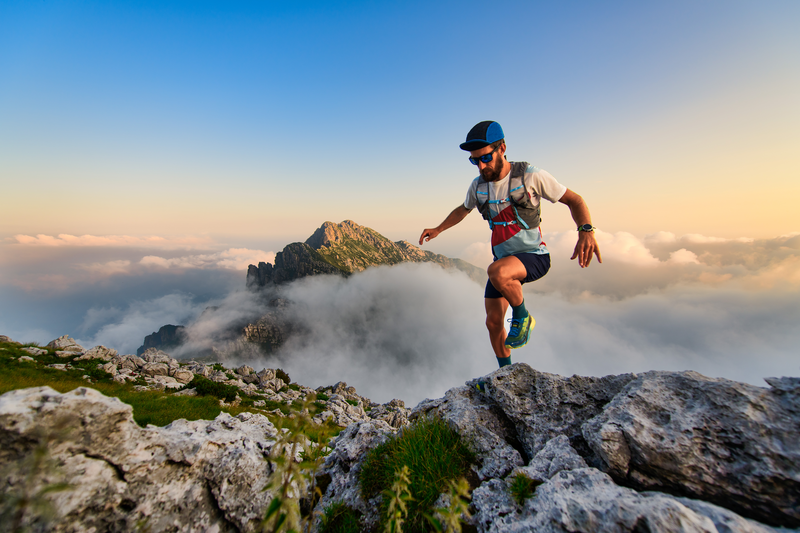
95% of researchers rate our articles as excellent or good
Learn more about the work of our research integrity team to safeguard the quality of each article we publish.
Find out more
REVIEW article
Front. Plant Sci. , 23 August 2022
Sec. Plant Pathogen Interactions
Volume 13 - 2022 | https://doi.org/10.3389/fpls.2022.969343
This article is part of the Research Topic Women in Plant Pathogen Interactions: 2022 View all 8 articles
Cell wall integrity is tightly regulated and maintained given that non-physiological modification of cell walls could render plants vulnerable to biotic and/or abiotic stresses. Expansins are plant cell wall-modifying proteins active during many developmental and physiological processes, but they can also be produced by bacteria and fungi during interaction with plant hosts. Cell wall alteration brought about by ectopic expression, overexpression, or exogenous addition of expansins from either eukaryote or prokaryote origin can in some instances provide resistance to pathogens, while in other cases plants become more susceptible to infection. In these circumstances altered cell wall mechanical properties might be directly responsible for pathogen resistance or susceptibility outcomes. Simultaneously, through membrane receptors for enzymatically released cell wall fragments or by sensing modified cell wall barrier properties, plants trigger intracellular signaling cascades inducing defense responses and reinforcement of the cell wall, contributing to various infection phenotypes, in which expansins might also be involved. Here, we review the plant immune response activated by cell wall surveillance mechanisms, cell wall fragments identified as responsible for immune responses, and expansin’s roles in resistance and susceptibility of plants to pathogen attack.
Throughout their lifetime plants interact with the microbiota that lives on and inside their tissues, including opportunistic or pathogenic microorganisms, which are controlled by plant physical barriers and multi-layered inducible defense responses (Lemanceau et al., 2017; Choi K. et al., 2021). The first barrier is the cuticle, mostly composed by cutin and epicuticular and intracuticular waxes localized on the aerial parts of the plants (Serrano et al., 2014). The secondary physical barrier is the cell wall, a dynamic and complex structure mainly composed of microfibrils of cellulose in the form of ß-1,4-linked glucan chains, interconnected with xyloglucan and embedded in a hydrated matrix of pectin (Wang et al., 2015; Cosgrove, 2018, 2022). Physicochemical characteristics of the cuticle and the cell wall impact on the composition of the plant microbiome for microbial selection and establishment (Bulgarelli et al., 2013; Aragón et al., 2017; Molina et al., 2021). To interact with their hosts, pathogens require specific activity levels of plant cell wall degrading enzymes such as pectate lyases, cellulases, xylanases, and proteases to digest the cell wall polysaccharides and structural proteins (Bellincampi et al., 2014; Mitsumasu et al., 2015). Digestion fragments play multiple roles in the development and pathogenesis of microbes, but they also trigger plant defense responses and determine the specificity of the plant-pathogen interaction. In particular, short polysaccharide oligomers derived from the cell wall belong to the type of self-recognized molecules known as Damage-Associated Molecular Patterns (DAMPs), and are potent defense-response inducers (Hou et al., 2019; Gigli-Bisceglia et al., 2020), and thus, their application could serve as a cheap and efficient priming treatment to boost plant resistance to prevent infection (Quintana-Rodriguez et al., 2018). For this reason, it is important to explore the characteristics of a range of active glycans such as their optimal activating degree of polymerization, defense-inducing concentration and mode of action, as well as identifying their membrane receptors (Molina et al., 2022). Computational models have for instance been used to determine detailed information of the possible conformation of known interactor pairs [for example receptor: CERK1, with ligand: 1,4-β-d-(GlcNAc)6] with the purpose of predicting and detecting receptors and the signaling pathways leading to resistance (del Hierro et al., 2021). A more challenging aspect of the study of cell wall integrity (CWI) surveillance mechanisms is the perception of modified physical properties and its translation into biochemical signals perceived by membrane receptors (Wolf, 2017). Unexpected changes in cell wall stiffness, surface tension, or barrier properties are in some instances caused by expansin proteins, which are non-enzymatic cell wall remodeling agents (Georgelis et al., 2014; Cosgrove, 2015, 2016). Ectopic expression, constitutive overexpression or exogenous addition of expansins affect plant resistance or susceptibility to infection either by direct modification of the cell wall or by triggering plant immune responses (Marowa et al., 2016; Narváez-Barragán et al., 2020). Here, we review aspects of CWI surveillance mechanisms focusing on stimuli from pathogen colonization, and on cell wall digestion fragments as inducers of defense responses and analyze how these relate to expansin-induced changes in host susceptibility to infection.
The plant cell wall is synthesized at different levels in a coordinated manner (Cosgrove, 2018). Cell wall polysaccharide content varies according to developmental status (Zablackis et al., 1995), but cellulose, formed at the cell surface by mobile cellulose synthase complexes (CSC), is the most abundant polysaccharide of the cell wall (Grones et al., 2019). In addition, the cell wall contains complex combinations of macromolecules, including hemicelluloses, pectin and lignin, constituting a dynamic structure that can change its composition and properties during plant development without compromising its integrity (Rongpipi et al., 2019). The cell wall is also modified in response to different types of stress (Bacete and Hamann, 2020; Rui and Dinneny, 2020). It is well known that alterations to the CWI activates plant defense responses that in some cases provoke resistance to pathogens (Srivastava et al., 2017; Engelsdorf et al., 2018; Li et al., 2019; Wolf, 2022).
Reduction of cellulose biosynthesis alters CWI inducing cell wall damage that is sensed through diverse mechanisms, including osmo- and mechano-perception, which activate signaling pathways impacting defense responses (Molina et al., 2022). The identity of the components acting upstream of the osmo- and mechano-receptors is practically unknown, and indeed there is little experimental evidence to relate them to responses to pathogens (Bacete and Hamann, 2020). Several microorganisms cause cell wall damage, and it is important to identify cell wall components that alert the cell of microbial invasion. The best characterized osmo-receptors in plants are the Arabidopsis thaliana histidine protein kinases (HPKs) (Pham et al., 2012). The A. thaliana genome contains eleven HPKs, of which five have been recognized as ethylene receptors, three as cytokinin receptors and the remaining three have no known activity as hormone receptors, although they participate in other signaling processes including responses to biotic and abiotic stresses (Pham et al., 2012; Hofmann et al., 2020). An additional mechanism to cope with physical forces is mediated by mechano-sensitive ion channels (MSIC) and mechano-sensitive like channels (MSL) located at the inner chloroplast envelope (Haswell and Meyerowitz, 2006; Bacete and Hamann, 2020). Based on structural homology with an MSIC originally described in E. coli, MSICs have been identified across all kingdoms. In A. thaliana, ten homologs exist (MSL for MscS-Like) located in the plasma, inner chloroplast envelope and inner mitochondrial membrane, and have functions related to osmoregulation and two processes clearly related to pathogen responses: cell death and the redox state (Guerringue et al., 2018; Bacete and Hamann, 2020; Schlegel and Haswell, 2020). Functional characterization of plant HPKs and MscS-Like channels is incipient and further research is needed.
Although diverse response pathways to perceived stimuli exist, most described cell wall-derived elicitor signaling pathways operate through receptor-like kinase (RLKs), which constitute one of the largest gene families in plants, with more than 600 members in A. thaliana (Jose et al., 2020). The basic structure of an RLK includes a variable extracellular domain (ECD), a transmembrane region and a cytosolic domain with kinase activity, for phosphorylation of various proteins during downstream signaling (Dievart et al., 2020). The largest subfamily of RLKs, which includes members essential for plant development and immune responses, is one for which the ECD is made up of leucine-rich repeats (LRR-RLK) (Haswell and Meyerowitz, 2006). This class of receptors generally heterodimerize with members of the subfamily SOMATIC EMBRYOGENESIS RECEPTOR KINASEs (SERKs), forming a receptor with two kinase domains that are self and hetero-phosphorylated at multiple residues (Zhou et al., 2019). Once activated, heteromeric complexes phosphorylate other components of the pathway forming a signaling cascade that includes Receptor-Like Cytoplasmic Kinases (RLCKs), Mitogen-Activated Protein Kinases (MAPKs) and/or calcium-dependent protein kinases (CDPKs), ultimately targeting transcription factors to drive gene expression changes in response to a particular ligand (Zhang et al., 2018). Regulation of these pathways includes the activity of pseudokinases and phosphatases, modulation of component abundance and ubiquitination and post-translational modifications, such as glycosylation, acetylation, etc. (Yuan et al., 2021). Thus, the diversity of responses controlled in this manner is enormous, difficult to study and harder to elucidate. Interestingly, other RLK subgroups contain extracellular domains with carbohydrate-binding motifs, being interesting candidates for sensing cell wall-related signals that potentially derive from interactions with pathogenic microorganisms (Zhu et al., 2021). The LRR-RLK family constitutes the largest plant-specific clade of the eukaryotic kinase superfamily and several of its members have essential roles in many aspects of plant development and immunity (Yang et al., 2016; Jose et al., 2020). Despite their importance in defense mechanisms, functional characterization of LRR-RLKs is incomplete, mainly due to high functional redundancy. Even so, some receptors have been extensively studied, generating important information about their role in plant immunity. Such is the case of BRASSINOSTEROID INSENSITIVE1 (BRI1) originally identified as the receptor for plant hormone brassinosteroid and more recently observed as being involved in plant immunity signaling (Lozano-Elena and Caño-Delgado, 2019; Choi J. H. et al., 2021). Heterodimerization of BRI1 with coreceptor BRI1-ASSOCIATED RECEPTOR KINASE1 (BAK1/SERK3) directs the transphosphorylation for Ca2+-dependent proteolytic cleavage of BAK1 that is critical for its location at the membrane and its activity to phosphorylate BOTRYTIS-INDUCED KINASE1 (BIK1), an essential control point in plant immunity (Lozano-Elena and Caño-Delgado, 2019; Zhou et al., 2019; Choi J. H. et al., 2021). BAK1 can form a co-receptor complex with MIK2 (LRR-RK MALE DISCOVERER 1-INTERACTING RECEPTOR LIKE KINASE2), reported as the receptor for SERINE RICH ENDOGENOUS PEPTIDE (SCOOP), which is required for resistance to Fusarium oxysporum, a root invading fungus causing vascular wilt disease through xylem colonization (Edel-Hermann and Lecomte, 2018). Interestingly, F. oxysporum proteome includes SCOOP-like sequences that seem to trigger plant immunity (Van der Does et al., 2017; Hou et al., 2021; Rhodes et al., 2021).
