Standard methods for the examination of water and wastewater. 18th ed (Washington, DC: American Public Health Association).
Arbuckle, K. E., Downing, J. A. (2001). The influence of watershed land use on lake n: P in a predominantly agricultural landscape. Limnol. Oceanogr. 46 (4), 970–975. doi: 10.4319/lo.2001.46.4.0970
Baker, S. M., Levinton, J. S., Kurdziel, J. P., Shumway, S. E. (1998). Selective feeding and biodeposition by zebra mussels and their relation to changes in phytoplankton composition and seston load. J. Shellfish Res. 17 (4), 1207–1214.
Bakker, E. S., Sarneel, J. M., Gulati, R. D., Liu, Z., van Donk, E. (2013). Restoring macrophyte diversity in shallow temperate lakes: biotic versus abiotic constraints. Hydrobiologia 710 (1), 23–37. doi: 10.1007/s10750-012-1142-9
Barko, J. W. (1982). Influence of potassium source (sediment vs. open water) and sediment composition on the growth and nutrition of a submersed freshwater macrophyte (Hydrilla verticillata) (L.f.) royle). Aquat. Bota. 12, 157–172. doi: 10.1016/0304-3770(82)90011-0
Barrow, J. L., Beisner, B. E., Giles, R., Giani, A., Domaizon, I., Gregory-Eaves, I. (2019). Macrophytes moderate the taxonomic and functional composition of phytoplankton assemblages during a nutrient loading experiment. Freshw. Biol. 64 (8), 1369–1381. doi: 10.1111/fwb.13311
Bartoń, K. (2022). MuMIn: Multi-model inference (R package version 1.46.0). Available at: https://CRAN.R-project.org/package=MuMIn.
Bastviken, D. T. E., Caraco, N. F., Cole, J. J. (1998). Experimental measurements of zebra mussel (Dreissena polymorpha) impacts on phytoplankton community composition. Freshw. Biol. 39 (2), 375–386. doi: 10.1046/j.1365-2427.1998.00283.x
Bergström, A.-K., Karlsson, J. (2019). Light and nutrient control phytoplankton biomass responses to global change in northern lakes. Global Change Biol. 25 (6), 2021–2029. doi: 10.1111/gcb.14623
Boegehold, A. G., Alame, K., Johnson, N. S., Kashian, D. R. (2019). Cyanobacteria reduce motility of quagga mussel (Dreissena rostriformis bugensis) sperm. Environ. Toxicol. Chem. 38 (2), 368–374. doi: 10.1002/etc.4305
Bolker, B. M., Brooks, M. E., Clark, C. J., Geange, S. W., Poulsen, J. R., Stevens, M. H. H., et al. (2009). Generalized linear mixed models: a practical guide for ecology and evolution. Trends Ecol. Evol. 24 (3), 127–135. doi: 10.1016/j.tree.2008.10.008
Borics, G., Nagy, L., Miron, S., Grigorszky, I., László-Nagy, Z., Lukács, B. A., et al. (2013). Which factors affect phytoplankton biomass in shallow eutrophic lakes? Hydrobiologia 714 (1), 93–104. doi: 10.1007/s10750-013-1525-6
Boucher-Carrier, O., Brisson, J., Abas, K., Duy, S. V., Sauvé, S., Kõiv-Vainik, M. (2022). Effects of macrophyte species and biochar on the performance of treatment wetlands for the removal of glyphosate from agricultural runoff. Sci. Total Environ. 838, 156061. doi: 10.1016/j.scitotenv.2022.156061
Briland, R. D., Stone, J. P., Manubolu, M., Lee, J., Ludsin, S. A. (2020). Cyanobacterial blooms modify food web structure and interactions in western lake Erie. Harmful Algae 92, 101586. doi: 10.1016/j.hal.2019.03.004
Brooks, M. E., Kristensen, K., van Benthem, K. J., Magnusson, A., Berg, C. W., Nielsen, A., et al (2017). Glmmtmb balances speed and flexibility among packages for zero-inflated generalized linear mixed modeling. R J 2 (2), 378–400. doi: 10.3929/ethz-b-000240890
