- 1Dr. Muhammad Ajmal Khan Institute of Sustainable Halophyte Utilization, University of Karachi, Karachi, Pakistan
- 2Institute of Plant Ecology, Research Centre for Bio Systems, Land Use, and Nutrition (IFZ), Justus-Liebig-University Giessen, Giessen, Germany
- 3Institute of Applied Microbiology, Research Centre for Bio Systems, Land Use, and Nutrition (IFZ), Justus-Liebig-University Giessen, Giessen, Germany
- 4Department of Biological and Environmental Sciences and Technologies (DiSTeBa), University of Salento, Lecce, Italy
- 5Department of Horticultural Sciences, Faculty of Agriculture and Environment, The Islamia University of Bahawalpur, Bahawalpur, Pakistan
- 6Department of Biology, College of Sciences, Taif University, Taif, Saudi Arabia
- 7Environment and Plant Protection Institute, Chinese Academy of Tropical Agricultural Science (CATAS), Haikou, China
- 8The UWA Institute of Agriculture, The University of Western Australia, Perth, WA, Australia
Growth stimulating bacteria help remediate dry arid soil and plant stress. Here, Pseudomonas sp. and Pantoea sp. we used to study the stress ecology of Hordeum vulgare and the environmental impact of water deficit on soil characteristics, growth, photosynthesis apparatus, mineral acquisition and antioxidiant defense. Plants inoculated with Pseudomonas or Pantoea had significantly higher (about 2 folds) soil carbon flux (soil respiration), chlorophyll levels (18%), net photosynthetic rate (33% in Pantoea and 54% in Pseudomonas), (44%) stomatal conductance than uninoculated plants in stressed conditions. Both bacterial strains improved leaf growth (23-29%) and root development under well-watered conditions but reduced around (25%) root biomass under drought. Plants inoculated with Pseudomonas or Pantoea under drought also increased of about 27% leaf respiration and transpiration (48%) but decreased water use efficiency, photoinhibition (91%), and the risk of oxidative stress (ETR/A) (49%). Drought stress increased most of the studied antioxidant enzymatic activities in the plants inoculated with Pseudomonas or Pantoea, which reduce the membrane damage and protect plants form oxidative defenses. Drought stress increased K+ acquisition around 50% in both shoots inoculated with Pseudomonas or Pantoea relative to non-stressed plants. Plants inoculated with Pseudomonas or Pantoea increased shoot Na+ while root Na+ only increased in plants inoculated with Pseudomonas in stressed conditions. Drought stress increased shoot Mg2+ in plants inoculated with Pseudomonas or Pantoea but did not affect Ca2+ relative to non-stressed plants. Drought stress increased about 70% K+/Na+ ratio only in plants inoculated with Pseudomonas relative to non-stressed plants. Our results indicate that inoculating barley with the studied bacterial strains increases plant biomass and can therefore play a role in the environmental remediation of drylands for food production.
Introduction
Irregular climate changes, the increasing population, and intensive agriculture are directly connected to land degradation and food shortages, inducing extreme weather events and environmental impacts in many countries (Munir et al., 2021). Increasing agricultural production, food security and protecting water reserves are critical for sustainable agriculture and environmental safety. Decreased water supply due to declining rainfall affects biological systems, nutrient supply, and crop productivity (Peña-Gallardo et al., 2019; Tyagi and Pandey, 2022). For example, barley (Hordeum vulgare L.) biomass and grain yield have substantially declined in arid regions. Limited food crop productivity is associated with reduced water and nutrient flux, causing significant economic losses and socio-economic issues (Kour and Yadav, 2022). New emerging agricultural techniques are acquired to overcome land degradation and increase crop biomass production (Pittelkow et al., 2015; Siddiqui et al., 2021). One strategy is to explore endophytic bacteria, which establish a symbiotic relationship with the host plant and synthesis of nutrients that offer favorable conditions to resist water stress in plants (Rahman et al., 2018; Kour and Yadav, 2022).
Microbial biotechnology is a promising approach for increasing edible plant biomass under stress conditions (Cardoso et al., 2018; Goudarzi et al., 2023). Microbial supplementation can significantly promote bioremediation, control phytopathogens, and increase plant physiological performance and productivity on degraded arid lands (Oleńska et al., 2020). Soil microbial supplementation is influenced by root exudates that produce different enzymes and metabolites, nutrient accumulation, and hormone production (Singh and Gupta, 2018; Zayed et al., 2022). Plant growth-promoting bacteria (PGPB) improve growth and can protect plants against biotic and abiotic stresses by producing volatile compounds, siderophores, growth hormones, biological nitrogen fixation, and reducing plant ethylene synthesis (Khatoon et al., 2020; Das et al., 2022). Identifying the signaling pathways governing the associations between plants and different PGPB can play an important role in improving agricultural production sustainably (Wiggins et al., 2022).
Changes in root development or leaf elongation can be modulated under drought stress but are closely associated with leaf metabolic status and growth portioning (Knutzen et al., 2015; Abideen et al., 2021). Plant-available nutrients (K+, Ca2+, Mg2+ and N) and carbon metablosim with water accessibility through osmotic balance are important mechanisms of plants for photosynthesis and leaf metabolites production under drought (Abdelaal et al., 2021b). However, the ecophysiological responses of plants to reach a new homeostasis after PGPB inoculation under water deficit are not well understood. Inoculation with PGPB could facilitate water uptake, protecting leaf desiccation and thus improving turgidity and plant growth (Abdelaal et al., 2021). Microbial inoculation improves plant ion flux and the synthesis and use of organic solutes for osmotic adjustments (Santander et al., 2017). In addition, microbial interactions improve stomatal regulation, leaf water use efficiency, and oxidative stress management (Paneque et al., 2016; Tak et al., 2021). Plants protect photosystem II (PSII) activity by regulating light harvesting mechanisms critical for biomass production (Saccon et al., 2022). Low water availability reduces the potential agricultural uses of arable land. However, soil and plants can be supplemented with beneficial bacteria to increase food production. The suitability of selected microbes depends on soil type, bacterial strain and concentration, plant species, and stress conditions. Plant microbiomes are integrated within the host into single units of evolution called holobionts. The seed endosphere is a little-investigated plant microhabitat, more recently receiving attention for its potential as a reservoir and vector of beneficial microbes (Berg and Raijmakers, 2018; Rahman et al., 2018). Seed endophytes have been detected in many crop plants, including cereals and legumes, and can improve plant growth and ecophysiological parameters (Alibrandi et al., 2018; Liu et al., 2020). In barley, seed endophytes increased plant biomass activities when used as inoculants (Rahman et al., 2018).
The two bacterial strains used in this work (Pantoea sp. “ITS group 2” and Pseudomonas sp. “ITS group 11”) were selected as best candidates among a series of new isolates from barley seeds (Rahman et al., 2018). They were demonstrated to be consistently associated to barley seed across a variety of cultivars and years (Rahman et al., 2018). Taxonomical identification was performed by 16S rRNA gene sequencing and the isolates were characterized at strain level by ITS polymorphism analysis. Due to their superior performance in barley growth promotion and biocontrol activity (Rahman et al., 2018), these two strains were chosen for the current study. Moreover, they showed ability to efficiently colonize barley roots upon seed germination (Rahman et al., 2018). This study investigates the potential of selected endophytes to improve biomass and physiology of barley under drought stress. The selection of bacterial strain and appropriate level in dry soil is the key component of this manuscript. Barley was selected due to its importance as a global staple food and the availability of preliminary data using seed endophytes as PGPB (Rahman et al., 2018). We tested the following hypotheses: 1) Bacterial inoculation enhances soil conditions (soil CO2 flux), barley growth, nutrient acquisition and plant survival under drought stress; 2) Water limitation improves stomatal resistance and photosynthesis by regulating antioxidiant defense in barley with bacterial inoculation.
Material and methods
Surface sterilization of seeds
Seeds were submerged in 70% ethyl alcohol (EtOH) for 5 minutes under gentle manual shaking before rinsing with sterile H2O for 5 minutes under manual shaking. Next, the seeds were immersed in a 1:1 mixture of Danklorix (~2.4% active NaClO) and disinfection solution (1 g Na2CO3, 30 g NaCl, 1.5 g NaOH per L distilled water) for 1 h at 25°C under mechanical shaking (90 rpm). Finally, the seeds were washed with sterile H2O for 10, 20, 30, 40, and 50 minutes at 25°C under mechanical shaking (90 rpm).
Inoculation of surface-sterilized seeds (bio-priming)
There were four treatments: (1) uninoculated, (2) inoculated with Pantoea sp. (ITS Group 2), (3) inoculated with Pseudomonas sp. (ITS Group 11), and (4) inoculated with E. coli. For each treatment, 170 seeds were immersed in ~35 mL of the corresponding bacterial suspension at the following concentrations:
The bacterial suspensions were obtained by diluting an overnight liquid culture (medium: CASO Bouillon) with sterile 0.03 M MgSO4. Seeds of the uninoculated treatment were immersed in 35 mL of sterile 0.03 M MgSO4. Inoculation with E. coli was used as an additional control to account for the possible effects of adding organic biomass.
Bacterial inoculation in soil and growth conditions
Twelve days after sowing of inoculated seed in soil, the pots were inoculated with 1 mL overnight liquid culture (~3 × 109 CFU mL–1) of the corresponding bacterium. Pots of the uninoculated treatment were amended with 1 mL sterile CASO Bouillon. The experiment was conducted in a greenhouse under controlled environmental conditions (average temperature 25 ± 2°C, relative humidity 50%, 16/8 h (light/dark) photoperiod, average daily light integral 200–250 μmol m–2 s–1 which equals to Daily Light Integral (DLI) 14.40 mol m-2 d-1). Seedlings were at 12 days transplanted into plastic tubes (20 cm length, 5 cm diameter) containing sand (50%), clay (30%), and gravel (20%), with nutrients supplied as Wuxal Super (Aglukon, Düsseldorf, Germany) for 10 days.
The water-holding capacity (WHC) of the potted soil was determined by using the method of Veihmeyer and Hendrickso 1931 (cited in Abideen et al., 2020a). The 100% WHC was used as the reference point for cultivation. Plants at 50% WHC (showed chronic stress and associated acclimation responses. Therefore, the water holding capacity was maintained around 50% for drought treatment in this study as described in Abideen et al. (2020). All plants were irrigated twice a week with Hoagland’s nutrient solution (Epstein, 1972). Plants were harvested after stress at 28 days, with leaf water relations and gas exchange parameters measured before the final harvest.
