- 1State Key Laboratory of Plant Physiology and Biochemistry, College of Agronomy and Biotechnology, National Maize Improvement Center, Center for Crop Functional Genomics and Molecular Breeding, China Agricultural University, Beijing, China
- 2Food Crops Research Institute, Xinjiang Academy of Agricultural Sciences, Urumqi, China
- 3Agricultural College, Inner Mongolia Agricultural University, Hohhot, China
The ZmCCT locus underlies both stalk-rot resistance and photoperiod sensitivity in maize (Zea mays L.). We previously introduced nine resistant ZmCCT haplotypes into seven elite but susceptible maize inbred lines (containing the haplotype H1) to generate 63 backcross families. Here, we continued backcrossing, followed by selfing, to develop 63 near-isogenic lines (NILs). We evaluated 22 of these NILs for stalk-rot resistance and flowering time under long-day conditions. Lines harboring the haplotype H5 outperformed the others, steadily reducing disease severity, while showing less photoperiod sensitivity. To demonstrate the value of haplotype H5 for maize production, we selected two pairs of NILs, 83B28H1/83B28H5 and A5302H1/A5302H5, and generated F1 hybrids with the same genetic backgrounds but different ZmCCT alleles: 83B28H1 × A5302H1, 83B28H1 × A5302H5, 83B28H5 × A5302H1, and 83B28H5 × A5302H5. We performed field trials to investigate yield/yield-related traits, stalk-rot resistance, flowering time, and drought/salt tolerance in these four hybrids. 83B28H5 × A5302H1 performed the best, with significantly improved yield, stalk-rot resistance, and drought tolerance compared to the control (83B28H1 × A5302H1). Therefore, the ZmCCT haplotype H5 has great value for breeding maize varieties with high yield potential, stalk-rot resistance, and drought tolerance.
Introduction
Increasing maize (Zea mays L.) output is crucial to meet the global demand for food security. However, both biotic and abiotic stress pose great threats to maize productivity. Breeding maize varieties with enhanced disease resistance and drought tolerance is the most effective and environmentally friendly way to ensure the security of maize production and supply.
Plants have evolved sophisticated defense responses against pathogens, including pattern-triggered immunity (PTI) and effector-triggered immunity (ETI). However, disease resistance is usually associated with a fitness penalty. In Arabidopsis thaliana, the R gene leads to a 5%–10% reduction in fitness in the absence of infection (Tian et al., 2003; Karasov et al., 2017). In crops, resistance (R) and susceptible (S) genes can play opposite roles in disease resistance and grain yield, with S genes decreasing disease resistance, but positively correlated with plant growth and development (Brown and Rant, 2013; Deng et al., 2017; Nelson et al., 2018). For example, Mildew resistance locus O (mlo) is derived from the loss of function of the dominant S gene (MLO), which confers durable, broad-spectrum resistance to powdery mildew in various species (Wang et al., 2014; Nekrasov et al., 2017). However, mlo-mediated resistance against powdery mildew is usually accompanied by a yield penalty (Büschges et al., 1997; Consonni et al., 2006), hampering the use of such resistance genes in crop breeding. Fortunately, the mildew-resistant mutant Tamlo-R32 was recently created by genome editing and harbors an activated Tonoplast monosaccharide transporter 3 (TaTMT3B) gene to rescue the Tamlo-related growth and yield penalty (Li et al., 2022). In addition, some ideal resistance genes appear to have no fitness cost and even increase yields (Li et al., 2017a; Wang et al., 2018). In rice, IDEAL PLANT ARCHITECTURE 1 (IPA1) not only improves rice yields by reducing the formation of unproductive tillers to increase grains per panicle, but also enhances disease resistance by activating the pathogen defense gene WRKY45 (WRKY DNA-binding protein 45; Wang et al., 2018). Thus, it is essential to evaluate the impact of a resistance gene on yield under normal and infected conditions before it can be appropriately applied for genetic improvement.
Pleiotropic genes are single genes (or a single locus) that affect two or more unrelated phenotypic traits (Solovieff et al., 2013) and numerous pleiotropic genes and QTLs have been reported in plants, whose functions range from growth and development to disease resistance (Liang et al., 2021). Most resistance genes in Arabidopsis have strong pleiotropic effects (Karasov et al., 2017). For example, the Arabidopsis thaliana typical coiled-coil (CC)-NBS-LRR (CNL) proteins Resistance to Pseudomonas syringae 5 (Rps5) and Resistance to P. syringae pv maculicola 1 (Rpm1) cause a fitness penalty when activated (Tian et al., 2003; Karasov et al., 2014). In maize, ZmNF-Y3A encodes a transcription factor that promotes early flowering and increases drought and heat stress tolerance by binding to different cis-elements in the promoters of various genes (Su et al., 2018).
In maize, a CCT (CONSTANS (CO), CO-LIKE, and TIMING OF CAB1) domain-containing transcription regulator gene, ZmCCT, was first reported to regulate flowering time under long-day conditions (Hung et al., 2012; Yang et al., 2013). Remarkably, ZmCCT was subsequently confirmed to be the causative gene at QTL-qRfg1 associated with maize resistance to Gibberella stalk rot (Yang et al., 2010; Wang et al., 2017). The non-TE ZmCCT allele (lacking a transposable element [TE] in its promoter) is resistant to stalk rot and has photoperiod sensitivity under long-day conditions (Yang et al., 2013; Wang et al., 2017). In addition, the non-TE ZmCCT increases primary branch number, spike length (Xu et al., 2017), and crown root number (Zhang et al., 2018). A new ZmCCT allele was recently discovered that contains a 4.2-kilobase (kb) TE inserted in the intron of a non-TE ZmCCT; this allele shortens flowering time in low-latitude regions (Zhong et al., 2021). Phylogenetic analysis of genes encoding CCT proteins and their orthologs showed that the closest genes to ZmCCT are Sb06g000570 in sorghum and Ghd7 (grain number, plant height, and heading date 7) in rice (Xue et al., 2008; Yang et al., 2013). The CCT domain-containing gene Ghd7 is involved in grain number, plant height, heading date (Xue et al., 2008), regulation of hormone metabolism, and biotic/abiotic stress responses (Weng et al., 2014). The finding that ZmCCT and Ghd7 share highly similar genomic features prompted us to explore whether ZmCCT could also improve yield or other agronomic traits under various environmental conditions.
Marker-assisted selection (MAS) has been successfully used to breed disease-resistant lines (Zhao et al., 2012; Konlasuk et al., 2015; Xu et al., 2020). We previously introgressed nine non-TE ZmCCT haplotypes into seven elite inbred maize lines by MAS (Li et al., 2017b). In the current study, we selected 22 pairs of NILs derived from advanced backcross generations and evaluated their disease resistance and photoperiod sensitivity. We then focused on two pairs of NILs, 83B28H1/83B28H5, and A5302 H1/A5302H5, to prepare four F1 hybrids with the same genetic backgrounds but different ZmCCT haplotypes. These F1 hybrids were evaluated for their yield/yield-related traits, stalk-rot resistance, and flowering time under normal and biotic/abiotic stress conditions to assess the value of haplotype H5 in the breeding of resistant maize varieties.
Materials and methods
Plant materials
We previously identified 15 haplotypes (H1 to H15) at the ZmCCT locus. Of these, only haplotype H1 contains a TE insertion in the promoter, whereas the other 14 lack a TE insertion (non-TE ZmCCT). Nine donor parents carrying different non-TE ZmCCT haplotypes (H3, H4, H5, H6, H7, H12, H13, H14, and H15) were previously crossed with and then backcrossed to seven elite inbred lines with haplotype H1 (Zheng58, Chang7-2, 83B28, A5302, Jing24, F349, and Yu87-1) to generate 63 BC5F1 families (Li et al., 2017b). In the current study, we continued backcrossing twice to obtain 63 BC7F1 families, which were selfed and grown at the experimental station of China Agricultural University (Beijing, N39°54′, E116°24′). The BC7F2 families were planted in the Hainan winter nursery (Hainan, Sanya, N18°21′, E109°10′) in 2017/2018. After genotyping, BC7F2 plants from each family with homozygous haplotype H1 or one of the non-TE ZmCCT haplotypes were continuously selfed to produce homozygous BC7F3 lines, which were grown at the experimental station of China Agricultural University to develop pairs of near-isogenic lines (NILs).
Due to limited resources, we chose five haplotypes (H3, H5, H6, H12, and H13) in the Zheng58 and Chang7-2 backgrounds and six haplotypes (H3, H5, H6, H7, H12, and H13) in the 83B28 and A5302 backgrounds for further study. We used the GoldenGate 6KSNP chip to survey the genome-wide SNPs. The genomic identities of two pairs of NILs, 83B28H1/83B28H5, and A5302H1/A5302H5, were estimated to be 96.86% and 93.71%, respectively. They were then crossed with one another to produce four F1 hybrids with the same genetic backgrounds but different ZmCCT haplotypes, including 83B28H1 × A5302H1, 83B28H1 × A5302H5, 83B28H5 × A5302H1, and 83B28H5 × A5302H5.
