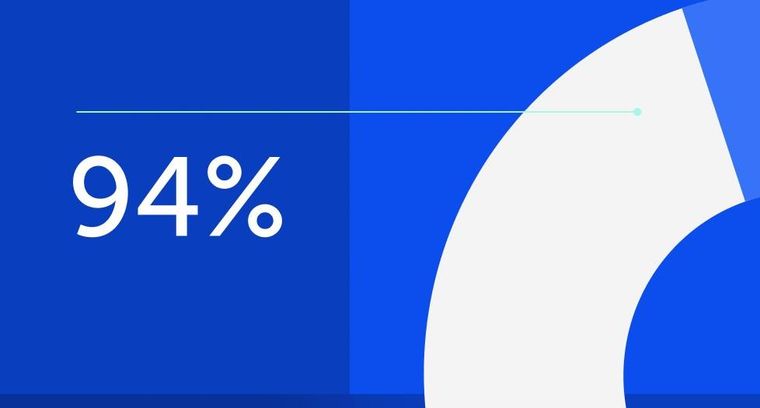
94% of researchers rate our articles as excellent or good
Learn more about the work of our research integrity team to safeguard the quality of each article we publish.
Find out more
REVIEW article
Front. Plant Sci., 29 September 2022
Sec. Plant Symbiotic Interactions
Volume 13 - 2022 | https://doi.org/10.3389/fpls.2022.985429
This article is part of the Research TopicBiostimulants in Agriculture II: Towards a Sustainable FutureView all 78 articles
Ensuring food and nutritional security, it is crucial to use chemicals in agriculture to boost yields and protect the crops against biotic and abiotic perturbations. Conversely, excessive use of chemicals has led to many deleterious effects on the environment like pollution of soil, water, and air; loss of soil fertility; and development of pest resistance, and is now posing serious threats to biodiversity. Therefore, farming systems need to be upgraded towards the use of biological agents to retain agricultural and environmental sustainability. Plants exhibit a huge and varied niche for endophytic microorganisms inside the planta, resulting in a closer association between them. Endophytic microorganisms play pivotal roles in plant physiological and morphological characteristics, including growth promotion, survival, and fitness. Their mechanism of action includes both direct and indirect, such as mineral phosphate solubilization, fixating nitrogen, synthesis of auxins, production of siderophore, and various phytohormones. Medicinal and aromatic plants (MAPs) hold a crucial position worldwide for their valued essential oils and several phytopharmaceutically important bioactive compounds since ancient times; conversely, owing to the high demand for natural products, commercial cultivation of MAPs is on the upswing. Furthermore, the vulnerability to various pests and diseases enforces noteworthy production restraints that affect both crop yield and quality. Efforts have been made towards enhancing yields of plant crude drugs by improving crop varieties, cell cultures, transgenic plants, etc., but these are highly cost-demanding and time-consuming measures. Thus, it is essential to evolve efficient, eco-friendly, cost-effective simpler approaches for improvement in the yield and health of the plants. Harnessing endophytic microorganisms as biostimulants can be an effective and alternative step. This review summarizes the concept of endophytes, their multidimensional interaction inside the host plant, and the salient benefits associated with endophytic microorganisms in MAPs.
Plants perform a range of fundamental functions in helping all distinct forms of living beings and release chemical signals to communicate with them. The roots give anchorage to the plant in soil and assist in the acquisition of water and nutrients, as well as produce chemical compounds that induce various types of interactions. This constitutes mutualism with the microorganisms that include fungi in mycorrhizal associations, endophytes, and plant growth-promoting rhizobacteria (PGPRs) as well as parasitism with pathogenic microorganisms, other plants, and herbivores (Badri et al., 2009). The plant roots discharge tremendous quantities of chemical compounds to fight pathogens and attract advantageous microorganisms (Badri and Vivanco, 2009). Such plant–microbe interactions occur at different trophic levels within a sophisticated arrangement of communities (Yan et al., 2019). Approximately 470 million years ago, the evolution of aquatic plants into terrestrial organisms was made possible by cooperating with soil microbes, and many of those microbe–plant interactions still persist (Bhattacharya and Medlin, 1998; Palmer et al., 2004; Ni et al., 2012; Williams et al., 2013). Some plant–microbe interactions are commensalism, where no harm is done to the plant, but the microbe gains some advantage. Several distinct interactions are advantageous to both partners (e.g., they are called mutualistic). Lastly, another group of microorganisms are pathogens and parasites to their host plants. In all the incidents, the microbe and the plant have established the ability to communicate. The microorganism recognizes and responds to the chemical signaling molecules produced by the plants. This usually results in the discharge of microbial compounds that are in turn identified by the plant, thereby generating a two-way “communication” that uses a molecular lexicon. Once a plant–microbe relationship begins, microbes and plants continue to observe their partner’s physiology and coordinate their activities accordingly.
Medicinal and aromatic plants (MAPs) hold a crucial position within people’s healthcare systems throughout the world. Until the arrival of advanced medicines, an oversized population in emerging nations has traditionally relied upon the products obtained from plants, particularly from forests. Numerous medicinal and aromatic crops are being exploited for economic uses. Approximately 12.5% of the more than 422,000 plant species have been universally documented for medicinal properties; however, only a couple of hundreds are known to be in cultivation (Schippmann et al., 2002). There is a need to grow MAPs to maintain their steady supply and conservation amidst decreasing stocks from natural sources and rising global interest. Apart from increasing farmers’ income, MAPs cultivation also acts as insurance crops against the climate extremes. Its cultivation for essential oils and several phytopharmaceutically important compounds is an age-old aspect of agriculture; conversely, owing to the high demand for natural products, commercial cultivation of MAPs is on the upswing. Furthermore, the vulnerability to various biotic and abiotic perturbations (phytopathogens, drought, water-logging, salinity, temperature, etc.) enforces noteworthy production constraints to diminish both yield and overall quality of the crops. Until the arrival of today’s medicine, a large part of the population in developing nations traditionally depended on the products obtained from plants, particularly from forests, for administering human and livestock ailments. Some efforts have been made towards enhancing yields of plant crude drugs by improving crop varieties, cell cultures, transgenic plants, etc., but these are highly cost-demanding and time-consuming measures. Thus, it is vital to evolve efficient, eco-friendly, cost-effective simpler approaches for improvement in yield and health of the plants.
Plant growth-promoting endophytic microorganisms inhabit and proliferate inside the plants without any distinct symptoms of any diseases in-planta (Bacon et al., 2002), and their mechanism includes both direct and indirect actions such as mineral phosphate solubilization (Verma et al., 2001), fixating nitrogen (Alishahi et al., 2020), synthesis of auxins (Patten and Glick, 2002), production of siderophore (Lodewyckx et al., 2002), and various phytohormones (Costacurta and Vanderleyden, 1995). As many abiotic stresses induce multiple physiological disturbances, which include stomatal closure and stunted plant growth, this ultimately results in lesser crop yield. It has been well reported that ACC (1-aminocyclopropane-1-carboxylic acid) deaminase-containing microbes lower the effect of stress induced by higher ethylene levels of the host plant (Penrose et al., 2001; Sessitsch et al., 2005; Glick, 2005; Sun et al., 2009; Rashid et al., 2012). Therefore, microbial treatments can protect plants from the damaging effects of environmental perturbations (Ali et al., 2014). Changes in climatic conditions such as rainfall, ambient CO2, and varying temperatures affect agriculture through countless constraints resulting in either low yields or sometimes death of the plants. This review reveals the role of endophytes in improving agricultural sustainability, which can serve as a valuable approach toward green cultivation of MAPs and cost-effective drug production.
Endophytes, the Greek word having “endon” (within) and “phyton” (plant), was coined by De Bary (1879) for “any organism occurring within plant tissues”. Bacon and White (2000) have defined endophyte in the broadest and widely accepted manner that states that endophytes include those microbes colonizing living plant internal tissues and not causing any instant, obvious ill effects. Thus, most precisely, endophytes refer to microorganisms (fungi, bacteria, actinomycetes, etc.) that spend at least a part of their life cycle establishing a relationship with a plant that remains asymptomatic (Vanessa and Christopher, 2004). Microorganisms that require living cells to grow and complete their life cycle are known as “obligate” while the others that mainly thrive on the outside of the plant tissues are termed as “epiphytes” and sometimes may enter the plant endosphere, called “opportunistic” (Hardoim et al., 2008). In this interaction, both plant and endophytic microbes live together, providing profound benefits to each other (Ting et al., 2009). These endophytes are often rhizospheric in nature, and preferable sites for their attachment and subsequent entry into the host plant could be apical root zone with thin-walled surface root layers and basal root zones with small cracks (Gagné, 1987). They proliferate in the entire host plant (Hallmann et al., 1997a), and reside within the cells, vascular tissues, or intercellular spaces (Patriquin and Dobereiner, 1978; Jacobs et al., 1985; Bell et al., 1995). Endophytic microbes could also enter through the stomata and vertically transmit from parent to offspring via seeds while roots have maximum colonization through epidermis formed by lateral root emergence (Roos and Hattingh, 1983; Agrawal and Shende, 1987). Which community of microbes are friends and which are foes? It is decided by the immune system of the plant itself (Zeilinger et al., 2016). The “balanced antagonism” with asymptomatic colonization between the host plant and endophytic microbes clearly shows that endophytes can live within the plant without activating any host defense mechanism and improves its self-sustenance through the production of the plant-like substances (Schulz et al., 1999; Schulz and Boyle, 2005).
The study of plant–microbe interactions helps us acknowledge natural events that influence our daily lives and could benefit befalling in sustainable resources, a smaller influence on the atmosphere and surroundings, and control of environmental pollution. The benefits of using these interactions for biotechnological applications are huge. The utilization of the pre-existing plant–microbe interactions for the promotion of growth of the plant and biocontrol diminishes the use of unnatural synthetic pesticides and fertilizers, resulting in lowering input costs and, more importantly, reducing the influence of chemical nutrients and pesticides on existing useful fiora and fauna (Boddey et al., 2003; Elmer and Reglinski, 2006; Whipps and Gerhardson, 2007). The production of beneficial compounds of industrial and pharmaceutical importance through plant–microbe symbiosis reduces the requirement to supply expensive catalysts and precursors and is energy-saving (Wu et al., 2007; Del Giudice et al., 2008). Remediation by traditional methods is costly and laborious; however, plant–microbe remediation approaches are incredibly efficient and less interfering (Anderson et al., 1993). The carbon sequestration by the plant–rhizosphere methods is probably a sustainable approach for reducing atmospheric carbon (Wu et al., 2009).
As the name implies, endophytic microorganisms live within a plant in the intercellular spaces of various plant parts such as stems, roots, petioles, leaves, etc., without imposing any apparent symptoms of disease or ill health. The symbiotic relationship of the host and its endophytes has been studied in detail and is explained as the plant partner protecting and feeding the endophytes, which, “in return”, produce certain substances with bioactive capabilities (antiviral, plant growth promotion, antibacterial, antifungal, insecticidal, etc.) to augment the growth and competitiveness of the former under natural conditions. These endophytic entities defend their host plants from pathogens by secreting bioactive secondary metabolites under unfavorable environments (Azevedo et al., 2000; Strobel, 2003). The endophytic organisms are now recognized as a vital part of biodiversity, the allocation of endophytic microfiora varies with the difference according to its host. Zhang et al. (2006a) suggested that almost all of the vascular plants are known to harbor endophytic entities, especially those with medicinal values that are thought to be related to the formation of therapeutic products. Endophytic microbes are not entirely explored yet, but several investigations present them as an enormous therapeutic compound source. Worldwide, about 300,000 plants grown in an unexplored region are a host of at least one or more endophytic microbes (Araújo et al., 2001). Therefore, functionally diverse endophytes’ occurrence offers a key role in ecosystems at the most plentiful biodiversity (Strobel, 2003). Survival of the endophytic microbes inside the host cell can be for a long duration. The ability of these microbes to produce bioactive secondary metabolites makes them interesting candidates to be studied and exploited in biotechnological aspects (Casella et al., 2013) to add on to the existing wealth of secondary metabolites. Such interest has been reflected in a number of recent reviews showcasing the secondary metabolite-producing abilities of these wonder microbes (Müller, 2015). The growth and yield of several medicinal plants have been reported to be enhanced by many endophytes (Barnawal et al., 2012; Barnawal et al., 2014), especially by gene expression modulation regulated in important secondary metabolites’ biosynthesis. They have also been reported to impart tolerance to the plants against a range of biotic and abiotic stresses (Pandey et al., 2016a; Pandey et al., 2016b; Lata et al., 2018).
Chemical fertilizers’ indiscriminate use for boosting the productivity of crops is drastically destroying soil and environmental health (Tilman, 1998). Even then, the use of fertilizers is likely to increase further in agriculture to feed the ever-growing huge population (Vitousek et al., 1997; Frink et al., 1999). In the current scenario of global climate change, sustainable agriculture production proves to be a significant challenge. Some approaches such as the integration of microbes associated with plants in agriculture enhance the growth of the plant through different modes and alleviate several biotic and abiotic perturbations (Tanaka et al., 2005; Vega et al., 2008; Lata et al., 2018), and may serve as rescue practices under such situations.
Plants exhibit a huge and varied niche for microorganisms inside the planta resulting in a closer association between them. Endophytes inhabit the internal plant tissue comprising different bacterial and fungal species that collectively form the “plant endomicrobiome” and can trigger various physiological responses in the plant. Colonization and benefits associated with endophytic microorganisms in plants are presented in Figure 1.
Bacterial endophytes, sometimes considered a part of the population of rhizospheric microbes (Marquez-Santacruz et al., 2010), are present in various tissues such as the stem, root, leaf, tuber, and fruit, of different agricultural, horticultural, and forest species. As the endophytic bacteria are directly in contact with the plant tissues, they have the benefit of greater advantage than rhizospheric bacteria from the plants and offer more benefits to the plant other than bacteria in the rhizospheric region and outside the plants (Araujo et al., 2002). The wide-ranging and recurrent occurrence of such endophytic bacterial populations indicates that healthy plants recruit more endophytic populations (Hardoim et al., 2015) and provide these organisms with a huge and somewhat uncharted ecological niche.
The colonization of host-associated microbes occurs horizontally from the surroundings and might be vertical via parent to the progenies (Santoyo et al., 2016). Endophytic bacteria have several ways to enter inside the host plant tissues. Except for those microbes that are already established inside the seeds of the plant (Truyens et al., 2014), the most frequent point of entry of endophytic bacterial endophytes inside the host plant is via the cracks on the roots and wounded root tissues formed as a result of growth and development of the plant (Sprent and De Faria, 1989; Sørensen and Sessitsch, 2006). This allows leakage of plant metabolites, which attract more bacteria towards it (Hallmann et al., 1997a). Other points of entry of endophytes could be lenticels present in root and shoot periderm (Scott et al., 1996), radicles of germinating seeds, or lateral root hair cells. For example, Hallmann et al. (1997b) showed that Enterobacter asburiae JM22, an endophytic bacterium in cotton plants, produces enzymes capable of hydrolyzing cellulose in the cell wall, assisting the entry of the bacterium inside the host plant.