Soil water content, temperature and CO2 flux
Soil respiration was measured using an LI-8100 soil efflux chamber system (LI-COR Inc., Lincoln, USA) and a dark survey chamber (10 cm diameter) within 30 min after removing the plant tops from the pots. The survey chamber fitted onto the brims of the pots (Kammann et al., 2011). The offset of each pot (distance from the soil surface to the pot brim) was entered into the LI-8100 system software to calculate the correct system volume and soil CO2 efflux. Measurement time and observation delay were set to 60 and 20 s, respectively, to provide sufficient time for chamber volume mixing and CO2 release monitoring. The increase in CO2 concentration always exhibited a linear slope, with R2 > 0.99. This result validated the automatic calculation of CO2 flux using LI-8100 software using the ideal gas law and linear regression. The respiration value is the CO2 flux in µmol m−2 s−1. The soil water content and temperature were measured with the help of WET150 Multi-Parameter Soil Sensor.
Growth parameters
Shoot and root fresh weights (FW) were recorded immediately after harvest using weighing balance. Shoot and root samples were oven-dried at 80°C for 48 h to determine dry weights. Some fresh samples were also frozen immediately in liquid nitrogen and stored at –20°C for antioxidiant enzyme assays. Leaf area (whole plant basis) was measured with a portable area meter (LI-COR-3000C). At least five biological replicates were used.
Leaf gas exchange, chlorophyll and chlorophyll fluorescence
Leaf gas exchange parameters (net photosynthetic rate, respiration, stomatal conductance, intercellular CO2 concentration, transpiration rate, and water use efficiency (WUE) = net photosynthetic rate/stomatal conductance) were measured on fully expanded young leaves between 8 a.m to 4 pm. Steady state CO2/H2O leaf gas exchange readings were recorded using a LI-COR 6400XT photosynthesis system (LI-COR Inc., Lincoln, NE, USA) at 400 μmol mol–1 CO2 atmospheric concentrations, 30°C block temperature, ≤ 2 kPa vapor pressure deficit, and ~1,000 μmol m–2 s–1 light intensity. Estimated chlorophyll content was recorded with a SPAD 502 (Konica Minolta, Japan).
Chlorophyll fluorescence parameters were determined two days before plant harvest using a pulse modulated chlorophyll fluorescence meter (Junior PAM, Walz, Germany) on the same leaves used for CO2/H2O gas exchange measurements. Minimal (Fo) and maximal fluorescence (Fm) values were recorded on dark (25 min) adapted leaves to calculate the maximum photochemical quantum yield of PSII [(Fv/Fm = (Fm – Fo)/Fm)] according to the method of Kitajima and Butler (1975). Steady state (Fs), maximal (Fm′), and minimal fluorescence (Fo) were measured on light-adapted leaves. Effective photochemical quantum yield of PSII was calculated according to the formula [(Fm′ – Fs)/Fm′] as described by Genty et al. (1989). Non-photochemical quenching (NPQ) was calculated as NPQ = Fm′/(Fm′ – 1), formulated by Bilger and Bjorkman (1990). The electron transport rate (ETR) was calculated according to the formula described in Krall and Edwards (1992):
where PPFD is leaf photosynthetic photon flux density, 0.5 is the factor used to assume an equal amount of energy distribution between two photosystems (PSII and PSI), and 0.84 is the factor used to assume leaf absorbance. The risk of oxidative stress was determined as ETR/Agross, as described in Salazar-Parra et al. (2012).
Lipid peroxidation and enzyme assays
Malonyldialdehyde (MDA) levels was determined on fresh leaf as a damage (stress) marker using the method of Hernandez et al. (2001). The measurement of catalase (CAT, EC 1.11.1.6) activity was performed according to Aebi (1984). Ascorbate peroxidase (APX, EC 1.11.1.11) activity was determined Nakano & Asada, (1981). Activity of superoxide dismutase (SOD) was determined according to the method of Beyer and Fridovich, 1987. Glutathione reductase (GR, EC 1.6.4.2) activity was determined as performed by Foyer and Halliwell (1976). Guaiacol peroxidase (GPX, EC 1.11.17) activity was measured as described by Tatiana et al. (1999).
Analysis of Na+, K+, Mg2+, Ca2+
Dried shoot and root samples (20 mg) were extracted with 10 mL HNO3 (0.5%) in a water bath (80°C) for 12 h. Na+, K+, Mg2+, and Ca2+ concentrations were determined using an atomic absorption spectrometer (AAS PE2100, Perkin Elmer, United States, MA 02451, Waltham, 940). The K+/Na+ ratio was calculated and used to indicate K+ and Na+ ion selectivity for absorption and transport (Pitman, 1965).
Statistical analysis
Analysis of data (n = 5) was performed using SPSS (ver. 11) software, with significant differences among means (P< 0.05) assessed by Fisher’s protected least significance difference (LSD). The data were analyzed using two way analysis of variance (ANOVA) to identify significant effects, drought, bacteria and drought x bacteria interaction. of the experiment at P< 0.05. (Table S1).
Results
Soil water content, temperature, and CO2 flux
For soil data, two-way ANOVA showed a significant individual effect of both drought (D) and bacteria (B) but their interactions (D X B) The volumetric soil water content was monitored during the water deficit stress treatments. Soil inoculated with Pantoea displayed higher soil water contents under water deficit and well-watered conditions compared to control (no bacteria added). There were no change in soil temperature was observe in soil throughout the study regardless of the PGPR treatments. Inoculation with Pseudomonas or Pantoea both enhanced (about 2 folds) soil gas exchange (soil carbon flux) under water deficit and well-watered conditions than un-inoculated treatments (Figure 1).
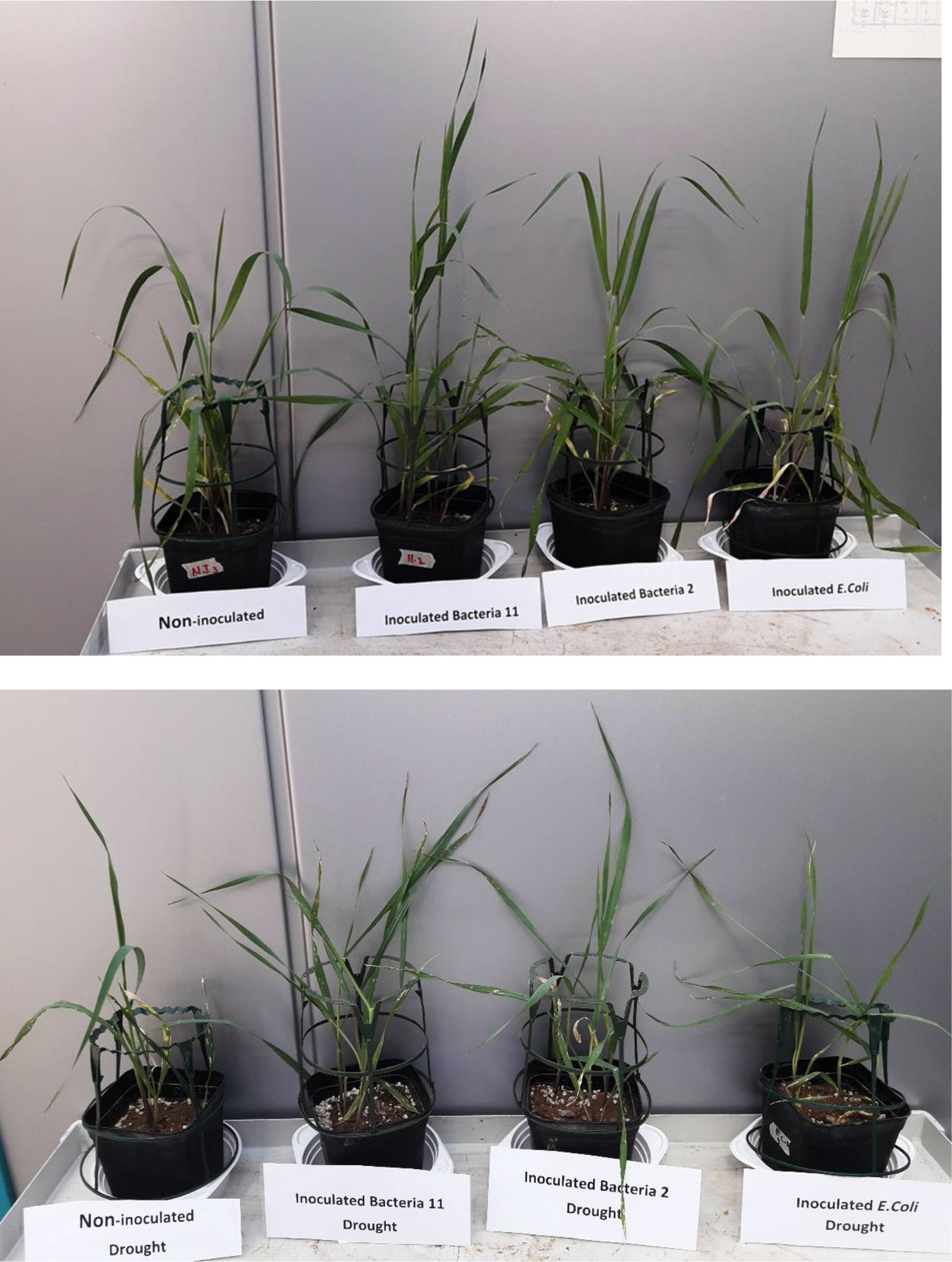
Figure 1 Shoot and root growth of drought-stressed and non-stressed (well-watered) Hordeum vulgare inoculated with three bacterial strains (Pseudomonas sp. (ITS Group 11), Pantoea sp. (ITS Group 2), or E. coli).
Plant growth
Plant total leaf fresh weight (FW) increased (23-29%) in non-stressed barley inoculated with Pseudomonas or Pantoea compared to other treatments. Water deficit caused a significant decreased in leaf FW, but the reduction was lower particularly in plants inoculated with Pseudomonas. Additionally, water deficit also reduced the stem and root FWs relative to well-watered plants. The inoculation of Pseudomonas or Pantoea under water deficit treatment caused a significant increase in the root length (1-2 folds) and 925%) leaf area compared to control plants (Figure 2).
