- 1Indian Agricultural Research Institute, Indian Council of Agricultural Research, New Delhi, India
- 2Central Potato Research Institute, Indian Council of Agricultural Research, Shimla, India
- 3Directorate of Groundnut Research, Indian Council of Agricultural Research, Ananthapur, India
- 4Farm Science Centre, Indian Institute of Horticultural Research, Indian Council of Agricultural Research, Gonikoppal, India
- 5National Research Centre for Banana, Indian Council of Agricultural Research, Tiruchirappalli, India
Photosynthesis, crop health and dry matter partitioning are among the most important factors influencing crop productivity and quality. Identifying variation in these parameters may help discover the plausible causes for crop productivity differences under various management practices and cropping systems. Thus, a 2-year (2019–2020) study was undertaken to investigate how far the integrated crop management (ICM) modules and cropping systems affect maize physiology, photosynthetic characteristics, crop vigour and productivity in a holistic manner. The treatments included nine main-plot ICM treatments [ICM1 to ICM4 – conventional tillage (CT)-based; ICM5 to ICM8 – conservation agriculture (CA)-based; ICM9 – organic agriculture (OA)-based] and two cropping systems, viz., maize–wheat and maize + blackgram–wheat in subplots. The CA-based ICM module, ICM7 resulted in significant (p < 0.05) improvements in the physiological parameters, viz., photosynthetic rate (42.56 μ mol CO2 m–2 sec–1), transpiration rate (9.88 m mol H2O m–2 sec–1) and net assimilation rate (NAR) (2.81 mg cm–2 day–1), crop vigour [NDVI (0.78), chlorophyll content (53.0)], dry matter partitioning toward grain and finally increased maize crop productivity (6.66 t ha–1) by 13.4–14.2 and 27.3–28.0% over CT- and OA-based modules. For maize equivalent grain yield (MEGY), the ICM modules followed the trend as ICM7 > ICM8 > ICM5 > ICM6 > ICM3 > ICM4 > ICM1 > ICM2 > ICM9. Multivariate and PCA analyses also revealed a positive correlation between physiological parameters, barring NAR and both grain and stover yields. Our study proposes an explanation for improved productivity of blackgram-intercropped maize under CA-based ICM management through significant improvements in physiological and photosynthetic characteristics and crop vigour. Overall, the CA-based ICM module ICM7 coupled with the maize + blackgram intercropping system could be suggested for wider adoption to enhance the maize production in semiarid regions of India and similar agroecologies across the globe.
Introduction
Photosynthesis, crop health and the accumulation and distribution of dry matter among different plant parts determine the level of crop yield (Rana et al., 2014). Hence, understanding the crop physiological and crop growth mechanism behind yield increase may help in the extrapolation of newly developed crop management technologies to other crops with judicious use of external inputs and farm resources. It has become more essential under the aegis of the UN’s Sustainable Development Goals (SDGs) to achieve zero hunger, good health and well-being, clean environment and resources and clean production with appropriate climate action (Harish et al., 2022). As per an estimate, the world population will increase from the current 7.9 to 9.7 billion by 2050, hence, requiring ∼70–100% increase in the production of major cereal crops (Guo Y. et al., 2021). Alike, the rice–wheat cropping system (RWCS), being a dominant cropping system in the South-Asian Indo-Gangetic plains region (IGPR), hinges food security of resource-poor and economically weak population (Choudhary et al., 2021, 2022). But there are several issues in RWCS in the South-Asian IGPR as indiscriminate use of chemical fertilisers, land degradation (salinisation/alkalisation), low resource-use efficiencies, water table decline and higher associated cost and energy in water extraction (Kumar et al., 2021, 2022; Harish et al., 2022), besides substantial weed management costs and the emergence of weed resistance (Dass et al., 2017; Choudhary et al., 2021), severe incidence of pests, diseases and minor pest resurgence, climatic vulnerabilities like heat/cold waves, terminal drought stress and other numerous production vulnerabilities that collectively threaten the sustainability of RWCS in IGPR (Paul et al., 2014; Bhatt et al., 2016; Pooniya et al., 2018, 2022). To overcome these vulnerabilities, one strategy could be to replace rice with maize, legumes and other underutilised crops that require less water and other resources (Choudhary et al., 2018, 2020; Harish et al., 2021, 2022; Singh et al., 2021, 2022a; Pooniya et al., 2022); and hence, diversify the system for better productivity, profitability and resource use.
With the advent of several high-yielding hybrids and bio-fortified cultivars, maize has become a highly competitive crop in replacing the RWCS in view of better farm productivity, profitability, sustainability and nutritional security (Yadav et al., 2015). Moreover, maize has a wide adaptability to diverse agroclimatic conditions making it a potential alternative (Sharma and Dass, 2012). However, there exists a yawning gap between developed countries and India for maize productivity (Yadav et al., 2015). Therefore, scientists in India are working hard to raise its productivity to the global level. Among important options, legume inclusion makes the cropping systems more profitable and resilient with improved soil health (Dass and Sudhishri, 2010; Weil and Brady, 2017; Choudhary et al., 2020). Likewise, legume as an intercrop could be a low-input strategy in agriculture for improving the food, nutritional, economical and environmental security of the small and marginal farm families (Maitra et al., 2021), besides reducing the risks of crop failure, improving the system sustainability, reducing the soil erosion and preventing nutrient leaching losses (Dass and Sudhishri, 2010; Nyawade et al., 2019). In order to improve the resource-use efficiency (RUE) and to facilitate the intercropping advantages like niche complementarity and enhanced system productivity, the selection of appropriate intercrop combinations with synergistic effects is highly essential (Choudhary et al., 2020). There are several reports on the use of blackgram as an intercrop in maize under conventional cultivation methods. In cereal forages, legume intercropping increases dry matter, crude protein and lowers neutral detergent fibre (Zhang et al., 2015). Maize intercropped with legumes, such as alfalfa, soybean, fababean, greengram, and blackgram, improves the RUE, N and P uptake, system productivity and net return compared to sole maize cropping by judicious usage of space and sunlight, resources, weed smothering, increased residue decomposition rate, rhizosphere microbial community and legume nodule activity (Sangakkara, 1994; Li et al., 2003; Zhang et al., 2011; Sun et al., 2014; Singh et al., 2021, 2022a,2022b; Tripathi et al., 2021). However, there is no study on blackgram intercropping in maize under different integrated crop management (ICM) modules. Hence, this prompted us to study how the blackgram intercropping in maize changes the growth, physiology and productivity of maize crop mediated by various ICM modules.
The Food and Agriculture Organization (FAO) has recently adopted the ICM as a holistic site-specific approach to crop husbandry that combines the sustainable tillage and land configuration practices and the integrated nutrient, weed, water and pest management practices to deliver the most efficient and safe farm output with long-term benefits while conserving/enhancing the natural resources (Varatharajan et al., 2019a; Choudhary et al., 2020; Biswakarma et al., 2021). Globally, ICM has gained importance because of numerous crop production and resource constraints associated with major cropping systems, viz., rice–wheat, maize–wheat, pigeonpea–wheat and soybean–wheat, etc., in India per se. The application of ICM techniques helps in raising RUE and system productivity (Choudhary et al., 2020). Earlier reports in major cropping systems of India like RWCS, maize–wheat cropping system (MWCS), soybean–wheat under ICM indicated that CA-based treatments recorded higher crop yields due to improved crop physiological and photosynthetic characteristics, modulation of microclimate and resilience to environmental stresses, enhanced soil fertility and favourable soil microbiome (Choudhary et al., 2018, 2020; Varatharajan et al., 2019a; Singh et al., 2020, 2021, 2022a; Biswakarma et al., 2021; Pooniya et al., 2022). Our extensive literature review revealed that the majority of earlier studies focused on individual components of crop management, i.e., crop establishment, tillage, nutrient, weed, water, energy management, etc.; meagre information is available on adopting the ICM technology by integrating all input- and production-related factors in the crops. The information pertaining to the combination/interaction effect of ICM and cropping system (sole vs. intercrop) in the MWCS under different tillage, crop establishment patterns, residue retention, nutrient management, etc., under intercropping is also inadequate. Therefore, the current study was designed for investigating this important research issue to bridge the knowledge gap and to decode the intrinsic interrelationship among leaf and photosynthetic parameters, dry matter partitioning (DMP) and maize productivity vis-à-vis resilience to environmental stresses. All this is expected to provide insights into understanding the mechanism of yield advantages under ICM in general and intercropping combinations of maize grown under MWCS of semiarid IGPR in South Asia. The overall objective of the study was to understand whether and how far do the ICM modules (tillage, fertiliser, irrigation and weed management, applied in combination) and cropping systems affect maize physiology, photosynthetic characteristics, crop vigour and productivity in a holistic manner.
Materials and methods
Experimental site, climate and soil
A field experiment was conducted in two consecutive cropping cycles (kharif season) during 2019 and 2020 in the research farm of ICAR-Indian Agricultural Research Institute, New Delhi [Latitude 28° 63’ N; Longitude 77° 15’ E; Altitude 228.6 m], under Indo-Gangetic plains region. The experimental site is located in a semiarid region with Typic Ustochrepts sandy loam alluvial soil with subtropical climate having hot, dry summers and cold winters. Mean weather parameters recorded in the two respective cropping seasons are presented in Table 1 and Figure 1. The experimental soil had a pH 8.23, soil organic carbon (SOC) 0.48% and available nitrogen (N), phosphorus (P) and potassium (K) contents of 194.1, 14.8 and 303.4 kg ha–1, respectively, for 0–15 cm soil layer as estimated using standard protocols (Rana et al., 2014).
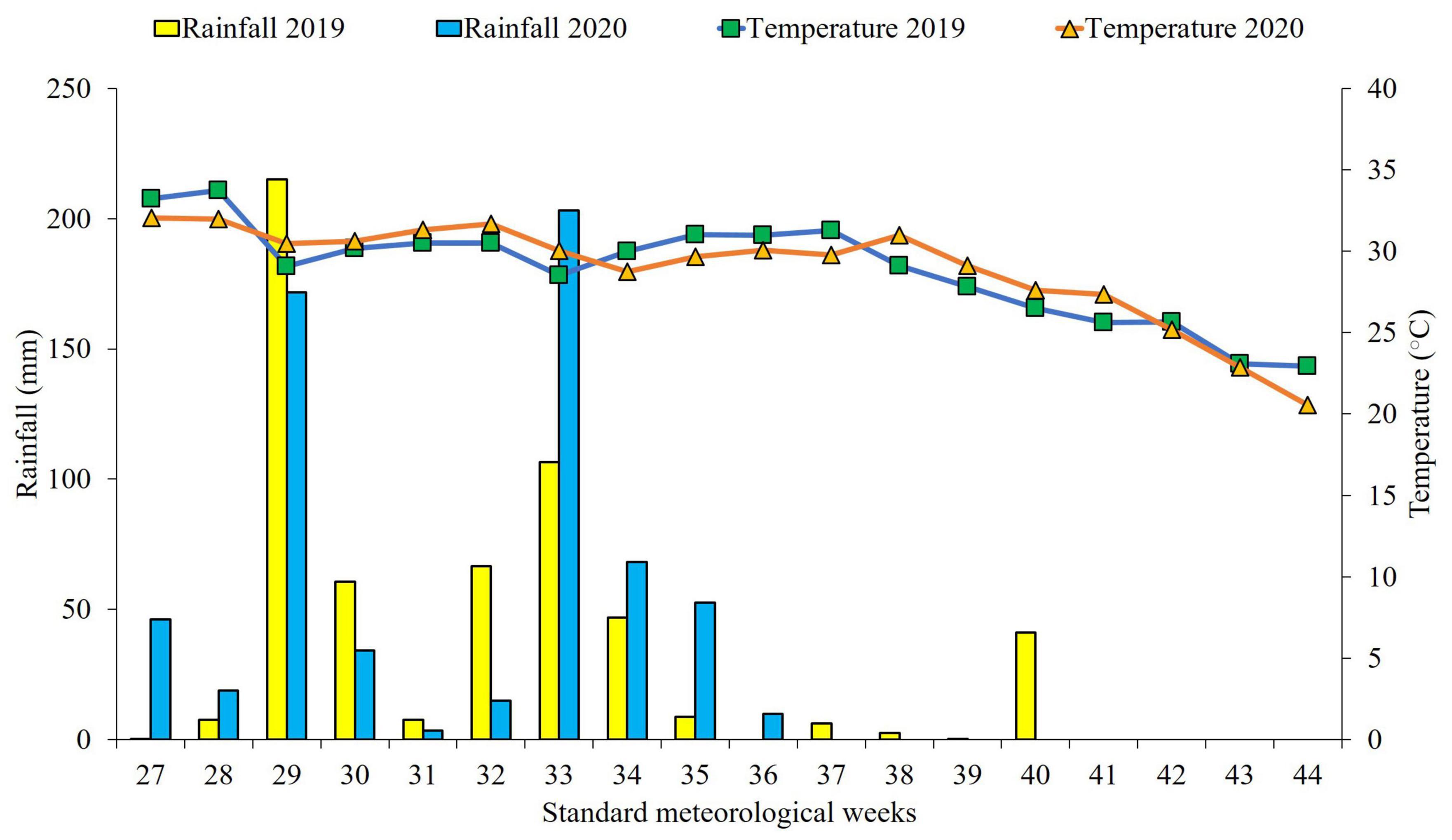
Figure 1. Weekly rainfall and mean temperature during crop growing seasons (2019–2020). [Source: Agro-meteorological Observatory, Division of Agricultural Physics, ICAR-IARI, New Delhi].
Experimental design
In the current study, nine main-plot treatments and two subplot treatments, all replicated thrice, were evaluated using a split-plot design. Main plots (42.0 m2 each) were assigned to nine ICM modules [ICM1 to ICM4 – conventional tillage (CT)-based; ICM5 to ICM8 – conservation agriculture (CA)-based; ICM9 – organic agriculture (OA)], while subplots (18.9 m2 each) were allotted to two cropping systems: (1) maize–wheat and (2) maize + blackgram–wheat. There was a total of 54 experimental units. Prior to the experiment, the field was under soybean–wheat cropping system for 4 years and ICM with the same layout. No synthetic fertilizer and crop protection chemical were used under OA (ICM9); only farmyard manure (FYM) was used as a nutrient source, with crop protection provided by botanicals, such as neem seed kernel extract. The detailed treatment description is presented in Table 2.
