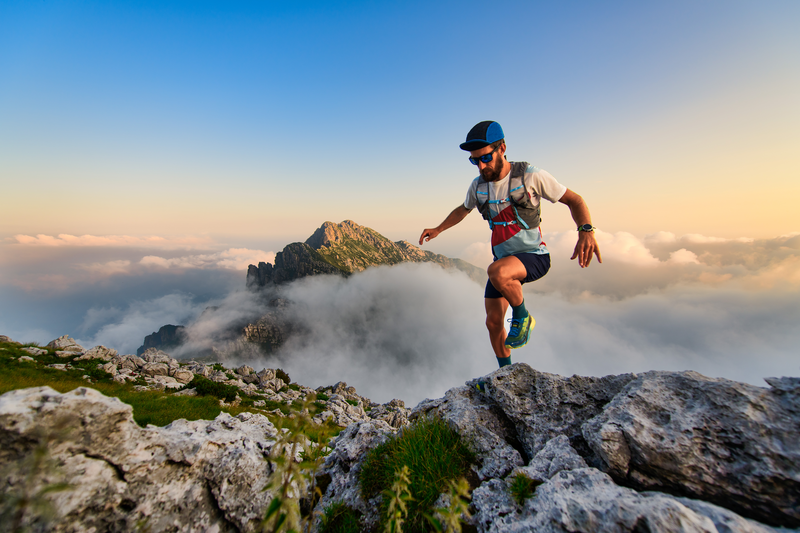
95% of researchers rate our articles as excellent or good
Learn more about the work of our research integrity team to safeguard the quality of each article we publish.
Find out more
REVIEW article
Front. Plant Sci. , 22 August 2022
Sec. Plant Abiotic Stress
Volume 13 - 2022 | https://doi.org/10.3389/fpls.2022.970339
Nitrogen is a vital nutrient for agricultural, and a defieciency of it causes stagnate cotton growth and yield penalty. Farmers rely heavily on N over-application to boost cotton output, which can result in decreased lint yield, quality, and N use efficiency (NUE). Therefore, improving NUE in cotton is most crucial for reducing environmental nitrate pollution and increasing farm profitability. Well-defined management practices, such as the type of sources, N-rate, application time, application method, crop growth stages, and genotypes, have a notable impact on NUE. Different N formulations, such as slow and controlled released fertilizers, have been shown to improve N uptake and, NUE. Increasing N rates are said to boost cotton yield, although high rates may potentially impair the yield depending on the soil and environmental conditions. This study comprehensively reviews various factors including agronomic and environmental constraints that influence N uptake, transport, accumulation, and ultimately NUE in cotton. Furthermore, we explore several agronomic and molecular approaches to enhance efficiency for better N uptake and utilization in cotton. Finally, this objective of this review to highlight a comprehensive view on enhancement of NUE in cotton and could be useful for understanding the physiological, biochemical and molecular mechanism of N in cotton.
Cotton is a dual-purpose crop grown for fiber and oil purposes (Ali et al., 2019). Under field conditions, various factors such as environmental conditions and agricultural management practices significantly influenced cotton growth and productivity. Nitrogen is one of the most important limiting variables for cotton growth, productivity, and quality, and it is required more than other vital nutrients (Khan et al., 2017a,b,c). Normally, farmers rely on the excessive application of N to enhance growth and crop yield (Dong et al., 2012). Nevertheless, excessive rates of N not only outcomes in stagnant growth, prolonged crop maturity, and reduction in productivity, but also reduce NUE and environmental pollution (Khan et al., 2021; Niu et al., 2021; Huang et al., 2022; Javed et al., 2022a,b; Kumar et al., 2022; Wang et al., 2022).
Nitrogen, when applied with the appropriate method, time, and rate, significantly increased plant biomass production by improving the physiological events in plants including chlorophyll contents. However, to increase the cotton yield and profitability, farmers largely depend on the excessive application of N which can cause late crop maturity, decrease NUE and yield, and overall reduction in farm productivity (Devkota et al., 2013; Lehrsch et al., 2015). The previously published reports claimed that administering the appropriate N doses can increase biomass production and physiological characteristics which improve the yield and quality of cotton (Chen et al., 2016; Niu et al., 2021). Moreover, other published studies also suggested that reducing the rate of N with appropriate agronomic management operation not only improves the NUE but also increases the lint yield and quality (Dong et al., 2012; Luo et al., 2018). Besides, straw and straw-derived biochar incorporation into the soil is one of the major alternatives to prevent the further increase in synthetic nitrogen fertilizer during crop production (Chen et al., 2015; Xia et al., 2016). Nonetheless, the overuse of chemical N fertilizer in the cotton production system has not been ameliorated. Consequently, an in-depth understanding of decreasing N rates and their effect on NUE and crop performance is still crucial for cotton production. In addition to N rates, N forms also directly influence the uptake, accumulation, and distribution of N in cotton plants (Chen et al., 2018). According to Liu et al. (2021), N in the form of urea affects the root growth in cotton, which in turn, influence the N uptake and its accumulation. Similarly, N in the forms of controlled and slow-release fertilizers (such as polymer-based, urease, and nitrification inhibitors) was also reported to enhance N uptake and its accumulation in plants (Antille and Moody, 2021).
In addition to N fertilization, some other agronomic factors such as sowing dates, planting density, and tillage practices also influence the N uptake and NUE in cotton. Sowing time is the most important manageable factor in cotton as it is imperative in determining the lint yield and quality and N uptake in cotton (Khan et al., 2017a,b,c). Planting the crop at the optimum time is imperative not only for greater above and below-ground ground biomass but also for adequate uptake of N (Khan et al., 2017a,b,c). The plant density affects crop growth and development by inflicting interference among plants for space, light, nutrients, and moisture (Stephenson et al., 2011). Since nutrient uptake and plant density are linearly correlated, increasing plant population may lead to enhanced N uptake in reproductive organs. According to Jiang et al. (2013), high plant density imparts more N uptake and N translocation from vegetative parts to reproductive tissues. Furthermore, tillage also influences the mineralization and later release of nutrients from the soil (Khan et al., 2017a,b,c). Among commonly used tillage practices, zero tillage (ZT) retains more crop residues which leads to a reduction in soil erosion and evaporation and an increase in NUE compared to conventional tillage (CT; Lal et al., 2007). Also, it has been stated that ZT recorded more N uptake by cotton as compared to the CT.
In recent years, various molecular approaches are being discussed for enhancing N uptake and NUE in cotton crops. Among these, traditional phenotyping techniques are time-consuming, labor-intensive, expensive, and provide low-throughput and non-reliable phenotypes, impeding cotton breeding (Furbank and Tester, 2011). However, modern-day’s automated phenomic approaches including metabolic and transcriptomic approaches, are precise, time-saving, and high-throughput and can circumvent the limitations of conventional breeding (Singhal et al., 2021). These approaches are being utilized for the effective identification of high-yielding cultivars with additional superior qualities that might be used in the genetic modification of cotton. Likewsie, precision N use, N metabolism, growth attributes, N modelling, N-scheduling, high responsive genotypes and genes related to N uptake and transporters can help in improving NUE. A number of plant growth regulators such as auxin (root growth), cytokinin (delayed senescence), gibberellic acid (stem elongation), and ABA (stress tolerance) crucial for cotton growth and optimum use of these PGRs can enhance NUE and growth. Morover, the genes related to N metabolism, uptake and transport in cotton is crucial for targeting the several biosynthetic pathways, and could enhance the NUE (Yang et al., 2014; Sabagh et al., 2021). In this review-based study, we discussed different agronomic-and environmental-related factors affecting N uptake, its accumulation in plants, and ultimately NUE in cotton. Furthermore, we also discussed various agronomic and molecular approaches to enhance efficiency for more N uptake and its utilization in cotton. The mechanism of N uptake, transport and accumulation in cotton further help in balanced N management and improving NUE.
Nitrogen accumulation in cotton crops is heavily dependent on crop growth phase, N application rates, N sources, plant species, and soil and environmental conditions (Yang et al., 2013). Nitrogen forms directly influence the accumulation and distribution of N in cotton plants, by affecting the root metabolism (Chen et al., 2018). For example, a recent study by Liu et al. (2021) stated that N when applied in the form of urea affects the root growth in cotton, which in turn, influence the N uptake and its accumulation. Similarly, N application in the forms of controlled and slow-release fertilizers such as polymer-based, urease, and nitrification inhibitors also reported enhancing N uptake and its accumulation in plants (Antille and Moody, 2021). Nitrogen supply from enhanced-efficiency nitrogenous fertilizers, such as 3,4-Dimethylpyrazole phosphate, has been well recognized to reduce N losses and enhance its efficiency in crops including cotton (Suter et al., 2020; de Paulo et al., 2021; Qiao et al., 2021). Recently, nano-fertilizers with unique nano properties (1–100 nm size of the particle) have gained popularity due to their higher nutrient use efficiency (Suppan, 2017). Sohair et al. (2018) reported the enhanced yield, yield components, and fiber properties of Egyptian cotton by nano-fertilizer applications.
N application rates also influence N uptake and its accumulation in plants. It is generally reported that increased N rates can increase the productivity of the cotton crop. For cotton crops, N application between 0 and 600 kg ha−1 is reported to positively influence the N accumulation, and reduce leaching and runoff losses (Yang et al., 2013). Normally, the cotton crop grows well when N is applied in the range of 50–412 kg ha−1 (Zurweller et al., 2019). In a recent field study, Wang et al. (2021a,b,c,d) recorded the highest N accumulation in cotton plants when N was applied at 300 kg ha−1. Furthermore, Hou et al. (2021) recorded the highest nitrogen accumulation in cotton when N was applied at 223.88 and 242.77 kg ha−1. However, excessive application of N causes soil and environmental pollution, degrading soil biological activities, nutrient imbalance, impairs growth, and ultimately crop productivity in terms of reduced yield and NUE (Ju et al., 2009; Liu et al., 2013; Chen et al., 2021). According to Macdonald et al. (2016), a high dose of N also causes leaching and runoff losses owing to reducing the availability of N in the rhizosphere. In addition, a deficiency of N reduced root growth (Chen et al., 2021). The root system in cotton directly influences the N use efficiency, which can be increased by regulating the root system (Chen et al., 2018, 2021). Nitrogen stress in cotton reduced the root growth by regulating cell wall growth, reducing cellular polysaccharide and glucan levels, increasing lignin contents, and expression of various genes involved in cell wall components such as EXPA17, EXLA1, LAC4, and NAC081 (Chen et al., 2021). Nitrogen application rates significantly influence the root structure, and moderate N application rates increase cotton root length and promote coordinates of the root-shoot relationship (Chen et al., 2020). Nitrogen stress also inhibits the carriage of auxin and increases lateral root elongation (Krouk et al., 2010). The change in the level of abscisic acid (ABA) and salicylic acid (SA) in lateral roots under varying N application rates were reported by Chen et al. (2021). Also, SA suppresses root development while promoting the growth of lateral plant roots and root primordium (Chen et al., 2021). Reduced root length in cotton was also associated with the activities of SA, JA, and ABA.
