- 1Institute of Soil and Environmental Sciences, University of Agriculture, Faisalabad, Pakistan
- 2Soil Bacteriology Section, Ayub Agricultural Research Institute, Faisalabad, Pakistan
- 3Institute of Molecular Biology and Biotechnology, The University of Lahore, Lahore, Pakistan
- 4Faculty of Life Sciences, University of Central Punjab, Lahore, Pakistan
- 5Agrovyzkum Rapotin Ltd., Rapotin, Czechia
- 6Department of Agrochemistry, Soil Science, Microbiology and Plant Nutrition, Faculty of AgriSciences, Mendel University in Brno, Brno, Czechia
- 7Institute of Chemistry and Technology of Environmental Protection, Faculty of Chemistry, Brno University of Technology, Brno, Czechia
- 8Institute for Environmental Studies, Faculty of Science, Charles University, Prague, Czechia
- 9Agricultural Research, Ltd., Troubsko, Czechia
Endophytic bacteria improve the growth, physiology, and metabolite profile of plants. They are known as potential biocontrol agents of soil-borne diseases. This study evaluated the effects of endophytic bacterial strains on growth, vase life, biochemical attributes, and antioxidant and nematicidal activities of French marigold (Tagetes patula). French marigold seeds were sole and consortium inoculated with three promising endophytic bacterial strains, Burkholderia phytofirmans (PsJN), Enterobacter sp. (MN17), and Bacillus sp. (MN54). The vase life of French marigold was promoted by 66.6% in the individual application of PsJN and 100% in plants treated with consortium compared to the uninoculated control. The shoot and root fresh weights were also increased by 65.9 and 68.7%, with the combined application of all three strains. The total phenolics, flavonoid, and protein contents were higher in consortium treatment with an increase of up to 38.0, 55.9, and 65.9%, respectively, compared to the uninoculated control. Furthermore, combined application of endophytic bacterial strains promoted DPPH radical scavenging, mortality of plant-parasitic nematodes, and ferric reducing antioxidant power activities with increase of up to 278.0, 103.8, and 178.0%, respectively, compared to uninoculated control. An increase in antioxidant activities of ascorbate peroxidase (APX), catalase (CAT), glutathione peroxidase (GPX), and superoxide dismutase (SOD) were observed up to 77.3, 86.0, 91.6, and 102.9%, respectively by combined application of endophytic bacterial strains. So, given the economic importance of floriculture crops, endophytic bacterial isolates studied here have shown a great potential for improving the productivity of cultivated ornamental French marigold.
Introduction
Floriculture contributes excellent value to the agricultural economy of Pakistan. Significant growth has been observed in this sector resulting in massive production of ornamental plants (Sudhagar, 2013; Khan et al., 2016). French marigold (Tagetes patula) is a commercial ornamental flower used in medicinal products for decoration and landscaping purposes (Bosma et al., 2003). It is also a repellent plant against various Lepidoptera, Coleoptera, Hemiptera, and plant-parasitic nematodes (PPNs; Fabrick et al., 2020). French marigold is a widely studied plant due to its allelopathic potential against PPNs (Hooks et al., 2010). Literature indicated that marigolds could be involved in the biocontrol of 14 genera of PPNs, including ectoparasitic, endoparasitic, and semi-endoparasitic nematodes (Suatmadji, 1969; Siddiqui and Alam, 1987). Marigold affects PPNs by acting as a poor host, producing allelopathic compounds, creating an antagonistic nematode environment, and behaving as a trap crop (Wang et al., 2001; Pudasaini et al., 2008). The expansion of environmentally safe techniques to inhibit PPNs growth seems attractive due to the environmental hazards of nematicides and chemical fumigants (Schneider et al., 2003).
The commercial production of ornamental plants is significant globally, including in Pakistan. Essential plant nutrients are vital in the quality production of seeds and flowers (Kashif, 2001). Over the past decades, extensive use of chemical fertilizers has negatively impacted the environment. In this regard, the application of bioinoculants has been gaining interest in the scientific communities (Zahir et al., 2004; Khan et al., 2019; Nazli et al., 2020). Several plant growth-promoting rhizobacteria (PGPR) have shown their potential to improve plant growth, crop yield, and quality (Ali et al., 2017; Mustafa et al., 2019; Umar et al., 2020). Such bioinoculants have direct effects on plant growth as these species facilitate nutrient uptake, fix atmospheric nitrogen (N), solubilize nutrients including phosphorus (P), potassium (K), and zinc (Zn), produce siderophores which solubilize and sequester iron, synthesize growth hormones (e.g., auxins, cytokinins, and gibberellins), and synthesize the enzymes that modulate plant growth and development (Gray and Smith, 2005; Khan and Bano, 2019; Saeed et al., 2019). These microbes enhance plant growth indirectly by reducing or eliminating the adverse effects of pathogenic microorganisms using numerous mechanisms that include the induction of host resistance to the pathogen (Van Loon, 2007; Khan, 2020; Khan et al., 2020; Nazli et al., 2020). Multiple studies have demonstrated the improvement in plant growth and development following seed or root inoculation with microbial strains capable of producing plant growth regulators (Zahir et al., 2004; Naseem et al., 2018; Ahmad et al., 2019b).
Certain aspects of PGPR interactions have been studied well, e.g., growth effects, nutrient availability, biocontrol of plant pathogens, tolerance of water stress, and other adverse environmental conditions. The microbiota within plant roots may significantly differ from that within the rhizosphere, indicating that the plants influence the microbial colonies inside their roots (Ali et al., 2017). Some endophytes can promote plant growth, and the mechanisms adopted by bacterial endophytes are similar to those used by rhizospheric bacteria (Santoyo et al., 2016). Within their plant host, the microbes named endophytes remain in the intercellular or intracellular region of healthy plant tissue throughout their complete life cycle, causing no harmful impact on the plant. By secreting phytohormones, endophytic bacteria promote the growth of their host. Endophytic bacteria can also promote the growth and yield of a plant by acting as biocontrol agents (Mustafa et al., 2019).
Flower senescence is the leading cause of short vase life and loss in quality which primarily determines its economic and ornamental value to establish a capital-incentive business (Van Doorn, 2002). Ethylene is a crucial stress regulatory phytohormone produced at low levels under normal circumstances and conferring beneficial effects on plant growth and development (Ahmad et al., 2020). However, in response to various stresses, there is often a significant increase in endogenous ethylene production that leaves adverse impacts on plant growth and is thought to be responsible for flower senescence (Van Doorn, 2001). Endophytic microbes having ACC-deaminase activity may naturally enhance plant growth under stress conditions by lowering ethylene production (Nascimento et al., 2012; Nazli et al., 2020). Flower senescence can be delayed by a reduction in ACC content, causing the synthesis of ethylene to a smaller extent (Moon and Ali, 2022). Previously, several studies have reported positive effects of PGPR and endophytic inoculation on plant growth promotion of different crops. However, the impact of microbial consortium inoculation remained neglected in the past, especially in horticultural crops. Moreover, there is a need to explore the effects of microbial inoculation on pharmacological and nematicidal activities of crops such as French marigold, which in the present study constitutes the further novelty of this work. Thus, we hypothesized that the endophytic bacterial inoculation in consortia might improve the growth and extends the vase life of French marigold; however, their effects on pharmacological and nematicidal activities may vary depending on the microbial sp. in question. The specific objectives of the present study were to evaluate the impact of different endophytic bacterial species on growth, flowering, and delay in flower senescence in French marigolds and the effects of bacterial endophytes on pharmacological and nematicidal activities of essential flower extract of French marigolds.
Results
Plant growth parameters
The present study shows the effects of endophytic bacteria strain Burkholderia phytofirmans PsJN, Enterobacter sp. MN17, and Bacillus sp. MN54 inoculation on French marigold growth and senescence. Almost all the growth parameters evaluated were significantly (p < 0.05) modified by four inoculating treatments.
Inoculation with endophytic bacteria caused a significant increase in plant height, shoot mass, root mass, and root length (Figure 1). All the five treatments statistically differed from each other. The increase in plant height up to 15.98 cm was recorded in the consortia, followed by MN17 showed an increase in plant height (14.33 cm) which was significantly different from control. The minimum plant height of 10.67 cm was found in control. The difference in plant height in PsJN, MN17, MN54, and consortia was quite evident compared to control. Data regarding shoot fresh weight revealed that application of endophytic bacterial strains significantly improved the shoot fresh weight plant–1. The maximum increase in shoot fresh weight up to 26 g plant–1 was recorded in the consortia application, followed by MN54, MN17, and PsJN showed an increase in shoot fresh weight up to 24.33, 22.00, and 19.68 g plant–1, respectively, compared to control (15.67 g plant–1; Figure 1).
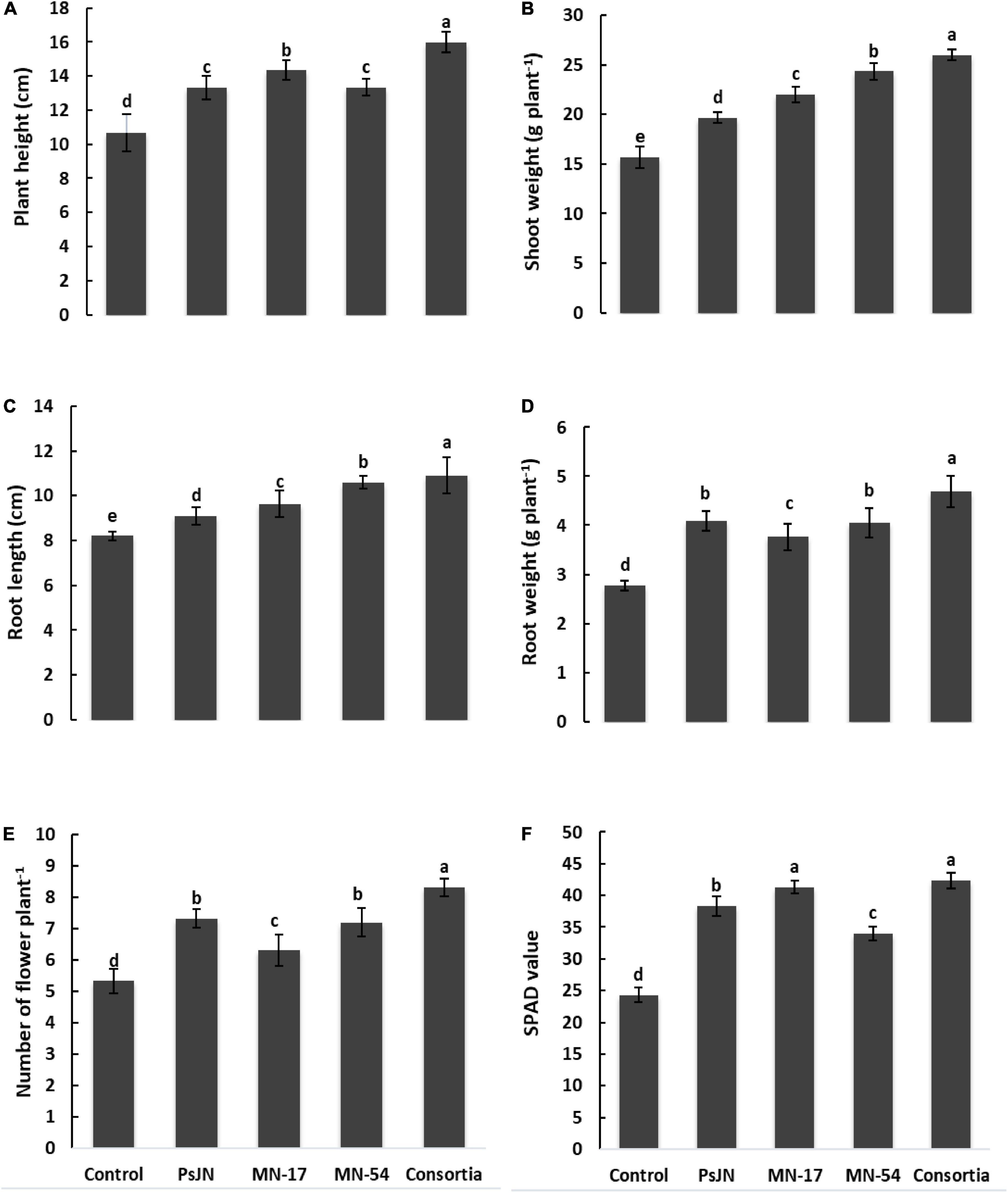
Figure 1. Effect of endophytic bacterial inoculation on (A) plant height, (B) shoot weight, (C) root length, (D) root weight, (E) number of flowers plant–1, and (F) SPAD value of French marigold. Error bars represent the standard error (SE). Bars with different letters are significantly different (P < 0.05) according to the LSD test.
Inoculation with MN54 significantly affects root length (10.60 cm) and root fresh weight (4.05 g plant–1), which was further increased in consortia application (10.90 cm and 4.69 g plant–1, respectively) as compared to control (8.2 cm and 2.78 g plant–1, respectively; Figure 1). Individual inoculation of PsJN and consortia significantly increased the number of flowers plant–1 up to 7.33 and 8.33, respectively, over control which reported 5.30 number of flowers plant–1.