Crop management
The experimental field was prepared using a tractor-drawn double-disc MB plough followed by two cultivator-cum-planker operations under CT. Whereas in CA, zero-tillage (ZT) plots were prepared by glyphosate spray (1 kg a.i. ha–1) 15 days before sowing. Maize (Zea mays) cultivar ‘PMH-1’ was sown at 70 × 20 cm spacing using 20 kg seed ha–1. The additive series intercropping technique was adopted maintaining 100% population of maize (base crop) in blackgram-intercropped subplots. Blackgram (Vigna mungo) cultivar ‘Pant-U-30’ was sown at a seed rate of 10 kg ha–1 to establish two rows of blackgram (at 35 × 10 cm spacing) between every two rows of maize. Both maize and maize + blackgram were sown at the same time (15th and 13th July in 2019 and 2020, respectively), while the blackgram was harvested earlier to maize in both years (30th and 27th September in 2019 and 2020, respectively). The maize was harvested in 25th and 22th October in 2019 and 2020, respectively. The wheat crop under cropping system was cultivated in the same year, and the period between wheat harvest and maize sowing was 3 months. The experimental treatments were repeated exactly in the same plots for both years to know the cumulative/additive effect of legume intercropping in the cropping systems. Three crop establishment patterns were followed among different ICM modules: (i) flatbed (FB), (ii) raised bed (RB) and (iii) permanent raised bed (PRB). The RB/PRB were prepared with a width of 70 cm. Crop residues from the preceding wheat crop were retained at 3 t ha–1 under CA-based modules (ICM5–ICM8) and OA module (ICM9). Three nutrient management schedules were followed in different ICM modules, viz., (i) 100% recommended dose of fertiliser (150:80:60 kg N:P2O5:K2O ha–1) (100% RDF), (ii) 75% RDF (112.5:60:45 kg N:P2O5:K2O ha–1) + NPK biofertiliser + arbuscular mycorrhizal (AM) fungi (75% RDF + NPK-bf + AMF) both in CT- and CA-based modules (ICM1–ICM8) and while (iii) FYM at 15 t ha–1 + NPK-bf + AM fungi (FYM15 + NPK-bf + AMF) in OA module (ICM9). Irrespective of treatments, the entire amounts of fertiliser P and K were basally applied, while N was supplied in three equal splits (at basal, knee-high and tasselling stage in maize). Although the number of irrigations was common for all treatments (3 and 4 irrigations were scheduled in 2019 and 2020, respectively), the depth of water applied in each irrigation varied with crop establishment patterns, i.e., (i) 60 mm in FB and (ii) 45 mm in RB/PRB (Table 2), measured and applied accurately using portable water meter installed in field channels. Blackgram (intercrop) was harvested 30 days earlier than maize harvest in both study years. The crop from the net-plot area (chosen at centre of the plot, by excluding border rows) was harvested manually using sickles and then sun-dried at threshing floor. Tractor-operated maize dehusker-cum-sheller was used to separate grains from corncob, while blackgram from intercropped plots was harvested, threshed and cleaned manually. The grains were sun-dried to 14% seed moisture, cleaned to record grain and stover yields of both maize and blackgram (Rana et al., 2014). Border rows were excluded from all samplings/recording observations to avoid any over/underestimation of parameters involved.
Leaf and photosynthetic characteristics and net assimilation rate
Leaf photosynthetic characteristics were measured using an infrared gas analyser (IRGA) LI-6400 XT that is a portable photosynthetic system (Li-COR, Lincoln, NE, USA), during the flowering stage on a clear sunny day between 9.00 and 11.00 h. The airflow rate through the chamber was 500 μmol s–1, the CO2 concentration was the ambient one, and relative humidity was 70–75%. Three plants per plot were randomly selected, and the measurements, viz., (i) net photosynthetic rate (Pn) – amount of CO2 consumed by leaves per unit area per unit time (μ mol CO2 m–2 sec–1), (ii) transpiration rate (Tr) – the amount of water consumed by leaves per unit area per unit time (m mol H2O m–2 sec–1), (iii) stomatal conductance (Gs) – gas exchange of stomata per unit area per unit time (mol H2O m–2 sec–1), were taken from its uppermost fully expanded leaf. The transpiration efficiency (TE) – the amount of CO2 assimilation per unit mass of water transpired [μ mol CO2 (m mol H2O)–1] – was computed using the following formula:
The net assimilation rate (NAR) (Williams, 1946) that represents the increase in dry weight per unit leaf area per unit time was calculated at 30-day intervals, starting from 30 DAS and continuing till 90 DAS.
Normalised difference vegetation index
Normalised difference vegetation index (NDVI) is a dimensionless vegetation index and an indicator of greenness vis-à-vis vigour of vegetation, used to evaluate the density of greenness and crop health. NDVI values were measured using a handheld crop sensor Trimble GreenSeeker® (Trimble, Sunnyvale, CA, USA). The instrument’s sensor emits red and infrared light and then measures the amount of light reflected from each segment. The average NDVI value of the respective plot was recorded from the sensor at 50 cm height above the crop canopy at 30-day intervals.
Leaf chlorophyll content (soil plant analysis development)
Chlorophyll content (SPAD) or greenness of the plant was measured using Konica-Minolta SPAD-502 chlorophyll meter (Minolta, Osaka, Japan). Ten plants per plot were randomly selected and tagged to measure the SPAD value at 30-day intervals. From each plant, three readings were recorded per leaf, and the mean was calculated to get the SPAD value of the respective treatment.
Plant dry matter partitioning
Five representative plants per plot were randomly selected for measuring DMP during the flowering and harvest stage. To record DMP of respective plant parts, i.e., leaves, stem, husk, corncob and kernels per plant were separated and shade-dried followed by oven drying (65 ± 2°C), weighed on achieving the constant weight and recorded in g plant–1 for respective plant part (Rana et al., 2014).
Leaf area index and leaf growth parameters
Five plants per plot were randomly selected for measuring leaf area at 30-day intervals using LI-3100C leaf area meter (Li-COR, Lincoln, NE, USA) as cm2 plant–1, and the leaf area index (LAI) was calculated (Rana et al., 2014). Based on plant dry matter and leaf area observations recorded, the following leaf growth indices were calculated using the formulas given below: leaf area ratio (Radford, 1967), leaf weight ratio, specific leaf area (Kvet et al., 1971), specific leaf weight (Pearce et al., 1968).
Days taken to different phenological stages
Ten plants per plot were selected randomly and tagged to observe number of days taken (after sowing) for the attainment of different phenological growth stages, viz., days taken to 50% tasselling and days taken to 50% silking.
Crop productivity
Maize equivalent grain yield
Maize equivalent grain yield (MEGY) denotes the financial sum of maize grain yield and MEGY of blackgram. Thus, MEGY of blackgram was determined using the following expression:
The minimum support price (decided by the Government of India) prevailing during the cropping season was used in above calculations, i.e., for maize INR 17600 (USD 235) and INR 18500 (USD 247) t–1 during 2019 and 2020, respectively, and similarly for blackgram INR 57000 (USD 760) and INR 60000 (USD 800) t–1 during 2019 and 2020, respectively.
Maize equivalent stover yield
Maize equivalent stover yield (MESY) (combined stover yield) denotes the sum of maize stover yield and MESY of blackgram. Thus, MESY of blackgram was calculated as per the following formula:
The stover prices were determined based on the prevailing local market prices. Both maize and blackgram stover were priced at INR 3000 (USD 40) t–1 during both the years.
Statistical analysis
The differences between the treatments were statistically analysed by ANOVA technique using JMP® from SAS. The significant difference between the mean values of treatments was determined by using the least significant difference (p < 0.05) and indicated by different letters. Standard error of mean values (SEm±) was provided in all appropriate places. Multivariate analysis (MVA) and principal component analysis (PCA) were carried out using JMP® from SAS to decipher the association between physiological parameters, crop vigour, plant growth, MEGY and MESY.
Results
Photosynthetic characteristics
Irrespective of the ICM treatments, net photosynthetic rate (Pn) values ranged from 34.3 to 42.9 μ mol CO2 m–2 sec–1 during the flowering stage for maize (Table 3). There was a significant (p < 0.05) difference between CT and CA, as well as between CA- and OA-based ICM modules. However, no significant difference existed between 100% RDF- and 75% RDF + NPK-bf + AMF-applied modules in any study years. CA-based ICM7 module [ZT + PRB + wheat crop residue retention (3 t ha–1) + 100% RDF + glyphosate-PP fb pendimethalin-PE fb tembotrione-POE + 3 irrigations (45 mm depth each) + need-based integrated crop protection] showed 19.3 and 14.3% enhancement in Pn over OA- and CT-based modules. Inclusion of blackgram as an intercrop influenced Pn in maize significantly (p < 0.05); intercropped maize exhibited 4.5% higher Pn than sole maize. The transpiration rate (Tr) values ranged between 9.02 and 9.92 m mol H2O m–2 sec–1. There was a significant (p < 0.05) difference between CA and OA, while Tr in CT and CA stood alike. The use of 100% RDF and 75% RDF + NPK-bf + AMF nutrient management did not differ significantly (p < 0.05) from each other. CA-based ICM7 module recorded 7.1 and 6.2% higher Tr than OA- and CT-based ICM modules, and the differences were significant statistically. Blackgram intercropping improved the Tr in maize by ∼2% over sole maize (Table 3).
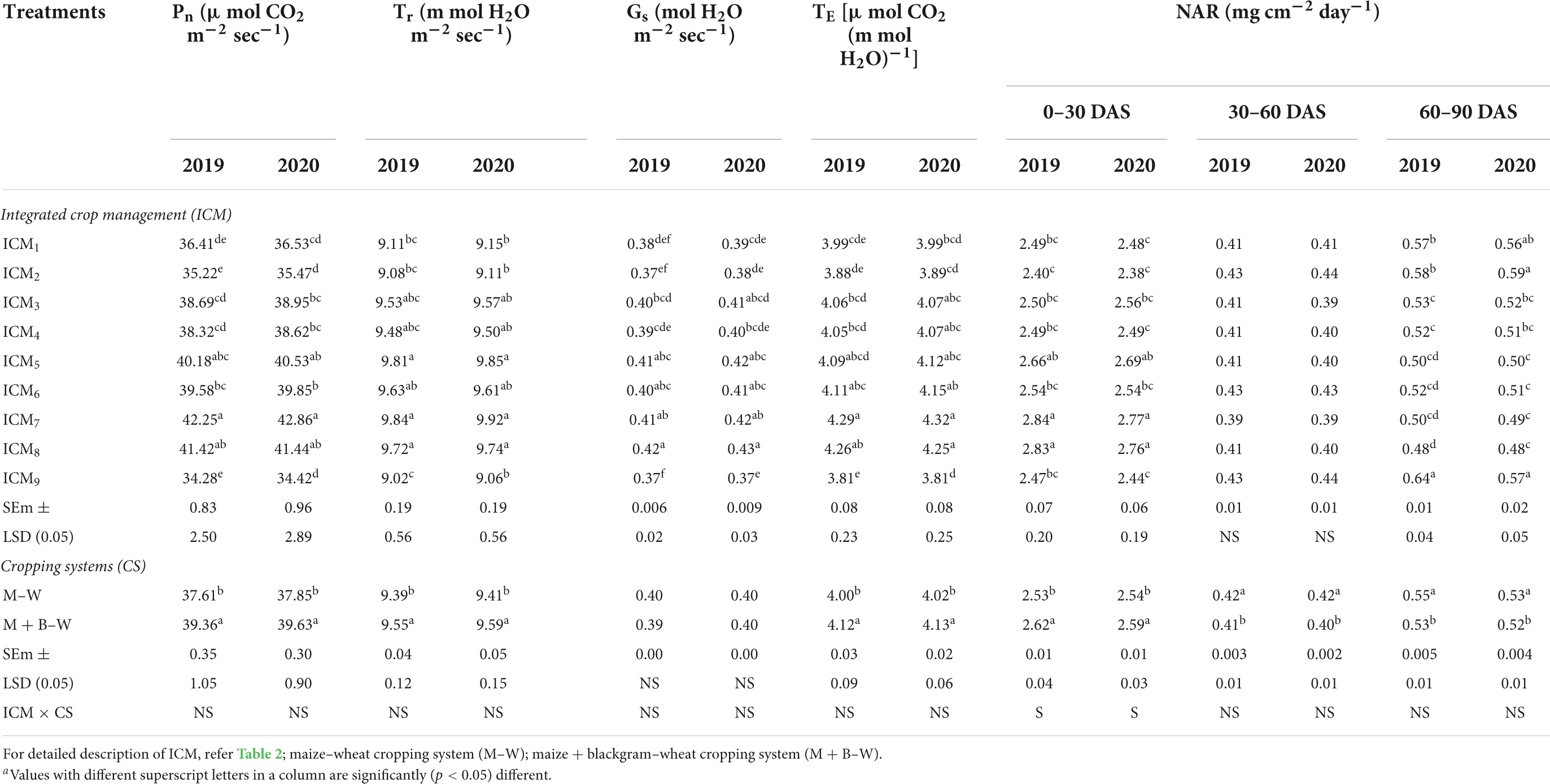
Table 3. Effect of ICM modules and cropping system on net photosynthetic rate (Pn), transpiration rate (Tr), stomatal conductance (Gs), transpiration efficiency (TE) at flowering stage and net assimilation rate (NAR) at 30-day intervals in maizea.
The stomatal conductance (Gs) values ranged from 0.37 to 0.43 mol H2O m–2 sec–1 during the flowering stage; CT, CA, and OA-based ICM modules exhibited significant (p < 0.05) differences in Gs during both years. The Gs was comparable between 100% RDF- and 75% RDF + NPK-bf + AMF-applied modules. However, the CA-based ICM8 module recorded ∼12.9 and 11.8% higher Gs than OA- and CT-based modules, respectively. Blackgram intercropping did not show any significant effect on Gs in maize. Transpiration efficiency (TE) values ranged between 3.81 and 4.32 μ mol CO2 (m mol H2O)–1. CA-based module ICM7 recorded significantly (p < 0.05) higher TE, which indicates 4.29–4.32 μ mol CO2 is fixed for every m mol H2O transpired. Blackgram intercropping (p < 0.05) caused significant improvement (∼3% increase) in TE of maize over sole maize. Further, ICM9, the OA-based ICM module, stood at the bottom level for all photosynthetic characteristics studied (Table 3). The ICM and CS interaction was non-significant for photosynthetic characteristics (Pn, Tr, Gs, and TE) of maize during both years.
Net assimilation rate
In general, the NAR ranged from 0.39 to 2.38 mg cm–1 day–1. Initially (0–30 DAS), NAR was high and later showed a falling trend (Table 3). Crop establishment techniques like FB, RB/PRB did not vary significantly in both CT- and CA-based ICM modules. Similarly, the nutrient management option 75% RDF + NPK-bf + AMF stood at par with 100% RDF-applied ICM modules in both CT- and CA-based ICM modules. But, among tillage options, the CA-PRB ICM module (ICM7 and ICM8) exhibited a significant advantage over CT-based ICM modules (ICM1 to ICM4) by assimilating more dry matter per unit leaf area per unit time. During the initial stages of growth (0–30 and 30–60 DAS), CA-based ICM modules exhibited higher NAR; however, during later growth stages (60–90 DAS), CT- and OA-based ICM modules showed significantly (p < 0.05) higher NAR. The OA-based ICM9 showed a low assimilation rate during early stages and significantly higher assimilation rate than CA-based ICM modules in later stages of growth. During 0–30 DAS, the CA-based ICM7 assimilated 2.84 and 2.77 mg of dry matter per cm2 of leaf area per day, which was 11.4 and 12.5% higher than CT-FB- and OA-based ICM modules. ICM modules did not show significant variation in NAR during 30–60 DAS. But during 60–90 DAS, OA-based ICM9 had significantly (p < 0.05) higher NAR, 0.64 and 0.57 mg dry matter per cm2 of leaf area per day during 2019 and 2020, respectively. Irrespective of the measurement interval, blackgram intercropping significantly altered the NAR in maize. Intercropped maize had significantly (p < 0.05) higher (1.9–3.4%) net assimilation though only for 0–30 DAS; at later stages, intercropped maize recorded lower. For the early growth stage (0–30 DAS) only, the ICM × cropping system (CS) interaction emerged significant (p < 0.05); both CA-based PRB modules (ICM7 and ICM8) had a comparable impact, although CT and OA depicted a much stronger interaction effect of blackgram intercropping than CA modules.