FEI1 and FEI2 are another pair of RLKs involved in CWI signaling; both interact with an arabinogalactan protein (SOS5/FLA4) involved in salinity tolerance and cellulose biosynthesis, as well as with at least two isoforms of AMINOCYCLOPROPANE 1-CARBOXYLIC ACID (ACC) SYNTHASE (ACS), thus relating CWI signaling directly with ethylene and indirectly with other growth regulators (ABA and auxins) (Engelsdorf et al., 2018; Seifert, 2021). Regarding the relationship of FEI proteins with defense responses, Botrytis cinerea [a necrotrophic fungus causative of the gray mold disease (Dean et al., 2012)] produces multiple siRNAs that interfere with the immune response, one of which (Bc-siR37) affects FEI2 transcription. In agreement with this observation, At-FEI2 mutant is hypersensitive to B. cinerea infection (Wang et al., 2017). Because of a possible requirement of THE1 for B. cinerea resistance, FEI1 and THE1 could operate in the same signaling pathway (Qu et al., 2017). However, these results were obtained with the hypermorphic allele the1-4, which could lead to misinterpretation of THE1 in resistance to pathogens (Merz et al., 2017).
The most studied RLK subfamily in relation to cell wall signaling, frequently associated with attack by pathogens, is the Catharanthus roseus Receptor-Like Kinase 1-Like (CrRLK1L) (Franck et al., 2018; Ge et al., 2019; Zhu et al., 2021). In A. thaliana, this subfamily consists of 17 members, of which seven have been functionally described (Ge et al., 2019). The CWI CrRLK1L sensor THESEUS1 (THE1), originally identified as a suppressor of the dwarfism phenotype that characterizes the cesa6prc1-1 mutant affected in cellulose synthase, provokes responses that share similarities to those activated by pathogen infections (Hématy et al., 2007; Merz et al., 2017). Cell signaling through THE1 results in the induction of callose and lignin synthesis and enrichment of homogalacturonan, as a compensatory mechanism for the cell wall weakening in A. thaliana (His et al., 2001; Caño-Delgado et al., 2003; Hématy et al., 2007; Bacete et al., 2018; Engelsdorf et al., 2018; Rui and Dinneny, 2020) involving jasmonate, ethylene and salicylic acid, which are hormones often related to plant immunity (Caño-Delgado et al., 2003; Hernández-Blanco et al., 2007; Hématy et al., 2007; Hamann et al., 2009; Shigenaga et al., 2017; Bacete et al., 2018; Engelsdorf et al., 2018). Particularly, in A. thaliana, impairment of cellulose synthesis enhance resistance to Ralstonia solanacearum [a wilt-causing, xylem invading bacteria (Peeters et al., 2013)], Plectosphaerella cucumerina [a root associated necrotrophic fungus (Carlucci et al., 2012)] and B. cinerea (Hernández-Blanco et al., 2007; Bacete et al., 2018; Engelsdorf et al., 2018). However, it seems that THE1-mediated defense responses are more related to the monitoring of CWI during plant cell growth and mechanical or hypo-osmotic stress, than to pattern-triggered immunity responses (Hématy et al., 2007; Engelsdorf et al., 2018). Cell wall damage induces oxidative bursts that can be misinterpreted as pathogen attack (Bacete et al., 2018). An ubiquitous family of secreted peptides known as RALFs (rapid alkalinization factors, which block proton channels, increase extracellular pH and stop cell growth) are ligands for different RLKs (Murphy and De Smet, 2014). Peptide RALF34 is perceived by THE1, which affects cell growth in response to cell wall damage (Gonneau et al., 2018). Interestingly, the RALFs represent a point of convergence/divergence between various signaling pathways potentially involved in the immune response (Zhang et al., 2020b). Several of these peptides are ligands of RLKs, being FERONIA (FER) undoubtedly the most widely studied (Ji et al., 2020) due to its involvement in processes such as gametophyte recognition during sexual reproduction, developmental processes, cell expansion, signaling of different hormones, tolerance to abiotic stress, and plant-pathogen interactions (Ji et al., 2020). In the last case, FER acts as a sensor of CWI upon necrotrophic pathogen cell wall digestive enzymes activity. Particularly, defects in the biosynthesis and modifications of pectin are sensed by FER, activating the ROP6 GTPase pathway that controls the formation of the puzzle-shaped pavement cells in A. thaliana (De Lorenzo et al., 2019; Lin et al., 2022). FER also has a function as a scaffold protein, recruiting a complex that includes the ELONGATION FACTOR THERMO UNSTABLE RECEPTOR (EFR), FLAGELLIN SENSING2 (FLS2), and BAK1 to initiate immune signaling (Stegmann et al., 2017). This scaffold function of FER depends on its interaction with RALF23. In A. thaliana the precursor of RALF23 can be processed by SITE-1 PROTEASE (S1P), preventing complex formation and compromising the immune response (Ji et al., 2020). To regulate immune responses, FER associates in a complex with LORELEI-LIKE GLYCOSYLPHOSPHATIDYLINOSITOLANCHORED PROTEIN1 and 2 (LLG1 and LLG2) and RALF23 (Xiao et al., 2019). A. thaliana fer mutants are resistant to Golovinomyces (syn. Erysiphe) orontii [fungal pathogen that causes powdery mildew (Braun et al., 2019)], suggesting that FER negatively regulates plant immunity to biotrophic pathogens (Kessler et al., 2010). Plant resistance seems to involve ET- and JA-mediated defense pathways since gene marker PDF1.2 (a plant defensin gene induced by both phytohormones) is highly expressed in fer mutants. However, because PR1 (a responsive marker gene for salicylic acid) is expressed at normal levels and the ROS burst is suppressed after the pathogen challenge, it has been postulated that powdery mildew resistance is independent of salicylic acid and ROS in fer mutants (Kessler et al., 2010). FER-mediated resistance mechanisms cannot be generalized, as fer mutants are susceptible to infections by Hyaloperonospora arabidopsidis, a biotrophic oomycete that provokes downy mildew (Coates and Beynon, 2010) and Colletotrichum higginsianum, a hemibiotrophic fungus causative of anthracnose disease (Kessler et al., 2010; Yan et al., 2018). Interestingly, during infection with F. oxysporum, FER is targeted by a RALF mimic secreted by the fungus (F-RALF) (Masachis et al., 2016; Thynne et al., 2017), provoking phosphorylation of H+–ADENOSINE TRIPHOSPHATASE 2 (AHA2) and activating a fungal activated mitogen-protein kinase (Fmk1 -indispensable for fungal pathogenicity), causing an increase of extracellular pH and defense-response inhibition (Segorbe et al., 2017; Nunez-Rodriguez et al., 2020). Similar RALFs are present in 26 species of phytopathogenic fungi, opening the possibility that RALF mimics-mediated inactivation of FER and/or other RLKs is employed by phytopathogens to override plant defense mechanisms (Masachis et al., 2016). Interestingly, in response to Pseudomonas syringae [a bacterial phytopathogen (Xin et al., 2018)], FER-induced immune signaling is independent of its kinase activity (Song et al., 2021), also Meloidogyne incognita [an endoparasitic nematode of the root vascular cylinder (Tapia-Vázquez et al., 2022) produces RALF mimics (MiRALF1/3) that directly bind the extracellular domain of FER, facilitating parasitism (Zhang et al., 2020a). Additionally, proteins ANX1 and ANX2, which are structurally related to FER, form a receptor-coreceptor complex with others RLKs, namely Buddha’s Paper Seal 1/2 (BUPS1/2), and LLG2/3, which perceives RALF signals to promote ROS production. This complex has already been related to the dynamics of pollen tube growth (Boisson-Dernier et al., 2009; Miyazaki et al., 2009), but ROS, in addition to their well-documented role as a wall-modifying agent, also act as a molecular signal that impacts both cell growth and immune responses. Interestingly ANX1 was re-identified in a genetic screen searching for components controlling plant immunity (Mang et al., 2017; Ge et al., 2019). In summary, FER functions can be associated to a positive or negative regulation of immune responses by binding to RALFs or modulating the assembly and activity of RLKs. Despite the many studies on the matter, much research remains to be done to identify FER interactors and the contribution of each one of them to the host-pathogen interaction.
Other types of kinases have been implicated in surveillance of the CWI, such is the case of wall-associated kinases (WAKs) that are distinguished from other receptors by the presence of unique epidermal growth factor (EGF) repeats in the extracellular domain. Up to now they are the only reported receptors with the ability to bind both native cell wall pectin during cell expansion and oligogalacturonides derived from mechanical and pathogen-provoked damage to the cell wall (Kohorn and Kohorn, 2012; Kohorn, 2016). The A. thaliana genome encodes five WAKs, 21 WAK-like genes (WAKL), and genes for truncated proteins or with EGF variations (Verica et al., 2003). WAKL genes have also been identified in several angiosperms including wheat, maize, rice, and tomato, and in the first three cases, they are involved in immune responses (Li et al., 2009; Yang et al., 2014; Zuo et al., 2015; Wu et al., 2020). Interestingly, other components of the WAK signaling pathway, the MAPK6 and the transcription factors EDS1 (ENHANCED DISEASE SUSCEPTIBILITY1) and PAD4, (PHYTOALEXIN DEFICIENT4), all of which are involved in the response to pathogens, seem to converge on this pathway (Joglekar et al., 2018; Dongus and Parker, 2021).
Cell wall leucine-rich repeat extensins (LRX) are a group of cell wall proteins harboring N-terminal leucine-rich repeats (LRR) predicted to bind ligands, and a highly glycosylated C-terminal extensin domain probably involved in the cross-linking of cell wall components, such as pectins. The presence of LRR and extensin domains place the LRX proteins in a great position to sense cell wall signals and transfer this information to downstream components. Three of the eleven LRX encoded in the A. thaliana genome, namely AtLRX 3/4/5, bind peptide RALF23, and interact as a complex with FER causing internalization (Herger et al., 2019). Therefore, LRX represent a link between the cell wall and plasma membrane signaling. The complex AtLRX 3/4/5-RALF23-FER was described in regulation of plant growth and salt stress tolerance but given the important implications of FER on plant immunity (Zhao et al., 2018; Herger et al., 2019, 2020), it is reasonable to think that similar complexes could operate in response to pathogens, although this is pending confirmation. Functional characterization of two A. thaliana xyloglucan-deficient mutants xxt1 and xxt2, affected in xylosyltransferase genes, revealed a link between the cell wall and transcriptional control of polar auxin transport mediated by PINFORMEDs (PINs) and AUX1 (Park and Cosgrove, 2012). Furthermore, promotion of xyloglucan-derived hepta- to nona-saccharides cleavage by fungal hemicellulases, regulate auxin-induced growth mediated by expansins (McDougall and Fry, 1990; Shavrukov and Hirai, 2016; Bacete and Hamann, 2020), through at least a cognate receptor (Claverie et al., 2018). However, auxin plays an important role in plant defense signaling, and altered expression of several genes involved in the immune responses, such as FEI1, FEI2, and FER downregulation, or WAK1 upregulation, in the xxt1 and xxtp2 mutants (Xiao et al., 2016), suggests that perturbations on xyloglucan content, are involved in the signaling processes.