Burks, R. L., Jeppesen, E., Lodge, D. M. (2001). Littoral zone structures as Daphnia refugia against fish predators. Limnol. Oceanogr. 46 (2), 230–237. doi: 10.4319/lo.2001.46.2.0230
Canfield, D., Shireman, J. V., Colle, D. E., Haller, W. T., Watkins, C. E., Maceina, M. J. (1984). Prediction of chlorophyll a concentrations in Florida lakes: Importance of aquatic macrophytes. Can. J. Fish. Aquat. Sci. 41 (3), 497–501. doi: 10.1139/f84-059
Carpenter, S. R., Caraco, N. F., Correll, D. L., Howarth, R. W., Sharpley, A. N., Smith, V. H. (1998). Nonpoint pollution of surface waters with phosphorus and nitrogen. Ecol. Appl. 8 (3), 559–568. doi: 10.1890/1051-0761(1998)008[0559:NPOSWW]2.0.CO;2
Chao, C., Lv, T., Wang, L., Li, Y., Han, C., Yu, W., et al. (2022). The spatiotemporal characteristics of water quality and phytoplankton community in a shallow eutrophic lake: Implications for submerged vegetation restoration. Sci. Total Environ. 821, 153460. doi: 10.1016/j.scitotenv.2022.153460
Chará-Serna, A. M., Epele, L. B., Morrissey, C. A., Richardson, J. S. (2019). Nutrients and sediment modify the impacts of a neonicotinoid insecticide on freshwater community structure and ecosystem functioning. Sci. Total Environ. 692, 1291–1303. doi: 10.1016/j.scitotenv.2019.06.301
Colvin, M. E., Pierce, C., Stewart, T. W. (2015). A food web modeling analysis of a Midwestern, USA eutrophic lake dominated by non-native common carp and zebra mussels. Ecol. Model. 312, 26–40. doi: 10.1016/j.ecolmodel.2015.05.016
Conley, D. J., Paerl, H. W., Howarth, R. W., Boesch, D. F., Seitzinger, S. P., Havens, K. E., et al. (2009). Controlling eutrophication: Nitrogen and phosphorus. Science 323(5917), 1014–1015. doi: 10.1126/science.1167755
Cook, S. C., Housley, L., Back, J. A., King, R. S. (2018). Freshwater eutrophication drives sharp reductions in temporal beta diversity. Ecology 99 (1), 47–56. doi: 10.1002/ecy.2069
Core Team., R. (2020). R: A language and environment for statistical computing (Vienna, Austria: R Foundation for Statistical Computing). Available at: https://www.R-project.org/.
Dionisio Pires, L. M., Bontes, B. M., Van Donk, E., Ibelings, B. W. (2005). Grazing on colonial and filamentous, toxic and non-toxic cyanobacteria by the zebra mussel Dreissena polymorpha. J. Plankton Res. 27 (4), 331–339. doi: 10.1093/plankt/fbi008
Du, X., Song, D., Ming, K., Yang, J., Jin, X., Wang, H., et al. (2022). Functional responses of phytoplankton assemblages to watershed land use and environmental gradients. Front. Ecol. E 9. doi: 10.3389/fevo.2021.819252
Fordham, D. A. (2015). Mesocosms reveal ecological surprises from climate change. PloS Biol. 13 (12), e1002323. doi: 10.1371/journal.pbio.1002323
Fujibayashi, M., Okano, K., Takada, Y., Mizutani, H., Uchida, N., Nishimura, O., et al. (2018). Transfer of cyanobacterial carbon to a higher trophic-level fish community in a eutrophic lake food web: fatty acid and stable isotope analyses. Oecologia 188 (3), 901–912. doi: 10.1007/s00442-018-4257-5
Gao, Y.-N., Liu, B.-Y., Xu, D., Zhou, Q.-H., Hu, C.-Y., Ge, F.-J., et al. (2011). Phenolic compounds exuded from two submerged freshwater macrophytes and their allelopathic effects on Microcystis aeruginosa. Pol. J. Environ. Stud. 20 (5), 1153–1159.