Normalised difference vegetation index
The Normalised Difference Vegetation Index (NDVI) ranged between 0.31 and 0.78, initially increased to the peak value at 60 DAS and then tapered gradually with the progression of plant growth (Table 4). NDVI varied significantly (p < 0.05) among CT, CA and OA-based modules. Various tillage, crop establishment techniques, nutrient management options and residue retention had a significant (p < 0.05) effect on maize NDVI. Irrespective of other variables, CA-based modules recorded higher NDVI than CT and OA modules. CA-PRB modules registered 24.1 and 35.2% greater NDVI values than CT-FB- and OA-based modules. At 30 DAS, all 100% RDF-applied modules recorded significantly higher NDVI over 75% RDF + NPK-bf + AMF-applied modules. Blackgram intercropping significantly (p < 0.05) improved the NDVI at 30 and 60 DAS, the increase being 14.7 and 3.3%, respectively, over sole maize. The OA ICM9 recorded significantly lower NDVI at all growth stages.
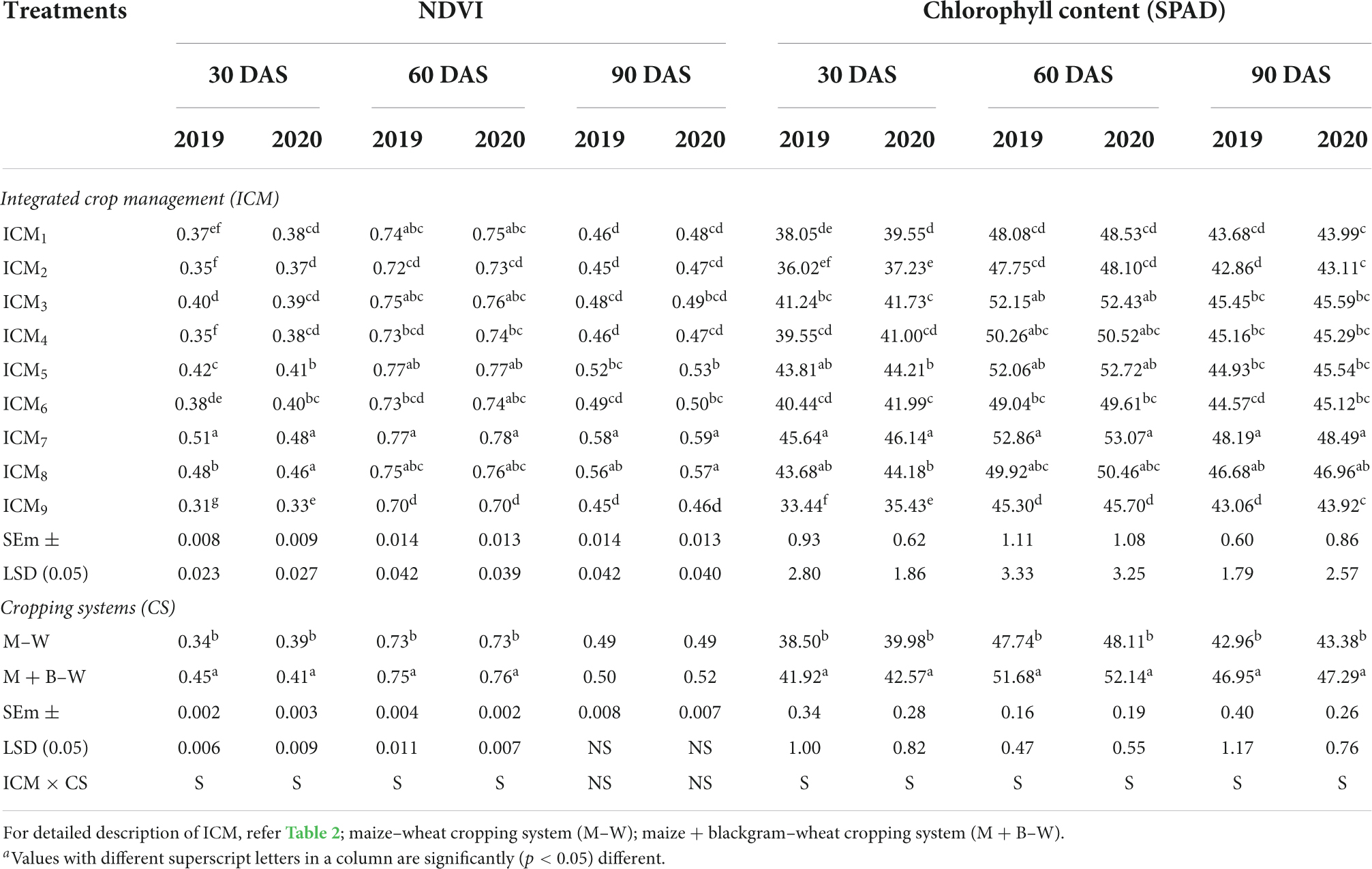
Table 4. Effect of different ICM modules and cropping system on Normalised Difference Vegetation Index (NDVI) and chlorophyll content (SPAD) in maize at 30-days intervala.
Chlorophyll content
The chlorophyll content (SPAD values) ranged from 33.4 to 53.1 across the ICM modules and at different intervals (Table 4). Chlorophyll content attained peak values at 60 DAS and later gradually decreased to its lowest at harvest. The SPAD values were similar between 100% RDF- and 75% RDF + NPK-bf + AMF-applied modules, except for OA-based ICM9 that recorded significantly (p < 0.05) lower SPAD than both 100 and 75% RDF-applied modules. CA-based ICM7 recorded SPAD values of 46.1, 53.1, and 48.5 at 30, 60, and 90 DAS, respectively, which were significantly (p < 0.05) higher than those recorded for CT- and OA-based modules. Over the entire crop duration, the association of blackgram as an intercrop improved the maize leaf chlorophyll content significantly (p < 0.05) over sole maize, where the increase was ∼7.1, 7.7, and 8.4% for 30, 60, and 90 DAS, respectively.
Integrated crop management and cropping system interaction effect for normalised difference vegetation index
The ICM × cropping system interaction effect was significant (p < 0.05) for maize NDVI (Table 5). At 30 DAS in the respective 2 years (2019 and 2020), the blackgram-intercropped maize under CT-, CA-, and OA-based modules had 2.7–29.3%, 2.4–25.4% and 13.9–20.6% higher NDVI than sole maize, respectively. Similarly, at 60 DAS blackgram-intercropped maize under CT-, CA- and OA-based modules had 1.4–5.4, 1.3–10.4, and 1.4–2.8% higher NDVI than sole maize, respectively. CA-based modules were superior to CT- and OA-based modules at all times (Table 5) under blackgram intercropping; the behaviour of CT- and OA-based ICM modules was alike under sole maize and blackgram intercropping.
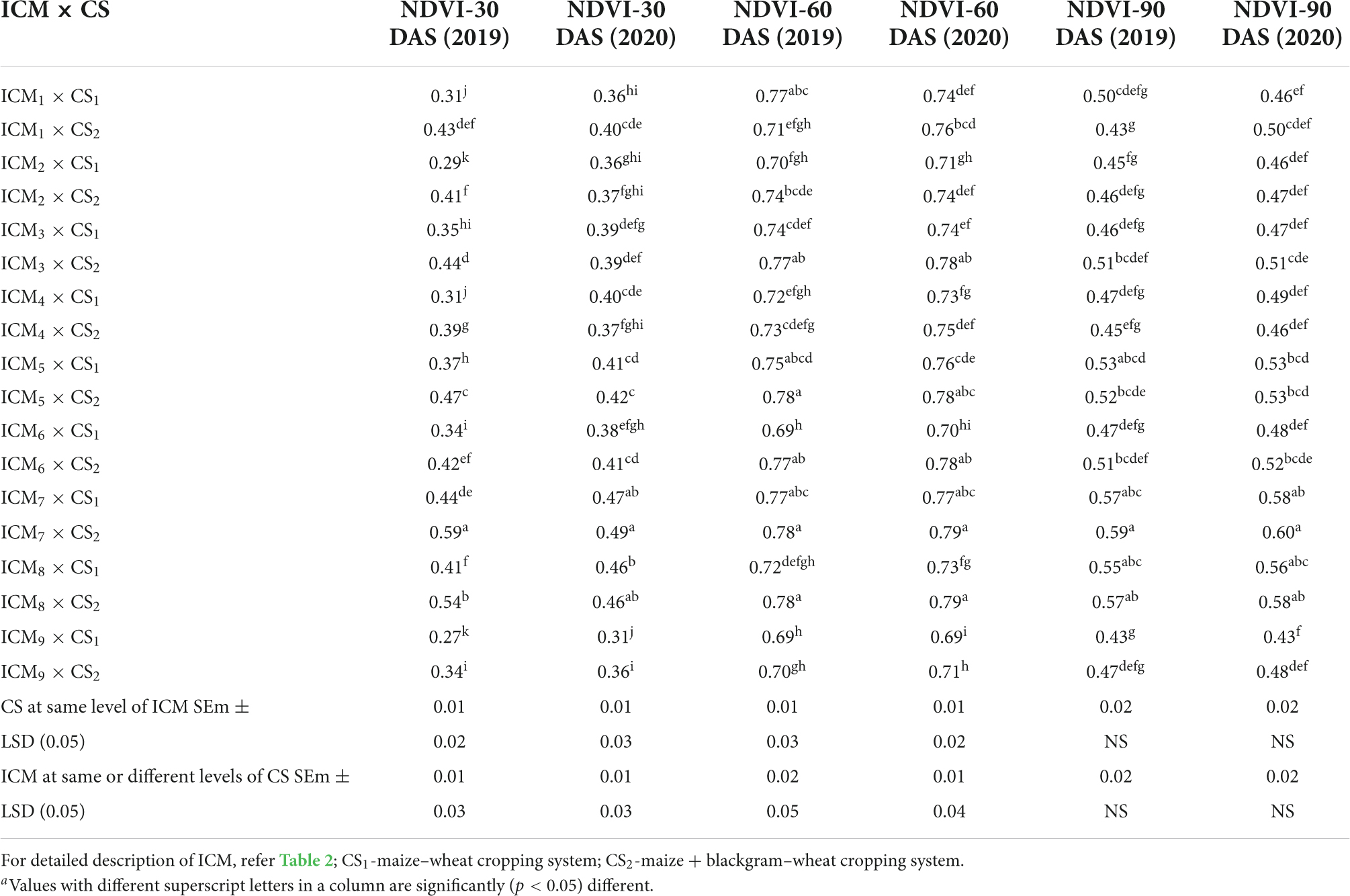
Table 5. Integrated crop management (ICM) modules × cropping system (CS) interaction effects on NDVI values of maize at 30-days interval under maizea.
Integrated crop management and cropping system interaction effect for soil plant analysis development
The ICM × cropping system interaction effect was significant (p < 0.05) for the chlorophyll content (SPAD) at all measurement times (Table 6). During 30 DAS, maize grown with blackgram intercropping under CT-, CA- and OA-based modules in the 2 years showed 2.1–13.9%, 1.4–11.0%, and 12.2–22.3% higher SPAD than sole maize, respectively. Similarly at 60 DAS, blackgram-intercropped maize under CT-, CA-, and OA-based ICM modules in the 2 years had 1.3–11.8, 6.6–11.0, and 12.2–13.5% higher SPAD than sole maize, respectively; the corresponding increase in SPAD values for 90 DAS was 1.1–12.2, 9.8–18.2, and 17.5–19.1% (Table 6). The highest SPAD value was found in blackgram-intercropped maize under ICM7 (55.6) at 60 DAS, and the lowest SPAD value was recorded in sole maize under ICM9 (29.2) at 30 DAS.
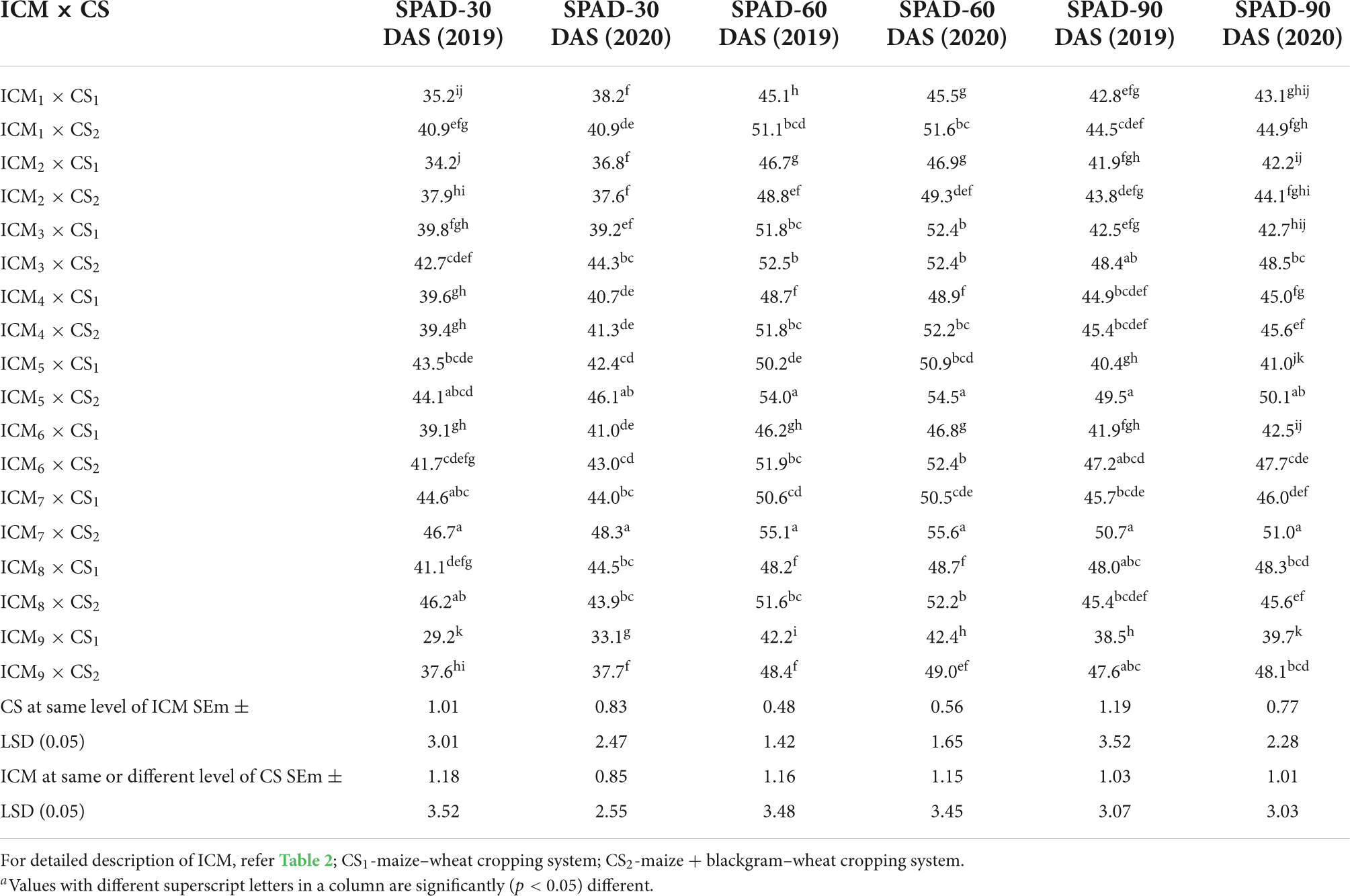
Table 6. Integrated crop management (ICM) modules × cropping system (CS) interaction effects on SPAD values of maize at 30-days interval DAS under maizea.
