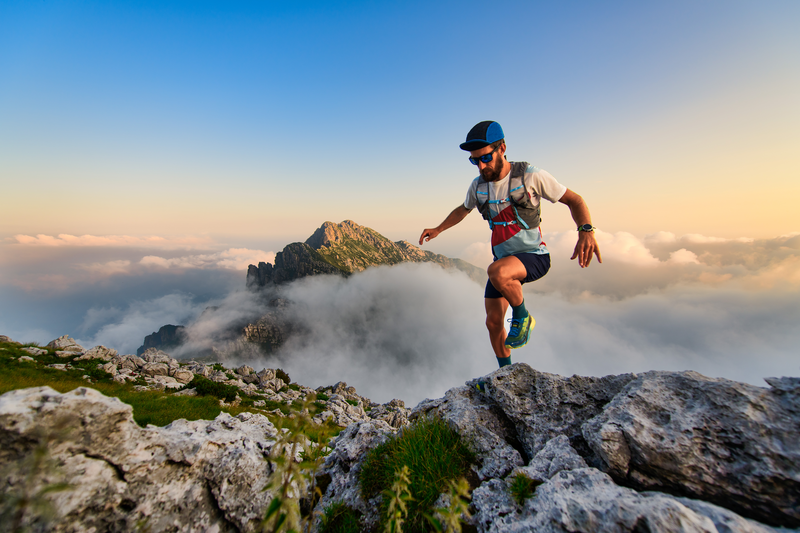
95% of researchers rate our articles as excellent or good
Learn more about the work of our research integrity team to safeguard the quality of each article we publish.
Find out more
ORIGINAL RESEARCH article
Front. Plant Sci. , 26 October 2022
Sec. Plant Breeding
Volume 13 - 2022 | https://doi.org/10.3389/fpls.2022.973065
Powdery mildew caused by Blumeria graminis f. sp. tritici (Bgt) is a destructive disease of wheat throughout the world. Host resistance is considered the most sustainable way to control this disease. Powdery mildew resistance gene Pm2b was mapped to the same genetic interval with Pm2a and PmCH1357 cloned previously, but showed different resistance spectra from them, indicating that they might be caused by different resistance genes or alleles. In this study, Pm2b was delimited to a 1.64 Mb physical interval using a large segregating population containing 4,354 F2:3 families of resistant parent KM2939 and susceptible cultivar Shimai 15. In this interval, TraesCS5D03G0111700 encoding the coiled-coil nucleotide-binding site leucine-rich repeat protein (CC-NBS-LRR) was determined as the candidate gene of Pm2b. Silencing by barley stripe mosaic virus-induced gene silencing (BSMV-VIGS) technology and two independent mutants analysis in KM2939 confirmed the candidate gene TraesCS5D03G0111700 was Pm2b. The sequence of Pm2b was consistent with Pm2a/PmCH1357. Subcellular localization showed Pm2b was located on the cell nucleus and plasma membrane. Pm2b had the highest expression level in leaves and was rapidly up-regulated after inoculating with Bgt isolate E09. The yeast two-hybrid (Y2H) and luciferase complementation imaging assays (LCI) showed that PM2b could self-associate through the NB domain. Notably, we identified PM2b interacting with the transcription factor TaWRKY76-D, which depended on the NB domain of PM2b and WRKY domain of TaWRKY76-D. TaWRKY76-D negatively regulated the resistance to powdery mildew in wheat. The specific KASP marker K529 could take the advantage of high-throughput and high-efficiency for detecting Pm2b and be useful in molecular marker assisted-selection breeding. In conclusion, cloning and disease resistance mechanism analysis of Pm2b provided an example to emphasize a need of the molecular isolation of resistance genes, which has implications in marker assisted wheat breeding.
Wheat (Triticum aestivum L., 2n=6x=42, AABBDD) is one of the most important food crops in the world and provides 20% of the total daily calories and protein consumed by humankind (Reynolds et al., 2012). Diseases and pests lead to a serious threat to the global production of wheat grain and food supply (Savary et al., 2019). Powdery mildew caused by Blumeria graminis f. sp. tritici (Bgt) is a destructive disease of wheat worldwide (Gao et al., 2018; Singh et al., 2016). Chemical prevention is usually used to control this disease, but will increase the cost, accelerate the variation of Bgt isolates and endanger the environment (Bennett, 1984; Bliffeld et al., 1999). Host resistance is the most economical, efficient, and environmental-friendly method to prevent powdery mildew (Koller et al., 2018; Sun et al., 2018). To date, 68 powdery mildew resistance genes (Pm) at 63 loci (Pm1-Pm68) (Pm8 = Pm17, Pm18 = Pm1c, Pm22 = Pm1e, Pm23 = Pm4c, and Pm31 = Pm21) have been identified from diversified genotypes (McIntosh et al., 2019; He et al., 2020).
Among the reported Pm loci, an interesting multi-allelic phenomenon is that seven Pm loci (Pm1a-Pm1e, Pm2a-Pm2c, Pm3a-3j, Pm4a-4d, Pm5a-5e, Pm24a-24b and Pm60-60b) have more than one resistance allele (Qie et al., 2019; Zou et al., 2022). Studies of multi-allelic race-specific R genes reveal that they evolve to recognize specific avirulence (Avr) alleles from the pathogen under strong diversifying selection (Rose et al., 2004; Bhullar et al., 2009; Kanzaki et al., 2012). For instance, 17 functional alleles confer race-specific resistance to powdery mildew at Pm3 locus (Bhullar et al., 2009; Brunner et al., 2010). PM3 belongs to the sub-group of nucleotide binding site and leucine rich repeat (NBS-LRR) proteins including an N-terminal coiled-coil (CC) domain. The CC domain is completely conserved among these alleles, while sequence exchange is detected in the NBS and LRR regions, indicating intragenic recombination or gene conversion between alleles (Bourras et al., 2015). Pm2 has been widely used in the world due to its significant resistance to powdery mildew in a long history (Jin et al., 2021). At Pm2 locus, several alleles have been identified from a series of diversified genotypes (Jin et al., 2018). These alleles have significantly different resistance spectra to Bgt isolates, which is crucial to the study of genetic diversity and variation of Pm2 and provides valuable resources for wheat disease resistance breeding. Pm2a, derived from American wheat cultivar Ulka, was cloned by mutant chromosome sequencing (MutChromSeq) for six ethylmethylsulfone (EMS)-induced mutants (Sánchez-Martín et al., 2016). PmCH1357, derived from wheat line CH1357, was map-based cloned combining with 90K SNP array. Further analysis showed that the sequence of PmCH1357 was completely consistent with that of Pm2a (Chen et al., 2019).
