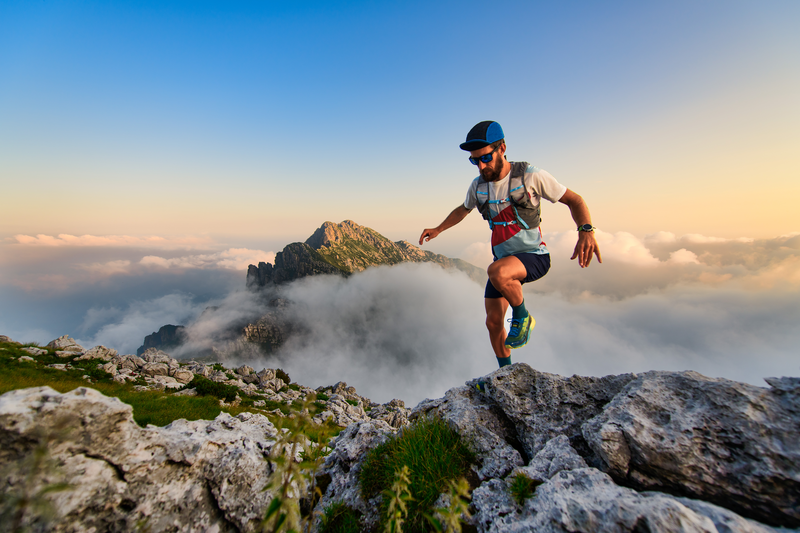
95% of researchers rate our articles as excellent or good
Learn more about the work of our research integrity team to safeguard the quality of each article we publish.
Find out more
ORIGINAL RESEARCH article
Front. Plant Sci. , 24 February 2023
Sec. Plant Abiotic Stress
Volume 14 - 2023 | https://doi.org/10.3389/fpls.2023.1018874
This article is part of the Research Topic Physiological, Biochemical and Molecular Approaches in Response to Abiotic Stresses in Plants View all 17 articles
Sucrose non-fermenting1-related protein kinase-2 (SnRK2) is a plant-specific protein kinase family and an important component of the abscisic acid (ABA) signaling pathway. However, there is a lack of relevant studies in blueberry (Vaccinium corymbosum). In this study, we identified six SnRK2 family members (from VcSnRK2.1 to VcSnRK2.6) in blueberries for the first time. In addition, we found that VcSnRK2.3 expression was not only positively correlated with fruit ripening but was also induced by ABA signaling. Transient expression in blueberry fruits also proved that VcSnRK2.3 promoted anthocyanin accumulation and the expression of anthocyanin synthesis-related genes such as VcF3H, VcDFR, VcANS, and VcUFGT. Transgenic Arabidopsis thaliana seeds and seedlings overexpressing VcSnRK2.3 showed anthocyanin pigmentation. Yeast two-hybrid assays (Y2H) and Bimolecular fluorescence complementation assays (BiFC) demonstrated that VcSnRK2.3 could interact with the anthocyanin positive regulator VcMYB1. Finally, VcSnRK2.3 was able to enhance the binding of VcMYB1 to the VcDFR promoter. Via regulation transcription of anthocyanin biosynthesis genes, VcSnRK2.3 promoted anthocyanin accumulation in blueberry. The above results suggest that VcSnRK2.3 plays an important role in blueberry anthocyanin synthesis, is induced by ABA, and can interact with VcMYB1 to promote anthocyanin biosynthesis in blueberry.
Blueberries (Vaccinium corymbosum, Ericaceae) are one of the most nutritious foods cultivated worldwide. In general, highbush blueberry (V. corymbosum L.) is considered to be a commercially important blueberry. Highbush blueberries include three types: southern highbush blueberry (SHB), half highbush blueberry (HHB), and northern highbush blueberry (NHB) (Yang et al., 2022). Blueberries are rich in flavonoids (mainly anthocyanins) and polyphenols (proanthocyanidins), which are recognized by the Food and Agriculture Organization of the United Nations (FAO) as one of the top five health foods for humans and have a promising commercial future. Anthocyanins have anti-cancer, anti-obesity, and anti-inflammatory effects, protect vision, prevent heart disease, improve immunity, and improve cognitive decline (Ma et al., 2018; Guan et al., 2021).
A change in fruit color is an important indicator of fruit maturity, which is mainly determined by anthocyanins, carotenoids and phenols (Ballester et al., 2010; Wang et al., 2020), and the anthocyanin concentration is a major factor that determines plant color. Anthocyanins are an important secondary metabolite of plants and are synthesized via the phenylalanine metabolic pathway (Allan et al., 2008; Jaakola, 2013; An et al., 2020a). Functionally, genes that affect anthocyanin biosynthesis are divided into two groups. The anthocyanin synthesis pathway’s essential structural genes make up one group, such as chalcone synthase (CHS), chalcone isomerase (CHI), lavanone 3-hydroxylase (F3H), dihydroflavonol 4-reductase (DFR), anthocyanidin synthase (ANS), and UDP-glucose flavonoid 3-O-glucosyltransferase (UFGT) (Boss et al., 1996; Ma et al., 2014). The other group consists of transcription factors that are not directly involved in anthocyanin synthesis but are used to regulate the expression of structural genes, such as MYB, bHLH and WD40, which usually form the transcriptional complex MYB-bHLH-WD40 (“MBW” transcription factor) (Petroni and Tonelli, 2011; Zhao et al., 2019). Previous studies have found that MYB75, MYB113 and MYB114 in Arabidopsis contribute to the accumulation of anthocyanins (Teng et al., 2005; Gonzalez et al., 2008; Chen et al., 2022). In apple, MdMYB1 and its alleles play important roles in anthocyanin biosynthesis (Ban et al., 2007; Espley et al., 2007). In recent studies, VcMYB1 was shown to function as a regulator of anthocyanin biosynthesis, and VcMYB1 positively regulates anthocyanin synthesis and accumulation by binding to the VcDFR and VcUFGT anthocyanin biosynthesis genes’ promoters (Tang et al., 2021; Zhang et al., 2021). In addition, it has been demonstrated that the transcription factors MYBA and MYBPA co-regulate anthocyanin biosynthesis in blueberries (Karppinen et al., 2021; Lafferty et al., 2022). Although many species’ anthocyanin synthesis pathways have been examined, and attempts have been made to identify their molecular mechanisms, few relevant studies have been carried out in blueberries.
Abscisic acid (ABA) is a sesquiterpenoid compound discovered in the 1960s (Bassaganya-Riera et al., 2010). In addition to being a crucial part in plant growth, development, and stress response, ABA is also a major regulator of ripening in non-climacteric fruits (Chen et al., 2020b; Zhang et al., 2021; Gupta et al., 2022). ABA has been shown to promote ripening and anthocyanin synthesis in many non-climacteric fruits, including strawberry, pear, sweet cherry, grape, cucumber, and blueberry, as well as climacteric fruits, such as tomato, peach, mango, and melon (Karppinen et al., 2018; Oh et al., 2018). Thus, fruit ripening is significantly influenced by ABA. In a recent study, silencing of VmNCED1, a key gene for ABA biosynthesis, resulted in down-regulation of the expression of key anthocyanin biosynthesis genes in blueberry (Karppinen et al., 2018). Inhibiting the expression of the essential ABA biosynthesis gene FaNCED1 in strawberries prevented ABA biosynthesis, and some fruit that was not colored could be supplemented with exogenous ABA, suggesting a molecular mechanism for the role of ABA in fruit ripening (Jia et al., 2011). Many transcription factors associated with anthocyanin accumulation and fruit ripening that are regulated by ABA have been discovered in studies. These transcription factors include MYB, bZIP, and bHLH (Shen et al., 2014; An et al., 2018; An et al., 2021). In apple, by regulating the MYB1-bHLH3 complex, ABI5 regulates the ABA-induced anthocyanin synthesis, and ABA treatment enhances the interaction between MdbHLH3 and MdMYB1 (An et al., 2021). ABA signaling plays an important regulatory role in fruit ripening, but the genes and key regulators involved in its downstream regulation of non-climacteric fruit ripening and anthocyanin accumulation are poorly understood.
