- 1Tianjin Key Laboratory of Water Resources and Environment, Tianjin Normal University, Tianjin, China
- 2College of Life Sciences, Nankai University, Tianjin, China
Symbiotic relationships with microbes may influence how plants respond to environmental change. In the present study, we tested the hypothesis that symbiosis with the endophytes promoted salt tolerance of the native grass. In the field pot experiment we compared the performance of endophyte-infected (E+) and endophyte-uninfected (E−) Leymus chinensis, a dominant species native to the Inner Mongolia steppe, under altered neutral and alkaline salt stresses. The results showed that under both neutral and alkaline salt stresses, endophyte infection significantly increased plant height, leaf length and fibrous root biomass. Under neutral salt stress, endophyte infection decreased Na+ content and Na+/K+ ratio (p=0.066) in the leaf sheath while increased Ca2+ and Mg2+ content in the rhizome. Under alkali salt stress, endophyte infection tended to increase K+ content in the fibrous root, enhance Mg2+ content in the fibrous root while reduce Na+/K+ ratio in the leaf blade in the 100 mmol/L alkali salt treatment. Although endophyte-infected L. chinensis cannot accumulate Na+ high enough to be halophytes, the observed growth promotion and stress tolerance give endophyte/plant associations the potential to be a model for endophyte-assisted phytoremediation of saline-alkaline soils.
Introduction
Soil salinization is a widespread issue throughout the world. It is estimated that more than 6 percent of the world’s land and 30 percent of the world’s irrigated areas suffer from salinity problems (Chaves et al., 2009). Soil salinization generally includes two kinds of stresses: neutral salt stress resulted from NaCl and Na2SO4, and alkali salt stress resulted from NaHCO3 and Na2CO3. Plants grown in neutral salt-affected soils are mainly subjected to water deficit, ion toxicity, and disorders of mineral nutrients (Reza Sabzalian and Mirlohi, 2010). Excess amount of salt ions in soils can damage plant roots as well as the aboveground plant parts, and appear to be a major constraint to plant and crop productivity (Parihar et al., 2015; Liu et al., 2022). Alkali salt stress causes not only the detrimental salt stress, but also induces high pH stress. The high pH soil surrounding the roots directly reduces the root activity, interferes with ion uptake, and breaks intracellular ion balances in plants (Guo et al., 2009; Kaiwen et al., 2020).
Saline soil rehabilitation can be performed by non-plant based, environment-friendly modifications (e.g., structural engineering alterations, leaching of salts) and plant-based remediation (e.g., phytoremediation) (Gutierrez-Gines et al., 2016). Besides halophytes, the use of plant varieties with elevated tolerance to salinity is another alternative in phytoremediation (Yin et al., 2014). Several studies have demonstrated that the local adaptation of plants to their environment is usually driven by closely associated microbes (Rodriguez and Redman, 2008; Porcel et al., 2011) and it has been documented that mutualistic symbiosis with rhizobia (Franzini et al., 2019) and arbuscular mycorrhizal fungi (Rodriguez et al., 2004; Ma et al., 2021) can reduce the negative effects of salinization and improve the salt tolerance of plants grown in saline soils. Mutualistic microbiota symbiosis may improve water absorption and osmotic regulation of host plants by alleviating the adverse effects of excess salt ion accumulations in host plants (Franzini et al., 2019).
Epichloë endophyte is a class of symbiotic fungi and mainly exists in the aboveground part of the plants. Plants serve as hosts and provide nutrients to their endophytes, and endophytic fungus protect them from biotic and abiotic stresses (Xia et al., 2015; Bastias et al., 2017; Shi et al., 2020), especially drought (Ren et al., 2011; Wang et al., 2015; Decunta et al., 2021; Manzur et al., 2022). Endophytic fungus confer drought tolerance to the host grass by increasing root growth, enhancing photosynthesis and osmotic adjustment (Richardson et al., 1992; Malinowski et al., 1998; Decunta et al., 2021). When growing in saline soils, plants are also exposed to drought stress. But limited studies about the effect of endophyte infection on salt tolerance of host grasses have focused on the neutral salt stress in a hydroponic system. In our previous study, we found that endophyte infection could significantly improve the tolerance of tall fescue to NaCl solution, a common lawn grass, by enhancing plant biomass and Na+ uptake ability of the host grass (Yin et al., 2014). Recently, Chen et al. (2021) found that endophyte infection ameliorated adverse effects of NaCl solution on Hordeum brevisubulatum, an important forage crop, by increasing the conducting tissues and endodermis thickness, which may help inhibiting water loss and the decrease of transport capacity. Endophyte-infected species occur in almost all habitats where grasses are common, including a large number of wild grasses, pasture grasses, lawn grasses, cultivated grains and their wild relatives, as well as weed grasses (Bacon, 1993; White, 1993; Clay and Schardl, 2002). The expression of salt tolerance in a saline hydroponic system could be different from expression in a saline soil-based system (Tavakkoli et al., 2012). Moreover, salinization and alkalization frequently co-occurred in soils, and the alkali stress expressed more serious growth inhibition than the neutral salt stress. However, little is known about whether endophyte infection can also improve the alkali tolerance of host plants.
Leymus chinensis, an important perennial rhizome grass, is widely distributed at eastern Eurasian steppe zone, from North Korea westward to Mongolia and northern China, and northwestward to Siberia. Due to excellent stress tolerance, rapid growth, high palatability and herbage production, L. chinensis is an economically and ecologically important forage grass (Lin et al., 2017; Liu et al., 2017). In the present study, endophyte-infected (E+) and uninfected (E−) L. chinensis were planted under neutral salt ranging from 0 to 400 mmol/L treatments or alkali salt ranging from 0 to 300 mmol/L treatments. The changes in the plant growth and photosynthesis, biomass allocation, and the accumulation of Na+, K+, Ca2+, Mg2+ and Na+/K+ in various plant parts were tested. Specifically, the following questions were addressed: (1) does neutral salt tolerance also exist in endophyte-infected wild grasses in soil-based system? And (2) does the endophyte improve alkali tolerance in the host grasses?
