- 1Integrated Molecular Plant Physiology Research, Department of Biology, University of Antwerp, Antwerp, Belgium
- 2Botany and Microbiology Department, Faculty of Science, Beni-Suef University, Beni-Suef, Egypt
- 3Department of Biology, College of Science, Princess Nourah bint Abdulrahman University, Riyadh, Saudi Arabia
- 4Ecosystem Management, Department of Biology, University of Antwerp, Antwerp, Wilrijk, Belgium
- 5Department of Botany and Microbiology, Faculty of Science, Cairo University, Giza, Egypt
Rice is a highly valuable crop consumed all over the world. Soil pollution, more specifically chromium (Cr), decreases rice yield and quality. Future climate CO2 (eCO2) is known to affect the growth and yield of crops as well as the quality parameters associated with human health. However, the detailed physiological and biochemical responses induced by Cr in rice grains produced under eCO2 have not been deeply studied. Cr (200 and 400 mg Cr6+/Kg soil) inhibited rice yield and photosynthesis in Sakha 106, but to less extend in Giza 181 rice cultivar. Elevated CO2 reduced Cr accumulation and, consequently, recovered the negative impact of the higher Cr dose, mainly in Sakha 106. This could be explained by improved photosynthesis which was consistent with increased carbohydrate level and metabolism (starch synthases and amylase). Moreover, these increases provided a route for the biosynthesis of organic, amino and fatty acids. At grain quality level, eCO2 differentially mitigated Cr stress-induced reductions in minerals (e.g., P, Mg and Ca), proteins (prolamin, globulin, albumin, glutelin), unsaturated fatty acids (e.g., C20:2 and C24:1) and antioxidants (phenolics and total antioxidant capacity) in both cultivars. This study provided insights into the physiological and biochemical bases of eCO2-induced grain yield and quality of Cr-stressed rice.
1 Introduction
Contamination with heavy metals (HMs) is a critical global environmental problem since their concentrations exceeded the allowable thresholds affecting the quality of soil and crops leading to human health problems (Rai et al., 2019). The naturally occurring heavy metal, chromium (Cr), is beneficial for some plants and animals in trace amounts, however, at higher concentrations it could be a hazardous environmental contaminant (Srivastava et al., 2021). According to Environmental Protection Agency and the International Agency for Research on Cancer, Cr has been classified among the top harmful environmental pollutants and human carcinogens (Tchounwou et al., 2012). The metallic forms, chromite (Cr III) and chromate (Cr VI) are the most stable forms of Cr available in the environment (Vitale et al., 1997). The valence state of Cr affects its toxicity, Cr VI is considered as the more soluble and mobile form at all pH conditions which enhances its bioavailability leading to higher toxicity in relation to Cr III (Oliveira, 2012). Cr III can be oxidized to Cr VI form in the cell which disturbs cell components and integrity (Sharma et al., 2020).
Natural sources and several anthropogenetic activities have resulted in high Cr release into water, soil, and air (Wakeel et al., 2020). Cr contaminated soils lead to phytotoxicity and substantial reduction in the growth and yield quality characteristics (Shahid et al., 2017). Morphological, biochemical, physiological, and molecular changes were reported in Arabidopsis thaliana as affected by Cr (Castro et al., 2007; Martínez-Trujillo et al., 2014; Eleftheriou et al., 2015). Plant root absorbs and accumulates Cr from the soil and induces its availability in the aerial plant parts, via an inactive pathway, consequently, this could affect human health via the food chain (Giri and Singh, 2017). Cr absorption was found to reduce the ability of plant roots for essential nutrients uptake (Wakeel et al., 2020). Once inside the plant, Cr induces phytotoxicity both by immediate Cr-plant interaction, leading to metabolic pathways alterations, and via generation and accumulation of reactive oxygen species (ROS), thus, oxidative damage (Arif et al., 2019; Wakeel et al., 2020).
Rice (Oryza sativa L.) is a highly valuable food crop for the world’s population. Rice is rich in antioxidants, proteins, carbohydrates, micronutrients, and certain fatty acids (Panhwar et al., 2015). It feeds about 50% of the world population, but is highly affected by stress factors such as HMs (Arif et al., 2019). An earlier study by Zhu et al. (2008) indicated minimal changes in grain milling quality and morphology in Cr and zinc contaminated soils. However, Basit et al. (2021) reported adverse effects in plant biomass and photosynthetic rate besides enhancement of ROS levels and antioxidant enzymes under Cr toxicity for two rice varieties. Cr toxicity reduced rice biomass, photosynthetic pigments, and seedling growth besides ATP content and related enzymes (Ma et al., 2016). Such adverse effects in rice quality and yield, when cultivated in Cr polluted soil, urge the needs to develop growth conditions aimed at mitigating this negative impact on plants for sustainable rice production.
Elevated CO2 (eCO2) level has a direct effect on crop growth and yield as well as crop quality parameters associated with human health and food safety (Luo et al., 2019). The greatest outcome of eCO2 on plants is photosynthetic rate increment, thus improving the carbon fixation that may provide the carbohydrate beyond the plant’s need leading to increased biomass and yield quality (Senthil-Nathan, 2021). Plant photosynthesis, biomass, growth, and grain yield were enhanced in rice as positive consequences to eCO2-induced carbon accumulation (Senthil-Nathan, 2021). Consequently, the equilibrium in carbon nutrients may regulate the distribution of plant secondary metabolites (Wang et al., 2019). Becker and Kläring (2016) indicated that eCO2 could enhance red leaf lettuce quality via improvements in the accumulation of antioxidant compounds due to increment in their precursors (soluble sugars). Exudation of dissolved organic carbon into the soil, by plant roots, is increased due to eCO2 (Rosado-Porto et al., 2021). Interestingly, previous studies confirmed the mitigating effect of eCO2 against plant oxidative stress under different environmental limitations (Pérez-López et al., 2009; Abdelgawad et al., 2015; Shabbaj et al., 2022). In this context, in soil contaminated with Cu and Cd, eCO2 had affected HMs distribution in plants and soil and consequently, affected the production safety and quality for rice and wheat grown there (Guo et al., 2011). Kim and Kang (2011) reported that eCO2 increased the pine seedlings’ biomass and ability in lead uptake, besides high changes in tissue metabolites were noted. eCO2 boosted the HMs detoxification system in C3 and C4 species under indium oxide nanoparticles (Shabbaj et al., 2022). The impact of eCO2 on some vegetables’ nutritional quality is well documented in a meta-analysis study that reported increased sugar, antioxidants, and several minerals in potato, tomato, and lettuce (Dong et al., 2018). Further, the mitigating action of CO2 on Cr VI phytotoxicity at the levels of growth and physiology of rice plants at the vegetative stage had been elucidated (AbdElgawad et al., 2022b). However, there is a lack of information regarding the clear interaction of eCO2 and Cr and their synchronous effect on rice yield and grain quality. To this end, as an expected consequence of the effect of eCO2 on plants grown in HMs contaminated soil, the current study was designed to fill the gap and investigate the ability of varied CO2 levels in mitigating the effect of Cr on grains’ yield and quality of two rice cultivars, Giza 181 and Sakha 106. Results afford a scientific background about rice grain quality under Cr stresses in relation to varying concentrations of CO2.
2 Materials and methods
2.1 Plant growth and treatments
Rice (Oryza sativa) seeds (cultivars Sakha 106 and Giza 181) were obtained from Agricultural Research Center, Giza, Egypt. These two cultivars were selected based on their differential responses to heavy metals stress. A homogenous lot of Oryza sativa were surface sterilized using sodium hypochlorite (5% v/v; for 20 min). Seeds were grown in a moist perlite. Then seedlings were transplanted into pots (14 cm high and 13 cm in diameter) filled with clay soil. A basal fertilizer was applied at the rate of 1.2 g urea (N content 46%) and 1.2 g of K2HPO4.3H2O. Pots were incubated in a controlled growth chamber (12 h of photoperiod, photosynthetically active radiation of 350 µmol photons m−2 s−1, 80% of humidity and 28/24°C Day/night temperatures). Rice cultivars were grown in 6 scenarios: 1) ambient CO2 (control, 410 ppm CO2 (aCO2)); 2) aCO2 + Cr VI (200 mg/kg soil); 3) aCO2 + Cr VI (400 mg/Kg soil); 4) elevated CO2 (eCO2, 620 ppm); 5) eCO2 + Cr VI (200 mg/Kg soil) and 6) eCO2 + Cr VI (400 mg/Kg soil). In order to minimize any bias among the cabinets, the experiment had been replicated once again with swapping the two CO2 levels among the cabinets. During the entire experiment, CO2 was supplied in the airflow of the cabinet and its concentration was continuously monitored with a CO2 analyser (WMA-4, PP Systems, Hitchin, UK). A preliminary experiment was conducted with gradient levels of Cr (50-500 mg/Kg soil) to select the most proper concentration of Cr VI. 80% soil water content was kept throughout the experiment. After 3 months dried rice grains were harvested for biochemical analyses. About 100 grains per treatment were milled by a polisher, then unbroken grains and bran were removed using a 1.5 mm sieve. The cleaned milled samples were used for the biochemical analyses. Part of the collected samples were kept in −80°C for enzymes activities investigations. Moreover, soil samples were compiled for chemical analyses.
2.2 Cr level analyses
The levels of Cr in rice grains were measured by flow injection hydride generation atomic absorption spectrophotometry (Welsch, 1990). To extract and investigate total Cr, the samples were digested, overnight at 120°C, in HNO3 and HCLO4. The digestion was stopped when the deep white fumes were released. After that, the levels of Cr were estimated using flow injection hydride generation atomic absorption spectrophotometry (FI-HG-AAS, Perkin Elmer A Analyst 400, CITY, USA) using external calibration (Welsch, 1990). The maximum sensitivity was obtained by using 10% HCl and 0.4% NaBH4.
2.3 Photosynthetic rate and pigment analysis
The light-saturated photosynthetic rate (Asat, μmol CO2 m−2 s−1) was estimated using (LI-COR LI-6400, LI-COR Inc., Lincoln, NE, USA) as outlined previously (AbdElgawad et al., 2015). LI-COR leaf chamber conditions were set according to the climate treatment. Leaf chamber conditions were controlled at 390 ppm CO2 and 23.5°C (block temperature) at saturating PAR (1500 μmol m−2 s−1) and ambient relative humidity for the current climate treatment. For elevated CO2 concentration, the conditions were controlled at 620 ppm.