Endophytic bacteria extend the vase life
The vase life of French marigold inoculated with a consortium of all three endophytic bacterial strains was 12 days compared to 6 days of control (Figure 2). Corresponding treatments of PsJN prolonged vase life by 10 days. Similarly, reduction in petal senescence was also significantly increased up to 8 days by MN17 and MN54. The inoculation with PsJN and consortia reported an increase in flower diameter up to 42.31 and 45.33 mm, respectively, compared to control (25.67 mm; Figure 2).
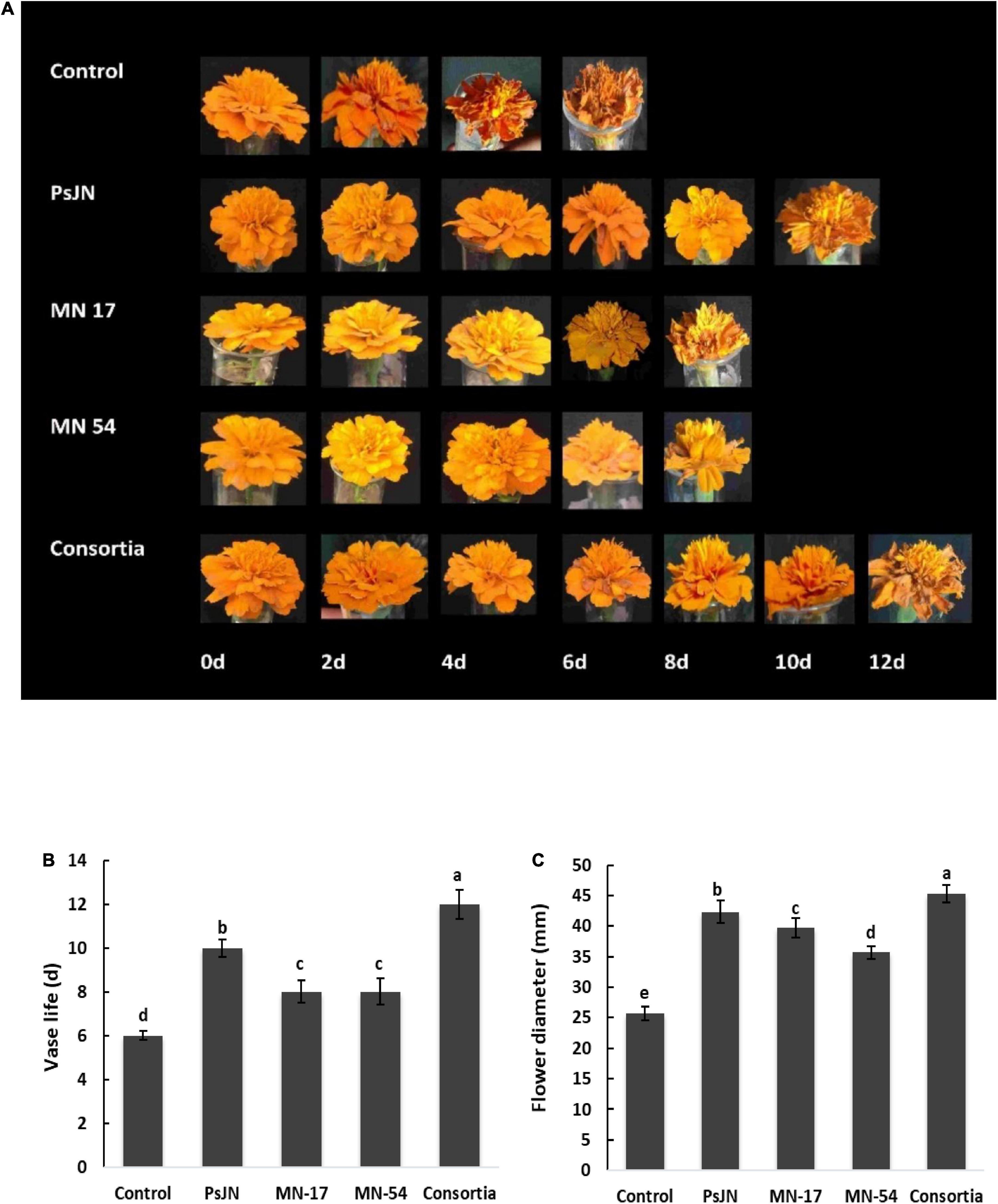
Figure 2. Effect of endophytic bacterial inoculation on (A,B) vase life and (C) maximum flower diameter of French marigold. Error bars represent the standard error (SE). Bars with different letters are significantly different (P < 0.05) according to the LSD test.
Photosynthetic parameters and SPAD index
The chlorophyll contents in terms of SPAD value were higher in endophytic bacterial strains treated plants compared to the uninoculated control (Figure 1). A maximum SPAD value of 42.33 was observed in consortium treated plants, followed by strain MN17 (41.30) over the uninoculated control (24.30). The consortium application gave a maximum increase of up to 36.84% in photosynthetic rate compared to the control (Figure 3). Strain PsJN inoculation also reported a better increase in the photosynthetic rate of up to 23.21% compared to the uninoculated control. A maximum increase in transpiration rate of up to 26.82% was observed due to consortium application. The application of PsJN also showed a better transpiration rate with an increase of up to 19.51% over uninoculated control (Figure 3). The consortium application showed a significant increase of up to 54.54 and 23.41% in stomatal and sub-stomatal conductance, respectively, compared to the untreated control.
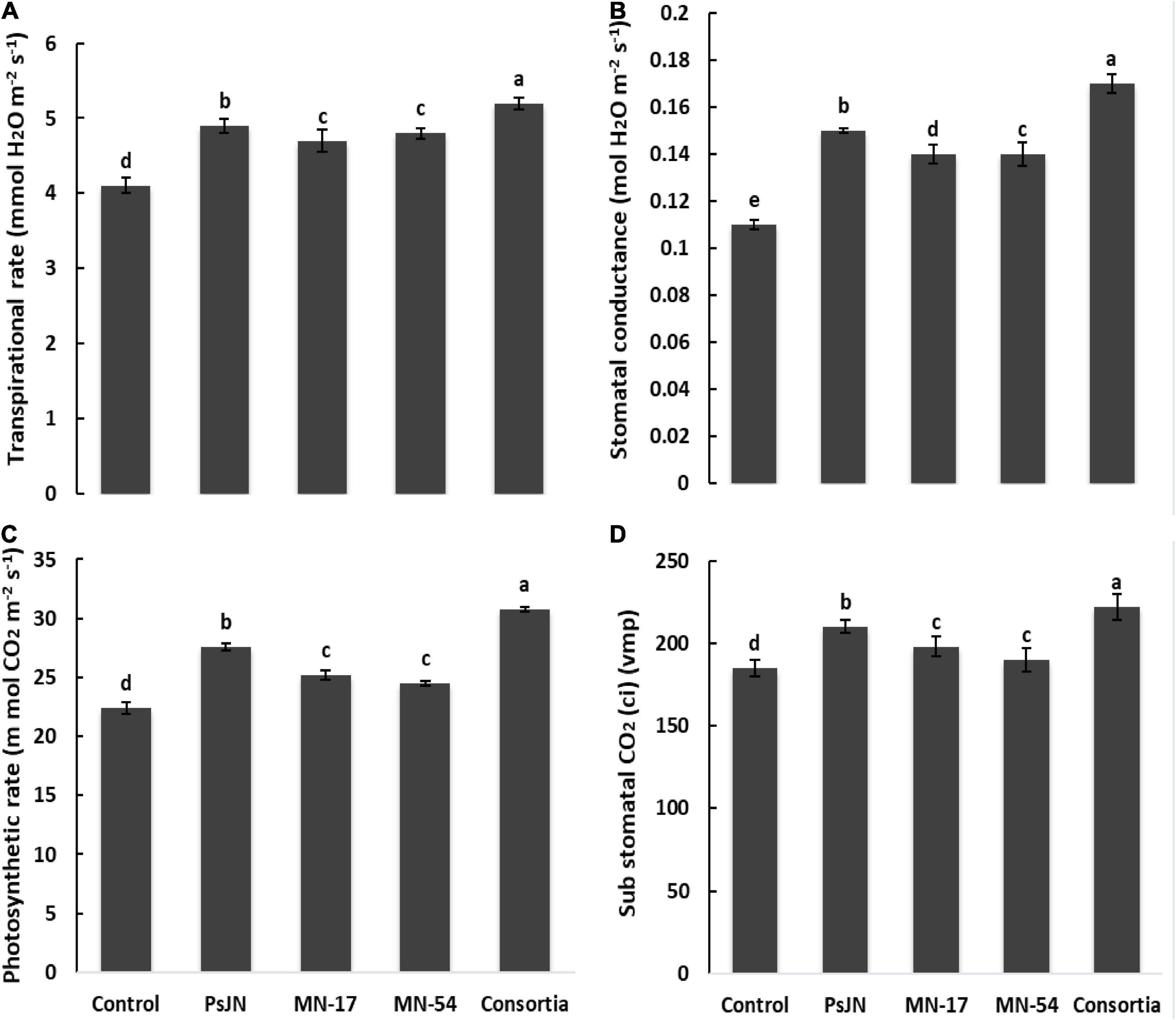
Figure 3. Effect of endophytic bacterial inoculation on (A) transpiration rate, (B) stomatal conductance, (C) photosynthetic rate, and (D) substomatal conductance of French marigold. Error bars represent the standard error (SE). Bars with different letters are significantly different (P < 0.05) according to the LSD test.
Biochemical and antioxidant parameters
The increase in total phenolic and flavonoid contents was observed due to inoculation with endophytic bacterial strains (Figure 4). The consortium application reported maximum phenolic contents of 602.67 mg g–1 followed by strain PsJN (596.0 mg g–1) than the uninoculated control (435.67 mg g–1; Figure 4). A maximum increase in total flavonoid contents of 304.30 mg g–1 was recorded in strain PsJN treated plants compared to the untreated control (157.32 mg g–1). The maximum protein contents of 6.27 μg ml–1 were observed in consortium treated plants, followed by PsJN inoculated plants which reported 5.67 μg ml–1 of total protein contents compared to the control having 3.78 μg ml–1 of total proteins contents (Figure 4).
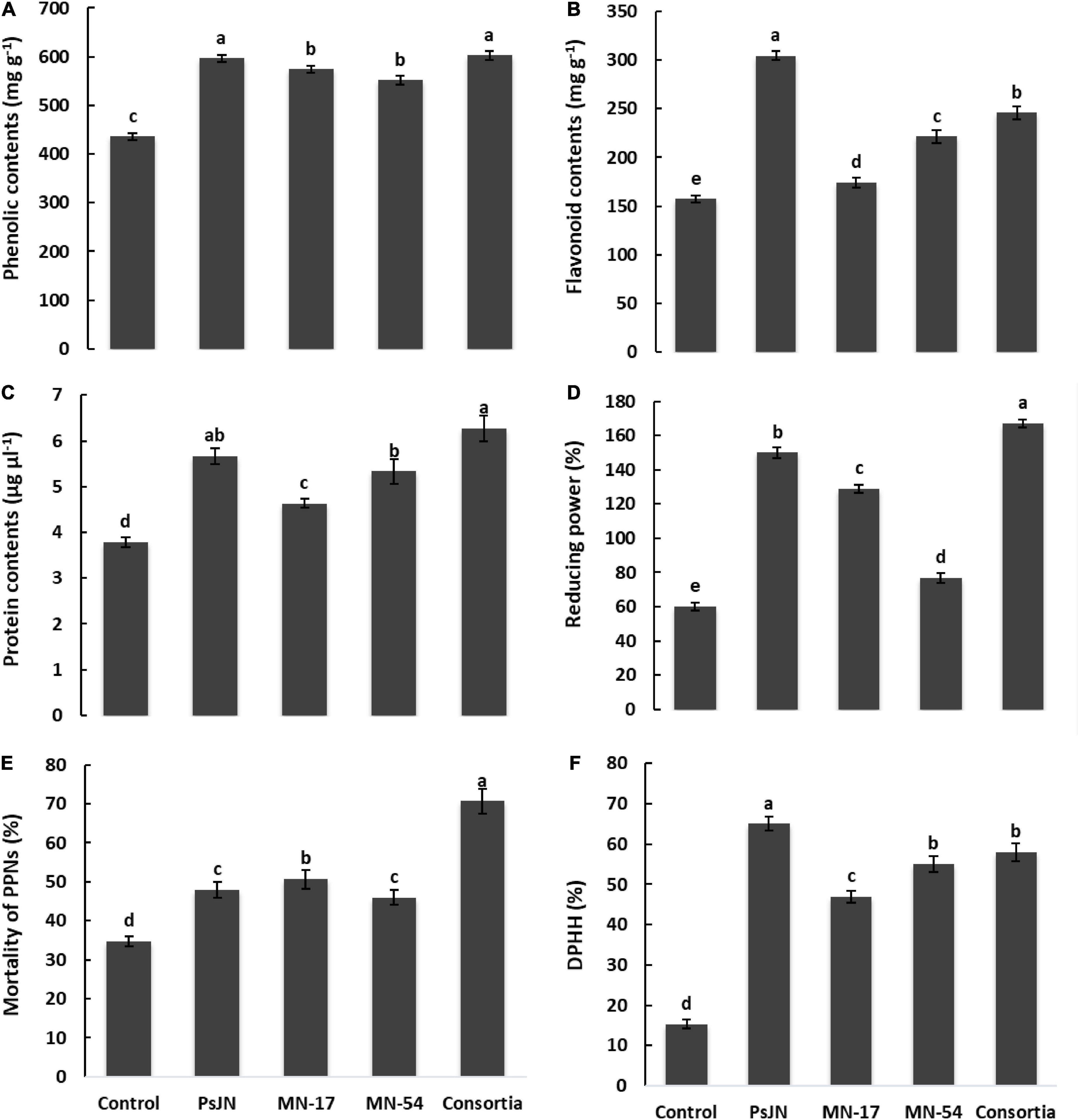
Figure 4. Effect of endophytic bacterial inoculation on (A) phenolic contents, (B) flavonoid contents, (C) protein contents, (D) ferric reducing power, (E) mortality of PPNs, and (F) DPPH radical scavenging activity of French marigold. Error bars represent the standard error (SE). Bars with different letters are significantly different (P < 0.05) according to the LSD test.