Materials and methods
Plant materials and growth conditions
In the middle and northeast parts of the Inner Mongolia steppe, L. chinensis is one of the most important dominant species. In the previous survey made by our lab (Wei et al., 2006; Zhu et al., 2013), the endophyte infection rate of L. chinensis in the Abaga Banner population was about 63.3%. Endophyte has been identified as Epichloë bromicola (Zhu et al., 2013). Thirty E+ and 30 E− plants were originally transplanted from different individuals in the same population from Abaga Banner of Inner Mongolia (43.90° N, 115.34° E). To avoid misleading 30 E+ and 30 E− plants were selected and maintained in the experimental field at Nankai University. After two years’ growth we randomly sampled tillers of approximately equal size for this experiment. During their growth, we clipped the plants repeatedly and kept them in vegetative growth. For this experiment, we transplanted 10 tillers of approximately equal size into a white plastic pot (23-cm diameter and 25-cm depth) filled with 5 kg of fluvial sand. Before conducting experiments, fluvial sand was washed with distilled water until the conductivity of the eluate was close to that of distilled water, and endophyte infection status were confirmed microscopically by examining leaf sheaths of each plant for the presence of fungal hyphae after staining with lactophenol aniline blue (Latch et al., 1984). The pots were all located in the experimental field at Nankai University, with transparent flashing above them. Plants were subjected to ambient light and temperature regimes. The plant growth conditions were maintained at a mean photoperiod of 15/9 h (light/dark) and a mean air temperature of 35/24°C (day/night) with an approximate 65% relative humidity. The positions of the pots were randomly rotated each week to minimize location effects. Salt Treatment
Here two neutral salts and two alkaline salts were considered simultaneously base on the salt components and characteristics of alkaline soil in northern China. A mixture of NaCl and Na2SO4 (molar ratio, 1: 1) was withheld to induce neutral salt condition, and another mixture of NaCl, Na2SO4, NaHCO3, and Na2CO3 (molar ratio, 1: 9: 9: 1) was withheld to induce alkaline salt treatment. Neutral salt treatment included four levels: 0, 200, 300, and 400 mmol/L. Alkali salt treatment included four levels: 0, 100, 200, and 300 mmol/L. Four salts concentrations were selected according to the tolerability of L. chinensis cultivar to the salt–alkaline stress and the varying ranges of salinity and pH in the soil. Each treatment was replicated five times. Different salt levels were achieved by the addition of distilled water containing the corresponding concentration of mixed salt. The control group was watered with distilled water. The salt solution was added once every two days, and its amount was 3 times the water field capacity of the planting sand so as to wash away the previous accumulated salt. Every morning, a soil moisture probe (ECH2O Check, Decagon Devices, Pullman, WA, USA) was inserted to a depth of 5 cm into each pot to record the volumetric water content percentage, and the lost water was supplemented with distilled water to maintain the soil water content at the field water capacity level. Two hundred milliliters Hoagland complete nutrient solution was supplemented twice a week. The experiment was performed from May 10 to August 23, and lasted 105 d.
Growth parameters and metal ions measurements
The tiller number, leaf number, plant height, leaf length and leaf width of each pot were counted or measured at the beginning and end of the experiment. At the end of the experiment, grass samples were separated into the aboveground plant parts (leaf blade and leaf sheaths) and the belowground plant parts (fibrous roots and rhizomes), weighed after drying in the oven at 80°C. Afterwards, the oven-dried samples were crushed with a grinder, and then placed in a muffle furnace and ashed at 650°C. The samples were digested with 5% nitric acid. The contents of Na+, K+, Ca2+, and Mg2+ in the solutions were determined by an inductively coupled plasma - optical emission spectrometry (ICP-OES, Perkin Elmer Optimal 8300).
Physiological indicators measurements
Specific leaf area (SLA) was calculated using two fully unfolded new leaves for each pot to measure their area and weigh their fresh and dry weights. Leaf moisture content (LWC) was calculated by fresh weight and dry weight of leaves. Net photosynthesis (Pn) was measured on second or third leaves using a portable photosynthesis system (LI-6400, LI-COR, Lincoln, USA). Leaf nitrogen content (LNC) was determined with an elemental analyzer (vario MACRO cube, Elementar, Germany). Photosynthetic nitrogen use efficiency (PNUE) was calculated as the ratio of CO2 assimilation rate to leaf nitrogen content. Chlorophyll a, chlorophyll b, and carotene in fresh leaves were extracted with solution (absolute ethanol and acetone, 1:1), and were assayed in a spectrophotometer (UV1800, Shimadzu, Japan) at wavelengths of 440, 645, and 663 nm. Photosynthetic pigment content was measured according to the procedure of Lin et al. (1999).
Statistical analyses
Data were analyzed using SPSS 16.0 statistical software (SPSS Inc., Chicago, IL, USA). The differences between endophyte-infected (E+) plants and uninfected (E−) plants, and between neutral salt or alkali salt treatments were tested by one-way ANOVA LSD test at a confidence interval of 95%. Interactions between endophyte status (E) and neutral salt stress (NSS) or alkali stress (ASS) on growth parameters and physiological indexes were tested by two-way analysis of variance (ANOVA) at a confidence interval of 95%.
Results
Plant growth and biomass allocation
At the end of the experiment, all plants except those treated with 300 mmol/L alkali salt survived, therefore we did not include 300 mmol/L treatment in the result. Both neutral salt and alkali salt stresses significantly inhibited tiller number, leaf number, plant height, SLA and LWC of L. chinensis, only alkali salt stress was more serious than neutral salt stress (Tables 1, 2). Under neutral salt treatments, endophyte infection significantly increased plant height and leaf length of the host (Figures 1A, B). Under alkali salt treatments, endophyte infection significantly increased plant height, leaf length and leaf width of the host (Figures 1C–E). The effect of endophyte status on the vegetative growth of L. chinensis in the same concentration (200 mM) of NSS and ASS treatments were shown in Table 2. The presence of endophyte significantly increased the plant height of L. chinensis, but neutral salt stress decreased the plant heights, and alkali salt stress decreased further. Salt stress decreased leaf length and width, and changed the size or area of leaf.