Gao, H., Qian, X., Wu, H., Li, H., Pan, H., Han, C. (2017). Combined effects of submerged macrophytes and aquatic animals on the restoration of a eutrophic water body–a case study of gonghu bay, lake taihu. Ecol. Eng. 102, 15–23. doi: 10.1016/j.ecoleng.2017.01.013
Gao, H., Song, Y., Lv, C., Chen, X., Yu, H., Peng, J., et al. (2015). The possible allelopathic effect of Hydrilla verticillata on phytoplankton in nutrient-rich water. Environ. Earth Sci. 73 (9), 5141–5151. doi: 10.1007/s12665-015-4316-8
Gazulha, V., Mansur, M. C. D., Cybis, L. F., Azevedo, S. M. F. O. (2012). Grazing impacts of the invasive bivalve Limnoperna fortunei (Dunker 1857) on single-celled, colonial and filamentous cyanobacteria. Braz. J. Biol. 72, 33–39. doi: 10.1590/S1519-69842012000100004
Gulati, R. D., Dionisio Pires, L. M., Van Donk, E. (2008). Lake restoration studies: Failures, bottlenecks and prospects of new ecotechnological measures. Limnologica 38 (3), 233–247. doi: 10.1016/j.limno.2008.05.008
Harrison, X. A., Donaldson, L., Correa-Cano, M. E., Evans, J., Fisher, D. N., Goodwin, C. E., et al. (2018). A brief introduction to mixed effects modelling and multi-model inference in ecology. PeerJ 6, e4794. doi: 10.7717/peerj.4794
Havens, K. E., James, R. T., East, T. L., Smith, V. H. (2003). N:P ratios, light limitation, and cyanobacterial dominance in a subtropical lake impacted by non-point source nutrient pollution. Environ. pollut. 122 (3), 379–390. doi: 10.1016/S0269-7491(02)00304-4
Heisler, J., Glibert, P. M., Burkholder, J. M., Anderson, D. M., Cochlan, W., Dennison, W. C., et al. (2008). Eutrophication and harmful algal blooms: A scientific consensus. Harmful Algae 8 (1), 3–13. doi: 10.1016/j.hal.2008.08.006
He, H., Ning, X., Chen, K., Li, Q., Li, K., Liu, Z., et al. (2021). Intraguild predation dampens trophic cascades in shallow aquatic mesocosms in the subtropics: Implications for lake restoration by biomanipulation. Freshw. Biol. 66 (8), 1571–1580. doi: 10.1111/fwb.13739
He, Y., Zhou, Y., Zhou, Z., Liu, Y., Xiao, Y., Long, L., et al. (2023). Allelopathic effect of pyrogallic acid on cyanobacterium Microcystis aeruginosa: The regulatory role of nitric oxide and its significance for controlling harmful algal blooms (HABs). Sci. Total Environ. 858, 159785. doi: 10.1016/j.scitotenv.2022.159785
Hillebrand, H., Dürselen, C. D., Kirschtel, D., Pollingher, U., Zohary, T. (1999). Biovolume calculation for pelagic and benthic microalgae. J. Phycol. 35 (2), 403–424. doi: 10.1046/j.1529-8817.1999.3520403.x
Horppila, J., Nurminen, L. (2003). Effects of submerged macrophytes on sediment resuspension and internal phosphorus loading in lake hiidenvesi (southern Finland). Water Res. 37 (18), 4468–4474. doi: 10.1016/S0043-1354(03)00405-6
Hu, H., Wei, Y. (2006). The freshwater algae of China-systematics, taxonomy and ecology. (Beijing: Science Press).
