- 1Graduate School of Science, Nagoya University, Nagoya, Japan
- 2Institute of Transformative Bio-Molecules (WPI-ITbM), Nagoya University, Nagoya, Japan
In plants, cytosolic and extracellular pH homeostasis are crucial for various physiological processes, including the uptake of macronutrients and micronutrients, cell elongation, cell expansion, and enzyme activity. Proton (H+) gradients and the membrane potential are generated by a H+ pump consisting of an active primary transporter. Plasma membrane (PM) H+-ATPase, a PM-localized H+ pump, plays a pivotal role in maintaining pH homeostasis in plant cells and extracellular regions. PM H+-ATPase activity is regulated by protein abundance and by post-translational modifications. Several stimuli have been found to activate the PM H+-ATPase through phosphorylation of the penultimate threonine (Thr) of the carboxy terminus. Light- and photosynthesis-induced phosphorylation of PM H+-ATPase are conserved phenomena among various plant species. In this work, we review recent findings related to PM H+-ATPase regulation in the photosynthetic tissues of plants, focusing on its mechanisms and physiological roles. The physiological roles of photosynthesis-dependent PM H+-ATPase activation are discussed in the context of nitrate uptake and cytoplasmic streaming in leaves.
Introduction
During photosynthesis plants capture energy from sunlight and convert carbon dioxide, into carbohydrate. Photosynthesis is a crucial physiological process for plant cells; the illumination of plant leaves induces numerous photosynthesis-dependent signaling pathways and maintains photosynthetic performance. Plant cell pH homeostasis is essential for various photosynthesis-induced physiological processes in plastids, as well as nutrient uptake via secondary transporters and channels across membranes. Plastidial pH homeostasis was recently reviewed by Trinh and Masuda (2022). Plant macronutrient availability greatly influences photosynthesis (Hester and Mendelssohn, 1990; Grossman and Takahashi, 2001). Therefore, pH homeostasis in plant cells promotes nutrient acquisition for both plant growth and the maintenance of photosynthesis.
Protons (H+) are translocated across the plasma membrane (PM) by PM H+-ATPase using energy provided by ATP hydrolysis. Many of the physiological roles of PM H+-ATPase in plants have been intensively studied such as stomatal opening in guard cells (Inoue and Kinoshita, 2017), seedling hypocotyl elongation (Hager, 2003), root elongation (Haruta et al., 2018), nutrient uptake by roots (Sondergaard et al., 2004), flower pollen tube growth (Hayashi and Palmgren, 2021), sugar loading in phloem sieve elements (DeWitt and Sussman, 1995), and seed dormancy alleviation (de Bont et al., 2019; Figure 1). The spatial and temporal regulation of PM H+-ATPase activity is critical for these physiological process. PM H+-ATPase activity is regulated by protein transcription and translation, as well as post-translational modification. Several environmental stimuli induce the phosphorylation of the penultimate threonine (Thr) on the carboxy terminus of PM H+-ATPase and subsequent binding of 14-3-3 protein to the region activate H+ pumping. The regulation of PM H+-ATPase activity by environmental stimuli and phytohormones was reviewed by Fuglsang and Palmgren (2021) and Miao et al. (2022); however, that of PM H+-ATPase activity in photosynthetic tissues is an emerging subject (Harada et al., 2002a; Okumura et al., 2012a,b). Therefore, we summarize recent findings regarding the regulatory mechanisms of PM H+-ATPase and its physiological roles in photosynthetic tissues.
