- 1College of Horticulture, China Agricultural University, Beijing, China
- 2College of Food Science and Nutritional Engineering, China Agricultural University, Beijing, China
Insects and animals are attracted to, and feed on ripe fruit, thereby promoting seed dispersal. As a vital vitamin and nutrient source, fruit make up an indispensable and enjoyable component of the human diet. Fruit ripening involves a series of physiological and biochemical changes in, among others, pigmentation, chlorophyll (Chl) degradation, texture, sugar accumulation, and flavor. Growing evidence indicates that the coordinated and ordered trait changes during fruit ripening depend on a complex regulatory network consisting of transcription factors, co-regulators, hormonal signals, and epigenetic modifications. As one of the predominant transcription factor families in plants and a downstream component of ethylene signaling, more and more studies are showing that APETALA2/ethylene responsive factor (AP2/ERF) family transcription factors act as critical regulators in fruit ripening. In this review, we focus on the regulatory mechanisms of AP2/ERFs in fruit ripening, and in particular the recent results on their target genes and co-regulators. We summarize and discuss the role of AP2/ERFs in the formation of key fruit-ripening attributes, the enactment of their regulatory mechanisms by interaction with other proteins, their role in the orchestration of phytohormone-signaling networks, and the epigenetic modifications associated with their gene expression. Our aim is to provide a multidimensional perspective on the regulatory mechanisms of AP2/ERFs in fruit ripening, and a reference for understanding and furthering research on the roles of AP2/ERF in fruit ripening.
Introduction
The fruit, which protects seeds and supports their development, begins ripening once the seeds mature. Ripe fruit encourages seed dispersal by attracting frugivorous animals, or through drying and dehiscence mechanisms (Forlani et al., 2019). As an essential source of sugars, vitamins, minerals, and antioxidants, fruit are desirable in the human diet. Fruit ripening is a complex process involving a series of physiological and biochemical changes in pigmentation, chlorophyll (Chl) degradation, texture, sugar accumulation, flavor formation, and so on, affecting fruit quality, postharvest life, and economic value (Chen et al., 2020).
Based on its ripening characteristics, fruit can be roughly categorized into climacteric and non-climacteric types. Climacteric fruit have concurrent peaks in respiration rate and ethylene production during ripening. Examples include apple, kiwi, tomato, banana, pear, peach, and mango. Non-climacteric fruit, such as strawberry, cherry, orange, and grape, do not display peaks in respiration rate or ethylene production during ripening (Gao et al., 2020). Although fruit development is co-regulated by a range of phytohormones, abscisic acid (ABA) and ethylene are generally considered to be the most critical ripening regulators. ABA can induce non-climacteric fruit ripening. Ethylene is essential for promoting climacteric fruit ripening (Chen et al., 2020).
Two ethylene-synthesis systems are found in fleshy fruit. System I contributes to the basal level of ethylene synthesis, and functions mainly during pre-ripening stages. System I is autoinhibitory, i.e., the perception of ethylene inhibits ethylene synthesis. In tomato (Solanum lycopersicum L.), AMINOCYCLOPROPANE-1-CARBOXYLIC ACID SYNTHASE 1A and 6 (SlACS1A and SlACS6) are involved in this process (Liu et al., 2015). System II is responsible for the burst of ethylene synthesis during climacteric fruit ripening and is autostimulated by ethylene signals; in tomato, this depends on SlACS1A, SlACS2, SlACS4, and 1-AMINOCYCLOPROPANE-1-CARBOXYLIC ACID OXIDASE 1 and 4 (SlACO1 and SlACO4) (Forlani et al., 2019). The intensity of the ethylene signal in system II is closely related to the onset of ripening and the achievement of full ripening in tomato (Huang et al., 2022).
Ethylene-signal transduction is conserved in climacteric and non-climacteric fruit (Figure 1). The receptors identified in Arabidopsis, including ETHYLENE RESPONSE 1/2 (ETR1/2), ETHYLENE RESPONSE SENSOR 1/2 (ERS1/2), and ETHYLENE INSENSITIVE 4 (EIN4), are located on the endoplasmic reticulum membrane. These receptors act as negative regulators of ethylene signaling. The tomato genome encodes more signal-transduction components than Arabidopsis, including 7 SlETRs, 4 SlCTRs (CONSTITUTIVE TRIPLE RESPONSE), 1 SlEIN2, 6 SlEILs (EIN3-Like), 4 SlEBFs (EIN3-BINDING F-BOX). SlETR3, SlETR4, and SlETR7 are the main receptor genes expressed during tomato ripening (Liu et al., 2015). A single amino acid change in the N-terminal ethylene-binding pocket of SlETR3 results in impaired fruit ripening, known as the NEVER-RIPENING (NR) mutant (Wilkinson et al., 1995). The function of ETR1 receptor proteins has been reported to be regulated by GREEN-RIPE (GR), a homolog of the negative ethylene-response regulator REVERSION-TO-ETHYLENE SENSITIVITY1 (RTE1) of Arabidopsis, which can affect ethylene signaling in tomato (Barry and Giovannoni, 2006). RESPONSE TO ANTAGONIST 1 (RAN1) plays a vital role in the delivery of copper to the ethylene receptors, required for ethylene binding (Binder et al., 2010). The protein TETRATRICOPEPTIDE REPEAT (SlTPR1) interacts with the ethylene receptors NR and ETR1 to regulate ethylene and auxin responses (Lin et al., 2008).
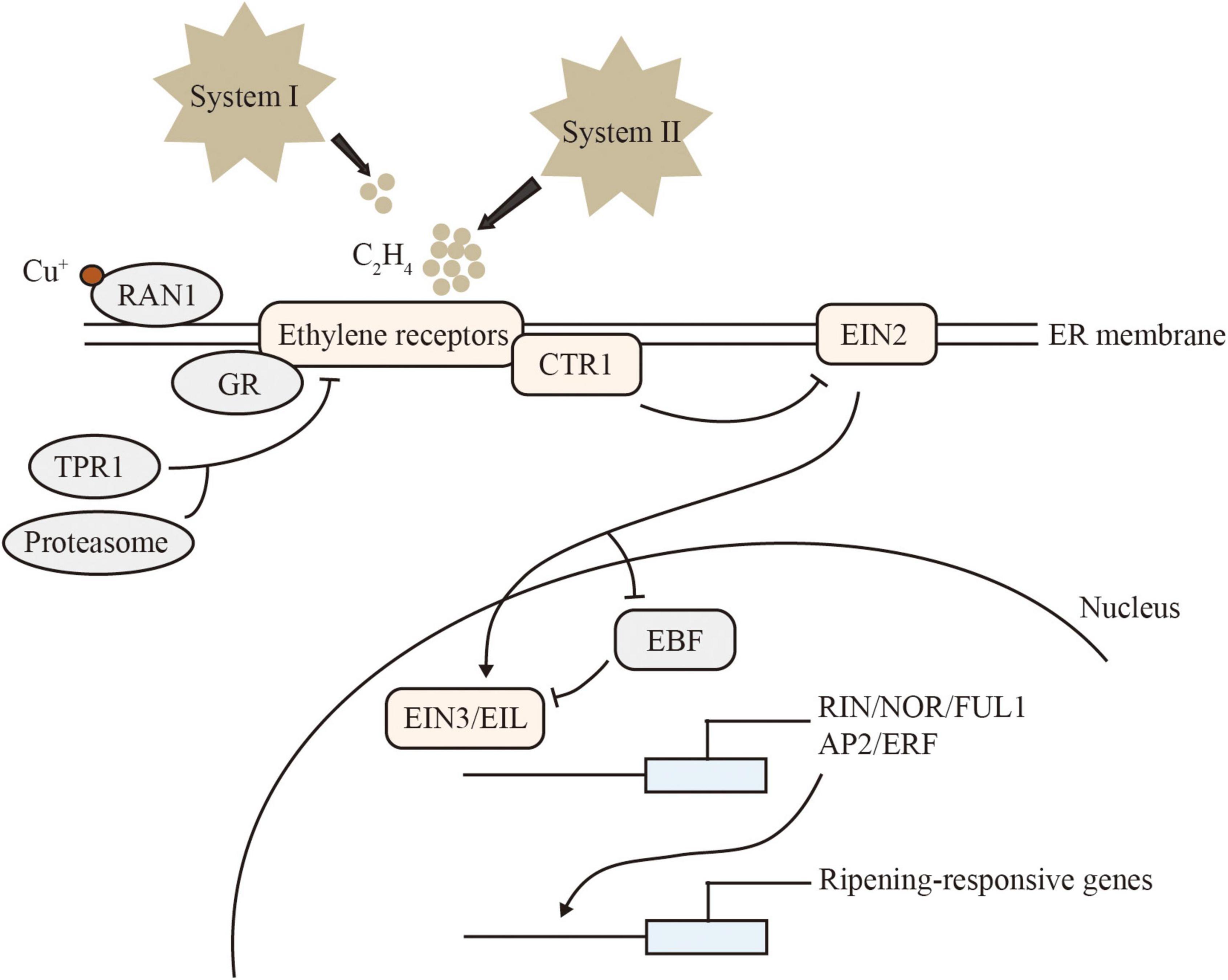
Figure 1. Model of ethylene signaling. CTR1 is a serine/threonine protein kinase that directly phosphorylates and inhibits EIN2 in the absence of ethylene. EIN2 contains multiple transmembrane domains at its N terminus and a cytoplasmic C terminus. In the presence of ethylene, the EIN2 C-terminal domain is cleaved and translocated into the nucleus. ETHYLENE INSENSITIVE3 BINDING F-BOX (EBF) proteins are responsible for targeting and degrading EIN3/EIL. EIN2 binds to and leads to the degradation of mRNAs encoding EBF1 and EBF2. In addition, EIN2 can regulate EIN3-dependent transcription. The accumulation and stabilization of EIN3/EIL lead to numerous transcriptional changes. Arrows represent activation, T-bars indicate repression.
Sensing ethylene inhibits receptor function, and relieves the inhibition of downstream pathways by the negative regulator CTR. Interestingly, a quantitative trait locus (QTL) containing a CTR1-Like gene and a putative DNA demethylase REPRESSOR OF SILENCING 1 (ROS1) gene in melon triggers climacteric ripening on a non-climacteric background. CRISPR/Cas9 knockout mutants of CTR1-Like on the climacteric genetic background show significant advances in ethylene production and ripening initiation but without affecting other important traits, such as flesh firmness (Giordano et al., 2022). EIN2 is the central positive regulator of ethylene signaling. Loss-of-function mutants of SlEIN2 obtained by CRISPR/Cas9 gene editing exhibit fully impaired ethylene signaling. Cessation of ripening in slein2 is partially rescued by slebf1 (Huang et al., 2022). In the presence of ethylene, the EIN2 C-terminal domain is cleaved and translocated into the nucleus to activate the transcription factor EIN3 and its homolog EIL, which in turn induce the expression of downstream ethylene-responsive genes and transcription factors APETALA2/ethylene-responsive factor (AP2/ERF) (Ju and Chang, 2015).
The simplicity and conservation of ethylene signaling cannot explain the diversity and specificity of the resultant physiological responses. However, because AP2/ERFs act downstream of the ethylene-signaling pathway, the various ripening-related traits induced by ethylene can be explained by the diversity of these transcription factors (Liu et al., 2015, 2016).