Field experimental design
In 2018 and 2019, the NILs were grown in Beijing, China, and their stalk-rot resistance was investigated after artificial inoculation. Each pair of NILs was planted adjacent to each other, and different pairs of NILs were planted in a completely random design. Each NIL was planted in three rows, with 17 plants per row in three replicates. In 2020 and 2021, the 22 pairs of NILs were investigated for flowering time in Beijing, and due to the COVID-19 pandemic, each NIL was planted in two rows without replicates. For the four hybrids grown at different locations over the years, a randomized block design was implemented in the field trials with three replicates. Each hybrid was grown in three rows, with 17 plants per row.
Preparation of Fusarium graminearum inoculum, field inoculation, and scoring of symptoms
The preparation of Fusarium graminearum inoculum and field inoculation was previously described in detail (Yang et al., 2010). The six-scale scoring system (0, 0.2, 0.4, 0.6, 0.8, and 1) was applied to investigate symptoms in the field. A disease severity index (DSI) was used to assess the stalk-rot severity for each line or hybrid. The DSI was calculated using the disease scales of all plants in the same line or hybrid as follows: DSI (%) = [∑(score × number of plants in score)]/total number of plants × 100.
Investigation of flowering time and other agronomic traits
We investigated three flowering-related traits per plant, including days to heading (DTH), days to anthesis (DTA), and days to silking (DTS) for the NILs and hybrids in 2020 and 2021 in Beijing. All hybrids were investigated for yield and yield-related traits from 2018 to 2020. At the harvest stage, ears were collected from healthy plants and dried in the sun for ~2 weeks. Five ear-related traits were then measured: ear length (EL), bare tip length (BTL), ear diameter (ED), kernel row number (KRN), and kernel number per row (KNPR). After threshing, the kernels were stored at room temperature for open-air drying for at least 2 weeks, and the hundred kernel weight (HKW) and kernel weight per ear (KWPE) were measured. Two plant morphological traits were also investigated from 2019 to 2021: plant height (PH) and ear height (EH).
Investigation of plant tolerance to drought and salt stress
The four hybrids were also planted in Xinjiang (from 2018 to 2020) and Ningxia (2018 and 2019), China to investigate yield and yield-related traits under drought and salt stress conditions, respectively. Xinjiang is an ideal place to test the drought tolerance of maize. Moreover, we employed drip irrigation under a mulch film, making it easy to control irrigation. For well-watered (control) conditions, the plants were irrigated regularly as needed. For drought stress conditions, the amount of irrigation water was reduced by half during the entire growth period compared to well-watered conditions, except for the first irrigation at the time of sowing. The Northeast of Ningxia Plain contains mostly alkaline soil, making it an excellent place to test plant tolerance to salt stress. We tested the soil salinity before sowing in 2018 and 2019. The salinity of 0–25-cm soil was 1.10% and 1.11% in 2018 and 2019, respectively, with a soil pH of 8.6 in 2019, indicating that the chosen site was an alkaline field with salt stress conditions.
Statistical analysis
For each pair of NILs, the two NILs differed solely at the ZmCCT locus, i.e., the resistant allele without the TE versus the susceptible allele with the TE insertion. Significant differences in DSI and flowering time between two NILs were analyzed by t-test. For the hybrids, all data were analyzed by one-way analysis of variance (ANOVA) with Duncan’s test and Tamhane’s test using IBM SPSS Statistics (version 21.0.0.0).
Results
Evaluation of stalk-rot resistance and flowering time in the NILs
We previously determined that all BC3F1 and BC5F1 plants with the non-TE ZmCCT showed dramatically reduced DSI (Li et al., 2017b). Of the NILs developed in the current study, we selected 22 lines to further examine stalk-rot resistance and flowering time in 2018 and 2019. Plants in the Zheng58 background with the non-TE ZmCCT haplotypes (except H13) showed DSI values of almost zero in both 2018 and 2019, i.e., almost no plants were diseased. By contrast, the haplotype H13 decreased the DSI by 13.32% and 17.45% in 2018 and 2019, respectively (Figure 1A). In the Chang7-2 background, the non-TE ZmCCT haplotypes also dramatically decreased the DSI values, with average decreases of 9.43% and 23.38% in 2018 and 2019, respectively (Figure 1B). However, in the 83B28 and A5302 backgrounds, five non-TE ZmCCT haplotypes (H5, H6, H7, H12, and H13) tested in 2018 and six (H3, H5, H6, H7, H12, and H13) tested in 2019 showed a certain degree of variation in stalk-rot resistance (Figures 1C,D), with only haplotypes H3 and H5 steadily reducing the DSI. In 2019, haplotype H3 reduced the DSI by 14.42% in the 83B28 background and 34.81% in the A5302 background. Likewise, in 2018 and 2019, haplotype H5 reduced the DSI by 9.20% and 8.77% in the 83B28 background and 24.74% and 22.87% in the A5302 background, respectively (Figures 1C,D). These results indicate that the reduction in the severity of maize stalk-rot disease is determined by a combination of the genetic background and ZmCCT haplotype.
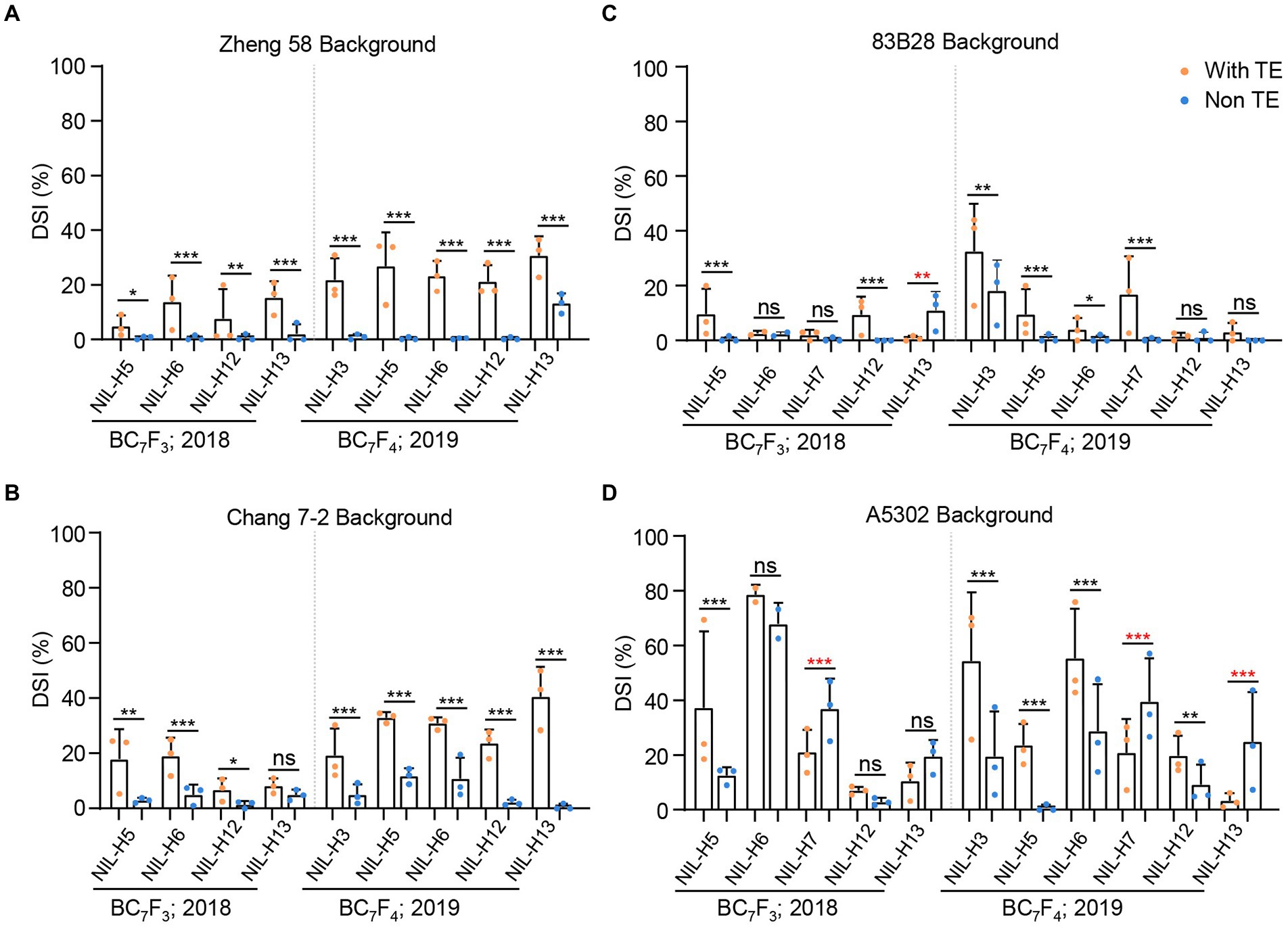
Figure 1. DSI of 22 NILs in four different backgrounds in 2018–2019 (Beijing). Five pairs of NILs include with TE ZmCCT homozygotes, and non-TE ZmCCT homozygotes in Zheng 58 (A), Chang 7–2 (B), 83B28 (C), and A5302 (D) background were investigated for DSI. NILs include with TE ZmCCT homozygotes: H1/H1, and non-TE ZmCCT homozygotes: H3/H3, H5/H5, H6/H6, H7/H7, H12/H12, or H13/H13 in different background were investigated for DSI. Values are mean ± standard deviation (s.d.). Asterisks indicate significant differences between H1/H1 plants and the other genotypes (two-tailed paired Student’s t-test, *p < 0.05, **p < 0.01, ***p < 0.001, ns, not significant).