In cotton, maximum N uptake largely occurred during 49–71 days after sowing, and the most quantity of N is accumulated during late growth stages, i.e., flowering and boll setting stages (Boquet and Breitenbeck, 2000; Yang et al., 2013). However, published reports have reported variable periods for N absorption in cotton crops. For example, Zhao et al. (2010) stated that cotton crop uptake maximum N between the period of 54–110 days after planting. Similarly, according to Wang et al. (2003), maximum N absorption takes place 90 days after planting. It is well stated that late N application is more beneficial to increase NUE and more N accumulation at the fruit developing stage in cotton crops (Tang et al., 2012). Basal N application in cotton is not recommended because this method losses 80% of applied N under field conditions, as compared to topdressing in which only 40% of losses are documented (Yang et al., 2013). In the same study, Yang et al. (2013) reported that maximum N accumulated at the reproductive stage in cotton because seeds are a major constituent of N than other organs. Recently, Che et al. (2021) recorded the highest N uptake and its accumulation when N was applied at 375 kg ha−1 at the flower boll stage in cotton under full irrigation supply.
N application rates and time of application, soil and environmental conditions, crop species, and other management practices influenced the N distribution in different plant organs. Plant roots play an essential role in N uptake and its transport (Bertoni, 2012). Different N forms and concentrations, soil properties, and root architecture can influence the N transport into the plant system (Khan et al., 2017a,b,c). Roots, which consume energy, mainly uptake N in the form of nitrate which is among the major forms of N present in the soil (Hu et al., 2014). Plants can uptake nitrate mainly through two systems/infinities: low-and high-affinity transporter systems by involving different transporter proteins such as nitrate transporters (NRT1 and NRT2) and peptides transporter proteins (Nacry et al., 2013; Wang et al., 2018). These proteins are activated through various genes such as putative NRT1/PTR genes including NRT1.4 and AtNRT1:4 in model Arabidopsis plants and different NPF genes in crop plants (Chiu et al., 2004; Tsay et al., 2007; Von Wittgenstein et al., 2014). Following uptake by plant roots, NO3-is reduced to NO2-by the nitrate reductase (NR) enzyme, and this NO2-is further reduced to NH4+ by the glutamate synthetase (GS) enzyme (North et al., 2009; Mokhele et al., 2012; Wang et al., 2018). Ammonia, plays an essential role in nitrogen metabolism, assimilated in cotton by the negotiation of different enzymes such as glutamine synthetase and glutamine oxoglutarate aminotransferase (Khan et al., 2017a,b,c). Most soil uptake nitrogen is assembled in vacuoles where it is reserved as an osmotic ion and then this nitrogen is let out in the cytosol for reduction. During the process of photorespiration in plants, nitrogen, in the form of glycine, is distributed for re-assimilation. At the reproductive stage, leaves can remobilize the nitrogen and transfer it to the reproductive plant parts through the hydrolysis of nitrogenous compounds including proteins (Khan et al., 2017a,b,c). The process of nitrogen metabolism is take place through different enzymes such as nitrate reductase (NR), nitrite reductase, and glutamate synthase (Ahmad and Abdin, 1999; Mokhele et al., 2012). Various transporters including AtNRT1.5 and AtNRT1.8 actively participated during the loading and unloading of nitrate from shoot vasculature/ root stele (Lin et al., 2008). According to Wang and Tsay (2011), another transporter AtNRT1.9 is involved during the loading of nitrate ions into the root phloem and facilitates in downward transport of nitrate in roots. The components, i.e., N metabolism, utilization, and uptake, related to NUE in cotton plants are schematically highlighted in Figure 1.
Figure 1. Major components involved in nitrogen use efficiency (NUE) in cotton plant. The nitrogen metabolism, uptake and utilization are crucial mechanisms involved in enhancing NUE in cotton. The activation of N transporters, plant growth indices, and N metabolizing pathways involved in facilitating N metabolism, uptake and utilization are highlighted.
Sowing time is considered to be the most important manageable factor in the cotton crop. It is an imperative factor in determining the yield and quality of lint as well as N uptake in cotton crops. Planting the crop at the optimal period is a must for higher above-and below-ground growth and appropriate N uptake. Sowing time greatly affects N status in cotton leaves, which is positively correlated with canopy photosynthetic ability (Khan et al., 2017a,b,c). Synchronization of crop N demands with its supply is significant for enhancing crop NUE. The requirement of N for a crop is largely associated to yield potential, which in turn is related to N supply during sowing time as well as later stages of crop growth (Yousaf et al., 2016). It has been reported that higher lint yield owing to early sowing was mainly attributed to adequate N uptake led to better boll formation, similarly, lint yield declined with late sowing due to the less time available to mature bolls (Bange et al., 2008). Sowing date also influences the availability, accumulation, distribution, and utilization of N (Yadvinder-Singh et al., 2005). Venugopalan et al. (2012) reported that N uptake and utilization efficiency were reduced in late sowing over the normal planting time of the Bt cotton.
The plant density affects crop growth and development by inflicting interference among plants for space, light, nutrients, and moisture (Stephenson et al., 2011). Since nutrient uptake and plant density are linearly correlated, increasing plant population may lead to enhanced N uptake in reproductive organs. High plant density imparts more N uptake and N translocation from vegetative parts to reproductive tissues (Jiang et al., 2013). It has been reported that cotton planted at 15 cm plant spacing resulted in higher N uptake over cotton planted at 22, 30, 37, and 45 cm spacing in the Multan region of Pakistan (Zaman et al., 2021). Similarly, Panhwar et al. (2018) also reported that close plant spacing in cotton led to higher leaf area, lint yield, chlorophyll contents, and N uptake. Further, investigations revealed that the highest N contents in cotton were recorded under high-density planting (12 plants/m2) and minimum N content was found under low-density planting (8 plants/m2) in the Wuhan region of China (Shah et al., 2021a,b). Plants with higher plant density benefited from enhanced N uptake during initial to peak flowering. Despite an accelerated rate of nutrient accumulation per unit area, plants with higher plant density might have accumulated lesser nutrients on a per plant basis (Xue et al., 2013). The N recovery in cotton was recorded the highest with narrow plant spacing as compared to wider plant spacing. This might be due to more exploration of soil under the close row and plant spacing (Venugopalan and Blaise, 2001).
Cotton is a long-duration crop that requires an adequate quantity of nutrients to be uptaken for successful growth and yield. The application of nutrients also not only affects the yield of cotton but also the uptake of N and NUE (Prasad, 2019). It has been reported that plant N uptake over the entire growth period of cotton improved with the increasing N application dose. However, agronomic efficiency and recovery efficiency of N declined with the increasing N application dose (Pengcheng et al., 2017). In Syria, it has been observed that maximum N uptake (354 kg N ha−1) in cotton was recorded with the application of 200 kg N ha−1. Further increase in the application dose of N diminished the N uptake of cotton, suggesting that increasing N input up to 200 kg N ha−1 led to an increase in N uptake (Janat, 2008). In China also the total N uptake in cotton increased with the N application rates. The total N uptake in N-applied cotton was about 46% greater than the cotton without N application (Chen et al., 2010). Similarly, in the Punjab region of Pakistan, application of N at 180 kg N ha−1 showed higher uptake of N in cotton as compared to 120 kg N ha−1 (Shah et al., 2021a,b).
It has been reported that supplementation of plant growth regulators in cotton crops improves the antioxidant defense systems, water use efficiency, nutrient availability, and uptake (Noreen et al., 2020). The application of humic acid imparts in releasing blocked or occluded phosphorus from soil collides and thus, increases its availability which improves N uptake and utilization, ultimately enhancing cotton yield (Khan et al., 2017a,b,c). Likewise, K+ content in cotton leaf and root increased by 20%–34% and 2%–8% respectively, due to the application of sodium nitroprusside at 300 μM (Kong et al., 2016). Application of salicylic acid at 100 μM to cotton seedlings for 3 days significantly improved Cu accumulation in roots, while reducing its translocation to aboveground parts (Mei et al., 2015). Supplementation of Brassinosteroids or putrescine to cotton plants resulted in enhanced uptake of N, P, and K compared to untreated plants (Ahmed et al., 2016). The application of Mepiquat chloride and Miantaijin increased the lint yield and K uptake in cotton in China. The plant growth regulators also improved the partial factor productivity, agronomic efficiency, and apparent recovery efficiency of K fertilizer (Yang et al., 2014).
Tillage is an important practice to obtain a good crop yield from agricultural land. Crop productivity depends on the nutrient applied or present in the soil. Tillage influences the mineralization and later release of nutrients from the soil. It has been demonstrated that zero tillage (ZT) retains crop residues which leads to a reduction in soil erosion and evaporation and an increase in NUE compared to conventional tillage (CT; Lal et al., 2007). It has been revealed that uptake of N by cotton was recorded significantly highest in ZT as compared to the CT. The adequate moisture content in ZT helped to restore high N in soil which led to improved uptake by cotton (Khan et al., 2021). Similarly, the highest uptake of N, P, and K in cotton was recorded in ZT as compared to CT in the Akola region of Maharashtra (Wagh et al., 2016). ZT can also influence the amounts of supplemental N needed to secure optimal cotton yields. Bronson et al. (2001) reported that 20–35 kg ha−1 additional N was required under ZT than with CT. It has been well explained that tillage incorporates crop residue and increases soil aeration, which serves to speed up the decomposition of both residue and existing organic matter and thereby enhance the release of N (Boquet et al., 2004). In the Nagpur region of Maharashtra cotton yield and partial factor productivity improved significantly due to the adoption of ZT. This was mainly due to a better soil hydro-thermal regime and improved weed control (Blaise, 2006).
A sustainable cropping system always maintains a balance between the input and output of N by imparting adequate uptake and a minimum loss from soil (Herridge et al., 2008). Investigations from the 2 years rotation of cotton–soybean resulted in higher productivity and NUE as compared to the cultivation of sole cotton (Blaise, 2011). The introduction of short-duration wheat varieties resulted in an intensification of the cotton-wheat cropping system by including legume crops in the rotation. Legumes can fix atmospheric N in considerable amounts in their root nodules and the integration of legumes in the existing cropping systems could minimize the N requirement of the system (Blaise, 2017). It has been demonstrated that legumes could facilitate the growth and productivity of non-legume crops through nutrient sharing as legumes not only fix atmospheric N for their consumption (Sitienei et al., 2017) but also for crops grown in succession (Hu et al., 2017). It has been reported that intercropping of cotton with mungbean enhanced the total N uptake, NUE, and PFP for N by 28%–45%, 27%–44%, and 16%–19% as compared to sole planting of cotton in northwest China (Liang et al., 2020). In the Indo-Gangetic plains of India intercropping of cowpea with cotton had higher production efficiency, N uptake, and NUE compared to sole cotton (Rajpoot et al., 2014).
Soil salinity is one of the abiotic factors affecting the growth, development, and photosynthesis in plants and is greatly correlated with osmotic stress, nutritional imbalance, and specific ion toxicity (Nazar et al., 2011). Nutrient uptake is drastically reduced under soil salinity stress, and it may inhibit nutrient translocation and eventually lead to a yield penalty (Pessarakli, 2001). It is well known that sodic or alkali soil contains huge amounts of carbonates and bicarbonates of Na+. It has been demonstrated that growing cotton in sodic soils resulted in less dry matter production as compared to cotton growing in non-sodic soils (Dodd et al., 2010). Inhibition of nutrient uptake and growth of cotton in sodic soils are largely related to the poor physical condition or soil structure of these soils, due to the huge content of Na+ in the soil solution (Dodd et al., 2010). Soil salinity is the biggest hurdle for seed germination and crop emergence. Cultivation of cotton in saline soils leads to reduced leaf area and poor seed germination (Dong, 2012). It has been reported that application of silicon fertilizer application could mitigate soil salinity stress and improve crop yield (Jinger et al., 2020).
