- 1Beijing Key Laboratory of Farmland Soil Pollution Prevention and Remediation, Key Laboratory of Plant-Soil Interactions of the Ministry of Education, China Agricultural University, Beijing, China
- 2Innovation Team of Remediation of Heavy Metal-Contaminated Farmlands, Agro-Environmental Protection Institute, Ministry of Agriculture and Rural Affairs, Tianjin, China
Selenium (Se) is an essential trace element for human and animal health, and toward an understanding of the uptake and translocation of Se in plants is important from the perspective of Se biofortification. In this study, we conducted hydroponic experiments to investigate the mechanisms of organic Se [selenomethionine (SeMet) and selenomethionine-oxide (SeOMet)] uptake, translocation, and the interactions between SeMet and SeOMet in rice. We also investigated differences in the dynamics of organic and inorganic Se uptake by rice roots. Concentration-dependent kinetic results revealed that SeMet uptake during a 1 h exposure was 3.19–16.0 times higher than that of three other Se chemical forms, with uptake capacity (Vmax) values ordered as follows: SeMet>SeOMet>selenite>selenate. Furthermore, time-dependent kinetic analysis revealed that SeMet uptake by roots and content in shoots were initially clearly higher than those of SeOMet, although the differences gradually diminished with prolonged exposure time; while no significant difference was found in the transfer factor of Se from rice roots to shoots between SeMet and SeOMet. Root uptake of SeOMet was significantly inhibited by carbonyl cyanide 3-chlorophenylhydrazone (CCCP) (30.4%), AgNO3 (41.8%), and tetraethylammonium chloride (TEACl) (45.6%), indicating that SeOMet uptake is a metabolically active process, and that it could be mediated via aquaporins and K+ channels. Contrarily, SeMet uptake was insensitive to CCCP, although markedly inhibited by AgNO3 (93.1%), indicating that rice absorbs SeMet primarily via aquaporins. Furthermore, Se uptake and translocation in rice treated simultaneously with both SeMet and SeOMet were considerably lower than those in rice treated with SeMet treatment alone and notably lower than the theoretical quantity, indicating interactions between SeMet and SeOMet. Our findings provide important insights into the mechanisms underlying the uptake and translocation of organic Se within plants.
Introduction
Selenium (Se), an essential micronutrient with respect to human and animal health, is a necessary component of more than 30 Se-containing proteins and enzymes in mammals and is associated with multiple properties, including antioxidative, immunological, and anticarcinogenic effects (Rayman, 2012; Avery and Hoffmann, 2018). Indeed, a deficiency in Se can lead to the risk of cardiovascular and cancer diseases (Hatfield et al., 2014). Although the World Health Organization (WHO) recommends a daily Se intake of 50–200 μg d–1 for adults, given marked differences in the soil contents of Se worldwide and the distribution of Se-poor soils in some notably populous regions, Se deficiency may afflict as many as one billion people globally (Combs, 2001; Haug et al., 2007).
Selenium has multiple beneficial effects on the plant growth at appropriate concentration, including the enhancement of antioxidant capacity and photosynthesis (Jiang et al., 2021; Lanza et al., 2021a); while excessive Se can be detrimental (Lanza et al., 2021b). Concerning its beneficial effects on plant growth and human health, plants can be strategically utilized to regulate the effects of Se on both. Consumption of Se-rich plant food is considered to be the most effective approach to increase human Se uptake (White and Broadley, 2009; Alfthan et al., 2015), and accordingly, in those regions characterized by Se-deficient soils, agronomic biofortification based on Se fertilization could be practiced to produce Se-rich crops, thereby enhancing human Se intake.
Among different factors influencing the accumulation of Se in plants, the uptake and translocation of this element are the most fundamental physiological aspects. Consequently, gaining an in-depth understanding of the associated processes and mechanisms is important from the perspective of developing Se biofortification strategies. In natural environment, a range of distinct chemical forms of Se exists, and among which, selenite (SeIV) and selenate (SeVI) are the two most abundant forms in soil, with selenite predominating in soils characterized by intermediate redox potentials and selenate predominating under aerobic and neutral to alkaline conditions (Elrashidi et al., 1987). Although both selenate and selenite can be absorbed from soil by plant roots, it appears that neither is taken up via Se-specific transporters. Selenate is typically taken up via sulfate transporters (Sors et al., 2005; Mehdawi et al., 2018), and selenite might be absorbed via silicon influx transporters (Zhao et al., 2010) or incorporated into an active process mediated by phosphate transporters (Li et al., 2008; Zhang et al., 2014). Upon uptake, most selenite is rapidly metabolized to organic Se compounds and retained within the root system, whereas selenate can be rapidly translocated to the shoots (Li et al., 2008; Huang et al., 2017; Gong et al., 2018; Yu et al., 2019). In some soils, Se is also present as organic forms, such as SeMet and SeCys (Kikkert and Berkelaar, 2013); however, compared with those of the inorganic forms of Se, the uptake mechanisms of organic forms of Se is less investigated.
Rice (Oryza sativa L.) is a staple food for nearly half of the world’s population and one of major sources of the dietary Se intake (Rayman et al., 2008). In this regard, in addition to the total concentration of Se in crops, Se speciation is of particular importance in terms of its different health benefits (Zhu et al., 2009). Research has shown that in the mature grains of rice, Se is present primarily in organic forms, and among which, selenomethionine (SeMet) predominates (Sun et al., 2010; Huang et al., 2018). SeMet can be incorporated into proteins either directly or non-specifically via the replacement of methionine and is thereby readily absorbed by humans (Fairweather-Tait et al., 2010). Moreover, the uptake rate of SeMet by plants is higher than that of either selenite or selenate (Kowalska et al., 2020; Wang M. K. et al., 2020). Selenomethionine-oxide (SeOMet) is a derivative obtained from the transformation of SeMet. In our previous study, we detected SeOMet in the soil solution extracted from natural and selenite-supplied soils (Li et al., 2010), and SeOMet was also found in plants growing in media supplemented with either selenite or SeMet (Li et al., 2008; Kowalska et al., 2020). However, the uptake of SeOMet in plant roots remain unclear, and differences between organic and inorganic Se uptake are insufficiently well documented. Since SeMet and SeOMet exist in the forms of uncharged molecules, we speculate that the uptake of these two Se forms might be mediated by aquaporins, with high uptake potential in plant roots. In addition, when supplied with different chemical forms of Se simultaneously, a non-additive effect on the uptake would occur, for example, the presence of selenite appeared to inhibit the uptake of selenate in plants (Li et al., 2008; Wang et al., 2019). Thus we speculate a certain interaction might occur between SeMet and SeOMet during uptake process. To gain further insights in these regards, we conducted a series of hydroponic experiments to investigate (1) differences between the dynamics of organic and inorganic Se uptake by rice roots, (2) the mechanisms associated with the uptake of SeMet and SeOMet by roots and their subsequent translocation in plant, and (3) the interactions between SeMet and SeOMet during the uptake and translocation processes. We anticipated that the findings of this study would provide a theoretical basis for increasing Se levels and accumulating more organic Se in crops.
Materials and methods
Plant culture
For the purposes of this study, we used the rice (Oryza sativa L.) cultivar Zhuliangyou120 (a common indica-type cultivar). Rice seeds were surface sterilized with 30% (v/v) H2O2 for 15 min, rinsed thoroughly with deionized water, soaked in saturated CaSO4 solution in the dark overnight, and then germinated in a 0.5 mM CaCl2 solution. Seven days after germination, rice plants were transplanted into plastic pots containing 3 L of modified ½ Kimura nutrient solution (Huang et al., 2015), with the following composition (mmol L–1): KNO3 0.091, KH2PO4 0.1, Ca (NO3)2⋅4H2O 0.183, MgSO4⋅7H2O 0.274, (NH4)2SO4 0.183, Fe (e1-EDTA 6.0 × 10–2, ZnSO4⋅7H2O 1.0 × 10–3, H3BO3 3.0 × 10–3, (NH4)6Mo7O24⋅4H2O 1.0 × 10–3, MnSO4⋅H2O 1.0 × 10–3 and CuSO4⋅5H2O 2.0 × 10–4. The pH of the solution was buffered with 2 mM 2-morpholinoethanesulfonic acid (MES) and adjusted to a value of 5.5 with either 1 mM KOH or HCl. The solution was renewed at 3-day intervals.