Plants have evolved two layered immune systems to prevent invading pathogens (Dodds and Rathjen, 2010; Dangl et al., 2013). The first layer -, triggered by perception of microbe-associated molecular patterns (MAMPs) or pathogen-associated molecular patterns (PAMPs), is known as pattern-triggered immunity (PTI) (Jones and Dangl, 2006; Boller and Felix, 2009; Boller and He, 2009). The second layer is that intracellular nucleotide-binding leucine-rich-repeat (NLR) directly or indirectly perceives pathogen effectors and leads to effector-triggered immunity (ETI) (Cui et al., 2015; Zhang et al., 2017). ETI is governed by NLR and often accompanied by the occurrence of cell death around pathogen infection sites, which is believed to limit the spread of pathogens (Dangl et al., 2013; Jones et al., 2016). In wheat, nine of 13 cloned Pm genes, including Pm1, Pm2a, Pm3, Pm5, Pm8, Pm17, Pm21, Pm41 and Pm60, encode NLR immune receptors that recognized pathogen effectors and activated ETI pathway. In general, NLRs are present in an inactive state without specific effectors (Praz et al., 2017). Intramolecular interactions could help to keep the NLR proteins in an autoinhibition state (Rairdan et al., 2008; Wang et al., 2015). However, the PTI and ETI pathways triggered by wheat powdery mildew resistance genes have been ambiguous.
NLR gene can regulate the disease resistance pathway by recruiting transcription factors (TFs) in plants. Transcription factors related to plant stress mainly include WRKY, MYB, NAC, bZIP and AP2/ERF. Plant WRKY TFs which are specific in higher plants play important roles in the regulation of transcriptional processes to modulate pathogen triggered cellular responses (Pandey and Somssich, 2009; Tsuda and Somssich, 2015). Some WRKY TFs, such as WRKY IIa subgroup members, have been shown to act as regulators of plant immunity. For instance, the chimeric protein AtWRKY52/RRS1, which possesses the dual structure and function of coiled-coil nucleotide-binding site leucine-rich repeat (CNL) protein and WRKY TF, interacts with the effector PopP2 in nucleus to be resistant to Ralstonia solanacearum in Arabidopsis (Tasset et al., 2022). Rice panicle blast gene Pb1 encodes a CNL disease resistance protein which depends on interacting with WRKY45 in the nucleus to regulate the resistance (Inoue et al., 2013). MLA10 interacts with HvWRKY1/HvWRKY2 through the CC domain to relieve the inhibition of HvWRKY1/HvWRKY2 on barley powdery mildew resistance, which makes barely plants resistant to powdery mildew (Maekawa et al., 2011). However, the interactions between Pm genes and transcription factors remain to be further elucidated in wheat.
Wheat breeding line KM2939 that carried Pm2b was highly resistant to powdery mildew at both seedling and adult stages. Pm2b was flanked by Xcfd81 and Xswgi011 with genetic distance 0.5 and 1.3 cM on the chromosome 5DS, respectively (Ma et al., 2015). In this study, we intend to map-based clone Pm2b, reveal the molecular mechanism of regulating resistance to powdery mildew by screening the interacted protein of PM2, then develop high-throughput and efficient markers to provide a reliable tool for marker-assisted selection breeding (MAS) of Pm2b.
The population of 4,354 F2:3 families, derived from a cross of the highly resistant wheat breeding line KM2939 and highly susceptible wheat cultivar Shimai 15 (SM15), was used to fine-map the powdery mildew resistance gene Pm2b. A collection of 133 wheat cultivars/lines was used to validate the specific marker of Pm2b in different genetic backgrounds (Table S5). Susceptible wheat cultivar Heng 4399 (H4399) was included in this study for maintaining and increasing Bgt isolates, and it was also used as the susceptible control. All plants were inoculated with the Bgt isolate E09 to assess the powdery mildew reaction in the greenhouse as previously described (Jin et al., 2021). When the pustules were fully developed on the first leaf of H4399 at about 14 days post inoculation (dpi), infection types (ITs) for each plant were assessed based on a 0-4 scale, and plants with ITs 0-2 were regarded as resistant and those with ITs 3 and 4 as susceptible (Si et al., 1987).
Microscopic analyses were performed as previously described (Wang et al., 2014). The 2 cm leaf segments were cut at 14 dpi with inoculating Bgt isolate E09 and fixed at 37°C for 24 h in 2 ml of Carnoy’s Fluid (ethanol: acetic acid, 3:1, v/v), then stained with 2 mL of 0.6% (w/v) Coomassie blue solution for 3 min. Excess dye was rinsed off carefully with distilled water, then determined the percentage of microcolonies formed from the total number of germinated spores. Samples were observed under an Olympus BX-53 microscope (Olympus, Japan).
The resistant and susceptible DNA bulks, which consisted of 30 homozygous resistant and 30 homozygous F2:3 families of the cross KM2939/SM15, were used in DNA-based bulked segregant Analysis (BSA) to validate polymorphic markers (Michelmore et al., 1991). Bulked segregant RNA-Sequencing (BSR-Seq) was conducted by Biomarker Technologies (Beijing, China). Thirty resistant and susceptible F2:3 families, represented by equal amounts of leaf tissues from each single plant of each family, were respectively selected randomly to compose the resistant bulk and susceptible bulk for BSR-Seq.
Total genomic DNA was extracted using cetyltrimethylammonium bromide (CTAB) method as previously described (Sharp et al., 1988). To convert candidate SNPs or Indels linked to Pm2b to available markers, 3 kb flanking sequences for each putatively linked SNPs or Indels were extracted from the IWGSC reference v2.1, used as reference sequence for developing marker. The primers were designed with software Primer 5.0.
Chi-squared (χ2) tests were performed to evaluate goodness of fit of observed phenotyping data from the expected segregation ratios of the F2:3 generations. The linkage relationship of polymorphic markers and Pm2b was established using Software MAPMAKER/Exp (version 3.0b) with the Kosambi map function and the logarithm of odd (LOD) score threshold was set at 3.0 (Kosambi, 1943). Putative genes were annotated with the TriAnnot pipeline and confirmed using the BLAST analysis tools available at NCBI and EnsemblPlants.
Barley stripe mosaic virus-induced gene silencing (BSMV-VIGS) was used to verify the gene function of Pm2b and TaWRKY76-D. The non-conserved-domain fragments of Pm2b and TaWRKY76-D were amplified with the specific primers (Table S1) from the cDNA of KM2939, digested with EcoRI/SalI, and reversely inserted into BSMV: γ vector to generate the recombinant vectors BSMV: Pm2b and BSMV: TaWRKY76-D. Using the system established by He et al. (2018) with the following steps: at 7-12 days after virus inoculation, the plants were treated with Bgt isolate E09. About 14 dpi later, the third leaves with virus symptoms were collected for phenotypic identification, staining with Commassie blue for observation of fungal development and expression analysis with the quantitative real-time PCR (qRT-PCR) (Table S1). At least 10 leaves were analyzed in each experiment to evaluate the effect of gene silencing.
About 5,000 seeds of KM2939 were treated with 0.8% Ethylmethylsulfone (EMS). Then, these seeds were sown in the field to generate M1 plants. To screen susceptible mutants, 30-50 seeds of each M2 family were evaluated for the response to Bgt isolate E09. The susceptible M2 plants were transplanted to the field to produce M3 generation for progeny testing to confirm the mutations. The genomic DNA sequence of candidate gene in each powdery mildew susceptible mutant was obtained by PCR with specific primers (Table S1), and then compared for nucleotide variations with wild type by multiple sequence alignment.