Failure of monsoon or paucity of water leads to drought stress which affects the growth and yield of the crops (Ahmad et al., 2021; Ali et al., 2021; Bahar et al., 2021; Chowdhary et al., 2021; Islam et al., 2021; Mahmood et al., 2021; Ahmad et al., 2022a,b; Akhtar et al., 2022). Reduced availability of moisture during the crop growing season is the major factor responsible for poor crop productivity (Noman et al., 2017). Photosynthetic and respiratory activities of crops are severely inhibited due to drought stress (Alghabari et al., 2016). Drought stress also plays a pivotal role in the poor mineral nutrition of plants since most of the plant nutrients are translocated along with water (Khawar et al., 2016). In open-field situations, the application of nutrients during drought conditions has no impact on crop growth since nutrients need adequate soil moisture to improve yield. A low concentration of K+ ions directly causes membrane damage and deformation of ionic homeostasis. Additionally, moisture scarcity affects the physiology of nutrients in plants and can retard the activity of nitrate reductase and glutamine synthetase associated with the assimilation of NH4+ to proteins (Rizhysky et al., 2004). In general, N uptake is improved, P uptake is reduced, and K remains unaffected during drought stress. Nevertheless, nutrient relations turn out to be more convoluted owing to interaction effects among different nutrients (Fahad et al., 2017).
Excess moisture also arrests the nutrient absorption, photosynthesis, growth, and reproductive development of cotton crops which ultimately results in loss of crop yield (Najeeb et al., 2015a). Waterlogging creates an anoxia situation that could be one of the imperative aspects accountable for yield penalty in cotton due to the production of ethylene in plant tissue under excess water conditions (Najeeb et al., 2015b). Inadequacy of particular nutrients in cotton leaves could lead to a decline in chlorophyll and photosynthetic activity. The application of particular nutrients could enhance photosynthesis and chlorophyll content (Ashraf et al., 2011). On the contrary, Zhou and Oosterhuis (2012) reported that the application of specific nutrients could not improve the photosynthetic activity of cotton crops facing waterlogged conditions and they have revealed that apart from nutrients inadequacy other factors are also accountable for reduced photosynthesis and leaf necrosis Waterlogging hindered cotton morphological, physiological, and biochemical processes (Armstrong and Drew, 2002). Excess moisture diminishes root growth, reduces H+-ATPase activity of plasma membrane, impedes nutrient acquisition, and triggers the activity of 1-aminocyclopropene-1-carboxlic acid in root tissues of cotton (Leblanc et al., 2008).
Temperature is one of the most important factors for agriculture production and is intimately connected (Reddy et al., 2017; Afzal et al., 2020; Ali et al., 2021; Haider et al., 2021). The temperature fluctuates or varies spatially and temporally over the cotton cultivating regions. The growth and productivity of cotton are tremendously decreased at low temperatures, especially during seed germination and crop emergence (Ashraf, 2002). Cotton crops experienced high temperatures lead to reducing the concentration of nutrients in different plant parts, though impacts may vary among nutrients and crop species (Shakoor et al., 2017). High temperature retards the production of antioxidants which accelerate the genesis of reactive oxygen species and thus imparts oxidative stress and nutrient deficiency in cotton (Sarwar et al., 2017). The activity of nitrate reductase involved in nutrient metabolism is also significantly diminished during heat stress (Hungria and Kaschuk, 2014). Under heat stress root density, root mass, and root volume is impaired which ultimately led to a reduction in total nutrient uptake (Basirirad, 2000).
Sunlight is an indispensable factor for photosynthesis in crop plants. Inadequacy of sunlight leads to impairment in the growth and development of crop plants. Cotton canopy experiencing low light has lower photosynthetic saturation compared to cotton canopy getting optimum light (Landivar et al., 2010). Very less information is available on the impact of light on nutrient uptake in cotton. However, some past studies have reported that total N P, K, S, Ca, and Mg concentrations in the cotton leaf blades increased by 19%, 29%, 22%, 22%, 13%, and 16%, respectively, in shaded plants compared with those of receiving optimum light (Zhao and Oosterhuis, 1998). The impact of different abiotic stresses on cotton morphological and yield attributes are presented in Table 1.
Table 1. Impact of different abiotic stresses on the morpho-physiological traits and yield of cotton.
Traditional phenotyping is time-consuming, labor-intensive, expensive, and provides low-throughput and non-reliable phenotypes, impeding cotton breeding (Furbank and Tester, 2011). However, the modern day’s automated phenomic approaches are precise, time-saving, and high-throughput and can circumvent the limitations of conventional breeding. These approaches are being utilized for the effective identification of high-yielding cultivars with additional superior qualities that might be used in the genetic modification of cotton. The advanced breeding, biotechnological, physiological, agronomical, and omics approaches for NUE enhancement in the cotton crop are highlighted in Figure 2.
Figure 2. Highlights the physiological, biochemical, biotechnological, agronomical, breeding and omics approaches for enhancing NUE in cotton.
Understanding the exact plant architecture is very crucial for improving cotton yield. For example, Paproki et al. (2011) applied a top-down approach for smart segmentation of cotton for automated analysis of phenotypic data to understand the architecture of the plant and further improvement of the 3D plant reconstruction in cotton. However, as cotton plants differ in shape, no single method is robust enough to offer correct segmentation in all circumstances.
Plant productivity may well be determined by understanding of plant growth dynamics. Plant growth modeling and functional analysis using a high-throughput phenotyping platform give a better understanding of energy distribution in the plant. Jiang et al. (2018) developed the ‘GPhenoVision’ system for evaluating the usefulness of the field-based high throughput phenotyping using plant growth and fiber yield traits for futuristic breeding programs and genomics studies in cotton. Another high throughput terrestrial LiDAR-based phenotyping system was developed by Sun et al. (2018) for accurate screening and understanding of growth trends and growth rate based on canopy height, projected canopy area, and plant volume of cotton plants through 3D surface modeling. Recently, CSM-CROPGRO model was used in cotton to evaluate the growth and development of the plant under varying nitrogen doses and planting dates (Ur Rahman et al., 2019).
Phenomic approaches have been used in several crops for precise identification of phenotypes with high agronomic and economic importance by using advanced crop sensing platforms and data analysis software. Thorp et al. (2015) investigated proximal hyperspectral sensing with a field for estimating leaf water content, specific leaf mass, leaf chlorophyll content, and leaf area index which could be utilized in cotton improvement. Phenomics data in association with a genome-wide association study (GWAS) on 56 morphological and 63 texture traits identified the 390 genetic loci in cotton (Li et al., 2020).
Adaptation to environmental stresses by a cotton plant directly determines its growth and yield. Understanding the accurate phenotypic and genotypic relationship makes it easier identification of stress-tolerant lines. Drought resistance is a complex trait governed by multiple genes. Li et al. (2020) through phenomics (using novel image-based indicators) and genomics (using Genome-Wide Association Studies) approach identified Gh_A04G0377 and Gh_A04G0378 candidate genes negatively regulating the drought resistance in cotton. The physiological, biochemical, and molecular mechanisms related to abiotic stress tolerance in cotton are highlighted in Figure 3.
Figure 3. Highlights the role of different transcription factors, genes, and functional traits in enhancing tolerance to drought, salinity, temperature, and waterlogging stresses.
Since nitrogen is a key ingredient of macromolecules such as proteins, enzymes, metabolites, signaling molecules, and plant hormones, it is one of the most significant factors limiting global cotton production. Metabolomics sheds light on the fundamental mechanisms of nitrogen absorption and assimilation in the plant, which ultimately determine its growth performance and productivity. Iqbal et al. (2020) through metabolic and transcriptomic approaches elucidated the major biosynthetic pathways and transcripts involved in Carbon and Nitrogen metabolism in cotton. Increased accumulation of 2-ketoglutarate, succinate, malate, etc. in the Ligon lintless-2 cotton mutant also indicated a higher nitrogen compound metabolism (Naoumkina et al., 2013).
N assimilation is associated with carbon metabolism and subsequent biomass yield. Carbon is metabolized into cellulose fibers in cotton. The first comprehensive study on changes in metabolites and associated transcripts involved in elongating fibers in Ligon lintless-2 mutant was reported by Naoumkina et al. (2013). They highlighted the number of factors and metabolic mechanisms involved in cotton fiber elongation. Similarly, Tuttle et al. (2015) have given a deep insight of cell wall transitions to secondary wall synthesis using deep-sequencing transcriptomics and non-targeted metabolomics approaches. They identified 206 fiber metabolites and 38,000 fiber-related transcripts and implicated that lignification is transcriptionally repressed during secondary cell wall synthesis in cotton. The roles of sterol and sphingolipid derivatives in the initiation of fiber cells have been identified through comparative metabolomics analysis in cotton (Wang et al., 2021a,b,c,d). Brown cotton fiber is a one-of-a-kind raw material made from naturally pigmented cotton. In a recent study, Wang et al. (2021a,b,c,d) elucidated the transcriptional regulatory network of proanthocyanidins biosynthesis and flavonoid metabolism in brown fiber variety (Zong 1–61) and white near-isogenic lines (RT) of cotton. They detected 10,891 differentially expressed genes about the brown pigmentation in cotton.
Various studies have reported stress-related metabolism in cotton crops. For example, Khizar et al. (2020) identified 241 Aspergillus leaf spot resistance-related metabolites belonging to phenylpropanoids, flavonoids, alkaloids, terpenoids, etc. through non-targeted metabolomics in cotton. Cotton yield is influenced by abiotic stress, particularly drought stress. Recently Zhang et al. (2021) identified a total of 537 metabolites (ABA, amino acids, Flavonoids, ROS, etc.) were detected at different stages of cotton cultivars, i.e., XLZ8 (sensitive) and G95079 (tolerant) under drought stress.
The assimilation of nitrogen is generally associated with photosynthetic efficiency and carbon content in the plant which is controlled by the mechanism involved in carbon-nitrogen balance (Ranathunge et al., 2014). This carbon-nitrogen ratio plays a crucial role in the proper growth and development of plants (Zheng, 2009). Various reports have demonstrated that the well-organized systemic pathways are involved in C and N metabolism that have a major impact on the growth yield and productivity of plants (Krapp and Traong, 2006). In this review information regarding gene regulation for N metabolism and its effect on plant growth and development, yield, productivity, and C and N metabolism has been taken into consideration (Li et al., 2016a,b). Furthermore, the carbon and nitrogen ratio plays a pivotal role in improving NUE, as it is essential for a plant to assimilate maximum carbon which enhances the plant’s capacity for nitrogen uptake and utilization. Similarly N content in plants can have a major impact on the carbon fixation metabolism of plants (Li et al., 2017), as N is required by plant in ample amount for production of photosynthetic enzymes, for example, Rubisco and PEP carboxylase. Decreased N accumulation and storage results in low carbon fixation in a plant (Li et al., 2017). Besides the carbon to nitrogen ratio, the outcome products of the GS and GOGAT cycles are also important for plants. Glutamate helps in nitrogen transportation, acts as a singling molecule, and also it provides a substrate for the synthesis of amino acids (Inthapanya et al., 2000). It also controls and regulates C and N pathways and ratios in plants. Enhanced expression of PEP carboxylase in plants resulted in increased glutamine synthesis (Inthapanya et al., 2000). The alterations in the relationship of source or sink in plant coordinated with proportionate changes in carbon or nitrogen ratio must be associated with environmental stimulants which include factors like nitrogen availability (Harvey, 1939). Although C-to-N ratios are responsible for proper plant growth and development, it also acts as a limiting factor while examining Nitrogen use efficiency in plants (Koutroubas and Ntanos, 2003). Nitrogen uptake, accumulation, and remobilization are greatly influenced by carbon metabolism, thus affecting NUE unless there is an increase in carbon content which is also confirmed by RNA sequencing studies conducted in cotton.