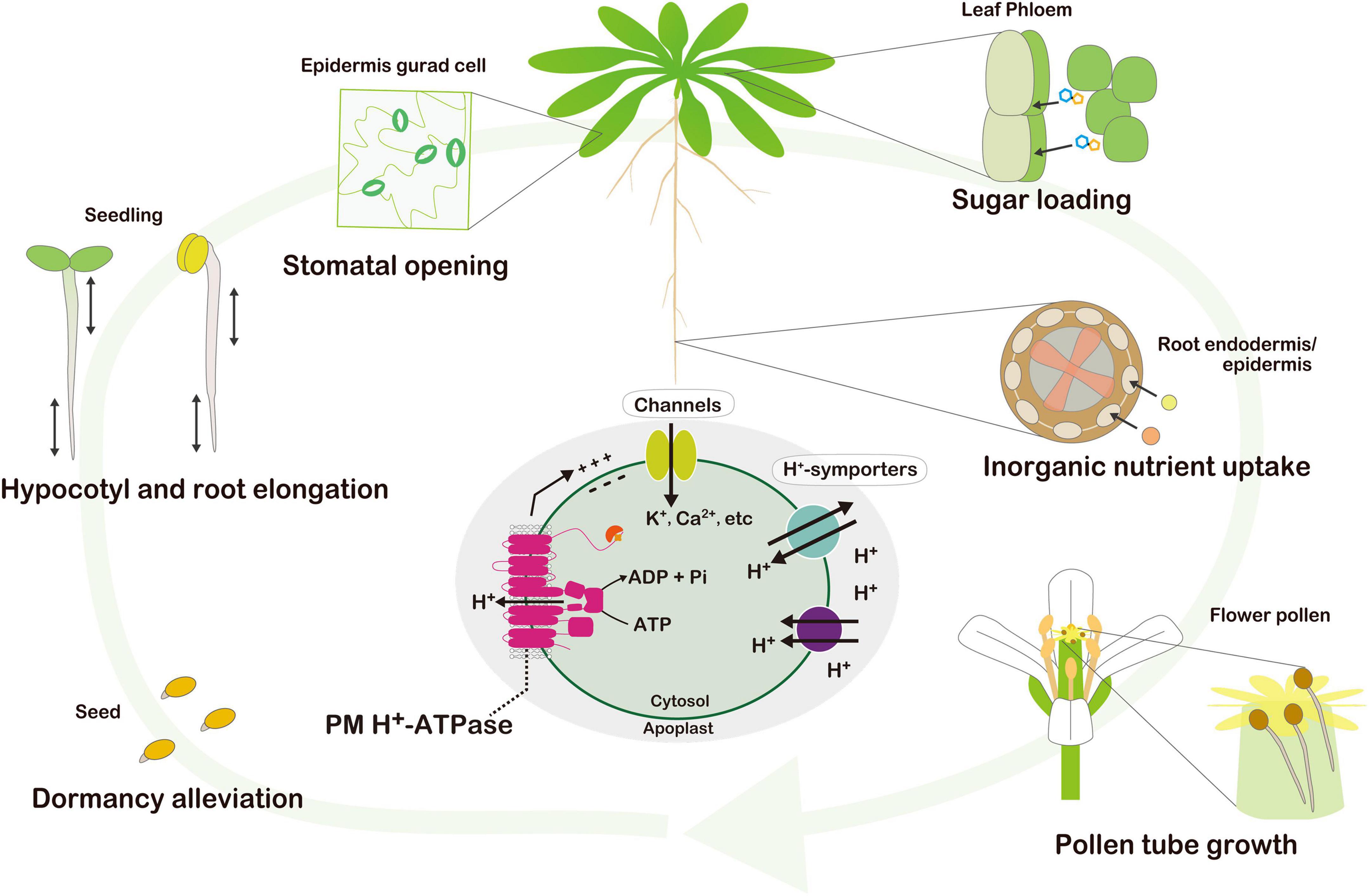
Figure 1. Physiological consequence of the PM H+-ATPase throughout the life of the plant. Seed dormancy alleviation, seedling hypocotyl and root elongation, stomatal guard cell opening, nutrient uptake in the root epidermis, sugar loading in phloem sieve elements, and flower pollen tube growth have been demonstrated to date. Active H+ pumping by PM H+-ATPase energizes the PM. Hyperpolarization and an H+ gradient across the PM, generated by H+ pumping, activate voltage-dependent channels and H+ symporters for substrate uptake.
Photosynthesis-driven PM H+-ATPase activation via phosphorylation and its roles
Photosynthesis-dependent activation of PM H+-ATPase in divergent plant species and tissues
Photosynthetically active radiation on photosynthetic tissues such as the thallus of Marchatia polymorpha, protonema of the moss Physcomitrella, and leaves of Arabidopsis, Vallisneria, rice, tobacco, and fava bean induces phosphorylation of the penultimate Thr of PM H+-ATPase (Okumura et al., 2012a,b, 2016; Harada et al., 2020). Two inhibitors of electron transport reactions in photosynthesis, 3-(3,4-dichlorophenyl)-1,1-dimethylurea (DCMU) and 2,5-dibromo-3-methyl-6-isopropyl-p-benzoquinone (DBMIB), have been shown to interrupt the light-induced phosphorylation of PM H+-ATPase (Okumura et al., 2012a,2016). Exogenous sugar supplementation to tissues in the dark induces the phosphorylation of PM H+-ATPase (Okumura et al., 2016). These results demonstrate that photosynthetic products are responsible for the activation of PM H+-ATPase via phosphorylation. Sucrose supplementation to Arabidopsis seedlings induces phosphorylation of the penultimate Thr of PM H+-ATPase, suggesting that the photosynthetic product-dependent phosphorylation of PM H+-ATPase is not limited to leaves (Niittylä et al., 2007).