Total RNA was extracted from wheat using TRIzol reagent (Invitrogen, USA). About 2 μg of RNA was used for reverse transcription with a FastQuant RT Kit (Tiangen, China). The qRT-PCR assays were performed using SYBR Premix Ex Taq (Takara, China) on the Bio-Rad CFX Connect real-time PCR system (BIO-RAD, USA). The relative expression of each gene was calculated as a fold change using the comparative CT method (Livak and Schmittgen, 2001). For each sample, three technical replications were analyzed. The TaActin was used as the internal control for normalization. Primers used in this study were listed in Table S1.
The full-length coding sequence (CDS) of Pm2b was amplified from KM2939 seedling cDNA sample and sub-cloned into the pCAMBIA1305-GFP vector (Han et al., 2020). For PM2b protein subcellular localization, Agrobacterium tumefaciens strain GV3101 harboring proper recombinant vectors constructs were infiltrated into N. benthamiana leaves. The fluorescence signal of green fluorescent protein (GFP) was observed at 48 h after infiltration under a Leica TCS SP5 Confocal Microscope (Leica, Germany). The CDS of TaWRKY76-D was amplified and sub-cloned into the pJIT163-GFP vector (Liu et al., 2020). For TaWRKY76-D protein, the recombinant plasmid was each introduced into wheat protoplasts using the polyethylene glycol (PEG)-mediated method as previously described (Liu et al., 2020). After incubation for 16 h at 22°C, GFP fluorescence was observed under the confocal microscope.
Protein isolation and western blotting were performed as previously described (Gao et al., 2020). In brief, 0.1 g of N. benthamiana leaves were collected at 48 hpi, then homogenized in 300 μl of plant extraction buffer (CWBIO, China). Proteins were separated on 10% SDS-PAGE gels followed by western blotting analysis using anti-GFP antibody (Abclonal, China).
Yeast two-hybrid (Y2H) assays were performed as previously described (Wu and Li, 2017). The indicated fragments were amplified and sub-cloned into the PXGY17, PXGY18, pGBKT7 or pGADT7 vectors to generate the recombinant vectors. After the co-transformation of pGBKT7-fragment-A and pGADT7-fragment-B plasmids into yeast AH109 cells, or the co-transformation of PXGY17-fragment-A and PXGY18-fragment-B plasmids into yeast NMY51 cells, the interaction between indicated proteins was determined by the growth of the co-transformants on a selection medium (SD/-Trp/-Leu/-His/-Ade). For Y2H screening, full-length CDS of Pm2b was fused to pGBKT7 vector for bait construction and the screening of a wheat cDNA library derived from Yangmai 158 with inoculating Bgt isolate E09.
The Luciferase complementation imaging (LCI) assays for the interaction between indicated proteins in N. benthamiana leaves were performed as described previously (Liu et al., 2017). The indicated fragments were fused with the N-terminal or C-terminal regions of the LUC reporter gene, respectively, and transformed into A. tumefaciens strain GV3101. Agrobacteria harboring the nLUC and cLUC derivative constructs were co-infiltrated into N. benthamiana, and the LUC activity was imaged and analyzed at 48 hpi using the NEWTON7.0 Bio. Plant Imaging System (Vilber Bio Imaging, France).
Wheat breeding line KM2939 was resistant with an IT 0 to Bgt isolate E09, while wheat cultivar SM15 was susceptible with IT 4. The 4,354 F2:3 families segregated as 1,107 homozygous resistant, 2,208 segregating, and 1,029 homozygous susceptible, which fitted a 1:2:1 ratio (χ2 = 3.99, df = 2, P = 0.14), indicating that Pm2b in KM2939 was a dominant powdery mildew resistance gene. To fine-map Pm2b, we first conducted the BSR-Seq analysis for KM2939, SM15, resistant and susceptible bulks. The 6.9 Gb, 9.0 Gb, 20.2 Gb and 23.1 Gb clean data were obtained from KM2939, SM15, resistant and susceptible bulks, respectively. The percentages of clean reads with a Q30 were 93.42%, 93.28%, 93.24% and 93.5%, respectively. The percentages of reads mapping to the reference genome were all more than 85%. These data indicated that the sequencing quality was high and suitable for subsequent analysis. A total of 1,198 candidate SNPs and InDels associated with the disease resistance were identified. The most abundant enrichment of the SNP loci was observed on chromosome 5D (Figure 1A). The density distribution of SNP loci showed a strong peak at the 42.41-46.86 Mb interval of the chromosome 5DS (Figure 1A), demonstrating a high probability of linkage with the resistance gene Pm2b in KM2939.
Figure 1 Map-based cloning of powdery mildew resistance gene Pm2b. (A) Distribution of polymorphic SNP loci between the resistant and susceptible bulks on 21 chromosomes and density of polymorphism SNP loci on chromosome 5D with 1 Mb intervals. (B) Linkage map of Pm2b. (C) Genomic structure of the candidate gene TraesCS5D03G0111700 in KM2939 and Shimai 15 (SM15). The green box represents the exon, the white box represents the intron, the gray box represents the deletion in SM15, the red box represents the seven missing bases and the number represents the position.
Based on sequence variations between resistant and susceptible bulks, three polymorphic markers XPm2b-map-1, XPm2b-map-2, and XPm2b-map-3 in the region were developed and showed consistent polymorphism in resistant and susceptible parents and DNA bulks. Combining with previously reported markers Xcfd81, Xswgi011, Xbwm20 and Xbwm25, these seven markers were used to validate in the 4,354 F2:3 families. Finally, Pm2b was flanked by Xcfd81 and Pm2b-map-2 with a physical region of 1.64 Mb, and marker Pm2b-map-3 was co-segregated with Pm2b (Figure 1B, Table S1). According to the fine-mapped interval and gene annotation of IWGSC_v2.1_HC_gene, there were 20 high confidence genes. However, only TraesCS5D03G0111700 existed variations in CDS between resistant parent KM2939 and susceptible parent SM15 (Figure 1C, Table S2). More importantly, TraesCS5D03G0111700 in SM15 contained a 7 bp deletion in the first exon, which formed a new termination site at the 422th triplet of the shifted reading frame (Figure 1C, Table S2). Therefore, TraesCS5D03G0111700 was the important candidate of Pm2b. And the sequence of TraesCS5D03G0111700 in resistant parent KM2939 was identical to Pm2a and PmCH1357 cloned previously.
To identify that TraesCS5D03G0111700 is essential to Pm2b resistance to powdery mildew, the silence effect of TraesCS5D03G0111700 in KM2939 was analyzed by BSMV-VIGS. The non-conserved-domain sequence, amplified by the primer Pm2b-VIGS, was designed as silencing target (Table S1). The qRT-PCR analysis showed that the expression level of TraesCS5D03G0111700 had a 66-95% decreasing in plants treated with BSMV: Pm2b, revealing a high efficiency of BSMV-VIGS system (Figure 2C). Fifteen of twenty plants which were treated with BSMV: Pm2b showed susceptible to Bgt isolate E09 with IT 4. However, no spore could be observed on leaves of empty vector BSMV: 00 (Figures 2A, B). The results indicated that silencing of TraesCS5D03G0111700 could compromise the resistance of KM2939.