Days taken to different phenological stages
For attaining 50% tasselling, on an average 51.8–56, 54.7–57.2, and 58.2–58.5 days were taken by sole maize grown under CA-, CT-, and OA-based ICM modules, respectively (Figure 2). The maize crop took 58.5–60.8, 60.0–64.3, and 65.2–65.3 days to achieve 50% silking under CA-, CT-, and OA-based ICM modules, respectively. Here, it is interesting to summarise that blackgram intercropping prolonged the vegetative growth of maize and, thus, postponed the occurrence of 50% tasselling and silking by about 2.1–3.6 and 1.9–2.7 days, respectively, compared to sole maize cropping, which may help in curtailing the effect of early thermal heat stress in summer months in semiarid climates, besides enhancing the photosynthetic efficiency of the intercropped maize crop.
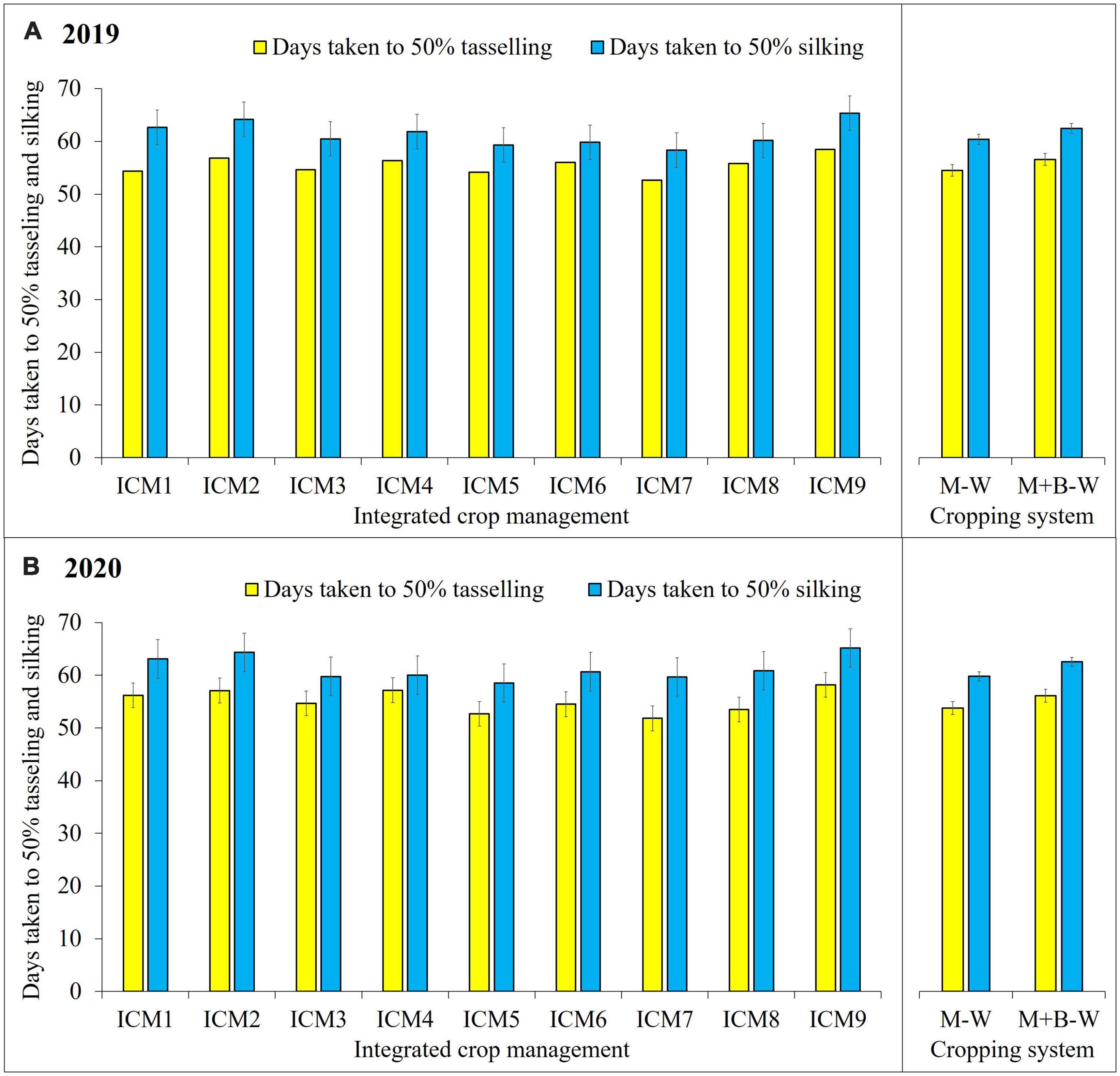
Figure 2. Effect of different ICM modules and cropping system on days taken to phenological stages of maize 2019 (A) and 2020 (B). The LSD0.05 is indicated by a bar above each column, and any treatment difference beyond the range of bar within each year is significantly different.
Dry matter partitioning
Leaf dry weight (LDW) and stem dry weight (SDW) ranged from 18.8 to 26.9 and 51.7 to 60.1 g plant–1. Both LDW and SDW were greater in CA-based ICM modules (Table 7). The OA-based ICM9 had the least LDW and SDW (Table 7). At harvest, the DMP among important plant parts as leaf, stem and kernels ranged between 16.4–21.4, 48.4–59.2, and 65.3–81 g plant–1, respectively (Figure 3). Kernel weight per plant was significantly (p < 0.05) higher under CA-based ICM modules, especially in ICM7 and ICM8. Dry matter accumulation (DMA) per plant forms the basis for DMP after flowering. The sum of DMP toward leaf, stem, husk, corncob and kernel was taken as DMA per plant. Thus, DMA was significantly (p < 0.05) higher under CA-based ICM modules (ICM5 to ICM8) (Figure 3); likewise, the DMP toward different plant parts was also higher under CA. And, the CA-based ICM7 had the highest DMP of 79.8 and 81 g kernel per plant during 2019 and 2020, respectively; while among the CT-based modules, ICM3 had significantly (p < 0.05) higher DMP to kernel 74.1 and 75.2 g per plant during 2019 and 2020, respectively. The least kernel DMP was observed in ICM9, i.e., 65.3 and 66.6 g per plant during 2019 and 2020, respectively. Similar trend was observed in DMP of leaf and stem, in the 2 years. Blackgram intercropping significantly (p < 0.05) improved the leaf (1.7–3.0%), stem (2.8–3.0%), and kernel (2.5%) weight per plant in 2019 and 2020, respectively (Figure 3).
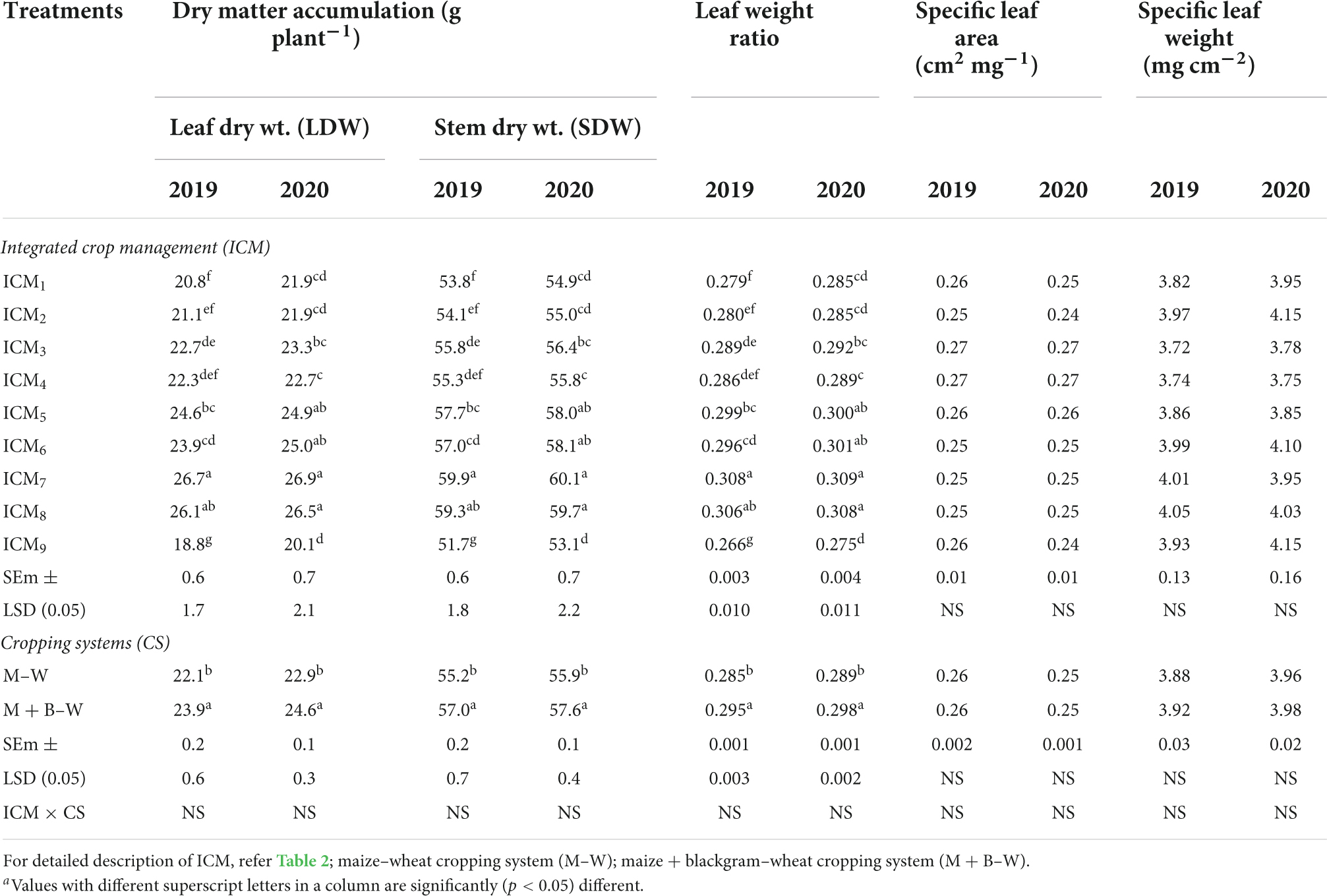
Table 7. Effect of ICM modules and cropping system on dry matter accumulation and leaf growth parameters of maize at flowering stagea.
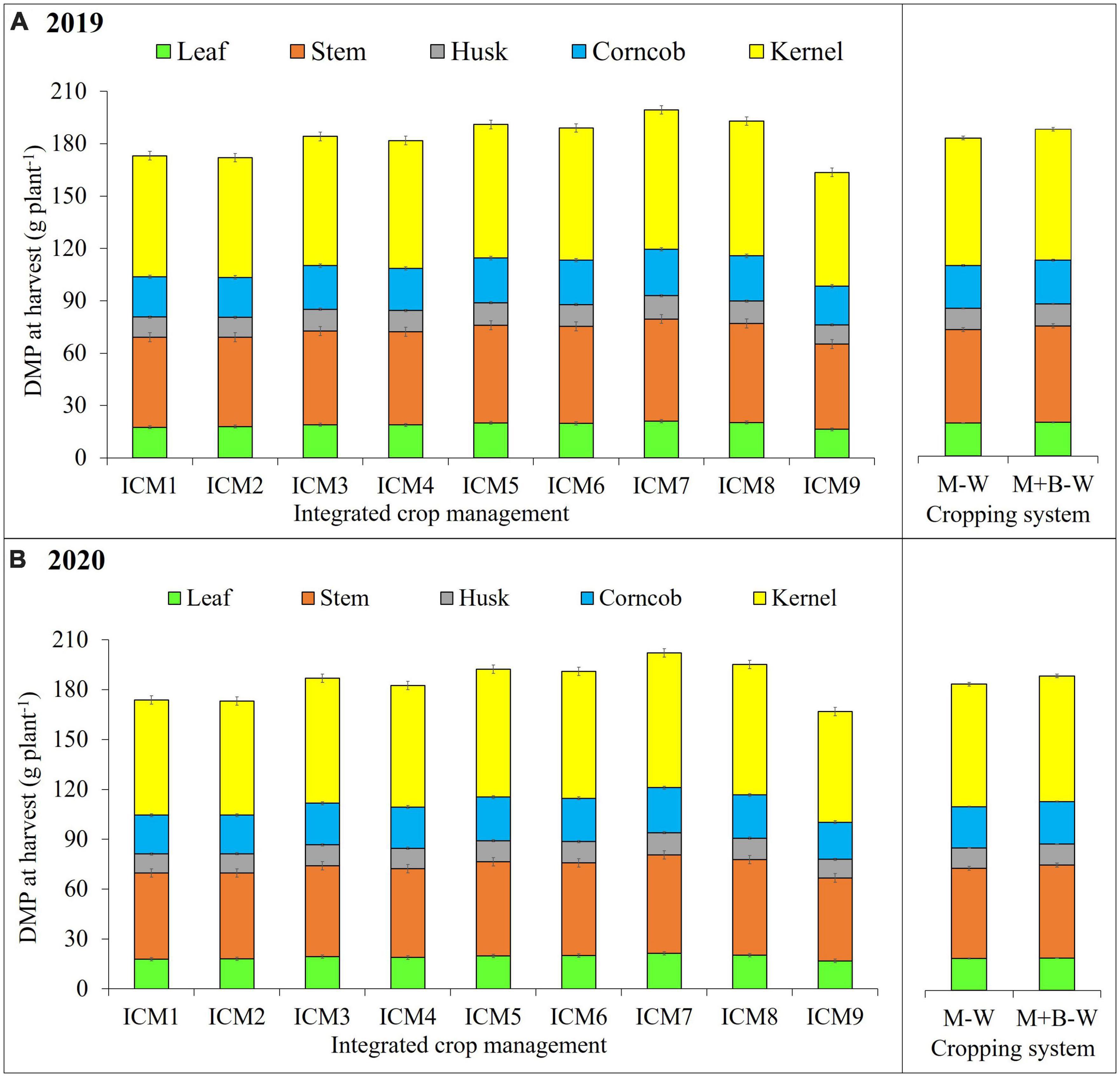
Figure 3. Effect of different ICM modules and cropping system on dry matter partitioning (DMP) of maize at harvest 2019 (A) and 2020 (B). The LSD0.05 is indicated by a bar above each column, and any treatment difference beyond the range of bar within each year is significantly different.