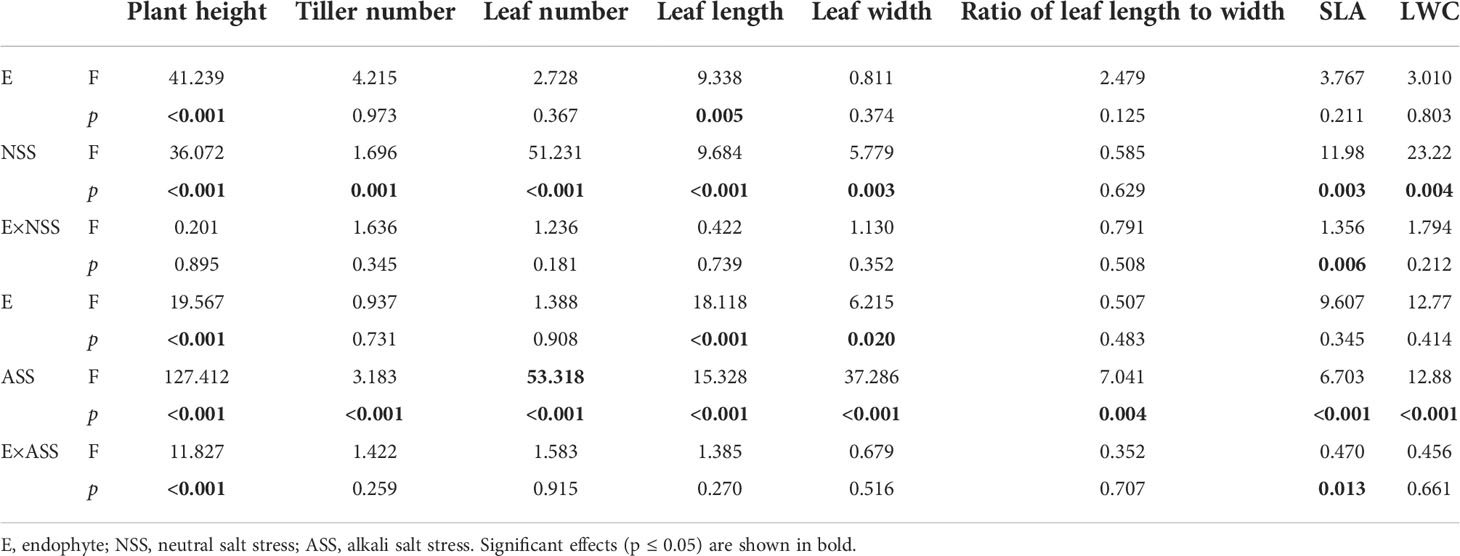
Table 1 ANOVA results for the effect of endophyte status, salt stress on vegetative growth of L. chinensis.
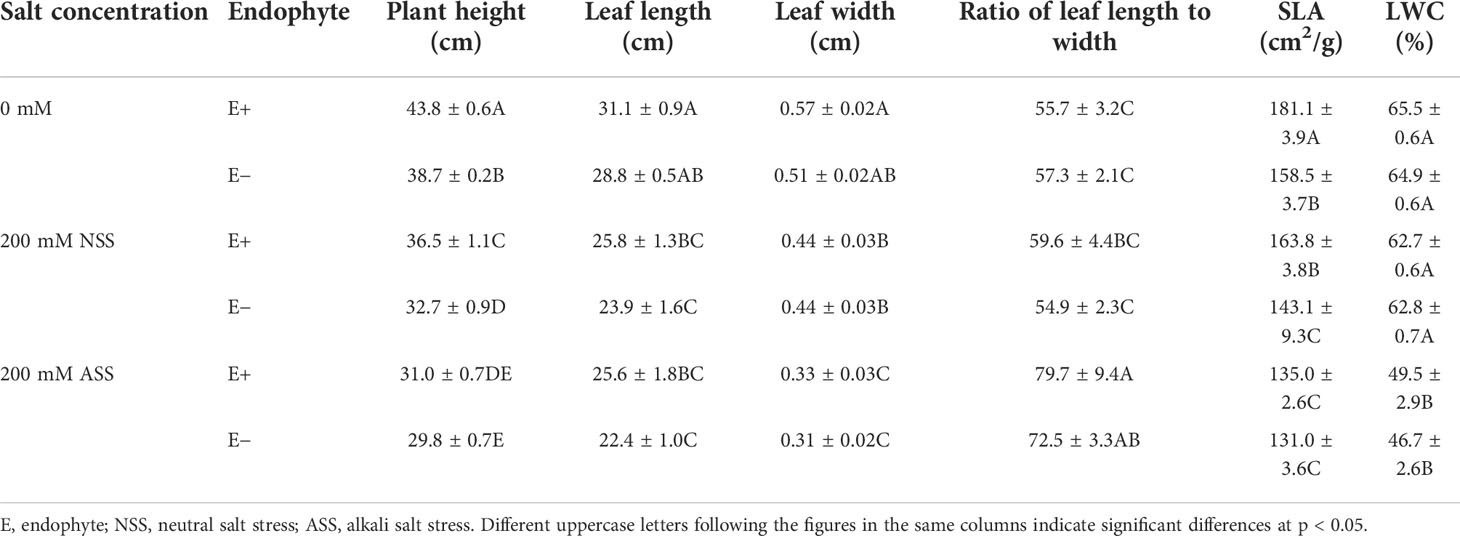
Table 2 The effect of endophyte status on the vegetative growth of L. chinensis in the same concentration (200 mM) of NSS and ASS treatments.