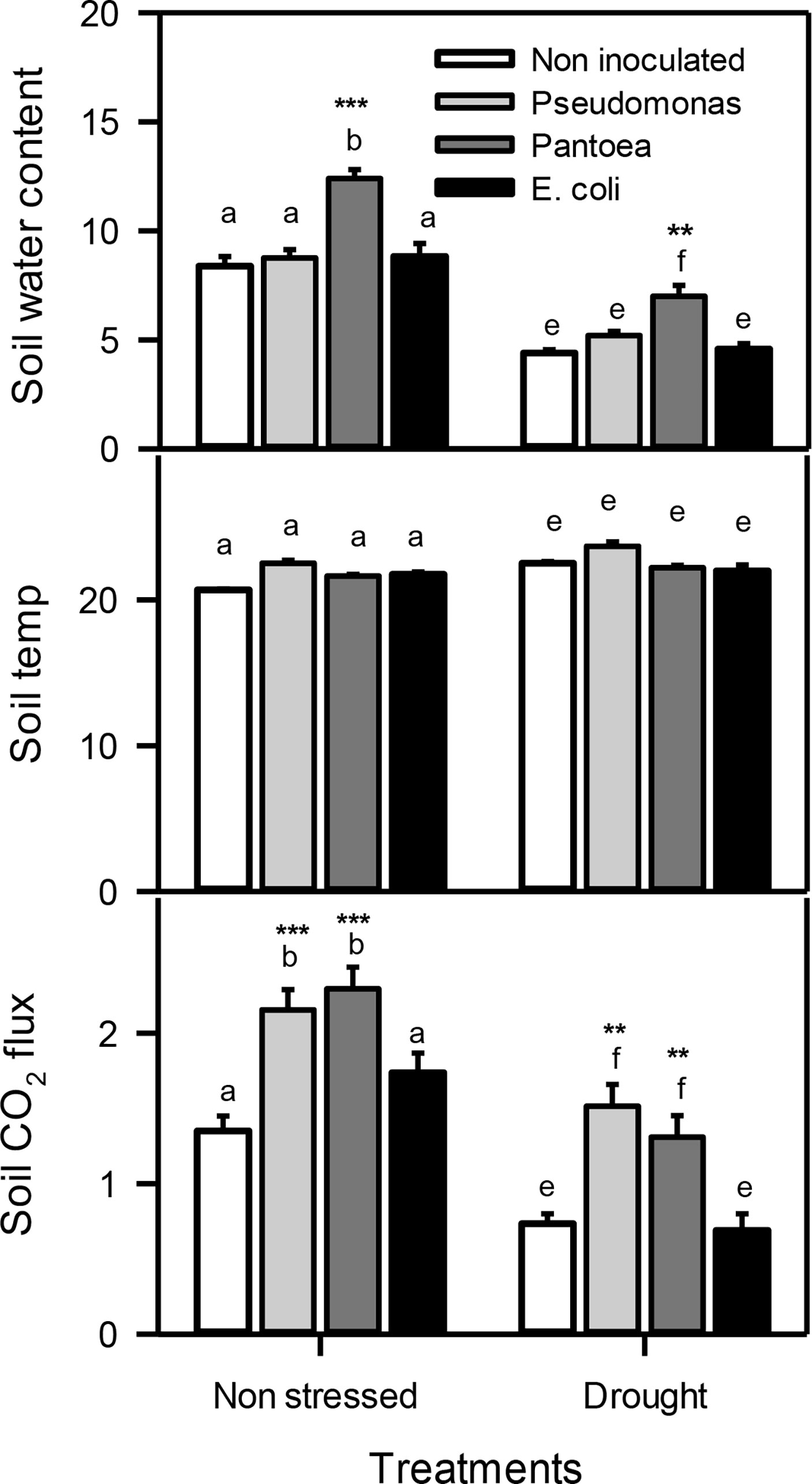
Figure 2 Soil water content, soil temperature (temp), and soil CO2 flux of drought-stressed and non-stressed (well-watered) Hordeum vulgare inoculated with three bacterial strains (Pseudomonas sp. (ITS Group 11), Pantoea sp. (ITS Group 2), or E. coli). F and P (***P< 0.001, **P< 0.01) values of the two-way ANOVAs are presented, drought, bacteria and drought x bacteria interaction. The lower case letters shows the significant differences among means (P< 0.05) assessed by Fisher’s protected least significance difference (LSD).
Leaf chlorophyll content, gas exchange, and chlorophyll fluorescence
About (18%) increase in total chlorophyll contents was detected in the well-watered and water-stressed plants inoculated with Pseudomonas or Pantoea than the control plants (Figure 3). Pseudomonas and Pantoea inoculation also increased net photosynthesis (33% in pentoa and 54% in Pseudomonas) while the reverse was true for E. coli (Table 1). Stomatal conductance increased about (44%) in drought-stressed plants inoculated with Pseudomonas. Internal CO2 concentration (Ci) decreased in well-watered plants inoculated with Pseudomonas or Pantoea, but in decline were prominent only with and water-stressed plants inoculated with Pseudomonas under drought. The Ci/Ca ratio decreased in well-watered plants inoculated with Pseudomonas but increased in water-stressed plants compare to other treatments (Table 1). Leaf transpiration increased about (27%) in water-stressed plants inoculated with Pseudomonas or Pantoea compare to other treatments (Table 1). Water use efficiency (WUE) increased in well-watered plants inoculated with Pseudomonas or Pantoea compare to control treatments, while rate of respiration rates increased in inoculated plants relative to uninoculated plants (Table 1).
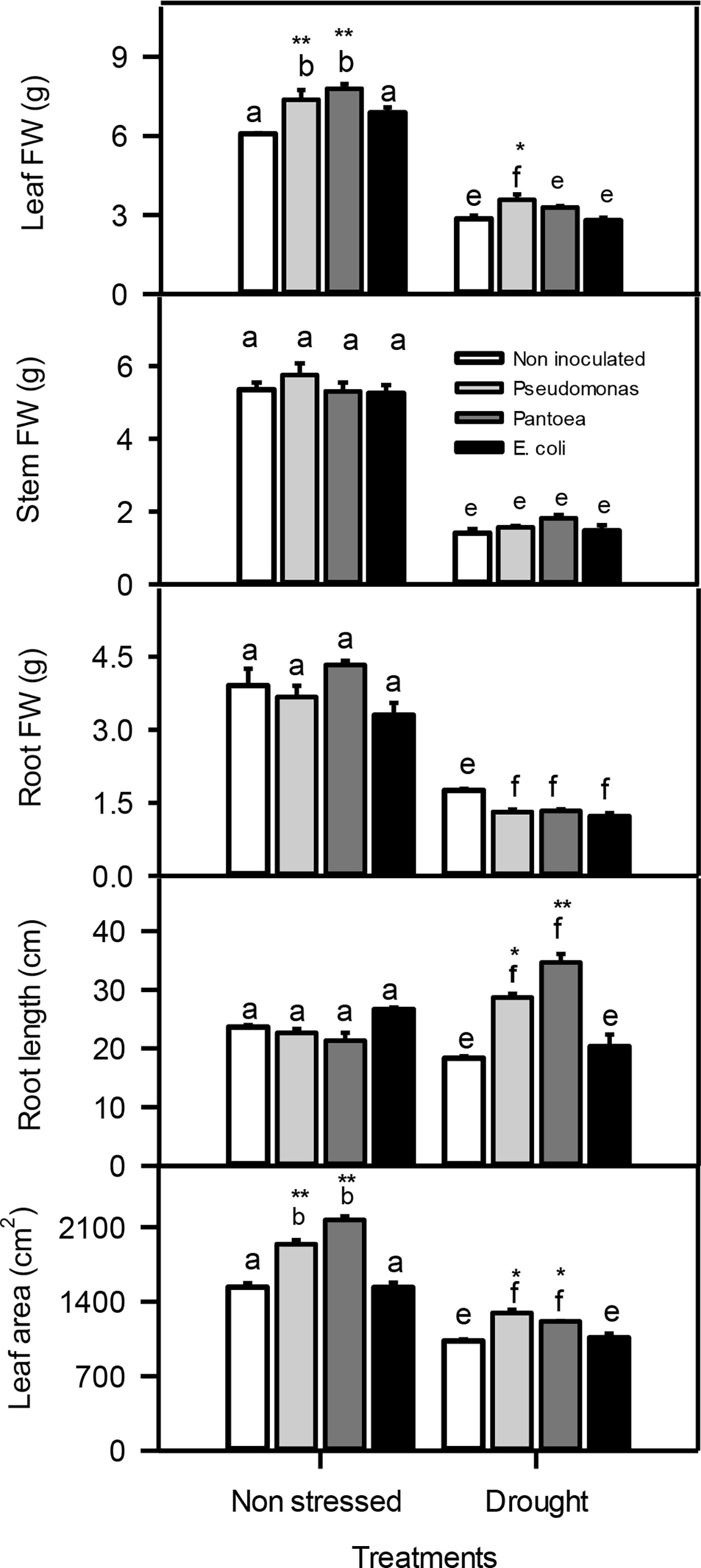
Figure 3 Leaf, stem, and root fresh weight (FW), root length, and leaf area of drought-stressed and non-stressed (well-watered) Hordeum vulgare inoculated with three bacterial strains (Pseudomonas sp. (ITS Group 11), Pantoea sp. (ITS Group 2), or E. coli). F and P (** P < 0.01, *P < 0.05) values of the two-way ANOVAs are presented, drought, bacteria and drought x bacteria interaction. The lower case letters shows the significant differences among means (P< 0.05) assessed by Fisher’s protected least significance difference (LSD).