Hydroponic experiments were conducted on a bench within a greenhouse of the China Agricultural University, Beijing. The conditions of the growth environment was as follows: day/night temperatures of 30 ± 2°C/23 ± 2°C; a light period of 14 h, with illumination provided by natural sunlight supplemented with sodium vapor lamps to maintain a light intensity of 240–350 μmol m–2 s–1; and a relative humidity of 60–70%.
Selenium sources
Selenite and selenate were obtained from Sigma-Aldrich (St Louis, MO, United States), SeMet was provided by Shanxi University, and SeOMet was prepared by reacting SeMet with 3% H2O2 under sonication for 1 h (Larsen et al., 2004).
Concentration-dependent kinetics of Se uptake
To evaluate the uptake capacities of rice roots with respect to organic and inorganic Se, we transferred 4-week-old rice plants to 250 mL of Se uptake solutions, each containing different chemical forms of Se, namely, selenite, selenate, SeMet, and SeOMet. For each of the different uptake solutions, a series of Se treatment solution were prepared with the Se concentration ranging from 0 to 20 μM (0, 0.1, 0.5, 1, 5, 10, and 20 μM at pH 5.5). Each treatment had four replicates with one plant per replicate. After 1 h of uptake incubation, rice roots were rinsed three times with deionized water and then transferred to 150-mL of ice-cold desorption solution (1 mM CaSO4 + 2 mM MES, pH 5.5) for 15 min to remove the Se adsorbed on root surfaces (Li et al., 2008). Following desorption, the rice roots were rinsed three times in deionized water and thereafter separated from the shoots. The root samples were oven-dried at 105°C for 30 min and 75n for 48 h, after which, they were weighed and used for determinations of Se concentrations.
Time-dependent kinetics of selenomethionine and selenomethionine-oxide uptake
This experiment was conducted to investigate the temporal patterns of organic Se uptake and translocation by rice. Four-week-old rice plants were transferred to 1-L plastic container (one plant per pot) containing uptake solutions [with normal nutrients (control) and supplemented with either 5 μM SeMet or 5 μM SeOMet (2 mM MES, pH 5.5)], to which they were exposed for 1, 3, 5, 18, 26, 48, or 72 h, a control treatment (without any Se) was also conducted. Each treatment had four replicates. Following organic Se absorption, the roots were rinsed with deionized water and desorbed as described previously. The roots and shoots were then oven-dried and analyzed for Se concentrations.
Effects of inhibitors on the uptake of selenomethionine and selenomethionine-oxide
To investigate the physiological processes and mechanisms of organic Se uptake, we examined the effects of the following inhibitors on the uptake of Se by rice: AgNO3, CoCl2, tetraethylammonium chloride (TEACl), 4,4-diisothiocyanatostilbene-2,2-disulfonic acid disodium salt hydrate (DIDS), and carbonyl cyanide 3-chlorophenylhydrazone (CCCP). AgNO3 is an aquaporin inhibitor that inhibits the water permeability of root cell plasma membranes (Niemietz and Tyerman, 2002), whereas CoCl2, TEACl, and DIDS act as inhibitors of Ca2+ (Harada and Shimazaki, 2009), K+ (White, 1995), and anion channels (Zhang et al., 2013), respectively, and the protonophore CCCP is a metabolic inhibitor. All inhibitors used in the study were obtained from Sigma-Aldrich (St Louis, MO, United States).
Four-week-old plants were transferred to uptake solutions containing 5 μM organic Se (SeMet or SeOMet) and different inhibitors (100 μM AgNO3, 5 mM TEACl, 5 mM CoCl2, 100 μM DIDS, or 1 μM CCCP, respectively), a control treatment (without any Se) was also conducted. CCCP was dissolved in ethanol and added to the solution at a final concentration of 0.01% (v/v) (Li et al., 2008). Consequently, we also included an additional control treatment containing 0.01% (v/v) ethanol. Four replicates were used for each treatment. After exposure for 1 h, the treated roots were rinsed with deionized water and the Se adsorbed on root surfaces was desorbed as described previously. Thereafter, the roots were oven-dried and analyzed for Se concentrations.
Effects of P or S starvation on selenomethionine and selenomethionine-oxide uptake and translocation
This experiment was conducted to investigate whether phosphorus (P) or sulfur (S) starvation would influence the uptake and translocation of organic Se by rice. Four-week-old plants were transferred to 1-L plastic containers and treated with normal, P-deficient, or S-deficient nutrient solutions for 7 days. In the P-deficient and S-deficient solution, MgSO4, ZnSO4, KH2PO4, or CuSO4 were replaced by the corresponding chloride salts. At the end of the treatment period, the plants were transferred to a normal nutrient solution (modified ½ Kimura nutrient solution), to which either 5 μM SeMet or 5 μM SeOMet was added, followed by incubation for a further 2 days, a control treatment (without any Se) was also conducted. Then, the roots were desorbed, rinsed, oven-dried, and analyzed for Se concentrations. Each treatment had four replicates.
Interactions between selenomethionine and selenomethionine-oxide
In this experiment, we sought to characterize the interactions between SeMet and SeOMet during their uptake and translocation in rice plants. Four-week-old plants were transferred to 250-mL containers, containing one of three different absorption solutions with the same total Se concentration (5 μM SeMet, 5 μM SeOMet, or 2.5 μM of both SeMet and SeOMet), a control treatment (without any Se) was also conducted. Each treatment had four replicates. After exposure for 1 h, the rice roots were rinsed three times with deionized water and desorbed as described previously. Thereafter, the roots and shoots were harvested, washed, oven-dried, and analyzed for Se concentrations.
Analysis of Se content
For Se content analyses, 0.2500 g dried root and shoot samples were digested with 8 mL HNO3 (Guaranteed reagent) using a CEM MARS5 microwave sample preparation system (CEM Corp., Matthews, NC, United States). A 4 mL volume of the digest solution was then mixed with 1 mL of 6 M HCl and heated at 95 for 2 h to reduce selenate to selenite. Concentrations of Se in the mixed solution were determined by atomic fluorescence spectrometry using an AFS-920 Dual-channel Atomic Fluorescence Spectrometer, (Beijing Jitian Instruments Co., Ltd., Beijing, China). For quality assurance, we simultaneously analyzed a certified reference material (GBW10014, cabbage) and blanks, the recovery of Se in GBW10014 was 85–110%.
Data analysis
Se uptake kinetics were described based on the Michaelis–Menten Equation:
where V represents the uptake rate [μg g–1 root DW (dry weight) h–1], Km represents the Michaelis constant (μM), Vmax represents the maximal uptake rate (μg g–1 root DW h–1), and C represents the substrate concentration (μM). The Michaelis–Menten equation is of particular value with respect to the evaluation of transporter-mediated uptake processes. Uptake capacity (Vmax) is the maximal transport rate when all available carrier sites are saturated, whereas substrate affinity (Km) is equal to the substrate concentration at which the reaction rate is half-maximal.
All results are expressed as mean values with corresponding standard errors (n = 4). Two-way analysis of variance (ANOVA) was performed to test the significance of the Se form, treatment time (or inhibitor type, nutrient status) and the interactions between them, by using SAS 9.3 statistical software with least significant difference (LSD, P < 0.05).
Results
Concentration-dependent kinetics of Se uptake
We found that uptake of the four assessed Se chemical forms by rice roots increased concomitant with an increase in the Se concentration of the uptake solution, all of which were satisfactorily described by the Michaelis–Menten equation (Figure 1 and Table 1). With the exception of SeMet, the influx of the remaining three chemical forms of Se into roots had features of saturating kinetics within the concentration range from 0 to 20 μM (Figure 1). The Michaelis–Menten kinetics curves also showed that, in the uptake solution containing 5–20 μM Se, the rate of SeMet uptake was 3.19–16.0 times higher than that of the other three Se chemical forms. In addition, we established that the chemical forms of Se had a marked effect (P < 0.05) on Vmax and Km values (Table 1). The calculated values of Vmax declined in the order of SeMet > SeOMet > selenite > selenate, thereby indicating that organic forms of Se, particularly SeMet, are characterized by a considerably higher uptake potential in rice roots than inorganic forms. Moreover, we found that the Km values calculated for selenate, SeMet, and SeOMet uptake were 2.42-, 2.64-, and 4.18-fold higher than those of selenite, respectively, indicating that rice roots have a higher affinity for selenite than for the other assessed Se chemical forms. Interestingly, we observed that the absorption kinetics of selenite and SeOMet in rice differed according to exogenous Se concentrations. Specifically, at Se concentrations between 0 and 10 μM, the rate of selenite uptake was higher than that of SeOMet (P > 0.05), whereas the opposite response (selenite < SeOMet) was detected at higher Se concentrations ranging from 10 to 20 μM (P > 0.05), and the two corresponding Michaelis–Menten curves had a single point of intersection at 10 μM (Figure 1).