Figure 2 Function verification of powdery mildew resistance gene Pm2b. Macroscopic phenotypes (A), microscopic phenotypes (B) and expression analysis of Pm2b (C) after silencing by barley stripe mosaic virus-induced gene silencing (BSMV-VIGS) of Pm2b in wheat line KM2939. Bar = 100 μm. Error bars represent SD of three independent experiments. Statistically significant differences (Student’s t-test): ns, not significant; **, P <0.01. (D) The analysis on two independent susceptible mutants of KM2939. The upper Figure indicated gene and protein structures of Pm2b, the middle Figure indicated the nucleotide and amino acid changes of Pm2b in two susceptible mutants M1 and M2, the below Figure indicated response of M1 and M2 to Blumeria graminis f. sp. tritici (Bgt) isolate E09.
To further verify the function of TraesCS5D03G0111700, we obtained two EMS independent susceptible mutants of KM2939 (Figure 2D). TraesCS5D03G0111700 was cloned from the two mutants to compare their differences with that of KM2939. Two SNPs were identified in the LRR domain of mutant M1, which caused Arg619Gln and Arg738Cys substitution. Two SNPs were identified in the LRR domain of mutant M2, which caused Cys1089Tyr and Val1165Met substitution (Figure 2D). In conclusion, TraesCS5D03G0111700 was the Pm2b using BSMV-VIGS and mutant analysis in KM2939.
The qRT-PCR was performed to identify the expression pattern of Pm2b in different tissues of KM2939 (Table S1). The transcript level of Pm2b was the highest in leaves, slightly lower in roots and substantially lower in stems, spikes and developing grains (Figure 3A). Next, we further used qRT-PCR to investigate the expression pattern of Pm2b in the KM2939 and SM15 leaves after inoculating with the Bgt isolate E09 at different time. As shown in Figure 3B, the transcript level of Pm2b was rapidly up-regulated and reached a peak at 4 h after inoculation which was about 10-fold compared with non-inoculated KM2939 at 0 h. However, the expression of Pm2b was declined and reversed to 0.51- to 0.14- fold from 8 to 12 h, compared with the inoculated KM2939 at 4 h. In contrast, the transcript level of Pm2b in SM15 did not change significantly after inoculation during 0-60 h, compared with KM2939 (Figure 3B).
Figure 3 Expression patterns of powdery mildew resistance gene Pm2b. (A) Tissue specific expression patterns of Pm2b in various KM2939 tissues. Seedling roots (SR), seedling stems (SS), seedling leaves (SL), roots at the elongation stage (RES), stems at the elongation stage (SES), leaves at the elongation stage (LES), roots at the heading stage (HR), stems at the heading stage (HS), leaves at the heading stage (HL), young spikes (YS), spikes at the heading stage (HSP) and grains (DG). (B) Expression pattern of Pm2b after inoculating with Blumeria graminis f. sp. tritici (Bgt) isolate E09 at 2, 4, 8, 12, 16, 20, 24, 26, 48 and 60 hours post inoculation (hpi). In B and C, normalized values of Pm2b expression relative to Actin were given as mean ± SD from three replicates. (C) Subcellular localization of Pm2b in Nicotiana benthamiana. Transient expression of GFP and Pm2b driven by CaMV 35S promoter in N. benthamiana. Bar = 10 μm. (D) Protein expression levels of Pm2b-R and Pm2b-S shown by western blotting. Total proteins were extracted from N. benthamiana leaves at 48 hpi and the tagged proteins were detected by western blotting using an anti-GFP antibody (α-GFP). Equal protein loading is shown by immunoblot detection of actin (α-Actin) throughout this article.
To identify the subcellular localization of PM2b, the CDS of Pm2b was fused with vector pCAMBIA1305-GFP and the recombinant plasmid was infiltrated into N. benthamiana leaves using Agrobacterium-mediated transient expression. As shown in Figure 3C, the GFP fluorescence patterns of the fused pCAMBIA1305-Pm2b-GFP proteins were distributed throughout the nucleus, cytoplasm and plasma membrane. Western blotting showed that Pm2b-S-GFP and Pm2b-R-GFP fusion proteins were properly expressed in N. benthamiana leaves (Figure 3D).
To identify whether the PM2b was self-associated, we conducted Y2H and LCI assays. Firstly, the CC, NB, LRR, CN, NL and PM2b domains were constructed into yeast vectors PXGY17 and PXGY18, respectively, to co-transformation yeast cells. These results suggested that PM2b could self-associate, NB domain self-associated and interacted with CN (Figure 4A). Then, using the LCI assays in N. benthamiana cells, it was found that the co-infiltration of Pm2b-nLUC and cLUC-Pm2b, NB-nLUC and cLUC-NB, Pm2b-NB and cLUC-CN resulted in luminescence generated by the complemented luciferase (Figure 4B). In conclusion, PM2b could self-associate through NB domain.
Figure 4 Interactions of different domains of PM2b. (A) The protein interaction of different domains of PM2b by yeast two-hybrid. Different domains of PM2b were fused to DNA binding domain (BD) and activation domain (AD) of GAL4, respectively. The yeast strains AH109 were serially diluted (100-10-3) before spotting on selection medium. SD/-Trp/-Leu, synthetic dextrose medium lacking Trp and Leu; SD/-Trp/-Leu/-His/-Ade, synthetic dextrose medium lacking Trp, Leu, His and Ade. (B) The protein interaction of different domains of PM2b by luciferase complementation imaging (LCI) assays. The N- or C-terminal fragment of LUC (nLUC or cLUC) was fused with the indicated proteins. The indicated constructs were co-expressed in Nicotiana benthamiana by agroinfiltration. Images of chemiluminescence were recorded by applying 0.5 mM luciferin 48 h after infiltration. Similar results were obtained in three biological replicates.
To identify additional components of Pm2b-mediated immune reaction, a cDNA library of Bgt-infected leaves was screened with the bait of PM2b. TaWRKY76-D was identified as a candidate interactor by PM2b bait (Table S3). Targeted Y2H analysis further confirmed that PM2b interacted with TaWRKY76-D, NB domain of PM2b interacted with TaWRKY76-D and PM2b interacted with WRKY domain of TaWRKY76-D, whereas PM2-SM15 did not interact with TaWRKY76-D (Figure 5A). We further performed a LCI assay in N. benthamiana cells to verify the interaction between PM2b and TaWRKY76-D. The co-infiltration of Pm2b-nLUC and cLUC-TaWRKY76-D, Pm2b-NB-nLUC and cLUC-TaWRKY76-D, Pm2b-nLUC and cLUC-WRKY-TaWRKY76-D resulted in luminescence generated by the complemented luciferase, which provided further evidence for interaction of PM2b and TaWRKY76-D in vivo (Figure 5B).