Reversible phosphorylation of proteins catalyzed by protein phosphatases and protein kinases is the main mechanism of post-translational regulation of protein activity and intracellular signal transduction in eukaryotes (Halford and Hey, 2009). Sucrose non-fermenting1-related protein kinase-2 (SnRK2) is one of the protein kinases involved in signal transduction in adversity (Boudsocq and Laurière, 2005). In Arabidopsis, the SnRK2 family contains ten members named SnRK2.1 to SnRK2.10 (Hrabak et al., 2003). And there are ten SnRK2 members in Oryza sativa and Triticum aestivum. Fragaria ananassa contains nine, Solanum tuberosum contains eight and Zea mays contains eleven (Boudsocq et al., 2004; Kobayashi et al., 2004; Han et al., 2015; Zhang et al., 2016; Bai et al., 2017). Subsequently, in many species, members of the SnRK2 family have been discovered, including Lycopersicon esculentum (Sun et al., 2011), Vitis vinifera (Boneh et al., 2012), Malus prunifolia (Shao et al., 2014), Prunus avium (Shen et al., 2017), Musa acuminata (Hu et al., 2017), Pyrus bretschneideri (Chen et al., 2020a), Fragaria vesca (Zhang et al., 2020).
SnRK2 is involved in osmotic stress and the ABA response and plays an important role in stress signal transduction (Yoshida et al., 2006; Huai et al., 2008; Cutler et al., 2010; Fujii et al., 2011). Studies on Arabidopsis have demonstrated that ABA elicits cellular responses by binding to the ABA receptor pyrabactin resistance1/PYR1-like/regulatory components of the ABA receptor (PYR/PYL/RCAR). ABA binding to the receptor protein PYR1/PYL/RCAR disables the inhibition of SnRK2 kinase by PP2C phosphatase and thus activates SnRK2 (Cutler et al., 2010; Moreno-Alvero et al., 2017; Islam et al., 2021). Activated SnRK2 then phosphorylates and regulates its downstream targets such as bZIP-like transcription factor ABF, slow anion channel 1 (SLAC1) and the MAPK signaling cascade pathway (Fujita et al., 2009; Geiger et al., 2009; de Zelicourt et al., 2016). Furthermore, SnRK2 plays key roles in plant growth and development and a range of stress reactions (Fujii et al., 2011; Fujita et al., 2013), including the modulation of fruit development and ripening and anthocyanin biosynthesis. For example, in strawberry, virus-induced gene silencing of the ABA receptor FaPYR1 delayed ripening and significantly decreased critical ABA signaling molecules, such as ABI1, ABI3, ABI4, ABI5, and SnRK2s (Chai et al., 2011). By contrast, FaABI1 interference resulted in the overexpression of most genes associated with fruit ripening, including ABI3, SnRK2s, and AREB1 (Jia et al., 2013). Previous studies identified FaSnRK2.6 as a member of the SnRK2-III family, and FaSnRK2.6 was a negative regulator of strawberry fruit ripening and anthocyanin accumulation (Han et al., 2015; Jia et al., 2022). Dehydration stress in sweet cherry resulted the expression of all PacSnRK2 and PacPP2C genes, and it also promoted the accumulation of ABA and anthocyanin (Shen et al., 2017). In addition, FvSnRK2.6 was found to mediate low-temperature-regulated anthocyanin accumulation in strawberry fruit (Mao et al., 2022).
Blueberries are rich in anthocyanins and have a high nutritional value. In this study, we selected blueberry (V. corymbosum “Duke”) as experimental material and identified its SnRK2 gene family members. In addition, we explored the regulatory effect of exogenous ABA on VcSnRK2.3. We also sought to determine the regulatory effect of VcSnRK2.3 on blueberry and to assess whether this protein participates in the control of ripening and anthocyanin synthesis in blueberry fruit, particularly to determine its interaction with VcMYB1. Our data provide a novel mechanism for blueberry anthocyanin biosynthesis and could potentially lead to improved ripening practices.
Nine-year-old blueberry (V. corymbosum “Duke”) plants collected from the small berry garden of the Fruit Tree Research Institute, Chinese Academy of Agricultural Sciences were used as experimental material. Experiments were conducted from March 2019 to February 2022 at the Ministry of Agriculture Key Laboratory of horticultural crop germplasm resources utilization, Institute of Fruit Tree Research, Chinese Academy of Agricultural Sciences (Xingcheng, Liaoning, China) and College of Horticulture Sciences, Shandong Agricultural University (Tai’an, Shandong, China). To ensure the consistency of the material, fruits were collected from the top of the inflorescence (first to mature). Samples were taken from plants under the same growth conditions for organ (root, stem, leaf, flower, and fruit)-specific expression. All samples were rapidly frozen in liquid nitrogen and stored at −80°C.
Blueberry transcriptome data obtained from Illumina and SMRT sequencing were used to identify the gene family members of SnRK2 in blueberry (Tang et al., 2021). The excavated VcSnRK2 protein sequences were analyzed using the Basic Local Alignment Search Tool (BLAST) program (http://www.ncbi.nlm.nih.gov/BLAST/) in the National Center for Biotechnology Information (NCBI) database. The results showed that the six VcSnRK2 sequences had high homology with the SnRK2 protein sequences of Solanum tuberosum, Arabidopsis thaliana and Zea mays. The six genes were named according to the Phylogenetic Tree Construction. If VcSnRK2 clusters with Solanum tuberosum, it is named after the same number, including VcSnRK2.1, 2.3, 2.4, and 2.5. For VcSnRK2.2 and 2.6, which do not cluster as closely with Solanum tuberosum, they are named sequentially according to their position on the chromosome in sequence.
The amino acid secondary structure of VcSnRK2 was predicted using the Simple Modular Architecture Research Tool (SMART) software program (http://smart.emb-lheidelberg.de/) through three rounds of PCR. The deduced amino acid sequences of VcSnRK2s were aligned using CLC Sequence Viewer 6.0 software with the default settings.
RNA was extracted from different organs and different fruit stages of blueberry plants, and Arabidopsis leaves using TaKaRa MiniBEST Plant RNA Extration Kit (TaKaRa, Shiga, Japan). qRT-PCR was performed with VcACTIN as a reference gene. Arabidopsis polyubiquitin 10 (AtUBQ10) was used as a reference gene. Single-stranded cDNA was obtained using a reverse transcription kit (TaKaRa, Shiga, Japan). 4 μg RNA was used as a template for cDNA synthesis. The procedure for cDNA synthesis was to incubate the reaction solution at 65°C for five minutes, 42°C for 60 minutes, and 70°C for 5 minutes. qPCR assays were performed on a CFX Connect real-time PCR assay system (Bio-Rad, USA) using the SYBR Green I chimeric fluorescence assay. The amplification reaction procedure was as follows: Stage 1 pre-denaturation, the mixture was reacted at 95°C for 30 sec, one cycle; Stage 2 cyclic reaction, 95°C for 10 sec, 60°C for 30 sec, 40 cycles; Stage 3 melting curve, 95°C for 15 sec, 60°C for 60 sec, 95°C for 15 sec, one cycle. The qPCR results were all analyzed using the comparative CT method. Three biological replicates and three technical replicates were conducted. All primers used in this study are listed in Supplementary Table S1.
Extraction methods for anthocyanins were based on previous studies (Latti et al., 2010; Pertuzatti et al., 2021). Separation, identification, and quantification of anthocyanins by high-performance liquid chromatography (HPLC) was conducted on an Agilent 1100 Series system (Agilent, Germany), equipped with DAD (G1315B), and an LC/MSD Trap VL (G2445C VL) electrospray ionization mass spectrometry (ESIMSn) system that was coupled to an Agilent ChemStation (version B.01.03) data-processing station (Castillo-Munãoz et al., 2009). Mass spectra data were processed with Agilent LC/MS Trap software (version 5.3). ESI-MSn was used to determine the anthocyanin profiles and the following parameters were employed: positive ionization mode; dry gas, N2, 11 mL/min; drying temperature, 350°C; nebulizer, 65 psi; capillary, –2500 V; capillary exit offset, 70 V; skimmer1, 20 V; skimmer 2, 6 V; compound stability, 100%; scan range, 50–1,200 m/z (Pertuzatti et al., 2016). For the purposes of quantification, DAD chromatograms were extracted at 520 nm for anthocyanins.
Agrobacterium tumefaciens GV3101 transformed the pRI101-AN vector with the open reading frame (ORF) after it had been cloned and inserted. Transformation of Agrobacterium into Arabidopsis by flower dip method. On MS media containing 50 mg L−1 kanamycin, T1 VcSnRK2.3 transgenic plants were selected. Until seed set, kanamycin-resistant T1 seedlings were cultivated in a growth chamber at 22°C and a 16 h day length. Seeds of T1 plants were planted and germinated to obtain T2 seedlings. T2 seedlings were also selected on MS medium containing 50 mg L−1 kanamycin.
Blueberry fruit injection assays were performed with reference to previous studies (An et al., 2019; Tang et al., 2021). The open reading frame of the SnRK2.3 gene was cloned into the pGreenII62-SK vector to obtain the VcSnRK2.3-pGreenII62-SK vector. Blueberry peels were injected with the mixed vector and Agrobacterium solutions.