The positive effect of tested endophytic strains and their consortium application on ferric reducing power of French marigold flower extract had been demonstrated in Figure 4. Strain PsJN treated plants reported a higher ferric reducing ability of 150%, which was further extended up to 167% in consortium treated plants compared to the uninoculated control having a ferric reducing power of 60% (Figure 3). Strains MN17 and MN54 also showed a better ferric reducing ability of 129 and 77%, respectively, compared to the uninoculated control. Inoculation with endophytic bacterial strains reported increased DPPH scavenging activity of French marigold flower extract compared to the uninoculated control (Figure 4). The consortium application reported higher DPPH scavenging activity of 58% than the uninoculated control of 15% DPPH scavenging activity.
The increase in antioxidant activities was observed due to endophytic bacterial strain over control treatment (Figure 5). Strain PsJN, MN17, and MN54 increased the ascorbate peroxidase (APX) to 31.9, 48.6, and 24.6%, respectively. However, consortium application increased the APX up to 77.3% over treatment set as control. Similarly, strain MN17 increased the catalase (CAT), glutathione peroxidase (GPX), and superoxide dismutase (SOD) up to 48.6, 60.2, and 65.8%, respectively. However, combined application of endophytic bacterial strains increased the CAT, GPX, and SOD activity to 86.0, 91.6, and 102.9%%, respectively, over control treatment.
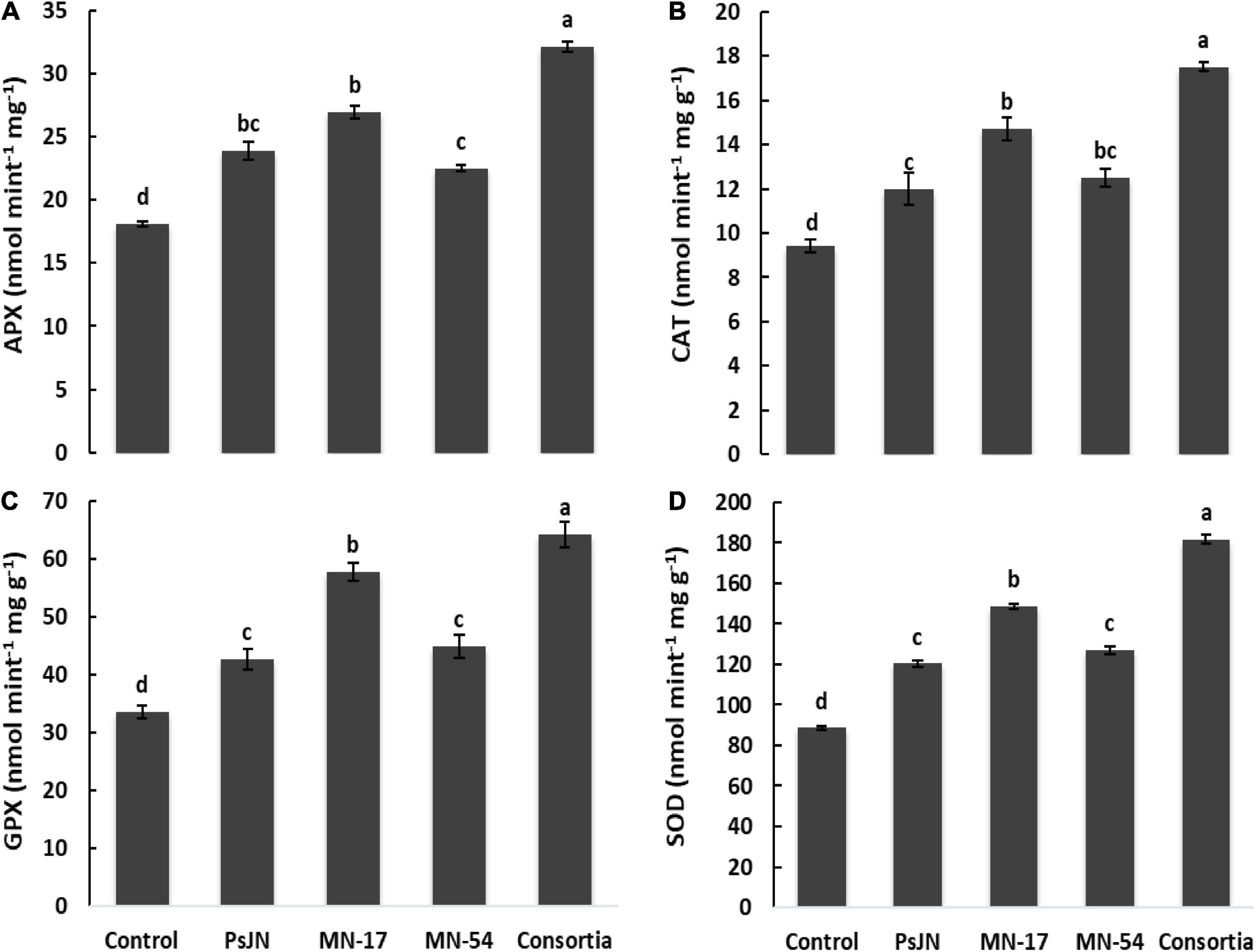
Figure 5. Effect of endophytic bacterial inoculation on (A) ascorbate peroxidase (APX), (B) catalase (CAT), (C) glutathione peroxidase (GPX), and (D) superoxide dismutase (SOD) activity of French marigold. Error bars represent the standard error (SE). Bars with different letters are significantly different (P < 0.05) according to the LSD test.
Nematicidal and hemolytic activities
French marigold flower extract from endophytic bacterial strains treated plants reported nematicidal activity against Meloidogyne incognita (Figure 4). The maximum nematicidal activity of up to 70.7% was recorded in consortium treatment, followed by strain MN17 which reported 50.7% higher nematicidal activity than uninoculated control. French flower extract of endophytic bacterial strains treated plants causes a reduction in hemolytic activity compared to the uninoculated control (Figure 6). A maximum decrease of 1.33, 1.30, and 1.30% in hemolytic activity of French flower extract was reported by strains PsJN, MN17, and MN54, respectively, over the uninoculated control of 2.6% hemolytic activity.
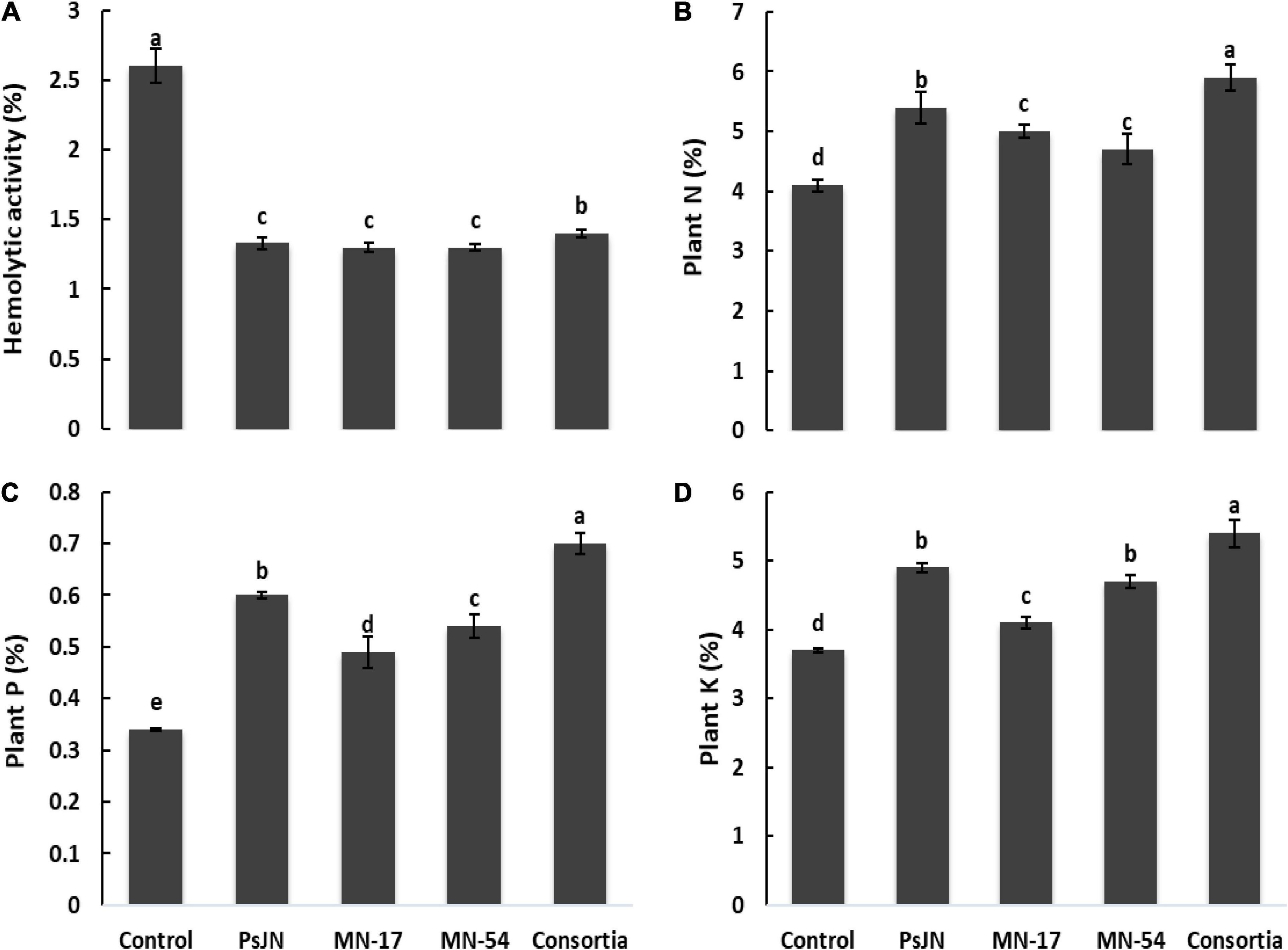
Figure 6. Effect of endophytic bacterial inoculation on (A) hemolytic activity, (B) plant N (%), (C) plant P (%), and (D) plant K (%) in French marigold. Error bars represent the standard error (SE). Bars with different letters are significantly different (P < 0.05) according to the LSD test.
Plant mineral contents
The N, P, and K contents in a shoot of French marigold were significantly promoted by inoculation with endophytic bacterial strains compared to the uninoculated control (Figure 6). The maximum increase of up to 43.9% in shoot N contents was observed in consortium treated plants followed by strain PsJN, which reported 37% higher shoot N contents compared to the uninoculated control. The maximum increase of up to 105.9 and 45.9% in shoot P and K contents, respectively, were obtained due to consortium application compared to the uninoculated control (Figure 6). Strain PsJN also reported a better increase of up to 60 and 33.3% in shoot P and K contents, respectively, over the uninoculated control.
Enumeration of endophytic bacteria in the rhizosphere, root, shoot, and flowers
Effective colonization of the applied strains was observed in the rhizosphere, root/shoot/leaves/flowers interior of French marigold (Figure 7). However, when used as a consortium, the persistence of selected strains was more enhanced relative to individual inoculation in the rhizosphere and tissue interior of French marigold plants. Inoculation with PsJN showed 4.49 × 105 CFU g–1 rhizosphere, 3.36 × 105 CFU g–1 root interior, 8.64 × 104 CFU g–1 shoot interior, and 8.04 × 104 CFU g–1 flowers bacterial population. However, the highest CFU g–1 dry weight of the inoculant strains was recovered from the rhizosphere (5.83 × 105), root (4.53 × 105), shoot (1.21 × 105), and flowers (9.80 × 104) in the consortium treatment.