Because CWI signaling pathway is controlled by the activity of different kinase types, it is valid to assume, for balanced purposes, the need for phosphatase activity. In fact, of the 32 members of the group of impotence rescue (ipr) mutants, identified as suppressors of the pollen tube (PT) bursting phenotype that characterizes the double anx1 anx2 mutant plants (Boisson-Dernier et al., 2015), one of them (ipr7) encodes for a Type One Protein Phosphatase (TOPP), named ATUNIS1 (AUN1) that, like its homolog AUN2, is a negative regulator of the CWI maintenance required for the tip growth of the PT (Franck et al., 2018). The possible participation of AUN1/AUN2 and other phosphatases in the CWI signaling pathways outside the tip-growth is an interesting hypothesis to be tested over the next few years.
The cell wall is a source of endogenous elicitors that warn of external danger (Keegstra, 2010; Scheller and Ulvskov, 2010; Mota et al., 2018). When broken down by microbes, polysaccharide fragments are detected by membrane Pattern Recognition Receptors (PRRs), triggering the above-mentioned signaling cascade events for the induction of responses to contain or eliminate the progress of invasion (Saijo et al., 2018). Both Microbe-Associated Molecular Patterns (MAMPs) and/or Damage-Associated Molecular Patterns (DAMPs) induce Pattern-Triggered Immunity (PTI), which can be amplified by feedback loops of endogenous peptides (or phytocytokines) synthesis that also activate PRRs (Gust et al., 2017; Ranf, 2018). PRRs are transmembrane single-pass proteins, with an RLK, or without an intracellular kinase domain and thus known as Receptor-Like Proteins (RLP). These receptors show specificity and high affinity (in the nanomolar range) for their ligands, which could be of protein origin, binding PRRs of the leucine rich repeat category; meanwhile PRRs with LysM motifs recognize N-acetylglucosamine and chitooligosaccharides derived from chitin. Some oligosaccharides are strong elicitors and their size, structure and origin have been reviewed (Zheng et al., 2020). Despite the specificity to various activators, downstream events include pathway crosstalk leading to a general PTI response. In the case of DAMP oligosaccharides, once they interact with their receptors, a fast response occurs resulting in some of the following events: intracellular Ca2+ transients, oxidative bursts, NO accumulation, phosphorylation events by kinase signaling cascades (MAPK) and cell wall reinforcement (Sun and Zhang, 2022). Induction of defense-related genes to produce secondary metabolites with antimicrobial activity and enzymes to digest the microbial wall structures (chitinases, ß-1-3 glucanases), and activation of the ethylene, salicylic acid and jasmonate pathways are late outcomes of the response. Several publications have reviewed PTI responses (Ranf, 2017, 2018; Yuan et al., 2021). In the following paragraphs we describe the induction of the plant defense triggered by the major cell wall-derived DAMPs.
To date, the best-characterized cell wall-derived DAMPs are oligogalacturonides (OGs). OGs are short molecules of α-1,4-linked galacturonosyl residues derived from digested pectin due to microbial activity or by wounding (Ferrari et al., 2013; Claverie et al., 2018; Voxeur et al., 2019). Different response outcomes to OGs depend on: the origin of the microbe involved in the degradation of pectin; plant species; degree of polymerization (DP); and chemical modification of the oligomer (for instance methyl or acetyl esterification). Active OGs are in an egg box conformation that is Ca2+-dependent with optimal DP >9, however, protoplasts have shown responses to large pectin fragments, suggesting that the active size is restricted to its capacity for free movement through the cell wall matrix for reaching the plasma membrane (Ferrari et al., 2013). Most work on OGs comes from fungal infection studies and much less is known from bacterial-plant interactions, but a pectate lyase from Xanthomonas campestris [a systemic xylem pathogen causing black rot disease (Vorhölter et al., 2012; Lowe-Power et al., 2018)] and a endopolygalacturonase from Pectobacterium carotovorum [soft rot disease causal agent (Mansfield et al., 2012)] produce OGs with weak inducing activity at low DP (2–4) but which is optimal at DP >8 (Davis et al., 1984; Norman et al., 1999). In A. thaliana and other species, OGs interact with receptor WALL-ASSOCIATED KINASE 1 (WAK1), a representative of WAK receptors containing an EGF-like ectodomain and intracellular Ser/Thr kinase domain, which in turn also binds Glycine Rich Protein 3 (GRP3) through a different binding domain, with a suggested desensitizing activity to control the response to OGs. WAK1 mainly binds non or low-methyl esterified OGs through interaction with five positive residues (arginines and lysines), four of which are located near to the N-terminal at the extracellular portion of the receptor and one further apart, which in combination allow high affinity for the ligand (Decreux et al., 2006). However, the general responses elicited by OGs in a wide range of plant species are accumulation of phytoalexins, callose deposition, production of ROS and NO that lead to resistance to B. cinerea in A. thaliana (Davis et al., 1986; Bellincampi et al., 2000; Galletti et al., 2008; Rasul et al., 2012; Ferrari et al., 2013; Pontiggia et al., 2020). For instance, Howlader et al. (2020) recently reported that spraying A. thaliana with oligosaccharides derived from partial hydrolysis of pectin (POS) confers resistance to Pseudomonas syringae pv. tomato DC3000 with an optimal concentration of 25 mg/L 3 days before inoculation. ROS, NO, and the expression of genes PR1, PR2, and PR5 all increased with the treatment.
Other cell wall-derived DAMPs are cello-oligosaccharides (or cellodextrins). Fewer reports exist for the eliciting capacities of cellulose and hemicellulose, but recent evidence has shown that oligomers from these polymers also trigger defense responses with some differences to those originated from OGs. Different outcomes relative to cellulose derivative burst have been observed in grapevine (Aziz et al., 2007), rice (Yang et al., 2021) and A. thaliana (Souza et al., 2017). Grapevine responded to cellodextrins with DP >7, which were strong inducers of cytosolic Ca2+ transients and of defensive enzymatic activity [chitinase and ß-1,3 glucanase (Aziz et al., 2007)]. In rice the cello oligosaccharides 31-β-D-cellobiosyl-glucose and 31-β-D-cellotriosyl-glucose are sensed by the CERK1-CEBiP receptor complex, inducing a burst of ROS (Yang et al., 2021). In the case of A. thaliana, no oxidative response was induced after cellobiose (or higher DP cellodextrins) treatment, but phosphorylation of MPK3 and MPK6 rapidly occurred (with a peak at 10 min), resulting in the induction of WRKY30 transcription factor and calcium transients. Cellobiose treatment also upregulates genes associated with phytohormone signaling: SAG101, PAD4 for SA; ACS7 for ET; and LOX3 and LOX4 for jasmonate but the treatment failed to deposit callose or suberin to reinforce the cell wall. Although perception of cellodextrins can saturate, the responsible PRR remains unknown, and analysis of the receptor involved in the response to cellulose indicates that THESEUS is not required in the cellobiose pathway (Aziz et al., 2007; Hématy et al., 2007). Compared to hepta cellodextrin in grapevine, cellobiose response induction in A. thaliana is weaker, but synergizes the response to chitin, flg22 and OGs when applied simultaneously, suggesting different signaling pathways and an auxiliary response to other danger signals (Souza et al., 2017). Additionally, oxidized and native cellulose- or cello-oligosaccharides (DP from 2 to 10), and C1- or C4-oxidized products oxidatively cleaved from cellulose by the microbial enzymes lytic polysaccharide monooxygenases (LPMOs), activate immunity in A. thaliana and tomato and induce resistance to B. cinerea. Upon treatment, two receptors, STRESS INDUCED FACTOR 2 and 4 (SIF2, SIF4), are induced and might form a complex with BAK1 and THE1 that detect these molecules (Zarattini et al., 2021).
Xyloglucan is the main hemicellulose of dicot plants, and in a report by Claverie et al. (2018) authors demonstrated that DP 7 heptamaloxyloglucan (and in minor concentrations also higher DPs) obtained from apple pomace is an elicitor (through a still unknown membrane receptor) in grapevine and in A. thaliana (Claverie et al., 2018). This oligoxyloglucan induces a fast dose-dependent phosphorylation of MAPKs within the first 10 min of treatment, but without a detectable oxidative burst. Late responses to the oligoxyloglucan include defense-related gene induction of the SA- and camalexin-pathways in A. thaliana and PAL (phenyl ammonia lyase) and STS (stilbene synthase) genes of the resveratrol biosynthesis pathway in grapevine (Claverie et al., 2018). A. thaliana mutants of the camalexin-, SA-, ET-, and JA-pathways confirmed their involvement to the presence of oligoxyloglucan as they failed to protect toward a challenge with B. cinerea (Claverie et al., 2018). Additionally, cell wall reinforcement with callose deposition is part of the responses induced by oligoxyloglucan (Claverie et al., 2018; Héloir et al., 2019). In the case of arabinoxylan, it was reported that pentose oligosaccharides with arabinose decorations as the pentasaccharide 33-α-L-arabinofuranosyl-xylotetraose (XA3XX) triggered Ca2+ transients, ROS burst, and induce MAPK3 and MAPK6 phosphorylation in A. thaliana (Mélida et al., 2020). Chemically synthesized mixed-linked glucans or MLGs [β-1,3/1,4-glucans; (1,3;1,4)-β-d-glucans] also trigger immunity, with the trisaccharide MLG43 [β-D-cellobiosyl-(1,3)-β-D-glucose] being the smallest of these molecules to act as a potent pathogen resistance inducer (Rebaque et al., 2021). Other species might also express receptors for MLG as MLG43 protected against oomycete, bacterial and fungal pathogens in A. thaliana, tomato, and pepper, which in this case would be detected as foreign molecule because MLG43 is absent in their cell walls, but present in the cell wall of some microorganisms (Barghahn et al., 2021).
Hyperinduction of immune responses activated by cell wall oligosaccharides is thought to be prevented by oligosaccharide oxidation of the (cellodextrins and oligogalacturonides) by FAD-dependent Berberine Bridge Enzyme-like (BBE-like) proteins with 27 members in A. thaliana, which render them inactive in this state. Oxidizing oligosaccharides also confer resistance to microorganisms that are unable to metabolize them (Benedetti et al., 2018; Locci et al., 2019). However, Zarattini et al. (2021) showed that C1-oxidized cellobionic acid is still able to induce high expression of defense genes.