Involvement of glycolysis and downstream metabolism in PM H+-ATPase phosphorylation regulation
Kinoshita et al. (2022) recently reported that glycolysis inhibition by a glucose analog, 2-deoxy glucose, suppresses the photosynthetic product-induced phosphorylation of PM H+-ATPase in Arabidopsis leaves, suggesting the involvement of glycolysis and downstream metabolism. As cytosolic ATP and NAD(P)H are end-products of glycolysis, respiration, and photosynthesis, it raised a question: whether the glycolysis and downstream metabolites are the direct stimuli on PM H+-ATPase, or the end-products are the key stimuli. Recent advance in biosensors of ATP/NADPH and a study using inducible silencing of specific metabolic enzyme may reveal an interesting connection between photosynthesis and glycolysis or respiration related to PM H+-ATPase regulation in leaves.
In addition, it is interesting to note that carbon availability and intact glycolysis are responsible for PM H+-ATPase activation in yeast (Mazón et al., 2015). Although the regulatory carboxy-terminus of PM H+-ATPase in yeast is shorter and structurally different compared to plant PM H+-ATPase, the response to carbon availability in cell is conserved phenomena. Taken together with the case of plant PM H+-ATPase activation in leaves, it would be more interesting to investigate what molecular components regulate PM H+-ATPase in response to carbon availability in cell, using both yeast and plants as the model.
Physiological significance of PM H+-ATPase in illuminated leaves
PM H+-ATPase activation may increase nitrate uptake in leaves
Maintaining the H+ gradient and membrane potential across the PM is essential for macronutrient and micronutrient uptake because numerous PM-localizing H+ symporter and voltage-dependent channels are involved in nutrient uptake from extracellular regions. The involvement of PM H+-ATPase in nutrient uptake was reviewed by Sondergaard et al. (2004). A mathematical model of the membrane transporter system, including PM H+-ATPase, was developed for the simulation of active coupling of H+ symporters and the H+ pump (Dreyer, 2021).
The putative physiological roles of photosynthesis-dependent PM H+-ATPase activation may be summarized by focusing on the relationship between PM H+-ATPase and macronutrient nitrate in leaves. The molecular mechanism of nitrate uptake in roots has been well studied because this process is the greatest limitation on plant nitrate acquisition (Xu et al., 2012). In Arabidopsis, concentrated nitrate is translocated from roots to the leaf apoplast via the xylem stream (Dechorgnat et al., 2011). However, nitrate import and translocation to leaves are important limitations on plant shoot growth (Chiu et al., 2004; Hsu and Tsay, 2013). Photosynthetic tissues generally invest large amounts of nitrogen in the synthesis of photosynthesis-related proteins, including rubisco, a light-harvesting complex (Evans and Clarke, 2019). Nitrate reduction in illuminated leaves is the key driving force for nitrate uptake to mesophyll cells (Cookson et al., 2005). Thus, mesophyll cells may require active nitrate uptake from the apoplast to compensate nitrogen availability during photosynthesis. Light-dependent nitrate uptake in roots and leaves has been suggested in soybean (Delhon et al., 1995). The involvement of the PM membrane potential in light-dependent nitrate uptake has been demonstrated using microelectrode recordings in Arabidopsis leaves (Cookson et al., 2005). Disks from nitrogen-starved cucumber leaves showed pH- and light-dependent increases in nitrate content, suggesting the involvement of PM H+-ATPase (Nikolic et al., 2012). Light- and PM H+-ATPase activation-induced nitrate uptake was also recently reported in Arabidopsis leaves grown on soil without nitrate starvation (Kinoshita et al., 2022).