APETALA2/ethylene responsive factor family, characteristic domains and binding specificities
AP2/ERF is a large class of transcription factors that is found mainly in plants, characterized by an AP2/ERF domain involved in DNA binding. This domain is approximately 60–70 amino acids in length and consists of a three-stranded β-sheet and one α-helix almost parallel to the sheet; arginine and tryptophan are the pivotal residues in the β-sheet for DNA binding (Allen et al., 1998). The AP2 domain was first identified in the Arabidopsis AP2 protein, which functions in flower development (Jofuku et al., 1994). AP2/ERF is generally considered plant-specific. However, homologs of the AP2 domain have been identified in the cyanobacterium Trichodesmium erythraeum, the ciliate Tetrahymena thermophila, and the viruses Enterobacteria phage Rb49 and Bacteriophage Felix 01, suggesting that plant AP2/ERFs may originate from horizontal transfer of HNH-AP2 endonuclease from bacteria or viruses via transposition and homing processes (Magnani et al., 2004).
Thanks to increasingly available genome data, hundreds of AP2/ERF genes have been identified from different plants, such as Arabidopsis thaliana (147, Nakano et al., 2006), tomato (Solanum lycopersicum; 146, Pirrello et al., 2012), apple (Malus × domestica; 259, Girardi et al., 2013), grapevine (Vitis vinifera; 132, Zhuang et al., 2009), pineapple (Ananas comosus L. Merr; 97, Zhang et al., 2021), longan (Dimocarpus longan Lour.; 125, Zhang S. et al., 2020), peach (Prunus persica; 131, Zhang et al., 2012), Chinese jujube (Ziziphus jujuba Mill.; 119, Zhang and Li, 2018), sweet orange (Citrus sinensis; 108, Ito et al., 2014), and others. Systematic analyses indicate that whole-genome duplication events, segmental duplication, and tandem duplication have contributed to the expansion of the AP2/ERF family in plants (Zhuang et al., 2009; Zhang et al., 2021). Duplicated genes evolve overlapping or distinct regulatory functions (Shoji and Yuan, 2021; Zhai et al., 2021), and expansion of the AP2/ERF family has brought about functional differentiation, leading to crucial roles for AP2/ERFs in a wide range of biological and physiological processes such as morphogenesis, defense responses, signal transduction, organ senescence, and metabolite regulation (Feng et al., 2020; Gao et al., 2020).
The Arabidopsis AP2/ERF superfamily is divided into four main categories: ERF (first discovered in Ethylene-Responsive Element-Binding proteins (EREBPs), AP2, Related to Abscisic Acid Insensitive 3/Viviparous 1 (RAV), and soloists (a few unclassified factors) (Nakano et al., 2006; Feng et al., 2020). The AP2 family usually contains two repeated AP2/ERF domains. In addition to one AP2/ERF domain, the RAV family also has one B3 domain, a DNA-binding domain that is conserved in other transcription factors, such as VP1/ABI3. The ERF family contains a single AP2/ERF domain. According to the conserved amino acid residues at positions 14 and 19 of this domain, the ERF family can be further divided into the ERF subfamily and the C-Repeat/Dehydration-Responsive Element Binding factors (CBF/DREB) subfamily (Sakuma et al., 2002). Based on the phylogenetic relationship and conserved motif characteristics, the ERF subfamily can be further divided into 12 phylogenetic groups, namely I to X, VI-L, and Xb-L (Nakano et al., 2006). In tomato, the ERF subfamily members are distributed into A–J groups (Liu et al., 2016).
AP2/ERF proteins bind directly to cis-acting elements on the target gene’s promoter, such as dehydration-responsive element/C-repeat element (DRE/CRT) with core sequence CCGAC, GCC box with core sequence AGCGCCC, and some other GC-rich motifs. The divergent DNA-binding specificities are associated with the amino acid residues of the AP2 domain, which affect the interaction of AP2/ERF proteins with DNA bases and phosphate backbones (Shoji et al., 2013). Furthermore, the AP2 and B3 domains of the RAV subfamily specifically recognize CAACA and CACCTG motifs, respectively (Kagaya et al., 1999). It has been reported that some ERFs can bind the ATCTA motif (Welsch et al., 2007) and vascular system-specific and wound-responsive cis-element (VWRE) (Sasaki et al., 2007). Nucleotides flanking the cis-element also enhance or weaken the binding affinity of AP2/ERF, thereby conferring different AP2/ERF binding specificities to target genes (Pirrello et al., 2012).
AP2/ERFs perform transcriptional activation or repression functions, mainly depending on the functional domain other than the DNA-binding domain. The hydrophobic amino acid-containing ERF-Associated Amphiphilic Repression (EAR) motif is the most dominant active repression domain identified in plants. It is widely found in AP2/ERFs with transcriptional repression function, and is mainly located in the C-terminal region of the protein, with conserved sequences LxLxL or DLNxxP (Ohta et al., 2001; Kagale et al., 2010). Moreover, a B3 Repression Domain (BRD) was identified in the RAV subfamily, containing the R/KLFGV conserved motif (Ikeda and Ohme-Takagi, 2009). The activation domains usually exhibit sequence divergence. The acidic amino acids are thought to be involved in transcriptional activation (Liu et al., 1999). An EDLL motif has been identified as a strong activation domain in group IX of the ERF family, containing several acidic amino acids spaced around hydrophobic leucines (Tiwari et al., 2012).
An N-terminal conserved MCGGAII/L domain has been identified in group VII of the ERF subfamily, involved in oxygen-sensing and N-end rule pathway-dependent protein degradation. Post-translational modifications of this domain can affect the activity of the transcription factors, thereby regulating the expression of core hypoxia responsive genes (Gibbs et al., 2011; Licausi et al., 2011). Group E members of ERF in tomato (corresponding to group VII in Arabidopsis) exhibit tight ripening-related expression and are thought to play a prominent role in ethylene- and RIN (RIPENING-INHIBITOR)/NOR (NON-RIPENING)-dependent ripening (Liu et al., 2016).
Role in fruit ripening
AP2/ERFs play critical roles in fruit ripening (Table 1). In tomato, 55 ERF subfamily members showed ripening-related expression patterns, 27 upregulated and 28 downregulated (Liu et al., 2016). AP2/ERFs are involved in textural changes, pigmentation, Chl degradation, and flavor formation via their regulation of downstream ripening-related genes.
Cell wall modification
The remarkable change in fruit texture at ripening is characterized by a process of remodeling cell wall structure and composition, coordinated by a series of cell wall-modifying enzymes. Among them, POLYGALACTURONASE (PG), PECTIN METHYLESTERASE (PME) and PECTATE LYASE (PL) are related to the metabolism of pectin, and XYLOGLUCAN ENDOTRANSGLUCOSYLASE/HYDROLASE (XTH) is related to xyloglucan and hemicellulose metabolism (Tucker et al., 2017). Recent studies have found that AP2/ERFs regulate the transcription of several cell wall-modifying genes. In persimmon (Diospyros kaki), DkERF8/16/19 bind to the DRE/CRT element of DkXTH9’s promoter and activate its transcription (Wang et al., 2017). DkERF8 and DkERF16 also activate DkXTH11 and EXPANSIN 4 (DkEXP4), respectively, via binding to their promoters (He et al., 2020).
In peach, PpeERF2 was found to bind directly to the PpePG1 promoter by yeast one-hybrid (Y1H) and electrophoretic mobility shift assay (EMSA), and further found to repress its expression by Agrobacterium infiltration (Wang et al., 2019a). During banana (Musa acuminata) ripening, MaDEAR1 represses the expression of MaEXP1/3, MaPG1, MaXTH10, MaPL3, and MaPME3 (Fan et al., 2016). In papaya (Carica papaya L.), CpERF9 binds to the GCC box of the CpPME1/2 and CpPG5 promoters and represses their expression (Fu et al., 2016, 2021). Notably, members of the F group characterized by the EAR motif have been widely found to inhibit cell wall-modifying gene expression. A recent study hypothesized that the rate of fruit softening depends on the balance between the ERF.F repressors and other activators. Another possibility is that ERF.F is inhibited after ripening begins, subsequently releasing the inhibition of cell wall-modification genes (Shi et al., 2022).
The G–C mutation in the EAR motif of apple MdERF4 impairs its transcriptional repression of MdERF3, thereby promoting ethylene production and loss of fruit firmness (Hu et al., 2020). Watermelon (Citrullus lanatus) ClERF4, associated with variations in fruit peel firmness, was identified via a combinatorial genetic map and bulk segregant analysis. An 11-bp indel and the neighboring single-nucleotide polymorphism in ClERF4 contributed to differences in rind hardness and cracking resistance (Liao et al., 2020). These findings suggest that ERF allelic mutations play an important role in textural fruit traits.
Cell wall modification contributes not only to softening but also to tissue expansion and growth. XTH is thought to be involved in maintaining the structural integrity of the cell wall during fruit development, while contributing to softening at the onset of ripening (Miedes and Lorences, 2009). This dual role is related to the dual biochemical function of XTH, with different members acting as xyloglucan endotransglucosylase (XET) or xyloglucan endohydrolase (XEH) (Rose et al., 2002). The expression and activity of several XTHs differ at different developmental stages of the fruit and are regulated by ethylene (Goulao et al., 2007; Tucker et al., 2017). It is assumed that AP2/ERFs participate in the change in cell wall state from fruit development through the onset of ripening to full ripeness by differentially regulating cell wall-modifying enzymes.
Color
Fruit color development at ripening depends mainly on the contents of flavonoids, carotenoids, and Chl (Wang et al., 2022). AP2/ERFs participate in fruit degreening by regulating Chl degradation. In citrus (Citrus reticulata), ethylene-induced CitERF6 and CitERF13 trigger Chl degradation by directly activating the expression of NON-YELLOW COLORING (CitNYC) and PHEOPHYTINASE (CitPPH), as identified by dual-luciferase and Y1H assay (Li et al., 2019). In apple, mutations in the coding region of MdERF17 affect peel degreening. Different numbers of serine repeats affect the transcription-regulatory activity of MdERF17 mutant alleles on Chl degradation-related genes, including MdPPH and MdNYC, as observed in a dual-luciferase reporter assay (Han et al., 2018). VvERF17 promotes Chl degradation in grape berry by activating several Chl catabolic genes, including CHLOROPHYLL-B-REDUCTASE (VvNOL), VvPPH, PHEOPHORBIDEα OXYGENASE (VvPAO), and RED CHLOROPHYLL CATABOLITE REDUCTASE (VvRCCR) (Lu et al., 2022).
Ethylene has been reported to regulate anthocyanin biosynthesis in many fruit (Ni et al., 2021). Ethylene treatment accelerates apple peel coloration, during which MdEIL1 activates MdMYB1 transcription. MdMYB1 promotes anthocyanin biosynthesis and activates the expression of MdERF3 (a key regulator of ethylene synthesis), providing positive feedback for ethylene signaling and fruit coloration (An et al., 2018). MdERF1B contributes to apple coloration by activating the transcription of MdMYB9 and MdMYB11 (Zhang J. et al., 2018). In strawberry (Fragaria × ananassa), FaRAV1 activates the expression of FaMYB10, an essential gene for anthocyanin biosynthesis, by binding to its promoter. FaRAV1 can also directly promote the transcription of CHALCONE SYNTHASE (FaCHS), FLAVANONE 3-HYDROXYLASE (FaF3H), DIHYDROFLAVONOL-4-REDUCTASE (FaDFR) and 3-GLYCOSYLTRANSFERASE (FaGT1), and enhance anthocyanin accumulation (Zhang Z. et al., 2020). Ethylene-induced PpERF105 activates the transcription of PpMYB140, which is a repressor of anthocyanin biosynthesis in pear (Pyrus spp.) (Ni et al., 2021).
