- 1Institute of Farmland Irrigation, Chinese Academy of Agriculture Sciences, Key Laboratory of Crop Water Use and Regulation, Ministry of Agriculture and Rural Affairs, Xinxiang, China
- 2College of Tobacco Science, Henan Agricultural University, Zhengzhou, China
- 3Guangli Irrigation Authority, Jiaozuo, China
Introduction: Extreme weather has occurred more frequently in recent decades, which results in more frequent drought disasters in the maize growing season. Severe drought often decreases remarkably plant growth and yield of maize, and even reduces significantly the quality of maize production, especially for waxy maize.
Results: To study the changes in plant growth, fresh ear yield, and fresh grain quality of waxy maize under water deficits occurring at different growth stages, and further strengthen the field water management of waxy maize, water deficit experiments were carried out under a rain shelter in 2019 and 2020. Water deficit treatments were imposed respectively at the V6–VT (DV6–VT), VT–R2 (DVT–R2), and R2–R3 (DR2–R3) stages of waxy maize, and treatment with non-water deficit in the whole growing season was taken as the control (CK). The lower limit of soil water content was 50% of field capacity for a water deficit period and 65% of field capacity for a non-water deficit period.
Results: In this study, water deficits imposed at V6–VT and VT–R2 stages decreased plant growth rate and leaf gas exchange parameters, accelerated leaf senescence, and limited ear growth of waxy maize, which resulted in 11.6% and 23.1% decreases in grains per ear, 19.4% and 7.3% declines in 100-grain weight, 20.3% and 14.2% losses in fresh ear yield in 2019 and 2020 growing seasons, respectively, while water deficit at R2–R3 stage had no significant effect on ear traits and fresh ear yield, but the fresh ear yield with husk of DR2–R3 decreased by 9.1% (P<0.05). The obvious water deficit imposed at the V6–VT and VT–R2 stages also lowered grain quality. Water deficits at the V6–VT and VT–R2 stages led to accelerated maturity, resulting in increased total protein, starch, and lysine content in grains at the R3 stage and decreased soluble sugar content. Principal component analysis revealed that when water deficits occurred in the waxy maize growing season, they firstly altered maize physiological processes, then affected ear characteristics and yield, and finally resulted in significant grain quality changes. In conclusion, a water deficit during V6–VT and VT–R2 not only reduced fresh ear yield but also adversely affected grain quality. However, water deficit during R2–R3 had little effect on total protein, starch, and soluble sugar content,but increased obviously lysine content.
Discussion: The above results suggested that avoiding serious water deficits at the V6–VT and VT–R2 stages of waxy maize while imposing a slight water deficit at the R2–R3 stage has not only little effects on fresh ear yield but also a remarkable improvement in grain quality.
Introduction
Drought is one of the main disasters affecting agricultural production around the world. Climate change has led to the aggravation of drought in many regions and significantly increased the frequency of extreme drought (IPCC, 2014). Since 1950, the land area affected by drought in China’s agricultural production has shown a gradual upward trend, and the loss of food due to drought is about 25–30 × 106 t, accounting for 60% of the total loss from natural disasters (Hao and Singh, 2015; Song et al., 2018).
Water deficit can lead to a large number of physiological stress reactions in plants, thus changing the physiological characteristics of plants, thereby affecting the growth of plants, and the yield and quality of final products (Wang and Frei, 2011). Under conditions of water deficit, plant cells will produce reactive oxygen species (ROS) due to oxidative damage and synthesize a large amount of malondialdehyde (MDA). Meanwhile, the enhanced activities of catalase (CAT), superoxide dismutase (SOD), and peroxidase (POD) prevent severe damage (Ye et al., 2020a). At the same time, soluble sugar, soluble protein, and proline content in plant cells will gradually increase to maintain normal cell osmotic pressure (Liu et al., 2015). Drought stress also significantly reduced the photosynthetic rate of maize leaves. On the one hand, stomatal opening would decrease under drought stress, leading to a decrease in CO2 supply and a decrease in the photosynthetic rate of maize leaves (Yao et al., 2012). On the other hand, peroxidation can reduce the activity of leaf photosynthetic enzymes (ribulose-1, 5-diphosphate carboxylase, and phosphoenolpyruvate carboxylase), resulting in a lower photosynthetic rate and final yield reduction of maize (Markelz et al., 2011; Ye et al., 2020a).
Water deficits can also affect the quality of crop products. Studies have shown that drought can reduce grain starch content and increase protein content in many crops (Wang and Frei, 2011; Thitisaksakul et al., 2012). Drought was shown to lower starch concentration in cassava tubers (Santisopasri et al., 2001), and water deficit during the flowering stage caused the process of starch accumulation in advance, and reduced the total starch accumulation (Yi et al., 2014). Some studies also showed that water deficit during the whole growth stage increased starch accumulation, starch accumulation rate, and the activities of key enzymes for starch synthesis (AGPase (glucose-1-ATP transferase), SS (starch synthase), and SBE (1,4-glucan branching enzyme)) at early filling stage in wheat, but decreased starch accumulation and amylose content at late filling stage (Dai et al., 2008; Singh et al., 2008). Studies also showed that drought stress increased total protein concentrations but decreased the contents of alcohol-soluble protein, glutenin, and oat protein contents in wheat grains (Begcy and Walia, 2015; Flagella et al., 2010; Ozturk and Aydin, 2004).
It was also reported that a water deficit reduced lysine content and increased protein content in maize grains and changed maize grain quality by increasing nitrogen, magnesium, zinc, and alcohol-soluble protein concentrations and reducing potassium and glutenin concentrations (Erbs et al., 2015). However, some studies suggested that drought at different growing stages had different effects on the grain quality of maize. Compared with normal irrigation, a drought at the whole growth stage was shown to decrease starch content by 3% and increase protein content in normal maize (Liu et al., 2013a). Sandhya et al. (2010) reported that drought stress at the seedling stage reduced the content of protein and starch in grains, while Ma et al. (2006) found that the content of protein and lysine in grains would be increased under moderate drought but decreased under excessive drought at the maize seedling stage. Drought imposed at the silking stage decreased starch content and increased protein content in maize grains (Wang and Frei, 2011; Thitisaksakul et al., 2012; Beckles and Thitisaksakul, 2014; Wang et al., 2021a). Drought stress at the flowering and post-anthesis stages both decreased grain protein content and fresh ear yield (decreased by 16.2%), but increased grain starch content in waxy maize (Zhao, 2017; Shi et al., 2018; Wang et al., 2021a). However, drought stress at the filling stage had no significant effects on the starch content but increased the protein content in the grains of fresh waxy maize. (Lu et al., 2015). In the process of grain formation, drought stress reduced the final starch content but increased the protein content (Wang et al., 2021b).
As a fresh-eating food, waxy maize places a high value on grain quality. Higher quality can bring better edible value and economic value (Wang et al., 2020). With the rapid improvement of citizen living standards, the planting area of fresh waxy maize increased significantly in the last decade in China. It can be expected that waxy maize will have a better market prospect and that the plantation area will continue to develop in the future. Although about 70% of the average annual rainfall of 582 mm occurred in the period of June to October in the Huang-Huai-Hai Plain (Si et al., 2020), most of the rainfall was given in several heavy rainstorms (Ma et al., 2016), which usually results in long periods without any effective rainfall and severe droughts during the maize growing season. The use of appropriate measurements and techniques is vital for high-yield, good-quality, and sustainable waxy maize production in the region. For the effects of water deficits on grain quality of waxy maize, most of the previous studies-imposed water deficits after flowering. They mainly explored the changes in grain quality of waxy maize at complete maturity under different water-deficit treatments. However, few studies have focused on the effects of water deficits occurring in the vegetative growth stage, especially on grain quality in the fresh stage. Therefore, the main objectives of this experiment were focused on: (1) clarifying the changes in plant growth, physiological characteristics, fresh ear yield, and fresh grain quality of waxy maize under water deficit at the jointing stage, flowering stage, and filling stage; and (2) revealing in detail the tolerance of waxy maize to water deficit at different growing stages for determining suitable water management during the waxy maize growing period. This research contributes to the rapid development of waxy maize production in the Huang-Huai-Hai Plain.
Materials and methods
Site description
The experiment was carried out in lysimeters under a large-scale rain shelter at the Xinxiang Comprehensive Experimental Station of the Chinese Academy of Agricultural Sciences located in Qiliying Town, Xinxiang, Henan, China (35°18′N, 113°54′E, 75 m a.s.l.) with temperate monsoon weather in the 2019 and 2020 maize growing seasons. All lysimeters are non-weighing with well-equipped irrigation and drainage systems. The dimensions of each lysimeter were 2.0 m wide × 3.33 m length × 2.0 m in depth. The top side of the steel outer frame of the lysimeter is 10 cm higher than the soil surface in the lysimeter to prevent runoff during rain or irrigation events. A total of 24 lysimeters were arranged in two rows under a rain shelter. There was a 2 m space between the rows and 20 cm between lysimeters in the same row. The physical and chemical properties of the top 40 cm of the soil layer are shown in Table 1. A mobile rain shelter was installed above the two rows of lysimeters and closed before a rainfall and opened after the rainfall. This was done to avoid the severe effects of natural rainfall on the experiment of signed water deficit at different stages in maize growing seasons. An automatic weather station (YM-HJ03, Handan Chuangmeng Electronic Technology Co., Ltd., Hebei, China) was set on the edge of the lysimeter area. The average daily temperature and accumulated precipitation during the whole growing season of waxy maize in the two experiment seasons are shown in Figure 1.
Experimental design
The experimental waxy maize variety was “Shenkenuo 1,” bred by the Shanghai Academy of Agricultural Sciences. The variety is a multi-resistant waxy maize variety with high taste quality and great planting promotion value (Wang et al., 2014). A total of 40 plants of waxy maize were maintained in each lysimeter, with row spacing of 60 cm and plant spacing of 30 cm. The experiment arranged only one factor (water deficit stage) with four treatment levels, i.e., water deficit occurred at V6–VT, VT–R2, and R2–R3 stages, and no water deficit in the whole waxy maize growing season (as CK), respectively. Following the soil water content arrangement shown in Xiao et al. (2011), no irrigation was carried out when the soil water contents were higher than 50% of field capacity during the process of water deficit treatment at the V6–VT, VT–R2, and R2–R3 stages of waxy maize, and irrigation was performed when the soil water content was reduced to or less than 50% of field capacity, or at the end of a growing stage with water deficit treatment. During the periods without arranging water deficit treatment, the soil water contents were maintained at more than 65% of field capacity, so the soil water contents in CK treatment were maintained at higher than 65% of field capacity in whole growing seasons. All four treatments were replicated three times. The planned soil water lower limits for all four treatments are shown in Table 2. The beginning date of each growth stage is shown in Table 3.