Because CWI is key for its barrier functions, modification by cell wall proteins can have distinct outcomes for pathogen susceptibility or resistance. Expansin’s importance in plant-microbe interactions is evidenced by a growing number of reports of expansin involvement in different phenotypes (Cosgrove, 2015). Expansins are a family of plant and microbial proteins with cell wall remodeling roles through their binding capacity to cellulose and other cell wall polysaccharides, and hypothetical non-catalytic disruptive activity of weak bonding between polysaccharides (McQueen-Mason and Cosgrove, 1994; Cosgrove, 2015, 2017). Different subclasses of plant expansins act in many physiological and developmental processes that involve controlled cell wall remodeling, and in particular their contribution in cell wall extension is well known (Cosgrove, 2016), yet expansins are also induced under several stressing conditions (Feng et al., 2019; Kong et al., 2019). Additionally, due to their prevalent induction observed in expression profiling analysis under stress, proteins classified as expansin-like type α and type β (subfamilies EXLA and EXLB), have been associated to cell wall modification resulting in (mainly abiotic) stress tolerance (Abuqamar et al., 2013; Marowa et al., 2016; Guimaraes et al., 2017; Chen et al., 2019; Muthusamy et al., 2020; Zhang et al., 2021; Morales-Quintana et al., 2022). Subfamily X (EXLX), includes microbial expansins, from bacteria, fungi and oomycetes, with structural similarity to ß-expansins (Kende et al., 2004; Nikolaidis et al., 2014). Very few reports on microbial expansin activity on the plant cell wall exist, probably in part due to the difficulty on quantifying their effect, although it is known that they also bind cellulose and plant cell walls, but show only weak extension activity in comparison to plant expansins (Kerff et al., 2008; Georgelis et al., 2014, 2015; Olarte-Lozano et al., 2014). As mentioned before, signs of cell wall modification due to microbial activity or induced by abiotic stress are detected by membrane receptors alerting the cell to mount defense and stress responses, sometimes simultaneously. For instance, ectopic overexpression of EXLB8 from Arachis in tobacco results in tolerance to water deficit and resistance to the white mold causative necrotrophic fungus [Sclerotinia sclerotiorum (Hegedus and Rimmer, 2005)], and to the nematode M. incognita, and to drought and simultaneous nematode infection (Brasileiro et al., 2021). Different cell wall nanomechanical properties (possibly stiffer, but more deformable leaves), brought about by EXLB8 overexpression, and consequently, possibly by other six endogenous EXL genes, was suggested as responsible of the phenotype hampering a successful infection. Various biotic and abiotic stress genes of the jasmonate, ethylene and abscisic acid pathways were also induced, activating a more general and unspecific priming of the host against pathogens with important lifestyle differences (Brasileiro et al., 2021). Contrarily, A. thaliana EXLA2 down-regulation provides resistance to B. cinerea and oxidative stress, although roots become sensitive to salt and cold when the medium contains ABA (Abuqamar et al., 2013). Susceptibility to pathogens might be a combination of a stiffer or more relaxed cell wall directly affecting colonization and the defense responses resulting from these changes, although to this day the trigger remains unknown. A clue as to the chemical nature of a possible ligand is exemplified by treatment of celery petioles and A. thaliana leaves with expansin Exl1 from Pectobacterium brasiliense strain BF45, which show resistance to a challenge of BF45 and B. cinerea 24 h after Exl1 infiltration (Narváez-Barragán et al., 2020). Because Exl1 binds to celery cell corners of cells surrounding xylem vessels, which is a site abundant in pectin and due to the seeming solubilization of a phenolic-substituted polysaccharide from isolated Swiss chard vascular bundles in vitro, it has been suggested that pectin could be the ligand triggering ROS bursts, and inducing genes of the jasmonate, ethylene and salicylic acid pathways (Tabuchi et al., 2011; Wang et al., 2016; Tovar-Herrera et al., 2018). More recently, Pi et al. (2022) identified a G-type lectin receptor-like kinase (ERK1, for expansin regulating kinase) that responds to the presence of expansin EXLX1 from Phytophthora capsici [one of the most harmful hemibiotrophic oomycetes (Pi et al., 2022)] in tobacco leaves, providing resistance to the infection. Although the ligand for the receptor remains to be found, this is a first step toward our understanding of the mechanisms underlying perception of wall modification by expansins.
Cell wall modification as a response to abiotic stress or due to pathogen activities induces defense responses resulting in priming of the host locally and systemically, in some cases involving common or shared pathways (Figure 1). Several studies have explored the importance of expansin proteins in this process, being of particular interest those that provide resistance to pathogens, opening the possibility for expansin treatment as a biotechnological application for crop improvement. However, given that a positive effect is not a general outcome, further investigation will tell which expansin treatment would modify the cell wall properties and subsequent defense responses in a manner that is favorable for the host. For this purpose, we need to integrate our knowledge on expansin activity (from both plant and microbial origins) with the mechanisms of the cell wall surveillance and responses to stress. Analysis of possible cell wall physical modification and changes in mechanical properties brought about by expansins, can be done combining traditional techniques with novel methods of cell wall imaging using fluorescence-based probes for different components recently developed (Michels et al., 2020; DeVree et al., 2021). This would shed light on whether resistance or susceptibility are a consequence of a direct cell wall alteration and how this affects microbial invasion, and it will tell whether some expansin types are better than others in providing resistance. With respect to microbial expansins many questions remain unanswered, for instance, which expansin treatment provides resistance (or susceptibility) and to which hosts. Most reverse genetics experiments of bacterial expansins indicate a requirement for proper colonization, except that of Exlx2 from C. michiganensis (Tancos et al., 2018), suggesting that similarly to plant expansins, microbial expansin treatment might result in different phenotypes, and thus, each case must be studied independently. Another important question is determining the identity of trigger stimuli that activate defenses after expansin acts on the cell wall. And finally, what pair of receptor-ligand (a detached polysaccharide fragment maybe) is responsible for intracellular signaling? Further research on this topic will be required to explore the biotechnological potential of expansins as possible biocontrol agents.
Figure 1. Defense responses activated by plant cell wall modification for pathogen resistance. Cell wall integrity (CWI) surveillance receptors, DAMPs and expansins interact with plant cell wall components and elicit defense responses leading, in some cases, to pathogen resistance. Stimuli from the cell wall is transduced to the cell interior through different pathways (arrows), for the induction of hormone-dependent signaling, NO, ROS and Ca2 + transients. CW, cell wall; PM, plasma membrane, ?: unknown receptor; OGs, oligosaccharides; POS, pectin oligosaccharides; COGs, cello-oligosaccharides; CB, cellobiose; CDs, cellodextrins; AX, arabinoxylan; MXG, heptamaloxyloglucan; AtEXLB8, Arabidopsis thaliana expansin-like B; PbExl1, Pectobacterium brasiliense expansin-like 1 protein; PcEXL1, Phytophthora capsici expansin-like 1 protein; THE1, THESEUS1 receptor; FER, FERONIA receptor; WAK1, WALL ASSOCIATED KINASE 1; CERK1-CEBiP, chitin-elicitor receptor kinase 1-chitin-elicitor binding protein receptor complex; ERK1, expansin-regulating kinase 1; NO, Nitric oxide; ROS, reactive oxygen species; MAPK, mitogen-activated protein kinases; SA, salicylic acid; JA, jasmonic acid; ET, ethylene. Unknown ligand,
Cellulose,
Hemicellulose,
Pectin,
Cello-oligosaccharides,
Hemicellulose derivatives,
Pectin derivatives. Figure created with BioRender.com.
All authors listed have made a substantial, direct, and intellectual contribution to the work, and approved it for publication.
This work was received funding from DGAPA (PAPIIT)-UNAM. Grants IN203720 to MS, IN209420 to AG-G, and IN203622 to CM-A.
We would like to thank Chris D. Wood for critical reading of this manuscript.
The authors declare that the research was conducted in the absence of any commercial or financial relationships that could be construed as a potential conflict of interest.
All claims expressed in this article are solely those of the authors and do not necessarily represent those of their affiliated organizations, or those of the publisher, the editors and the reviewers. Any product that may be evaluated in this article, or claim that may be made by its manufacturer, is not guaranteed or endorsed by the publisher.
