- State Key Laboratory of Crop Biology and College of Agronomy, Shandong Agricultural University, Tai’an, China
In order to explore the physiological mechanism of different yield of summer maize (Zea mays L.) hybrids with different growth duration, a field experiment was conducted to study the growth stage, leaf photosynthetic characteristics, dry matter accumulation (DMA), transport and distribution characteristics and yield of the early maturity hybrid Denghai 518 (DH518) and the mid-late maturity hybrid Denghai 605 (DH605) from 2017 to 2021. The results showed that the yield of DH605 was significantly higher than that of DH518. The growth period of DH518 was 7-10 days shorter and the days of the growth stage of the sowing-silking stage (R1) were 5-6 days shorter compared to that of DH605. The contribution to grain dry matter by leaf and stalk dry matter remobilization (DMRC) of DH518 was significantly higher than that of DH605. There was a significant negative correlation between pre-silking growth days and harvest index (HI). The 13C distribution to grains of DH518 was significantly higher than that of DH605, and the HI and the corresponding contribution of HI to yield was also higher than that of DH605. The light and temperature resource use efficiency from silking to physiological maturity stage of DH605 was significantly higher than that of DH518. The yield per GDD of DH605 increased by 7.25% than that of DH518. At post-silking, the duration of higher leaf area index (DLAI) (>56 days) and active photosynthesis duration (APD) (>50 days) of DH605 were longer compared with that of DH518, and the average plant growth rate was 7.15% higher than that of DH518, which significantly increased the DMA of DH605. Therefore, the significant reduction of DH518 yield compared with DH605 was not due to the shortening of the growth stage of sowing-R1, but the lower light and temperature resource use efficiency from silking to physiological maturity stage.
Introduction
Maize has become the largest cereal food crop in China since 2013, which was responsible for 22% of the global maize output (Liu et al., 2017; FAO, 2020). Increasing maize production is critical to ensuring global food and energy security in China (Liu et al., 2020). In a global crisis like COVID-19, food insecurity and disparity can become a serious problem not only in densely populated developing countries (Guha and Chandra, 2021; Odunitan-Wayas et al., 2021) but also in some developed countries (O’Hara and Toussaint, 2021; Oncini, 2021). Under the wheat-maize double-cropping model in the North China Plain, light and heat resources are not enough, so it is impossible to guarantee sufficient maize grain filling duration and late grain dehydration time (Sun et al., 2007; Wang et al., 2012). In order to increase yield, farmers are accustomed to plant mid-late maturity or late maturity hybrids for a long time (Edwards et al., 2005). However, with the wide application of the whole mechanized maize planting mode, the hybrids with a long growth period and slow dehydration in the later stage are increasingly unsuitable for production requirements. Due to the shortening of the growth period, the yield of early maturity hybrids is lower than that of middle-late maturity hybrids (Zhao et al., 2021). Therefore, how to improve the yield of early maturity hybrids becomes an urgent problem to be solved.
Aboveground dry matter accumulation (DMA) and harvest index (HI) are two simple and valuable characteristics for evaluating plant traits and improving yield (Donald and Hamblin, 1976). The characteristics of DMA, distribution, and transfer during the growth period are the important factors to determine the yield of maize (Saidou et al., 2003). The fundamental way to achieve a high yield is to increase DMA and distribution to grains (i.e. HI) as much as possible (Chen, 1994). With the upgrading of hybrids, the DMA per plant also increased, especially at post-silking (Hu et al., 1998). The post-silking DMA accounts for 50% of the total DMA at R6, which is mainly supplied to the grain (Rajcan and Tollenaar, 1999; Tollenaar et al., 2004; Lee and Tollenaar, 2007). In addition, the HI has improved (Echarte and Andrade, 2003; Hou et al., 2012; Ma et al., 2014). However, other studies have shown that the HI does not change continuously over time (Meghji et al., 1984; Tollenaar, 1989). Therefore, it is necessary to explore the differences between DMA and HI and their contribution to the yield of summer maize hybrids with different growth duration.
Leaves are the decisive organs for photosynthesis in terrestrial plants (Piazza et al., 2005). Higher LAI, longer active photosynthesis duration and slower leaf senescence play a positive role in the increase of DMA and yield after silking (Ma and Dwyer, 1998; Borrell et al., 2001; Duvick, 2005). Temperature plays an important role in the growth and development of crops (Lin et al., 2011). It affects the synthesis and distribution of photosynthetic matter in maize (Ptaszynska and Silesia, 2008) and the duration of growth period (Ileleji et al., 2007). In addition, the light and temperature resource use efficiency of high-yield maize hybrids are also higher (Slattery and Ort, 2021). DH605 is one of the most popular maize hybrids in China at present, and DH518 is a newly released hybrid that is being promoted. DH605 (mid-late hybrid) and DH518 (mid-early hybrid) have different growth duration, which are widely representative (Ma et al., 2022). Therefore, we analyzed the yield, accumulated temperature demand characteristics, leaf photosynthetic characteristics, and DMA and distribution characteristics of different summer maize hybrids to explore the effects of the changes in the growth duration on DMA and distribution and yield. We aimed to determine: a) whether the changes in growth period affect the contribution of dry matter and HI to yield, and b) what is the physiological mechanism of yield difference of summer maize hybrids with different growth duration. These results will provide an important reference for improving the yield of early maturity hybrids adapted to mechanical grain harvest.
Materials and methods
Plant materials and experimental design
Field experiments were conducted at an experimental farm of Shandong Agricultural University (36.09°N, 117.09°E) from 2017 to 2021. The weather conditions of the summer maize growing season in the planting area are shown in Figure 1. The early maturity hybrid Denghai 518 (DH518) and the mid-late maturity hybrid Denghai 605 (DH605) were used as test materials with a row spacing of 60 cm and a planting density of 75,000 ha-1. Both hybrids are widely planted in the province of Shandong, China. The hybrid maturity is classified as 113 d for DH605 and 103 d for DH518. The growing season duration (from sowing to harvest dates) for two hybrids in different years were shown in Table 2. Each treatment was repeated three times, in a completely randomized design, and the plot area was 54 m2 (9 m × 6 m). The N, P, and K fertilizers were applied as base fertilizer: 84 kg ha-1 N (urea, 46% N), 52.5 kg ha-1 P2O5 (calcium superphosphate, 17% P2O5), and 67.5 kg ha-1 K2O (muriate of potash, 60% K2O). At the ninth leaf stage (V9) 126 kg ha-1 N fertilizer (urea, 46% N) was applied as supplementary fertilizer.
Investigation of growth stage and calculation of the growing degree days
After sowing, the date on which the plant reached the sixth leaf stage (V6), the 12th leaf stage (V12), silking stage (R1), milk stage (R3), 45 days after silking (R5) and physiological maturity stage (R6) were observed and recorded. The R6 is defined as the date when the black layer forms at the base of the kernel and the milk line has disappeared. The weather conditions data were provided by the Agricultural Experimental Station of Shandong Agricultural University. GDD was calculated as follows (Gregory and Wilhelm, 1997):
where Tmax is the daily maximum temperature, Tmin is the daily minimum temperature, Tbase is the maize growth base temperature (10 °C).
Leaf area index
Six typical plants were signed from each plot at V6, V12, R1, R3, R5 and R6 stage to measure and calculate leaf area and LAI, according to the method of (Montgomery, 1911).
Chlorophyll content
Ten typical plants leaves SPAD value was measured as leaf chlorophyll content at V6, V12, VT, R3 and R6 stage. SPAD value of functional leaf was metered using a chlorophyll meter (SPAD-502, Minolta, Japan), and replicated at least eight times (Wu et al., 1998).
Leaf gas exchange parameters
The net photosynthetic rates (Pn), stomatal conductance (Gs) and intercellular CO2 concentration (Ci) were measured in the middle of five ear leaves representational in each treatment from 10:00 to 12:00 at R1, R3 and R5 stage by using a portable infrared gas analyzer (CIRAS-III, PP System, Hansatech, USA). The active photosynthesis duration (APD) and the duration of higher LAI (DLAI) was defined by (Zhang and Cheng, 1992) as the number of days when Pn and LA decrease from the maximum to 50%.
Accumulation, distribution and transport of dry matter
5 randomly selected plants from each plot were sampled at each physiological stage. At R1 and R6 stage, plants were separated into leaf, stalk (at R1 and R6 stage), and reproductive parts (bract, cob, and grain at R6 stage) for analysis. Plant samples were killed at 105 °C for 30min, then dried at 80 °C to constant weight, and the dry matter weight was measured. Post-silking DMA in aboveground biomass (PoSDMA), Pre-silking and post-silking DMA ratioin aboveground biomass (PrSDMAR and PoSDMAR), dry matter remobilization efficiency (DMRE) of vegetative tissues, and contribution to grain dry matter by leaf and stalk dry matter remobilization (DMRC) were estimated according to the following formulas (Mi et al., 2003; Chen et al., 2014; Chen et al., 2015b):
Harvest index was calculated as per (Ciampitti et al., 2013):
13C Pulse labeling, sampling and analysis
At R1 stage, five plants were selected for 13CO2 labeling and five for reference from the different summer maize hybrids in 2017, 2018, 2020 and 2021. The ear leaf was placed in the labeling chamber (a transparent plastic oven bag sealed at both ends) and 60 mL of 13CO2 air was pumped into the labeling chamber. After one hour, the bags were removed from the plants. At R6 stage, three labeled 13CO2 plants and three reference plants without labeled 13CO2 were separated into stalk, leaf, grain and others. These samples were dried, weighed, and ball-milled for analysis (Schussler and Westgate, 1994).
Yield and yield components
Thirty ears from the middle three rows of each plot were sampled to measure yield and yield components. Maize kernels with 14% moisture content were determined.
Data analysis
Microsoft Excel 2016 (Microsoft, Redmond, WA, USA) and SigmaPlot 12.5 (Systat Software, Inc., Richmond, CA, USA) were used for data processing and plotting, curve expert 1.4 for model fitting. Differences between years or hybrids were compared using ANOVA and Student’s t test with p< 0.05 (LSD 0.05). Correlation were carried out according to the method of Piepho (2018). Correlations and regression analyses were estimated as Pearson’s correlation coefficient. The main effects of year, hybrid, and their interactions were tested for the grain yield, dry matter remobilization, DMRC, Grain/leaf and HI using IBM SPSS Statistics 21 (IBM Corporation, Armonk, NY, USA).
Results
Yield
There was no significant year × hybrids interaction effects on the yield (Table 1). The yield of DH605 was significantly higher and was increased by 3.4-7.3%, compared with that of DH518. The increase in maize yield was mainly due to the significant increase in the 1,000-grain weight of DH605 (Table 1). There was no significant difference in the number of ears and grain number per ear (except in 2019) among summer maize hybrids with different growth duration (Table 1). The effective accumulated temperature of DH605 after silking was 53.1 °C lower than that of DH518 in 2019 because the sowing date is more than 6 days later than that of other years, resulting in a decrease in 1000-grain weight of DH605 (Table 2).
