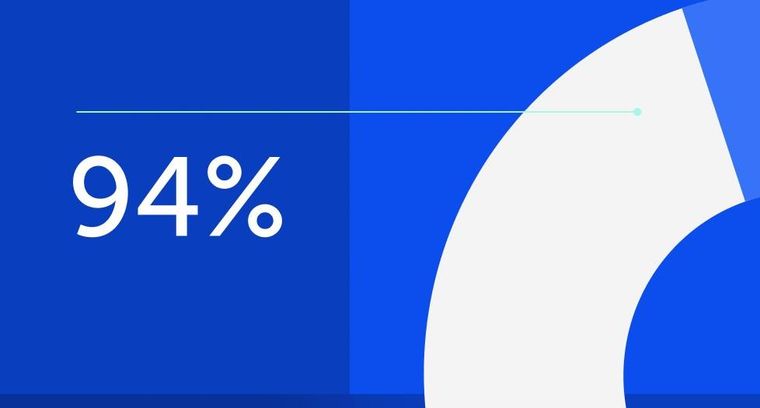
94% of researchers rate our articles as excellent or good
Learn more about the work of our research integrity team to safeguard the quality of each article we publish.
Find out more
ORIGINAL RESEARCH article
Front. Plant Sci., 10 February 2023
Sec. Crop and Product Physiology
Volume 14 - 2023 | https://doi.org/10.3389/fpls.2023.1006245
Quantifying the effects of various environmental conditions on maize leaf number is essential to understanding the environmental adaptations and population structure of maize plants and for enhancing maize productivity. In this study, seeds of three temperate-adapted maize cultivars, each belonging to different maturity classes, were sown on eight different dates. Sowing dates ranged from the middle of April to early July, which allowed us to cover a wide range of environmental conditions. Random forest regression and multiple regression models with variance partitioning analyses were used to assess the effects of environmental factors on the number of leaves and their distributions on maize primary stems. We demonstrated that the total leaf number (TLN) increased in the three cultivars in the following order: FK139 < JNK728 < ZD958, and variations in TLN for each cultivar were 1.5, 1.76, and 2.75 leaves, respectively. The variation in TLN was ascribed to changes in LB (leaf number below the primary ear), which were higher than variations in LA (leaf number above the primary ear). Variations in TLN and LB were mainly affected by the photoperiod during growth stages V7 to V11, and differences in TLN and LB in response to different photoperiods ranged from 1.34 to 2.95 leaves h-l. Variations in LA was mainly affected by temperature-related factors. Therefore, the results of this study enhanced our current understanding of key environmental conditions that affect maize leaf numbers, and provides scientific support for the benefits of adjusting sowing dates and selecting suitable cultivars to mitigate the effects of climate change on maize production.
Crop growth is strongly affected by external environmental factors (Craufurd and Wheeler, 2009; Poorter et al., 2016), such as temperature (Lizaso et al., 2018), solar radiation (Shi et al., 2022), water availability (Nakayama and Bucks, 1991) and photoperiod (Craufurd and Wheeler, 2009), all of which ultimately lead to uncertainties for crop production (Tsimba et al., 2013a). Therefore, a great challenge in crop production is identifying critical meteorological factors affecting crop growth and yield. For instance, when the same variety of maize was planted at different latitudes, the resulting TLN varied by 5 or more leaves (Liu et al., 2020). Changes in leaf number are important as they affect key growth and developmental processes, leaf area, and the overall canopy morphology of maize plants, which affects plant biomass accumulation and yield; as such, leaf number can be used to assess regional adaptations among different maize cultivars (Allen et al., 1973; Ellis et al., 1992; Bonhomme et al., 1994; Lafitte and Edmeades, 1997; Li et al., 2016; Liu et al., 2020). Leaf number is also a useful metric for modeling maize phenology and ontogeny (Bonhomme et al., 1991; Birch et al., 1998b).
Maize leaves develop from stem apical meristems, which usually occurs before tassel initiation (Bonhomme et al., 1991). Environmental factors affect the leaf initiation rate and duration, two important traits that ultimately determine the final leaf number (Tollenaar and Hunter, 1983). Many researchers have investigated variations in leaf number between different maize cultivars in response to environmental factors, such as photoperiod and air temperature (Russell and Stuber, 1983; Tollenaar and Hunter, 1983; Warrington and Kanemasu, 1983; Ellis et al., 1992; Liu et al., 2020), diurnal temperature range (Bonhomme et al., 1991), rate of changing daylength (Bonhomme et al., 1991), and incident photosynthetic photon flux density (Tollenaar, 1999).
Most studies investigating changes in maize leaf number in response to environmental factors have been focused on field experiments in multiple locations or altering sowing dates (Bonhomme et al., 1991; Birch et al., 1998a; Giauffret et al., 2000; Liu et al., 2020), however some studies have also used controlled-environment cabinets to more tightly dissect the effects of different environmental factors on maize leaf number (Allison and Daynard, 1979; Chen et al., 2015; Francis et al., 1969; Stevenson and Goodman, 1972; Tollenaar, 1999), and a few others used combined field based and climate controlled experiments (Russell and Stuber, 1985; Mungoma and Pollak, 1991). However, between field based and controlled-environment experiments, controlled-environment studies consistently obtain lower photothermal ratios (the ratio between the daily amount of light and daily temperature), and the correlation between controlled-environment and field phenotypic data is very low (Poorter et al., 2016). Hence, it is questionable whether responses quantified in a controlled environment can accurately reflect field performance (White et al., 2012; Poorter et al., 2016). Furthermore, there are experimental limitations to controlling so many field-related factors; nearly all research conducted under field conditions has focused on just one or two environmental factors for which pertinent information is lacking, and ignored how interactions between multiple meteorological factors can affect maize leaf number.
