- 1Department of Sustainable Crop Production, Università Cattolica del Sacro Cuore, Piacenza, Italy
- 2HORTA S.r.l., Piacenza, Italy
Introduction: Pruning wounds are the main entry points for fungi causing grapevine trunk diseases (GTDs). Several studies identified factors influencing the temporal dynamics of wound susceptibility, which include the fungal species and inoculum dose, weather conditions, grape variety, pruning date, and so forth. Here, we conducted a quantitative analysis of literature data to synthesise outcomes across studies and to identify the factors that most affect the length of pruning wound susceptibility.
Methods: We extracted data on the frequency at which the inoculated wounds showed GTD symptoms or an inoculated pathogen was reisolated following artificial inoculation at the time of pruning or in the following days. A negative exponential model was fit to these data to describe changes in wound susceptibility as a function of time since pruning, in which the rate parameter changed depending on specific factors.
Results and Discussion: The results show that wound susceptibility is high at the time of pruning, and they remain susceptible to invasion by GTD fungi for months after pruning. Infection incidence on wounds was higher for fungi associated with Botryosphaeria dieback than those associated with Eutypa dieback or Esca complex, and wound susceptibility decreased faster for Eutypa dieback than for other GTD agents. Grapevine variety and pruning season also affected the wound susceptibility period. Sauvignon Blanc remains susceptible to GTDs longer than other varieties. We also found that the time of pruning can affect infection dynamics, especially for more susceptible varieties. The results increase our understanding of GTD epidemiology and should help growers control infections.
1 Introduction
Grapevine trunk diseases (GTDs) cause serious economic losses to the grape industry worldwide (Fontaine et al., 2016b; Mondello et al., 2018a). The casual agents include a wide range of taxonomically distant fungi (Gramaje et al., 2018; Mondello et al., 2018b) that can affect the plant alone or together. In addition to causing external symptoms on foliage and clusters, these pathogens can cause internal wood discoloration. An unpredictable discontinuity in the expression of symptoms is a characteristic of these diseases (Mugnai et al., 1999). GTDs include a range of diseases that affect adult and young vines. Esca complex, Botryosphaeria dieback, and Eutypa dieback are considered major GTDs of adult vines (Claverie et al., 2020).
The Esca complex has been associated with a number of phylogenetically diverse fungi (Mugnai et al., 1999), including both Ascomycota and Basidiomycota. Esca-associated ascomycetes include the vascular pathogens Phaeomoniella chlamydospora and Phaeoacremonium minimum (syn. Pm. aleophilum) (Úrbez-Torres et al., 2014), and other species of Phaeoacremonium. Wood-decay basidiomycetes include Fomitiporia mediterranea in Europe (Moretti et al., 2021), and other pathogens belonging to the genera Fomitiporella, Fomitiporia, Inocutis, Inonotus, Stereum, and Phellinus in non-European countries (Cloete et al., 2011; White et al., 2011); these fungi have been isolated from infected vine trunks, but their role in the disease aetiology has not been completely understood (Surico et al., 2006; Bertsch et al., 2013; Gramaje et al., 2018), and is being reconsidered in recent years.
Botryosphaeria dieback is caused by more than 20 species in the Botryosphaeriaceae family, including Botryosphaeria dothidea, Lasiodiplodia theobromae, Dothoriella viticola, Neofusicoccum parvum, N. australe, N. luteum, N. ribis, Diplodia seriata, and D. mutila (Van Niekerk et al., 2004; Taylor et al., 2005; Úrbez-Torres and Gubler, 2009; Amponsah et al., 2012b; Bertsch et al., 2013). Eutypa dieback is caused by Eutypa lata and other Diatrypaceae species (Trouillas and Gubler, 2010; Luque et al., 2012). These pathogens can be recovered from the affected wood alone or in combination with other fungi, such as Pa. chlamydospora, Pm. aleophilum, Sphaeropsis malorum, and Diaporthe ampelina (Péros et al., 1999).
GTD symptoms are multifaced and include dieback of spurs and arms, discoloration or internal streaking of wood, sectorial wood necrosis and white rot; since plants can be affected by multiple fungi at the same time, some symptoms can overlap among GTDs (Gramaje et al., 2018). Wood discoloration and decay result from a number of structural and physiological changes caused by cellulolytic and ligninolytic enzymes produced by the fungi, vascular occlusion due to gels and gums secreted by the affected xylem parenchyma cells, or necrosis of xylem parenchyma cells as the result of fungal toxins (Bertsch et al., 2013; Claverie et al., 2020). All of these changes lead to an alteration of xylem vessel functions, which results in reduced water and nutrient movement (Mugnai et al., 1999; Sparapano et al., 2000; Andolfi et al., 2011). A detailed description of symptoms in relation to the different GTDs has been recently reported by (Mondello et al., 2018b). Leaves, from which the GTD fungi have never been isolated (Bertsch et al., 2013), also show a variety of symptoms, which have been also described (Mugnai et al., 1999; Amborabé et al., 2001; Mondello et al., 2018b); wood and xylem vessel alteration, fungal toxins and deposition of secondary metabolites all contribute to the expression of disease symptoms (Tabacchi et al., 2000; Bruno et al., 2007). The epidemiology of GTDs is not fully understood. The fungi are known to spread via airborne spores (Billones-baaijens et al., 2017), splashing rain (Larignon and Dubos, 2000), or arthropods (Moyo et al., 2014; Kalvelage et al., 2021), even though details on the spread of inoculum in the vineyard are not wholly explained. Spores germinate, and germ tubes enter vine tissues through any type of wound (Graniti et al., 2000; Makatini, 2014) that exposes the xylem; fungi then colonise the wood tissue by growing in and around xylem vessels and parenchyma cells. Pruning wounds are considered a relevant entry point for GTD fungi (Gramaje et al., 2018), and protection of pruning wounds in the period of susceptibility is considered important for reducing fungal penetration into the wood (Halleen et al., 2010; Di Marco et al., 2022).
A number of studies have investigated the dynamics of pruning wound susceptibility to fungal invasion (Petzoldt et al., 1981; Munkvold and Marois, 1995; Eskalen et al., 2007; Serra et al., 2008; Amponsah, 2010; Úrbez-Torres and Gubler, 2011; Amponsah et al., 2014; Luque et al., 2014; Ayres et al., 2016; Elena and Luque, 2016). All of these studies indicate that pruning wounds are highly susceptible to fungal invasion at the time of pruning and that susceptibility declines over the following weeks. These studies were conducted for ascomycetes but not for basidiomycetes, probably because the role of the latter fungi in GTDs has been reconsidered only recently.
These studies collectively show that the wound susceptibility and its decrease over time are influenced by the fungal species, weather conditions, geographical region, grape variety, trellis system, endophytic bacterial microbiome, pruning date, the total surface area of pruning wounds, and vineyard management practices (Larignon and Dubos, 2000; Gu et al., 2005; Borgo et al., 2008; Úrbez-Torres and Gubler, 2011; Bertsch et al., 2013; Billones-Baaijens et al., 2013a; Andreini et al., 2014; Luque et al., 2014; Shafi, 2016; Lecomte et al., 2018; Martínez-Diz et al., 2020; Bekris et al., 2021; Henderson et al., 2021). Wound susceptibility is also influenced by plant response to pruning (Gubler et al., 2005). When a plant is cut or mechanically damaged, a response takes place at both cellular and tissue levels, which include biochemical changes like phenol accumulation, phytoalexin production, synthesis of hydrolytic enzymes, and cell wall reinforcement with suberin and/or lignin (Mundy and Manning, 2011); for example, high lignin production in cell walls has been reported to reduce susceptibility to E. lata (Rolshausen et al., 2008). The wound-inducible polyphenol oxidase (PPO) is also related to plant resistance and the production of the above compounds, as reviewed by Fontaine et al. (2016a). In addition to the biochemical changes, the xylem responds to wounding with gel closures in winter and tyloses in summer (Mundy and Manning, 2011). Because of this complexity, synthesizing the knowledge on the susceptibility of pruning wounds to fungal invasion is difficult.
Claverie et al. (2020) recently conducted a literature analysis and provided qualitative information on factors affecting pruning wound susceptibility to GTD fungi. To date, however, a quantitative analysis of the literature data has not been performed. Quantitative analysis of the results coming from different studies is becoming important to increase the statistical power of single studies and for summarising the available scientific knowledge on a topic (Noordzij et al., 2009). This is also true for plant pathology, where “formal” meta-analysis and other quantitative analyses of literature data are increasingly being used (Madden and Paul, 2011; Ngugi et al., 2011; Philibert et al., 2012; Scherm et al., 2014; Ji et al., 2022).
In this study, we performed a systematic literature search and extracted quantitative data from published papers with the following goals: (i) to quantitatively appraise and synthetise outcomes across studies on the dynamics of wound susceptibility to GTD fungi; (ii) to determine whether wound susceptibility depends on the pruning season, grapevine variety, the pathogen that is involved, or by the interaction of these factors; and (iii) to identify knowledge gaps that require further research.
2 Materials and methods
2.1 Systematic literature search
A systematic literature search was conducted in the Web of Science (WoS) Databases (which include the Web of Science Core Collection, BIOSIS, Derwert, KCI-Korean, Russian Science, SciELO, Data Citation index, and MEDLINE®)(Clarivate Analytics, 2020) using the following search string: Grapevine AND Trunk AND Disease$ AND Wound$ AND (susceptibility OR age). The operator AND indicates that the words must occur simultaneously in the search results, while OR indicates that the selected study must contain any of the terms separated by the operator; the dollar sign ($) represents zero or one character (e.g., “wound” or “wounds”). No restrictions on language or publication date were used. The search detected a total of 79 papers that were exported to EndNote™ online (Clarivate Analytics, online) and that were first screened based on the title and abstract. To be selected, papers had to first satisfy the following criteria: (i) the papers were published in peer-reviewed scientific journals, conference proceedings, scientific reports, or peer-reviewed theses; (ii) they describe experiments in which pruning wounds were artificially inoculated with GTD pathogens at different times after pruning; (iii) they contain quantitative information in the main text, tables, or figures on the percentage of inoculated wounds that were infected (e.g., by observing internal symptoms, or by reisolating the fungal pathogen after artificial inoculation); and (iv) the studies had an experimental design with replicates and were conducted with potted plants or field plants. The papers fulfilling these eligibility criteria were considered to be of potential interest, and the full-text manuscripts were retrieved and reviewed. Additionally, a cross reference-based search of relevant studies was performed to include reports of other studies that might have been eligible for the review and to reduce the risk of missing relevant information due to the choice of search terms. This procedure is shown in Figure 1 as a PRISMA flow diagram (Koricheva and Gurevitch, 2014).
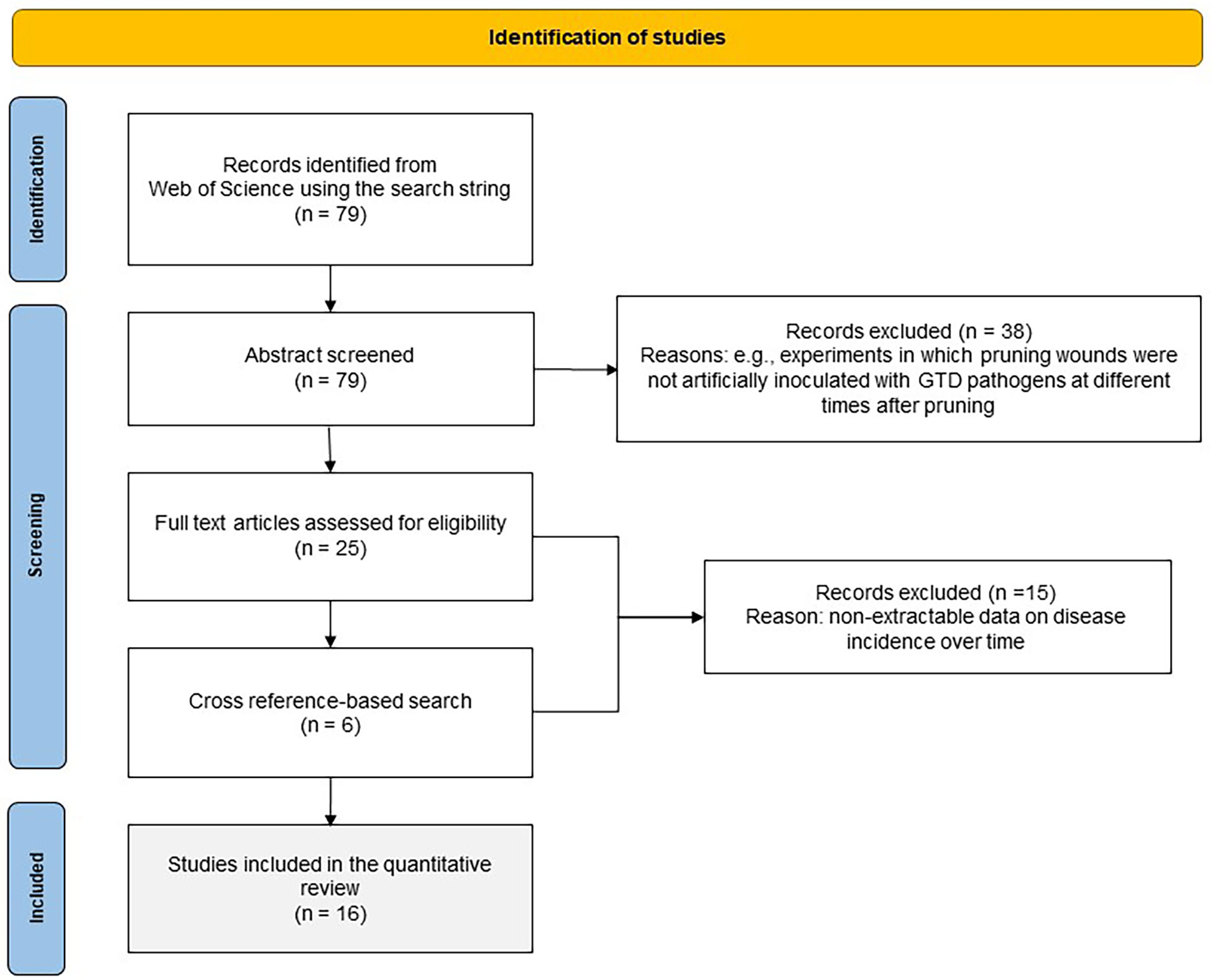
Figure 1 PRISMA flow diagram showing the procedure used for selection of studies for the quantitative analysis.