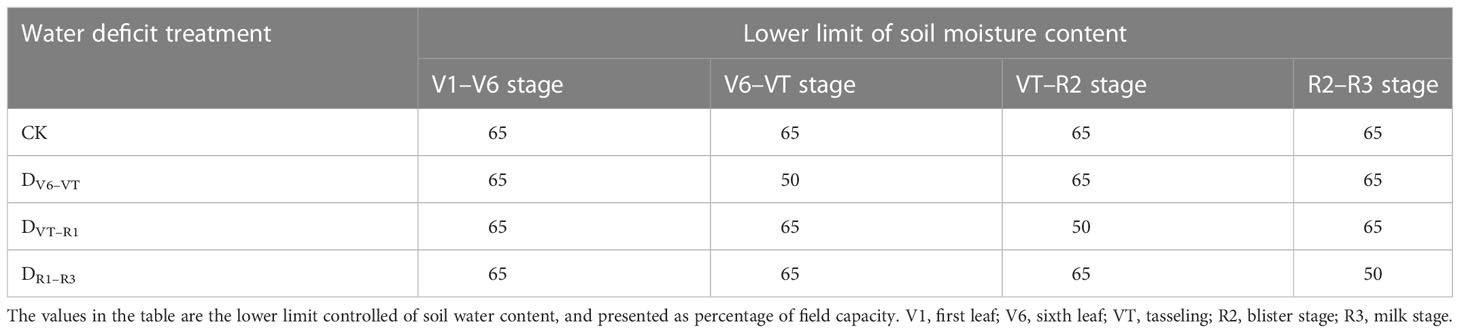
Table 2 Designed low limit of soil water content for different treatments at different waxy maize growing stages.
Measurement set-up
Measurements of soil water content
Soil water content (SWS, cm−3 cm−3) in the 0–100 cm soil layer was measured in real time with Insentek sensors (Oriental Zhigan Technology Ltd., Zhejiang, China) with a 10 cm increment. The sensor parameters were shown in Qin et al. (2019).
Measurements of plant height and leaf area index
At the six-leaf stage of waxy maize, three representative waxy maize plants with similar growth status were selected and marked in each lysimeter. The plant height and the leaf length and largest leaf width of all leaves on the three marked plants were measured at the end of each water deficit period of V6–VT, VT–R2, and R2–R3 in 2019 and 2020, and the leaf area index of each lysimeter was calculated by using Eq. (1) (Huang et al., 2022).
where LAI is the leaf area index (dimensionless), Lij is the leaf length (cm) of the jth leaf on ith plant, Wij is the largest width (cm) of the jth leaf on ith plant, m is the measured number of plants, n is the number of leaves per plant, N is the plant number on a lysimeter, and S is the soil surface area of a lysimeter (cm2).
Measurements of gas exchange
A Li-6400 portable photosynthesis analyzer (LI-COR, USA) was used to measure the gas exchange parameters (including net photosynthetic rate (Pn), stomatal conductance (Gs), transpiration rate (Tr), and intercellular CO2 concentration (Ci)) of the ear leaves on the marked plants at the end of each water deficit period of V6–VT, VT–R2, and R2–R3 in 2019 and 2020. Measurements were carried out between 9:00 and 11:00 a.m. on a sunny day. A SPAD-502 portable chlorophyll meter (Konica Minolta Holdings, Inc., Japan) was used to measure the SPAD value of the ear leaves on the marked plants at the end of the three water deficit periods (Huang et al., 2022). The leaf water use efficiency (LWUE) was calculated with Eq. (2) (Huang et al., 2022).
Determination of enzyme activities and osmotic adjustment substances in waxy maize leaves
Five ear leaves of waxy maize in each lysimeter were sampled at the R3 stage in 2019 for determining the soluble protein content (determined with the BCA protein method), soluble sugar content (with the anthrone colorimetry method), proline content (with the ninhydrin method), and malondialdehyde (MDA, determined with the thiobarbituric acid method) contents of ear leaves of waxy maize. The methods of determining the activities of antioxidant enzymes such as superoxide dismutase (SOD, NBT method), peroxidase (POD, guaiacol method), and catalase (CAT, ammonium molybdate method) also were consistent with those described by Huang et al. (2022).
Fresh ear grain yield and ear characters
Fresh ears with husks were taken on all lysimeters (the harvest date is shown in Table 2) at the end of the R3 stage in the 2019 and 2020 seasons. A total of 20 representative ears were sampled on each lysimeter to measure the yield of fresh ears with husks and the yield of fresh ears without husks. Ear characters such as ear length, ear diameter, bald tip length, grain rows per ear, grains per row, and grains per ear were also determined simultaneously by averaging the relevant values of the 20 sample ears. After threshing, three groups of 100 grains were randomly sampled to determine the 100-grain weight for each experimental lysimeter.
Grain quality
The waxy maize grains were collected at late R3 stages for determining fresh grain quality using the method described by Huang et al. (2022) in the 2019 and 2020 seasons. The soluble sugar content (determined with the anthrone colorimetric method), starch content (with the anthrone-sulfuric acid method), total protein content (with the total nitrogen content method, total protein content = total nitrogen content × 6.25), and lysine content (with the ninhydrin chromogenic method) of waxy maize grains were measured for each sample. The contents of amylopectin, amylose, gliadin, gluten, albumin, and globulin were measured by the Sanshu Bio-Tech company in China. The amylose content of starch was determined using a colorimetric amylose content assay (Knutson and Grove, 1994). The amylose content was analyzed using the GPC-RI-MALLs system developed by Park et al. (2007). Glutenins and gliadins were extracted and quantitated subsequently from two biological replicates by reverse-phase ultra-performance liquid chromatography (RP-UPLC) according to a method described by Han et al. (2015), and the sample size was modified in minor ways according to the protein concentration of waxy maize grain. The albumin and globulin content was analyzed using an automatic microplate reader (Multiskan GO, Thermo, USA).
Statistical analysis
The effects of water deficits imposed at different stages on the waxy maize growth index, grain yield, yield components, and grain quality were analyzed by analysis of variance using the General Linear Model procedure (GLM) in SPSS 19.0 (IBM Inc., Chicago, IL, USA). Duncan’s new multiple range difference method was used to test the significance of the difference at the P<0.05 level. Figures were drawn with Origin 2017 (OriginLab, USA). Principal component analysis was used to determine the comprehensive impact of the water deficit.
Results
Effects of water deficits on plant height and leaf area index
Plant height and leaf area index (LAI) of waxy maize varied significantly with growing stages and growing seasons (P<0.01; Table 4). The results of the two growing seasons showed that plant height and LAI decreased most significantly under the water deficit imposed at the V6–VT and VT–R2 stages (Table 4), and the change trends in the two growing seasons were basically the same (Figure 2). At the end of the water deficit period of V6–VT and VT–R2, all plant heights and LAI values of DV6–VT and DVT–R2 were significantly lower than those of the CK treatment. Compared with CK, the plant height under DV6–VT and DVT–R2 treatments at the R3 stage decreased by 10.7% and 9.0% (P<0.05), and the LAI decreased by 22.4% and 19.5% (P<0.05), respectively. The DR2–R3 treatment did not exhibit significant effects on plant height and LAI, but because of temperature, light, and other reasons factors, the plant height of waxy maize in 2019 was higher than in 2020. It was clearly indicated that a water deficit imposed at the V6–VT and VT–R2 stages may severely limit the plant growth and leaf development of waxy maize.
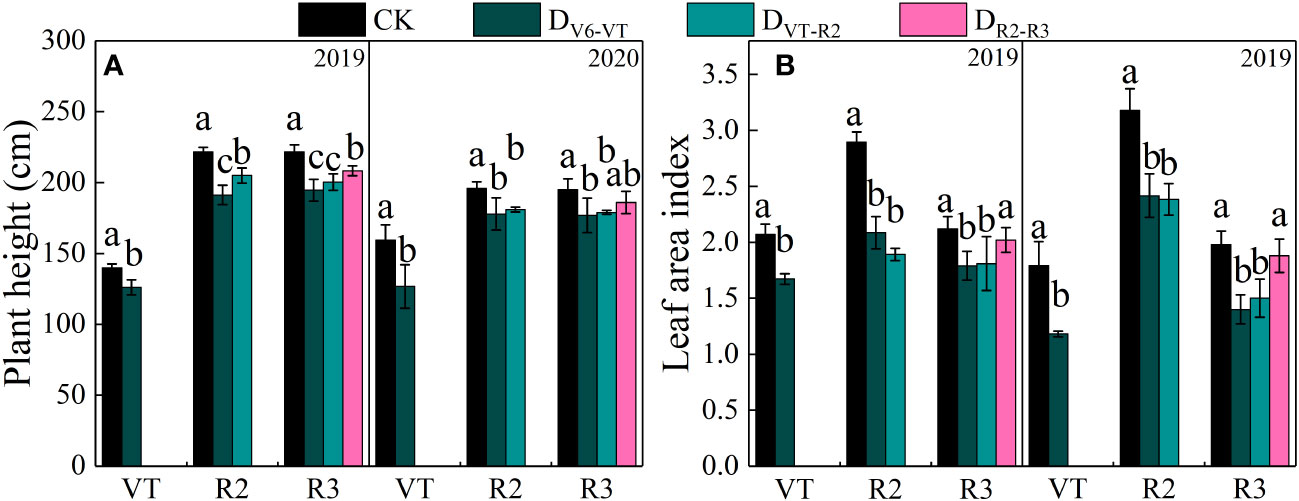
Figure 2 Plant heights and LAI of waxy maize under water deficits at different growing stages in the 2019 and 2020 seasons. Different lowercase letters during the same year indicated significant at 0.05 level. The X axes are years. CK, control; DV6–VT, water deficit from six leaf stage (V6) to tasseling stage (VT); DVT–R2, water deficit from tasseling stage to blister stage (R2); DR2–R3, water deficit from blister stage to milk stage (R3); VT, tasseling stage; R2, blister stage; R3, milk stage. (A) plant height of waxy maize; (B) leaf area index of waxy maize.
Effects of water deficits on MDA, antioxidant enzymes, and osmotic adjustment substances in maize leaves
MDA content and antioxidant enzyme activities in waxy maize leaves under different water-deficit treatments are shown in Figure 3. Compared with CK, the MDA content in DV6–VT and DVT–R2 increased by 40.8% and 46.0%, respectively (P<0.05, Figure 3A), while the MDA content in DR2–R3 was significantly decreased by 30.1% (P<0.05). These results indicated that the recovery of plants in the DV6–VT and DVT–R2 treatments was weak after re-watering. It may be the main reason that changes in CAT were insignificant under DV6–VT treatment, and the activities of SOD and POD were significantly decreased by 26.8% and 25.8%, respectively, compared with CK (P<0.05). The oxidative damage to waxy maize plants still appeared obviously at the milk stage, even after a long-term release of the water deficit imposed at the jointing stage. DVT–R2 significantly increased CAT by 33.6% (P<0.05) but decreased significantly SOD and POD by 19.1% and 18.4% (P<0.05), which indicated that the oxidative damage caused by the water deficit at the VT–R2 stage can only be alleviated to a certain extent. DR2–R3 exhibited less oxidative damage and obvious increases in SOD, CAT, and POD by 24.5%, 83.8%, and 24.5%, respectively (P<0.05), which indicated that the damage caused by water deficit at the R2–R3 stage may have been almost completely alleviated at the milk stage.