Carotenoids are another major pigment component. The expression of MdAP2-34 promotes carotenoid accumulation in apple callus and fruit. MdAP2-34 can directly activate the expression of PHYTOENE SYNTHASE 2 (MdPSY2-1), which is a key gene in carotenoid biosynthesis (Dang et al., 2021). In citrus (C. sinensis) fruit, ethylene-induced CsERF061 activates the expression of 10 carotenoid-related genes—LYCOPENEβ- CYCLASE (LCYb2), PSY1, PHYTOENE DESATURASE (PDS), CAROTENOID ISOMERASE (CRTISO), LCYb1, β-CAROTENE HYDROXYLASE (BCH), ZEAXANTHIN EPOXIDASE (ZEP), 9-CIS-EPOXYCAROTENOID DIOXYGENASE (NCED3), CAROTENOID CLEAVAGE DIOXYGENASE (CCD1), and CCD4, thus enhancing carotenoid synthesis through multitarget regulation (Zhu et al., 2021).
Aroma
Volatile esters are the main components of the aroma of strawberry, apple, banana, and other fruit. Alcohol acyltransferase (AAT) is considered the key gene in ester biosynthesis and has a significant effect on aroma formation. Ethylene is thought to affect ester biosynthesis in apple by regulating AAT (Defilippi et al., 2005). Several ERF genes have been shown to be associated with the expression of AAT in strawberry via transcriptome analysis and weight co-expression network analysis (WGCNA). Overexpression of FveERF indeed activates AAT gene expression and ester accumulation in strawberry (Fragaria vesca) fruit (Li et al., 2020). Ethylene treatment increased volatile production in banana fruit, consistent with upregulation of volatile biosynthetic genes at the transcriptional level, including PYRUVATE DECARBOXYLASE (PDC) and ALCOHOL DEHYDROGENASE (ADH) (Yang et al., 2011). Chromatin immunoprecipitation (ChIP)-qPCR and EMSA revealed that banana MaDREB2 binds directly to the promoters of MaADH1 and MaPDC (Kuang et al., 2017).
The monoterpene E-geraniol and sesquiterpene (+)-valencene are important volatile compounds for flavor formation in sweet orange. CitERF71 binds directly to the promoter of TERPENE SYNTHASE 16 (CitTPS16) and activates its expression to regulate E-geraniol biosynthesis in citrus fruit (Li X. et al., 2017). Dual-luciferase assays indicated that ethylene-induced CitAP2.10 transactivates CsTPS1, regulating (+)-valencene biosynthesis (Shen et al., 2016). Transcriptome and biochemical analyses of AP2/ERF PaWRI1-2 revealed functions associated with fatty acid accumulation in avocado fruit (Persea americana Mill.), affecting fruit quality and nutritional value (Ge et al., 2021). In apple pericarp, MdERF3 binds to the DRE motif of α-FARNESENE SYNTHASE (MdAFS) and activates its expression to promote biosynthesis of α-farnesene, which is related to insect attraction and plant defense (Wang et al., 2020). In banana, the transcriptional activator MaERF9 interacts physically with the transcriptional repressor DNA BINDING WITH ONE FINGER 23 (MaDOF23) to antagonistically regulate the expression of two aroma-related genes, BRANCHED-CHAIN AMINO ACID TRANSAMINASE (MaCAT) and PYRUVATE DECARBOXYLASE (MaPDC) (Feng et al., 2016). These findings suggest that AP2/ERFs play a vital role in regulating fruit ripening-related aroma development.
APETALA2/ethylene responsive factor interacts with other proteins in regulating fruit ripening
Transcription factors can interact with many different proteins, including other transcription factors, co-regulators, and components of basal transcription complexes, resulting in effects on transcription factor cell localization, protein stability, protein–protein interactions, and DNA binding (Whitmarsh and Davis, 2000). Physical interactions between AP2/ERFs and other proteins have been widely reported, involving processes such as cell wall modification, anthocyanin synthesis, and flavor accumulation. In persimmon, DkERF8 and DkERF16, previously mentioned as direct regulators of cell wall modifications, interact with the DkNAC9 protein. Moreover, DkNAC9 binds directly to the promoter of ENDO-1,4-β-D-GLUCANASE (DkEGase1) and activates its expression, revealing a synergistic role for ERF and NAC in cell wall remodeling (Wu et al., 2020).
In apple, MdERF1B interacts with MdMYB9, MdMYB1, and MdMYB11 (all related to apple anthocyanin biosynthesis) proteins in yeast two-hybrid (Y2H), pull-down, and bimolecular fluorescence complementation (BiFC) assays, and binds to their promoters (Zhang J. et al., 2018). MdERF38 interacts with MdMYB1, which enhances the binding of MdMYB1 to the promoters of anthocyanin-biosynthesis genes (An et al., 2020). PyERF3 affects anthocyanin accumulation in pear by interacting with PyMYB114 (Yao et al., 2017). Pp4ERF24 and Pp12ERF96 interact with PpMYB114 and enhance PpMYB114-mediated UDP GLUCOSE:FLAVONOID 3-O-GLUCOSYLTRANSFERASE (PpUFGT) expression (Ni et al., 2019). CitRAV1 interacts with CitERF33 in citrus, enhancing the transcriptional activation of CHALCONE ISOMERASE (CitCHIL1) by CitERF33 and promoting flavonoid accumulation (Zhao et al., 2021). These findings provide insight into how ethylene regulates anthocyanin synthesis. In strawberry, FaERF#9 indirectly activates the expression of QUINONE OXIDOREDUCTASE (FaQR) by forming an ERF–MYB complex with FaMYB98, thus promoting the biosynthesis of 4-hydroxy-2,5-dimethyl-3(2H)-furanone and flavor accumulation (Zhang Y. et al., 2018).
AP2/ERFs with the EAR motif respond to various biotic and abiotic stresses in plants, including salinity, wounding, low temperature, drought and pathogens (Dong and Liu, 2010; Lu et al., 2011; Dong et al., 2012, 2015). EAR is the most common transcriptional-repression motif identified in plants. A total of 219 candidate transcriptional regulators with EAR motifs were identified in Arabidopsis, belonging to 21 different transcription-regulator families. The LxLxL type accounted for most of them (72%) (Kagale et al., 2010). EAR motif-mediated transcriptional repression is one of the principal mechanisms of gene regulation in plants, mainly through physical interactions with other co-repressors, including SWITCH INDEPENDENT 3 (SIN3, Song et al., 2005), SIN3 ASSOCIATED POLYPEPTIDE 18 (SAP18, Hill et al., 2008), TOPLESS/TOPLESS-RELATED (TPL/TPR), and histone deacetylases (Deng et al., 2022).
In apple, MdTPL4 and HISTONE DEACETYLASE 19 (MdHDA19) are recruited by the EAR motif-containing MdERF4, and the protein complex inhibits MdACSa expression, thereby affecting ethylene synthesis and fruit ripening (Hu et al., 2022a). In tomato, SlERF.F12 (with both types of EAR motifs) represses the expression of multiple ethylene-synthesis and cell wall-degradation genes by recruiting TPL2 and HDA1/HDA3 (Deng et al., 2022). In banana, MaERF11, with the EAR motif, recruits MaHDA1 and represses the expression of a range of ripening-related genes through histone deacetylation (Han et al., 2016). The EAR motif works in conjunction with both TPL and histone deacetylase, supporting a model in which it mediates transcriptional repression by recruiting chromatin remodelers. Moreover, conserved residues adjacent and integral to the EAR motif are involved in the post-translational regulation (Kagale et al., 2010). For example, serine and threonine residues within and around the EAR motifs are regulated by phosphorylation (Kagale and Rozwadowski, 2010).
Post-translational modifications are essential regulatory mechanisms in plants that respond to extracellular signaling molecules and environmental changes, resulting in rapid changes in protein status and transcriptional activity (Whitmarsh and Davis, 2000). In apple, MdERF17 interacts with and is phosphorylated by MAP KINASE4 (MdMPK4-14G). MdERF17 mutants with different numbers of serine repeats display diverse phosphorylation profiles. Phosphorylation of MdERF17 by MdMPK4-14G is necessary to regulate peel Chl degradation (Wang et al., 2022). Moreover, BTB AND TAZ DOMAIN PROTEIN MdBT2, with ubiquitinase activity, accelerates MdERF38 protein degradation and reduces MdERF38-promoted anthocyanin biosynthesis in apple coloration (An et al., 2020).
APETALA2/ethylene responsive factor in plant hormone signaling
A complex network of hormonal crosstalk coordinates fruit ripening. Early research on ripening-related hormones focused on ethylene, especially in climacteric fruits. Ethylene treatment promotes a climacteric rise in banana, and upregulation of MaERF9 and downregulation of MaERF11 are thought to contribute to fruit-ripening regulation (Xiao et al., 2013). In fig (Ficus carica L.), exogenous ethephon treatment results in accelerated fruit ripening; downregulation of the high expression of AP2/ERFs was found in ethephon-treated fruit 2 and 4 days after the treatment except for two genes, and the ethephon-induced AP2/ERF repression generally ended 6 days after the application (Cui et al., 2021).
AP2/ERFs are not only regulated by ethylene; they also regulate ethylene synthesis (Table 2). In pear, ERF24 binds directly to the promoter of ACO54 and activates its expression, while overexpression of ACO54 also increases the expression of ERF24 (Hao et al., 2018). In apple, MdERF2 binds to the promoter of MdERF3 and represses its expression, thereby reducing MdERF3-promoted transcription of MdACS1. Moreover, MdERF2 binds directly to the promoter of MdACS1 and represses its transcription, resulting in inhibition of ethylene biosynthesis (Li et al., 2016). MdMYB1 can promote the expression of MdERF3, revealing a synergistic regulation mechanism (An et al., 2018). In banana, MaERF11 binds to the promoters of MaACS1 and MaACO1 and represses their expression, whereas MaERF9 activates MaACO1 transcription (Xiao et al., 2013). In peach, ethylene-induced PpERF.A16 enhances ethylene biosynthesis by directly activating the expression of PpACS1 and PpACO1. At the same time, PpERF.A16 is transcriptionally regulated by PpNAC.A59 (Guo et al., 2021).
Several ERFs regulate ethylene synthesis and fruit ripening in tomato. SlERF6 silencing by RNAi increases ethylene levels and carotenoid content during fruit ripening (Lee et al., 2012). APETALA2a (AP2a) negatively regulates fruit ripening. RNAi repression of SlAP2a results in overproduction of ethylene and altered carotenoid accumulation (Chung et al., 2010). Overexpression of SlERF.B3-SRDX (a chimeric dominant repressor version) shows contrasting effects on fruit ripening, resulting in delayed ripening onset, enhanced ethylene production and fruit softening, and reduced carotenoid biosynthesis. Consistent with the phenotypes, the expression of ripening-related genes is highly induced, such as ethylene-synthesis genes ACS2, ACS4 and ACO1, softening gene PG2A, developmental regulators RIN, NOR and CNR, and a set of ERF genes (Liu et al., 2013, 2014).