Furthermore, significant alterations were also observed in the metabolic pathways of different amino acids which include phenylalanine, tryptophan, tyrosine cysteine, methionine metabolism, alanine, glutamate, aspartate, glycine, serine and threonine, and “starch and sucrose metabolism” similarly glycolysis – gluconeogenesis also acts as an important factor in carbon metabolism which was further restored and was highly expressed in the shoot of CCRI-69 when N was supplied after a certain interval of time within 0–6 h. Furthermore, 4 DEGs associated with pentose phosphate shunt were highly expressed in the shoot of CCRI-69.
NUE amelioration is considered to be a crucial challenge in a cotton crop that has to be overcome. Plenty of research work has been done to improve cotton NUE that mainly focused on crop cultivation techniques (Zhang et al., 2018) and now it has been directed towards NUE evaluation of cotton varieties and their application in breeding programs (Iqbal et al., 2020). These strategies adopted to increase NUE in the cotton crop are time-consuming, laborious, and costly so alternative approaches are being utilized. Therefore, the transgenic methodology is now preferred to enhance agronomic traits and has received great success in cotton. The success of Bt cotton for insect resistance (Zafar et al., 2020) is a great example of a transgenic crop. Therefore, determination and genetic alteration of major NUE-efficient genes become a crucial step towards improving cotton NUE.
It has been reported by experiment that Arabidopsis NLP7 gene integration in cotton helps to increase NUE. The AtNLP7 over-expressed genes lines in cotton exhibited significantly better growth in seedlings under different N levels with improved seedling biomass, wider leaf, and healthy roots, which was directly useful during the seedling establishment process. The field trial indicated that higher expression of AtNLP7 greatly improved both cotton NUE and yield. It was also observed that AtNLP7 genes are also related to N uptake and assimilation in cotton. Its integration into the genome of cotton resulted in increased uptake of N uptake and a higher assimilation rate therefore useful in imparting cotton with high NUE and a better yield (Jan et al., 2022).
Several studies provide evidence that there are in general 60, 61, and 105 NLP genes in G. arboreum, G. raimondii, and G. hirsutum, approximately (Magwanga et al., 2019). BLAST results were evident to prove that there are 7 orthologs of AtNLP7 that are found in cotton. A list of these important 7 genes includes GhNLP2, 4, 5, 6, 7, 8, and GhNLP9. furthermore, the NREs sequence in the promoter (2 kb) of these cotton genes is identical to those observed in the case of Arabidopsis which indicates that AtNLP7 could be responsible for activating the up-regulation of cotton genes by associating with NRE. Similarly, various regulatory sequences have been discovered that are useful in improving NUE, e.g., SNF1-related kinase in tomato (Wang et al., 2012), bHLH T.F in soybean (Chiasson et al., 2014), NAC T.F present in wheat (Borrill et al., 2017). These genes can be successfully used to develop transgenic cotton with enhanced NUE.
Fixation of N and C are regulated in association, which is the indication that NUE, along with N accumulations, also affects C metabolism (Chardon et al., 2012). Thus, therefore it is evident enough to prove that optimization in plant growth, development, and yield includes concurrent amelioration in both N and C use efficiencies. It was observed in research conducted by Yu et al., 2016 that association among C and N accumulation resulted in higher storage of C and N in transgenic cotton lines where AtNLP7 gene was highly expressed. The high expression level of AtNLP7 resulted in higher chlorophyll in transgenic cotton which may have a direct effect on the C-fixation ability of cotton (Yu et al., 2016). A proper N/C ratio is highly useful in increasing NUE.
The AtNLP7-increased NUE in the cotton crop was further assisted by the data of total N and C content and two biochemical metabolite markers chiefly glutamate and glutamine for nitrogen accumulation and utilization. High levels of nitrogen and carbon content were observed in transgenic cotton as compared with non-transgenic cotton. The net glutamate contents of both non-transgenic and transgenic plants were higher at a higher level of KNO3. On the other hand, glutamine (Gln) contents increased exceptionally in transgenic cotton than in control while net Gln quantity increased on high supply of N as compared with lower quantity of N. These results show that cotton having enhanced expression of AtNLP7 gene will improve nitrogen use efficiency in cotton and provide an alternative approach as compared to breeding and agronomic methodologies (Jan et al., 2022).
These data can support by the expression studies of nitrogen metabolism marker genes which include GhNRT1.1, GhNRT1.2, GhNRT2.1, GhNRT2.2, GhNIA1, GhAMT1, GhAMT2, and GhGST1.1, which were expressed in transgenic lines shows that the activity of AtNLP7 gene is conserved in cotton crop (Jan et al., 2022). Some genes related to NUE in cotton plants are highlighted in Table 2.
Cotton is a major source of high-quality natural fiber for textile industries globally however, its production is directly associated with the NUE, which relies on the management practices like source, rate, time, and mode of N administration, growth stage, and genotypic constitution. Excess N dose reduction, timely application, and gradual and controlled N release may be beneficial for improved NUE in cotton. A mulidimension approach to enhance NUE in cotton crop highlighted in Figure 4. Further, the usage of N efficient genotypes can enhance NUE and cotton yield while reducing the possibility of excess N wastage, and environmental pollution simultaneously. Furthermore, overexpression of various target genes like Arabidopsis NLP, NLP7, and AtNLP7 can be extremely useful in increasing NUE, plant growth, and fiber yield in cotton even under poor N availability. Although several conventional studies on manipulating single or multiple genes to improve NUE have been reported in a variety of crops, the use of modern days advanced genomic tools like whole genome sequencing and omics could be the thrust areas for futuristic research in cotton. In addition, the use of wild genotypes may be an appropriate alternative to improve NUE by retaining the resistance against major pests and diseases in cotton.
Figure 4. Highlights the multidimensional approaches (agronomical, biochemical and molecular) to enhance NUE in cotton plant.
ANS, TJ, RKS, RS, DW, SH, HA, DJ, HP, NRA, RYG, and MJ together designed the scope of the manuscript, wrote individual chapters, and prepared the figures. All authors contributed to the article and approved the submitted version.
The authors thank Smart Health Initiative and Red Sea Research Center, Division of Biological and Environmental Sciences and Engineering, King Abdullah University of Science and Technology, Thuwal, Saudi Arabia for financially supporting the current research. The authors also extend their appreciation to Alexandria University for supporting the current research.
The authors declare that the research was conducted in the absence of any commercial or financial relationships that could be construed as a potential conflict of interest.
All claims expressed in this article are solely those of the authors and do not necessarily represent those of their affiliated organizations, or those of the publisher, the editors and the reviewers. Any product that may be evaluated in this article, or claim that may be made by its manufacturer, is not guaranteed or endorsed by the publisher.
Afzal, I., Imran, S., Javed, T., and Basra, S. M. A. (2020). Evaluating the integrative response of moringa leaf extract with synthetic growth promoting substances in maize under early spring conditions. S. Afr. J. Bot. 132, 378–387.
Ahmad, A., and Abdin, M. Z. (1999). NADH: nitrate reductase and NAD(P)H: nitrate reductase activities in mustard seedlings. Plant Sci. 143, 1–8. doi: 10.1016/S0168-9452(99)00016-3
Ahmad, A., Aslam, Z., Hussain, S., Javed, T., Hussain, S., Bashir, S., et al. (2022a).Soil application of wheat straw vermicompost enhances morpho-physiological attributes and antioxidant defense in wheat under drought stress. Front. Environ. Sci. 387.
Ahmad, A., Aslam, Z., Javed, T., Hussain, S., Raza, A., Shabbir, R., et al. (2022b). Screening of wheat (Triticum aestivum L.) genotypes for drought tolerance through agronomic and physiological response. Agronomy 12:287.
Ahmad, A., Aslam, Z., Naz, M., Hussain, S., Javed, T., Aslam, S., et al. (2021). Exogenous salicylic acid-induced drought stress tolerance in wheat (Triticum aestivum L.) grown under hydroponic culture. PloS one 16:e0260556.
Ahmed, H., Darwish, E., and Alobaidy, M. G. (2016). Impact of putrescine and 24-epibrassinolide on growth, yield and chemical constituents of cotton (Gossypium barbadense L.) plant grown under drought stress conditions. Asian J. Plant Sci. 16, 9–23. doi: 10.3923/ajps.2017.9.23
Ahmad, S., Khan, N., Iqbal, M. Z., Hussain, A., and Hassan, M. (2002). Salt tolerance of cotton (Gossypium hirsutum L.). Asian J. Plant Sci. 1, 715–719. doi: 10.3923/ajps.2002.715.719
Akhtar, G., Faried, H. N., Razzaq, K., Ullah, S., Wattoo, F. M., Shehzad, M. A., et al. (2022). Chitosan-induced physiological and biochemical regulations confer drought tolerance in pot marigold (Calendula officinalis L.). Agronomy 12:474.
Alghabari, F., Muhammad, Z. I., Abdul, K., Saddam, H., Ihsanullah, D., Fahad, S., et al. (2016). Gibberellin-sensitive Rht alleles confer tolerance to heat and drought stresses in wheat at booting stage. J. Cereal Sci. 70, 72–78. doi: 10.1016/j.jcs.2016.05.016
Ali, A., Chu, N., Ma, P., Javed, T., Zaheer, U., Huang, M. T., et al. (2021). Genome-wide analysis of mitogen-activated protein (MAP) kinase gene family expression in response to biotic and abiotic stresses in sugarcane. Physiologia plantarum 171, 86–107.
Ali, M. A., Farooq, J., Batool, A., Zahoor, A., Azeem, F., Mahmood, A., et al. (2019). Cotton production in Pakistan. Cotton Prod., 249–276. doi: 10.1002/9781119385523.ch12
Ali, M. M., Waleed Shafique, M., Gull, S., Afzal Naveed, W., Javed, T., Yousef, A. F., et al. (2021). Alleviation of heat stress in tomato by exogenous application of sulfur. Horticulturae 7:21.
Alishah, O., and Ahmadikhah, A. (2009). The effects of drought stress on improved cotton varieties in Golesatn Province of Iran. Int. J. Plant Prod. 3, 17–25.
Antille, D. L., and Moody, P. W. (2021). Nitrogen use efficiency indicators for the Australian cotton, grains, sugar, dairy and horticulture industries. Environ. Sustainability Indic. 10:100099. doi: 10.1016/j.indic.2020.100099
Armstrong, W., and Drew, M. C. (2002). “Root growth and metabolism under oxygen deficiency,” in Plant Roots: The Hidden Half. eds. Y. Waisel, A. Eshel, and U. Kafkaf (New York, NY: Marcel Dekker).
Ashraf, M. (2002). Salt tolerance of cotton: some new advances. Crit. Rev. Plant Sci. 21, 1–30. doi: 10.1016/S0735-2689(02)80036-3
Ashraf, M., Ahmad, A., Qurainy, F., and Ashraf, M. Y. (2011). Alleviation of waterlogging stress in upland cotton (Gossypium hirsutum L.) by exogenous application of potassium in soil and as a foliar spray. Crop Pasture Sci. 62, 25–38. doi: 10.1071/CP09225
Bahar, A. A., Faried, H. N., Razzaq, K., Ullah, S., Akhtar, G., Amin, M., et al. (2021). Potassium-induced drought tolerance of potato by improving morpho-physiological and biochemical attributes. Agronomy 11:2573.