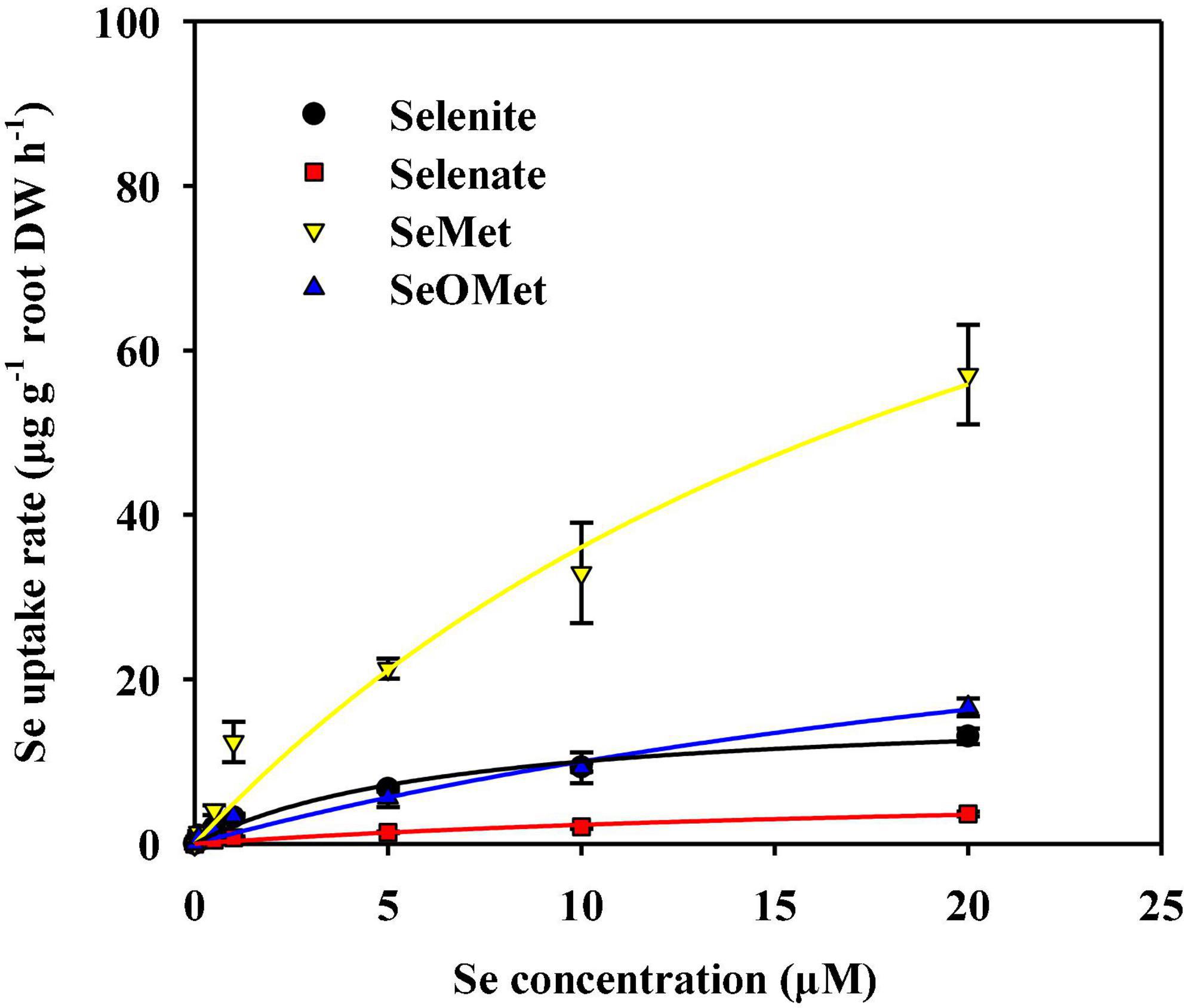
Figure 1. Concentration-dependent kinetics for the influx of different forms of Se into rice roots within 1 h. Data are presented as mean ± SE (n = 4). The curves represent the fitted Michaelis–Menten kinetics.
Time-dependent kinetics of organic Se uptake and translocation
Our time-dependent analysis of organic Se uptake revealed it was significantly affected by Se form, treatment time, and their interaction between these two factors (P < 0.001). And the Se in rice plants in the control treatment was below the detection level (the same below). Generally, the uptake rate of Se as SeMet was higher than that of the SeOMet form at all assessed time points, although the differences narrowed with time. For example, although SeMet uptake rate after exposure for up to 26 h was 1.56–7.19-fold higher than that of SeOMet, however, after exposure for 48 h, the uptake rate of Se as SeMet was only 9.60–32.8% higher (Figure 2A). Furthermore, irrespective of the chemical forms, the uptake rate of Se increased with a prolongation of treatment time, although the increase in SeMet uptake rate declined with time and that of SeOMet remained relatively constant. In neither case, however, did the rate of Se uptake reach a plateau during the assessed treatment period. A similar tendency was obtained with respect to the total Se uptake: within 26 h, the total Se in SeMet treatments was 1.89–7.09-fold higher than that of SeOMet treatments; while the difference was only 8.82–19.8% after 48 h (Figure 2B).
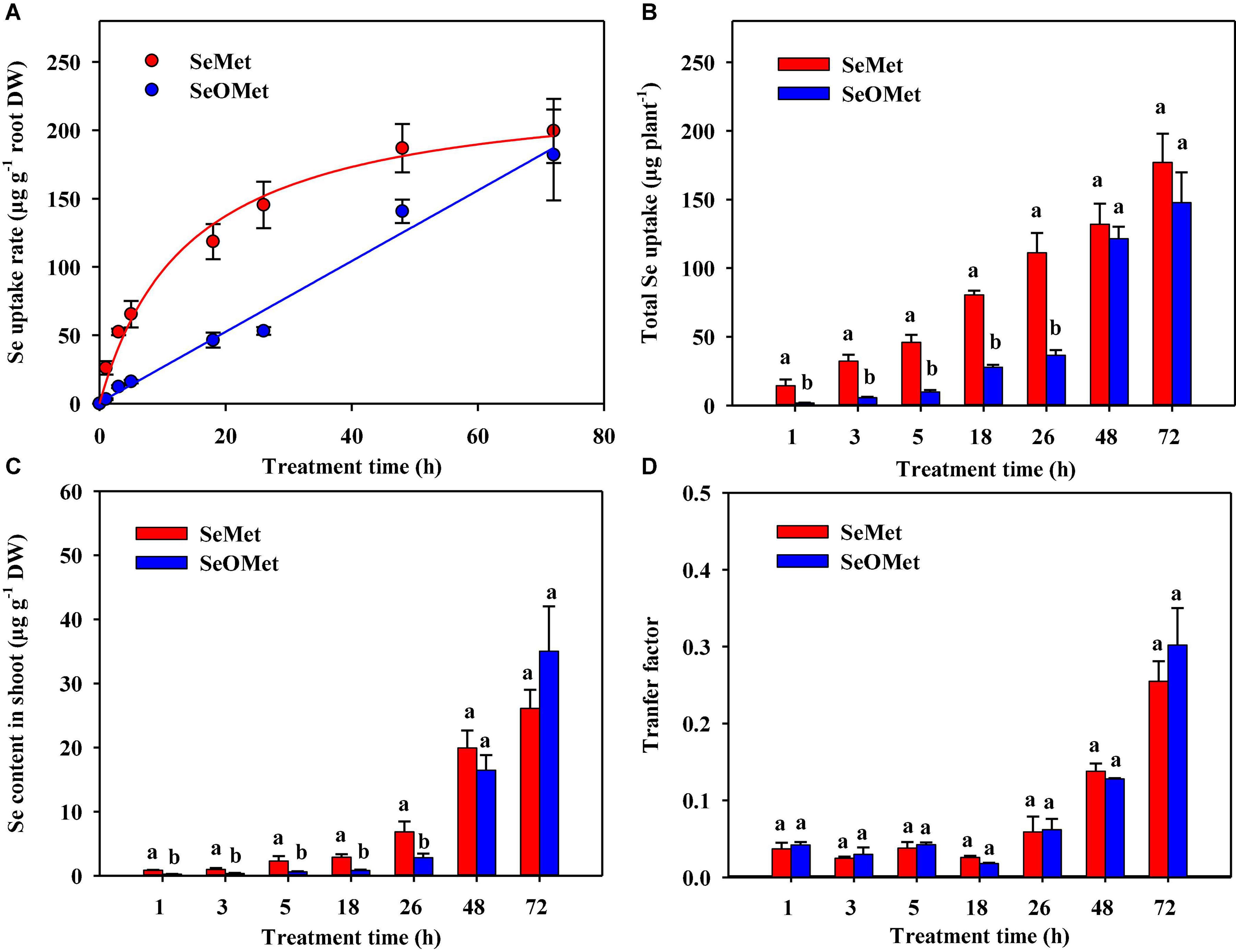
Figure 2. Time-dependent kinetics of the uptake of organic Se by rice roots (A), total Se uptake (B), the contents of Se in rice shoots (C), and the transfer factor of Se from roots to shoots (D). Data are presented as mean ± SE (n = 4). Different lowercase letters above bars indicate significant differences between SeMet and SeOMet treatments in individual treatment times (P < 0.05).