Figure 5 Protein-protein interactions between PM2b and TaWRKY76-D. (A) Yeast two-hybrid assay showed the interaction between PM2b and TaWRKY76-D. BD: GAL4 DNA binding domain; AD: GAL4 activation domain. The yeast strains AH109 were serially diluted (100-10-3) before spotting on selection medium. SD/-Trp/-Leu, synthetic dextrose medium lacking Trp and Leu; SD/-Trp/-Leu/-His/-Ade, synthetic dextrose medium lacking Trp, Leu, His and Ade. (B) Luciferase complementation imaging (LCI) assays showed the interaction between PM2b and TaWRKY76-D in Nicotiana benthamiana. nLUC, N-terminal part of LUC; cLUC, C-terminal part of LUC. The nLUC and cLUC derivative constructs were coexpressed in N. benthamiana by agroinfiltration. Images of chemiluminescence were recorded by applying 0.5 mM luciferin 48 h after infiltration. Similar results were obtained in three biological replicates.
To investigate the subcellular localization of TaWRKY76-D in wheat protoplasts, the CDS of the TaWRKY76-D was fused in-frame with the gene encoding GFP, and the resulting constructs were introduced into wheat protoplasts. As shown in Figure 6A, the GFP fluorescence pattern of the fused TaWRKY76-D protein was distributed on nucleus.
Figure 6 Subcellular localization and barley stripe mosaic virus-induced gene silencing (BSMV-VIGS) of TaWRKY76-D. (A) Subcellular localization of TaWRKY76-D protein in wheat protoplast. Transient expression of GFP and TaWRKY76-D driven by CaMV 35S promoter in wheat protoplast. Bar = 7.5 μm. (B–G) TaWRKY76-D negatively regulates wheat resistance to powdery mildew (Blumeria graminis f. sp. tritici, Bgt). Inoculated with Bgt isolate E09 at 14 days post inoculation, macroscopic (B), microscopic (C) phenotypes and micro-colony index (D) after silencing by barley stripe mosaic virus-induced gene silencing (BSMV-VIGS) of TaWRKY76-D in wheat cultivar Shimai 15 (SM15), macroscopic (E), microscopic phenotypes (F) and micro-colony index (G) after silencing by BSMV-VIGS of TaWRKY76-D in wheat line KM2939. Bar = 1 mm, Error bars represent SD of three independent experiments. Statistically significant differences (Student’s t-test): ns, not significant; **, P <0.01.
To identify the function of TaWRKY76-D in wheat immunity against powdery mildew, we conducted BSMV-VIGS to silence TaWRKY76-D in resistant parent KM2939 and susceptible parent SM15, respectively. The non-conserved-domain sequence of TaWRKY76-D was designed as silencing target (Table S1). The amounts of spores on the leaf of SM15 treated with BSMV: TaWRKY76-D reduced almost one half compared with that treated with BSMV: 00 (Figures 6B–D). No spore was observed on the leaves of KM2939 treated with BSMV: TaWRKY76-D and BSMV: 00 (Figures 6E–G). The TaWRKY76-D transcript levels were reduced by 66-86% and 78-85% in SM15 and KM2939 treated with BSMV: TaWRKY76-D, respectively (Figure S1). Thus, silencing of TaWRKY76-D increased powdery mildew resistance to the Bgt isolate E09.
To determine the variations of Pm2b locus, we cloned and sequenced the entire genomic region of Pm2 from 15 wheat cultivars/lines which were reported to carry Pm2 alleles, and seven susceptible wheat cultivars without any allele of Pm2 (Table S4). The results showed that the sequences of 15 documented Pm2 alleles were identical to Pm2b, whereas the sequences of seven susceptible cultivars were the same as Pm2-SM15 (Table S4). There were several non-synonymous SNPs between KM2939 and SM15 in the first exon of NLR (Table S2). According to these differential SNPs, a high-throughput and high-efficiency KASP (Kompetitive Allele Specific PCR) marker K529 based on the variation of c.556C>A was developed to detect the presence of Pm2b. Subsequently, 133 wheat cultivars/lines from the main wheat producing regions were tested with marker K529. K529 could consistently amplify specific genotypes differed from KM2939 in 105 out of 133 wheat accessions (Figure S2, Table S5). Therefore, comparing with the common PCR markers established previously, KASP marker K529 could be used in MAS with high throughput and efficiency for detecting Pm2b in those wheat genetic backgrounds.
Recent studies showed that 393 of 659 powdery mildew-resistant accessions carried Pm2, including 83 cultivars, 252 advanced breeding lines, three landraces and 33 introduced cultivars (Jin et al., 2021). Cloning and revealing the disease resistance mechanism of Pm2 could provide theoretical basis for its application in production. Pm2a and PmCH1357 were cloned by different means and their sequences were identical (Sánchez-Martín et al., 2016; Chen et al., 2019). But the further studies of Pm2 will be needed to verify its function and determine the disease resistance mechanism. In the current study, we cloned Pm2b from wheat breeding line KM2939 using map-based cloning combined with BSR-Seq analysis. Subsequently, the function of Pm2b was verified by BSMV-VIGS assay and mutant analysis in KM2939. Sequence alignment showed that the genomic sequence of Pm2b was completely identical with Pm2a/PmCH1357. In conclusion, the cloning and function verification of Pm2 further facilitated its application in wheat powdery mildew resistance breeding. In addition, in this study, the marker K529 was developed based on the SNP on the first exon of Pm2b between resistant and susceptible parents and was verified in 133 wheat cultivars/lines. The marker K529 was able to identify Pm2b in a high throughput and efficiency scale during the process of MAS.
Several studies have shown that R gene-mediated disease resistance against pathogens depended on the formation of heterologous or homologous polymers in plants (Jones et al., 2016). For instance, two different CC-NBS-LRR genes form a paired NB-LRR receptor, which is required for resistance to leaf rust resistance in tetraploid and hexaploid wheat (Loutre et al., 2009). In a recent example, the NB-LRR gene PigmR confers broad-spectrum resistance to rice blast in Magnaporthe oryzae, whereas the paired NB-LRR PigmS suppresses PigmR-mediated resistance by competitively affecting PigmR homodimerization to regulate resistance (Deng et al., 2017). In barley, MLA receptors self-associate in vivo, but self-association appears to be independent of effector-triggered receptor activation, further study showed dimeric CC module in downstream immune signaling (Maekawa et al., 2011). In the current study, we found that PM2b could form homologous dimer through NB and NB domain interaction. The results showed that Pm2 induced a strong hypersensitive cell death response upon recognition of AvrPm2 whereas no hypersensitive reaction was observed when Pm2 was co-expressed with the GUS control in N. benthamiana (Praz et al., 2017). It was speculated that Pm2b existed in the form of homologous polymers and maintained autoinhibition in the absence of pathogen effector, and Pm2b function might depend on directly or indirectly recognizing the specific effector AvrPm2 to activate the immunity.