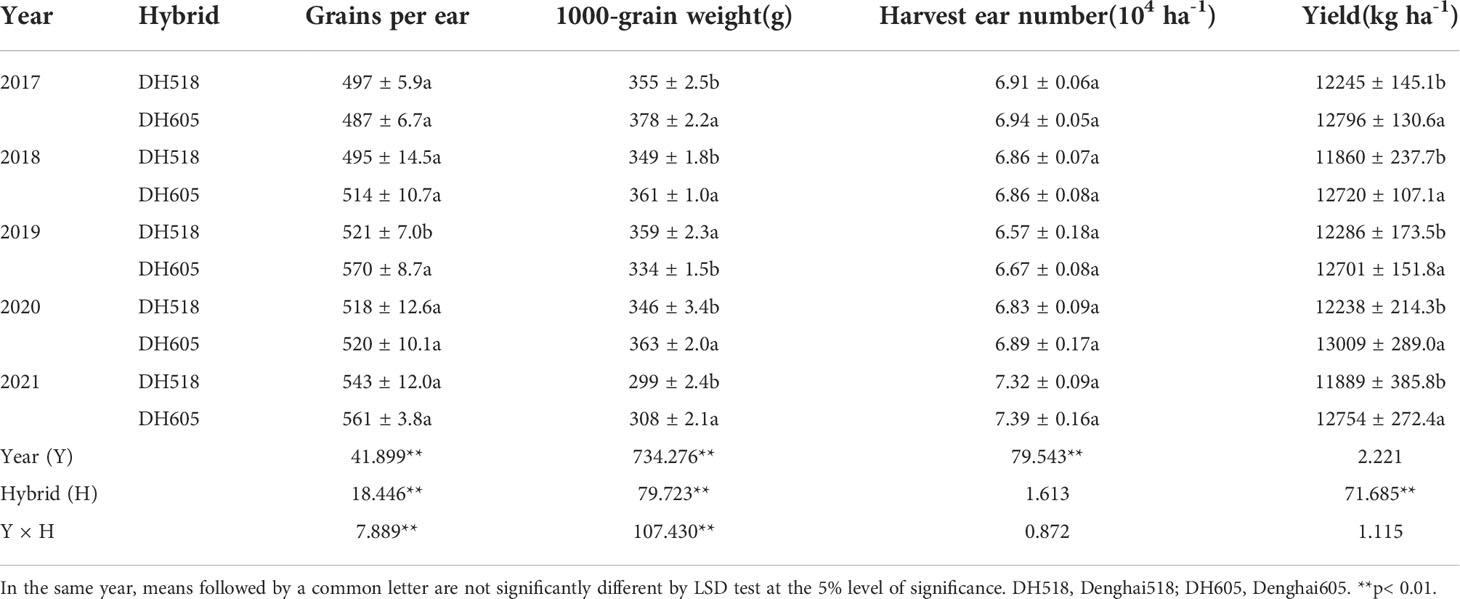
Table 1 Grain yield and yield components of summer maize hybrids with different growth duration from 2017 to 2021.
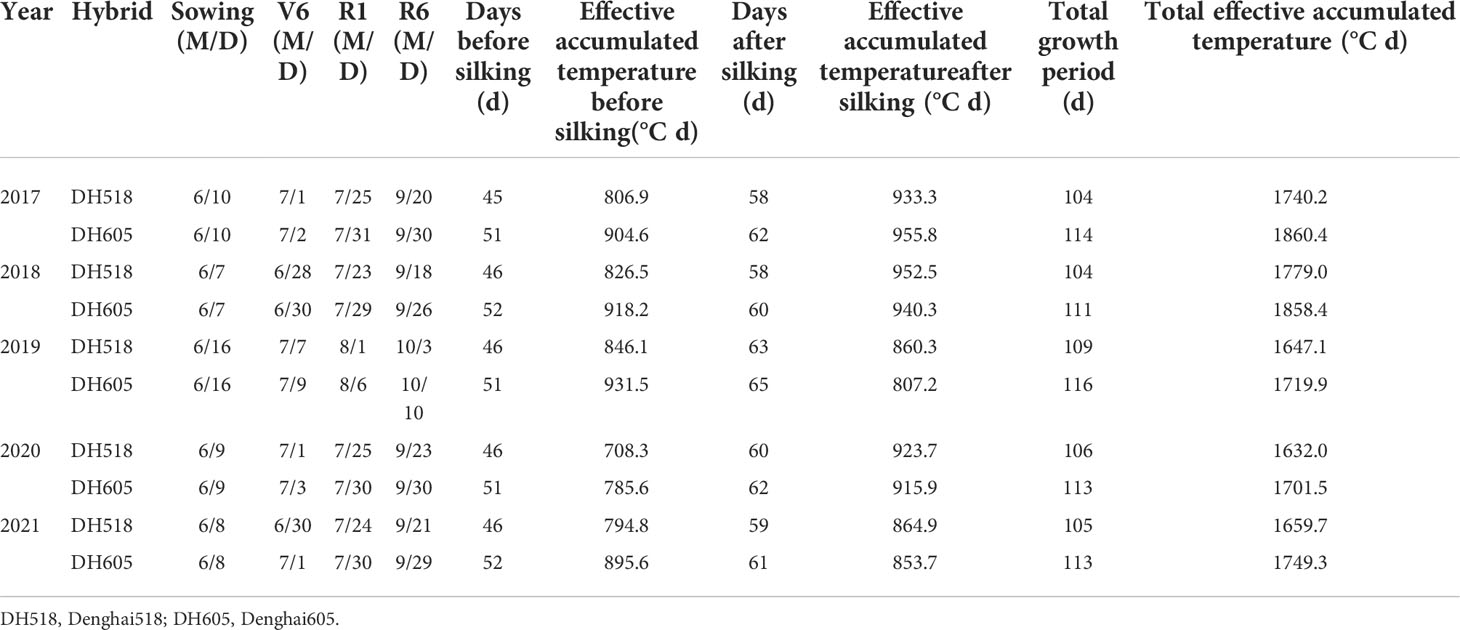
Table 2 Growth process and effective accumulated temperature of summer maize hybrids with different growth duration from 2017 to 2021.
Growth process and demand characteristics of GDD
The growth period of DH518 was shorter than that of DH605, and different period was mainly from V6 to R1 stage. The growth period of DH518 was 7-10 days shorter than that of DH605 and the pre-silking GDD was significantly lower. The pre-silking days and GDD of DH605 were increased 5-6 days and 77.3-100.8°C than those of DH518. There was no significant difference in post-silking GDD among different hybrids (Table 2). At post-silking, the yield per GDD of DH605 (12.73 kg ha-1 (°C d)-1) was significantly higher than that of DH518 (11.87 kg ha-1 (°C d)-1).
Dry matter accumulation
The PrSDMA and PoSDMA of DH605 were significantly higher than DH518. Compared with DH518, the PrSDMA and PoSDMA of DH605 increased by 9.7% and 10.2% respectively (Figure 2A). There was no significant difference between PrSDMAR and PoSDMAR of summer maize hybrids with different growth duration. The PoSDMA of the two hybrids was higher than the PrSDMA. Summer maize hybrids with different growth duration accumulated 40% of the total plant dry matter from sowing to R1 (Figure 2B), which indicated This shows that hybrids with higher PrSDMA had greater PoSDMA production potential. The DMA of various organs of DH605 was significantly higher than that of DH518. The DMA of grain, leaf, stalk and other organs of DH605 at R6 increased by 5.3-9.7%, 12.1-22.4%, 21.6-33.0% and 9.4-13.5%, respectively, compared to DH518. (Figure 2C)
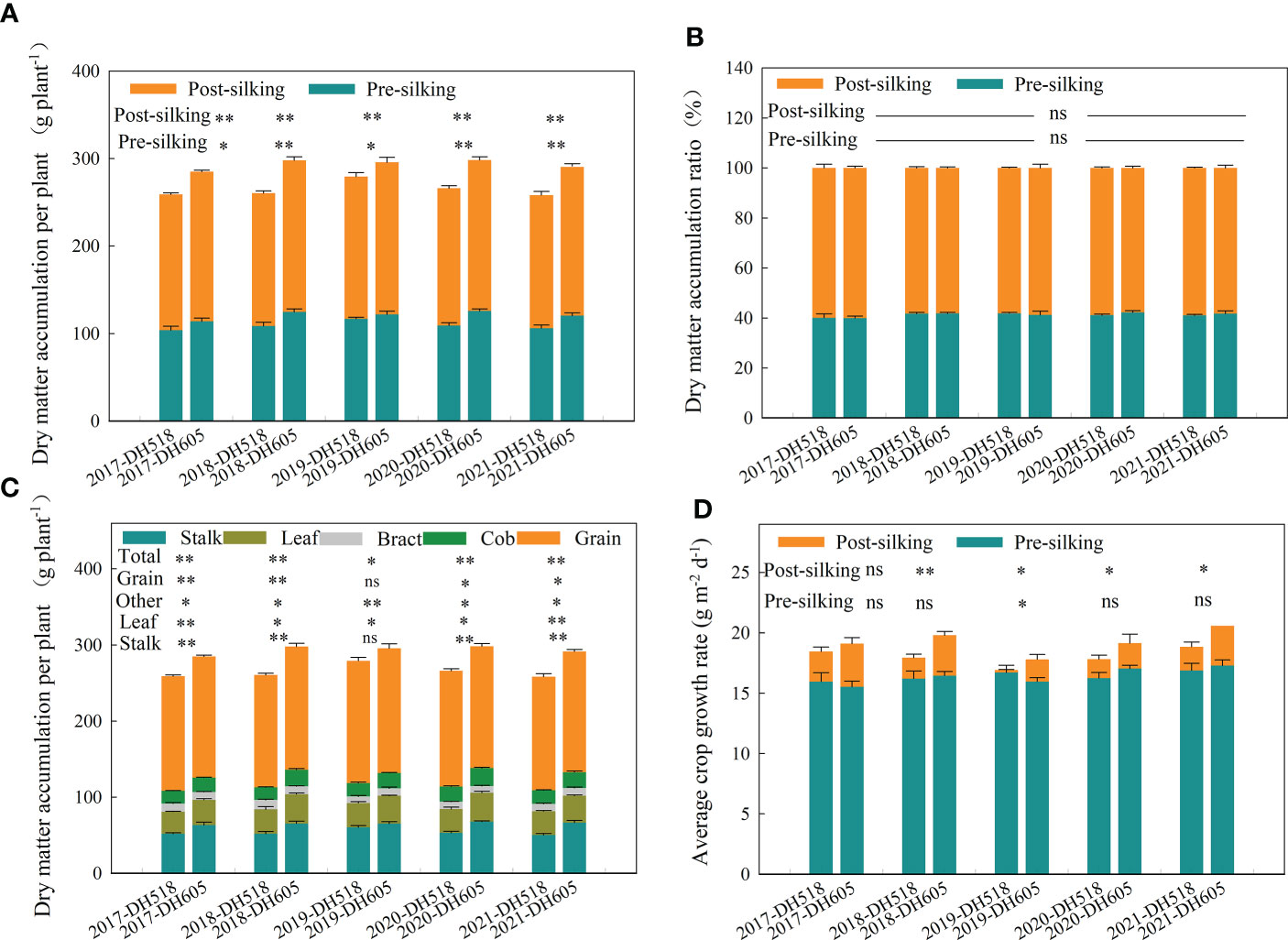
Figure 2 Above-ground biomass distribution and average crop growth rate of summer maize hybrids with different growth duration (2017-2021). 2017, 2018, 2019, 2020 and 2021 were different years; DH518, Denghai518; DH605, Denghai605. (A) Pre-silking and post-silking dry matter accumulation in aboveground biomass. Pre-silking and post-silking were the dry matter accumulation in aboveground biomass in that stage. (B) Pre-silking and post-silking dry matter accumulation ratio in aboveground biomass. Pre-silking and post-silking were the dry matter accumulation ratio` in aboveground biomass in that stage. (C) The dry matter distribution of different summer hybrids. Grain leaf and stalk were dry matter accumulation of each organ per plant; total were total dry matter accumulation per plant; other were dry matter accumulation of bract and cob per plant. (D) Average crop growth rate of different hybrids. Pre-silking and post-silking were average crop growth rate in that stage. ** and * indicated significantly different at the 0.01 and 0.05 probability levels, respectively; ns means there is no significantly different at the 0.05 probability levels, respectively.