2.4 Antioxidants analysis
To extract polyphenols and flavonoids, a known weight of fine powdered plant tissues was homogenized in 80% ethanol. The slurry was centrifuged (5000 g for 15 min), and the clear extract was used to quantify total phenolics and flavonoids using Folin-Ciocalteu and AlCl3 methods, respectively. Additionally, tocopherols were extracted in hexane, followed by evaporation using CentriVap concentrator (Labconco, Kansas, USA), and the dry pellet was resuspended in hexane. At the end of the extraction, tocopherols were separated, and their levels were determined by HPLC (Shimadzu, ‘s Hertogenbosch, The Netherlands) coupled with a fluorometric detector (excitation at 290 nm and emission at 330 nm). Tocopherols were separated on normal phase conditions, Particil Pac 5 μm column material, length 250 mm, i.d. 4.6 mm. The mobile phase was applied at a flow rate of 0.45 ml min−1. Dimethyl tocol (DMT) was used as internal standard (5 ppm). Data were analyzed with Shimadzu Class VP 6.14 software.
In 80% ethanol extract, the total antioxidant capacity (TAC, FRAP) was determined. Centrifugation was done at 14000 g, 4°C, for 25 min, and then the FRAP test [TPTZ (0.01 mM) in HCl (0.04 mM), acetate buffer (0.3 M, pH3.6), and FeCl3.6H2O (0.02 M)] was performed by using Trolox (0 to 650 M), as already described (Benzie and Strain, 1996).
2.5 Sugar metabolism
Sugars levels in rice grain were extracted in 50 mM TAE buffer, pH 7.5, supplemented with a mixture of polyclar (0.15%), Na azide (0.02%), PMSF (2 mM), mercapto-ethanol (1 mM), mannitol (10 mM), and NaHSO3 (12 mM). The mixture was centrifuged (15000 g, 4°C, 10 min). Afterwards, a part of the mixture was incubated at 90°C for 5 min, then it was allowed to cool down. Centrifugation was done again (14,000 g, 4°C, 5 min), then the clear supernatant was moved to a mixed bed Dowex column of 300 µL Dowex H+, 300 µL Dowex Ac–; both 100–200 mesh. Thereafter, elution was done with ddH2O, and quantification of different sugars, (glucose, sucrose, raffinose, and fructose) was done by using (HPAEC-PAD) (Verspreet et al., 2012). Sugar separation was done on CarboPac MA1 column. The flow rate of 0.3 mL min−1 of the eluent NaOH gradient (250–700 mM) was applied. The quantification of existing sugars was carried out by comparing the peak areas obtained from calibration curve with those of the corresponding authentic external standards. The internal standard maltotriose which is not naturally present in the samples was used to control the quality of extraction and purification.
For determination of the activities of sugar-related enzymes in rice grains, the samples were extracted in HEPES buffer (100 mM HEPES pH 8.2, 10 mM EDTA, 5 mM MgCl2, 15 mM KCl, 2 mM sodium diethyl dithiocarbamate, 5 mM β-mercaptoethanol, 1% PPV) by MagNALyser (Kerr et al., 1985). After centrifugation at 14,000 g and 4°C for 15 min, the clear supernatant was used for activity determination., starch synthase activity was done in a mixture containing glycogen and citrate (Nishi et al., 2001). Meanwhile, amylase activity was detected in a starch solution containing of I2/KI (0.05%) in HCl (0.05% as well), and then the reading was taken at 620 nm (Madany and Khalil, 2017).
2.6 Protein content
Total proteins were quantified by using the modified semimicro-Kjeldahl methods (Bremner and Hauck, 1982). Albumin, globulin, glutelin and prolamin were assessed using the methods described by Kumamaru et al. (1988). Prolamin contents were determined using Bradford Protein Assay Kit and glutelin content was determined using a bicinchoninic acid Kit.
2.7 Organic acids
Organic acids were detected in rice grains by using HPLC. The HPLC system consisted of a liquid chromatographer (Dionex, USA) and a detector (LED, ultimate 3000), in addition to a pump (LPG-3400A), a column thermostat (TCC-3000SD) and an autosampler (EWPS-3000SI). Separation of organic acids was conducted through an Aminex HPH-87 H (300 × 7.8 mm) column coupled with IG Cation H (30 × 4.6) precolumn of Bio-Red firm (at 65°C). Samples were eluted using 0.001 N sulfuric acid (0.6 mL min-1) and the detection was done at 210 nm. Data analysis and interpretation were done using chromeleon v.6.8 computer software (Hamad et al., 2015).
2.8 Amino acids levels and metabolism
Amino acids were analyzed according to AbdElgawad et al. (2014). Extraction was done by using 100 mg of grain samples in 5 mL of 80% ethanol, and then centrifugation was done (14,000 ×g, 25 min). Afterwards, the supernatant was taken and resuspended in chloroform (5 mL). Detection and quantification of amino acids were done by using UPLC (Waters Acquity, TQD). The aqueous phase was filtered through two Millipore micro filters (0.2 μM pore size). A fixed volume of filtrated supernatant was diluted with the internal standard deuterium labelled l-glutamine- 2,3,3,4,4-d5 (C/D/N Isotopes INC, Pointe-Claire, Quebec). Free amino acids were separated on BEH amide column. A gradient mobile phase system consisted of [A: ammonium formate (84%), acetonitrile (10%) acid and formic (6%)], and [B: acetonitrile and formic acid (2%)] at flow rate of 0.3 mL min−1.
2.9 Fatty acids
Fatty acids levels were determined in grains of treated and non-treated plants by using GC/MS (Hewlett Packard, USA). A know weight of the powdered grains was extracted in chloroform/methanol (2:1, v/v) at 25°C and the lipophilic fractions were centrifuged at 16,000 rpm for 30 min (Hassan et al., 2018). To normalize the extraction efficiency, nonadecanoic acid was used as an internal standard. GC/MS analysis was performed on a Hewlett Packard 6890, MSD 5975 mass spectrometer (Hewlett Packard, Palo Alto, CA, USA), with an HP -5 MS column (30 m × 0.25 mm × 0.25 mm). A 1.0-μL sample was injected using a split mode (split ratio, 1:10). Helium gas was used as a carrier gas at a flow rate of 1.5 mL/min. An electron ionization mode with ionization energy of 70 eV was used for MS detection. The injector and MS transfer line temperatures were set at 220 and 290°C, respectively. The mass scan ranged from 50 to 550 m/z with an Em voltage, 1035 V. The quantification of fatty acids was conducted by comparison of the mass spectrometric ion signal of the target molecule with that of an identical standard. The fatty acid content in the sample is then calculated from the standard curve using analyte/internal standard ion yield ratios (Hassan et al., 2018).
2.10 Statistics
The results were expressed as mean ± SD (standard deviation) and analyzed by two-way ANOVA using IBM SPSS Statistical 23 software package (SPSS® Inc., Chicago, IL, USA). Statistical significance of the mean data was assessed by post-hoc TukeyHSD (p ≤ 0.05).
3 Results
3.1 Growth and photosynthesis
Soil contamination with Cr greatly inhibited the biomass production and the photosynthetic rate in both rice cultivars, while these reductions were less severe in Giza 181 (Figure 1). Under Cr free conditions, eCO2 improved the biomass and photosynthetic rate in both cultivars, where Sakha 106 is the most responsive. Further, eCO2 effectively recovered the hazardous impact of Cr on growth and photosynthesis of rice plants (Table S1). Such mitigating action of eCO2 was more obvious in Sakha 106.
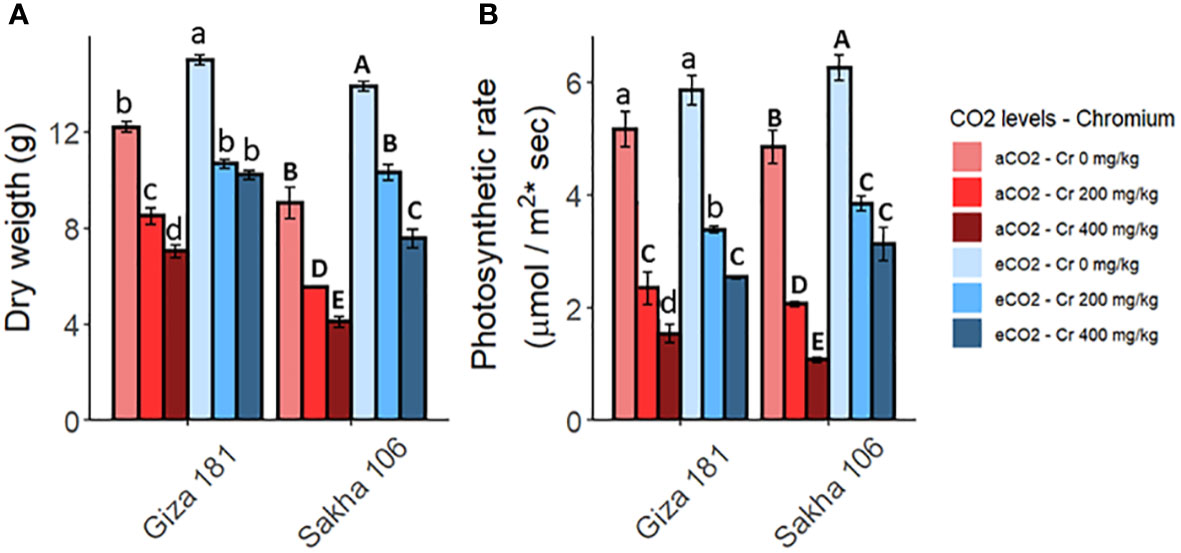
Figure 1 Effect of Chromium (Cr) exposure on dry biomass (A) and the rate of photosynthesis (B) in rice plants grown under either aCO2 (410 ppm) or eCO2 (620 ppm). Values are presented as means± standard error of 4 independent replicates. Different letters within the same cultivar represent significant differences between means (Tukey’s Test; P < 0.05).
3.2 Accumulation of Cr6+ and grain yield
The accumulation of Cr6+ in the grains of the two rice cultivars is increased by increasing the concentration of Cr in the soil (Figure 2). However, Sakha 106 cultivar accumulated higher levels of Cr6+ in their grains, compared to Giza 181. The co-application of eCO2 with Cr decreased the accumulation of Cr6+ in the grains of both cultivars (Table S1). For instance, eCO2 treatment decreased the levels of Cr6+ by about 35 and 20% in grains of Sakha 106 and Giza 181, respectively, at the sever dose of Cr (400 mg Cr6+/Kg soil).