Bange, M. P., Caton, S. J., and Milroy, S. P. (2008). Managing yields of high fruit retention in transgenic cotton (Gossypium hirsutum L.) using sowing date. Aust. J. Agric. Res. 59, 733–741. doi: 10.1071/AR07423
Basirirad, H. (2000). Kinetics of nutrient uptake by roots: responses to global change. New Phytol. 147, 155–169. doi: 10.1046/j.1469-8137.2000.00682.x
Bertoni, G. (2012). A nitrate transporter for both roots and shoots. Plant Cell 24, 1. doi: 10.1105/tpc.112.240110
Blaise, D. (2006). Effect of tillage systems on weed control, yield and fibre quality of upland (Gossypium hirsutum L.) and Asiatic tree cotton (G. arboreum L.). Soil Till. Res. 91, 207–216. doi: 10.1016/j.still.2005.12.005
Blaise, D. (2011). Tillage and green manure effects on Bt transgenic cotton (Gossypium hirsutum L.) hybrid grown on rainfed Vertisols of Central India. Soil Till. Res. 114, 86–96. doi: 10.1016/j.still.2011.04.008
Blaise, D. (2017). “Cotton based cropping systems,” in Enhancing Nutrient Use Efficiency. eds. K. Ramesh, A. K. Biswas, B. Lakaria, S. Srivastava, and A. K. Patra (New Delhi: New India Publishing Agency), 369–384.
Boquet, D. J., and Breitenbeck, G. A. (2000). Nitrogen rate effect on partitioning of nitrogen and dry matter by cotton. Crop Sci. 40, 1685–1693. doi: 10.2135/cropsci2000.4061685x
Boquet, D. J., Hutchinson, R. L., and Breitenbeck, G. A. (2004). Long-term tillage, cover crop, and nitrogen rate effects on cotton: yield and fiber properties. Agron. J. 96, 1436–1442. doi: 10.2134/agronj2004.1436
Borrill, P., Harrington, S. A., and Uauy, C. (2017). Genome-wide sequence and expression analysis of the NAC transcription factor family in polyploid wheat. G3: genes, genomes. Genetics 7, 3019–3029. doi: 10.1534/g3.117.043679
Bronson, K. F., Onken, A. B., Keeling, J. W., Booker, J. D., and Torbert, H. A. (2001). Nitrogen response in cotton as affected by tillage system and irrigation level. Soil Sci. Soc. Am. J. 65, 1153–1163. doi: 10.2136/sssaj2001.6541153x
Chardon, F., Noël, V., and Masclaux-Daubresse, C. (2012). Exploring NUE in crops and in Arabidopsis ideotypes to improve yield and seed quality. J. Exp. Bot. 63, 3401–3412. doi: 10.1093/jxb/err353
Che, Z., Wang, J., and Li, J. (2021). Effects of water quality, irrigation amount and nitrogen applied on soil salinity and cotton production under mulched drip irrigation in arid Northwest China. Agric. Water Manag. 247:106738. doi: 10.1016/j.agwat.2021.106738
Chen, G., Chen, Y., Zhao, G. H., Cheng, W. D., and Guo, S. W. (2015). Do high nitrogen use efficiency rice cultivars reduce nitrogen losses from paddy fields. Agric. Ecosyst. Environ. 209, 26–33. doi: 10.1016/j.agee.2015.03.003
Chen, W., Hou, Z., Wu, L., Liang, Y., and Wei, C. (2010). Effects of salinity and nitrogen on cotton growth in arid environment. Plant Soil 326, 61–73. doi: 10.1007/s11104-008-9881-0
Chen, J., Liu, S. D., Zhang, S. P., Ge, C. W., Shen, Q., Ma, H. J., et al. (2020). Nitrogen modulates cotton root morphology by affecting abscisic acid (ABA) and salicylic acid (SA) content. Arch. Agron. Soil Sci. 67, 1722–1738. doi: 10.1080/03650340.2020.1807518
Chen, Z., Tao, X., Khan, A., Tan, D. K., and Luo, H. (2018). Biomass accumulation, photosynthetic traits and root development of cotton as affected by irrigation and nitrogen-fertilization. Front. Plant Sci. 9: 173. doi: 10.3389/fpls.2018.00173
Chen, J., Wang, Z., Liu, S., Zhang, S., Ge, C., Shen, Q., et al. (2021). Nitrogen stress inhibits root growth by regulating cell wall and hormone changes in cotton (Gossypium hirsutum L.). J. Agron. Crop Sci. 207, 1006–1023. doi: 10.1111/jac.12538
Chen, B. L., Yang, H. K., Song, W. C., Liu, C. Y., Xu, J., Zhao, W. Q., et al. (2016). Effect of N fertilization rate on soil alkalihydrolyzable N, subtending leaf N concentration, fiber yield, and quality of cotton. Crop J. 4, 323–330. doi: 10.1016/j.cj.2016.03.006
Chiasson, D. M., Loughlin, P. C., Mazurkiewicz, D., Mohammadidehcheshmeh, M., Fedorova, E. E., Okamoto, M., et al. (2014). Soybean SAT1 (symbiotic ammonium transporter 1) encodes a bHLH transcription factor involved in nodule growth and NH4+ transport. Proc. Natl. Acad. Sci. 111, 4814–4819. doi: 10.1073/pnas.1312801111
Chiu, C. C., Lin, C. S., Hsia, A. P., Su, R. C., Lin, H. L., and Tsay, Y. F. (2004). Mutation of a nitrate transporter, AtNRT1: 4, results in a reduced petiole nitrate content and altered leaf development. Plant Cell Physiol. 45, 1139–1148. doi: 10.1093/pcp/pch143
Chowdhury, M. K., Hasan, M. A., Bahadur, M. M., Islam, M. R., Hakim, M. A., Iqbal, M. A., et al. (2021). Evaluation of drought tolerance of some wheat (Triticum aestivum L.) genotypes through phenology, growth, and physiological indices. Agronomy 11:1792.
de Paulo, E. N., Galindo, F. S., Rabêlo, F. H. S., Frazão, J. J., and Lavres, J. (2021). Nitrification inhibitor 3, 4-Dimethylpyrazole phosphate improves nitrogen recovery and accumulation in cotton plants by reducing NO3− leaching under 15N-urea fertilization. Plant Soil 469, 259–272. doi: 10.1007/s11104-021-05169-4
Devkota, M., Martius, C., Lamers, J. P. A., Sayre, K. D., Devkota, K. P., and Vlek, P. L. G. (2013). Tillage and nitrogen fertilization effects on yield and nitrogen use efficiency of irrigated cotton. Soil Till Res. 134, 72–82. doi: 10.1016/j.still.2013.07.009
Dodd, K., Guppy, C., Lockwood, P., and Rochester, I. (2010). The effect of sodicity on cotton plant response to solutions containing high sodium concentrations. J. Plant Soil 330, 239–249. doi: 10.1007/s11104-009-0196-6
Dong, H. (2012). Technology and field management for controlling soil salinity effects on cotton. Aust. J. Crop. Sci. 6, 333–341.
Dong, H. Z., Li, W. J., Enejia, A. E., and Zhang, D. M. (2012). Nitrogen rate and plant density effects on yield and late-season leaf senescence of cotton raised on a saline field. Field Crop Res. 126, 137–144. doi: 10.1016/j.fcr.2011.10.005
Fahad, S., Bajwa, A. A., Nazir, U., Anjum, S. A., Farooq, A., Zohaib, A., et al. (2017). Crop production under drought and heat stress: plant responses and management options. Front. Plant Sci. 8:1147. doi: 10.3389/fpls.2017.01147
Feng, L., Tang, G., Xu, W., Gu, M., and Geng, Z. (2020). Biochar effect-mediated ammonium transporter genes and GDH may be the core of improvement nitrogen assimilation in cotton seedling. bioRxiv doi: 10.1101/2020.02.22.960997
Furbank, R. T., and Tester, M. (2011). Phenomics–technologies to relieve the phenotyping bottleneck. Trends Plant Sci. 16, 635–644. doi: 10.1016/j.tplants.2011.09.005
Guo, W., Liu, R., Zhou, Z., Chen, B., and Xu, N. (2010). Waterlogging of cotton cells for caution with N fertilization, acta Agric. Scand. Sect. B-Soil Plant Sci. 60, 450–459. doi: 10.1080/09064710903136818
Haider, S., Rehman, S., Ahmad, Y., Raza, A., Tabassum, J., Javed, T., et al. (2021). In silico characterization and expression profiles of heat shock transcription factors (HSFs) in maize (Zea mays L.). Agronomy 11:2335.
Harvey, P. H. (1939). Herdeitary variation in plant nutrition. Genetics 24, 437–461. doi: 10.1093/genetics/24.4.437
Herridge, D. F., Peoples, M. B., and Boddey, R. M. (2008). Global inputs of biological nitrogen fixation in agricultural systems. Plant Soil 311, 1–18. doi: 10.1007/s11104-008-9668-3
Hodges, H. F., Reddy, K. R., McKinnon, J. M., and Reddy, V. R. (1993). Temperature Effects on Cotton. Mississippi Agricultural and Forestry Experiment Station. Miss: MS, Mississippi State University.
Hou, X., Xiang, Y., Fan, J., Zhang, F., Hu, W., Yan, F., et al. (2021). Evaluation of cotton N nutrition status based on critical N dilution curve, N uptake and residual under different drip fertigation regimes in southern Xinjiang of China. Agric. Water Manag. 256:107134. doi: 10.1016/j.agwat.2021.107134
Hu, Y., Fernández, V., and Ma, L. (2014). Nitrate transporters in leaves and their potential roles in foliar uptake of nitrogen dioxide. Front. Plant Sci. 5:360. doi: 10.3389/fpls.2014.00360
Hu, F., Zhao, C., Feng, F., Chai, Q., Mu, Y., and Zhang, Y. (2017). Improving N management through intercropping alleviates the inhibitory effect of mineral N on nodulation in pea. Plant Soil 412, 235–251. doi: 10.1007/s11104-016-3063-2
Huang, P., Shen, F., Abbas, A., Wang, H., Du, Y., Du, D., et al. (2022). Effects of different nitrogen forms and competitive treatments on the growth and antioxidant system of wedelia trilobata and wedelia chinensis under high nitrogen concentrations. Front. Plant Sci. 13.
Hungria, M., and Kaschuk, G. (2014). Regulation of N2 fixation and NO3−/NH4+ assimilation in nodulated and N-fertilized Phaseolus vulgaris L. exposed to high temperature stress. Environ. Exp. Bot. 98, 32–39. doi: 10.1016/j.envexpbot.2013.10.010
Inthapanya, P., Sihavong, P., Sihathep, V., Chanphengsay, M., Fukai, S., and Basnayake, J. (2000). Genotype differences in nutrient uptake and utilization for grain yield production of rainfed lowland rice under fertilised and non-fertilised condition. Field Crop Res. 65, 1–14. doi: 10.1016/S0378-4290(99)00065-9
Iqbal, A., Huiping, G., Xiangru, W., Hengheng, Z., Xiling, Z., and Meizhen, S. (2022). Genome-wide expression analysis reveals involvement of asparagine synthetase family in cotton development and nitrogen metabolism. BMC Plant Biol. 22, 122–114. doi: 10.1186/s12870-022-03454-7
Iqbal, A., Qiang, D., Zhun, W., Xiangru, W., Huiping, G., Hengheng, Z., et al. (2020). Growth and nitrogen metabolism are associated with nitrogen-use efficiency in cotton genotypes. Plant Physiol. Biochem. 149, 61–74. doi: 10.1016/j.plaphy.2020.02.002
Islam, M. R., Sarker, B. C., Alam, M. A., Javed, T., Alam, M. J., Zaman, M. S. U., et al. (2021). Yield stability and genotype environment interaction of water deficit stress tolerant Mung Bean (Vigna radiata L. Wilczak) genotypes of Bangladesh. Agronomy 11:2136.