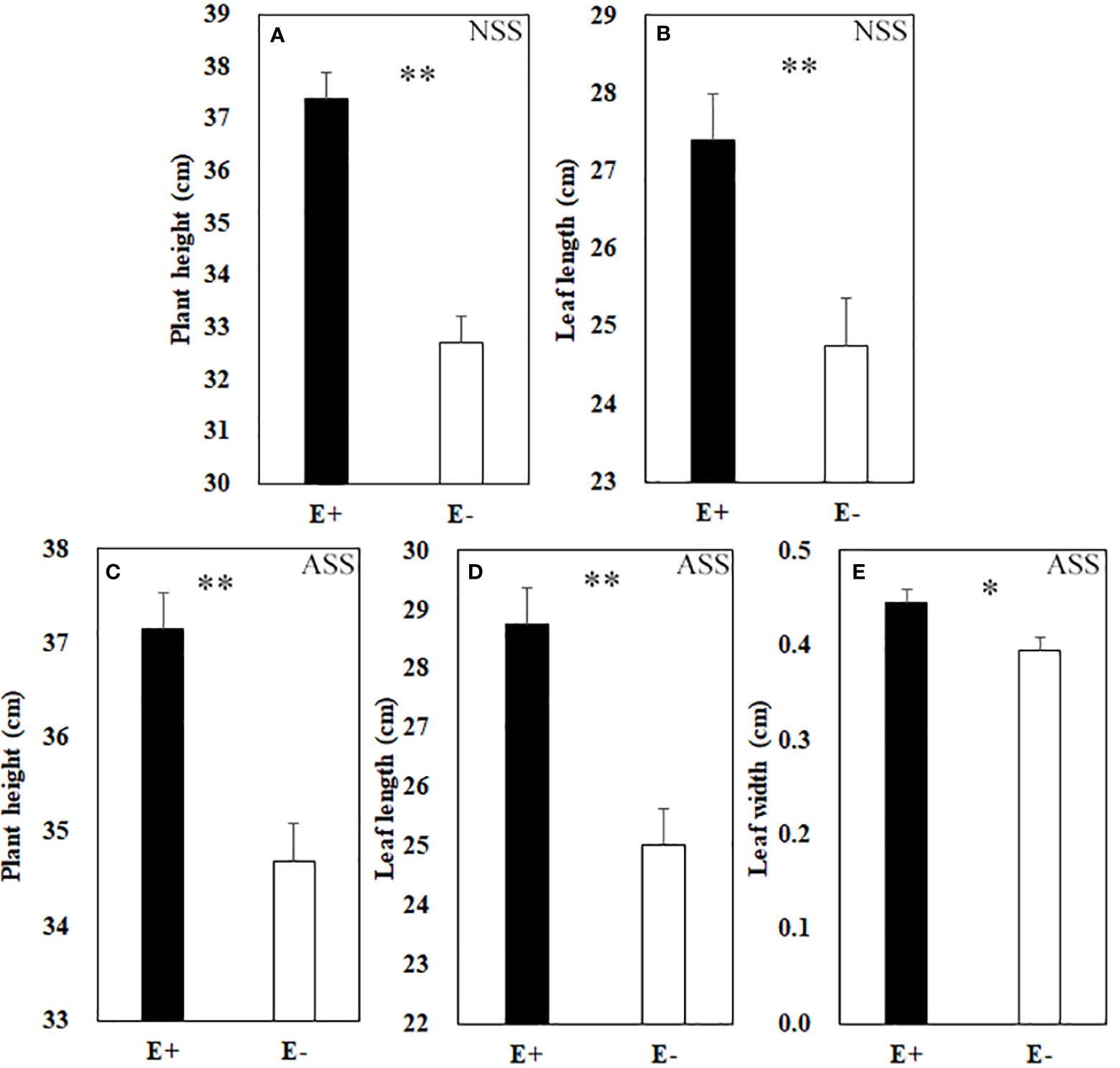
Figure 1 Plant height (A) and leaf length (B) under neutral salt stress (NSS) as well as plant height (C), leaf length (D) and leaf width (E) under alkaline salt stress (ASS) of endophyte infected (E+) and uninfected (E−) L. chinensis. Values are mean ± standard error. * and ** indicate significant difference at p < 0.05 and p < 0.01, respectively.
Both neutral and alkali salt stresses significantly inhibited total biomass, above- and belowground biomasses of L. chinensis, only alkali salt stress was more serious than neutral salt stress (Tables 2 and 3). Under neutral salt treatment, both belowground biomass and fibrous root biomass were significantly higher in E+ plants than those in E− plants. For alkali salt treatment, the fibrous root biomass was also higher in E+ plants than that in E− plants under 200 mmol/L treatment (Figures 2A–C). Pn rates in µmol/(m2.s) were not significantly influenced by endophyte status (p=0.073) (Table 3). However, when SLA was considered together Pn rates in µmol/(g.s) in E+ plants were significantly enhanced than those in E− plants (p=0.025) (Figure 2D).