When assessed at time points prior to 26 h, the contents of Se in the shoots of plants treated with SeOMet were between 58.6 and 72.3% lower than those in the plants treated with SeMet. However, in response to prolonged exposure for 48 and 72 h, the differences between these two Se chemical forms became non-significant (Figure 2C). In order to evaluate the root-to-shoot translocation ability of different Se sources, the transfer factor was introduced in the study. In general, irrespective of the Se treatments, the transfer factor of Se from rice roots to shoots showed an increasing trend with increase of the exposure time, varied from 0.025 to 0.302; while the lowest transfer factor was appeared at 18 h. In addition, there was no significant difference of the transfer factor between SeMet and SeOMet treatments at all exposure time (Figure 2D).
Effects of inhibitors on the uptake rate of organic Se
To gain further insights into the uptake process, we examined the effects of exposure to four specific inhibitors on uptake of the two assessed chemical forms of organic Se following 1 h exposures. Our observations revealed that Se uptake rate was significantly affected by Se form, inhibitor type, and their interaction between these two factors (Figure 3, P < 0.001). Generally, the uptake of Se as SeMet by rice roots was substantially higher than that as SeOMet, the former being 1.25–18.5-fold higher than the latter. Compared with the control, the exposure to AgNO3 significantly reduced the uptake of Se as SeMet and SeOMet by 93.1 and 41.8%, respectively (P < 0.05); whereas, although TEACl significantly inhibited the uptake of Se as SeOMet by 45.7% (P < 0.05), no significant inhibitory effect was detected in the uptake of SeMet. Contrastingly, neither CoCl2 nor DIDS had any significant inhibitory effects on Se uptake as either SeMet or SeOMet.
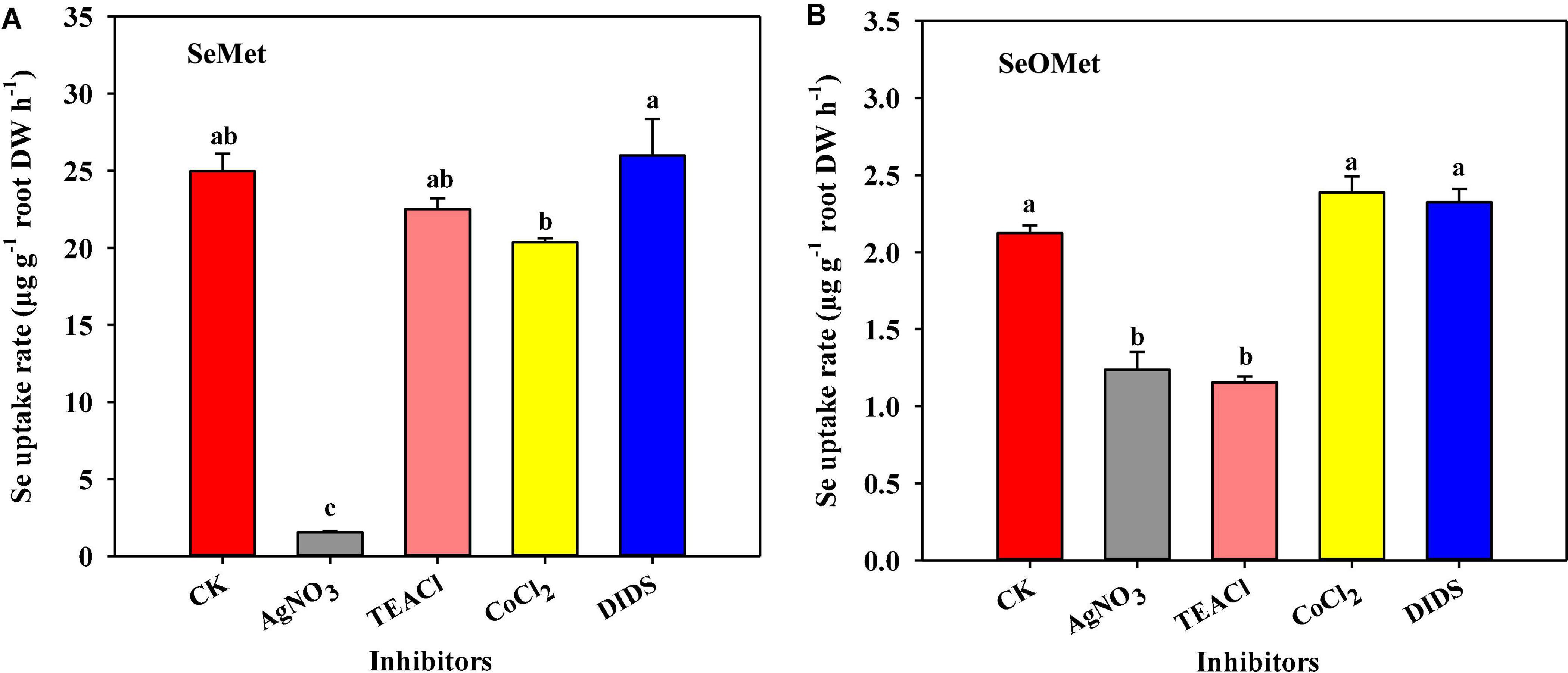
Figure 3. Effect of different specific inhibitors on the uptake of Se by rice supplied with SeMet (A) and SeOMet (B). Data are presented as mean + SE (n = 4). Different lowercase letters above bars indicate significant differences among the inhibitor treatments (P < 0.05).
Moreover, whereas the exposure to the metabolic inhibitor CCCP had no significant effect on the uptake of Se as SeMet, a significant reduction of 30.4% was detected for SeOMet form. The presence of ethanol in this treatment had no significant effect on Se uptake (Figure 4).