We also investigated the flowering-related traits DTH, DTA, and DTS in the same sets of NILs. In the Zheng58 background, all non-TE ZmCCT haplotypes were more sensitive to long-day conditions than the TE haplotype H1 and delayed DTH, DTA, and DTS in Beijing by an average of 5.0, 7.0, and 10.6 days, respectively (Supplementary Figure 1A). Notably, the non-TE ZmCCT haplotypes in the Chang7-2 background were even more sensitive to long-day conditions than those in the Zheng58 background, resulting in further delays in DTH, DTA, and DTS by an average of 10.6, 11.6, and 13.3 days, respectively (Supplementary Figure 1B). By contrast, non-TE ZmCCT haplotypes in the 83B28 and A5302 backgrounds were less sensitive to long-day conditions in Beijing and did not show significant differences in most flowering-related traits. However, there were some subtle differences among the non-TE ZmCCT haplotypes, with haplotypes H3 and H5 showing no or less sensitivity to photoperiod in the 83B28 and A5302 backgrounds during 2020 and 2021 (Figures 2A,B).
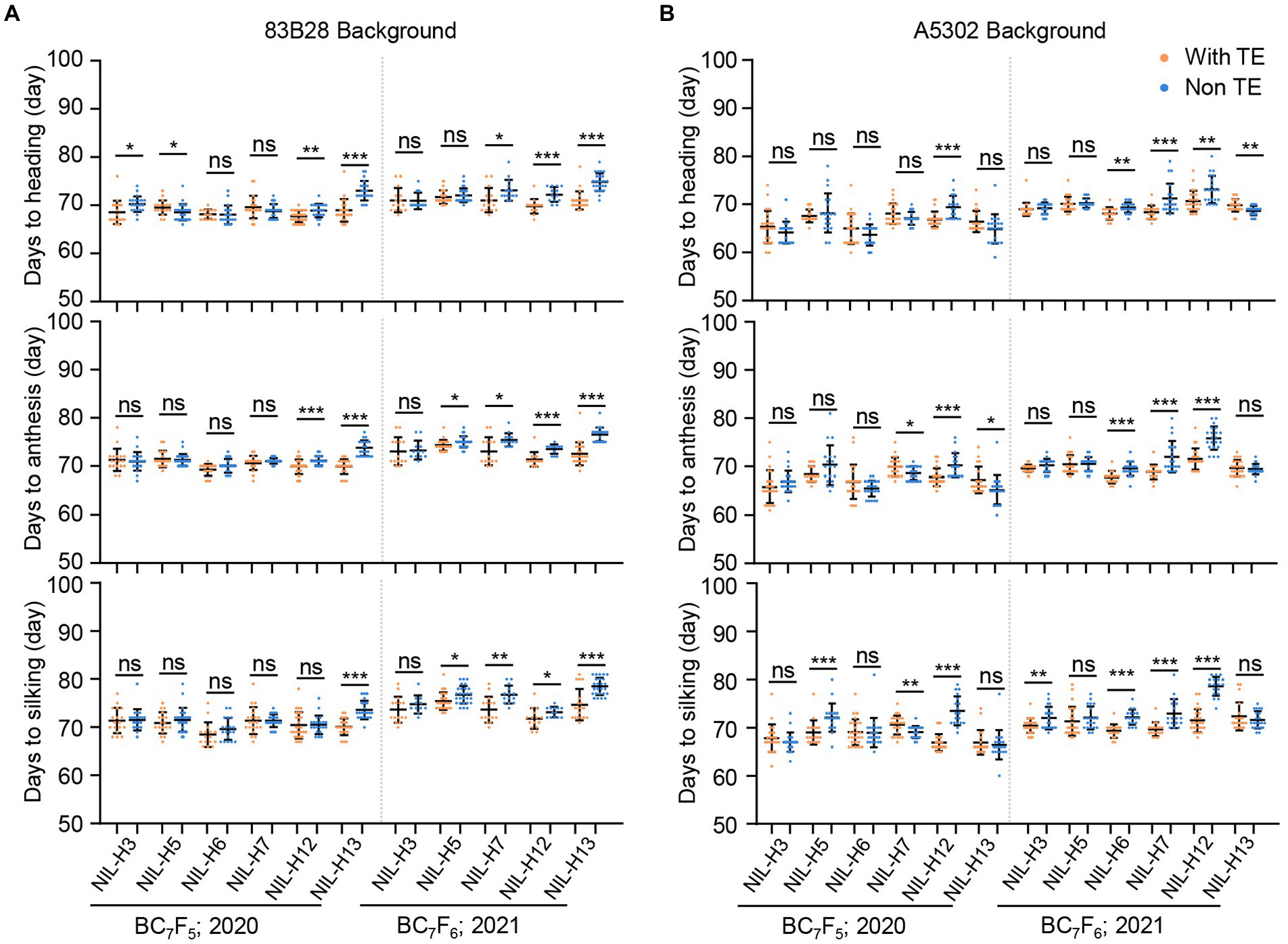
Figure 2. Twenty-two pairs of NILs in 83B28 (A) and A5302 (B) background were investigated for flowering time in 2020–2021 Beijing under long-day conditions. The genotypes of every pair of NILs were with TE ZmCCT homozygotes: H1/H1, and non-TE ZmCCT homozygotes: H3/H3, H5/H5, H6/H6, H7/H7, H12/H12, or H13/H13. Values are mean ± standard deviation (s.d.). Asterisks indicate significant differences between H1/H1 plants and the other genotypes (two-tailed paired Student’s t-test, *p < 0.05, **p < 0.01, ***p < 0.001, ns, not significant).
Of the non-TE ZmCCT haplotypes tested, H5 performed the best, with high and stable stalk-rot resistance as well as less or no photoperiod sensitivity in the 83B28 and A5302 backgrounds, suggesting it would be valuable for breeding stalk-rot-resistant varieties.
Stalk-rot resistance and flowering times of four F1 hybrids
We used two pairs of NILs, 83B28H1/83B28H5 and A5302H1/A5302H5, to generate four F1 hybrids. Overall, the F1 hybrids exhibited higher stalk-rot resistance than the parental lines in both 2018 and 2019, presumably due to heterosis. Among the four F1 hybrids, 83B28H5 × A5302H1 and 83B28H5 × A5302H5 only produced a few diseased plants. Compared to 83B28H1 × A5302H1, these two hybrids showed significantly lower DSI values, with an average reduction of 6.98% and 8.68%, respectively (Figure 3A; Table 1). However, there was no significant difference in the DSI values between 83B28H1 × A5302H5 and 83B28H1 × A5302H1 (Figure 3A). These findings suggest that the maternal parental line plays a decisive role in stalk-rot resistance and that the homozygous ZmCCT haplotype H5/H5 does not further increase stalk-rot resistance compared to the heterozygous ZmCCT haplotype H5/H1.
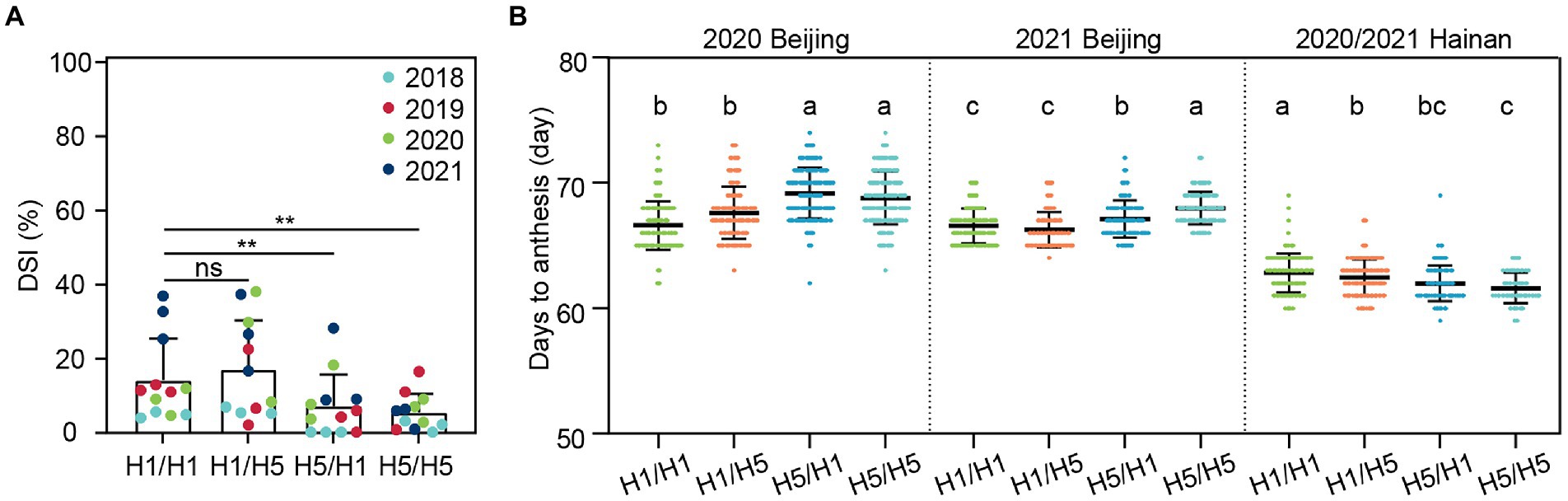
Figure 3. Evaluation of DSI and flowering time of four hybrids. In 2018–2021, the DSI (A) was investigated after artificial inoculated, each spot represents one replication of field trial (two-tailed paired Student’s t-test, **p < 0.01, ns, not signifcant). The flowering time (B) of four hybrids was investigated in 2020–2021 Beijing and 2020/2021 Hainan, which represents long-day conditions and short-day conditions, respectively. H1/H1, H1/H5, H5/H1, and H5/H5 are genotypes of four hybrids representing 83B28H1 × A5302H1, 83B28H1 × A5302H5, 83B28H5 × A5302H1, and 83B28H5 × A5302H5, respectively. Values are mean ± standard deviation (s.d.). Different letters indicate significant differences among hybrids (p < 0.05, one-way ANOVA, Tamhane test).