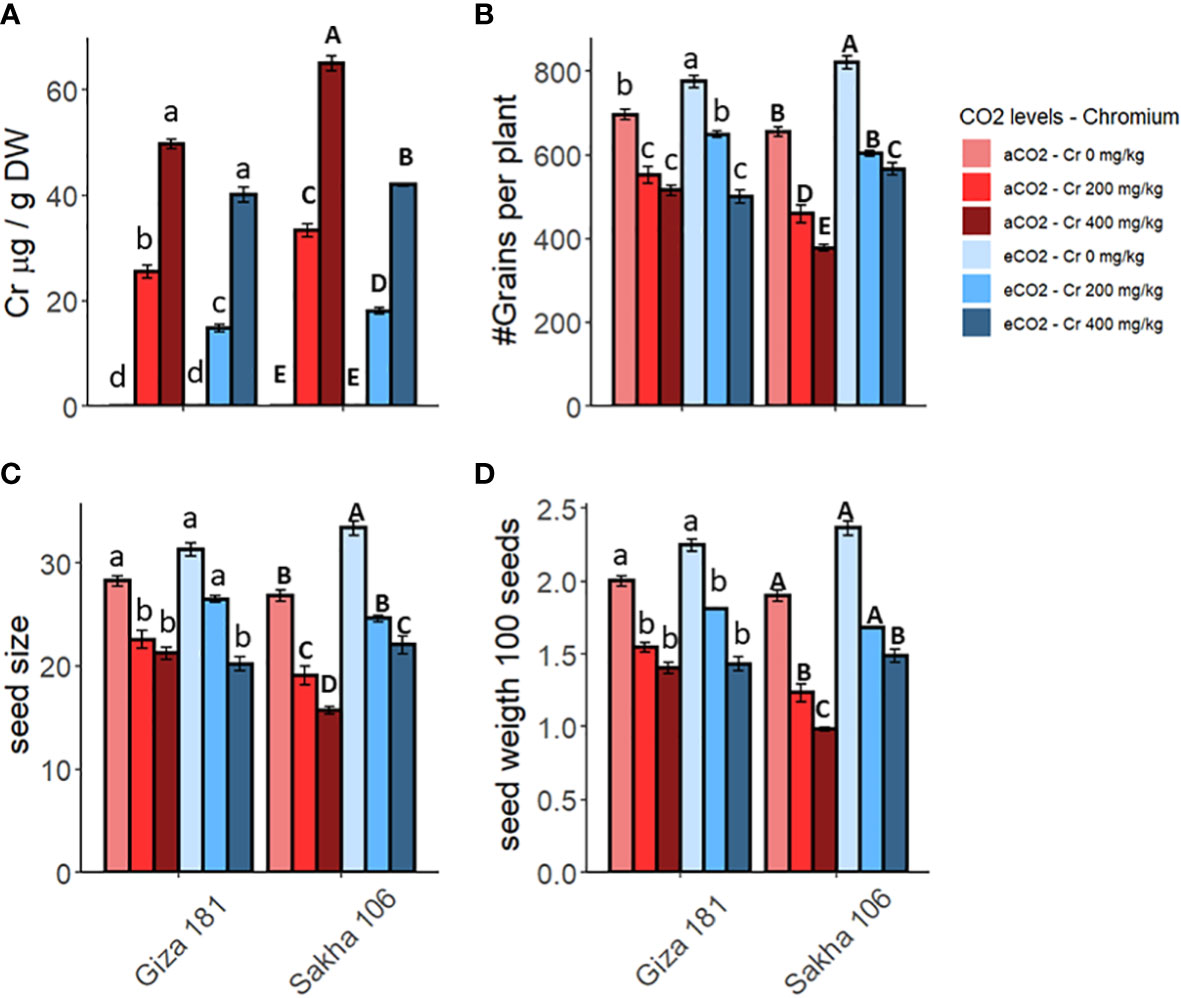
Figure 2 Effect of Chromium (Cr) exposure on Cr accumulation (A) and yield parameters (B–D) of rice grains produced under either aCO2 (410 ppm) or eCO2 (620 ppm). Values are presented as means ± standard error of 4 independent replicates. Different letters within the same cultivar represent significant differences between means (Tukey's Test; P < 0.05).
Expectedly, soil contamination with Cr significantly inhibited the yield parameters of both rice cultivars, specially under the higher dose of Cr (Figure 2). However, yield of Giza 181 cultivar was less affected by Cr accumulation as compared with that of Sakha 106. In this regard, the higher dose of Cr caused 25, 30 and 26% reductions in the numbers of grains per plant, grain size, and grain weight, respectively, in Giza 181 cultivar compared to 41, 48 and 42% in Sakha 106. eCO2 caused a positive impact on the measured yield parameters in both cultivars. Further, the co-application of eCO2 significantly recovered the negative impact of the lower Cr concentration on both cultivars (Table S1) and that for the higher Cr dose in Sakha 106 only.
3.3 Elemental composition of rice grains
In general, Cr toxicity considerably retard the elemental composition of rice grains and such effect was more sever at the higher dose of Cr, where Sakha cultivar was the most affected (Table 1). For instance, 400 mg Cr6+/Kg soil caused significant reductions in the levels of P, S, K, Ca, Zn and Mn in both cultivars and that for Mg, Fe and Cu in Sakha 106 only. In absence of Cr stress, eCO2 significantly improved the levels of P in Giza 181 and that for Mg and Ca in both cultivars. eCO2 significantly recovered the inhibitory action of Cr stress on the levels of most of the detected elements in both cultivars.
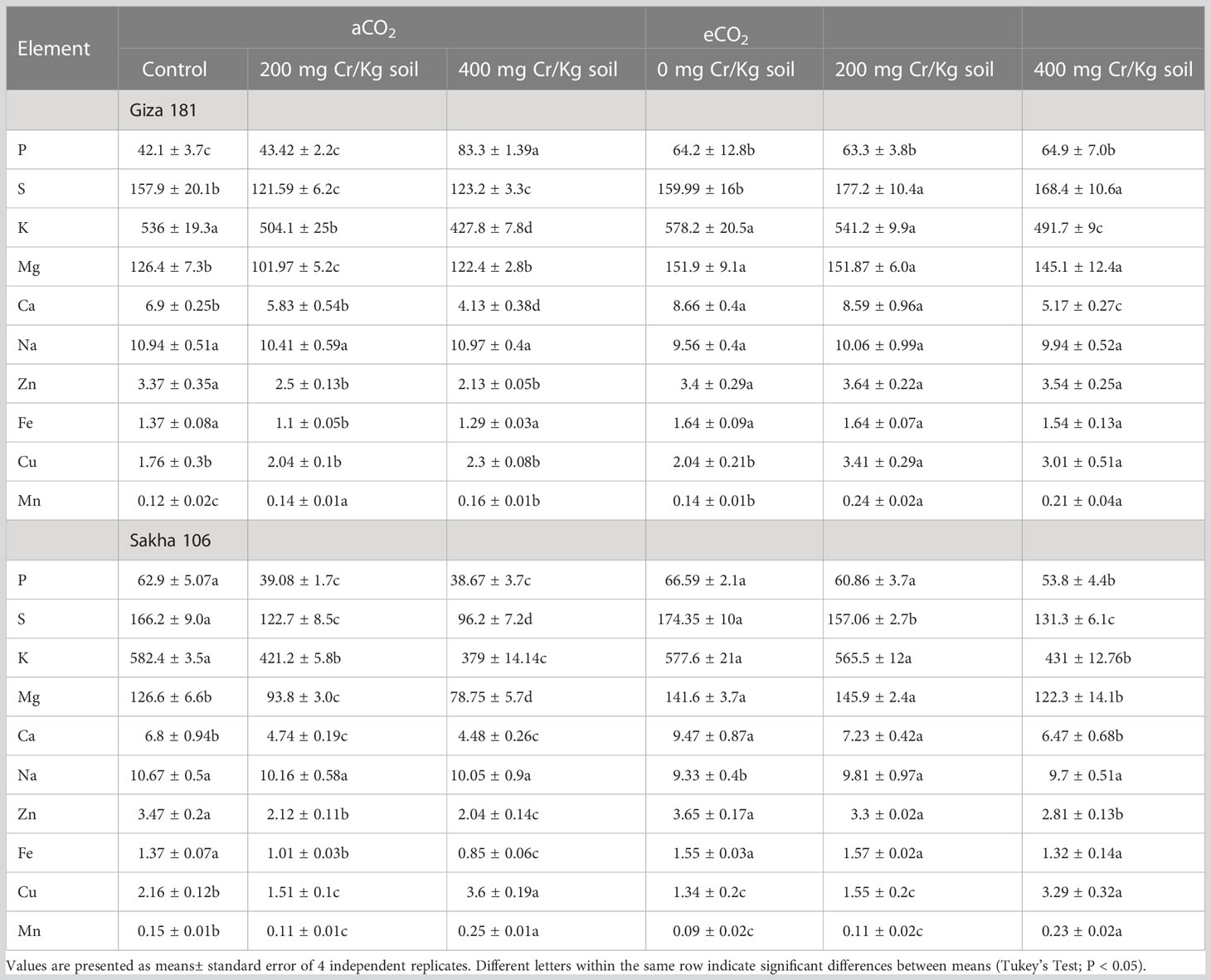
Table 1 Effect of Chromium (Cr) exposure on elemental composition of rice grains produced under either aCO2 (410 ppm) or eCO2 (620 ppm).
3.4 Grain protein content
Cr stress significantly decreased the accumulation of prolamin, globulin, albumin, glutelin and total proteins in grains of Giza 181 and to more extent in Sakha 106 (Figure 3). For instance, Cr at concentration of 400 mg/Kg soil decreased the prolamin, globulin and total protein of Sakha 106 and Giza 181 by about 59, 40 and 44%, and 29, 20 and 22%, respectively, as compared with their respective controls. eCO2 alone treatment improved the accumulation of globulin, glutelin, prolamin, and total protein in the grains of Sakha 106, but had no significant impact on the protein profile of Giza 181. Under Cr stress, eCO2 efficiently recovered the adverse effects of Cr on accumulation of prolamin, globulin and total protein, especially in Sakha 106. In this regard, at sever Cr stress, the levels of grain prolamin and globulin in Sakha 106 and Giza 181 plants grown under eCO2 were 1.7 and 1.4-fold, and 1.3 and 1.2-fold, respectively, higher than those found in grains produced under aCO2.