Leaf nitrogen availability greatly influences photosynthetic activity (Makino, 2011). Therefore, we speculate that photosynthesis activates PM H+-ATPase to compensate the nitrate pool, which is substantially reduced and acclimated during light illumination (Figure 2). Future studies should track nitrate flux within the cell to improve our understanding of the positive relationship between photosynthesis and PM H+-ATPase.
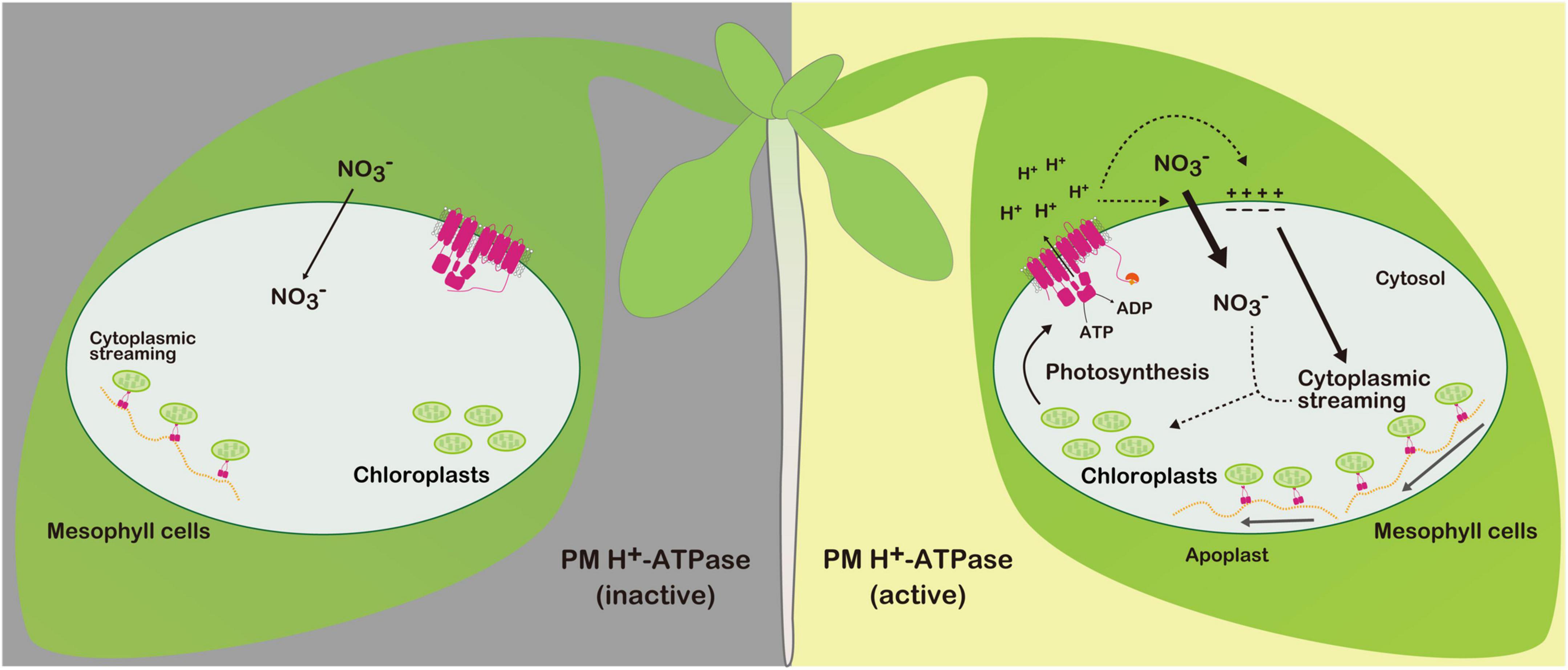
Figure 2. Photosynthesis-induced PM H+-ATPase activation and its physiological roles in mesophyll cells. Photosynthesis activates PM H+-ATPase via phosphorylation of the penultimate threonine (Thr). Activated PM H+-ATPase increases nitrate influx and cytoplasmic streaming under light illumination. Efficient nitrate influx and redistribution of chloroplast via cytoplasmic streaming by organelle-associated actin/myosin system may support photosynthesis. Under overnight dark conditions, the penultimate Thr of PM H+-ATPase is dephosphorylated, thereby inactivating PM H+-ATPase.