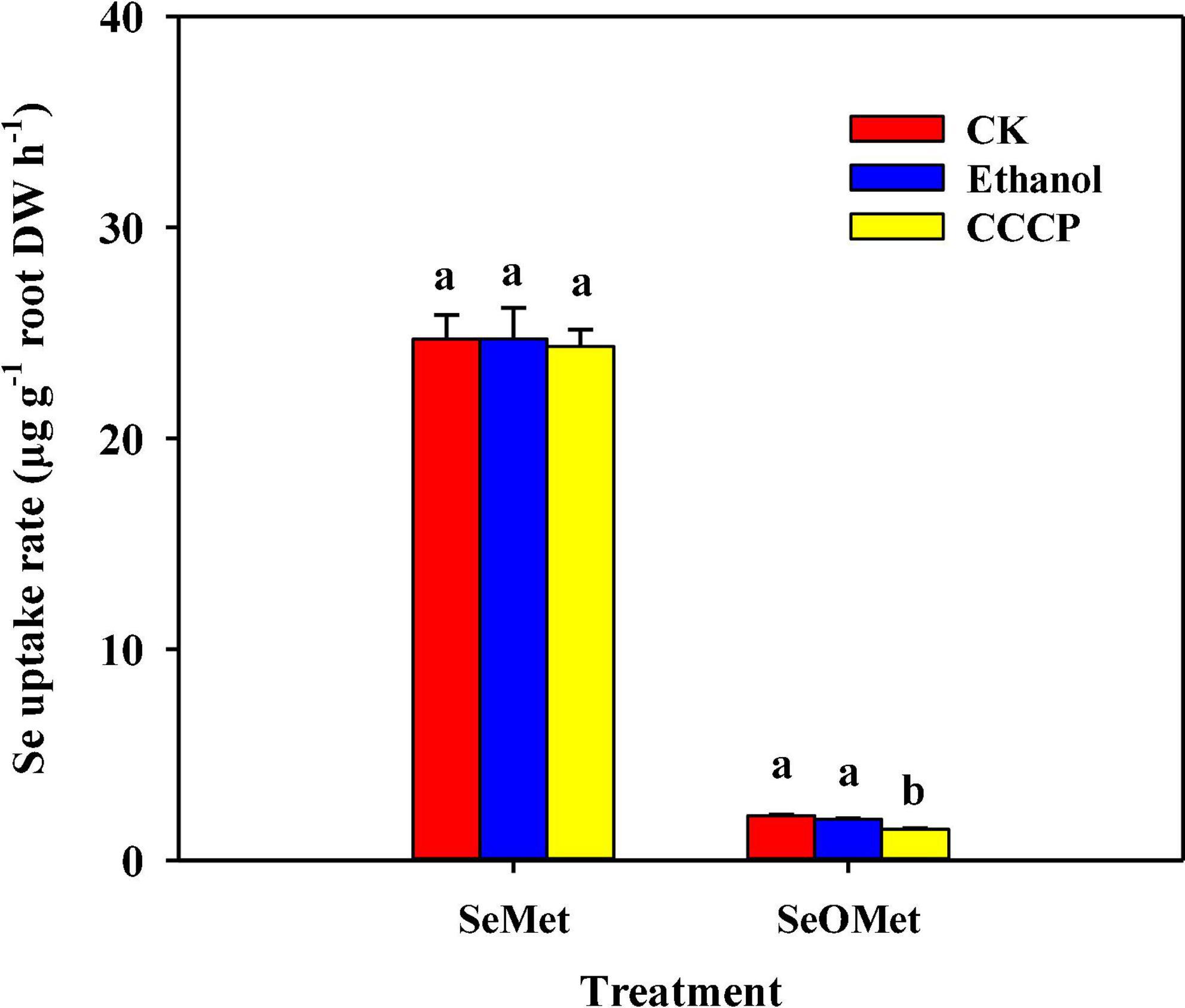
Figure 4. Effect of the respiratory inhibitor CCCP on the uptake of Se by rice supplied with different forms of organic Se. Data are presented as mean + SE (n = 4). Different lowercase letters above bars indicate significant differences among inhibitor treatments in individual Se treatment forms (P < 0.05).
Effects of P or S starvation on organic Se uptake and translocation
This experiment was designed to determine the effects of major macronutrients P and S on the uptake and translocation of different organic chemical forms of Se. Rice plants were grown in normal, S-deficient, or P-deficient medium for 7 days, after which, they were transferred to media containing either 5 μM SeMet or SeOMet for a further 2 days. To evaluate the efficiency of Se translocation, Se distribution in shoot (%) was measured as the proportion of Se allocated to rice shoots. Interestingly, we found that whereas the uptake of Se by roots was significantly affected by Se forms (P < 0.05) but not by S or P status (P > 0.05), Se distribution in shoot (%) was significantly affected by nutrient status (P < 0.05) but not by Se forms (P > 0.05). Moreover, the contents of Se in shoots were significantly affected by both Se forms (P < 0.05) and the nutrient status (P < 0.05) (Table 2).
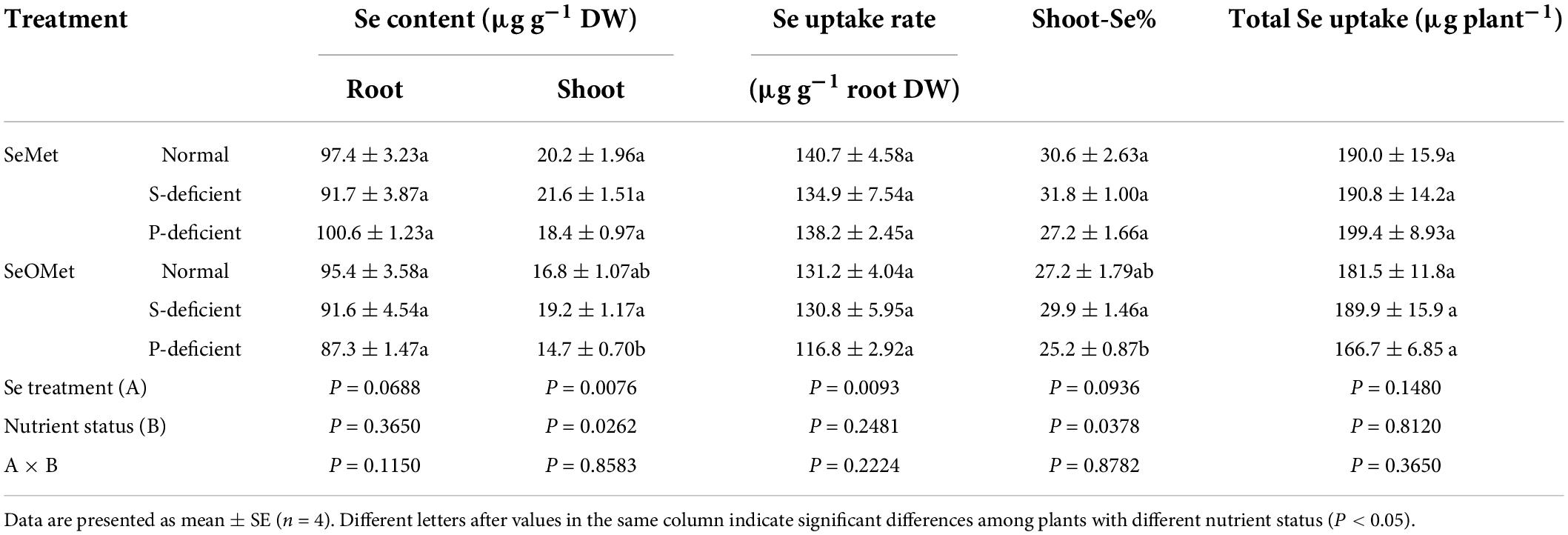
Table 2. Effect of Se chemical forms supplied and nutrient status on the content, uptake rate, and proportion of Se allocated to rice shoot.
In general, the contents of Se detected in rice plants, Se uptake rate, total uptake of Se, and distribution in shoots were higher in plants administered SeMet (although not all the detected differences were significant). Furthermore, compared with the normal treatment, neither P nor S deficiencies appeared to have any substantial effects on either the uptake or translocation of Se by rice, although we did observe a non-significant 14.9% reduction in the uptake of Se as SeOMet in response to a deficiency in P (Table 2).
Interactions between selenomethionine and selenomethionine-oxide
The results of interaction experiment revealed that Se uptake and translocation in rice were significantly affected by the interactions between SeMet and SeOMet (Table 3). We found that both root Se content and uptake in rice treated with both SeMet and SeOMet forms at the same total Se concentration (SeMet + SeOMet) were 90.9 and 92.5%, respectively, lower than those in plants exposed to SeMet only, although no significant differences were detected between SeMet + SeOMet and SeOMet treatments.
The efficiency of Se translocation during 1 h treatments was also expressed in terms of Se distribution in shoot (%). However, we were able to detect Se only in the shoots of those plants treated with SeMet treatment, which accounted for 16.9% of the total Se. In addition, the total uptake of Se by the roots of plants exposed to SeMet was between 11.4- and 11.7-fold higher than that in the SeOMet and SeMet+SeOMet treatments (P < 0.001). According to the calculations described by Longchamp et al. (2013) and Wang et al. (2019), in the absence of an interaction between two Se chemical forms, the total uptake of Se by roots should theoretically be 5.01 μg in the SeMet + SeOMet treatment. However, our findings indicated a total Se uptake of only ∼15% of the theoretical quantity in plants exposed to a mixture of SeMet and SeOMet, which accordingly tends to indicate a non-additive effect. This suggests that during uptake and translocation in rice, SeMet and SeOMet may interact to a certain extent.
Data are presented as the mean ± SE (n = 4). Different letters after values within the same column indicate a significant difference among the treatments (P < 0.05).