We also investigated the flowering-related traits of the four hybrids under long-day (Beijing, 2020) and short-day (Hainan, 2020/2021) conditions. In Beijing, the H5-containing parental lines 83B28H5 and A5302H5 did not show detectible photoperiod sensitivity (Figures 2A,B), whereas the hybrids 83B28H5 × A5302H1 and 83B28H5 × A5302H5 showed 1.4- and 1.8-day delays in flowering time (average of DTH, DTA, and DTS), respectively, compared to 83B28H1 × A5302H1. By contrast, the three flowering-related traits of the 83B28H1 × A5302H5 hybrid did not significantly differ from those of 83B28H1 × A5302H1 (Figure 3B; Supplementary Figure 2). In Hainan, the hybrid with two H5 alleles (83B28H5 × A5302H5) showed earlier flowering times than the hybrids with a single H5 (83B28H5 × A5302H1 and 83B28H1 × A5302H5), while 83B28H1 × A5302H1 showed the latest flowering time (Figure 3B; Supplementary Figure 2). Taken together, these results indicate that hybrids with the heterozygous genotype at ZmCCT (H5/H1) can help meet the breeding goal of improved maize stalk-rot resistance with little or no photoperiod sensitivity under long-day conditions.
The genetic effects of H5 on yield of the hybrids under infected conditions
To test the genetic effect of the haplotype H5 on yield under infected conditions, we planted the four hybrids in Beijing and performed artificial inoculation in the field, with no inoculation as a control. In 2018, hybrids 83B28H5 × A5302H1 and 83B28H5 × A5302H5 had significantly higher kernel weight per ear (KWPE) than 83B28H1 × A5302H1 under infected conditions, with increases of 16.6% and 11.4%, respectively, whereas the KWPE of 83B28H1 × A5302H5 did not significantly differ from that of 83B28H1 × A5302H1 (Figure 4). To confirm these findings, we repeated the experiment in Beijing in 2019 and added a no inoculation control. Compared to 83B28H1 × A5302H1, 83B28H5 × A5302H1 showed a significantly higher KWPE under both infected and non-infected conditions, increasing by 7.4% and 8.0%, respectively. By contrast, the other two hybrids, 83B28H1 × A5302H5 and 83B28H5 × A5302H5, did not show significant improvements in KWPE. In 2020, the KWPE of 83B28H5 × A5302H1 was not significantly improved compared with 83B28H1 × A5302H1, likely due to environmental effects (Figure 4).
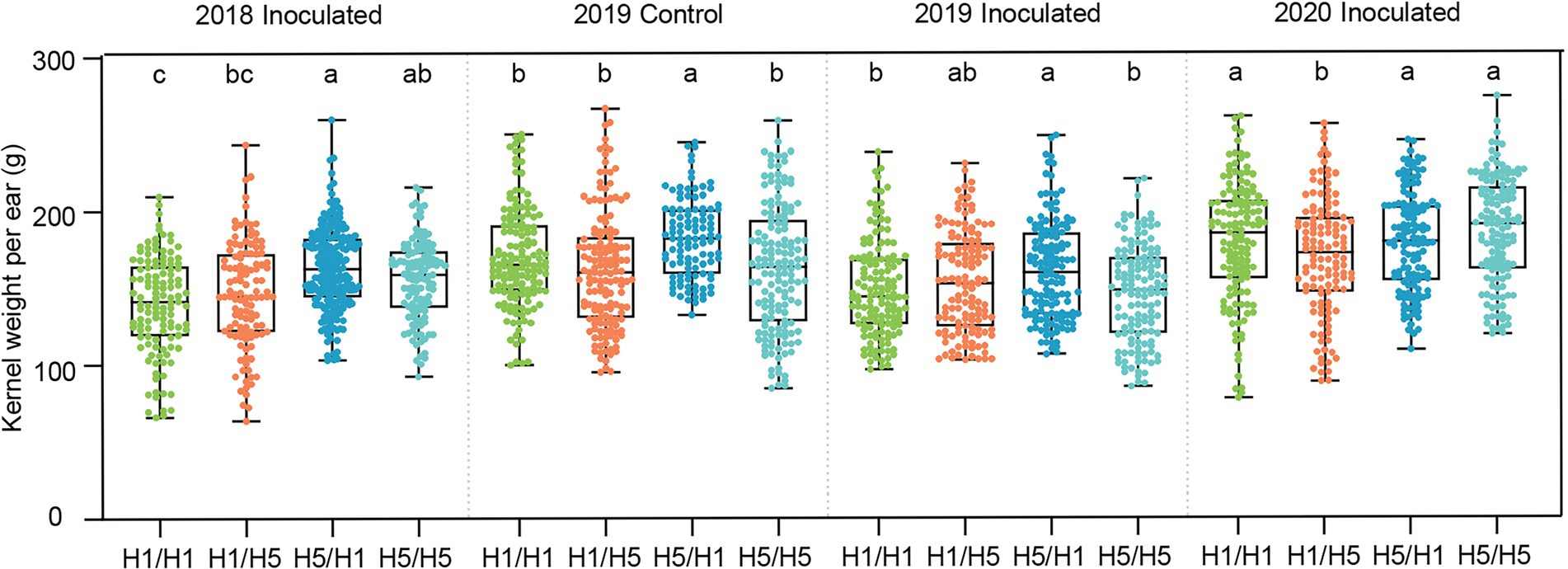
Figure 4. The kernel weight per ear of hybrids in Beijing under control and biotic stress. H1/H1, H1/H5, H5/H1, and H5/H5 are genotypes of four hybrids represent 83B28H1 × A5302H1, 83B28H1 × A5302H5, 83B28H5 × A5302H1, and 83B28H5 × A5302H5, respectively. Boxes show mean and quartile, whiskers represent max to min. Different letters indicate significant differences among hybrids (p < 0.05, one-way ANOVA, Tamhane’s test and Duncan’s test).
Consistent with its yield, the 83B28H5 × A5302H1 hybrid generally performed better than the control (83B28H1 × A5302H1) for yield-related traits, including ear length (EL), bare tip length (BTL), ear diameter (ED), kernel row number (KRN), kernel number per row (KNPR), and hundred kernel weight (HKW). In 2018, 83B28H5 × A5302H1 had greater EL, KNPR, and ED values than those of 83B28H1 × A5302H1 (Supplementary Figure 3). The hybrid 83B28H5 × A5302H5 had better BTL, ED, and KRN but worse HKW values than the control (Supplementary Figure 3). In 2019, 83B28H5 × A5302H1 had greater ED and HKW values than 83B28H1 × A5302H1 under normal conditions but showed no significant differences in any yield-related traits compared to 83B28H1 × A5302H1 under infected conditions (Supplementary Figure 3). By contrast, 83B28H1 × A5302H5 and 83B28H5 × A5302H5 showed better BTL and KRN but worse EL, KPR, and HKW values than the control under both infected and normal conditions (Supplementary Figure 3). In 2020, 83B28H5 × A5302H1 had higher EL and KPR values but lower ED, KRN, and HKW values than those of 83B28H1 × A5302H1, resulting in no difference in KWPE (Supplementary Figure 3; Figure 4). Taken together, these findings indicate that 83B28H5 × A5302H1 performed better than the other hybrids in terms of yield and yield-related traits.
The genetic effects of H5 on yield under abiotic stress conditions
We tested the yield and yield-related traits of the hybrids under drought stress conditions in Xinjiang from 2018 to 2020 and salt stress conditions in Ningxia in 2018 and 2019. Under normal irrigation conditions in Xinjiang from 2018 to 2020, 83B28H5 × A5302H1 and 83B28H5 × A5302H5 showed significantly higher KWPE compared to 83B28H1 × A5302H1 and 83B28H1 × A5302H5 (Figure 5). Under drought stress, 83B28H5 × A5302H1 showed the best KWPE among the four hybrids in 2018, with an increase of 9.7% compared to the control (83B28H1 × A5302H1), but with no significant difference in this value in 2019 and 2020. The hybrid 83B28H5 × A5302H5 showed a higher KWPE value in 2018 but lower values in 2019 and 2020 compared to the control. The KWPE of 83B28H1 × A5302H5 did not significantly differ from the control value from 2018 to 2019 but was lower than the control value in 2020 (Figure 5). Overall, 83B28H5 × A5302H1 performed the best among the four hybrids.