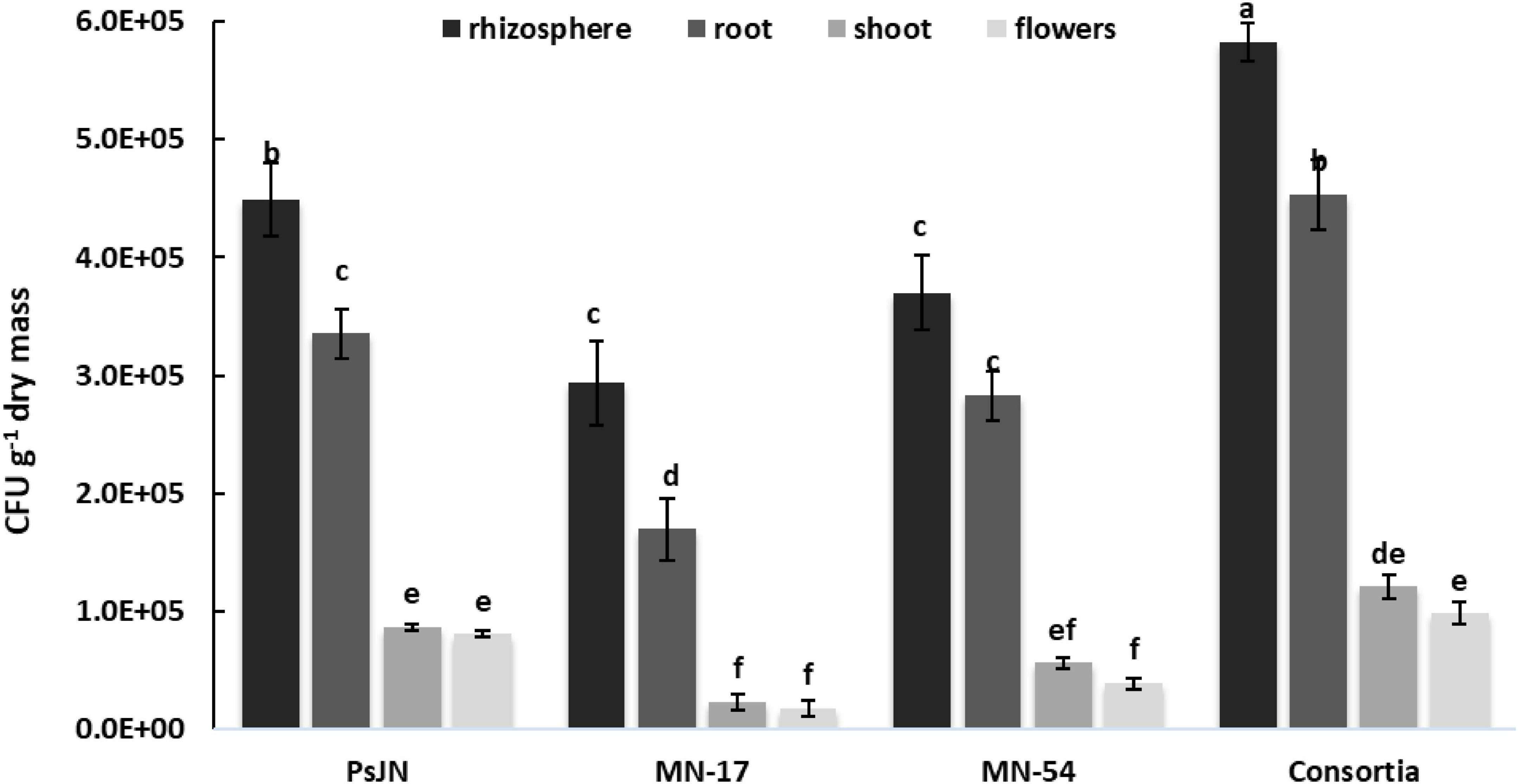
Figure 7. Persistence of selected endophytic strains in the rhizosphere, root, shoot, leaves, and flowers of French marigold. Error bars represent the standard error (SE). Bars with different letters are significantly different (P < 0.05) according to the LSD test.
Pearson correlation and principal component analysis
A significant positive correlation was observed in growth attributes, vase life, biochemical parameters, physiological parameters, biological activities, and plant mineral contents (Figure 8).
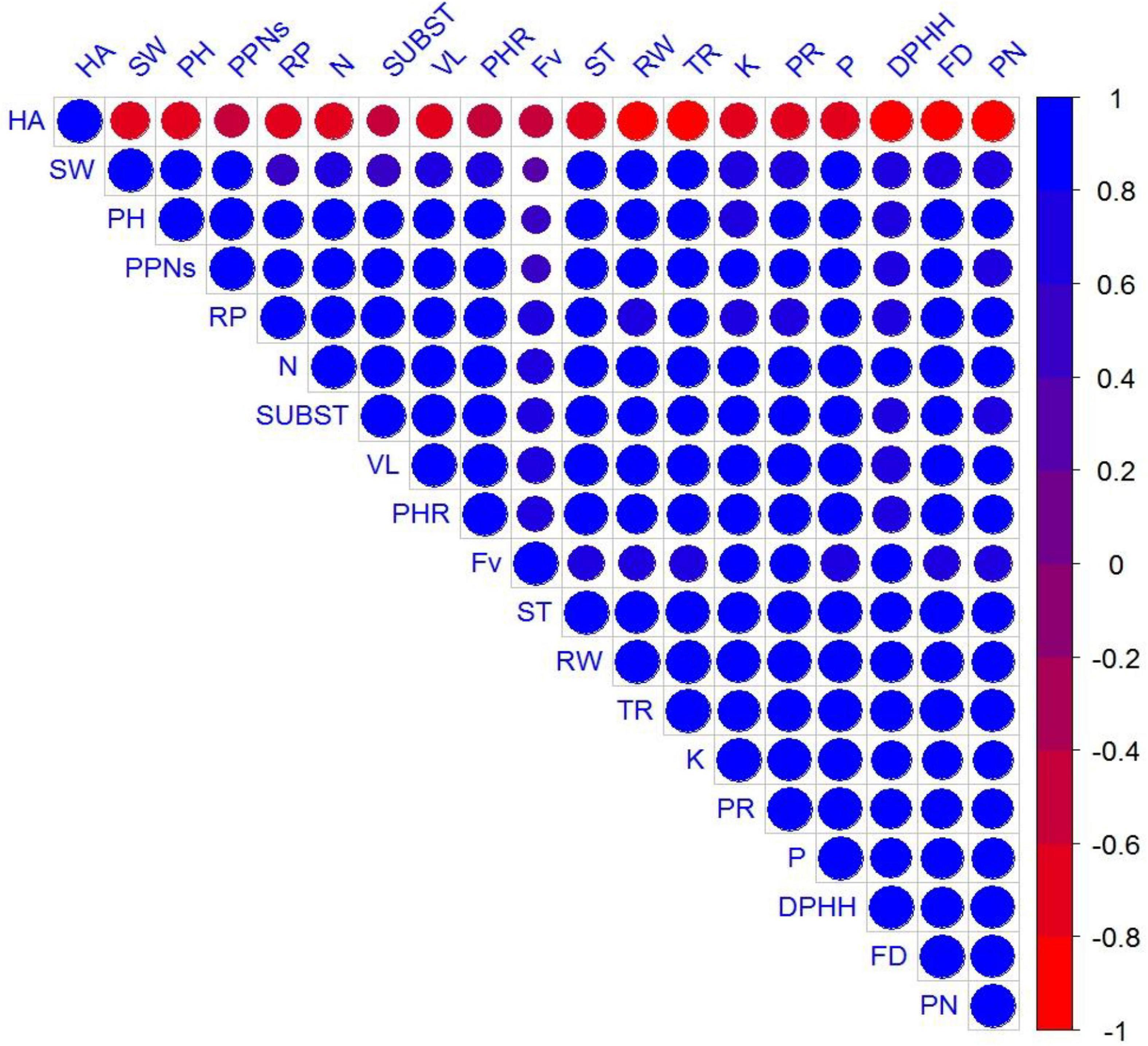
Figure 8. Represents correlation among measured parameters, where abbreviations of the correlation matrix are plant height (PH), shoot weight (SW), root weight (RW), flower diameter (FD), total flavonoids contents (FV), phenolic contents (PN), protein contents (PR), DPHH scavenging activity (DPHH), hemolytic activity (HA), vase life (VL), reducing power (RP), mortality of PPNs (PPNs), transpiration rate (TR), photosynthetic rate (PHR), stomatal conductance (ST), sub stomatal conductance (SUBST), plant N (N), plant P (P), and plant K (K).
Principal component analysis (PCA) revealed interrelationships between different variables. The score and loading plots of PCA on some crucial traits of French marigold are shown in Figures 9A,B. Among all the components, the first two components viz. PC1 (Dim1) and PC2 (Dim2) exhibited maximum contribution and accounted for 91.5% of the total dataset. Of which PC1 contributed 85.7% while PC2 contributed 5.8%, respectively. All applied treatments were successfully separated by the first two principal components (Figure 9A). The distribution of the treatments gave a clear indication that endophytic bacterial strains sole or their consortium application had significant effects on studied attributes of French marigold compared to control. The sole application of endophytic bacterial strains was displaced more from control, and the consortium treatment was more displaced from control, indicating that it has a more pronounced effect. The first group of variables with which PC 1 is positively correlated includes plant height (PH), shoot weight (SW), root weight (RW), flower diameter (FD), total flavonoids contents (FV), phenolic contents (PN), protein contents (PR), DPHH scavenging activity (DPHH), hemolytic activity (HA), vase life (VL), reducing power (RP), mortality of PPNs (PPNs) transpiration rate (TR), photosynthetic rate (PHR), stomatal conductance (ST), sub stomatal conductance (SUBST), plant N (N), plant P (P), and plant K (K).
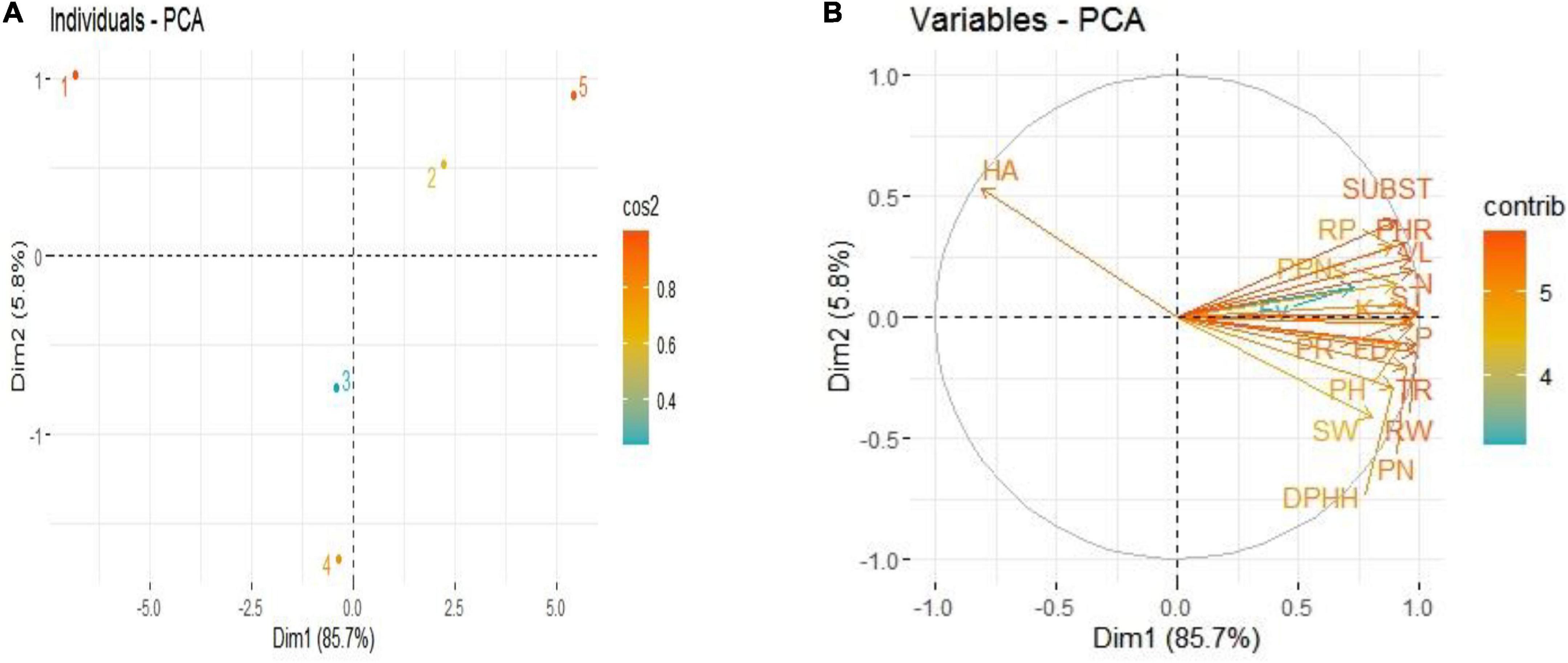
Figure 9. Score plots (A) and loading plots (B) of PCA on different attributes of French marigold by application of Endophytic bacteria alone and in combination. Score plots (A) represent the separation of treatments as (1) Control, (2) PsJN inoculation, (3) MN 17, (4) MN 54, and (5) Consortia. The abbreviations of loading plots (B) are plant height (PH), shoot weight (SW), root weight (RW), flower diameter (FD), total flavonoids contents (FV), phenolic contents (PN), protein contents (PR), DPHH scavenging activity (DPHH), hemolytic activity (HA), vase life (VL), reducing power (RP), mortality of PPNs (PPNs), transpiration rate (TR), photosynthetic rate (PHR), stomatal conductance (ST), sub stomatal conductance (SUBST), plant N (N), plant P (P), and plant K (K).
