- 1College of Agronomy, Sichuan Agricultural University, Chengdu, China
- 2Institute of Chinese Medicine Resources and Cultivation, Sichuan Academy of Traditional Chinese Medicine Sciences, Chengdu, China
- 3College of Grassland Science and Technology, Sichuan Agricultural University, Chengdu, China
- 4Dry Grain Department, Sichuan General Popularization Centre of Agricultural Technique, Chengdu, China
- 5College of Landscape Architecture, Sichuan Agricultural University, Chengdu, China
- 6Department of Agronomy, University of Agriculture, Faisalabad, Pakistan
- 7Department of Agronomy, The Islamia University of Bahawalpur, Punjab, Pakistan
- 8Department of Agronomy, Pir Mehr Ali Shah Arid Agriculture University, Rawalpindi, Pakistan
Assessing the spatial distribution of organic matter and total nitrogen in soil is essential for management and optimum utilization of fertilizers. Therefore, the present field experiment was conducted to evaluate the impact of different planting pattern arrangements on the spatial distribution of soil total nitrogen and organic matter content under a maize/soybean strip relay intercropping system. The planting was arranged in a manner such that soil sampling could be done from continuous maize/soybean relay strip intercropping (MS1), maize/soybean relay strip intercropping in rotation (MS2), traditional maize/soybean intercropping (MS3), sole maize (M), sole soybean (S), and fallow land (FL) from 2018 to 2020. The results showed significant variations for soil organic matter and total nitrogen content under different planting pattern arrangements of maize and soybean in the strip relay intercropping system. Across all systems, the highest soil organic matter (29.19 g/kg) and total nitrogen (10.19 g/kg) were recorded in MS2. In contrast, the lowest soil organic matter (1.69 g/kg) and total nitrogen (0.64 g/kg) were observed in FL. Soil organic matter and total nitrogen in MS2 increased by 186.45% and 164.06%, respectively, when compared with FL. Soil organic matter and total nitrogen in MS2 increased by 186.45% and 164.06%, respectively, when compared with FL. Furthermore, under MS2, the spatial distribution of soil organic matter was higher in both maize and soybean crop rows as compared with other cropping patterns, whereas the soil total nitrogen was higher under soybean rows as compared with maize in all other treatment. However, correlation analysis of the treatments showed variations in organic matter content. It can be concluded that different planting patterns can have varying effects on soil organic matter and total nitrogen distribution under the strip relay intercropping system. Moreover, it is recommended from this study that MS2 is a better planting pattern for the strip relay intercropping system, which can increase the spatial distribution of soil organic matter and total nitrogen, thereby improving soil fertility, C:N ratio, and crop production. This study will serve as a foundation towards the scientific usage of chemical fertilizers in agricultural sector.
1. Introduction
Soil organic matter and total nitrogen content are important indexes to evaluate soil fertility and soil quality. These indexes are essential sources and sinks of the global carbon cycle and have become one of the research hotspots in soil and environmental sciences (Johannes et al., 2020; Roberta et al., 2020; Wu et al., 2021). Although soil organic matter and total nitrogen content only account for a small part of the total soil volume, they play a vital role in balancing soil fertility, environmental protection, and sustainable agricultural production (Struijk et al., 2020). China feeds approximately 20% of the world’s population, with less than 9% of the world’s arable land area (Guo et al., 2010). The world’s population is increasing rapidly; therefore, it must produce more from the limited arable land to meet the needs of the growing population (Altieri, 1999; Fan et al., 2012). Strengthening the utilization of chemical fertilizers is an essential step in obtaining bumper crop yields (Hassanein et al., 2019; Mohamed et al., 2020); nevertheless, the indiscriminate use of fertilizers has led to increased soil nutrient imbalance in various regions of the world, notably many Asian countries (Zhou et al., 2017; Wang et al., 2020). In economically developed areas of China, a markedly disproportionate dose of fertilizers is being administered, i.e., averaging 339 kg/hm2, which is 1.29 times higher than the national average of 262 kg/hm2; however, in underdeveloped areas, the fertilization rate is only 178 kg/hm2 (Cho, 2007; Zhang and Zhang, 2008; Zhang et al., 2013).
Regarding the limited utilization of fertilizer resources, China adopted the concept of ecological agriculture in the last century (Shao et al., 2019; Tourn et al., 2019). In this regard, a lot of research on soil nutrient status has been carried out, and intercropping of different crops was one of the priorities. Intercropping is a cropping pattern in which two or more crops are cultivated simultaneously on the same piece of farmland (Godfray et al., 2010), which proves to be economically, ecologically, and socially profitable (Du et al., 2018; Gitari et al., 2020). According to statistics, the universal intercropping area is more than 1,109 hm2, which is about 3% of the total cultivated area (Li et al., 2007; Gautam et al., 2014). Common intercropping patterns principally comprise intercropping or relay strip intercropping, and strip intercropping refers to growing two or more crops in strips within a specific width, allowing alternate planting of different crops. The main difference between intercropping and strip intercropping lies in the fact that, in strip intercropping, different kinds of crops are not grown in a single row, but two or more rows of the same crop are grown together; one crop is formed in a “strip” and the interval is cultivated with another “strip” of crop, having a relatively fixed line number, line spacing, and strip width. Strip intercropping can make full use of limited land resources, improve the nutrient absorption and utilization efficiency of crops, and enhance soil fertility as well as soil quality (Zhang et al., 2019; Ahmed et al., 2020).
Under intercropping, soil organic matter and total nitrogen content, like other soil characteristics, have high spatial variabilities in various regions. Similarly, in China, there are significant differences in soil organic matter and nitrogen contents at different spatial locations at the same time (Liu et al., 2011; Yao et al., 2019). The changes in soil organic matter and total nitrogen content are dependent on farming practices such as fertilization, incorporation of crop residues, crop rotations, soil utilization, and tillage method (Huang et al., 2007). The tillage method has a great influence on soil organic matter and total nitrogen content. It was reported that intercropping could enhance the distribution and content of soil organic matter and total nitrogen (Leonard et al., 2012). Cereal/legume intercropping is widely recognized as a sustainable agricultural production system, as it can improve the symbiotic nitrogen fixation of legumes and reduce the input of chemical fertilizers (Yao et al., 2019). Intercropping of maize and soybean represents a new cereal/legume pattern, which farmers are rapidly adopting in Southwest China (Yao et al., 2019). However, there is no available literature on soil organic matter and total nitrogen spatial distribution in the maize/soybean strip relay intercropping system so far. Therefore, the objectives of the present study were to identify better management practices that could optimize land use efficiency; to understand the mechanism underlying the increased soil fertility under maize/soybean relay strip intercropping systems, especially Southwest China and similar areas; and, to quantify the relationship between soil organic matter, total nitrogen content, and planting patterns in a maize/soybean strip relay intercropping system. The results will serve as a foundation for the scientific usage of chemical fertilizers in the maize/soybean relay strip intercropping system.