Autofluorescent proteins (AFP) could be an important method as well as a tool for the visualization of the biofilm to study plant–microbe interaction. These visualization techniques also include gene expression studies using GFP (green fluorescent proteins) in which the GFP gene is integrated into the chromosome of bacteria and a plasmid containing GFP cloned cells which are visualized by confocal or epifluorescence or laser scanning microscopy (Villacieros et al., 2003; Germaine et al., 2004). In the β-glucuronidase (GUS) reporter system, staining helps in the visualization of bacterial movement and gene expression in the rhizosphere and phyllosphere, and entrance and site of pathogens can be studied by IVET (in vivo expression technology) (Preston et al., 2001; Leveau and Lindow, 2001; Zhang et al., 2006a).
For the recruitment of the endophytes, the host plant establishes symbiosis with large soil microbial diversity. Initially, attachment of the endophytic fungi might occur on the surface of roots and form structures called appressorium (Yedidia et al., 1999). After that, these attachments penetrate the outer root system and internally colonize the plant tissues (Viterbo and Chet, 2006; Nogueira-Lopez et al., 2018). Endophytic fungi mostly use two types of diffusion patterns; in the primary mechanism, fungi are vertically transmitted into progeny seed from maternal plants by which the offspring gets infected (Gagic et al., 2018). The endophytic fungi transmission among the host plants and the offspring is brought about under appropriate environmental conditions when infected seeds germinate and the endophytic fungi present inside the seed enter the seedlings after the germination of seeds (Hodgson et al., 2014).
Generally, endophytic fungi initiate from the nutrient-rich atmosphere of the rhizosphere, which also has insects and animal feeding processes, and air floating fungal spores (Rodriguez et al., 2009; Sasse et al., 2018). Many of the endophytic fungal microbes are transmitted through spores or hyphal fragments horizontally in aboveground tissues, by insects or herbivores (biotic) or rain or wind (abiotic dispersion agents) from plant to plant, thus establishing communication of fungal endophytes between several plant hosts (Wiewiora et al., 2015).
Microscopic observations of tomato roots on early colonization by the endophytic fungus Trichoderma illustrated no disturbance in cell integrity during this process (Chacón et al., 2007). However, in cucumber roots colonized by endophytes, various phenomena such as enhanced chitinase activity, necrosis of the penetration peg, and fluorescent products’ formation in the intercellular spaces were observed. This phenomenon might be due to copious extracellular enzyme production by endophytic fungi (Suryanarayanan et al., 2012).
Not much information exists about the mechanisms of endophyte-mediated plant growth enhancement. Endophytes can mediate plant growth improvement both directly and indirectly (Figure 2). As rhizospheric bacterium initiates its development into endophytes, it is supposed that endophytes can maintain their characteristics inside the host plant.
Endophytic microbes help host plants directly in many ways to promote the plant’s growth by improving the uptake of essential nutrients, which ultimately increases overall crop yield (Muthukumarasamy et al., 2002). A typical example is nitrogen fixation by particular endophytes in leguminous crops (Stacey et al., 2006). Additionally, several researchers have stated that endophytic bacteria effectively associate with non-leguminous crops to form a synergistic association intended for nitrogen fixation (Bhattacharjee et al., 2008; Saravanan et al., 2008; Mattos et al., 2008; Govindarajan et al., 2008; Oliveira et al., 2009). Plant growth enhancement may occur via many approaches that include plant hormone production, like gibberellins, IAA (indole-3-acetic acid), ethylene, and cytokinin regulation and activity. Many endophytes have shown to exhibit an activity of enzyme ACC (1-aminocyclopropane-1-carboxylate) deaminase to modulate physiology by reducing ethylene content (Hardoim et al., 2008; Barnawal et al., 2012) since ethylene in plant growth inhibitive hormone. In previous studies, Burkholderia phytofirmans, a plant growth-promoting endophytic strain, has been proven to enhance the yields of numerous crops (Lazarovits and Nowak, 1997; Sharma and Nowak, 1998; Sessitsch et al., 2005; Barka et al., 2006). Similarly, Mattos et al. (2008) showed that Burkholderia kururiensis, an endophytic bacterium, enhances IAA hormone production. Penicillium citrinum strain generated a large amount of physiologically active gibberellins than Gibberlla fujikuroi (wild type), which offers to generate a biologically active source of gibberellic acid (GA3) (Khan et al., 2008). Various studies showed that a variety of fungal endophytes promoted height, biomass, and tiller number in numerous crops (Spiering et al., 2006; Zhang et al., 2007; Lowe et al., 2008). Stagonospora spp., a seed-borne endophytic fungus, increased the yield of Phragmites australis (Cav.) Trin. ex Steud. (Ernst et al., 2003), and endophytic fungus promoted growth in peppermint (Mucciarelli et al., 2003). Some examples of endophytic microbes’ associated benefits in medicinal and aromatic plants are presented in Table 1.
Plants muddle through various unfavorable environmental conditions or abiotic stresses like cold, drought, hyper-salty situations, or pathogenesis. Endophytic microbes help the plant conquer such perturbations through some indirect mechanisms, which also promote the buildup of secondary metabolites (including drugs or important medicinal components) in plants.
The endophytic microbes support the host plants to defeat the before-mentioned stresses by some indirect mechanisms. They are also effective in bioremediation by various means, like they decrease heavy metal stress (Zhang et al., 2012), eliminating harmful greenhouse gases (Stępniewska and Kuźniar, 2013), and restricting the development of pests on plants (Azevedo et al., 2000). Endophytic microbes also support phytoremediation by reducing metal phytotoxicity. Simultaneously, for plants containing endophytes with requisite metabolic capabilities and degradation pathways for reducing phytotoxicity and magnifying degradation, the plant–endophyte relationships can be equipped to remediate wastelands and groundwater (Weyens et al., 2009). Rajkumar et al. (2009) reported that endophytic bacteria assist in promoting the extraction of heavy metals. Endophytes are also effective in the degradation of polyaromatic hydrocarbon (PAH). Radwan (2009) documented the phytoremediation of oily soils using rhizobacteria, which appears to be a cheap and environmentally friendly method of cleaning the environment.
Endophytic microorganisms have the ability to enhance plant resistance systems against pathogen infestation through antagonistic activity (Miller et al., 2002; Gunatilaka, 2006). Many studies showed that endophytes have a crucial role in regulating the gene expression of the host, modulating physiological responses and plant defense-related pathways (Van Bael et al., 2012; Estrada et al., 2013; Salam et al., 2017). Khare et al. (2016) illustrated that jasmonic acid and salicylic acid could greatly contribute to plant stress responses against phytopathogens. Ren and Dai (2012) described that inoculation with Gilmaniella sp. AL12 induces jasmonic acid defense responses against pathogenic fungi in Atractylodes lancea (Thunb.) DC. The gibberellins produced by endophytes enhance insect and phytopathogens’ resistance via salicylic and jasmonic acid pathways (Waqas et al., 2015a). An endophyte, Fusarium solani, elicits induced systemic resistance against a fungal pathogen, Septoria lycopersici, by stimulating gene expression linked to the pathogenesis (Kavroulakis et al., 2007). Foliar endophytic fungi, Colletotrichum tropicale, inoculated in Theobroma cacao (L.) enhance tolerance to Phytophthora (Mejía et al., 2008). The endophytic bacteria produce several antimicrobial compounds that can increase the resistance of the plants to various phytopathogenic fungi, bacteria, nematodes, etc. Endophytic Pseudomonas putida BP25 associated with black pepper inhibits a range of phytopathogens, viz., Rhizoctonia solani, Phytophthora capsici, Gibberella moniliformis, Pythium myriotylum, Radopholus similis, and Colletotrichum gloeosporioides by the production of several compounds (Sheoran et al., 2015). Macrophomina phaseolina causes charcoal rot disease in different crops and has been reported to be restricted by siderophore-producing Rhizobium (Arora et al., 2001). An endophyte, Pseudomonas fluorescens, having antagonistic effects against Vertcillium was isolated by Mercado-Blanco et al. (2004) from olive plant roots. Yong et al. (1994) established the role of endophyte Fusarium spp. in the enhancement of growth and terpenoid content in Euphorbia pekinensis Rupr.
The endophytic fungus Phomopsis cassia associated with Cassia spectabilis enhances tolerance against phytopathogenic fungi Cadosporium sphaerospermum and C. cladosporioides by producing cadinane sesquiterpenoids, which are toxic to pathogens (Silva et al., 2006). Similarly, Wang et al. (2012) reported that an endophyte, Chaetomium globosum L18, inhibits pathogenic fungi by synthesizing some toxic chemicals in Curcuma wenyujin Y. H. Chen & C. Ling. In another study, Cao et al. (2009) identified endophytic microbes Choiromyces aboriginum, Stachybotrys elegans, and Cylindrocarpon associated produces cell wall-degrading enzymes to kill pathogenic fungi in Phragmites australis (Cav.) Trin. ex Steud. Furthermore, an endophytic fungus, Trichothecium roseum, has a toxic chemical, “trichothecin”, which enhances tolerance to pathogenic fungi in Maytenus hookeri Loes. (Zhang et al., 2010). Similarly, Bacillus subtilis and Myxormia sp. also enhance tolerance against pathogenic fungi Fusarium oxysporum and F. solani in Angelica sinensis (Oliv.) Diels (Yang et al., 2012).
Endophytic microbes Chaetomium cochliodes, Cladosporium cladosporioides, and Trichoderma viride enhance insect resistance in creeping thistle via producing some chemicals toxic to pathogens (Gange et al., 2012). Similarly, Sumarah et al. (2010) reported that fungal endophytes enhance resistance against Choristoneura fumiferana insect in red spruce (Picea rubens Sarg.). An endophyte, Leucocoprinus gongylophorus, increases insect resistance by synthesizing some chemicals antagonistic to ants’ fungal symbiont (Bittleston et al., 2011). Furthermore, the endophyte Chaetomium Ch1001 associated with cucumber enhances tolerance against root-knot nematode by producing abscisic acid affecting the motility of the second-stage juveniles of insects (Yan et al., 2011). According to the study undertaken by Gómez-Vidal et al. (2009), endophytes Beauveria bassiana, Lecanicillium dimorphum, and L. cf. Psalliotae increase insect resistance to Phoenix dactylifera by modulating the expression of cell division-related proteins in the host plant. Strobel et al. (1999) reported that in thunder god vine (Triptergyium wilfordii Hook. f.), an endophye, Cryptosporiopsis cf. quercina, produces “cryptocin” and “cryptocandin” toxic to pathogenic fungi Pyricularia oryzae in host plant. Daungfu et al. (2019) recently identified several endophytes, Bacillus subtilis LE24, Bacillus amyloliquefaciens LE109, and Bacillus tequilensis PO80, isolated from citrus plant having antagonistic effects against pathogens, which might be helpful in the biocontrol of pathogens. All these findings strongly confirm that the presence of endophytes in the host has the potential to increase their tolerance to pathogens through several mechanisms. Conversely, in resistance stimulation to disease facilitation, the process wherein endophytes invade plant tissues affects endophyte–pathogen interactions, perhaps producing facilitation (positive induction of pathogens), negatively strengthening host resistance, or having no effect at all (Suryanarayanan et al., 2009; Schmidt et al., 2014; Adame-Alvarez et al., 2014). These require further research for confirmation.
Abiotic stressful conditions, viz., drought, water-logging, salinity, cold, heat, and heavy metal toxicity, may cause adverse effects on soil and environmental health (Wang et al., 2003; Khare and Arora, 2015). Endophytes have a vital function in increasing tolerance against abiotic perturbations in plants (Wani et al., 2016). Waqas et al. (2012) reported that stomatal closure mediated by abscisic acid (ABA) might have a key function in the regulation of plant growth by reducing other abiotic stresses, including osmotic stress.
Under drought stress, an endophytic bacterium, Sinorhizobium meliloti, upregulated FeSOD and CU/ZnSOD, promoting drought tolerance in Medicago sativa (L.) (Naya et al., 2007). Similarly, Arbuseular mycorrhiza improves nutrient absorption and modifies Salvia’s metabolic activities to boost drought tolerance (Meng and He, 2011). An endophytic microbe, Trichoderma hamatum DIS 219b, delayed drought-induced alterations in stomatal conductance and net photosynthesis, promoting drought tolerance in Theobroma cacao (L.) (Bae et al., 2009). According to Sziderics et al. (2007), a fungal endophyte, Piriformospora indica, enhances osmotic stress tolerance via encoding enzyme ACC oxidase and lipid transfer protein. Wang et al. (2009) reported that Arbuseular mycorrhiza and Penicillium griseofulvum reduce injury of water stress by increasing the activity of protective enzymes and osmotica contents, thereby enhancing tolerance against salt and drought stress in Glycyrrhiza uralensis. Liu et al. (2011) reported that soluble protein concentration and peroxidase activity (POD) are altered by Chaetomium globosum and Botrytis sp. Under salt stress in Chrysanthemum morifolium (Ramat.) Hemsl. A fungal endophyte, Yarrowia lipolytica, promotes salinity stress tolerance in Euphorbia milii Des Moul. (Jan et al., 2019).
Moreover, ABA-mediated signaling pathways and their biosynthesis during salinity stress are altered by several microbes in the plant endosphere and may help plant growth. B. phytofirmans (PsJN) altered gene expression for a cell surface signaling element, which passes signals to bacteria about the alteration in environmental conditions and consequently improves their metabolism (Sheibani-Tezerji et al., 2015). Fernandez et al. (2012) reported in PsJN bacterized grapevine that stress-induced gene expression in addition to metabolite levels improved over control at a lower temperature by balancing carbohydrate metabolism. Recently, de Zélicourt et al. (2018) reported that Enterobacter sp. (SA187), an endophyte, colonizes Arabidopsis root and shoot tissues and stimulates tolerance against salt stress by synthesizing KMBA (2-keto-4-methylthiobutyric acid). Márquez et al. (2007) reported that at high soil temperatures, Curvularia protuberate, an endophytic fungus, has been linked to Dichanthelium lanuginosum for its survival. Wan et al. (2012) reported that endophytes can also reduce the heavy metal-promoted oxidative injury. Earlier studies suggested endophytic bacteria’s function in enhancing tolerance to metal toxicity through diverse mechanisms such as sequestration, intracellular accumulation, and extracellular precipitation or alteration of toxic metal ions to a minimum or non-toxic form (Ma et al., 2016; Mishra et al., 2017). Luo et al. (2012) discovered that a bacterial endophyte, Bacillus sp. SLS18, reduces heavy metal toxicity via root tillers and biomass accumulation in Solanum nigrum (L.) and Phytolacca acinosa Roxb. Recently, Domka et al. (2019) identified an endophytic fungus, Mucor sp., which enhances metal toxicity tolerance in Arabidopsis arinosa (L.) Lawalrée. Some examples of endophytes enhancing the tolerance of crop plants against abiotic and biotic stresses are presented in Table 2.