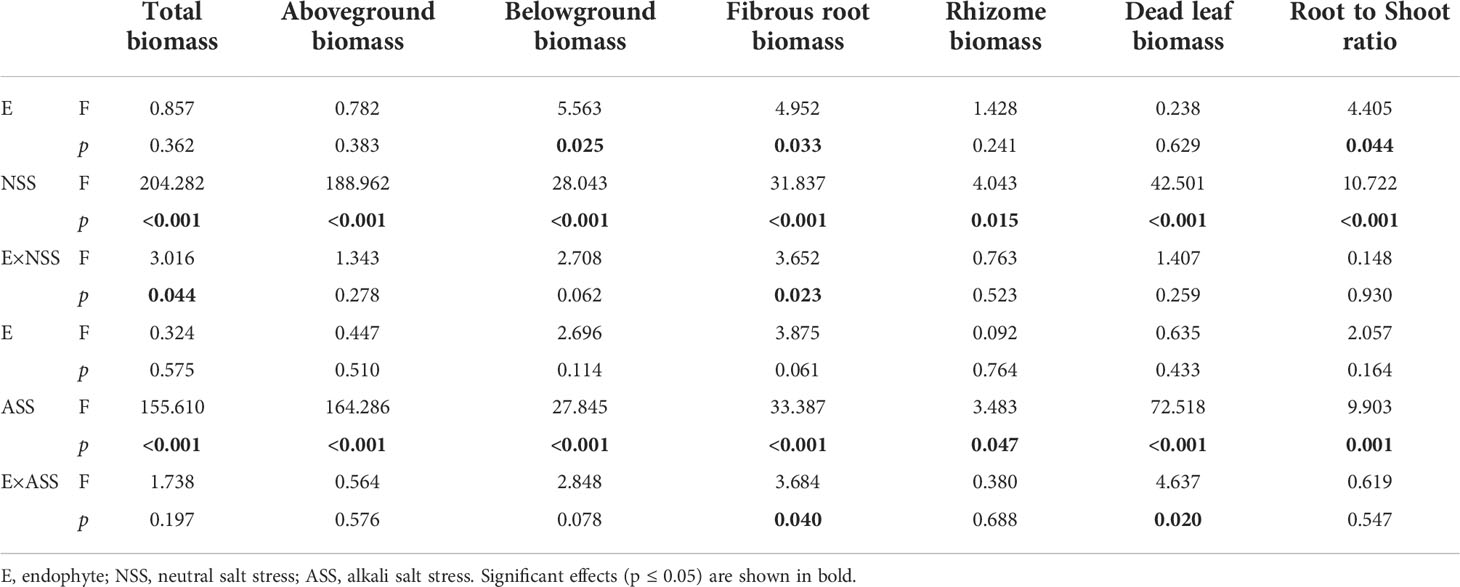
Table 3 ANOVA results for the effect of endophyte status and salt stress on biomass allocation of L. chinensis.
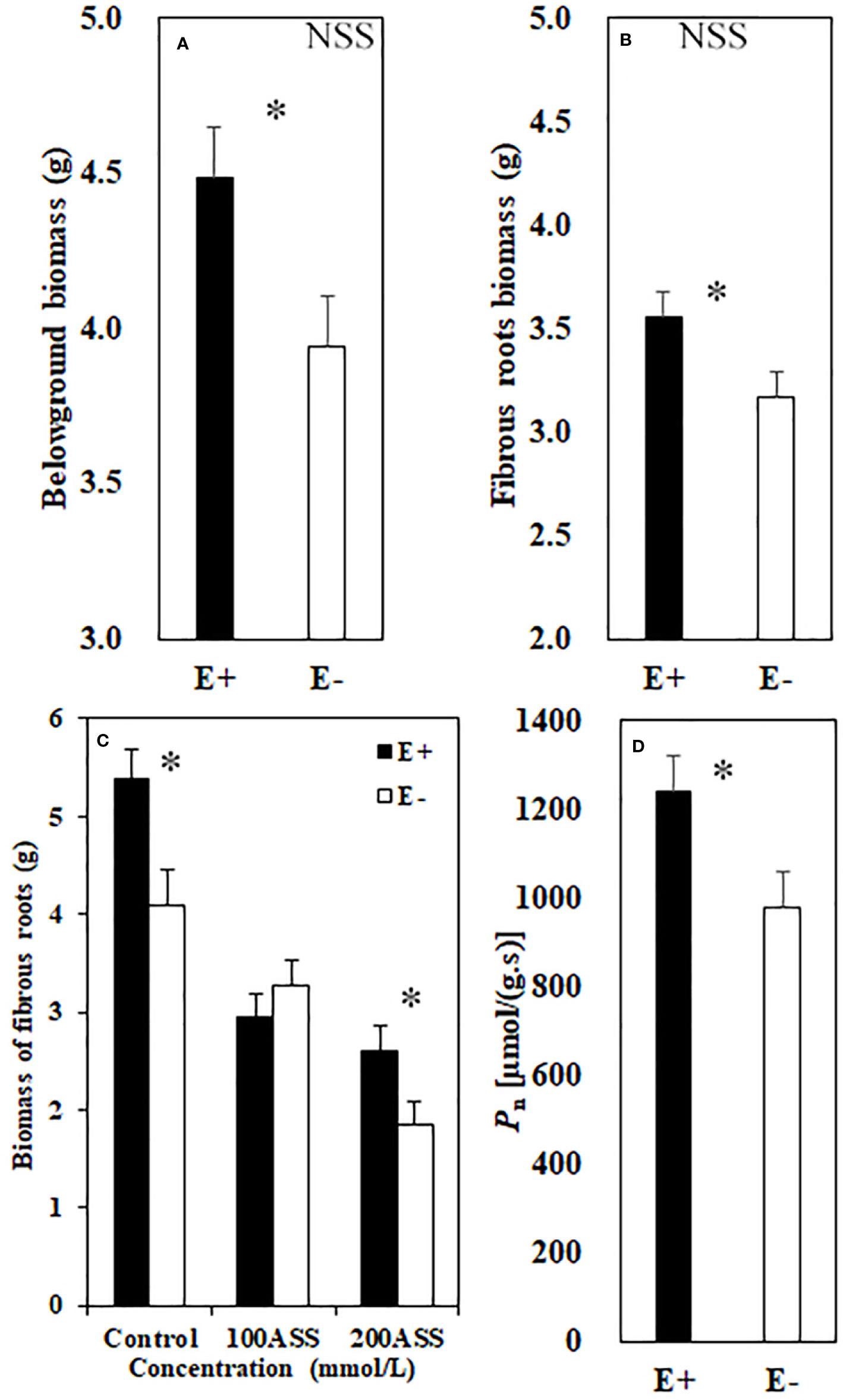
Figure 2 Belowground biomass (A) and fibrous root biomass (B) under neutral salt stress (NSS) and fibrous root biomass (C) under alkali salt stress (ASS) of endophyte infected (E+) and uninfected (E−) L. chinensis. Pn rate (D). Values are mean ± standard error. * indicates significant difference at p < 0.05.