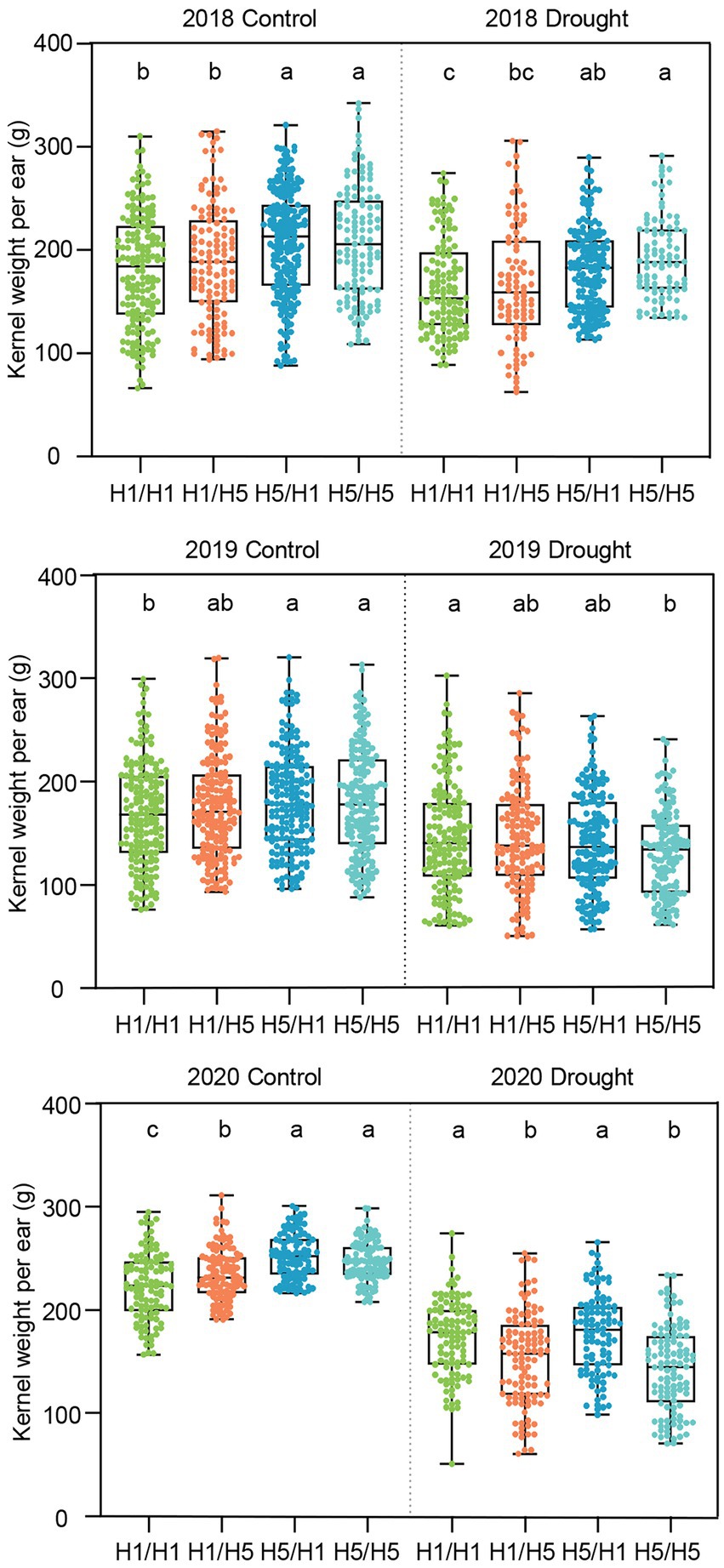
Figure 5. The kernel weight per ear of hybrids in 2018–2020 Xinjiang under control and drought stress. Figures above show the KWPE of hybrids in 2018, 2019, and 2020, respectively. H1/H1, H1/H5, H5/H1, and H5/H5 are genotypes of four hybrids represent 83B28H1 × A5302H1, 83B28H1 × A5302H5, 83B28H5 × A5302H1, and 83B28H5 × A5302H5, respectively. Boxes show mean and quartile, whiskers represent max to min. Different letters indicate significant differences among hybrids (p < 0.05, one-way ANOVA, Duncan’s test, and Tamhane’s test).
Under salt stress in Ningxia, 83B28H5 × A5302H1 and 83B28H5 × A5302H5 had lower KWPE values in 2018 but higher values in 2019 compared to 83B28H1 × A5302H1. The KWPE of 83B28H1 × A5302H5 did not significantly differ from the control in 2018 but was higher than the control value in 2019 (Figure 6). Unlike under F. graminearum inoculation and drought stress conditions, 83B28H1 × A5302H5 showed the best performance under salt stress conditions, suggesting that the haplotype H5 employs different mechanisms in response to different stress conditions.
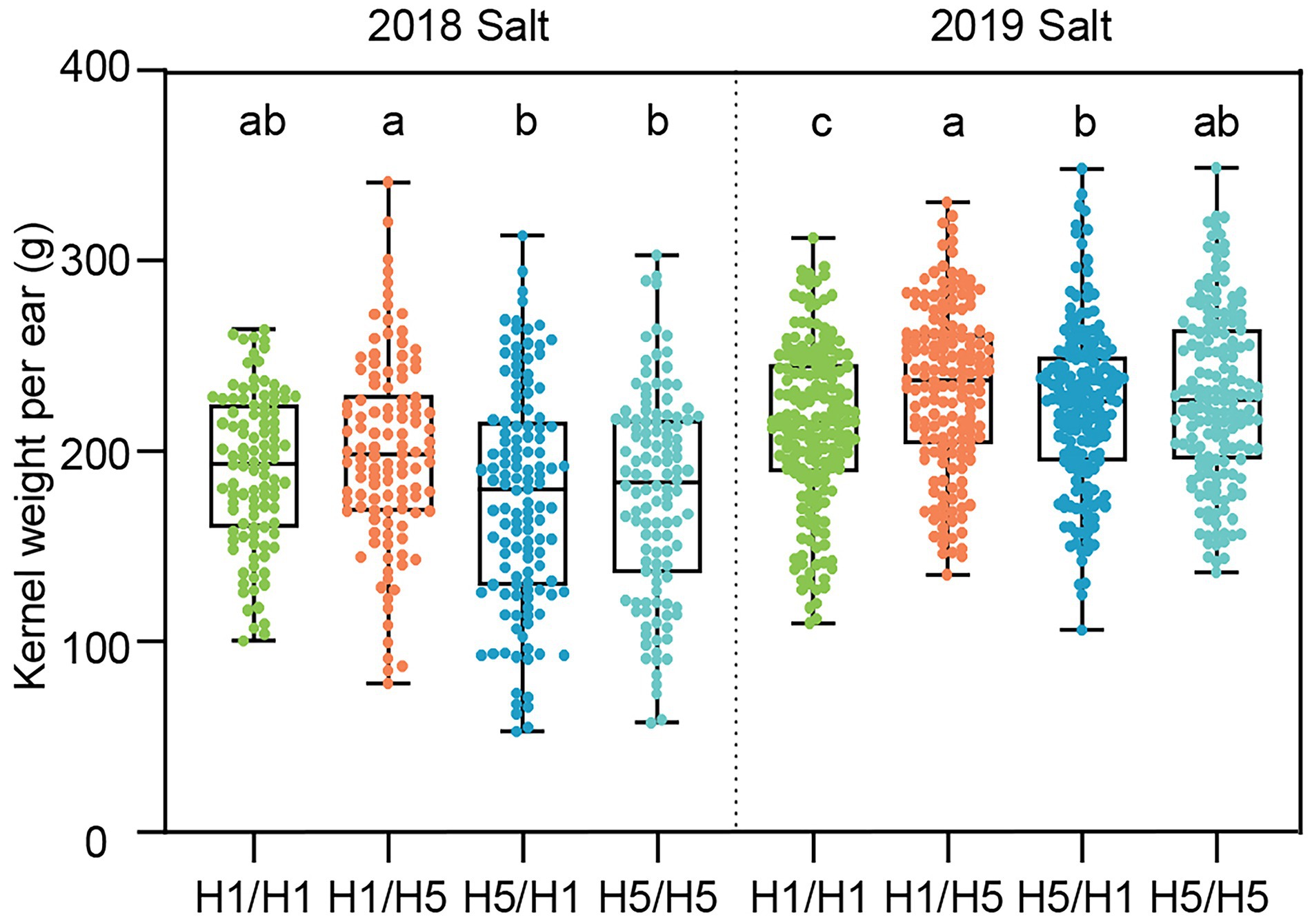
Figure 6. The yield-related traits of hybrids in 2018–2019 Ningxia under salt stress conditions. H1/H1, H1/H5, H5/H1, and H5/H5 are genotypes of four hybrids represent 83B28H1 × A5302H1, 83B28H1 × A5302H5, 83B28H5 × A5302H1, and 83B28H5 × A5302H5, respectively. Boxes show mean and quartile, whiskers represent max to min. Different letters indicate significant differences among hybrids (p < 0.05, one-way ANOVA, Duncan’s test, and Tamhane’s test).