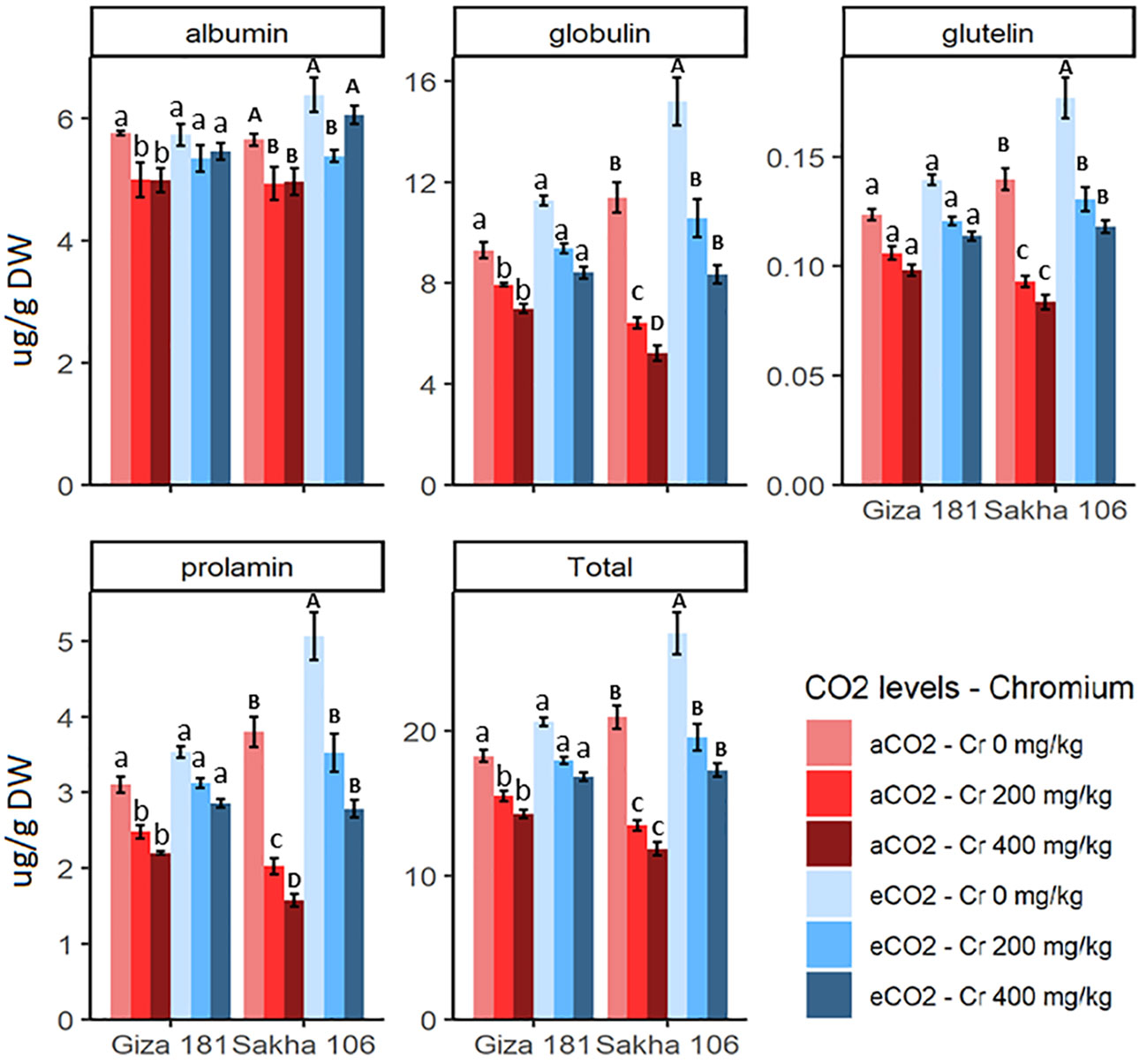
Figure 3 Effect of Chromium (Cr) exposure on protein profile of rice grains produced under either aCO2 (410 ppm) or eCO2 (620 ppm). Values are presented as means± standard error of 4 independent replicates. Different letters within the same cultivar represent significant differences between means (Tukey's Test; P < 0.05).
3.5 Sugars and organic acids
Unlike soluble sugars, Cr stress reduced the starch levels and inhibited the activities of starch synthase in both cultivars (Table 2). In unstressed plants, eCO2 had no significant impact on the different sugar fractions in Sakha 106 but improved the soluble sugars in Giza 181. However, in Cr-stressed plants, eCO2 decreased the Cr-induced accumulation in soluble sugars in Giza 181 and recovered the inhibition in the activities of starch synthase in both cultivars (Table S1).
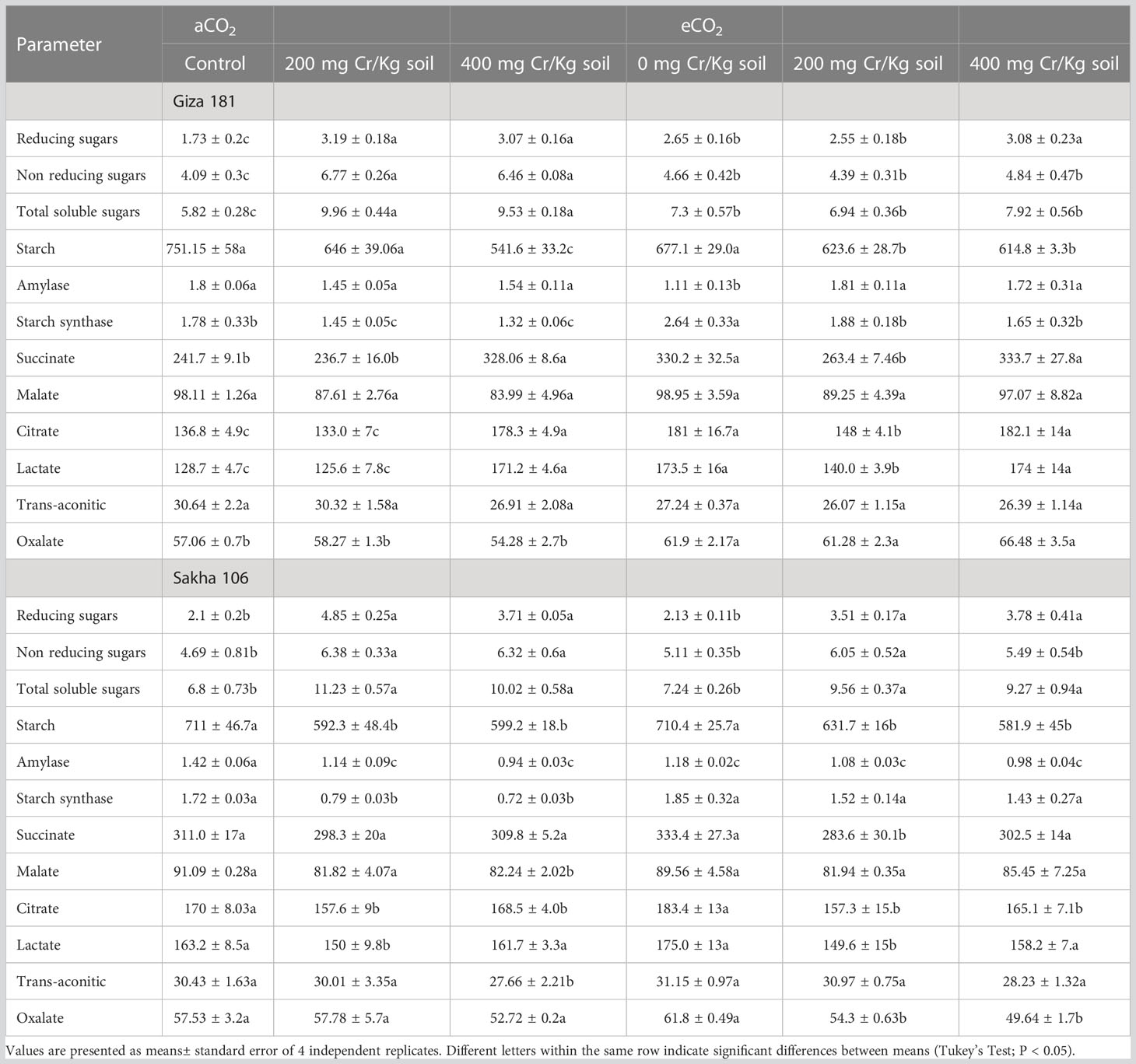
Table 2 Effect of Chromium (Cr) exposure on the levels of sugars and organic acids and the activities of sugar metabolizing enzymes in rice grains produced under either aCO2 (410 ppm) or eCO2 (620 ppm).
The lower dose of Cr did not exert any significant effect on the concentrations of organic acids in the two cultivars (Table 2). The higher dose of Cr, however, improved the accumulation of succinate, citrate and lactate, in Giza 181 and decreased the levels of malate, citrate and trans-aconitate in Sakha 106. eCO2 had no significant effect on the levels of organic acids in Sakha 106 plants grown, however in Giza 181 eCO2 improved the levels of succinate, citrate, lactate and oxalate. Further, eCO2 increased the levels of oxalate in Giza 181 and that for malate and trans-aconitate in Sakha 106 plants grown under 400 mg Cr/Kg soil.
3.6 Amino acids composition
Despite the variability in the response to Cr stress among the two cultivars, some amino acids showed distinctive patterns of response (Table 3). For instance, lysin and threonine were improved in both cultivars, while valine was sharply decreased. Cultivar specific responses were also recorded in response to eCO2 alone treatment. For example, lysine, histidine and aspartate were improved in Sakha 106 but not affected in Giza 181. On contrary, the levels of serine and cystine were decreased in Sakha 106 and unchanged in Giza 181. Under Cr stress, eCO2 improved the accumulation of lysine and histidine in both cultivars. Unlike in Giza 181, eCO2 decreased the levels of aspartate, valine and tyrosine in Cr-stressed Sakha 106 plants. On the other hand, eCO2 decreased the levels of glycine and arginine in Giza 181, but not Sakha 106, under Cr stress.