The cut-off date for publications to be considered for inclusion in this quantitative analysis was 25 July 2022.
2.2 Data extraction
Data on the percentage of pruning wounds that showed internal disease symptoms or from which the pathogen was re-isolated following artificial inoculation (hereafter referred to as “disease incidence”) at different times after pruning were extracted from the original papers. All the inoculations were done by depositing a spore suspension (concentration provided as conidia/mL, or ascospores/spores per wound) on the pruning wound surface. Additional details can be found in Supplementary Material S3.
Data in the main text or tables were extracted directly, while data in graphs were extracted with WebPlotDigitalizer version 4.3 (Rohatgi, 2020), an online tool that supports the extraction of numerical data from different types of graphs in a PNG or JPEG format. Unfortunately, it was not possible to extract data concerning the within-study variance because only five original articles contained information on the residual error component and the number of replicates (González-Domínguez et al., 2018). To retrieve missing information, we contacted the corresponding authors of the papers were contacted via email, but we received only a few replies, in which the authors declared they are not able to provide us with the original data or missing information, with only one exception.
Extracted data were organised in a database containing the selected studies (i.e., articles), cases within a study (i.e., single location or year or variety inoculated with a single pathogen), age of the pruning wounds (the time between pruning and pathogen inoculation, as defined before), and the disease incidence (as defined before). The time at which the pathogens were artificially inoculated on pruning woods was expressed as days after pruning (DAP); the day of pruning was considered as time zero (t0). For instance, in an experiment in which pruning wounds were inoculated with a pathogen immediately after pruning and then 7, 14, 21, and 28 days later, the times were referred to as t0, t7, t14, t21, and t28.
Relevant information on each case was also included in the database in order to extract subsets of data for considering the following main factors that could potentially affect the relationship between disease incidence and DAP were the pruning period (season), identity of the GTD, identity of the inoculated pathogen, and grapevine variety. The pruning period included three “categories”: early-season pruning (i.e., November for the Northern Hemisphere, no data for the Southern Hemisphere were found), mid-season pruning, (i.e., December and January for the Northern Hemisphere and June for the Southern Hemisphere), and late-season pruning (i.e., February and March for the Northern Hemisphere and July and August for the Southern Hemisphere). Identity of GTD included three categories: the Esca complex (EC, cases in which pruning wounds were inoculated with Pm. minimum or Pa. chlamydospora), Botryosphaeria dieback (BD, cases in which pruning wounds were inoculated with D. seriata, L. theobromae, N. parvum, or N. luteum), and Eutypa dieback (ED, cases in which pruning wounds were inoculated with E. lata (previously named E. armeniacae). There are eight identities for the inoculated pathogens: Pa. chlamydospora, Pm. minimum, D. seriata, L. theobromae, N. parvum, N. luteum and E. lata. Ten grapevine varieties: Cabernet Sauvignon, Chardonnay, Chenin Blanc, Merlot, Sauvignon Blanc, Thompson Seedless, Grenache, Pinot Noir, Shiraz, or Tempranillo.
2.3 Data analysis
Since it was not possible to extract data on the within-study variance of the different studies, it was impossible to fit a multivariate random effects model via linear (mixed-effects) models, and perform a formal meta-analysis. Therefore, we made a quantitative analysis of pooled data coming from the different studies considering the among-study variability only. The weaknesses of this approach are discussed in the next paragraphs.
We first used boxplots (Williamson et al., 1989) to study the effects of the main factors on the disease incidence at the time of pruning (t0). To assess the differences between the main factors at t0, we used generalised linear models (GLMs). GLMs provide regression analysis and analysis of variance for one dependent variable by one or more factors, allowing the test of the null hypotheses about the effects of other factors on the means of various groupings of a single dependent variable (Neal and Allgar, 2005). Because there was under-dispersion of data (i.e., lower variability than expected by the binomial model), a quasibinomial family distribution was used. The function glht (general linear hypotheses) was used for post hoc comparisons to compute contrasts between each combination of the factors by using Tukey’s method. The raw data used are available as Supplementary Material S1.
To study the temporal dynamics of pruning wound susceptibility, the disease incidence at t0 was set at 1, and the disease incidences at the following inoculation times were rescaled to 1 and expressed on a 0 to 1 scale. For instance, if the disease incidence was 85% for wounds inoculated at t0, and was 55%, 32%, 10%, and 3% for wounds inoculated at 7, 14, 21, and 28 DAP, then t0 = 1.00, t7 = 0.65 (i.e., 55/85), t14 = 0.38 (i.e., 32/85), t21 = 0.12 (i.e., 10/85), and t28 = 0.04 (i.e., 3/85).
Average (and standard error) of the rescaled disease incidence in the different cases was then calculated for each timing after pruning (DAP) and fit to a negative exponential equation in the following form:
where Yt is the rescaled disease incidence (dependent variable, from 0 to 1) at time t; t is the time of fungal inoculation after pruning (independent variable, in days: DAP); parameter a is the rate at which Y decreases as t increases, which was estimated for all data, main factors, and interactions (for a total of 71 estimates) by using nonlinear regression procedures (nls); standard errors of the parameter a given by the nls function were used to calculate the confidence interval of the predicted Y values.
Goodness-of-fit of equations [1] were calculated as follows: NSE (Nash-Sutcliffe efficiency), i.e., the ratio of the mean square error to the variance in the observed data, subtracted from unity (when the error is zero, NSE = 1, and the equation provides a perfect fit); W index of agreement, i.e., the ratio between mean square error to total potential error (W = 1 represents a perfect fit); root mean square error (RMSE), i.e., the fit standard error of the regression, which is calculated as the square root of the mean square error (RMSE represents the average distance of real data from the fitted line); coefficient of residual mass (CRM), which is a measure the tendency of the model to over- or underestimate the observed data (a negative CRM indicates a tendency of the model toward overestimation); R2, which represents the proportion of experimental variability explained by the selected model (R2 = 1 represents a perfect fit); and the concordance correlation coefficient (CCC), which is calculated as a measure of model accuracy (the CCC is the product of the Pearson product-moment correlation coefficient between observed and predicted values, and the coefficient Cb indicates the difference between the best-fitting line and the perfect agreement line; CCC ranges from -1 to 1, with perfect agreement at 1 (Nash and Sutcliffe, 1970; Loague and Green, 1991; Madden et al., 2007).
To determine whether the temporal dynamics of pruning wound susceptibility were significantly affected by the categories of the main factors and their two-way interactions (three-way interactions were not considered because the number of studies would have been too low), the following null hypothesis was tested: the slope a of equation [1] estimated for a category of a main factor (e.g., category EC for the main factor GTD) was not significantly different from that of another category of the same factor (e.g., EC vs. BD). The latter null hypothesis was tested as follows. Equation [1] was expressed in its linear form through data transformation through the natural logarithm function (ln), and the intercept of the linearised equation was set at ln(1 + 1) shifting the y-values by ln(2). Significant differences between slopes of the linearised, shifted functions were finally tested using the lsmeans package; slopes were extracted using the function lstrends and then compared through the function pairs, which returns a matrix consisting of t-tests for each comparison. There were 529 comparisons in total, and these are shown in Supplementary Material S2.
All statistical analyses were conducted with R studio Version 1.4.1717 (RStudio Team, 2021).
3 Results
3.1 Literature search and database overview
The literature search provided 79 studies, of which 41 were selected for full text analysis. The others were disregarded because they were not considered relevant to this study as they failed to meet the above-mentioned criteria. Two additional papers were selected via cross-referencing. After full text analysis, 16 papers were used for quantitative data extraction. The selected papers were published between 1980 and 2022, and were conducted in Australia, New Zealand, California, the USA, South Africa, Italy, Spain, and France; most were performed in the Northern Hemisphere. The key information of these 16 studies is summarised in Table 1; further information is available as Supplementary Material S3. Since each study considered multiple fungi, years, or vineyards, there were 154 cases in our dataset, for a total of 1110 single data values for disease incidence.
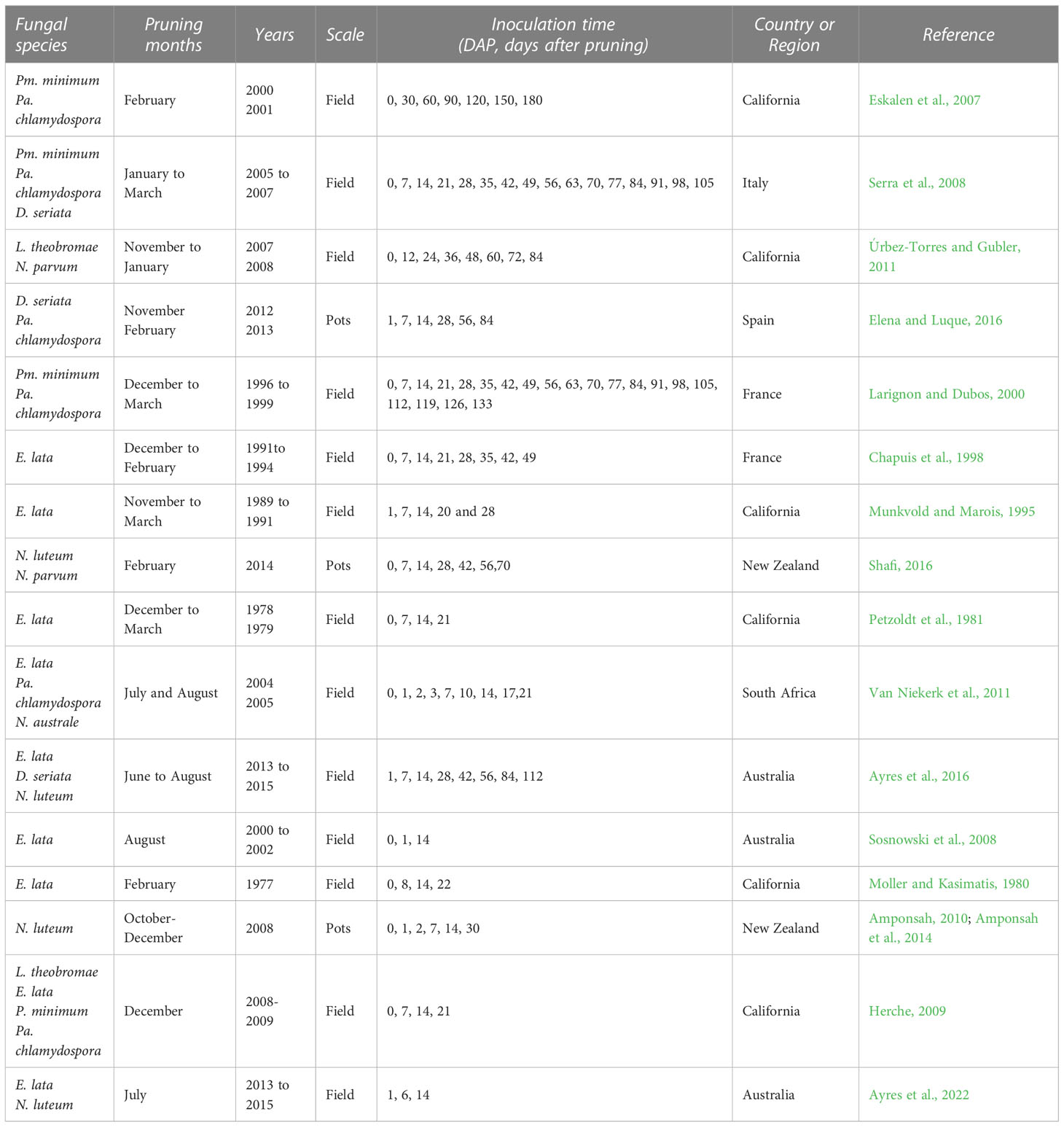
Table 1 Main characteristics of the studies selected for the analysis (further information is available in Supplementary Material – S3).
In the selected studies, wounds were inoculated at various times after pruning with a maximum of 10 months (Eskalen et al., 2007) on different grapevine varieties and in experiments conducted either in the field or with potted plants located outdoors, for 1 to 4 consecutive years. Three major GTDs were considered, including six studies for the Esca complex (in which the artificial inoculation was conducted with Pm. minimum or Pa. chlamydospora, nine for Botryosphaeria dieback (with D. seriata, L. theobromae, N. parvum, or N. luteum), and nine for Eutypa dieback (with E. lata) (Table 1). Pruning dates varied between November and March for the Northern Hemisphere, and between June and August for the Southern Hemisphere. In only two studies (Amponsah et al., 2014; Shafi, 2016), vines were pruned during the vegetative period; the data from these two studies were used in the whole dataset but not in the analysis of the pruning period.
3.2 Susceptibility of wounds at the time of pruning
In the whole dataset, the median value of disease incidence (wounds being infected or showing GTDs symptoms following artificial inoculation with GTDs fungi) at the time of pruning (t0) was 64.0%; the variability, however, was very high with extremes ranging from 0 to 100%, and with 50% of the data ranging from 40.3 to 80.0% (Figure 2). When the whole dataset was split based on the pruning period, the disease incidence for wounds inoculated at t0 was significantly higher for wounds inoculated at mid-pruning than at late pruning (P< 0.001), with early pruning showing intermediate values (Figure 2). When the whole dataset was split based on the GTD, the incidence of successful infections was significantly lower for Eutypa dieback than for Botryosphaeria dieback (P = 0.032), with intermediate values for Esca complex agents. When the whole dataset was split based on grape variety (Figure 3), disease incidence was lower for Chenin Blanc and Merlot than for other varieties; for Chenin Blanc and Merlot,< 40% of inoculated wounds became infected (Figure 3). Because of high variability among experiments and fewer data availability for Grenache, Pinot noir, Tempranillo, and Shiraz, no significant differences were found in the pairwise comparisons between these varieties (P > 0.058).