Many studies have suggested that effective measures to cope with the impending effects of global climate change on maize crop production are to adjust the sowing date to allow for acclimation to increasing temperatures (Huang et al., 2020; Liu et al., 2013b; Zhang et al., 2020). However, altering the sowing date also changes the photoperiod to which maize seedlings are exposed during the growing period, and the effects of this on maize growth and yield have not been accurately studied (Tsimba et al., 2013b). In this study, we conducted a series of sowing date experiments that cover a wide range of temperature, photoperiod, and radiative conditions to explore the comprehensive effects of multiple meteorological factors on variations in maize leaf number. This study’s objectives are to investigate variations in leaf number below the primary ear (LB), leaf number above the primary ear (LA), total leaf number (TLN), and whether differences in LB and LA contribute to TLN. We also aimed to elucidate the effects of multiple meteorological factors on LB and LA in association with TLN, and identify which meteorological factors occurring at which growth stages are vital in determining maize leaf numbers.
Field experiments were conducted from 2018 to 2020 at Xinxiang Experimental Station, Chinese Academy of Agriculture Sciences (35°18′N, 113°54′E), Henan province. Maize was grown almost entirely under irrigated conditions. The average annual number of frost-free days was about 260 d, the average annual accumulated temperature was about 2700-3000 °. The soil texture at the site was clay loam (ISSS Classification, International Soil Science Society) with 12.6 g kg-1 of organic material, 61.2 mg kg-1 of available N, 16.2 mg kg-1 of available P, 110.0 mg kg-1 of available K, and a pH of 8.21.
The election of the genotypes of this study was mainly based on maize maturity, as this a key trait affecting the distribution of different cultivars within existing production areas, which could be associated with the impact of environmental factors on maize leaf numbers. Therefore, three temperate-adapted maize cultivars, FK139, JNK728, and ZD958, with different maturity periods and germplasm origins (Table 1) were planted from 2018 to 2020. The required quantities of ≥0 °-accumulated-temperature through a season of growth for the three hybrids were 2700 °, 2800 °, and 3100 °, respectively.
Treatments for field experiments in each year consisted of eight sowing dates (Table 2) to cover a wide range of weather regimes associated with each date and still allow crops time to reach physiological maturity. The eight sowing dates are denoted as: SD1, SD2, SD3, SD4, SD5, SD6, SD7, and SD8.
Two or three maize seeds per hole were planted at a soil depth of 5.0 cm, and the resulting seedlings were thinned at the V3 stage to a target density of 75,000 plants ha-1 with an equal spacing of 0.6 m. Each plot was 9 m long and 7.2 m wide, and each consisted of 12 rows. We established a randomized complete block design with three replications for each treatment in this experiment. Before sowing, the plot was finely prepared and moistened. Experimental plots were irrigated after sowing and fertilized according to soil analysis recommendations, typically at rates of 225 kg of N ha-1, 173 kg of P2O5 ha-1, and 150 kg of K2O ha-1. Fertilizers were applied once before sowing to avoid nutrient limitations. Irrigation was applied as required to maintain soil moisture near field capacity to avoid any degree of water stress. Crops were protected from pests, pathogens, and weeds according to local farm management practices.
Prior to assessing leaf stages and recording maize development, the timing of seedling emergence was recorded from seeds planted in three of the 12 rows (per plot) and within a 1 m length of each row. Once 50% of the viable seeds emerged from the soil, the seedling emergence stage (V1) was recorded. Twenty healthy maize plants were chosen from among the emerged seedlings to assess leaf stages. Leaf stages were defined by the number of leaves with visible tips in a whorl, and the number of visible leaf tips was recorded daily for each treatment (Zadoks et al., 1974). When the nth number of leaf tips in a whorl was observed, a new leaf stage of maize was recorded as Vn (i.e. at stage V1 one leaf tip is visible, and so on). Leaves 6 and 12 were paint-marked to use as references for leaf number.
Before the first leaf had senesced 20, 50, and 50 plants from the center rows were selected for leaf number counting in the 2018, 2019, and 2020 crop seasons, respectively. Per treatment, plants that appeared uniform in growth at the silking stage were chosen at random and then tagged by red paint for convenient and accurate counting of TLN, LB, and LA.
Half-hourly data collections were made for the meteorological variables, including air temperature and solar radiation at the field site, using a “WatchDog” Data Logger (Spectrum Technologies, Inc., USA) during the vegetative growth stage of maize. Sensors to measure solar radiation and air temperature were placed 2 m above ground level. Photoperiod (sunrise to sunset and civil twilight) was computed using the method described by Forsythe et al. (1995).
The number of leaves appearing along the main stem of a plant can be used to examine maize phenology (Zadoks et al., 1974). Here, three counts of leaf numbers (TLN, LB, and LA) were counted prior to maize plants initiating tassel formation (Ellis et al., 1992). Tassel initiation reportedly occurs at a leaf stage that is numerically equal to 50% of the final leaf number per plant (Tollenaar and Hunter, 1983). The average TLNs of our three cultivars, FK139, JNK728, and ZD958, were 17.26, 18.19, and 19.75 leaves, respectively. The final leaf numbers at the tassel initiation stage for each cultivar were approximately 8.6, 9.1, and 9.9 leaves, respectively. The topmost axillary meristem is initiated shortly after tassel initiation (Lejeune and Bernier, 1996). So in this study we focused on the stages from seedling emergence to V11. We divided the different maize developmental stages from seedling emergence (V1) to V11. In order to determine the most accurate sensitivity period, we divided the cycles from V1 to V11 into different developmental stage combinations, such as stages V1 to V2, V1 to V3, V1 to V4, V1 to V5…V2 to V3 stage, V2 to V4 stage, V2 to V5 stage … V10 to V11 stage, and so on. In total, 55 different developmental stage combinations were used to determine the sensitivity of each stage to different weather variables. Combinations of each of the 55 stages and each of the six meteorological factors—mean air temperature (MT), average maximum air temperature (Tmax), average minimum air temperature (Tmin), average temperature range (Tr), photoperiod (PP), and photosynthetically active radiation (PAR)—produced 330 combinations of variables for this study. The effects of weather variables on different developmental stages correlated strongly with one another, which could lead to a serious multicollinearity problem in the traditional statistical models, for instance stepwise multiple regression and linear regression analyses haven’t eliminated latent multicollinearity. Random forest is not influenced by multicollinearity issues (Dormann et al., 2013). Hence, in this study to avoid multicollinearity issues in the data analysis, the random forest regression model was used to determine the combined factors that were likely responsible for variation in our three counts of leaf numbers (Dormann et al., 2013; Trivedi et al., 2016). For the random forest models, we used each of the three counts as response variables and the 330 combined variables as predictors. We used percentage increases in the MSE (mean squared error) of variables to assess the importance of the combined factors. Higher MSE% values indicated greater involvement of a variable (Breiman, 2001). The 10 most critically important combined factors were selected based on the MSE%.