DMRE and DMRC of leaf or stalk
The DMR of leaf and DMRE of leaf and stalk of DH605 were significantly lower than those of DH518, but there was no significant difference in DMR of stalk between two hybrids. Compared with DH605, the DMRE of leaf and stalk of DH518 increased by 8.0-11.2% and 5.1-5.9%, respectively (Table 3). The DMRC of leaf or stalk of DH518 was significantly higher than that of DH605, and the contribution to grain dry matter by PoSDMA of different summer maize hybrids was 90.0-93.6%, which was significantly higher than DMRC. (Table 4).
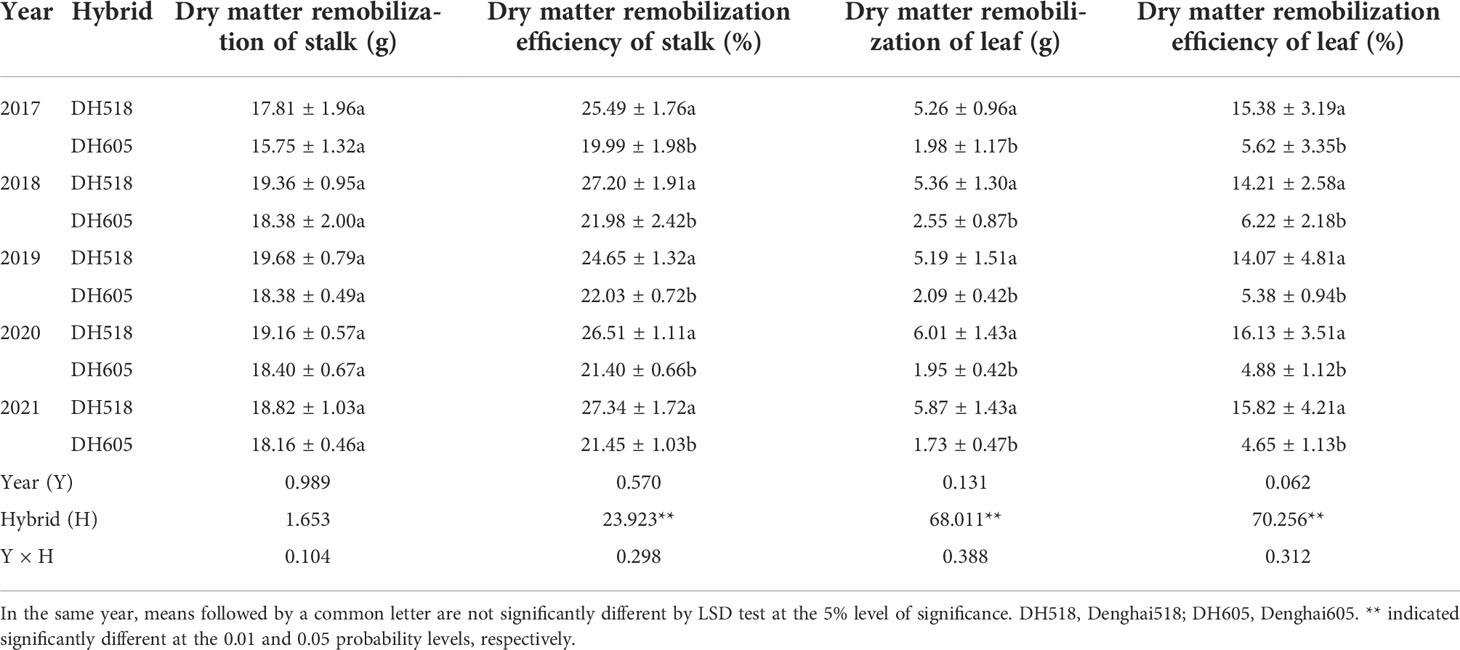
Table 3 Dry matter remobilization of vegetative tissues of summer maize hybrids with different growth duration from 2017 to 2021.
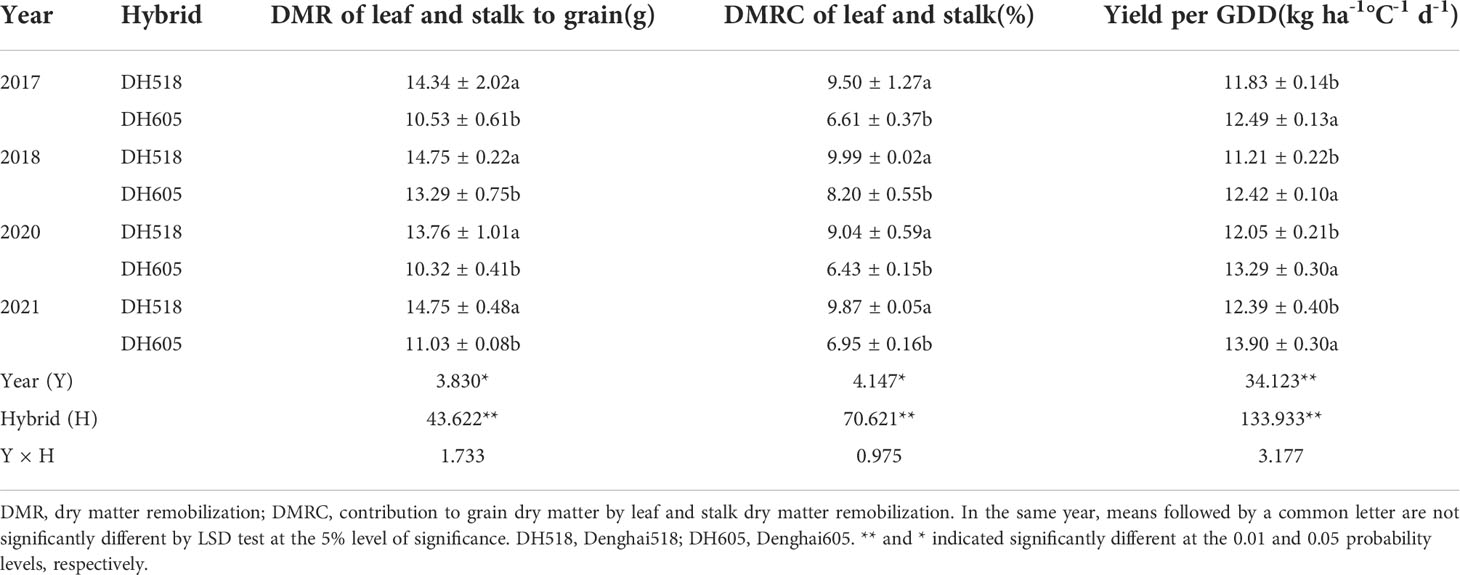
Table 4 Contribution to grain dry matter by leaf and stalk dry matter remobilization of summer maize hybrids with different growth duration (2017-2018; 2020-2021).
Compared with DH605, the 13C distribution to grains of DH518 increased by 3.4-4.4%, while the 13C distribution in stalks decreased by 2.6-11.2%. The distribution of 13C in different organs of different summer maize hybrids is as follows: grain>others>stalk>leaf. The proportion of 13C in ear is more than 60% (Figure 3).
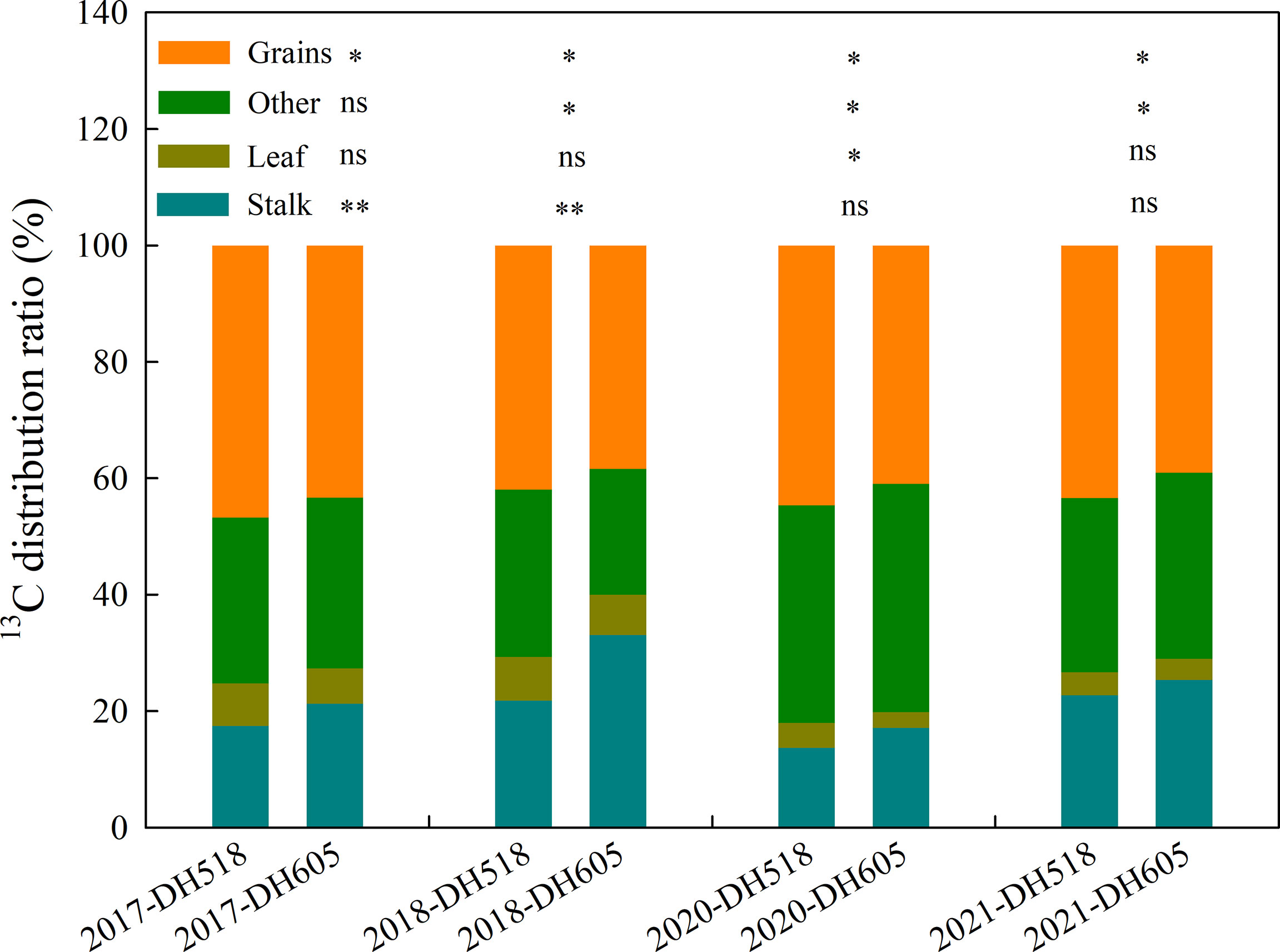
Figure 3 13C distribution to organs of summer maize hybrids with different growth duration (2017-2018; 2020-2021). 2017, 2018, 2019, 2020 and 2021 were different years; DH518, Denghai518; DH605, Denghai605. Grain, leaf and stalk were 13C distribution ratio to each organ; other were 13C distribution ratio of bract and cob. ** and * indicated significantly different at the 0.01 and 0.05 probability levels, respectively; ns means there is no significantly different at the 0.05 probability levels, respectively.