Physiological indicators
Significant effects of neutral salt or alkali salt stresses on chlorophyll a, carotenoid and the net photosynthetic rate of leaves were observed in the present study, while these indexes were not influenced by endophyte status (Table 4). Endophyte infection significantly enhanced LNC under neutral salt treatment, yet did not affect LNC under alkali salt treatment (Figures 3A, B). Both neutral salt and alkaline salt stresses had significant effects on PNUE, while endophyte infection had no significant effect on PNUE.
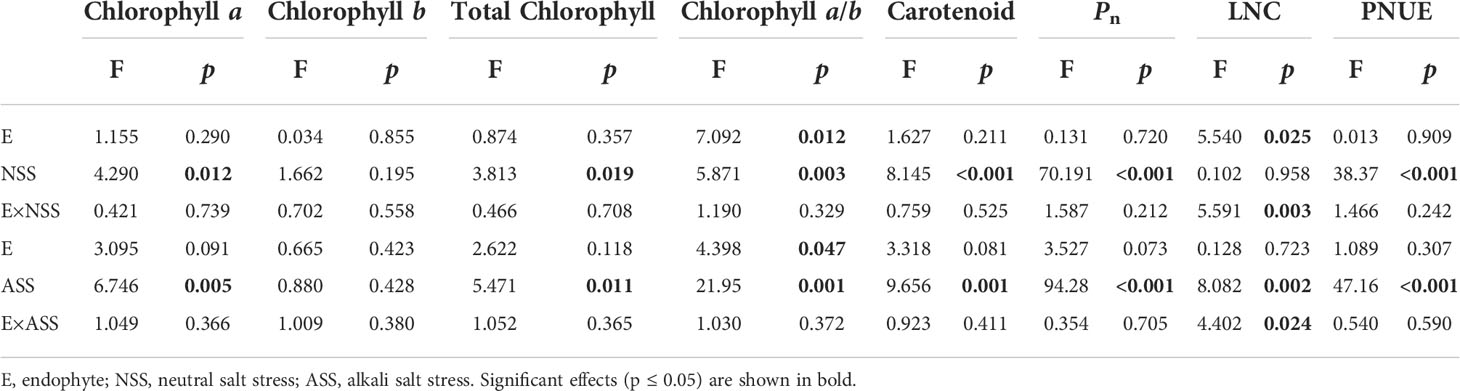
Table 4 ANOVA results for the effect of endophyte status and salt stress on photosynthetic pigment content and photosynthetic indicators.
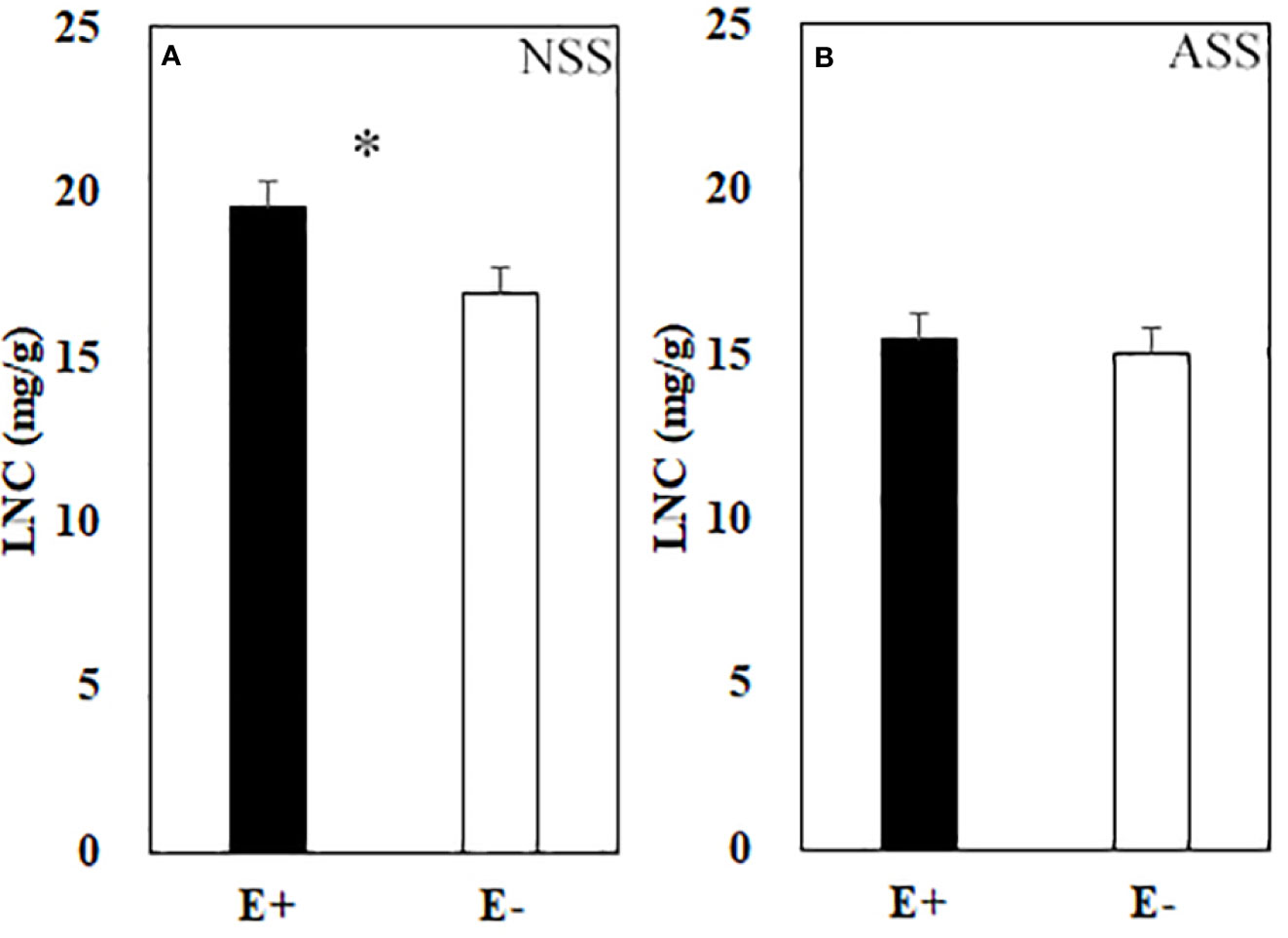
Figure 3 Leaf nitrogen content (LNC) of endophyte infected (E+) and uninfected (E−) L. chinensis under neutral salt stress (A) and alkali salt stress (B). Values are mean ± standard error. * means significant difference at p < 0.05.