Significant effects of each predictor on response variables were estimated using the R package ‘rfPermute’ (version 4.1.1; http://www.r-project.org/).
A multiple regression model with variance partitioning analysis was also used to evaluate the importance of the top 10 critically important combined factors identified by the random forest analysis, and to identify key combined factors influencing variations observed in any of the three leaf counts using the lm and calc.relimp functions in the “relaimpo” R package (version 4.1.1; http://www.r-project.org/).
Analyses of variance (ANOVA) was used to estimate the effects of the sowing date, year, cultivar, and their correlations with the three maize leaf numbers of interest using R (version 4.1.1; http://www.r-project.org/). Significant effects were determined by P-values from the F-test and variation partitioning was determined based on the sum of squares. The packages “ggplot2” (version 4.1.1; http://www.r-project.org/), Microsoft Visio 2003, and Excel 2016 were used to produce figures. In order to present the data concisely, the notations *, **, and *** are used to indicate significance levels at 0.01 < P < 0.05, 0.001 < P < 0.01, and P < 0.001, respectively. In addition, ns refers to P ≥ 0.05.
Meteorological conditions measured during crop vegetative growth in the 2018 to 2020 maize growing seasons are shown in Figure 1. The time from emergence to the silking period was between 42-60 d for all experiments, and the duration of this timeframe became significantly shortened with later sowing dates. Maize plants developed from the middle of April to early July, and were covered to a broad range of photoperiod, thermal, and radiative conditions. More specifically, during the studied developmental period from seedling emergence to tassel initiation (stages V1 to V11, which occurred at about 20 days after seedling emergence), the average PP ranged from 13.76 to 14.46 h/d, the MT ranged from 21.20 to 29.38 °, the Tmax ranged from 26.93 to 34.47 °, the Tmin ranged from 10.02 to 26.70 °, the Tr ranged from 8.96 to 16.21 °, and the PAR ranged from 8.20 to 11.18 MJ m-2d-1 (Figure 1). Meteorological conditions exhibited similar values and trends during the period from seedling emergence to silking.
Figure 1 Time-course of meteorological conditions during maize vegetative growth. (A–C) respectively represent the meteorological conditions of 2018, 2019, and 2020, and the below horizontal bars indicate the duration (days) of maize phenological periods: black-bars indicate the time from sowing to emergence (V1), red-bars indicate the time fromV1 to V11 (The period of V1 to V11 nearly the time of maize leaf differentiation), blue-bars indicate the time fromV11 to silking (Xu et al., 2023).
As shown in Figure 2, the TLN ranged from 16.40 to 21.10; the mean value of the TLN was 18.39 across all cultivars and treatments (n=2880). The TLN significantly differed among cultivars (Figure 3); the TLNs of FK139, JNK728, and ZD958 were 17.27 (n = 960), 18.19 (n = 960), and 19.76 (n = 960) leaves, respectively. LB exhibited the same trends in variation observed for TLN, wherein it started out high and decreased in accordance with the delay in sowing date. The LB ranged from 10.80 to 15.48 leaves, and the mean value of LB was 12.86 (n = 2880) leaves for all treatments. The LB for each cultivar significantly increased in the following order: 11.57 (FK139, n = 960), 12.41 (JNK728, n = 960), and 14.61 (ZD958, n = 960). The TLN and LB of ZD958 were significantly higher than the corresponding values of the other two cultivars. Variations in LA were lower than the observed variations in TLN and LB. The average LA was 5.51 leaves (n = 2880) among all treatments. The mean LA for each cultivar significantly increased in the following order: 5.14 (ZD958, n = 960), 5.66 (FK139, n = 960), and 5.71 (JNK728, n = 960). In contrast to the TLN and LB data where FK139 had the lowest mean values, ZD958 had the lowest mean LA compared to the other two cultivars.
Figure 2 Variation observed in leaf counts relative to sowing dates. A, variation in TLN; B, variation in LB; C, variation in LA.
Figure 3 The total leaf number among different maize cultivars. ****are used to indicate significance levels at P < 0.0001.
The TLNs of the cultivars selected for this study were widely variable. The degrees of variation of TLN in FK139, JNK728, and ZD958 were 1.5, 1.76, and 2.75 leaves, respectively. The TLN was significantly lower for the earlier and later sowing dates than when seeds were sown in the middle of the season.
Across the three crop seasons, LB significantly differed among cultivars, and the changes in LB variation across sowing dates coincided with the changes in TLN variation. The variations in LB for FK139, JNK728, and ZD958 were 1.30, 1.28, and 2.33 leaves, respectively. The variation of TLNs and LBs could explain 61% and 49% of the maize maximum leaf area, respectively (Figure 4). Therefore, the difference of 1 or 2 leaves can significantly alter the maize canopy and this may affect biomass accumulation and grain yield.