Jan, S. U., Liaqat, A., Zhu, Y., Li, J., Zhang, H., Abdalla, M., et al. (2022). Arabidopsis NLP7 improves nitrogen use efficiency and yield in cotton. J. Cotton Res. 5, 1–10. doi: 10.1186/s42397-021-00110-7
Janat, M. (2008). Response of cotton to irrigation methods and nitrogen fertilization: yield components, water-use efficiency, nitrogen uptake, and recovery. Commun. Soil Sci. Plant Anal. 39, 2282–2302. doi: 10.1080/00103620802292293
Javed, T., Indu, I., Singhal, R. K., Shabbir, R., Shah, A. N., Kumar, P., et al. (2022a). Recent advances in agronomic and physio-molecular approaches for improving nitrogen use efficiency in crop plants. Front. Plant Sci. 13:877544. doi: 10.3389/fpls.2022.877544
Javed, T., Zhou, J. R., Li, J., Hu, Z. T., Wang, Q. N., and Gao, S. J. (2022b). Identification and expression profiling of wrky family genes in sugarcane in response to bacterial pathogen infection and nitrogen implantation dosage. Front. Plant Sci. 13:917953.
Jiang, Y., Li, C., Robertson, J. S., Sun, S., Xu, R., and Paterson, A. H. (2018). GPhenoVision: a ground mobile system with multi-modal imaging for field-based high throughput phenotyping of cotton. Sci. Rep. 8, 1–15. doi: 10.1038/s41598-018-19142-2
Jiang, W., Wang, K., Wu, Q., Dong, S., Liu, P., and Zhang, J. (2013). Effects of narrow plant spacing on root distribution and physiological nitrogen use efficiency in summer maize. Crop J. 1, 77–83. doi: 10.1016/j.cj.2013.07.011
Jinger, D., Devi, M. T., Dhar, S., Dass, A., Sharma, V. K., Vijayakumar, S., et al. (2020). Silicon application mitigates abiotic stresses in rice: a review. Indian J. Agric. Sci. 90, 2043–2050.
Ju, X. T., Xing, G. X., Chen, X. P., Zhang, S. L., Zhang, L. J., Liu, X. J., et al. (2009). Reducing environmental risk by improving N management in intensive Chinese agricultural systems. Proc. Natl. Acad. Sci. 106, 3041–3046. doi: 10.1073/pnas.0813417106
Khan, N. U., Khan, A. A., Goheer, M. A., Shafique, I., Hussain, S., Hussain, S., et al. (2021). Effect of Zero and Minimum Tillage on Cotton Productivity and Soil Characteristics under Different Nitrogen Application Rates. Sustainability 13:13753. doi: 10.3390/su132413753
Khan, A., Najeeb, U., Wanga, L., Tanb, D. K. Y., Yanga, G., Munsifc, F., et al. (2017a). Planting density and sowing date strongly influence growth and lint yield of cotton crops. Field Crops Res. 209, 129–135. doi: 10.1016/j.fcr.2017.04.019
Khan, A., Tan, D. K. Y., Afridi, M. Z., Luo, H., Tung, S. A., Ajab, M., et al. (2017b). Nitrogen fertility and abiotic stresses management in cotton crop: a review. Environ. Sci. Pollut. Res. 24, 14551–14566. doi: 10.1007/s11356-017-8920-x
Khan, A., Tan, D. K. Y., Munsif, F., Afridi, M. Z., Shah, F., Wei, F., et al. (2017c). Nitrogen nutrition in cotton and control strategies for greenhouse gas emissions: a review. Environ. Sci. Pollut. Res. 24, 23471–23487. doi: 10.1007/s11356-017-0131-y
Khawar, J., Mubshar, H., Fahad, S., Muhammad, F., Ali, A. B., Hesham, A., et al. (2016). Economic assessment of different mulches in conventional and water-saving rice production systems. Environ. Sci. Pollut. Res. 23, 9156–9163. doi: 10.1007/s11356-016-6162-y
Khizar, M., Shi, J., Saleem, S., Liaquat, F., Ashraf, M., Latif, S., et al. (2020). Resistance associated metabolite profiling of aspergillus leaf spot in cotton through non-targeted metabolomics. PLoS One 15:e0228675, 5. doi: 10.1371/journal.pone.0228675
Khorsandi, F., and Anagholi, A. (2009). Reproductive compensation of cotton after salt stress relief at different growth stages. J. Agron. Crop Sci. 195, 278–283. doi: 10.1111/j.1439-037X.2009.00370.x
Kong, X., Wang, T., Li, W., Tang, W., Zhang, D., and Dong, H. (2016). Exogenous nitric oxide delays salt induced leaf senescence in cotton (Gossypium hirsutum L.). Acta Physiol. Plant. 38, 61. doi: 10.1007/s11738-016-2079-9
Koutroubas, S. D., and Ntanos, D. A. (2003). Genotypic differences for grain yield and nitrogen utilization in dicaand japonica rice under Mediterranean conditions. Field Crop Res. 83, 251–260. doi: 10.1016/S0378-4290(03)00067-4
Krapp, A., and Traong, H. N. (2006). Regulation of C/N interaction in model plant species. J. Crop Improv. 15, 127–173. doi: 10.1300/J411v15n02_05
Krouk, G., Lacombe, B., Bielach, A., Perrine-Walker, F., Malinska, K., Mounier, E., et al. (2010). Nitrate-regulated auxin transport by NRT1. 1 defines a mechanism for nutrient sensing in plants. Dev. Cell 18, 927–937. doi: 10.1016/j.devcel.2010.05.008
Kumar, R., Pareek, N. K., Kumar, U., Javed, T., Al-Huqail, A. A., Rathore, V. S., et al. (2022). Coupling effects of nitrogen and irrigation levels on growth attributes, nitrogen use efficiency, and economics of cotton. Front. Plant Sci. 13.
Lal, R., Reicosky, D. C., and Hanson, J. D. (2007). Evolution of the plow over 10,000 years and the rationale for no-till farming. Soil Till. Res. 93, 1–12. doi: 10.1016/j.still.2006.11.004
Landivar, J. A., Reddy, K. R., and Hodges, H. F. (2010). “Physiological simulation of cotton growth and yield,” in Physiology of Cotton. eds. J. Stewart, D. M. Oosterhuis, J. J. Heitholt, and J. Mauney (New York, NY: Springer), 319–331.
Leblanc, A., Renault, H., Lecourt, J., Etienne, P., Deleu, C., and Le, D. E. (2008). Elongation changes of exploratory and root hair systems induced by aminocyclopropane carboxylic acid and aminoethoxyvinylglycine affect nitrate uptake and BnNrt2. 1 and BnNrt1. 1 transporter gene expression in oilseed rape. Plant Physiol. 146, 1928–1940. doi: 10.1104/pp.107.109363
Lehrsch, G. A., Brown, B., Lentz, R. D., Johnson-Maynard, J. L., and Leytem, A. B. (2015). Compost and manure effects on Sugarbeet nitrogen uptake, nitrogen recovery, and nitrogen use efficiency. Agron. J. 107, 1155–1166. doi: 10.2134/agronj14.0507
Li, B., Chen, L., Sun, W., Wu, D., Wang, M., Yu, Y., et al. (2020). Phenomics-based GWAS analysis reveals the genetic architecture for drought resistance in cotton. Plant Biotechnol. J. 18, 2533–2544. doi: 10.1111/pbi.13431
Li, Q., Ma, X. J., Cheng, Q. B., Dou, P., Yu, D. H., Luo, Y. H., et al. (2016a). Effects of nitrogen application on nitrogen utilization and nitrogen balance in field of maize cultivars with different low nitrogen tolerance. J. Soil Water Conserv. 30, 171–176.
Li, Q., Ma, X. J., Cheng, Q. B., Dou, P., Yu, D. H., Luo, Y. H., et al. (2016b). Effects of nitrogen fertilizer on post-silking dry matter production and leaves function characteristics of low-nitrogen tolerance maize. Chin. J. Eco-Agric. 24, 17–26. doi: 10.13930/j.cnki.cjea.150744
Li, Q., Wu, Y. W., Chen, W., Jin, R., Kong, F. L., Ke, Y. P., et al. (2017). Cultivar differences in root nitrogen uptake ability of maize hybrids. Front. Plant Sci. 8:1060. doi: 10.3389/fpls.2017.01060
Liang, J., He, Z., and Shi, W. (2020). Cotton/mung bean intercropping improves crop productivity, water use efficiency, nitrogen uptake, and economic benefits in the arid area of Northwest China. Agric. Water Manag. 240:106277. doi: 10.1016/j.agwat.2020.106277
Lin, Y. F., Jing, S. R., Lee, D. Y., Chang, Y. F., and Shih, K. C. (2008). Nitrate removal from groundwater using constructed wetlands under various hydraulic loading rates. Bioresour. Technol. 99, 7504–7513. doi: 10.1016/j.biortech.2008.02.017
Liu, L., Bi, X. Y., Sheng, S., Gong, Y. Y., Pu, W. X., Ke, J., et al. (2021). Evidence that exogenous urea acts as a potent cue to alleviate ammonium-inhibition of root system growth of cotton plant (Gossypium hirsutum). Physiol. Plant. 171, 137–150. doi: 10.1111/ppl.13222
Liu, J., Meng, Y., Chen, J., Lv, F., Ma, Y., Chen, B., et al. (2015). Effect of late planting and shading on cotton yield and fiber quality formation. Field Crop Res. 183, 1–13. doi: 10.1016/j.fcr.2015.07.008
Liu, X., Zhang, Y., Han, W., Tang, A., Shen, J., Cui, Z., et al. (2013). Enhanced nitrogen deposition over China. Nature 494, 459–462. doi: 10.1038/nature11917
Luo, Z., Liu, H., Li, W. P., Zhao, Q., Dai, J. L., Tian, L. W., et al. (2018). Effects of reduced nitrogen rate on cotton yield and nitrogen use efficiency as mediated by application mode or plant density. Field Crop Res. 218, 150–157. doi: 10.1016/j.fcr.2018.01.003
Lv, F., Liu, J., Ma, Y., Chen, J., Keyoumu Abudurezikekey, A., Wang, Y., et al. (2013). Effect of shading on cotton yield and quality on different fruiting branches. Crop Sci. 53, 2670–2678. doi: 10.2135/cropsci2013.03.0170
Macdonald, B. C. T., Chang, Y. F., Nadelko, A., Tuomi, S., and Glover, M. (2016). Tracking fertiliser and soil nitrogen in irrigated cotton: uptake, losses and the soil N stock. Soil Research 55, 264–272. doi: 10.1071/SR16167
Magwanga, R. O., Kirungu, J. N., Lu, P., Cai, X., Zhou, Z., Xu, Y., et al. (2019). Map-based functional analysis of the GhNLP genes reveals their roles in enhancing tolerance to N-deficiency in cotton. Int. J. Mol. Sci. 20, 4953. doi: 10.3390/ijms20194953
Mahmood, T., Ahmar, S., Abdullah, M., Iqbal, M. S., Yasir, M., Khalid, S., et al. (2021). Genetic potential and inheritance pattern of phenological growth and drought tolerance in cotton (Gossypium hirsutum L.) Front. Plant Sci. 1999.