Photosynthetic characteristics
The dynamic variation in the post-silking population leaf area was shown in Figure 4. The overall change trend of the LAI of DH518 and DH605 were the same, which gradually increased from V6-R1 stage, at R1 the LAI was the highest and gradually decreased after R1 (Figure 4). The LAI of DH605 at the R1 and post-silking was significantly higher than that of DH518. Compared with DH518, the LAI of DH605 in R1, R3 and R6 increased by 10.8-19.0%, 9.6-15.1% and 12.7-23.6%, respectively. The change of post-silking LAI conformed to the Gaussian model. The analysis showed that the LAImax of the two hybrids appeared 3-8 days after silking. The DLAI of DH605 was more than 56 days, while that of DH518 was less than 51 days, which significantly accelerated the rapid decay process of DH518. Canopy photosynthetic capacity is a comprehensive reflection of Pn and LAI. The post-silking canopy photosynthetic capacity of DH605 was significantly higher than that of DH518. Compared with DH518, the canopy photosynthetic capacity of DH605 in R1, R3 and R5 increased by 17.0-25.9%, 11.5-22.8% and 79.5-104.3%, respectively. The SPAD value of DH605 was significantly higher than that of DH518 at R6, and the SPAD value of DH605 increased by 21.9-59.7% compared with DH518 at R6. There was no significant difference between different hybrids before R3 (Figure 4).
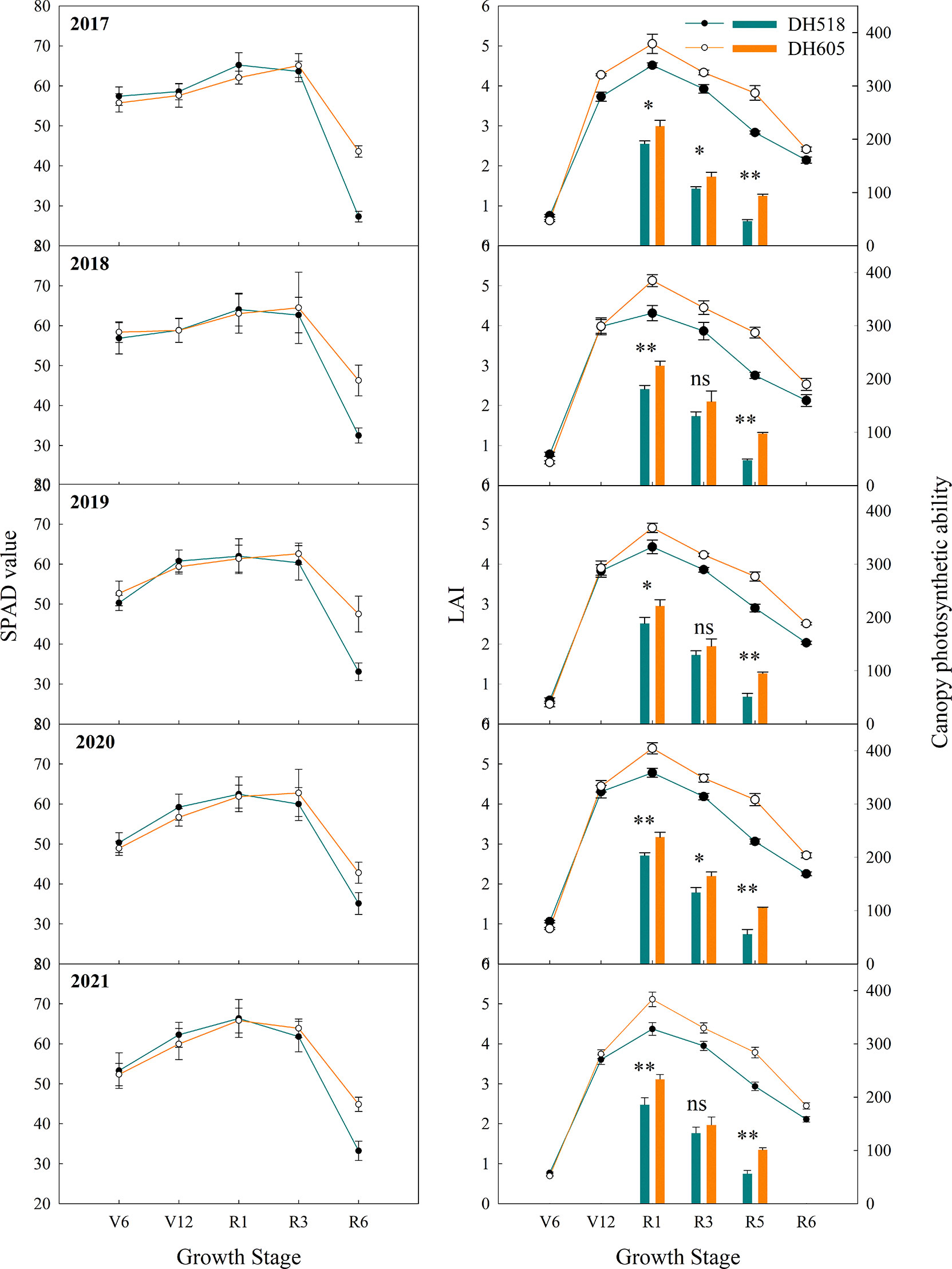
Figure 4 Chlorophyll relative content, leaf area index and canopy photosynthesis ability of summer maize hybrids with different growth duration (2017-2021). 2017, 2018, 2019, 2020 and 2021 were different years; DH518, Denghai518; DH605, Denghai605. V6, V12, R1, R3 and R6 were the sixth leaf stage, the 12th leaf stage, silking stage, milk stage and physiological maturity stage, respectively. ** and * indicated significantly different at the 0.01 and 0.05 probability levels, respectively. ns means there is no significantly different at the 0.05 probability levels.
The Pn, Gs and Ci of ear leaf of summer maize hybrids with different growth duration were not significantly different at R1 and R3 stages (Figure 5). The Pn and Gs of DH605 was significantly higher than those of DH518, and the Ci of DH605 was significantly lower than that of DH518. Compared with DH605, the Pn and Gs of DH518 decreased by 28.2-33.3% and 22.7-35.1%, respectively, and the Ci increased by 14.4-65.7%, indicating that the decrease of Pn in the later stage of DH518 was limited by stomatal factors and the decline of mesophyll cell function. The linear fitting of Pn after full expansion of ear leaf showed that the APD of DH605 was more than 10 days longer than that of DH518 (Table 5).
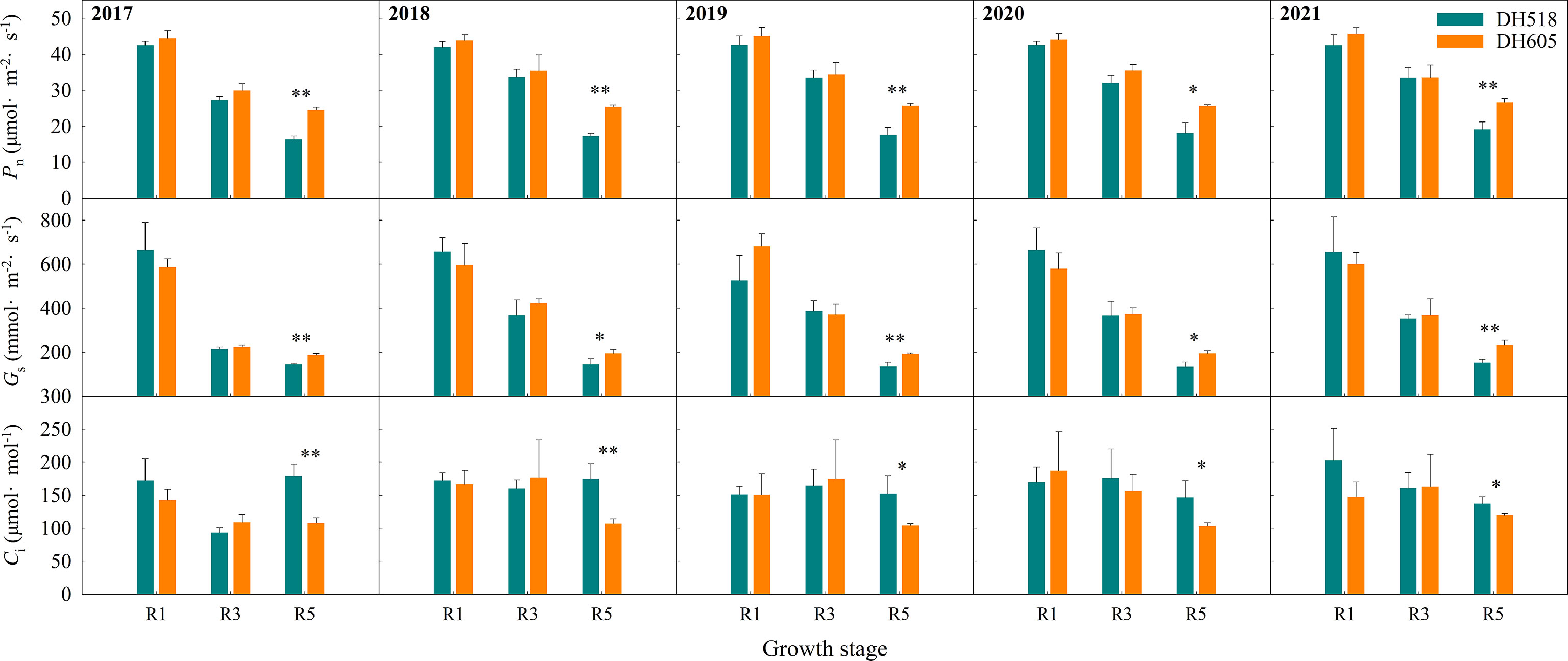
Figure 5 Post-silking changes of gas exchange parameters in leaves of summer maize hybrids with different growth duration (2017-2021). 2017, 2018, 2019, 2020 and 2021 were different years; DH518, Denghai518; DH605, Denghai605. R1, R3 and R5 were silking stage, milk stage and 45 days after silking. Pn, the net photosynthetic rates; Gs, stomatal conductance; Ci, intercellular CO2 concentration. ** and * indicated significantly different at the 0.01 and 0.05 probability levels, respectively.