Discussion
Selenium uptake kinetics in rice root
The capacity of plants to take up Se from soil depends on plant species, soil Se concentration and form, and environmental conditions (including pH, Eh, and organic matter content). A number of previous studies have reported the uptake kinetics of different Se chemical forms in plant roots. For example, Huang et al. (2015) observed that the uptake of selenite into rice roots was considerably more rapid than that of selenate, with the value of Vmax for selenite influx (102 μg⋅g–1 root h–1 DW) being approximately 6.5-fold higher than that for selenate (13.7 μg⋅g–1 root h–1 DW), with Km values of 16.2 and 11.3 μM for selenite and selenate, respectively. Similarly, Hu et al. (2018) demonstrated that, for Se uptake by wheat roots, selenite (Vmax: 25.6 μg⋅g–1 root h–1 DW) is characterized by a higher uptake potential than nanoselenium (Vmax: 10.1 μg⋅g–1 root h–1 DW). Moreover, Zhang et al. (2019) obtained a Vmax value of 132 μg⋅g–1 root h–1 DW for the uptake of SeMet. In the present study, we found that rice are characterized by a higher uptake potential (Vmax) for organic Se than for inorganic forms, showing a descending order of SeMet > SeOMet > selenite > selenate (Figure 1 and Table 1). Similarly, Wang M. K. et al. (2020) demonstrated that the roots of maize (Zea mays L.) had a higher uptake of organic Se (SeMet, MeSeCys, and SeCys) than inorganic Se (selenite and selenate) when supplied with 0.01 or 1 mg⋅L–1 Se. Consistently, Kikkert and Berkelaar (2013) found that the rate of SeMet uptake by the wheat roots was considerably higher than that of either selenite or selenate and conjectured that this difference could be attributable to the differences in the activities of their respective transporters. Moreover, in the present study, the differences in the rates of selenite and SeOMet uptake by rice roots varied according to the Se concentration exogenously applied Se (Figure 1). Likewise, Kikkert and Berkelaar (2013) found that the rate of selenite uptake was 60% lower than that of selenate, when supplied at a Se concentration of 0.5 μM but was 3.6 times higher when supplied with 5 μM Se. Collectively, these findings tend to indicate that the Se uptake capacity of plants might also be influenced by the interaction between the Se chemical form and the Se exposure level.
Regarding the two organic Se compounds (SeMet and SeOMet), this study showed that the uptake rate of SeMet was significantly greater than that of SeOMet during the initial 26 h of treatments (Figures 1, 2), although the extent of the observed differences narrowed when measured at 48 and 72 h (Figure 2). We speculated that this pattern reflected the fact that during the latter part of the treatment period, SeMet uptake was gradually approaching a level of saturation at a decreasing rate. Additionally, the effects of exposure time on organic Se absorption could be attributed to the transformation of SeMet to SeOMet in rice roots or within the rhizosphere, a conjecture that is supported by previous findings indicating that SeOMet is detectable in the roots of lettuce (Lactuca sativa L.) exposed to SeMet (Kowalska et al., 2020). Similarly, in a previous study, we detected both SeMet and SeOMet in the roots of selenite-treated wheat (Li et al., 2008), thereby indicating an occurrence of the oxidative transformation of Se in plants.
The mechanisms of selenomethionine and selenomethionine-oxide uptake
To investigate the physiological processes associated with the uptake of SeMet and SeOMet by rice and the underlying mechanisms, effects of selected inhibitors on Se uptake have been assessed in this study. Among these, CCCP is a respiratory inhibitor that promotes a dissipation of the proton motive force across membranes. We found that whereas the uptake of SeOMet is sensitive to CCCP, but unaffected on SeMet (Figure 3). These observations contrast with the findings of previous hydroponic studies, which have revealed that CCCP significantly inhibits the uptake of SeMet by rice and wheat, thereby tending to indicate that SeMet is taken up by an energy-dependent symport process (Abrams et al., 1990; Zhang et al., 2019). We suspect that the disparity between the findings of these different studies could be due to differences in the respective exposure times (only 1 h in this study), which should be confirmed by further studies. Nevertheless, our findings do indicate that SeOMet uptake is a metabolically active process requiring selective binding sites and metabolic energy.
Furthermore, the findings of our specific inhibitor treatments indicate that SeMet and SeOMet are taken up into rice roots via different channels. Among the other inhibitors we studied, AgNO3 is a potential inhibitor of aquaporins of plant origin and partially inhibit the uptake of selenite (Zhang et al., 2006) and Nano-Se (Wang M. K. et al., 2020). The mechanism of its inhibit function is that silver reacts with the sulfhydryl group of a cysteine and also with a histidine, thereby resulting in a gating of the targeted aquaporins (Niemietz and Tyerman, 2002). The other three assessed inhibitors, TEACl, CoCl2, and DIDS, are recognized as specific inhibitors of K+ (White, 1995), Ca2+ (Harada and Shimazaki, 2009), and anionic channels, respectively. In the present study, we found that the addition of AgNO3 to the uptake solution significantly reduced the rate of SeMet uptake by 93.1% (Figure 3), thereby providing evidence that rice absorbs SeMet mainly via aquaporins. With respect to SeOMet, we observed that both AgNO3 (41.8%) and TEACl (45.6%) can significantly inhibit uptake by rice roots (Figure 3), which might indicate that the influx of SeOMet is mediated via both aquaporins and K+ channels. However, exposure to CoCl2 and DIDS exhibited no appreciable effects on Se uptake, which would accordingly tend to indicate that the uptake of SeOMet and SeMet is associated with neither Ca2+ nor anion channels.
Depriving plants of S significantly increases the uptake of selenate, whereas P starvation induces significant increases in selenite uptake (Li et al., 2008). And there is a competition between selenate and sulfate for uptake by roots (de Souza Cardoso et al., 2022). In the present study, we found that neither S nor P deficiency had the effect of promoting the uptake of SeMet or SeOMet by rice roots (Table 2), which might indicate that the uptake of organic Se is independent of sulfate or phosphate transporters. Conversely, under P-deficient conditions, a slight reduction was observed in the root uptake of Se as SeOMet (Table 2). In this regard, phosphorylation is one of the factors associated with the regulation of K+ channel activity (Yu et al., 2006), and thus, a reduction in SeOMet uptake under P-deficient conditions is attributable to diminished K+ channels activity, which needs to be confirmed by further in-depth molecular studies.
The translocation of selenomethionine and selenomethionine-oxide from roots to shoots
In rice exposed to different sources of Se for 48 h, we found that the proportions of Se distributed in the shoots of rice supplied with the two assessed organic forms of Se ranged from 25.2 to 31.8% (Table 2), which are lower than the values we previously recorded in plants supplied with selenate, although slightly higher than those in plants treated with selenite (Huang et al., 2015). In this regard, the findings of several studies on rice and wheat indicated that most of the selenate taken up by roots is subsequently translocated to the shoots (Wang et al., 2015). Conversely, having been absorbed by roots, selenite is rapidly converted to organic forms, such as SeMet, MeSeCys, and SeOMet, which reduces mobility (da Silva et al., 2020). Sulfate transporters such as Sultr2;1, Sultr3;5, and Sultr1;3, are the main transporters involved in the translocation of selenate from roots to shoots (Maruyama-Nakashita, 2017; Mehdawi et al., 2018), whereas phosphorus transporters such as OsPT8 transport selenite in plants (Song et al., 2017). Furthermore, Zhang et al. (2019) found that NRT1.1B, a member of the peptide transporter family, mediates the transport activity of SeMet, whereas in maize, Wang K. et al. (2020) recorded that the values of Se distribution in shoot (%) decreased in the order of selenate treatment > selenite treatment > SeMet treatment when supplied as 0.01 mg L–1 Se and in the order of selenate treatment > SeMet treatment > selenite treatment when plants were supplied with 0.1 mg L–1 Se. Moreover, Kowalska et al. (2020) found that the translocation of Se from the roots to leaves of lettuce supplied with SeMet was 3.65 times higher than that in lettuce supplied with selenite. These phenomena can presumably be attributed to the differing capacities of the transporters of different Se chemical forms. In the present study, no significant difference was found in the transfer factor of Se from rice roots to shoots between SeMet and SeOMet (Figure 2D and Table 2), indicating the similar transportation ability of SeMet and SeOMet in rice plants; while the lowest transfer factor at 18 h might be due to the lowest transpiration rate when treated for 18 h (5:00 am), since the transportation of micro-element from roots to shoots is mainly driven by transpiration (Van der Vliet et al., 2007). Furthermore, we also found that the contents of Se were higher in the shoots of plants supplied with SeMet than in those of plants receiving SeOMet treatment when exposed for up to 26 h (Figure 2C and Table 3), although the differences were found to gradually diminish with a prolongation of exposure (Figure 2C and Table 2). This effect of exposure time on organic Se accumulation in shoots is conceivably associated with the transformation of Se in rice plants.