2. Materials and methods
2.1. Experimental location
Field experiments were conducted from 2018 to 2020 at the research farm of Sichuan Agricultural University in Ya’an city located in Southwest Sichuan Province of China (101°56′26″E, 28°51′10″N). This region comprised of a hilly and mountainous topography (Figure 1). The climate was humid subtropical monsoon, with an average annual temperature of 16.2°C, an average annual rainfall of 1,250 to 1,750 mm, an average annual sunshine duration of 1,005 hours, and an average annual frost-free period of 300 days. The soil type was gley soils according to FAO-UNESCO 1988 (Rolf and Eddy de, 2011) with pH 6.6, organic matter 29.8 g·kg−1, total nitrogen 1.6 g·kg−1, total phosphorus 1.28 g·kg−1, and total potassium 14.28 g·kg−1.
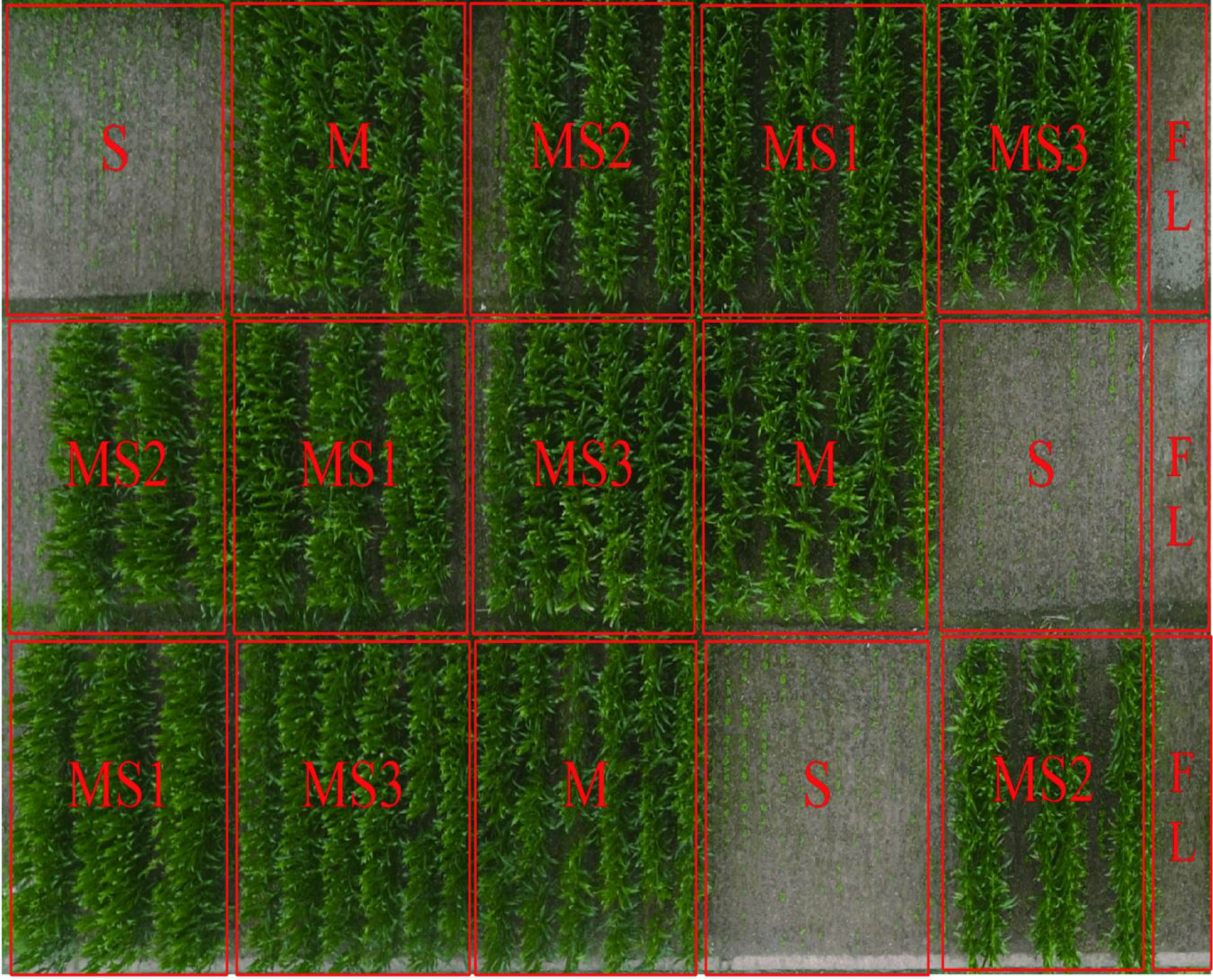
Figure 1 Location of the research site aerial photo. Note: MS1, MS2, MS3, M, S, FL represent the Continuous planting of maize/soybean relay strip intercropping, Planting of maize/soybean relay strip inter-cropping in rotation, Traditional maize/soybean inter-cropping, Sole maize planting, Sole soybean planting, Fallow land, respectively.
2.2. Experimental design and treatments
The experimental design was a randomized block design with three replications (Figure 2). In this experiment, maize variety Denghai-605 and soybean variety Nandou-12 were used. Treatments were arranged MS1 = Continuous maize/soybean relay strip intercropping, MS2 = maize/soybean relay strip intercropping in rotations, MS3 = Traditional maize/soybean intercropping (MS3: A conventional planting method in Southwest of China), M = Sole maize, S = Sole soybean, or FL = Fallow land. The size of the experimental plots under MS1, MS2, MS3, M, and S was 6 × 6 (36 m2), whereas the plot size for FL was 2 × 6 (12 m2). The total width of MS1 and MS2 was “160 cm + 40 cm”, i.e., the relay intercropping combination of two crop strips with a total width of 200 cm, consisting of two rows of maize and two rows of soybean with a 40-cm row width for maize and soybean, and 60-cm spacing between the adjacent rows of maize and soybean. MS3 had a total width of 100 cm with a 1:1 row ratio, and distance between maize/soybean rows was 50 cm. In sole planting of maize and soybean, the distance between two rows was 100 cm for maize and 50 cm for soybean. (Note: The difference between MS2 treatment and MS1 treatment was that the maize belt and soybean belt were rotated in MS2, i.e., maize belts turned into soybean and soybean belts turned into maize each year.)