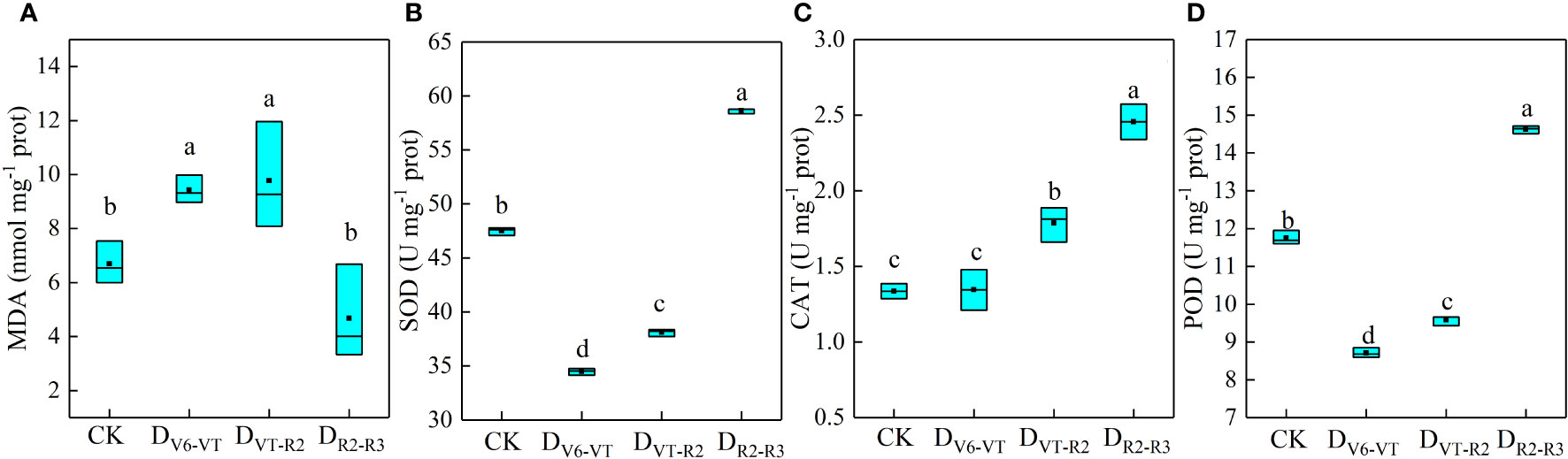
Figure 3 MDA contents and antioxidant enzyme activities of waxy maize leaves under water deficits at different growing stages in the 2019 season. Lowercase letters indicate the difference of different treatments at 0.05 level; MDA, malonaldehyde; SOD, superoxide dismutase; CAT, catalase; POD, peroxidase. the box from bottom to top indicated the lower quartile, median and upper quartile respectively, and the middle black box indicated the mean value. CK, control; DV6–VT, water deficit from six leaf stage (V6) to tasseling stage (VT); DVT–R2, water deficit from tasseling stage to blister stage (R2); DR2–R3, water deficit from blister stage to milk stage (R3). (A) MDA content of leaves; (B) SOD activities of leaves; (C) CAT activities of leaves; (D) POD activities of leaves.
The soluble protein, soluble sugar, and free proline in waxy maize leaves at the R3 stage are shown in Figure 4. Results showed that the recovery of waxy maize plants after re-watering was poor under DV6–VT and DVT–R2 treatments. Compared with CK, the soluble sugars and soluble proteins in the DV6–VT treatment increased by 95.4% and 38.1% (P<0.05) and by 42.0% and 26.4% (P<0.05) in the DVT–R2 treatment, respectively. These increases were beneficial to maintaining cell water potential under water deficits, reducing leaf water loss, and improving leaf water use efficiency. The DV6–VT treatment had the highest levels of soluble sugar, soluble protein, and proline. Meanwhile, the soluble sugar content in the DR2–R3 treatment increased by 30.9% (P<0.05), but the soluble protein decreased by 19.1% compared with the CK treatment. It was further indicated that a water deficit in the V6–VT stage had the greatest impact on the relevant index in waxy maize leaves and the smallest effects from a water deficit in the R2–R3 stage.
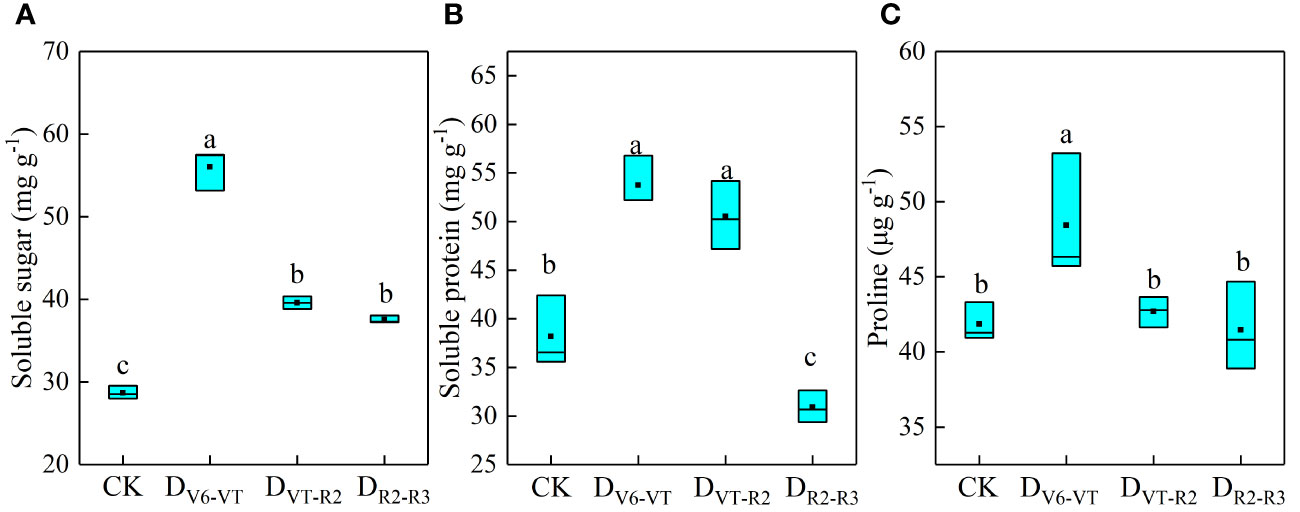
Figure 4 Contents of osmotic adjustment substances of waxy maize leaves under water deficits imposed at different growing stages in the 2019 season. Lowercase letters indicate the difference of different treatments at 0.05 level; the box from bottom to top indicated the lower quartile, median and upper quartile respectively, and the middle black box indicated the mean value. CK, control; DV6–VT, water deficit from six leaf stage (V6) to tasseling stage (VT); DVT–R2, water deficit from tasseling stage to blister stage (R2); DR2–R3, water deficit from blister stage to milk stage (R3). (A) soluble sugar contents of leaves; (B) soluble protein contents of leaves; (C) proline contents of leaves.
Effects of water deficits on photosynthetic characteristics
Under water deficit, plants usually reduced water loss by closing partially stomates, while the photosynthetic recovery of waxy maize was different after release from the water deficit imposed at different growing stages (Figure 5). The changes in SPAD and photosynthetic characteristics in the two growing seasons were basically the same (Figure 5). After water deficit at the V6–VT stage, SPAD and gas exchange parameters of leaves decreased, but leaf water use efficiency (LWUE) increased. After a water deficit at the VT–R2 stage, Pn, Gs, Ci, and Tr of leaves decreased significantly. Compared with CK, the DV6–VT treatment reduced the SPAD of waxy maize leaves (Figure 5A), and the DV6–VT and DVT–R2 treatments significantly decreased Pn, Gs, and Tr (Figures 5B, C, E) and Ci of waxy maize leaves at the R3 stage. Both DV6–VT and DVT–R2 treatments increased LWUE significantly (Figure 5F). But no significant differences in Pn, Ci, Gs, Tr, and LWUE between DR2–R3 and CK were investigated. With the postponement of the water deficit stage, Pn, Gs, and Tr showed an increasing trend, while Ci showed a trend of decreasing at the beginning and then increasing later (Figure 5D), while LWUE showed a firmly decreasing trend (Figure 5F).
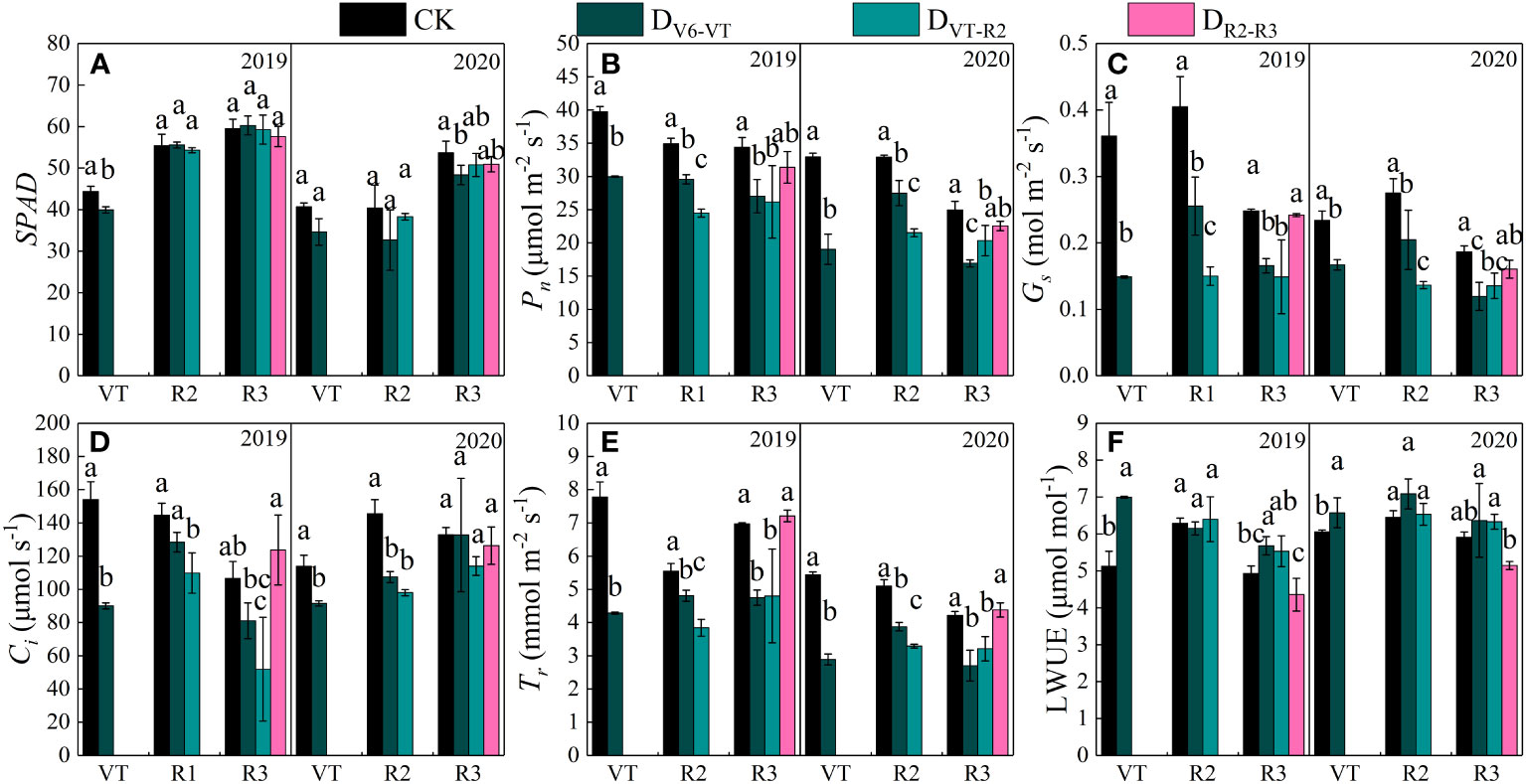
Figure 5 Photosynthetic characteristics of waxy maize leaves under water deficits at different growing stages in the 2019 and 2020 seasons. Different lowercase letters during the same stage indicated significant at 0.05 level. SPAD, leaf chlorophyll content index; Pn, net photosynthetic rate; Gs, stomatal conductance; Ci, intercellular CO2 concentration; Tr, transpiration rate; LWUE, leaf water use efficiency. The X axes are years. CK, control; DV6–VT, water deficit from six leaf stage (V6) to tasseling stage (VT); DVT–R2, water deficit from tasseling stage to blister stage (R2); DR2–R3, water deficit from blister stage to milk stage (R3). (A) SAPD value of leaves; (B) net photosynthetic rate of leaves, Pn; (C) stomatal conductivity of leaves, Gs; (D) intercellular CO2 concentration of leaves, Ci; (E) transpiration rate of leaves, Tr; (F) leaf water use efficiency of leaves, LWUE.