In addition, we established that the uptake and translocation of Se by rice plants maintained in the growth medium treated with both SeMet + SeOMet were considerably lower than the theoretical quantities (Table 3), indicating an interaction between SeMet and SeOMet when both Se forms are supplied simultaneously. In this regard, previous studies have reported non-additive effects in the uptake and translocation of different Se chemical forms, with the coexisting selenite being found to inhibit selenate uptake and translocation (Li et al., 2008; Wang et al., 2019). Similarly, in the present study, we found that the presence of SeOMet appeared to suppress the uptake and translocation of SeMet in rice. We speculate that these observations could be explained in terms of a preferential absorption by plants, whereby an optimal absorption strategy is adopted based on intrinsic synergistic activities in response to mixed supplies of different Se chemical forms, thus conserving energy required for subsequent Se assimilation (Versini et al., 2016). However, this needs to be verified in further studies.
Conclusion
In this study, we demonstrated that the uptake and translocation of Se by rice were significantly influenced by both Se chemical forms and treatment time. Compared with inorganic forms, organic Se exhibited a higher uptake potential over the course of a 1 h exposure, with recorded uptake capacity (Vmax) values declining in the order of SeMet > SeOMet > selenite > selenate. Furthermore, analysis of the time-dependent kinetics of organic Se uptake by roots revealed that, regardless of the duration of exposure in Se-treated growth media, the uptake of SeMet was invariably higher than that of SeOMet, whereas difference between the uptake of these two forms narrowed with time. A similar tendency was detected with respect to the Se in rice shoots. In addition, examination of the effects of selected inhibitors on Se uptake indicated that SeOMet uptake is an energy-dependent symport process and that SeOMet could be imported by rice roots via aquaporins and K+ channels. In contrast, the uptake of SeMet by roots appears to be mediated primarily via aquaporins. We also found that when simultaneously supplied with both SeOMet and SeMet, SeOMet appeared to inhibit the uptake and translocation of SeMet.
Data availability statement
The original contributions presented in this study are included in the article/Supplementary material, further inquiries can be directed to the corresponding author.
Author contributions
QW, YW, and HL designed the research and wrote the manuscript. QW performed the experiments. LK helped in data analysis. QH helped in sample determination. All authors read, reviewed, and approved the manuscript.
Funding
This work was supported by the National Natural Science Foundation of China (no. 41907146) and the Special Fund for Agro-scientific Research in the Public Interest of China (no. 201303106).
Conflict of interest
The authors declare that the research was conducted in the absence of any commercial or financial relationships that could be construed as a potential conflict of interest.
Publisher’s note
All claims expressed in this article are solely those of the authors and do not necessarily represent those of their affiliated organizations, or those of the publisher, the editors and the reviewers. Any product that may be evaluated in this article, or claim that may be made by its manufacturer, is not guaranteed or endorsed by the publisher.
Supplementary material
The Supplementary Material for this article can be found online at: https://www.frontiersin.org/articles/10.3389/fpls.2022.970480/full#supplementary-material
References
Abrams, M. M., Shennan, C., Zasoski, R. J., and Burau, R. G. (1990). Selenomethionine uptake by wheat seedlings. Agron. J. 82, 1127–1130.
Alfthan, G., Eurola, M., Ekholm, P., Venäläinen, E. R., Root, T., Korkalainen, K., et al. (2015). Effects of nationwide addition of selenium to fertilizers on foods, and animal and humanhealth in Finland: from deficiency to optimal selenium status of the population. J. Trace Elem. Med. Biol. 31, 142–147. doi: 10.1016/j.jtemb.2014.04.009
Avery, J. C., and Hoffmann, P. R. (2018). Selenium, selenoproteins, and immunity. Nutrients 10:1203.
da Silva, D. F., Cipriano, P. E., de Souza, R. R., Júnior, M. S., da Silva, R. F., Faquin, V., et al. (2020). Anatomical and physiological characteristics of Raphanus sativus L. submitted to different selenium sources and forms application. Sci. Hortic. 260:108839.
de Souza Cardoso, A. A., de Lima Gomes, F. T., Antonio, J. R. R., Guilherme, L. R. G., Liu, J. P., Li, L., et al. (2022). Sulfate availability and soil selenate adsorption alleviate selenium toxicity in rice plants. Environ. Exp. Bot. 201:104971.
Elrashidi, M. A., Adriano, D. C., Workman, S. M., and Lindsay, W. L. (1987). Chemical equilibria of selenium in soils: a theoretical development. Soil Sci. 144, 141–152.
Fairweather-Tait, S. J., Collings, R., and Hurst, R. (2010). Selenium bioavailability: current knowledge and future research requirements. Am. J. Clin. Nutr. 91, 1484s–1491s. doi: 10.3945/ajcn.2010.28674J
Gong, R., Ai, C., Zhang, B., and Cheng, X. (2018). Effect of selenite on organic selenium speciation and selenium bioaccessibility in rice grains of two Se-enriched rice cultivars. Food Chem. 264, 443–448. doi: 10.1016/j.foodchem.2018.05.066
Harada, A., and Shimazaki, K. (2009). Measurement of changes in cytosolic Ca2+ in Arabidopsis guard cells and mesophyll cells in response to blue light. Plant Cell Physiol. 50, 360–373. doi: 10.1093/pcp/pcn203
Hatfield, D. L., Tsuji, P. A., Carlson, B. A., and Gladyshev, V. N. (2014). Selenium and selenocysteine: roles in cancer, health, and development. Trends Biochem. Sci. 39, 112–120.
Haug, A., Graham, R. D., Christophersen, O. A., and Lyons, G. H. (2007). How to use the world’s scarce selenium resources efficiently to increase the selenium concentration in food. Microb. Ecol. Health Dis. 19, 209–228. doi: 10.1080/08910600701698986
Hu, T., Li, H. F., Li, J. X., Zhao, G. S., Wu, W. L., Liu, L. P., et al. (2018). Absorption and bio-transformation of selenium nanoparticles by wheat seedlings (Triticum aestivum L.). Front. Plant Sci. 9:597. doi: 10.3389/fpls.2018.00597
Huang, G. X., Ding, C. F., Yu, X. Y., Yang, Z., Zhang, T. L., and Wang, X. X. (2018). Characteristics of time-dependent selenium biofortification of rice (Oryza sativa L.). J. Agric. Food Chem. 66, 12490–12497. doi: 10.1021/acs.jafc.8b04502
Huang, Q. Q., Wang, Q., Wan, Y. N., Yu, Y., Jiang, R. F., and Li, H. F. (2017). Application of X-ray absorption near edge spectroscopy to the study of the effect of sulphur on selenium uptake and assimilation in wheat seedlings. Biol. Plant 61, 726–732.
Huang, Q. Q., Yu, Y., Wang, Q., Luo, Z., Jiang, R. F., and Li, H. F. (2015). Uptake kinetics and translocation of selenite and selenate as affected by iron plaque on root surfaces of rice seedlings. Planta 241, 907–916. doi: 10.1007/s00425-014-2227-7
Jiang, H. Y., Lin, W. Q., Jiao, H. P., Liu, J. G., Chan, L., Liu, X. Y., et al. (2021). Uptake, transport, and metabolism of selenium and its protective effects against toxic metals in plants: a review. Metallomics 13:mfab040.
Kikkert, J., and Berkelaar, E. (2013). Plant uptake and translocation of inorganic and organic forms of selenium. Arch. Environ. Con. Tox. 65, 458–465.
Kowalska, I., Smolen, S., Czernicka, M., Halka, M., Keska, K., and Pitala, J. (2020). Effect of selenium form and salicylic acid on the accumulation of selenium speciation forms in hydroponically grown lettuce. Agriculture 10:584.
Lanza, M. G. D. B. L., de Carvalho, H. W. P. B., and dos Reis, A. R. (2021a). Roles of selenium in mineral plant nutrition: ROS scavenging responses against abiotic stresses. Plant Physiol. Biochem. 164, 27–43. doi: 10.1016/j.plaphy.2021.04.026
Lanza, M. G. D. B. L., Silva, V. M., Montanha, G. S., Lavres, J., de Carvalho, H. W. P. B., and dos Reis, A. R. (2021b). Assessment of selenium spatial distribution using μ-XFR in cowpea (Vigna unguiculata (L.) Walp.) plants: integration of physiological and biochemical responses. Ecotox. Environ. Saf. 207:11216. doi: 10.1016/j.ecoenv.2020.111216
Larsen, E. H., Hansen, M., Paulin, H., Moesgaard, S., and Reid, M. (2004). Speciation and bioavailability of selenium in yeast-based intervention agents used in cancer chemoprevention studies. J. Aoac. Int. 87, 225–232.