Abuqamar, S., Ajeb, S., Sham, A., Enan, M. R., and Iratni, R. (2013). A mutation in the expansin-like A2 gene enhances resistance to necrotrophic fungi and hypersensitivity to abiotic stress in Arabidopsis thaliana. Mol. Plant Pathol. 14, 813–827. doi: 10.1111/mpp.12049
Aragón, W., Reina-Pinto, J. J., and Serrano, M. (2017). The intimate talk between plants and microorganisms at the leaf surface. J. Exp. Bot. 68, 5339–5350. doi: 10.1093/jxb/erx327
Aziz, A., Gauthier, A., Bézier, A., Poinssot, B., Joubert, J.-M., Pugin, A., et al. (2007). Elicitor and resistance-inducing activities of β-1,4 cellodextrins in grapevine, comparison with β-1,3 glucans and α-1,4 oligogalacturonides. J. Exp. Bot. 58, 1463–1472. doi: 10.1093/jxb/erm008
Bacete, L., and Hamann, T. (2020). The role of mechanoperception in plant cell wall integrity maintenance. Plants 9:574. doi: 10.3390/plants9050574
Bacete, L., Mélida, H., Miedes, E., and Molina, A. (2018). Plant cell wall-mediated immunity: Cell wall changes trigger disease resistance responses. Plant J. 93, 614–636. doi: 10.1111/tpj.13807
Barghahn, S., Arnal, G., Jain, N., Petutschnig, E., Brumer, H., and Lipka, V. (2021). Mixed linkage β-1,3/1,4-glucan oligosaccharides induce defense responses in Hordeum vulgare and Arabidopsis thaliana. Front. Plant Sci. 12:682439. doi: 10.3389/fpls.2021.682439
Bellincampi, D., Cervone, F., and Lionetti, V. (2014). Plant cell wall dynamics and wall-related susceptibility in plant–pathogen interactions. Front. Plant Sci. 5:228. doi: 10.3389/fpls.2014.00228
Bellincampi, D., Dipierro, N., Salvi, G., Cervone, F., and De Lorenzo, G. (2000). Extracellular H2O2 induced by oligogalacturonides is not involved in the inhibition of the auxin-regulated rolB gene expression in tobacco leaf explants1. Plant Physiol. 122, 1379–1386. doi: 10.1104/pp.122.4.1379
Benedetti, M., Verrascina, I., Pontiggia, D., Locci, F., Mattei, B., De Lorenzo, G., et al. (2018). Four Arabidopsis berberine bridge enzyme-like proteins are specific oxidases that inactivate the elicitor-active oligogalacturonides. Plant J. 94, 260–273. doi: 10.1111/tpj.13852
Boisson-Dernier, A., Franck, C. M., Lituiev, D. S., and Grossniklaus, U. (2015). Receptor-like cytoplasmic kinase MARIS functions downstream of CrRLK1L-dependent signaling during tip growth. Proc. Natl. Acad. Sci. U.S.A. 112, 12211–12216. doi: 10.1073/pnas.1512375112
Boisson-Dernier, A., Roy, S., Kritsas, K., Grobei, M. A., Jaciubek, M., Schroeder, J. I., et al. (2009). Disruption of the pollen-expressed FERONIA homologs ANXUR1 and ANXUR2 triggers pollen tube discharge. Development 136, 3279–3288. doi: 10.1242/dev.040071
Brasileiro, A. C. M., Lacorte, C., Pereira, B. M., Oliveira, T. N., Ferreira, D. S., Mota, A. P. Z., et al. (2021). Ectopic expression of an expansin-like B gene from wild Arachis enhances tolerance to both abiotic and biotic stresses. Plant J. 107, 1681–1696. doi: 10.1111/tpj.15409
Braun, U., Shin, H. D., Takamatsu, S., Meeboon, J., Kiss, L., Lebeda, A., et al. (2019). Phylogeny and taxonomy of Golovinomyces orontii revisited. Mycol. Prog. 18, 335–357. doi: 10.1007/s11557-018-1453-y
Bulgarelli, D., Schlaeppi, K., Spaepen, S., Ver Loren van Themaat, E., and Schulze-Lefert, P. (2013). Structure and functions of the bacterial microbiota of plants. Annu. Rev. Plant Biol. 64, 807–838. doi: 10.1146/annurev-arplant-050312-120106
Caño-Delgado, A., Penfield, S., Smith, C., Catley, M., and Bevan, M. (2003). Reduced cellulose synthesis invokes lignification and defense responses in Arabidopsis thaliana. Plant J. 34, 351–362. doi: 10.1046/j.1365-313x.2003.01729.x
Carlucci, A., Raimondo, M. L., Santos, J., and Phillips, A. J. (2012). Plectosphaerella species associated with root and collar rots of horticultural crops in southern Italy. Persoonia 28, 34–48. doi: 10.3767/003158512x638251
Chen, Y., Zhang, B., Li, C., Lei, C., Kong, C., Yang, Y., et al. (2019). A comprehensive expression analysis of the expansin gene family in potato (Solanum tuberosum) discloses stress-responsive expansin-like B genes for drought and heat tolerances. PLoS One 14:e0219837. doi: 10.1371/journal.pone.0219837
Choi, J. H., Oh, E. S., and Oh, M. H. (2021). Phosphorylation of BIK1 is critical for interaction with downstream signaling components. Genes Genomics 43, 1269–1276. doi: 10.1007/s13258-021-01148-2
Choi, K., Khan, R., and Lee, S.-W. (2021). Dissection of plant microbiota and plant-microbiome interactions. J. Microbiol. 59, 281–291. doi: 10.1007/s12275-021-0619-5
Claverie, J., Balacey, S., Lemaître-Guillier, C., Brulé, D., Chiltz, A., Granet, L., et al. (2018). The cell wall-derived xyloglucan is a new DAMP triggering plant immunity in Vitis vinifera and Arabidopsis thaliana. Front. Plant Sci. 9:1725. doi: 10.3389/fpls.2018.01725
Coates, M. E., and Beynon, J. L. (2010). Hyaloperonospora arabidopsidis as a pathogen model. Annu. Rev. Phytopathol. 48, 329–345. doi: 10.1146/annurev-phyto-080508-094422
Cosgrove, D. J. (2015). Plant expansins: Diversity and interactions with plant cell walls. Curr. Opin. Plant Biol. 25, 162–172. doi: 10.1016/j.pbi.2015.05.014
Cosgrove, D. J. (2016). Catalysts of plant cell wall loosening. F1000Res. 5, F1000FacultyRev–119. doi: 10.12688/f1000research.7180.1
Cosgrove, D. J. (2017). Microbial expansins. Annu. Rev. Microbiol. 71, 479–497. doi: 10.1146/annurev-micro-090816-093315
Cosgrove, D. J. (2018). Diffuse growth of plant cell walls. Plant Physiol. 176, 16–27. doi: 10.1104/pp.17.01541
Cosgrove, D. J. (2022). Building an extensible cell wall. Plant Physiol. 189, 1246–1277. doi: 10.1093/plphys/kiac184
Davis, K. R., Darvill, A. G., Albersheim, P., and Dell, A. (1986). Host-pathogen interactions 1: XXIX. Oligogalacturonides released from sodium polypectate by endopolygalacturonic acid lyase are elicitors of phytoalexins in soybean. Plant Physiol. 80, 568–577. doi: 10.1104/pp.80.2.568
Davis, K. R., Lyon, G. D., Darvill, A. G., and Albersheim, P. (1984). Host-pathogen interactions 1: XXV. Endopolygalacturonic acid lyase from Erwinia carotovora elicits phytoalexin accumulation by releasing plant cell wall fragments. Plant Physiol. 74, 52–60. doi: 10.1104/pp.74.1.52
De Lorenzo, G., Ferrari, S., Giovannoni, M., Mattei, B., and Cervone, F. (2019). Cell wall traits that influence plant development, immunity, and bioconversion. Plant J. 97, 134–147. doi: 10.1111/tpj.14196
Dean, R., Van Kan, J. A. L., Pretorius, Z. A., Hammond-Kosack, K. E., Di Pietro, A., Spanu, P. D., et al. (2012). The Top 10 fungal pathogens in molecular plant pathology. Mol. Plant Pathol. 13, 804–804. doi: 10.1111/j.1364-3703.2012.00822.x
Decreux, A., Thomas, A., Spies, B., Brasseur, R., Cutsem, P. V., and Messiaen, J. (2006). In vitro characterization of the homogalacturonan-binding domain of the wall-associated kinase WAK1 using site-directed mutagenesis. Phytochemistry 67, 1068–1079. doi: 10.1016/j.phytochem.2006.03.009
del Hierro, I., Mélida, H., Broyart, C., Santiago, J., and Molina, A. (2021). Computational prediction method to decipher receptor–glycoligand interactions in plant immunity. Plant J. 105, 1710–1726. doi: 10.1111/tpj.15133
DeVree, B. T., Steiner, L. M., Głazowska, S., Ruhnow, F., Herburger, K., Persson, S., et al. (2021). Current and future advances in fluorescence-based visualization of plant cell wall components and cell wall biosynthetic machineries. Biotechnol. Biofuels 14:78. doi: 10.1186/s13068-021-01922-0
Dievart, A., Gottin, C., Périn, C., Ranwez, V., and Chantret, N. (2020). Origin and Diversity of plant receptor-like kinases. Annu. Rev. Plant Biol. 71, 131–156. doi: 10.1146/annurev-arplant-073019-025927
Dongus, J. A., and Parker, J. E. (2021). EDS1 signalling: At the nexus of intracellular and surface receptor immunity. Curr. Opin. Plant Biol. 62:102039. doi: 10.1016/j.pbi.2021.102039
Edel-Hermann, V., and Lecomte, C. (2018). Current status of Fusarium oxysporum formae speciales and races. Phytopathology 109, 512–530. doi: 10.1094/PHYTO-08-18-0320-RVW
Engelsdorf, T., Gigli-Bisceglia, N., Veerabagu, M., McKenna, J. F., Vaahtera, L., Augstein, F., et al. (2018). The plant cell wall integrity maintenance and immune signaling systems cooperate to control stress responses in Arabidopsis thaliana. Sci. Signal. 11:eaao3070. doi: 10.1126/scisignal.aao3070
Feng, X., Xu, Y., Peng, L., Yu, X., Zhao, Q., Feng, S., et al. (2019). TaEXPB7-B, a β-expansin gene involved in low-temperature stress and abscisic acid responses, promotes growth and cold resistance in Arabidopsis thaliana. J. Plant Physiol. 240:153004. doi: 10.1016/j.jplph.2019.153004
Ferrari, S., Savatin, D., Sicilia, F., Gramegna, G., Cervone, F., and De Lorenzo, G. (2013). Oligogalacturonides: Plant damage-associated molecular patterns and regulators of growth and development. Front. Plant Sci. 4:49. doi: 10.3389/fpls.2013.00049
Franck, C. M., Westermann, J., and Boisson-Dernier, A. (2018). Plant malectin-like receptor kinases: From cell wall integrity to immunity and beyond. Annu. Rev. Plant Biol. 69, 301–328. doi: 10.1146/annurev-arplant-042817-040557
Galletti, R., Denoux, C., Gambetta, S., Dewdney, J., Ausubel, F. M., De Lorenzo, G., et al. (2008). The AtrbohD-mediated oxidative burst elicited by oligogalacturonides in Arabidopsis is dispensable for the activation of defense responses effective against Botrytis cinerea. Plant Physiol. 148, 1695–1706. doi: 10.1104/pp.108.127845
Ge, Z., Dresselhaus, T., and Qu, L.-J. (2019). How CrRLK1L receptor complexes perceive RALF signals. Trends Plant Sci. 24, 978–981. doi: 10.1016/j.tplants.2019.09.002
Georgelis, N., Nikolaidis, N., and Cosgrove, D. J. (2014). Biochemical analysis of expansin-like proteins from microbes. Carbohydr. Polym. 100, 17–23. doi: 10.1016/j.carbpol.2013.04.094
Georgelis, N., Nikolaidis, N., and Cosgrove, D. J. (2015). Bacterial expansins and related proteins from the world of microbes. Appl. Microbiol. Biotechnol. 99, 3807–3823. doi: 10.1007/s00253-015-6534-0
Gigli-Bisceglia, N., Engelsdorf, T., and Hamann, T. (2020). Plant cell wall integrity maintenance in model plants and crop species-relevant cell wall components and underlying guiding principles. Cell. Mol. Life Sci. 77, 2049–2077. doi: 10.1007/s00018-019-03388-8
Gonneau, M., Desprez, T., Martin, M., Doblas, V. G., Bacete, L., Miart, F., et al. (2018). Receptor kinase THESEUS1 is a rapid alkalinization factor 34 receptor in Arabidopsis. Curr. Biol. 28, 2452.e–2458.e. doi: 10.1016/j.cub.2018.05.075
Grones, P., Raggi, S., and Robert, S. (2019). FORCE-ing the shape. Curr. Opin. Plant Biol. 52, 1–6. doi: 10.1016/j.pbi.2019.05.008