Hwang, S.-J., Kim, H.-S., Shin, J.-K., Oh, J.-M., Kong, D.-S. (2004). Grazing effects of a freshwater bivalve (Corbicula leana prime) and large zooplankton on phytoplankton communities in two Korean lakes. Hydrobiologia 515 (1), 161–179. doi: 10.1023/B:HYDR.0000027327.06471.1e
Jackson, M. C., Loewen, C. J. G., Vinebrooke, R. D., Chimimba, C. T. (2016). Net effects of multiple stressors in freshwater ecosystems: a meta-analysis. Global Change Biol. 22 (1), 180–189. doi: 10.1111/gcb.13028
Jeppesen, E., Meerhoff, M., Jacobsen, B. A., Hansen, R. S., Søndergaard, M., Jensen, J. P., et al. (2007b). Restoration of shallow lakes by nutrient control and biomanipulation–the successful strategy varies with lake size and climate. Hydrobiologia 581 (1), 269–285. doi: 10.1007/s10750-006-0507-3
Jeppesen, E., Moss, B., Bennion, H., Carvalho, L., DeMeester, L., Feuchtmayr, H., et al. (2010). “Interaction of climate change and eutrophication,” in Climate change impacts on freshwater ecosystems. Eds. Kernan, M., Battarbee, R. W., Moss, B. (Oxford: Wiley-Blackwell), 119–151. doi: 10.1002/9781444327397.ch6
Jeppesen, E., Søndergaard, M., Meerhoff, M., Lauridsen, T. L., Jensen, J. P. (2007a). Shallow lake restoration by nutrient loading reduction–some recent findings and challenges ahead. Hydrobiologia 584, 239–252. doi: 10.1007/s10750-007-0596-7
Jespersen, A. M., Christoffersen, K. (1987). Measurements of chlorophyll-a from phytoplankton using ethanol as extraction solvent. Arch. Hydrobiologie 109 (3), 445–454. doi: 10.1127/archiv-hydrobiol/109/1987/445
Knopik, J. M., Newman, R. M. (2018). Transplanting aquatic macrophytes to restore the littoral community of a eutrophic lake after the removal of common carp. Lake Reservoir Manage. 34 (4), 365–375. doi: 10.1080/10402381.2018.1477885
Lawrence, M. (2016). Ez: Easy analysis and visualization of factorial experiments (R Package Version 4.4-0). Available at: https://CRAN.R-project.org/package=ez.
Legendre, P., Gallagher, E. D. (2001). Ecologically meaningful transformations for ordination of species data. Oecologia 129 (2), 271–280. doi: 10.1007/s004420100716
Le Moal, M., Gascuel-Odoux, C., Ménesguen, A., Souchon, Y., Étrillard, C., Levain, A., et al. (2019). Eutrophication: A new wine in an old bottle? Sci. Total Environ. 651, 1–11. doi: 10.1016/j.scitotenv.2018.09.139
Li, H., Li, Y., Huang, D., Zhang, L., Lu, J., Zhang, J. (2021a). The response mechanism of Hydrilla verticillata and leaf epiphytic biofilms to depth and nutrient removal. Environ. Sci. pollut. R. 28 (35), 49032–49041. doi: 10.1007/s11356-021-14131-x
Liu, H., Liu, G., Xing, W. (2021). Functional traits of submerged macrophytes in eutrophic shallow lakes affect their ecological functions. Sci. Total Environ. 760, 143332. doi: 10.1016/j.scitotenv.2020.143332
Liu, X., Zhang, G., Sun, G., Wu, Y., Chen, Y. (2019). Assessment of lake water quality and eutrophication risk in an agricultural irrigation area: A case study of the chagan lake in northeast China. Water 11 (11), 2380. doi: 10.3390/w11112380