Li, H. F., Lombi, E., Stroud, J. L., Mcgrath, S. P., and Zhao, F. J. (2010). Selenium speciation in soil and rice: influence of water management and se fertilization. J. Agric. Food Chem. 58, 11837–11843.
Li, H. F., McGrath, S. P., and Zhao, F. J. (2008). Selenium uptake, translocation and speciation in wheat supplied with selenate or selenite. New Phytol. 178, 92–102.
Longchamp, M., Angeli, N., and Castrec-Rouelle, M. (2013). Selenium uptake in Zea mays, supplied with selenate or selenite under hydroponic conditions. Plant Soil 362, 107–117.
Maruyama-Nakashita, A. (2017). Metabolic changes sustain the plant life in low-sulfur environments. Curr. Opin. Plant Biol. 39, 144–151. doi: 10.1016/j.pbi.2017.06.015
Mehdawi, A. F. E., Jiang, Y., Guignardi, Z. S., Esmat, A., Pilon, M., Pilon-Smits, E. A., et al. (2018). Influence of sulfate supply on selenium uptake dynamics and expression of sulfate/selenate transporters in selenium hyperaccumulator and nonhyperaccumulator Brassicaceae. New Phytol. 217, 194–205. doi: 10.1111/nph.14838
Niemietz, C. M., and Tyerman, S. D. (2002). New potent inhibitors of aquaporins: silver and gold compounds inhibit aquaporins of plant and human origin. FEBS Lett. 531, 443–447. doi: 10.1016/s0014-5793(02)03581-0
Rayman, M. P., Infante, H. G., and Sargent, M. (2008). Food-chain selenium and human health: spotlight on speciation. Br. J. Nut. 100, 238–253. doi: 10.1017/S0007114508922522
Song, Z., Shao, H., Huang, H., Shen, Y., Wang, L., Wu, F., et al. (2017). Overexpression of the phosphate transportergene OsPT8 improves the Pi and selenium contents in Nicotiana tabacum. Environ. Exp. Bot. 137, 158–165.
Sors, T. G., Ellis, D. R., and Salt, D. E. (2005). Selenium uptake, translocation, assimilation and metabolic fate in plants. Photosynth. Res. 86, 373–389.
Sun, G. X., Liu, X., Williams, P. N., and Zhu, Y. G. (2010). Distribution and translocation of selenium from soil to grain and its speciation in paddy rice (Oryza sativa L.). Environ. Sci. Technol. 44, 6706–6711. doi: 10.1021/es101843x
Van der Vliet, L., Peterson, C., and Hale, B. (2007). Cd accumulation in roots and shoots of durum wheat: the roles of transpiration rate and apoplastic bypass. J. Exp. Bot. 58, 2939–2947. doi: 10.1093/jxb/erm119
Versini, A., Di Tullo, P., Aubry, E., Bueno, M., Thiry, Y., Pannier, F., et al. (2016). Influence of Se concentrations and species in hydroponic cultures on Se uptake, translocation and assimilation in non-accumulator ryegrass. Plant Physiol. Biochem. 108, 372–380. doi: 10.1016/j.plaphy.2016.07.029
Wang, K., Wang, Y. Q., Li, K., Wan, Y. N., Wang, Q., Zhuang, Z., et al. (2020). Uptake, translocation and biotransformation of selenium nanoparticles in rice seedlings (Oryza sativa L.). J. Nanobiotechnol. 18:103. doi: 10.1186/s12951-020-00659-6
Wang, M. K., Dinh, Q. T., Qi, M. X., Wang, M., Yang, W. X., Zhou, F., et al. (2020). Radicular and foliar uptake, and xylem- and phloem-mediated transport of selenium in maize (Zea mays L.): a comparison of five Se exogenous species. Plant Soil 446, 111–123.
Wang, M. K., Peng, Q., Zhou, F., Yang, W. X., Dinh, Q. T., and Liang, D. L. (2019). Uptake kinetics and interaction of selenium species in tomato (Solanum lycopersicum L.) seedlings. Environ. Sci. Pollut. Res. 26, 9730–9738. doi: 10.1007/s11356-019-04182-6
Wang, P., Menzies, N. W., Lombi, E., McKenna, B. A., James, S., Tang, C., et al. (2015). Synchrotron-based X-ray absorption near-edge spectroscopy imaging for laterally resolved speciation of selenium in fresh roots and leaves of wheat and rice. J. Exp. Bot. 66, 4795–4806. doi: 10.1093/jxb/erv254
White, P. J. (1995). Separation of K+- and Cl–selective ion channels from rye roots on a continuous sucrose density gradient. J. Exp. Bot. 46, 361–376.
White, P. J., and Broadley, M. R. (2009). Biofortification of crops with seven mineral elements often lacking in human diets–iron, zinc, copper, calcium, magnesium, selenium and iodine. New Phytol. 182, 49–84.
Yu, L., Becker, D., Levi, H., Moshelion, M., Hedrich, R., Lotan, I., et al. (2006). Phosphorylation of SPICK2, an AKT2 channel homologue from Samanea motor cells. J. Exp. Bot. 57, 3583–3594. doi: 10.1093/jxb/erl104
Yu, Y., Liu, Z., Luo, L. Y., Fu, P. N., Wang, Q., and Li, H. F. (2019). Selenium uptake and biotransformation in Brassica rapa supplied with selenite and selenate: a hydroponic work with HPLC speciation and RNA-sequencing. J. Agric. Food Chem. 67, 12408–12418. doi: 10.1021/acs.jafc.9b05359
Zhang, L. H., Hu, B., Deng, K., Gao, X. K., Sun, G. X., Zhang, Z. L., et al. (2019). NRT1.1B improves selenium concentrations in rice grains by facilitating selenomethinone translocation. Plant Biotechnol. J. 17, 1058–1068. doi: 10.1111/pbi.13037
Zhang, L. H., Hu, B., Li, W., Che, R. H., Deng, K., Li, H., et al. (2014). OsPT2, a phosphate transporter, is involved in the active uptake of selenite in rice. New Phytol. 201, 1183–1191. doi: 10.1111/nph.12596
Zhang, L. H., Shi, W. M., and Wang, X. C. (2006). Difference in selenite absorption between high- and low-selenium rice cultivars and its mechanism. Plant Soil 282, 183–193.
Zhang, X. C., Gao, H. J., Zhang, Z. Z., and Chun, W. X. (2013). Influences of different ion channel inhibitors on the absorption of fluoride in tea plants (Camellia sinesis L.). Plant Growth Regul. 69, 99–106.
Zhao, X. Q., Mitani, N., Yamaji, N., Shen, R. F., and Ma, J. F. (2010). Involvement of silicon influx transporter OsNIP2;1 in selenite uptake in rice. Plant Physiol. 153, 1871–1877. doi: 10.1104/pp.110.157867
Keywords: rice, selenomethionine, selenomethionine-oxide, uptake kinetics, transport, interaction
Citation: Wang Q, Kong L, Huang Q, Li H and Wan Y (2022) Uptake and translocation mechanisms of different forms of organic selenium in rice (Oryza sativa L.). Front. Plant Sci. 13:970480. doi: 10.3389/fpls.2022.970480
Received: 16 June 2022; Accepted: 02 August 2022;
Published: 22 August 2022.
Edited by:
Zhi-Qing Lin, Southern Illinois University Edwardsville, United StatesReviewed by:
José Lavres Junior, University of São Paulo, BrazilBarbara Hawrylak-Nowak, University of Life Sciences in Lublin, Poland
Copyright © 2022 Wang, Kong, Huang, Li and Wan. This is an open-access article distributed under the terms of the Creative Commons Attribution License (CC BY). The use, distribution or reproduction in other forums is permitted, provided the original author(s) and the copyright owner(s) are credited and that the original publication in this journal is cited, in accordance with accepted academic practice. No use, distribution or reproduction is permitted which does not comply with these terms.
*Correspondence: Yanan Wan, d2FueWFuYW5AY2F1LmVkdS5jbg==