Table 2 Host medicinal plants with enhanced defense responses conferred by endophytic microorganisms.
The diversity of bioactive compounds varies according to the habitats of the host plants; for example, in a tropical rain forest, limited resource availability leads to great competition among plants and their endophytes; thus, selection pressure is at the peak, resulting in the production of many novel molecules as compared to temperate forests (Reddell and Gordon, 2000). Isolation of indigenous microbes from different plant parts and their interaction with the host plant may divulge applicant microbes to promote plant growth and as biocontrol agents. Such microbial inoculants have the potential to provide resistance against environmental perturbations without affecting the indigenous microbial equilibrium (Palaniyandi et al., 2013).
Many endophytic microbes can synthesize a notable variety of secondary metabolites like antioxidant, anticancer, immunosuppressive, antidiabetic, antioomycete, antifungal, antibacterial, antiviral, and nematicidal agents (Gunatilaka, 2006; Zhang et al., 2006b; Verma et al., 2009; Aly et al., 2011). Shimizu (2011) reported that endophytic actinobacteria synthesize antibiotics, which is useful in plant growth promotion and improves the tolerance of plants to stress. Endophytes also have been in the limelight during the last decade or so because of their capability in producing several secondary metabolites that are bioactive (Tan and Zou, 2001; Schulz et al., 2002; Strobel, 2003; Prado et al., 2013). These compounds extracted from endophytes belong to the various chemical groups like xanthones, terpenoids, phenols, steroids, benzopyranones, isocoumarins, chinones, cytochalasins, tetralones, and enniatines (Schulz et al., 2002). Sometimes, these endophytic microbes may cause variation in well-known structural compounds like fungal steroids, ergosterol, or plant hormone indole-3-acetic acid (Lu et al., 2000). Several endophytes can also be attributed to providing protection of plants against pests because of the compounds occurring in them (Poling et al., 2008).
There are many reports that have shown that host secondary metabolism can be induced by endophytes, but such interaction has not been much explored. Qawasmeh et al. (2012) reported that when endophytes (from the Clavicipitaceae family) interact with grasses, there is a production of phenolic compounds, which are mainly defense-related. However, secondary metabolites could remain unchanged or get reduced depending on which type of endophyte interacts with the host plant. A well-known example of a high-demanding anticancerous molecule is “taxol” isolated from Taxomyces andreanae, a taxol-producing fungal endophyte of Taxus species (Stierle et al., 1995). An example showed that bacterial endophytes have methanol dehydrogenase genes, which were known to express furanone biosynthesis and localized especially in vascular tissues of strawberry receptacles and plant achenes cells (Nasopoulou et al., 2014). Likewise, Koskimaki et al. (2009) have reported that fungal endophyte Paraphaeosphaeria sp. increased the accumulation and biosynthesis of flavan-3-ols phenolic acids and oligomeric proanthocyanidins in Vaccinium myrtillus. In Artemisia annua (L.), an endophytic bacterium, Pseudonocardia sp., has been reported to increase artemisinin synthesis by upregulating the cytochrome P450 monooxygenase (CYP71AVI) and cytochrome P450 oxidoreductase (CPR) genes, and this also activated a defense mechanism (Wang et al., 2006). It has also been found that endophytes may act as upregulators of the specific gene expression for tissue-specific roles, e.g., foliar endophytes enhanced primary metabolites, crop yields could be increased by root endophytes, and endophytes isolated from the capsule could upregulate key genes of benzylisoquinoline alkaloid (BIA) biosynthesis in Papaver somniferum (L.) (Pandey et al., 2016a). Tiwari et al. (2010) found an improved content and yield of essential oils in holy basil. In the Chinese medicinal plant Atractylodes lancea (Thunb.) DC., the endophytic bacterium P. fluorescens could enhance the generation of ROS (reactive oxygen species), which resulted in an increase of sesquiterpenoids (Zhou et al., 2015). Endophytic bacteria Aranicola proteolyticus, Bacillus cereus, B. thuringiensis, B. licheniformi, and Serratia liquefaciens recovered from Pinellia ternata (Thunb.) Makino could produce inosine and guanosine alkaloids similar to their host plant in fermentation media (Liu et al., 2015). Tiwari et al. (2013) found that endophyte Micrococcus sp. and Staphylococcus sciuri inoculated plant had significantly higher amounts of ajmalicine, serpentine, and vindoline in Catharanthus roseus (L.) G. Don. Kumar et al. (2014) reported that the endophytic bacterium Azotobactor chroococcum could enhance the yield of curcumin in rhizomes of turmeric.
Endophytic microflora encompasses a high potential for the synthesis of an ample range of unidentified, undepicted novel secondary metabolites within or without host plants. It is needed to identify which type of mechanism or cryptic genes and what circumstances for the evolution of the genome are involved in the synthesis of novel compounds in endophytes as well as in-planta. Some examples of endophytes producing secondary metabolites in host plants are presented in Table 3.
Phytoremediation is the most efficient and eco-friendly system for restoring natural soil conditions when various environmental pollutants have contaminated it. In the past two decades, endophytes’ usage in the phytoremediation of diverse environmental pollutants has received more attention (Sheng et al., 2008; McGuinness and Dowling, 2009; Weyens et al., 2009; Weyens et al., 2010; Hur et al., 2011; Segura and Ramos, 2013; Wei et al., 2014; Anyasi and Atagana, 2018). Such research has shown the potential of plant–microbe interaction in the restoration of polluted regions and may be helpful in designing efficient environmental pollutant removal systems. One of the reasons could be attributed to the complicated relationship between endophytes and their hosts. In contrast, the other reason has to do with the fact that it is practically impossible to comprehend the mechanisms of existence and situations of endophytes to be able to replicate them. It is impractical to exaggerate the role of endophytes in both management and harnessing the natural environment. Unfortunately, the holistic view of these taxa has been constrained by them. Such constraints include the fact that the process of isolation of endophyte relies on culture dependence, while several microorganisms exist that cannot be cultured. This emphasizes nonculture-dependent innovation as the barrier to understanding endophytes.
The most frequently used inoculation technique in agriculture includes the use of endophytes; culture, facilitated by a carrier, is combined with the synthetically manufactured sticky seeds and sown. Moreover, several commercial formulations include liquid cultures directly applied or with the granular fertilizer or seed applications. Other techniques have also been used, including seed priming, seed coating, foliar spraying, root dipping, pelleting, and direct soil application (O’Callaghan, 2016). Given the presence of endophytic microbes, seed inoculation can accomplish this goal. Still, its effectiveness is constrained by the lengthy engaging period, subsequent physical abrasion, and competition with other soil microbes. Root dipping or seedlings treated with microbial suspension are also susceptible to contamination and handling issues. The application of endophytes in bulk populations is made possible by pelleting or direct soil application. However, there is still an issue with the lack of homogeneity in the field and exposure to environmental perturbations. Despite using every conventional method, the microbes still need to endure for a few weeks before they may enter the plant after root hair emergence. Even while some endophytes’ facultative character suggests the prospect of further colonization if they can endure in the rhizosphere, the ecological constraint exists. Seed priming with a predetermined duration can help the microbe’s entrance during imbibitions. However, several scientific and technical difficulties are still associated with applying endophytes to seeds via coatings, sprays, granules, and capsules. Moreover, a seemingly simple procedure like plate counting also seems to have some technical problems, e.g., the “viable but not culturable” bacteria may not be detected by plate counting.
The formulation should facilitate the microorganism’s penetration and colonization of the host while minimizing dosage and cost. It should also increase microbe establishment in the soil and close to or on the plant. The application of endophytes requires a thorough knowledge of the physicochemical and biological environment, including the phyllosphere, soil, seed surface, and rhizosphere, as well as the cultivation and formulation of the biologicals to prolong shelf life. In the context of mycorrhiza, progress has been made in understanding the molecular plant–microbe interaction that needs to be integrated into novel formulation and application strategies. In other circumstances, such endophytic entomopathogenic fungi do not clearly understand how they invade and colonize. Basic research studies only use straightforward water-spore mixtures instead of more advanced and practical application methods.
Furthermore, designing formulations having high microbial inoculant concentration and survivability during storage is crucial for developing potent inoculants. Since it is impossible to test out every potential combination of parameters throughout the formulation process, it is challenging to determine the most critical variables. Nevertheless, it is challenging to maintain sterility throughout the formulation process for an extended period, which could lead to contamination. Even though we can find entomopathogenic fungi in nature in this form, their poor recovery rate from plants reveals that these organisms do not occur naturally.
Plant–microbe interactions benefit the all-embracing vicinity of agricultural applications. Microorganisms are abundantly present in nature and primarily colonized in-planta. Plant–endophyte interaction is mostly considered beneficial, having profound effects on the physiology of the host plant and the overall performance by promoting growth, development, and imparting fitness to the host plants against different biotic and abiotic stresses. They play a vital function in agricultural sustainability by providing eco-friendly inputs to enhance crop productivity and quality while minimizing harmful chemical fertilizers. The study of these plant–microbe interactions helps us acknowledge natural events that influence our daily lives and could benefit befalling in sustainable resources, a smaller influence on the atmosphere and surroundings, and control of environmental pollution. The benefits of using these interactions for biotechnological applications are huge. The utilization of the pre-existing plant–microbe interactions for the promotion of growth of the plant and biocontrol diminishes the use of unnatural synthetic pesticides and fertilizers, resulting in lowering input costs and, more importantly, reducing the influence of chemical nutrients and pesticides on existing useful fiora and fauna. Moreover, the production of beneficial compounds of industrial and pharmaceutical importance through plant–microbe symbiosis reduces the requirement to supply expensive catalysts and precursors and is energy-saving. In recent years, MAPs are being paid considerable attention worldwide due to their vast economic potential, primarily in the field of herbal medicine. Until the arrival of advanced medicines, an oversized population in emerging nations has traditionally relied upon the products obtained from plants. Furthermore, about 12.5% of the more than 422,000 plant species have been universally documented for medicinal properties; however, only a couple of hundreds are known to be in cultivation. There is a need to grow MAPs to maintain their steady supply and conservation amidst decreasing stocks from natural sources and rising global interest.
Endophytic microorganisms are tissue specific in nature; their establishment and functionality within the host are affected by several factors such as tissue type, host’s genotype, and surrounding conditions. The lack of knowledge about the widespread presence of endophytic microorganisms’ communities in plant tissues has been a hindrance in advancing research on endophytes in various fields. It should be noted that the development of successful endophyte application technologies would fully depend on improving our understanding of how they enter and colonize in-planta. Consequently, to guarantee reproducibility, reliable methods of endophytic inoculum delivery should be developed for better productivity of MAPs. Leveraging the relationship between plants and endophytes can be crucial for advancing sustainable development (Ryan et al., 2008); extensive research investigations are required to accept or refute this hypothesis. Therefore, in-depth future studies are needed to demonstrate an improved comprehension of the organism in its host to advance the viability of endophyte-assisted biological applications, especially in the field. The persistent reliance on the deployment of a generic method in their processing was inferred to be a hindrance to the capability to fully grasp the interaction between endophytes and their host concerning their utilization in biological activities. Since most organisms prefer to eschew them due to transformations, they cannot be recognized using those general techniques. Thus, using complex molecular processes in their processing will lead to a better understanding and enable the use of the endophytic application in agriculture/food processing, medicine, and environmental management.
AK and AT conceived and planned this review article. AT and PP wrote the original draft of the manuscript. ST helped in review and data collection. PP prepared the figures. All authors reviewed and agreed on the final version.
We are thankful to the Director of CSIR-Central Institute of Medicinal and Aromatic Plants for providing the necessary facilities to carry out the research work. AT is grateful to the Department of Science and Technology, India for the INSPIRE fellowship.
The authors declare that the research was conducted in the absence of any commercial or financial relationships that could be construed as a potential conflict of interest.
All claims expressed in this article are solely those of the authors and do not necessarily represent those of their affiliated organizations, or those of the publisher, the editors and the reviewers. Any product that may be evaluated in this article, or claim that may be made by its manufacturer, is not guaranteed or endorsed by the publisher.