Plant WRKY transcription factors play important roles in the regulation of transcriptional processes to modulate pathogen-triggered cellular responses (Tsuda and Somssich, 2015; Han et al., 2020). For instance, barley HvWRKY1 and HvWRKY2 were shown to interact with MLA which encoded a NLR receptor in the nucleus to derepress barley immunity and potentiate ETI responses (Shen et al., 2007). In Arabidopsis, the RPS4 and RRS1 proteins formed a paired NB-LRR receptor, which was required for resistance to bacterial and fungal pathogens. In this immune receptor complex, the WRKY domain of RRS1 served as a decoy to bind the pathogen effector and initiated resistance signaling mediated by RPS4 (Le Roux et al., 2015; Sarris et al., 2015). In our study, we identified a WRKY transcription factor TaWRKY76-D by Y2H, and PM2b could physically interact with TaWRKY76-D through NB domain and WRKY domain interaction. Gene silencing of TaWRKY76-D showed that it negatively regulated powdery mildew resistance in wheat. It was likely that Pm2b interacted with TaWRKY76-D to remove the inhibition of TaWRKY76-D on downstream genes. Previous study reported that PM2 could interact with its avirulent gene AVRPM2 directly or indirectly to induce strong hypersensitive reaction in N. benthamiana, when infected with Bgt isolates (Praz et al., 2017). We speculated that AVRPM2 enhanced the interaction between PM2 and TaWRKY76-D to strengthen the resistance to powdery mildew. Similarly, WRKY76-D could facilitate the recognition of PM2 and AVRPM2 through interacting with PM2 to initiate the disease resistance response. However, silencing TaWRKY76-D did not fully gain absolute resistance to powdery mildew. Therefore, TaWRKY76-D could be the important component in PM2b-mediated powdery mildew resistance pathway.
In conclusion, we cloned powdery mildew resistance gene Pm2b from resistant line KM2939. The function of Pm2b was verified by barley stripe mosaic virus-induced gene silencing technology and loss-of-function mutants. Pm2b encoded the coiled-coil nucleotide-binding site leucine-rich repeat protein. PM2b could self-associate through the NB domain. Notably, we identified PM2b interacting with the transcription factor TaWRKY76-D, which depended on the NB domain of PM2b and WRKY domain of TaWRKY76-D. TaWRKY76-D negatively regulated the resistance to powdery mildew. In addition, we developed a KASP marker K529 which could take the advantage of high-throughput and high-efficiency for detecting Pm2b and be useful in molecular marker assisted-selection breeding. This study could provide theoretical basis for wheat disease resistance breeding.
The original contributions presented in the study are included in the article/Supplementary Material. Further inquiries can be directed to the corresponding authors.
DA and LL conceived the project. YJ, HL, and DA designed the experiments. YJ, HL, TG, LX, GH, PM, XL, YZ, and JF performed experiments. YJ and HL analyzed data and wrote the manuscript. DA and LL edited the manuscript and supervised the project. All authors contributed to the article and approved the submitted version.
This research was supported by the National Key Research and Development Program of China (2021YFD1200600), the National Natural Science Foundation of China (32272105), and the Hebei Province Key Research and Development Program (22326306D).
The authors are grateful to Hailiang Mao from Huazhong Agricultural University of China for providing cDNA library of Yangmai 158.
The authors declare that the research was conducted in the absence of any commercial or financial relationships that could be construed as a potential conflict of interest.
The reviewer PY declared a shared affiliation with the authors XL, YZ, JF, LL to the handling editor at the time of the review.
All claims expressed in this article are solely those of the authors and do not necessarily represent those of their affiliated organizations, or those of the publisher, the editors and the reviewers. Any product that may be evaluated in this article, or claim that may be made by its manufacturer, is not guaranteed or endorsed by the publisher.
The Supplementary Material for this article can be found online at: https://www.frontiersin.org/articles/10.3389/fpls.2022.973065/full#supplementary-material
Supplementary Figure 1 | Relative expression of TaWRKY76 after silencing by barley stripe mosaic virus-induced gene silencing of TaWRKY76 in wheat cultivar Shimai 15 (A) and line KM2939 (B). Error bars represent SD of three independent experiments. Statistically significant differences (Student’s t-test): ns, not significant; **, P <0.01.
Supplementary Figure 2 | Partial genotyping results of marker K529 by Kompetitive Allele Specific PCR (KASP) assay in 133 wheat cultivar/lines. Orange circles represent the accessions that carry Pm2b (allele1/allele1), blue squares represent the accessions that did not carry Pm2b (allele2/allele2), and black diamonds represent the nontemplate control.
Bennett, F. G. A. (1984). Resistance to powdery mildew in wheat: a review of its use in agriculture and breeding programmes. Plant Pathol. 33, 279–300. doi: 10.1111/j.1365-3059.1984.tb01324.x
Bhullar, N. K., Street, K., Mackay, M., Yahiaoui, N., Keller, B. (2009). Unlocking wheat genetic resources for the molecular identification of previously undescribed functional alleles at the Pm3 resistance locus. P. Natl. Acad. Sci. 106, 9519–9524. doi: 10.1073/pnas.0904152106
Bliffeld, M., Mundy, J., Potrykus, I., Fütterer, J. (1999). Genetic engineering of wheat for increased resistance to powdery mildew disease. Theor. Appl. Genet. 98, 1079–1086. doi: 10.1007/s001220051170
Boller, T., Felix, G. (2009). A renaissance of elicitors: perception of microbeassociated molecular patterns and danger signals by pattern-recognition receptors. Annu. Rev. Plant Biol. 60, 379–406. doi: 10.1146/annurev.arplant.57.032905.105346
Boller, T., He, S. Y. (2009). Innate immunity in plants: An arms race between pattern recognition receptors in plants and effectors in microbial pathogens. Science 324, 742–744. doi: 10.1126/science.1171647