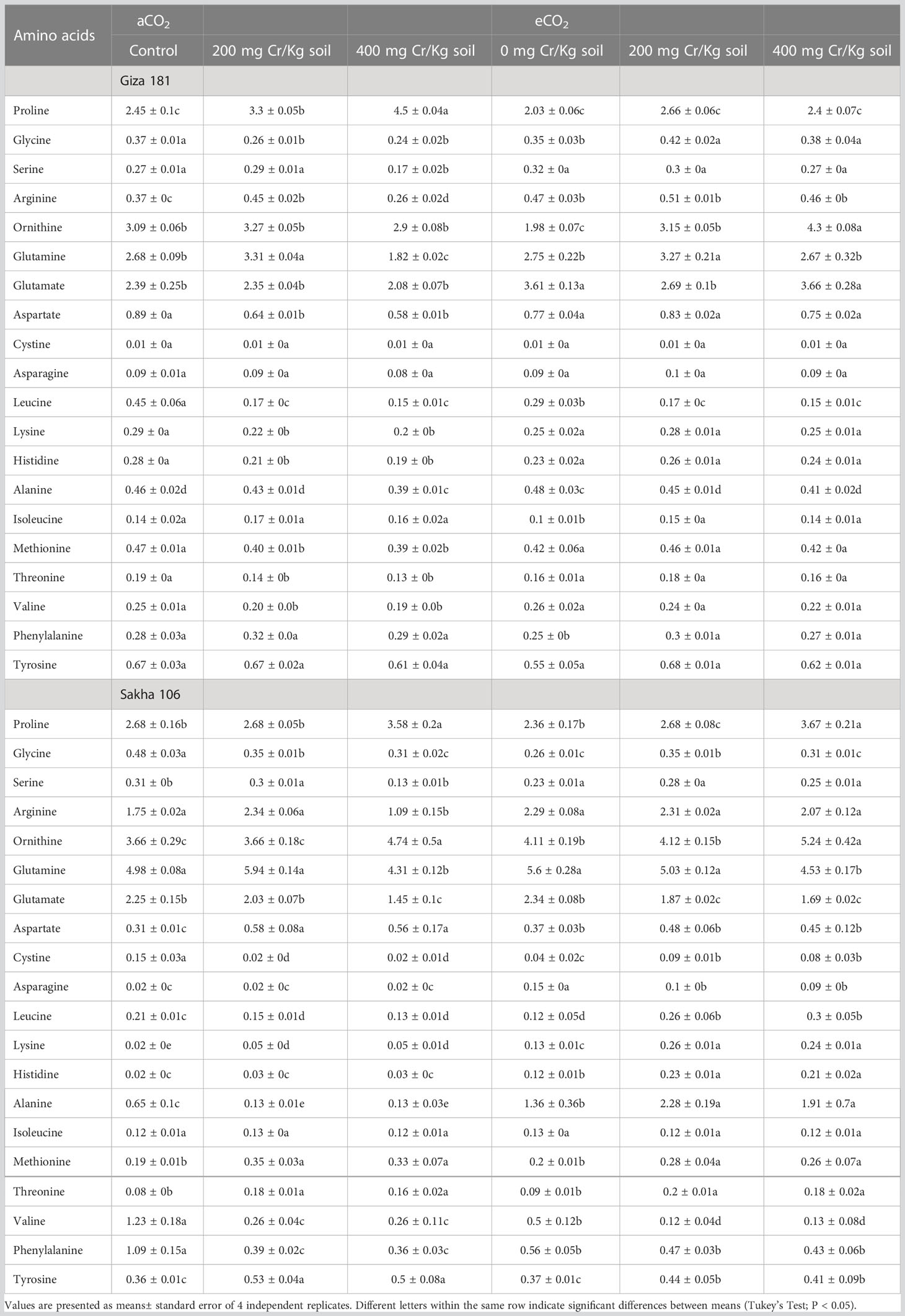
Table 3 Effect of Chromium (Cr) exposure on amino acids profile of rice grains produced under either aCO2 (410 ppm) or eCO2 (620 ppm).
3.7 Fatty acids composition
Cr stress, at both levels, significantly decreased the accumulation of the majority of the detected saturated and unsaturated fatty acids in both rice cultivars (Table 4). However, the negative impact of Cr stress on the total unsaturated acids was more evident on Giza 181, specially at the higher dose of Cr. On contrary, eCO2 alone treatment exerted a positive impact on the accumulation of most of the individual fatty acids in Sakha 106 and to less extent in Giza 181. Interestingly, Cr stressed plants grown under eCO2 accumulated higher levels of the unsaturated fatty acids C20:2 and C24:1, as compared to their respective Cr alone treatments. Further, eCO2 improved the accumulation of C16:1, C16:2, C16:3, C18:1 and C18:3 in Cr-stressed Sakha 106.
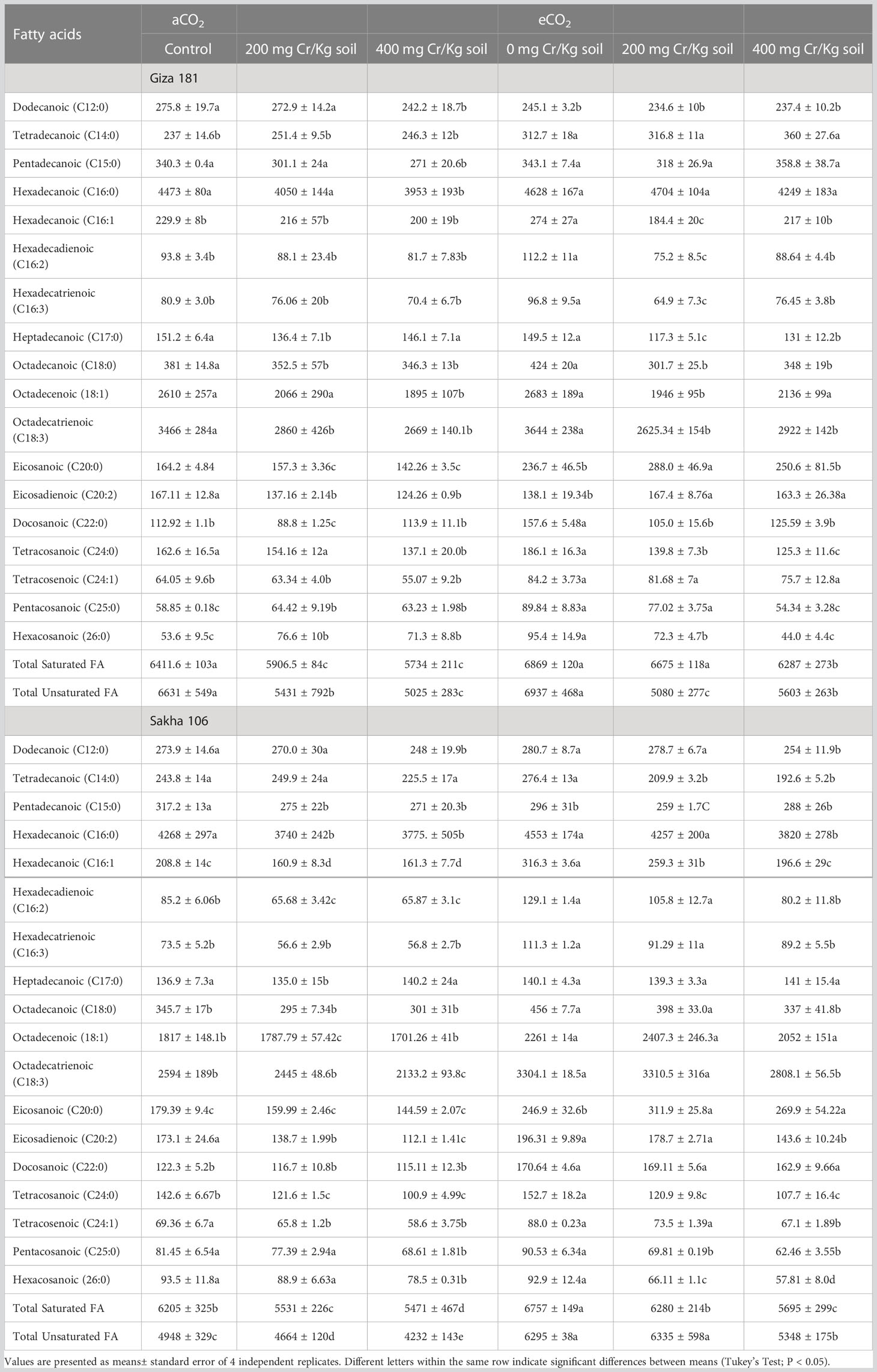
Table 4 Effect of Chromium (Cr) exposure on fatty acids profile of rice grains produced under either aCO2 (410 ppm) or eCO2 (620 ppm).
3.8 Molecular antioxidants and total antioxidant capacity
The impact of Cr toxicity on accumulation of total phenolics and TAC was more sever at the higher Cr concentration and was more evident for Sakha 106 cultivar (Figure 4). For instance, Sakha 106 plants grown in soil contaminated with 400 mg Cr6+/Kg soil showed about 39, 43 and 49% reductions in the content of total phenolics and the FRAP and ABTS radical scavenging activities, respectively, as compared with 21, 26 and 34% inhibitions in case of Giza 181. The sever Cr dose improved the total tocopherols in Giza 181 but not in Sakha 106, however it reduced the gamma tocopherol in both cultivars. eCO2 alone treatment improved the total tocopherols in Giza 181 but had no significant effects on the levels of flavonoids and total phenolics or the FRAP and ABTS radical scavenging activities in both cultivars. However, under Cr stress, eCO2 mitigated the negative impacts of Cr on phenolics accumulation and TAC in both cultivars.
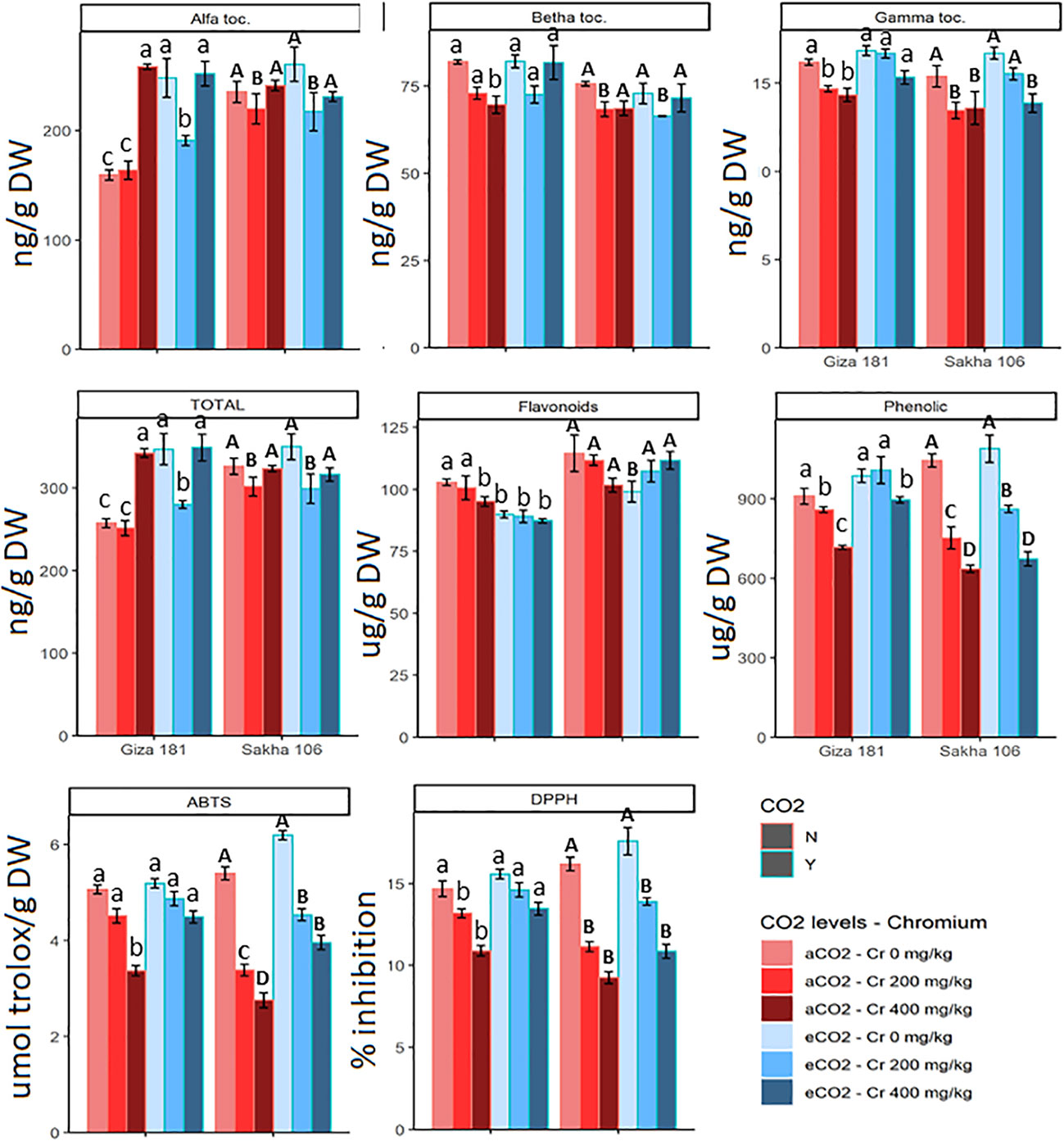
Figure 4 Effect of Chromium (Cr) exposure on the levels of molecular antioxidants and total antioxidant capacities of rice grains produced under either aCO2 (410 ppm) or eCO2 (620 ppm). Values are presented as means± standard error of 4 independent replicates. Different letters within the same cultivar represent significant differences between means (Tukey's Test; P < 0.05).