When comparing yield-related traits among the four hybrids in Xinjiang, each hybrid showed similar trends under normal and drought conditions (Supplementary Figure 4). In 2018, 83B28H5 × A5302H1 had significantly higher EL, lower BTL, and higher KPR than 83B28H1 × A5302H1 under both normal and drought conditions (Supplementary Figure 4). Similarly, 83B28H5 × A5302H5 showed better EL, BTL, KPR, and ED values, whereas 83B28H1 × A5302H5 only showed improved ED and KRN values (Supplementary Figure 4). In 2019, 83B28H5 × A5302H1 showed higher HKW than 83B28H1 × A5302H1 under drought stress but no significant improvements in any other yield-related trait (Supplementary Figure 4). In 2020, 83B28H5 × A5302H1 had higher EL, lower BTL, and higher HKW but lower ED compared to 83B28H1 × A5302H1 under drought stress, while 83B28H5 × A5302H5 showed higher EL and lower BTL but lower ED vs. the control (Supplementary Figure 4). Finally, 83B28H1 × A5302H5 had a similar performance for yield-related traits compared to the control (83B28H1 × A5302H1; Supplementary Figure 4).
We also compared the yield-related traits of the hybrids under salt stress in Ningxia. The hybrid 83B28H5 × A5302H1 had significantly smaller ED than 83B28H1 × A5302H1, and 83B28H1 × A5302H5 had significantly higher KRN than 83B28H1 × A5302H1 (Supplementary Figure 5). For the remaining yield-related traits, no significant differences were detected among the four hybrids in 2018 and 2019 (Supplementary Figure 5). These yield-related traits underlie the KWPE performance of the four hybrids.
Morphological traits of the hybrids
To better understand the genetic effects of haplotype H5 on plant morphological traits, we investigated the plant height (PH) and ear height (EH) of the hybrids under different environmental conditions. In 2019, 83B28H5 × A5302H1 had significantly higher PH and EH than 83B28H1 × A5302H1 in Beijing (normal and infected conditions), Xinjiang (normal conditions), and Ningxia (salt stress; Figure 7). In Beijing and Xinjiang in 2020, 83B28H5 × A5302H1 and 83B28H5 × A5302H5 had significantly higher PH than 83B28H1 × A5302H1 and 83B28H1 × A5302H5 (Supplementary Figure 6). Finally, 83B28H5 × A5302H1 had significantly higher EH than 83B28H1 × A5302H5, while the EH values of 83B28H5 × A5302H5 and 83B28H1 × A5302H1 fell between those of 83B28H5 × A5302H1 and 83B28H1 × A5302H5 (Supplementary Figure 6). In conclusion, haplotype H5 significantly increased PH and EH in the hybrid 83B28H5 × A5302H1.
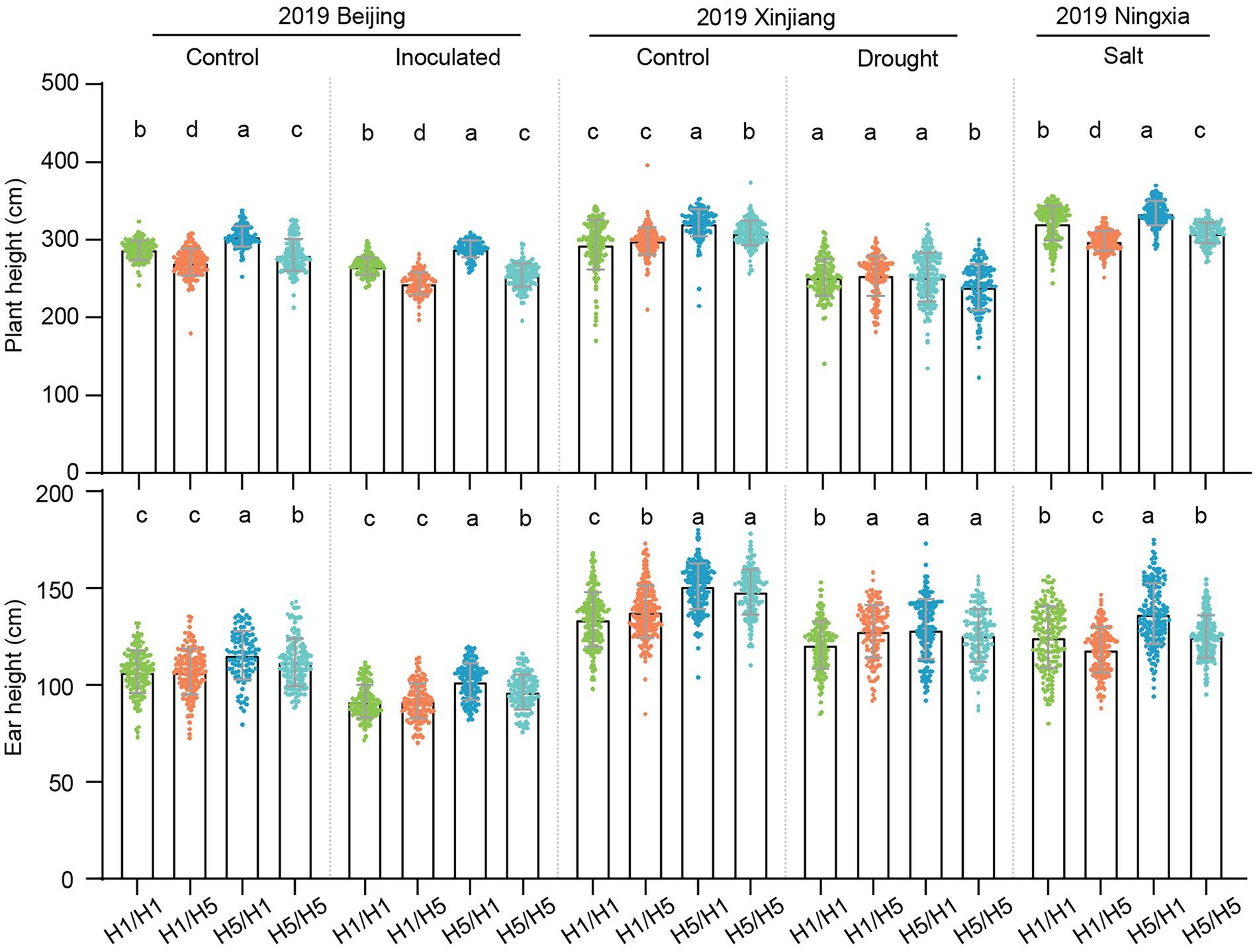
Figure 7. Plant architecture traits of hybrids in 2019 under control, inoculated, drought, and salt conditions. H1/H1, H1/H5, H5/H1, and H5/H5 are genotypes of four hybrids representing 83B28H1 × A5302H1, 83B28H1 × A5302H5, 83B28H5 × A5302H1, and 83B28H5 × A5302H5, respectively. Values are mean ± standard deviation (s.d.). Different letters indicate significant differences (p < 0.05, one-way ANOVA, Tamhane’s test, and Duncan’s test).
Discussion
CCT domain genes are associated with crop yield
Increasing the resilience of crops is important in light of the changing climate (Juliana et al., 2019). Plant disease resistance usually compromises plant fitness (Brown, 2002; Yang et al., 2012; Li et al., 2016). The pleiotropic effects of ZmCCT on disease resistance and delayed flowering make it possible to have no yield penalty. We previously examined the performance of the progeny of a cross between 83B28H5/H1 and A5302H4/H1, whose F1 hybrids could be divided into non-TE (H4 and H5), TE (H1), and heterozygous genotypes at ZmCCT (Li et al., 2017b). This experiment did not clearly explain the genetic effects of H5 or H4, and the test was only conducted for 1 year. In the current study, to explore the use of H5 for maize resistance breeding, we obtained four hybrids by single crossing among two pairs of NILs: 83B28H1/83B28H5 and A5302H1/A5302H5. The use of four hybrids allowed us to clearly observe the specific effects of different combinations of alleles under various conditions. The DSI values were significantly lower for 83B28H5 × A5302H1 and 83B28H5 × A5302H5 compared to the other two hybrids. Moreover, 83B28H5 × A5302H1 had significantly higher KWPE than the other three hybrids under both normal and infected conditions. In Xinjiang, 83B28H5 × A5302H1 and 83B28H5 × A5302H5 had higher KWPE values than the other two hybrids under well-watered conditions, while 83B28H5 × A5302H1 had no yield penalty compared to 83B28H1 × A5302H1 under drought stress. Overall, 83B28H5 × A5302H1 showed the best performance among the four hybrids.
ZmCCT expression is induced in roots in response to F. graminearum, confers stalk-rot resistance, and exhibits photoperiod sensitivity in leaves to delay flowering time (Yang et al., 2013; Wang et al., 2017). We propose that ZmCCT has different inducers in different tissues (leaves or roots), similar to the transcription factor gene IPA1. This may partly explain why the haplotype H5 has no yield penalty compared to other disease resistance genes, or in some cases (like 83B28H5 × A5302H1) even increases yield, like IPA1 (Wang et al., 2018). The haplotype H5 was slightly sensitive to photoperiod in the 83B28 and A5302 backgrounds; the underlying mechanism deserves further investigation. In wheat, the CCT domain gene TaCol-B5 also has pleiotropic effects: It increases the number of spikelet nodes per spike, as well as the number of tillers and spikes, leading to increased grain yield (Zhang et al., 2022). CCT domain genes have obvious convergent effects in rice (Ghd7), maize (ZmCCT), and wheat (TaCol-B5), all of which are pleiotropic genes that regulate plant growth, development, stress responses, yield performance, and other agronomic traits (Xue et al., 2008; Weng et al., 2014; Zhang et al., 2022).