Bourras, S., Mcnally, K. E., Ben-David, R., Parlange, F., Roffler, S., Praz, C. R., et al. (2015). Multiple avirulence loci and allele-specific effector recognition control the Pm3 race-specific resistance of wheat to powdery mildew. Plant Cell 27, 2991–3012. doi: 10.1105/tpc.15.00171
Brunner, S., Hurni, S., Streckeisen, P., Mayr, G., Albrecht, M., Yahiaoui, N., et al. (2010). Intragenic allele pyramiding combines different specificities of wheat Pm3 resistance alleles. Plant J. 64, 433–445. doi: 10.1111/j.1365-313x.2010.04342.x
Chen, F., Jia, H. Y., Zhang, X. J., Qiao, L. Y., Li, X., Zheng, J., et al. (2019). Positional cloning of PmCH1357 reveals the origin and allelic variation of the Pm2 gene for powdery mildew resistance in wheat. Crop J. 7, 771–783. doi: 10.1016/j.cj.2019.08.004
Cui, H., Tsuda, K., Parker, J. E. (2015). Effector-triggered immunity: from pathogen perception to robust defense. Annu. Rev. Plant Biol. 66, 487–511. doi: 10.1146/annurev-arplant-050213-040012
Dangl, J. L., Horvath, D. M., Staskawicz, B. J. (2013). Pivoting the plant immune system from dissection to deployment. Science 341, 746–751. doi: 10.1126/science.1236011
Deng, Y. W., Zhai, K. R., Xie, Z., Yang, D. Y., Zhu, X. D., Liu, J. Z., et al. (2017). Epigenetic regulation of antagonistic receptors confers rice blast resistance with yield balance. Science 355, 962–965. doi: 10.1126/science.aai8898
Dodds, P. N., Rathjen, J. P. (2010). Plant immunity: towards an inte-grated view of plant-pathogen interactions. Nat. Rev. Genet. 11, 539–548. doi: 10.1038/nrg2812
Gao, A., Hu, M., Gong, Y., Dong, R., Jiang, Y., Zhu, S. (2020). Pm21 CC domain activity modulated by intramolecular interactions is implicated in cell death and disease resistance. Mol. Plant Pathol. 21, 975–984. doi: 10.1111/mpp.12943
Gao, H. Y., Niu, J. S., Li, S. P. (2018). Impacts of wheat powdery mildew on grain yield & quality and its prevention and control methods. Am. J. Agric. For. 6, 141–147. doi: 10.11648/j.ajaf.20180605.14
Han, X. Y., Zhang, L., Zhao, L. F., Xue, P. Y., Qi, T., Zhang, C. L., et al. (2020). SnRK1 phosphorylates and destabilizes WRKY3 to enhance barley immunity to powdery mildew. Plant Commun. 1, 100083. doi: 10.1016/j.xplc.2020.100083
He, H. G., Liu, R. K., Ma, P. T., Du, H. N., Zhang, H. H., Wu, Q. H., et al. (2020). Characterization of Pm68, a new powdery mildew resistance gene on chromosome 2BS of Greek durum wheat TRI 1796. Theor. Appl. Genet. 134, 53–62. doi: 10.1007/s00122-020-03681-2
He, H. G., Zhu, S. Y., Zhao, R. H., Jiang, Z. N., Ji, Y. Y., Ji, J., et al. (2018). Pm21, encoding a typical CC-NBS-LRR protein, confers broad-spectrum resistance to wheat powdery mildew disease. Mol. Plant 11, 879–882. doi: 10.1016/j.molp.2018.03.004
Inoue, H., Hayashi, N., Matsushita, A., Liu, X. Q., Nakayama, A., Sugano, S., et al. (2013). Blast resistance of CC-NB-LRR protein Pb1 is mediated by WRKY45 through protein-protein interaction. P. Natl. Acad. Sci. 110, 9577–9582. doi: 10.1073/pnas.1222155110
Jin, Y. L., Shi, F. Y., Liu, W. H., Fu, X. Y., Gu, T. T., Han, G. H., et al. (2021). Resistance identification and genes detection of powdery mildew and leaf rust in 2978 wheat accessions. Plant Dis. 105, 3900–3908. doi: 10.1094/pdis-03-21-0532-re
Jin, Y. L., Xu, H. X., Ma, P. T., Fu, X. Y., Song, L. P., Xu, Y. F., et al. (2018). Characterization of a new Pm2 allele associated with broad-spectrum powdery mildew resistance in wheat line subtil. Sci. Rep. 8, 475. doi: 10.1038/s41598-017-18827-4
Jones, J. D. G., Dangl, J. L. (2006). The plant immune system. Nature 444, 323–329. doi: 10.1038/nature05286
Jones, J. D. G., Vance, R. E., Dangl, J. L. (2016). Intracellular innate immune surveillance devices in plants and animals. Science 354, aaf63. doi: 10.1126/science.aaf6395
Kanzaki, H., Yoshida, K., Saitoh, H., Fujisaki, K., Hirabuchi, A., Alaux, L., et al. (2012). Arms race co-evolution of Magnaporthe oryzae AVR-pik and rice Pik genes driven by their physical interactions. Plant J. 72, 894–907. doi: 10.1111/j.1365-313X.2012.05110.x
Koller, T., Brunner, S., Herren, G., Hurni, S., Keller, B. (2018). Pyramiding of transgenic Pm3 alleles in wheat results in improved powdery mildew resistance in the field. Theor. Appl. Genet. 131, 861–871. doi: 10.1007/s00122-017-3043-9
Kosambi, D. D. (1943). The estimation of map distances from recombination values. Ann. Hum. Genet. 12, 172. doi: 10.1111/j.1469-1809.1943.tb02321.x
Le Roux, C., Huet, G., Jauneau, A., Cambor De, L., Trémousaygue, D., Kraut, A., et al. (2015). A receptor pair with an integrated decoy converts pathogen disabling of transcription factors to immunity. Cell 161, 1074–1088. doi: 10.1016/j.cell.2015.04.025
Liu, J., Cheng, X., Liu, P., Sun, J. (2017). miR156-targeted SBP-box transcription factors interact with DWARF53 to regulate TEOSINTE BRANCHED1 and BARREN STALK1 expression in bread wheat. Plant Physiol. 174, 1931–1948. doi: 10.1104/pp.17.00445
Liu, H., Li, H. F., Hao, C. Y., Wang, K., Wang, Y. M., Qin, L., et al. (2020). TaDA1, a conserved negative regulator of kernel size, has an additive effect with TaGW2 in common wheat (Triticum aestivum l.). Plant Biotechnol. J. 18, 1330–1342. doi: 10.1111/pbi.13298
Livak, K. J., Schmittgen, T. D. (2001). Analysis of relative gene expression data using real-time quantitative PCR and the 2-△△CT method. Methods 25, 402–408. doi: 10.1006/meth.2001.1262
Loutre, C., Wicker, T., Travella, S., Galli, P., Scofield, S., Fahima, T., et al. (2009). Two different CC-NBS-LRR genes are required for Lr10-mediated leaf rust resistance in tetraploid and hexaploid wheat. Plant J. 60, 1043–1054. doi: 10.1111/j.1365-313X.2009.04024.x
Maekawa, T., Cheng, W., Spiridon, L. N., Töller, A., Lukasik, E., Saijo, Y., et al. (2011). Coiled-coil domain-dependent homodimerization of intracellular barley immune receptors defines a minimal functional module for triggering cell death. Cell Host Microbe 9, 187–199. doi: 10.1016/j.chom.2011.02.008
Ma, P. T., Xu, H. X., Xu, Y. F., Li, L. H., Qie, Y. M., Luo, Q. L., et al. (2015). Molecular mapping of a new powdery mildew resistance gene Pm2b in Chinese breeding line KM2939. Theor. Appl. Genet. 128, 613–622. doi: 10.1007/s00122-015-2457-5
McIntosh, R. A., Dubcovsky, J., Rogers, W. J., Xia, X. C., Raupp, W. J. (2019). “Catalogue of 505 gene symbols for wheat: 2019 supplement,” in Annual wheat 506. Ed. Raupp, W. J. (Manhattan, USA: Newsletter), p98–113.