Abd Allah, E. F., Hashem, A., Alqarawi, A. A., Bahkali, A. H., Alwhibi, M. S. (2015). Enhancing growth performance and systemic acquired resistance of medicinal plant sesbania sesban (L.) merr using arbuscular mycorrhizal fungi under salt stress. Saudi J. Biol. Sci. 22 (3), 274–283. doi: 10.1016/j.sjbs.2015.03.004
Adame-Alvarez, R. M., Mendiola-Soto, J., Heil, M. (2014). Order of arrival shifts endophyte-pathogen interactions in bean from resistance induction to disease facilitation. FEMS Microbiol. Lett. 355, 100–107. doi: 10.1111/1574-6968.12454
Agarwal, S., Shende, S. T. (1987). Tetrazolium reducing microorganisms inside the root of brassica species. Curr. Sci. 56, 187–188. Available at: https://www.currentscience.ac.in/Volumes/56/04/0187.pdf
Ahmad, N., Hamayun, M., Khan, S. A., Khan, A. L., Lee, I. J., Shin, D. H. (2010). Gibberellin-producing endophytic fungi isolated from monochoria vaginalis. J. Microbiol. Biotech. 20 (12), 1744–1749. Available at: https://koreascience.kr/article/JAKO201018860405953.page
Ali, S., Charles, T. C., Glick, B. R. (2014). Amelioration of high salinity stress damage by plant growth-promoting bacterial endophytes that contain ACC deaminase. Plant Physiol. Biochem. 80, 160–167. doi: 10.1016/j.plaphy.2014.04.003
Ali, S., Khan, S. A., Hamayun, M., Iqbal, A., Khan, A. L., Hussain, A., et al. (2019). Endophytic fungi from caralluma acutangula can secrete plant growth promoting enzymes. Fresenius Environ. Bull. 28, 2688–2696. Available at: https://www.cabdirect.org/cabdirect/abstract/20193515732
Alishahi, F., Alikhani, H. A., Khoshkholgh-Sima, N. A., Etesami, H. (2020). Mining the roots of various species of the halophyte suaeda for halotolerant nitrogen-fixing endophytic bacteria with the potential for promoting plant growth. int. J. Microbiol. 23, 415–427. doi: 10.1007/s10123-019-00115-y
Aly, A. H., Debbab, A., Proksch, P. (2011). Fungal endophytes: unique plant inhabitants with great promises. Appl. Microbiol. Biotechnol. 90 (6), 1829–1845. doi: 10.1007/s00253-011-3270-y
Ambrosini, A., Beneduzi, A., Stefanski, T., Pinheiro, F. G., Vargas, L. K., Passaglia, L. M. (2012). Screening of plant growth promoting rhizobacteria isolated from sunflower (Helianthus annuus l.). Plant Soil 356 (1-2), 245–264. doi: 10.1007/s11104-011-1079-1
Amna, T., Puri, S. C., Verma, V., Sharma, J. P., Khajuria, R. K., Musarrat, J., et al. (2006). Bioreactor studies on the endophytic fungus entrophospora infrequens for the production of an anticancer alkaloid camptothecin. Can. J. Microbiol. 52 (3), 189–196. doi: 10.1139/w05-122
Anderson, T. A., Guthrie, E. A., Walton, B. T. (1993). Bioremediation in the rhizosphere. Environ. Sci. Technol. 27 (13), 2630–2636. doi: 10.1021/es00049a001
Anyasi, R. O., Atagana, H. I. (2018). Profiling of plants at petroleum contaminated site for phytoremediation. Int. J. Phytorem. 20, 352–361. doi: 10.1080/15226514.2017.1393386
Araújo, W. L., Maccheroni, W. C., Aguilar-Vildoso, C. I., Barroso, P. A., Saridakis, H. O., Azevedo, J. L. (2001). Variability and interactions between endophytic bacteria and fungi isolated from leaf tissues of citrus rootstocks. Can. J. Microbiol. 47 (3), 229–236. doi: 10.1139/w00-146
Araujo, W. L., Marcon, J., Maccheroni, W., van Elsas, J. D., van Vuurde, J. W., Azevedo, J. L. (2002). Diversity of endophytic bacterial populations and their interaction with xylella fastidiosa in citrus plants. Appl. Environ. Microbiol. 68 (10), 4906–4914. doi: 10.1128/AEM.68.10.4906-4914.2002
Arora, N. K., Kang, S. C., Maheshwari, D. K. (2001). Isolation of siderophore-producing strains of rhizobium meliloti and their biocontrol potential against macrophomina phaseolina that causes charcoal rot of groundnut. Curr. Sci. 81, 673–677. Available at: https://www.currentscience.ac.in/Volumes/81/06/0673.pdf
Azevedo, J. L., Maccheroni, W., Jr., Pereira, J. O., de Araújo, W. L. (2000). Endophytic microorganisms: a review on insect control and recent advances on tropical plants. Electron. J. Biotechnol. 3 (1), 15–16. doi: 10.2225/vol3-issue1-fulltext-4
Babu, A. G., Shea, P. J., Sudhakar, D., Jung, I. B., Oh, B. T. (2015). Potential use of pseudomonas koreensis AGB-1 in association with miscanthus sinensis to remediate heavy metal (loid)-contaminated mining site soil. J. Environ. Manage. 151, 160–166. doi: 10.1016/j.jenvman.2014.12.045
Bacon, C. W., Glenn, A. E., Hinton, D. M. (2002). “Isolation, in planta detection and culture of endophytic bacteria and fungi,” in Manual of environmental microbiology, 2nd edn. Eds. Hurst, C. J., Crawford, R. L., McInerney, M. J., Knudsen, G. R., Stetzenbach, L. D. (Washington DC: ASM Press).
Bacon, C. W., White, J. (2000). Microbial endophytes (New York, Basel, Switzerland: CRC Press). doi: 10.1201/9781482277302
Badri, D. V., Vivanco, J. M. (2009). Regulation and function of root exudates. Plant Cell Environ. 32, 666–681. doi: 10.1111/j.1365-3040.2009.01926.x
Badri, D. V., Weir, T. L., van der Lelie, D., Vivanco, J. M. (2009). Rhizosphere chemical dialogues: plant–microbe interactions. Curr. Opin. Biotechnol. 20 (6), 642–650. doi: 10.1016/j.copbio.2009.09.014
Bae, H., Sicher, R. C., Kim, M. S., Kim, S. H., Strem, M. D., Melnick, R. L., et al. (2009). The beneficial endophyte trichoderma hamatum isolate DIS 219b promotes growth and delays the onset of the drought response in theobroma cacao. J. Exp. Bot. 60 (11), 3279–3295. doi: 10.1093/jxb/erp165
Barazani, O., von Dahl, C. C., Baldwin, I. T. (2007). Sebacina vermifera promotes the growth and fitness of nicotiana attenuata by inhibiting ethylene signaling. Plant Physiol. 144 (2), 1223–1232. doi: 10.1104/pp.107.097543
Barka, E. A., Nowak, J., Clément, C. (2006). Enhancement of chilling resistance of inoculated grapevine plantlets with a plant growth-promoting rhizobacterium, burkholderia phytofirmans strain PsJN. Appl. Environ. Microbiol. 72 (11), 7246–7252. doi: 10.1128/AEM.01047-06
Barnawal, D., Bharti, N., Maji, D., Chanotiya, C. S., Kalra, A. (2012). 1-Aminocyclopropane-1-carboxylic acid (ACC) deaminase-containing rhizobacteria protect ocimum sanctum plants during waterlogging stress via reduced ethylene generation. Plant Physiol. Biochem. 58, 227–235. doi: 10.1016/j.plaphy.2012.07.008
Barnawal, D., Bharti, N., Maji, D., Chanotiya, C. S., Kalra, A. (2014). ACC deaminase-containing arthrobacter protophormiae induces NaCl stress tolerance through reduced ACC oxidase activity and ethylene production resulting in improved nodulation and mycorrhization in pisum sativum. J. Plant Physiol. 171 (11), 884–894. doi: 10.1016/j.jplph.2014.03.007
Bell, C. R., Dickie, G. A., Harvey, W. L. G., Chan, J. W. Y. F. (1995). Endophytic bacteria in grapevine. Can. J. Microbiol. 41 (1), 46–53. doi: 10.1139/m95-006
Bernardi, D. I., das Chagas, F. O., Monteiro, A. F., dos Santos, G. F., de Souza Berlinck, R. G. (2019). “Secondary metabolites of endophytic actinomycetes: Isolation, synthesis, biosynthesis, and biological activities,” in Progress in the chemistry of organic natural products, vol. 108. (Springer Nature Switzerland AG: Springer, Cham), 207–296. doi: 10.1007/978-3-030-01099-7_3
Bhattacharjee, R. B., Singh, A., Mukhopadhyay, S. N. (2008). Use of nitrogen-fixing bacteria as biofertiliser for non-legumes: prospects and challenges. Appl. Microbiol. Biotechnol. 80 (2), 199–209. doi: 10.1007/s00253-008-1567-2
Bhattacharya, D., Medlin, A. L. (1998). Algal phylogeny and the origin of land plants. Plant Physiol. 116 (1), 9–15. doi: 10.1104/pp.116.1.9
Bilal, L., Asaf, S., Hamayun, M., Gul, H., Iqbal, A., Ullah, I., et al. (2018). Plant growth promoting endophytic fungi asprgillus fumigatus TS1 and fusarium proliferatum BRL1 produce gibberellins and regulates plant endogenous hormones. Symbiosis 76 (2), 117–127. doi: 10.1007/s13199-018-0545-4
Bittleston, L. S., Brockmann, F., Wcislo, W., Van Bael, S. A. (2011). Endophytic fungi reduce leaf-cutting ant damage to seedlings. Biol. Lett. 7 (1), 30–32. doi: 10.1098/rsbl.2010.0456
Boddey, R. M., Urquiaga, S., Alves, B. J., Reis, V. (2003). Endophytic nitrogen fixation in sugarcane: present knowledge and future applications. Plant Soil 252 (1), 139–149. doi: 10.1023/A:1024152126541
Cao, R., Liu, X., Gao, K., Mendgen, K., Kang, Z., Gao, J., et al. (2009). Mycoparasitism of endophytic fungi isolated from reed on soilborne phytopathogenic fungi and production of cell wall-degrading enzymes in vitro. Curr. Microbiol. 59 (6), 584–592. doi: 10.1007/s00284-009-9477-9
Cao, X., Li, J., Zhou, L. (2007). Determination of diosgenin content of the endophytic fungi from Paris polyphylla var. yunnanensis by using an optimum ELISA. Nat. Prod. Res. 19, 1020–1023. doi: 10.3969/j.issn.1001-6880.2007.06.025
Casella, T. M., Eparvier, V., Mandavid, H., Bendelac, A., Odonne, G., Dayan, L., et al. (2013). Antimicrobial and cytotoxic secondary metabolites from tropical leaf endophytes: Isolation of antibacterial agent pyrrocidine c from lewia infectoria SNB-GTC2402. Phytochemistry 96, 370–377. doi: 10.1016/j.phytochem.2013.10.004
Castillo, U. F., Strobel, G. A., Ford, E. J., Hess, W. M., Porter, H., Jensen, J. B., et al. (2002). Munumbicins, wide-spectrum antibiotics produced by streptomyces NRRL 30562, endophytic on kennedia nigriscansaaThe GenBank accession number for the sequence determined in this work is AY127079. Microbiology 148 (9), 2675–2685. doi: 10.1099/00221287-148-9-2675
Chacón, M. R., Rodríguez-Galán, O., Benítez, T., Sousa, S., Rey, M., Llobell, A., et al. (2007). Microscopic and transcriptome analyses of early colonization of tomato roots by trichoderma harzianum. Int. Microbiol. 10 (1), 19–27. doi: 10.2436/20.1501.01.4
Chakravarthi, B. V. S. K., Das, P., Surendranath, K., Karande, A. A., Jayabaskaran, C. (2008). Production of paclitaxel by fusarium solani isolated from taxus celebica. J. Biosci. 33 (2), 259–267. doi: 10.1007/s12038-008-0043-6
Chen, J. X., Dai, C. C., Li, X., Tian, L. S., Xie, H. (2008). Endophytic fungi screening from atracty lancea and inoculating into the host plantlet. Guihaia 28 (2), 256–260. doi: 10.3969/j.issn.1000-3142.2008.02.022
Chen, X. M., Guo, S. X. (2005). Effects of four species of endophytic fungi on the growth and polysaccharide and alkaloid contents of dendrobium nobile. Zhongguo Zhong Yao Za Zhi 30 (4), 253–257. doi: 10.3321/j.issn:1001-5302.2005.04.003
Chen, X. M., Guo, S. X., Wang, C. L. (2005). Effects of four endophytic fungi on the growth and polysaccharide content of anoectochilus roxburghii (Wall.) lindl. Chin. Pharm. J. 40 (1), 13–16. doi: 10.3321/j.issn:1001-2494.2005.01.006
Chen, X., Sang, X., Li, S., Zhang, S., Bai, L. (2010). Studies on a chlorogenic acid-producing endophytic fungi isolated from eucommia ulmoides oliver. j. Ind. microbiol. Biotechnol 37 (5), 447–454. doi: 10.1007/s10295-010-0690-0
Chen, B., Wang, M., Hu, Y., Lin, Z., Yu, R., Huang, L. (2011a). Preliminary study on promoting effects of endophytic fungi to growth of rehmannia glutinosa. Chin. J. Integr. Med. 36, 1137–1140. doi: 10.4268/cjcmm20110906
Chen, M., Yang, L., Li, Q., Shen, Y., Shao, A., Lin, S., et al. (2011b). Volatile metabolites analysis and molecular identification of endophytic fungi bn12 from cinnamomum camphora chvar. Borneol. Zhongguo Zhongyao Zazhi 36 (23), 3217–3221. doi: 10.4268/cjcmm20112301
Chithra, S., Jasim, B., Sachidanandan, P., Jyothis, M., Radhakrishnan, E. K. (2014). Piperine production by endophytic fungus colletotrichum gloeosporioides isolated from piper nigrum. Phytomedicine 21 (4), 534–540. doi: 10.1016/j.phymed.2013.10.020
Chutima, R., Dell, B., Vessabutr, S., Bussaban, B., Lumyong, S. (2011). Endophytic fungi from pecteilis susannae (L.) rafin (Orchidaceae), a threatened terrestrial orchid in Thailand. Mycorrhiza 21 (3), 221–229. doi: 10.1007/s00572-010-0327-1
Costacurta, A., Vanderleyden, J. (1995). Synthesis of phytohormones by plant-associated bacteria. Crit. Rev. Microbiol. 21 (1), 1–18. doi: 10.3109/10408419509113531
Cui, Y., Yi, D., Bai, X., Sun, B., Zhao, Y., Zhang, Y. (2012). Ginkgolide b produced endophytic fungus (Fusarium oxysporum) isolated from ginkgo biloba. Fitoterapia 83 (5), 913–920. doi: 10.1016/j.fitote.2012.04.009
Daungfu, O., Youpensuk, S., Lumyong, S. (2019). Endophytic bacteria isolated from citrus plants for biological control of citrus canker in lime plants. Trop. Life Sci. Res. 30 (1), 73. doi: 10.21315/tlsr2019.30.1.5
De Bary, A. (1879). “Die erscheinung der symbiose (Vol. 121),” in Strassburg: Verlag von Karl J (Strasbourg, France: Trübner). doi: 10.1515/9783111471839