4 Discussion
Rice represents the major source for carbohydrates in the diet of about one half of the world population. Unfortunately, the production of rice is threatened by the presence of contaminants in water and soils. In this regard, Cr has been reported as a major pollutant that affect the yield and quality of rice grains in affected areas (Ma et al., 2016; Basit et al., 2021). eCO2 has been reported to ameliorate the negative impacts of some heavy metals, e.g., Hg, Cu, Cd and Pb, on several plant species (Guo et al., 2011; Kim and Kang, 2011; Saleh et al., 2021). In a recent study, AbdElgawad et al. (2022b) had addressed the mitigating action of CO2 on Cr VI phytotoxicity at the levels of growth and physiology of rice plants at the vegetative stage, however the implication on the quality of rice grains had not been studied. Herein, we have evaluated the mitigating action of eCO2 on the yield and quality of two rice cultivars, Giza 181 and Sakha 106, grown under chromate (Cr VI), the most stable and bioavailable form of Cr in the environment (Vitale et al., 1997; Oliveira, 2012).
Cr is inactively absorbed by the plant roots and negatively affect the uptake of essential mineral nutrients, accordingly, this will reduce the plant growth (Wakeel et al., 2020). The present data showed that Cr stress significantly inhibited the whole plant biomass of both rice cultivars at the harvest time, in a concentration dependent manner. This reduction could be ascribed to the sharp inhibition noted in the photosynthetic rate of rice plants as affected by Cr (Figure 1). Similarly, Cr application in soil (100 to 500 mg kg−1) markedly reduced the growth, photosynthetic pigments, and photosynthetic rate of rice plants (Hussain et al., 2018; AbdElgawad et al., 2022b). Further, Basit et al. (2021) reported that Cr adversely affected the biomass production and photosynthetic rate and induced the accumulation of ROS and cell damage. Notably, eCO2 mitigated the negative impact of Cr on dry matter. eCO2 has been reported to improve the growth of HMs stressed plants by enhancing the photosynthetic C assimilation, as a substrate for Rubisco, and by inhibiting photorespiration, thereby, reducing the production of H2O2 and the provoked cell damage (Saleh et al., 2019; AbdElgawad et al., 2022a). In accordance, the present results indicated that eCO2 significantly ameliorated the hazardous impact of Cr on the photosynthetic rate of both cultivars with Sakha 106 is the more responsive to eCO2. Such impact was confirmed by PCA which reveals a separation on the bases of Cr treatment on the PC1, which explains 31.8% of the variance, meanwhile Cr combined with eCO2 treatments seems closer to the control plants (Figure 5).
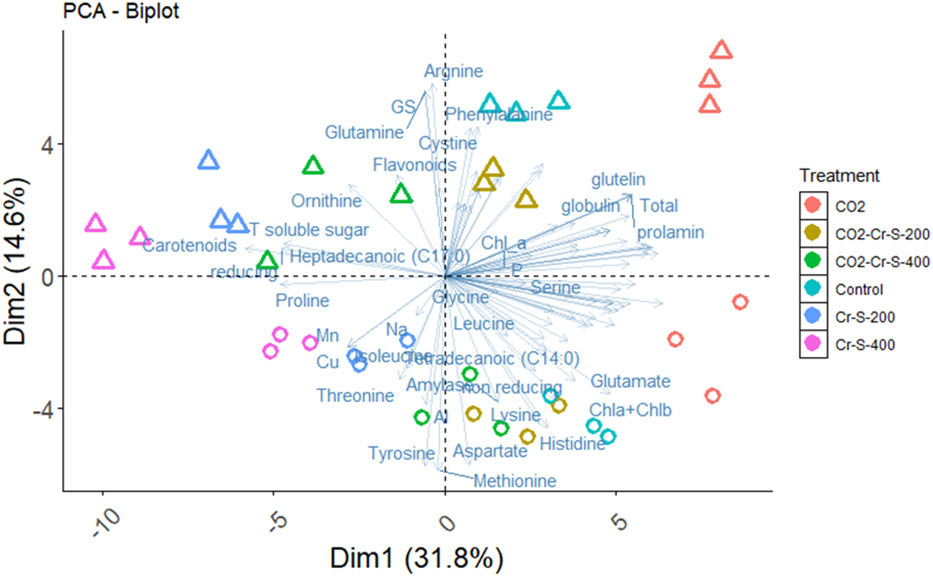
Figure 5 Principal component analysis (PCA) of biochemical parameters of rice grains produced under Chromium (Cr) and either aCO2 (410 ppm) or eCO2 (620 ppm). Variances explained by the first two components (PC1 and PC2) appear in parentheses.
Similar to the present results, the accumulation of Cr in grains and the reduction in yield of rice plants as affected by soil pollution with Cr has been reported (Sundaramoorthy et al., 2010; Zhou et al., 2019). In consistence with its ameliorating action against the Cr-induced reduction in growth and photosynthesis, eCO2 caused a notable increase in the yield parameters of both rice cultivars grown under Cr and reduced the accumulation of Cr in the produced grains. Similarly, eCO2 has been recorded to enhance the yield and ear number in rice grown in Hg polluted soil (Mao et al., 2021). Despite the absence of literature regarding the impact of eCO2 on the accumulation of Cr in rice grains, Guo et al. (2011) reported that grains of rice and wheat plants grown in soils polluted with Cd or Cu accumulated higher Cd and less Cu compared to those produced under aCO2. On the other hand, the ability of eCO2 to reduce the accumulation of Cr in vegetative tissues of rice has been recently reported (AbdElgawad et al., 2022b). Further, AbdElgawad et al. (2022a) has pointed to the ability of eCO2, alone or combined with arbuscular mycorrhizal fungi, to reduce the accumulation of AsIII in wheat and soybean plants. They have ascribed the reduced uptake of these HMs under eCO2 to the lower stomatal conductance and the higher exudation of polyphenols and organic acid into the soil, relative to the control. This explanation was supported by the fact that polyphenols and organic acids can form complexes with HMs, thereby, reduced their bioavailability (Schwab et al., 2005; Campbell and Nordstrom, 2014).
The nutritional quality of rice grains is related to their carbohydrate, protein and lipid compositions (Yibo et al., 2022). In addition, secondary metabolites, e.g., polyphenols, and minerals, e.g., Fe and Zn, that exist in the rice grain are beneficial to human health (Zhao et al., 2020). To assess the impact of Cr and/or eCO2 on the nutritional value of rice grains we have performed a metabolic profiling of carbohydrates, proteins, amino acids, fatty acids, minerals and antioxidant metabolites. The results indicated a negative impact for Cr stress, especially at the highest dose, on the grains’ nutritional value in Giza 181 and to more extent in Sakha 106 in term of the following: 1) increased accumulation of Cr; 2) reduced starch content; 3) decreased levels of individual and total proteins and several amino acids; 4) reduced levels of several unsaturated fatty acids; 5) lower levels of mineral nutrients such as Fe and Zn; 6) reduced content of polyphenols and TAC. Interestingly, growing plants under eCO2 efficiently antagonized the adverse effects of Cr on the nutritional value of rice grains. In this regard, Sharma et al. (1995) reported that Cr VI (0.05-1.00 mM) greatly reduced the grain yield of T. aestivum plants, where at the highest dose, the plants failed to form seeds. Maize plants treated with Cr VI at concentrations of 30-150 μmol L-1 showed increased accumulation of Cr in grains and decreased yield attributes, in a dose dependent manner (Anjum et al., 2017). Further, Singh et al. (2020) have reported that growing two varieties of chickpea in soil treated with Cr VI (60 μM and 120 μM) significantly reduced the yield attributes (e.g. pod number, grain yield per plant) and grain proteins.
In fact, most carbon that integrate to the nutritional components of the rice grain is imported as photoassimilates from the photosynthetic leaves, particularly the flag leaf, during the grain-filling, (Yoshida, 1981). Further, the correlation between C assimilation and yield in rice is likely (Sharkey et al., 2000). Thus, the negative impact of Cr on the yield and nutritional value of rice grains could be a logical consequence of its adverse action on plant biochemical attributes. In this regard, Cr has been reported to induce oxidative damage and to inhibit many physiological and biochemical aspects in plants, including photosynthesis and C and N metabolism (Sangwan et al., 2014; Shahid et al., 2017; Hussain et al., 2018). Further, by its negative impact on starch synthesizing enzymes, e.g., starch synthase, Cr could retard the sink strength of the developed grains, thus affecting its filling with C and N metabolites (Mohapatra et al., 2009). On the other hand, the ameliorating impact of eCO2 against Cr-induced reduction in yield and nutritional compositions could be attributed to the enhancement of photosynthetic C assimilation, which will improve the biosynthesis of photoassimilates and hence retrieve the source strength. In this regard, Mao et al. (2021) reported that eCO2 significantly promoted the light saturated CO2 assimilation rate in rice plants, during both flowering and grain filling periods, which improved the yield attributes. Högy et al. (2009) revealed that eCO2 caused enhancements in the levels of non-structural carbohydrates and lipids but decreased the protein content in wheat grains. Elevated CO2 improved grain size and weight but did not significantly affect its nitrogen and amylose content in wild and domesticated rice genotypes (Rahman et al., 2021). Besides eCO2 has found to enhance the activity of starch synthesizing enzymes and improve the accumulation of starch in wheat and maize plants grown under mercuric oxide nanoparticles (AbdElgawad et al., 2020). Thus, it could be hypothesized that the positive impact of eCO2 on the nutritional value of grains is more evident under stressful conditions that retard the source strength of the photosynthetic leaves and/or the sink strength of the developing grains.