Michelmore, R. W., Paran, I., Kesseli, R. V. (1991). Identification of markers linked to disease-resistance genes by bulked segregant analysis: a rapid method to detect markers in specific genomic regions by using segregating populations. Proc. Natl. Acad. Sci. U.S.A. 88, 9828–9832. doi: 10.1073/pnas.88.21.9828
Pandey, S. P., Somssich, I. E. (2009). The role of WRKY transcription factors in plant immunity. Plant Physiol. 150, 1648–1655. doi: 10.1104/pp.109.138990
Praz, C. R., Bourras, S., Zeng, F., Sanchez-Martin, J., Menardo, F., Xue, M. F., et al. (2017). AvrPm2 encodes an RNase-like avirulence effector which is conserved in the two different specialized forms of wheat and rye powdery mildew fungus. New Phytol. 213, 1301–1314. doi: 10.1111/nph.14372
Qie, Y. M., Sheng, Y., Xu, H. X., Jin, Y. L., Ma, F. F., Li, L. H., et al. (2019). Identification of a new powdery mildew resistance gene pmDHT at or closely linked to the Pm5 locus in the Chinese wheat landrace dahongtou. Plant Dis. 103, 2645–2651. doi: 10.1094/pdis-02-19-0401-re
Rairdan, G. J., Collier, S. M., Sacco, M. A., Baldwin, T. T., Boettrich, T., Moffett, P. (2008). The coiled-coil and nucleotide binding domains of the potato rx disease resistance protein function in pathogen recog-nition and signaling. Plant Cell 20, 739–751. doi: 10.1105/tpc.107.056036
Reynolds, M., Foulkes, J., Furbank, R., Griffiths, S., King, J., Murchie, E., et al. (2012). Achieving yield gains in wheat. Plant Cell Environ. 35, 1799–1823. doi: 10.1111/j.1365-3040.2012.02588.x
Rose, L. E., Bittner-Eddy, P. D., Langley, C. H., Holub, E. B., Michelmore, R. W., Beynon, J. L. (2004). The maintenance of extreme amino acid diversityat the disease resistance gene, RPP13, in Arabidopsis thaliana. Genetics 166, 1517–1527. doi: 10.1534/genetics.166.3.1517
Sánchez-Martín, J., Steuernagel, B., Ghosh, S., Herren, G., Hurni, S., Adamski, N., et al. (2016). Rapid gene isolation in barley and wheat by mutant chromosome sequencing. Genome Biol. 17, 221. doi: 10.1186/s13059-016-1082-1
Sarris, P. F., Duxbury, Z., Huh, S. U., Ma, Y., Segonzac, C., Sklenar, J., et al. (2015). A plant immune receptor detects pathogen effectors that target WRKY transcription factors. Cell 161, 1089–1100. doi: 10.1016/j.cell.2015.04.024
Savary, S., Willocquet, L., Pethybridge, S. J., Esker, P., McRoberts, N., Nelson, A. (2019). The global burden of pathogens and pests on major food crops. Nat. Ecol. Evol. 3, 430–439. doi: 10.1038/s41559-018-0793-y
Sharp, P. J., Kreis, M., Shewry, P. R., Gale, M. D. (1988). Location of β-amylase sequences in wheat and its relatives. Theor. Appl. Genet. 75, 286–290. doi: 10.1007/bf00303966
Shen, Q. H., Saijo, Y., Mauch, S., Biskup, C., Bieri, S., Keller, B., et al. (2007). Nuclear activity of MLA immune receptors links isolate-specific and basal disease-resistance responses. Science 315, 1098–1103. doi: 10.1126/science.1136372
Si, Q. M., Zhang, X. M., Duan, X. Y., Sheng, B. X. (1987). Identification of isolates of Blumeria graminis f. sp. tritici. Sci. Agric. Sin. 20, 64–70. doi: 10.1146/annurev-phyto-080615-095835
Singh, R. P., Singh, P. K., Rutkoski, J., Hodson, D. P., He, X. Y., Jørgensen, L. N., et al. (2016). Disease impact on wheat yield potential and prospects of genetic control. Annu. Rev. Phytopathol. 54, 303–322. doi: 10.1146/annurev-phyto-080615-095835
Sun, H. G., Hu, J. H., Song, W., Qiu, D., Cui, L., Wu, P. P., et al. (2018). Pm61: A recessive gene for resistance to powdery mildew in wheat landrace xuxusanyuehuang identified by comparative genomics analysis. Theor. Appl. Genet. 131, 2085–2097. doi: 10.1007/s00122-018-3135-1
Tasset, C., Bernoux, M., Jauneau, A., Pouzet, C., Brière, C., Kieffer-Jacquinod, S., et al. (2022). Correction: Autoacetylation of the Ralstonia solanacearum effector PopP2 targets a lysine residue essential for RRS1-r-mediated immunity in Arabidopsis. PLoS Pathog. 18, e1010368. doi: 10.1371/journal.ppat.1001202
Tsuda, K., Somssich, I. E. (2015). Transcriptional networks in plant immunity. New Phytol. 206, 932–947. doi: 10.1111/nph.13286
Wang, Y., Cheng, X., Shan, Q., Zhang, Y., Liu, J. X., Gao, C. X., et al. (2014). Simultaneous editing of three homoeoalleles in hexaploid bread wheat confers heritable resistance to powdery mildew. Nat. Biotechnol. 32, 947–951. doi: 10.1038/nbt.2969
Wang, G. F., Ji, J., El-Kasmi, F., Dangl, J. L., Johal, G., Balint-Kurti, P. J. (2015). Molecular and functional analyses of a maize autoactive NB-LRR protein identify precise structural requirements for activity. PLoS Pathog. 11, e1004674. doi: 10.1371/journal.ppat.1004674
Wu, X. Y., Li, T. (2017). A casein kinase II phosphorylation site in AtYY1 affects its activity, stability, and function in the ABA response. Front. Plant Sci. 8. doi: 10.3389/fpls.2017.00323
Zhang, X., Dodds, P. N., Bernoux, M. (2017). What do we know about NOD-like receptors in plant immunity? Annu. Rev. Phytopathol. 55, 205–229. doi: 10.1146/annurev-phyto-080516-035250
Keywords: Pm2b, TaWRKY76-D, wheat, MAS, powdery mildew
Citation: Jin Y, Liu H, Gu T, Xing L, Han G, Ma P, Li X, Zhou Y, Fan J, Li L and An D (2022) PM2b, a CC-NBS-LRR protein, interacts with TaWRKY76-D to regulate powdery mildew resistance in common wheat. Front. Plant Sci. 13:973065. doi: 10.3389/fpls.2022.973065
Received: 19 June 2022; Accepted: 12 October 2022;
Published: 26 October 2022.
Edited by:
Daolong Dou, Nanjing Agricultural University, ChinaReviewed by:
Ping Yang, Institute of Crop Sciences, Chinese Academy of Agricultural Sciences, ChinaCopyright © 2022 Jin, Liu, Gu, Xing, Han, Ma, Li, Zhou, Fan, Li and An. This is an open-access article distributed under the terms of the Creative Commons Attribution License (CC BY). The use, distribution or reproduction in other forums is permitted, provided the original author(s) and the copyright owner(s) are credited and that the original publication in this journal is cited, in accordance with accepted academic practice. No use, distribution or reproduction is permitted which does not comply with these terms.
*Correspondence: Lihui Li, bGlsaWh1aUBjYWFzLmNu; Diaoguo An, ZGdhbkBzanppYW0uYWMuY24=
†These authors have contributed equally to this work
Disclaimer: All claims expressed in this article are solely those of the authors and do not necessarily represent those of their affiliated organizations, or those of the publisher, the editors and the reviewers. Any product that may be evaluated in this article or claim that may be made by its manufacturer is not guaranteed or endorsed by the publisher.
Research integrity at Frontiers
Learn more about the work of our research integrity team to safeguard the quality of each article we publish.