The regulatory role of ethylene in fruit ripening is affected by the signaling network formed by interactions with other hormones and transcription factors (Kou et al., 2021), the latter serving as correlated connectors for the crosstalk (Li et al., 2022). In tomato, the ethylene-responsive factor SlPti4 regulates fruit ripening by affecting ABA metabolism and signaling, and silencing SlPti4 results in increased ABA accumulation and decreased ethylene release (Sun et al., 2018). In peach, PpeERF2 binds to and represses the transcription of two ABA-biosynthesis genes, PpeNCED2/3 (Wang et al., 2019a). In contrast, PpERF3 transactivates PpNCED2/3, thereby increasing ABA biosynthesis (Wang et al., 2019b).
Crosstalk between auxin and ethylene is necessary for fruit development and ripening. Downregulation of AUXIN-RESPONSIVE FACTOR 2 (ARF2) in tomato, a downstream factor in auxin signaling, results in ripening defects, reduced climacteric ethylene synthesis and delayed ripening. SlARF2-RNAi lines showed significant downregulation of ethylene receptor genes ETR3 and ETR4, and 12 and 5 AP2/ERFs were found downregulated and upregulated, respectively, in the tomato fruit (Hao et al., 2015). In apple, 4 mM auxin naphthaleneacetic acid treatment before the commercial harvest stage induced ethylene synthesis and fruit ripening; MdARF5 activated the transcription of MdERF2, two MdACSs and MdACO1 by directly binding to their promoters (Yue et al., 2020). Ethephon treatment reduced free indole acetic acid (IAA) content in pear; during this process, PuERF2 activated the transcription of GRETCHEN HAGEN 3 (PuGH3.1), and PuGH3.1 conjugated free IAA to inactive IAA-amide (Yue et al., 2019). In tomato, SlERF.B3 integrates ethylene and auxin signaling by directly binding to the promoter of SlIAA27; ectopic expression of SlERF.B3 results in phenotypes similar to those of SlIAA27-downregulated lines, such as elongated primary root and remarkably increased lateral root formation (Liu et al., 2018). A positive feedback loop of ripening regulation was revealed in peach: PpIAA1, which can be upregulated by both ethylene and auxin, promoted the expression of PpACS1 and PpNCED2/3. PpERF4 activated the transcription of PpIAA1 and physically interacted with PpIAA1 protein, thereby enhancing the latter’s transcription-activation ability (Wang et al., 2021).
Although most studies on jasmonate (JA) have focused on responses to biotic and abiotic stresses, recent studies have revealed its involvement in fruit-ripening regulation. In apple, the transcription factor MYELOCYTOMATOSIS-RELATED PROTEINS 2 (MdMYC2), involved in JA signaling, binds directly to the promoters of MdERF3, MdACS1, and MdACO1 and activates their expression. MdMYC2 also physically interacts with MdERF2, thereby reducing the latter’s transcriptional repression of MdERF3 and MdACS1, and increasing ethylene production (Li T. et al., 2017). MdERF4 physically interacts with JASMONATE ZIM-DOMAIN (JAZ) to form a repressor complex that acts as a molecular link between ethylene and JA signaling (Hu et al., 2022b).
Regulation of APETALA2/ethylene responsive factor expression by epigenetic mechanisms
Epigenetic modifications affect mainly DNA methylation, as well as the methylation, acetylation, phosphorylation, and ubiquitination status of histones (Giovannoni et al., 2017). Examination of the role of DNA methylation during ripening in several fruit crops has revealed the occurrence of global epigenome reprogramming. For example, 5-azacytidine, a general inhibitor of DNA (cytosine-5) methyltransferase, promotes early ripening of immature tomatoes. Using whole-genome bisulfite sequencing, 52,095 differentially methylated regions (DMRs) were identified, representing 1% of the genome. During tomato fruit ripening, DNA methylation levels at the 5′ end of genes generally declined across the genome. The demethylation events promoted binding of a master ripening-related regulator, RIN, to promoters of a series of ripening genes, including several AP2/ERFs (Zhong et al., 2013).
Overall loss of DNA methylation was also found during strawberry ripening. A total of 2766 DMRs were identified, of which 466 were hypermethylated and 2,300 were hypomethylated (Cheng et al., 2018). In contrast, a global increase in DNA methylation was found during orange fruit ripening, which led to the repression or activation of hundreds of genes. The application of DNA-methylation inhibitor interfered with orange fruit ripening, indicating that DNA hypermethylation is essential for proper ripening (Huang et al., 2019). Increased methylation activity during fruit ripening has also been found in apple (El-Sharkawy et al., 2015).
The activator PpERF61 promotes volatile linalool synthesis in peach fruit by directly binding to DRE/CRT elements on the promoters of PpTPS1/3. Ripening-induced expression of PpERF61 was associated with DNA demethylation of its promoter (Wei et al., 2022). AP2/ERFs are sensitive to methylation at their binding sites (O’Malley et al., 2016) but overall, the role of DNA methylation in AP2/ERF regulation remains poorly understood. Considering the prevalence of changes in DNA methylation during fruit ripening and the extensive regulation by AP2/ERF of ripening genes, it is reasonable to assume that DNA methylation plays a vital role in the AP2/ERF regulation mechanism, but further study is required.
Post-translational modifications of histones, including methylation, acetylation, phosphorylation and ubiquitination, regulate gene expression by affecting chromatin conformation. Increasing evidence suggests that histone modifications play an important role in fruit-ripening regulation (Li et al., 2022). Studies of several fruit crops have found that histone modifiers are preferentially or specifically expressed in fruit and perform phasic differences, suggesting that they are involved in the regulation of fruit development (Aiese Cigliano et al., 2013; Xu et al., 2015; Gallusci et al., 2016). The acetylation status of histones has been best studied. This is a reversible mark regulated by histone acetyltransferases and histone deacetylases, usually related to genes’ transcriptional activity (Shen et al., 2015).
In tomato fruit, inhibition of SlHDA3 or SlHDA1 expression causes significant upregulation of several ethylene-biosynthesis genes and cell wall-modification genes, resulting in accelerated ripening and reduced storability (Guo et al., 2017b,2018). However, inhibition of SlHDT3, which belongs to the HD2 family of histone deacetylases, has the opposite effect (Guo et al., 2017a). Recent studies have found that histone modifiers, such as histone deacetylases, are recruited by AP2/ERFs in fruit-ripening regulation. In apple, MdHDA19 is recruited to the MdERF4–MdTPL4 complex and inhibits fruit ripening by reducing the expression of MdACSa (Hu et al., 2022a). In tomato, the SlERF.F12–TPL2–HDA1/HDA3 protein complex represses the expression of ripening-related genes, including ACS2, ACS4, PG2a, and PL. These examples of transcriptional repression rely on reduced levels of histone acetylation marks H3K9Ac and H3K27Ac in the promoter regions of the target genes (Deng et al., 2022). In banana, MaERF11 recruits MaHDA1 to repress the expression of ethylene-biosynthesis and cell wall-degradation genes (Han et al., 2016). As already noted, histone deacetylases play an essential role in the EAR motif-mediated mechanism of transcriptional repression.
MicroRNAs (miRNAs) are an important class of endogenous small RNAs in plants; they target mRNA through complementary base pairing and induce gene silencing by inhibiting translation or initiating mRNA degradation. The miRNAs are essential regulators, at the post-transcriptional level, of various biological processes in plants, such as development and stress responses (He et al., 2022). Mature miRNAs are usually 20–24 nucleotides in length, and differences in length lead to distinct functions (Manavella et al., 2012). MiR172–AP2 is a conserved miRNA-target module in plants. A previous study in Arabidopsis found that miR172 specifically targets the mRNA of AP2 (Chen, 2004). There is increasing evidence that this module plays a crucial role in fruit ripening. In tomato, miR172 specifically targets SlAP2a. Overexpression of miR172 represses SlAP2a expression, resulting in enhanced ethylene biosynthesis and coloration (Chung et al., 2020). In apple, MdAP2_1a, targeted by miR172, transactivates MdMYB10 and positively regulates anthocyanin biosynthesis. Overexpression of miR172 represses the expression of MdAP2_1a, thereby inhibiting anthocyanin accumulation (Ding et al., 2022). A study on an apple breeding population revealed a transposon insertional allele of miR172 with reduced miR172 expression which was associated with large fruit. Overexpression of miR172 negatively affected fruit development and fruit size (Yao et al., 2015). Moreover, in Arabidopsis, AP2 positively regulated miR156 but negatively regulated miR172, and both miRNAs influenced AP2 expression, indicating that AP2 and miRNAs have complex direct feedback loops in plants (Yant et al., 2010).
Plant genomes encode a considerable number of long non-coding RNAs (lncRNAs)—usually over 200 nucleotides in length with no discernable coding potential—which play an important role in essential biological processes (Chekanova, 2015). The lncRNAs affect all elements of genes, such as promoters, untranslated regions, exons, introns, and terminators, and control gene expression at the levels of chromatin accessibility, transcription, splicing, and translation (Wierzbicki et al., 2021). In apple, the lncRNA MdLNC499, located near MdERF109, was identified as a cis-regulator of MdERF109 expression by Promoter:β-glucuronidase reporter analysis and Hi-C sequencing. Promoter cis-element analysis found the presence of a W-box element in MdLNC499 promoter, which is regulated by MdWRKY1. The MdWRKY1–MdLNC499–MdERF109 transcriptional cascade was reconstructed in apple fruit and callus by transient expression and stable transformation. MdERF109 promotes coloration by directly binding to anthocyanin-related gene promoters, including MdCHS, MdUFGT, and BASIC HELIX–LOOP–HELIX 3 (MdbHLH3) (Ma et al., 2021).
Conclusion and perspective
The AP2/ERF superfamily is a large class of transcription factors in plants that exhibit coordinated changes in expression during fruit ripening. AP2/ERFs play critical regulatory roles in intrinsic and extrinsic quality development during fruit ripening (Figure 2). However, our understanding of the molecular mechanisms of AP2/ERF is still limited, especially with respect to the identification of co-regulators, and the influence of post-translational and epigenetic modifications. With the application of modern molecular biology and high-throughput sequencing technology, such as chromatin immunoprecipitation, DNA affinity purification sequencing, immunoprecipitation coupled with mass spectrometry analysis, next-generation sequencing, genome-wide association studies, the new gene-editing system CRISPR/Cas9, and so on, future dissection and exploration of the AP2/ERF regulatory network will broaden our understanding of quality formation during fruit ripening. In the 1990s, great effort was made to improve tomato storability by deciphering key genes in ethylene synthesis. Today, functional analyses of many ripening-related AP2/ERFs has allowed us to regulate the formation and maintenance of specific quality traits more precisely, while avoiding the interference of other, unexpected quality traits. In the future, altering the function and expression of specific AP2/ERF family members through synthetic biology techniques will provide new approaches to improving internal and external fruit quality and storability.