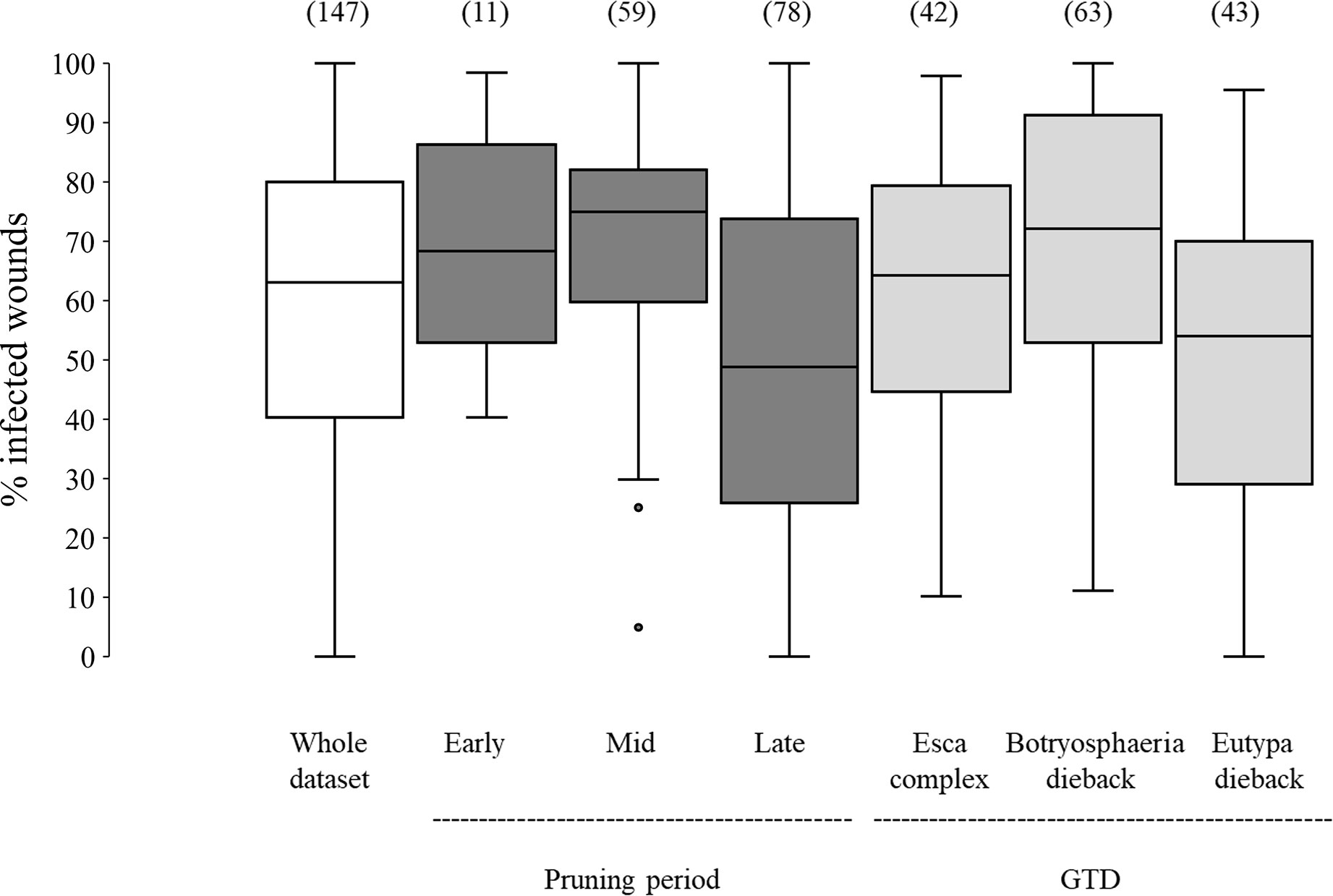
Figure 2 Susceptibility of the pruning wounds at the time of pruning (t0) (expressed as the % of the wounds that became infected after being artificially inoculated with fungi associated with grape trunk diseases [GTDs] for the whole dataset (the white box), and for subsets of data concerning pruning periods (the dark-grey boxes) and GTDs (the light-grey boxes). The values in brackets indicate the number of observations available for each subset. Whiskers indicate the lowest and highest values (excluding outliers, represented by black dots); 25% of the data fall below the lower quartile value, and 75% of the data fall below the upper quartile value. The median marks the mid-point of the data and is shown by the line that divides the box into two parts.
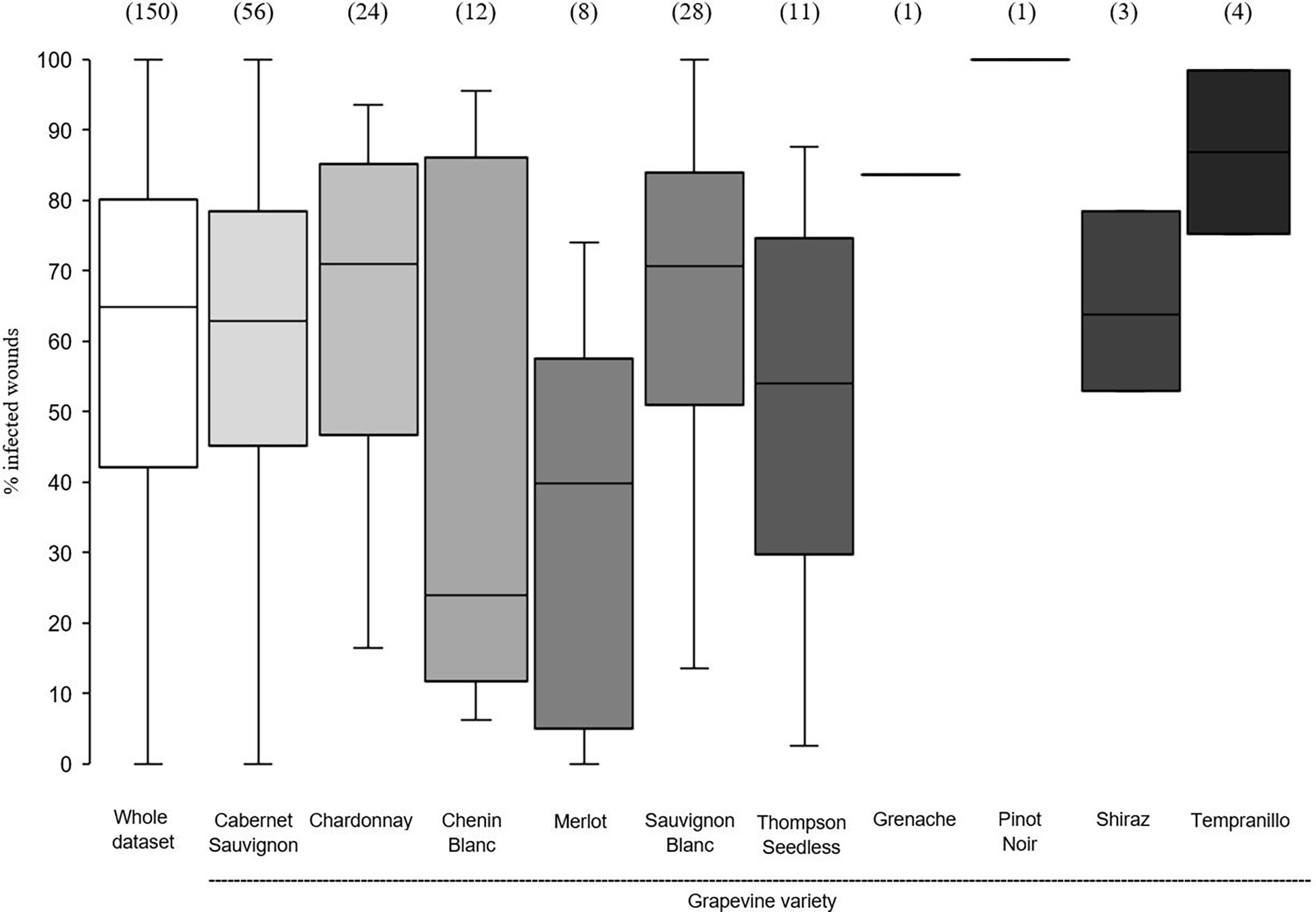
Figure 3 Susceptibility of the pruning wounds at the time of pruning (t0) (expressed as the % of the wounds that became infected after being artificially inoculated with fungi associated with grape trunk diseases [GTDs] for the whole data set (the white box) and for subsets of data concerning grapevine varieties. The values in brackets indicate the number of observations available for each subset. Whiskers indicate the lowest and highest values (excluding outliers, represented by black dots); 25% of the data fall below the lower quartile value, and 75% of the data fall below the upper quartile value. The median marks the mid-point of the data and is shown by the line that divides the box into two parts.
3.3 Changes in wound susceptibility over time
In the whole dataset, the disease incidence declined over time after pruning (DAP) when the GTD fungi were inoculated (Figure 4). Equation [1] fit the data with a = 0.019 ± 0.002, R2 = 0.74, CCC = 0.85, NSE = 0.68, W = 0.92, RMSE = 0.16, and CRM = -0.031. The analysis of data distribution with respect to the fit and its confidence interval showed that > 50% of the observed data overlapped with the predicted interval, with some overestimation between 20 and 30 DAP, and underestimation between 90 and 120 DAP (Figure 4).
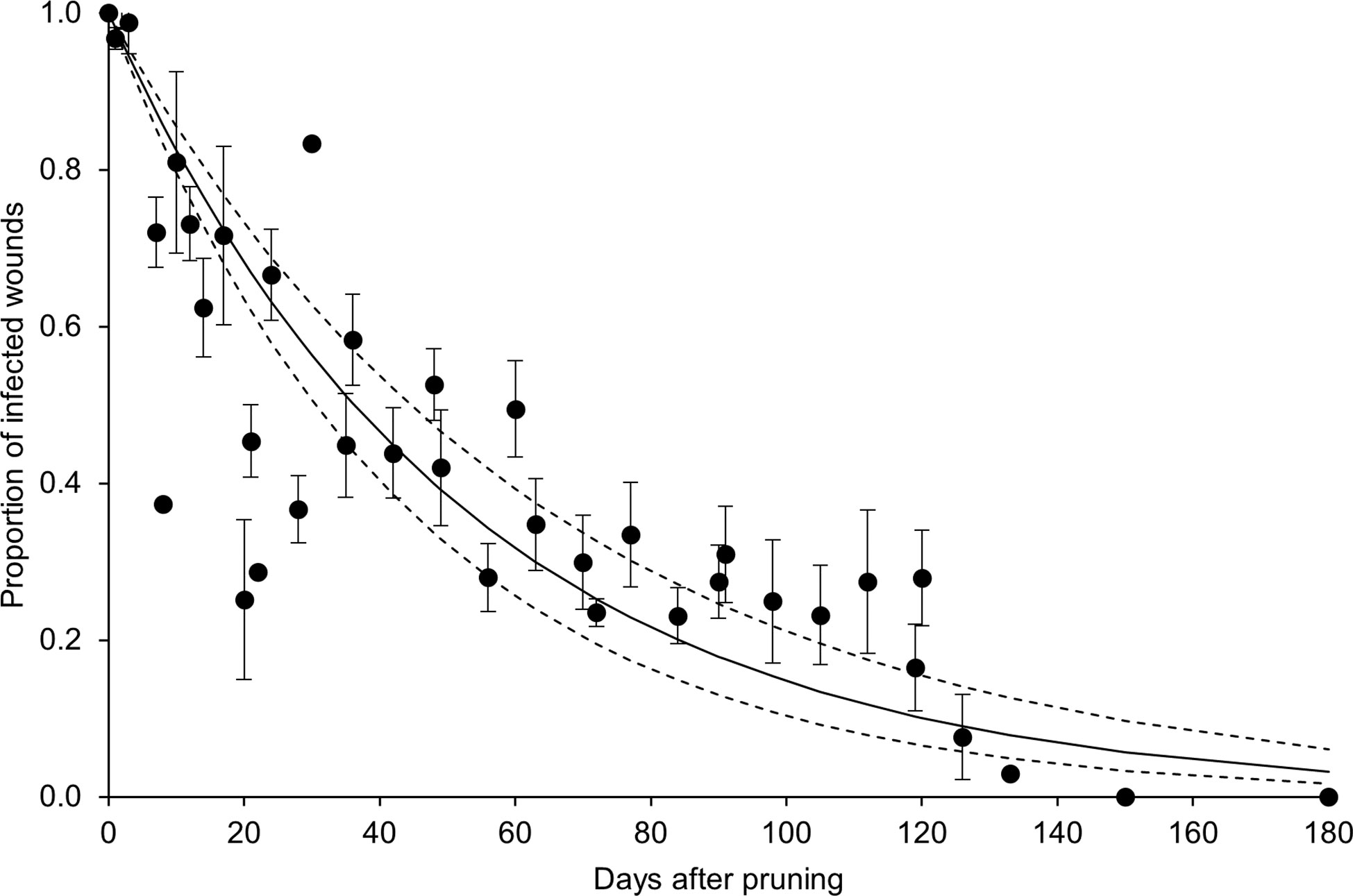
Figure 4 Proportion of pruning wounds that became infected as a function of DAP (number of days after pruning when the wounds were inoculated with fungi associated with grape trunk diseases [GTDs]) for the whole dataset shown in Table 1. Dots are averages of the observed values for each day, and whiskers represent the standard error. The full line shows the fit of the observed data with equation [1] (see text and Table 2), and the dashed lines show the upper and lower intervals of the distribution (i.e., a ± standard error).
When the whole dataset was divided according to the main factors (pruning period, GTD, grape variety, and fungal species) and their interactions, for a total of 70 combinations, equation [1] fit the observed data rather well with the following ranges of values: R2 (0.25 to 1); NSE (0.1 to 0.99); W (0.46 to 1); RMSE (0.02 to 0.72); CRM (-0.08 to 0.12); and CCC (0.3 to 1) (Tables 2–5).