Discussion
Chemical inhibitors such as silver thiosulphate (Veen and van de Geijn, 1978; Hyde et al., 2020), cyclic olefin norbornadiene (Reid and Wu, 1992; Depaepe and Van Der Straeten, 2020), and L-a-(aminoethoxyvinyl)-glycine (Nayani et al., 1998; Yu et al., 2022) are applied to inhibit plant ethylene production and to promote vase life of ethylene-sensitive flowers. However, the use of different chemicals has a variety of drawbacks associated with their application rate and method and high costs. Applying bio-effectors containing beneficial microorganisms instead of synthetic chemicals improves plant growth by supplying plant nutrients and may sustain environmental health and soil productivity. In the present study, three promising bioinoculants, including B. phytofirmans strain PsJN, Enterobacter sp. strain MN17, and Bacillus sp. strain MN54, were applied to French marigold seeds in the forms of sole inoculation and their consortium treatments. The treated plants were grown in a pot trial to investigate the effects of endophytic bacterial strains on plant attributes in terms of vase life, physiological, biochemical, antioxidant, and biological activities. By adopting the current biotechnological approach, we hypothesized that bioinoculants could competently dwell in the French marigold rhizosphere and improve the quality of their flowers in terms of their growth, vase life, chemical constituents, physiological, and biological activities (Dal Cortivo et al., 2020).
Endophytes improve the growth attributes of French marigold plants
To sustain ornamental crop production under limited available resources, the greenhouse growers need to reduce the cost of production in terms of chemical fertilizers without losing crop quality. PGPR can improve plant growth in a resource-poor environment by improving plant nutrient availability. In the current study, the application of endophytic bacterial strains in sole inoculation and/or consortium treatment enhances the plant growth of French marigold in terms of plant height, root length, shoot weight, and root weight (Figure 1). The French marigold plants treated with a consortium of strains B. phytofirmans (PsJN), Enterobacter sp. (MN17), and Bacillus sp. (MN54) showed maximum increase in plant growth attributes compared to sole inoculation that may be due to accumulative and synergistic effect of endophytic bacterial strains that boosted the plant growth through involving their direct and indirect impact (Doty et al., 2009; Suherman and Anggoro, 2011; El-Deeb et al., 2012; Mustafa et al., 2019; Aziz et al., 2020; Nordstedt and Jones, 2020; South et al., 2021). These strains showed their potential in vitro plant growth-promoting (PGP) attributes, including production of indole-3-acetic acid and siderophore, solubilization of nutrients, and nitrogen fixation, which our research group previously reported (Samreen et al., 2019; Naveed et al., 2020a; Sabir et al., 2020). Similarly, Puri et al. (2020) said that Caballeronia sordidicola isolated from spruce seedlings in low-fertility soil possess PGP attributes and promoted plant growth attributes of spruce and pine tree seedlings. In this study, the increase in plant growth of French marigold due to the application of a consortium of endophytic bacterial strains might also be due to the production of phytohormones, including auxins, gibberellins, ethylene, cytokinin, and abscisic acid that can stimulate plant growth as chemical messengers. These hormones are essential in regulating plant growth and development in plants by regulating the process of organogenesis, cell division, expansion, and differentiation (Ryu and Patten, 2008).
Endophytes improve physiological attributes of French marigold plants
In this study, the application of endophytic bacterial strains promoted plant physiological attributes, including chlorophyll contents, transpiration rate, photosynthetic rate, and stomatal and sub-stomatal conductance compared to the uninoculated control (Figures 1, 3). The beneficial influence of endophytic bacterial strains was more dominant in consortium treatment than in sole inoculation of strains. These beneficial effects of plant-bacteria interaction were previously reported by various researchers under numerous environmental stresses, including drought (Naveed et al., 2014), salinity (Ahmad et al., 2014), heavy metals (Naveed et al., 2020a,b), and hydrocarbon toxicity (Ali et al., 2020). Increased physiological attributes due to bacterial inoculation improved plant growth and biomass production of French marigolds. The increase in chlorophyll content due to a consortium of endophytic strains could be related to the established factor of higher enzymatic activities such as catalase and peroxidase (Singh, 1988; Kavino et al., 2010). The plant-associated bacteria logically boosted enzymatic activity due to their abundant biomass, higher metabolic activity, and extracellular enzyme production. They are also involved in plant defense by eliciting multiple antioxidant enzymes and counteracting oxidative stress by reactive oxygen species (Prasanna et al., 2013, 2017; Ibiang et al., 2017). The consortium of endophytic strains could be involved in regulating the transpiration of water and penetration of CO2 into the leaf by maintaining optimum moisture content. Such physiological phenomena could be helpful in rainfed cultivation by closing stoma and reducing water loss by preventing transpiration.
The application of PGPR showed their great prospect for improving nutrient availability and reducing the excessive application of chemical fertilizer. In the current study, inoculation with endophytic bacterial strains improves N, P, and K concentration in a shoot of marigold flower plants (Figure 5). The increase in nutrient concentration was more prominent in consortium treatment as compared to the sole application of endophytic bacterial strains. This increase in nutrient concentration could be due to the ability of endophytic bacterial strains to increase the bioavailability of nutrients in the soil and to improve its uptake and accumulation. Previously, we have reported the tested strains viz. B. phytofirmans (PsJN), Enterobacter sp. (MN17), and Bacillus sp. (MN54) in vitro ability to solubilize nutrients, especially P might improve its bioavailability in soil and enhance uptake and accumulation in plants shoot (Samreen et al., 2019; Naveed et al., 2020a; Sabir et al., 2020). Such nutrient solubilizing bacterial strains might also have the ability to solubilize other nutrients, including K and zinc, which can be possible in the current study as we have observed the increase in K concentration in plants of the marigold flower. Our results are similar to the findings of Khanghahi et al. (2018), Mumtaz et al. (2018, 2020), and Ahmad et al. (2019a). Such nutrients solubilizing bacteria adopted a variety of mechanisms, including acidolysis, reduction in pH, enzymolysis, and complexation through extracellular polysaccharides (Welch et al., 1999; Parmar and Sindhu, 2013). The mechanisms of association of plant and endophytic bacterial strains are not precise for mineral solubilization that might result from the secretion of organic acids of ligands specific to elements (Duangpaeng et al., 2012). Such ligands can alter substrate pH and enhance insoluble compounds’ chelation (Forchetti et al., 2010; Khan et al., 2020). The free-living rhizosphere and endophytic bacteria also demonstrated N-fixation in roots of nonleguminous crops (Nag et al., 2020) which might be valid for the current endophytic bacteria strains that showed an increase in N concentration in marigold flower plants. The precise mechanism of association of free-living endophytic bacteria with plant species is not well-known. It may be due to the presence of plant-specific root exudates that recruits plant-available bacteria and carry the microbiota from one generation to another (Toju et al., 2018; Van Deynze et al., 2018; Eyre et al., 2019).
Endophytes improve the vase life of French marigold flowers
Bacteria associated with plants improve the quality of their flowers. In the present study, the uninoculated control revealed the lowest flower quality in terms of their numbers, diameters, and vase life (Figures 1, 2) which might be due to higher ethylene production during the flower ripening process. Higher ethylene production at ripening time causes several stress changes, including rapid loss of chlorophyll, proteolysis, loss of catalase activity, and increased membrane permeability (Ranwala and Miller, 2005). However, the increase in these flower’s quality attributes of marigold was observed due to the sole and/or consortium inoculation with endophytic bacterial strains, which might be due to the ability of these bacteria to produce ACC-deaminase that reduces the ethylene level in plants and delay flower ripening (Moon and Ali, 2022). This ethylene reduction in plants by ACC-deaminase-producing bacteria is a critical property that enables interference with the physiological processes of the host plant (Eun et al., 2019; Ali et al., 2022). Various commercially available chemicals such as potassium permanganate, ultraviolet lamps, activated charcoal, and catalytic oxidizers inhibit the ethylene concentration in fruits and flowers to improve vase life (Ebrahimi et al., 2021). However, improving the vase life of flowers through applying rhizosphere and endophytic bacteria is a novel biotechnological approach that is quite efficient in increasing flower quality and composition.
Endophytes improve biochemical and antioxidant attributes of flowers extract
We reported the increase in the total content of phenolics, flavonoids, and protein in French marigold flower extract due to inoculation with endophytic bacterial strain (Figure 4). The consortium of endophytes mediated responses in French marigold flower extract promoted these metabolites in flower extract that played a cumulative, synergistic role in the enhancement of plant growth and flower quality. Previously, various researchers showed evidence of total proteins, phenolics, and flavonoid contents in plant-microbe interaction (Weir et al., 2004; Brencic and Winans, 2005; Bhattacharya et al., 2010). The flavonoid and phenolic contents in the extract of French marigolds could quench free radicals and act as antioxidants involved in anti-inflammatory and anti-carcinogenic responses (Biglari et al., 2008). We observed higher flavonoid and phenolic contents in marigold flower extract due to the consortium application of endophytic bacterial strains that could manifest higher antioxidant capacities. Further, consortium application also reported a higher increase in antioxidant activities in terms of DPPH radical scavenging, APX, CAT, GPX, and SOD activity and ferric reducing power of extract from French marigold flowers (Figure 4). Li et al. (2007) analyzed the 11 Chinese cultivars of marigold flowers extracted with ethanol, ethyl acetate, and n-hexane through high-performance liquid chromatography-mass spectrometry. They reported marked variation in total phenols, flavonoids, antioxidants, and radical-scavenging activities in the tested marigold cultivars.
Similarly, Duan et al. (2007) and Liu et al. (2009) reported polyphenols extracted from lychee-fruit pericarp to possess the scavenging activity against DPPH free radical, superoxide anions, and hydroxyl radicals. Additionally, a reduction in hemolytic activity was observed in the current study due to inoculation with sole and demonstrating the ability of endophytic bacterial strains to reduce the hemolytic activity of French marigold flower extract. The reduction in hemolytic activity was more in sole inoculation than in a consortium which might be due to the application of a single organism in sole inoculation with endophytic bacteria. The reduction in hemolytic activity due to inoculation can be due to the increased production of antioxidants because of bacterial application in French marigold plants.
Endophytes improve the nematicidal activity of French marigold flowers extract
Some marigold varieties are resistant to PPNs due to their allelopathic potential (Wang et al., 2007; Hooks et al., 2010). A blue-fluorescing compound called α-terthienyl in marigold plants was recognized for its allelopathic potential against nematodes, insects, fungi, and viruses and cytotoxic activities (Zechmeister and Sease, 1947; Wang et al., 2007). In this study, endophytic bacterial strains promoted the nematicidal activity of extract from French marigold flowers compared to the uninoculated control (Figure 5). This increase in nematicidal activity could be due to the role of endophytic bacterial strains in enhancing the production of nematicidal phytocompounds specially α-terthienyl. Various rhizospheric and endophytic bacterial strains are also well-known for their potential role in the biocontrol of phytopathogen and are involved in indirect plant growth promotion (Mhatre et al., 2019; Gamalero and Glick, 2020), which might be true for current endophytic bacterial strains. Sturz and Kimpinski (2004) isolated several bacterial endophytes from African and French marigolds that showed their nematicidal activities against Pratylenchus penetrans in soils. Such plant bacterization could be a potential candidate for non-residual and environment-friendly pesticides that will boost the plant’s defense metabolites to reduce the pathogens population in the root zone (Nivsarkar et al., 2001).
Materials and methods
Preparation of endophytic bacterial inoculum
Three pre-isolated endophytic PGP bacterial strains, viz. B. phytofirmans PsJN (Naveed et al., 2020a), Enterobacter sp. MN17 (Sabir et al., 2020), and Bacillus sp. MN54 (Samreen et al., 2019) were collected from Environmental Science Laboratory, Institute of Soil and Environmental Sciences (ISES), University of Agriculture Faisalabad (UAF), Pakistan. These strains were grown separately in Luria-Bertani (LB) broth containing tryptone (10 g L–1), yeast extract (5 g L–1), and NaCl (10 g L–1) at 28 ± 1°C and 100 rpm for 48 h in an orbital shaking incubator (Firstek Scientific, Japan). The optical density (OD) at 600 nm of each broth was adjusted to 0.5 using a spectrophotometer (Gene Quant Pro, Gemini BV, Netherlands) to obtain a uniform population of bacteria [108–109 colony forming units (CFU) ml–1].
Seed bacterization
The peat as a carrier material was sterilized at a pressure of 138 kPa and temperature of 121°C for 30 min and inoculated with bacterial broth culture. The peat-based inoculum was incubated at 28 ± 2°C by adding a 10% sugar solution to increase the microbial populations. For inoculation, the desired suspension of inoculum (108–109 CFU ml–1; 250 ml kg–1 peat) was mixed with sterilized peat and incubated for 24 h at 28 ± 2°C before use for seed coating (seed to peat ratio 1.25:1 w/w). Marigold seed dressing was prepared with the inoculated peat mixed with 10% sterilized sugar (sucrose) solution in a 10:1 ratio (Saeed et al., 2019). In the case of un-inoculated control, seeds were coated with the sterilized peat treated with broth, and 10 % fixed sugar solution.