Del Giudice, L., Massardo, D. R., Pontieri, P., Bertea, C. M., Mombello, D., Carata, E., et al. (2008). The microbial community of vetiver root and its involvement into essential oil biogenesis. Environ. Microbiol. 10 (10), 2824–2841. doi: 10.1111/j.1462-2920.2008.01703.x
Deng, B. W., Liu, K. H., Chen, W. Q., Ding, X. W., Xie, X. C. (2009). Fusarium solani, tax-3, a new endophytic taxol-producing fungus from taxusá chinensis. World J. Microbiol. Biotechnol. 25 (1), 139. doi: 10.1007/s11274-008-9876-2
de Zélicourt, A., Synek, L., Saad, M. M., Alzubaidy, H., Jalal, R., Xie, Y., et al. (2018). Ethylene induced plant stress tolerance by enterobacter sp. SA187 is mediated by 2-keto-4-methylthiobutyric acid production. PLoS. Genet. 14 (3), e1007273. doi: 10.1371/journal.pgen.1007273
Domka, A., Rozpądek, P., Ważny, R., Turnau, K. (2019). Mucor sp.–an endophyte of brassicaceae capable of surviving in toxic metal-rich sites. J. Basic Microbiol. 59 (1), 24–37. doi: 10.1002/jobm.201800406
Duan, L. I., Liwei, G., Hong, Y. (2009). Isolation and identification of producing endophytic fungi of berberine from the plant phellodendron amurense. J. Anhui. Agric. Sci. 37 (22), 10340–10341, 10350. doi: 10.3969/j.issn.0517-6611.2009.22.007
Elmer, P. A. G., Reglinski, T. (2006). Biosuppression of botrytis cinerea in grapes. Plant Pathol. 55 (2), 155–177. doi: 10.1111/j.1365-3059.2006.01348.x
Ernst, M., Mendgen, K. W., Wirsel, S. G. (2003). Endophytic fungal mutualists: seed-borne stagonospora spp. enhance reed biomass production in axenic microcosms. Mol. Plant Microbe Interact. 16 (7), 580–587. doi: 10.1094/MPMI.2003.16.7.580
Estrada, G. A., Baldani, V. L. D., de Oliveira, D. M., Urquiaga, S., Baldani, J. I. (2013). Selection of phosphate-solubilizing diazotrophic herbaspirillum and burkholderia strains and their effect on rice crop yield and nutrient uptake. Plant Soil 369 (1-2), 115–129. doi: 10.1007/s11104-012-1550-7
Fernandez, O., Theocharis, A., Bordiec, S., Feil, R., Jacquens, L., Clément, C., et al. (2012). Burkholderia phytofirmans PsJN acclimates grapevine to cold by modulating carbohydrate metabolism. Mol. Plant Microbe Interact. 25 (4), 496–504. doi: 10.1094/MPMI-09-11-0245
Frink, C. R., Waggoner, P. E., Ausubel, J. H. (1999). Nitrogen fertilizer: retrospect and prospect. Proc. Natl. Acad. Sci. U.S.A. 96 (4), 1175–1180. doi: 10.1073/pnas.96.4.1175
Gagic, M., Faville, M. J., Zhang, W., Forester, N. T., Rolston, M. P., Johnson, R. D., et al. (2018). Seed transmission of epichloë endophytes in lolium perenne is heavily influenced by host genetics. Front. Plant Sci. 9, 1580. doi: 10.3389/fpls.2018.01580
Gagné, S., Richard, C., Rousseau, H., Antoun, H. (1987). Xylem-residing bacteria in alfalfa roots. Can. J. Microbiol. 33 (11), 996–1000. doi: 10.1139/m87-175
Gangadevi, V., Murugan, M., Muthumary, J. (2008). Taxol determination from pestalotiopsis pauciseta, a fungal endophyte of a medicinal plant. Chin. J. Biotechnol. 24 (8), 1433–1438. doi: 10.1016/S1872-2075(08)60065-5
Gangadevi, V., Muthumary, J. (2009). Taxol production by pestalotiopsis terminaliae, an endophytic fungus of terminalia arjuna (arjun tree). biotechnol. Appl. Biochem. 52 (1), 9–15. doi: 10.1042/BA20070243
Gange, A. C., Eschen, R., Wearn, J. A., Thawer, A., Sutton, B. C. (2012). Differential effects of foliar endophytic fungi on insect herbivores attacking a herbaceous plant. Oecologia 168 (4), 1023–1031. doi: 10.1007/s00442-011-2151-5
Germaine, K., Keogh, E., Garcia-Cabellos, G., Borremans, B., van der Lelie, D., Barac, T., et al. (2004). Colonisation of poplar trees by GFP expressing bacterial endophytes. FEMS Microbiol. Ecol. 48 (1), 109–118. doi: 10.1016/j.femsec.2003.12.009
Glick, B. R. (2005). Modulation of plant ethylene levels by the bacterial enzyme ACC deaminase. FEMS Microbiol. Lett. 251 (1), 1–7. doi: 10.1016/j.femsle.2005.07.030
Gómez-Vidal, S., Salinas, J., Tena, M., Lopez-Llorca, L. V. (2009). Proteomic analysis of date palm (Phoenix dactylifera l.) responses to endophytic colonization by entomopathogenic fungi. Electrophoresis 30 (17), 2996–3005. doi: 10.1002/elps.200900192
Govindarajan, M., Balandreau, J., Kwon, S. W., Weon, H. Y., Lakshminarasimhan, C. (2008). Effects of the inoculation of burkholderia vietnamensis and related endophytic diazotrophic bacteria on grain yield of rice. Microb. Ecol. 55 (1), 21–37. doi: 10.1007/s00248-007-9247-9
Guan, S., Grabley, S., Groth, I., Lin, W., Christner, A., Guo, D., et al. (2005). Structure determination of germacrane-type sesquiterpene alcohols from an endophyte streptomyces griseus subsp. Magn. Reson. Chem. 43 (12), 1028–1031. doi: 10.1002/mrc.1710
Gunatilaka, A. L. (2006). Natural products from plant-associated microorganisms: distribution, structural diversity, bioactivity, and implications of their occurrence. J. Natural Prod. 69 (3), 509–526. doi: 10.1021/np058128n
Guo, S., Wang, Q. (2001). Character and action of good strain on stimulating seed germination of gastrodia elata. Jun Wu Xi Tong 20 (3), 408–412. doi: 10.3969/j.issn.1672-6472.2001.03.023
Guo, D. D., Xu, C. X., Quan, J. S., Song, C. K., Jin, H., Kim, D. D., et al. (2009). Synergistic anti-tumor activity of paclitaxel-incorporated conjugated linoleic acid-coupled poloxamer thermosensitive hydrogel in vitro and in vivo. Biomaterials 30 (27), 4777–4785. doi: 10.1016/j.biomaterials.2009.05.051
Hallmann, Q. A., Hallmann, J. (1997b). Bacterial endophytes in cotton: location and interaction with other plant-associated bacteria. Can. J. Microbiol. 43 (3), 254–259. doi: 10.1139/m97-035
Hallmann, J., Quadt-Hallmann, A., Mahaffee, W. F., Kloepper, J. W. (1997a). Bacterial endophytes in agricultural crops. Can. J. Microbiol. 43 (10), 895–914. doi: 10.1139/m97-131
Hardoim, P. R., Van Overbeek, L. S., Berg, G., Pirttilä, A. M., Compant, S., Campisano, A., et al. (2015). The hidden world within plants: ecological and evolutionary considerations for defining functioning of microbial endophytes. M. M. B. R. 79 (3), 293–320. doi: 10.1128/MMBR.00050-14
Hardoim, P. R., van Overbeek, L. S., van Elsas, J. D. (2008). Properties of bacterial endophytes and their proposed role in plant growth. Trends Microbiol. 16 (10), 463–471. doi: 10.1016/j.tim.2008.07.008
Hodgson, S., de Cates, C., Hodgson, J., Morley, N. J., Sutton, B. C., Gange, A. C. (2014). Vertical transmission of fungal endophytes is widespread in forbs. Ecol. Evol. 4 (8), 1199–1208. doi: 10.1002/ece3.953
Hur, M., Kim, Y., Song, H. R., Kim, J. M., Choi, Y. I., Yi, H. (2011). Effect of genetically modified poplars on soil microbial communities during the phytoremediation of waste mine tailings. Appl. Environ. Microbiol. 77, 7611–7619. doi: 10.1128/AEM.06102-11
Jacobs, M. J., Bugbee, W. M., Gabrielson, D. A. (1985). Enumeration, location, and characterization of endophytic bacteria within sugar beet roots. Can. J. Bot. 63 (7), 1262–1265. doi: 10.1139/b85-174
Jan, F. G., Hamayun, M., Hussain, A., Jan, G., Iqbal, A., Khan, A., et al. (2019). An endophytic isolate of the fungus yarrowia lipolytica produces metabolites that ameliorate the negative impact of salt stress on the physiology of maize. BMC Microbiol. 19 (1), 1–10. doi: 10.1186/s12866-018-1374-6
Ju, Z., Wang, J., Pan, S. L. (2009). Isolation and preliminary identification of the endophytic fungi which produce hupzine a from four species in hupziaceae and determination of huperzine a by HPLC. Fudan Univ. J. Med. Sci. 4, 017. doi: 10.3969/j.issn.1672-8467.2009.04.015
Kavroulakis, N., Ntougias, S., Zervakis, G. I., Ehaliotis, C., Haralampidis, K., Papadopoulou, K. K. (2007). Role of ethylene in the protection of tomato plants against soil-borne fungal pathogens conferred by an endophytic fusarium solani strain. J. Exp. Bot. 58 (14), 3853–3864. doi: 10.1093/jxb/erm230
Khan, S. A., Hamayun, M., Yoon, H., Kim, H. Y., Suh, S. J., Hwang, S. K., et al. (2008). Plant growth promotion and penicillium citrinum. BMC Microbiol. 8 (1), 1–10. doi: 10.1186/1471-2180-8-231
Khare, E., Arora, N. K. (2015). “Effects of soil environment on field efficacy of microbial inoculants,” in Plant microbes symbiosis: applied facets (New Delhi: Springer), (pp. 353–381). doi: 10.1007/978-81-322-2068-8_19
Khare, E., Kim, K., Lee, K. J. (2016). Rice OsPBL1 (ORYZA SATIVA ARABIDOPSIS PBS1-LIKE 1) enhanced defense of arabidopsis against pseudomonas syringae DC3000. Eur. J. Plant Pathol. 146 (4), 901–910. doi: 10.1007/s10658-016-0968-9
Koskimäki, J. J., Hokkanen, J., Jaakola, L., Suorsa, M., Tolonen, A., Mattila, S., et al. (2009). Flavonoid biosynthesis and degradation play a role in early defense responses of bilberry (Vaccinium myrtillus) against biotic stress. Eur. J. Plant Pathol. 125 (4), 629. doi: 10.1007/s10658-009-9511-6
Kour, A., Shawl, A. S., Rehman, S., Sultan, P., Qazi, P. H., Suden, P. (2008). Isolation and identification of an endophytic strain of fusarium oxysporum producing podophyllotoxin from juniperus recurva. World J. Microbiol. Biotechnol. 24 (7), 1115–1121. doi: 10.1007/s11274-007-9582-5
Kumaran, R. S., Muthumary, J., Hur, B. K. (2008). Taxol from phyllosticta citricarpa, a leaf spot fungus of the angiosperm citrus medica. J. Biosci. Bioeng. 106 (1), 103–106. doi: 10.1263/jbb.106.103
Kumaran, R. S., Muthumary, J., Kim, E. K., Hur, B. K. (2009). ). production of taxol from phyllosticta dioscoreae, a leaf spot fungus isolated from hibiscus rosa-sinensis. Biotechnol. Bioprocess Eng. 14 (1), 76–83. doi: 10.1007/s12257-008-0041-4
Kumar, A., Singh, R., Giri, D. D., Singh, P. K., Pandey, K. D. (2014). Effect of azotobacter chroococcum CL13 inoculation on growth and curcumin content of turmeric (Curcuma longa l.). Int. J. Curr. Microbiol. Appl. Sci. 3 (9), 275–283.
Kusari, S., Lamshöft, M., Zühlke, S., Spiteller, M. (2008). An endophytic fungus from hypericum perforatum that produces hypericin. J. Natural Prod. 71 (2), 159–162. doi: 10.1021/np070669k
Kusari, S., Zühlke, S., Spiteller, M. (2011). An endophytic fungus from Camptotheca acuminata that produces camptothecin and analogues. J. Nat. Prod. 72 (1), 2–7. doi: 10.1021/np800455b
Kushwaha, R. K., Singh, S., Pandey, S. S., Kalra, A., Babu, C. V. (2019a). Fungal endophytes attune withanolide biosynthesis in withania somnifera, prime to enhanced withanolide a content in leaves and roots. World J. Microbiol. Biotechnol. 35 (2), 20. doi: 10.1007/s11274-019-2593-1
Kushwaha, R. K., Singh, S., Pandey, S. S., Rao, D. V., Nagegowda, D. A., Kalra, A., et al. (2019b). Compatibility of inherent fungal endophytes of withania somnifera with trichoderma viride and its impact on plant growth and withanolide content. J. Plant Growth Regul. 38 (4), 1228–1242. doi: 10.1007/s00344-019-09928-7
Lata, R., Chowdhury, S., Gond, S. K., White, J. F., Jr (2018). Induction of abiotic stress tolerance in plants by endophytic microbes. Lett. Appl. Microbiol. 66 (4), 268–276. doi: 10.1111/lam.12855
Lazarovits, G., Nowak, J. (1997). Rhizobacteria for improvement of plant growth and establishment. HortSci 32 (2), 188–192. doi: 10.21273/HORTSCI.32.2.188
Leveau, J. H., Lindow, S. E. (2001). Appetite of an epiphyte: quantitative monitoring of bacterial sugar consumption in the phyllosphere. Proc. Natl. Acad. Sci. 98 (6), 3446–3453. doi: 10.1073/pnas.061629598
Li, C. (2007). Fermentation conditions of sinopodophyllum hexandrum endophytic fungus on production of podophyllotoxin. Food Ferment. Ind. 33 (9), 28.
Li, A. R., Guan, K. Y. (2007). Mycorrhizal and dark septate endophytic fungi of pedicularis species from northwest of yunnan province, China. Mycorrhiza 17 (2), 103–109. doi: 10.1007/s00572-006-0081-6
Limón, R. I., Peñas, E., Torino, M. I., Martínez-Villaluenga, C., Dueñas, M., Frias, J. (2015). Fermentation enhances the content of bioactive compounds in kidney bean extracts. Food Chem. 172, 343–352. doi: 10.1016/j.foodchem.2014.09.084
Liu, K., Ding, X., Deng, B., Chen, W. (2009). Isolation and characterization of endophytic taxol-producing fungi from taxus chinensis. J. Ind. Microbiol. Biotechnol. 36 (9), 1171. doi: 10.1007/s10295-009-0598-8
Liu, Y., Liu, W., Liang, Z. (2015). Endophytic bacteria from pinellia ternata, a new source of purine alkaloids and bacterial manure. Pharm. Biol. 53 (10), 1545–1548. doi: 10.3109/13880209.2015.1016580
Liu, X., Song, W., Zhang, K., Ye, Y. (2011). Effects of two kinds of endophytic fungi infection on water stress of seedlings of chrysanthemum morifolium. Acta Hortic. Sin. 38 (2), 335–342.
Li, W., Zhou, J., Lin, Z., Hu, Z. (2007). Study on fermentation condition for production of huperzine a from endophytic fungus 2F09P03B of huperzia serrata. Chin. Med. Biotechnol. 2 (4), 254–259.