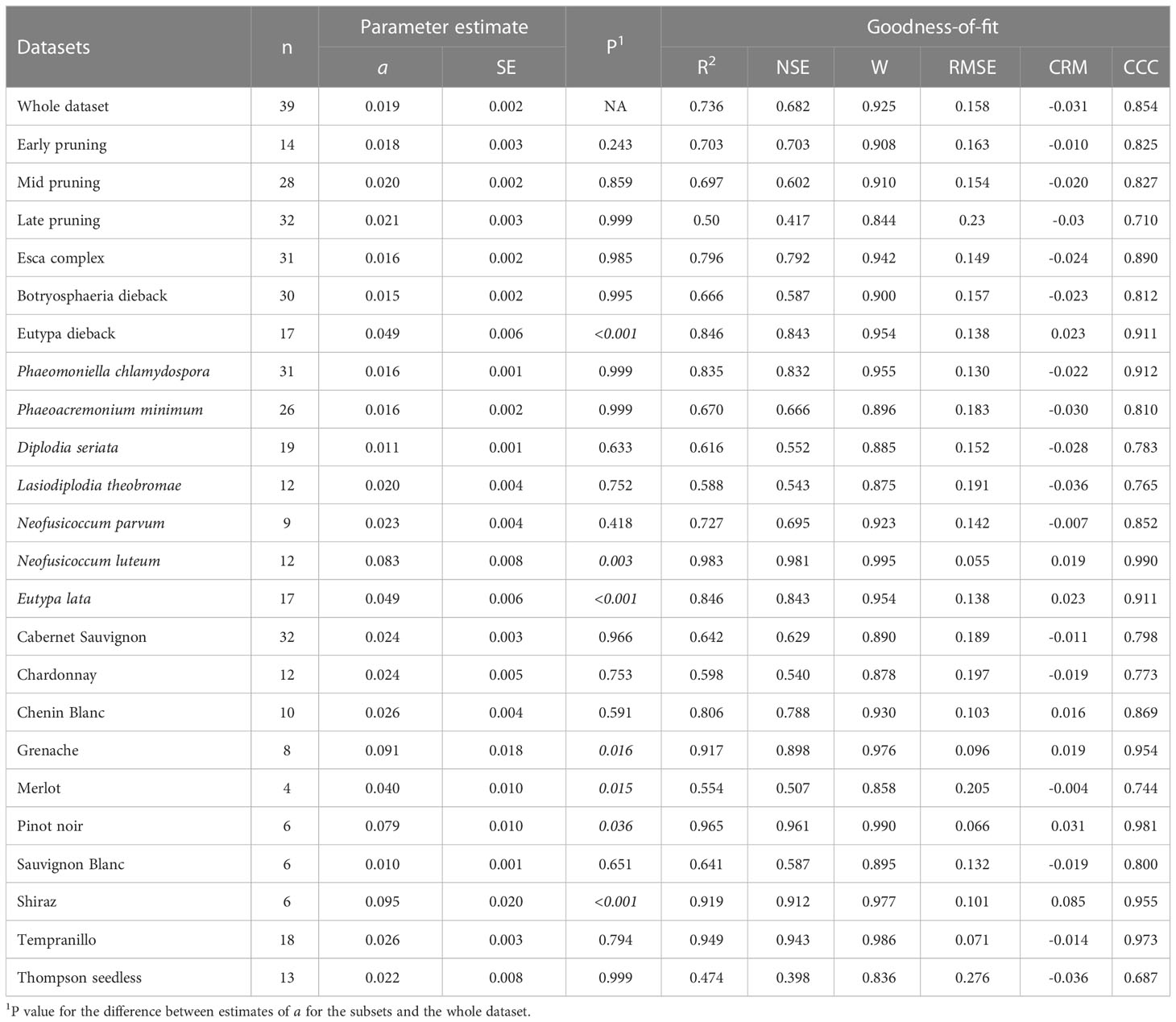
Table 2 Parameters and statistics of goodness-of-fit for equation [1] (see text), which predicts pruning wound susceptibility as a function of time after pruning when wounds were inoculated, for the whole dataset obtained from the studies in Table 1, and for subsets of data concerning different categories of the following main factors: pruning period, disease, species inoculated to wounds, and grape variety.
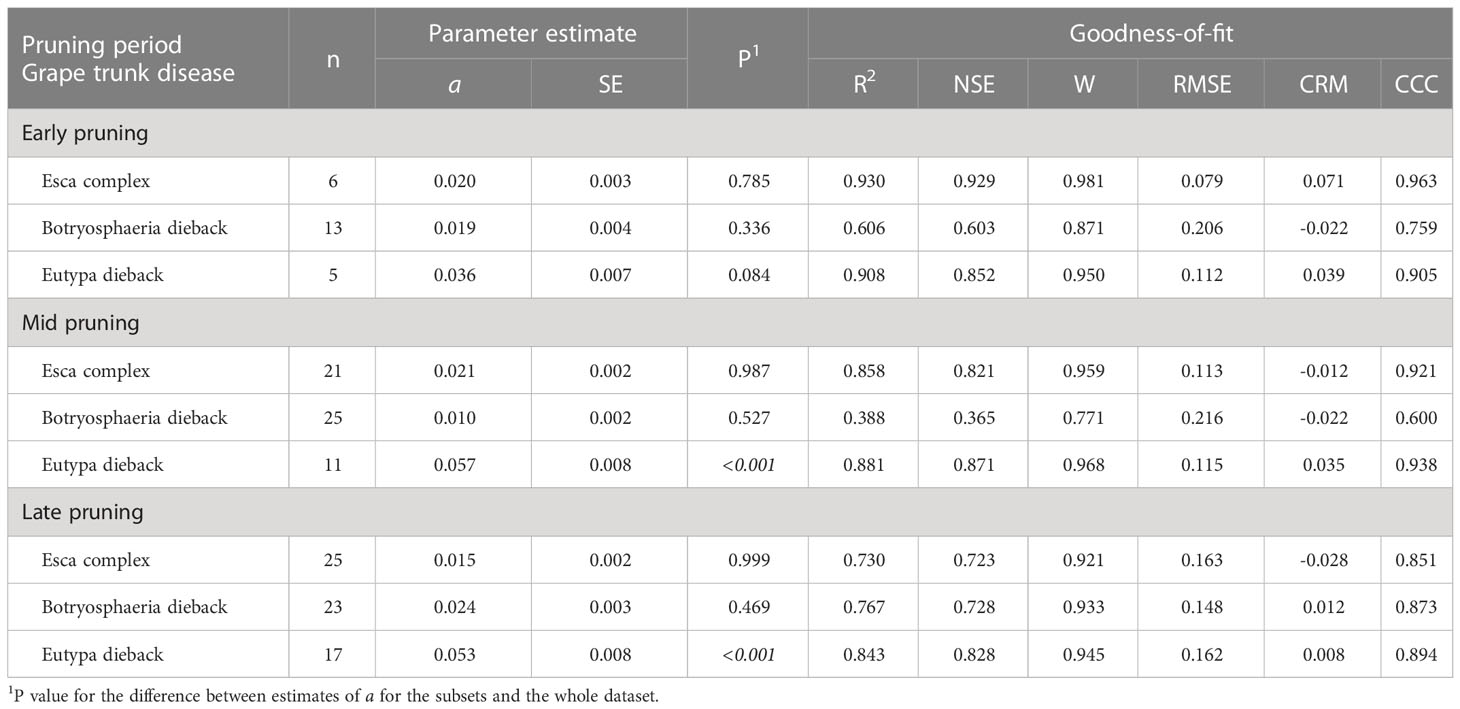
Table 3 Parameters and statistics of goodness-of-fit for equation [1] (see text), which predicts pruning wound susceptibility as a function of time after pruning when wounds were inoculated for subsets of the data in Table 1 that concern the interaction between pruning period and grape trunk disease.
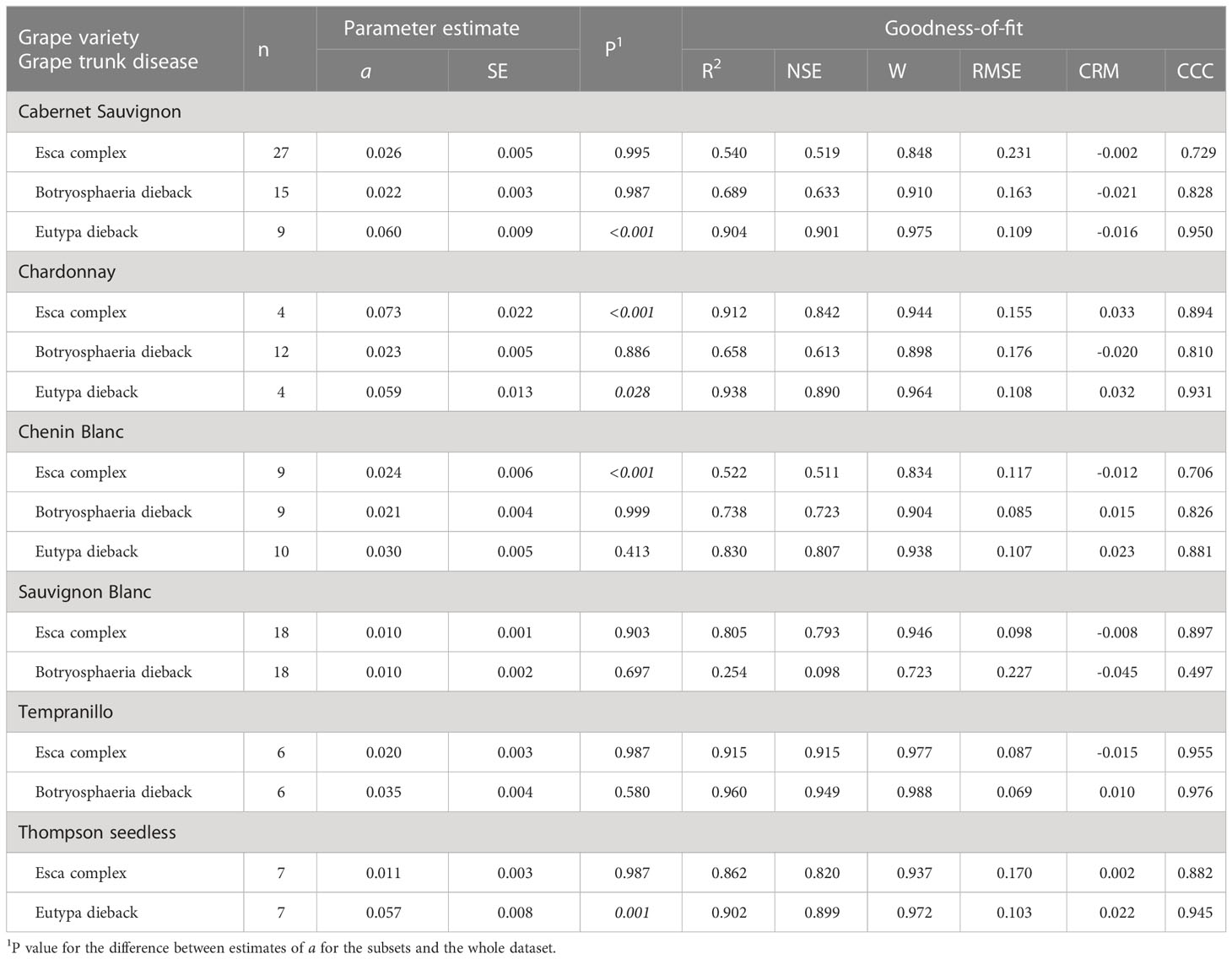
Table 4 Parameters and statistics of goodness-of-fit for equation [1] (see text), which predicts pruning wound susceptibility as a function of time after pruning when wounds were inoculated for subsets of the data that concern the interaction between grape variety and grape trunk disease.
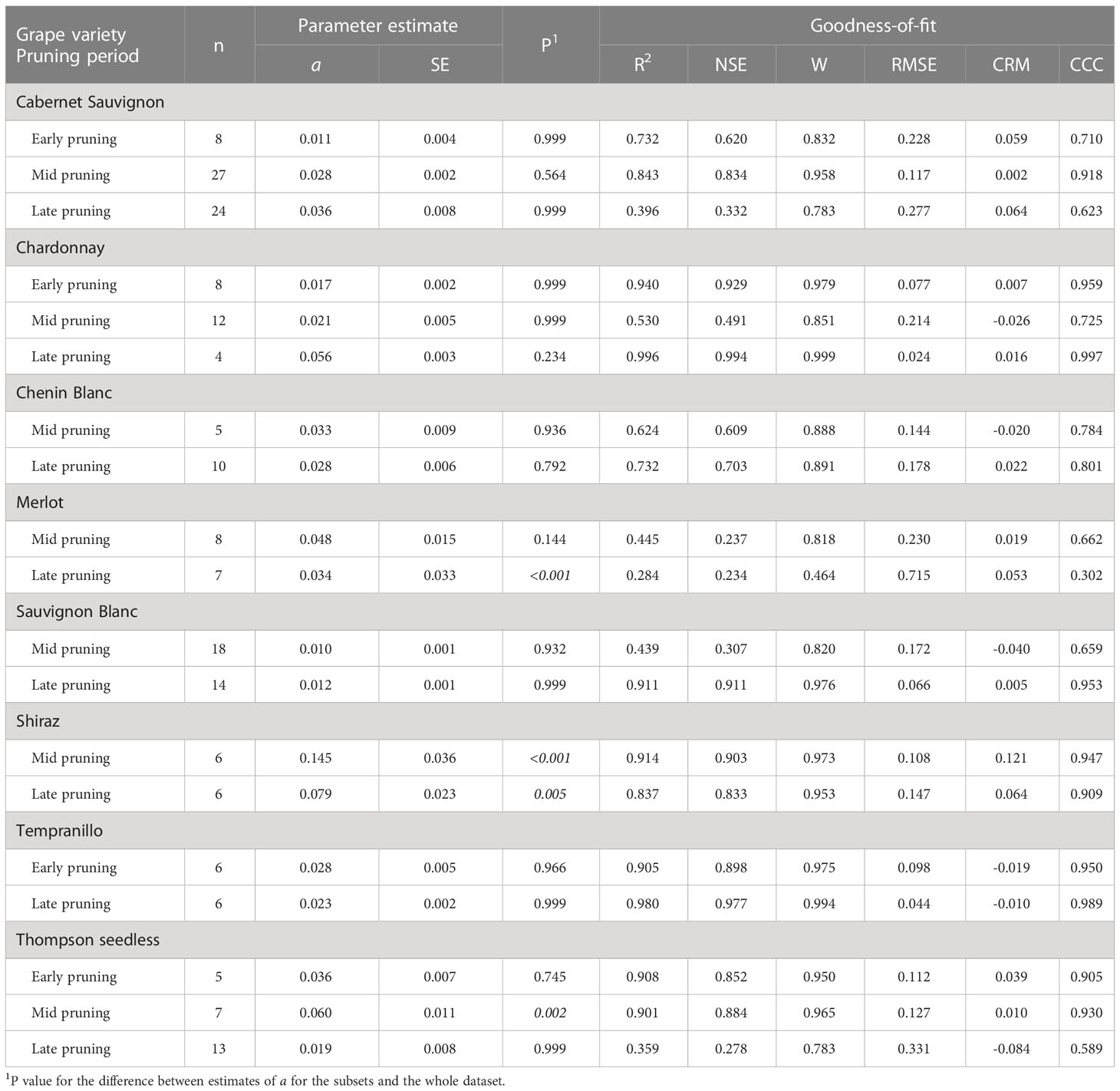
Table 5 Parameters and statistics of goodness-of-fit for equation [1] (see text), which predicts pruning wound susceptibility as a function of time after pruning when wounds were inoculated for subsets of the data that concern the interaction between grape variety and pruning period.