Guerringue, Y., Thomine, S., and Frachisse, J.-M. (2018). Sensing and transducing forces in plants with MSL10 and DEK1 mechanosensors. FEBS Lett. 592, 1968–1979. doi: 10.1002/1873-3468.13102
Guimaraes, L. A., Mota, A. P. Z., Araujo, A. C. G., de Alencar Figueiredo, L. F., Pereira, B. M., de Passos Saraiva, M. A., et al. (2017). Genome-wide analysis of expansin superfamily in wild Arachis discloses a stress-responsive expansin-like B gene. Plant Mol. Biol. 94, 79–96. doi: 10.1007/s11103-017-0594-8
Gust, A. A., Pruitt, R., and Nürnberger, T. (2017). Sensing danger: Key to activating plant immunity. Trends Plant Sci. 22, 779–791. doi: 10.1016/j.tplants.2017.07.005
Hamann, T., Bennett, M., Mansfield, J., and Somerville, C. (2009). Identification of cell-wall stress as a hexose-dependent and osmosensitive regulator of plant responses. Plant J. 57, 1015–1026. doi: 10.1111/j.1365-313X.2008.03744.x
Haswell, E. S., and Meyerowitz, E. M. (2006). MscS-like proteins control plastid size and shape in Arabidopsis thaliana. Curr. Biol. 16, 1–11. doi: 10.1016/j.cub.2005.11.044
Hegedus, D. D., and Rimmer, S. R. (2005). Sclerotinia sclerotiorum: When “to be or not to be” a pathogen? FEMS Microbiol. Lett. 251, 177–184. doi: 10.1016/j.femsle.2005.07.040
Héloir, M.-C., Adrian, M., Brulé, D., Claverie, J., Cordelier, S., Daire, X., et al. (2019). Recognition of Elicitors in grapevine: From MAMP and DAMP perception to induced resistance. Front. Plant Sci. 10:1117. doi: 10.3389/fpls.2019.01117
Hématy, K., Sado, P.-E., Van Tuinen, A., Rochange, S., Desnos, T., Balzergue, S., et al. (2007). A receptor-like kinase mediates the response of Arabidopsis cells to the inhibition of cellulose synthesis. Curr. Biol. 17, 922–931. doi: 10.1016/j.cub.2007.05.018
Herger, A., Dünser, K., Kleine-Vehn, J., and Ringli, C. (2019). Leucine-Rich repeat extensin proteins and their role in cell wall sensing. Curr. Biol. 29, R851–R858. doi: 10.1016/j.cub.2019.07.039
Herger, A., Gupta, S., Kadler, G., Franck, C. M., Boisson-Dernier, A., and Ringli, C. (2020). Overlapping functions and protein-protein interactions of LRR-extensins in Arabidopsis. PLoS Genet. 16:e1008847. doi: 10.1371/journal.pgen.1008847
Hernández-Blanco, C., Feng, D. X., Hu, J., Sánchez-Vallet, A., Deslandes, L., Llorente, F., et al. (2007). Impairment of cellulose synthases required for Arabidopsis secondary cell wall formation enhances disease resistance. Plant Cell 19, 890–903. doi: 10.1105/tpc.106.048058
His, I., Driouich, A., Nicol, F., Jauneau, A., and Höfte, H. (2001). Altered pectin composition in primary cell walls of korrigan, a dwarf mutant of Arabidopsis deficient in a membrane-bound endo-1,4-β-glucanase. Planta 212, 348–358. doi: 10.1007/s004250000437
Hofmann, A., Müller, S., Drechsler, T., Berleth, M., Caesar, K., Rohr, L., et al. (2020). High-level expression, purification and initial characterization of recombinant Arabidopsis histidine kinase AHK1. Plants 9:304. doi: 10.3390/plants9030304
Hou, S., Liu, D., Huang, S., Luo, D., Liu, Z., Xiang, Q., et al. (2021). The Arabidopsis MIK2 receptor elicits immunity by sensing a conserved signature from phytocytokines and microbes. Nat. Commun. 12:5494. doi: 10.1038/s41467-021-25580-w
Hou, S., Liu, Z., Shen, H., and Wu, D. (2019). Damage-associated molecular pattern-triggered immunity in plants. Front. Plant Sci. 10:646. doi: 10.3389/fpls.2019.00646
Howlader, P., Bose, S. K., Zhang, C., Jia, X., Wang, W., and Yin, H. (2020). Pectin oligosaccharide (POS) induce resistance in Arabidopsis thaliana through salicylic acid-mediated signaling pathway against Pseudomonas syringae pv.tomato DC3000. Physiol. Mol. Plant Pathol. 110:101483. doi: 10.1016/j.pmpp.2020.101483
Ji, D., Chen, T., Zhang, Z., Li, B., and Tian, S. (2020). Versatile roles of the receptor-like kinase Feronia in plant growth, development and host-pathogen interaction. Int. J. Mol. Sci. 21:7881. doi: 10.3390/ijms21217881
Joglekar, S., Suliman, M., Bartsch, M., Halder, V., Maintz, J., Bautor, J., et al. (2018). Chemical activation of EDS1/PAD4 signaling leading to pathogen resistance in Arabidopsis. Plant Cell Physiol. 59, 1592–1607. doi: 10.1093/pcp/pcy106
Jose, J., Ghantasala, S., and Roy Choudhury, S. (2020). Arabidopsis transmembrane receptor-like kinases (RLKs): A bridge between extracellular signal and intracellular regulatory machinery. Int. J. Mol. Sci. 21:4000. doi: 10.3390/ijms21114000
Kende, H., Bradford, K., Brummell, D., Cho, H. T., Cosgrove, D., Fleming, A., et al. (2004). Nomenclature for members of the expansin superfamily of genes and proteins. Plant Mol. Biol. 55, 311–314. doi: 10.1007/s11103-004-0158-6
Kerff, F., Amoroso, A., Herman, R., Sauvage, E., Petrella, S., Filée, P., et al. (2008). Crystal structure and activity of Bacillus subtilis YoaJ (EXLX1), a bacterial expansin that promotes root colonization. Proc. Natl. Acad. Sci. U.S.A. 105, 16876–16881. doi: 10.1073/pnas.0809382105
Kessler, S. A., Shimosato-Asano, H., Keinath, N. F., Wuest, S. E., Ingram, G., Panstruga, R., et al. (2010). Conserved molecular components for pollen tube reception and fungal invasion. Science 330, 968–971. doi: 10.1126/science.1195211
Kohorn, B. D. (2016). Cell wall-associated kinases and pectin perception. J. Exp. Bot. 67, 489–494. doi: 10.1093/jxb/erv467
Kohorn, B., and Kohorn, S. (2012). The cell wall-associated kinases, WAKs, as pectin receptors. Front. Plant Sci. 3:88. doi: 10.3389/fpls.2012.00088
Kong, Y., Wang, B., Du, H., Li, W., Li, X., and Zhang, C. (2019). GmEXLB1, a soybean expansin-like B gene, alters root architecture to improve phosphorus acquisition in Arabidopsis. Front. Plant Sci. 10:808. doi: 10.3389/fpls.2019.00808
Lemanceau, P., Barret, M., Mazurier, S., Mondy, S., Pivato, B., Fort, T., et al. (2017). “Chapter five – plant communication with associated microbiota in the spermosphere, rhizosphere and phyllosphere,” in Advances in botanical research, ed. G. Becard (San Diego, CA: Academic Press), 101–133. doi: 10.1016/bs.abr.2016.10.007
Li, H., Zhou, S.-Y., Zhao, W.-S., Su, S.-C., and Peng, Y.-L. (2009). A novel wall-associated receptor-like protein kinase gene, OsWAK1, plays important roles in rice blast disease resistance. Plant Mol. Biol. 69, 337–346. doi: 10.1007/s11103-008-9430-5
Li, N., Han, X., Feng, D., Yuan, D., and Huang, L.-J. (2019). Signaling crosstalk between salicylic acid and ethylene/jasmonate in plant defense: Do we understand what they are whispering? Int. J. Mol. Sci. 20:671. doi: 10.3390/ijms20030671
Lin, W., Tang, W., Pan, X., Huang, A., Gao, X., Anderson, C. T., et al. (2022). Arabidopsis pavement cell morphogenesis requires FERONIA binding to pectin for activation of ROP GTPase signaling. Curr. Biol. 32, 497.e–507.e. doi: 10.1016/j.cub.2021.11.030
Locci, F., Benedetti, M., Pontiggia, D., Citterico, M., Caprari, C., Mattei, B., et al. (2019). An Arabidopsis berberine bridge enzyme-like protein specifically oxidizes cellulose oligomers and plays a role in immunity. Plant J. 98, 540–554. doi: 10.1111/tpj.14237
Lowe-Power, T. M., Khokhani, D., and Allen, C. (2018). How Ralstonia solanacearum exploits and thrives in the flowing plant xylem environment. Trends Microbiol. 26, 929–942. doi: 10.1016/j.tim.2018.06.002
Lozano-Elena, F., and Caño-Delgado, A. I. (2019). Emerging roles of vascular brassinosteroid receptors of the BRI1-like family. Curr. Opin. Plant Biol. 51, 105–113. doi: 10.1016/j.pbi.2019.06.006
Mang, H., Feng, B., Hu, Z., Boisson-Dernier, A., Franck, C. M., Meng, X., et al. (2017). Differential regulation of two-tiered plant immunity and sexual reproduction by ANXUR receptor-like kinases. Plant Cell 29, 3140–3156. doi: 10.1105/tpc.17.00464
Mansfield, J., Genin, S., Magori, S., Citovsky, V., Sriariyanum, M., Ronald, P., et al. (2012). Top 10 plant pathogenic bacteria in molecular plant pathology. Mol. Plant Pathol. 13, 614–629. doi: 10.1111/j.1364-3703.2012.00804.x
Marowa, P., Ding, A., and Kong, Y. (2016). Expansins: Roles in plant growth and potential applications in crop improvement. Plant Cell Rep. 35, 949–965. doi: 10.1007/s00299-016-1948-4
Masachis, S., Segorbe, D., Turrà, D., Leon-Ruiz, M., Fürst, U., El Ghalid, M., et al. (2016). A fungal pathogen secretes plant alkalinizing peptides to increase infection. Nat. Microbiol. 1:16043. doi: 10.1038/nmicrobiol.2016.43
McDougall, G. J., and Fry, S. C. (1990). Xyloglucan oligosaccharides promote growth and activate cellulase: Evidence for a role of cellulase in cell expansion 1. Plant Physiol. 93, 1042–1048. doi: 10.1104/pp.93.3.1042
McQueen-Mason, S., and Cosgrove, D. J. (1994). Disruption of hydrogen bonding between plant cell wall polymers by proteins that induce wall extension. Proc. Natl. Acad. Sci. U.S.A. 91, 6574–6578. doi: 10.1073/pnas.91.14.6574
Mélida, H., Bacete, L., Ruprecht, C., Rebaque, D., del Hierro, I., López, G., et al. (2020). Arabinoxylan-oligosaccharides act as damage associated molecular patterns in plants regulating disease resistance. Front. Plant Sci. 11:1210. doi: 10.3389/fpls.2020.01210
Merz, D., Richter, J., Gonneau, M., Sanchez-Rodriguez, C., Eder, T., Sormani, R., et al. (2017). T-DNA alleles of the receptor kinase THESEUS1 with opposing effects on cell wall integrity signaling. J. Exp. Bot. 68, 4583–4593. doi: 10.1093/jxb/erx263
Michels, L., Gorelova, V., Harnvanichvech, Y., Borst, J. W., Albada, B., Weijers, D., et al. (2020). Complete microviscosity maps of living plant cells and tissues with a toolbox of targeting mechanoprobes. Proc. Natl. Acad. Sci. U.S.A. 117, 18110–18118. doi: 10.1073/pnas.1921374117
Mitsumasu, K., Seto, Y., and Yoshida, S. (2015). Apoplastic interactions between plants and plant root intruders. Front. Plant Sci. 6:617. doi: 10.3389/fpls.2015.00617
Miyazaki, S., Murata, T., Sakurai-Ozato, N., Kubo, M., Demura, T., Fukuda, H., et al. (2009). ANXUR1 and 2, sister genes to FERONIA/SIRENE, are male factors for coordinated fertilization. Curr. Biol. 19, 1327–1331. doi: 10.1016/j.cub.2009.06.064
Molina, A., Miedes, E., Bacete, L., Rodríguez, T., Mélida, H., Denancé, N., et al. (2021). Arabidopsis cell wall composition determines disease resistance specificity and fitness. Proc. Natl. Acad. Sci. U.S.A. 118:e2010243118. doi: 10.1073/pnas.2010243118
Molina, A., O’Neill, M. A., Darvill, A. G., Etzler, M. E., Mohnen, D., Hahn, M. G., et al. (2022). “Free glycans as bioactive molecules,” in Essentials of glycobiology, eds A. Varki, R. D. Cummings, J. D. Esko, P. Stanley, G. W. Hart, M. Aebi, et al. (Cold Spring Harbor, NY: Cold Spring Harbor Laboratory Press), 539–548.