Effects of water deficits on ear traits
The results of the ANOVA in two growing seasons showed that there were significant differences in grains per ear and 100-grain weight under different water deficits imposed at different growth stages of waxy maize (P<0.01; Table 4). The 100-grain weight of DV6–VT was significantly lower than those of other treatments, decreasing by 19.4% compared with CK (P<0.05; Figure 6A). Meanwhile, the grain yield per ear of the DVT–R2 treatment was the lowest and was 23.1% lower than that of CK (P<0.05; Figure 6B).
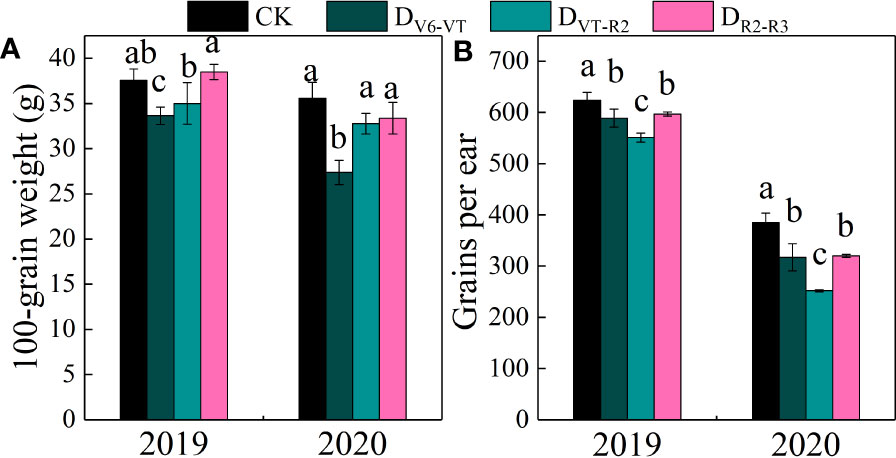
Figure 6 100-grain weight and grains per ear of waxy maize under water deficits at different growing stages in the 2019 and 2020 seasons. Different lowercase letters during the same stage indicated significant at 0.05 level. The X axes are years. CK, control; DV6–VT, water deficit from six leaf stage (V6) to tasseling stage (VT); DVT–R2, water deficit from tasseling stage to blister stage (R2); DR2–R3, water deficit from blister stage to milk stage (R3). (A) 100-grain weight of fresh grains; (B) grains per ear of fresh ear.
Ear length, ear diameter, bald tip length, grain rows per ear, and grains per row significantly varied with water deficit treatments (P<0.01; Table 4; Figures 7A–E). The results of multiple comparisons in two growing seasons showed that the ear length of DV6–VT, compared with CK, decreased the most obviously, followed by DVT–R2. Meanwhile, the ear diameter under the DVT–R2 treatment decreased the most significantly, and grain rows per ear and grains per row of DVT–R2 decreased significantly due to the decrease in ear length and ear diameter (Table 4). Compared with CK, the ear length of DV6–VT and DVT–R2 decreased by 12.7% and 8.5%, respectively (P<0.05; Figure 7A), while the ear diameter, grain rows per ear, and grains per row of DVT–R2 decreased by 3.2%, 12.6%, and 16.8%, respectively (P<0.05; Figures 7B, D, E). It was the decrease in grain rows per ear and grains per row that resulted in the decrease in grains per ear. Due to the stage differences in imposed water deficit stages, the maturity dates of ears were obviously different, which resulted in different grain moisture contents at harvest. The grain moisture content of DV6–VT treatment was significantly lower than that of CK, while no remarkable differences were investigated between the grain moisture contents of DVT–R2 or DR2–R3 treatments and that of CK at the end of R3 stage (Figure 7F).
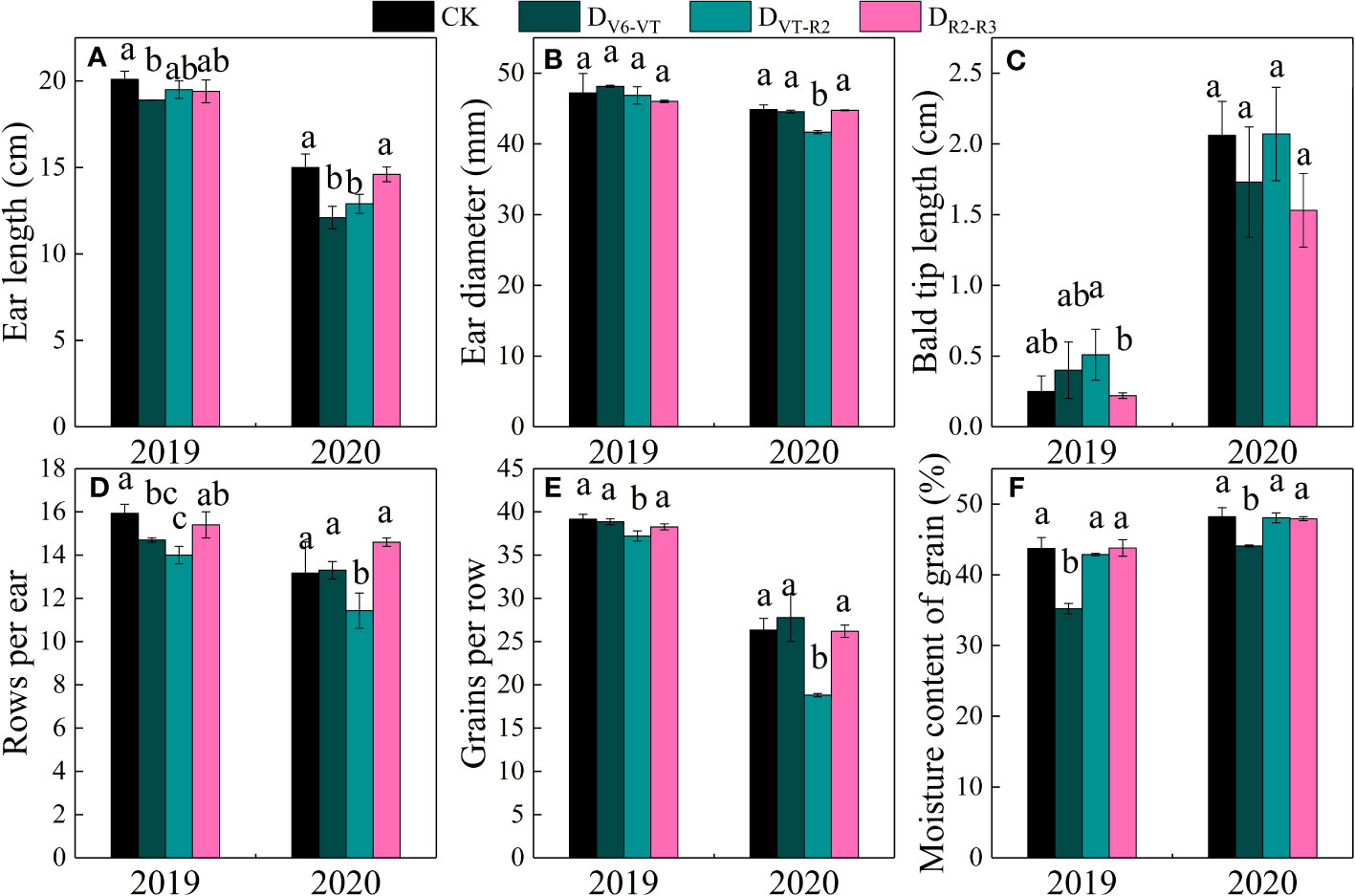
Figure 7 Ear traits of waxy maize under water deficits at different growing stages in the 2019 and 2020 seasons. Different lowercase letters during the same stage indicated significant at 0.05 level. The X axes are years. CK, control; DV6–VT, water deficit from six leaf stage (V6) to tasseling stage (VT); DVT–R2, water deficit from tasseling stage to blister stage (R2); DR2–R3, water deficit from blister stage to milk stage (R3). (A) ear length; (B) ear diameter; (C) bald tip length; (D) rows per ear; (E) grains per row; (F) moisture content of grains.
Effects of water deficits on fresh ear yield
Fresh ear yield with husks (HFY) and fresh ear yield (FY) were affected very significantly by the water deficit stage and growing seasons (P<0.01; Table 4). The ear yield of waxy maize varied under different water deficit treatments. Water deficit at the V6–VT stage showed the greatest effect on the ear yield, followed by those at the VT–R2 stage, and the least effect under water deficit at the R2–R3 stage (Figure 8). Compared with those under CK, mainly due to the varying grain number per ear and 100-grain weight under water deficit at different stages, the HFY and FY decreased by 22.0% and 20.3% under DV6–VT (P<0.05), by 14.3% and 14.2% under DVT–R2 (P<0.05), and both less than 10.0% under DR2–R3, respectively.
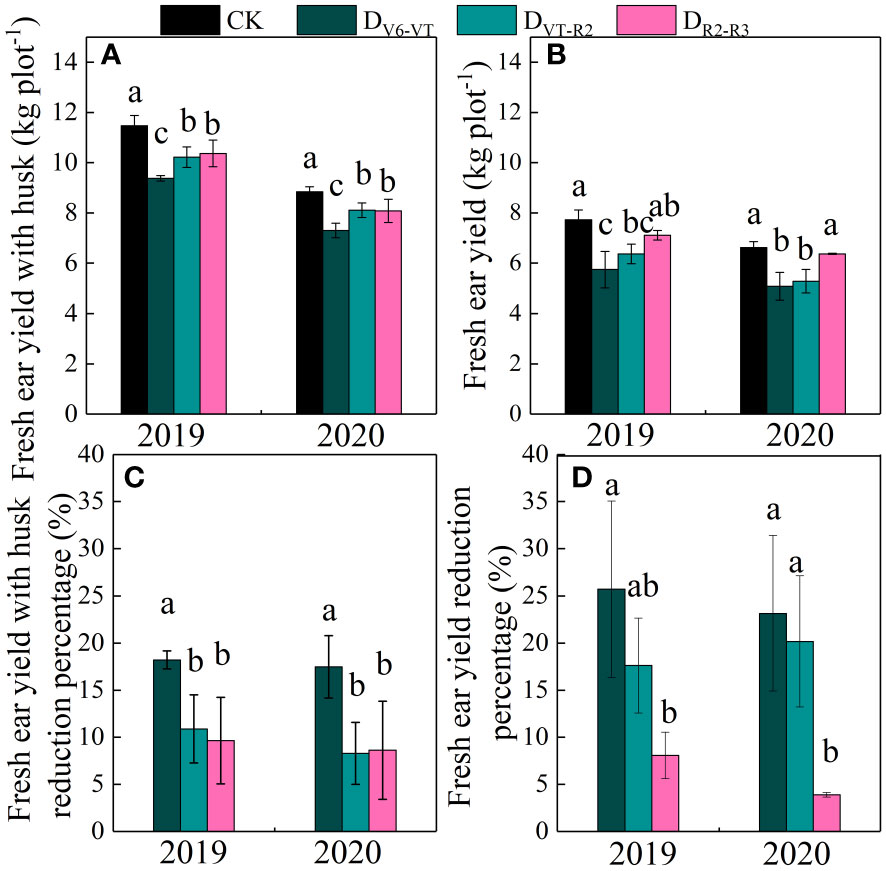
Figure 8 Ear yields of waxy maize under water deficits at different growing stages in the 2019 and 2020 seasons. Different lowercase letters during the same stage indicated significant at 0.05 level. The X axes are years. CK, control; DV6–VT, water deficit from six leaf stage (V6) to tasseling stage (VT); DVT–R2, water deficit from tasseling stage to blister stage (R2); DR2–R3, water deficit from blister stage to milk stage (R3). (A) fresh ear yield with husk; (B) fresh ear yield; (C) fresh ear yield with husk reduction percentage; (D) fresh ear yield reduction percentage.