Liu, H., Zhou, W., Li, X., Chu, Q., Tang, N., Shu, B., et al. (2020). How many submerged macrophyte species are needed to improve water clarity and quality in Yangtze floodplain lakes? Sci. Total Environ. 724, 138267. doi: 10.1016/j.scitotenv.2020.138267
Li, Y., Wang, L., Chao, C., Yu, H., Yu, D., Liu, C. (2021b). Submerged macrophytes successfully restored a subtropical aquacultural lake by controlling its internal phosphorus loading. Environ. pollut. 268, 115949. doi: 10.1016/j.envpol.2020.115949
Lürling, M., van Geest, G., Scheffer, M. (2006). Importance of nutrient competition and allelopathic effects in suppression of the green alga Scenedesmus obliquus by the macrophytes Chara, Elodea and Myriophyllum. Hydrobiologia 556 (1), 209–220. doi: 10.1007/s10750-005-1168-3
MacIsaac, H. J., Sprules, G., Johannson, O. E., Leach, J. H. (1992). Filtering impacts of larval and sessile zebra mussels (Dreissena polymorpha) in western lake Erie. Oecologia 92 (1), 30–39. doi: 10.1007/bf00317259
Mantzouki, E., Visser, P. M., Bormans, M., Ibelings, B. W. (2016). Understanding the key ecological traits of cyanobacteria as a basis for their management and control in changing lakes. Aquat. Ecol. 50 (3), 333–350. doi: 10.1007/s10452-015-9526-3
Mohamed, Z. A. (2017). Macrophytes-cyanobacteria allelopathic interactions and their implications for water resources management–a review. Limnologica 63, 122–132. doi: 10.1016/j.limno.2017.02.006
Nakagawa, S., Schielzeth, H. (2013). A general and simple method for obtaining R2 from generalized linear mixed-effects models. Methods Ecol. Evol. 4 (2), 133–142. doi: 10.1111/j.2041-210x.2012.00261.x
Oksanen, J., Blanchet, F. G., Friendly, M., Kindt, R., Legendre, P., McGlinn, D., et al. (2020). Vegan: Community ecology package (R package version 2.5-7). Available at: https://CRAN.R-project.org/package=vegan.
Peng, X., Lin, Q., Liu, B., Huang, S., Yan, W., Zhang, L., et al. (2022). Effect of submerged plant coverage on phytoplankton community dynamics and photosynthetic activity in situ. J. Environ. Manage. 301, 113822. doi: 10.1016/j.jenvman.2021.113822
Phillips, G., Pietiläinen, O. P., Carvalho, L., Solimini, A., Lyche Solheim, A., Cardoso, A. C. (2008). Chlorophyll–nutrient relationships of different lake types using a large European dataset. Aquat. Ecol. 42 (2), 213–226. doi: 10.1007/s10452-008-9180-0
Puijalon, S., Bouma, T. J., Douady, C. J., van Groenendael, J., Anten, N. P. R., Martel, E., et al. (2011). Plant resistance to mechanical stress: evidence of an avoidance–tolerance trade-off. New Phytol. 191 (4), 1141–1149. doi: 10.1111/j.1469-8137.2011.03763.x
Rangel, L. M., Silva, L. H. S., Faassen, E. J., Lürling, M., Ger, K. A. (2020). Copepod prey selection and grazing efficiency mediated by chemical and morphological defensive traits of cyanobacteria. Toxins 12 (7), 465. doi: 10.3390/toxins12070465
Rao, Q., Su, H., Deng, X., Xia, W., Wang, L., Cui, W., et al. (2020). Carbon, nitrogen, and phosphorus allocation strategy among organs in submerged macrophytes is altered by eutrophication. Front. Plant Sci. 11. doi: 10.3389/fpls.2020.524450
Redfield, A. C. (1958). The biological control of chemical factors in the environment. Am. Sci. 46 (3), 205–221.