The pruning period (as a main factor) was not significantly related to the dynamics of wound susceptibility, because the slope parameters calculated for early (a = 0.018), mid (a = 0.020), and late (a = 0.021) pruning were not significantly different from a based on the whole dataset (P > 0.243; Table 2) or from each other, with P > 0.485 (Figure 5). Disease as a main factor, on the contrary, showed a significant effect. Wound susceptibility decreased over a shorter period after pruning for Eutypa dieback (a = 0.049) than for the Esca complex (a = 0.016) or Botryosphaeria dieback (a = 0.015), with P< 0.001 (Table 2 and Figure 6). For Botryosphaeria dieback, a significant interaction between pruning period x GTD (Table 3) showed that the susceptibility decreased faster for late pruning (a=0.024) and early pruning (a = 0.019), which were not significantly different from each other (P = 0.999), than for mid-pruning (a = 0.010; P = 0.021 and 0.029, respectively) (Supplementary Material S2).
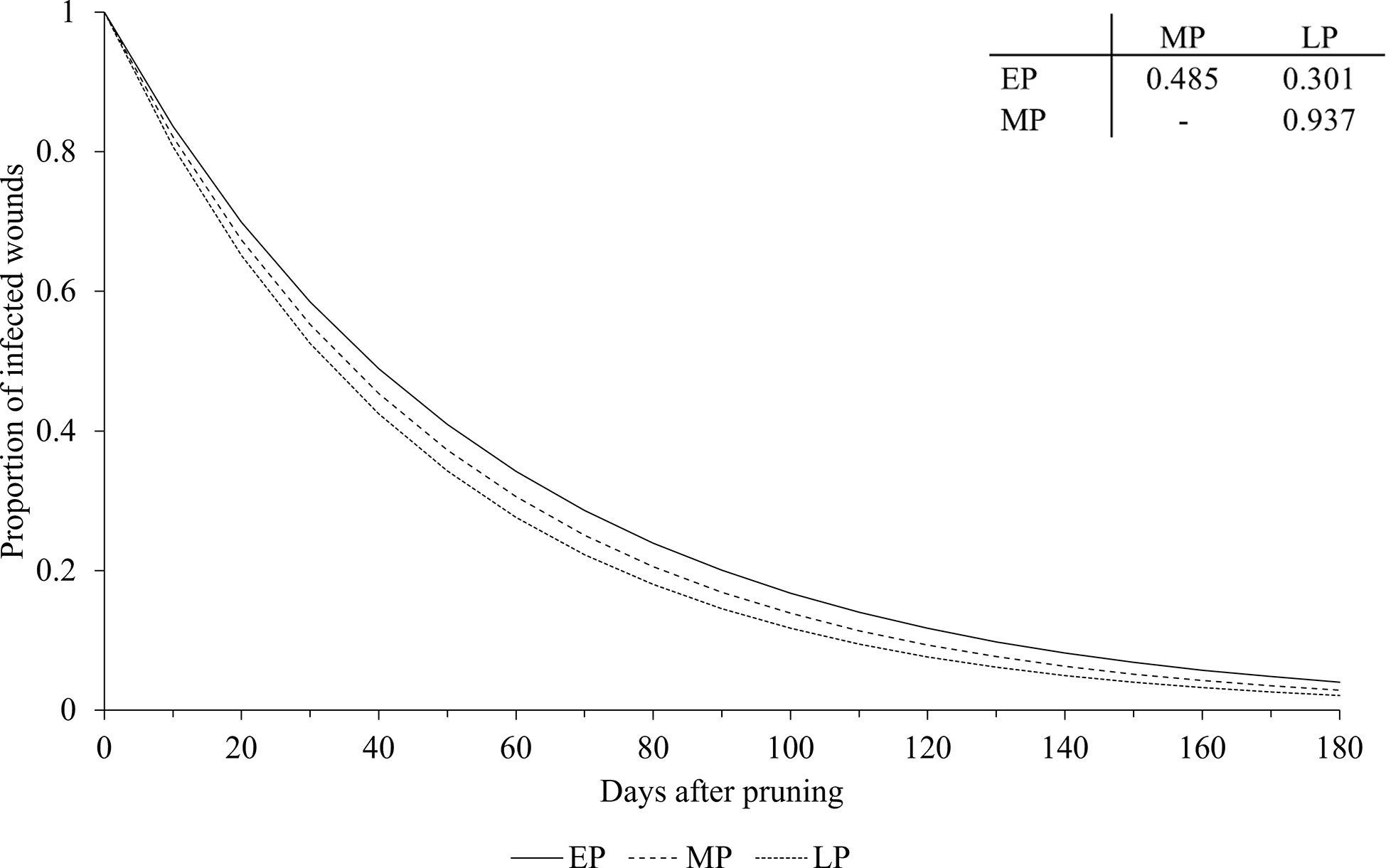
Figure 5 Effect of pruning period on the relationship between the proportion of pruning wounds that became infected (Y axis) and the time after pruning (DAP) when the wounds were artificially inoculated with GTD fungi (X axis). Early pruning period (EP - full line), mid pruning period (MP - dashed line), and late pruning period (LP - dotted line). Lines were predicted by equation [1] (see text). The table shows the P values for the differences between the estimates of parameter a in equation [1] (see Table 2 for estimates of a for pruning periods).
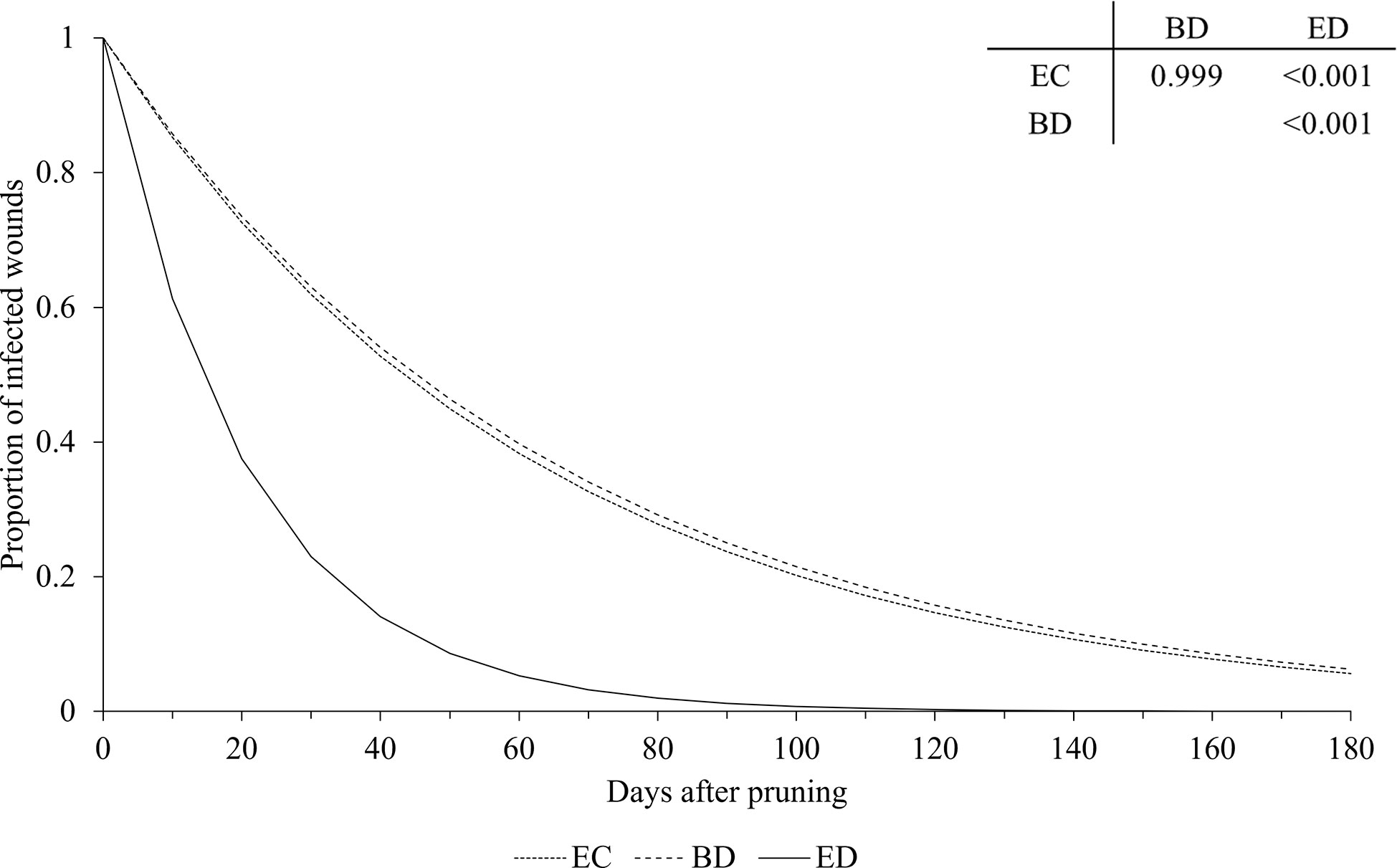
Figure 6 Effect of GTDs on the relationship between the proportion of pruning wounds that became infected (Y axis) and the time after pruning (DAP) when the wounds were artificially inoculated with GTD fungi (X axis). Esca complex (EC - dotted line), Botryosphaeria dieback (BD - dashed line), and Eutypa dieback (ED - full line). Lines were predicted by equation [1] (see text). The table shows the P values for the differences between estimates of parameter a of equation [1] (see Table 2 for estimates of a for the GTDs).
Wound susceptibility decreased faster when pruning wounds were inoculated with E. lata (a = 0.049) than with other fungal species (a ranged from a minimum of 0.011 for D. seriata to a maximum of 0.023 for N. parvum, Table 2; with P< 0.001, Supplementary Material S2), with the exception of N. luteum (a = 0.083; P = 0.019). The latter species was also different from the two main species involved in the Esca complex, Pa. chlamydospora and Pm. minimum (a = 0.016 for both species; P = 0.001 and 0.002, respectively), and from D. seriata (a = 0.011; P< 0.001), which is part of the same GTD (i.e., Botryosphaeria dieback).
Wounds were susceptible for a shorter period for Shiraz, Grenache, Pinot noir, and Merlot (in decreasing order, with a ranging from 0.095 to 0.040) than for Cabernet Sauvignon, Chardonnay, Chenin Blanc, Tempranillo, and Thompson seedless (in decreasing order, with a ranging from 0.026 to 0.022); the pairwise comparisons between varieties in the first and second of these groups were in most cases significant groups (Table 2 and Supplementary Material S2). Sauvignon Blanc had the longest period of wound susceptibility (a = 0.010), and was different from the first group of varieties but not from the second. No significant differences were found for the pruning period within each variety (P > 0.593), with the exception of Thompson Seedless, in which the susceptibility of pruning wound declined slower with late pruning (a = 0.019) than with early or mid-pruning (a = 0.036 and 0.060, respectively; P = 0.001) (Table 3 and Supplementary Material S2). Nevertheless, differences were detected between varieties within the same pruning seasons. Wounds of Sauvignon Blanc pruned in mid-season (a = 0.01) were susceptible for a longer time than wounds of Cabernet Sauvignon (a = 0.028; P = 0.02) or Thomson seedless (a = 0.06; P< 0.001) pruned in the same season. Differences among varieties were not significant with late-season pruning (Supplementary Material S2). No data were available for early-pruned Sauvignon Blanc.
Differences were also found for some varieties or pruning seasons among GTDs. For Cabernet Sauvignon, the decrease in wound susceptibility over time after pruning was faster for Eutypa dieback (a = 0.060) than for Botryosphaeria dieback or the Esca complex (a = 0.022 and 0.026, respectively; P< 0.001) (Table 4 and Supplementary Material S2). Similarly, decline in wound susceptibility for Thompson seedless was faster for Eutypa dieback (a = 0.057) than for Esca complex (a = 0.011; P< 0.001) (no data available for Botryosphaeria dieback). For Chardonnay, on the contrary, the decline in wound susceptibility was faster for the Esca complex (a = 0.073) than for Botryosphaeria dieback (a = 0.023; P = 0.003) (Supplementary Material S2). Among the GTDs, the decline in wound susceptibility was faster for Botryosphaeria dieback with late-season pruning (a = 0.024) than with mid-season pruning (a = 0.01; P = 0.002) (Table 5 and Supplementary Material S2).
When the estimates of a for main factors and interactions were compared with the estimate of a for the whole dataset (a = 0.019), significant differences were found as indicated in Tables 2–5.
4 Discussion
Since the 1970s, researchers have investigated the susceptibility of pruning wounds to GTD fungi as a function of DAP (number of days after pruning when the wounds were inoculated) and other factors. It is commonly accepted that the age of pruning wounds has a considerable effect on the susceptibility of wounds to GTD fungi, i.e., wound susceptibility decreases as the time increases between pruning and inoculation. The decline in susceptibility over time has been related to changes in environmental conditions affecting biological and physiological wound responses (Petzoldt et al., 1981; Eskalen et al., 2007; Úrbez-Torres and Gubler, 2011; Ayres et al., 2016). Many other factors affect wound susceptibility to infection, including grapevine variety, the pathogen that has been inoculated, pruning season, and other environmental conditions (Van Niekerk et al., 2011). As a consequence, the available information on the changes in wound susceptibility over time is inconsistent.
In this work, we conducted a quantitative analysis of the published data in order to synthesise the available information (Ferrer, 1998; Harrison, 2011; Ji et al., 2022). The studies analysed in this work included a wide variety of data: several grape varieties inoculated with different fungi, in different seasons, at different times after pruning, and in different countries around the world (including both the Northern and Southern Hemispheres). This variability well represents the complexity of the topic and highlights the need for synthesis. Because the studies included in our analysis involved artificial inoculation of wounds with fungi, disease incidence of wounds was probably higher than would occur with natural inoculation given that the inoculum dose is likely to be much higher with artificial than with natural inoculation (Elena and Luque, 2016). In support of this inference, pathogen recovery following artificial inoculation was lower with 10 ascospores per wound than with 100 or 1000 ascospores per wound (Elena et al., 2015; Ayres et al., 2022). The effect of inoculum dose and inoculation method used in different studies was not considered as a separate factor in this analysis because of the considerable variability among studies (see Supplementary Material S3 for information on the inoculum of each study); therefore, possible effects of inoculum dose and inoculation method on parameter a estimation was not considered.