Effects of water deficits on grain quality
Total protein, grain soluble sugar, and starch contents in waxy maize grains varied significantly with the different water deficit stage treatments and growing seasons (P<0.01; Table 4). Water deficit increased the contents of total protein, starch, and lysine in fresh waxy maize grains, but reduced the content of soluble sugar in fresh waxy maize grains. However, the effects of water deficits at different growing stages on grain quality were obviously different, and the changing trends in the two growing seasons were consistent (Figure 9). The soluble sugar content of the DV6–VT treatment was the lowest due to a 31.6% decrease over that of CK (Figure 9B; P<0.05), followed by the DVT–R2 treatment with a decrease of 14.1% (P<0.05), while the decrease was 4.0% under the DR2–R3 treatment. The total protein content and starch content were the greatest under the DV6–VT treatment (Figures 9A, C) with an increase of 8.5% and 66.5%, respectively (P<0.05) than those under CK, respectively (P<0.05). The following were those under the DVT–R2 treatment with an increase of 7.1% and 20.1% (P<0.05), and under the DR2–R3 treatment with an increase of 2.0% and 1.5% under the DR2–R3 treatment. Compared with the CK treatment, the DV6–VT, DVT–R2, and DR2–R3 treatments increased the lysine content in grains by 16.7%, 23.8%, and 16.7%, respectively.
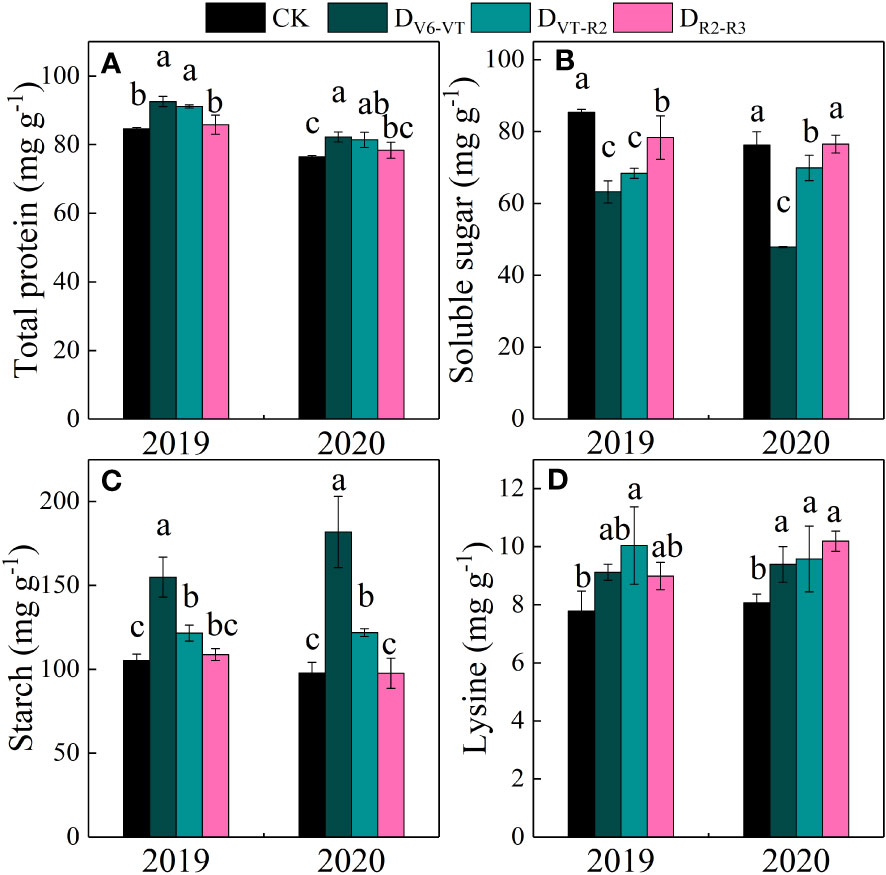
Figure 9 Grain quality traits of waxy maize under water deficits at different growing stages in the 2019 and 2020 seasons. Different lowercase letters during the same stage indicated significant at 0.05 level. The X axes are years. CK, control; DV6–VT, water deficit from six leaf stage (V6) to tasseling stage (VT); DVT–R2, water deficit from tasseling stage to blister stage (R2); DR2–R3, water deficit from blister stage to milk stage (R3). (A) total protein of fresh grains; (B) soluble sugar of fresh grains; (C) starch of fresh grains; (D) Lysine of fresh grains.
Figure 9 indicated that the water deficit at the V6–VT stage had the most significant effects on the total protein and starch content of waxy maize grains, so the changes in amylose, amylopectin, and component protein content of waxy maize grains under the DV6–VT treatment were selected as representative to show the effects of water deficit on grain quality index (Table 5). Compared with CK, the amylose and amylopectin content under the DV6–VT treatment increased by 15.9% and 92.5% (P<0.05), the contents of glutelin and globulin decreased by 9.1% and 56.9%, respectively (P<0.05), while the contents of alcohol-soluble protein and albumin increased by 24.0% and 59.9%, respectively (P<0.05).
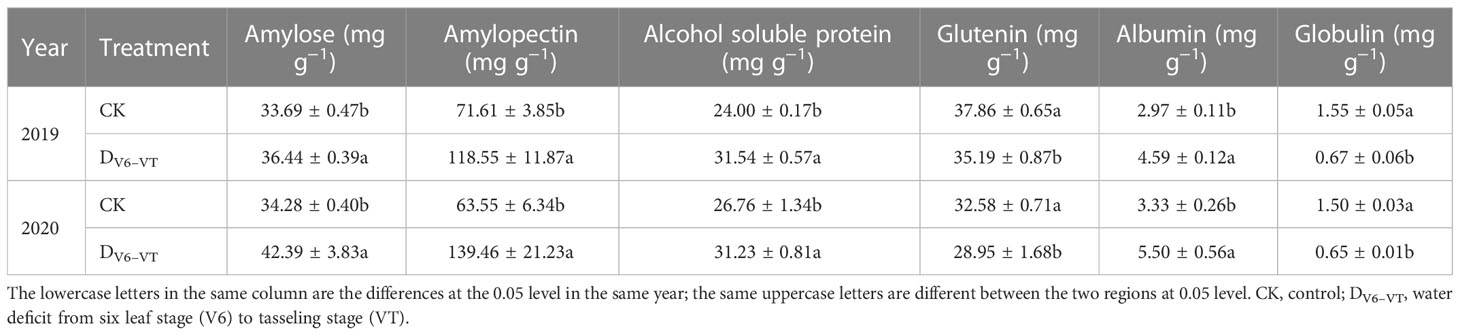
Table 5 Effects of water deficit imposed at jointing stage on starch and protein contents of waxy maize grains in the 2019 and 2020 growing seasons.
Principal component analysis
Principal component analysis (PCA) was performed on all measured indexes of waxy maize in the two growing seasons. Three principal components (PC1, PC2, and PC3) were extracted in 2019 and 2020 (λ >1), and the eigenvalues (λ) of principal component 1 (PC1) in 2019 and 2020 were 18.88 and 13.37, and explained 69.9% and 66.9% of the total variation, respectively. λ of PC2 in 2019 and 2020 were 5.19 and 4.22, which contributed 19.2% and 21.1% of the total variation, respectively. λ of PC3 in 2019 and 2020 were 2.93 and 2.41, which explained 10.9% and 12.0% of the total variation, respectively (Table 6). The largest loading variable in 2019 was CAT, followed by bald tip length, SPAD, lysine, and ear diameter. The largest loading variable in 2020 was Ci, followed by ear length, ear diameter, grain number per ear, and fresh ear yield with husks. These results indicated that leaf physiology traits were most sensitive to water deficit, followed by ear traits and fresh ear yield in waxy maize (Figure 10).
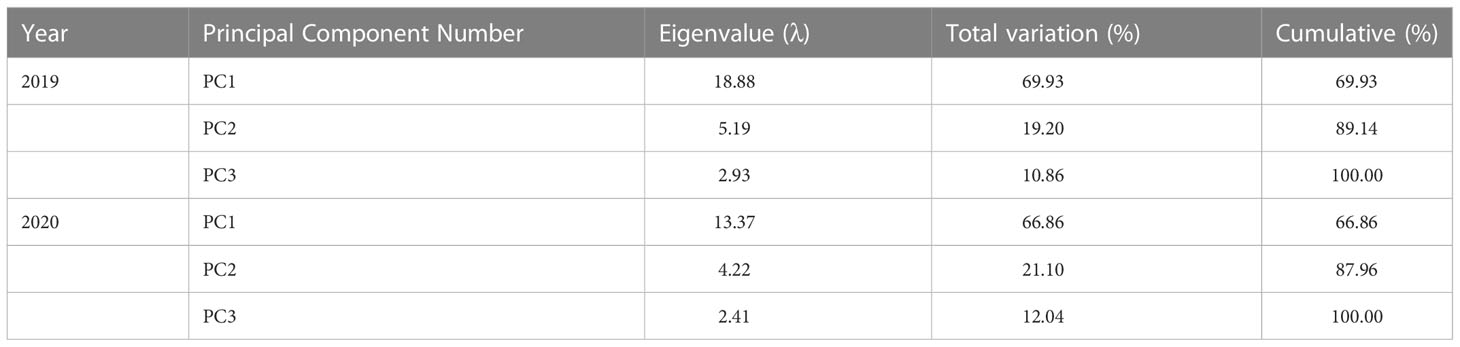
Table 6 Eigenvalues and variances of principal component analysis in the 2019 and 2020 growing seasons.