The Y2H experiment was performed according to Clontech’s instructions. The ORF, N-terminus, and C-terminus of VcSnRK2.3 and the ORF of MdMYB1 were inserted into pGAD and pGBD vectors. The plasmids were co-transformed into yeast strain Y2H Gold (TaKaRa, Beijing, China) by using the lithium acetate method with reference to Veries et al. For transformation, 4-5 μl of plasmid and 50 μg of denatured salmon sperm vector DNA are mixed. Then 500 μl of polyethylene glycol (PEG) lithium acetate solution is added. After incubation 20 μl DMSO is added and incubated again. The transformed yeast strains were grown on medium lacking Leucine and Tryptophan (SD-L/-T), Leucine, Tryptophan, Histidine and Adenine (SD-L/-T/-H/-A) or Leucine, Tryptophan, Histidine, Adenine and X-gal (SD-T/-L/-H/-A+X-gal) at 28°C for 3 days.
The ORF and C-terminus of VcSnRK2.3 and the ORF of MdMYB1 were inserted into YFPC or YFPN to generate the VcSnRK2.3-C-YFPC, VcSnRK2.3-YFPC and VcMYB1-YFPN plasmids. The constructs were transformed into Agrobacterium GV3101. The specified plasmid-carrying Agrobacterium solution was incubated with prepared tobacco leaves for 30 minutes. After 2 days of darkness at 24°C, the transformed tobacco leaves were observed under a Confocal microscope (Zeiss LSM 780, Lena, Germany). The excitation wavelength of YFP is 510 nm, and the emission wavelength is 527 nm.
VcDFR promoter sequences were obtained from pre-lab data (Tang et al., 2021). Tobacco transient expression assays were carried out according to previous studies (Shang et al., 2010; An et al., 2017). The VcDFRpro : Luc reporter construct was created by amplifying the VcDFR promoter and cloning it into the pGreenII0800-LUC vector. The ORFs of VcMYB1 and VcSnRK2.3 were cloned into the pGreenII62-SK vector to generate 35Spro : VcMYB1 and 35Spro : VcSnRK2.3, respectively. Transformed leaves were sprayed with 100 mM luciferin and placed in the dark for 5 minutes before being examined for luminescence. The LUC pictures were collected using a charge-coupled device-imaging apparatus (NightOWL LB983 in conjunction with Indigo software).
Effector constructs were generated from Agrobacterium GV3101 strains containing the pRI101-VcSnRK2.3 and pRI101-VcMYB1 genes. The reporter constructs were generated using the promoter sequences of VcDFR cloned upstream of the β-glucuronidase (GUS) reporter gene in the pCAMBIA1301 vector. Agrobacterium GV3101 strains carrying pRI101-VcSnRK2.3 and pRI101-VcMYB1 were co-injected into the abaxial surface of tobacco leaves. Infected leaves were grown in growth chambers for 3-4 days and then analyzed for GUS activity. Proteins were extracted from infected leaves and fluorescence was measured with a fluorometer (VersaFluor Fluorometer, Bio-Rad) (http://www.bio-rad.com) with reference to Jefferson et al., 1987 and Bowling et al., 1994.
Based on the blueberry transcriptome data, we obtained six blueberry SnRK2 gene family members, named VcSnRK2.1 to VcSnRK2.6. We analyzed the physicochemical properties of the blueberry SnRK2 gene family members using the online software ProtParam. Table 1 shows that the amino acid lengths of the six blueberry SnRK2 proteins, ranging from 340 (VcSnRK2.1) AAs to 362 (VcSnRK2.3) AAs, and the molecular weights ranged from 38.56 kDa (VcSnRK2.1) to 41.04 (VcSnRK2.3) kDa. In addition, the pI was in the range of 4.75 (VcSnRK2.6) to 5.62 (VcSnRK2.4). To investigate the structural features of the SnRK2 protein sequences, we conducted a comparative analysis of the amino acid sequences of the blueberry SnRK2 proteins (Figure 1A). The results showed that all family members contain ATP binding domains and a protein kinase activation loop and were relatively conserved (Boudsocq et al., 2004; Han et al., 2015).
Figure 1 Sequence alignments and phylogenetic analysis of SnRK2.3. (A) Sequence alignments of the deduced amino acid sequences of VcSnRK2.1 to VcSnRK2.6. The same color shading indicates the same amino acid residues. The deduced amino acid sequences of VcSnRK2s were aligned using CLC Sequence Viewer 6 software with the default settings. The predicted functional domains are boxed. (B) Phylogenic analysis of VcSnRK2 and its homologs in Arabidopsis, Solanum tuberosum and Zea mays. VcSnRK2.3 is denoted by the black spot. SnRK2 of Arabidopsis, Solanum tuberosum and Zea mays (AtSnRK2.1: AT5G08590.1; AtSnRK2.2: AT3G50500.2; AtSnRK2.3: AT5G66880.1; AtSnRK2.4: AT1G10940.2; AtSnRK2.5: AT5G63650.1; AtSnRK2.6: AT4G33950.1; AtSnRK2.7: AT4G40010.1; AtSnRK2.8: AtSnRK2.9: AT2G23030.1; AtSnRK2.10: AT1G60940.1; StSnRK2.1: JX280911; StSnRK2.1: JX280911; StSnRK2.2: JX280912; StSnRK2.3: JX280913; StSnRK2.4: JX280914; StSnRK2.5: JX280915; StSnRK2.6: JX280916; StSnRK2.7: JX280917; StSnRK2.8: JX280918; ZmSnRK2.1: EU676033.1; ZmSnRK2.2: EU676034.1; ZmSnRK2.3: EU676035.1; ZmSnRK2.4: EU676036.1; ZmSnRK2.5: EU676037.1; ZmSnRK2.6: EU676038.1; ZmSnRK2.7: EU676039.1; ZmSnRK2.8: EU676040.1; ZmSnRK2.10: EU676041.1; ZmSnRK2.11: EU676042.1).
In addition, to investigate the evolutionary relationship between the blueberry SnRK2 gene and SnRK2 members in other plants, we downloaded the entire SnRK2 gene sequence from the NCBI website for Arabidopsis, Solanum tuberosum, and Zea mays. The Protein BLAST program was used to obtain homologs of Arabidopsis, Solanum tuberosum and Zea mays SnRK2. The evolutionary tree was constructed in MEGA-X software using neighbor- joining, which contained a total of 34 SnRK2 protein sequences. Based on the evolutionary results, VcSnRK2.3 had the highest similarity to StSnRK2.3 and AtSnRK2.6 (Figure 1B).
To analyze the expression of each member of the blueberry VcSnRK2 subfamily at different fruiting stages, we examined the expression of VcSnRK2 during the green, pink, and blue fruit stages of blueberry using quantitative real-time PCR analysis (qRT-PCR). According to Figure 2A, the expression of VcSnRK2.3 increased significantly when blueberry fruit ripened from the green fruit stage, indicating a possible positive regulatory relationship between VcSnRK2.3 and blueberry fruit ripening. In contrast, the expression of VcSnRK2.4 decreased significantly with fruit ripening, and the expression of VcSnRK2.1 and VcSnRK2.5 decreased slightly. For VcSnRK2.2 and VcSnRK2.6, we did not observe any correlation with fruit ripening. Thus, we hypothesized that VcSnRK2.3 could positively regulate the ripening of blueberry fruits. And further experiments were designed to verify its function.
Figure 2 Temporospatial pattern of VcSnRK2 expression in blueberry fruits. (A) Quantitative real-time PCR (qRT-PCR) analysis of VcSnRK2.1 to VcSnRK2.6 expression in blueberry fruits at different developmental stages. Green fruits (40 days after flowering), pink fruits (65 days after flowering), and blue fruits (80 days after flowering). The qRT-PCR was performed with VcGAPDH as a reference gene. Different letters above the bars indicate a significant difference based on one-way ANOVA (P < 0.05). Values are the means + SD of three biological replicates. (B) qRT-PCR analysis of VcSnRK2.3 expression in different organs of blueberry plants. Total RNA was isolated from organs (roots, stems, leaves, flowers and fruits). The qRT-PCR was performed with VcGAPDH as a reference gene. Values are the means + SD of three biological replicate.
Therefore, we investigated the distribution of VcSnRK2.3 in different organs of blueberry, and qRT-PCR analysis was carried out on sample materials. The results showed that VcSnRK2.3 genes were expressed in roots, stems, leaves, flowers and fruits. The expression of VcSnRK2.3 was stem > fruit > root > leaf > flower (Figure 2B). Because the relative expression of VcSnRK2.3 was high in blueberry stems and fruits, we speculated that SnRK2.3 may play a relatively important role in stems and fruits.