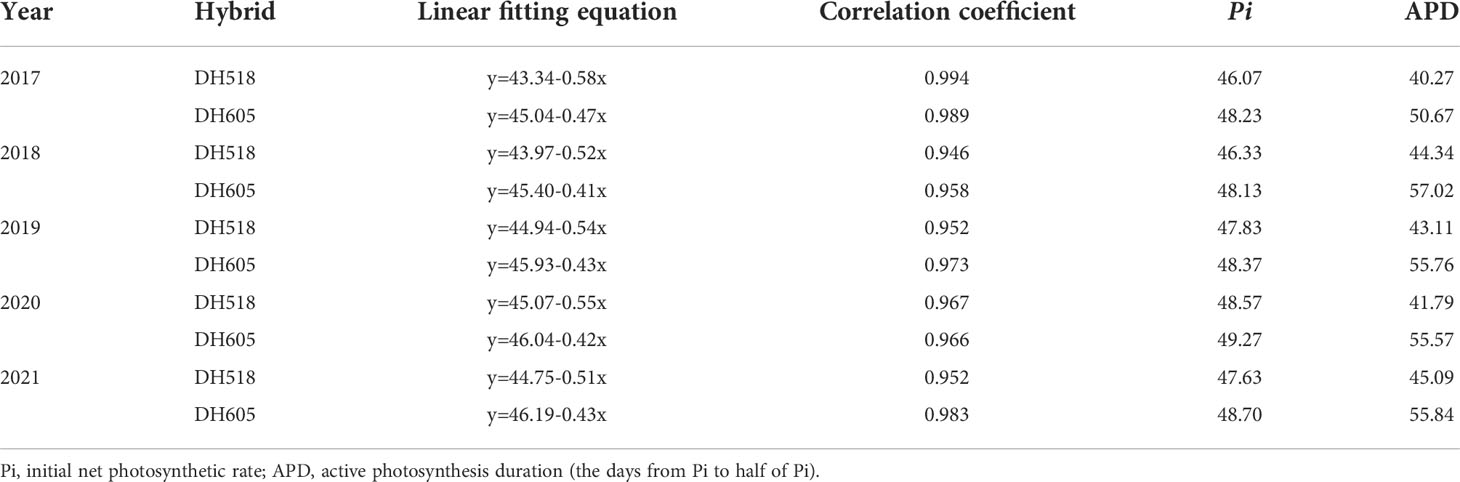
Table 5 Active photosynthesis duration of summer maize hybrids with different growth duration from 2017 to 2021.
The correlation analysis
Correlation analyses showed different relationships between yield and DMA and HI of summer maize hybrids with different growth duration. The yield of DH518 was positively correlated with dry matter and harvest index. DH518 reached significant levels in 2017 and 2020, and DH605 reached significant levels in 2018,2020 and 2021 (Table 6). The HI was negatively correlated with the days from sowing to R1 (r=0.922, p<0.01), but not with the days of R1-R6 (p>0.05) (Figure S1).
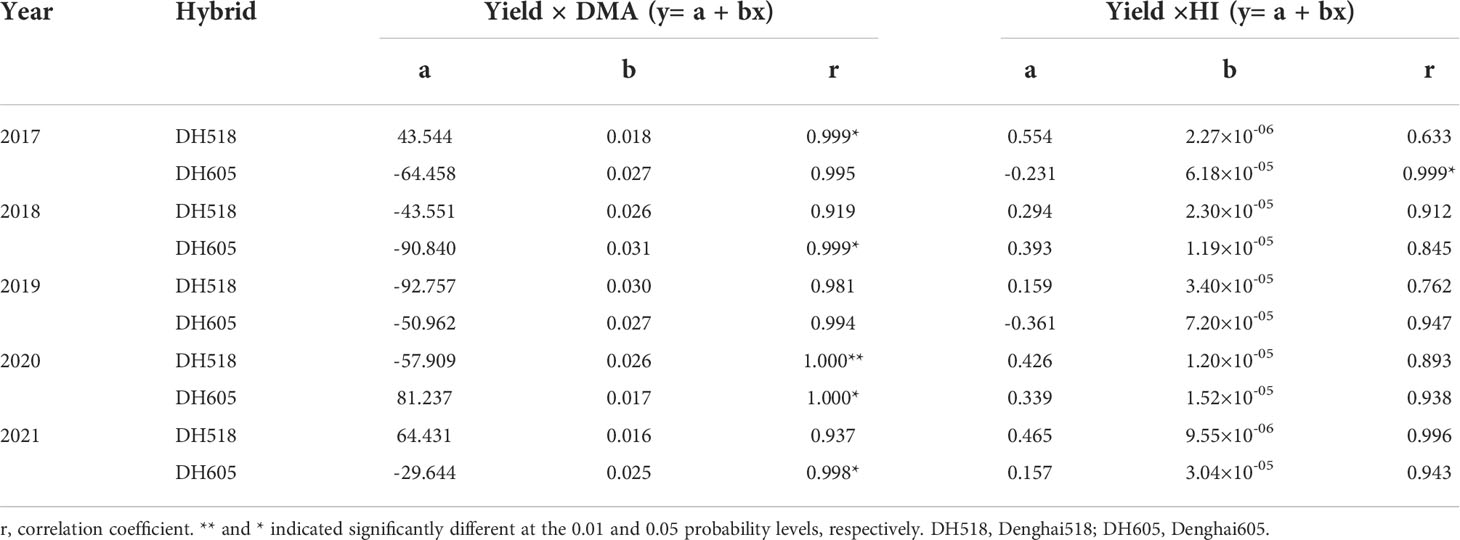
Table 6 Regression analysis between grain yield and DMA and HI of summer maize hybrids with different growth duration from 2017 to 2021.
Discussion
The light and temperature resource use efficiency from silking to physiological maturity stage of different summer maize hybrids
In the past few decades, breeding programs in pursuit of higher yields have extended the fertility period of maize (Valentinuz and Tollenaar, 2004; Luque et al., 2006). Compared with early maturity hybrids, the senescence of mid-late maturity hybrids occurs slower (Valentinuz and Tollenaar, 2004), which shows that the post-silking chlorophyll activity steady phase (RSP) of mid-late maturity hybrids lasted longer (Ding et al., 2005). Our results showed that the decline rate of SPAD value of DH605 was lower than that of DH518 after silking, which greatly delayed the senescence of leaves (Figure 4). Late maturity hybrids have higher leaf area index, longer photosynthesis time, slower leaf senescence and higher DMA rate at filling stage (Ma and Dwyer, 1998; Borrell et al., 2001; Duvick, 2005), which has a positive effect on the increase of yield (Rajcan and Tollenaar, 1999; Valentinuz and Tollenaar, 2004; Ding et al., 2005). The photosynthetic characteristics of leaves are closely related to the yield (Motto, 1987; Ma and Dwyer, 1998). DH605 had larger leaf area than that of DH518. The time of DH605 entering the rapid decay stage was more than 15 days later than that of DH518 (Figure 4). The DLAI of DH605 was 5 days and the APD of DH605 was more than 10 days longer than that of DH518 (Table 7). There is no doubt that the yield of mid-late maturity hybrids is higher than that of early maturity hybrids (Scott and Hector, 1997; Morinaka et al., 2006; Table 1), not only because of the extension of growth period (Table 2), but also because DH605 has higher light and temperature resource use efficiency. When the GDD was increased by 1 °C, the yield of DH605 was higher than that of DH518 by 0.86kg ha-1. Therefore, the light and temperature resource use efficiency from silking to physiological maturity stage is key to determine the yield of summer maize hybrids with different growth duration. In the future, in the research on the yield of summer maize hybrids with different growth duration, we should pay attention not only to the changes of growth period of different hybrids, but also to the differences of light and temperature resource use efficiency from silking to physiological maturity stage. In addition, it is necessary to further explore the physiological and molecular mechanisms that cause this difference, such as changes in the expression of genes and metabolic enzymes related to plant senescence.
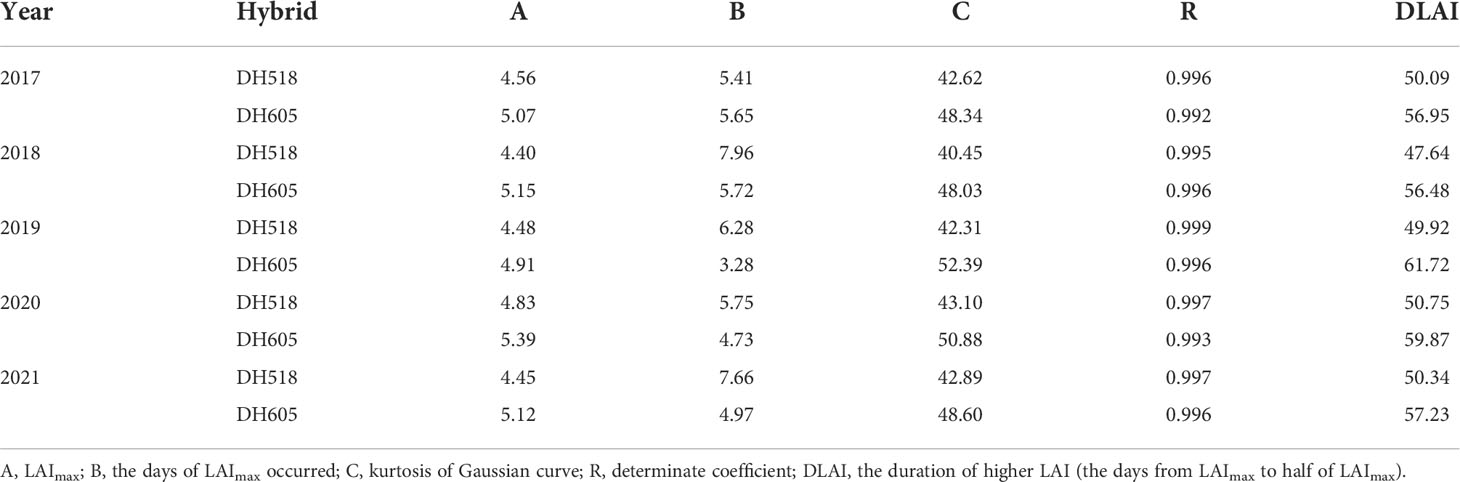
Table 7 Characteristic parameters of LAI fixt by Gaussian Model of summer maize hybrids with different growth duration from 2017 to 2021.