Pot experiment and treatment plan
A pot experiment was conducted in the net-house, ISES, UAF, Pakistan, to evaluate the potential of endophytic bacteria to improve flowering, pharmacological activities, and delay of flower senescence of French marigold. The soil used for the experiment was collected from the field, air dried, thoroughly mixed, passed through a 2-mm sieve, and analyzed for various physical and chemical characteristics. The soil was sandy clay loam, having pH, 7.88; EC, 1.38 dS m–1; organic matter, 0.78%; total nitrogen, 0.034%; available phosphorus, 7.80 mg kg–1 and extractable potassium, 117 mg kg–1. French marigold seeds were surface sterilized by dipping in 70% ethanol for 2 min and treated with 1.5% NaClO for 5 min, followed by washing three times with sterile distilled water (1 min each time). The efficacy of surface sterilization was checked by culturing seeds and aliquots of the final rinse in LB agar plates where no growth was observed. The experiment contained the following treatments: (1) Control, (2) PsJN inoculation, (3) MN-17 inoculation, (4) MN-54 inoculation, and (5) Consortia of PsJN, MN-17, and MN-54. Surface-disinfected marigold seeds were coated with different endophytic bacterial treated slurry. In the control treatment, slurry for seed coating was prepared using sterilized LB broth. Pots were arranged in the net-house using a completely randomized design with three replications of each treatment. The plants were harvested after 60 days during full bloom flowering and further processed immediately for growth, physiological, and pharmacological analysis.
Assessment of growth parameters and vase life
The end of vase life was determined as the time in which 50% of open flower petals had wilted. The vase life of French marigold flowers was recorded by the number of days from the day that cut flowers were put in distilled water and incubated at room temperature (24°C for 12 days) until they had no ornamental value (underwent a color change, wilt, or loose turgidity). The mean value of the vase life of all flowers in each was calculated as the average vase life for each treatment. Plant agronomic parameters such as plant height, shoot, root fresh weight, root length, number of flowers plant–1, and flower diameter were recorded after harvesting the French marigold plants.
Physiological parameters
Plant physiological parameters of both treated and untreated plants were recorded at mid-day (between 10:00 and 14:00). A portable infrared gas analyzer [IRGA (CI-340) Germany] was used (at 1,200–1,400 μmol m–2 s–1 photosynthetic photon flux density) to measure transpiration rate, photosynthetic rate, stomatal, and substomatal conductance. Relative chlorophyll contents SPAD index of the second leaf from apex were recorded through Chlorophyll meter at vegetative stage.
Estimation of total flavonoid, phenolic acid, and protein contents
To determine total flavonoid contents, 0.5 ml of French marigold flowers extract was mixed with 2 ml of distilled water and 0.15 ml of NaNO2 (5%) solution and incubated for 6 min. After that, 0.15 ml of 10%, AlCl3 solution was added and incubated for 6 min, followed by adding NaOH (4%) solution to the mixture. The volume of the reaction mixture was made up to 5 ml by adding methanol. The absorbance of the reaction mixture was taken at 510 nm after incubation for 15 min. Total flavonoid contents of the extracts were expressed as catechin equivalents from the linear regression curve of catechin (Park et al., 2008). The total phenolic compounds in French marigold flowers extract were determined by the Folin–Ciocalteu method (Bärloche and Graça, 2020). The calibration curve was prepared with different concentrations of gallic acid. To estimate total protein contents, 0.1 ml of French marigold flower extract and 0.1 ml of NaOH (2N) were mixed and hydrolyzed at 100°C. The freshly prepared complex-forming reagent (1 ml) consisting of Na2CO3 (2%), CuSO4.5H2O (1%), and KNAC4H4O6.4H2O (2%) was added to cooled hydrolysate (Lowry et al., 1951). After 10 min of incubation, 0.1 ml of Folin reagent was added through a vortex and incubated for 30 min. Total protein contents were estimated by taking absorbance at 750 nm (Lowry et al., 1951).
DPPH radical scavenging and antioxidant power assays
The antioxidant activity of French marigold extract was estimated through DPPH radical scavenging assay as performed by Yen and Chen (1995) with slight modifications. The freshly prepared 1 ml of DPPH solution was added to 3 ml of marigold flower extracts at different concentrations and kept for 30 min in the dark. After incubation, absorbance was noted at 517 nm. A low absorbance of the reaction mixture indicates a high radical scavenging activity. The antioxidant activity of butylated hydroxytoluene and ascorbic acid was analyzed as standards. The solution without marigold flower extract was used as a control. The inhibition of DPPH radical samples was calculated as follows.
where, AC, absorbance of control; AS, absorbance of sample.
The ferric reducing power of French marigold flower extract that reflected their antioxidant activity was determined using Fe3+ and Fe2+ reduction assay (Benzie and Strains, 1996). The 1 ml of French marigold flowers extract in methanol was added to 2.5 ml of sodium phosphate buffer (0.2 M; PBS) and 2.5 ml of K3Fe(CN)6 (1%) solution. The solution was incubated at 50°C for 20 min on a vortex shaker, followed by adding 2.5 ml of trichloroacetic acid (10%). The final volume of 2.5 ml after centrifugation was diluted up to 5.0 ml with deionized water, and absorbance was read at 700 nm.
For antioxidant enzyme determination, frozen leaf material was homogenized in potassium phosphate buffer (0.2 M, pH 7) ice-cold solution with ethylene diamine tetra acetic acid (EDTA) (0.1 mM). The activity of APX was measured by a decline in spectrophotometer absorbance (290 nm wavelength) due to the reduction of ascorbate by H2O2 (Nakano and Asada, 1981). The activity of CAT enzymes was observed by a diminution in the spectrophotometer absorbance (240 nm wavelength) owing to H2O2 loss (Cakmak and Marschner, 1992). The activity of GPX was observed by spectrophotometric absorbance (340 nm wavelength) due to the reaction of a sodium azide, glutathione, and GPX solution into a β-NADPH (nicotinamide adenine dinucleotide phosphate) (Aebi, 1983). The activity of SOD enzyme was estimated by spectrophotometric absorbance (420 nm wavelength) due to reaction of enzyme extract with sodium phosphate, EDTA and pyrogallol (Roth and Gilbert, 1984).
Hemolytic and nematicidal activities
Hemolytic activity of French marigold extracts was assayed through the method of Powell et al. (2000). The human blood cells (3 ml) were gently poured into a sterile falcon tube and washed three times with chilled PBS (5 ml) by centrifuging the tubes for 5 min. The red blood cells (180 μl) were gently mixed with French marigold flower extract (20 μl) and centrifuged for 5 min. The supernatant (100 μl) was diluted with chilled sterile PBS (900 μl). Triton X-100 was run as a positive control, and PBS was taken as a negative control in triplicate. Absorbance was taken at 576 nm through ELISA plate reader.
The nematicidal activity of endophytic bacterial strain treated French marigold flowers extract was evaluated. A root-knot nematode M. incognita at juveniles J2 stage was obtained by incubating nematode egg masses in tap water at 27°C in the dark. They were collected every 2 days and concentrated in small volumes of sterilized water by filtering through 1 μm Whatman filters and collecting them after repeated washes (Molinari, 2009). The soil stages juveniles of nematodes were separately transferred to oil solutions from French marigold flower extract in Petri dishes. Each treatment had 100 nematodes in triplicate. Nematodes in distilled water and 0.05% Tween solutions were served as checks. The Petri dishes were kept at room temperature (28 ± 2°C). Numbers of un-hatched and M. incognita juveniles were daily recorded for 16 days, and immobile soil stages of nematode were counted after 24 and 72 h (Abd-Elgawad and Omer, 1995). Each time, the nematodes were transferred in aerated distilled water, and then the active nematodes were counted after a day.
Plant analysis
Post-harvest plant biomass (above and below ground) was obtained after drying whole plants at 65°C for 72 h, and samples were wet digested by following the method of Wolf (1982). The plant digested samples were analyzed for N, P, and K concentration by following the technique of Estefan et al. (2013). The N contents in plant digest were determined through the Kjeldahl method. The P concentration was estimated through a colorimetric method, while the K concentration was determined using a flame photometer.
Persistence of endophytic bacteria in the rhizosphere, root, shoot, and flowers
The rhizosphere and endophytic persistence of selected bacterial strains were determined by dilution and plate counting technique. For colonization assay, rhizospheric soil was collected, and soil slurry was prepared at a ratio of 1:5 (soil: NaCl 0.9%) following agitation for 30 min at room temperature. After sedimentation, serial dilutions up to 10–6 were plated onto a 10% tryptic soy agar medium. Colonies were counted after incubating the plates at 28 ± 1°C for 2-days, and the colonization value was determined following the equation.
Similarly, 1 g of surface-sterilized samples of each plant tissue (root, shoot, leaves, and flowers) were homogenized in 5 ml 0.9% saline buffer using a sterile mortar and pestle. After settling the solid fraction, serial dilutions up to 10–5 were spread on a TSA medium. Twenty-five visible colonies were selected per treatment randomly, and their identity with that of inoculant strain was confirmed by restriction fragment length polymorphism (RFLP) analysis of the 16S–23S rRNA intergenic spacer (IGS) region (Naveed et al., 2014).
Statistical analysis
Data for different growth and yield attributes were collected and analyzed statistically using software “Statistix 8.1®” version. Means were compared by using least significant difference (LSD) test (Steel et al., 1997) at a 5% probability level. Origin Pro 9.1 software was used for graphs, and Pearson correlation, PCA was performed using R-software.
Conclusion
The current study concluded that French marigold plants inoculated with endophytic bacteria improve plant growth, physiology, nutrient uptake, and vase life compared to the uninoculated control. They also improve the metabolites, antioxidant enzyme activity, and nematicidal activities of extract from French marigold flowers. The application of endophytic bacteria is also involved in reducing the hemolytic activity of extract from French marigold flowers. The consortium application reported a significantly more significant improvement in French marigold attributes than sole inoculation. Consortium application of such compatible promising endophytic bacteria could benefit the horticulture industry by providing evidence that beneficial bacteria adopted as an effective tool to reduce fertilizers input and improve plant metabolites profile and pharmaceutical quality of ornamental plants.
Data availability statement
The original contributions presented in the study are included in the article/supplementary material, further inquiries can be directed to the corresponding authors.
Author contributions
MN, AM, and MB: conceptualization. SH and MR: methodology and formal analysis. SH, MR, MM, and ZS: software. MN, OM, TH, and JH: validation. MN, MB, AK, and JH: resources. SH, ZS, and MN: data curation. SH, MN, and MR: writing—original draft preparation. MN and AM: writing—review and editing. TH, MB, JH, and AM: supervision. MN: project administration. AK, JH, AM, MB, and MN: funding acquisition. All authors have read and agreed to the published version of the manuscript.
Funding
This work was supported by the Ministry of Agriculture of the Czech Republic, institutional support MZE-RO1218 and MZE-RO1722, and by the Ministry of Education, Youth and Sports of the Czech Republic, grant number: FCH-S-22-8001.
Conflict of interest
Author JH was employed by Agrovyzkum Rapotin Ltd. Author AK was employed by Agricultural Research, Ltd.
The remaining authors declare that the research was conducted in the absence of any commercial or financial relationships that could be construed as a potential conflict of interest.
Publisher’s note
All claims expressed in this article are solely those of the authors and do not necessarily represent those of their affiliated organizations, or those of the publisher, the editors and the reviewers. Any product that may be evaluated in this article, or claim that may be made by its manufacturer, is not guaranteed or endorsed by the publisher.
References
Abd-Elgawad, M. M., and Omer, E. A. (1995). Effect of essential oils of some medicinal plants on phytonematodes. Anz. Schädlingskd. Pfl. Umwelt . 68, 82–84. doi: 10.1007/BF01908429
Aebi, H. E. (1983). “Catalase,” in Methods of enzymatic analysis, ed. H. U. Bergmeyer (Weinhem: Verlag Chemie), 273–286.
Ahmad, M., Adil, Z., Hussain, A., Mumtaz, M. Z., Nafees, M., Ahmad, I., et al. (2019a). Potential of phosphate solubilizing Bacillus strains for improving growth and nutrient uptake in mungbean and maize crops. Pak. J. Agri. Sci. 56, 283–289. doi: 10.3390/agronomy9100621
Ahmad, M., Naseer, I., Hussain, A., Zahid Mumtaz, M., Mustafa, A., Hilger, T. H., et al. (2019b). Appraising endophyte–plant symbiosis for improved growth, nodulation, nitrogen fixation and abiotic stress tolerance: an experimental investigation with chickpea (Cicer arietinum L.). Agron 9:621. doi: 10.21162/PAKJAS/19.7455
Ahmad, M., Wang, X., Hilger, T. H., Luqman, M., Nazli, F., Hussain, A., et al. (2020). Evaluating biochar-microbe synergies for improved growth, yield of maize, and post-harvest soil characteristics in a semi-arid climate. Agron 10:1055. doi: 10.3390/agronomy10071055
Ahmad, M., Zahir, Z. A., Nadeem, S. M., Nazli, F., Jamil, M., and Jamshaid, M. U. (2014). Physiological response of mung bean to Rhizobium and Pseudomonas based biofertilizers under salinity stress. Pak. J. Agri. Sci. 51, 555–562.