ABA has been shown to promote anthocyanin accumulation during blueberry fruit ripening. In our study, ABA treatment promoted the synthesis of blueberry anthocyanins. The color of blueberry fruits gradually changed from green to pink as the treatment continued within the 24 h period (Figure 3A). In addition, when compared to the control (CK) treatment, the anthocyanin content of ripen blueberry fruits was considerably higher following ABA treatment (Figure 3B). To investigate the response of blueberry VcSnRK2.3 to ABA, we measured the expression of VcSnRK2.3 after ABA treatment of blueberry fruits. As shown in Figure 3C, the control (CK) treatment had no significant effect on the expression of VcSnRK2.3 in fruits. The expression of VcSnRK2.3 increased gradually and then decreased slightly with the increase in the ABA treatment time, and the highest value at 12 h was 2.8 times that of the CK treatment (Figure 3C). The results indicated that VcSnRK2.3 was strongly induced by ABA, which is in line with other discoveries (Boudsocq et al., 2004; Kulik et al., 2011).
Figure 3 ABA and CK treatment in blueberry fruits. (A) Isolated blueberry fruits were treated with 100 μM ABA immersion at 0, 3, 6, 12, and 24 h. CK indicates the negative control (no treatment). Scale bar = 8 mm. (B) Anthocyanin contents of ripe blueberry fruits. Different letters above the bars indicate a significant difference based on a t-test (P < 0.05). Values are the means + SD of three biological replicates. (C) qRT-PCR analysis of VcSnRK2.3 relative expression in blueberry fruits. Different letters above the bars indicate a significant difference based on a t-test (P < 0.05). Values are the means + SD of three biological replicates.
In strawberry, fruit ripening and anthocyanin accumulation were shown to be negatively regulated by FaSnRK2.6 (Han et al., 2015). To study the function of VcSnRK2.3 and validate its effect on anthocyanin biosynthesis, we carried out heterologous expression in Arabidopsis (ecotype Columbia) under CaMV-35S control. Compared with the wild-type (WT), transgenic Arabidopsis had red pigmentation in seeds 8–10 days after flowering (Figure 4A). The cotyledons and hypocotyls of Arabidopsis seedlings grown from the above seeds were also pigmented (Figure 4B). The total anthocyanin content of WT Arabidopsis was 1.513 mg/kg, while the mean value for total anthocyanin contents of VcSnRK2.3 Arabidopsis (VcSnRK2.3-L1, L2) was 119.91 mg/kg (Figure 4C). In addition, RNA was extracted from the above plants and examined using qRT-PCR. The results demonstrated that overexpression of VcSnRK2.3 up-regulated the expression of primary genes involved in the flavonoid biosynthetic pathway, including AtPAP1, At4CL, AtCHS, AtCHI, AtDFR and AtANS (Figure 4D). Among them, the transgenic Arabidopsis plants had the highest expression of structural genes AtDFR and AtANS.
Figure 4 Functional analysis of VcSnRK2.3 in a heterologous system. (A) Seeds of Arabidopsis thaliana (ecotype Columbia) plants wild-type (WT) and overexpressing 35S:VcSnRK2.3 (VcSnRK2.3-L1, L2). Scale bar = 1 mm. (B) Arabidopsis plants wild-type and overexpressing 35S:VcSnRK2.3 (VcSnRK2.3-L1, L2). Scale bar = 5 mm. (C) Anthocyanin contents of Arabidopsis plants overexpressing 35S:VcSnRK2.3 (VcSnRK2.3-L1, L2) and the wild-type. Different asterisks above the bars indicate significant differences based on a t-test (P < 0.01). Values are the means + SD of three biological replicates. (D) Relative expression levels of VcSnRK2.3, AtPAP1, AtPAL, At4CL, AtCHS, AtCHI, AtDFR and AtANS in Arabidopsis plants overexpressing 35S:VcSnRK2.3 (VcSnRK2.3-L1, L2) and the wild-type. All values were calculated based on the housekeeping gene AtUBQ10. Different asterisks above the bars indicate significant differences based on a t-test (P < 0.01). Values are the means + SD of three biological replicates.
To further demonstrate the function of VcSnRK2.3, we carried out Agrobacterium-mediated transient transformation assay in blueberry fruits 40 days after flowering. The control was the pGreenII62-SK empty vector, and the experimental group was the VcSnRK2.3-pGreenII62-SK vector (VcSnRK2.3-L1, L2 and L3). The results showed that overexpression of VcSnRK2.3 facilitated anthocyanin accumulation in blueberry injection sites (Figure 5A). And the phenotype can be observed two days after gene overexpression and can last until fruit ripening. The total anthocyanin content, including the levels of delphinidin, cyanidin, petunidin, peonidin, and malvidin, was significantly increased (Figure 5B). In addition, compared with the pGreenII62-SK vector, the expression levels of VcMYB1 and anthocyanin genes such as VcF3H, VcDFR, VcANS, and VcUFGT were considerably increased by the overexpression of VcSnRK2.3 (Figure 5C). Therefore, based on the above two experiments, we might infer that VcSnRK2.3 can promote not only anthocyanin biosynthesis but also the expression of VcMYB1.
Figure 5 Functional analysis of VcSnRK2.3 in blueberry. (A) Blueberry fruits overexpressing pGreenII62-SK and VcSnRK2.3-pGreenII62-SK (VcSnRK2.3-L1, L2 and L3). Scale bar = 5 mm. (B) Anthocyanin contents of fruits overexpressing VcSnRK2.3-pGreenII62-SK (VcSnRK2.3-L1, L2 and L3) and pGreenII62-SK. Different English letters above the bars indicate a significant difference based on a t-test (P < 0.05). Values are the means + SD of three biological replicates. (C) Relative expression levels of VcSnRK2.3, VcMYB1, VcF3H, VcDFR, VcANS and VcUFGT in overexpressed VcSnRK2.3-pGreenII62-SK (VcSnRK2.3-L1, L2 and L3) and pGreenII62 -SK fruits. The qRT-PCR served as a reference gene with VcGAPDH. Different letters above the bars indicate a significant difference based on a t-test (P < 0.05). Values are the means + SD of three biological replicates.
The above results demonstrated that VcSnRK2.3 can promote VcMYB1 expression and anthocyanin accumulation in blueberry fruits (Figure 5). Therefore, Y2H assays were carried out to confirm the interaction between VcSnRK2.3 and VcMYB1. After transforming VcMYB1-pGBD and VcSnRK2.3-pGAD into yeast cells, the results showed that VcMYB1-pGBD and VcSnRK2.3-pGAD as well as VcMYB1-pGBD and VcSnRK2.3-N-pGAD were able to grow on SD/-Leu-Trp -His-Ade medium (Figure 6A). This indicated that VcSnRK2.3 interacts with VcMYB1 in yeast cells, and the action site is located at the N-terminus of VcSnRK2.3. In addition, the interaction between VcSnRK2.3 and VcMYB1 was tested by BiFC assay. As shown in Figure 6B, when VcSnRK2.3-YFPC and VcMYB1-YFPN were co-expressed, the YFP fluorescent signals could be detected. In contrast, other combinations such as VcSnRK2.3-YFPC + YFPN, YFPC + VcMYB1- YFPN, VcSnRK2.3-C-YFPC + VcMYB1-YFPN, and VcSnRK2.3-C-YFPC + YFPN did not detect fluorescent signals (Figure 6B). Therefore, it was further demonstrated that VcSnRK2.3 and VcMYB1 interacted with each other.
Figure 6 VcSnRK2.3 interacts with VcMYB1. (A) Yeast two-hybrid assays. The N-terminus of VcSnRK2.3 interacts specifically with VcMYB1. Yeast grown in SD-Leu/-Trp (-L/-T) medium, SD-Leu/-Trp/-His/-Ade (-L/-T/-H/-A) medium or SD-Trp/-Leu/-His/-Ade supplemented with X-gal (-T/-L/-H/-A+X-gal) medium. (B) Bimolecular fluorescence complementation assays. The open reading frames of VcSnRK2.3 and VcMYB1 were fused to the C-terminus part of YFP and the N-terminus part of YFP. Scale bar = 10 µm. A representative image was presented after this experiment was carried out three times with similar results.