Mei, L., Daud, M. K., Ullah, N., Ali, S., Khan, M., Malik, Z., et al. (2015). Pretreatment with salicylic acid and ascorbic acid significantly mitigate oxidative stress induced by copper in cotton genotypes. Environ. Sci. Pollut. Res. 22, 9922–9931. doi: 10.1007/s11356-015-4075-9
Meng, Z., Meng, Z., Zhang, R., Liang, C., Wan, J., Wang, Y., et al. (2015). Expression of the rice arginase gene OsARG in cotton influences the morphology and nitrogen transition of seedlings. PLoS One 10:e0141530. doi: 10.1371/journal.pone.0141530
Mokhele, B., Zhan, X., Yang, G., and Zhang, X. (2012). Nitrogen assimilation in crop plants and its affecting factors. Can. J. Plant Sci. 92, 399–405. doi: 10.4141/cjps2011-135
Nacry, P., Bouguyon, E., and Gojon, A. (2013). Nitrogen acquisition by roots: physiological and developmental mechanisms ensuring plant adaptation to a fluctuating resource. Plant Soil 370, 1–29. doi: 10.1007/s11104-013-1645-9
Najeeb, U., Bange, M. P., Tan, D. K. Y., and Atwell, B. J. (2015b). Consequences of waterlogging in cotton and opportunities for mitigation of yield losses. AoB Plants 7:plv080. doi: 10.1093/aobpla/plv080
Najeeb, U., Tan, D. K. Y., and Bange, M. P. (2015a). Inducing waterlogging tolerance in cotton via an anti-ethylene agent aminoethoxyvinyl glycine application. Arch. Agron. Soil Sci. 62, 1136–1146. doi: 10.1080/03650340.2015.1113403
Naoumkina, M., Hinchliffe, D. J., Turley, R. B., Bland, J. M., and Fang, D. D. (2013). Integrated metabolomics and genomics analysis provides new insights into the fiber elongation process in Ligon lintless-2 mutant cotton (Gossypium hirsutum L.). BMC Genomics 14, 155–116. doi: 10.1186/1471-2164-14-155
Nazar, R., Iqbal, N., Masood, A., Syeed, S., and Khan, N. A. (2011). Understanding the significance of sulfur in improving salinity tolerance in plants. Environ. Exp. Bot. 70, 80–87. doi: 10.1016/j.envexpbot.2010.09.011
Niu, J., Gui, H., Iqbal, A., Zhang, H., Dong, Q., Pang, N., et al. (2021). N-use efficiency and yield of cotton (G. hirsutumn L.) are improved through the combination of N-fertilizer reduction and N-efficient cultivar. Agronomy 11, 55. doi: 10.3390/agronomy11010055
Noman, A., Fahad, S., Aqeel, M., Ali, U., Amanullah, A. S., Baloch, S. K., et al. (2017). Mi RNAs: major modulators for crop growth and development under abiotic stresses. Biotechnol. Lett. 39, 685–700. doi: 10.1007/s10529-017-2302-9
Noreen, S., Mahmood, S., Faiz, S., and Akhter, S. (2020). “Plant growth regulators for cotton production in changing environment,” in Cotton Production and Uses Agronomy, Crop Protection, and Postharvest Technologies. eds. S. Ahmad and M. Hasanuzzaman (Singapore: Springer Nature Singapore), 199–144.
North, K. A., Ehlting, B., Koprivova, A., Rennenberg, H., and Kopriva, S. (2009). Natural variation in Arabidopsis adaptation to growth at low nitrogen conditions. Plant Physiol. Biochem. 47, 912–918. doi: 10.1016/j.plaphy.2009.06.009
Oosterhuis, D. M., and Snider, J. L. (2011). “High temperature stress on floral development and yield of cotton,” in Stress Physiology in Cotton. ed. D. M. Oosterhuis (Cordova, TN: The Cotton Foundation)
Panhwar, R. B., Akbar, A., Panhwar, B. U., Panhwar, G. A., and Bai-li, F. (2018). Effects of plant spacing and nitrogen fertilizer levels on cotton yield and growth. Int. J. Sci. Environ. Technol. 7, 313–324.
Paproki, A., Fripp, J., Salvado, O., Sirault, X., Berry, S., and Furbank, R. (2011). Automated 3D segmentation and analysis of cotton plants. in 2011 International Conference on Digital Image Computing: Techniques and Applications 555–560. IEEE.
Peng, J., Liu, J., Zhang, L., Luo, J., Dong, H., Ma, Y., et al. (2016). Effects of soil salinity on sucrose metabolism in cotton leaves. PLoS One 11:e0156241. doi: 10.1371/journal.pone.0156241
Pengcheng, L. I., Helin, D., Aizhong, L., Jingran, L., Miao, S., Yabing, L., et al. (2017). Effects of nitrogen rate and split application ratio on nitrogen use and soil nitrogen balance in cotton field. Pedosphere 27, 769–777. doi: 10.1016/S1002-0160(17)60303-5
Pessarakli, M. (2001). “Physiological responses of cotton (Gossypium hirsutum L.) to salt stress,” in Handbook of Plant and Crop Physiology. ed. M. Pessarakli (New York, NY: Marcel Dekker), 681–696.
Prasad, R. (2019). Text Book of Field Crops Production Commercial Crops. Vol. II. Indian Council of Agricultural Research. New Delhi.
Qiao, C., Mia, S., Wang, Y., Hou, J., and Xu, B. (2021). Assessing the effects of nitrification inhibitor dmpp on acidification and inorganic n leaching loss from tea (Camellia sinensis L.) cultivated soils with increasing urea–n rates. Sustainability 13, 994. doi: 10.3390/su13020994
Rajpoot, S. K., Rana, D. S., Rana, K. S., and Sepat, S. (2014). Effect of methods of crop establishment over productivity and economics of Bt cotton (Gossypium hirsutum)-based intercroppingsystem. Indian J. Agron. 59, 489–492.
Ranathunge, K., El-kereamy, A., Gidda, S., Bi, Y.-M., and Rothstein, S. J. (2014). AMT1; 1 transgenic rice plants with enhanced NH4+ permeability show superior growth and higher yield under optimal and suboptimal NH4+ conditions. J. Exp. Bot. 65, 965–979. doi: 10.1093/jxb/ert458
Reddy, K. R., Brand, D., Wijewardana, C., and Gao, W. (2017). Temperature effects on cotton seedling emergence, growth, and development. Agron. J. 109, 1379–1387. doi: 10.2134/agronj2016.07.0439
Rizhysky, L., Liang, H., Shuman, J., Shulaev, V., Davletova, S., and Mittlerm, R. (2004). When defense pathways collide: the response of Arabidopsis to a combination of drought and heat stress. J. Plant Physiol. 134, 1683–1696. doi: 10.1104/pp.103.033431
Sabagh, A. E., Mbarki, S., Hossain, A., Iqbal, M. A., Islam, M. S., Raza, A., et al. (2021). Potential role of plant growth regulators in administering crucial processes Against abiotic stresses. Front. Agron. 3:648694 doi: 10.3389/fagro.2021.648694
Sarwar, M., Saleem, M. F., Najeeb, U., Shakeel, A., Ali, S., and Bilal, M. F. (2017). Hydrogen peroxide reduces heat-induced yield losses in cotton (Gossypium hirsutum L.) by protecting cellular membrane damage. J. Agron. Crop Sci. 203, 429–441. doi: 10.1111/jac.12203
Shah, A. N., Wu, Y., Iqbal, J., Tanveer, M., Bashir, S., Rahman, S. U., et al. (2021a). Nitrogen and plant density effects on growth, yield performance of two different cotton cultivars from different origin. J. King Saud Univ. Sci 33, 101512. doi: 10.1016/j.jksus.2021.101512
Shah, A. N., Wua, Y., Tanveer, M., Hafeez, A., Tung, S. A., Ali, S., et al. (2021b). Interactive effect of nitrogen fertilizer and plant density on photosynthetic and agronomical traits of cotton at different growth stages. Saudi J. Biol. Sci. 28, 3578–3584. doi: 10.1016/j.sjbs.2021.03.034
Shakoor, A., Saleem, M. F., Anjum, S. A., Wahid, M. A., and Saeed, M. T. (2017). Effect of heat stress and benzoic acid as foliar application on earliness and nutrients uptake in cotton. J. Agric. Res. 55, 15–28.
Singhal, R. K., Saha, D., Skalicky, M., Mishra, U. N., Chauhan, J., Behera, L. P., et al. (2021). Crucial cell signaling compounds crosstalk and integrative multi-omics techniques for salinity stress tolerance in plants. Front. Plant Scie. 12:670369. doi: 10.3389/fpls.2021.670369
Sitienei, R. C., Onwonga, R. N., Lelei, J. J., and Kamoni, P. (2017). Use of Dolichos (Lablab purpureus L.) and combined fertilizers enhance soil nutrient availability and maize (Zea Mays L.) yield in farming systems of Kabete sub-county. Kenya. Agric. Sci. Res. J. 7, 41–61.
Sohair, E. E. D., Abdall, A. A., Amany, A. M., and Faruque HMd, H. R. (2018). Evaluation of nitrogen. Phosphorus and potassium Nano-fertilizers on yield, yield components and Fiber properties of Egyptian cotton (Gossypium Barbadense L.). J. Plant Sci. Crop Protec. 1, 208.
Stephenson, D. O., Barber, T., and Bourland, F. M. (2011). Effect of twin-row planting pattern and plant density on cotton growth, yield, and fiber quality. J. Cotton Sci. 15, 243–250.
Sun, S., Li, C., Paterson, A. H., Jiang, Y., Xu, R., Robertson, J. S., et al. (2018). In-field high throughput phenotyping and cotton plant growth analysis using LiDAR. Front. Plant Sci. 9:16. doi: 10.3389/fpls.2018.00016
Suppan, S. (2017). “Applying nanotechnology to fertilizer: rationales, research, risks and regulatory challenges,” in The Institute for Agriculture and Trade Policy works locally and globally, Brazil
Suter, H., Lam, S. K., Walker, C., and Chen, D. (2020). Enhanced efficiency fertilisers reduce nitrous oxide emissions and improve fertiliser 15N recovery in a southern Australian pasture. Sci. Total Environ. 699:134147. doi: 10.1016/j.scitotenv.2019.134147
Tang, H. Y., Yang, G. Z., Zhang, X. L., and Siddique, K. (2012). Improvement of fertilizer N recovery by allocating more N for later application in cotton (Gossypium hirsutum L.). Int. J. Basic Appl. Sci. 12, 32–37.