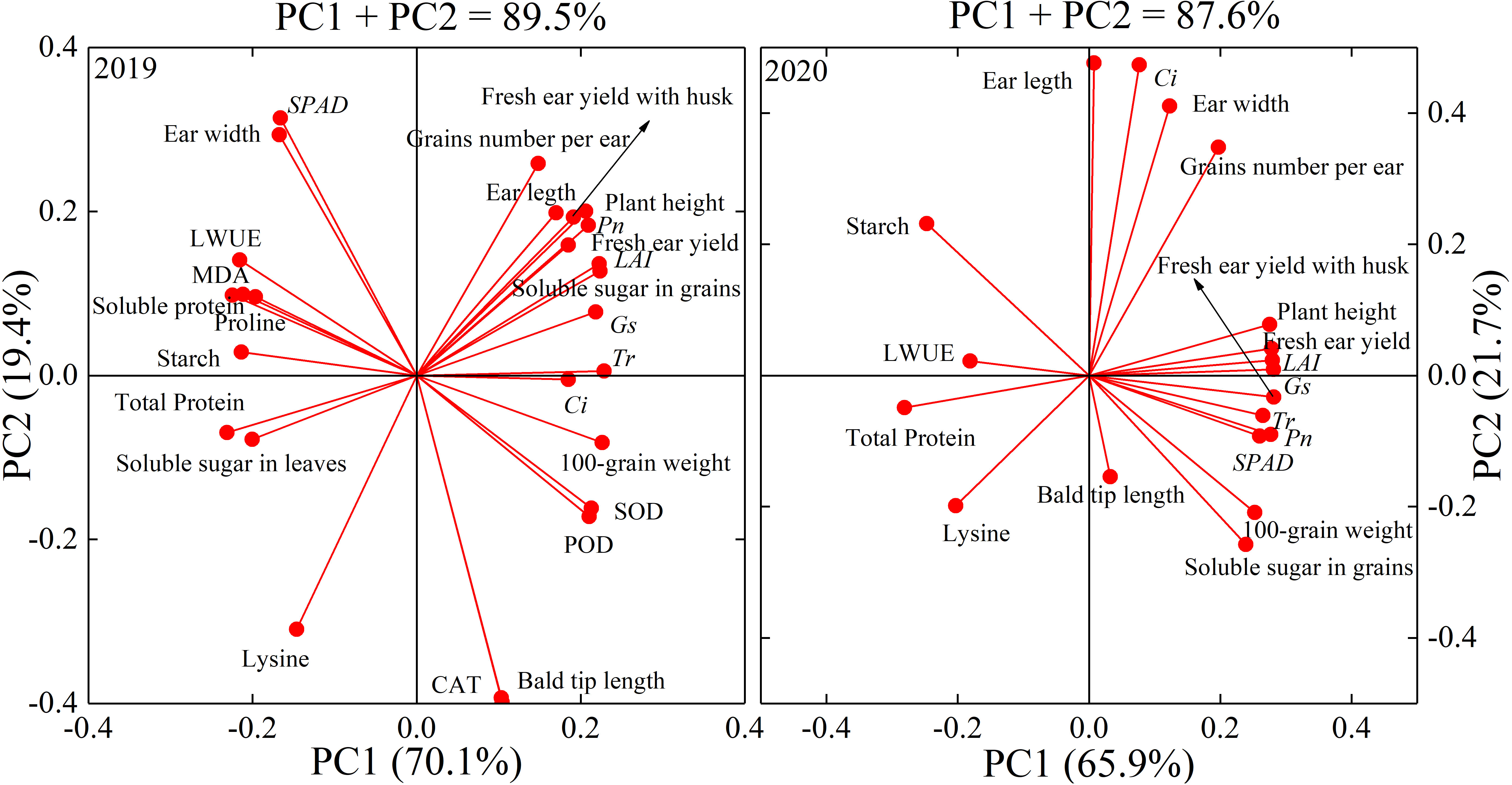
Figure 10 Loading diagram of principal component analysis in the 2019 and 2020 seasons. LAI, leaf area index; MDA, malonaldehyde; SOD, superoxide dismutase; CAT, catalase; POD, peroxidase; SPAD, leaf chlorophyll content index; Pn, net photosynthetic rate; Gs, stomatal conductance; Ci, intercellular CO2 concentration; Tr, transpiration rate; LWUE, leaf water use efficiency. PCn indicates the extracted principal component.
Discussion
Effects of water deficits at different growing stages on leaf physiological characteristics of waxy maize
When plants are subjected to water stress, a large amount of reactive oxygen species will accumulate in the tissues, which will break the mechanism of reactive oxygen species production and scavenging, triggering the production of MDA by cell membrane peroxidation (Liu et al., 2015). Meanwhile, plant cells produce many kinds of antioxidant enzymes such as SOD, CAT, and POD to scavenge reactive radicals (Simova et al., 2008). Studies have shown that the accumulation of MDA varies with growing stages and that the longer a water deficit lasted, the more MDA was accumulated (Liu, 2013b). In our study, the MDA content in waxy maize leaves under the DV6–VT and DVT–R2 treatments was significantly higher than that under the CK treatment at the R3 stage, and the MDA value under DV6–VT was greater than that under DVT–R2. Our results are similar to the results of Li et al. (2017), which indicated that the magnitude of MDA accumulation at the jointing stage was greater than that at the filling stage. On the one hand, SOD, CAT, and POD activities in the cells increased to scavenge excessive reactive oxygen species (Liu et al., 2015). In this study, both SOD and POD activities were significantly lower in the DV6–VT treatment compared with those in CK, mainly due to the inhibition of SOD and POD activities by excessive MDA (Liu, 2013b). But SOD, CAT, and POD activities in the DR2–R3 treatment increased significantly, which indicated that the plant functions recovered better after re-watering from the R2 to the R3 water deficit. Bu et al. (2009) also demonstrated an increase in protective enzyme activity after re-watering. Meanwhile, the increases in proline and total carbohydrate contents under water deficit are beneficial to protect maize plant tissues from oxidative damage (Bu et al., 2009; Anjum et al., 2016). Previous studies have shown that proline and MDA are in a reciprocal relationship, with proline accumulation helpful for reducing MDA damage to the plant, while soluble sugars and soluble proteins increase beneficial for maintaining cellular osmotic pressure (Liu et al., 2015; Li et al., 2017). In our study, the soluble sugar, soluble protein, and proline contents of waxy maize leaves under DV6–VT were significantly higher than those under CK at the R3 stage, while these contents under both DVT–R2 and DR2–R3 were similar to those under the CK treatment. Even though the soluble sugar content increased after re-watering (Bu et al., 2009), the damage caused by the water deficit imposed at the V6–VT stage remained unrecovered. After re-watering, the recovery of antioxidant enzyme activity was very poor, photosynthesis was adversely affected, and an irreversible effect resulted in waxy maize plants grown under the DV6–VT treatment in our study, mainly due to the long water deficit period (approximately 20 d, see Table 3) in the V6–VT stage.
Photosynthesis is the main physiological process driving plant growth and is highly sensitive to water deficits (Chaves et al., 2009). Water deficits reduce Pn, Tr, and Gs, which in turn reduce maize plant biomass and grain yield (Ali and Ashraf, 2011; De Carvalho et al., 2011; Li et al., 2018). In our study, Pn and Gs decreased significantly under both the DV6–VT and DVT–R2 treatments with nearly the same variation pattern, which was very similar to those results from Cai et al. (2017). Ci was also significantly reduced in the DVT–R2 treatment, mainly due to stomatal limitation, but was not significantly affected in the DV6–VT treatment. SPAD value decreased obviously under the DV6–VT treatment, because reactive oxygen species generated by water deficit severely degraded severely chlorophyll pigments (Anjum et al., 2016) and significantly decreased chlorophyll content (Ye et al., 2020b). Water deficit also may reduce photosynthetic enzyme (PEPCase, RuBPase) activity (Ye et al., 2020a), and it caused a remarkable reduction in photosynthetic rate under the DV6–VT treatment in this study. Reduced Gs also limited transpiration and resulted in a significant decrease in Tr under the DV6–VT and DVT–R2 treatments. But LWUE increased when plants were exposed to a water deficit, due mainly to the obvious reduction in transpiration and water consumption (Yao et al., 2012).
Effects of water deficits at different growing stages on yield and yield traits of waxy maize
The reduction of photosynthetic rate under water deficit may lead to a reduction of plant photoassimilate deposition and ultimately the loss of grain yield (Kimaro et al., 2015; Ye et al., 2020a; Ulfat et al., 2021). Previous studies have shown that a water deficit during grain filling reduced the grain fresh weight, water content, and grains per ear of waxy maize (Guo et al., 2022). In this study, fresh ear yield with husk and fresh ear yield were significantly reduced, and the grain number per ear and 100-grain weight also showed some reducing trends under the DV6–VT and DVT–R2 treatments. The most obvious decrease in grain number per ear was investigated in the DVT–R2 treatment, while the most remarkable decrease in 100-grain weight occurred in the DV6–VT treatment. Previous results already indicated that a water deficit imposed at the tasseling, flowering, and filling stages could reduce the grain number per ear and grain weight of waxy maize, resulting in yield loss (Sun, 2014). Similar to the results of Xiao et al. (2011), the yield loss under treatment with a water deficit that occurred at the jointing stage was less than that under a water deficit imposed at the filling stage. The yield loss trend under water deficit may be described as that the yield loss reduces gradually as the period of water shortage becomes later and later. The yield reduction was mainly caused by the decrease in grain number per ear and 100-grain weight (Wen, 2020). Yang et al. (2019) also showed that a water deficit imposed at the tasseling stage significantly reduced the grain number per ear and 100-grain weight of fresh waxy maize, mainly due to the decreases in pollen dispersal and grain filling rates. Water deficit not only affects the formation and vitality of pollen but also affects the differentiation of maize spikelets (Li et al., 2022), resulting in a decrease in the number of rows per ear, grain number per ear, and final yield reduction. This study showed that high accumulation of MDA in leaves resulted in quicker leaf senescence, and then decreases in LAI, photosynthesis rate, and dry matter accumulation under the DV6–VT treatment. The greatest reductions in 100-grain weight and grain moisture content were investigated under DV6–VT in this study, indicating that the DV6–VT treatment caused the process of starch accumulation in advance and led to poor filling quality. The Pn values in 2020 were generally lower than those in 2019, mainly due to less radiation and a lower temperature in 2020 (Figure 5B), which resulted in a lower 100-grain weight and less dry matter accumulation in 2020 (Gao et al., 2017). The worse radiation environment in the 2020 growing season also led to a decrease in pollen viability and a subsequent decline in grains per ear, which may be the main reason for the remarkable differences in fresh ear yields between the two growing seasons (Zhou et al., 2013).