DFR plays a crucial role in the biosynthesis of anthocyanins. When the expression level of DFR increased, the anthocyanin content in plants increased (An et al., 2020b). A previous study showed that MdMYB1 promotes the synthesis of anthocyanins by directly binding to the promoter sequences of MdDFR and MdUF3GT (An et al., 2018). To verify the action of the VcDFR promoter by VcSnRK2.3 and VcMYB1, we carried out firefly luciferase complementation assay (Figure 7A). The results showed that the luciferase signal was highest when the VcDFR promoter was co-injected with VcSnRK2.3 and VcMYB1, and the signal was second highest when the VcDFR promoter was co-injected with VcMYB1. Both were significantly higher than the luciferase signal of the control group (Figure 7B).
Figure 7 Regulation of VcDFRpro by VcMYB1 and VcSnRK2.3. (A) Firefly luciferase complementation assay analysis showed that VcSnRK2.3 and VcMYB1 can activate VcDFR promoter activity in tobacco (Nicotiana tabacum) leaves. (a) VcDFRpro : Luc+35Spro : VcMYB1+ 35Spro : VcSnRK2.3, (b) VcDFRpro : Luc+35Spro : VcSnRK2.3, (c) VcDFRpro : Luc +35Spro:empty, (d) VcDFRpro : Luc+35Spro : VcMYB1. Scale bar = 10 mm. (B) Quantitative analysis of luminescence intensity. The value for column c (VcDFRpro : Luc+35Spro:empty) was set to 1. Different asterisks above the bars indicate significant differences based on a t-test (**P < 0.01). Values are the means + SD of three biological replicates. (C) Analysis of GUS activity. Effector vectors containing VcSnRK2.3 and VcMYB1 and a reporter vector containing the VcDFR promoter were co-injected into wild-type tobacco leaves. VcSnRK2.3 and VcMYB1 can activate VcDFR promoter activity. Co-injection of VcSnRK2.3 and VcMYB1 largely increased the activity of the VcDFR promoter. Empty vector used as the reference. Different asterisks above the bars indicate significant differences based on a t-test (**P < 0.01, *P < 0.05). Values are the means + SD of three biological replicates.
In a different assay, as shown in Figure 7C, the VcDFR promoter region was fused to the GUS gene as a reporter marker. The GUS activity was highest when the VcDFR promoter was co-injected with VcSnRK2.3 and VcMYB1. And the GUS activity was also significantly higher than control when the VcDFR promoter was injected with VcSnRK2.3 or VcMYB1 alone. These results suggested that the presence of VcSnRK2.3 up-regulated the binding of VcMYB1 to the target promoter and activated the transcription of the anthocyanin biosynthesis genes.
Blueberry fruits contain a large amount of anthocyanins, which are not only beneficial to human health but also improve the tolerance of plants to oxidative and drought stresses, but the biosynthesis and metabolic mechanisms of blueberry anthocyanin biosynthesis are poorly studied (Chalker-Scott, 1999; Kalt et al., 2020). Moreover, in previous studies on genes related to anthocyanin synthesis in blueberries, most of them focused on the “MBW” transcription factor and paid less attention to other genes. In this study, we identified a novel signaling component VcSnRK2.3 that is strongly induced by ABA signaling and acts as a positive regulator of anthocyanin synthesis.
SnRK2 is a family of plant-specific protein kinases, and its members are widely distributed in plants. In this study, we identified six SnRK2 members from blueberry, and the evolutionary results showed that blueberry SnRK2 family members are divided into three subfamilies as in Arabidopsis, Solanum tuberosum, and Zea mays (Figure 1B). OST1 is closely associated with osmotic stress in plants such as Arabidopsis and Zea mays and enhances plant drought tolerance mainly by promoting stomatal closure and reducing the rate of plant water loss (Boudsocq et al., 2004; Huai et al., 2008). In this study, we found that VcSnRK2.3 has high homology with OST1. Previous studies have shown that FaSnRK2.6, PacSnRK2, and FvSnRK2.6 can be induced by drought and temperature signals to mediate anthocyanin synthesis in fruits (Han et al., 2015; Shen et al., 2017; Mao et al., 2022). Therefore, in this study, VcSnRK2.3 was overexpressed in Arabidopsis, and the results showed that the total anthocyanin content as well as anthocyanin synthesis genes increased significantly (Figure 4). This suggested that VcSnRK2.3 can regulate anthocyanin accumulation in Arabidopsis.
In plant like apple and blueberry, the MYB1 gene has been found to promote fruit ripening and anthocyanin accumulation. It also enhances the expression of genes involved in anthocyanin synthesis, such as DFR, ANS, and UFGT (An et al., 2018; Tang et al., 2021). In our study, transient injection of VcSnRK2.3 in blueberry fruits was able to increase the anthocyanin content and expression of genes involved in the process of anthocyanin synthesis, including VcF3H, VcDFR, VcANS, and VcUFGT (Figure 5). Interestingly, transient injection also increased the expression of MYB1. Therefore, we hypothesized that the promotion effect of VcSnRK2.3 on VcMYB1 expression is a branch of the blueberry anthocyanin biosynthetic pathway, and there is an interaction between VcSnRK2.3 and VcMYB1.
Most previous studies focused on the interaction of SnRK2 with upstream signals of the ABA signaling pathway, while there are few studies on downstream transcription factors and targets. In strawberry plants, FvMAPK3 activated acted as a downstream target of FvSnRK2.6, phosphorylating FvMYB10 and reducing its transcriptional activity (Mao et al., 2022). Therefore, in this study, we explored the interaction of VcSnRK2.3 with MYB1, a positive regulator of anthocyanin biosynthesis, as a way to enrich the mechanism of blueberry anthocyanin synthesis. Yeast two-hybrid and bimolecular fluorescence complementation analyses showed that the ATP binding domain or protein kinase activation domain of the N-terminal, the VcSnRK2.3 protein could interact with the MYB1 gene (Figure 6). This provided us with a new idea of anthocyanin biosynthesis in blueberry and enriches the network of anthocyanin synthesis in blueberry. In our study, MYB1 transcription factor has been shown to interact with VcSnRK2.3 to positively regulate blueberry anthocyanin synthesis. However, the mechanism by which both regulate downstream anthocyanin synthesis-related structural genes is unclear. Therefore, we conducted further experiments to demonstrate this process.
Previous studies have shown that VcMYB1 can bind to the VcDFR promoter and directly control the expression of structural genes, thus, promoting anthocyanin biosynthesis (Tang et al., 2021). In blueberries overexpressing VcSnRK2.3, the DFR gene was the most differentially expressed of the anthocyanin synthesis-related genes compared to the wild type. (Figure 5C). Therefore, we hypothesized that DFR structural genes play a major role in regulating anthocyanin synthesis in blueberry fruits. The results showed that fluorescence and higher GUS activity were detected in the presence of VcMYB1 alone, demonstrating that VcMYB1 can promote anthocyanin synthesis by initiating the expression of VcDFR. More importantly, the fluorescence effect and GUS activity were more significant when VcSnRK2.3 and VcMYB1 were co-expressed (Figure 7). The reason is that the presence of VcSnRK2.3 enhanced the binding of VcMYB1 to the target promoter, thus increasing the transcriptional activity of the anthocyanin biosynthesis structural gene. In the study of MdWRKY40 and MdERF38, MdMYB1 not only bound to the MdDFR and MdUF3GT promoter regions, but the presence of MdWRKY40 and MdERF38 enhanced the binding of MdMYB1 to its target promoter (An et al., 2019; An et al., 2020a). This may be similar to the regulatory model of this study, where the presence of an exogenous gene regulates the transcriptional activity of MYB on the target genes, thus ultimately promoting anthocyanin accumulation in the plants.
The three key ABA receptor proteins PYR/PYL/PCAR, PP2C, and SnRK2, as well as the downstream transcription factors, make up the ABA signaling pathway. This is the more well-known signaling pathway ABA-PYR/PYL/RCARs-PP2Cs-SnRK2s (Li et al., 2016; Wu et al., 2016). In this study, we investigated the effect of VcSnRK2.3 overexpression on blueberry anthocyanin biosynthesis and its interaction with VcMYB1 in an attempt to find a novel pathway to regulate anthocyanin anabolism in blueberry. Previous studies on anthocyanin synthesis have focused on transcription factors such as MYB and bHLH and less on ABA signal transduction pathway genes. Therefore, through this study, we investigated the ABA signaling pathway gene SnRK2, thus broadening our understanding of genes related to anthocyanin synthesis. In addition, our study has enriched the knowledge of the ripening process of blueberry fruits and laid the foundation for elucidating the ripening mechanism of non-climacteric plants and other fruit trees. ABA signaling pathway genes have been identified in many plants, but there are few studies on the effects of SnRK2 downstream targets and transcription factors on anthocyanin metabolism, which may be further explored in the future.