PM H+-ATPase activation may increase cytoplasmic streaming
Because photosynthesis-dependent PM H+-ATPase activation is a conserved phenomenon across numerous plant species, it is valuable to dissect the physiological roles of PM H+-ATPase in photosynthetic tissues using different types of plant species. For example, mesophyll cells of the aquatic monocot Vallisneria show cytoplasmic streaming and active H+ pumping under red light illumination (Harada et al., 2002a,b). Subsequently, phosphorylation of the penultimate Thr of PM H+-ATPase was confirmed to be responsible for light-induced H+ pumping, and cytoplasmic streaming was revealed to be dependent on the H+ pumping (Harada et al., 2020). Cytoplasmic streaming is thought to be generated by association of myosin/actin system with organelle (Shimmen and Yokota, 2004). Modified cytoplasmic streaming via introducing improved myosin XI into Arabidopsis has been suggested to contribute to regulating cell size (Tominaga and Ito, 2015), which implies that constant redistribution of chloroplasts via light-induced cytoplasmic streaming contributes to cell growth by facilitating photosynthesis (Figure 2). Considering that PM H+-ATPase activity is also important for cell elongation and expansion in various plant species, it would be interesting to investigate the evolution of the mechanism by which photosynthesis controls divergent physiologies through the regulation of PM H+-ATPase activity.
Conclusions and perspectives
In this review, the relationship between photosynthesis and PM H+-ATPase was discussed in the context of the positive roles of active PM H+-ATPase in mesophyll cells. Photosynthesis energizes the PM, and therefore may activate nitrate uptake and cytoplasmic streaming to maintain efficient photosynthesis (Figure 2).
Macronutrient and micronutrient availability (i.e., nitrogen, phosphorus, potassium, and magnesium) greatly affects photosynthesis activity (Makino, 2011; Tränkner et al., 2018; Sitko et al., 2019). The uptake of these molecules may be facilitated by the H+ gradient or membrane potential. Thus, the physiological roles of photosynthesis-dependent PM H+-ATPase in macronutrient and micronutrient uptake should be further investigated in the context of a win-win scenario for photosynthesis and the H+ pump. Notably, the H+ pump, photosynthesis, nutrient acquisition, and cytoplasmic streaming are conserved in numerous plant species. Therefore, further investigation of the molecular mechanisms underlying these conserved phenomena will open new avenues for the elucidation of these evolutionary questions.
Author contributions
SNK and TK designed the concept. SNK wrote the manuscript and drew the figures. Both authors reviewed and approved the final version of the manuscript.
Funding
This work was supported by a Japan Society for the Promotion of Science (JSPS) grant-in-aid to SNK (Research Fellow No. 19J20450) and the Ministry of Education, Culture, Sports, Science and Technology (MEXT) grants-in-aid for scientific research to TK (Nos. 20H05687 and 20H05910).
Acknowledgments
We thank Koji Takahashi, Shin-ichiro Inoue, and Yuki Hayashi (Nagoya University) for providing their generous support.
Conflict of interest
The authors declare that the research was conducted in the absence of any commercial or financial relationships that could be construed as a potential conflict of interest.
Publisher’s note
All claims expressed in this article are solely those of the authors and do not necessarily represent those of their affiliated organizations, or those of the publisher, the editors and the reviewers. Any product that may be evaluated in this article, or claim that may be made by its manufacturer, is not guaranteed or endorsed by the publisher.
References
Chiu, C. C., Lin, C. S., Hsia, A. P., Su, R. C., Lin, H. L., and Tsay, Y. F. (2004). Mutation of a nitrate transporter, AtNRT1:4, results in a reduced petiole nitrate content and altered leaf development. Plant Cell Physiol. 45, 1139–1148. doi: 10.1093/pcp/pch143
Cookson, S. J., Williams, L. E., and Miller, A. J. (2005). Light–dark changes in cytosolic nitrate pools depend on nitrate reductase activity in Arabidopsis leaf cells. Plant Physiol. 138, 1097–1105. doi: 10.1104/pp.105.062349
de Bont, L., Naim, E., Arbelet-Bonnin, D., Xia, Q., Palm, E., Meimoun, P., et al. (2019). Activation of plasma membrane H+-ATPases participates in dormancy alleviation in sunflower seeds. Plant Sci. 280, 408–415. doi: 10.1016/j.plantsci.2018.12.015
Dechorgnat, J., Nguyen, C. T., Armengaud, P., Jossier, M., Diatloff, E., Filleur, S., et al. (2011). From the soil to the seeds: The long journey of nitrate in plants. J. Exp. Bot. 62, 1349–1359. doi: 10.1093/jxb/erq409
Delhon, P., Gojon, A., Tillard, P., and Passama, L. (1995). Diurnal regulation of NO3 uptake in soybean plants I. Changes in NO3 influx, efflux, and N utilization in the plant during the day/night cycle. J. Exp. Bot. 46, 1585–1594.