Morales-Quintana, L., Tapia-Valdebenito, D., Castro, R. I., Rabert, C., Larama, G., Gutiérrez, A., et al. (2022). Characterization of the cell wall component through thermogravimetric analysis and its relationship with an expansin-like protein in Deschampsia antarctica. Int. J. Mol. Sci. 23:5741. doi: 10.3390/ijms23105741
Mota, T. R., Oliveira, D. M., Rogério Marchiosi, O., and Ferrarese-Filho Santos, W. D. (2018). Plant cell wall composition and enzymatic deconstruction. AIMS Bioengineering 5, 63–77. doi: 10.3934/bioeng.2018.1.63
Murphy, E., and De Smet, I. (2014). Understanding the RALF family: A tale of many species. Trends Plant Sci. 19, 664–671. doi: 10.1016/j.tplants.2014.06.005
Muthusamy, M., Kim, J. Y., Yoon, E. K., Kim, J. A., and Lee, S. I. (2020). BrEXLB1, a Brassica rapa expansin-like B1 gene is associated with root development, drought stress response, and seed germination. Genes 11:404. doi: 10.3390/genes11040404
Narváez-Barragán, D. A., Tovar-Herrera, O. E., Torres, M., Rodríguez, M., Humphris, S., Toth, I. K., et al. (2020). Expansin-like Exl1 from pectobacterium is a virulence factor required for host infection, and induces a defence plant response involving ROS, and jasmonate, ethylene and salicylic acid signalling pathways in Arabidopsis thaliana. Sci. Rep. 10:7747. doi: 10.1038/s41598-020-64529-9
Nikolaidis, N., Doran, N., and Cosgrove, D. J. (2014). Plant expansins in bacteria and fungi: Evolution by horizontal gene transfer and independent domain fusion. Mol. Biol. Evol. 31, 376–386. doi: 10.1093/molbev/mst206
Norman, C., Vidal, S., and Palva, E. T. (1999). Oligogalacturonide-mediated induction of a gene involved in jasmonic acid synthesis in response to the cell-wall-degrading enzymes of the plant pathogen Erwinia carotovora. Mol. Plant Microbe Interact. 12, 640–644. doi: 10.1094/MPMI.1999.12.7.640
Nunez-Rodriguez, J. C., Ruiz-Roldán, C., Lemos, P., Membrives, S., and Hera, C. (2020). The phosphatase Ptc6 is involved in virulence and MAPK signalling in Fusarium oxysporum. Mol. Plant Pathol. 21, 206–217. doi: 10.1111/mpp.12889
Olarte-Lozano, M., Mendoza-Nuñez, M. A., Pastor, N., Segovia, L., Folch-Mallol, J., and Martínez-Anaya, C. (2014). PcExl1 a novel acid expansin-like protein from the plant pathogen Pectobacterium carotovorum, Binds cell walls differently to BsEXLX1. PLoS One 9:e95638. doi: 10.1371/journal.pone.0095638
Park, Y. B., and Cosgrove, D. J. (2012). Changes in cell wall biomechanical properties in the xyloglucan-deficient xxt1/xxt2 mutant of Arabidopsis. Plant Physiol. 158, 465–475. doi: 10.1104/pp.111.189779
Peeters, N., Guidot, A., Vailleau, F., and Valls, M. (2013). Ralstonia solanacearum, a widespread bacterial plant pathogen in the post-genomic era. Mol. Plant Pathol. 14, 651–662. doi: 10.1111/mpp.12038
Pham, J., Liu, J., Bennett, M. H., Mansfield, J. W., and Desikan, R. (2012). Arabidopsis histidine kinase 5 regulates salt sensitivity and resistance against bacterial and fungal infection. New Phytol. 194, 168–180. doi: 10.1111/j.1469-8137.2011.04033.x
Pi, L., Yin, Z., Duan, W., Wang, N., Zhang, Y., Wang, J., et al. (2022). A G-type lectin receptor-like kinase regulates the perception of oomycete apoplastic expansin-like proteins. J. Integr. Plant Biol. 64, 183–201. doi: 10.1111/jipb.13194
Pontiggia, D., Benedetti, M., Costantini, S., De Lorenzo, G., and Cervone, F. (2020). Dampening the DAMPs: How plants maintain the homeostasis of cell wall molecular patterns and avoid hyper-immunity. Front. Plant Sci. 11:613259. doi: 10.3389/fpls.2020.613259
Qu, S., Zhang, X., Song, Y., Lin, J., and Shan, X. (2017). THESEUS1 positively modulates plant defense responses against Botrytis cinerea through GUANINE EXCHANGE FACTOR4 signaling. J. Integr. Plant Biol. 59, 797–804. doi: 10.1111/jipb.12565
Quintana-Rodriguez, E., Duran-Flores, D., Heil, M., and Camacho-Coronel, X. (2018). Damage-associated molecular patterns (DAMPs) as future plant vaccines that protect crops from pests. Sci. Hortic. 237, 207–220. doi: 10.1016/j.scienta.2018.03.026
Ranf, S. (2017). Sensing of molecular patterns through cell surface immune receptors. Curr. Opin. Plant Biol. 38, 68–77. doi: 10.1016/j.pbi.2017.04.011
Ranf, S. (2018). Pattern recognition receptors—versatile genetic tools for engineering broad-spectrum disease resistance in crops. Agronomy 8:134.
Rasul, S., Dubreuil-Maurizi, C., Lamotte, O., Koen, E., Poinssot, B., Alcaraz, G., et al. (2012). Nitric oxide production mediates oligogalacturonide-triggered immunity and resistance to Botrytis cinerea in Arabidopsis thaliana. Plant Cell Environ. 35, 1483–1499. doi: 10.1111/j.1365-3040.2012.02505.x
Rebaque, D., del Hierro, I., López, G., Bacete, L., Vilaplana, F., Dallabernardina, P., et al. (2021). Cell wall-derived mixed-linked β-1,3/1,4-glucans trigger immune responses and disease resistance in plants. Plant J. 106, 601–615. doi: 10.1111/tpj.15185
Rhodes, J., Yang, H., Moussu, S., Boutrot, F., Santiago, J., and Zipfel, C. (2021). Perception of a divergent family of phytocytokines by the Arabidopsis receptor kinase MIK2. Nat. Commun. 12:705. doi: 10.1038/s41467-021-20932-y
Rongpipi, S., Ye, D., Gomez, E. D., and Gomez, E. W. (2019). Progress and Opportunities in the characterization of cellulose – an important regulator of cell wall growth and mechanics. Front. Plant Sci. 9:1894. doi: 10.3389/fpls.2018.01894
Rui, Y., and Dinneny, J. R. (2020). A wall with integrity: Surveillance and maintenance of the plant cell wall under stress. New Phytol. 225, 1428–1439. doi: 10.1111/nph.16166
Saijo, Y., Loo, E. P., and Yasuda, S. (2018). Pattern recognition receptors and signaling in plant-microbe interactions. Plant J. 93, 592–613. doi: 10.1111/tpj.13808
Scheller, H. V., and Ulvskov, P. (2010). Hemicelluloses. Annu. Rev. Plant Biol. 61, 263–289. doi: 10.1146/annurev-arplant-042809-112315
Schlegel, A. M., and Haswell, E. S. (2020). Charged pore-lining residues are required for normal channel kinetics in the eukaryotic mechanosensitive ion channel MSL1. Channels 14, 310–325. doi: 10.1080/19336950.2020.1818509
Segorbe, D., Di Pietro, A., Pérez-Nadales, E., and Turrà, D. (2017). Three Fusarium oxysporum mitogen-activated protein kinases (MAPKs) have distinct and complementary roles in stress adaptation and cross-kingdom pathogenicity. Mol. Plant Pathol. 18, 912–924. doi: 10.1111/mpp.12446
Seifert, G. J. (2021). The FLA4-FEI pathway: A unique and mysterious signaling module related to cell wall structure and stress signaling. Genes 12:145. doi: 10.3390/genes12020145
Serrano, M., Torres, M., Coluccia, F., L’Haridon, F., and Metraux, J.-P. (2014). The cuticle and plant defense to pathogens. Front. Plant Sci. 5:274. doi: 10.3389/fpls.2014.00274
Shavrukov, Y., and Hirai, Y. (2016). Good and bad protons: Genetic aspects of acidity stress responses in plants. J. Exp. Bot. 67, 15–30. doi: 10.1093/jxb/erv437
Shigenaga, A. M., Berens, M. L., Tsuda, K., and Argueso, C. T. (2017). Towards engineering of hormonal crosstalk in plant immunity. Curr. Opin. Plant Biol. 38, 164–172. doi: 10.1016/j.pbi.2017.04.021
Song, Y., Wilson, A. J., Zhang, X.-C., Thoms, D., Sohrabi, R., Song, S., et al. (2021). FERONIA restricts Pseudomonas in the rhizosphere microbiome via regulation of reactive oxygen species. Nat. Plants 7, 644–654. doi: 10.1038/s41477-021-00914-0
Souza, C. A., Li, S., Lin, A. Z., Boutrot, F., Grossmann, G., Zipfel, C., et al. (2017). Cellulose-derived oligomers act as damage-associated molecular patterns and trigger defense-like responses. Plant Physiol. 173, 2383–2398. doi: 10.1104/pp.16.01680
Srivastava, A. K., Zhang, W., Schneider, J., Rogach, A. L., Chigrinov, V. G., and Kwok, H.-S. (2017). Photoaligned nanorod enhancement films with polarized emission for liquid-crystal-display applications. Adv. Mater. 29:1701091. doi: 10.1002/adma.201701091
Srivastava, V., McKee, L.S., and Bulone, V. (2017). “Plant cell walls,” in eLS (Chichester: John Wiley & Sons Ltd). doi: 10.1002/9780470015902.a0001682.pub3
Stegmann, M., Monaghan, J., Smakowska-Luzan, E., Rovenich, H., Lehner, A., Holton, N., et al. (2017). The receptor kinase FER is a RALF-regulated scaffold controlling plant immune signaling. Science 355, 287–289. doi: 10.1126/science.aal2541
Sun, T., and Zhang, Y. (2022). MAP kinase cascades in plant development and immune signaling. EMBO Rep. 23:e53817. doi: 10.15252/embr.202153817
Tabuchi, A., Li, L.-C., and Cosgrove, D. J. (2011). Matrix solubilization and cell wall weakening by β-expansin (group-1 allergen) from maize pollen. Plant J. 68, 546–559. doi: 10.1111/j.1365-313X.2011.04705.x
Tancos, M. A., Lowe-Power, T. M., Peritore-Galve, F. C., Tran, T. M., Allen, C., and Smart, C. D. (2018). Plant-like bacterial expansins play contrasting roles in two tomato vascular pathogens. Mol. Plant Pathol. 19, 1210–1221. doi: 10.1111/mpp.12611