Accumulation, distribution and transport of dry matter in different summer maize hybrids
Grain filling of cereal crops depends on two sourcesof photosynthate, current photosynthate transferred directly to the grain and redistribution of photosynthate from reserve pools in vegetative tissues (Johnson and Tanner, 1972; Pheloung and Siddique, 1991; Kobata et al., 1992; Schnyder, 1993; Blum et al., 1994; Yang and Zhang, 2006). The characteristics of PrSDMR and PrSDMRC of different summer maize hybrids have been widely studied (Ning et al., 2013; Horacio et al., 2014; Chen et al., 2015a). However, the calculation of the proportion of DMRE and pre-silking and post-silking DMRC are not accurate. For example, about 40% of the PrSDMA was used to meet the development of other organs such as cob and bract (Figure 3), which was not taken into account in most articles. Therefore,13C pulse labeling technique was used to investigate the dry matter distribution characteristics of summer maize hybrids with different growth duration in this experiment. The 13C distribution to grains of DH518 was significantly higher than that of DH605, and the contribution rate to yield of HI and the HI of DH518 was significantly higher than that of DH605 (Table 8; Figure S2). This may be related to the activities of related enzymes in the process of sucrose transport, and higher amylase and SPS activities can promote starch degradation and photosynthetic redistributed from stalk to grain (Wang and Zhang, 2020). The next step could be to focus on the changes in the activities of these related enzymes to explain the reasons for the higher HI of DH518. This indicates that DH518 distributes more photosynthate from stalks to grains to ensure grain development. Non-structural carbohydrates stored in vegetative tissues can be remobilized and exported to grains during grain filling (Yang et al., 2001), but their contribution is limited (Cliquet et al., 1990). In wheat, 7-36% of the yield comes from the PrSDMR, and most of the yield comes from current photosynthate transferred directly (Austin et al., 1977; Papakosta and Gagianas, 1991; Masoni et al., 2007). The duration of sowing-R1 were 5-6 days shorter than that of DH605 (Table 1). The results based on 13C pulse labeling showed that the DMRC of DH518 was significantly higher than that of DH605 (Table 4)., which indicated that the shortening of sowing-R1 had no negative effect on yield. Most of the grain dry matter of maize comes from the photosynthetic products produced during grain filling (Tollenaar et al., 2004; Lee and Tollenaar, 2007), which is supported by the post-silking slight decrease of dry matter in vegetative tissues (Table 3). The PoSDMA of DH605 was significantly higher than that of DH518 (Figure 2). More PoSDMA may eventually make a significant contribution to higher yield. Our results showed that the contribution rate of PoSDMA to the yield of different summer maize hybrids was more than 90% (Table 4), which played a decisive role in the increase of yield.
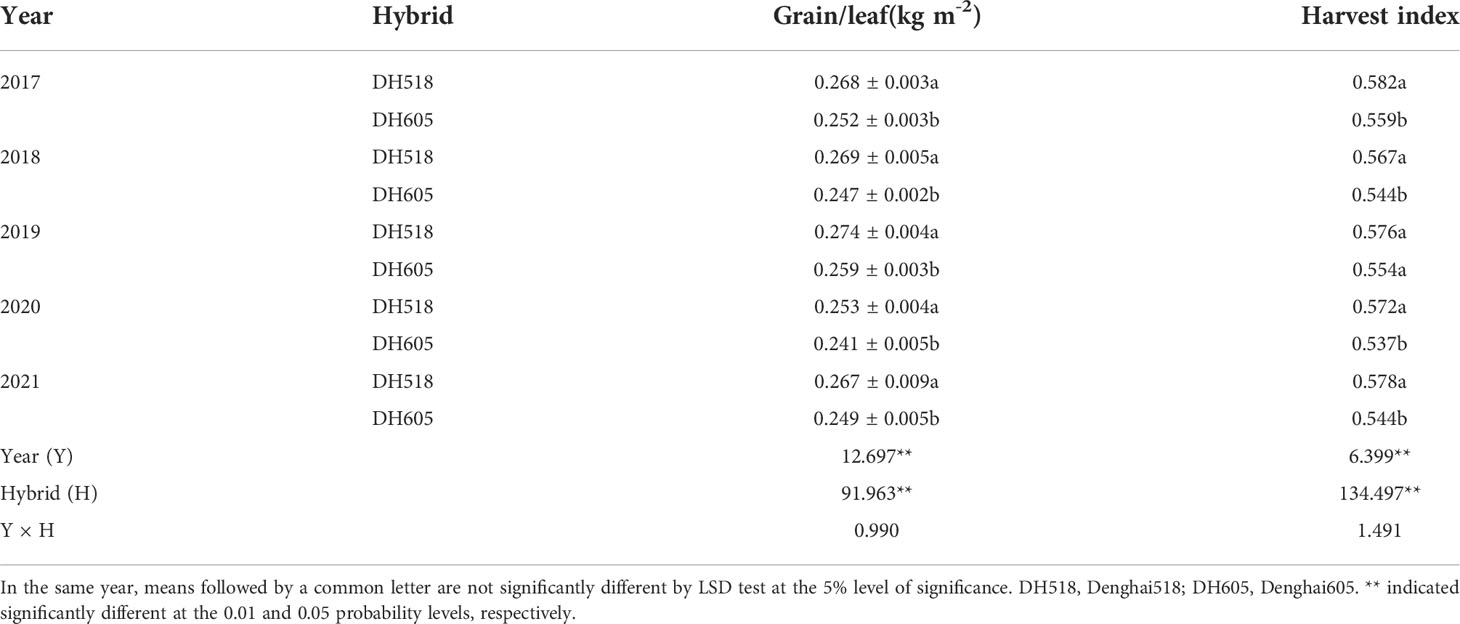
Table 8 Grain/leaf and harvest index of summer maize hybrids with different growth duration from 2017 to 2021.
HI and DMA of summer maize hybrids with different growth duration
The effects of HI and DMA on yield varied with crop species. The wheat yield was mainly attributed to the increase in HI (Nass, 1980; Brancourt-Hulmel et al., 2003; Xiao et al., 2012; Lo Valvo et al., 2017). In rice, the increase in yield before 1980 was related to the increase in HI, while after 1980 that was due to the increase in biomass production (Peng et al., 2000), which was similar to the succession of Chinese Japonica hybrids (Xiong et al., 2011). So far, hybrid rice and super rice have higher yields, which was mainly due to an increase in DMA rather than an increase in HI (Yang et al., 2006). However, maize as a C4 plant is different from most small-grain crops. Our results showed that the increase in DH518 yield in different years was mainly due to the increase in DMA and HI, while the increase in the yield of DH605 in different years was mainly depended on the increase in DMA. The grain/leaf ratio and HI of the population could be a better way to reflect the source-sink relationship of the population (Rajcan and Tollenaar, 1999). According to the theoretical value of the target yield of 15,000kg ha-1 and the LAI was 5-6 when intercepting 95% solar radiation, the grain-leaf ratio was 0.3-0.25 (Wang, 2008). The grain/leaf ratio of different summer maize hybrids was higher than 0.25 kg·m-2 (Table 8). On the premise of increasing the grain-leaf ratio, increasing the density and “expanding the pool and strengthening the source” can make the leaves per unit area to supply more grains and further increase the yield. With the increase of density, the DMA of maize per unit area increased significantly (Duvick, 2005; Li et al., 2015). Our results showed that the contribution rate of DMA to yield of different summer maize hybrids was higher than that of HI (Figure S2). Our previous studies have confirmed that the yield of DH518 was significantly lower than that of DH605 under 75,000 ha-1 planting density. There was no significant difference between the yield of DH518 at 90,000 plants ha-1 and that of DH605 at 75,000 plants ha-1, both of the two treatment yields were significantly higher than that of DH518 under 75,000 ha-1 planting density. (Wan et al., 2018; Figure 6 and S3). Therefore, the yield loss caused by the decrease of the light and temperature resource use efficiency from silking to physiological maturity stage could be made up by increasing the planting density of DH518 to increase the population DMA and light and temperature resource use efficiency.
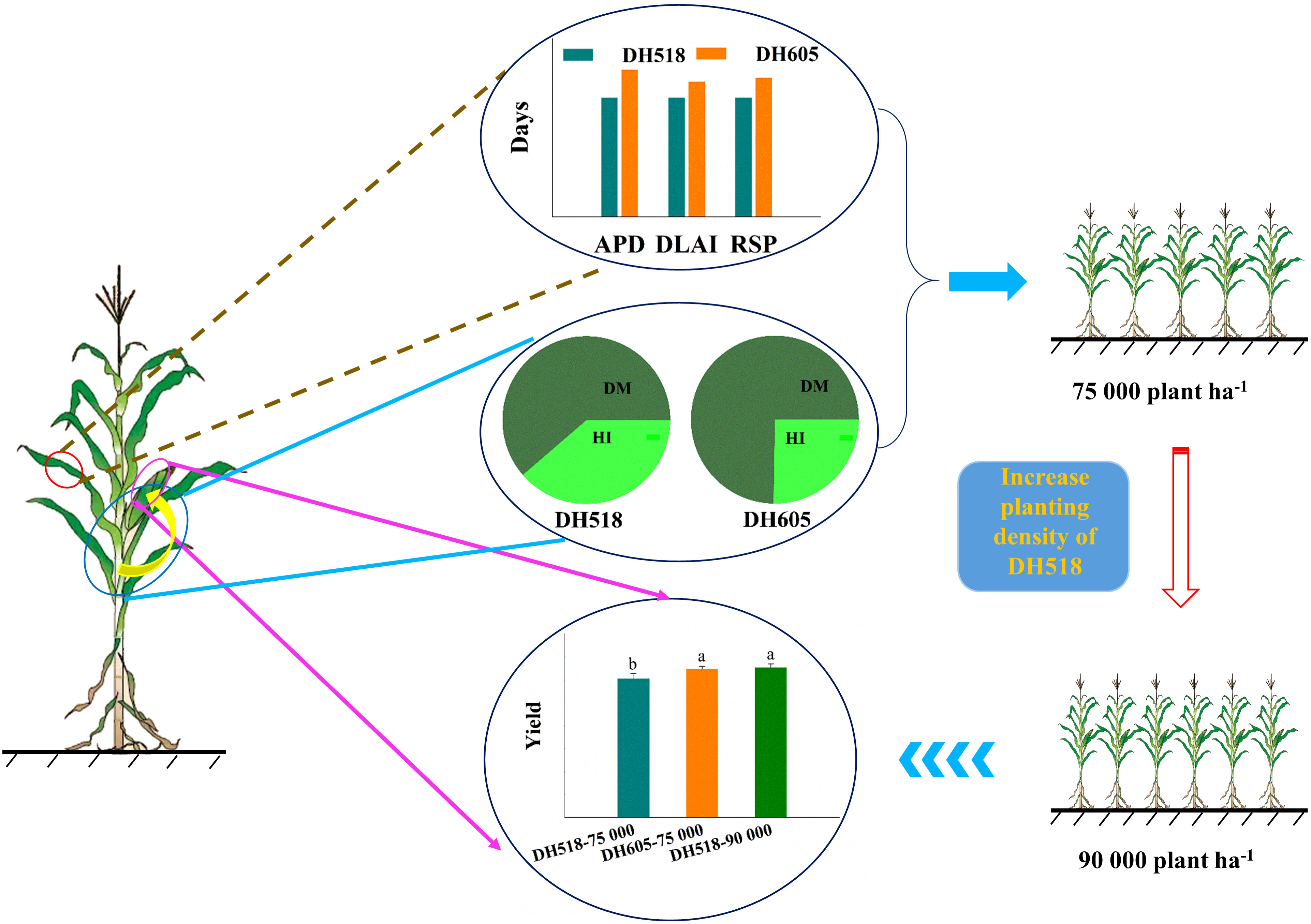
Figure 6 Grain yield, photosynthetic characteristics and contribution of dry matter accumulation and harvest index to yield of summer maize hybrids with different growth duration. The shortening of active photosynthesis duration, the duration of higher LAI and chlorophyll relative steady phase of early-maturing hybrids reduced the post-silking utilization efficiency of light and temperature resources and negatively affected the yield. Increasing planting density of early-maturing hybrids and increasing dry matter accumulation of population could significantly increase yield, and there was no significant difference between early-maturing hybrids and middle-late maturing hybrids. DH518, Denghai518; DH605, Denghai605. ADP, active photosynthesis duration; DLAI, the duration of higher LAI; RSP, chlorophyll relative steady phase. Different letters on bars indicate significant differences among treatments at p< 0.05 using LSD test.