Different basal fertilizers, including urea (CH4N2O, including 46% N), calcium superphosphate [Ca(H2PO4)2H2O, including 14% P2O5], and potassium chloride (KCl, including 52% K2O) were used for maize and soybean. Maize was fertilized with pure nitrogen at 120 kg/ha, P2O5 at 105 kg/ha, and K2O at 135 kg/ha, and soybean was fertilized with pure nitrogen at 60 kg/ha, P2O5 at 63 kg/ha, and K2O at 52.5 kg/ha in 2018, 2019, and 2020. Maize crop was sown on 24 March, 23 March, and 29 March, in 2018, 2019, and 2020, respectively, and harvested on 25 July, 6 August, and 8 August in 2018, 2019, and 2020, respectively. Soybean was sown on 7 June, 8 June, and 13 June, in 2018, 2019, and 2020, respectively, and harvested on 30 October, 23 October, and 22 October 22 in 2018, 2019, and 2020, respectively.
2.3. Sample collection and measurement
In this experiment, soil samples from all the cropping patterns were collected after soybean harvesting. For soil sampling, the fixed-point sampling procedure was adopted to collect the soil sample from 0 to 20 cm soil layer (Figure 3). To collect the sample, a soil core was inserted vertically into the ground. All the collected soil samples were mixed, and approximately 1 kg of soil was taken for further analysis. Undisturbed soil samples were placed in a tray and stored in a clean indoor ventilation area for natural air drying. After drying, samples were put into a sample bag for the determination of soil organic matter and total nitrogen. All sample bags were labeled with a number, sampling place, soil type, sampling depth, sampling date, and time.
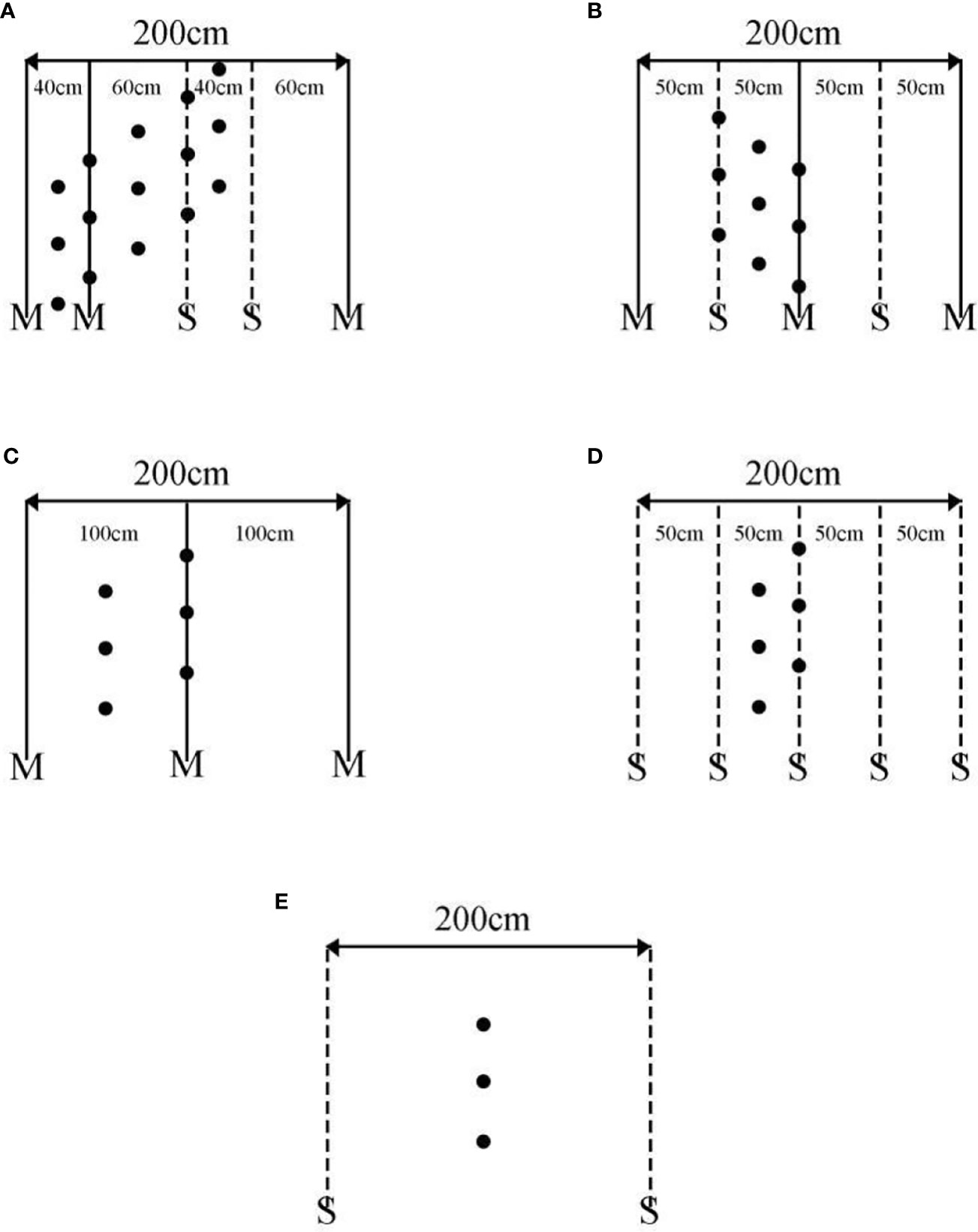
Figure 3 Spatial distribution of soil sample sites in different cropping patterns. (A) Maize/soybean relay strip intercropping (continuous and rotation cropping), (B) traditional maize/soybean intercropping, (C) maize monoculture, (D) soybean monoculture, and (E) fallow land. M, sole maize planting; S, sole soybean planting; ●, for the soil sample collection point.
2.3.1. Soil organic matter and total nitrogen content determination
The soil organic matter (SOM) was determined by the potassium dichromate volumetric method – external heating method (Geng and Wei, 2020).
where c (mol/L) is the molar concentration of consuming ferrous sulfate, V0 is the volume (ml) of consuming ferrous sulfate in a blank test, V is the volume (ml) of consuming ferrous sulfate in the titrating soil sample, 0.003 is ¼ mmol/g of carbon, 10,724 is the conversion coefficient from soil organic carbon to organic matter, 1.1 is a correction factor (the oxidation rate in this method is 90%), m (g) is the air dry soil quality, and k is the coefficient of drying soil to drying soil.
The total nitrogen content (TN) was determined by the Kjeldahl method (Wu, 2004).
Where V0 is the volume (ml) of standard acid used for titrating the sample, V is the volume (ml) of normal acid used for titrating the blank, C is the normal acid concentration (mol/L), 14 is the molar mass of N (g/mol), and W is the sample weight (g).