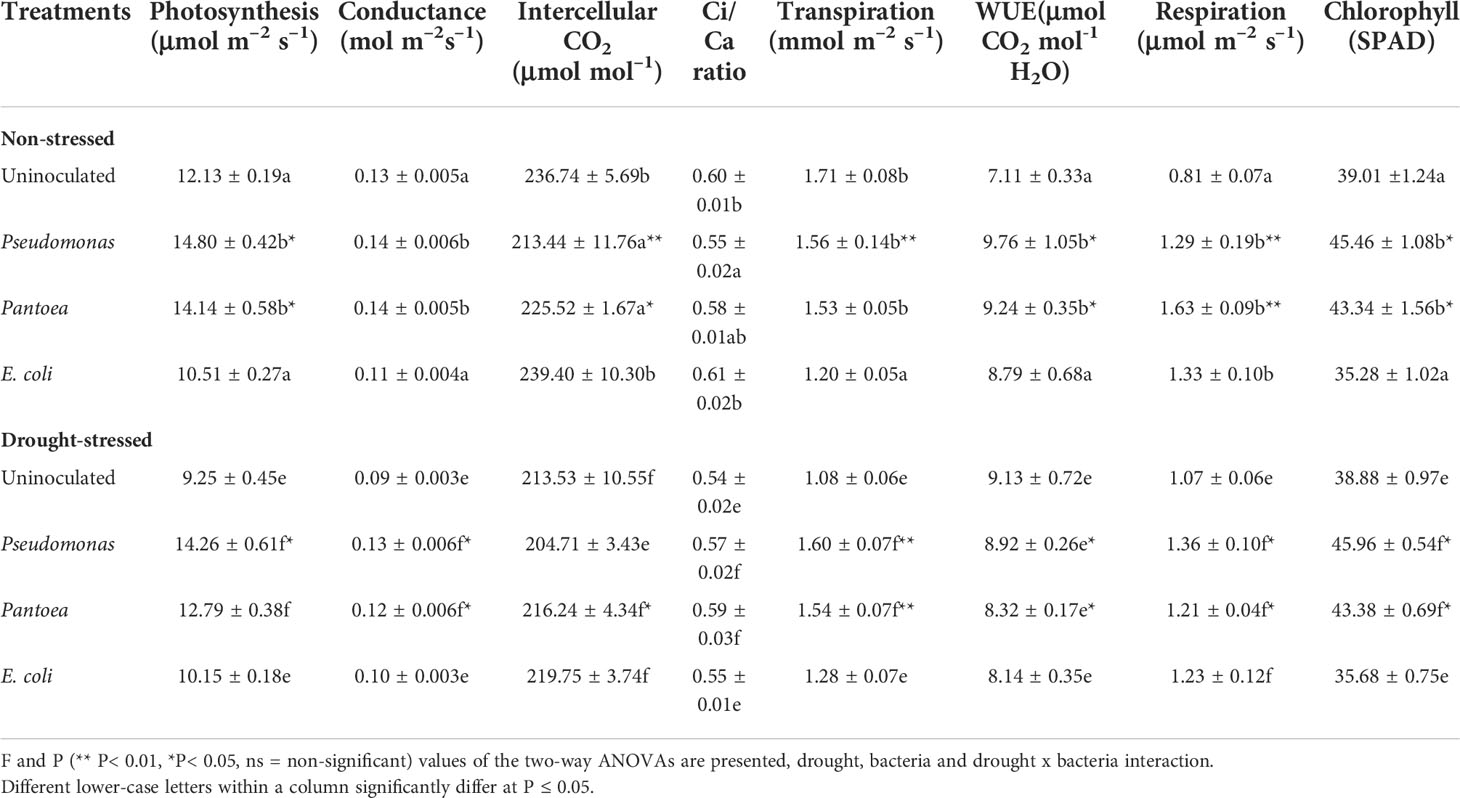
Table 1 Photosynthesis, stomatal conductance, intercellular carbon dioxide (Ci) and ratio of intercellular CO2 with atmospheric CO2 (Ci/Ca), transpiration, water use efficiency (WUE), and respiration of drought-stressed and non-stressed (well-watered) Hordeum vulgare inoculated with three bacterial strains (Pseudomonas sp. (ITS Group 11), Pantoea sp. (ITS Group 2), or E. coli).
Bacterial inoculation did not change the potential quantum yield of PSII (Fv/Fm) under well-watered or water deficit conditions (Table 2). ETR did not change in well-watered plants inoculated with Pseudomonas or Pantoea but increased in plants inoculated with Pantoea relative to uninoculated plants under drought. NPQ increased in well-watered and water-stressed plants inoculated with Pseudomonas or Pantoea (Table 2). Photoinhibition and ETR/A ratios decreased (photoinhibition 91% and 49% ETR/A) in barley plants inoculated with Pseudomonas or Pantoea compared to control under well-watered and water-deficit conditions (Table 2).
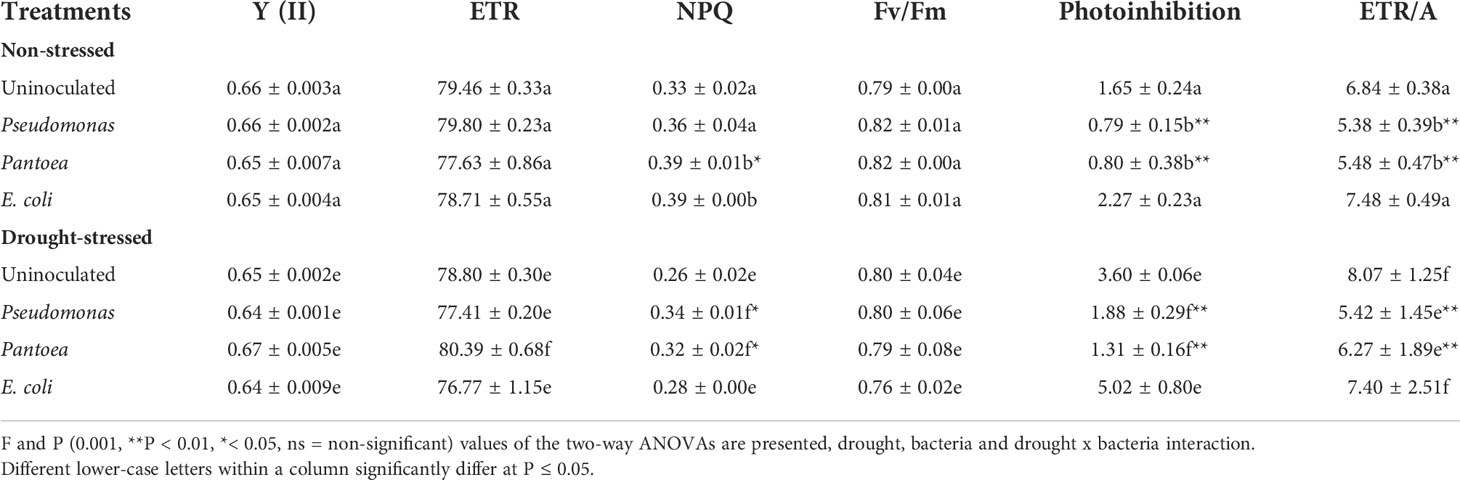
Table 2 Chlorophyll florescence parameters (photochemical efficiency of photosystem II [Y (II)], electron transport rate (ETR), non-photochemical quenching (NPQ), maximum photosynthetic efficiency of PSII (Fv/Fm), photoinhibition and oxidative stress (ETR/A)) of drought-stressed and non-stressed (well-watered) Hordeum vulgare inoculated with three bacterial strains (Pseudomonas sp. (ITS Group 11), Pantoea sp. (ITS Group 2), or E. coli).
Antioxidant enzymes
Well-watered and drought-stress inoculated plants accumulated lower SOD enzyme activities than uninoculated plants. Plants inoculated with Pseudomonas or Pantoea enhanced CAT activities under well-watered conditions and decreased CAT activities under drought stress than uninoculated plants (Figure 4). Plants inoculated with Pseudomonas or Pantoea had higher APX enzyme activities under drought stress relative to uninoculated plants. Plants inoculated with Pseudomonas or Pantoea had higher GPX activities under well-watered conditions relative to uninoculated plants (Figure 4). Well-watered and drought-stressed inoculated plants improved GR activities than uninoculated plants(Figure 4). Interestingly, MDA contents decreased in well-watered and drought-stressed plants inoculated with Pseudomonas or Pantoea (Figure 4).
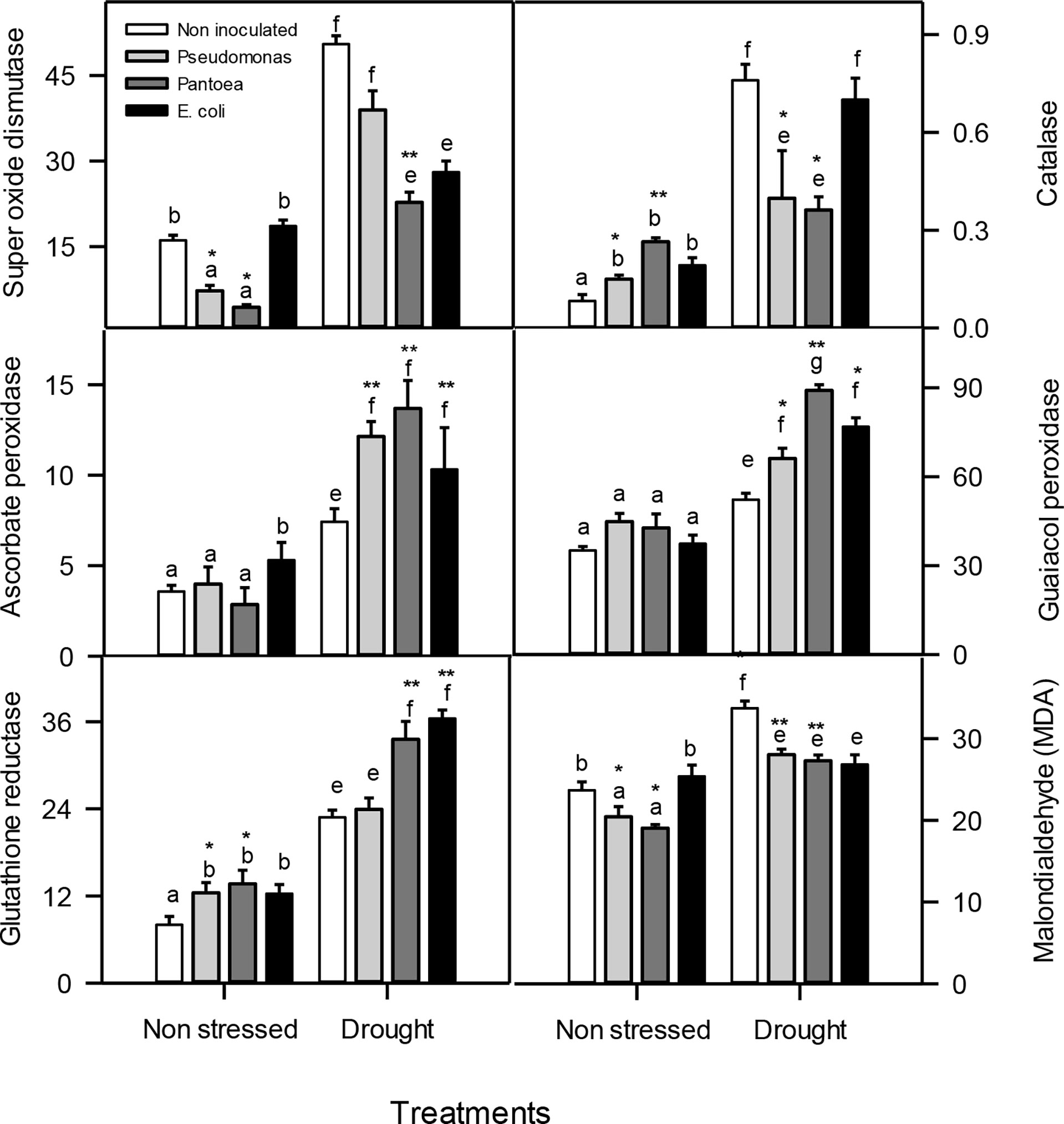
Figure 4 Antioxidant enzyme levels (μmol mg-1 prot min-1) FW—superoxide dismutase (SOD), catalase (CAT), ascorbate peroxidase (APX), guaiacol peroxidase (GPOX), glutathione reductase (GR), and malondealdehyde (MDA (μmol g-1 FW))—in drought-stressed and non-stressed (well-watered) Hordeum vulgare inoculated with three bacterial strains (Pseudomonas sp. (ITS Group 11), Pantoea sp. (ITS Group 2), or E. coli). F and P (** P< 0.01, *P< 0.05) values of the two-way ANOVAs are presented, drought, bacteria and drought x.The lower case letters shows the significant differences among means (P< 0.05) assessed by Fisher’s protected least significance difference (LSD).