DeWitt, N. D., and Sussman, M. R. (1995). Immunocytological localization of an epitope-tagged plasma membrane proton pump (H+-ATPase) in phloem companion cells. Plant Cell 7, 2053–2067. doi: 10.1105/tpc.7.12.2053
Dreyer, I. (2021). Nutrient cycling is an important mechanism for homeostasis in plant cells. Plant Physiol. 187, 2246–2261.
Fuglsang, A. T., and Palmgren, M. (2021). Proton and calcium pumping P-type ATPases and their regulation of plant responses to the environment. Plant Physiol. 187, 1856–1875.
Grossman, A., and Takahashi, H. (2001). Macronutrient utilization by photosynthetic eukaryotes and the fabric of interactions. Annu. Rev. Plant Physiol. Plant Mol. Biol. 52, 163–210. doi: 10.1146/annurev.arplant.52.1.163
Hager, A. (2003). Role of the plasma membrane H+-ATPase in auxin-induced elongation growth: Historical and new aspects. J. Plant Res. 116, 483–505. doi: 10.1007/s10265-003-0110-x
Harada, A., Okazaki, Y., and Takagi, S. (2002a). Photosynthetic control of the plasma membrane H+-ATPase in Vallisneria leaves. I. Regulation of activity during light-induced membrane hyperpolarization. Planta 214, 863–869. doi: 10.1007/s00425-001-0689-x
Harada, A., Fukuhara, T., and Takagi, S. (2002b). Photosynthetic control of the plasma membrane H+-ATPase in Vallisneria leaves. II. Presence of putative isogenes and a protein equipped with a C-terminal autoinhibitory domain. Planta 214, 870–876. doi: 10.1007/s00425-001-0690-4
Harada, A., Okazaki, Y., Kinoshita, T., Nagai, R., and Takagi, S. (2020). Role of proton motive force in photoinduction of cytoplasmic streaming in Vallisneria mesophyll cells. Plants 9:376. doi: 10.3390/plants9030376
Haruta, M., Tan, L. X., Bushey, D. B., Swanson, S. J., and Sussman, M. R. (2018). Environmental and genetic factors regulating localization of the plant plasma membrane H+-ATPase. Plant Physiol. 176, 364–377.
Hayashi, M., and Palmgren, M. (2021). The quest for the central players governing pollen tube growth and guidance. Plant Physiol. 185, 682–693. doi: 10.1093/plphys/kiaa092
Hester, M. W., and Mendelssohn, I. A. (1990). Effects of macronutrient and micronutrient additions on photosynthesis, growth parameters, and leaf nutrient concentrations of Uniola paniculata and Panicum amarum. Bot. Gaz. 151, 21–29.
Hsu, P. K., and Tsay, Y. F. (2013). Two phloem nitrate transporters, NRT1.11 and NRT1.12, are important for redistributing xylem-borne nitrate to enhance plant growth. Plant Physiol. 163, 844–856. doi: 10.1104/pp.113.226563
Inoue, S., and Kinoshita, T. (2017). Blue light regulation of stomatal opening and the plasma membrane H+-ATPase. Plant Physiol. 174, 531–538.