Changes in cation contents and K+/Na+ ratio
Both neutral salt stress and alkali salt stresses had significant effects on Na+, Ca2+, Mg2+, K+ contents and Na+/K+ ratio in L. chinensis (Table 5 and 6). Under neutral salt stress, endophyte infection significantly reduced Na+ content in leaf sheaths, while enhanced Ca2+ and Mg2+contents in rhizomes (Figures 4A–C). Endophyte infection tended to decrease Na+/K+ ratio in the leaf sheath (p=0.066) (Figure 4D). Under alkali salt stress, endophyte infection tended to enhance K+ in the fibrous root. Endophyte infection increased Mg2+ content in the fibrous root while decreased Na+/K+ ratio in the leaf blade in the 100 mmol/L alkali salt treatment (Figures 4E–G).
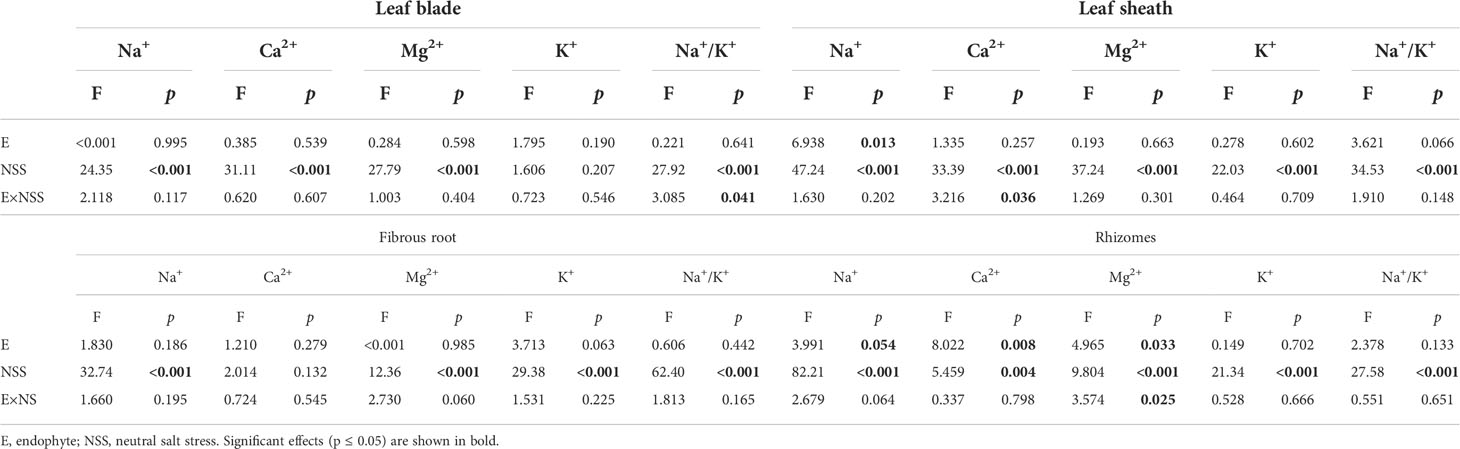
Table 5 ANOVA results for the effect of endophyte status and neutral salt stress on ion contents and K+/Na+ ratio in shoots and roots.
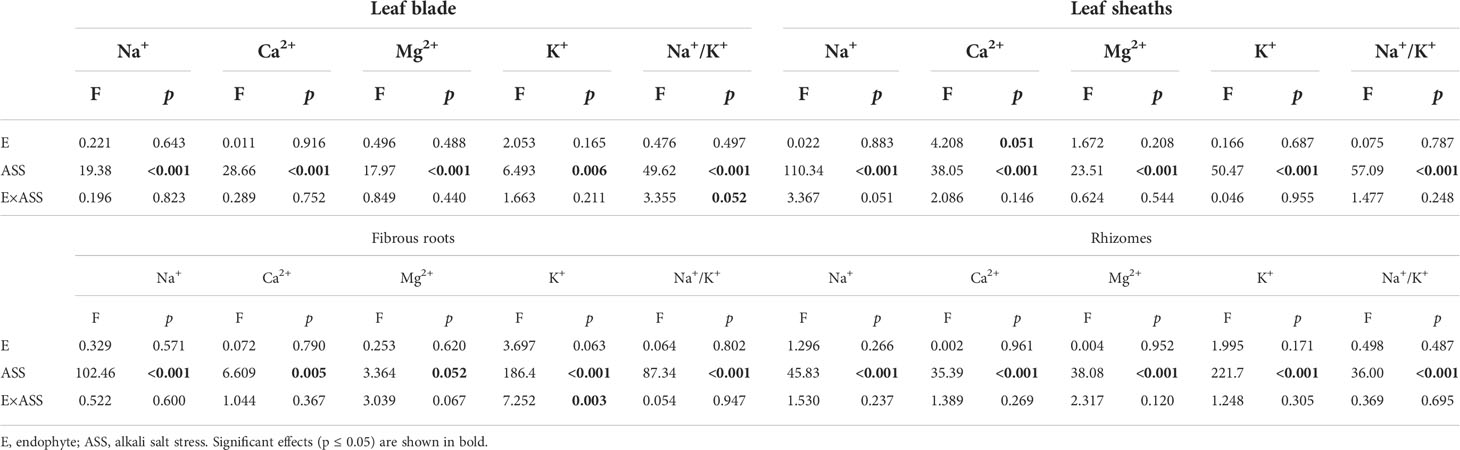
Table 6 ANOVA results for the effect of endophyte status and alkali salt stress on ion contents and K+/Na+ ratio in shoots and roots.