Figure 4 Relationship between leaf number variables and maximum leaf area at the silking stage. (A, B represent the relationship between the maximum leaf area and TLN, LB, respectively).
LA also significantly differed between cultivars. The variations in LA for FK139, JNK728, and ZD958 were 0.88, 0.93, and 1.44 leaves, respectively. There were small differences in LA variation between the sowing, thus the LA remained relatively stable in comparison with the LB and TLN.
As shown in Figure 5, the three cultivars exhibited wide variation in the three variables representing leaf number. Variation in TLN for FK139, JNK728, and ZD958 ranged from 0.01 to 0.87, 0.04 to 0.99, and 0.01 to 1.41 leaves, respectively. Variation in LB for FK139, JNK728, and ZD958 ranged between 0.04 to 0.82, 0.02 to 0.77, and 0.09 to 1.48 leaves, respectively. The respective ranges in variation of LA for FK139, JNK728, and ZD958 were 0 to 0.49, 0.02 to 0.50, and 0.01 to 0.77 leaves.
Figure 5 Relationships between variations in leaf number variables. Variation of LA is the absolute value of LA of a treatment minus the average value of LA of a cultivar; variation of LB is the absolute value of LB of a treatment minus the average value of LB of a cultivar; and variation of TLN is the absolute value of TLN of a treatment minus the average value of TLN of a cultivar. R2adj, adjusted R square.
The correlation between variation in LB and variation in TLN was significant (p<0.01), and the adjusted R square values of FK139, JNK728, and ZD958 were 0.43, 0.30, and 0.67, respectively. In contrast, correlations between LA variation, TLN variation, and LB variation were insignificant at p>0.05. All of these results indicate that changes in TLN are more closely correlated with changes in LB.
We used random forest regression analysis (Figure 6) to identify the major meteorological factors affecting changes in leaf number between the three cultivars and across different sowing dates. Variations in TLN, LB, and LA were jointly affected by photoperiod, PAR, and temperature-related factors. Variation of TLN was strongly associated with photoperiod and temperature-related factors. Variations in LB were primarily influenced by photoperiod, while variations in LA were mainly affected by temperature-related factors.
Figure 6 Relative contributions (percent increase of the MSE) of various meteorological factors as drivers of variations in TLN, LB, and LA of the three maize cultivars. Percent increases in the MSE of different variables were used to estimate the importance of these predictors, and higher MSE% values indicated greater importance of the predictors. MSE, mean squared error (A, FK139; B, JNK728; C, ZD958). * and ** are used to indicatesignificance levels at 0.01 < P < 0.05, 0.001 < P < 0.01, and P < 0.001,respectively. In addition, ns refers to P ≥ 0.05.
We also evaluated the responses of each cultivar to the various meteorological factors. Evidently, FK139 and JNK728 were more sensitive to photoperiod and temperature-related factors, while ZD958 was more sensitive to photoperiod during maize growth.
In addition, multivariate regression analysis was conducted to validate the observations of the random forest regression analysis and to clearly identify the most crucial meteorological factors that significantly affected the leaf number responses of the three cultivars (Figure 7). The TLNs of FK139, JNK728, and ZD958 were mainly affected by PP, Tmax, and PP in the V10 to V11 (R2adj=0.23, P < 0.05), V7 to V8 (R2adj =0.13, P < 0.05) and V7 to V11 (R2adj =0.44, P < 0.05) stages, respectively.
Figure 7 Key meteorological factors during crucial growth stages affecting leaf number responses of three maize cultivars. (A–C) respectively represent the TLN, LB, and LA of FK139; (D–F) respectively represent the TLN, LB, and LA of JNK728; and (G–I) respectively represent the TLN, LB, and LA of ZD958.
The LBs of FK139, JNK728, and ZD958 were mainly affected by the Tmax in the V6–V8 stages (R2adj =0.34, P < 0.05), PP in the V7–V10 stages (R2adj =0.29, P < 0.05) and PP in the V7–V10 stages (R2adj =0.54, P < 0.05). The variation in TLN correlated in large part with the variation in LB, and photoperiod appeared to have the greatest influence on variations in TLN and LB. Photoperiod sensitivity, defined as the number of leaves that developed per hour increase of photoperiod, varied among cultivars, and sensitivities of TLN and LB to photoperiod ranged from 1.34 to 2.95 leaves h-l. Hence, photoperiod was the main driver of the variation observed in maize TLN.
Temperature-related factors during later growth stages primarily affected the LA of the three tested cultivars. These factors were the Tmin in the V10–V11 stage (R2adj =0.21, P < 0.05), Tr in the V3–V5 stages (R2adj =0.15, P < 0.05) and Tmax in the V10–V11 stage (R2adj =0.15, P < 0.05). Overall, the variation of LA and the distribution of data for the three leaf number responses were mainly affected by temperature-related factors.
Consistent with the findings of previous research, we observed that adjusting the sowing date can expose a crop to a broad range of environmental conditions (Tsimba et al., 2013b; Bonelli et al., 2016; Zhang et al., 2019). As previously reported, maize TLN per plant varied relative to different sowing dates (Birch et al., 1998a; Tsimba et al., 2013b), and higher leaf numbers were recorded for the summer plantings (Chase and Nanda, 1967). The degrees of variation in TLN and LB were almost identical. Moreover, variations in TLN was primarily attributed to variations in LB. The degree of variation in LA was smaller than that of the LB or TLN, which has also been reported in earlier papers (Warrington and Kanemasu, 1983; Tollenaar, 1999; Liu et al., 2020). In contrast to the patterns we observed for variation in LA and LB in conventional maize, Subedi and Ma (2005) reported greater variation in LA than in LB for leafy hybrid maize.