Our quantitative analysis showed a significant influence of the considered influencing factors on the infection incidence of GTD fungi inoculated at the time of pruning and on the rate of decline in infection incidence over time. Changes in wound susceptibility to fungal invasion after pruning followed a negative exponential distribution, a probability distribution that describes the time between events in a Poisson process, i.e., a process in which events occur continuously and independently at a constant average rate. In our case, the events consisted of the number of times wounds became infected over the total number of cases that wounds were inoculated at different times after pruning (i.e., disease incidence), and the constant average rate was the equation parameter a in equation [1]. We estimated a for the whole data set and for sub-sets referring to specific influencing factors (e.g., pruning period, identity of the GTD, etc.). In equation [1], a is related to the time required for the disease incidence to halve, so that the higher the estimate of a, the shorter the time required for disease incidence to decline from 1.0 to 0.5. To our knowledge, this is the first time an equation was fit to experimental data describing the temporal changes in wound susceptibility after pruning.
Equation [1] fit the whole dataset with R2 = 0.74; based on this equation, wounds remained susceptible to invasion by GTD fungi for months after pruning, and< 50% of the wounds remained susceptible at 6 weeks after pruning (Figure 4). We retrieved only one study in which data were collected for more than 5 months after pruning (Eskalen et al., 2007); in the latter study, no infection resulted from inoculations of 5-month-old or older pruning wounds.
The fit of equation [1] showed that the different factors (i.e., GTDs, pruning periods, grape varieties), and their interactions had a significant effect on the estimates of parameter a, so that the different curves showed a different pattern in the wound susceptibility over time. Therefore, the data dispersion around the fit of equation [1] for the whole dataset (Figure 4) was determined by specific factors that our quantitative analysis was able to identify. These factors are discussed in the following sections, as well as their possible implications for the practical management of GTDs.
Some data dispersion around the fitted line, however, remained unexplained by our quantitative analysis. Indeed, other factors may influence wound infection by GTD fungi and contribute to the imperfect fit of equation [1] to the observed data, which were not considered in our study because of a lack of information in the literature; they include the age of the wood that was pruned, the size of the cut surface, the extent of wound healing, the trellis system, the rootstock, plant vigor. Because most of the studies included in our analysis were conducted in vineyards, natural colonisation of pruning wounds by GTD fungi may have occurred in the period between two artificial inoculations. It follows that the incidence of GTD in the vineyard and weather conditions that may lead to infection and expression of disease symptoms, may also be a source of variability in the data that we used (Van Niekerk et al., 2011). Further studies aimed at clarifying the effect of all the above sources of variability in the response to fungal infection through pruning wounds should be carried out under controlled environmental conditions, in which the factor of interest can be evaluated by maintaining the others constant.
4.1 Effect of the GTDs
Our analysis showed that, at the time of pruning, disease incidence of wounds was higher for the fungi associated with Botryosphaeria dieback than for those associated with Esca complex or Eutypa dieback, which generally agrees with previous reports (Rolshausen et al., 2010; Amponsah et al., 2014; Ayres et al., 2016). In addition, pruning wounds remained susceptible to infection longer when inoculated with Botryosphaeria dieback fungi or Esca fungi than with Eutypa dieback fungi, except in the case of Neofusicoccum luteum, for which wound susceptibility decreased faster than for other Botryosphaeriaceae and E. lata. A shorter susceptibility of wounds to E. lata infection than to infection by other GTDs was observed by Ayres et al. (2016) and Herche (2006), but not by Elena and Luque (2016), who observed a similar decrease in wound susceptibility for Esca and Botryosphaeria dieback. Our result overall confirmed the potential threat of Botryosphaeriaceae species once they have established in a vineyard (Rolshausen et al., 2010) as well as the increasing prevalence of these species among the GTDs (Guerin-Dubrana et al., 2019).
An important limitation of our study is that no studies were found for the basidiomycetes involved in the EC. A possible reason is that these fungi have been isolated from infected vine trunks, but often with an incomplete understanding of their role in the disease aetiology (Surico et al., 2006; Bertsch et al., 2013; Gramaje et al., 2018). With the advent of metagenomics, reports on basidiomycetes are increasing (Del Frari et al., 2019; Bruez et al., 2020) as well as the studies on their role in symptom development (Cholet et al., 2021; Moretti et al., 2021; Pacetti et al., 2021); recently Brown et al. (2020) suggested that basidiomycetes may not require infection by P. chlamydospora in order to extensively colonize the wood. Studies on the susceptibility of pruning wounds to infection by basidiomycetes are then needed.
4.2 Effect of pruning season
Reports on the effect of pruning season on the susceptibility of pruning wounds to infection by GTD fungi are inconsistent. In California, USA, for instance, Petzoldt et al. (1981) found that pruning wounds made in December remained susceptible to infection by E. lata for a longer time than pruning wounds made in March. Similarly, Úrbez-Torres and Gubler (2011) showed that wounds were susceptible to L. theobromae and N. parvum for a longer time when pruning was done in early winter (with susceptibility lasting up to 84 days) than in early spring (with susceptibility lasting up to 12 days). Furthermore, Munkvold and Marois (1995) found that the percentage of wounds infected decreased faster with time when pruning was done in January or March than in previous months. Studies conducted in Spain, in contrast, documented a faster decrease in wound susceptibility when pruning was done in autumn compared to late pruning, but only when wounds were inoculated with D. seriata (Luque et al., 2014; Elena and Luque, 2016). Some studies reported that fresh pruning wounds are susceptible to infection regardless of the pruning season (Eskalen et al., 2007), and that adjustment of pruning season is ineffective in controlling wood diseases (Serra et al., 2008). Based on the differences among findings from experiments carried out under different conditions, Van Niekerk et al. (2011) concluded that the susceptibility of pruning wounds to infection may be less related to the time of year when pruning is done than to other factors, such as the disease investigated or more specifically the fungi implicated.
In our analysis, however, infection incidence for wounds inoculated at the time of pruning was overall lower when vines were pruned later in the season, and the reduction in the infection incidence over time after pruning for fungi causing Botryosphaeria dieback was faster when pruning was done in the late season than in earlier pruning periods. Úrbez-Torres et al. (2010) found that there was less spore dispersal by the Botryosphaeriaceae in the late dormant season than in winter, and this may contribute to the result of a lower incidence of wound infection with late versus early pruning.
We, therefore, confirm that the time of pruning is relevant for reducing infection of susceptible varieties and by fungi causing Botryosphaeria dieback, with late pruning being preferred, aware that seasonal variation might occur between regions caused mainly by climatic differences (Gramaje et al., 2018). Advantages of late pruning have been associated with “vine bleeding” in early spring, which can temporarily inhibit fungal penetration into the xylem vessels (Larignon and Dubos, 2000; Serra et al., 2008; Martínez-Diz et al., 2020) and can flush away fungal spores from the wound surface (Martínez-Diz et al., 2020). In addition, the low temperatures of winter may reduce the rate at which wounded tissues heal, increasing the time that they remain susceptible to infection (Petzoldt et al., 1981; Munkvold and Marois, 1995; Rolshausen et al., 2010). In contrast to our overall results, our analysis indicated that wounds of Thompson Seedless remained susceptible for a longer time when pruned later than earlier in the season (mid-season pruning), and this deserves further study.
4.3 Effect of the grape variety
In our quantitative analysis, differences were found among grape varieties for infection incidence of wounds inoculated at pruning (t0) and after pruning. For the t0 data, however, data variability and unbalanced replications caused some under-dispersion in the GLM model (Sellers and Morris, 2017). Additional data are needed to determine whether the degree to which the probability of a pruning wound becoming infected is influenced by grape variety, and to which extent.
Our analysis showed that pruning wounds remained susceptible to GTDs for a longer time on Sauvignon Blanc than on other varieties. Sauvignon Blanc was previously reported to be especially susceptible to GTDs (Borgo et al., 2008; Bertsch et al., 2013; Murolo and Romanazzi, 2014; Sosnowski et al., 2022). Similarly, wounds of Cabernet Sauvignon remained susceptible to infection for a longer time than wounds of other red varieties, such as Grenache, Pinot noir, Merlot, or Shiraz. Differences in the susceptibility of grapevines to GTDs may be related to a variety of factors such as differences in sensitivity to fungal toxins, the rate of toxin degradation in wood tissue, and the time required for recognition of the fungal invasion and consequent activation of defense mechanisms (Wagschal et al., 2008), which involve stilbenes, other polyphenols, and proteins involved in primary metabolism (Borgo et al., 2016; Fontaine et al., 2016a; Fontaine et al., 2016b). Xylem morphology, and especially xylem vessel size may also contribute to differences in susceptibility among varieties (Pouzoulet et al., 2014; Fischer and Peighami Ashnaei, 2019; Claverie et al., 2020). It is also important to notice that a higher number of data was available for Cabernet Sauvignon than for Grenache, Pinot noir, Merlot and Shiraz so that our estimation of parameter a would be more accurate for Cabernet Sauvignon than for other varieties. Additional work is then needed to add robustness to our interpretations.
5 Limitations
Following the general objectives of systematic literature search and quantitative analysis of literature data, our work makes a synthesis of published information and projects some relevant aspects that need further research. The number of studies we found and used is limited and most of them did not include complete information on the experimental variability, thus not permitting the calculation of the effect sizes and the execution of a formal meta-analysis. Unfortunately, this limitation has been frequently faced by other authors (Koricheva and Gurevitch, 2014; Nakagawa et al., 2017). Meta-analysis is used to combine common effect sizes across studies and accounts for statistical non-independence, which occurs when data points (or effect sizes) are related to each other (for example when multiple points or effect sizes come from a single study). Failing to account for non-independence is generally considered a limitation that can lead to a loss of information or erroneous conclusions (Nakagawa et al., 2017). Even though the execution of a formal meta-analysis would lead to more robust conclusions about the susceptibility of pruning wounds to infection by GTD fungi, our manuscript represents a useful assessment of the information published so far.
Our work also suffers from the incomplete knowledge about GTDs we discussed before. Systematic literature review, however, does not have the ambition of elucidating all the aspects related to a topic, but it is useful for identifying knowledge gaps and orientating further research. Complex interactions between cultivar, biogeography, wood healing, the wood fungal pathobiome, pruning time, and environmental conditions, need further investigations.
Furthermore, our work does not claim that pruning wounds are the sole or most important infection pathway for GTD fungi. Other wood injuries caused by frost, hail, and mechanical harvesting can also act as points of entry. Soil is also considered a possible reservoir of inoculum, and roots an entry point for Phaeoacremonium spp., Pa. chlamydospore (Whiteman et al., 2002; Whiteman et al., 2005; Agustì-Brisach et al., 2013), and D. seriata (Whitelaw-Weckert et al., 2006; Whitelaw-Weckert et al., 2013), but for other Botryosphaeriaceae has not been observed (Amponsah et al., 2012a; Billones-Baaijens et al., 2013b; Billones-Baaijens and Savocchia, 2019). Soil is also a source of inoculum for black-foot pathogens (Halleen et al., 2006; Agustí-Brisach and Armengol, 2013; Agustì-Brisach et al., 2013; Agustí-Brisach et al., 2014) but as most GTD fungi utilize air-borne inoculum, its role as a reservoir of inoculum needs to be further investigated (Billones-Baaijens and Savocchia, 2019; Claverie et al., 2020).
Data availability statement
The original contributions presented in the study are included in the article/Supplementary Material. Further inquiries can be directed to the corresponding author.
Author contributions
MCR was the principal investigator. MCR and SEL extracted the data. MCR and VR conducted the statistical analysis and drafted the manuscript. VR, IS and SEL edited and revised the manuscript. All authors contributed to the article and approved the submitted version.
Funding
This work was supported by the Project “GRAPEvine TRUnk diSeases forecasTing towards a sustainable viticulture in Montenegro and Italy (GRAPETRUST)” started in 2023 and funded by the Foreign Affairs Ministry (IT) and Ministry of Science (ME) within the International collaboration framework between Italy and Montenegro.
Acknowledgments
We are grateful to Dr Giorgia Fedele, researcher at the Università Cattolica del Sacro Cuore, Dr Elisa González-Domínguez, HORTA S.r.l., and Dr Simona Giosuè, HORTA S.r.l., for their valuable suggestions regarding statistical analysis. The authors also wish to thank Dr Laura Mugnai, Professor at the University of Florence, for critically reading the manuscript and Dr Serra Salvatorica, Professor at the University of Sassari, for sharing the original data of her work. Maria Chiara Rosace conducted this study as a part of her PhD project, supported by the Doctoral School on the Agro-Food System (Agrisystem) of Università Cattolica del Sacro Cuore (Italy).
Conflict of interest
Author Sara E. Legler is employed by HORTA. S.r.l.
The remaining authors declare that the research was conducted in the absence of any commercial or financial relationships that could be construed as a potential conflict of interest.
Publisher’s note
All claims expressed in this article are solely those of the authors and do not necessarily represent those of their affiliated organizations, or those of the publisher, the editors and the reviewers. Any product that may be evaluated in this article, or claim that may be made by its manufacturer, is not guaranteed or endorsed by the publisher.
Supplementary material
The Supplementary Material for this article can be found online at: https://www.frontiersin.org/articles/10.3389/fpls.2023.1063932/full#supplementary-material
References
Agustí-Brisach, C., Armengol, J. (2013). Black-foot disease of grapevine: An update on taxonomy, epidemiology and management strategies. Phytopathol. Mediterr. 52, 245–261.