Leaf characteristics
Leaf weight ratio (LWR) indicates the ratio between LDW and whole plant dry weight. The higher LWR denotes a higher proportion of leaf in plant dry weight and hence greater photosynthetic area of plant. Crop establishment pattern and nutrient management option stood at par, excluding OA-based ICM9. CA-based ICM modules resulted in significantly (p < 0.05) higher LWR than CT and OA (Table 7). Intercropped maize recorded significantly higher (3.0–3.4%) LWR than sole maize. Both ICM and CS did not exert any significant (p < 0.05) effect on specific leaf area (SLA) and specific leaf weight (SLW) measured during the flowering stage. The interaction effect of ICM and CS on LWR, SLA and SLW was non-significant during both years (Table 7).
Leaf area index and leaf area ratio
Leaf area index (LAI) range was 1.84–2.23, 3.42–4.88, and 2.68–3.67 at 30, 60, and 90 DAS, respectively (Table 8). Similarly, leaf area ratio (LAR) range was 94.0–106.5, 66.5–78.6, and 24.6–28.9 cm2 g–1 at 30, 60, and 90 DAS, respectively. Both ICM and CS had a significant (p < 0.05) effect on LAI and LAR in both years. CA-based ICM7 caused significantly (p < 0.05) greater LAI (2.19, 4.83 and 3.63) at 30, 60, and 90 DAS, which was ∼9.1, 18.8, and 14.3% higher than CT-FB-based ICM modules and ∼14.4, 28.7, and 25.9% higher than OA ICM9 module, respectively. The CA-based ICM modules exhibited significantly (p < 0.05) lower LAR at 30 DAS, while the OA-based ICM9 had significantly (p < 0.05) lower LAR at 60 and 90 DAS (Table 8). Sole maize had 104.8 cm2 leaf area g–1 of LDW, which was higher at 30 DAS, while intercropped maize had 75.3 and 28.6 cm2 leaf area g–1 of LDW, which was significantly (p < 0.05) higher than sole maize during 60 and 90 DAS, respectively. For both LAI and LAR, ICM modules had a significant (p < 0.05) interaction with CS up to 60 DAS, but it was non-significant at 90 DAS. Blackgram-intercropped maize had 3.2, 4.6, and 7.2% greater LAI than sole maize at 30 DAS while on interaction with ICM, under CT, CA and OA, respectively. Similarly, at 60 DAS, blackgram-intercropped maize had 5.2, 6.6, and 12.5% higher LAI than sole maize under CT, CA, and OA, respectively. At 30 DAS, the LAR of OA- and CT-based ICM modules was significantly (p < 0.05) higher than CA-based ICM modules. But at 60 DAS, the LAR of CA- PRB-, CA-FB- and CT-RB-based ICM modules stood at par.
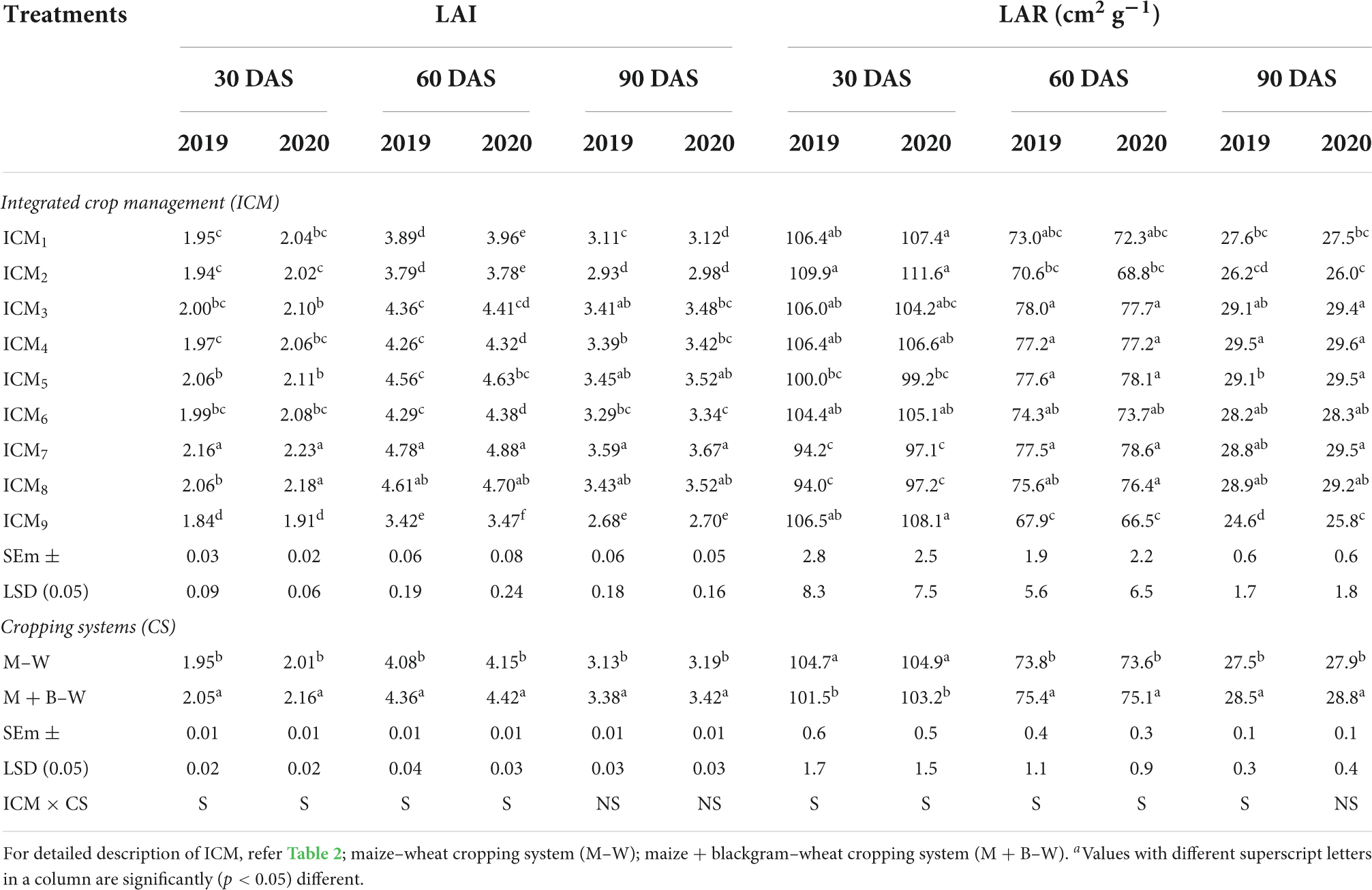
Table 8. Effect of ICM modules and cropping system on leaf area index (LAI) and leaf area ratio (LAR) of maize at 30-days intervala.
The ICM and cropping system interaction effect was significant (p < 0.05) for maize LAI and LAR only during 30 and 60 DAS. Irrespective of tillage, crop establishment methods and nutrient/water management options, at 30 and 60 DAS, the maize under blackgram-intercropped ICM modules recorded significantly higher LAI than sole maize during both years. While at 30 DAS, the blackgram-intercropped maize recorded significantly lower LAR than sole maize, except CA-PRB (ICM7 and ICM8), where the maize + blackgram intercropping had significantly higher LAR. At 60 DAS, maize crop under ICM modules with blackgram intercropping had higher LAR than sole maize. At 90 DAS, the ICM and cropping system interaction was non-significant for LAI and LAR, during both years.
Crop productivity
Combined maize equivalent grain yield
Combined MEGY was significantly (p < 0.05) influenced by both ICM modules and cropping systems (Figure 4). The MEGY was highest in ICM7 (6.6 and 6.71 t ha–1), while the remaining ICM modules followed the decreasing order as ICM8 > ICM5 > ICM6 > ICM3 > ICM4 > ICM1 > ICM2 > ICM9. Nutrient management options like 100% RDF and 75% RDF + NPK-bf + AM fungi stood on par with each other. Under various crop establishment techniques, MEGY was significantly (p < 0.05) higher in CA-PRB-induced ICM modules followed by CA- FB-, CT-RB- and CT-FB-based ICM modules. It is vividly evident from Figure 4 that CA-based ICM modules were superior to CT- and OA-based ICM modules during both years of study. The MEGY of CA-based ICM7 was 13.8 and 27.9% higher than CT-FB- and OA-based ICM modules. The MEGY of blackgram-intercropped maize was 6.49 and 6.66 t ha–1, which was higher by 16.9 and 17.9% over sole maize in 2019 and 2020, respectively. ICM and CS had a significant (p < 0.05) interaction effect on MEGY only during 2020. The CA-PRB ICM7 module in combination with blackgram intercropping recorded highest MEGY (7.32 t ha–1), which was 16.7% higher than sole maize under ICM7 module.
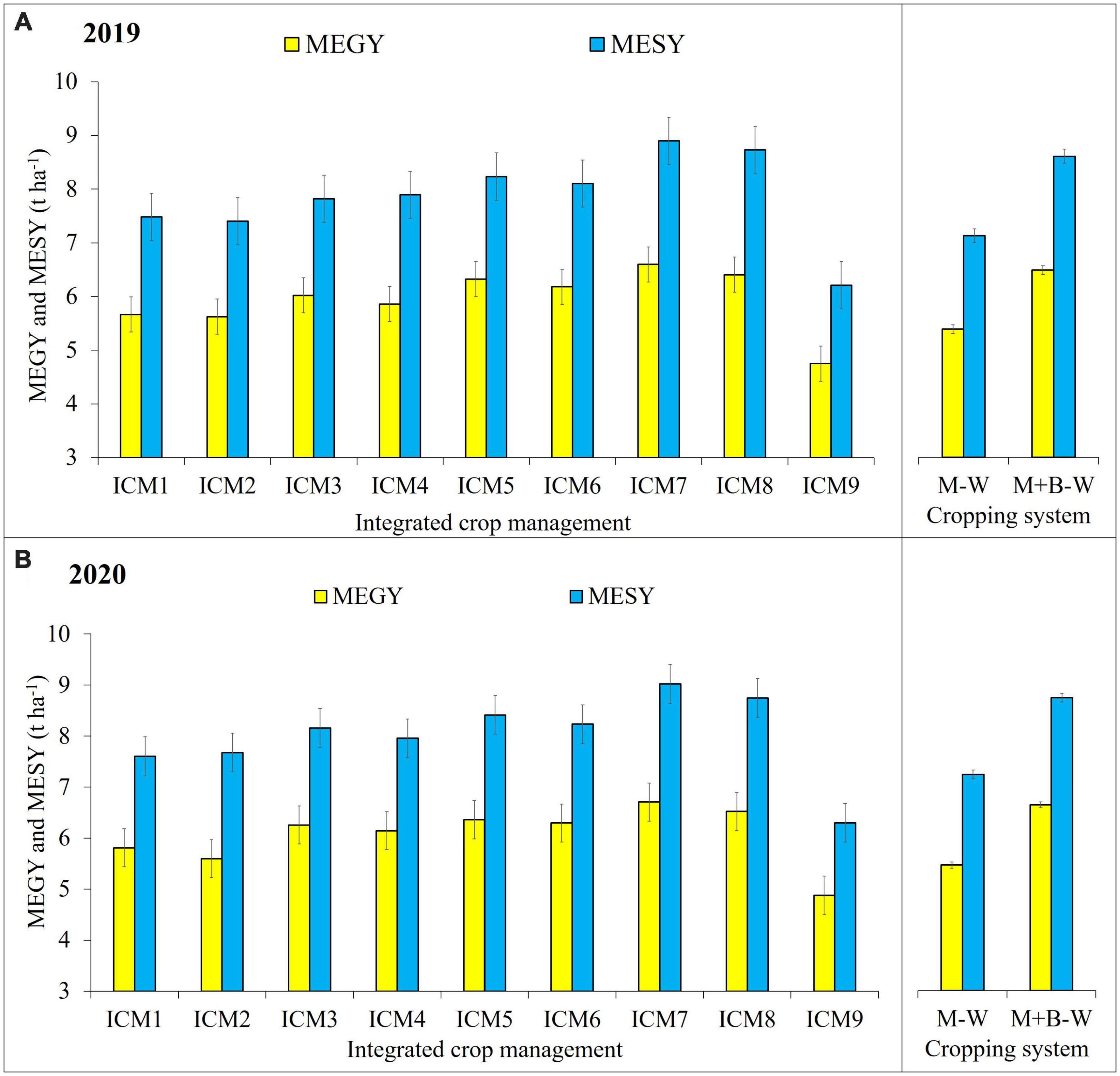
Figure 4. Effect of different ICM modules and cropping system on maize equivalent grain yield (MEGY) and maize equivalent stover yield (MESY) 2019 (A) and 2020 (B). The LSD0.05 is denoted by a bar above each column, and any treatment beyond the range of bar within each year is significantly different.
Combined stover yield (maize equivalent stover yield)
The ICM modules and CS had a significant effect on MESY, whose general trend was similar to that of MEGY (Figure 4). The MESY under different nutrient management options were on par, except for the OA-based ICM9 (where 15 t ha–1 FYM was applied instead of RDF), which recorded significantly (p < 0.05) lower stover yield in 2019 and 2020, respectively (6.21 and 6.3 t ha–1). The CA-based ICM7 recorded higher MESY (8.9 and 9.02 t ha–1) than CT. Also, the ICM modules with RB/PRB land configuration proved superior to FB-based ICM modules under CT, CA and OA. Blackgram-intercropped maize produced 8.6 and 8.77 t ha–1 of MESY against MESY of 7.13 and 7.26 t ha–1 obtained from sole maize during 2019 and 2020, respectively. During 2020, ICM and CS had a significant (p < 0.05) interaction effect on MESY. The blackgram-intercropped ICM7 module recorded highest MESY (9.98 t ha–1), which was 21.6% higher than sole maize under ICM7 module.
Multivariate and principal component analysis
The MVA and PCA were performed to understand the strength and degree of correlation among physiological parameters, crop growth, health and productivity (MEGY and MESY). In general, all observed parameters were found positively correlated with MESY and MEGY, except for the negative correlation with the NAR (Figure 5). Positive correlations included those of combined grain yield (MEGY), with chlorophyll (SPAD) at 60 DAS (r = 0.79), LAI (r = 0.79), Pn (r = 0.69), NDVI (r = 0.68), Tr (r = 0.54). At 60 DAS, a strong negative correlation was observed in NAR, with LAR (r = –0.86), LAI (r = –0.63). A strong positive correlation was found between MEGY and MESY with a correlation coefficient of 0.97. Similarly, Pn was positively correlated with several parameters, including SPAD (r = 0.67), Tr (r = 0.79), and LAI (r = 0.83).