Minerals analysis
Shoot Ca2+ increased in well-watered plants inoculated with Pseudomonas or Pantoea compared to other treatments. Root Ca2+ increased in well-watered plants inoculated with Pseudomonas or Pantoea and water-stressed plants except Pseudomonas in water-stressed plants (Table 3). Shoot Mg2+ levels enhanced in well-watered and drought-stressed plants inoculated with Pseudomonas and Pantoea. Root Mg2+ increased in well-watered plants inoculated with Pseudomonas but did not change under drought stress (Table 3). Shoot and root K+ and the shoot and root K+/Na+ ratios increased in well-watered and drought-stressed plants inoculated with Pseudomonas and Pantoea, relative to control treatments (Table 3). Shoot Na+ increased in well-watered plants inoculated with Pantoae but decreased with plants inoculated with Pseudomonas. Shoot Na+ increased in drought-stressed plants inoculated with Pantoea, Pseudomonas relative to uninoculated plants. Well-watered inoculated plants accumulated higher root Na+ than uninoculated plants compared to control (Table 3). Plants under water deficit had higher root Na+ than well-watered plants, particularly those inoculated with Pseudomonas. (Table 3).
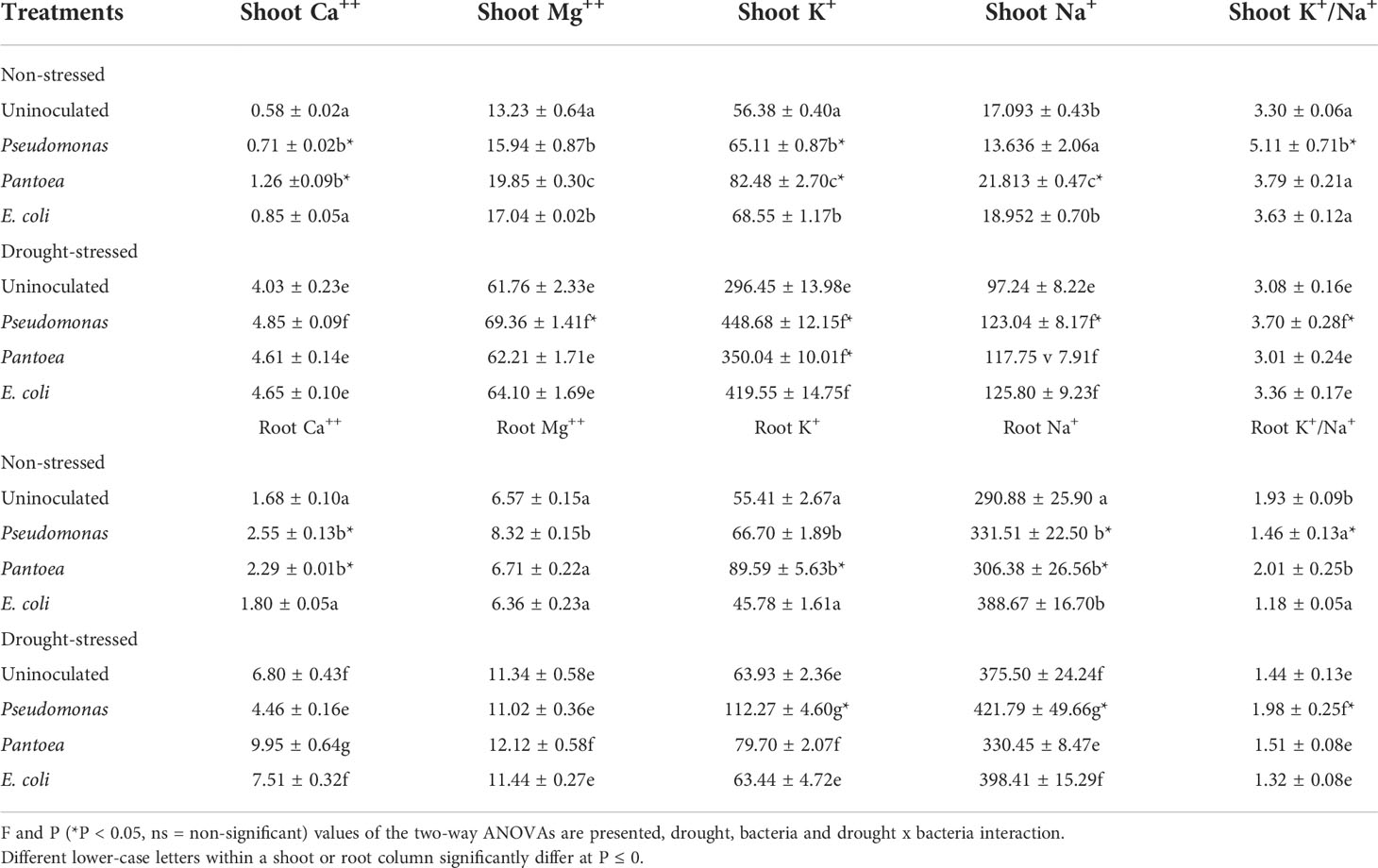
Table 3 Shoot and root cation (Ca++, Mg++, K+, Na+) concentrations (mmol kg–1 FW) and shoot K+/Na+ ratio of drought-stressed and non-stressed (well-watered) Hordeum vulgare inoculated with three bacterial strains (Pseudomonas sp. (ITS Group 11), Pantoea sp. (ITS Group 2), or E. coli).
Discussions
Drought is a major abiotic factor that inhibits crop yields but association of seed-associated bacterial endophytes of Hordeum vulgare are beneficial for rhizosphere soil health and its proper application, relieved the adverse effects of water deficit on barley grown under water limited areas to ensure productivity of such an important food crops (Chandra et al., 2021; Tyagi and Pandey, 2022; Kour and Yadav, 2022). In this study, bacterial inoculation enhanced both soil carbon flux and soil moisture under drought stress, regulating the ecophysiological performance (growth, net photosynthesis, and mineral acquisition) of barley seedlings. Stimulation of soil carbon flux due to Pseudomonas and Pantoea inoculation in dry arid areas in barley was might be associated with increased soil respiration that triggers higher microbial activity. Higher soil metabolic output with microbial inoculation indicates that barley cultivation with PGPB is a suitable strategy for enhancing carbon sequestration and thus contributing to climate change mitigation (Radicetti et al., 2020). Improvement of soil parameters especially soil water acquisition (especially Pantoea treatment) under stressed and non-stressed conditions was also as reported for maize (Naseem and Bano, 2014; Goudarzi et al., 2023), which has been associated with exopolysaccharides (EPS) production. EPS significantly enhance plant growth (Naseem and Bano, 2014; Zayed et al., 2022) by colonizing plant roots, forming hydrophilic biofilms, and providing plant immune response (Sun et al., 2022). In addition, PGPB= use several mechanisms to improve plant growth such as maintaining sufficient nutrient supply or regulating hormone levels (Forni et al., 2017; Siddiqui et al., 2021). In this study, the introduced bacterial endophytes Pseudomonas and Pantoea emerged as mediators for enhancing total foliage biomass, as reported in Capsicum annuum (Figure 2) (Datta et al., 2011; Lin et al., 2020). Application of microbes can improve fruit quality by upregulating nitrogen metabolism and producing specific hormones that trigger water and mineral balance and promote belowground biomass (Ahemad and Kibret, 2014). In the present study, Pseudomonas (under drought and control both condition) and Pantoea inoculations (well water condition) increased leaf fresh biomass and other growth parameters which was reported earlier in maize under water deficit condition (Jeong et al., 2021). Plants inoculated with Pseudomonas or Pantoea improved plant root elongation under water deficit compared to the other treatments. Higher root production under drought suggests that barley seeds benefit from soil microbe/plant interactions with Pseudomonas and Pantoea endophytes to access optimum water and nutrient which is critical for biomass production (Lin et al., 2020; Verma et al., 2021). Increased root development from microbial amendments can also support seedling emergence and long-term survival of barley in poorly degraded, dry areas that appear futile for agriculture and thus improve sustainable agriculture to avoid food insecurity (Calvo et al., 2017; Abdelfadil et al., 2022). In addition, it was reported that PGPB strains enhance phytohormone production and other signals to modify root system architecture, such as increased lateral root branching and root hair development (Siddiqui et al., 2022). The proper root modification stimulated the leaf development that favors the photosynthesis and the activity of photochemical reaction. Chlorophyll is the main photosynthetic pigment that was stimulated due to PGPB application in barley under drought-stressed and well-watered conditions due to increased nutrient acquisition, as reported elsewhere (Dawwam et al., 2013; Dao et al., 2020). Enhanced synthesis of chlorophyll pigments and their accessory components improve photosynthetic rate as well asPSII efficiency and the protein-pigment complex function. Plants inoculated with PGPR developed drought tolerance, suggesting that plants treated with bacterial strains enhance CO2 assimilation and reduce water release by leaves (Armada et al., 2015; Mehrasa et al., 2022). In addition, under suboptimum conditions, plants release some photosynthetic assimilates as root exudates, which helps maintain bacterial colonization in the root zone, promoting mutual benefits such as increased plant resistance against abiotic stress (Samaniego-Gámez et al., 2016). It was reported that PGPR elevate photosynthesis in in plants by regulating endogenous sugar/abscisic acid signaling (Sati et al., 2021). In the present study, plants inoculated with Pseudomonas and Pantoea increased gas exchange, respiration, stomatal conductance and leaf transpiration under drought-stressed and well-watered conditions. However, they only increased WUE under well-watered conditions (Table 3), indicating that bacterial-inoculated plants improve plant growth by enhancing photosynthetic performance (Chandra et al., 2021). It was reported that bacterial strains in the root zone synthesize auxins (indole-3-acetic acid/indole acetic acid/IAA) that increase tissue cell division, photosynthetic pigment synthesis, and photosynthesis (Ahemad and Kibret, 2014; Mitra et al., 2022). In tobacco, it was established that CO2 produced in roots was transported to shoots for photosynthesis via the vascular system instead of stomata (Andrade et al., 2022). It was suggested that endophyte colonization changed the host plant’s photosynthetic apparatus, increasing the activity of light harvesting complexes and enhancing photosynthetic performance (Chaturvedi et al., 2022). Similarly, Liu et al. (2021) indicated that seed endophytes stimulate PSII efficiency in plants.