5 Conclusion
Based on the results of the present study it could be concluded that Cr stress significantly inhibited the growth and photosynthetic and grain yield and quality of rice plants. However, growing plants under of eCO2 could mitigate the negative effects of Cr at the levels of growth, physiology, and yield production. Cr exposure reduces the quality of rice grains by inducing the accumulation of Cr and reducing the levels of nutritionally important metabolites such as starch, proteins, unsaturated fatty acids, antioxidant molecules, and minerals. Interestingly, eCO2 reduced the accumulation of Cr in rice grains and improved the grain quality under Cr toxicity. To this end, growing rice under CO2-enriched environment could reduce the toxicity hazards of Cr and support the production and quality of the produced grains.
Data availability statement
The raw data supporting the conclusions of this article will be made available by the authors, without undue reservation.
Author contributions
Conception and design of study: HA, JvD, GB, AS. Acquisition of data: HA, JvD, AS. Analysis and/or interpretation of data: AM, MA, JvD, AS. Drafting the manuscript: HA, AM, AS. Revising the manuscript critically for important intellectual content: HA, JvD, AS, GB. All authors contributed to the article and approved the submitted version.
Funding
This research has been supported by Princess Nourah bint Abdulrahman University Researchers Supporting Project number (PNURSP2023R101), Princess Nourah bint Abdulrahman University, Riyadh, Saudi Arabia.
Conflict of interest
The authors declare that the research was conducted in the absence of any commercial or financial relationships that could be construed as a potential conflict of interest.
Publisher’s note
All claims expressed in this article are solely those of the authors and do not necessarily represent those of their affiliated organizations, or those of the publisher, the editors and the reviewers. Any product that may be evaluated in this article, or claim that may be made by its manufacturer, is not guaranteed or endorsed by the publisher.
Supplementary material
The Supplementary Material for this article can be found online at: https://www.frontiersin.org/articles/10.3389/fpls.2023.1019859/full#supplementary-material
References
AbdElgawad, H., De Vos, D., Zinta, G., Domagalska, M. A., Beemster, G. T. S., Asard, H. (2015). Grassland species differentially regulate proline concentrations under future climate conditions: An integrated biochemical and modelling approach. New Phytol. 208, 354–369. doi: 10.1111/nph.13481
AbdElgawad, H., El-Sawah, A. M., Mohammed, A. E., Alotaibi, M. O., Yehia, R. S., Selim, S., et al. (2022a). Increasing atmospheric CO2 differentially supports arsenite stress mitigating impact of arbuscular mycorrhizal fungi in wheat and soybean plants. Chemosphere 296, 134044. doi: 10.1016/j.chemosphere.2022.134044
Abdelgawad, H., Farfan-vignolo, E. R., Vos, D., Asard, H. (2015). Elevated CO2 mitigates drought and temperature-induced oxidative stress differently in grasses and legumes. Plant Sci. 231, 1–10. doi: 10.1016/j.plantsci.2014.11.001
AbdElgawad, H., Hassan, Y. M., Alotaibi, M. O., Mohammed, A. E., Saleh, A. M. (2020). C3 and C4 plant systems respond differently to the concurrent challenges of mercuric oxide nanoparticles and future climate CO2. Sci. Total Environ. 749, 142356. doi: 10.1016/j.scitotenv.2020.142356
AbdElgawad, H., Peshev, D., Zinta, G., den Ende, W., Janssens, I. A., Asard, H. (2014). Climate extreme effects on the chemical composition of temperate grassland species under ambient and elevated CO2: A comparison of fructan and non-fructan accumulators. PloS One 9, e92044. doi: 10.1371/journal.pone.0092044
AbdElgawad, H., Sheteiwy, M. S., Saleh, A. M., Mohammed, A. E., Alotaibi, M. O., Beemster, G. T. S., et al. (2022b). Elevated CO2 differentially mitigates chromium (VI) toxicity in two rice cultivars by modulating mineral homeostasis and improving redox status. Chemosphere 307, 135880. doi: 10.1016/j.chemosphere.2022.135880
Anjum, S. A., Ashraf, U., Imran, K., Tanveer, M., Shahid, M., Shakoor, A., et al. (2017). Phyto-toxicity of chromium in maize: Oxidative damage, osmolyte accumulation, anti-oxidative defense and chromium uptake. Pedosphere 27, 262–273. doi: 10.1016/S1002-0160(17)60315-1
Arif, N., Sharma, N. C., Yadav, V., Ramawat, N., Dubey, N. K., Tripathi, D. K., et al. (2019). Understanding heavy metal stress in a rice crop: Toxicity, tolerance mechanisms, and amelioration strategies. J. Plant Biol. 62, 239–253. doi: 10.1007/s12374-019-0112-4
Basit, F., Chen, M., Ahmed, T., Shahid, M., Noman, M., Liu, J., et al. (2021). Seed priming with brassinosteroids alleviates chromium stress in rice cultivars via improving ROS metabolism and antioxidant defense response at biochemical and molecular levels. Antioxidants 10, 1089. doi: 10.3390/antiox10071089
Becker, C., Kläring, H.-P. (2016). CO2 enrichment can produce high red leaf lettuce yield while increasing most flavonoid glycoside and some caffeic acid derivative concentrations. Food Chem. 199, 736–745. doi: 10.1016/j.foodchem.2015.12.059
Benzie, I. F. F., Strain, J. J. (1996). The ferric reducing ability of plasma (FRAP) as a measure of “antioxidant power”: The FRAP assay. Anal. Biochem. 239, 70–76. doi: 10.1006/abio.1996.0292
Bremner, J. M., Hauck, R. D. (1982). Advances in methodology for research on nitrogen transformations in soils. Nitrogen Agric. soils 22, 467–502. doi: 10.2134/agronmonogr22.c13
Campbell, K. M., Nordstrom, D. K. (2014). Arsenic speciation and sorption in natural environments. Rev. Mineral Geochem. 79, 185–216. doi: 10.2138/rmg.2014.79.3
Castro, R. O., Trujillo, M. M., Bucio, J. L., Cervantes, C., Dubrovsky, J. (2007). Effects of dichromate on growth and root system architecture of Arabidopsis thaliana seedlings. Plant Sci. 172, 684–691. doi: 10.1016/j.plantsci.2006.11.004
Dong, J., Gruda, N., Lam, S. K., Li, X., Duan, Z. (2018). Effects of elevated CO2 on nutritional quality of vegetables: A review. Front. Plant Sci. 9. doi: 10.3389/fpls.2018.00924
Eleftheriou, E. P., Adamakis, I. D. S., Panteris, E., Fatsiou, M. (2015). Chromium-induced ultrastructural changes and oxidative stress in roots of Arabidopsis thaliana. Int. J. Mol. Sci. 16, 15852–15871. doi: 10.3390/ijms160715852
Giri, S., Singh, A. K. (2017). Human health risk assessment due to dietary intake of heavy metals through rice in the mining areas of singhbhum copper belt, India. Environ. Sci. pollut. Res. 24, 14945–14956. doi: 10.1007/s11356-017-9039-9
Guo, H., Zhu, J., Zhou, H., Sun, Y., Yin, Y., Pei, D., et al. (2011). Elevated CO2 levels affects the concentrations of copper and cadmium in crops grown in soil contaminated with heavy metals under fully open-air field conditions. Environ. Sci. Technol. 45, 6997–7003. doi: 10.1021/es2001584
Hamad, I., AbdElgawad, H., Al Jaouni, S., Zinta, G., Asard, H., Hassan, S., et al. (2015). Metabolic analysis of various date palm fruit (Phoenix dactylifera l.) cultivars from Saudi Arabia to assess their nutritional quality. Molecules 20, 13620–13641. doi: 10.3390/molecules200813620
Hassan, M. O., Saleh, A. M., AbdElgawad, H. (2018). Sonchus oleraceus residue improves nutritive and health-promoting value of common bean ( Phaseolus vulgaris l.): A metabolic study. J. Agric. Food Chem. 66, 2092–2100. doi: 10.1021/acs.jafc.7b05821
Högy, P., Wieser, H., Köhler, P., Schwadorf, K., Breuer, J., Franzaring, J., et al. (2009). Effects of elevated CO2 on grain yield and quality of wheat: Results from a 3-year free-air CO2 enrichment experiment. Plant Biol. 11, 60–69. doi: 10.1111/j.1438-8677.2009.00230.x
Hussain, A., Ali, S., Rizwan, M., Zia ur Rehman, M., Hameed, A., Hafeez, F., et al. (2018). Role of zinc–lysine on growth and chromium uptake in rice plants under cr stress. J. Plant Growth Regul. 37, 1413–1422. doi: 10.1007/s00344-018-9831-x
Kerr, P. S., Rufty, T. W., Huber, S. C. (1985). Endogenous rhythms in photosynthesis, sucrose phosphate synthase activity, and stomatal resistance in leaves of soybean (Glycine max [L.] merr.). Plant Physiol. 77, 275–280. doi: 10.1104/pp.77.2.275
Kim, S., Kang, H. (2011). Effects of elevated CO2 and Pb on phytoextraction and enzyme activity. Water. Air. Soil pollut. 219, 365–375. doi: 10.1007/s11270-010-0713-5
Kumamaru, T., Satoh, H., Iwata, N., Omura, T., Ogawa, M., Tanaka, K. (1988). Mutants for rice storage proteins. Theor. Appl. Genet. 76, 11–16. doi: 10.1007/BF00288825
Luo, X. S., Zhang, D., Hu, Z., Liu, C., Zhao, Z., Sun, W., et al. (2019). Effects of elevated carbon dioxide on metal transport in soil-crop system: Results from a field rice and wheat experiment. J. Soils Sediments 19, 3742–3748. doi: 10.1007/s11368-019-02329-z
Madany, M. M. Y., Khalil, R. R. (2017). Seed priming with ascorbic acid or calcium chloride mitigates the adverse effects of drought stress in sunflower (Helianthus annuus l.) seedlings. Egypt J. Exp. Biol. 13, 119–133. doi: 10.5455/egyj