In conclusion, we identified a new protein VcSnRK2.3 in blueberries that is involved in the regulation of blueberry anthocyanin biosynthesis. VcSnRK2.3 expression not only positively correlated with fruit ripening but could also be induced by ABA signaling. In addition, VcSnRK2.3 can interact with VcMYB1 to promote anthocyanin accumulation by enhancing the binding of VcMYB1 to the VcDFR promoter.
The datasets presented in this study can be found in online repositories. The names of the repository/repositories and accession number(s) can be found below: https://www.ncbi.nlm.nih.gov/, OP626311 OP626312 OP626313 OP626314 OP626315 OP626316.
YS conceived and designed the experiments. XW, QT and YS performed the research. XW, QT, YS, FC, HL, and HZ analyzed the data. YS and XW wrote the paper. All authors contributed to the article and approved the submitted version.
This study was supported by the Central Public-interest Scientific Institution Basal Research Fund (No.Y2022PT15), the Chinese Academy of Agricultural Sciences-Agricultural Science and Technology Innovation Program (CAAS-ASTIP).
We appreciate the experimental assistance provided by Xiu-hong An (Hebei Agricultural University).
The authors declare that the research was conducted in the absence of any commercial or financial relationships that could be construed as a potential conflict of interest.
The reviewer XL declared a shared affiliation with the authors to the handling editor at the time of the review.
All claims expressed in this article are solely those of the authors and do not necessarily represent those of their affiliated organizations, or those of the publisher, the editors and the reviewers. Any product that may be evaluated in this article, or claim that may be made by its manufacturer, is not guaranteed or endorsed by the publisher.
The Supplementary Material for this article can be found online at: https://www.frontiersin.org/articles/10.3389/fpls.2023.1018874/full#supplementary-material
Allan, A. C., Hellens, R. P., Laing, W. A. (2008). MYB transcription factors that colour our fruit. Trends. Plant Sci. 13, 99–102. doi: 10.1016/j.tplants.2007.11.012
An, J. P., Qu, F. J., Yao, J. F., Wang, X. N., You, C. X., Wang, X. F., et al. (2017). The bZIPtranscription factor MdHY5 regulates anthocyanin accumulation and nitrate assimilation in apple. Hortic. Res. 4, 17023. doi: 10.1038/hortres.2017.23
An, J. P., Wang, X. F., Zhang, X. W., Xu, H. F., Bi, S. Q., You, C. X., et al. (2020b). An apple MYB transcription factor regulates cold tolerance and anthocyanin accumulation and undergoes MIEL1-mediated degradation. Plant Biotechnol. J. 18, 337–353. doi: 10.1111/pbi.13201
An, J. P., Yao, J. F., Xu, R. R., You, C. X., Wang, X. F., Hao, Y. J. (2018). Apple bZIP transcription factor MdbZIP44 regulates abscisic acid-promoted anthocyanin accumulation. Plant Cell Environ. 41, 2678–2692. doi: 10.1111/pce.13393
An, J. P., Zhang, X. W., Bi, S. Q., You, C. X., Wang, X. F., Hao, Y. J. (2020a). The ERF transcription factor MdERF38 promotes drought stress-induced anthocyanin biosynthesis in apple. Plant J. 101, 573–589. doi: 10.1111/tpj.14555
An, J. P., Zhang, X. W., Liu, Y. J., Wang, X. F., You, C. X., Hao, Y. J. (2021). ABI5 regulates ABA-induced anthocyanin biosynthesis by modulating the MYB1-bHLH3 complex in apple. J. Exp. Bot. 72, 1460–1472. doi: 10.1093/jxb/eraa525
An, J. P., Zhang, X. W., You, C. X., Bi, S. Q., Wang, X. F., Hao, Y. J. (2019). MdWRKY40 promotes wounding-induced anthocyanin biosynthesis in association with MdMYB1 and undergoes MdBT2-mediated degradation. New Phytol. 224, 380–395. doi: 10.1111/nph.16008
Bai, J., Mao, J., Yang, H., Khan, A., Fan, A., Liu, S., et al. (2017). Sucrose non-ferment 1 related protein kinase 2 (SnRK2) genes could mediate the stress responses in potato (Solanum tuberosum l.). BMC Genet. 18, 41. doi: 10.1186/s12863-017-0506-6
Ballester, A. R., Molthoff, J., de Vos, R., Hekkert, B., Orzaez, D., Fernández-Moreno, J. P., et al. (2010). Biochemical and molecular analysis of pink tomatoes: Deregulated expression of the gene encoding transcription factor SlMYB12 leads to pink tomato fruit color. Plant Physiol. 152, 71–84. doi: 10.1104/pp.109.147322
Ban, Y., Honda, C., Hatsuyama, Y., Igarashi, M., Bessho, H., Moriguchi, T. (2007). Isolation and functional analysis of a MYB transcription factor gene that is a key regulator for the development of red coloration in apple skin. Plant Cell Physiol. 48, 958–970. doi: 10.1093/pcp/pcm066
Bassaganya-Riera, J., Skoneczka, J., Kingston, D., Krishnan, A., Misyak, S., Guri, A., et al. (2010). Mechanisms of action and medicinal applications of abscisic acid. Curr. Med. Chem. 17, 467–478. doi: 10.2174/092986710790226110
Boneh, U., Biton, I., Schwartz, A., Ben-Ari, G. (2012). Characterization of the ABA signal transduction pathway in vitis vinifera. Plant Sci. 187, 89–96. doi: 10.1016/j.plantsci.2012.01.015
Boss, P. K., Davies, C., Robinson, S. P. (1996). Analysis of the expression of anthocyanin pathway genes in developing vitis vinifera l. cv Shiraz grape berries and the implications for pathway regulation. Plant Physiol. 111, 1059–1066. doi: 10.1104/pp.111.4.1059
Boudsocq, M., Barbier-Brygoo, H., Laurière, C. (2004). Identification of nine sucrose nonfermenting 1-related protein kinases 2 activated by hyperosmotic and saline stresses in arabidopsis thaliana. J. Biol. Chem. 279, 41758–41766. doi: 10.1074/jbc.M405259200
Boudsocq, M., Laurière, C. (2005). Osmotic signaling in plants: Multiple pathways mediated by emerging kinase families. Plant Physiol. 138, 1185–1194. doi: 10.1104/pp.105.061275
Bowling, S. A., Guo, A., Cao, H., Gordon, A. S., Klessig, D. F., Dong, X. (1994). A mutation in arabidopsis that leads to constitutive expression of systemic acquired resistance. Plant Cell. 6, 1845–1857. doi: 10.1105/tpc.6.12.1845
Castillo-Munãoz, N., Goímez-Alonso, S., Garciía-Romero, E., Gómez, M. V., Velders, A. H., Hermosiín-Gutieírrez, I. (2009). Flavonol 3-o-glycosides series of vitis vinifera cv. Petit verdot red wine grapes. J. Agric. Food Chem. 57, 209–219. doi: 10.1021/jf802863g
Chai, Y. M., Jia, H. F., Li, C. L., Dong, Q. H., Shen, Y. Y. (2011). FaPYR1 is involved in strawberry fruit ripening. J. Exp. Bot. 62, 5079–5089. doi: 10.1093/jxb/err207
Chalker-Scott, L. (1999). Environmental significance of anthocyanins in plant stress responses. Photochem. Photobiol. 70, 1–9. doi: 10.1111/j.1751-1097.1999.tb01944.x
Chen, K., Li, G. J., Bressan, R. A., Song, C. P., Zhu, J. K., Zhao, Y. (2020b). Abscisic acid dynamics, signaling, and functions in plants. J. Integr. Plant Biol. 62, 25–54. doi: 10.1111/jipb.12899
Chen, R. C., Mao, L. C., Guan, W. L., Wei, X. B., Huang, Z. H., Wu, Y. Y. (2022). ABA-mediated miR5290 promotes anthocyanin biosynthesis by inhibiting the expression of FaMADS1 in postharvest strawberry fruit. Postharvest Biol. Technol. 189, 111934. doi: 10.1016/j.postharvbio.2022.111934
Chen, G., Wang, J., Qiao, X., Jin, C., Duan, W., Sun, X., et al. (2020a). Genome-wide survey of sucrose non-fermenting 1-related protein kinase 2 in rosaceae and expression analysis of PbrSnRK2 in response to ABA stress. BMC Genomics 21, 781. doi: 10.1186/s12864-020-07201-w