Kinoshita, N. S., Suzuki, T., Kiba, T., Sakakibara, H., and Kinoshita, T. (2022). Photosynthetic-product-dependent activation of plasma membrane H+-ATPase and nitrate uptake in Arabidopsis leaves. bioRxiv [Preprint]. doi: 10.1101/2022.06.30.498222
Makino, A. (2011). Photosynthesis, grain yield, and nitrogen utilization in rice and wheat. Plant Physiol. 155, 125–129. doi: 10.1104/pp.110.165076
Mazón, M. J., Eraso, P., and Portillo, F. (2015). Specific phosphoantibodies reveal two phosphorylation sites in yeast Pma1 in response to glucose. FEMS Yeast Res. 15:fov030. doi: 10.1093/femsyr/fov030
Miao, R., Russinova, E., and Rodriguez, P. L. (2022). Tripartite hormonal regulation of plasma membrane H+-ATPase activity. Trends Plant Sci. 27, 588–600. doi: 10.1016/j.tplants.2021.12.011
Niittylä, T., Fuglsang, A. T., Palmgren, M. G., Frommer, W. B., and Schulze, W. X. (2007). Temporal analysis of sucrose-induced phosphorylation changes in plasma membrane proteins of Arabidopsis. Mol. Cell. Proteomics 6, 1711–1726. doi: 10.1074/mcp.M700164-MCP200
Nikolic, M., Cesco, S., Monte, R., Tomasi, N., Gottardi, S., Zamboni, A., et al. (2012). Nitrate transport in cucumber leaves is an inducible process involving an increase in plasma membrane H+-ATPase activity and abundance. BMC Plant Biol. 12:66. doi: 10.1186/1471-2229-12-66
Okumura, M., Inoue, S., Kuwata, K., and Kinoshita, T. (2016). Photosynthesis activates plasma membrane H+-ATPase via sugar accumulation. Plant Physiol. 171, 580–589. doi: 10.1104/pp.16.00355
Okumura, M., Inoue, S., Takahashi, K., Ishizaki, K., Kohchi, T., and Kinoshita, T. (2012a). Characterization of the plasma membrane H+-ATPase in the liverwort Marchantia polymorpha. Plant Physiol. 159, 826–834.
Okumura, M., Takahashi, K., Inoue, S., and Kinoshita, T. (2012b). Evolutionary appearance of the plasma membrane H+-ATPase containing a penultimate threonine in the bryophyte. Plant Signal. Behav. 7, 7–11. doi: 10.4161/psb.20936
Shimmen, T., and Yokota, E. (2004). Cytoplasmic streaming in plants. Curr. Opin. Cell Biol. 16, 68–72.
Sitko, K., Gieroń, Ż., Szopiński, M., Zieleźnik-Rusinowska, P., Rusinowski, S., Pogrzeba, M., et al. (2019). Influence of short-term macronutrient deprivation in maize on photosynthetic characteristics, transpiration and pigment content. Sci. Rep. 9:14181. doi: 10.1038/s41598-019-50579-1
Sondergaard, T. E., Schulz, A., and Palmgren, M. G. (2004). Energization of transport processes in plants. Roles of the plasma membrane H+-ATPase. Plant Physiol. 136, 2475–2482.
Tominaga, M., and Ito, K. (2015). The molecular mechanism and physiological role of cytoplasmic streaming. Curr. Opin. Plant Biol. 27, 104–110.
Tränkner, M., Tavakol, E., and Jákli, B. (2018). Functioning of potassium and magnesium in photosynthesis, photosynthate translocation and photoprotection. Physiol. Plant. 163, 414–431. doi: 10.1111/ppl.12747
Trinh, M. D. L., and Masuda, S. (2022). Chloroplast pH homeostasis for the regulation of photosynthesis. Front. Plant Sci. 13:919896. doi: 10.3389/fpls.2022.919896
Keywords: leaves, pH homeostasis, photosynthesis, PM H+-ATPase, proton pump, transporters
Citation: Kinoshita SN and Kinoshita T (2022) A win-win scenario for photosynthesis and the plasma membrane H+ pump. Front. Plant Sci. 13:982485. doi: 10.3389/fpls.2022.982485
Received: 30 June 2022; Accepted: 28 July 2022;
Published: 12 August 2022.
Edited by:
Paul F. Devlin, University of London, United KingdomReviewed by:
Pedro Rodriguez, Spanish National Research Council (CSIC), SpainCopyright © 2022 Kinoshita and Kinoshita. This is an open-access article distributed under the terms of the Creative Commons Attribution License (CC BY). The use, distribution or reproduction in other forums is permitted, provided the original author(s) and the copyright owner(s) are credited and that the original publication in this journal is cited, in accordance with accepted academic practice. No use, distribution or reproduction is permitted which does not comply with these terms.
*Correspondence: Satoru N. Kinoshita, kinoshita.satoru.r8@s.mail.nagoya-u.ac.jp; Toshinori Kinoshita, kinoshita@bio.nagoya-u.ac.jp