Bacterial inoculation of pepper plants increased ETR and NPQ which could be a consequence of the positive effect of PGPB (Samaniego-Gámez et al., 2016). Further, NPQ helped minimize the over-synthesis of O2 in PSII antenna, increasing NPQ in plants inoculated with bacterial strains to reduce excess light energy (Radhakrishnan and Baek, 2017). In our study, bacterial inoculation increased Fv/Fm under well-watered conditions but increased ETR and NPQ under drought stress. Interestingly, drought stress produced higher photoinhibition and ETR/A ratios than well-watered conditions but were substantially lower in plants inoculated with Pseudomonas and Pantoea than the other treatments. ETR increases due to high oxidation of the quinone acceptor (Qa) and its excitation energy, reducing oxidative damage (Garcia-Caparros et al., 2021). Yang et al. (2017) reported that PGPR provoke systemic tolerance of plants during abiotic stress (salt and drought). Abiotic stresses such as drought increase ROS formation, causing oxidative stress (Chiappero et al., 2019). Increased ROS production affects plants due to the oxidation of photosynthetic pigments in membrane lipids, proteins, and nucleic acids (Jajic et al., 2015; Mukarram et al., 2021). The upregulation of antioxidant enzymes, such as SOD and APX, is a significant plant response to drought Mukarram et al., 2021). Increased CAT, GR, and APX activities were reported in drought-stressed Ocimun basilicum inoculated with PGPR (Chiappero et al., 2019). In the present study, drought stress increased SOD, CAT, APX, GPX, and GR activities, and MDA content. Drought stress and bacterial inoculation combined reduced ROS production, as indicated by the decreased SOD activity and MDA content and increased APX, GPX, and GR activities.
Leaf growth and metabolite production are important parameters under water deficiency (Zulfiqar et al., 2020). In the present study, leaf area increased under drought-stressed and well-watered conditions, which may be linked to P uptake triggered by Pseudomonas or Pantoea inoculation, as reported for maize (Chaudhary et al., 2022). Microbial inoculum improves nutritional assimilation (N, P and K contents) in plants relative to uninoculated plants, possibly because the soil microbes compensate for nutrient deficiency, enhancing plant growth in nutrient-deficient environments (Bargaz et al., 2018). In the present study, Pseudomonas inoculation under well-watered conditions increased shoot and root K+ concentration but decreased shoot Na+ concentration. Sequestration of Na+ in roots and higher uptake of K+ in leaves increased the shoot K+/Na+ ratio in well-watered plants inoculated with Pseudomonas, as reported elsewhere for maize (Shahzad et al., 2022). Similarly, under water deficit, Pseudomonas preferentially increased shoot K+, retained root Na+, and enhanced the shott K+/Na+ ratio relative to the other treatments. De Inoculation of Pseudomonas increased seedling growth in low fertile soil by compensating for nutrient deficiency through the synthesis of plant growth-promoting hormones at the root interface, stimulating root development and increasing soil water and nutrient absorption (Amora-Lazcano et al., 2022; Mehrasa et al., 2022).
In addition, Ca2+ is critical for plant growth, playing an important role in cell wall and membrane development, photosynthesis and ion homeostasis and acting as a signaling molecule in the cytosol (Shabbir et al., 2022). Recently, Ahmed et al. (2021) showed that Ca2+ acts as a signaling agent, enhancing plant stress resistance in unfavorable environmental conditions. In the present study, plant Ca2+ concentration increased substantially in bacterial-treated plants compared to uninoculated plants and even under drought stress. In addition to Ca2+, Mg2+ plays an important role in carbohydrate partitioning, CO2 fixation during photosynthesis, and reactive oxygen species (ROS) formation (Tewari et al., 2021). Mg2+ increases root surface area and overall root growth, enhancing photosynthetic assimilate synthesis and transport and carbohydrate translocation, alleviating drought stress (Alrashidi et al., 2022). In the present study, shoot Mg2+ increased in drought-stressed plants inoculated with Pseudomonas while root Mg2+ did not change. Well-watered inoculated plants increased shoot Mg2+ relative to uninoculated plants, particularly in plants inoculated with Pantoea. Well-watered plants inoculated with Pseudomonas increased root Mg2+ relative to uninoculated plants.
Conclusions
Our results suggest that the appropriate selection of endophytes and their respective response is important for inducing drought stress resistance in barley. Pseudomonas and Pantoea inoculations improved growth, metal acquisition, photosynthesis, and oxidative stress tolerance in drought-stressed barley. The improved biomass production and crop yield with endophytic bacterial inoculation could be a solution for growing barley on poorly degraded lands. Further research is needed to confirm our findings under field conditions in saline and waterlogged areas to unlock the full potential of PGPB on crop performance.
Data availability statement
The raw data supporting the conclusions of this article will be made available by the authors, without undue reservation.
Author contributions
ZA, MC and SR: Conceptualization, Investigation. ZA and SR: Formal analysis, Methodology, Writing- original draft. MC, FaZ, H-WK, KH, FeZ and KHMS: Supervision, Conceptualization, Resources, Writing – review and editing, Funding acquisition. WD and FaZ: Formal analysis. All authors contributed to the article and approved the submitted version.
Acknowledgments
This work was supported by the DAAD (The German Academic Exchange Service) Fund (grant P21067). The authors also acknowledge Taif University Researchers Supporting Project number (TURSP-2020/94), Taif University, Taif, Saudi Arabia.
Conflict of interest
The authors declare that the research was conducted in the absence of any commercial or financial relationships that could be construed as a potential conflict of interest.
Publisher’s note
All claims expressed in this article are solely those of the authors and do not necessarily represent those of their affiliated organizations, or those of the publisher, the editors and the reviewers. Any product that may be evaluated in this article, or claim that may be made by its manufacturer, is not guaranteed or endorsed by the publisher.
Supplementary material
The Supplementary Material for this article can be found online at: https://www.frontiersin.org/articles/10.3389/fpls.2022.980046/full#supplementary-material
References
Abdelaal, K., AlKahtani, M., Attia, K., Hafez, Y., Király, L., Künstler, A. (2021). The role of plant growth-promoting bacteria in alleviating the adverse effects of drought on plants. Biology 10 (6), 520. doi: 10.3390/biology10060520
Abdelfadil, M. R., Taha, M. H., El-Hadidi, M., Hamza, M. A., Youssef, H. H., Khalil, M., et al. (2022). Clay chips and beads capture in situ barley root microbiota and facilitate in vitro long-term preservation of microbial strains. FEMS Microbiol. Ecol. 98 (7), fiac064. doi: 10.1093/femsec/fiac064
Abideen, Z., Koyro, H. W., Huchzermeyer, B., Ahmed, M., Zulfiqar, F., Egan, T., et al. (2021). Phragmites karka plants adopt different strategies to regulate photosynthesis and ion flux in saline and water deficit conditions. Plant Biosystems-An. Int. J. Dealing. All. Aspects. Plant Biol. 155 (3), 524–534. doi: 10.1080/11263504.2020.1762783
Abideen, Z., Koyro, H. W., Huchzermeyer, B., Ansari, R., Zulfiqar, F., Gul (2020a). Ameliorating effects of biochar on photosynthetic efficiency and antioxidant defence of phragmites karka under drought stress. Plant Biol. 22 (2), 259–266. doi: 10.1111/plb.13054
Abideen, Z., Koyro, H. W., Huchzermeyer, B., Gul, B., Khan, M. A. (2020b). Impact of a biochar or a biochar-compost mixture on water relation, nutrient uptake and photosynthesis of Phragmites karka. Pedosphere 30 (4), 466–477. doi: 10.1016/S1002-0160(17)60362-X
Ahemad, M., Kibret, M. (2014). Mechanisms and applications of plant growth promoting rhizobacteria: current perspective. J. King. Saud. Univ-Sci. 26 (1), 1–20. doi: 10.1016/j.jksus.2013.05.001
Ahmed, M. Z., Hussain, T., Gulzar, S., et al. (2021). Calcium improves the leaf physiology of salt treated Limonium stocksii: A floriculture crop. Sci. Hortic. 285, 110190. doi: 10.1016/j.scienta.2021.110190
Alibrandi, P., Cardinale, M., Rahman, M. M., et al. (2018). The seed endosphere of Anadenanthera colubrina is inhabited by a complex microbiota, including methylobacterium spp. and staphylococcus spp. with potential plant-growth promoting activities. Plant Soil. 422 (1), 81–99. doi: 10.1007/s11104-017-3182-4
Alrashidi, A. A., Alhaithloul, H. A. S., Soliman, M. H., Attia, M. S., Elsayed, S. M., SADEK, A. M., et al. (2022). Role of calcium and magnesium on dramatic physiological and anatomical responses in tomato plants. Notulae. Bot. Horti. Agrobotanici. Cluj-Napoca. 50 (1), 12614–12614. doi: 10.15835/nbha50112614
Amora-Lazcano, E., Quiroz-González, H. J., Osornio-Ortega, C. I., Cruz-Maya, J. A., Jan-Roblero, J. (2022). Plant growth-promoting bacteria belonging to the genera pseudomonas and bacillus improve the growth of sorghum seedings in a low-nutrient soil. Bot. Sci. 100 (1), 55–66. doi: 10.17129/botsci.2841
Armada, E., Azcón, R., López-Castillo, O. M., et al. (2015). Autochthonous arbuscular mycorrhizal fungi and bacillus thuringiensis from a degraded Mediterranean area can be used to improve physiological traits and performance of a plant of agronomic interest under drought conditions. Plant Physiol. Biochem. 73, 64–74. doi: 10.1016/j.plaphy.2015.03.004
Bargaz, A., Lyamlouli, K., Chtouki, M., et al. (2018). Soil microbial resources for improving fertilizers efficiency in an integrated plant nutrient management system. Front. Microbiol. 9, 1606. doi: 10.3389/fmicb.2018.01606
Berg, G., Raijmakers, J. M. (2018). Saving seed microbiomes. ISME. J. 12 (5), 1167–1170. doi: 10.1038/s41396-017-0028-2
Beyer, W. F., Fridovich, I. (1987). Assaying for superoxide dismutase activity: some large consequences of minor changes in conditions. Anal. Biochem. 161, 559–566.