According to the results of the random forest regression analysis, variations in TLN, LB, and LA of each cultivar were affected jointly by photoperiod, PAR, and temperature-related factors, largely confirming the conclusions of a previous study (Lejeune and Bernier, 1996), and photoperiod was a primary determinant of maize TLN and LB. Moreover, previous reports have shown that the effects of photoperiod on maize can occur as early as the V3 (leaf collar appearance) stage (Chen et al., 2015) and that maize leaf number is affected by photoperiod during the V5 and V7 stages (Tollenaar and Hunter, 1983). Excluding FK139, the results of this study showed that the photoperiod-sensitive interval for maize leaf number occurred approximately between stages V7 to V11, the duration of which was approximately 10.2 days; all durations were within the range found by a previous study where the photoperiod-induced phase varied from 0 to greater than 17 days (Rood and Major, 1980). One important finding of this research was that variations in LA were mainly affected by temperature-related factors, and to a lesser extent photoperiod and PAR, and the duration of the sensitive period for LA responses to temperature-related factors was estimated to be about 2.4 days. This finding is in line with findings of previous studies showing that temperature and photoperiod affected the variation of LA (Warrington and Kanemasu, 1983; Lejeune and Bernier, 1996), but is in contrast to previous results showing LA was not affected by photoperiod (Tollenaar, 1999).
Differentiation of maize leaves from stem apical meristems occurs before tassel initiation (Bonhomme et al., 1991), and the leaf number of maize plants is sensitive to photoperiod mainly between the time of emergence to tassel initiation. We assumed that maize was insensitive to photoperiod before the V7 stage (Wu et al., 2008; Craufurd and Wheeler, 2009). Environmental factors affect the leaf initiation rate and duration of leaf initiation, and these two leaf traits ultimately determine the final leaf number. The leaf initiation rate was constant from seedling emergence until tassel initiation (Warrington and Kanemasu, 1983). In this study, TLN and LB increased with increasing photoperiod, indicating that the photoperiod extension increased the leaf initiation duration and ultimately increased the maize leaf number (Birch et al., 1998a; Giauffret et al., 2000). On the other hand, variation of LA was mainly affected by temperature-related factors, suggesting that LA and LB are independently controlled at the genetic level (Li et al., 2016). The LAs of our three cultivars ranged from 4.46 to 6.15 leaves, which partly supports results of a previous report demonstrating that maize axillary meristems and leaf primordia at the apical meristem are initiated at the same rate but with a constant delay of 5.6–7.0 plastochrons (Lejeune and Bernier, 1996). Less variation in LA can reduce self-shading and shading by neighbors that inherently occurs in a maize crop (Subedi and Ma, 2005). Accordingly, a combination of environmental conditions affect the position of the maize primary ear and drive variations of LA and LB, consistent with findings that photoperiod, temperature, and irradiance may alter maize apical dominance which induces abortion of the topmost ear, affecting the primary ear position (Lejeune and Bernier, 1996; Lejeune et al., 1998).
Maize leaf number is an accessible morphological index with higher environmental plasticity, which can be used to determine maize population structure, and is also widely used as a measure of anthesis time, an indicator crucial to local environmental adaptation (Li et al., 2016; Zhang et al., 2019; Liu et al., 2020). Previous studies about the sensitive period during which maize leaf number is influenced by photoperiod and temperature-related factors are mainly based on developmental stages as defined by the number of visible leaf collars (Tollenaar and Hunter, 1983; Wu et al., 2008; dos Santos et al., 2022). In contrast, our results of sensitive periods are based on the number of visible leaf tips. The duration of successive appearances of new leaf tips was about 2 days that shorter than the period of successive appearances of new leaf collars, which is in accordance with findings involving twelve early maturing inbred corn lines reared under controlled-environmental conditions (Rood and Major, 1980). Differences in photoperiod during the sensitivity phase varied less than two hours but the maize leaf number varied by up to 5 leaves (Liu et al., 2013a; Liu et al., 2020). In our current study, a photoperiod during the sensitivity phase that varied less than one hour (0.7 h, Figure 1) led to significant variation in maize leaf, especially for the cultivar ZD958, the leaf number of which varied by up to 2.75 leaves, so the effects of photoperiod on leaf number may not be negligible. In addition, the sensitivities of TLN and LB to photoperiod (1.34 to 2.95 leaves h-l, Figure 7) were over the range reported by previous studies (Warrington and Kanemasu, 1983; Russell and Stuber, 1985; Bonhomme et al., 1991; Birch et al., 1998a), suggesting that basing the sensitive period on the number of visible leaf tips may more accurately reflect the real characteristics of some maize cultivars. The response in maize leaf number to photoperiod and temperature-related factors varied with season (Birch et al., 1998a), cultivar, and experimental location (Bonhomme et al., 1991). This study was conducted in the North China Plain, an area with a large sowing window (Huang et al., 2021) that differed in temperature, photoperiod, and radiative conditions. To the best of our knowledge this is the first study to use random forest regression analysis to determine major meteorological factors influencing the variability of maize leaf number and leaf positional distribution. A major advantage of the random forest regression is that its predictive power is not influenced by multicollinearity issues (Dormann et al., 2013). We also used a multiple regression model with variance partitioning analysis to validate the random forest analysis outcomes and identify key meteorological factors affecting variations in TLN, LB, and LA.