Lodewyckx, C., Vangronsveld, J., Porteous, F., Moore, E. R., Taghavi, S., Mezgeay, M., et al. (2002). ). endophytic bacteria and their potential applications. Crit. Rev. Plant Sci. 21 (6), 583–606. doi: 10.1080/0735-260291044377
Lowe, K. F., Bowdler, T. M., Hume, D. E., Casey, N. D., Tapper, B. A. (2008). The effect of endophyte on the performance of irrigated perennial ryegrasses in subtropical Australia. Aust. J. Agric. Res. 59 (6), 567–577. doi: 10.1071/AR08019
Lu, L., He, J., Yu, X., Li, G., Zhang, X. (2006). Studies on isolation and identification of endophytic fungi strain SC13 from harmaceutical plant Sabina vulgaris ant. and metabolites. Xi Bei Nong Ye Xue Bao 15, 85–89. doi: 10.3969/j.issn.1004-1389.2006.05.021
Luo, S., Xu, T., Chen, L., Chen, J., Rao, C., Xiao, X., et al. (2012). Endophyte-assisted promotion of biomass production and metal-uptake of energy crop sweet sorghum by plant-growth-promoting endophyte bacillus sp. SLS18. Appl. Microbiol. Biotechnol. 93 (4), 1745–1753. doi: 10.1007/s00253-011-3483-0
Lu, H., Zou, W. X., Meng, J. C., Hu, J., Tan, R. X. (2000). New bioactive metabolites produced by colletotrichum sp., an endophytic fungus in artemisia annua. Plant Sci. 151 (1), 67–73. doi: 10.1016/S0168-9452(99)00199-5
Maehara, S., Simanjuntak, P., Maetani, Y., Kitamura, C., Ohashi, K., Shibuya, H. (2012). Ability of endophytic filamentous fungi associated with cinchona ledgeriana to produce cinchona alkaloids. J. Nat. Med. 67 (2), 421–423. doi: 10.1007/s11418-012-0701-8
Márquez, L. M., Redman, R. S., Rodriguez, R. J., Roossinck, M. J. (2007). A virus in a fungus in a plant: three-way symbiosis required for thermal tolerance. Science 315 (5811), 513–515. doi: 10.1126/science.1136237
Marquez-Santacruz, H. A., Hernandez-Leon, R., Orozco-Mosqueda, M. D. C., Velazquez-Sepulveda, I., Santoyo, G. (2010). Diversity of bacterial endophytes in roots of Mexican husk tomato plants (Physalisixocarpa) and their detection in the rhizosphere. Genet. Mol. Res. 9 (4), 2372–2380. doi: 10.4238/vol9-4gmr921
Mattos, K. A., Pádua, V. L., Romeiro, A., Hallack, L. F., Neves, B. C., Ulisses, T. M., et al. (2008). Endophytic colonization of rice (Oryza sativa l.) by the diazotrophic bacterium burkholderia kururiensis and its ability to enhance plant growth. Anais Da Acad. Bras. Ciências 80 (3), 477–493. doi: 10.1590/S0001-37652008000300009
Ma, Y., Zhang, C., Oliveira, R. S., Freitas, H., Luo, Y. (2016). Bioaugmentation with endophytic bacterium E6S homologous to achromobacter piechaudii enhances metal rhizoaccumulation in host sedum plumbizincicola. Front. Plant Sci. 7, 75. doi: 10.3389/fpls.2016.00075
McGuinness, M., Dowling, D. (2009). Plant-associated bacterial degradation of toxic organic compounds in soil. Int. J. Environ. Res. Public Health 6, 2226–2247. doi: 10.3390/ijerph6082226
Mehmood, A., Hussain, A., Irshad, M., Hamayun, M., Iqbal, A., Khan, N. (2019). In vitro production of IAA by endophytic fungus aspergillus awamori and its growth promoting activities in zea mays. Symbiosis 77 (3), 225–235. doi: 10.1007/s13199-018-0583-y
Mejía, L. C., Rojas, E. I., Maynard, Z., Van Bael, S., Arnold, A. E., Hebbar, P., et al. (2008). Endophytic fungi as biocontrol agents of theobroma cacao pathogens. Biol. Control 46 (1), 4–14. doi: 10.1016/j.biocontrol.2008.01.012
Meng, J. J., He, X. L. (2011). Effects of AM fungi on growth and nutritional contents of salvia miltiorrhiza bge. under drought stress. J. Agricult. Univ. Hebei 34 (1), 51–61. doi: 10.3969/j.issn.1000-1573.2011.01.011
Mercado-Blanco, J., Rodrıguez-Jurado, D., Hervás, A., Jiménez-Dıaz, R. M. (2004). Suppression of verticillium wilt in olive planting stocks by root-associated fluorescent pseudomonas spp. Biol. Control 30 (2), 474–486. doi: 10.1016/j.biocontrol.2004.02.002
Miller, J. D., Mackenzie, S., Foto, M., Adams, G. W., Findlay, J. A. (2002). Needles of white spruce inoculated with rugulosin-producing endophytes contain rugulosin reducing spruce budworm growth rate. Myco. Res. 106 (4), 471–479. doi: 10.1017/S0953756202005671
Ming, Q., Han, T., Li, W., Zhang, Q., Zhang, H., Zheng, C., et al. (2011). Tanshinone IIA and tanshinone I production by trichoderma atroviride D16, an endophytic fungus in salvia miltiorrhiza. Phytomedicine 19 (3-4), 330–333. doi: 10.1016/j.phymed.2011.09.076
Misganaw, G., Simachew, A., Gessesse, A. (2019). Endophytes of finger millet (Eleusine coracana) seeds. Symbiosis 78 (3), 203–213. doi: 10.1007/s13199-019-00607-5
Mishra, J., Singh, R., Arora, N. K. (2017). Alleviation of heavy metal stress in plants and remediation of soil by rhizosphere microorganisms. Front. Microbiol. 8, 1706. doi: 10.3389/fmicb.2017.01706
Mucciarelli, M., Scannerini, S., Bertea, C., Maffei, M. (2003). In vitro and in vivo peppermint (Mentha piperita) growth promotion by nonmycorrhizal fungal colonization. New Phytol. 158 (3), 579–591. doi: 10.1046/j.1469-8137.2003.00762.x
Müller, J. L. (2015). Plants and endophytes: equal partners in secondary metabolite production? Biotechnol. Let. 37 (7), 1325–1334. doi: 10.1007/s10529-015-1814-4
Muthukumarasamy, R., Revathi, G., Seshadri, S., Lakshminarasimhan, C. (2002). Gluconacetobacter diazotrophicus (syn. acetobacter diazotrophicus), a promising diazotrophic endophyte in tropics. Curr. Sci. 83, 137–145. Available at: https://www.currentscience.ac.in/Volumes/83/02/0137.pdf
Nasopoulou, C., Pohjanen, J., Koskimäki, J. J., Zabetakis, I., Pirttilä, A. M. (2014). Localization of strawberry (Fragaria x ananassa) and methylobacterium extorquens genes of strawberry flavor biosynthesis in strawberry tissue by in situ hybridization. J. Plant Physiol. 171 (13), 1099–1105. doi: 10.1016/j.jplph.2014.03.018
Naya, L., Ladrera, R., Ramos, J., González, E. M., Arrese-Igor, C., Minchin, F. R., et al. (2007). The response of carbon metabolism and antioxidant defenses of alfalfa nodules to drought stress and to the subsequent recovery of plants. Plant Physiol. 144 (2), 1104–1114. doi: 10.1104/pp.107.099648
Ni, T., Yue, J., Sun, G., Zou, Y., Wen, J., Huang, J. (2012). Ancient gene transfer from algae to animals: mechanisms and evolutionary significance. BMC Evol. Biol. 12 (1), 1–10. doi: 10.1186/1471-2148-12-83
Nogueira-Lopez, G., Greenwood, D. R., Middleditch, M., Winefield, C., Eaton, C., Steyaert, J. M., et al. (2018). The apoplastic secretome of trichoderma virens during interaction with maize roots shows an inhibition of plant defense and scavenging oxidative stress secreted proteins. Front. Plant Sci. 9, 409. doi: 10.3389/fpls.2018.00409
O’Callaghan, M. (2016). Microbial inoculation of seed for improved crop performance: issues and opportunities. Appl. Microbiol. Biotechnol. 100, 5729–5746. doi: 10.1007/s00253-016-7590-9
Oliveira, A. L. M., Stoffels, M., Schmid, M., Reis, V. M., Baldani, J. I., Hartmann, A. (2009). Colonization of sugarcane plantlets by mixed inoculations with diazotrophic bacteria. Eur. J. Soil Biol. 45 (1), 106–113. doi: 10.1016/j.ejsobi.2008.09.004
Palaniyandi, S. A., Yang, S. H., Zhang, L., Suh, J. W. (2013). Effects of actinobacteria on plant disease suppression and growth promotion. Appl. Microbiol. Biotechnol. 97 (22), 9621–9636. doi: 10.1007/s00253-013-5206-1
Palmer, J. D., Soltis, D. E., Chase, M. W. (2004). The plant tree of life: an overview and some points of view. Am. J. Bot. 91 (10), 1437–1445. doi: 10.3732/ajb.91.10.1437
Pandey, S. S., Singh, S., Babu, C. V., Shanker, K., Srivastava, N. K., Kalra, A. (2016a). Endophytes of opium poppy differentially modulate host plant productivity and genes for the biosynthetic pathway of benzylisoquinoline alkaloids. Planta 243 (5), 1097–1114. doi: 10.1007/s00425-016-2467-9
Pandey, S. S., Singh, S., Babu, C. V., Shanker, K., Srivastava, N. K., Shukla, A. K., et al. (2016b). Fungal endophytes of catharanthus roseus enhance vindoline content by modulating structural and regulatory genes related to terpenoid indole alkaloid biosynthesis. Sci. Rep. 6, 26583. doi: 10.1038/srep26583
Pan, X. W., Han, L., Zhang, Y. H., Chen, D. F., Simonsen, H. T. (2015). Sclareol production in the moss physcomitrella patens and observations on growth and terpenoid biosynthesis. Plant Biotechnol. Rep. 9 (3), 149–159. doi: 10.1007/s11816-015-0353-8
Patriquin, D. G., Dobereiner, J. (1978). Light microscopy observations of tetrazolium-reducing bacteria in the endorhizosphere of maize and other grasses in Brazil. Can. J. Microbiol. 24 (6), 734–742. doi: 10.1139/m78-122
Patten, C. L., Glick, B. R. (2002). Role of pseudomonas putida indoleacetic acid in development of the host plant root system. Appl. Environ. Microbiol. 68 (8), 3795–3801. doi: 10.1128/AEM.68.8.3795-3801.2002
Penrose, D. M., Moffatt, B. A., Glick, B. R. (2001). Determination of 1-aminocycopropane-1-carboxylic acid (ACC) to assess the effects of ACC deaminase-containing bacteria on roots of canola seedlings. Can. J. Microbiol. 47 (1), 77–80. doi: 10.1139/w00-128
Poling, S. M., Wicklow, D. T., Rogers, K. D., Gloer, J. B. (2008). Acremonium zeae, a protective endophyte of maize, produces dihydroresorcylide and 7-hydroxydihydroresorcylides. J. Agricult. Food Chem. 56 (9), 3006–3009. doi: 10.1021/jf073274f
Prado, R., Vendramim, J. D., Bicalho, K. U., dos Santos Andrade, M., Fernandes, J. B., de Andrade Moral, R., et al. (2013). Annona mucosa Jacq.(Annonaceae): a promising source of bioactive compounds against sitophilus zeamais Mots.(Coleoptera: Curculionidae). J. Stored Prod. Res. 55, 6–14. doi: 10.1016/j.jspr.2013.06.001
Preston, G. M., Bertrand, N., Rainey, P. B. (2001). Type III secretion in plant growth-promoting pseudomonas fluorescens SBW25. Mol. Microbiol. 41 (5), 999–1014. doi: 10.1046/j.1365-2958.2001.02560.x
Qawasmeh, A., Obied, H. K., Raman, A., Wheatley, W. (2012). Influence of fungal endophyte infection on phenolic content and antioxidant activity in grasses: interaction between lolium perenne and different strains of neotyphodium lolii. J. Agricult. Food Chem. 60 (13), 3381–3388. doi: 10.1021/jf204105k
Qiu, M., Xie, R. S., Shi, Y., Zhang, H., Chen, H. M. (2010). Isolation and identification of two flavonoid-producing endophytic fungi from ginkgo biloba l. Ann. Microbiol. 60 (1), 143–150. doi: 10.1007/s13213-010-0016-5
Radwan, S. (2009). “Phytoremediation for oily desert soils,” in Advances in applied bioremediation (Berlin, Heidelberg: Springer), (pp. 279–298). doi: 10.1007/978-3-540-89621-0_15
Rajkumar, M., Ae, N., Freitas, H. (2009). Endophytic bacteria and their potential to enhance heavy metal phytoextraction. Chemosphere 77 (2), 153–160. doi: 10.1016/j.chemosphere.2009.06.047
Rashid, S., Charles, T. C., Glick, B. R. (2012). Isolation and characterization of new plant growth-promoting bacterial endophytes. Appl. Soil Ecol. 61, 217–224. doi: 10.1016/j.apsoil.2011.09.011
Reddell, P., Gordon, V. (2000). Lessons from nature’: can ecology provide new leads in the search for novel bioactive chemicals from tropical rainforests?. in biodiversity: New leads for the pharmaceutical and agrochemical industries. Proc Int Meeting Held 5-8 September 1999 Univ St Andrews UK, 205–212. doi: 10.1039/9781847550231-00205
Rehman, S., Shawl, A. S., Kour, A., Andrabi, R., Sudan, P., Sultan, P., et al. (2008). An endophytic neurospora sp. from nothapodytes foetida producing camptothecin. Appl. Biochem. Microbiol. 44 (2), 203–209. doi: 10.1134/S0003683808020130
Ren, C. G., Dai, C. C. (2012). Jasmonic acid is involved in the signaling pathway for fungal endophyte-induced volatile oil accumulation of atractylodes lancea plantlets. BMC Plant Biol. 12 (1), 128. doi: 10.1186/1471-2229-12-128
Rodriguez, R. J., White, J. F., Jr., Arnold, A. E., Redman, A. R. A. (2009). Fungal endophytes: diversity and functional roles. New Phytol. 182 (2), 314–330. doi: 10.1111/j.1469-8137.2009.02773.x
Roos, I. M., Hattingh, M. J. (1983). Scanning electron microscopy of pseudomonas syringae pv, morsprunorum on sweet cherry leaves. J. Phytopathol. 108 (1), 18–25. doi: 10.1111/j.1439-0434.1983.tb00559.x
Ryan, P. R., Germaine, K., Franks, A., Ryan, D. J., Dowling, D. N. (2008). Bacterial endophytes: Recent developments and applications. FEMS microbiol. Lett 278, 1–9. doi: 10.1111/j.1574-6968.2007.00918.x
Sørensen, J., Sessitsch, A. (2006). “Plant-associated bacteria lifestyle and molecular interactions,” in Modern soil microbiology, 2nd edn. Eds. Van Elsas, J. D., Jansson, J. K., Trevors, J. T., Nannipieri, P. (Boca Raton: CRC press), 211–236.