Effects of water deficits at different growing stages on grain quality of waxy maize
Grain quality was closely related to leaf physiology and yield. Studies have shown that an increase in wheat grain protein content is closely associated with a decrease in yield under water deficit (Ozturk and Aydin, 2004; Flagella et al., 2010). It is also indicated that the starch content of grain was also closely related to yield in barley (Worch et al., 2011). In this experiment with waxy maize, the DV6–VT and DVT–R2 treatments increased the total protein contents while decreasing the soluble sugar contents. In addition, the starch and lysine contents were increased by the water deficit imposed at all growing stages. Several studies have shown that water deficit at the grain formation stage may reduce the starch content and increase the total protein content of waxy maize grain (Sun, 2014; Wang et al., 2021b). The increase in protein content was mainly due to the concentration effect of reduced biomass under water deficits (Rotundo and Westgate, 2009). Ma et al. (2006) also showed that the protein and lysine contents of maize grains increased under moderate water deficit conditions. The changes in protein and lysine contents in this study showed very similar trends to those in Ma et al. (2006). DV6–VT resulted in increases in glutenin and albumin contents, but decreases in alcoholic-soluble protein and globulin contents in grains. For the grain quality of waxy maize, the effects of water deficit on grain starch content in this study were opposite to those shown in some previous studies (Sun, 2014; Wang et al., 2021b), maybe mainly because the previous results were investigated from matured grains, whereas fresh grains were used in this study. Debon et al. (1998) and Silva et al. (2001) found that the increase in the starch concentration of fresh fruit of potato was mainly due to the concentration effect caused by the decrease in water content in fresh fruit. Guo et al. (2022) and Shi et al. (2018) also showed an increase in starch content in fresh waxy maize grains under drought stress and indicated that acceleration of grain filling and decreases in grain water content under drought (Figure 7F) might be the main reasons for the starch content increase. Meanwhile, water deficit caused a significantly increase in abscisic acid (ABA) content in maize plant tissues, and then led to the activity increases of four key enzymes related closely to converting soluble sugar into starch (Zhang et al., 2012). Yi et al. (2014) indicated that a water deficit imposed at V6–VT advanced the starch accumulation process in sorghum, and Dai et al. (2008) also indicated that a water deficit increased starch accumulation in wheat at the early filling stage. In our study, both amylose and amylopectin increased under water deficit at the V6–VT period, and the percentage of amylopectin increase was much greater than that of amylose. Water deficit reduced the expression of starch branching enzymes SEBI and SBEIIb genes, which resulted in decreases in amylose and amylopectin content, and then the final total starch content (Wu et al., 2022). The results of PCA (Figure 10) showed that the antioxidant enzymes and gas exchange parameters in leaves were items affected early by water deficit, and ear growth, grain number per ear, 100-grain weight, the water content of fresh grains were affected lately, and fresh ear yield and grain quality as the finally affected items.
Conclusion
The water deficit imposed at the V6–VT stage limits severely the growth of waxy maize plants in the vegetative stage, reduces the 100-grain weight of waxy maize, and results in a significant reduction in fresh ear yield and grain starch accumulation, mainly due to accelerated grain ripening. A water deficit imposed at the VT–R2 stage affects the flowering and pollination of waxy maize plants and results in a significant reduction in grain number per ear and the final ear yield. However, a moderate water deficit imposed at the R2–R3 stage had little effect on growth and development, fresh ear yield, total protein, soluble sugar, and starch content in fresh grains of waxy maize but had significant effects on increasing the lysine content in fresh grains. The results in this study also suggested that more in-depth and comprehensive studies on the effects of water deficit duration imposed at different growing stages should be performed, and suitable techniques for reducing loss of yield and quality caused by water deficit should be developed.
Data availability statement
The raw data supporting the conclusions of this article will be made available by the authors, without undue reservation.
Author contributions
CH: investigation, data curation, formal analysis, visualization, and writing—original draft preparation. AQ: conceptualization, methodology, data curation, formal analysis, writing, and manuscript reviews. YG and SM: methodology, writing—editing and funding acquisition. ZuL, BZ, and DN: provided guidance and manuscript reviews. WG and MS: investigation. KZ and ZiL: conceptualization, writing—editing, supervision, project administration, and funding acquisition. All authors contributed to the article and approved the submitted version.
Funding
This research was jointly supported by the China Agriculture Research System (CARS-02-18), the Key R&D and promotion projects in Henan Province (212102110291 and 222102110163), the Henan Provincial Natural Science Foundation (202300410553), the Agricultural Science and Technology Innovation Program (ASTIP), the National Natural Science Foundation of China (32101856), and the Key Project of Water Conservancy Science and Technology in Henan Province (Key Technology Innovation and Application of Agricultural Intensive and Efficient Water Use in Irrigation Areas).
Conflict of interest
The authors declare that the research was conducted in the absence of any commercial or financial relationships that could be construed as a potential conflict of interest.
Publisher’s note
All claims expressed in this article are solely those of the authors and do not necessarily represent those of their affiliated organizations, or those of the publisher, the editors and the reviewers. Any product that may be evaluated in this article, or claim that may be made by its manufacturer, is not guaranteed or endorsed by the publisher.
References
Ali, Q., Ashraf, M. (2011). Induction of drought tolerance in maize (Zea mays l.) due to exogenous application of trehalose: Growth, photosynthesis, water relations and oxidative defence mechanism. J. Agron. Crop Sci. 197, 258–271. doi: 10.1111/j.1439-037X.2010.00463.x
Anjum, S. A., Tanveer, M., Ashraf, U., Hussain, S., Shahzad, B., Khan, I., et al. (2016). Effect of progressive drought stress on growth, leaf gas exchange, and antioxidant production in two maize cultivars. Environ. Sci. pollut. R. 23 (17), 17132–17141. doi: 10.1007/s11356-016-6894-8
Beckles, D. M., Thitisaksakul, M. (2014). How environmental stress affects starch composition and functionality in cereal endosperm. Die Stärke 66 (1-2), 58–71. doi: 10.1002/star.201300212
Begcy, K., Walia, H. (2015). Drought stress delays endosperm development and misregulates genes associated with cytoskeleton organization and grain quality proteins in developing wheat seeds. Plant Sci. 240, 109–119. doi: 10.1016/j.plantsci.2015.08.024
Bu, L. D., Zhang, R. H., Han, M. M., Xu, J. Q., Chang, Y. (2009). The physiological mechanism of compensation effect in maize leaf by re-watering after drought stress. Acta Agr. Bor-Occid. Sin. 8 (2), 88–92.
Cai, F., Mi, N., Ji, R. P., Zhao, X. L., Shi, K. Q., Yang, Y., et al. (2017). Effects of drought stress and subsequent rewatering on major physiological parameters of spring maize during the key growth periods. Chin. J. Appl. Ecol. 28 (11), 3643–3652. doi: 10.13287/j.1001-9332.201711.015
Chaves, M. M., Flexas, J., Pinheiro, C. (2009). Photosynthesis under drought and salt stress: Regulation mechanisms from whole plant to cell. Ann. Bot. 103 (4), 551–560. doi: 10.1093/aob/mcn125
Dai, Z. M., Wang, Z. L., Zhang, M., Li, W. Y., Yan, S. H., Cai, R. G., et al. (2008). Starch accumulation and activities of enzymes involved in starch synthesis in grains of wheat grown under irrigation and rain-fed conditions. Sci. Agr. Sinica. 46 (5), 34–37. doi: 10.1016/0921-8696(86)90292-6
Debon, S. J., Tester, R. F., Millam, S., Davies, H. V. (1998). Effect of temperature on the synthesis, composition and physical properties of potato microtuber starch. J. Sci. Food Agr. 76 (4), 599–607. doi: 10.1002/(SICI)1097-0010(199804)76:43.0.CO;2-F
De Carvalho, R. C., Cunha, A., Da Silva, J. M. (2011). Photosynthesis by six Portuguese maize cultivars during drought stress and recovery. Acta Physiol. Plant 33, 359–374. doi: 10.1007/s11738-010-0555-1
Erbs, M., Manderscheid, R., Hüther, L., Schenderlein, A., Wieser, H., Dänicke, S., et al. (2015). Free-air CO2 enrichment modifies maize quality only under drought stress. Agron. Sustain. Dev. 35 (1), 203–212. doi: 10.1007/s13593-014-0226-5
Flagella, Z., Giuliani, M. M., Giuzio, L., Volpi, C., Masci, S. (2010). Influence of water deficit on durum wheat storage protein composition and technological quality. Eur. J. Agron. 33 (3), 197–207. doi: 10.1016/j.eja.2010.05.006
Gao, J., Shi, J. G., Dong, S. T., Liu, P., Zhao, B., Zhang, J. W. (2017). Effect of different light intensities on root characteristics and grain yield of summer maize (Zea mays l.). Sci. Agr. Sin. 50 (11), 2104–2113. doi: 10.3864/j.issn.0578-1752.2017.11.016
Guo, J., Qu, L. L., Wang, L. F., Lu, W. P., Lu, D. L. (2022) Effects of post-silking drought stress degree on grain yield and quality of waxy maize. J. Sci. Food Agr. 1–11. doi: 10.1002/jsfa.12250
Han, C., Lu, X., Yu, Z., Li, X., Ma, W., Yan, Y. (2015). Rapid separation of seed gliadins by reversed-phase ultraperformance liquid chromatography (RP-UPLC) and its application in wheat variety and germplasm identification. Biosci. Biotech. Bioch. 79 (5), 808–815. doi: 10.1080/09168451.2014.998618
Hao, Z. C., Singh, V. P. (2015). Drought characterization from a multivariate perspective: A review. J. Hydrol. 527, 668–678. doi: 10.1016/j.jhydrol.2015.05.031
Huang, C., Zhang, W. Q., Wang, H., Gao, Y., Ma, S. T., Qin, A. Z., et al. (2022). Effects of waterlogging at different stages on growth and ear quality of waxy maize. Agr. Water Manage. 266, 207603. doi: 10.1016/j.agwat.2022.107603
IPCC (2014). “Contribution of working group II to the fifth assessment report of the intergovernmental panel on climate change,” in Climate change 2014: Impacts, adaptation, and vulnerability. part a: Global and sectoral aspects. Eds. Field, C. B., Barros, V. R., Dokken, D. J., Mach, K. J., Mastrandrea, M. D. (Cambridge, United Kingdom and New York, NY, USA: Cambridge University Press).
Kimaro, A. A., Mpanda, M., Rioux, J., Aynekulu, E., Shaba, S., Thiong’o, M., et al. (2015). Is conservation agriculture 'climate-smart' for maize farmers in the highlands of Tanzania. Nutr. Cycl. agroecosys. 105 (3), 217–228. doi: 10.1007/s10705-015-9711-8
Knutson, C., Grove, M. (1994). Rapid method for estimation of amylose in maize starches. Cereal. Chem. 71 (5), 469–471.
Li, T. L., Hu, X. T., Wang, W. E., Du, B., Ma, W. G. (2017). Effects of water stress on proline and malondialdehyde content in leaves of spring maize. Water Saving Irrig. 6), 34–37.