Tapia-Vázquez, I., Montoya-Martínez, A. C., De Los Santos-Villalobos, S., Ek-Ramos, M. J., Montesinos-Matías, R., and Martínez-Anaya, C. (2022). Root-knot nematodes (Meloidogyne spp.) a threat to agriculture in Mexico: Biology, current control strategies, and perspectives. World J. Microbiol. Biotechnol. 38:26. doi: 10.1007/s11274-021-03211-2
Thynne, E., Saur, I. M. L., Simbaqueba, J., Ogilvie, H. A., Gonzalez-Cendales, Y., Mead, O., et al. (2017). Fungal phytopathogens encode functional homologues of plant rapid alkalinization factor (RALF) peptides. Mol. Plant Pathol. 18, 811–824. doi: 10.1111/mpp.12444
Tovar-Herrera, O. E., Rodríguez, M., Olarte-Lozano, M., Sampedro-Guerrero, J. A., Guerrero, A., Pinto-Cámara, R., et al. (2018). Analysis of the binding of expansin Exl1, from Pectobacterium carotovorum, to plant Xylem and comparison to EXLX1 from Bacillus subtilis. ACS Omega 3, 7008–7018. doi: 10.1021/acsomega.8b00406
Van der Does, D., Boutrot, F., Engelsdorf, T., Rhodes, J., McKenna, J. F., Vernhettes, S., et al. (2017). The Arabidopsis leucine-rich repeat receptor kinase MIK2/LRR-KISS connects cell wall integrity sensing, root growth and response to abiotic and biotic stresses. PLoS Genet. 13:e1006832. doi: 10.1371/journal.pgen.1006832
Verica, J. A., Chae, L., Tong, H., Ingmire, P., and He, Z.-H. (2003). Tissue-specific and developmentally regulated expression of a cluster of tandemly arrayed cell wall-associated kinase-like kinase genes in Arabidopsis. Plant Physiol. 133, 1732–1746. doi: 10.1104/pp.103.028530
Vorhölter, F.-J., Wiggerich, H.-G., Scheidle, H., Sidhu, V. K., Mrozek, K., Küster, H., et al. (2012). Involvement of bacterial TonB-dependent signaling in the generation of an oligogalacturonide damage-associated molecular pattern from plant cell walls exposed to Xanthomonas campestris pv. Campestris pectate lyases. BMC Microbiol. 12:239. doi: 10.1186/1471-2180-12-239
Voxeur, A., Habrylo, O., Guénin, S., Miart, F., Soulié, M.-C., Rihouey, C., et al. (2019). Oligogalacturonide production upon Arabidopsis thaliana–Botrytis cinerea interaction. Proc. Natl. Acad. Sci. U.S.A. 116, 19743–19752. doi: 10.1073/pnas.1900317116
Wang, M., Weiberg, A., Dellota, E., Yamane, D., and Jin, H. (2017). Botrytis small RNA Bc-siR37 suppresses plant defense genes by cross-kingdom RNAi. RNA Biol. 14, 421–428. doi: 10.1080/15476286.2017.1291112
Wang, T., Chen, Y., Tabuchi, A., Cosgrove, D. J., and Hong, M. (2016). The target of β-Expansin EXPB1 in maize cell walls from binding and solid-state NMR studies. Plant Physiol. 172, 2107–2119. doi: 10.1104/pp.16.01311
Wang, T., Park, Y. B., Cosgrove, D. J., and Hong, M. (2015). Cellulose-pectin spatial contacts are inherent to never-dried Arabidopsis primary cell walls: Evidence from solid-state nuclear magnetic resonance. Plant Physiol. 168, 871–884. doi: 10.1104/pp.15.00665
Wolf, S. (2017). Plant cell wall signalling and receptor-like kinases. Biochem. J. 474, 471–492. doi: 10.1042/bcj20160238
Wolf, S. (2022). Cell wall signaling in plant development and defense. Annu. Rev. Plant Biol. 73, 323–353. doi: 10.1146/annurev-arplant-102820-095312
Wu, X., Bacic, A., Johnson, K. L., and Humphries, J. (2020). The role of Brachypodium distachyon wall-associated kinases (WAKs) in cell expansion and stress responses. Cells 9:2478. doi: 10.3390/cells9112478
Xiao, C., Zhang, T., Zheng, Y., Cosgrove, D. J., and Anderson, C. T. (2016). Xyloglucan deficiency disrupts microtubule stability and cellulose biosynthesis in Arabidopsis, altering cell growth and morphogenesis. Plant Physiol. 170, 234–249. doi: 10.1104/pp.15.01395
Xiao, Y., Stegmann, M., Han, Z., DeFalco, T. A., Parys, K., Xu, L., et al. (2019). Mechanisms of RALF peptide perception by a heterotypic receptor complex. Nature 572, 270–274. doi: 10.1038/s41586-019-1409-7
Xin, X.-F., Kvitko, B., and He, S. Y. (2018). Pseudomonas syringae: What it takes to be a pathogen. Nat. Rev. Microbiol. 16, 316–328. doi: 10.1038/nrmicro.2018.17
Yan, Y., Yuan, Q., Tang, J., Huang, J., Hsiang, T., Wei, Y., et al. (2018). Colletotrichum higginsianum as a model for understanding host–pathogen interactions: A review. Int. J. Mol. Sci. 19:2142. doi: 10.3390/ijms19072142
Yang, C., Liu, R., Pang, J., Ren, B., Zhou, H., Wang, G., et al. (2021). Poaceae-specific cell wall-derived oligosaccharides activate plant immunity via OsCERK1 during Magnaporthe oryzae infection in rice. Nat. Commun. 12:2178. doi: 10.1038/s41467-021-22456-x
Yang, K., Qi, L., and Zhang, Z. (2014). Isolation and characterization of a novel wall-associated kinase gene TaWAK5 in wheat (Triticum aestivum). Crop J. 2, 255–266. doi: 10.1016/j.cj.2014.04.010
Yang, Y., Labbé, J., Muchero, W., Yang, X., Jawdy, S. S., Kennedy, M., et al. (2016). Genome-wide analysis of lectin receptor-like kinases in Populus. BMC Genom. 17:699. doi: 10.1186/s12864-016-3026-2
Yuan, M., Ngou, B. P. M., Ding, P., and Xin, X.-F. (2021). PTI-ETI crosstalk: An integrative view of plant immunity. Curr. Opin. Plant Biol. 62:102030. doi: 10.1016/j.pbi.2021.102030
Zablackis, E., Huang, J., Muller, B., Darvill, A. G., and Albersheim, P. (1995). Characterization of the cell-wall polysaccharides of Arabidopsis thaliana leaves. Plant Physiol. 107, 1129–1138. doi: 10.1104/pp.107.4.1129
Zarattini, M., Corso, M., Kadowaki, M. A., Monclaro, A., Magri, S., Milanese, I., et al. (2021). LPMO-oxidized cellulose oligosaccharides evoke immunity in Arabidopsis conferring resistance towards necrotrophic fungus B. cinerea. Commun. Biol. 4:727. doi: 10.1038/s42003-021-02226-7
Zhang, B., Chang, L., Sun, W., Ullah, A., and Yang, X. (2021). Overexpression of an expansin-like gene, GhEXLB2 enhanced drought tolerance in cotton. Plant Physiol. Biochem. 162, 468–475. doi: 10.1016/j.plaphy.2021.03.018
Zhang, M., Su, J., Zhang, Y., Xu, J., and Zhang, S. (2018). Conveying endogenous and exogenous signals: MAPK cascades in plant growth and defense. Curr. Opin. Plant Biol. 45, 1–10. doi: 10.1016/j.pbi.2018.04.012
Zhang, X., Peng, H., Zhu, S., Xing, J., Li, X., Zhu, Z., et al. (2020a). Nematode-encoded RALF Peptide mimics facilitate Parasitism of plants through the FERONIA receptor kinase. Mol. Plant 13, 1434–1454. doi: 10.1016/j.molp.2020.08.014
Zhang, X., Yang, Z., Wu, D., and Yu, F. (2020b). RALF–FERONIA signaling: Linking plant immune response with cell growth. Plant Commun. 1:100084. doi: 10.1016/j.xplc.2020.100084
Zhao, C., Zayed, O., Yu, Z., Jiang, W., Zhu, P., Hsu, C.-C., et al. (2018). Leucine-rich repeat extensin proteins regulate plant salt tolerance in Arabidopsis. Proc. Natl. Acad. Sci. U.S.A. 115, 13123–13128. doi: 10.1073/pnas.1816991115
Zheng, F., Chen, L., Zhang, P., Zhou, J., Lu, X., and Tian, W. (2020). Carbohydrate polymers exhibit great potential as effective elicitors in organic agriculture: A review. Carbohydr. Polym. 230:115637. doi: 10.1016/j.carbpol.2019.115637
Zhou, J., Wang, P., Claus, L. A. N., Savatin, D. V., Xu, G., Wu, S., et al. (2019). Proteolytic processing of SERK3/BAK1 regulates plant immunity, development, and cell death. Plant Physiol. 180, 543–558. doi: 10.1104/pp.18.01503
Zhu, S., Fu, Q., Xu, F., Zheng, H., and Yu, F. (2021). New paradigms in cell adaptation: Decades of discoveries on the CrRLK1L receptor kinase signalling network. New Phytol. 232, 1168–1183. doi: 10.1111/nph.17683
Keywords: expansin, cell wall oligosaccharides, microbial pathogen, defense response, resistance/susceptibility
Citation: Narváez-Barragán DA, Tovar-Herrera OE, Guevara-García A, Serrano M and Martinez-Anaya C (2022) Mechanisms of plant cell wall surveillance in response to pathogens, cell wall-derived ligands and the effect of expansins to infection resistance or susceptibility. Front. Plant Sci. 13:969343. doi: 10.3389/fpls.2022.969343
Received: 14 June 2022; Accepted: 11 July 2022;
Published: 23 August 2022.
Edited by:
Giulia Malacarne, Fondazione Edmund Mach, ItalyReviewed by:
Aurélien Boisson-Dernier, Institut Sophia Agrobiotech, FranceCopyright © 2022 Narváez-Barragán, Tovar-Herrera, Guevara-García, Serrano and Martinez-Anaya. This is an open-access article distributed under the terms of the Creative Commons Attribution License (CC BY). The use, distribution or reproduction in other forums is permitted, provided the original author(s) and the copyright owner(s) are credited and that the original publication in this journal is cited, in accordance with accepted academic practice. No use, distribution or reproduction is permitted which does not comply with these terms.
*Correspondence: Claudia Martinez-Anaya, Y2xhdWRpYS5tYXJ0aW5lekBpYnQudW5hbS5teA==
Disclaimer: All claims expressed in this article are solely those of the authors and do not necessarily represent those of their affiliated organizations, or those of the publisher, the editors and the reviewers. Any product that may be evaluated in this article or claim that may be made by its manufacturer is not guaranteed or endorsed by the publisher.
Research integrity at Frontiers
Learn more about the work of our research integrity team to safeguard the quality of each article we publish.