Cutler, S. R., Rodriguez, P. L., Finkelstein, R. R., Abrams, S. R. (2010). Abscisic acid: Emergence of a core signaling network. Annu. Rev. Plant Biol. 61, 651–679. doi: 10.1146/annurev-arplant-042809-112122
de Zelicourt, A., Colcombet, J., Hirt, H. (2016). The role of MAPK modules and ABA during abiotic stress signaling. Trends. Plant Sci. 21, 677–685. doi: 10.1016/j.tplants.2016.04.004
Espley, R. V., Hellens, R. P., Putterill, J., Stevenson, D. E., Kutty-Amma, S., Allan, A. C. (2007). Red colouration in apple fruit is due to the activity of the MYB transcription factor, MdMYB10. Plant J. 49, 414–427. doi: 10.1111/j.1365-313X.2006.02964.x
Fujii, H., Verslues, P. E., Zhu, J. K. (2011). Arabidopsis decuple mutant reveals the importance of SnRK2 kinases in osmotic stress responses in vivo. Proc. Natl. Acad. Sci. U. S. A. 108, 1717–1722. doi: 10.1073/pnas.1018367108
Fujita, Y., Nakashima, K., Yoshida, T., Katagiri, T., Kidokoro, S., Kanamori, N., et al. (2009). Three SnRK2 protein kinases are the main positive regulators of abscisic acid signaling in response to water stress in arabidopsis. Plant Cell Physiol. 50, 2123–2132. doi: 10.1093/pcp/pcp147
Fujita, Y., Yoshida, T., Yamaguchi-Shinozaki, K. (2013). Pivotal role of the AREB/ABF-SnRK2 pathway in ABRE-mediated transcription in response to osmotic stress in plants. Physiol. Plant 147, 15–27. doi: 10.1111/j.1399-3054.2012.01635.x
Geiger, D., Scherzer, S., Mumm, P., Stange, A., Marten, I., Bauer, H., et al. (2009). Activity of guard cell anion channel SLAC1 is controlled by drought-stress signaling kinase-phosphatase pair. Proc. Natl. Acad. Sci. U. S. A. 106, 21425–21430. doi: 10.1073/pnas.0912021106
Gonzalez, A., Zhao, M., Leavitt, J. M., Lloyd, A. M. (2008). Regulation of the anthocyanin biosynthetic pathway by the TTG1/bHLH/Myb transcriptional complex in arabidopsis seedlings. Plant J. 53, 814–827. doi: 10.1111/j.1365-313X.2007.03373.x
Guan, R., Van Le, Q., Yang, H., Zhang, D., Gu, H., Yang, Y., et al. (2021). A review of dietary phytochemicals and their relation to oxidative stress and human diseases. Chemosphere. 271, 129499. doi: 10.1016/j.chemosphere.2020.129499
Gupta, K., Wani, S. ,. H., Razzaq, A., Skalicky, M., Samantara, K., Gupta, S., et al. (2022). Abscisic acid: Role in fruit development and ripening. Front. Plant Sci. 10. doi: 10.3389/fpls.2022.817500
Halford, N. G., Hey, S. J. (2009). Snf1-related protein kinases (SnRKs) act within an intricate network that links metabolic and stress signalling in plants. Biochem. J. 419, 247–259. doi: 10.1042/bj20082408
Han, Y., Dang, R., Li, J., Jiang, J., Zhang, N., Jia, M., et al. (2015). SUCROSE NONFERMENTING1-RELATED PROTEIN KINASE2.6, an ortholog of OPEN STOMATA1, is a negative regulator of strawberry fruit development and ripening. Plant Physiol. 167, 915–930. doi: 10.1104/pp.114.251314
Hrabak, E. M., Chan, C. W., Gribskov, M., Harper, J. F., Choi, J. H., Halford, N., et al. (2003). The arabidopsis CDPK-SnRK superfamily of protein kinases. Plant Physiol. 132, 666–680. doi: 10.1104/pp.102.011999
Hu, W., Yan, Y., Shi, H., Liu, J., Miao, H., Tie, W., et al. (2017). The core regulatory network of the abscisic acid pathway in banana: Genome-wide identification and expression analyses during development, ripening, and abiotic stress. BMC Plant Biol. 17, 145. doi: 10.1186/s12870-017-1093-4
Huai, J., Wang, M., He, J., Zheng, J., Dong, Z., Lv, H., et al. (2008). Cloning and characterization of the SnRK2 gene family from zea mays. Plant Cell Rep. 27, 1861–1868. doi: 10.1007/s00299-008-0608-8
Islam, M., Inoue, T., Hiraide, M., Khatun, N., Jahan, A., Kuwata, K., et al. (2021). Activation of SnRK2 by raf-like kinase ARK represents a primary mechanism of ABA and abiotic stress responses. Plant Physiol. 185, 533–546. doi: 10.1093/plphys/kiaa046
Jaakola, L. (2013). New insights into the regulation of anthocyanin biosynthesis in fruits. Trends. Plant Sci. 18, 477–483. doi: 10.1016/j.tplants.2013.06.003
Jefferson, R. A., Kavanagh, T. A., Bevan, M. W. (1987). GUS fusions: beta-glucuronidase as a sensitive and versatile gene fusion marker in higher plants. EMBO. J. 6, 3901–3907. doi: 10.1002/j.1460-2075.1987.tb02730.x
Jia, H. F., Chai, Y. M., Li, C. L., Lu, D., Luo, J. J., Qin, L., et al. (2011). Abscisic acid plays an important role in the regulation of strawberry fruit ripening. Plant Physiol. 157, 188–199. doi: 10.1104/pp.111.177311
Jia, M., Li, X., Wang, W., Li, T., Dai, Z., Chen, Y., et al. (2022). SnRK2 subfamily I protein kinases regulate ethylene biosynthesis by phosphorylating HB transcription factors to induce ACO1 expression in apple. New Phytol. 234, 1262–1277. doi: 10.1111/nph.18040
Jia, H. F., Lu, D., Sun, J. H., Li, C. L., Xing, Y., Qin, L., et al. (2013). Type 2C protein phosphatase ABI1 is a negative regulator of strawberry fruit ripening. J. Exp. Bot. 64, 1677–1687. doi: 10.1093/jxb/ert028
Kalt, W., Cassidy, A., Howard, L. R., Krikorian, R., Stull, A. J., Tremblay, F., et al. (2020). Recent research on the health benefits of blueberries and their anthocyanins. Adv. Nutr. 11, 224–236. doi: 10.1093/advances/nmz065
Karppinen, K., Lafferty, D. J., Albert, N. W., Mikkola, N., McGhie, T., Allan, A. C., et al. (2021). MYBA and MYBPA transcription factors co-regulate anthocyanin biosynthesis in blue-coloured berries. New Phytol. 232, 1350–1367. doi: 10.1111/nph.17669
Karppinen, K., Tegelberg, P., Häggman, H., Jaakola, L. (2018). Abscisic acid regulates anthocyanin biosynthesis and gene expression associated with cell wall modification in ripening bilberry (Vaccinium myrtillus l.) fruits. Front. Plant Sci. 9.1259 doi: 10.3389/fpls.2018.01259
Kobayashi, Y., Yamamoto, S., Minami, H., Kagaya, Y., Hattori, T. (2004). Differential activation of the rice sucrose nonfermenting1-related protein kinase2 family by hyperosmotic stress and abscisic acid. Plant Cell. 16, 1163–1177. doi: 10.1105/tpc.019943
Kulik, A., Wawer, I., Krzywińska, E., Bucholc, M., Dobrowolska, G. (2011). SnRK2 protein kinases–key regulators of plant response to abiotic stresses. OMICS. 15, 859–872. doi: 10.1089/omi.2011.0091
Lafferty, D. J., Espley, R. V., Deng, C. H., Günther, C. S., Plunkett, B., Turner, J. L., et al. (2022). Hierarchical regulation of MYBPA1 by anthocyanin- and proanthocyanidin-related MYB proteins is conserved in vaccinium species. J. Exp. Bot. 73, 1344–1356. doi: 10.1093/jxb/erab460
Latti, A. K., Jaakola, L., Riihinen, K. R., Kainulainen, P. (2010). Anthocyanin and flavonol variation in bog bilberries (Vaccinium uliginosum l.) in Finland. J. Agric. Food Chem. 58, 427–433. doi: 10.1021/jf903033m
Li, D., Mou, W., Luo, Z., Li, L., Limwachiranon, J., Mao, L., et al. (2016). Developmental and stress regulation on expression of a novel miRNA, fan-miR73, and its target ABI5 in strawberry. Sci. Rep. 6, 28385. doi: 10.1038/srep28385
Ma, D., Sun, D., Wang, C., Li, Y., Guo, T. (2014). Expression of flavonoid biosynthesis genes and accumulation of flavonoid in wheat leaves in response to drought stress. Plant Physiol. Biochem. 80, 60–66. doi: 10.1016/j.plaphy.2014.03.024