2.3.2. Soil organic matter and total nitrogen reference standards
At present, there are many soil nutrient grading standards in China (Geng and Wei, 2020). The results of this experiment mainly refer to the national soil nutrient classification standard (Table 1). The Chinese soil nutrient classification standard divides soil organic matter and soil nutrient into six grades from 1 to 6. Soil organic matter and soil nutrient are the highest in grade 1 and the lowest in grade 6 (Wu, 2004). Furthermore, the spatial variation of soil organic matter and nutrient availability in China is relatively high. For example, soil organic matter in China can be as high as 200 g/kg or more, and as low as 5 g/kg or less, and the total nitrogen content can be as high as 35 g/kg and as low as 5 g/kg (Zhang et al., 2008). Therefore, further refinement of the soil organic matter and soil nutrient grade is needed to compare differences in soil nutrient grading in China (Yan et al., 2017).
The classification of coefficient of variation: coefficient of variation is considered weak under <10%, moderate between 10% and 100%, and strong when it is >100% (Duan, 2000; Zhang et al., 2003).
2.4. Data statistics and analysis
All the experimental data were managed in Microsoft Excel 2016, and the figures were constructed with Origin Pro 2018. Differences between intercropping systems and soil organic matter and total nitrogen content were identified by analyzing variance (ANOVA) using SPSS 22.0 software (SPSS Inc., Chicago, IL, USA). The mean values were compared with a least significant difference (LSD) test at the p < 0.01 significance level. Linear regression techniques were used to describe the relationships between soil organic matter and total nitrogen content. The effectiveness of cropping patterns was determined by regression analysis with p-values (Tukey’s test) and the coefficient of determination (R2).
3. Results
3.1. Soil organic matter content and spatial distribution
The different planting patterns showed significant (p < 0.01) variations for soil organic matter content in both maize and soybean at all sampling times across the 3 years of this experiment (Table 2 and Figure 4). During the 3 years, the average SOM of each treatment order was MS2 > MS1 > MS3 > S > M > FL (Table 2 and Figure 4). Furthermore, it was observed that the maximum soil organic matter (39.72 g kg−1) was recorded in MS2, whereas the minimum soil organic matter (8.71 g kg−1) was recorded in FL per system. The soil organic matter content in MS2 increased by 186.45% when compared with FL (Table 2 and Figure 4). At the same time, the spatial distribution of organic matter in maize and soybean rows was most dense in MS2. The obtained results were also graded by the coefficient of variation, where we found that MS1, MS2, MS3, M, and S demonstrated moderate variations when compared, while FL showed weak variation, and overall MS2 showed the most significant variations (Table 2).
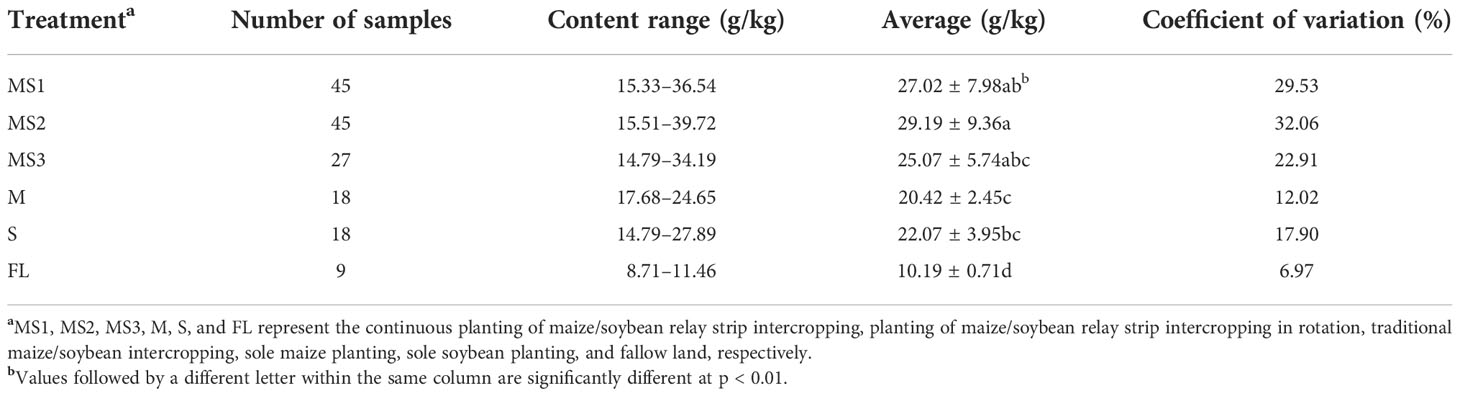
Table 2 Soil organic matter content under different planting patterns of maize and soybean in 2018–2020.

Figure 4 Spatial distribution of soil organic matter under different planting patterns of maize and soybean. MS1, MS2, MS3, M, S, and FL represent the continuous planting of maize/soybean relay strip intercropping, planting of maize/soybean relay strip intercropping in rotation, traditional maize/soybean intercropping, sole maize planting, sole soybean planting, and fallow land, respectively.
3.2. Total soil nitrogen content and spatial distribution
Across all treatments, MS2 exhibited the most significant variation in total soil nitrogen content (Table 3 and Figure 5). On average, the order of different treatments was MS2 > MS1 > MS3 > S > M > FL, which revealed that the minimum soil nitrogen content was under FL (0.64g/kg), and the maximum soil nitrogen content was recorded under MS2 (1.69 g/kg) (Table 3 and Figure 5). However, under MS2, the spatial distribution of soil total nitrogen in soybean rows was higher when compared with maize rows (Table 3 and Figure 5). The results showed that the maximum total soil nitrogen content (2.47 g kg−1) was recorded in MS2, while the minimum total soil nitrogen content (0.55 g kg−1) was recorded in S or FL, and total nitrogen in MS2 increased by 164.06% in contrast with FL (Table 3). Under all planting patterns, the spatial distribution of soil total nitrogen content in maize rows was lower as compared with soybean rows; however, the average maximum and minimum total nitrogen and organic matter content was almost identical under various planting patterns. Therefore, it could be speculated that organic matter and total nitrogen had a strong correlation with each other. The results showed that the MS2 planting pattern was most beneficial for soil total nitrogen accumulation.