Results of this study have diverse implications for future research on maize physiology and eco-physiology, especially in modeling maize phenology and ontogeny (Bonhomme et al., 1991; Carberry and Abrecht, 1991; Birch et al., 1998a; Jones and Kirniry, 1986). Furthermore, adjusting the sowing date and selecting late-maturing cultivars, are two cost-effective options to contend with potential negative consequences of climate change (Liu et al., 2013b; Huang et al., 2020; Zhang et al., 2020). Adjusting the sowing date can expose maize to different environmental conditions; previous studies paid more attention to temperature, solar radiation, and water availability on maize production and ignored the effects of photoperiod, especially in relation to maize leaf number. It is true that some leaf numbers of some maize cultivars (FK139, JNK728) are insensitive to changing environment factors, while some cultivars such as ZD958 are sensitive to this change, which should be considered in studies and to develop models on the effects of adjusting the sowing date. Importantly, we determined that the photoperiod during the photoperiod-sensitive phase of maize development, which can be altered by adjusting sowing date, is one of the key factors affecting maize leaf number. A previous study indicated that the average photoperiod, ranging from just 13.7-15.6 h, during the photoperiod-sensitive phase of maize development was responsible for increasing total leaf numbers from 18.7 to 23.7 (Liu et al., 2013a). Therefore, more attention should be paid to variations of photoperiod during the photoperiod-sensitive phase of maize development. Moreover, based on our empirical evidence, when maize farmers select cultivars and adjust the sowing dates, they must consider each cultivar’s sensitivity to photoperiod to avoid dramatic decreases in leaf number. These reductions in leaf number may affect leaf area and the amount of incident radiation intercepted by the maize canopy, which ultimately influences maize assimilate production and maize grain yield (Lafitte and Edmeades, 1997; Liu et al., 2020). Further investigations into the effects of photoperiod on maize yield are necessary to improve the current understanding maize production and responses to different environmental conditions.
Our study revealed that changing the sowing date can expose maize to different environmental regimes in terms of temperature, photoperiod, and solar radiation, and significantly influenced maize leaf numbers of the tested cultivars. The variation in TLN was attributed to variations in LB, both of which were greater than variations in LA. Variations in TLN and LB were mainly affected by photoperiod, particularly from growth stages V7 to V11, and this photoperiod sensitivity varied between cultivars. Variation in LA was mainly affected by temperature-related factors. Identifying key environmental conditions affecting leaf number variation in maize can provide theoretical references for assessing regional adaptations, developing breeding strategies, optimizing sowing dates of a given variety, and optimizing critical processes for maize crop modeling.
The raw data supporting the conclusions of this article will be made available by the authors, without undue reservation.
HX: Data curation, formal analysis, resources, software, visualization, writing – original draft. BM: Data curation, formal analysis, resources, software, visualization, writing – original draft. KW: Writing – review & editing, project administration, methodology. JX: Data curation, visualization. PH: Data curation, visualization. SL: Conceptualization, funding acquisition, supervision, validation, writing – review & editing, project administration, methodology. RX: Conceptualization, funding acquisition, supervision, validation, writing – review & editing, project administration, methodology. All authors contributed to the article and approved the submitted version.
This work was sponsored by the National Key Research and Development Program of China (2017YFD0300302), the National Maize Industrial Technology System in China (CARS-02-25) and the Agricultural Science and Technology Innovation Program (CAAS-ZDRW202004).
We are grateful to Ma Pingping (Xinxiang County, Henan Province), Liu Yuanhang (Institute of Crop Sciences, Chinese Academy of Agricultural Sciences), and Li Yanan (JiNing Normal University) for their assistance with the experiments performed at Xinxiang Experimental Station, Chinese Academy of Agriculture Sciences. We also thank the editors and reviewers for helping us to improve the manuscript.
The authors declare that the research was conducted in the absence of any commercial or financial relationships that could be construed as a potential conflict of interest.
All claims expressed in this article are solely those of the authors and do not necessarily represent those of their affiliated organizations, or those of the publisher, the editors and the reviewers. Any product that may be evaluated in this article, or claim that may be made by its manufacturer, is not guaranteed or endorsed by the publisher.
Allen, J. R., McKee, G. W., McGahen, J. H. (1973). Leaf number and maturity in hybrid corn. Agron. J. 65, 233–235. doi: 10.2134/agronj1973.00021962006500020014x
Allison, J. C. S., Daynard, T. B. (1979). Effect of change in time of flowering, induced by altering photoperiod or temperature, on attributes related to yield in maize. Crop Sci. 19, 1–4. doi: 10.2135/cropsci1979.0011183X001900010001x
Birch, C. J., Hammer, G. L., Rickert, K. G. (1998a). Temperature and photoperiod sensitivity of development in five cultivars of maize (Zea mays l.) from emergence to tassel initiation. Field Crop Res. 55, 93–107. doi: 10.1016/S0378-4290(97)00062-2
Birch, C. J., Rickert, K. G., Hammer, G. L. (1998b). Modelling leaf production and crop development in maize (Zea mays l.) after tassel initiation under diverse conditions of temperature and photoperiod. Field Crop Res. 58, 81–95. doi: 10.1016/S0378-4290(98)00087-2
Bonelli, L. E., Monzon, J. P., Cerrudo, A., Rizzalli, R. H., Andrade, F. H. (2016). Maize grain yield components and source-sink relationship as affected by the delay in sowing date. Field Crop Res. 198, 215–225. doi: 10.1016/j.fcr.2016.09.003
Bonhomme, R., Derieux, M., Edmeades, G. O. (1994). Flowering of diverse maize cultivars in relation to temperature and photoperiod in multilocation field trials. Crop Sci. 34, 156–164. doi: 10.2135/cropsci1994.0011183X003400010028x
Bonhomme, R., Derieux, M., Kiniry, J. R., Edmeades, G. O., Ozier.-Lafontaine, H. (1991). Maize leaf number sensitivity in relation to photoperiod in multilocation field trials. Agron. J. 83, 153–157. doi: 10.2134/agronj1991.00021962008300010035x
Carberry, P. S., Abrecht, D. G. (1991). “Tailoring crop models to the semiarid tropics,” in Climatic risk in crop production: Models and management for the semiarid tropics and subtropics. Eds. Muchow, R. C., Bellamy, J. A. (Wallingford, UK: C.A.B. International), 157–182.