Richardson, J., Feuchtmayr, H., Miller, C., Hunter, P. D., Maberly, S. C., Carvalho, L. (2019). Response of cyanobacteria and phytoplankton abundance to warming, extreme rainfall events and nutrient enrichment. Global Change Biol. 25 (10), 3365–3380. doi: 10.1111/gcb.14701
Rong, Y., Tang, Y., Ren, L., Taylor, W. D., Razlutskij, V., Naselli-Flores, L., et al. (2021). Effects of the filter-feeding benthic bivalve Corbicula fluminea on plankton community and water quality in aquatic ecosystems: A mesocosm study. Water 13 (13), 1827. doi: 10.3390/w13131827
Søndergaard, M., Jeppesen, E., Lauridsen, T. L., Skov, C., Van Nes, E. H., Roijackers, R., et al. (2007). Lake restoration: successes, failures and long-term effects. J. Appl. Ecol. 44 (6), 1095–1105. doi: 10.1111/j.1365-2664.2007.01363.x
Søndergaard, M., Johansson, L. S., Lauridsen, T. L., Jørgensen, T. B., Liboriussen, L., Jeppesen, E. (2010). Submerged macrophytes as indicators of the ecological quality of lakes. Freshw. Biol. 55 (4), 893–908. doi: 10.1111/j.1365-2427.2009.02331.x
Sala, O. E., Chapin, F. S., III, Armesto, J. J., Berlow, E., Bloomfield, J., Dirzo, R., et al. (2000). Global biodiversity scenarios for the year 2100. Science 287 (5459), 1770–1774. doi: 10.1126/science.287.5459.1770
Sand-Jensen, K., Borum, J. (1991). Interactions among phytoplankton, periphyton, and macrophytes in temperate freshwaters and estuaries. Aquat. Bota. 41 (1), 137–175. doi: 10.1016/0304-3770(91)90042-4
Schindler, D. W. (1977). Evolution of phosphorus limitation in lakes. Science 195 (4275), 260–262. doi: 10.1126/science.195.4275.260
Segurado, P., Almeida, C., Neves, R., Ferreira, M. T., Branco, P. (2018). Understanding multiple stressors in a Mediterranean basin: Combined effects of land use, water scarcity and nutrient enrichment. Sci. Total Environ. 624, 1221–1233. doi: 10.1016/j.scitotenv.2017.12.201
Smith, V. H. (1983). Low nitrogen to phosphorus ratios favor dominance by blue-green algae in lake phytoplankton. Science 221 (4611), 669–671. doi: 10.1126/science.221.4611.669
Smith, V. H., Joye, S. B., Howarth, R. W. (2006). Eutrophication of freshwater and marine ecosystems. Limnol. Oceanogr 51 (1part2), 351–355. doi: 10.4319/lo.2006.51.1_part_2.0351
Sprung, M., Rose, U. (1988). Influence of food size and food quantity on the feeding of the mussel Dreissena polymorpha. Oecologia 77 (4), 526–532. doi: 10.1007/BF00377269
Stenton-Dozey, J., Brown, A. C. (1992). Clearance and retention efficiency of natural suspended particles by the rock-pool bivalve Venerupis corrugatus in relation to tidal availability. Mar. Ecol. Prog. Ser. 82, 175–186. doi: 10.3354/meps082175
Stewart, R. I. A., Dossena, M., Bohan, D. A., Jeppesen, E., Kordas, R. L., Ledger, M. E., et al. (2013). Mesocosm experiments as a tool for ecological climate-change research. Adv. Ecol. Res. 48, 1–181. doi: 10.1016/B978-0-12-417199-2.00002-1
Su, H., Chen, J., Wu, Y., Chen, J., Guo, X., Yan, Z., et al. (2019). Morphological traits of submerged macrophytes reveal specific positive feedbacks to water clarity in freshwater ecosystems. Sci. Total Environ. 684, 578–586. doi: 10.1016/j.scitotenv.2019.05.267
Van den Brink, P. J., Braak, C. J. F. T. (1999). Principal response curves: Analysis of time-dependent multivariate responses of biological community to stress. Environ. Toxicol. Chem. 18 (2), 138–148. doi: 10.1002/etc.5620180207
van Donk, E., van de Bund, W. J. (2002). Impact of submerged macrophytes including charophytes on phyto- and zooplankton communities: allelopathy versus other mechanisms. Aquat. Bota. 72 (3), 261–274. doi: 10.1016/S0304-3770(01)00205-4
Vaughn, C. A., Hoellein, T. J. (2018). Bivalve impacts in freshwater and marine ecosystems. Annu. Rev. Ecol. Evol. Syst. 49 (1), 183–208. doi: 10.1146/annurev-ecolsys-110617-062703
Wang, L.-X., Zhang, L., Zhang, Y.-X., Jin, C.-Y., Lu, C.-M., Wu, G.-R. (2006). The inhibitory effect of Hydrilla verticillata culture water on Microcystic aeruginosa and its mechanism. J. Plant Physiol. Mol. Biol. 32 (6), 672–678.