Conclusion
The yield of DH605 was significantly higher than that of DH518. The difference in the growth period of different hybrids was mainly in the V6 to R1 stage. Compared to DH518, the higher light and temperature resource use efficiency from silking to physiological maturity stage of DH605 led to higher yield. The shortening of the growth stage of DH518 from sowing to R1 stage could make up for the yield loss by increasing the harvest index. The yield of DH518 could be increased by reasonably increasing planting density. Therefore, it is necessary to further study the carbon transport process of the plant to explain the association between the shortened growth period and the increased HI of early maturity hybrid. This study can provide a feasible research direction for the breeding and high yield and high efficiency cultivation of early maturity summer maize in the future.
Data availability statement
The original contributions presented in the study are included in the article/Supplementary Material. Further inquiries can be directed to the corresponding author.
Author contributions
JyZ: Data curation, writing—original draft, visualization, and investigation. BR, BZ, and PL: Supervision. JwZ: Conceptualization, writing—review and editing, and funding acquisition. All authors contributed to the article and approved the submitted version.
Funding
This work was supported by Province Key Research and Development Program of Shandong (2021LZGC014-2), National Natural Science Foundation of China (32172115), the earmarked fund for CARS (CARS-02-21).
Conflict of interest
The authors declare that the research was conducted in the absence of any commercial or financial relationships that could be construed as a potential conflict of interest.
Publisher’s note
All claims expressed in this article are solely those of the authors and do not necessarily represent those of their affiliated organizations, or those of the publisher, the editors and the reviewers. Any product that may be evaluated in this article, or claim that may be made by its manufacturer, is not guaranteed or endorsed by the publisher.
Supplementary material
The Supplementary Material for this article can be found online at: https://www.frontiersin.org/articles/10.3389/fpls.2022.992311/full#supplementary-material
Supplementary Figure 1 | Relationships between days at different growth duration and HI of summer maize (2017-2021). HI, harvest index. R1, silking stage; R6, physiological maturity stage. ** indicated significantly different at the 0.01 probability levels, ns means not significant at the level of p< 0.05.
Supplementary Figure 2 | Contribution rates of DM and HI to grain yield (2017-2021). DH518, Denghai518; DH605, Denghai605. DMA, dry matter accumulation; HI, harvest index.
Supplementary Figure 3 | Yield of summer maize hybrids with different growth duration (2016-2017). DH518, Denghai518; DH605, Denghai605. 75 000 and 90 000 were the planting density. Different letters on bars indicate significant differences among treatments at p< 0.05 using LSD test.
References
Austin, R. B., Edrich, J. A., Ford, M. A., Blackwell, R. D. (1977). The fate of dry matter, carbohydrates and 14C lost from the leaves and stems of wheat during grain filling. Ann. Bot. 41, 1309–1321. doi: 10.1093/oxfordjournals.aob.a085419
Blum, A., Sinmena, B., Mayer, J., Golan, G., Shpiler, L. (1994). Stem reserve mobilization supports wheat-grain filling under heat stress. Funct. Plant Biol. 21, 771–781. doi: 10.1071/PP9940771
Borrell, A. K., Hammer, G. L., Oosterom, E. V. (2001). Stay-green: a consequence of the balance between supply and demand for nitrogen during grain filling. Ann. Appl. Biol. 138, 91–95. doi: 10.1111/j.1744-7348.2001.tb00088.x
Brancourt-Hulmel, M., Doussinault, G., Lecomte, C., Bérard, P., Le Buanec, B., Trottet, M. (2003). Genetic improvement of agronomic traits of winter wheat cultivars released in France from 1946 to 1992. Crop Sci. 43, 37–45. doi: 10.2135/cropsci2003.3700
Chen, G. P. (1994). Dry matter production and distribution. J. Maize Sci. 2, 48–53. doi: cnki:sun:ymkx.0.1994-01-013
Chen, K. R., Kumudini, S. V., Tollenaar, M., Vyn, T. J. (2015a). Plant biomass and nitrogen partitioning changes between silking and maturity in newer versus older maize hybrids. Field Crops Res. 2015, 315–328. doi: 10.1016/j.fcr.2015.08.013
Chen, Y. L., Xiao, C. X., Chen, X. C., Li, Q., Zhang, J., Chen, F. J., et al. (2014). Characterization of the plant traits contributed to high grain yield and high grain nitrogen concentration in maize. Field Crops Res. 159, 1–9. doi: 10.1016/j.fcr.2014.01.002
Chen, Y. L., Xiao, C. X., Wu, D., Xia, T., Chen, Q., Chen, F. J., et al. (2015b). Effects of nitrogen application rate on grain yield and grain nitrogen concentration in two maize hybrids with contrasting nitrogen remobilization efficiency. Eur. J. Agron. 62, 79–89. doi: 10.1016/j.eja.2014.09.008
Ciampitti, I. A., Camberato, J. J., Murrel, S. C., Vyn, T. J. (2013). Maize nutrient accumulation and partitioning in response to plant density and nitrogen rate: i. macronutrients. Agron. J. 105, 783–795. doi: 10.2134/agronj2012.0467
Cliquet, J., Deléens, E., Mariotti, A. (1990). C and n mobilization from stalk and leaves during kernel filling by 13C and 15N tracing in Zea mays l. Plant Physiol. 94, 1547–1553. doi: 10.1104/pp.94.4.1547
Ding, L., Wang, K. J., Jiang, G. M., Liu, M. Z., Niu, S. L., Gao, L. M. (2005). Post-anthesis changes in photosynthetic traits of maize hybrids released in different years. Field Crops Res. 93, 108–115. doi: 10.1016/j.fcr.2004.09.008
Donald, C. M., Hamblin, J. (1976). The biological yield and harvest index of cereals as agronomic and plant breeding criteria. Adv. Agro. 28, 361–405. doi: 10.1016/S0065-2113(08)60559-3
Duvick, D. N. (2005). The contribution of breeding to yield advances in maize (Zea mays l.). Adv. Agron. 86, 83–145. doi: 10.1016/S0065-2113(05)86002-X
Echarte, L., Andrade, F. H. (2003). Harvest index stability of argentinean maize hybrids released between 1965 and 1993. Field Crops Res. 82, 1–12. doi: 10.1016/S0378-4290(02)00232-0
Edwards, J. T., Purcell, L. C., Vories, E. D. (2005). Light interception and yield potential of short-season maize (Zea mays l.) hybrids in the midsouth. Agron. J. 97, 225–234. doi: 10.1051/agro:2004062
FAO (2020) FAOSTAT–agriculture database. Available at: https://faostat.fao.org/.
Gregory, S. M., Wilhelm, W. W. (1997). Growing degree-days: one equation, two interpretations. Agr. For. Meteorol. 87, 1291–1300. doi: 10.1016/S0168-1923(97)00027-0
Guha, S., Chandra, H. (2021). Measuring disaggregate level food insecurity via multivariate small area modelling: evidence from rural districts of uttar pradesh, India. Food Secur. 13, 597–615. doi: 10.1007/s12571-021-01143-1
Horacio, A. A., Eduardo, A. T., Mariana, A., María, S. Z., Fernando, H. A., Juan, J. G. (2014). Carbon assimilation, leaf area dynamics, and grain yield in contemporary earlier- and later-senescing maize hybrids. Eur. J. Agron. 59, 29–38. doi: 10.1016/j.eja.2014.05.007
Hou, P., Gao, Q., Xie, R., Li, S., Meng, Q., Kirkby, E. A., et al. (2012). Grain yields in relation to n requirement: Optimizing nitrogen management for spring maize grown in China. Field Crops Res. 129, 1–6. doi: 10.1016/j.fcr.2012.01.006
Hu, C. H., Dong, S. T., Wang, K. J., Sun, Q. Q. (1998). Evolution of maize cultivars in different ages in China: II Evolution of material production characteristics. J. Maize Sci. 6, 49–53. doi: cnki:sun:ymkx.0.1998-03-014
Ileleji, K. E., Maier, D. E., Woloshuk, C. P. (2007). Evaluation of different temperature management strategies for suppression of zamias in stored maize. J. Stored Prod. Res. 43, 480–488. doi: 10.1016/j.jspr.2007.01.002
Johnson, D. R., Tanner, L. W. (1972). Calculation of the rate and duration of grain filling in corn (Zea mays l.). Crop Sci. 12, 485–486. doi: 10.2135/cropsci1972.0011183X001200040028x
Kobata, T., Palta, J. A., Turner, N. C. (1992). Rate of development of post-anthesis water deficits and grain filling of spring wheat. Crop Sci. 32, 1238–1242. doi: 10.2135/cropsci1992.0011183X003200050035x
Lee, E. A., Tollenaar, M. (2007). Physiological basis of successful breeding strategies for maize grain yield. Crop Sci. 47, 202–215. doi: 10.2135/cropsci2007.04.0010IPBS
Lin, X. E., Ji, H. Q., Niu, J. H., Hu, Y. M., Fu, Z. J., Liu, Z. H., et al. (2011). Cloning one CIPK gene from a thermo-sensitive genic self-incompatible line in maize expressing under different temperatures. Sci. Agric. Sin. 10, 813–819. doi: 10.1016/S1671-2927(11)60066-7
Liu, B., Chen, X., Meng, Q., Yang, H., Van Wart, J. (2017). Estimating maize yield potential and yield gap with agro-climatic zones in China–distinguish irrigated and rainfed conditions. Agr. For. Meteorol. 239, 108–117. doi: 10.1016/j.agrformet.2017.02.035
Liu, W. M., Hou, P., Liu, G. Z., Yang, Y. S., Guo, X. X., Ming, B., et al. (2020). Contribution of total dry matter and harvest index to maize grain yield–a multisource data analysis. Food Energy Secur. 9, 1–12. doi: 10.1002/fes3.256
Li, J., Xie, R. Z., Wang, K. R., Ming, B., Guo, Y. Q., Zhang, G. Q., et al. (2015). Variations in maize dry matter, harvest index, and grain yield with plant density. Agron. J. 107, 829–834. doi: 10.2134/agronj14.0522
Lo Valvo, P., Miralles, D. J., Serrago, R. A. (2017). Genetic progress in Argentine bread wheat hybrids released between 1918 and 2011: Changes in physiological and numerical yield components. Field Crops Res. 221, 314–321. doi: 10.1016/j.fcr.2017.08.014
Luque, S. F., Cirilo, A. G., Otegui, M. E. (2006). Genetic gains in grain yield and related physiological attributes in Argentine maize hybrids. Field Crops Res. 95, 383–397. doi: 10.1016/j.fcr.2005.04.007
Ma, B. L., Dwyer, M. L. (1998). Nitrogen uptake and use in two contrasting maize hybrids differing in leaf senescence. Plant Soil. 199, 283–291. doi: 10.1023/A:1004397219723
Ma, Z. T., Ren, B. Z., Zhao, B., Liu, P., Zhang, J. W. (2022). Increasing grain yield, nitrogen use efficiency of summer maize and reducing greenhouse gas emissions by applying urea ammonium nitrate solution. Agron. J. 114, 948–960. doi: 10.1002/agj2.20942
Masoni, A., Ercoli, L., Mariotti, M., Arduini, I. (2007). Post-anthesis accumulation and remobilization of dry matter, nitrogen and phosphorus in durum wheat as affected by soil type. Eur. J. Agron. 26, 179–186. doi: 10.1016/j.eja.2006.09.006
Ma, D. L., Xie, R. Z., Niu, X. K., Li, S. K., Long, H. L., Liu, Y. E. (2014). Changes in the morphological traits of maize genotypes in China between the 1950s and 2000s. Eur. J. Agron. 58, 1–10. doi: 10.1016/j.eja.2014.04.001
Meghji, M. R., Dudley, J. W., Lambert, R. J., Sprague, G. F. (1984). Inbreeding depression, inbred and hybrid grain yields, and other traits of maize genotypes representing three Eras1. Crop Sci. 24, 545. doi: 10.2135/cropsci1984.0011183x002400030028x
Mi, G., Liu, J., Chen, F., Zhang, F., Cui, Z., Liu, X. (2003). Nitrogen uptake and remobilization in maize hybrids differing in leaf senescence. J. Plant Nutr. 26, 237–247. doi: 10.1081/PLN-120016507
Montgomery, E. G. (1911). “Correlation studies in corn,” in 24th annual report (Nebraska, Mo, USA: Agricultural Experiment Station), 108–159.