Bilger, W., Bjorkman, O. (1990). Role of the xanthophyll cycle in photo-protection elucidated by measurements of light-induced absorbance changes, fluorescence and photosynthesis in leaves of Hedera canariensis. Photosynth. Res. 25 (3), 173–185. doi: 10.1007/BF00033159
Calvo, P., Watts, D. B., Kloepper, J. W., et al. (2017). Effect of microbial-based inoculants on nutrient concentrations and early root morphology of corn (Zea mays). J. Plant Nutrit. Soil Sci. 180 (1), 56–70. doi: 10.1002/jpln.201500616
Cardoso, P., Alves, A., Silveira, P., Sá, C., Fidalgo, C., Freitas, R., et al. (2018). Bacteria from nodules of wild legume species: Phylogenetic diversity, plant growth promotion abilities and osmotolerance. Sci. Total Environ. 645, 1094–1102. doi: 10.1016/j.scitotenv.2018.06.399
Chandra, P., Wunnava, A., Verma, P., et al. (2021). Strategies to mitigate the adverse effect of drought stress on crop plants-influences of soil bacteria: A review. Pedosphere 31 (3), 496–509. doi: 10.1016/S1002-0160(20)60092-3
Chaturvedi, H., Singh, B., Jajoo, A., Prakash, A. (2022). Shielding of photosynthetic apparatus by consortia of bacterial endophytes in tomato plants suffering from fusarium wilt. Front. Agron., 33. doi: 10.3389/fagro.2022.831731
Chaudhary, P., Khati, P., Chaudhary, A., Maithani, D., Kumar, G., Sharma, A. (2021). Cultivable and metagenomic approach to study the combined impact of nanogypsum and pseudomonas taiwanensis on maize plant health and its rhizospheric microbiome. PloS One 16 (4), e0250574. doi: 10.1371/journal.pone.0250574
Chiappero, J., Del Rosario Cappellari, L., Alderete, L. G. S. (2019). Plant growth promoting rhizobacteria improve the antioxidant status in Mentha piperita grown under drought stress leading to an enhancement of plant growth and total phenolic content. Indust. Crop Prod. 4, 831731. doi: 10.1016/j.indcrop.2019.111553
Dao, G., Wang, S., Wang, X., Chen, Z., Wu, Y., Wu, G., et al. (2020). Enhanced scenedesmus sp. growth in response to gibberellin secretion by symbiotic bacteria. Sci. Total Environ. 740, 140099. doi: 10.1016/j.scitotenv.2020.140099
Das, P. P., Singh, K. R., Nagpure, G., Mansoori, A., Singh, R. P., Ghazi, I. A., et al. (2022). Plant-soil-microbes: A tripartite interaction for nutrient acquisition and better plant growth for sustainable agricultural practices. Environ. Res. 214, 113821. doi: 10.1016/j.envres.2022.113821
Datta, M., Palit, R., Sengupta, C., et al. (2011). Plant growth promoting rhizobacteria enhance growth and yield of chilli (Capsicum annuum l.) under field conditions. Aust. J. Crop Sci. 5 (5), 531–536.
Dawwam, G. E., Elbeltagy, A., Emara, H. M., et al. (2013). Beneficial effect of plant growth promoting bacteria isolated from the roots of potato plant. Ann. Agric. Sci. 58 (2), 195–201. doi: 10.1016/j.aoas.2013.07.007
Epstein, E. (1972). “Mineral nutrition of plants: Principles and perspectives,” in Physiological genetics of plant nutrition (New York: John Wiley), 325–344.
Forni, C., Duca, D., Glick, B. R. (2017). Mechanisms of plant response to salt and drought stress and their alteration by rhizo-bacteria. Plant Soil. 410 (1-2), 335–356. doi: 10.1007/s11104-016-3007-x
Foyer, C. H., Halliwell, B. (1976). The presence of glutathione and glutathione reductase in chloroplasts: a proposed role in ascorbic acid metabolism. Planta 133, 21–25.
Garcia-Caparros, P., De Filippis, L., Gul, A., Hasanuzzaman, M., Ozturk, M., Altay, V., et al. (2021). Oxidative stress and antioxidant metabolism under adverse environmental conditions: a review. Bot. Rev. 87 (4), 421–466. doi: 10.1007/s12229-020-09231-1
Genty, B., Briantais, J. M., Baker, N. R. (1989). The relationship between the quantum yield of photosynthetic electron transport and quenching of chlorophyll fluorescence. Biochim. Biophy. Acta 990 (1), 87–92. doi: 10.1016/S0304-4165(89)80016-9
Glick, B. R. (2012). Plant growth-promoting bacteria: mechanisms and applications. Scientifica. 1–15. doi: 10.6064/2012/963401
Goudarzi, T., Tabrizi, L., Alikhani, H. A., Nazeri, V., Najafi, F. (2023). Phytostimulation properties of indigenous plant growth-promoting bacteria from licorice (Glycyrrhiza glabraL.): Benefits for seed germination and seedling growth. Int. J. Hortic. Sci. 10 (1), 53–68. 1f178abc60e3c8a8b19fe12cbfd2528a.pdf
Hernández, J. A., Talavera, J. M., Martínez‐Gómez, P., Dicenta, F, Sevilla, F. (2001). Response of antioxidative enzymes to plum pox virus in two apricot cultivars. Physiologia Plantarum 111 (3), 313–321.
Jajic, I., Sarna, T., Strzalka, K. (2015). Senescence, stress, and reactive oxygen species. Plants 4 (3), 393–411. doi: 10.3390/plants4030393
Jeong, S., Kim, T. M., Choi, B., Kim, Y., Kim, E. (2021). Invasive lactuca serriola seeds contain endophytic bacteria that contribute to drought tolerance. Sci. Rep. 11 (1), 1–12. doi: 10.1038/s41598-021-92706-x
Kammann, C. I., Linsel, S., Gößling, J. W., Koyro, H. W. (2011). Influence of biochar on drought tolerance of chenopodium quinoa willd and on soil–plant relations. Plant Soil 345 (1), 195–210. doi: 10.1007/s11104-011-0771-5
Kang, S. H., Cho, H. S., Cheong, H., et al. (2007). Two bacterial entophytes eliciting both plant growth promotion and plant defense on pepper (Capsicum annuum l.). J. Microbiol. Biotechnol. 17 (1), 96–103.
Khatoon, Z., et al. (2020). Unlocking the potential of plant growth-promoting rhizobacteria on soil health and the sustainability of agricultural systems. J. Environ. Manag. 273. doi: 10.1016/j.jenvman.2020.111118
Kitajima, M., Butler, W. L. (1975). Quenching of chlorophyll fluorescence and primary photochemistry in chloroplasts by di-bromo-thymo-quinone. Biochimi. Biophys. Acta 376 (1), 105–115. doi: 10.1016/0005-2728(75)90209-1
Knutzen, F., Meier, I. C., Leuschner, C. (2015). Does reduced precipitation trigger physiological and morphological drought adaptations in European beech (Fagus sylvatica l.)? comparing provenances across a precipitation gradient. Tree Physiol. 35 (9), 949–963. doi: 10.1093/treephys/tpv057
Kour, D., Yadav, A. N. (2022). Bacterial mitigation of drought stress in plants: Current perspectives and future challenges. Curr. Microbiol. 79 (9), 1–19. doi: 10.1007/s00284-022-02939-w
Krall, J. P., Edwards, G. E. (1992). Relationship between photosystem II activity and CO2 fixation in leaves. Physiol. Plant 86 (1), 180–187. doi: 10.1111/j.1399-3054.1992.tb01328.x
Lehmann, J., Rillig, M. C., Thies, J., et al. (2011). Biochar effects on soil biota-a review. Soil Biol. Biochem. 43 (9), 1812–1836. doi: 10.1016/j.soilbio.2011.04.022
Lin, Y., Watts, D. B., Kloepper, J. W., et al. (2020). Influence of plant growth promoting rhizobacteria on corn growth under drought stress. Commun. Soil Sci. Plant 51 (2), 250–264. doi: 10.1080/00103624.2019.1705329
Liu, B., Jing, D., Liu, F., Ma, H., Liu, X., Peng, L. (2021). Serendipita indica alleviates drought stress responses in walnut (Juglans regia l.) seedlings by stimulating osmotic adjustment and antioxidant defense system. Appl. Microbiol. Biotechnol. 105 (23), 8951–8968. doi: 10.1007/s00253-021-11653-9
Liu, J., Qi, W., Li, Q., et al. (2020). Exogenous phosphorus-solubilizing bacteria changed the rhizo-sphere microbial community indirectly. Biotechnology 10 (4), 1–11.
Mehrasa, H., Farnia, A., Kenarsari, M. J., Nakhjavan, S. (2022). Endophytic bacteria and SA application improve growth, biochemical properties, and nutrient uptake in white beans under drought stress. J. Soil Sci. Plant Nutr., 1–12. doi: 10.1007/s42729-022-00884-y
Mitra, D., Mondal, R., Khoshru, B., Senapati, A., Radha, T. K., Mahakur, B., et al. (2022). Actinobacteria-enhanced plant growth, nutrient acquisition, and crop protection: Advances in soil, plant, and microbial multifactorial interactions. Pedosphere 32 (1), 149–170. doi: 10.1016/S1002-0160(21)60042-5
Mukarram, M., Choudhary, S., Kurjak, D., Petek, A., Khan, M. M. A. (2021). Drought: Sensing, signalling, effects and tolerance in higher plants. Physiol. Plantarum. 172 (2), 1291–1300. doi: 10.1111/ppl.13423
Munir, N., Hasnain, M., Roessner, U., Abideen, Z. (2021). Strategies in improving plant salinity resistance and use of salinity resistant plants for economic sustainability. Crit. Rev. Environ. Sci. Technol. 52 (12), 2150–2196. doi: 10.1080/10643389.2021.1877033
Nakano, Y., Asada, K. (1981). Hydrogen peroxide is scavenged by ascorbate-specific peroxidase in spinach chloroplasts. Plant Cell Physiol. 22, 867–880.