Salam, N., Khieu, T. N., Liu, M. J., Vu, T. T., Chu-Ky, S., Quach, N. T., et al. (2017). Endophytic actinobacteria associated with dracaena cochinchinensis lour.: isolation, diversity, and their cytotoxic activities. BioMed. Res. Int. 2017, 1308563. doi: 10.1155/2017/1308563
Santoyo, G., Moreno-Hagelsieb, G., del Carmen Orozco-Mosqueda, M., Glick, B. R. (2016). Plant growth-promoting bacterial endophytes. Microbiol. Res. 183, 92–99. doi: 10.1016/j.micres.2015.11.008
Saravanan, V. S., Madhaiyan, M., Osborne, J., Thangaraju, M., Sa, T. M. (2008). Ecological occurrence of gluconacetobacter diazotrophicus and nitrogen-fixing acetobacteraceae members: their possible role in plant growth promotion. Microb. Ecol. 55 (1), 130–140. doi: 10.1007/s00248-007-9258-6
Sasse, J., Martinoia, E., Northen, T. (2018). Feed your friends: do plant exudates shape the root microbiome? Trends Plant Sci. 23 (1), 25–41. doi: 10.1016/j.tplants.2017.09.003
Sato, F., Kumagai, H. (2013). Microbial production of isoquinoline alkaloids as plant secondary metabolites based on metabolic engineering research. Proc. Jpn. Acad. B.: Phys. Biol. Sci. 89 (5), 165–182. doi: 10.2183/pjab.89.165
Schippmann, U., Leaman, D. J., Cunningham, A. B. (2002). Impact of cultivation and gathering of medicinal plants on biodiversity: Global trends and issues (Rome, Italy: Inter-Department Working Group on Biology Diversity for Food and Agriculture, FAO). Available at: http://www.fao.org/3/aa010e/AA010E00.pdf.
Schmidt, R., Köberl, M., Mostafa, A., Ramadan, E. M., Monschein, M., Jensen, K. B., et al. (2014). Effects of bacterial inoculants on the indigenous microbiome and secondary metabolites of chamomile plants. Front. Microbiol. 5. doi: 10.3389/fmicb.2014.00064
Schulz, B., Boyle, C. (2005). The endophytic continuum. Mycol. Res. 109 (6), 661–686. doi: 10.1017/S095375620500273X
Schulz, B., Boyle, C., Draeger, S., Römmert, A. K., Krohn, K. (2002). Endophytic fungi: a source of novel biologically active secondary metabolites. Mycol. Res. 106 (9), 996–1004. doi: 10.1017/S0953756202006342
Schulz, B., Römmert, A. K., Dammann, U., Aust, H. J., Strack, D. (1999). The endophyte-host interaction: a balanced antagonism? Mycol. Res. 103 (10), 1275–1283. doi: 10.1017/S0953756299008540
Scott, R. I., Chard, J. M., Hocart, M. J., Lennard, J. H., Graham, D. C. (1996). Penetration of potato tuber lenticels by bacteria in relation to biological control of blackleg disease. Potato Res. 39 (3), 333–344. doi: 10.1007/BF02357937
Segura, A., Ramos, J. L. (2013). Plant-bacteria interactions in the removal of pollutants. Curr. Opin. Biotechnol. 24, 467–473. doi: 10.1016/j.copbio.2012.09.011
Sessitsch, A., Coenye, T., Sturz, A. V., Vandamme, P., Barka, E. A., Salles, J. F., et al. (2005). Burkholderia phytofirmans sp. nov., a novel plant-associated bacterium with plant-beneficial properties. Int. J. Syst. Evol. Microbiol. 55 (3), 1187–1192. doi: 10.1099/ijs.0.63149-0
Shah, S., Shrestha, R., Maharjan, S., Selosse, M. A., Pant, B. (2019b). Isolation and characterization of plant growth-promoting endophytic fungi from the roots of dendrobium moniliforme. Plants 8 (1), 5. doi: 10.3390/plants8010005
Shah, S., Thapa, B. B., Chand, K., Pradhan, S., Singh, A., Varma, A., et al. (2019a). Piriformospora indica promotes the growth of the in-vitro-raised cymbidium aloifolium plantlet and their acclimatization. Plant Signal. Behav. 14 (6), 1596716. doi: 10.1080/15592324.2019.1596716
Sharma, V. K., Nowak, J. (1998). Enhancement of verticillium wilt resistance in tomato transplants by in vitro co-culture of seedlings with a plant growth promoting rhizobacterium (Pseudomonas sp. strain PsJN). Can. J. Microbiol. 44 (6), 528–536. doi: 10.1139/w98-017
Sheibani-Tezerji, R., Rattei, T., Sessitsch, A., Trognitz, F., Mitter, B. (2015). Transcriptome profiling of the endophyte burkholderia phytofirmans PsJN indicates sensing of the plant environment and drought stress. MBio 6 (5), e00621–e00615. doi: 10.1128/mBio.00621-15
Sheng, X. F., Xia, J. J., Jiang, C. Y., He, L. Y., Qian, M. (2008). Characterization of heavy metal-resistant endophytic bacteria from rape (Brassica napus) roots and their potential in promoting the growth and lead accumulation of rape. Environ. pollut. 156, 1164–1170. doi: 10.1016/j.envpol.2008.04.007
Sheoran, N., Nadakkakath, A. V., Munjal, V., Kundu, A., Subaharan, K., Venugopal, V., et al. (2015). Genetic analysis of plant endophytic pseudomonas putida BP25 and chemo-profiling of its antimicrobial volatile organic compounds. Microbiol. Res. 173, 66–78. doi: 10.1016/j.micres.2015.02.001
Shimizu, M. (2011). “Endophytic actinomycetes: biocontrol agents and growth promoters,” in Bacteria in agrobiology: Plant growth responses (Springer), 201–220, Berlin, Heidelberg: Springer-Verlag. doi: 10.1007/978-3-642-20332-9_10
Shrestha, K., Strobel, G. A., Shrivastava, S. P., Gewali, M. B. (2001). Evidence for paclitaxel from three new endophytic fungi of Himalayan yew of Nepal. Planta Med. 67 (04), 374–376. doi: 10.1055/s-2001-14307
Shweta, S., Zuehlke, S., Ramesha, B. T., Priti, V., Kumar, P. M., Ravikanth, G., et al. (2010). Endophytic fungal strains of fusarium solani, from apodytes dimidiata e. mey. ex arn (Icacinaceae) produce camptothecin, 10-hydroxycamptothecin and 9-methoxycamptothecin. Phytochemistry 71 (1), 117–122. doi: 10.1016/j.phytochem.2009.09.030
Silva, G. H., Teles, H. L., Zanardi, L. M., Young, M. C. M., Eberlin, M. N., Hadad, R., et al. (2006). Cadinane sesquiterpenoids of phomopsis cassiae, an endophytic fungus associated with cassia spectabilis (Leguminosae). Phytochemistry 67 (17), 1964–1969. doi: 10.1016/j.phytochem.2006.06.004
Soldan, R., Mapelli, F., Crotti, E., Schnell, S., Daffonchio, D., Marasco, R., et al. (2019). Bacterial endophytes of mangrove propagules elicit early establishment of the natural host and promote growth of cereal crops under salt stress. Microbiol. Res. 223, 33–43. doi: 10.1016/j.micres.2019.03.008
Spiering, M. J., Greer, D. H., Schmid, J. A. N. (2006). Effects of the fungal endophyte, neotyphodium lolii, on net photosynthesis and growth rates of perennial ryegrass (Lolium perenne) are independent of in planta endophyte concentration. Ann. Bot. 98 (2), 379–387. doi: 10.1093/aob/mcl108
Sprent, J. I., De Faria, S. M. (1989). “Mechanisms of infection of plants by nitrogen fixing organisms,” in Nitrogen fixation with non-legumes (Dordrecht: Springer), (pp. 3–(pp11). doi: 10.1007/978-94-009-0889-5_1
Stępniewska, Z., Kuźniar, A. (2013). Endophytic microorganisms–promising applications in bioremediation of greenhouse gases. Appl. Microbiol. Biotechnol. 97 (22), 9589–9596. doi: 10.1007/s00253-013-5235-9
Stacey, G., Libault, M., Brechenmacher, L., Wan, J., May, G. D. (2006). Genetics and functional genomics of legume nodulation. Curr. Opin. Plant Biol. 9 (2), 110–121. doi: 10.1016/j.pbi.2006.01.005
Stierle, A., Strobel, G., Stierle, D., Grothaus, P., Bignami, G. (1995). The search for a taxol-producing microorganism among the endophytic fungi of the pacific yew, taxus brevifolia. J. Nat. Prod 58 (9), 1315–1324. doi: 10.1021/np50123a002
Strobel, G. A. (2003). Endophytes as sources of bioactive products. Microb. Inf. 5 (6), 535–544. doi: 10.1016/S1286-4579(03)00073-X
Strobel, G., Daisy, B., Castillo, U., Harper, J. (2004). Natural products from endophytic microorganisms. J. Nat. Prod. 67 (2), 257–268. doi: 10.1021/np030397v
Strobel, G. A., Miller, R. V., Martinez-Miller, C., Condron, M. M., Teplow, D. B., Hess, W. M. (1999). Cryptocandin, a potent antimycotic from the endophytic fungus cryptosporiopsis cf. quercina. Microbiology 145 (8), 1919–1926. doi: 10.1099/13500872-145-8-1919
Strobel, G., Stierle, A., Stierle, D., Hess, W. M. (1993). Taxomyces andreanae, a proposed new taxon for a bulbilliferous hyphomycete associated with pacific yew (Taxus brevifolia). Mycotaxon 47, 71–80. Available at: https://www.cabdirect.org/cabdirect/abstract/19932339243
Sumarah, M. W., Puniani, E., Sørensen, D., Blackwell, B. A., Miller, J. D. (2010). Secondary metabolites from anti-insect extracts of endophytic fungi isolated from picea rubens. Phytochemistry 71 (7), 760–765. doi: 10.1016/j.phytochem.2010.01.015
Sun, Y., Cheng, Z., Glick, B. R. (2009). The presence of a 1-aminocyclopropane-1-carboxylate (ACC) deaminase deletion mutation alters the physiology of the endophytic plant growth-promoting bacterium burkholderia phytofirmans PsJN. FEMS Microbiol. Lett. 296 (1), 131–136. doi: 10.1111/j.1574-6968.2009.01625.x
Sun, D., Ran, X., Wang, J. (2008). Isolation and identification of a taxol-producing endophytic fungus from podocarpus. Wei Sheng Wu Xue Bao 48 (5), 589. doi: 10.3321/j.issn:0001-6209.2008.05.005
Suryanarayanan, T. S., Thirunavukkarasu, N., Govindarajulu, M. B., Gopalan, V. (2012). Fungal endophytes: an untapped source of biocatalysts. Fungal Div. 54 (1), 19–30. doi: 10.1007/s13225-012-0168-7
Suryanarayanan, T. S., Thirunavukkarasu, N., Govindarajulu, M. B., Sasse, F., Jansen, R., Murali, T. S. (2009). Fungal endophytes and bioprospecting. Fungal Biol. Rev. 23, 9–19. doi: 10.1016/j.fbr.2009.07.001
Sziderics, A. H., Rasche, F., Trognitz, F., Sessitsch, A., Wilhelm, E. (2007). Bacterial endophytes contribute to abiotic stress adaptation in pepper plants (Capsicum annuum l.). Can. J. Microbiol. 53 (11), 1195–1202. doi: 10.1139/W07-082
Tanaka, A., Tapper, B. A., Popay, A., Parker, E. J., Scott, B. (2005). A symbiosis expressed non-ribosomal peptide synthetase from a mutualistic fungal endophyte of perennial ryegrass confers protection to the symbiotum from insect herbivory. Mol. Microbiol. 57 (4), 1036–1050. doi: 10.1111/j.1365-2958.2005.04747.x
Tang, M. J., Meng, Z. X., Guo, S. X., Chen, X. M., Xiao, P. G. (2008). Effects of endophytic fungi on the culture and four enzyme activities of anoectochilus roxburghii. Chin. Pharm. J. 43, 890–893. doi: 10.3321/j.issn:1001-2494.2008.12.003
Tan, R. X., Zou, W. X. (2001). Endophytes: a rich source of functional metabolites. Nat. Prod. Rep. 18 (4), 448–459. doi: 10.1039/b100918o
Tilman, D. (1998). The greening of the green revolution. Nature 396 (6708), 211–212. doi: 10.1038/24254
Ting, A. S. Y., Meon, S., Kadir, J., Radu, S., Singh, G. (2009). Induced host resistance by non-pathogenic fusarium endophyte as a potential defense mechanism in fusarium wilt management of banana. Pest Technol. 3 (1), 67–72.