Morinaka, Y., Sakamoto, T., Inukai, Y., Agetsuma, M., Kitano, H., Ashikari, M., et al. (2006). Morphological alteration caused by brassinosteroid insensitivity increases the biomass and grain production of rice. Plant Physiol. 141, 924–931. doi: 10.1104/pp.106.077081
Motto, M. (1987). Genotype-dependent leaf senescence in maize: inheritance and effects of pollination-prevention. Plant Physiol. 85, 720–725. doi: 10.1104/pp.85.3.720
Nass, H. G. (1980). Harvest index as a selection criterion for grain yield in two spring wheat crosses grown at two population densities. Can. J. Plant Sci. 60, 1141–1146. doi: 10.4141/cjps80-166
Ning, P., Li, S., Yu, P., Zhang, Y., Li, C. (2013). Post-silking accumulation and partitioning of dry matter, nitrogen, phosphorus and potassium in maize hybrids differing in leaf longevity. Field Crops Res. 144, 19–27. doi: 10.1016/j.fcr.2013.01.020
Odunitan-Wayas, F. A., Alaba, O. A., Lambert, E. V. (2021). Food insecurity and social injustice: the plight of urban poor African immigrants in south Africa during the COVID-19 crisis. Glob. Public Health 16, 1–4. doi: 10.1080/17441692.2020.1854325
O’Hara, S., Toussaint, E. C. (2021). Food access in crisis: food security and COVID-19. Ecol. Econ. 180, 106859. doi: 10.1016/j.ecolecon.2020.106859
Oncini, F. (2021). Food support provision in COVID-19 times: a mixed method study based in greater Manchester. Agric. Hum. Val. 38, 1201–1213. doi: 10.1007/s10460-021-10212-2
Papakosta, D. K., Gagianas, A. A. (1991). Nitrogen and dry matter accumulation, remobilization, and losses for Mediterranean wheat during grain filling. Agron. J. 83, 864–870. doi: 10.2134/agronj1991.00021962008300050018x
Peng, S. B., Laza, R. C., Visperas, R. M., Sanico, A. L., Cassman, K. G., Khush, G. S. (2000). Grain yield of rice cultivars and lines developed in the Philippines since 1966. Crop Sci. 40, 307–314. doi: 10.2135/cropsci2000.402307x
Pheloung, P., Siddique, K. (1991). Contribution of stem dry matter to grain yield in wheat cultivars. Funct. Plant Biol. 18, 53–64. doi: 10.1071/PP9910053
Piazza, P., Jasinski, S., Tsiantis, M. (2005). Evolution of leaf developmental mechanisms. New Phytol. 167, 693–710. doi: 10.1111/j.1469-8137.2005.01466.x
Ptaszynska, G., Silesia, H. (2008). Yield variation of maize hybrids with different growing period in climatic conditions of central wielkopolska region. Dulwich Centre Review. 7, 93. doi: 10.1063/1.1692753
Rajcan, I., Tollenaar, M. (1999). Source: sink ratio and leaf senescence in maize: I.Dry matter accumulation and partitioning during grain filling. Field Crops Res. 60, 245–253. doi: 10.1016/s0378-4290(98)00142-7
Saidou, A., Janssen, B. H., Temmingh, E. J. M. (2003). Effects of soil properties, mulch and NPK fertilizer on maize yields and nutrient budgets on ferralitic soils in southern Benin. Agr Ecosyst. Environ. 100, 265–273. doi: 10.1016/S0167-8809(03)00184-1
Schnyder, H. (1993). The role of carbohydrate storage and redistribution in the source-sink relations of wheat and barley during grain filling - a review. New Phytol. 123, 233–245. doi: 10.2307/2557991
Schussler, J. R., Westgate, M. E. (1994). Increasing assimilate reserves does not prevent kernel abortion at low water potential in maize. Crop Sci. 34, 1569–1576. doi: 10.2135/cropsci1994.0011183X003400060028x
Scott, C. C., Hector, J. B. (1997). Using a chlorophylls meter to estimate specific leaf nitrogen of tropical maize during vegetative growth. Agron. J. 89, 557–562. doi: 10.2134/agronj1997.00021962008900040004x
Slattery, R. A., Ort, D. R. (2021). Perspectives on improving light distribution and light use efficiency in crop canopies. Plant Physiol. 185, 34–48. doi: 10.1093/plphys/kiaa006
Sun, H. Y., Zhang, X. Y., Chen, S. Y., Pei, D., Liu, C. M. (2007). Effects of harvest and sowing time on the performance of the rotation of winter wheat-summer maize in the north China plain. Ind. Crops Prod. 25, 239–247. doi: 10.1016/j.indcrop.2006.12.003
Tollenaar, M. (1989). Genetic improvement in grain yield of commercial maize hybrids grown in Ontario from 1959 to 1988. Crop Sci. 29, 1365–1371. doi: 10.2135/cropsci1989.0011183X002900060007x
Tollenaar, M., Ahmadzadeh, A., Lee, E. A. (2004). Physiological basis of heterosis for grain yield in maize. Crop Sci. 44, 2086–2094. doi: 10.2135/cropsci2004.2086
Valentinuz, O. R., Tollenaar, M. (2004). Vertical profile of leaf senescence during thegrain-filling period in older and newer maize hybrids. Crop Sci. 44, 827–834. doi: 10.2135/cropsci2004.8270
Wang, Y. J. (2008). Study on population quality and individual physiology function of super high-yielding maize (Zea mays l.) (Taian Shandong Agric. Univ). doi: 10.7666/d.y1374885
Wang, J., Wang, E., Yang, X., Zhang, F., Hong, Y. (2012). Increased yield potential of wheat-maize cropping systalk in the north China plain by climate change adaptation. Climatic Change. 113, 825–840. doi: 10.1007/s10584-011-0385-1
Wang, G., Zhang, J. (2020). Carbohydrate, hormone and enzyme regulations of rice grain filling under post-anthesis soil drying. Environ. Exp. Bot. 178, 104165. doi: 10.1016/j.envexpbot.2020.104165
Wan, Z. H., Ren, B. Z., Zhao, B., Liu, P., Zhang, J. W. (2018). Grain filling and dehydration characteristics of summer maize hybrids with different growth duration and effect of plant density. Acta Agron. Sin. 44, 1517–1526. doi: 10.3724/SP.J.1006.2018.01517
Wu, F. B., Wu, L. H., Xu, F. H. (1998). Chlorophyll meter to predict nitrogen sidedress requirements for short-season cotton (Gossypium nirsutum l.). Field Crops Res. 56, 309–314. doi: 10.1016/S0378-4290(97)00108-1
Xiao, Y. G., Qian, Z. G., Wu, K., Liu, J. J., Xia, X. C., Ji, W. Q., et al. (2012). Genetic gains in grain yield and physiological traits of winter wheat in Shandong province, China, from 1969 to 2006. Crop Sci. 52, 44–56. doi: 10.2135/cropsci2011.05.0246
Xiong, J., Chen, G. L., Wang, S. H., Ding, Y. F. (2011). The difference in grain yield and plant type among typical japonica hybrids in different years in jiangsu province. J. Nanjing Agric. Univ. 34, 1–6. doi: 10.7685/j.issn.1000-2030.2011.05.001
Yang, J. C., Wang, P., Liu, L. J., Wang, Z. Q., Zhu, Q. S. (2006). Evolution characteristics of grain yield and plant type for mid-season indica rice cultivars. Acta Agron. Sin. 07, 949–955. doi: 10.3321/j.issn:0496-3490.2006.07.001
Yang, J., Zhang, J. (2006). Grain filling of cereals under soil drying. New Phytol. 169, 223–236. doi: 10.1111/j.1469-8137.2005.01597.x
Yang, J. C., Zhang, J. H., Wang, Z. Q., Zhu, Q. S., Wang, W. (2001). Remobilization of carbon reserves in response to water deficit during grain filling of rice. Field Crops Res. 71, 47–55. doi: 10.1016/S0378-4290(01)00147-2
Zhang, R. X., Cheng, Z. Q. (1992). Preliminary study on the net photosynthetic active duration of leaf in wheat. J. Nanjing Normal Univ. (Natural Sci. Edition). 15, 76–86. doi: cnki:sun:jsnb.0.1990-01-000
Keywords: maize, harvest index (HI), resource use efficiency (RUE), yield, dry matter
Citation: Zhao J, Ren B, Zhao B, Liu P and Zhang J (2022) Yield of summer maize hybrids with different growth duration determined by light and temperature resource use efficiency from silking to physiological maturity stage. Front. Plant Sci. 13:992311. doi: 10.3389/fpls.2022.992311
Received: 12 July 2022; Accepted: 05 September 2022;
Published: 29 September 2022.
Edited by:
Hans-Peter Kaul, University of Natural Resources and Life Sciences Vienna, AustriaReviewed by:
Ritesh Kumar, Boyce Thompson Institute (BTI), United StatesMuhiuddin Faruquee, International Rice Research Institute, Bangladesh
Hans-Peter Piepho, University of Hohenheim, Germany
Copyright © 2022 Zhao, Ren, Zhao, Liu and Zhang. This is an open-access article distributed under the terms of the Creative Commons Attribution License (CC BY). The use, distribution or reproduction in other forums is permitted, provided the original author(s) and the copyright owner(s) are credited and that the original publication in this journal is cited, in accordance with accepted academic practice. No use, distribution or reproduction is permitted which does not comply with these terms.
*Correspondence: Jiwang Zhang, jwzhang@sdau.edu.cn