Thorp, K. R., Gore, M. A., Andrade-Sanchez, P., Carmo-Silva, A. E., Welch, S. M., White, J. W., et al. (2015). Proximal hyperspectral sensing and data analysis approaches for field-based plant phenomics. Comput. Electron. Agric. 118, 225–236. doi: 10.1016/j.compag.2015.09.005
Tsay, Y. F., Chiu, C. C., Tsai, C. B., Ho, C. H., and Hsu, P. K. (2007). Nitrate transporters and peptide transporters. FEBS Lett. 581, 2290–2300. doi: 10.1016/j.febslet.2007.04.047
Tuttle, J. R., Nah, G., Duke, M. V., Alexander, D. C., Guan, X., Song, Q., et al. (2015). Metabolomic and transcriptomic insights into how cotton fiber transitions to secondary wall synthesis, represses lignification, and prolongs elongation. BMC Genomics 16, 477–428. doi: 10.1186/s12864-015-1708-9
Ur Rahman, M. H., Ahmad, A., Wajid, A., Hussain, M., Rasul, F., Ishaque, W., et al. (2019). Application of CSM-CROPGRO-cotton model for cultivars and optimum planting dates: evaluation in changing semi-arid climate. Field Crops Res 238, 139–152. doi: 10.1016/j.fcr.2017.07.007
Venugopalan, M. V., and Blaise, D. (2001). Effect of planting density and nitrogen levels on the productivity and nitrogen use efficiency of rainfed upland cotton (Gossypium Hirsutum). Indian J. Agron. 46, 346–353.
Venugopalan, M. V., Deshmukh, R., Hebbar, K. B., and Tandulkar, N. R. (2012). Productivity and nitrogenous efficiency yardsticks in conventional and Bt cotton hybrids on rainfed Vertisols. Indian J. Agric. Sci. 82, 641–644.
Von Wittgenstein, N. J., Le, C. H., Hawkins, B. J., and Ehlting, J. (2014). Evolutionary classification of ammonium, nitrate, and peptide transporters in land plants. BMC Evol. Biol. 14, 1–17. doi: 10.1186/1471-2148-14-11
Wagh, N. S., Katkar, R. N., and Kharche, V. K. (2016). Effect of tillage and nutrient management on seed cotton yield, yield contributing characters and total uptake by cotton. Asian J. Soil Sci. 11, 277–285. doi: 10.15740/HAS/AJSS/11.2/277-285
Wang, X., Cai, X., Xu, C., Wang, S., Dai, S., and Wang, Q. (2018). Nitrate accumulation and expression patterns of genes involved in nitrate transport and assimilation in spinach. Molecules 23, 2231. doi: 10.3390/molecules23092231
Wang, X., Deng, Z., Zhang, W., Meng, Z., Chang, X., and Lv, M. (2017). Effect of waterlogging duration at different growth stages on the growth, yield and quality of cotton. PLoS One 12:e0169029. doi: 10.1371/journal.pone.0169029
Wang, M., Hasegawa, T., Beier, M., Hayashi, M., Ohmori, Y., Yano, K., et al. (2021b). Growth and nitrate reductase activity are impaired in rice osnlp 4 mutants supplied with nitrate. Plant Cell Physiol. 62, 1156–1167. doi: 10.1093/pcp/pcab035
Wang, J., Hussain, S., Sun, X., Zhang, P., Javed, T., Dessoky, E. S., et al. (2022). Effects of nitrogen application rate under straw incorporation on photosynthesis, productivity and nitrogen use efficiency in winter wheat. Front. Plant Sci. 13, 862088–862088.
Wang, R., Ji, S., Zhang, P., Meng, Y., Wang, Y., Chen, B., et al. (2016). Drought effects on cotton yield and fiber quality on different fruiting branches. 2016. Crop Sci. 56, 1265–1276. doi: 10.2135/cropsci2015.08.0477
Wang, K., Li, S., Cao, L., Song, G., Chen, G., and Cao, S. (2003). A preliminary study on dynamics and models of N, P, K absorption in high yield cotton in Xinjiang. Sci. Agric. Sin. 36, 775–780.
Wang, Q., Meng, Q., Xu, F., Chen, Q., Ma, C., Huang, L., et al. (2021c). Comparative metabolomics analysis reveals sterols and sphingolipids play a role in cotton fiber cell initiation. Int. J. Mol. Sci. 22, 11438. doi: 10.3390/ijms222111438
Wang, X., Peng, F., Li, M., Yang, L., and Li, G. (2012). Expression of a heterologous SnRK1 in tomato increases carbon assimilation, nitrogen uptake and modifies fruit development. J. Plant Physiol. 169, 1173–1182. doi: 10.1016/j.jplph.2012.04.013
Wang, Y. Y., and Tsay, Y. F. (2011). Arabidopsis nitrate transporter NRT1.9 is important in phloem nitrate transport. Plant Cell 23, 1945–1957. doi: 10.1105/tpc.111.083618
Wang, H., Wu, L., Wang, X., Zhang, S., Cheng, M., Feng, H., et al. (2021a). Optimization of water and fertilizer management improves yield, water, nitrogen, phosphorus and potassium uptake and use efficiency of cotton under drip fertigation. Agric. Water Manag. 245:106662. doi: 10.1016/j.agwat.2020.106662
Wang, Z., Zhang, X., He, S., Rehman, A., Jia, Y., Li, H., et al. (2021d). Transcriptome co-expression network and metabolome analysis identifies key genes and regulators of Proanthocyanidins biosynthesis in Brown cotton. Front. Plant Sci. 12:822198. doi: 10.3389/fpls.2021.822198
Xia, B., Ren, X. M., Du, M. W., Xu, D. Y., Yin, X. F., Tian, X. L., et al. (2016). Nitrogen rate and nitrogen use efficiency of cotton harvested by machine in the field with stalks recycling in the Yellow River Valley region. Cott. Sci. 28, 315–323.
Xue, J., Li, M. Y., Ning, S., Wei-shao, S., and Zhou, Z. (2013). Effects of planting density on uptake and utilization of NPK of transgenic cotton. Plant Nutr. Fert. 19, 179–186.
Yadvinder-Singh, S., Singh, B., and Timsina, J. (2005). Crop residue management for nutrient cycling and improving soil productivity in rice-based cropping systems in the tropics. Adv. Agron. 85, 269–407. doi: 10.1016/S0065-2113(04)85006-5
Yang, G. Z., Chu, K. Y., Tang, H. Y., Nie, Y. C., and Zhang, X. L. (2013). Fertilizer 15N accumulation, recovery and distribution in cotton plant as affected by N rate and split. J. Integr. Agric. 12, 999–1007. doi: 10.1016/S2095-3119(13)60477-3
Yang, F., Dua, M., Tiana, X., Enejic, A. E., Duana, L., and Lia, Z. (2014). Plant growth regulation enhanced potassium uptake and use efficiency in cotton. Field Crops Res. 163, 109–118. doi: 10.1016/j.fcr.2014.03.016
Yousaf, M., Li, X., Zhang, Z., Ren, T., Cong, R., Ata-Ul-Karim, S. T., et al. (2016). Nitrogen fertilizer management for enhancing crop productivity and nitrogen use efficiency in a rice-oilseed rape rotation system in China. Front. Plant Sci. 7:1496. doi: 10.3389/fpls.2016.01496
Yu, L. H., Wu, S. J., Peng, Y. S., Liu, R. N., Chen, X., Zhao, P., et al. (2016). Arabidopsis EDT 1/HDG 11 improves drought and salt tolerance in cotton and poplar and increases cotton yield in the field. Plant Biotechnol. J. 14, 72–84. doi: 10.1111/pbi.12358
Zafar, M. M., Razzaq, A., Farooq, M. A., Rehman, A., Firdous, H., Shakeel, A., et al. (2020). Genetic variation studies of ionic and within boll yield components in cotton (Gossypium Hirsutum L.) Under salt stress. J. Nat. Fibers, 1–20. doi: 10.1080/15440478.2020.1838996
Zaman, I., Ali, M., Shahzad, K., Tahir, M. S., Matloob, A., Ahmad, W., et al. (2021). Effect of plant spacing on growth, physiology, yield and fiber quality attributes of cotton genotypes under nitrogen fertilization. Agronomy 11, 2589. doi: 10.3390/agronomy11122589
Zhang, X., Chen, J., Feng, K., Wang, N., Zhang, S., Ma, H., et al. (2021). Widely targeted metabolomics reveals the different metabolic changes in leaves and roots of two cotton varieties under drought stress. J. Agron. Crop Sci. 207, 1041–1049. doi: 10.1111/jac.12527
Zhang, H., Yu, C., Kong, X., Hou, D., Gu, J., Liu, L., et al. (2018). Progressive integrative crop managements increase grain yield, nitrogen use efficiency and irrigation water productivity in rice. Field Crop Res. 215, 1–11. doi: 10.1016/j.fcr.2017.09.034
Zhao, S., Lin, Z., Li, X., Xu, W., Li, Y., Hou, S., et al. (2010). Study on absorption law of nitrogen, phosphorus and potassium of cotton plant under different nitrogen application rate. Xinjiang Agric. Sci. 47, 141–145.
Zhao, D., and Oosterhuis, D. M. (1998). Influence of shade on mineral nutrient status of field-grown cotton. J. Plant Nutr. 21, 1681–1695. doi: 10.1080/01904169809365513
Zhao, D., Reddy, K. R., Kakani, V. G., Koti, S., and Gao, W. (2005). Physiological causes of cotton fruit abscission under conditions of high temperature and enhanced ultraviolet-B radiation. Physiol. Plant. 124, 189–199. doi: 10.1111/j.1399-3054.2005.00491.x
Zheng, Z. L. (2009). Carbon and nitrogen nutrient balance signaling in plants. Plant Signal. Behav. 4, 584–591. doi: 10.4161/psb.4.7.8540
Zhou, Z. Q., and Oosterhuis, M. D. (2012). Physiological mechanism of nitrogen mediating cotton (Gossypium hirsutum L.) seedlings growth under water-stress conditions. American J. Plant Sci. 03, 721–730. doi: 10.4236/ajps.2012.36087
Keywords: abiotic stresses, climate change, cotton, nitrogen use efficiency, physiological responses GhNRT1.1, GhNRT1.2, GhNRT2.1, GhNRT2.2
Citation: Shah AN, Javed T, Singhal RK, Shabbir R, Wang D, Hussain S, Anuragi H, Jinger D, Pandey H, Abdelsalam NR, Ghareeb RY and Jaremko M (2022) Nitrogen use efficiency in cotton: Challenges and opportunities against environmental constraints. Front. Plant Sci. 13:970339. doi: 10.3389/fpls.2022.970339
Received: 15 June 2022; Accepted: 20 July 2022;
Published: 22 August 2022.
Edited by:
Iftikhar Ali, Institute of Genetics and Developmental Biology (CAS), ChinaReviewed by:
Pushpendra Koli, Murdoch University, Australia Asif Iqbal, University of Agriculture, PakistanCopyright © 2022 Shah, Javed, Singhal, Shabbir, Wang, Hussain, Anuragi, Jinger, Pandey, Abdelsalam, Ghareeb and Jaremko. This is an open-access article distributed under the terms of the Creative Commons Attribution License (CC BY). The use, distribution or reproduction in other forums is permitted, provided the original author(s) and the copyright owner(s) are credited and that the original publication in this journal is cited, in accordance with accepted academic practice. No use, distribution or reproduction is permitted which does not comply with these terms.
*Correspondence: Adnan Noor Shah, YW5zLjc4NkB5YWhvby5jb20=; Depeng Wang, d2FuZ2RlcGVuZ0BseXUuZWR1LmNu
†These authors have contributed equally to this work
Disclaimer: All claims expressed in this article are solely those of the authors and do not necessarily represent those of their affiliated organizations, or those of the publisher, the editors and the reviewers. Any product that may be evaluated in this article or claim that may be made by its manufacturer is not guaranteed or endorsed by the publisher.
Research integrity at Frontiers
Learn more about the work of our research integrity team to safeguard the quality of each article we publish.