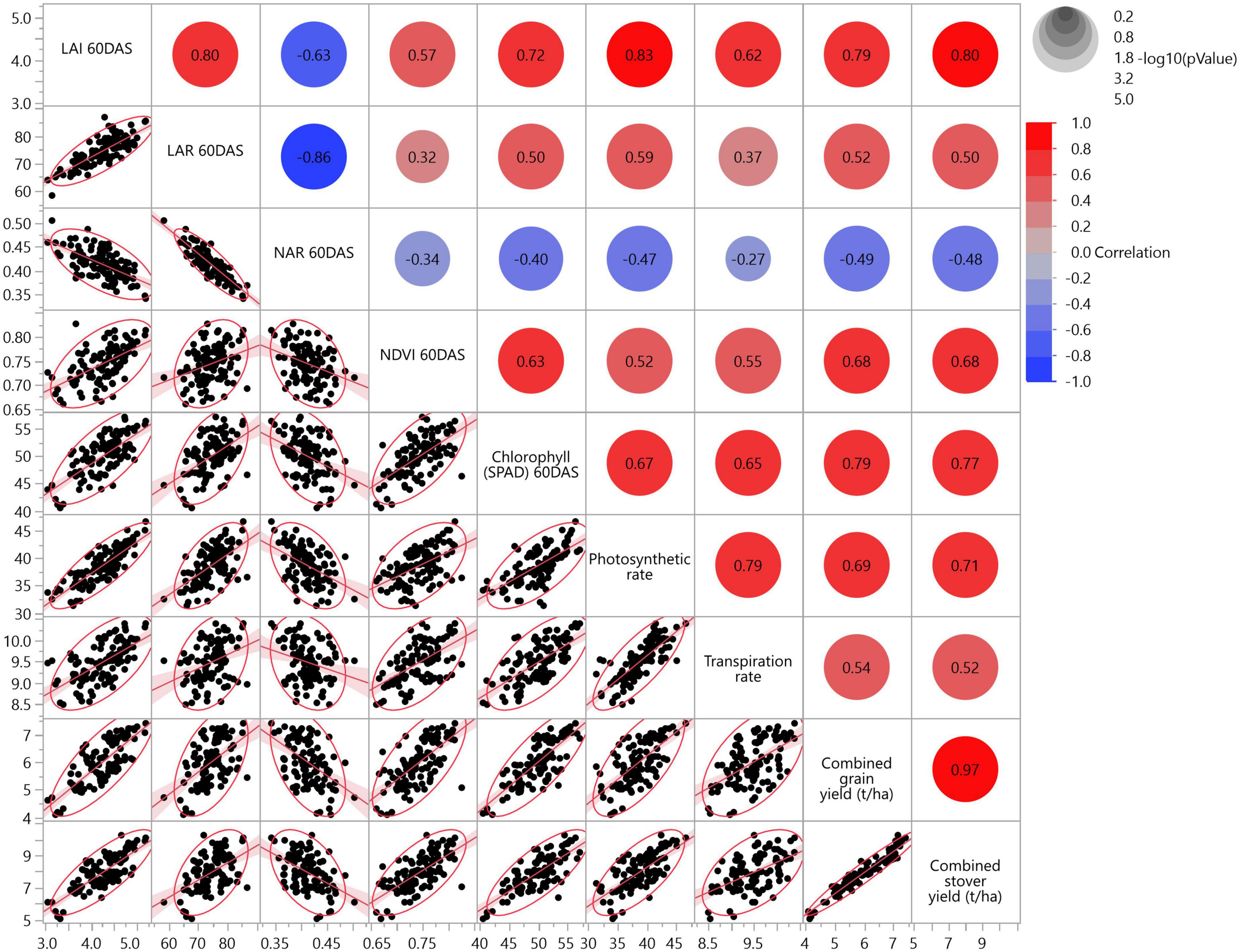
Figure 5. Multivariate analysis showing correlation among various crop physiological (LAI, LAR, NAR, NDVI and SPAD at 60 DAS) and photosynthetic characteristics (flowering stage, 60 DAS), MEGY and MESY in maize from two cropping seasons (2019 and 2020). The lower triangle shows scatter plot matrix with line fit, and the upper triangle shows the significant circles with correlation coefficient (p < 0.05) (2 years’ pooled data).
Moreover, PCA illustrates the significant effect of individual treatments (ICM and CS) and among various observed parameters (Figure 6). The PCA shows the superiority of blackgram-intercropped maize over sole maize. Similarly, all CA-based modules (ICM5–ICM8) and CT-RB modules (ICM3 and ICM4) performed more or less similar in improving the maize productivity by improving all physiological, crop vigour and growth parameters (Figure 6). All physiological parameters as Pn, Tr, SPAD and NDVI were closely related to MEGY and MESY, except LAR and NAR which were found to be far from being related.
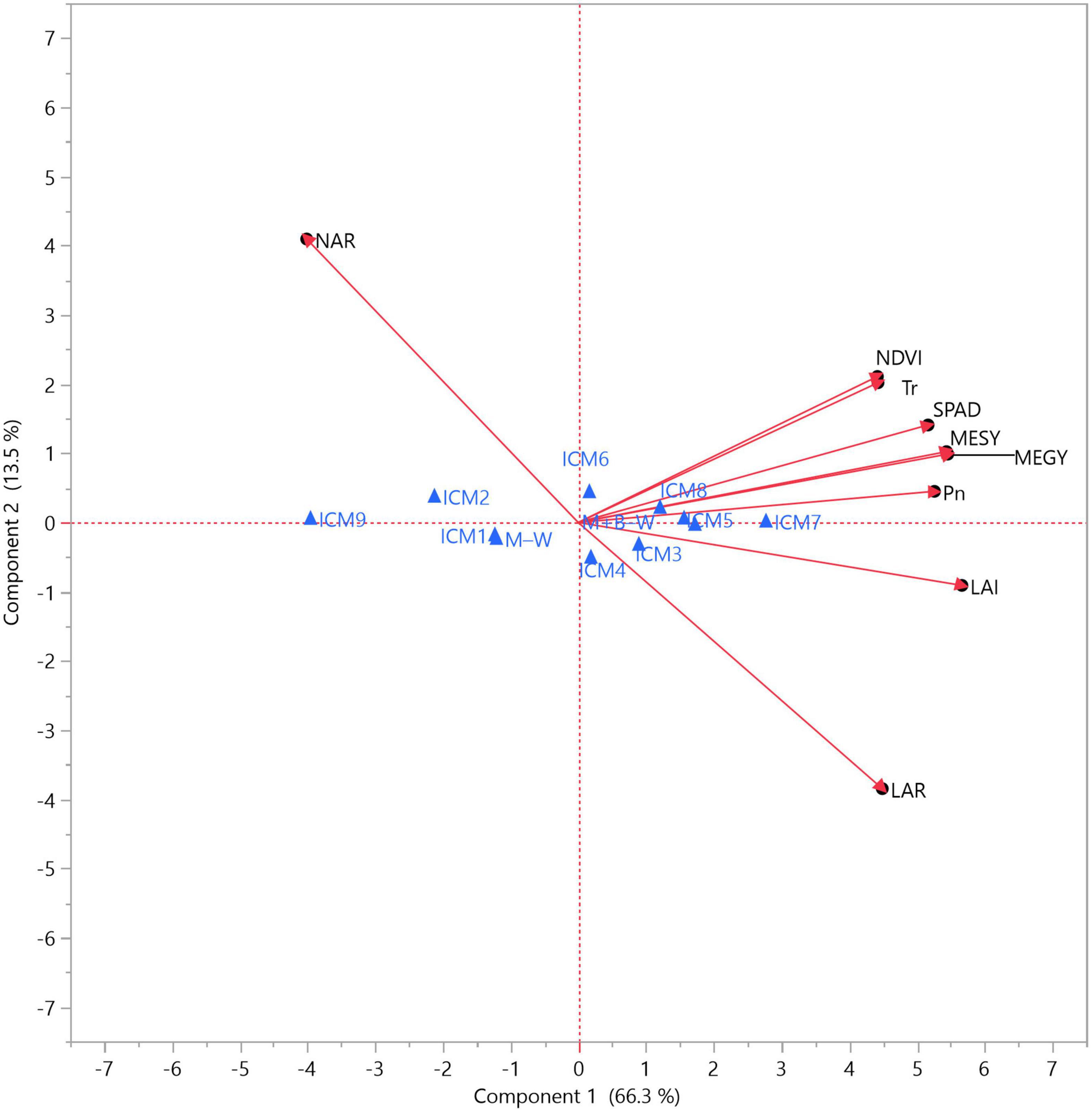
Figure 6. Principal component analysis (PCA) biplots on impact of ICM and CS on various crop physiological (LAI, LAR, NAR, NDVI, and SPAD at 60 DAS) and photosynthetic characteristics (flowering stage, 60 DAS), MEGY and MESY in maize from two cropping seasons (2019 and 2020). Blue triangle indicates main- and subplot treatments, i.e., CT (ICM1–ICM4), CA (ICM5–ICM8), and OA (ICM9); maize–wheat cropping system (M–W); maize + blackgram–wheat cropping system (M + B–W).
Discussion
Photosynthetic characteristics
Grain formation and crop productivity immensely depend on the photosynthetic characteristics of the crop (Long et al., 2006; Dass and Chandra, 2013; Kumari et al., 2017; Choudhary et al., 2022). In our study, we found that ICM practices significantly influenced the maize photosynthetic characteristics. Maize under CA-based ICM modules showed significant (p < 0.05) improvement in net photosynthetic rate (Pn), transpiration rate (Tr), stomatal conductance (Gs) and transpiration efficiency (TE); the magnitude of improvement ranged from 9.0 to 9.2% for Pn, 4.5 to 4.6% for Tr, 6.0 to 6.1% for Gs and 4.6 to 4.9% for TE over CT-based ICM modules, in 2019 and 2020, respectively. Blackgram intercropping had a positive influence on the maize crop and substantially improved all its photosynthetic characteristics as Pn, Tr, and TE over sole maize by increasing LAI, LAR, and LWR (Li et al., 2019). Blackgram intercropping favours maize through (i) reducing N leaching to deeper soil layers (Whitmore and Schröder, 2007; Ding et al., 2021), (ii) symbiotic nitrogen fixation (Ibrahimi et al., 2017; Weil and Brady, 2017) and (iii) smothering and suppressing the weed growth (Saudy, 2015; Choudhary and Choudhury, 2018; Choudhary et al., 2020; Gu et al., 2021), which ultimately improves soil N content. Many studies have shown that increased soil N enhances photosynthetic properties and NAR of maize (Zhang et al., 2014; Guo Y. et al., 2021; Guo F. et al., 2021). Under normal ecosystem, legumes can fix up to 28.0–84.1 kg N ha–1 (Kebede, 2021), while the amounts of nitrogen transfer from legume intercrop to companion crop vary between 21.6 and 50.9 kg N ha–1 (Mallarino et al., 1990; Fustec et al., 2010; Thilakarathna et al., 2016). In most cases, the ICM modules under the 100% RDF nutrition management option performed similarly to the 75% RDF + NPK-bf + AMF, demonstrating that the remaining 25% of nutrient requirements could be met through the synergistic action of microbial consortia and AMF (Mishra et al., 2021; Singh et al., 2021, 2022a; Khan, 2022). Similarly, the combined action of NPK-bf and AMF on P solubilisation and P mobilisation might have increased P bioavailability and shoot and root growth (Suri et al., 2011a,b; Kumar et al., 2015). The enhancement in root growth and root exploratory area due to mycorrhizal mycelia growth under AMF-applied plots may further lead to enhanced nutrient acquisition, plant growth and photosynthetic characteristics of the crop (Kumar et al., 2021, 2022; Harish et al., 2022). Similarly, the ZT system under CA-based ICM modules leads to improved nutrient bioavailability and soil fertility, soil structure, moisture conservation, microclimate modulation and more favourable soil microbiome under the harsh summer season of semiarid IGPR coinciding with an early vegetative phase of maize. All these circumstances resulted in improved plant growth and photosynthetic characteristics in maize under CA-based ICM modules (Choudhary et al., 2018, 2020; Varatharajan et al., 2019a,b; Singh et al., 2020, 2021, 2022a,2022b; Kumar et al., 2021, 2022).
Crop growth and physiological parameters
In the current study, the ICM combining different tillage and crop establishment techniques, nutrient management scenarios and crop residue retention had a significant (p < 0.05) impact on crop growth and health, as measured by NDVI and SPAD. The higher is the NDVI, the better is the plant water conditions and, hence, greener and thicker/denser is the crop canopy (Varatharajan et al., 2019a; Harish et al., 2021). That is why the sole maize or maize + blackgram intercropping in CA-based residue retained PRB plots with 100% RDF application (ICM5, ICM7) was greener, denser and with less water stress than OA-based ICM9 or CT-based FB plots with 75% RDF + NPK-bf + AMF allocation (ICM2, ICM4). Likewise, it is evident from our research that CA with PRB and 100% RDF application significantly (p < 0.05) improved the chlorophyll content (SPAD greenness) of maize plants than CT/OA with FB and 75% RDF + NPK-bf + AMF (Saudy, 2014). This was because of the improved nutrient bioavailability, lower water stress, superior soil microbial activity and favourable soil microclimate for nutrient uptake (Singh et al., 2021, 2022a; Harish et al., 2022). Further, the nutrient released from slow decomposing residues retained on CA plots might have positively impacted crop health over the entire crop duration (Jayaraman et al., 2021). ICM and cropping system interaction for NDVI and SPAD also reiterates the above facts. At the same time, the improved degradation of residue retained and the subsequent nutrients release along with improved soil microbial activity under CA might increase the available nutrients in the soil and ensure nutrient supply to maize as and when required (Sarkar et al., 2020; Jayaraman et al., 2021; Kumar et al., 2022). This might reduce the requirement for additional nutrient supplements from legume intercrop. Hence, the effect of legume intercropping was more prominent in CT/OA than CA, which denotes less available nutrients in CT/CA, which are supplemented through legume inclusion as intercrop.
Maize and blackgram intercropping caused significant (p < 0.05) improvement in LAI, LAR and LWR, while SLA and SLW did not change significantly (p < 0.05). Similarly, ICM exerted a significant (p < 0.05) improvement in DMP at the harvest stage (Figures 3A,B). Blackgram intercropping expressed its significant influence on DMA and translocation (leaf, stem, kernel) by improving maize photosynthesis and growth. In the current study, we found that maize under CA-based ICM plots had significantly higher DMA than CT and OA. Under CA, the higher DMA in leaves and stem before flowering might have helped in improving the photosynthates translocation from leaves and stem toward the kernel in post-anthesis and grain-filling stages due to enhanced N enrichment and bioavailability (Dhillon et al., 2018). Thus, increased kernel DMA suggests the improved photosynthates’ translocation efficiency from leaves and stem to sink (kernel) during grain filling. Similarly, blackgram intercropping increased the maize kernel DMP, as a result of N rhizodeposition from legume roots and improved soil nitrogen bioavailability (Fustec et al., 2010; Amanullah et al., 2021). The DMA under intercropping system also depends on the intercrop, its growth habit and competitive efficiency. In contrast to this, some researchers also reported contrasting results that intercropping negatively affected DMA and productivity of base crop (Huang et al., 2019, Arshad, 2021). Thus, the selection of the right intercrop components is very important, even though the spatial arrangement of crops may reduce competition to some extent (Dass and Sudhishri, 2010). Legume intercrop serves as an additional source of carbon input for better soil microbial activity and nutrient cycling (Choudhary et al., 2020; Singh et al., 2021, 2022a). Thus, CA and legume intercropping combinations may lead to improved soil fertility in the long run, which might contribute substantially toward the improved crop growth, DMA and DMP in the crops (Bantie, 2019). Hence, maize under CA-based ICM modules with blackgram intercropping had significantly (p < 0.05) higher leaf area per gram of plant dry weight (LAR) and leaf weight to plant dry weight (LWR), which denotes a higher proportion of leaf in whole plant dry weight. Eventually, this results in higher light interception, photosynthetic area and NAR, which in turn increases final crop productivity (Long et al., 2006). The role of crop growth and vigour in improving the photosynthetic characteristics and MEGY and MESY can be supported from our findings based on the correlation among LAI, LAR, NDVI, Pn and SPAD with MEGY and MESY, as well as multivariate (Figure 5) and PCA analysis (Figure 6).