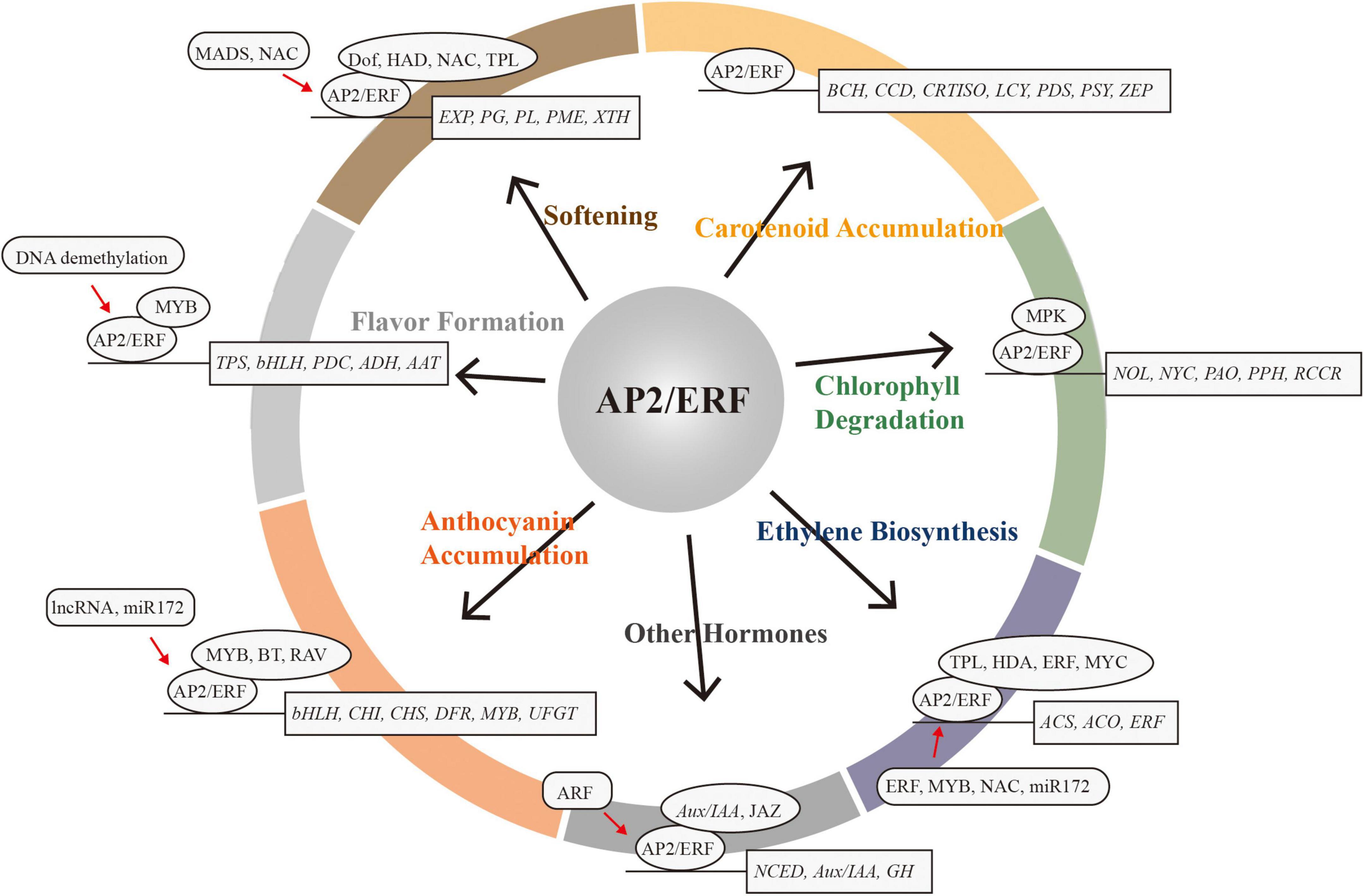
Figure 2. The AP2/ERF superfamily acts as a critical regulator in fruit ripening. Target genes are in rectangles. Protein–protein interactions or co-regulators are in ovals. Red arrows represent expression regulation by AP2/ERF.
Author contributions
HM planned the review manuscript. YZ, ZF, YC, XG, SC, and HM prepared the manuscript. All authors have read and approved the manuscript for publication.
Funding
This work was supported by the National Natural Science Foundation of China project NSFC (31372007).
Conflict of interest
The authors declare that the research was conducted in the absence of any commercial or financial relationships that could be construed as a potential conflict of interest.
Publisher’s note
All claims expressed in this article are solely those of the authors and do not necessarily represent those of their affiliated organizations, or those of the publisher, the editors and the reviewers. Any product that may be evaluated in this article, or claim that may be made by its manufacturer, is not guaranteed or endorsed by the publisher.
References
Aiese Ciglid, R., Sanseverino, W., Cremona, G., Ercolano, M. R., Conicella, C., and Consiglio, F. M. (2013). Genome-wide analysis of histone modifiers in tomato: gaining an insight into their developmental roles. BMC Genom. 14:57. doi: 10.1186/1471-2164-14-57
Allen, M. D., Yamasaki, K., Ohme-Takagi, M., Tateno, M., and Suzuki, M. (1998). A novel mode of DNA recognition by a β-sheet revealed by the solution structure of the GCC-box binding domain in complex with DNA. EMBO J. 17, 5484–5496. doi: 10.1093/emboj/17.18.5484
An, J. P., Wang, X. F., Li, Y. Y., Song, L. Q., Zhao, L. L., You, C. X., et al. (2018). EIN3-LIKE1, MYB1, and ETHYLENE RESPONSE FACTOR3 act in a regulatory loop that synergistically modulates ethylene biosynthesis and anthocyanin accumulation. Plant Physiol. 178, 808–823. doi: 10.1104/pp.18.00068
An, J. P., Zhang, X. W., Bi, S. Q., You, C. X., Wang, X. F., and Hao, Y. J. (2020). The ERF transcription factor MdERF38 promotes drought stress-induced anthocyanin biosynthesis in apple. Plant J. 101, 573–589. doi: 10.1111/tpj.14555
Barry, C. S., and Giovannoni, J. J. (2006). Ripening in the tomato Green-ripe mutant is inhibited by ectopic expression of a protein that disrupts ethylene signaling. Proc. Natl. Acad. Sci. U S A 103, 7923–7928. doi: 10.1073/pnas.0602319103
Binder, B. M., Rodriguez, F. I., and Bleecker, A. B. (2010). The copper transporter RAN1 is essential for biogenesis of ethylene receptors in Arabidopsis. J. Biol. Chem. 285, 37263–37270. doi: 10.1074/jbc.M110.170027
Chekanova, J. A. (2015). Long non-coding RNAs and their functions in plants. Curr. Opin. Plant Biol. 27, 207–216. doi: 10.1016/j.pbi.2015.08.003
Chen, T., Qin, G., and Tian, S. (2020). Regulatory network of fruit ripening: current understanding and future challenges. New Phytol. 228, 1219–1226. doi: 10.1111/nph.16822
Chen, X. (2004). A microRNA as a translational repressor of APETALA2 in Arabidopsis flower development. Science 303, 2022–2025. doi: 10.1126/science.1088060
Cheng, J., Niu, Q., Zhang, B., Chen, K., Yang, R., Zhu, J. K., et al. (2018). Downregulation of RdDM during strawberry fruit ripening. Genome Biol. 19, 212. doi: 10.1186/s13059-018-1587-x
Chung, M. Y., Nath, U. K., Vrebalov, J., Gapper, N., Lee, J. M., Lee, D. J., et al. (2020). Ectopic expression of miRNA172 in tomato (Solanum lycopersicum) reveals novel function in fruit development through regulation of an AP2 transcription factor. BMC Plant Biol. 20:283. doi: 10.1186/s12870-020-02489-y
Chung, M. Y., Vrebalov, J., Alba, R., Lee, J., McQuinn, R., Chung, J. D., et al. (2010). A tomato (Solanum lycopersicum) APETALA2/ERF gene, SlAP2a, is a negative regulator of fruit ripening. Plant J. 64, 936–947. doi: 10.1111/j.1365-313X.2010.04384.x
Cui, Y., Zhai, Y., Flaishman, M., Li, J., Chen, S., Zheng, C., et al. (2021). Ethephon induces coordinated ripening acceleration and divergent coloration responses in fig (Ficus carica L.) flowers and receptacles. Plant Mol. Biol. 105, 347–364. doi: 10.1007/s11103-020-01092-x
Dang, Q., Sha, H., Nie, J., Wang, Y., Yuan, Y., and Jia, D. (2021). An apple (Malus domestica) AP2/ERF transcription factor modulates carotenoid accumulation. Hortic. Res 8, 223. doi: 10.1038/s41438-021-00694-w
Defilippi, B. G., Kader, A. A., and Dandekar, A. M. (2005). Apple aroma: alcohol acyltransferase, a rate limiting step for ester biosynthesis, is regulated by ethylene. Plant Sci. 168, 1199–1210. doi: 10.1016/j.plantsci.2004.12.018
Deng, H., Chen, Y., Liu, Z., Liu, Z., Shu, P., Wang, R., et al. (2022). SlERF.F12 modulates the transition to ripening in tomato fruit by recruiting the co-repressor Topless and histone deacetylases to repress key ripening genes. Plant Cell 34, 1250–1272. doi: 10.1093/plcell/koac025
Ding, T., Tomes, S., Gleave, A. P., Zhang, H., Dare, A. P., Plunkett, B., et al. (2022). microRNA172 targets APETALA2 to regulate flavonoid biosynthesis in apple (Malus domestica). Hortic. Res [Epub ahead of print]. doi: 10.1093/hr/uhab007
Dong, C. J., and Liu, J. Y. (2010). The Arabidopsis EAR-motif-containing protein RAP2. 1 functions as an active transcriptional repressor to keep stress responses under tight control. BMC Plant Biol. 10:47. doi: 10.1186/1471-2229-10-47
Dong, L., Cheng, Y., Wu, J., Cheng, Q., Li, W., Fan, S., et al. (2015). Overexpression of GmERF5, a new member of the soybean EAR motif-containing ERF transcription factor, enhances resistance to Phytophthora sojae in soybean. J. Exp. Bot. 66, 2635–2647. doi: 10.1093/jxb/erv078
Dong, W., Ai, X., Xu, F., Quan, T., Liu, S., and Xia, G. (2012). Isolation and characterization of a bread wheat salinity responsive ERF transcription factor. Gene 511, 38–45. doi: 10.1016/j.gene.2012.09.039
El-Sharkawy, I., Liang, D., and Xu, K. (2015). Transcriptome analysis of an apple (Malus × domestica) yellow fruit somatic mutation identifies a gene network module highly associated with anthocyanin and epigenetic regulation. J. Exp. Bot. 66, 7359–7376. doi: 10.1093/jxb/erv433
Fan, Z. Q., Kuang, J. F., Fu, C. C., Shan, W., Han, Y. C., Xiao, Y. Y., et al. (2016). The banana transcriptional repressor MaDEAR1 negatively regulates cell wall-modifying genes involved in fruit ripening. Front. Plant Sci. 7:1021. doi: 10.3389/fpls.2016.01021
Feng, B. H., Han, Y. C., Xiao, Y. Y., Kuang, J. F., Fan, Z. Q., Chen, J. Y., et al. (2016). The banana fruit Dof transcription factor MaDof23 acts as a repressor and interacts with MaERF9 in regulating ripening-related genes. J. Exp. Bot. 67, 2263–2275. doi: 10.1093/jxb/erw032
Feng, K., Hou, X. L., Xing, G. M., Liu, J. X., Duan, A. Q., Xu, Z. S., et al. (2020). Advances in AP2/ERF super-family transcription factors in plant. Crit. Rev. Biotechnol. 40, 750–776. doi: 10.1080/07388551.2020.1768509
Forlani, S., Masiero, S., and Mizzotti, C. (2019). Fruit ripening: the role of hormones, cell wall modifications, and their relationship with pathogens. J. Exp. Bot. 70, 2993–3006. doi: 10.1093/jxb/erz112
Fu, C. C., Chen, H. J., Gao, H. Y., Wang, S. L., Wang, N., Jin, J. C., et al. (2021). Papaya CpMADS4 and CpNAC3 co-operatively regulate ethylene signal genes CpERF9 and CpEIL5 during fruit ripening. Postharvest Biol. Technol. 175, 111485. doi: 10.1016/j.postharvbio.2021.111485
Fu, C. C., Han, Y. C., Qi, X. Y., Shan, W., Chen, J. Y., Lu, W. J., et al. (2016). Papaya CpERF9 acts as a transcriptional repressor of cell-wall-modifying genes CpPME1/2 and CpPG5 involved in fruit ripening. Plant Cell Rep. 35, 2341–2352. doi: 10.1007/s00299-016-2038-3
Gallusci, P., Hodgman, C., Teyssier, E., and Seymour, G. B. (2016). DNA methylation and chromatin regulation during fleshy fruit development and ripening. Front. Plant Sci. 7:807. doi: 10.3389/fpls.2016.00807
Gao, J., Zhang, Y., Li, Z., and Liu, M. (2020). Role of ethylene response factors (ERFs) in fruit ripening. Food Qual. Safety 4, 15–20. doi: 10.1093/fqsafe/fyz042
Ge, Y., Zang, X., Yang, Y., Wang, T., and Ma, W. (2021). In-depth analysis of potential PaAP2/ERF transcription factor related to fatty acid accumulation in avocado (Persea americana Mill.) and functional characterization of two PaAP2/ERF genes in transgenic tomato. Plant Physiol. Biochem. 158, 308–320. doi: 10.1016/j.plaphy.2020.11.016
Gibbs, D. J., Lee, S. C., Isa, N. M., Gramuglia, S., Fukao, T., Bassel, G. W., et al. (2011). Homeostatic response to hypoxia is regulated by the N-end rule pathway in plants. Nature 479, 415–418. doi: 10.1038/nature10534