Chase, S. S., Nanda, D. K. J. (1967). Number of leaves and maturity classification in Zea mays l. Crop Sci. 7, 431–432. doi: 10.2135/cropsci1967.0011183X000700050007x
Chen, Q., Zhong, H., Fan, X. W., Li, Y. Z. (2015). An insight into the sensitivity of maize to photoperiod changes under controlled conditions. Plant Cell Environ. 38, 1479–1489. doi: 10.1111/pce.12361
Craufurd, P. Q., Wheeler, T. R. (2009). Climate change and the flowering time of annual crops. J. Exp. Bot. 60, 2529–2539. doi: 10.1093/jxb/erp196
Dormann, C. F., Elith, J., Bacher, S., Buchmann, C., Carl, G., Carré, G., et al. (2013). Collinearity: a review of methods to deal with it and a simulation study evaluating their performance. Ecography 36, 27–46. doi: 10.1111/j.1600-0587.2012.07348.x
dos Santos, C. L., Abendroth, L. J., Coulter, J. A., Nafziger, E. D., Suyker, A., Yu, J., et al. (2022). Maize leaf appearance rates: A synthesis from the united states corn belt. Front. Plant Sci. 13. doi: 10.3389/fpls.2022.872738
Ellis, R. H., Summerfield, R. J., Edmeades, G. O., Roberts, E. H. (1992). Photoperiod, leaf number, and interval from tassel initiation to emergence in diverse cultivars of maize. Crop Sci. 32, 398–403. doi: 10.2135/cropsci1992.0011183X003200020024x
Forsythe, W. C., Rykiel C.OMMAJr., E. J., Stahl, R. S., Wu, H.-i., Schoolfield, R. M. (1995). A model comparison for daylength as a function of latitude and day of year. Ecol. Modell 80, 87–95. doi: 10.1016/0304-3800(94)00034-F
Francis, C., Grogan, C., Sperling, D. J. (1969). Identification of photoperiod insensitive strains of maize (Zea mays l.). Crop Sci. 9, 675–677. doi: 10.2135/cropsci1970.0011183X001000050001x
Giauffret, C., Lothrop, J., Dorvillez, D., Gouesnard, B., Derieux, M. J. (2000). Genotype× environment interactions in maize hybrids from temperate or highland tropical origin. Crop Sci. 40, 1004–1012. doi: 10.2135/cropsci2000.4041004x
Huang, M., Wang, J., Wang, B., Liu, D., Feng, P., Yu, Q., et al. (2021). Assessing maize potential to mitigate the adverse effects of future rising temperature and heat stress in China. Agric. For. Meteorol. 311, 108673. doi: 10.1016/j.agrformet.2021.108673
Huang, M., Wang, J., Wang, B., Liu, D., Yu, Q., He, D., et al. (2020). Optimizing sowing window and cultivar choice can boost china’s maize yield under 1.5° c and 2° c global warming. Environ. Res. Lett. 15, 024015. doi: 10.1088/1748-9326/ab66ca
Jones, C. A., Kiniry, J. R. (1986). CERES-maize: a simulation model of maize growth and development (College Station: Texas A&M University Press).
Lafitte, H. R., Edmeades, G. O. (1997). Temperature effects on radiation use and biomass partitioning in diverse tropical maize cultivars. Field Crop Res. 49, 231–247. doi: 10.1016/S0378-4290(96)01005-2
Lejeune, P., Bernier, G. J. (1996). Effect of environment on the early steps of ear initiation in maize (Zea mays l.). Plant Cell Environ. 19, 217–224. doi: 10.1111/j.1365-3040.1996.tb00243.x
Lejeune, P., Prinsen, E., Van Onckelen, H., Bernier, G. (1998). Hormonal control of ear abortion in a stress-sensitive maize (Zea mays) inbred. Aust. J. Plant Physiol. 25, 481–488. doi: 10.1071/PP97154
Li, D., Wang, X., Zhang, X., Chen, Q., Xu, G., Xu, D., et al. (2016). The genetic architecture of leaf number and its genetic relationship to flowering time in maize. New Phytol. 210, 256–268. doi: 10.1111/nph.13765
Liu, W., Ming, B., Xie, R., Liu, G., Wang, K., Yang, Y., et al. (2020). Change in maize final leaf numbers and its effects on biomass and grain yield across China. Agriculture 10, 411. doi: 10.3390/agriculture10090411
Liu, Y., Xie, R., Hou, P., Li, S., Zhang, H., Ming, B., et al. (2013a). Phenological responses of maize to changes in environment when grown at different latitudes in China. Field Crops Res. 144, 192–199. doi: 10.1016/j.fcr.2013.01.003
Liu, Z., Hubbard, K. G., Lin, X., Yang, X. (2013b). Negative effects of climate warming on maize yield are reversed by the changing of sowing date and cultivar selection in northeast China. Glob. Change Biol. 19, 3481–3492. doi: 10.1111/gcb.12324
Lizaso, J. I., Ruiz-Rarnos, M., Rodriguez, L., Gabaldon-Leal., C., Oliveira, J. A., Lorite, I. J., et al. (2018). Impact of high temperatures in maize: Phenology and yield components. Field Crop Res. 216, 129–140. doi: 10.1016/j.fcr.2017.11.013
Mungoma, C., Pollak, L. M. (1991). Photoperiod sensitivity in tropical maize accessions, early inbreds, and their crosses. Crop Sci. 31, 388–391. doi: 10.2135/cropsci1991.0011183X003100020032x
Nakayama, F. S., Bucks, D. A. (1991). Water quality in drip/trickle irrigation: A review. Irrigation Sci. 12, 187–192. doi: 10.1007/BF00190522
Poorter, H., Fiorani, F., Pieruschka, R., Wojciechowski, T., van der Putten, W. H., Kleyer, M., et al. (2016). Pampered inside, pestered outside? differences and similarities between plants growing in controlled conditions and in the field. New Phytol. 212, 838–855. doi: 10.1111/nph.14243
Rood, S. B., Major, D. J. (1980). Responses of early corn inbreds to photoperiod. Crop Sci. 20, 679–682. doi: 10.2135/cropsci1980.0011183X002000060001x