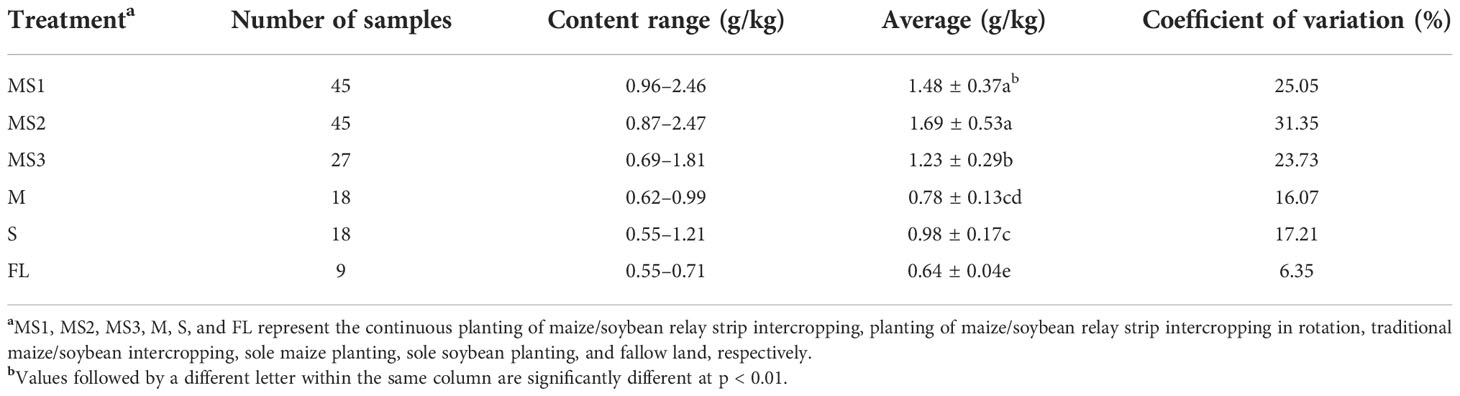
Table 3 Total nitrogen content under different planting arrangements of maize and soybean in 2018–2020.

Figure 5 Spatial distribution of soil total nitrogen content under different planting patterns of maize and soybean. MS1, MS2, MS3, M, S, and FL represent the continuous planting of maize/soybean relay strip intercropping, planting of maize/soybean relay strip intercropping in rotation, traditional maize/soybean intercropping, sole maize planting, sole soybean planting, and fallow land, respectively.
The correlation analysis revealed that there was moderate variation in soil total nitrogen under MS1, MS2, MS3, M, and S, and weak variation in FL; the most significant variation was observed under MS2 (R2 = 0.96).
3.3. Correlation between soil organic matter and total nitrogen
Based on the results of soil organic matter and total nitrogen content in the six treated soils, the correlation equation and coefficient of determination between them could be obtained from Table 4 and Figure 6. The relationship between soil organic matter (x) and total nitrogen (y) was unevenly linear. Six linear regression equations for soil organic matter and total nitrogen, and their coefficient of determinations were obtained (Table 4). The regression equation of the entire test area was y = 0.06x − 0.08, and the coefficient of determination was R2 = 0.87 (Table 4). Furthermore, the correlation between all treatments (planting patterns) for soil organic matter and total nitrogen was significant, where MS1, MS2, MS3, and FL were closer to 1. At the same time, the soil C:N ratio was significantly different among all treatments (p < 0.01), and the highest C:N ratio (25.22) was recorded in MS2 (Table 4). These results indicated that the correlation between soil organic matter and total nitrogen in different planting methods was significant and positively correlated.
4. Discussion
4.1. Variations in soil organic matter content
The soil organic matter content is mainly influenced by land-use management (Demir and Ersoy, 2020; Mishra et al., 2020; Zhao et al., 2020), especially the management of different vegetation in the soil (Welegedara et al., 2020). The percentage of organic matter in shrub soil, grassland, and forest soil, within 1 m depth, was 33%, 42%, and 50%, respectively, which were significantly correlated with the type of vegetation (Eghdami et al., 2019; Yeasmin et al., 2020). Similarly, in this study, there were differences in soil organic matter content between maize and soybean planting patterns. During the 3 years, the average SOM of each treatment order was MS2 > MS1 > MS3 > S > M > FL (Table 2 and Figure 4). This phenomenon might be associated with different planting patterns and crop residues of maize and soybean in the field, thereby contributing toward enhanced soil organic matter accumulation in MS2. Different land-use patterns lead to different soil cultivation, soil physical or chemical properties, and soil fertility. These variations directly affect the decomposition and transformation of soil organic matter in different soils (Lai et al., 2016; Segura et al., 2019; Liu et al., 2020). Furthermore, increased organic matter contents under different treatments in this study indicated that soil organic matter played a vital role in soil fertility under the strip relay intercropping system. Our results are consistent with the study of King et al. (2020).
4.2. Variations in soil total nitrogen content
The soil total nitrogen content reflects the soil potential capacity to provide nutrients for vegetation, which, together with soil organic matter and its dynamic balance, constitutes an essential index of soil fertility (Marco et al., 2011; Alhaj et al., 2019; Dai et al., 2020; Li et al., 2020; Meng, 2020). Nitrogen is considered to be blood for crop growth and development because its absorption and utilization can promote crop growth and increase the crop yield (Hua et al., 2020). Nitrogen competition is significantly higher among crop species; however, in the cereal/legumes intercropping system, it was decreased due to the nitrogen fixation mechanism of legumes, contributing toward increased nitrogen availability for absorption and utilization by cereals. Ta and Faris (1987) found that clover increased nitrogen absorption and utilization by 25% in cereals under intercropping. Broadbent (1981) reported that white clover improved the nitrogen absorption and utilization by 80% in ryegrass under intercropping. Du et al. (2019) and Raza et al. (2019) found that in the maize and soybean intercropping system, the nitrogen uptake of maize was increased by 17%–21%, which was mainly attributed to soybean nitrogen fixation. Similarly, this study demonstrated that soil total nitrogen content in maize/soybean strip intercropping was higher than sole cropping (Table 3 and Figure 5). This might be related to soybean nitrogen fixation and increased total organic matter. It was found that soil nitrogen content and spatial distribution were significantly different between intercropping and monoculture, p < 0.01. The content and spatial distribution of soil nitrogen in the contour maps were reflected by the grading color, and the difference was obvious. Soil total nitrogen was the highest (2.47 g·kg−1) in MS2 treatment and the lowest (0.55 g·kg−1) in S and FL treatment (Table 3 and Figure 5). Furthermore, this study showed that when legumes and non-legumes were intercropped, legumes’ nitrogen fixation could benefit the nitrogen absorption and uptake in non-legumes, thus promoting the growth and development of non-leguminous crops. However, the amount of nitrogen fixation depends on the different legume and non-legume intercropping combinations and different crop varieties, planting patterns, and growth habits of various crops (Gabriela et al., 2021). The biological nitrogen fixation of legumes can not only improve the nitrogen absorption and utilization of non-legumes, but can also promote growth and development, reduce crop dependence on non-renewable resources, and increase land equivalent ratio and land interest rate (Zhou et al., 2017; Chen et al., 2019). Similarly, this study demonstrated that maize/soybean relay strip intercropping has a positive effect on soil total nitrogen contents.
4.3. Relationship between soil organic matter and soil total nitrogen content
The soil C:N ratio not only plays a vital role in soil organic matter decomposition but is also an essential factor of soil quality evaluation, as it determines the organic matter effectiveness that improves the soil structure, enhances the carbon fixation, and increases the soil potential as a “source/sink” of atmospheric CO2 and nitrogen regulation (Bewket and Stroosnijder, 2003; Hagedorn et al., 2010). It is generally believed that during the initial stage of mineralization, organic matter having a C:N ratio > 30 cannot produce nitrogen. If organic matter has a C:N ratio < 15 at the beginning of mineralization, the amount of adequate nitrogen will exceed microorganism assimilation in the soil, thereby making it possible for plants to obtain adequate nitrogen from organic matter mineralization (Sun et al., 2020; Veronika et al., 2020).