Ali, M. A., Naveed, M., Mustafa, A., and Abbas, A. (2017). “The good, the bad, and the ugly of rhizosphere microbiome,” in Probiotics and Plant Health, eds V Kumar, M Kumar, S Sharma, and R Prasad (Singapore: Springer), 253–290. doi: 10.1007/978-981-10-3473-2_11
Ali, M. H., Sattar, M. T., Khan, M. I., Naveed, M., Rafique, M., Alamri, S., et al. (2020). Enhanced growth of mungbean and remediation of petroleum hydrocarbons by Enterobacter sp. MN17 and biochar addition in diesel contaminated soil. Appl. Sci. 10:8548. doi: 10.3390/app10238548
Ali, S., Moon, Y. S., Hamayun, M., Khan, M. A., Bibi, K., and Lee, I. J. (2022). Pragmatic role of microbial plant biostimulants in abiotic stress relief in crop plants. J Plant Interact. 17, 705–718. doi: 10.1080/17429145.2022.2091801
Aziz, M. Z., Yaseen, M., Naveed, M., Wang, X., Fatima, K., Saeed, Q., et al. (2020). Polymer-Paraburkholderia phytofirmans PsJN coated diammonium phosphate enhanced microbial survival, phosphorous use efficiency, and production of wheat. Agron 10:1344. doi: 10.3390/agronomy10091344
Bärloche, R. F., and Graça, M. A. (eds) (2020). “Total phenolics,” inMethods to Study Litter Decomposition. doi: 10.1007/978-3-030-30515-4_18
Benzie, I. F., and Strains, J. J. (1996). The ferric reducing ability of plasma (FRAP) as a measure of “Antioxidant Power”: the FRAP assay. Anal. Biochem. 239, 70–76. doi: 10.1006/abio.1996.0292
Bhattacharya, A., Sood, P., and Citovsky, V. (2010). The roles of plant phenolics in defence and communication during Agrobacterium and Rhizobium infection. Mol. Plant Pathol. 11, 705–719. doi: 10.1111/j.1364-3703.2010.00625.x
Biglari, F., AlKarkhi, A. F. M., and Easa, A. M. (2008). Antioxidant activity and phenolic content of various date palm (Phoenix dactylifera) fruits from Iran. Food Chem. 107, 1636–1641. doi: 10.1016/j.foodchem.2007.10.033
Bosma, T. L., Dole, J. M., and Maness, N. O. (2003). Optimizing marigold (Tagetes erecta L.) petal and pigment yield. Crop Sci. 43, 2118–2124. doi: 10.2135/cropsci2003.2118
Brencic, A., and Winans, S. C. (2005). Detection of and response to signals involved in host–microbe interactions by plant-associated bacteria. Microbiol. Mol. Biol. Rev. 69, 155–194. doi: 10.1128/MMBR.69.1.155-194.2005
Cakmak, I., and Marschner, H. (1992). Magnesium deficiency and high light intensity enhance activities of superoxide dismutase, ascorbate peroxidase and glutathione reductase in bean leaves. Plant Physiol. 98, 1222–1227. doi: 10.1104/pp.98.4.1222
Dal Cortivo, C., Ferrari, M., Visioli, G., Lauro, M., Fornasier, F., Barion, G., et al. (2020). Effects of seed-applied biofertilizers on rhizosphere biodiversity and growth of common wheat (Triticum aestivum L.) in the field. Front. Plant Sci. 11:72. doi: 10.3389/fpls.2020.00072
Depaepe, T., and Van Der Straeten, D. (2020). Tools of the ethylene trade: a chemical kit to influence ethylene responses in plants and its use in agriculture. Small Methods 4:1900267. doi: 10.1002/smtd.201900267
Doty, S. L., Oakley, B., Xin, G., Kang, J. W., Singleton, G., Khan, Z., et al. (2009). Diazotrophic endophytes of native black cottonwood and willow. Symbiosis 47, 23–33. doi: 10.1007/BF03179967
Duan, X., Jiang, Y., Su, X., Zhang, Z., and Shi, J. (2007). Antioxidant properties of anthocyanins extracted from litchi (Litchi chinensis Sonn.) fruit pericarp tissues in relation to their role in the pericarp browning. Food Chem. 101, 1365–1371. doi: 10.1016/j.foodchem.2005.06.057
Duangpaeng, A., Phetcharat, P., Chanthapho, S., Boonkantong, N., and Okuda, N. (2012). The study and development of endophytic bacteria for enhancing organic rice growth. Proc.Engin. 32, 172–176. doi: 10.1016/j.proeng.2012.01.1253
Ebrahimi, A., Khajavi, M. Z., Ahmadi, S., Mortazavian, A. M., Abdolshahi, A., Rafiee, S., et al. (2021). Novel strategies to control ethylene in fruit and vegetables for extending their shelf life: a review. Int. J. Environ. Sci. Technol. 21, 1–2.
El-Deeb, B., Bazaid, S., Gherbawy, Y., and Elhariry, H. (2012). Characterization of endophytic bacteria associated with rose plant (Rosa damascena trigintipeta) during flowering stage and their plant growth promoting traits. J. Plant Interact. 7, 248–253. doi: 10.1080/17429145.2011.637161
Estefan, G., Sommer, R., and Ryan, J. (2013). Methods of Soil, Plant, and Water Analysis: A Manual for the West Asia and North Africa region. Beirut: International Center for Agricultural Research in the Dry Areas (ICARDA).
Eun, H. D., Ali, S., Jung, H., Kim, K., and Kim, W. C. (2019). Profiling of ACC synthase gene (ACS11) expression in Arabidopsis induced by abiotic stresses. Appl. Biol. Chem. 62, 1–11. doi: 10.1186/s13765-019-0450-4
Eyre, A. W., Wang, M., Oh, Y., and Dean, R. A. (2019). Identification and characterization of the core rice seed microbiome. Pbiome 3, 148–157. doi: 10.1094/PBIOMES-01-19-0009-R
Fabrick, J. A., Yool, A. J., and Spurgeon, D. W. (2020). Insecticidal activity of marigold Tagetes patula plants and foliar extracts against the hemipteran pests, Lygus hesperus and Bemisia tabaci. PLoS One 15:e0233511. doi: 10.1371/journal.pone.0233511
Forchetti, G., Masciarelli, O., Izaguirre, M. J., Alemano, S., Alvarez, D., and Abdala, G. (2010). Endophytic bacteria improve seedling growth of sunflower under water stress, produce salicylic acid, and inhibit growth of pathogenic fungi. Curr.Microbiol. 61, 485–493. doi: 10.1007/s00284-010-9642-1
Gamalero, E., and Glick, B. R. (2020). The use of plant growth-promoting bacteria to prevent nematode damage to plants. Biology 9:381. doi: 10.3390/biology9110381
Gray, E. J., and Smith, D. L. (2005). Intracellular and extracellular PGPR: commonalities and distinctions in the plant-bacterium signaling processes. Soil Biol. Biochem. 37, 395–412. doi: 10.1016/j.soilbio.2004.08.030
Hooks, C. R., Wang, K. H., Ploeg, A., and McSorley, R. (2010). Using marigold (Tagetes spp.) as a cover crop to protect crops from plant-parasitic nematodes. Appl. Soil Ecol. 46, 307–320. doi: 10.1016/j.apsoil.2010.09.005
Hyde, P. T., Guan, X., Abreu, V., and Setter, T. L. (2020). The anti-ethylene growth regulator silver thiosulfate (STS) increases flower production and longevity in cassava (Manihot esculenta Crantz). Plant Growth Regul. 90, 441–453. doi: 10.1007/s10725-019-00542-x
Ibiang, Y. B., Mitsumoto, H., and Sakamoto, K. (2017). Bradyrhizobia and Arbuscular Mycorrhizal fungi modulate manganese iron, phosphorus and polyphenols in soyabean [Glycine max (L.) Merr.] under excess zinc. Environ. Exp. Bot. 137, 1–13. doi: 10.1016/j.envexpbot.2017.01.011
Kashif, N. (2001). Effect of NPK on Growth and Chemical Effect on Vase Life Of Zinnia. M.Sc. Thesis, Pir Mehr Ali Shah Arid Agriculture University, Rawalpindi, Pakistan.
Kavino, M., Harish, S., Kumar, N., Saravanakumar, D., and Samiyappan, R. (2010). Effect of chitinolytic PGPR on growth, yield and physiological attributes of banana (Musa spp.) under field conditions. Appl. Soil Ecol. 45, 71–77. doi: 10.1016/j.apsoil.2010.02.003
Khan, A. L., Halo, B. A., Elyassi, A., Ali, S., Al-Hosni, K., Hussain, J., et al. (2016). Indole acetic acid and ACC deaminase from endophytic bacteria improves the growth of Solanum lycopersicum. Electron. J. Biotechnol. 21, 58–64. doi: 10.1016/j.ejbt.2016.02.001
Khan, N. (2020). Plant-microbial interactions and their role in sustainable agriculture and sustainability of agriculture soils. Recent Pat. Food Nutr. Agri. 11, 94–95. doi: 10.2174/221279841102200806152933
Khan, N., and Bano, A. (2019). Exopolysaccharide producing rhizobacteria and their impact on growth and drought tolerance of wheat grown under rainfed conditions. PLoS One 14:e0222302. doi: 10.1371/journal.pone.0222302
Khan, N., Bano, A., and Babar, M. D. (2019). The stimulatory effects of plant growth promoting rhizobacteria and plant growth regulators on wheat physiology grown in sandy soil. Arch. Microbiol. 201, 769–785. doi: 10.1007/s00203-019-01644-w
Khan, N., Bano, A., and Curá, J. A. (2020). Role of beneficial microorganisms and salicylic acid in improving rainfed agriculture and future food safety. Microorganisms 8:1018. doi: 10.3390/microorganisms8071018
Khanghahi, M. Y., Pirdashti, H., Rahimian, H., Nematzadeh, G. A., and Sepanlou, M. G. (2018). Nutrient use efficiency and nutrient uptake promoting of rice by potassium solubilizing bacteria (KSB). Cereal Res. Commun. 46, 739–750. doi: 10.1556/0806.46.2018.042
Li, W., Gao, Y., Zhao, J., and Wang, Q. (2007). Phenolic, flavonoid, and lutein ester content and antioxidant activity of 11 cultivars of chinese marigold. J. Agric. Food Chem. 55, 8478–8484. doi: 10.1021/jf071696j
Liu, S. C., Lin, J. T., Wang, C. K., Chen, H. Y., and Yang, D. J. (2009). Antioxidant properties of various solvent extracts from lychee (Litchi chinensis Sonn.) flowers. Food Chem. 114, 577–581. doi: 10.1016/j.foodchem.2008.09.088
Lowry, O. H., Rosebrough, N. J., Farr, A. L., and Randall, R. J. (1951). Protein measurement with the Folin Phenol reagent. J. Biol. Chem. 193, 265–275. doi: 10.1016/S0021-9258(19)52451-6
Mhatre, P. H., Karthik, C., Kadirvelu, K., Divya, K. L., Venkatasalam, E. P., Srinivasan, S., et al. (2019). Plant growth promoting rhizobacteria (PGPR): a potential alternative tool for nematodes bio-control. Biocat. Agri. Biotechnol. 1, 119–128. doi: 10.1016/j.bcab.2018.11.009
Molinari, S. (2009). “Bioassays on plant-nematode interactions,” in Plant Bioassays, eds S. S. Narwal, D. A. Sampietro, C. A. N. Catalàn, M. A. Vattuone, and B. Politycka (New Delhi: Studium Press LLC), 293–326.
Moon, Y. S., and Ali, S. (2022). A fruitful decade of bacterial ACC deaminase biotechnology: a pragmatic approach towards abiotic stress relief in plants. Theor. Exp. Plant Physiol. 34:104. doi: 10.1007/s40626-022-00237-1
Mumtaz, M. Z., Ahmad, M., Jamil, M., Asad, S. A., and Hafeez, F. (2018). Bacillus strains as potential alternate for zinc biofortification of maize grains. Int. J. Agric. Biol. 1, 1779–1786.
Mumtaz, M. Z., Malik, A., Nazli, F., Latif, M., Zaheer, A., Ali, Q., et al. (2020). Potential of zinc solubilizing Bacillus strains to improve growth, yield, and quality of maize (Zea mays). Int. J. Agric. Biol. 1, 691–698.
Mustafa, A., Naveed, M., Saeed, Q., Ashraf, M. N., Hussain, A., Abbas, T., et al. (2019). “Application potentials of plant growth promoting rhizobacteria and fungi as an alternative to conventional weed control methods,” in Sustainable Crop Production, eds S Imadi, K Shazadi, and A Gul doi: 10.5772/intechopen.86339
Nag, P., Shriti, S., and Das, S. (2020). Microbiological strategies for enhancing biological nitrogen fixation in nonlegumes. J. Appl. Microbiol. 129, 186–198. doi: 10.1111/jam.14557
Nakano, Y., and Asada, K. (1981). Hydrogen peroxide is scavenged by ascorbate-specific peroxidase in spinach chloroplasts. Plant Cell Physiol. 22, 867–880.