Wetzel, R. G., Likens, G. E. (2000). Limnological analyses. 3rd edition. (New York: Springer-Verlag).
White, J. D., Sarnelle, O. (2014). Size-structured vulnerability of the colonial cyanobacterium, Microcystis aeruginosa, to grazing by zebra mussels (Dreissena polymorpha). Freshw. Biol. 59 (3), 514–525. doi: 10.1111/fwb.12282
Wu, L., Culver, D. A. (1991). Zooplankton grazing and phytoplankton abundance: an assessment before and after invasion of Dreissena polymorpha. J. Great Lakes Res. 17 (4), 425–436. doi: 10.1016/S0380-1330(91)71378-6
Yu, J., Xia, M., He, H., Jeppesen, E., Guan, B., Ren, Z., et al. (2020). The host mussel Sinanodonta woodiana alleviates negative effects of a small omnivorous fish (Acheilognathus macropterus) on water quality: A mesocosm experiment. Freshw. Sci. 39 (4), 752–761. doi: 10.1086/711295
Zhang, T. T., He, M., Wu, A. P., Nie, L. W. (2012). Inhibitory effects and mechanisms of Hydrilla verticillata (Linn.f.) royle extracts on freshwater algae. B. Environ. Contam. Tox. 88 (3), 477–481. doi: 10.1007/s00128-011-0500-z
Zhang, Y., Jeppesen, E., Liu, X., Qin, B., Shi, K., Zhou, Y., et al. (2017). Global loss of aquatic vegetation in lakes. Earth-Sci. Rev. 173, 259–265. doi: 10.1016/j.earscirev.2017.08.013
Zhang, X., Liu, Z., Jeppesen, E., Taylor, W. D. (2014). Effects of deposit-feeding tubificid worms and filter-feeding bivalves on benthic-pelagic coupling: Implications for the restoration of eutrophic shallow lakes. Water Res. 50, 135–146. doi: 10.1016/j.watres.2013.12.003
Zhang, X., Zhen, W., Jensen, H. S., Reitzel, K., Jeppesen, E., Liu, Z. (2021). The combined effects of macrophytes (Vallisneria denseserrulata) and a lanthanum-modified bentonite on water quality of shallow eutrophic lakes: A mesocosm study. Environ. pollut. 277, 116720. doi: 10.1016/j.envpol.2021.116720
Zhu, X., Dao, G., Tao, Y., Zhan, X., Hu, H. (2021). A review on control of harmful algal blooms by plant-derived allelochemicals. J. Hazard. Mater. 401, 123403. doi: 10.1016/j.jhazmat.2020.123403
Keywords: biomanipulation, control of cyanobacteria, eutrophication, filer-feeding bivalves, submerged macrophytes
Citation: Du X, Song D, Wang H, Yang J, Liu H and Huo T (2023) The combined effects of filter-feeding bivalves (Cristaria plicata) and submerged macrophytes (Hydrilla verticillate) on phytoplankton assemblages in nutrient-enriched freshwater mesocosms. Front. Plant Sci. 14:1069593. doi: 10.3389/fpls.2023.1069593
Received: 14 October 2022; Accepted: 02 January 2023;
Published: 23 January 2023.
Edited by:
Eric Marechal, UMR5168 Laboratoire de Physiologie Cellulaire Vegetale (LPCV), FranceCopyright © 2023 Du, Song, Wang, Yang, Liu and Huo. This is an open-access article distributed under the terms of the Creative Commons Attribution License (CC BY). The use, distribution or reproduction in other forums is permitted, provided the original author(s) and the copyright owner(s) are credited and that the original publication in this journal is cited, in accordance with accepted academic practice. No use, distribution or reproduction is permitted which does not comply with these terms.
*Correspondence: Tangbin Huo, tbhuo@163.com