Giordano, A., Santo Domingo, M., Quadrana, L., Pujol, M., Martin-Hernandez, A. M., and Garcia-Mas, J. (2022). CRISPR/Cas9 gene editing uncovers the roles of CONSTITUTIVE TRIPLE RESPONSE 1 and REPRESSOR OF SILENCING 1 in melon fruit ripening and epigenetic regulation. J. Exp. Bot. 73, 4022–4033. doi: 10.1093/jxb/erac148
Giovannoni, J., Nguyen, C., Ampofo, B., Zhong, S., and Fei, Z. (2017). The epigenome and transcriptional dynamics of fruit ripening. Annu. Rev. Plant Biol. 68, 61–84. doi: 10.1146/annurev-arplant-042916-040906
Girardi, C. L., Rombaldi, C. V., Dal Cero, J., Nobile, P. M., Laurens, F., Bouzayen, M., et al. (2013). Genome-wide analysis of the AP2/ERF superfamily in apple and transcriptional evidence of ERF involvement in scab pathogenesis. Sci. Hortic. 151, 112–121. doi: 10.1016/j.scienta.2012.12.017
Goulao, L. F., Santos, J., de Sousa, I., and Oliveira, C. M. (2007). Patterns of enzymatic activity of cell wall-modifying enzymes during growth and ripening of apples. Postharvest Biol. Technol. 43, 307–318. doi: 10.1016/j.postharvbio.2006.10.002
Guo, J. E., Hu, Z., Li, F., Zhang, L., Yu, X., Tang, B., et al. (2017a). Silencing of histone deacetylase SlHDT3 delays fruit ripening and suppresses carotenoid accumulation in tomato. Plant Sci. 265, 29–38. doi: 10.1016/j.plantsci.2017.09.013
Guo, J. E., Hu, Z., Zhu, M., Li, F., Zhu, Z., Lu, Y., et al. (2017b). The tomato histone deacetylase SlHDA1 contributes to the repression of fruit ripening and carotenoid accumulation. Sci. Rep. 7, 1–10. doi: 10.1038/s41598-017-08512-x
Guo, J. E., Hu, Z., Yu, X., Li, A., Li, F., Wang, Y., et al. (2018). A histone deacetylase gene, SlHDA3, acts as a negative regulator of fruit ripening and carotenoid accumulation. Plant Cell Rep. 37, 125–135. doi: 10.1007/s00299-017-2211-3
Guo, Z. H., Zhang, Y. J., Yao, J. L., Xie, Z. H., Zhang, Y. Y., Zhang, S. L., et al. (2021). The NAM/ATAF1/2/CUC2 transcription factor PpNAC.A59 enhances PpERF.A16 expression to promote ethylene biosynthesis during peach fruit ripening. Hortic. Res 8, 209. doi: 10.1038/s41438-021-00644-6
Han, Y. C., Kuang, J. F., Chen, J. Y., Liu, X. C., Xiao, Y. Y., Fu, C. C., et al. (2016). Banana transcription factor MaERF11 recruits histone deacetylase MaHDA1 and represses the expression of MaACO1 and expansins during fruit ripening. Plant Physiol. 171, 1070–1084. doi: 10.1104/pp.16.00301
Han, Z., Hu, Y., Lv, Y., Rose, J. K., Sun, Y., Shen, F., et al. (2018). Natural variation underlies differences in ETHYLENE RESPONSE FACTOR17 activity in fruit peel degreening. Plant Physiol. 176, 2292–2304. doi: 10.1104/pp.17.01320
Hao, P. P., Wang, G. M., Cheng, H. Y., Ke, Y. Q., Qi, K. J., Gu, C., et al. (2018). Transcriptome analysis unravels an ethylene response factor involved in regulating fruit ripening in pear. Physiol. Planta. 163, 124–135. doi: 10.1111/ppl.12671
Hao, Y., Hu, G., Breitel, D., Liu, M., Mila, I., Frasse, P., et al. (2015). Auxin response factor SlARF2 is an essential component of the regulatory mechanism controlling fruit ripening in tomato. PLoS Genet. 11:e1005649. doi: 10.1371/journal.pgen.1005649
He, M., Kong, X., Jiang, Y., Qu, H., and Zhu, H. (2022). MicroRNAs: emerging regulators in horticultural crops. Trends Plant Sci [Epub ahead of print]. doi: 10.1016/j.tplants.2022.03.011
He, Y., Xue, J., Li, H., Han, S., Jiao, J., and Rao, J. (2020). Ethylene response factors regulate ethylene biosynthesis and cell wall modification in persimmon (Diospyros kaki L.) fruit during ripening. Postharvest Biol. Technol. 168, 111255. doi: 10.1016/j.postharvbio.2020.111255
Hill, K., Wang, H., and Perry, S. E. (2008). A transcriptional repression motif in the MADS factor AGL15 is involved in recruitment of histone deacetylase complex components. Plant J. 53, 172–185. doi: 10.1111/j.1365-313X.2007.03336.x
Hu, Y., Han, Z., Sun, Y., Wang, S., Wang, T., Wang, Y., et al. (2020). ERF4 affects fruit firmness through TPL4 by reducing ethylene production. Plant J. 103, 937–950. doi: 10.1111/tpj.14884
Hu, Y., Han, Z., Wang, T., Li, H., Li, Q., Wang, S., et al. (2022a). Ethylene response factor MdERF4 and histone deacetylase MdHDA19 suppress apple fruit ripening through histone deacetylation of ripening-related genes. Plant Physiol. 188, 2166–2181. doi: 10.1093/plphys/kiac016
Hu, Y., Sun, H., Han, Z., Wang, S., Wang, T., Li, Q., et al. (2022b). ERF4 affects fruit ripening by acting as a JAZ interactor between ethylene and jasmonic acid hormone signaling pathways. Hortic. Plant J doi: 10.1016/j.hpj.2022.01.002
Huang, H., Liu, R., Niu, Q., Tang, K., Zhang, B., Zhang, H., et al. (2019). Global increase in DNA methylation during orange fruit development and ripening. Proc. Natl. Acad. Sci. USA 116, 1430–1436. doi: 10.1073/pnas.1815441116
Huang, W., Hu, N., Xiao, Z., Qiu, Y., Yang, Y., Yang, J., et al. (2022). A molecular framework of ethylene-mediated fruit growth and ripening processes in tomato. Plant Cell [Epub ahead of print]. doi: 10.1093/plcell/koac146
Ikeda, M., and Ohme-Takagi, M. (2009). A novel group of transcriptional repressors in Arabidopsis. Plant Cell Physiol. 50, 970–975. doi: 10.1093/pcp/pcp048
Ito, T. M., Polido, P. B., Rampim, M. C., Kaschuk, G., and Souza, S. G. H. (2014). Genome-wide identification and phylogenetic analysis of the AP2/ERF gene superfamily in sweet orange (Citrus sinensis). Genet. Mol. Res. 13, 7839–7851. doi: 10.4238/2014.September.26.22
Jofuku, K. D., Den Boer, B. G., Van Montagu, M., and Okamuro, J. K. (1994). Control of Arabidopsis flower and seed development by the homeotic gene APETALA2. Plant Cell 6, 1211–1225. doi: 10.1105/tpc.6.9.1211
Ju, C., and Chang, C. (2015). Mechanistic insights in ethylene perception and signal transduction. Plant Physiol. 169, 85–95. doi: 10.1104/pp.15.00845
Kagale, S., Links, M. G., and Rozwadowski, K. (2010). Genome-wide analysis of ethylene-responsive element binding factor-associated amphiphilic repression motif-containing transcriptional regulators in Arabidopsis. Plant Physiol. 152, 1109–1134. doi: 10.1104/pp.109.151704
Kagale, S., and Rozwadowski, K. (2010). Small yet effective: the ethylene responsive element binding factor-associated amphiphilic repression (EAR) motif. Plant Signal. Behav. 5, 691–694. doi: 10.4161/psb.5.6.11576
Kagaya, Y., Ohmiya, K., and Hattori, T. (1999). RAV1, a novel DNA-binding protein, binds to bipartite recognition sequence through two distinct DNA-binding domains uniquely found in higher plants. Nucleic Acids Res. 27, 470–478. doi: 10.1093/nar/27.2.470
Kou, X., Feng, Y., Yuan, S., Zhao, X., Wu, C., Wang, C., et al. (2021). Different regulatory mechanisms of plant hormones in the ripening of climacteric and non-climacteric fruits: a review. Plant Mol. Biol. 107, 477–497. doi: 10.1007/s11103-021-01199-9
Kuang, J. F., Chen, J. Y., Liu, X. C., Han, Y. C., Xiao, Y. Y., Shan, W., et al. (2017). The transcriptional regulatory network mediated by banana (Musa acuminata) dehydration-responsive element binding (MaDREB) transcription factors in fruit ripening. New Phytol. 214, 762–781. doi: 10.1111/nph.14389
Lee, J. M., Joung, J. G., McQuinn, R., Chung, M. Y., Fei, Z., Tieman, D., et al. (2012). Combined transcriptome, genetic diversity and metabolite profiling in tomato fruit reveals that the ethylene response factor SlERF6 plays an important role in ripening and carotenoid accumulation. Plant J. 70, 191–204. doi: 10.1111/j.1365-313X.2011.04863.x
Li, S. J., Xie, X. L., Liu, S. C., Chen, K. S., and Yin, X. R. (2019). Auto- and mutual-regulation between two CitERFs contribute to ethylene-induced citrus fruit degreening. Food Chem. 299, 125163. doi: 10.1016/j.foodchem.2019.125163
Li, T., Jiang, Z., Zhang, L., Tan, D., Wei, Y., Yuan, H., et al. (2016). Apple (Malus domestica) MdERF2 negatively affects ethylene biosynthesis during fruit ripening by suppressing MdACS1 transcription. Plant J. 88, 735–748. doi: 10.1111/tpj.13289
Li, T., Xu, Y., Zhang, L., Ji, Y., Tan, D., Yuan, H., et al. (2017). The jasmonate-activated transcription factor MdMYC2 regulates ETHYLENE RESPONSE FACTOR and ethylene biosynthetic genes to promote ethylene biosynthesis during apple fruit ripening. Plant Cell 29, 1316–1334. doi: 10.1105/tpc.17.00349
Li, X., Xu, Y., Shen, S., Yin, X., Klee, H., Zhang, B., et al. (2017). Transcription factor CitERF71 activates the terpene synthase gene CitTPS16 involved in the synthesis of E-geraniol in sweet orange fruit. J. Exp. Bot. 68, 4929–4938. doi: 10.1093/jxb/erx316
Li, X., Wang, X., Zhang, Y., Zhang, A., and You, C. X. (2022). Regulation of fleshy fruit ripening: from transcription factors to epigenetic modifications. Hortic. Res [Epub ahead of print]. doi: 10.1093/hr/uhac013