Our results showed that the variation coefficient of organic matter and total nitrogen content in each treatment was moderate (Table 4). This might be due to the difference between organic matter and total nitrogen content. Furthermore, this could also be influenced by (1) the contrast of parent material and soil texture at the experimental site; (2) the particularity of remote terrain in the field; (3) impact of crop vegetation; and (4) climatic factors. Meanwhile, the contents of organic matter and total nitrogen in soil samples were compared, and it was found that the spatial difference of organic matter and total nitrogen was significant, and there was a significant positive correlation between organic matter and total nitrogen (Table 4). The results of the present study will serve as a guideline for rational fertilization in agricultural production, thereby contributing toward appropriate fertilizer usage, improved utilization rate of nutrients, and enhanced crop yield from low and medium soils.
5. Conclusions
Taken together, the findings of the current study elucidated the effect of different planting pattern arrangements on soil organic matter and soil nitrogen content under the maize/soybean strip relay intercropping system. It was found that different planting patterns of maize and soybean strip relay intercropping significantly affected soil organic matter and total nitrogen content in soil. The findings revealed that the highest soil organic matter and total nitrogen content was recorded in MS2, while the lowest soil organic matter and total nitrogen content was recorded in FL. Furthermore, under MS2, the spatial distribution of soil organic matter was higher in both maize and soybean crop rows as compared with other cropping patterns, whereas the soil total nitrogen was higher in soybean rows as compared with maize in all other treatments. However, correlation analysis of the treatments showed variations in organic matter content. Moreover, it is recommended that MS2 is a better planting pattern for the strip relay intercropping system, which can increase the spatial distribution of soil organic matter and total nitrogen, thereby improving the soil fertility, C:N ratio, and crop production.
Data availability statement
The original contributions presented in the study are included in the article/supplementary material. Further inquiries can be directed to the corresponding authors.
Author contributions
XT and WY conceived and designed the research. MH, KC, JX, MA, and AS conducted the experiments. XT and KC evaluated the data. JX and MA provided different chemical reagents and experimental material. Paper writing was completed by XT, WY, and MH. KC reviewed and edited the manuscript. All authors contributed to the article and approved the submitted version.
Funding
This research was supported by the National Key Research and Development Program of China (2016YFD0300209 and 2016YFD0300602), the National Nature Science Foundation (31571615), the Program on Industrial Technology System of National Soybean (CARS-04-PS19), the Sichuan Department of Science and Technology Miao Zi Project (2016RZ0061), the Sichuan Province to Guide Local Science and Technology Development Project (2018SZYD0003), the Classification of Project (2022YFN0045), and the Key Project at Central Government Level: The Ability Establishment of Sustainable Use for Valuable Chinese Medicine Resources (2060302).
Conflict of interest
The authors declare that the research was conducted in the absence of any commercial or financial relationships that could be construed as a potential conflict of interest.
Publisher’s note
All claims expressed in this article are solely those of the authors and do not necessarily represent those of their affiliated organizations, or those of the publisher, the editors and the reviewers. Any product that may be evaluated in this article, or claim that may be made by its manufacturer, is not guaranteed or endorsed by the publisher.
References
Ahmed, A., Aftab, S., Hussain, S., Cheema, H. N., Yang, W. (2020). Nutrient accumulation and distribution assessment in response to potassium application under maize–soybean intercropping system. Agronomy 10, 725. doi: 10.3390/agronomy10050725
Alhaj, H. Y., Guo, X., Wang, Z., Shaghaleh, H., Chen, S., Hassan, A., et al. (2019). Effects of irrigation regime and soil clay content and their interaction on the biological yield, nitrogen uptake and nitrogen-use efficiency of rice grown in southern China. Agric. Water Manage 213, 934–946. doi: 10.1016/j.agwat.2018.12.017
Altieri, M. A. (1999). The ecological role of biodiversity in agroecosystems. Agricult. Ecosyst. Environ. 74, 19–31. doi: 10.1016/S0167-8809(99)00028-6
Bewket, W., Stroosnijder, L. (2003). Effects of agroecological land use succession on soil properties in chemoga watershed, blue Nile basin, Ethiopia. Geoderma 111, 85–98. doi: 10.1016/S0016-7061(02)00255-0
Broadbent, F. E. (1981). Methodology for nitrogen transformation and balance in soil. Plant Soil 58, 383–399. doi: 10.1007/BF02180064
Chen, P., Song, C., Liu, X. M., Zhou, L., Yang, H., Zhang, X., et al. (2019). Yield advantage and nitrogen fate in an additive maize/soybean relay intercropping system. Sci. Total Environ. 657, 987–999. doi: 10.1016/j.scitotenv.2018.11.376
Cho, S. E. (2007). Effects of spatial variability of soil properties on slope stability. Eng. Geol. 92, 97–109. doi: 10.1016/j.enggeo.2007.03.006
Dai, L., Fu, R., Guo, X., Du, Y., Cao, G. (2020). Long-term grazing exclusion greatly improve carbon and nitrogen store in an alpine meadow on the northern qinghai-Tibet plateau. Catena 197, 104955. doi: 10.1016/j.catena.2020.104955
Demir, Y., Ersoy, M. (2020). Effect of land use and topographic factors on soil organic carbon content and mapping of organic carbon distribution using regression kriging method. Carpathian J. Earth Environ. Sci. 15 (3), 311–322. doi: 10.26471/cjees/2020/015/131
Duan, J. (2000). Soil variation and its process simulation on the loess plateau (Beijing: China Agriculture Press), 90–91.
Du, J., Han, T., Gai, J., Yong, T., Sun, X., Wang, X., et al. (2018). Maize/soybean strip intercropping: Achieved a balance between high productivity and sustainability. J. Integr. Agric. 17, 747–754. doi: 10.1016/S2095-3119(17)61789-1
Du, Q., Zhou, L., Chen, P., Liu, X., Yong, T. (2019). Relay-intercropping soybean with maize maintains soil fertility and increases nitrogen recovery efficiency by reducing nitrogen input. Crop J. 8, 1–22. doi: 10.1016/j.cj.2019.06.010
Eghdami, H., Azhdari, G., Lebailly, P., Azadi, H. (2019). Impact of land use changes on soil and vegetation characteristics in fereydan, Iran. Agriculture 9, 58. doi: 10.3390/agriculture9030058
Fan, M., Shen, J., Yuan, L., Jiang, R., Zhang, F. (2012). Improving crop productivity and resource use efficiency to ensure food security and environmental quality in China. J. Exp. Biol. 63, 13–24. doi: 10.1093/jxb/err248
Gabriela, C. S., Edmilson, J. A., Fabrício, R., Ivani, P. O., Gláucia, M. B.A., Cesar, A. S., et al. (2021). Biological n fixation and n transfer in an intercropping system between legumes and organic cherry tomatoes in succession to green corn. Agriculture 11 (8), 690. doi: 10.3390/agriculture11080690
Gautam, P., Lal, B., Rana, R. (2014). Intercropping: An alternative pathway for sustainable agriculture alternative pathway for sustainable agriculture. Popular Kheti 1, 216–220.