Ma, L., Sun, Z., Zeng, Y., Luo, M., Yang, J. (2018). Molecular mechanism and health role of functional ingredients in blueberry for chronic disease in human beings. Int. J. Mol. Sci. 19 (9), 2785 doi: 10.3390/ijms19092785
Mao, W., Han, Y., Chen, Y., Sun, M., Feng, Q., Li, L., et al. (2022). Low temperature inhibits anthocyanin accumulation in strawberry fruit by activating FvMAPK3-induced phosphorylation of FvMYB10 and degradation of chalcone synthase 1. Plant Cell. 34, 1226–1249. doi: 10.1093/plcell/koac006
Moreno-Alvero, M., Yunta, C., Gonzalez-Guzman, M., Lozano-Juste, J., Benavente, J. L., Arbona, V., et al. (2017). Structure of ligand-bound intermediates of crop ABA receptors highlights PP2C as necessary ABA Co-receptor. Mol. Plant 10, 1250–1253. doi: 10.1016/j.molp.2017.07.004
Oh, H. D., Yu, D. J., Chung, S. W., Chea, S., Lee, H. J. (2018). Abscisic acid stimulates anthocyanin accumulation in 'Jersey' highbush blueberry fruits during ripening. Food Chem. 244, 403–407. doi: 10.1016/j.foodchem.2017.10.051
Pertuzatti, P. B., Barcia, M. T., Gómez-Alonso, S., Godoy, H. T., Hermosin-Gutierrez, I. J. F. C. (2021). Phenolics profiling by HPLC-DAD-ESI-MSn aided by principal component analysis to classify rabbiteye and highbush blueberries. Food Chem. 340, 127958. doi: 10.1016/j.foodchem.2020.127958
Pertuzatti, P. B., Barcia, M. T., Rebello, L. P. G., Gómez-Alonso, S., Duarte, R. M. T., Duarte, M. C. T., et al. (2016). Antimicrobial activity and differentiation of anthocyanin profiles of rabbiteye and highbush blueberries using HPLC–DAD–ESI-MSn and multivariate analysis. J. Funct. Foods. 26, 506–516. doi: 10.1016/j.jff.2016.07.026
Petroni, K., Tonelli, C. (2011). Recent advances on the regulation of anthocyanin synthesis in reproductive organs. Plant Sci. 181, 219–229. doi: 10.1016/j.plantsci.2011.05.009
Shang, Y., Yan, L., Liu, Z. Q., Cao, Z., Mei, C., Xin, Q., et al. (2010). The mg-chelatase h subunit of arabidopsis antagonizes a group of WRKY transcription repressors to relieve ABA-responsive genes of inhibition. Plant Cell. 22, 1909–1935. doi: 10.1105/tpc.110.073874
Shao, Y., Qin, Y., Zou, Y., Ma, F. (2014). Genome-wide identification and expression profiling of the SnRK2 gene family in malus prunifolia. Gene. 552, 87–97. doi: 10.1016/j.gene.2014.09.017
Shen, X., Guo, X., Zhao, D., Zhang, Q., Jiang, Y., Wang, Y., et al. (2017). Cloning and expression profiling of the PacSnRK2 and PacPP2C gene families during fruit development, ABA treatment, and dehydration stress in sweet cherry. Plant Physiol. Biochem. 119, 275–285. doi: 10.1016/j.plaphy.2017.08.025
Shen, X., Zhao, K., Liu, L., Zhang, K., Yuan, H., Liao, X., et al. (2014). A role for PacMYBA in ABA-regulated anthocyanin biosynthesis in red-colored sweet cherry cv. Hong deng (Prunus avium l.). Plant Cell Physiol. 55, 862–880. doi: 10.1093/pcp/pcu013
Sun, L., Wang, Y.P., Chen, P., Ren, J., Ji, K., Li, Q., Leng, P. (2011). Transcriptional regulation of SlPYL, SlPP2C, and SlSnRK2 gene families encoding ABA signal core components during tomato fruit development and drought stress. J Exp Bot. 62(15), 5659–5669. doi: 10.1093/jxb/err252
Tang, Q., Chi, F. M., Liu, H. D., Zhang, H. J., Song, Y. (2021). Single-molecule real-time and illumina sequencing to analyze transcriptional regulation of flavonoid synthesis in blueberry. Front. Plant Sci. 12. doi: 10.3389/fpls.2021.754325
Teng, S., Keurentjes, J., Bentsink, L., Koornneef, M., Smeekens, S. (2005). Sucrose-specific induction of anthocyanin biosynthesis in arabidopsis requires the MYB75/PAP1 gene. Plant Physiol. 139, 1840–1852. doi: 10.1104/pp.105.066688
Wang, Y., Li, W., Chang, H., Zhou, J., Luo, Y., Zhang, K., et al. (2020). SRNAome and transcriptome analysis provide insight into strawberry fruit ripening. Genomics. 112, 2369–2378. doi: 10.1016/j.ygeno.2020.01.008
Wu, Q., Zhang, X., Peirats-Llobet, M., Belda-Palazon, B., Wang, X., Cui, S., et al. (2016). Ubiquitin ligases RGLG1 and RGLG5 regulate abscisic acid signaling by controlling the turnover of phosphatase PP2CA. Plant Cell. 28, 2178–2196. doi: 10.1105/tpc.16.00364
Yang, W., Guo, Y., Liu, M., Chen, X., Xiao, X., Wang, S., et al. (2022). Structure and function of blueberry anthocyanins: A review of recent advances. J. Funct. Foods. 88, 104864. doi: 10.1016/j.jff.2021.104864
Yoshida, R., Umezawa, T., Mizoguchi, T., Takahashi, S., Takahashi, F., Shinozaki, K. (2006). The regulatory domain of SRK2E/OST1/SnRK2.6 interacts with ABI1 and integrates abscisic acid (ABA) and osmotic stress signals controlling stomatal closure in arabidopsis. J. Biol. Chem. 281, 5310–5318. doi: 10.1074/jbc.M509820200
Zhang, H., Li, W., Mao, X., Jing, R., Jia, H. (2016). Differential activation of the wheat SnRK2 family by abiotic stresses. Front. Plant Sci. 7. doi: 10.3389/fpls.2016.00420
Zhang, Y. L., Lin-Wang, K., Albert, N. W., Elborough, C., Espley, R. V., Andre, C. M., et al. (2021). Identification of a strong anthocyanin activator, VbMYBA, from berries of vaccinium bracteatum thunb. Front. Plant Sci. 12. doi: 10.3389/fpls.2021.697212
Zhang, Y., Ye, Y., Jiang, L., Lin, Y., Gu, X., Chen, Q., et al. (2020). Genome-wide characterization of Snf1-related protein kinases (SnRKs) and expression analysis of SnRK1.1 in strawberry. Genes (Basel). 11 (4), 427. doi: 10.3390/genes11040427
Keywords: SnRK2, ABA signaling pathway, fruit ripening, anthocyanin synthesis, VcMYB1, Vaccinium corymbosum
Citation: Wang X, Tang Q, Chi F, Liu H, Zhang H and Song Y (2023) Sucrose non-fermenting1-related protein kinase VcSnRK2.3 promotes anthocyanin biosynthesis in association with VcMYB1 in blueberry. Front. Plant Sci. 14:1018874. doi: 10.3389/fpls.2023.1018874
Received: 14 August 2022; Accepted: 10 February 2023;
Published: 24 February 2023.
Edited by:
Giselle Camargo Mendes, Instituto Federal de Santa Catarina (IFSC), BrazilReviewed by:
Dong Fang Ma, Yangtze University, ChinaCopyright © 2023 Wang, Tang, Chi, Liu, Zhang and Song. This is an open-access article distributed under the terms of the Creative Commons Attribution License (CC BY). The use, distribution or reproduction in other forums is permitted, provided the original author(s) and the copyright owner(s) are credited and that the original publication in this journal is cited, in accordance with accepted academic practice. No use, distribution or reproduction is permitted which does not comply with these terms.
*Correspondence: Yang Song, c29uZ3lhbmdAY2Fhcy5jbg==
†These authors share first authorship
Disclaimer: All claims expressed in this article are solely those of the authors and do not necessarily represent those of their affiliated organizations, or those of the publisher, the editors and the reviewers. Any product that may be evaluated in this article or claim that may be made by its manufacturer is not guaranteed or endorsed by the publisher.
Research integrity at Frontiers
Learn more about the work of our research integrity team to safeguard the quality of each article we publish.