Russell, W. K., Stuber, C. W. (1983). Effects of photoperiod and temperatures on the duration of vegetative growth in maize. Crop Sci. 23, 847–850. doi: 10.2135/cropsci1983.0011183X002300050008x
Russell, W. K., Stuber, C. W. (1985). Genotype×photoperiod and genotype×temperature interactions for maturity in maize. Crop Sci. 25, 152–158. doi: 10.2135/cropsci1985.0011183X002500010037x
Shi, D., Huang, Q., Liu, Z., Liu, T., Su, Z., Guo, S., et al. (2022). Radiation use efficiency and biomass production of maize under optimal growth conditions in northeast China. Sci. Total Environ. 2022, 836. doi: 10.1016/j.scitotenv.2022.155574
Stevenson, J., Goodman, M. J. (1972). Ecology of exotic races of maize. i. leaf number and tillering of 16 races under four temperatures and two photoperiods. Crop Sci. 12, 864–868. doi: 10.2135/cropsci1972.0011183X001200060045x
Subedi, K. D., Ma, B. L. (2005). Ear position, leaf area, and contribution of individual leaves to grain yield in conventional and leafy maize hybrids. Crop Sci. 45, 2246–2257. doi: 10.2135/cropsci2004.0653
Tollenaar, M. (1999). Duration of the grain-filling period in maize is not affected by photoperiod and incident PPFD during the vegetative phase. Field Crop Res. 62, 15–21. doi: 10.1016/S0378-4290(98)00170-1
Tollenaar, M., Hunter, R. B. (1983). A photoperiod and temperature sensitive period for leaf number of maize. Crop Sci. 23, 457–460. doi: 10.2135/cropsci1983.0011183X002300030004x
Trivedi, P., Delgado-Baquerizo, M., Trivedi, C., Hu, H., Anderson, I. C., Jeffries, T. C., et al. (2016). Microbial regulation of the soil carbon cycle: Evidence from gene–enzyme relationships. ISME J. 10, 2593–2604. doi: 10.1038/ismej.2016.65
Tsimba, R., Edmeades, G. O., Millner, J. P., Kemp, P. D. (2013a). The effect of planting date on maize grain yields and yield components. Field Crop Res. 150, 135–144. doi: 10.1016/j.fcr.2013.05.028
Tsimba, R., Edmeades, G. O., Millner, J. P., Kemp, P. D. (2013b). The effect of planting date on maize: Phenology, thermal time durations and growth rates in a cool temperate climate. Field Crop Res. 150, 145–155. doi: 10.1016/j.fcr.2013.05.028
Warrington, I. J., Kanemasu, E. T. (1983). Corn growth response to temperature and photoperiod. III. leaf number. Agron. J. 75, 762–766. doi: 10.2134/agronj1983.00021962007500050010x
White, J. W., Kimball, B. A., Wall, G. W., Ottman, M. J. (2012). Cardinal temperatures for wheat leaf appearance as assessed from varied sowing dates and infrared warming. Field Crop Res. 137, 213–220. doi: 10.1016/j.fcr.2012.08.013
Wu, L., Wang, T., Ku, L., Huang, Q., Sun, Z., Xia, Z., et al. (2008). Determination of the photoperiod-sensitive inductive phase in maize with leaf numbers and morphologies of stem apical meristem. Agr Sci. China 7, 554–560. doi: 10.1016/S1671-2927(08)60052-8
Xu, H. G., Ming, B., Wang, K. R., Xue, J., Hou, P., Li, S. K., et al. (2023). Quantitative analysis of maize leaf collar appearance rates. Plant Physiol Bioch. doi: 10.1016/j.plaphy.2023.01.016 (In press)
Zadoks, J. C., Chang, T. T., Konzak, C. F. (1974). A decimal code for the growth stages of cereals. Weed Res. 14, 415–421. doi: 10.1111/j.1365-3180.1974.tb01084.x
Zhang, Z. X., Christensen, M., Nan, Z. B., Whish, J. P. M., Bell, L. W., Wang, J. F., et al. (2019). Plant development and solar radiation interception of four annual forage plants in response to sowing date in a semi-arid environment. Ind. Crop Prod. 131, 41–53. doi: 10.1016/j.indcrop.2019.01.028
Keywords: photoperiod, temperature-related factors, maize, leaf number, leaf positional distribution
Citation: Xu H, Ming B, Wang K, Xue J, Hou P, Li S and Xie R (2023) The effects of photoperiod and temperature-related factors on maize leaf number and leaf positional distribution in the field. Front. Plant Sci. 14:1006245. doi: 10.3389/fpls.2023.1006245
Received: 29 July 2022; Accepted: 23 January 2023;
Published: 10 February 2023.
Edited by:
Luis A. N. Aguirrezabal, National University of Mar del Plata, ArgentinaReviewed by:
Samir Alahmad, The University of Queensland, AustraliaCopyright © 2023 Xu, Ming, Wang, Xue, Hou, Li and Xie. This is an open-access article distributed under the terms of the Creative Commons Attribution License (CC BY). The use, distribution or reproduction in other forums is permitted, provided the original author(s) and the copyright owner(s) are credited and that the original publication in this journal is cited, in accordance with accepted academic practice. No use, distribution or reproduction is permitted which does not comply with these terms.
*Correspondence: Shaokun Li, bGlzaGFva3VuQGNhYXMuY24=; Ruizhi Xie, eGllcnVpemhpQGNhYXMuY24=
Disclaimer: All claims expressed in this article are solely those of the authors and do not necessarily represent those of their affiliated organizations, or those of the publisher, the editors and the reviewers. Any product that may be evaluated in this article or claim that may be made by its manufacturer is not guaranteed or endorsed by the publisher.
Research integrity at Frontiers
Learn more about the work of our research integrity team to safeguard the quality of each article we publish.