Li, X. J., Kang, S. Z., Zhang, X. T., Li, F. S., Lu, H. N. (2018). Deficit irrigation provokes more pronounced responses of maize photosynthesis and water productivity to elevated CO2. Agr. Water Manage. 195, 71–83. doi: 10.1016/j.agwat.2017.09.017
Li, H. W., Tiwari, M., Tang, Y. L., Wang, L. J., Yang, S., Long, H. C., et al. (2022). Metabolomic and transcriptomic analyses reveal that sucrose synthase regulates maize pollen viability under heat and drought stress. Ecotox. Environ. Safe 246, 114191. doi: 10.1016/j.ecoenv.2022.114191
Liu, Y. H. (2013b). Physiological responses and adaptation of summer maize (Zea mays l) to water stress during different growth periods. J. Arid Land Resour. Environ. 27 (2), 171–175. doi: 10.13448/j.cnki.jalre.2013.02.009
Liu, L. M., Klocke, N., Yan, S. P., Rogers, D., Schlegel, A., Lamm, F., et al. (2013a). Impact of deficit irrigation on maize physical and chemical properties and ethanol yield. Cereal. Chem. 90, 453–462. doi: 10.1094/CCHEM-07-12-0079-R
Liu, C., Li, Z. T., Yang, K. J., Xu, J. Y., Wang, Y. F., Zhao, C. J., et al. (2015). Effects of water stress and subsequent rehydration on physiological characteristics of maize (Lea mays) with different drought tolerance. J. Plant Physiol. 51 (5), 702–708. doi: 10.13592/j.cnki.ppj.2015.0038
Lu, D., Cai, X., Zhao, J., Shen, X., Lu, W. (2015). Effects of drought after pollination on grain yield and quality of fresh waxy maize. J. Sci. Food Agr. 95 (1), 210–215. doi: 10.1002/jsfa.6709
Ma, X. L., Cui, Z. H., Chen, J., Xiao, C. L., Zhang, L. J., Wang, Q. X. (2006). Effect of drought stress during seedling stage on the content of grain crude protein, and lysine of maize. J. Maize Sci. 14 (2), 71–74. doi: 10.13597/j.cnki.maize.science.2006.02.026
Markelz, R. J. C., Strellner, R. S., Leakey, A. D. B. (2011). Impairment of C4 photosynthesis by drought is exacerbated by limiting nitrogen and ameliorated by elevated [CO2] in maize. J. Exp. Bot. 62, 3235–3246. doi: 10.1093/jxb/err056
Ma, Y. P., Sun, L. L., Ma, X. Q. (2016). Ecophysiological responses of summer maize to drought and waterlogging in Huang-Huai-Hai plain. Agr. Res. Arid Area 34 (4), 85–93. doi: 10.7606/j.issn.1000-7601.2016.04.13
Ozturk, A., Aydin, F. (2004). Effect of water stress at various growth stages on some quality characteristics of winter wheat. J. Agron. Crop Sci. 190 (2), 93–99. doi: 10.1046/j.1439-037X.2003.00080.x
Park, I. M., Ibanez, A. M., Shoemaker, C. F. (2007). Rice starch molecular size and its relationship with amylose content. Starch-Stärke 59 (2), 69–77. doi: 10.1002/star.200600568
Qin, A., Ning, D., Liu, Z., Sun, B., Zhao, B., Xiao, J., et al. (2019). Insentek sensor: An alternative to estimate daily crop evapotranspiration for maize plants. Water 11 (1), 25. doi: 10.3390/w11010025
Rotundo, J. L., Westgate, M. E. (2009). Meta-analysis of environmental effects on soybean seed composition. Field Crop Res. 110 (2), 147–156. doi: 10.1016/j.fcr.2008.07.012
Sandhya, V. S. K. Z., Ali, S. Z., Grover, M., Reddy, G., Venkateswarlu, B. (2010). Effect of plant growth promoting pseudomonas spp. on compatible solutes, antioxidant status and plant growth of maize under drought stress. Plant Growth Regul. 62 (1), 21–30. doi: 10.1007/s10725-010-9479-4
Santisopasri, V., Kurotjanawong, K., Chotineeranat, S., Piyachomkwan, K., Sriroth, K., Oates, C. G. (2001). Impact of water stress on yield and quality of cassava starch. Ind. Crop Prod. 13, 115–129. doi: 10.1016/S0926-6690(00)00058-3
Shi, L. J., Wen, Z. R., Zhang, S. B., Wang, J., Lu, W. P., Lu, D. L. (2018). Effects of water deficit at flowering stage on yield and quality of fresh waxy maize. Acta Agron. Sin. 44 (8), 1205–1211. doi: 10.3724/SP.J.1006.2018.01205
Silva, J. A. B., Otoni, W. C., Martinez, C. A., Dias, L. M., Silva, M. (2001). Microtuberization of andean potato species (solanum spp.) as affected by salinity. Sci. Hortic-Amsterdam 89 (2), 91–101. doi: 10.1016/S0304-4238(00)00226-0
Simova, S. L., Demirevska, K., Petrova, T., Tsenov, N., Feller, U. (2008). Antioxidative protection in wheat varieties under severe recoverable drought at seedling stage. Plant Soil Environ. 54, 529–536. doi: 10.17221/427-PSE
Si, Z., Muhammad, Z., Mehmood, F., Wang, G., Gao, Y., Duan, A. (2020). Effects of nitrogen application rate and irrigation regime on growth, yield, and water-nitrogen use efficiency of drip-irrigated winter wheat in the north China plain. Agr. Water Manage. 231, 106002. doi: 10.1016/j.agwat.2020.106002
Singh, S., Singh, G., Singh, P., Singh, N. (2008). Effect of water stress at different stages of grain development on the characteristics of starch and protein of different wheat varieties. Food Chem. 108, 130–139. doi: 10.1016/j.foodchem.2007.10.054
Song, H., Li, Y., Zhou, L., Xu, Z., Zhou, G. (2018). Maize leaf functional responses to drought episode and rewatering. Agr. For. Meteorol. 249, 57–70. doi: 10.1016/j.agrformet.2017.11.023
Sun, X. L. (2014). Effects of soil moisture stress during grain filling on grain yield and starch quality of waxy maize (Yangzhou, China: Yangzhou Univ).
Thitisaksakul, M., Jiménez, R. C., Arias, M. C., Beckles, D. M. (2012). Effects of environmental factors on cereal starch biosynthesis and composition. J. Cereal. Sci. 56 (1), 67–80. doi: 10.1016/j.jcs.2012.04.002
Ulfat, A., Shokat, S., Li, X., Fang, L., Großkinsky, D. K., Majid, S. A., et al. (2021). Elevated carbon dioxide alleviates the negative impact of drought on wheat by modulating plant metabolism and physiology. Agr. Water Manage. 250, 106804. doi: 10.1016/j.agwat.2021.106804
Wang, Y., Frei, M. (2011). Stressed food-the impact of abiotic environmental stresses on crop quality. Agr. Ecosyst. Environ. 141 (3-4), 271–286. doi: 10.1016/j.agee.2011.03.017
Wang, J., Fu, P. X., Lu, W. P., Lu, D. L. (2020). Application of moderate nitrogen levels alleviates yield loss and grain quality deterioration caused by post-silking heat stress in fresh waxy maize. Crop J. 8 (6), 1081–1092. doi: 10.1016/j.cj.2019.11.007
Wang, H., Lu, Y. L., Sun, D. P., Yu, D. S., Shi, B., Lin, J. Y., et al. (2014). Breeding of a new waxy maize cultivar ‘Shenkenuo 1’. Acta Agr. Shanghai 30 (6), 50–53.
Wang, J., Mao, Y., Huang, T., Lu, W., Lu, D. (2021b). Water and heat stresses during grain formation affect the physicochemical properties of waxy maize starch. J. Sci. Food Agr. 101 (4), 1331–1339. doi: 10.1002/jsfa.10743
Wang, L., Yan, Y., Lu, W., Lu, D. (2021a). Application of exogenous phytohormones at silking stage improve grain quality under post-silking drought stress in waxy maize. Plants 10 (1), 48. doi: 10.3390/plants10010048
Worch, S., Rajesh, K., Harshavardhan, V. T., Pietsch, C., Sreenivasulu, N. (2011). Haplotyping, linkage mapping and expression analysis of barley genes regulated by terminal drought stress influencing seed quality. BMC Plant Biol. 11 (1), 1–14. doi: 10.1186/1471-2229-11-10
Wu, W. H., Qu, J. Z., Blennow, A., Herburger, K., Hebelstrup, K. H., Guo, K., et al. (2022). The effects of drought treatments on biosynthesis and structure of maize starches with different amylose content. Carbohyd. Polym. 297, 120045. doi: 10.1016/j.carbpol.2022.120045
Xiao, J. F., Liu, Z. D., Liu, Z. G., Nan, J. Q. (2011). Effects of drought at different growth stages and different water availabilities on growth and water consumption characteristics of summer maize. J. Maize Sci. 19 (4), 54–58, 64. doi: 10.13597/j.cnki.maize.science.2011.04.025
Yang, H., Gu, X. T., Ding, M. Q., Lu, W. P., Lu, D. L. (2019). Activities of starch synthetic enzymes and contents of endogenous hormones in waxy maize grains subjected to post-silking water deficit. Sci. Rep-Uk 9 (1), 1–8. doi: 10.1038/s41598-019-43484-0
Yao, C. X., Zhang, S. Q., Yan, X. J. (2012). Effects of drought and re-watering on photosynthetic characteristics of maize leaf. Res. Soil Water Conserv. 19 (3), 278–283.
Ye, Y. X., Lu, D. L., Wang, F. B., Chen, X. H., Qi, M. Y., He, R., et al. (2020b). Effects of exogenous trehalose on physiological characteristics in waxy maize seedlings under drought stress. J. Maize Sci. 28 (3), 80–86. doi: 10.13597/j.cnki.maize.science.20200310
Ye, Y. X., Wen, Z. R., Yang, H., Lu, W. P., Lu, D. L. (2020a). Effects of post-silking water deficit on the leaf photosynthesis and senescence of waxy maize. J. Integr. Agr. 19 (9), 2216–2228. doi: 10.1016/S2095-3119(20)63158-6
Yi, B., Zhou, Y. F., Gao, M. Y., Zhang, Z., Han, Y. (2014). Effect of drought stress during flowering stage on starch accumulation and starch synthesis enzymes in sorghum grains. J. Integr. Agr. 13 (11), 2399–2406. doi: 10.1016/S2095-3119(13)60694-2
Zhang, H., Li, H., Yuan, L., Wang, Z., Yang, J., Zhang, J. (2012). Post-anthesis alternate wetting and moderate soil drying enhances activities of key enzymes in sucrose-to-starch conversion in inferior spikelets of rice. J. Exp. Bot. 63 (1), 215–227. doi: 10.1093/jxb/err263
Zhao, Q. (2017). Selection of waxy maize materials and comprehensive evaluation (Changchun, China: Jilin Agr. Univ).
Zhou, W. X., Wang, X. P., Mu, X. Y., Li, C. H. (2013). Effects of low-light stress on Male and female flower development and pollination and fructification ability of different maize (Zea mays l.) genotypes. Acta Agron. Sin. 39 (11), 2065–2073. doi: 10.3724/SP.J.1006.2013.02065
Keywords: waxy maize, water deficit, leaf physiological characteristic, fresh ear yields, grain quality
Citation: Huang C, Qin A, Gao Y, Ma S, Liu Z, Zhao B, Ning D, Zhang K, Gong W, Sun M and Liu Z (2023) Effects of water deficit at different stages on growth and ear quality of waxy maize. Front. Plant Sci. 14:1069551. doi: 10.3389/fpls.2023.1069551
Received: 14 October 2022; Accepted: 09 January 2023;
Published: 27 January 2023.
Edited by:
Jiwang Zhang, Shandong Agricultural University, ChinaReviewed by:
Muhammad Ishaq Asif Rehmani, Ghazi University, PakistanWangfeng Zhang, Shihezi University, China
Copyright © 2023 Huang, Qin, Gao, Ma, Liu, Zhao, Ning, Zhang, Gong, Sun and Liu. This is an open-access article distributed under the terms of the Creative Commons Attribution License (CC BY). The use, distribution or reproduction in other forums is permitted, provided the original author(s) and the copyright owner(s) are credited and that the original publication in this journal is cited, in accordance with accepted academic practice. No use, distribution or reproduction is permitted which does not comply with these terms.
*Correspondence: Kai Zhang, zhangkai@henau.edu.cn; Zhandong Liu, liuzhandong@caas.cn
†These authors have contributed equally to this work