83B28H5 × A5302H1 and 83B28H1 × A5302H5 show opposite effects
Among the four hybrids, 83B28H5 × A5302H1 had better KWPE values than the others under both pathogen-infected and drought conditions, while 83B28H1 × A5302H5 showed better performance under salt stress conditions. In all circumstances, the effects of 83B28H5 × A5302H1 on yield-related traits were opposite those of 83B28H1 × A5302H5. Whereas 83B28H5 × A5302H1 tended to show higher EL and KNPR values, 83B28H1 × A5302H5 tended to show higher KRN. In addition, the plant architecture traits of 83B28H5 × A5302H1 were opposite those of 83B28H1 × A5302H5. Compared to 83B28H1 × A5302H1, 83B28H5 × A5302H1 showed significantly greater PH, while 83B28H1 × A5302H5 generally showed lower PH. The flowering time of 83B28H5 × A5302H1 was significantly delayed in Beijing in 2020 and 2021, whereas that of 83B28H1 × A5302H5 was not. Why do these two hybrids exhibit such different characteristics even though they contain the same single haplotype H5? A recent study indicated that maternal and cytoplasmic effects influence multiple traits in durum wheat, such as resistance to Zymoseptoria tritici, plant height, and thousand kernel weight (Hassine et al., 2022). Therefore, perhaps, the haplotype H5 has maternal effects on the hybrids.
The relationships of flowering time, plant height, and yield
Alleles that delay flowering time generally increase plant height, such as Ghd7 and DTH8 (Days to heading 8) in rice (Xue et al., 2008; Wei et al., 2010), gmap1 (homozygous quadruple mutant of APETALA 1) in soybean (Chen et al., 2020), and lfy1 (leafy 1), ZmCCT9, and ZmMADS69 (MADS-box transcription factor) in maize (Cui et al., 2017; Huang et al., 2018; Liang et al., 2019). In some cases, alleles that increase plant height also increase yield, as in Ghd7 and OsMPH1 (MYB-like gene of plant height 1; Zhang et al., 2017). It is reasonable to associate a longer vegetative stage with more biomass: The taller the plant, the higher the grain yield. However, in other cases, such as zmm28 (AP1-FUL-Like MADS-Box Gene) in maize, increased yield is independent of plant height (Wu et al., 2019). In a study of 280 genotypic combinations within the same population, earlier flowering times were generally associated with reduced plant height and ear weight, although 11 optimal genotypic combinations were also identified (Xiao et al., 2021). In the current study, 83B28H5 × A5302H1 showed delayed flowering time under long-day conditions and increased PH and KWPE under all conditions except salt stress. These traits conferred by the haplotype H5 must be intrinsically related.
The haplotype H5 of ZmCCT is a versatile maize resistance gene
In summary, the hybrid 83B28H5 × A5302H1 with a single haplotype H5 showed an ~2-day delay in flowering time, increased stalk-rot resistance, and improved yield and yield-related traits under both biotic and abiotic stress conditions. From the perspective of maize breeding, an ~2-day delay in flowering time has little impact on farmers, but the yield and resilience of this crop under different conditions are important. Therefore, the haplotype H5 is valuable due to its ability to optimize the trade-off between stress resistance and plant growth. However, the haplotype H5 did not lead to better performance under salt stress, reminding us of the limits of using the haplotype H5 in maize breeding.
In the current study, we also generated four hybrids from the cross between Zheng58H1/Zheng58H5 and Chang7-2H1/Chang7-2H5. However, the hybrids with the haplotype H5 exhibited much stronger photoperiod sensitivity than their parental lines and were therefore excluded from further study. While conducting this study, the haplotype H5 was introgressed into a number of elite maize inbred lines in China. The stalk-rot resistance of these lines greatly improved, and grain yield routinely increased by 5%–10%. For example, the original maize variety MC812 (developed by the Beijing Academy of Agricultural and Forestry Sciences) had a diseased plant rate of 19.25% and a yield of 12,936 kg per hectare, in contrast to the improved MC812, with a diseased plant rate of 1.79% and a yield of 13,818 kg per hectare. In conclusion, the haplotype H5 will be quite valuable for maize breeding for stalk-rot resistance and improved grain yield. This is a good example to exploit natural genetic variation to uncouple growth and defense trade-offs (He et al., 2022).
Data availability statement
The original contributions presented in the study are included in the article/Supplementary material, further inquiries can be directed to the corresponding author.
Author contributions
MX and LT designed the study, analyzed, and interpreted the data, and wrote and revised the manuscript. LT conducted the experiments. MY, MZ, and JY participated in field experiments and partial traits data collection. MZ helped in data analysis and data visualization. YL provided the seed resources. MX supervised the project. All authors contributed to the article and approved the submitted version.
Funding
This study was supported by Jiangsu province’s Seed Industry Revitalization project [JBGS(2021)002] and Yunnan Provincial Science and Technology Department (202005AF150026).
Acknowledgments
We extend our thanks to the Crop Functional Genomics and Molecular Breeding Center of China Agricultural University for their strong support in the field trial of salt tolerance.
Conflict of interest
The authors declare that the research was conducted in the absence of any commercial or financial relationships that could be construed as a potential conflict of interest.
Publisher’s note
All claims expressed in this article are solely those of the authors and do not necessarily represent those of their affiliated organizations, or those of the publisher, the editors and the reviewers. Any product that may be evaluated in this article, or claim that may be made by its manufacturer, is not guaranteed or endorsed by the publisher.
Supplementary material
The supplementary material for this article can be found online at: https://www.frontiersin.org/articles/10.3389/fpls.2022.984527/full#supplementary-material
References
Brown, J. K. (2002). Yield penalties of disease resistance in crops. Curr. Opin. Plant Biol. 5, 339–344. doi: 10.1016/s1369-5266(02)00270-4
Brown, J. K. M., and Rant, J. C. (2013). Fitness costs and trade-offs of disease resistance and their consequences for breeding arable crops. Plant Pathol. 62, 83–95. doi: 10.1111/ppa.12163
Büschges, R., Hollricher, K., Panstruga, R., Simons, G., Wolter, M., Frijters, A., et al. (1997). The barley Mlo gene: a novel control element of plant pathogen resistance. Cell 88, 695–705. doi: 10.1016/s0092-8674(00)81912-1
Chen, L., Nan, H., Kong, L., Yue, L., Yang, H., Zhao, Q., et al. (2020). Soybean AP1 homologs control flowering time and plant height. J. Integr. Plant Biol. 62, 1868–1879. doi: 10.1111/jipb.12988
Consonni, C., Humphry, M. E., Hartmann, H. A., Livaja, M., Durner, J., Westphal, L., et al. (2006). Conserved requirement for a plant host cell protein in powdery mildew pathogenesis. Nat. Genet. 38, 716–720. doi: 10.1038/ng1806
Cui, M., Jia, B., Liu, H., Kan, X., Zhang, Y., Zhou, R., et al. (2017). Genetic mapping of the leaf number above the primary ear and its relationship with plant height and flowering time in maize. Front. Plant Sci. 8:1437. doi: 10.3389/fpls.2017.01437
Deng, Y., Zhai, K., Xie, Z., Yang, D., Zhu, X., Liu, J., et al. (2017). Epigenetic regulation of antagonistic receptors confers rice blast resistance with yield balance. Science 355, 962–965. doi: 10.1126/science.aai8898
Hassine, M., Bnejdi, F., Bahri, B. A., Tissaoui, S., Mougou-Hamdane, A., Guesmi, M., et al. (2022). Detection of maternal and cytoplasmic effects on resistance to Zymoseptoria tritici in durum wheat. Biomed. Res. Int. 2022, 8497417–8497416. doi: 10.1155/2022/8497417
He, Z., Webster, S., and He, S. Y. (2022). Growth-defense trade-offs in plants. Curr. Biol. 32, R634–R639. doi: 10.1016/j.cub.2022.04.070
Huang, C., Sun, H., Xu, D., Chen, Q., Liang, Y., Wang, X., et al. (2018). ZmCCT9 enhances maize adaptation to higher latitudes. Proc. Natl. Acad. Sci. U. S. A. 115, E334–E341. doi: 10.1073/pnas.1718058115