Ma, J., Lv, C., Xu, M., Chen, G., Lv, C., Gao, Z. (2016). Photosynthesis performance, antioxidant enzymes, and ultrastructural analyses of rice seedlings under chromium stress. Environ. Sci. pollut. Res. 23, 1768–1778. doi: 10.1007/s11356-015-5439-x
Mao, Q., Tang, L., Ji, W., Rennenberg, H., Hu, B., Ma, M. (2021). Elevated CO2 and soil mercury stress affect photosynthetic characteristics and mercury accumulation of rice. Ecotoxicol. Environ. Saf. 208, 111605. doi: 10.1016/j.ecoenv.2020.111605
Martínez-Trujillo, M., Méndez-Bravo, A., Ortiz-Castro, R., Hernández-Madrigal, F., Ibarra-Laclette, E., Ruiz-Herrera, L. F., et al. (2014). Chromate alters root system architecture and activates expression of genes involved in iron homeostasis and signaling in Arabidopsis thaliana. Plant Mol. Biol. 86, 35–50. doi: 10.1007/s11103-014-0210-0
Mohapatra, P. K., Sarkar, R. K., Kuanar, S. R. (2009). Starch synthesizing enzymes and sink strength of grains of contrasting rice cultivars. Plant Sci. 176, 256–263. doi: 10.1016/j.plantsci.2008.11.001
Nishi, A., Nakamura, Y., Tanaka, N., Satoh, H. (2001). Biochemical and genetic analysis of the effects of amylose-extender mutation in rice endosperm. Plant Physiol. 127, 459–472. doi: 10.1104/pp.010127.BEII
Oliveira, H. (2012). Chromium as an environmental pollutant: Insights on induced plant toxicity. J. Bot. 2012, 1–8. doi: 10.1155/2012/375843
Panhwar, Q. A., Naher, U. A., Radziah, O., Shamshuddin, J., Razi, I. M., Dipti, S. S., et al. (2015). Quality and antioxidant activity of rice grown on alluvial soil amended with zn, Cu and Mo. South Afr. J. Bot. 98, 77–83. doi: 10.1016/j.sajb.2015.01.021
Pérez-López, U., Robredo, A., Lacuesta, M., Sgherri, C., Muñoz-Rueda, A., Navari-Izzo, F., et al. (2009). The oxidative stress caused by salinity in two barley cultivars is mitigated by elevated CO2. Physiol. Plant 135, 29–42. doi: 10.1111/j.1399-3054.2008.01174.x
Rahman, S., Copeland, L., Atwell, B. J., Roberts, T. H. (2021). Elevated CO2 differentially affects the properties of grain from wild and domesticated rice. J. Cereal Sci. 100, 103227. doi: 10.1016/j.jcs.2021.103227
Rai, P. K., Lee, S. S., Zhang, M., Tsang, Y. F., Kim, K. H. (2019). Heavy metals in food crops: Health risks, fate, mechanisms, and management. Environ. Int. 125, 365–385. doi: 10.1016/j.envint.2019.01.067
Rosado-Porto, D., Ratering, S., Cardinale, M., Maisinger, C., Moser, G., Deppe, M., et al. (2021). Elevated atmospheric CO2 modifies mostly the metabolic active rhizosphere soil microbiome in the giessen FACE experiment. Microb. Ecol 83(3), 619–634. doi: 10.1007/s00248-021-01791-y
Saleh, A. M., Hassan, Y. M., Habeeb, T. H., Alkhalaf, A. A., Hozzein, W. N., Selim, S., et al. (2021). Interactive effects of mercuric oxide nanoparticles and future climate CO2 on maize plant. J. Hazard Mater. 401. doi: 10.1016/j.jhazmat.2020.123849
Saleh, A. M., Hassan, Y. M., Selim, S., AbdElgawad, H. (2019). NiO-nanoparticles induce reduced phytotoxic hazards in wheat (Triticum aestivum l.) grown under future climate CO2. Chemosphere 220, 1047–1057. doi: 10.1016/J.CHEMOSPHERE.2019.01.023
Sangwan, P., Kumar, V., Joshi, U. N. (2014). Effect of chromium (VI) toxicity on enzymes of nitrogen metabolism in clusterbean (Cyamopsis tetragonoloba l.). Enzyme Res. 2014. doi: 10.1155/2014/784036
Schwab, A. P., He, Y., Banks, M. K. (2005). The influence of organic ligands on the retention of lead in soil. Chemosphere 61, 856–866. doi: 10.1016/j.chemosphere.2005.04.098
Senthil-Nathan, S. (2021). Effects of elevated CO2 on resistant and susceptible rice cultivar and its primary host, brown planthopper (BPH), nilaparvata lugens (Stål). Sci. Rep. 11, 8905. doi: 10.1038/s41598-021-87992-4
Shabbaj, I. I., Abdelgawad, H., Balkhyour, M. A., Tammar, A., Madany, M. M. Y. (2022). Elevated CO2 differentially mitigated oxidative stress induced by indium oxide nanoparticles in young and old leaves of C3 and C4 crops. Antioxidants 11. doi: 10.3390/antiox11020308
Shahid, M., Shamshad, S., Rafiq, M., Khalid, S., Bibi, I., Niazi, N. K., et al. (2017). Chromium speciation, bioavailability, uptake, toxicity and detoxification in soil-plant system: A review. Chemosphere 178, 513–533. doi: 10.1016/j.chemosphere.2017.03.074
Sharkey, T. D., Laporte, M. M., Kruger, E. L. (2000). “Will increased photosynthetic efficiency lead to increased yield in rice?,” in Studies in plant science 7, 73–86. (Elsevier)
Sharma, D. C., Chatterjee, C., Sharma, C. P. (1995). Chromium accumulation and its effects on wheat (Triticum aestivum l. cv. HD 2204) metabolism. Plant Sci. 111, 145–151. doi: 10.1016/0168-9452(95)04230-R
Sharma, A., Kapoor, D., Wang, J., Shahzad, B., Kumar, V., Bali, A. S., et al. (2020). Chromium bioaccumulation and its impacts on plants: An overview. Plants 9. doi: 10.3390/plants9010100
Singh, D., Sharma, N. L., Singh, C. K., Kumar Sarkar, S., Singh, I., Lal Dotaniya, M. (2020). Effect of chromium (VI) toxicity on morpho-physiological characteristics, yield, and yield components of two chickpea (Cicer arietinum l.) varieties. PloS One 15. doi: 10.1371/journal.pone.0243032
Srivastava, D., Tiwari, M., Dutta, P., Singh, P., Chawda, K., Kumari, M., et al. (2021). Chromium stress in plants: Toxicity, tolerance and phytoremediation. Sustain 13. doi: 10.3390/su13094629
Sundaramoorthy, P., Chidambaram, A., Ganesh, K. S., Unnikannan, P., Baskaran, L. (2010). Chromium stress in paddy:(i) nutrient status of paddy under chromium stress;(ii) phytoremediation of chromium by aquatic and terrestrial weeds. C. R. Biol. 333, 597–607. doi: 10.1016/j.crvi.2010.03.002
Tchounwou, P. B., Yedjou, C. G., Patlolla, A. K., Sutton, D. J. (2012). Heavy metal toxicity and the environment. EXS 101, 133–164. doi: 10.1007/978-3-7643-8340-4_6
Verspreet, J., Pollet, A., Cuyvers, S., Vergauwen, R., den Ende, W., Delcour, J. A., et al. (2012). A simple and accurate method for determining wheat grain fructan content and average degree of polymerization. J. Agric. Food Chem. 60, 2102–2107. doi: 10.1021/jf204774n
Vitale, R. J., Mussoline, G. R., Rinehimer, K. A. (1997). Environmental monitoring of chromium in air, soil, and water. Regul. Toxicol. Pharmacol. 26, S80–S85. doi: 10.1006/rtph.1997.1144
Wakeel, A., Xu, M., Gan, Y. (2020). Chromium-induced reactive oxygen species accumulation by altering the enzymatic antioxidant system and associated cytotoxic, genotoxic, ultrastructural, and photosynthetic changes in plants. Int. J. Mol. Sci. 21. doi: 10.3390/ijms21030728
Wang, J., Liu, X., Zhang, X., Li, L., Lam, S. K., Pan, G. (2019). Changes in plant c, n and p ratios under elevated [CO2] and canopy warming in a rice-winter wheat rotation system. Sci. Rep. 9. doi: 10.1038/s41598-019-41944-1
Welsch, F. P. (1990). Trace elements determination of arsenic and selenium using continuous-flow hydride generation atomic absorption spectrophotometry (HG-AAS). Qual. Assur. Man. branch geochem., 38–45.
Yibo, C., Zhidong, W., Chongrong, W., Hong, L., Daoqiang, H., Degui, Z., et al. (2022). Comparisons of metabolic profiles for carbohydrates, amino acids, lipids, fragrance and flavones during grain development in indica rice cultivars. Rice Sci. 29, 155–165. doi: 10.1016/j.rsci.2022.01.004
Zhao, M., Lin, Y., Chen, H. (2020). Improving nutritional quality of rice for human health. Theor. Appl. Genet. 133, 1397–1413. doi: 10.1007/s00122-019-03530-x
Zhou, J., Chen, H., Tao, Y., Thring, R. W., Mao, J. (2019). Biochar amendment of chromium-polluted paddy soil suppresses greenhouse gas emissions and decreases chromium uptake by rice grain. J. Soils Sediments 19, 1756–1766. doi: 10.1007/s11368-018-2170-5
Keywords: chromium, rice, yield, pollution, stress, eCO2
Citation: AbdElgawad H, Mohammed AE, van Dijk JR, Beemster GTS, Alotaibi MO and Saleh AM (2023) The impact of chromium toxicity on the yield and quality of rice grains produced under ambient and elevated levels of CO2. Front. Plant Sci. 14:1019859. doi: 10.3389/fpls.2023.1019859
Received: 15 August 2022; Accepted: 26 January 2023;
Published: 07 March 2023.
Edited by:
Dr. Subhan Danish, Bahauddin Zakariya University, PakistanReviewed by:
Amardeep Singh Virdi, Amity University Punjab, IndiaDomancar Orona-Tamayo, Centro de Innovación Aplicada en Tecnologías Competitivas (CIATEC), Mexico
Copyright © 2023 AbdElgawad, Mohammed, van Dijk, Beemster, Alotaibi and Saleh. This is an open-access article distributed under the terms of the Creative Commons Attribution License (CC BY). The use, distribution or reproduction in other forums is permitted, provided the original author(s) and the copyright owner(s) are credited and that the original publication in this journal is cited, in accordance with accepted academic practice. No use, distribution or reproduction is permitted which does not comply with these terms.
*Correspondence: Ahmed M. Saleh, YXNhbGVoQHNjaS5jdS5lZHUuZWc=; Afrah E. Mohammed, YWZhbW9oYW1tZWRAcG51LmVkdS5zYQ==
†These authors have contributed equally to this work