Li, Z., Wang, Z., Wang, K., Liu, Y., Hong, Y., Chen, C., et al. (2020). Co-expression network analysis uncovers key candidate genes related to the regulation of volatile esters accumulation in Woodland strawberry. Planta 252, 55. doi: 10.1007/s00425-020-03462-7
Liao, N., Hu, Z., Li, Y., Hao, J., Chen, S., Xue, Q., et al. (2020). Ethylene-responsive factor 4 is associated with the desirable rind hardness trait conferring cracking resistance in fresh fruits of watermelon. Plant Biotechnol. J. 18, 1066–1077. doi: 10.1111/pbi.13276
Licausi, F., Kosmacz, M., Weits, D. A., Giuntoli, B., Giorgi, F. M., Voesenek, L. A., et al. (2011). Oxygen sensing in plants is mediated by an N-end rule pathway for protein destabilization. Nature 479, 419–422. doi: 10.1038/nature10536
Lin, Z., Arciga-Reyes, L., Zhong, S., Alexander, L., Hackett, R., Wilson, I., et al. (2008). SlTPR1, a tomato tetratricopeptide repeat protein, interacts with the ethylene receptors NR and LeETR1, modulating ethylene and auxin responses and development. J. Exp. Bot. 59, 4271–4287. doi: 10.1093/jxb/ern276
Liu, L., White, M. J., and MacRae, T. H. (1999). Transcription factors and their genes in higher plants: functional domains, evolution and regulation. Eur. J. Biochem. 262, 247–257. doi: 10.1046/j.1432-1327.1999.00349.x
Liu, M., Chen, Y., Chen, Y., Shin, J. H., Mila, I., Audran, C., et al. (2018). The tomato Ethylene Response Factor Sl-ERF.B3 integrates ethylene and auxin signaling via direct regulation of Sl-Aux/IAA27. New Phytol. 219, 631–640. doi: 10.1111/nph.15165
Liu, M., Diretto, G., Pirrello, J., Roustan, J. P., Li, Z., Giuliano, G., et al. (2014). The chimeric repressor version of an Ethylene Response Factor (ERF) family member, Sl-ERF.B3, shows contrasting effects on tomato fruit ripening. New Phytol. 203, 206–218. doi: 10.1111/nph.12771
Liu, M., Gomes, B. L., Mila, I., Purgatto, E., Peres, L. E., Frasse, P., et al. (2016). Comprehensive profiling of ethylene response factor expression identifies ripening-associated ERF genes and their link to key regulators of fruit ripening in tomato. Plant Physiol. 170, 1732–1744. doi: 10.1104/pp.15.01859
Liu, M., Pirrello, J., Chervin, C., Roustan, J. P., and Bouzayen, M. (2015). Ethylene control of fruit ripening: revisiting the complex network of transcriptional regulation. Plant Physiol. 169, 2380–2390. doi: 10.1104/pp.15.01361
Liu, M., Pirrello, J., Kesari, R., Mila, I., Roustan, J. P., Li, Z., et al. (2013). A dominant repressor version of the tomato Sl-ERF.B3 gene confers ethylene hypersensitivity via feedback regulation of ethylene signaling and response components. Plant J. 76, 406–419. doi: 10.1111/tpj.12305
Lu, J., Ju, H., Zhou, G., Zhu, C., Erb, M., Wang, X., et al. (2011). An EAR-motif-containing ERF transcription factor affects herbivore-induced signaling, defense and resistance in rice. Plant J. 68, 583–596. doi: 10.1111/j.1365-313X.2011.04709.x
Lu, S., Zhang, M., Zhuge, Y., Fu, W., Ouyang, Q., Wang, W., et al. (2022). VvERF17 mediates chlorophyll degradation by transcriptional activation of chlorophyll catabolic genes in grape berry skin. Environ. Exp. Bot. 193, 104678. doi: 10.1016/j.envexpbot.2021.104678
Ma, H., Yang, T., Li, Y., Zhang, J., Wu, T., Song, T., et al. (2021). The long noncoding RNA MdLNC499 bridges MdWRKY1 and MdERF109 function to regulate early-stage light-induced anthocyanin accumulation in apple fruit. Plant Cell 33, 3309–3330. doi: 10.1093/plcell/koab188
Magnani, E., Sjölander, K., and Hake, S. (2004). From endonucleases to transcription factors: evolution of the AP2 DNA binding domain in plants. Plant Cell 16, 2265–2277. doi: 10.1105/tpc.104.023135
Manavella, P. A., Koenig, D., and Weigel, D. (2012). Plant secondary siRNA production determined by microRNA-duplex structure. Proc. Natl. Acad. Sci. USA 109, 2461–2466. doi: 10.1073/pnas.1200169109
Miedes, E., and Lorences, E. P. (2009). Xyloglucan endotransglucosylase/hydrolases (XTHs) during tomato fruit growth and ripening. J. Plant Physiol. 166, 489–498. doi: 10.1016/j.jplph.2008.07.003
Nakano, T., Suzuki, K., Fujimura, T., and Shinshi, H. (2006). Genome-wide analysis of the ERF gene family in Arabidopsis and rice. Plant Physiol. 140, 411–432. doi: 10.1104/pp.105.073783
Ni, J., Bai, S., Zhao, Y., Qian, M., Tao, R., Yin, L., et al. (2019). Ethylene response factors Pp4ERF24 and Pp12ERF96 regulate blue light-induced anthocyanin biosynthesis in ‘Red Zaosu’ pear fruits by interacting with MYB114. Plant Mol. Biol. 99, 67–78. doi: 10.1007/s11103-018-0802-1
Ni, J., Premathilake, A. T., Gao, Y., Yu, W., Tao, R., Teng, Y., et al. (2021). Ethylene-activated PpERF105 induces the expression of the repressor-type R2R3-MYB gene PpMYB140 to inhibit anthocyanin biosynthesis in red pear fruit. Plant J. 105, 167–181. doi: 10.1111/tpj.15049
Ohta, M., Matsui, K., Hiratsu, K., Shinshi, H., and Ohme-Takagi, M. (2001). Repression domains of class II ERF transcriptional repressors share an essential motif for active repression. Plant Cell 13, 1959–1968. doi: 10.1105/tpc.010127
O’Malley, R. C., Huang, S. S. C., Song, L., Lewsey, M. G., Bartlett, A., Nery, J. R., et al. (2016). Cistrome and epicistrome features shape the regulatory DNA landscape. Cell 165, 1280–1292. doi: 10.1016/j.cell.2016.04.038
Pirrello, J., Prasad, B. C., Zhang, W., Chen, K., Mila, I., Zouine, M., et al. (2012). Functional analysis and binding affinity of tomato ethylene response factors provide insight on the molecular bases of plant differential responses to ethylene. BMC Plant Biol. 12:190. doi: 10.1186/1471-2229-12-190
Rose, J. K., Braam, J., Fry, S. C., and Nishitani, K. (2002). The XTH family of enzymes involved in xyloglucan endotransglucosylation and endohydrolysis: current perspectives and a new unifying nomenclature. Plant Cell Physiol. 43, 1421–1435. doi: 10.1093/pcp/pcf171
Sakuma, Y., Liu, Q., Dubouzet, J. G., Abe, H., Shinozaki, K., and Yamaguchi-Shinozaki, K. (2002). DNA-binding specificity of the ERF/AP2 domain of Arabidopsis DREBs, transcription factors involved in dehydration-and cold-inducible gene expression. Biochem. Biophys. Res. Comm. 290, 998–1009. doi: 10.1006/bbrc.2001.6299
Sasaki, K., Mitsuhara, I., Seo, S., Ito, H., Matsui, H., and Ohashi, Y. (2007). Two novel AP2/ERF domain proteins interact with cis-element VWRE for wound-induced expression of the Tobacco tpoxN1 gene. Plant J. 50, 1079–1092. doi: 10.1111/j.1365-313X.2007.03111.x
Shen, S. L., Yin, X. R., Zhang, B., Xie, X. L., Jiang, Q., Grierson, D., et al. (2016). CitAP2.10 activation of the terpene synthase CsTPS1 is associated with the synthesis of (+)-valencene in ‘Newhall’ orange. J. Exp. Bot. 67, 4105–4115. doi: 10.1093/jxb/erw189
Shen, Y., Wei, W., and Zhou, D. X. (2015). Histone acetylation enzymes coordinate metabolism and gene expression. Trends Plant Sci. 20, 614–621. doi: 10.1016/j.tplants.2015.07.005
Shi, Y., Li, B. J., Su, G., Zhang, M., Grierson, D., and Chen, K. S. (2022). Transcriptional regulation of fleshy fruit texture. J. Integr. Plant Biol [Epub ahead of print]. doi: 10.1111/jipb.13316
Shoji, T., Mishima, M., and Hashimoto, T. (2013). Divergent DNA-binding specificities of a group of ETHYLENE RESPONSE FACTOR transcription factors involved in plant defense. Plant Physiol. 162, 977–990. doi: 10.1104/pp.113.217455
Shoji, T., and Yuan, L. (2021). ERF gene clusters: working together to regulate metabolism. Trends Plant Sci. 26, 23–32. doi: 10.1016/j.tplants.2020.07.015
Song, C. P., Agarwal, M., Ohta, M., Guo, Y., Halfter, U., Wang, P., et al. (2005). Role of an Arabidopsis AP2/EREBP-type transcriptional repressor in abscisic acid and drought stress responses. Plant Cell 17, 2384–2396. doi: 10.1105/tpc.105.033043
Sun, Y., Liang, B., Wang, J., Kai, W., Chen, P., Jiang, L., et al. (2018). SlPti4 affects regulation of fruit ripening, seed germination and stress responses by modulating ABA signaling in tomato. Plant Cell Physiol. 59, 1956–1965. doi: 10.1093/pcp/pcy111
Tiwari, S. B., Belachew, A., Ma, S. F., Young, M., Ade, J., Shen, Y., et al. (2012). The EDLL motif: a potent plant transcriptional activation domain from AP2/ERF transcription factors. Plant J. 70, 855–865. doi: 10.1111/j.1365-313X.2012.04935.x
Tucker, G., Yin, X., Zhang, A., Wang, M., Zhu, Q., Liu, X., et al. (2017). Ethylene and fruit softening. Food Qual. Safety 1, 253–267. doi: 10.1093/fqsafe/fyx024
Wang, M. M., Zhu, Q. G., Deng, C. L., Luo, Z. R., Sun, N. J., Grierson, D., et al. (2017). Hypoxia-responsive ERFs involved in postdeastringency softening of persimmon fruit. Plant Biotechnol. J. 15, 1409–1419. doi: 10.1111/pbi.12725