Naseem, H., Bano, A. (2014). Role of plant growth-promoting rhizo-bacteria and their exo-polysaccharide in drought tolerance of maize. J. Plant Interact. 9 (1), 689–701. doi: 10.1080/17429145.2014.902125
Oleńska, E., Małek, W., Wójcik, M., Swiecicka, I., Thijs, S., Vangronsveld, J. (2020). Beneficial features of plant growth-promoting rhizobacteria for improving plant growth and health in challenging conditions: A methodical review. Sci. Total Environ. 743, 140682. doi: 10.1016/j.scitotenv.2020.140682
Paneque, M., José, M., Franco-Navarro, J. D., et al. (2016). Effect of biochar amendment on morphology, productivity and water relations of sunflower plants under non-irrigation conditions. Catena 147, 280–287. doi: 10.1016/j.catena.2016.07.037
Peña-Gallardo, M., Vicente-Serrano, S. M., Domínguez-Castro, F., et al. (2019). The impact of drought on the productivity of two rainfed crops in Spain. Nat. Hazard. Earth Syst. Sci. 19 (6), 1215–1234. doi: 10.5194/nhess-19-1215-2019
Pitman, M. G. (1965). Sodium and potassium uptake by seedlings of Hordeum vulgare. aust. J. Biol. Sci. 18 (1), 10–24. doi: 10.1071/BI9650010
Pittelkow, C. M., Liang, X., Linquist, B. A., et al. (2015). Productivity limits and potentials of the principles of conservation agriculture. Nature 517 (7534), 365–368. doi: 10.1038/nature13809
Radhakrishnan, R., Baek, K. H. (2017). Physiological and biochemical perspectives of non-salt tolerant plants during bacterial interaction against soil salinity. Plant Physiol. Biochem. 116, 116–126. doi: 10.1016/j.plaphy.2017.05.009
Radicetti, E., Campiglia, E., Langeroodi, A.S., Zsembeli, J., Mendler-Drienyovszki, N., Mancinelli, R. (2020). Soil carbon dioxide emissions in eggplants based on cover crop residue management. Nutrient Cycling in Agroecosystems 118 (1)39.
Rahman, M. M., Flory, E., Koyro, H. W., Abideen, Z., Schikora, A., Suarez, C., et al. (2018). Consistent associations with beneficial bacteria in the seed endo-sphere of barley (Hordeum vulgare l.). Systemat. Appl. Microbiol. 41 (4), 386–398. doi: 10.1016/j.syapm.2018.02.003
Saccon, F., Wilson, S., Morey-Burrows, F. S., Ruban, A. V. (2022). Quantifying the long-term interplay between photoprotection and repair mechanisms sustaining photosystem II activity. Biochem. J. 479 (5), 701–717. doi: 10.1042/BCJ20220031
Salazar-Parra, C., Aguirreolea, J., Sánchez-Díaz, M., et al. (2012). Climate change (elevated CO2, elevated temperature and moderate drought) triggers the antioxidant enzymes’ response of grapevine cv. tempranillo, avoiding oxidative damage. Physiol. Plant 144 (2), 99–110. doi: 10.1111/j.1399-3054.2011.01524.x
Samaniego-Gámez, B. Y., Garruña, R., Tun-Suárez, J. M., Kantun-Can, J., Reyes-Ramírez, A., Cervantes-Díaz, L.. (2016). Bacillus spp. inoculation improves photosystem II efficiency and enhances photosynthesis in pepper plants. Chilean. J. Agric. Res. 76 (4), 409–416. doi: 10.4067/S0718-58392016000400003
Santander, C., Aroca, R., Ruiz-Lozano, J. M., Olave, J., Cartes, P., Borie, F., et al. (2017). Arbuscular mycorrhiza effects on plant performance under osmotic stress. Mycorrhiza 27 (7), 639–657. doi: 10.1007/s00572-017-0784-x
Sati, D., Pande, V., Pandey, S. C., Samant, M. (2021). Recent advances in PGPR and molecular mechanisms involved in drought stress resistance. J. Soil Sci. Plant Nutr., 1–19. doi: 10.1007/s42729-021-00724-5
Shabbir, R., Javed, T., Hussain, S., Ahmar, S., Naz, M., Zafar, H., et al. (2022). Calcium homeostasis and potential roles to combat environmental stresses in plants. South Afr. J. Bot. 148, 683–693. doi: 10.1016/j.sajb.2022.05.038
Shahzad, B., Rehman, A., Tanveer, M., Wang, L., Park, S. K., Ali, A. (2022). Salt stress in brassica: effects, tolerance mechanisms, and management. J. Plant Growth Regul. 41 (2), 781–795. doi: 10.1007/s00344-021-10338-x
Siddiqui, Z. S., Wei, X., Umar, M., Abideen, Z., Zulfiqar, F., Chen, J., et al. (2021). Scrutinizing the application of saline endophyte to enhance salt tolerance in rice and maize plants. Front. Plant Sci. 12, 770084–770084. doi: 10.3389/fpls.2021.770084
Singh, J. S., Gupta, V. K. (2018). Soil microbial biomass: a key soil driver in management of ecosystem functioning. Sci. Total Environ. 634, 497–500. doi: 10.1016/j.scitotenv.2018.03.373
Sun, L., Cheng, L., Ma, Y., Lie, P., Wang, R., Gu, Y., et al (2022). Exopolysaccharides from Pantoea alhagi NX-11 specifically improve its root colonization and rice salt resistance. Int. J. Biol. Macromol. 209, 396–404.
Tak, Y., Yadav, V. K., Gautam, C., Kumar, R., Kaur, M. (2021). “Drought stress alleviation in plants by soil microbial interactions,” in Microbiological activity for soil and plant health management (Singapore: Springer), 133–159.
Tatiana, Z., Yamashita, K., Matsumoto, H. (1999). Iron deficiency induced changes in ascorbate content and enzyme activities related to ascorbate metabolism in cucumber roots. J. Soil Sci. Plant Nutr. 21(1), 832–859.
Tewari, R. K., Yadav, N., Gupta, R., Kumar, P. (2021). Oxidative stress under macronutrient deficiency in plants. J. Soil Sci. Plant Nutr. 21(1), 832–859.
Tiwari, R. K., Lal, M. K., Kumar, R., Chourasia, K. N., Naga, K. C., Kumar, D., Das, S. K., et al. (2021). Mechanistic insights on melatonin-mediated drought stress mitigation in plants. Physiologia Plantarum 172 (2), 1212–1226.
Truyens, S., Weyens, N., Cuypers, A., Vangronsveld, J. (2015). Bacterial seed endophytes: genera, vertical transmission and interaction with plants. Environmental Microbiology Reports 7 (1), 40–50.
Tyagi, M., Pandey, G. C. (2022). Physiology of heat and drought tolerance in wheat: An overview. J. Cereal Res. 14 (1), 13–25. doi: 10.25174/2582-2675/2022,122868
Verma, S. K., Sahu, P. K., Kumar, K., Pal, G., Gond, S. K., Kharwar, R. N., et al. (2021). Endophyte roles in nutrient acquisition, root system architecture development and oxidative stress tolerance. J. Appl. Microbiol. 131 (5), 2161–2177. doi: 10.1111/jam.15111
Wiggins, G., Thomas, J., Rahmatallah, Y., Deen, C., Haynes, A., Degon, Z., et al. (2022). Common gene expression patterns are observed in rice roots during associations with plant growth-promoting bacteria, herbaspirillum seropedicae and azospirillum brasilense. Sci. Rep. 12 (1), 1–11. doi: 10.1038/s41598-022-12285-3
Wu, S. C., Cao, Z. H., Li, Z. G., Cheung, K. C., Wong, M. H. (2005). Effects of biofertilizer containing N-fixer, P and K solubilizers and AM fungi on maize growth: a greenhouse trial. Geoderma 125 (1-2), 155–166.
Yang, Y., Wang, N., Guo, X., Zhang, Y., Ye, B.. (2017). Comparative analysis of bacterial community structure in the rhizosphere of maize by high-throughput pyro sequencing. PloS One 12 (5), e0178425. doi: 10.1371/journal.pone.0178425
Zhang, H., Xie, X., Kim, M. S., Kornyeyev, D. A., Holaday, S., Paré, P. W. (2008). Soil bacteria augment Arabidopsis photosynthesis by decreasing glucose sensing and abscisic acid levels in planta. The Plant Journal 56 (2), 264–273.
Zayed, A., Mansour, M. K., Sedeek, M. S., Habib, M. H., Ulber, R., Farag, M. A. (2022). Rediscovering bacterial exopolysaccharides of terrestrial and marine origins: novel insights on their distribution, biosynthesis, biotechnological production, and future perspectives. Crit. Rev. Biotechnol. 42 (4), 597–617. doi: 10.1080/07388551.2021.1942779
Keywords: bacterial inoculation, ecophysiology, endophyte (DSE), oxidative stress, photosynthesis
Citation: Abideen Z, Cardinale M, Zulfiqar F, Koyro H-W, Rasool SG, Hessini K, Darbali W, Zhao F and Siddique KHM (2022) Seed Endophyte bacteria enhance drought stress tolerance in Hordeum vulgare by regulating, physiological characteristics, antioxidants and minerals uptake. Front. Plant Sci. 13:980046. doi: 10.3389/fpls.2022.980046
Received: 28 June 2022; Accepted: 05 September 2022;
Published: 05 October 2022.
Edited by:
Shah Fahad, The University of Haripur, PakistanReviewed by:
Aamir Hamid Khan, Huazhong Agricultural University, ChinaAdnan Rasheed, Jiangxi Agricultural University, China
Zhenhua Wei, Northwest A & F University, China
Hyungmin Tony Rho, National Taiwan University, Taiwan
Copyright © 2022 Abideen, Cardinale, Zulfiqar, Koyro, Rasool, Hessini, Darbali, Zhao and Siddique. This is an open-access article distributed under the terms of the Creative Commons Attribution License (CC BY). The use, distribution or reproduction in other forums is permitted, provided the original author(s) and the copyright owner(s) are credited and that the original publication in this journal is cited, in accordance with accepted academic practice. No use, distribution or reproduction is permitted which does not comply with these terms.
*Correspondence: Zainul Abideen, WnVhYmlkZWVuQHVvay5lZHUucGs=; Kadambot H.M. Siddique, a2FkYW1ib3Quc2lkZGlxdWVAdXdhLmVkdS5hdQ==