Gitari, H. I., Nyawade, S. O., Kamau, S., Karanja, N. N., Schulte-Geldermann, E. (2020). Revisiting intercropping indices with respect to potato-legume intercropping systems. Field Crop Res. 258, 107957. doi: 10.1016/j.fcr.2020.107957
Godfray, H. C., Beddington, J. R., Crute, I. R., Haddad, L., Lawrence, D., Muir, J. F., et al. (2010). Food security: the challenge of feeding 9 billion people. Science 327 (5967), 812–818.
Guo, J. H., Liu, X. J., Zhang, Y., Shen, J. L., Han, W. X., Zhang, W. F., et al. (2010). Significant acidification in major Chinese croplands. Science 327, 1008–1010. doi: 10.1126/science.1182570
Hagedorn, F., Spinnler, D., Bundt, M., Blaser, P., Siegwolf, R. (2010). The input and fate of new c in two forest soils under elevated CO2. Global Change Biol. 9 (6), 862–872. doi: 10.1046/j.1365-2486.2003.00638.x
Hassanein, A., Mesbah, E., Soliman, F., Ebrahim, T. (2019). Effect of nitrogen rates, biofertilizers and foliar urea application on yield and yield components of maize (Zea mays, l.). J. Plant Production 10, 53–58. doi: 10.21608/jpp.2019.36203
Hua, W., Luo, P., An, N., Cai, F., Han, X. (2020). Manure application increased crop yields by promoting nitrogen use efficiency in the soils of 40-year soybean-maize rotation. Sci. Rep. 10 (1), 14882. doi: 10.1038/s41598-020-71932-9
Huang, B., Sun, W., Zhao, Y., Zhu, J., Yang, R., Zou, Z., et al. (2007). Temporal and spatial variability of soil organic matter and total nitrogen in an agricultural ecosystem as affected by farming practices. Geoderma 139 (3-4), 336–345. doi: 10.1016/j.geoderma.2007.02.012
Johannes, W. M. P., Peter., S., Bo, M., Jørgen, E. O. (2020). Legacy effects of soil fertility management on cereal dry matter and nitrogen grain yield of organic arable cropping systems - ScienceDirect. Eur. J. Agron. 122, 126169. doi: 10.1016/j.eja.2020.126169
King, A. E., Ali, G. A., Gillespie, A. W., Wagner-Riddle, C. (2020). Soil organic matter as catalyst of crop resource capture. Front. Environ. Sci. 8, 50. doi: 10.3389/fenvs.2020.00050
Lai, Z., Zhang, Y., Liu, J., Wu, B., Qin, S., Fa, K. (2016). Fine-root distribution, production, decomposition, and effect on soil organic carbon of three revegetation shrub species in northwest China. For. Ecol. Manage. 359, 381–388. doi: 10.1016/j.foreco.2015.04.025
Leonard, R., Marc, C., Justice, N., Giller, K. E. (2012). Maize-grain legume intercropping is an attractive option for ecological intensification that reduces climatic risk for smallholder farmers incentral Mozambique. Field Crops Res. 136, 12–22. doi: 10.1016/j.fcr.2012.07.014
Li, L., Li, S. M., Sun, J. H., Zhou, L. L., Bao, X. G., Zhang, H. G., et al. (2007). Diversity enhances agricultural productivity via rhizosphere phosphorus facilitation on phosphorus-deficient soils. P. China Basic Sci. 104, 11192–11196. doi: 10.1073/pnas.0704591104
Liu, M., Liu, M., Li, P., Yang, J., Chang, Q. (2020). Variations in soil organic carbon decompositions of different land use patterns on the tableland of loess plateau. Environ. Sci. pollut. Res. 27 (1), 1–16. doi: 10.1007/s11356-019-07099-2
Liu, W., Su, Y., Yang, R., Yang, Q., Fan, G. (2011). Temporal and spatial variability of soil organic matter and total nitrogen in a typical oasis cropland ecosystem in arid region of Northwest China. Environ. Earth Sci. 64 (8), 2247–2257. doi: 10.1007/s12665-011-1053-5
Li, Z., Zeng, Z., Tian, D., Wang, J., Fu, Z., Zhang, F., et al. (2020). Global patterns and controlling factors of soil nitrification rate. Global Change Biol. 26 (7), 4147–57. doi: 10.1111/gcb.15119
Marco, M., Tek, B. S., Bàrberi, P., Antichi, D., Risaliti, R. (2011). Long-term effect of tillage, nitrogen fertilization and cover crops on soil organic carbon and total nitrogen content. Soil Tillage Res. 114 (2), 165–174. doi: 10.1016/j.still.2011.05.001
Meng, T. (2020). Effects of tillage methods on soil organic carbon and total nitrogen content in the loess plateau. Asian Agric. Res. 12, 29–31. doi: CNKI:SUN:AAGR.0.2020-02-006
Mishra, G., Sarkar, A., Das, J. (2020). Changes in soil organic carbon lability along the altitudinal variations in three land use types of meghalaya, India. Environ. Sustain. 3, 325–331. doi: 10.1007/s42398-020-00120-2
Mohamed, A. A., Younis, S. M., Ibrahim, M. M. (2020). Effects of different tillage methods and fertilizer on soil carbon, emission of co2, and maize yield. Plant Arch. 20, 9265–9276.