Agustì-Brisach, C., Gramaje, D., Garcìa-Jiménez, J., Armengol, J. (2013). Detection of black-foot and Petri disease pathogens in soils of grapevine nurseries and vineyards using bait plants. Plant Soil 364, 5–13. doi: 10.1007/sl
Agustí-Brisach, C., Mostert, L., Armengol, J. (2014). Detection and quantification of ilyonectria spp. associated with black-foot disease of grapevine in nursery soils using multiplex nested PCR and quantitative PCR. Plant Pathol. 63, 316–322. doi: 10.1111/ppa.12093
Amborabé, B. E., Fleurat-Lessard, P., Bonmort, J., Roustan, J. P., Roblin, G. (2001). Effects of eutypine, a toxin from eutypa lata, on plant cell plasma membrane: Possible subsequent implication in disease development. Plant Physiol. Biochem. 39, 51–58. doi: 10.1016/S0981-9428(00)01216-X
Amponsah, N. T. (2010). Epidemiology of botryosphaeriaceous species associated with grapevines in new zealand. doctor of philosophy thesis (Christchurch, New Zealand: Lincoln University).
Amponsah, N. T., Jones, E. E., Ridgway, H. J., Jaspers, M. V. (2012a). Susceptibility of grapevine tissues to neofusicoccum luteum conidial infection. Plant Pathol. 61, 719–729. doi: 10.1111/j.1365-3059.2011.02548.x
Amponsah, N. T., Jones, E., Ridgway, H. J., Jaspers, M. V. (2012b). Evaluation of fungicides for the management of botryosphaeria dieback diseases of grapevines. Pest Manage. Sci. 68, 676–683. doi: 10.1002/ps.2309
Amponsah, N. T., Jones, E. E., Ridgway, H. J., Jaspers, M. V. (2014). Factors affecting neofusicoccum luteum infection and disease progression in grapevines. Australas. Plant Pathol. 43, 547–556. doi: 10.1007/s13313-014-0294-7
Andolfi, A., Mugnai, L., Luque, J., Surico, G., Cimmino, A., Evidente, A. (2011). Phytotoxins produced by fungi associated with grapevine trunk diseases. Toxins. (Basel). 3, 1569–1605. doi: 10.3390/toxins3121569
Andreini, L., Cardelli, R., Bartolini, S., Scalabrelli, G., Viti, R. (2014). Esca symptoms appearance in vitis vinifera l.: Influence of climate, pedo-climatic conditions and rootstock/cultivar combination. Vitis - J. Grapevine. Res. 53, 33–38.
Ayres, M., Billones-Baaijens, R., Savocchia, S., Scott, E., Sosnowski, M. (2016). Susceptibility of pruning wounds to grapevine trunk disease pathogens. Wine Vitic. J. 48, 48–50.
Ayres, M. R., Billones-Baaijens, R., Savocchia, S., Scott, E. S., Sosnowski, M. R. (2022). Critical timing of fungicide application for pruning wound protection to control grapevine trunk diseases. Aust. J. Grape Wine Res. 28, 70–74. doi: 10.1111/AJGW.12517
Bekris, F., Vasileiadis, F., Papadopoulou, E., Samaras, A., Testempasis, S., Gkizi, D., et al. (2021). Grapevine wood microbiome analysis identifies key fungal pathogens and potential interactions with the bacterial community implicated in grapevine trunk disease appearance. Environ. Microbiomes. 16, 1–17. doi: 10.1186/s40793-021-00390-1
Bertsch, C., Ramírez-Suero, M., Magnin-Robert, M., Larignon, P., Chong, J., Abou-Mansour, E., et al. (2013). Grapevine trunk diseases: Complex and still poorly understood. Plant Pathol. 62, 243–265. doi: 10.1111/j.1365-3059.2012.02674.x
Billones-baaijens, B. R., Ayres, M., Savocchia, S., Sosnowski, M. (2017). Monitoring inoculum dispersal by grapevine trunk disease pathogens using spore traps. Wine Vitic. J. 46–50.
Billones-Baaijens, R., Jones, E. E., Ridgway, H. J., Jaspers, M. V. (2013a). Susceptiblity of common rootstock and scion varieties of grapevines to botryosphaeriaceae species. Australas. Plant Pathol. 43, 25–31. doi: 10.1007/s13313-013-0228-9
Billones-Baaijens, R., Ridgway, H. J., Jones, E. E., Jaspers, M. V. (2013b). Inoculum sources of botryosphaeriaceae species in new Zealand grapevine nurseries. Eur. J. Plant Pathol. 135, 159–174. doi: 10.1007/s10658-012-0075-5
Billones-Baaijens, R., Savocchia, S. (2019). A review of botryosphaeriaceae species associated with grapevine trunk diseases in Australia and new Zealand. Australas. Plant Pathol. 48, 3–18. doi: 10.1007/s13313-018-0585-5
Borgo, M., Bellotto, D., Dal Cortivo, G., Zanzotto, A. (2008). Sensibilità varietale al mal dell’esca della vite nel veneto. ATTI. Giornate. Fitopatol. 2, 223–230.
Borgo, M., Pegoraro, G., Sartori, E. (2016). Susceptibility of grape varieties to esca disease. Bio Web Conf. 7, 1041. doi: 10.1051/bioconf/20160701041
Brown, A. A., Lawrence, D. P., Baumgartner, K. (2020). Role of basidiomycete fungi in the grapevine trunk disease esca. Plant Pathol. 69, 205–220. doi: 10.1111/ppa.13116
Bruez, E., Vallance, J., Gautier, A., Laval, V., Compant, S., Maurer, W., et al. (2020). Major changes in grapevine wood microbiota are associated with the onset of esca, a devastating trunk disease. Environ. Microbiol. 22, 5189–5206. doi: 10.1111/1462-2920.15180
Bruno, G., Sparapano, L., Graniti, A. (2007). Effects of three esca-associated fungi on vitis vinifera l.: IV. diffusion through the xylem of metabolites produced by two tracheiphilous fungi in the woody tissue of grapevine leads to esca-like symptoms on leaves and berries. Physiol. Mol. Plant Pathol. 71, 106–124. doi: 10.1016/j.pmpp.2007.12.004
Chapuis, L., Richard, L., Dubos, B. (1998). Variation in susceptibility of grapevine pruning wound to infection by eutypa lata in south-western France. Plant Pathol. 47, 463–472. doi: 10.1046/j.1365-3059.1998.00258.x
Cholet, C., Bruez, É., Lecomte, P., Barsacq, A., Martignon, T., Giudici, M., et al. (2021). Plant resilience and physiological modifications induced by curettage of esca-diseased grapevines. OENO. One 55, 153–169. doi: 10.20870/OENO-ONE.2021.55.1.4478
Claverie, M., Notaro, M., Fontaine, F., Wéry, J. (2020). Current knowledge on grapevine trunk diseases with complex etiology: A systemic approach. Phytopathol. Mediterr. 59, 29–53. doi: 10.14601/Phyto-11150
Cloete, M., Fourie, P., Damm, U., Crous, P., Mostert, L. (2011). Fungi associated with die-back symptoms of apple and pear trees, a possible inoculum source of grapevine trunk disease pathogens. Phytopathol. Mediterr. 50, S176–S190.
Del Frari, G., Gobbi, A., Aggerbeck, M. R., Oliveira, H., Hansen, L. H., Ferreira, R. B. (2019). Characterization of the wood mycobiome of vitis vinifera in a vineyard affected by esca. spatial distribution of fungal communities and their putative relation with leaf symptoms. Front. Plant Sci. 10. doi: 10.3389/fpls.2019.00910
Di Marco, S., Metruccio, E. G., Moretti, S., Nocentini, M., Carella, G., Pacetti, A., et al. (2022). Activity of trichoderma asperellum strain ICC 012 and trichoderma gamsii strain ICC 080 toward diseases of esca complex and associated pathogens. Front. Microbiol. 12. doi: 10.3389/FMICB.2021.813410
Elena, G., Luque, J. (2016). Seasonal susceptibility of grapevine pruning wounds and cane colonization in catalonia, Spain following artificial infection with diplodia seriata and phaeomoniella chlamydospora. Plant Dis. 100, 1651–1659. doi: 10.1094/PDIS-10-15-1186-RE
Elena, G., Sosnowski, M. R., Ayres, M. R., Lecomte, P., Benetreau, C., Garcia-Figueres, F., et al. (2015). Effect of the inoculum dose of three grapevine trunk pathogens on the infection of artificially inoculated pruning wounds. Phytopathol. Mediterr. 54, 345–354. doi: 10.14601/Phytopathol_Mediterr-16010
Eskalen, A., Feliciano, A. J., Gubler, W. D. (2007). Susceptibility of grapevine pruning wounds and symptom development in response to infection by phaeoacremonium aleophilum and phaeomoniella chlamydospora. Plant Dis. 91, 1100–1104. doi: 10.1094/PDIS-91-9-1100
Ferrer, R. (1998). Graphical methods for detecting bias in meta-analysis. Fam. Med. - Kansas. 30, 579–583.
Fischer, M., Peighami Ashnaei, S. (2019). Grapevine, esca complex, and environment: The disease triangle. Phytopathol. Mediterr. 58, 17–37. doi: 10.13128/Phytopathol_Mediterr-25086
Fontaine, F., Gramaje, D., Armengol, J., Smart, R., Nagy, Z. A., Borgo, M., et al. (2016a). Grapevine trunk diseases. a review. Int. Organ. Vine. Wine 25, 1–24.
Fontaine, F., Pinto, C., Vallet, J., Clément, C., Gomes, A. C., Spagnolo, A. (2016b). The effects of grapevine trunk diseases (GTDs) on vine physiology. Eur. J. Plant Pathol. 144, 707–721. doi: 10.1007/s10658-015-0770-0
González-Domínguez, E., Fedele, G., Caffi, T., Delière, L., Sauris, P., Gramaje, D., et al. (2018). A network meta-analysis provides new insight into fungicide scheduling for the control of botrytis cinerea in vineyards. Pest Manage. Sci. 75, 324–332. doi: 10.1002/ps.5116
Gramaje, D., Urbez-Torres, J. R., Sosnowski, M. R. (2018). Managing grapevine trunk diseases with respect to etiology and epidemiology: Current strategies and future prospects. Plant Dis. 102, 12–39. doi: 10.1094/PDIS-04-17-0512-FE
Graniti, A., Surico, G., Mugnai, L. (2000). Esca of grapevine: A disease complex or a complex of diseases? Phytopathol. Mediterr. 39, 16–20. doi: 10.14601/Phytopathol_Mediterr-1539
Gu, S., Cochran, R. C., Du, G., Hakim, A., Fugelsang, K. C., Ledbetter, J., et al. (2005). Effect of training-pruning regimes on eutypa dieback and performance of “Cabernet sauvignon” grapevines. J. Hortic. Sci. Biotechnol. 80, 313–318. doi: 10.1080/14620316.2005.11511936
Gubler, W., Rolshausen, P., Trouillase, F., Urbez, J., Voegel, T. (2005). Grapevine trunk diseases in California. Pract. Winer. Vineyard. 1–9, 1–9.
Guerin-Dubrana, L., Fontaine, F., Mugnai, L. (2019). Grapevine trunk disease in European and Mediterranean vineyards: occurrence, distribution and associated disease-affecting cultural factors. Phytopathol. Mediterr. 58, 49–71. doi: 10.13128/Phytopathol_Mediterr-25153
Halleen, F., Fourie, P. H., Crous, P. W. (2006). A review of black foot disease of grapevine. Phytopathol. Mediterr. 45, 55–67.
Halleen, F., Fourie, P. H., Lombard, P. J. (2010). Protection of grapevine pruning wounds against eutypa lata by biological and chemical methods. South Afr. J. Enol. Vitic. 31, 125–132. doi: 10.21548/31-2-1409
Harrison, F. (2011). Getting started with meta-analysis. Methods Ecol. Evol. 2, 1–10. doi: 10.1111/j.2041-210X.2010.00056.x
Henderson, B., Sosnowski, M. R., McCarthy, M. G., Scott, E. S. (2021). Incidence and severity of eutypa dieback in grapevines are related to total surface area of pruning wounds. Aust. J. Grape Wine Res. 27, 87–93. doi: 10.1111/AJGW.12465
Herche, R. (2006). Control strategies for trunk diseases of grapevine (Vitis vinifera L.). Thesis. (California: University of the Pacific).
Herche, R. (2009). Control strategies for Trunk Diseases of Grapevine (Vitis vinifera L.). Thesis. (California: University of the Pacific).
Ji, D. T., Salotti, M. I., Altieri, M. V., Li, P. M., Rossi, P. V. (2022). Temperature-dependent growth and spore germination of fungi causing grapevine trunk diseases: quantitative analysis of literature data. Plant Dis. doi: 10.1094/PDIS-09-22-2249-RE
Kalvelage, E. M., Voegele, R. T., Fischer, M. (2021). Dissemination of esca-related pathogens in German vineyards: do arthropods play roles in vectoring spores? Phytopathol. Mediterr. 60, 467–478. doi: 10.36253/phyto-12948
Koricheva, J., Gurevitch, J. (2014). Uses and misuses of meta-analysis in plant ecology. J. Ecol. 102, 828–844. doi: 10.1111/1365-2745.12224
Larignon, P., Dubos, B. (2000). Preliminary studies on the biology of phaeoacremonium. Phytopathol. Mediterr. 39, 184–189.
Lecomte, P., Diarra, B., Carbonneau, A., Rey, P., Chevrier, C. (2018). Field suppression of fusarium wilt disease in banana by the combined application of native endophytic and rhizospheric bacterial isolates possessing multiple functions. Phytopathol. Mediterr. 57, 472–487. doi: 10.14601/Phytopathol_Mediterr-22025
Loague, K., Green, R. E. (1991). Statistical and graphical methods for evaluating solute transport models: Overview and application. J. Contam. Hydrol. 7, 51–73. doi: 10.1016/0169-7722(91)90038-3
Luque, J., Elena, G., Garcia-Figueres, F., Reyes, J., Barrios, G., Legorburu, F. J. (2014). Natural infections of pruning wounds by fungal trunk pathogens in mature grapevines in Catalonia (Northeast Spain). Aust. J. Grape Wine Res. 20, 134–143. doi: 10.1111/ajgw.12046
Luque, J., Garcia-Figueres, F., Legorburu, F. J., Muruamendiaraz, A., Armengol, J., Trouillas, F. P. (2012). Species of diatrypaceae associated with grapevine trunk diseases in Eastern Spain. Phytopathol. Mediterr. 51, 528–540. doi: 10.14601/Phytopathol_Mediterr-9953
Madden, L. V., Hughes, G., van den Bosch, F. (2007). The study of plant disease epidemics (St. Paul, Minnesota: The American Phytopathological Society). doi: 10.1094/9780890545058
Madden, L. V., Paul, P. A. (2011). Meta-analysis for evidence synthesis in plant disease epidemiology and management meta-analysis for evidence synthesis in plant pathology: An overview e-xtra *. Phytopathology 101, 16–30. doi: 10.1094/PHYTO-03-10-0069
Makatini, G. J. (2014). The role of sucker wounds as portals for grapevine trunk pathogen infections Thesis. (South Africa: University of Stellenbosch).