Crop productivity
Significant (p < 0.05) improvement in plant growth and photosynthetic characteristics, NDVI, SPAD and better DMP led to increase in the maize yield under intercropping system than the sole maize cultivation. The RB/PRB planting had an efficient and denser crop canopy with higher light capture and use efficiency than FB planting, which might have helped in yield enhancement. Likewise, crop residue retained under CA-PRB reduced evaporation losses which led to improved crop productivity and water-use efficiency (WUE) even with less amount of water applied (45 mm/irrigation) compared to FB (60 mm/irrigation). However, likely greater evaporation loss and higher competition from weeds resulting from less denser crop canopy under FB-based ICM modules eventually led to reduction in crop productivity and WUE. Besides, intercropped blackgram increases the photosynthetically active radiation (PAR) interception by utilising the transmitted radiation from maize crop by actively growing and shading the ground (Awal et al., 2006; Liu et al., 2017). Thus, the intercropping increases the MEGY of the system. In addition, atmospheric nitrogen fixation, nutrient exudates from legume roots under blackgram intercropping might have been beneficial in increasing the grain and stover yield of maize (Kakraliya et al., 2018; Pooniya et al., 2018). The intercropped maize has a substantial advantage over the sole maize cultivation in higher light interception from understorey blackgram due to the increased LAI and land covering (Rajpoot et al., 2018). This, in turn, increases light-use efficiency and crop productivity of intercropping system than sole crop (Kermah et al., 2017; Rajpoot et al., 2018). Additional intercrop yield further improves the land-use efficiency by improving the land equivalent ratio (Xu et al., 2020; Feng et al., 2021). By boosting the rhizospheric N and P cycling through microbial populations, the CA practices enhance soil available N and P (Singh et al., 2021, 2022a,2022b). Furthermore, under the maize–legume intercropping system, the legume component considerably influences the carbon, nitrogen and phosphorus metabolism in the soil, hence, improving nutrient bioavailability to both the base crop and the intercrop (Guo Y. et al., 2021; Singh et al., 2021, 2022a). Likewise, the crop residue retention and its decomposition under CA-based ICM modules might have improved the SOC, nutrient bioavailability, soil moisture retention, microclimate modulation and biological activity, hence all resulting in greater growth, photosynthesis and finally crop productivity (Varatharajan et al., 2019b; Choudhary et al., 2020; Singh et al., 2020, 2021, 2022a,b; Harish et al., 2022). Furthermore, the ZT system under CA-based ICM leads to favourable characteristics, such as reduced resource competition, root aeration and high fertiliser use efficiency, which might have resulted in higher grain output than the CT-based ICM modules (Harish et al., 2021, 2022). Lesser farm machinery trafficking, better root aeration and improved soil health parameters lead to improved shoot and root growth, which again might have contributed in enhancing the maize productivity in CA plots (Varatharajan et al., 2019b; Kumar et al., 2021, 2022). It is also noticed that there is lesser water stagnation after heavy rains vis-à-vis enhanced moisture retention during dry spells in maize under the CA system (Choudhary et al., 2018, 2020; Harish et al., 2021, 2022). In nutshell, all these favourable conditions under the CA-based modules led to overall superior physiological and photosynthetic characteristics with less environmental stress which collectively resulted in better crop productivity.
Conclusion
The present study provides major insights into the positive impacts of the ICM modules and legume intervention on crop physiological and photosynthetic characteristics and productivity of sole and intercropped maize. Among the ICM modules, conservation agriculture (CA)-based ICM was superior to conventional tillage (CT)- and organic agriculture (OA)-based ICM for all the studied parameters, including overall productivity. In general, the CA-based ICM module ICM7 proved superior with significant improvements in photosynthetic rate, transpiration rate and NAR, crop vigour, DMP to the grain and finally maize productivity by 13.4–14.2% and 27.3–28.0% higher than the CT- and OA-based modules in 2019 and 2020, respectively. Nutrient management options like 100% RDF and 75% RDF + NPK-bf + AMF also stood on par with each other. Furthermore, blackgram intercropping in maize played a significant role in improving maize productivity over sole maize due to improved crop physiological and photosynthetic characteristics. Likewise, MEGY was significantly (p < 0.05) higher in CA-PRB-based ICM modules followed by CA-FB-, CT-RB- and CT-FB-based ICM modules. The MEGY of blackgram-intercropped maize was higher by 16.9–17.9% over sole maize in the current study. The MEGY followed the trend of ICM7 > ICM8 > ICM5 > ICM6 > ICM3 > ICM4 > ICM1 > ICM2 > ICM9. Overall, crop physiological and photosynthetic characteristics and maize productivity were found to be significantly higher in the CA-based ICM7 module with blackgram intercropping; hence, this technology package can be adopted widely to enhance the maize production in maize-growing semiarid regions of India and South Asia.
Data availability statement
The original contributions presented in this study are included in the article/supplementary material, further inquiries can be directed to the corresponding author/s.
Author contributions
TV: conceptualisation, methodology formulation and implementation, resource, review, and original draft preparation. AD: conceptualisation, project administration, methodology formulation and implementation, and review and editing. AC: conceptualisation, project administration, methodology formulation, and review and editing. SS and KS: results compilation and draft preparation. VP, SP, AS, PK, and TD: review of literature and basic analysis. GR: results compilation and draft preparation. MH: results compilation and draft preparation and editing. SD and RS: data collection and processing and original draft preparation. RR: review of literature and results compilation. KK: editing of original and revised versions of the manuscript. KSS: data collection and processing and original draft preparation. All authors contributed to the article and approved the submitted version.
Acknowledgments
The authors were thankful to ICAR-Indian Agricultural Research Institute, New Delhi, for arranging necessary experimental field and laboratory facilities. TV was thankful to the University Grants Commission (UGC), New Delhi, for providing Senior Research Fellowship during the course of study.
Conflict of interest
The authors declare that the research was conducted in the absence of any commercial or financial relationships that could be construed as a potential conflict of interest.
Publisher’s note
All claims expressed in this article are solely those of the authors and do not necessarily represent those of their affiliated organizations, or those of the publisher, the editors and the reviewers. Any product that may be evaluated in this article, or claim that may be made by its manufacturer, is not guaranteed or endorsed by the publisher.
Abbreviations
ANOVA, analysis of variance; AMF, arbuscular mycorrhizal fungi; CA, conservation agriculture; CS, cropping system; CT, conventional tillage; DAP, diammonium phosphate; DAS, days after sowing; DMA, dry matter accumulation; DMP, dry matter partitioning; FB, flatbed; Gs, stomatal conductance; ha, hectare; ICM, integrated crop management; IGPR, Indo-Gangetic plains region; IRGA, infrared gas analyser; K, potassium; MEBY, maize equivalent biological yield; MEGY, maize equivalent grain yield; MESY, maize equivalent stover yield; MVA, multivariate analysis; MWCS, maize–wheat cropping system; N, nitrogen; NAR, net assimilation rate; NDVI, normalised difference vegetation index; NPK-bf, NPK biofertiliser; LAI, leaf area index; LAR, leaf area ratio; LDW, leaf dry weight; LWR, leaf weight ratio; OA, organic agriculture; P, phosphorus; Pn, net photosynthetic rate; PAR, photosynthetically active radiation; PCA, principal component analysis; PRB, permanent raised bed; RB, raised bed; RUE, resource-use efficiency; RDF, recommended dose of fertilizer; RWCS, rice–wheat cropping system; SDW, stem dry weight; SLA, specific leaf area; SOC, soil organic carbon; SLW, specific leaf weight; SPAD, soil plant analysis development; Tr, leaf transpiration rate; t ha–1, tonnes ha–1; ZT, zero tillage.
References
Amanullah, Khalid, S., Khalil, F., Elshikh, M. S., Alwahibi, M. S., Alkahtani, J., et al. (2021). Growth and dry matter partitioning response in cereal-legume intercropping under full and limited irrigation regimes. Sci. Rep. 11:12585. doi: 10.1038/s41598-021-92022-4
Arshad, M. (2021). Fortnightly dynamics and relationship of growth, dry matter partition and productivity of maize based sole and intercropping systems at different elevations. Eur. J. Agron. 130:126377. doi: 10.1016/j.eja.2021.126377
Awal, M. A., Koshi, H., and Ikeda, T. (2006). Radiation interception and use by maize/peanut intercrop canopy. Agric. For. Meteorol. 139, 74–83. doi: 10.1016/j.agrformet.2006.06.001
Bantie, Y. (2019). Conservation agriculture based annual intercropping system for sustainable crop production: A review. Indian J. Ecol. 46, 235–249.
Bhatt, R., Kukal, S., Arora, S., Busari, M., and Yadav, M. (2016). Sustainability issues on rice-wheat cropping system. Int. Soil Water Conserv. Res. 4, 67–71. doi: 10.1016/j.iswcr.2015.12.001
Biswakarma, N., Pooniya, V., Zhiipao, R. R., Kumar, D., Verma, A. K., Shivay, Y. S., et al. (2021). Five years integrated crop management in direct seeded rice-zero till wheat rotation of NW India: Effects on soil carbon dynamics, crop yields, water productivity and economic profitability. Agric. Ecosyst. Environ. 318:107492. doi: 10.1016/j.agee.2021.107492
Choudhary, A. K., Bana, R. S., and Pooniya, V. (2018). Integrated Crop Management Practices For Enhancing Productivity, Resource-Use Efficiency, Soil Health And Livelihood Security. New Delhi: ICAR-IARI.
Choudhary, A. K., Sood, P., Rahi, S., Yadav, D. S., Thakur, O. C., Siranta, K. R., et al. (2022). Rice productivity, Zn-biofortification and nutrient-use efficiency as influenced by Zn-fertilization under conventional transplanted-rice and the system of rice intensification. Front. Environ. Sci. 10:869194. doi: 10.3389/fenvs.2022.869194
Choudhary, A. K., Varatharajan, T., Rohullah, Bana, R. S., Pooniya, V., Dass, A., et al. (2020). Integrated crop management technology for enhanced productivity, resource-use efficiency and soil health in legumes-A review. Indian J. Agric. Sci. 90, 1839–1849.
Choudhary, A. K., Yadav, D. S., Sood, P., Rahi, S., Arya, K., Thakur, S. K., et al. (2021). Post-emergence herbicides for effective weed management, enhanced wheat productivity, profitability and quality in NW Himalayas: A ‘participatory-mode’ technology development and dissemination. Sustainability 13:5425. doi: 10.3390/su13105425
Choudhary, V. K., and Choudhury, B. U. (2018). A staggered maize–legume intercrop arrangement influences yield, weed smothering and nutrient balance in the eastern himalayan region of india. Exp. Agric. 54, 181–200. doi: 10.1017/S0014479716000144
Dass, A., and Chandra, S. (2013). Irrigation, spacing and cultivar effects on net photosynthetic rate, dry matter partitioning and productivity of rice under system of rice intensification in Mollisols of northern India. Exp. Agric. 49, 504–523. doi: 10.1017/S0014479713000252
Dass, A., Shekhawat, K., Choudhary, A. K., Sepat, S., Rathore, S. S., Mahajan, G., et al. (2017). Weed management in rice using crop competition-a review. Crop Prot. 95, 45–52. doi: 10.1016/j.cropro.2016.08.005
Dass, A., and Sudhishri, S. (2010). Intercropping in fingermillet (Eleusine coracana) with pulses for enhanced productivity, resource conservation and soil fertility in uplands of southern Orissa. Indian J. Agron. 55, 89–94. doi: 10.1007/s10705-010-9375-3
Dhillon, B. S., Sharma, P. K., and Choudhary, A. K. (2018). Influence of staggered sown spring sunflower (Helianthus annuus L.) at varying intra-row spacing and applied-N on pre- and post-anthesis N dynamics and dry matter partitioning in Indo-Gangetic plains region. Commun. Soil Sci. Plant Anal. 49, 2002–2015. doi: 10.1080/00103624.2018.1492602
Ding, Y., Huang, X., Li, Y., Liu, H., Zhang, Q., Liu, X., et al. (2021). Nitrate leaching losses mitigated with intercropping of deep-rooted and shallow-rooted plants. J. Soils Sediments 21, 364–375. doi: 10.1007/s11368-020-02733-w
Feng, C., Sun, Z., Zhang, L., Feng, L., Zheng, J., Bai, W., et al. (2021). Maize/peanut intercropping increases land productivity: A meta-analysis. Field Crops Res. 270:108208. doi: 10.1016/j.fcr.2021.108208
Fustec, J., Lesuffleur, F., Mahieu, S., and Cliquet, J. B. (2010). Nitrogen rhizodeposition of legumes-A review. Agron. Sustain. Dev. 30, 57–66. doi: 10.1051/agro/2009003
Gu, C., Bastiaans, L., Anten, N. P. R., Makowski, D., and van der Werf, W. (2021). Annual intercropping suppresses weeds: A meta-analysis. Agric. Ecosyst. Environ. 322:107658. doi: 10.1016/j.agee.2021.107658
Guo, Y., Yin, W., Fan, H., Fan, Z., Hu, F., Yu, A., et al. (2021). Photosynthetic physiological characteristics of water and N coupling for enhanced high-density tolerance and increased yield of maize in arid irrigation regions. Front. Plant Sci. 12:34630472. doi: 10.3389/fpls.2021.726568
Guo, F., Wang, M., Si, T., Wang, Y., Zhao, H., Zhang, X., et al. (2021). Maize-peanut intercropping led to an optimization of soil from the perspective of soil microorganism. Arch. Agron. Soil Sci. 67, 1986–1999. doi: 10.1080/03650340.2020.1818725
Harish, M. N., Choudhary, A. K., Dass, A., Singh, V. K., Pooniya, V., and Varatharajan, T. (2021). Tillage and phosphorus management in maize (Zea mays L.) under maize-wheat cropping system. Indian J. Agric. Sci. 91, 117–122.
Harish, M. N., Choudhary, A. K., Kumar, S., Dass, A., Singh, V. K., Sharma, V. K., et al. (2022). Double zero-tillage and foliar-P fertilization coupled with microbial-inoculants lead to improved maize productivity and quality in a maize–wheat rotation of semi-arid agro-ecology. Sci. Rep. 12:3161. doi: 10.1038/s41598-022-07148-w
Huang, C. D., Liu, Q. Q., Li, X. L., and Zhang, C. C. (2019). Effect of intercropping on maize grain yield and yield components. J. Integr. Agric. 18, 1690–1700. doi: 10.1016/S2095-3119(19)62648-1
Ibrahimi, F., Rana, K. S., Choudhary, A. K., Dass, A., Ehsan, Q., and Noorzai, A. U. (2017). Effect of varieties and planting geometry on growth, yield and profitability of Kharif mungbean (Vigna radiata) in southern Afghanistan. Ann. Agric. Res. 38, 185–193.