Nascimento, F. X., Brigido, C., Glick, B. R., Oliveira, S., and Alho, L. (2012). Mesorhizobium ciceri LMS-1 expressing an exogenous 1-aminocyclopropane-1-carboxylate (ACC) deaminase increases its nodulation abilities and chickpea plant resistance to soil constraints. Lett. Appl. Microbiol. 55, 15–21. doi: 10.1111/j.1472-765X.2012.03251.x
Naseem, H., Ahsan, M., Shahid, M. A., and Khan, N. (2018). Exopolysaccharides producing rhizobacteria and their role in plant growth and drought tolerance. J. Basic Microbiol. 58, 1009–1022. doi: 10.1002/jobm.201800309
Naveed, M., Bukhari, S. S., Mustafa, A., Ditta, A., Alamri, S., El-Esawi, M. A., et al. (2020a). Mitigation of nickel toxicity and growth promotion in sesame through the application of a bacterial endophyte and zeolite in nickel contaminated soil. Int. J. Environ. Res. Public Health. 17:8859. doi: 10.3390/ijerph17238859
Naveed, M., Mustafa, A., Azhar, S. Q., Kamran, M., Zahir, Z. A., and úñez-Delgado, A. N. (2020b). Burkholderia phytofirmans PsJN and tree twigs derived biochar together retrieved Pb-induced growth, physiological and biochemical disturbances by minimizing its uptake and translocation in mung bean (Vigna radiata L.). J. Environ.Manag. 257:109974. doi: 10.1016/j.jenvman.2019.109974
Naveed, M., Hussain, M. B., Zahir, Z. A., Mitter, B., and Sessitsch, A. (2014). Drought stress amelioration in wheat through inoculation with Burkholderia phytofirmans strain PsJN. Plant Growth Regul. 73, 121–131. doi: 10.1007/s10725-013-9874-8
Nayani, S., Mayak, S., and Glick, B. R. (1998). Effect of plant growth-promoting rhizobacteria on senescence of flower petals. Indian J. Exp. Biol. 36, 836–839.
Nazli, F., Mustafa, A., Ahmad, M., Hussain, A., Jamil, M., Wang, X., et al. (2020). A review on practical application and potentials of phytohormone producing plant growth-promoting Rhizobacteria for inducing heavy metal tolerance in crops. Sustainability 12:9056. doi: 10.3390/su12219056
Nivsarkar, M., Cherian, B., and Padh, H. (2001). Alpha-terthienyl: a plant-derived new generation insecticide. Curr. Sci. 25, 667–672.
Nordstedt, N. P., and Jones, M. L. (2020). Isolation of rhizosphere bacteria that improve quality and water stress tolerance in greenhouse ornamentals. Front. Plant Sci. 16:826. doi: 10.3389/fpls.2020.00826
Park, Y.-S., Jung, S.-T., Kang, S.-G., Heo, B. K., Arancibia-Avila, P., Toledo, F., et al. (2008). Antioxidants and proteins in ethylene-treated kiwifruits. Food Chem. 107, 640–648. doi: 10.1016/j.foodchem.2007.08.070
Parmar, P., and Sindhu, S. S. (2013). Potassium solubilization by rhizosphere bacteria: influenc of nutritional and environmental conditions. J. Microbiol. Res. 3, 25–31.
Powell, W. A., Catranis, C. M., and Maynard, C. A. (2000). Design of self-processing antimicrobial peptides for plant protection. Lett. Appl. Microbiol. 31, 163–168. doi: 10.1046/j.1365-2672.2000.00782.x
Prasanna, R., Chaudhary, V., Gupta, V., Babu, S., Kumar, A., Singh, R., et al. (2013). Cyanobacteria mediated plant growth promotion and bioprotection against Fusarium wilt in tomato. Eur. J. Plant Pathol. 136, 337–353. doi: 10.1007/s10658-013-0167-x
Prasanna, R., Ramakrishnan, B., Simranjit, K., Ranjan, K., Kanchan, A., Hossain, F., et al. (2017). Cyanobacterial and rhizobial inoculation modulates the plant physiological attributes and nodule microbial communities of chickpea. Arch. Microbiol. 199, 1311–1323. doi: 10.1007/s00203-017-1405-y
Pudasaini, M. P., Viaene, N., and Moens, M. (2008). Hatching of the root-lesion nematode, Pratylenchus penetrans, under the influence of temperature and host. Nematology 10, 47–54. doi: 10.1163/156854108783360078
Puri, A., Padda, K. P., and Chanway, C. P. (2020). In vitro and in vivo analyses of plant-growth-promoting potential of bacteria naturally associated with spruce trees growing on nutrient-poor soils. Appl. Soil Ecol. 149:103538. doi: 10.1016/j.apsoil.2020.103538
Ranwala, A. P., and Miller, W. B. (2005). Effects of cold storage on postharvest leaf and flower quality of potted Oriental-, Asiatic-and LA-hybrid lily cultivars. Sci. Hortic. 105, 383–392. doi: 10.1016/j.scienta.2005.01.031
Reid, M. S., and Wu, M. (1992). Ethylene and flower senescence. Plant Growth Regul. 11, 37–43. doi: 10.1007/BF00024431
Roth, E. F., and Gilbert, H. S. (1984). The pyrogallol assay for superoxide dismutase: absence of a glutathione artifact. Anal. Biochem 137, 50–53. doi: 10.1016/0003-2697(84)90344-0
Ryu, R., and Patten, C. L. (2008). Aromatic amino acid dependent expression of indole-3-pyruvate decarboxylase is regulated by 4 TyrR in Enterobacter cloacae UW5. Am. Soc. Microbiol. Suppl 21, 1–35. doi: 10.1128/JB.00804-08
Sabir, A., Naveed, M., Bashir, M. A., Hussain, A., Mustafa, A., Zahir, Z. A., et al. (2020). Cadmium mediated phytotoxic impacts in Brassica napus: managing growth, physiological and oxidative disturbances through combined use of biochar and Enterobacter sp. MN17. J. Environ. Manag. 265:110522. doi: 10.1016/j.jenvman.2020.110522
Saeed, Z., Naveed, M., Imran, M., Bashir, M. A., Sattar, A., Mustafa, A., et al. (2019). Combined use of Enterobacter sp. MN17 and zeolite reverts the adverse effects of cadmium on growth, physiology and antioxidant activity of Brassica napus. PLoS One 14:e0213016. doi: 10.1371/journal.pone.0213016
Samreen, T., Zahir, Z. A., Naveed, M., and Asghar, M. (2019). Boron tolerant phosphorus solubilizing Bacillus spp. MN-54 improved canola growth in alkaline calcareous soils. Int. J. Agric. Biol. 21, 538–546.
Santoyo, G., Moreno-Hagelsieb, G., del Carmen Orozco-Mosqueda, M., and Glick, B. R. (2016). Plant growth-promoting bacterial endophytes. Microbiol. Res. 1, 92–99. doi: 10.1016/j.micres.2015.11.008
Schneider, S. M., Rosskopf, E. N., Leesch, J. G., Chellemi, D. O., Bull, C. T., and Mazzola, M. (2003). Alternatives to methyl bromide pre plant and post-harvest. Pest Manag. Sci. 59, 814–826. doi: 10.1002/ps.728
Siddiqui, M. A., and Alam, M. M. (1987). Control of plant-parasitic nematodes by intercropping with Tagetes minuta. Nematol. Medit. 15, 205–211.
Singh, H. G. (1988). “Sulphur management in fine texured calcareous soils,” in Proceedings of the TSI – FAI Symposium, (New Delhi: Sulphur in Indian Agriculture), 9–1.
South, K. A., Nordstedt, N. P., and Jones, M. L. (2021). Identification of plant growth promoting rhizobacteria that improve the performance of greenhouse-grown petunias under low fertility conditions. Plants 10:1410. doi: 10.3390/plants10071410
Steel, R. G. D., Torrie, J. H., and Dickey, D. A. (1997). Principles and Procedures of Statistics. A Biometric Approach, 3rd Edn. New York, NY: McGraw-Hill Book Co.
Sturz, A. V., and Kimpinski, J. (2004). Endoroot bacteria derived from marigolds (Tagetes spp.) can decrease soil population densities of root-lesion nematodes in the potato root zone. Plant Soil 262, 241–249. doi: 10.1023/B:PLSO.0000037046.86670.a3
Suatmadji, R. W. (1969). Studies on the Effect of Tagetes Species on Plant Parasitic Nematodes. Netherlands: H. Veenman en Zonen, 132.
Sudhagar, S. (2013). Production and marketing of cut flower (Rose and Gerbera) in Hosur Taluk. Int. J. Bus. Manag. Invent. 2, 15–25.
Suherman Anggoro, D. D. (2011). Producing slow release urea by coating with starch/acrylic acid in fluid bed spraying. Int. J. Eng. Tech. 11, 77–80.
Toju, H., Peay, K. G., Yamamichi, M., Narisawa, K., Hiruma, K., Naito, K., et al. (2018). Core microbiomes for sustainable agroecosystems. Nat. Plants 4, 247–257. doi: 10.1038/s41477-018-0139-4
Umar, W., Ayub, M. A., UrRehman, M. Z., Ahmad, H. R., Farooqi, Z. U. R., Shahzad, A., et al. (2020). “Nitrogen and phosphorus use efficiency in agroecosystems,” in Resources Use Efficiency in Agriculture, eds R. S Meena, M. K Jhariya, and S Kumar doi: 10.1007/978-981-15-6953-1_7 (Singapore: Springer), 213–257.
Van Deynze, A., Zamora, P., Delaux, P. M., Heitmann, C., Jayaraman, D., Rajasekar, S., et al. (2018). Nitrogen fixation in a landrace of maize is supported by a mucilage-associated diazotrophic microbiota. PLoS Biol. 16:e2006352. doi: 10.1371/journal.pbio.2006352
Van Doorn, W. G. (2001). Categories of petal senescence and abscission: a re-evaluation. Ann. Bot. 87, 447–456. doi: 10.1006/anbo.2000.1357
Van Doorn, W. G. (2002). Effect of ethylene on flower abscission: a survey. Ann. Bot. 89, 689–693. doi: 10.1093/aob/mcf124
Van Loon, L. C. (2007). “Plant responses to plant growth-promoting rhizobacteria,” in New Perspectives and Approaches in Plant Growth-Promoting Rhizobacteria Res, eds P. Bakker, J. M Raaijmakers, G Bloemberg, and M Höfte doi: 10.1007/978-1-4020-6776-1_2 (Dordrecht: Springer), 243–254.
Veen, H., and van de Geijn, S. C. (1978). Mobility and ionic form of silver as related to longevity of cut carnations. Planta 140, 93–96. doi: 10.1007/BF00389386
Wang, K. H., Hooks, C. R., and Ploeg, A. (2007). Protecting crops from nematode pests: using marigold as an alternative to chemical nematicides. Plant Dis. 35, 1–6.
Wang, K.-H., Sipes, B. S., and Schmitt, D. P. (2001). Suppression of Rotylenchulus reniformis by Crotalaria juncea. Brassica napus, and Tagetes erecta. Nematropica 31, 235–249.
Weir, T. L., Park, S. W., and Vivanco, J. M. (2004). Biochemical and physiological mechanisms mediated by allelochemicals. Curr. Opin. Plant Biol. 7:479. doi: 10.1016/j.pbi.2004.05.007
Welch, S., Barker, W., and Banfield, J. (1999). Microbial extracellular polysaccharides and plagioclase dissolution. Geochim. Cosmochim. Acta 63, 1405–1419. doi: 10.1016/S0016-7037(99)00031-9
Wolf, B. (1982). A comprehensive system of leaf analysis and its use for diagnosing crop nutrient status. Commun. Soil Sci. Plant Anal. 13:10351059. doi: 10.1080/00103628209367332
Yen, G. C., and Chen, H. Y. (1995). Antioxidant activity of various tea extracts in relation to their antimutagenicity. J. Agric. Food chem. 43, 27–32. doi: 10.1021/jf00049a007
Yu, Y. B., Adams, D. O., and Yang, S. F. (2022). Reprint of: 1-Aminocyclopropanecarboxylate synthase, a key enzyme in ethylene biosynthesis. Arch. Biochem. Biophys. 726:109238. doi: 10.1016/j.abb.2022.109238
Zahir, Z. A., Arshad, M., and Frankenberger, J. W. T. (2004). Plant growth promoting rhizobacteria application and perspectives in agriculture. Adv. Agron. 81, 97–168. doi: 10.1016/S0065-2113(03)81003-9
Keywords: allelopathic effect, antioxidant activity, endophytic bacteria, metabolites, plant-parasitic nematodes, Tagetes patula, vase life
Citation: Naveed M, Hafeez S, Rafique M, Mumtaz MZ, Subhani Z, Holatko J, Hammerschmiedt T, Malicek O, Mustafa A, Kintl A and Brtnicky M (2022) Plant-endophyte mediated improvement in physiological and bio-protective abilities of marigold (Tagetes patula). Front. Plant Sci. 13:993130. doi: 10.3389/fpls.2022.993130
Received: 13 July 2022; Accepted: 16 August 2022;
Published: 09 September 2022.
Edited by:
Ravinder Kumar, Central Potato Research Institute (ICAR), IndiaReviewed by:
Hamza Sohail, Huazhong Agricultural University, ChinaSajid Ali, Yeungnam University, South Korea
Copyright © 2022 Naveed, Hafeez, Rafique, Mumtaz, Subhani, Holatko, Hammerschmiedt, Malicek, Mustafa, Kintl and Brtnicky. This is an open-access article distributed under the terms of the Creative Commons Attribution License (CC BY). The use, distribution or reproduction in other forums is permitted, provided the original author(s) and the copyright owner(s) are credited and that the original publication in this journal is cited, in accordance with accepted academic practice. No use, distribution or reproduction is permitted which does not comply with these terms.
*Correspondence: Muhammad Naveed, bXVoYW1tYWQubmF2ZWVkQHVhZi5lZHUucGs=; Adnan Mustafa, YWRuYW5tdXN0YWZhNzgwQGdtYWlsLmNvbQ==