Martínez-Diz, M., Eichmeier, A., Spetik, M., Bujanda, R., Díaz-Fernández, Á., Díaz-Losada, E., et al. (2020). Grapevine pruning time affects natural wound colonization by wood-invading fungi. Fungal Ecol. 48. doi: 10.1016/j.funeco.2020.100994
Moller, WJ, Kasimatis, A N. (1980). Protection of grapevine pruning wounds from Eutypa dieback. Plant Dis. 64 (3), 278–280.
Mondello, V., Larignon, P., Armengol, J., Kortekamp, A., Vaczy, K., Prezman, F., et al. (2018a). Management of grapevine trunk diseases: knowledge transfer, current strategies and innovative strategies adopted in Europe. Phytopathol. Mediterr. 57, 369–383. doi: 10.14601/Phytopathol_Mediterr-23942
Mondello, V., Songy, A., Battiston, E., Pinto, C., Coppin, C., Trotel-Aziz, P., et al. (2018b). Grapevine trunk diseases: A review of fifteen years of trials for their control with chemicals and biocontrol agents. Plant Dis. 102, 1189–1217. doi: 10.1094/PDIS-08-17-1181-FE
Moretti, S., Pacetti, A., Pierron, R., Kassemeyer, H.-H., Fischer, M., Péros, J.-P., et al. (2021). Fomitiporia mediterranea m. fisch., the historical esca agent: A comprehensive review on the main grapevine wood rot agent in Europe. Phytopathol. Mediterr. 60, 351–379. doi: 10.36253/phyto-13021
Moyo, P., Allsopp, E., Roets, F., Mostert, L., Halleen, F. (2014). Arthropods vector grapevine trunk disease pathogens. Phytopathology 104, 1063–1069. doi: 10.1094/PHYTO-11-13-0303-R
Mugnai, L., Graniti, A., Surico, G. (1999). Esca (Black measles) and brown wood-streaking: Two old and elusive diseases of grapevines. Plant Dis. 83, 404–418. doi: 10.1094/PDIS.1999.83.5.404
Mundy, D. C., Manning, M. A. (2011). Physiological response of grapevines to vascular pathogens: A review. New Zeal. Plant Prot. 64, 7–16. doi: 10.30843/nzpp.2011.64.5974
Munkvold, G. P., Marois, J. J. (1995). Factors associated with variation in susceptibility of grapevine pruning wounds to infection by eutypa lata. Ecol. Epidemiol. 85, 249–256.
Murolo, S., Romanazzi, G. (2014). Effects of grapevine cultivar, rootstock and clone on esca disease. Australas. Plant Pathol. 43, 215–221. doi: 10.1007/s13313-014-0276-9
Nakagawa, S., Noble, D. W. A., Senior, A. M., Lagisz, M. (2017). Meta-evaluation of meta-analysis: Ten appraisal questions for biologists. BMC Biol. 15, 1–14. doi: 10.1186/s12915-017-0357-7
Nash, J. E., Sutcliffe, J. V. (1970). River flow forecasting through conceptual models - part I - a discussion of principles. J. Hydrol. 10, 282–290. doi: 10.1016/0022-1694(70)90255-6
Neal, R. D., Allgar, V. L. (2005). Sociodemographic factors and delays in the diagnosis of six cancers: Analysis of data from the “National survey of NHS patients: Cancer”. Br. J. Cancer 92, 1971–1975. doi: 10.1038/sj.bjc.6602623
Ngugi, H. K., Esker, P. D., Scherm, H., Ngugi, H. K. (2011). Meta-analysis for evidence synthesis in plant disease epidemiology and management meta-analysis to determine the effects of plant disease management measures: Review and case studies on soybean and apple. Phytopathol. Symp. 101, 31. doi: 10.1094/PHYTO-03-10-0068
Noordzij, M., Hooft, L., Dekker, F. W., Zoccali, C., Jager, K. J. (2009). Systematic reviews and meta-analyses: When they are useful and when to be careful. Kidney Int. 76, 1130–1136. doi: 10.1038/ki.2009.339
Pacetti, A., Moretti, S., Pinto, C., Compant, S., Farine, S., Bertsch, C., et al. (2021). Trunk surgery as a tool to reduce foliar symptoms in diseases of the esca complex and its influence on vine wood microbiota. J. Fungi. 7, 521. doi: 10.3390/JOF7070521
Péros, J. P., Jamaux-Despréaux, I., Berger, G., Gerba, D. (1999). The potential importance of diversity in eutypa lata and co-colonising fungi in explaining variation in development of grapevine dieback. Mycol. Res. 103, 1385–1390. doi: 10.1017/S0953756299008291
Petzoldt, C. H., Moller, W. J., Sall, M. A. (1981). Eutypa dieback of grapevine: Seasonal differences in infection and duration of susceptibility of pruning wounds. Phytopathology 71, 540. doi: 10.1094/phyto-71-540
Philibert, A., Loyce, C., Makowski, D. (2012). Assessment of the quality of meta-analysis in agronomy. Agric. Ecosyst. Environ. 148, 72–82. doi: 10.1016/J.AGEE.2011.12.003
Pouzoulet, J., Pivovaroff, A. L., Santiago, L. S., Rolshausen, P. E. (2014). Can vessel dimension explain tolerance toward fungal vascular wilt diseases in woody plants? lessons from dutch elm disease and esca disease in grapevine. Front. Plant Sci. 5. doi: 10.3389/fpls.2014.00253
Rohatgi, A. (2020) WebPlotDigitizer - web based tool to extract data from plots, images, and maps (Accessed November 25, 2020).
Rolshausen, P. E., Greve, L. C., Labavitch, J. M., Mahoney, N. E., Molyneux, R. J., Gubler, W. D. (2008). Pathogenesis of eutypa lata in grapevine: Identification of virulence factors and biochemical characterization of cordon dieback. Phytopathology 98, 222–229. doi: 10.1094/PHYTO-98-2-0222
Rolshausen, P. E., Úrbez-Torres, J. R., Rooney-Latham, S., Eskalen, A., Smith, R. J., Gubler, W. D. (2010). Evaluation of pruning wound susceptibility and protection against fungi associated with grapevine trunk diseases. Am. J. Enol. Vitic. 61, 113–119. doi: 10.5344/ajev.2010.61.1.113
RStudio Team (2021). RStudio: Integrated development environment for r. Boston, MA: PBC. Available at: http://www.rstudio.com/.
Scherm, H., Thomas, C. S., Garrett, K. A., Olsen, J. M. (2014). Meta-analysis and other approaches for synthesizing structured and unstructured data in plant pathology. Annu Rev Phytopathol. 52, 453–476. doi: 10.1146/ANNUREV-PHYTO-102313-050214
Sellers, K. F., Morris, D. S. (2017). Underdispersion models: Models that are “under the radar” underdispersion models: Models that are “under the radar”. Commun. Stat. Methods 46, 12075–12086. doi: 10.1080/03610926.2017.1291976
Serra, S., Mannoni, M. A., Ligios, V. (2008). Studies on the susceptibility of pruning wounds to infection by fungi involved in grapevine wood diseases in Italy. Phytopathol. Mediterr. 47, 234–246. doi: 10.14601/Phytopathol_Mediterr-2727
Shafi, A. (2016). Botryosphaeria dieback in vineyards: conidial dispersal, infection, disease development and control (New Zealand: Doctor of Philosophy Thesis, Lincoln University, Christchurch).
Sosnowski, MR, Creaser, ML, Wicks, T J, Lardner, R, Scott, ES (2008). Protection of grapevine pruning wounds from infection by Eutypa lata. Aust. J. Grape Wine Res. 14 (2), 134–142.
Sosnowski, M. R., Ayres, M. R., McCarthy, M. G., Scott, E. S. (2022). Winegrape cultivars (Vitis vinifera) vary in susceptibility to the grapevine trunk pathogens eutypa lata and diplodia seriata. Aust. J. Grape Wine Res. 14, 134–142. doi: 10.1111/AJGW.12531
Sparapano, L., Bruno, G., Graniti, A. (2000). Effects on plants of metabolites produced in culture by phaeoacremonium chlamydosporum, p. aleophilum and fomitiporia punctata. Phytopathol. Mediterr. 39, 169–177. doi: 10.1400/57840
Surico, G., Mugnai, L., Marchi, G. (2006). Older and more recent observations on esca: A critical overview. Phytopathol. Mediterr. 45, 68–86. doi: 10.14601/Phytopathol_Mediterr-1847
Tabacchi, R., Fkyera, A., Poliart, C., Dubin, G.-M. (2000). Phytotoxins from fungi of esca of grapevine. Phytopathol. Mediterr. 39, 156–161.
Taylor, A., Hardy, G. E. S. J., Wood, P., Burgess, T. (2005). Identification and pathogenicity of botryosphaeria species associated with grapevine decline in Western Australia. Australas. Plant Pathol. 34, 187–195. doi: 10.1071/AP05018
Trouillas, F. P., Gubler, W. D. (2010). Pathogenicity of diatrypaceae species in grapevines in California. Plant Dis. 94, 867–872. doi: 10.1094/PDIS-94-7-0867
Úrbez-Torres, JR, Battani, M, Bettiga, LJ, Gispert, C, Mc Gourty, G, Roncoroni, J, Smith, RJ, Verdegaal, P, Gubler, WD, et al (2010). Botryosphaeriaceae species spore-trapping studies in California vineyards. Plant Disease 94, 6, 717–724. doi: 10.1094/PDIS-94-6-0717
Úrbez-Torres, J. R., Gubler, W. D. (2009). Pathogenicity of botryosphaeriaceae species isolated from grapevine cankers in California. Plant Dis. 93, 584–592. doi: 10.1094/PDIS-93-6-0584
Úrbez-Torres, JR, Battani, M, Bettiga, LJ, Gispert, C, Mc Gourty, G, Roncoroni, J, et al (2009). Botryosphaeriaceae species spore-trapping studies in California vineyards. Plant Dis. 94 (6), 717–724. doi: 10.1094/PDIS-94-6-0717
Úrbez-Torres, J. R., Gubler, W. D. (2011). Susceptibility of grapevine pruning wounds to infection by lasiodiplodia theobromae and neofusicoccum parvum. Plant Pathol. 60, 261–270. doi: 10.1111/j.1365-3059.2010.02381.x
Úrbez-Torres, J. R., Haag, P., Bowen, P., O’Gorman, D. T. (2014). Grapevine trunk diseases in British Columbia: Incidence and characterization of the fungal pathogens associated with black foot disease of grapevine. Plant Dis. 98, 456–468. doi: 10.1094/PDIS-05-13-0524-RE
Van Niekerk, J. M., Crous, P. W., Groenewald, J. Z., Fourie, P. H., Halleen, F. (2004). DNA Phylogeny, morphology and pathogenicity of botryosphaeria species on grapevines. Mycologia 96, 781–798. doi: 10.1080/15572536.2005.11832926
Van Niekerk, J. M., Halleen, F., Fourie, P. H. (2011). Temporal susceptibility of grapevine pruning wounds to trunk pathogen infection in south African grapevines. Phytopathol. Mediterr. 50, S139–S150. doi: 10.14601/Phytopathol_Mediterr-8575
Wagschal, I., Abou-Mansour, E., Petit, A., Clement, C., Fontaine, F. (2008)Wood diseases of grapevine: A review on eutypa dieback and esca. In: Plant-microbe interactions (Kerala, India: Research Signpost) (Accessed December 21, 2020).
White, C. L., Halleen, F., Fischer, M., Mostert, L. (2011). Characterisation of the fungi associated with esca diseased grapevines in south Africa. Phytopathol. Mediterr. 50, 204–223. doi: 10.14601/Phytopathol_Mediterr-8983
Whitelaw-Weckert, M. A., Rahman, L., Appleby, L. M., Hall, A., Clark, A. C., Waite, H., et al. (2013). Co-Infection by botryosphaeriaceae and ilyonectria spp. fungi during propagation causes decline of young grafted grapevines. Plant Pathol. 62, 1226–1237. doi: 10.1111/ppa.12059
Whitelaw-Weckert, M. A., Sergeeva, V., Priest, M. J. (2006). Botryosphaeria stevensii infection of pinot noir grapevines by soil-root transmission. Australas. Plant Pathol. 35, 369–371. doi: 10.1071/AP06034
Whiteman, S. A., Jaspers, M. V., Stewart, A., Ridgway, H. J. (2002). Detection of phaeomoniella chlamydospora in soil using speciesspecific PCR. Hortic. Pathol. 55, 139–145. doi: 10.30843/nzpp.2002.55.3943
Whiteman, S., Jaspers, M., Stewart, A., Ridgway, H. (2005). “Soil as a source of phaeomoniella chlamydospora (causal agent of Petri disease),” in Abstracts of the 4th international workshop on grapevine trunk diseases, vol. 78. (Stellenbosch, South Africa: University of Stellenbosch).
Keywords: artificial inoculation, Botryosphaeria dieback, Esca complex, Eutypa dieback, fungal pathogens
Citation: Rosace MC, Legler SE, Salotti I and Rossi V (2023) Susceptibility of pruning wounds to grapevine trunk diseases: A quantitative analysis of literature data. Front. Plant Sci. 14:1063932. doi: 10.3389/fpls.2023.1063932
Received: 07 October 2022; Accepted: 01 February 2023;
Published: 23 February 2023.
Edited by:
Christopher Michael Wallis, United States Department of Agriculture, United StatesReviewed by:
K. W. Thilini Chethana, Mae Fah Luang University, ThailandErin Galarneau, Agricultural Research Service (USDA), United States
Copyright © 2023 Rosace, Legler, Salotti and Rossi. This is an open-access article distributed under the terms of the Creative Commons Attribution License (CC BY). The use, distribution or reproduction in other forums is permitted, provided the original author(s) and the copyright owner(s) are credited and that the original publication in this journal is cited, in accordance with accepted academic practice. No use, distribution or reproduction is permitted which does not comply with these terms.
*Correspondence: Maria Chiara Rosace, bWFyaWFjaGlhcmEucm9zYWNlQHVuaWNhdHQuaXQ=