Tiwari, R., Awasthi, A., Mall, M., Shukla, A. K., Srinivas, K. S., Syamasundar, K. V., et al. (2013). Bacterial endophyte-mediated enhancement of in planta content of key terpenoid indole alkaloids and growth parameters of catharanthus roseus. Ind. Crops Prod. 43, 306–310. doi: 10.1016/j.indcrop.2012.07.045
Tiwari, R., Kalra, A., Darokar, M. P., Chandra, M., Aggarwal, N., Singh, A. K., et al. (2010). Endophytic bacteria from ocimum sanctum and their yield enhancing capabilities. Curr. Microbiol. 60 (3), 167–171. doi: 10.1007/s00284-009-9520-x
Truyens, S., Jambon, I., Croes, S., Janssen, J., Weyens, N., Mench, M., et al. (2014). The effect of long-term cd and Ni exposure on seed endophytes of agrostis capillaris and their potential application in phytoremediation of metal-contaminated soils. Int. J. Phytoremed. 16 (7-8), 643–659. doi: 10.1080/15226514.2013.837027
Van Bael, S. A., Seid, M. A., Wcislo, W. T. (2012). Endophytic fungi increase the processing rate of leaves by leaf-cutting ants (Atta). Ecol. Entomol. 37 (4), 318–321. doi: 10.1111/j.1365-2311.2012.01364.x
Vanessa, M. C., Christopher, M. M. F. (2004). Analysis of the endophytic actinobacterial population in the roots of wheat (Triticum aestivum l.) by terminal restriction fragment length polymorphism and sequencing of 16S rRNA clones. Appl. Environ. Microbiol. 70 (3), 1787–1794. doi: 10.1128/AEM.70.3.1787-1794.2004
Vega, F. E., Posada, F., Aime, M. C., Pava-Ripoll, M., Infante, F., Rehner, S. A. (2008). Entomopathogenic fungal endophytes. Biol. Control 46 (1), 72–82. doi: 10.1016/j.biocontrol.2008.01.008
Venkatachalam, R., Subban, K., Paul, M. (2008). Taxol from botryodiplodia theobromae (BT 115)-an endophytic fungus of taxus baccata. J. Biotechnol. 136, S189–S190. doi: 10.1016/j.jbiotec.2008.07.1823
Verma, V. C., Gond, S. K., Kumar, A., Mishra, A., Kharwar, R. N., Gange, A. C. (2009). Endophytic actinomycetes from azadirachta indica a. juss.: isolation, diversity, and anti-microbial activity. Microb. Ecol. 57 (4), 749–756. doi: 10.1007/s00248-008-9450-3
Verma, S. C., Ladha, J. K., Tripathi, A. K. (2001). Evaluation of plant growth promoting and colonization ability of endophytic diazotrophs from deep water rice. J. Biotechnol. 91 (2-3), 127–141. doi: 10.1016/S0168-1656(01)00333-9
Villacieros, M., Power, B., Sánchez-Contreras, M., Lloret, J., Oruezabal, R. I., Martín, M., et al. (2003). Colonization behaviour of pseudomonas fluorescens and sinorhizobium meliloti in the alfalfa (Medicago sativa) rhizosphere. Plant Soil 251 (1), 47–54. doi: 10.1023/A:1022943708794
Viterbo, A. D. A., Chet, I. (2006). TasHyd1, a new hydrophobin gene from the biocontrol agent trichoderma asperellum, is involved in plant root colonization. Mol. Plant Pathol. 7 (4), 249–258. doi: 10.1111/j.1364-3703.2006.00335.x
Vitousek, P. M., Mooney, H. A., Lubchenco, J., Melillo, J. M. (1997). Human domination of earth’s ecosystems. Science 277 (5325), 494–499. doi: 10.1126/science.277.5325.494
Wang, W. X., Barak, T., Vinocur, B., Shoseyov, O., Altman, A. (2003). “Abiotic resistance and chaperones: possible physiological role of SP1, a stable and stabilizing protein from populus,” in Plant biotechnology 2002 and beyond (Dordrecht: Springer), (pp. 439–443). doi: 10.1007/978-94-017-2679-5_91
Wang, P., Kong, F., Wei, J., Wang, Y., Wang, W., Hong, K., et al. (2014). Alkaloids from the mangrove-derived actinomycete jishengella endophytica 161111. Mar. Drugs 12 (1), 477–490. doi: 10.3390/md12010477
Wang, L., Liu, L., Han, S. Z. (2009). Screening and identification of antimicrobe activity of endophytic fungus in glycyrrhiza uralensis. Biotechnol. Bull. 6, 034.
Wang, Y., Xu, L., Ren, W., Zhao, D., Zhu, Y., Wu, X. (2012). Bioactive metabolites from chaetomium globosum L18, an endophytic fungus in the medicinal plant curcuma wenyujin. Phytomedicine 19 (3-4), 364–368. doi: 10.1016/j.phymed.2011.10.011
Wang, J. W., Zheng, L. P., Tan, R. X. (2006). The preparation of an elicitor from a fungal endophyte to enhance artemisinin production in hairy root cultures of artemisia annua l. Sheng Wu Gong Cheng Xue Bao 22 (5), 829–834. doi: 10.3321/j.issn:1000-3061.2006.05.023
Wani, S. H., Kumar, V., Shriram, V., Sah, S. K. (2016). Phytohormones and their metabolic engineering for abiotic stress tolerance in crop plants. Crop J. 4 (3), 162–176. doi: 10.1016/j.cj.2016.01.010
Wan, Y., Luo, S., Chen, J., Xiao, X., Chen, L., Zeng, G., et al. (2012). Effect of endophyte-infection on growth parameters and cd-induced phytotoxicity of cd-hyperaccumulator solanum nigrum l. Chemosphere 89 (6), 743–750. doi: 10.1016/j.chemosphere.2012.07.005
Waqas, M., Khan, A. L., Hamayun, M., Shahzad, R., Kang, S. M., Kim, J. G., et al. (2015a). Endophytic fungi promote plant growth and mitigate the adverse effects of stem rot: an example of penicillium citrinum and aspergillus terreus. J. Plant Interact. 10 (1), 280–287. doi: 10.1080/17429145.2015.1079743
Waqas, M., Khan, A. L., Hamayun, M., Shahzad, R., Kim, Y. H., Choi, K. S., et al. (2015b). Endophytic infection alleviates biotic stress in sunflower through regulation of defense hormones, antioxidants and functional amino acids. Eur. J. Plant Pathol. 141 (4), 803–824. doi: 10.1007/s10658-014-0581-8
Waqas, M., Khan, A. L., Kamran, M., Hamayun, M., Kang, S. M., Kim, Y. H., et al. (2012). Endophytic fungi produce gibberellins and indoleacetic acid and promotes host-plant growth during stress. Mol. (Basel) 17 (9), 10754–10773. doi: 10.3390/molecules170910754
Wei, J., Liu, X., Wang, Q., Wang, C., Chen, X., Li, H. (2014). Effect of rhizodeposition on pyrene bioaccessibility and microbial structure in pyrene and pyrene-lead polluted soil. Chemosphere 97, 92–97. doi: 10.1016/j.chemosphere.2013.09.105
Weyens, N., Cross, S., Dupae, J., Newman, L., van der Lelie, D., Carleer, R., et al. (2010). Endophytic bacteria improve phytoremediation of Ni and TCE co-contamination. Environ. pollut. 158, 2422–2427. doi: 10.1016/j.envpol.2010.04.004
Weyens, N., van der Lelie, D., Taghavi, S., Vangronsveld, J. (2009). Phytoremediation: plant–endophyte partnerships take the challenge. Curr. Opin. Biotechnol. 20 (2), 248–254. doi: 10.1016/j.copbio.2009.02.012
Whipps, J., Gerhardson, B. (2007). Biological pesticides for control of seed-and soil-borne plant pathogens. Modern Soil Microbiol. CRC Press Boca Raton pp, 479–501. Available at: https://cir.nii.ac.jp/crid/1570854175613702528
Wiewiora, B., Żurek, G., Pańka, D. (2015). Is the vertical transmission of neotyphodium lolii in perennial ryegrass the only possible way to the spread of endophytes? PloS One 10 (2), e0117231. doi: 10.1371/journal.pone.0117231
Williams, T. A., Foster, P. G., Cox, C. J., Embley, T. M. (2013). An archaeal origin of eukaryotes supports only two primary domains of life. Nature 504 (7479), 231–236. doi: 10.1038/nature12779
Wu, C. H., Bernard, S. M., Andersen, G. L., Chen, W. (2009). Developing microbe–plant interactions for applications in plant-growth promotion and disease control, production of useful compounds, remediation and carbon sequestration. Microb. Biotechnol. 2 (4), 428–440. doi: 10.1111/j.1751-7915.2009.00109.x
Wu, L., Han, T., Li, W., Jia, M., Xue, L., Rahman, K., et al. (2013). Geographic and tissue influences on endophytic fungal communities of taxus chinensis var. mairei in China. Curr. Microbiol. 66 (1), 40–48. doi: 10.1007/s00284-012-0235-z
Wu, J. Y., Ng, J., Shi, M., Wu, S. J. (2007). Enhanced secondary metabolite (tanshinone) production of salvia miltiorrhiza hairy roots in a novel root–bacteria coculture process. Appl. Microbiol. Biotechnol. 77 (3), 543–550. doi: 10.1007/s00253-007-1192-5
Yang, N. Y., Jiang, S., Shang, E. X., Tang, Y. P., Duan, J. A. (2012). A new phenylpentanamine alkaloid produced by an endophyte bacillus subtilis isolated from angelica sinensis. J. Chem. Res. 36 (11), 647–647. doi: 10.3184/174751912X13469254685262
Yang, X., Shiping, G., Lingqi, Z., Hua, S. (2003). Select of producing podophyllotoxin endophytic fungi from podophyllin plant. Nat. Prod. Res. Devel. 15 (5), 419–422. doi: 10.3969/j.issn.1001-6880.2003.05.012
Yan, X. N., Sikora, R. A., Zheng, J. W. (2011). Potential use of cucumber (Cucumis sativus l.) endophytic fungi as seed treatment agents against root-knot nematode meloidogyne incognita. J. Zhejiang Univ. Sci. 12 (3), 219–225. doi: 10.1631/jzus.B1000165
Yan, L., Zhu, J., Zhao, X., Shi, J., Jiang, C., Shao, D. (2019). Beneficial effects of endophytic fungi colonization on plants. Appl. Microbiol. Biotechnol. 103 (8), 3327–3340. doi: 10.1007/s00253-019-09713-2
Yedidia, I., Benhamou, N., Chet, I. (1999). Induction of defense responses in cucumber plants (Cucumis sativus l.) by the biocontrol agent trichoderma harzianum. Appl. Environ. Microbiol. 65 (3), 1061–1070. doi: 10.1128/AEM.65.3.1061-1070.1999
Yin, H., Chen, J. L. (2008). Sipeimine-producing endophytic fungus isolated from: Fritillaria ussuriensis. Z. Für Naturforschung C. 63 (11-12), 789–793. doi: 10.1515/znc-2008-11-1202
Yong, Y., Dai, C., Gao, F., Yang, Q., Zhao, M. (1994). Effects of endophytic fungi on growth and two kinds of terpenoids for euphorbia pekinensis. Chin. Trad. Herbal. Drugs 40, 18–22. Available at: https://pesquisa.bvsalud.org/portal/resource/pt/wpr-579810
Yu, X. M., Guo, S. X. (2000). Establishment of symbiotic system for anoectochilus roxburghii (Wall.) lindl. and endophytic fungi. Zhongguo Zhong Yao Za Zhi 25 (2), 81–83. doi: 10.3321/j.issn:1001-5302.2000.02.006
Zeilinger, S., Gupta, V. K., Dahms, T. E., Silva, R. N., Singh, H. B., Upadhyay, R. S., et al. (2016). Friends or foes? emerging insights from fungal interactions with plants. FEMS Microbiol. Rev. 40 (2), 182–207. doi: 10.1093/femsre/fuv045
Zeng, S., Shao, H., Zhang, L. (2004). An endophytic fungus producing a substance analogous to podophyllotoxin isolated from diphylleia sinensis. J. Microbiol. 24, 1–2. doi: 10.3969/j.issn.1005-7021.2004.04.001
Zhang, H., Bai, X., Wu, B. (2012). Evaluation of antimicrobial activities of extracts of endophytic fungi from artemisia annua. Bangladesh J. Pharmacol. 7 (2), 120–123. doi: 10.3329/bjp.v7i2.10951
Zhang, X. X., George, A., Bailey, M. J., Rainey, P. B. (2006b). The histidine utilization (hut) genes of pseudomonas fluorescens SBW25 are active on plant surfaces, but are not required for competitive colonization of sugar beet seedlings. Microbiology 152 (6), 1867–1875. doi: 10.1099/mic.0.28731-0
Zhang, X., Li, G., Ma, J., Zeng, Y., Ma, W., Zhao, P. (2010). Endophytic fungus trichothecium roseum LZ93 antagonizing pathogenic fungi in vitro and its secondary metabolites. J. Microbiol. 48 (6), 784–790. doi: 10.1007/s12275-010-0173-z
Zhang, C. L., Liu, S. P., Lin, F. C., Kubicek, C. P., Druzhinina, I. S. (2007). Trichoderma taxi sp. nov., an endophytic fungus from Chinese yew taxus mairei. FEMS Microbiol. Lett. 270 (1), 90–96. doi: 10.1111/j.1574-6968.2007.00659.x
Zhang, H. W., Song, Y. C., Tan, R. X. (2006a). Biology and chemistry of endophytes. Nat. Prod. Rep. 23 (5), 753–771. doi: 10.1039/b609472b
Zhang, J., Wang, C., Guo, S., Chen, J., Xiao, P. (1999). Studies on the plant hormones produced by 5 species of endophytic fungi isolated from medicinal plants (Orchidacea). Zhongguo Yi Xue Ke Xue Yuan Xue Bao 21 (6), 460–465.
Zhang, P., Zhou, P. P., Yu, L. J. (2009). An endophytic taxol-producing fungus from taxus media, cladosporium cladosporioides MD2. Curr. Microbiol. 59 (3), 227. doi: 10.1007/s00284-008-9270-1
Zhou, X., Wang, Z., Jiang, K., Wei, Y., Lin, J., Sun, X., et al. (2007). Screening of taxol-producing endophytic fungi from taxus chinensis var. mairei. Appl. Biochem. Microbiol. 43 (4), 439–443. doi: 10.1134/S000368380704014X
Zhou, S. L., Yang, F., Lan, S. L., Xu, N., Hong, Y. H. (2009). Huperzine a producing conditions from endophytic fungus in SHB huperzia serrata. J. Microbiol. 3, 32–36. doi: 10.3969/j.issn.1005-7021.2009.03.006
Keywords: plant-microbe interaction, plant growth promotion, secondary metabolites, stress tolerance, medicinal plants, endophytic microorganisms
Citation: Tripathi A, Pandey P, Tripathi SN and Kalra A (2022) Perspectives and potential applications of endophytic microorganisms in cultivation of medicinal and aromatic plants. Front. Plant Sci. 13:985429. doi: 10.3389/fpls.2022.985429
Received: 03 July 2022; Accepted: 07 September 2022;
Published: 29 September 2022.
Edited by:
Maurizio Ruzzi, University of Tuscia, ItalyReviewed by:
Satish Kumar Verma, Banaras Hindu University, IndiaCopyright © 2022 Tripathi, Pandey, Tripathi and Kalra. This is an open-access article distributed under the terms of the Creative Commons Attribution License (CC BY). The use, distribution or reproduction in other forums is permitted, provided the original author(s) and the copyright owner(s) are credited and that the original publication in this journal is cited, in accordance with accepted academic practice. No use, distribution or reproduction is permitted which does not comply with these terms.
*Correspondence: Arpita Tripathi, YXJwaXRhdHJpcGF0aGkwMzkxQGdtYWlsLmNvbQ==
†These authors have contributed equally to this work and share first authorship
Disclaimer: All claims expressed in this article are solely those of the authors and do not necessarily represent those of their affiliated organizations, or those of the publisher, the editors and the reviewers. Any product that may be evaluated in this article or claim that may be made by its manufacturer is not guaranteed or endorsed by the publisher.
Research integrity at Frontiers
Learn more about the work of our research integrity team to safeguard the quality of each article we publish.