Jayaraman, S., Sinha, N. K., Mohanty, M., Hati, K. M., Chaudhary, R. S., Shukla, A. K., et al. (2021). Conservation tillage, residue management, and crop rotation effects on soil major and micro-nutrients in semi-arid Vertisols of India. J. Soil Sci. Plant Nutr. 21, 523–535. doi: 10.1007/s42729-020-00380-1
Kakraliya, S. K., Singh, U., Bohra, A., Choudhary, K. K., Kumar, S., Meena, R. S., et al. (2018). “Nitrogen and legumes: A meta-analysis,” in Legumes For Soil Health And Sustainable Management, eds R. S. Meena, A. Das, G. S. Yadav, and R. Lal (Berlin: Springer), 277–314. doi: 10.1007/978-981-13-0253-4_9
Kebede, E. (2021). Contribution, utilization, and improvement of legumes-driven biological nitrogen fixation in agricultural systems. Front. Sustain. Food Syst. 5:767998. doi: 10.3389/fsufs.2021.767998
Kermah, M., Franke, A. C., Adjei-Nsiah, S., Ahiabor, B. D. K., Abaidoo, R. C., and Giller, K. E. (2017). Maize-grain legume intercropping for enhanced resource use efficiency and crop productivity in the Guinea savanna of northern Ghana. Field Crops Res. 213, 38–50. doi: 10.1016/j.fcr.2017.07.008
Khan, S. T. (2022). Consortia-based microbial inoculants for sustaining agricultural activities. Appl. Soil Ecol. 176:104503. doi: 10.1016/j.apsoil.2022.104503
Kumar, A., Rana, K. S., Choudhary, A. K., Bana, R. S., Sharma, V. K., Gupta, G., et al. (2022). Sole- or dual-crop basis residue-mulching and Zn-fertilization lead to improved productivity, rhizo-modulation and soil health in zero-tilled pigeonpea–wheat cropping system. J. Soil Sci. Plant Nutr. 22, 1193–1214. doi: 10.1007/s42729-021-00723-6
Kumar, A., Rana, K. S., Choudhary, A. K., Bana, R. S., Sharma, V. K., Prasad, S., et al. (2021). Energy budgeting and carbon footprints of zero-tilled pigeonpea–wheat cropping system under sole or dual crop basis residue mulching and Zn-fertilization in a semiarid agro-ecology. Energy 231:120862. doi: 10.1016/j.energy.2021.120862
Kumar, A., Suri, V. K., Choudhary, A. K., Yadav, A., Kapoor, R., Sandal, S., et al. (2015). Growth behavior, nutrient harvest index and soil fertility in okra-pea cropping system as influenced by AM fungi, applied phosphorus and irrigation regimes in Himalayan acid Alfisol. Commun. Soil Sci. Plant Anal. 46, 2212–2233. doi: 10.1080/00103624.2015.1069323
Kumari, K., Dass, A., Sudhishri, S., Kaur, R., and Rani, A. (2017). Yield components, yield and nutrient uptake pattern in maize under varying irrigation and N levels. Indian J. Agron. 62, 104–107.
Kvet, J., Ondok, J. P., Necas, J., and Jarvis, P. G. (1971). “Methods of growth analysis,” in Plant Photosynthetic Production: Manual And Methods, eds Z. Sesthk, J. Catsky, and P. G. Jarvis (The Hague: Dr. W. Junk N. V. Publishers), 343–384.
Li, L., Zhang, F., Li, X., Christie, P., Sun, J., Yang, S., et al. (2003). Interspecific facilitation of nutrient uptake by intercropped maize and faba bean. Nutr. Cycl. Agroecosyst. 65, 61–71. doi: 10.1023/A:1021885032241
Li, Y. H., Shi, D. Y., Li, G. H., Zhao, B., Zhang, J. W., Liu, P., et al. (2019). Maize/peanut intercropping increases photosynthetic characteristics, 13C-photosynthate distribution, and grain yield of summer maize. J. Integr. Agric. 18, 2219–2229. doi: 10.1016/S2095-3119(19)62616-X
Liu, X., Rahman, T., Yang, F., Song, C., Yong, T., Liu, J., et al. (2017). PAR Interception and Utilization in Different Maize and Soybean Intercropping Patterns. PLoS One 12:e0169218. doi: 10.1371/journal.pone.0169218
Long, S. P., Zhu, X. G., Naidu, S. L., and Ort, D. R. (2006). Can improvement in photosynthesis increase crop yields? Plant Cell Environ. 29, 315–330. doi: 10.1111/j.1365-3040.2005.01493.x
Maitra, S., Hossain, A., Brestic, M., Skalicky, M., Ondrisik, P., Gitari, H., et al. (2021). Intercropping-A low input agricultural strategy for food and environmental security. Agronomy 11:343. doi: 10.3390/agronomy11020343
Mallarino, A. P., Wedin, W. F., Perdomo, C. H., Goyenola, R. S., and West, C. P. (1990). Nitrogen transfer from white clover, red clover, and birdsfoot trefoil to associated grass. Agron. J. 82, 790–795. doi: 10.2134/agronj1990.00021962008200040027x
Mishra, M., Singh, S. K., and Kumar, A. (2021). “Microbial consortia: approaches in crop production and yield enhancement,” in Microbiome Stimulants for Crops, eds J. White, A. Kumar, and S. Droby (Cambridge: Woodhead Publishing), 293–303. doi: 10.1016/B978-0-12-822122-8.00013-3
Nyawade, S. O., Gachene, C. K. K., Karanja, N. N., Gitari, H. I., Schulte-Geldermann, E., and Parker, M. L. (2019). Controlling soil erosion in smallholder potato farming systems using legume intercrops. Geoderma Reg. 17:e00225. doi: 10.1016/j.geodrs.2019.e00225
Paul, J., Choudhary, A. K., Suri, V. K., Sharma, A. K., Kumar, V., and Shobhna. (2014). Bioresource nutrient recycling and its relationship with biofertility indicators of soil health and nutrient dynamics in rice-wheat cropping system. Commun. Soil Sci. Plant Anal. 45, 912–924. doi: 10.1080/00103624.2013.867051
Pearce, R. B., Brown, R. H., and Blaser, R. E. (1968). Photosynthesis of alfalfa leaves as influenced by age and environment. Crop Sci. 8, 677–680. doi: 10.2135/cropsci1968.0011183X000800060011x
Pooniya, V., Choudhary, A. K., Bana, R. S., Swarnalaxami, K., Pankaj, Rana, D. S., et al. (2018). Influence of summer legume residue-recycling and varietal diversification on productivity, energetics and nutrient dynamics in basmati rice-wheat cropping system of western Indo-Gangetic plains. J. Plant Nutr. 41, 1491–1506. doi: 10.1080/01904167.2018.1458868
Pooniya, V., Zhiipao, R. R., Biswakarma, N., Kumar, D., Shivay, Y. S., Babu, S., et al. (2022). Conservation agriculture based integrated crop management sustains productivity and economic profitability along with soil properties of the maize-wheat rotation. Sci. Rep. 12:1962. doi: 10.1038/s41598-022-05962-w
Radford, P. J. (1967). Growth analysis formulae - Their use and abuse. Crop Sci. 7, 171–175. doi: 10.2135/cropsci1967.0011183X000700030001x
Rajpoot, S. K., Rana, D. S., and Choudhary, A. K. (2018). Bt-cotton–vegetable-based intercropping systems as influenced by crop establishment method and planting geometry of Bt-cotton in Indo-Gangetic plains region. Curr. Sci. 115, 516–522. doi: 10.18520/cs/v115/i3/516-522
Rana, K. S., Choudhary, A. K., Sepat, S., Bana, R. S., and Dass, A. (2014). Methodological and Analytical Agronomy. New Delhi: Indian Agricultural Research Institute New Delhi.
Sangakkara, R. (1994). Growth, yield and nodule activity of mungbean intercropped with maize and cassava. J. Sci. Food Agric. 66, 417–421. doi: 10.1002/jsfa.2740660322
Sarkar, S., Skalicky, M., Hossain, A., Brestic, M., Saha, S., Garai, S., et al. (2020). Management of crop residues for improving input-use efficiency and agricultural sustainability. Sustainability 12:9808. doi: 10.3390/su12239808
Saudy, H. S. (2014). Chlorophyll meter as a tool for forecasting wheat N requirements after application of herbicides. Arch. Agron. Soil Sci. 60, 1077–1090. doi: 10.1080/03650340.2013.866226
Saudy, H. S. (2015). Maize-cowpea intercropping as an ecological approach for N-use rationalization and weed suppression. Arch. Agron. Soil Sci. 61, 1–14. doi: 10.1080/03650340.2014.920499
Sharma, A. R., and Dass, A. (2012). “Maize,” in Text Book of Crop Production, ed. R. Prasad (Copenhagen: DKMA), 98–136.
Singh, U., Choudhary, A. K., and Sharma, S. (2020). Comparative performance of conservation agriculture vis-a-vis organic and conventional farming in enhancing plant attributes and rhizospheric bacterial diversity in Cajanus cajan: A field study. Eur. J. Soil Biol. 99:103197. doi: 10.1016/j.ejsobi.2020.103197
Singh, U., Choudhary, A. K., and Sharma, S. (2021). Agricultural practices modulate the bacterial communities, and nitrogen cycling bacterial guild in rhizosphere: Field experiment with soybean. J. Sci. Food Agric. 101, 2687–2695. doi: 10.1002/jsfa.10893
Singh, U., Choudhary, A. K., and Sharma, S. (2022a). A 3-year field study reveals that agri-management practices drive the dynamics of dominant bacterial taxa in the rhizosphere of Cajanus cajan. Symbiosis 86, 215–227. doi: 10.1007/s13199-022-00834-3
Singh, U., Choudhary, A. K., Varatharajan, T., and Sharma, S. (2022b). Agricultural management practices affect the abundance of markers of phosphorus cycle in soil: Case study with pigeonpea and soybean. J. Soil Sci. Plant Nutr. doi: 10.1007/s42729-022-00863-3 [Epub ahead of print].
Sun, B. R., Peng, Y., Yang, H., Li, Z. J., Gao, Y. Z., Wang, C., et al. (2014). Alfalfa/maize intercropping provides a feasible way to improve yield and economic incomes in farming and pastoral areas of northeast China. PLoS One 9:e110556. doi: 10.1371/journal.pone.0110556
Suri, V. K., Choudhary, A. K., Chander, G., and Verma, T. S. (2011a). Influence of VAM fungi and applied-P on root colonization in wheat and plant nutrient dynamics in a phosphorus-deficient acid Alfisol of western Himalayas. Commun. Soil Sci. Plant Anal. 42, 1177–1186. doi: 10.1080/00103624.2011.566962
Suri, V. K., Choudhary, A. K., Chander, G., Gupta, M. K., and Dutt, N. (2011b). Improving phosphorus use through co-inoculation of VAM fungi and PSB in maize in an acid Alfisol. Commun. Soil Sci. Plant Anal. 42, 2265–2273. doi: 10.1080/00103624.2011.602451
Thilakarathna, M. S., McElroy, M. S., Chapagain, T., Papadopoulos, Y. A., and Raizada, M. N. (2016). Belowground N transfer from legumes to non-legumes under managed herbaceous cropping systems. A review. Agron. Sustain. Dev. 36:58. doi: 10.1007/s13593-016-0396-4
Tripathi, S. C., Venkatesh, K., Meena, R. P., Chander, S., and Singh, G. P. (2021). Sustainable intensification of maize and wheat cropping system through pulse intercropping. Sci. Rep. 11:18805. doi: 10.1038/s41598-021-98179-2
Varatharajan, T., Choudhary, A. K., Pooniya, V., Dass, A., and Harish, M. N. (2019a). Integrated crop management practices for enhancing crop productivity, profitability, production-efficiency and monetary-efficiency in pigeonpea (Cajanus cajan) in Indo-Gangetic plains region. Indian J. Agric. Sci. 89, 559–563.
Varatharajan, T., Choudhary, A. K., Pooniya, V., Dass, A., Meena, M. C., Gurung, B., et al. (2019b). Influence of ICM practices on yield, PAR-interception, resource-use-efficiency and energetics in pigeonpea in north-Indian plains. J. Environ. Biol. 40, 1204–1210. doi: 10.22438/jeb/40/6/MRN-1073
Weil, R., and Brady, N. (2017). The Nature and Properties of Soils. London: Pearson Education Limited.
Whitmore, A. P., and Schröder, J. J. (2007). Intercropping reduces nitrate leaching from under field crops without loss of yield: A modelling study. Eur. J. Agron. 27, 81–88. doi: 10.1016/j.eja.2007.02.004
Williams, S. R. F. (1946). “Methods of growth analysis,” in Plant Photosynthetic Production. Manual of Methods, eds Z. Sesták, J. Catský, and P. G. Jarvis (The Hague: Drow, Jenk N.U. Publishers), 348–391.
Xu, Z., Li, C., Zhang, C., Yu, Y., van der Werf, W., and Zhang, F. (2020). Intercropping maize and soybean increases efficiency of land and fertilizer N use: A meta-analysis. Field Crops Res. 246:107661. doi: 10.1016/j.fcr.2019.107661
Yadav, D. S., Choudhary, A. K., Sood, P., Thakur, S. K., Rahi, S., and Arya, K. (2015). Scaling-up of maize productivity, profitability and adoption through frontline demonstration technology-transfer programme using promising maize hybrids in Himachal Pradesh. Ann. Agric. Res. 36, 331–338.
Zhang, G. G., Yang, Z. B., and Dong, S. T. (2011). Interspecific competitiveness affects the total biomass yield in an alfalfa and corn intercropping system. Field Crops Res. 124, 66–73. doi: 10.1016/j.fcr.2011.06.006
Zhang, J., Yin, B., Xie, Y., Li, J., Yang, Z., and Zhang, G. (2015). Legume-cereal intercropping improves forage yield, quality and degradability. PLoS One 10:e0144813. doi: 10.1371/journal.pone.0144813
Keywords: integrated crop management, blackgram intercropping, conservation agriculture, cropping systems, dry matter partitioning, photosynthetic rate
Citation: Varatharajan T, Dass A, Choudhary AK, Sudhishri S, Pooniya V, Das TK, Rajanna GA, Prasad S, Swarnalakshmi K, Harish MN, Dhar S, Singh R, Raj R, Kumari K, Singh A, Sachin KS and Kumar P (2022) Integrated management enhances crop physiology and final yield in maize intercropped with blackgram in semiarid South Asia. Front. Plant Sci. 13:975569. doi: 10.3389/fpls.2022.975569
Received: 22 June 2022; Accepted: 31 August 2022;
Published: 23 September 2022.
Edited by:
Min Huang, Hunan Agricultural University, ChinaReviewed by:
Nester Mashingaidze, One Acre Fund, RwandaLorenzo Barbanti, University of Bologna, Italy
Copyright © 2022 Varatharajan, Dass, Choudhary, Sudhishri, Pooniya, Das, Rajanna, Prasad, Swarnalakshmi, Harish, Dhar, Singh, Raj, Kumari, Singh, Sachin and Kumar. This is an open-access article distributed under the terms of the Creative Commons Attribution License (CC BY). The use, distribution or reproduction in other forums is permitted, provided the original author(s) and the copyright owner(s) are credited and that the original publication in this journal is cited, in accordance with accepted academic practice. No use, distribution or reproduction is permitted which does not comply with these terms.
*Correspondence: Anchal Dass, YW5jaGFsX2RAcmVkaWZmbWFpbC5jb20=; Anil K. Choudhary, YW5pbGhwYXUyMDEwQGdtYWlsLmNvbQ==
†These authors share first authorship