Raza, M. A., Feng, L., van der Werf, W., Nasir, I., Imran, K., Hassan, M. J., et al. (2019). Optimum leaf defoliation: A new agronomic approach for increasing nutrient uptake and land equivalent ratio of maize soybean relay intercropping system. Field Crop Res. 244, 107647. doi: 10.1016/j.fcr.2019.107647
Roberta, B., Andrea, F., Stefano, S., Federico, A., Federico, C., Stefania, C. M., et al. (2020). Cover crops during transition to no-till maintain yield and enhance soil fertility in intensive agro-ecosystems. Field Crop Res. 255, 107871. doi: 10.1016/j.fcr.2020.107871
Rolf, S., Eddy de, P. (2011). Organic carbon in soils of central Asia–status quo and potentials for sequestration. Plant Soil 338 (1/2), 273–288. doi: 10.1007/s11104-010-0479-y
Segura, J. H., Sparrman, M. B., Serk, T., Öquist, H. (2019). Boreal Tree species affect soil organic matter composition and saprotrophic mineralization rates. Plant Soil 441, 173–190. doi: 10.1007/s11104-019-04105-x
Shao, Y., Chen, Z., Xiao, H., Zhu, Z., Li, B. (2019). Integrating environmental parameters and economic benefits to analyze the ecological agriculture (EA) application in the mountain rice paddy system of chongqing, China. Environ. Sci. Europe 31, 1–10. doi: 10.1186/s12302-019-0204-2
Struijk, M., Whitmore, A. P., Mortimer, S. ,. R., Tom, S. (2020). Obtaining more benefits from crop residues as soil amendments by application as chemically heterogeneous mixtures. Soil 6, 1–22. doi: 10.5194/soil-6-467-2020
Sun, Y., Wang, C., Chen, H. Y. H., Ruan, H. (2020). Responses of C:N stoichiometry in plants, soil, and microorganisms to nitrogen addition. Plant Soil 456, 1–11. doi: 10.1007/s11104-020-04717-8
Ta, T., Faris, M. (1987). Species variation in the fixation and transfer of nitrogen from legumes to associated grasses. Plant Soil 98, 265–274. doi: 10.1007/BF02374830
Tourn, S. N., Videla, C. C., Studdert, G. A. (2019). Ecological agriculture intensification through crop-pasture rotations does improve aggregation of southeastern-pampas mollisols. Soil Tillage Res. 195, 104411. doi: 10.1016/j.still.2019.104411
Veronika, J. A., Petra, S. B. C., Jan, F. A. D. (2020). Foliage C:N ratio, stage of organic matter decomposition and interaction with soil affect microbial respiration and its response to c and n addition more than C:N changes during decomposition. Appl. Soil Ecol. 152, 103568. doi: 10.1016/j.apsoil.2020.103568
Wang, P. P., Han, Y. J., Zhang, Y. (2020). Characteristics of change and influencing factors of the technical efficiency of chemical fertilizer use for agricultural production in China. Resour. Sci. 42, 1764–1776. doi: 10.18402/resci.2020.09.11
Welegedara, N. P. Y., Grant, R. F., Quideau, S. A., Landhäusser, S. M., Merlin, M., Lloret, E. (2020). Modelling plant water relations and net primary productivity as affected by reclamation cover depth in reclaimed forestlands of northern Alberta. Plant Soil 446 (1/2), 627–654. doi: 10.1007/s11104-019-04363-9
Wu, X., Wang, Y., Sun, S. (2021). Long-term fencing decreases plant diversity and soil organic carbon concentration of the zoige alpine meadows on the eastern Tibetan plateau. Plant Soil 458 (1), 191–200. doi: 10.1007/s11104-019-04373-7
Yan, X., Jin, J., Liang, M. (2017). Fertilizer use efficiencies and yield-increasing rates of grain crops in China. Soils 49, 1067–1077. doi: 10.13758/j.cnki.tr.2017.06.001
Yao, X., Yu, K., Deng, Y., Liu, J., Lai, Z. (2019). Spatial variability of soil organic carbon and total nitrogen in the hilly red soil region of southern China. J. Forestry Res. 31, 2385–2394. doi: 10.1007/s11676-019-01014-8
Yeasmin, S., Jahan, E., Molla, M. A., Islam, A., Chungopast, S. (2020). Effect of land use on organic carbon storage potential of soils with contrasting native organic matter content. Int. J. Agron. 2020, 1–9. doi: 10.1155/2020/8042961
Zhang, S. J., He, Y., Fang, H. (2003). Spatial variability of soil properties in the field based on GPS and GIS. Trans. CSAE 19, 39–44. doi: 1002-6819(2003)02-0039-06
Zhang, Z. Z., Qin, S. J., Na, L. I., Guo, L. W., Ning, T. Y., Chen, G. Q. (2013). Effects of sub soiling and n fertilizer application on dry matter accumulation,nitrogen use efficiency and yield of summer maize. J. Plant Nutr. Fertilizer 19, 790–798. doi: 10.11674/zwyf.14526
Zhang, F. S., Wang, J. Q., Zhang, W. F., Cui, Z. L., Ma, W., Chen, X., et al. (2008). Present situation of fertilizer utilization rate of main grain crops in China and improve ways. Acta Pedologica Sin. 5, 915–924.
Zhang, M., Wang, N., Zhang, J., Hu, Y., Sun, G. (2019). Soil physicochemical properties and the rhizosphere soil fungal community in a mulberry (Morus alba L.)/Alfalfa (Medicago sativa l.) intercropping system. Forests 10, 167. doi: 10.3390/f10020167
Zhang, Z., Zhang, W. (2008). The situation and trend of fertilizer application in China. Phosphate&Compound Fertilizer 23, 9–12. doi: 10.1007/s11368-020-02809-7
Zhao, Z., Zhao, Z., Fu, B., Wang, J., Tang, W. (2020). Characteristics of soil organic carbon fractions under different land use patterns in a tropical area. J. Soils Sediments 21 (2), 1–9. doi: 10.1007/s11368-020-02809-7
Zhou, L., Fu, Z., Du, Q., Chen, P., Yang, W., Yong, T. (2017). Effects of reduced n fertilization on crop n uptake, soil ammonia oxidation and denitrification bacteria diversity in Maize/Soybean relay strip intercropping system. Scientia Agricultura Sin. 50, 1076–1087. doi: 10.3864/j.issn.0578-1752.2017.06.009
Keywords: intercropping, soil organic matter and total nitrogen, spatial distribution, maize (Zea mays L.), soybean
Citation: Te X, Hassan MJ, Cui K, Xiao J, Aslam MN, Saeed A, Yang W and Ali S (2022) Effect of different planting pattern arrangements on soil organic matter and soil nitrogen content under a maize/soybean strip relay intercropping system. Front. Plant Sci. 13:995750. doi: 10.3389/fpls.2022.995750
Received: 16 July 2022; Accepted: 14 November 2022;
Published: 15 December 2022.
Edited by:
Janusz Zwiazek, University of Alberta, CanadaReviewed by:
Shen Yuan, Huazhong Agricultural University, ChinaMohammed Antar, McGill University, Macdonald Campus, Canada
Copyright © 2022 Te, Hassan, Cui, Xiao, Aslam, Saeed, Yang and Ali. This is an open-access article distributed under the terms of the Creative Commons Attribution License (CC BY). The use, distribution or reproduction in other forums is permitted, provided the original author(s) and the copyright owner(s) are credited and that the original publication in this journal is cited, in accordance with accepted academic practice. No use, distribution or reproduction is permitted which does not comply with these terms.
*Correspondence: Xiao Te, 2018101003@stu.sicau.edu.cn; Wenyu Yang, msiyangwy@sicau.edu.cn
†These authors have contributed equally to this work