Hung, H. Y., Shannon, L. M., Tian, F., Bradbury, P. J., Chen, C., Flint-Garcia, S. A., et al. (2012). ZmCCT and the genetic basis of day-length adaptation underlying the postdomestication spread of maize. Proc. Natl. Acad. Sci. U. S. A. 109, E1913–E1921. doi: 10.1073/pnas.1203189109
Juliana, P., Poland, J., Huerta-Espino, J., Shrestha, S., Crossa, J., Crespo-Herrera, L., et al. (2019). Improving grain yield, stress resilience and quality of bread wheat using large-scale genomics. Nat. Genet. 51, 1530–1539. doi: 10.1038/s41588-019-0496-6
Karasov, T. L., Chae, E., Herman, J. J., and Bergelson, J. (2017). Mechanisms to mitigate the trade-Off between growth and defense. Plant Cell 29, 666–680. doi: 10.1105/tpc.16.00931
Karasov, T. L., Kniskern, J. M., Gao, L., DeYoung, B. J., Ding, J., Dubiella, U., et al. (2014). The long-term maintenance of a resistance polymorphism through diffuse interactions. Nature 512, 436–440. doi: 10.1038/nature13439
Konlasuk, S., Xing, Y. X., Zhang, N., Zuo, W. L., Zhang, B. Q., Tan, G. Q., et al. (2015). ZmWAK, a quantitative resistance gene to head smut in maize, improves yield performance by reducing the endophytic pathogen Sporisorium reiliana. Mol. Breed. 35:174. doi: 10.1007/s11032-015-0325-2
Li, S., Lin, D., Zhang, Y., Deng, M., Chen, Y., Lv, B., et al. (2022). Genome-edited powdery mildew resistance in wheat without growth penalties. Nature 602, 455–460. doi: 10.1038/s41586-022-04395-9
Li, Y., Tong, L., Deng, L., Liu, Q., Xing, Y., Wang, C., et al. (2017b). Evaluation of ZmCCT haplotypes for genetic improvement of maize hybrids. Theor. Appl. Genet. 130, 2587–2600. doi: 10.1007/s00122-017-2978-1
Li, X., Yang, D. L., Sun, L., Li, Q., Mao, B., and He, Z. (2016). The systemic acquired resistance regulator OsNPR1 attenuates growth by repressing Auxin signaling through promoting IAA-Amido synthase expression. Plant Physiol. 172, 546–558. doi: 10.1104/pp.16.00129
Li, W., Zhu, Z., Chern, M., Yin, J., Yang, C., Ran, L., et al. (2017a). A natural allele of a transcription factor in Rice confers broad-Spectrum blast resistance. Cell 170, 114.e15-126.e15. doi: 10.1016/j.cell.2017.06.008
Liang, Y., Liu, Q., Wang, X., Huang, C., Xu, G., Hey, S., et al. (2019). ZmMADS69 functions as a flowering activator through the ZmRap2.7-ZCN8 regulatory module and contributes to maize flowering time adaptation. New Phytol. 221, 2335–2347. doi: 10.1111/nph.15512
Liang, Y., Liu, H. J., Yan, J., and Tian, F. (2021). Natural variation in crops: realized understanding, continuing promise. Annu. Rev. Plant Biol. 72, 357–385. doi: 10.1146/annurev-arplant-080720-090632
Nekrasov, V., Wang, C., Win, J., Lanz, C., Weigel, D., and Kamoun, S. (2017). Rapid generation of a transgene-free powdery mildew resistant tomato by genome deletion. Sci. Rep. 7:482. doi: 10.1038/s41598-017-00578-x
Nelson, R., Wiesner-Hanks, T., Wisser, R., and Balint-Kurti, P. (2018). Navigating complexity to breed disease-resistant crops. Nat. Rev. Genet. 19, 21–33. doi: 10.1038/nrg.2017.82
Solovieff, N., Cotsapas, C., Lee, P. H., Purcell, S. M., and Smoller, J. W. (2013). Pleiotropy in complex traits: challenges and strategies. Nat. Rev. Genet. 14, 483–495. doi: 10.1038/nrg3461
Su, H., Cao, Y., Ku, L., Yao, W., Cao, Y., Ren, Z., et al. (2018). Dual functions of ZmNF-YA3 in photoperiod-dependent flowering and abiotic stress responses in maize. J. Exp. Bot. 69, 5177–5189. doi: 10.1093/jxb/ery299
Tian, D., Traw, M. B., Chen, J. Q., Kreitman, M., and Bergelson, J. (2003). Fitness costs of R-gene-mediated resistance in Arabidopsis thaliana. Nature 423, 74–77. doi: 10.1038/nature01588
Wang, Y., Cheng, X., Shan, Q., Zhang, Y., Liu, J., Gao, C., et al. (2014). Simultaneous editing of three homoeoalleles in hexaploid bread wheat confers heritable resistance to powdery mildew. Nat. Biotechnol. 32, 947–951. doi: 10.1038/nbt.2969
Wang, C., Yang, Q., Wang, W., Li, Y., Guo, Y., Zhang, D., et al. (2017). A transposon-directed epigenetic change in ZmCCT underlies quantitative resistance to Gibberella stalk rot in maize. New Phytol. 215, 1503–1515. doi: 10.1111/nph.14688
Wang, J., Zhou, L., Shi, H., Chern, M., Yu, H., Yi, H., et al. (2018). A single transcription factor promotes both yield and immunity in rice. Science 361, 1026–1028. doi: 10.1126/science.aat7675
Wei, X., Xu, J., Guo, H., Jiang, L., Chen, S., Yu, C., et al. (2010). DTH8 suppresses flowering in rice, influencing plant height and yield potential simultaneously. Plant Physiol. 153, 1747–1758. doi: 10.1104/pp.110.156943
Weng, X., Wang, L., Wang, J., Hu, Y., Du, H., Xu, C., et al. (2014). Grain number, plant height, and heading date 7 is a central regulator of growth, development, and stress response. Plant Physiol. 164, 735–747. doi: 10.1104/pp.113.231308
Wu, J., Lawit, S. J., Weers, B., Sun, J., Mongar, N., Van Hemert, J., et al. (2019). Overexpression of zmm28 increases maize grain yield in the field. Proc. Natl. Acad. Sci. U. S. A. 116, 23850–23858. doi: 10.1073/pnas.1902593116
Xiao, Y., Jiang, S., Cheng, Q., Wang, X., Yan, J., Zhang, R., et al. (2021). The genetic mechanism of heterosis utilization in maize improvement. Genome Biol. 22:148. doi: 10.1186/s13059-021-02370-7
Xu, Z., Hua, J., Wang, F., Cheng, Z., Meng, Q., Chen, Y., et al. (2020). Marker-assisted selection of qMrdd8 to improve maize resistance to rough dwarf disease. Breed. Sci. 70, 183–192. doi: 10.1270/jsbbs.19110
Xu, G., Wang, X., Huang, C., Xu, D., Li, D., Tian, J., et al. (2017). Complex genetic architecture underlies maize tassel domestication. New Phytol. 214, 852–864. doi: 10.1111/nph.14400
Xue, W., Xing, Y., Weng, X., Zhao, Y., Tang, W., Wang, L., et al. (2008). Natural variation in Ghd7 is an important regulator of heading date and yield potential in rice. Nat. Genet. 40, 761–767. doi: 10.1038/ng.143
Yang, Q., Li, Z., Li, W., Ku, L., Wang, C., Ye, J., et al. (2013). CACTA-like transposable element in ZmCCT attenuated photoperiod sensitivity and accelerated the postdomestication spread of maize. Proc. Natl. Acad. Sci. U. S. A. 110, 16969–16974. doi: 10.1073/pnas.1310949110
Yang, D. L., Yao, J., Mei, C. S., Tong, X. H., Zeng, L. J., Li, Q., et al. (2012). Plant hormone jasmonate prioritizes defense over growth by interfering with gibberellin signaling cascade. Proc. Natl. Acad. Sci. U. S. A. 109, E1192–E1200. doi: 10.1073/pnas.1201616109
Yang, Q., Yin, G., Guo, Y., Zhang, D., Chen, S., and Xu, M. (2010). A major QTL for resistance to Gibberella stalk rot in maize. Theor. Appl. Genet. 121, 673–687. doi: 10.1007/s00122-010-1339-0
Zhang, X., Jia, H., Li, T., Wu, J., Nagarajan, R., Lei, L., et al. (2022). TaCol-B5 modifies spike architecture and enhances grain yield in wheat. Science 376, 180–183. doi: 10.1126/science.abm0717
Zhang, Y., Yu, C., Lin, J., Liu, J., Liu, B., Wang, J., et al. (2017). OsMPH1 regulates plant height and improves grain yield in rice. PLoS One 12:e0180825. doi: 10.1371/journal.pone.0180825
Zhang, Z., Zhang, X., Lin, Z., Wang, J., Xu, M., Lai, J., et al. (2018). The genetic architecture of nodal root number in maize. Plant J. 93, 1032–1044. doi: 10.1111/tpj.13828
Zhao, X., Tan, G., Xing, Y., Wei, L., Chao, Q., Zuo, W., et al. (2012). Marker-assisted introgression of qHSR1 to improve maize resistance to head smut. Mol. Breed. 30, 1077–1088. doi: 10.1007/s11032-011-9694-3
Keywords: ZmCCT, hybrids, yield, stalk-rot resistance, drought tolerance
Citation: Tong L, Yan M, Zhu M, Yang J, Li Y and Xu M (2022) ZmCCT haplotype H5 improves yield, stalk-rot resistance, and drought tolerance in maize. Front. Plant Sci. 13:984527. doi: 10.3389/fpls.2022.984527
Edited by:
Zuhua He, Center for Excellence in Molecular Plant Sciences (CAS), ChinaReviewed by:
Jianbing Yan, Huazhong Agricultural University, ChinaJianfeng Weng, Institute of Crop Sciences (CAAS), China
Copyright © 2022 Tong, Yan, Zhu, Yang, Li and Xu. This is an open-access article distributed under the terms of the Creative Commons Attribution License (CC BY). The use, distribution or reproduction in other forums is permitted, provided the original author(s) and the copyright owner(s) are credited and that the original publication in this journal is cited, in accordance with accepted academic practice. No use, distribution or reproduction is permitted which does not comply with these terms.
*Correspondence: Mingliang Xu, bXh1QGNhdS5lZHUuY24=