Wang, Q., Liu, H., Zhang, M., Liu, S., Hao, Y., and Zhang, Y. (2020). MdMYC2 and MdERF3 positively co-regulate α-farnesene biosynthesis in apple. Front. Plant Sci 11:512844. doi: 10.3389/fpls.2020.512844
Wang, S., Wang, T., Li, Q., Xu, C., Tian, J., Wang, Y., et al. (2022). Phosphorylation of MdERF17 by MdMPK4 promotes apple fruit peel degreening during light/dark transitions. Plant Cell 34, 1980–2000. doi: 10.1093/plcell/koac049
Wang, X., Pan, L., Wang, Y., Meng, J., Deng, L., Niu, L., et al. (2021). PpIAA1 and PpERF4 form a positive feedback loop to regulate peach fruit ripening by integrating auxin and ethylene signals. Plant Sci. 313, 111084. doi: 10.1016/j.plantsci.2021.111084
Wang, X., Zeng, W., Ding, Y., Wang, Y., Niu, L., Yao, J. L., et al. (2019a). Peach ethylene response factor PpeERF2 represses the expression of ABA biosynthesis and cell wall degradation genes during fruit ripening. Plant Sci. 283, 116–126. doi: 10.1016/j.plantsci.2019.02.009
Wang, X., Zeng, W., Ding, Y., Wang, Y., Niu, L., Yao, J. L., et al. (2019b). PpERF3 positively regulates ABA biosynthesis by activating PpNCED2/3 transcription during fruit ripening in peach. Hortic. Res 6, 19. doi: 10.1038/s41438-018-0094-2
Wei, C., Li, M., Cao, X., Jin, Z., Zhang, C., Xu, M., et al. (2022). Linalool synthesis related PpTPS1 and PpTPS3 are activated by transcription factor PpERF61 whose expression is associated with DNA methylation during peach fruit ripening. Plant Sci. 317, 111200. doi: 10.1016/j.plantsci.2022.111200
Welsch, R., Maass, D., Voegel, T., Dellapenna, D., and Beyer, P. (2007). Transcription factor RAP2.2 and its interacting partner SINAT2: stable elements in the carotenogenesis of Arabidopsis leaves. Plant Physiol. 145, 1073–1085. doi: 10.1104/pp.107.104828
Whitmarsh, A. J., and Davis, R. J. (2000). Regulation of transcription factor function by phosphorylation. Cell. Mol. Life Sci. 57, 1172–1183. doi: 10.1007/pl00000757
Wierzbicki, A., Blevins, T., and Swiezewski, S. (2021). Long noncoding RNAs in plants. Annu. Rev. Plant Biol. 72, 245–271. doi: 10.1146/annurev-arplant-093020-035446
Wilkinson, J. Q., Lanahan, M. B., Yen, H. C., Giovannoni, J. J., and Klee, H. J. (1995). An ethylene-inducible component of signal transduction encoded by never-ripe. Science 270, 1807–1809. doi: 10.1126/science.270.5243.1807
Wu, W., Wang, M. M., Gong, H., Liu, X. F., Guo, D. L., Sun, N. J., et al. (2020). High CO2/hypoxia-induced softening of persimmon fruit is modulated by DkERF8/16 and DkNAC9 complexes. J. Exp. Bot. 71, 2690–2700. doi: 10.1093/jxb/eraa009
Xiao, Y. Y., Chen, J. Y., Kuang, J. F., Shan, W., Xie, H., Jiang, Y. M., et al. (2013). Banana ethylene response factors are involved in fruit ripening through their interactions with ethylene biosynthesis genes. J. Exp. Bot. 64, 2499–2510. doi: 10.1093/jxb/ert108
Xu, J., Xu, H., Liu, Y., Wang, X., Xu, Q., and Deng, X. (2015). Genome-wide identification of sweet orange (Citrus sinensis) histone modification gene families and their expression analysis during the fruit development and fruit-blue mold infection process. Front. Plant Sci. 6:607. doi: 10.3389/fpls.2015.00607
Yang, X., Song, J., Fillmore, S., Pang, X., and Zhang, Z. (2011). Effect of high temperature on color, chlorophyll fluorescence and volatile biosynthesis in green-ripe banana fruit. Postharvest Biol. Technol. 62, 246–257. doi: 10.1016/j.postharvbio.2011.06.011
Yant, L., Mathieu, J., Dinh, T. T., Ott, F., Lanz, C., Wollmann, H., et al. (2010). Orchestration of the floral transition and floral development in Arabidopsis by the bifunctional transcription factor APETALA2. Plant Cell 22, 2156–2170. doi: 10.1105/tpc.110.075606
Yao, G., Ming, M., Allan, A. C., Gu, C., Li, L., Wu, X., et al. (2017). Map-based cloning of the pear gene MYB114 identifies an interaction with other transcription factors to coordinately regulate fruit anthocyanin biosynthesis. Plant J. 92, 437–451. doi: 10.1111/tpj.13666
Yao, J. L., Xu, J., Cornille, A., Tomes, S., Karunairetnam, S., Luo, Z., et al. (2015). A micro RNA allele that emerged prior to apple domestication may underlie fruit size evolution. Plant J. 84, 417–427. doi: 10.1111/tpj.13021
Yue, P., Lu, Q., Liu, Z., Lv, T., Li, X., Bu, H., et al. (2020). Auxin-activated MdARF5 induces the expression of ethylene biosynthetic genes to initiate apple fruit ripening. New Phytol. 226, 1781–1795. doi: 10.1111/nph.16500
Yue, P., Wang, Y., Bu, H., Li, X., Yuan, H., and Wang, A. (2019). Ethylene promotes IAA reduction through PuERFs-activated PuGH3.1 during fruit ripening in pear (Pyrus ussuriensis). Postharvest Biol. Technol. 157, 110955. doi: 10.1016/j.postharvbio.2019.110955
Zhai, Y., Cui, Y., Song, M., Vainstein, A., Chen, S., and Ma, H. (2021). Papain-like cysteine protease gene family in fig (Ficus carica L.): genome-wide analysis and expression patterns. Front. Plant Sci. 12:994. doi: 10.3389/fpls.2021.681801
Zhang, C. H., Shangguan, L. F., Ma, R. J., Sun, X., Tao, R., Guo, L., et al. (2012). Genome-wide analysis of the AP2/ERF superfamily in peach (Prunus persica). Genet. Mol. Res. 11, 4789–4809. doi: 10.4238/2012.October.17.6
Zhang, H., Pan, X., Liu, S., Lin, W., Li, Y., and Zhang, X. (2021). Genome-wide analysis of AP2/ERF transcription factors in pineapple reveals functional divergence during flowering induction mediated by ethylene and floral organ development. Genomics 113, 474–489. doi: 10.1016/j.ygeno.2020.10.040
Zhang, J., Xu, H., Wang, N., Jiang, S., Fang, H., Zhang, Z., et al. (2018). The ethylene response factor MdERF1B regulates anthocyanin and proanthocyanidin biosynthesis in apple. Plant Mol. Biol. 98, 205–218. doi: 10.1007/s11103-018-0770-5
Zhang, Y., Yin, X., Xiao, Y., Zhang, Z., Li, S., Liu, X., et al. (2018). An ETHYLENE RESPONSE FACTOR-MYB transcription complex regulates furaneol biosynthesis by activating QUINONE OXIDOREDUCTASE expression in strawberry. Plant Physiol. 178, 189–201. doi: 10.1104/pp.18.00598
Zhang, S., Zhu, C., Lyu, Y., Chen, Y., Zhang, Z., Lai, Z., et al. (2020). Genome-wide identification, molecular evolution, and expression analysis provide new insights into the APETALA2/ethylene responsive factor (AP2/ERF) superfamily in Dimocarpus longan Lour. BMC Genom. 21:62. doi: 10.1186/s12864-020-6469-4
Zhang, Z., Shi, Y., Ma, Y., Yang, X., Yin, X., Zhang, Y., et al. (2020). The strawberry transcription factor FaRAV1 positively regulates anthocyanin accumulation by activation of FaMYB10 and anthocyanin pathway genes. Plant Biotechnol. J. 18, 2267–2279. doi: 10.1111/pbi.13382
Zhang, Z., and Li, X. (2018). Genome-wide identification of AP2/ERF superfamily genes and their expression during fruit ripening of Chinese jujube. Sci. Rep. 8, 1–16. doi: 10.1038/s41598-018-33744-w
Zhao, C., Liu, X., Gong, Q., Cao, J., Shen, W., Yin, X., et al. (2021). Three AP2/ERF family members modulate flavonoid synthesis by regulating type IV chalcone isomerase in citrus. Plant Biotechnol. J. 19, 671–688. doi: 10.1111/pbi.13494
Zhong, S., Fei, Z., Chen, Y. R., Zheng, Y., Huang, M., Vrebalov, J., et al. (2013). Single-base resolution methylomes of tomato fruit development reveal epigenome modifications associated with ripening. Nat. Biotechnol. 31, 154–159. doi: 10.1038/nbt.2462
Zhu, K., Sun, Q., Chen, H., Mei, X., Lu, S., Ye, J., et al. (2021). Ethylene activation of carotenoid biosynthesis by a novel transcription factor CsERF061. J. Exp. Bot. 72, 3137–3154. doi: 10.1093/jxb/erab047
Keywords: AP2/ERF, fruit ripening, transcription regulation, protein interaction, phytohormone, epigenetic regulation
Citation: Zhai Y, Fan Z, Cui Y, Gu X, Chen S and Ma H (2022) APETALA2/ethylene responsive factor in fruit ripening: Roles, interactions and expression regulation. Front. Plant Sci. 13:979348. doi: 10.3389/fpls.2022.979348
Received: 27 June 2022; Accepted: 29 July 2022;
Published: 18 August 2022.
Edited by:
Luciano Freschi, University of São Paulo, BrazilReviewed by:
Julien Pirrello, École Nationale Supérieure Agronomique de Toulouse, FranceZhang Zongying, Shandong Agricultural University, China
Lida Fuentes-Viveros, Pontifical Catholic University of Valparaíso, Chile
Copyright © 2022 Zhai, Fan, Cui, Gu, Chen and Ma. This is an open-access article distributed under the terms of the Creative Commons Attribution License (CC BY). The use, distribution or reproduction in other forums is permitted, provided the original author(s) and the copyright owner(s) are credited and that the original publication in this journal is cited, in accordance with accepted academic practice. No use, distribution or reproduction is permitted which does not comply with these terms.
*Correspondence: Huiqin Ma, hqma@cau.edu.cn