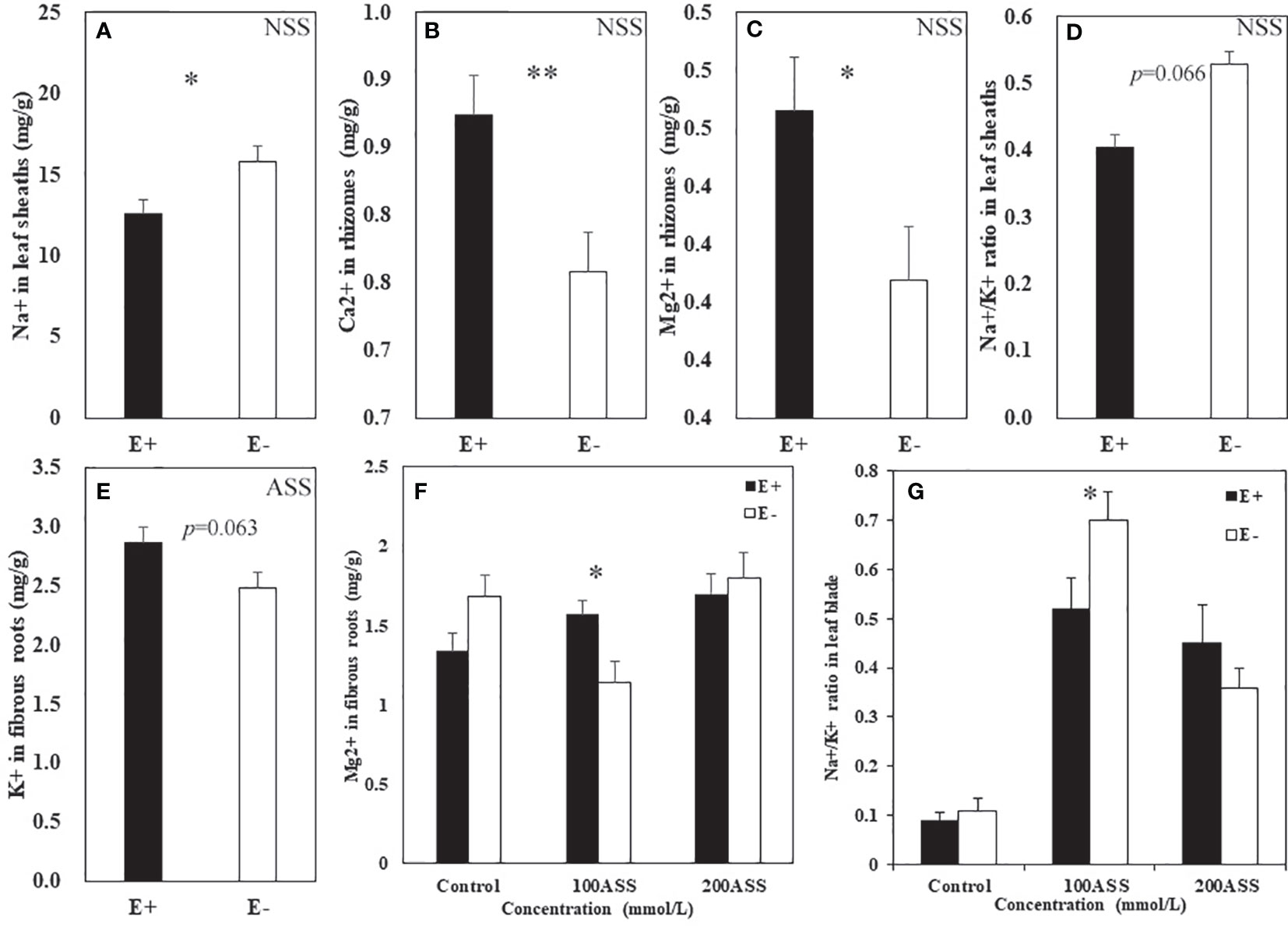
Figure 4 Na+, Ca2+, Mg2+, K+ contents and Na+/K+ ratio in shoots and roots of endophyte infected (E+) and uninfected (E−) L. chinensis under neutral salt (A–D) and alkali salt (E–G) stresses. (A) Sheath Na+, (B) Rhizomes Ca2+, (C) Rhizomes Mg2+, (D) Sheath Na+/K+ ratio; (E) Fibrous root K+, (F) Fibrous root Mg2+, (G) Blade Na+/K+ ratio. Values are mean ± standard error. * and ** indicate significant difference at p < 0.05 and p < 0.01, respectively.
Discussion
Both alkali salt and neutral salt stresses involved in deleterious effects of salinity on plant growth; the adverse effects of high pH alkaline solutions on plant growth were more severe than those of low pH salt treatments. High pH is the key characteristic of alkali salt stress that is different from salt stress. The responses of L. chinensis were significantly correlated not only with salinity but also with the pH of the alkalinity treatment. It has been reported that alkali salt produced a stronger stress than neutral salt in both halophytes and glycophytes (Yang et al., 2009; Li et al., 2010; Gong et al., 2014). In the present study, L. chinensis survived under 400 mmol/L neutral salt treatment, yet died under 300 mmol/L alkali salt treatment, which was consistent with previous reports.
It has been well documented that Epichloë endophyes could ameliorate drought stress for both agronomically important forage species (Clay and Schardl, 2002; Saikkonen et al., 2006) and wild grass species (Ren and Clay, 2009; Liu et al., 2017). In the hydroponic experiment, the beneficial effects of Epichloë endophytes on NaCl tolerance has been demonstrated in several studies (Reza Sabzalian and Mirlohi, 2010; Yin et al., 2014; Chen et al., 2021). However, expressions of salinity tolerance in hydroponic systems might different with the performance in the soil. Tavakkoli et al. (2010); Tavakkoli et al. (2012) compared salt tolerance of barley under three different growing conditions (hydroponics, potted soil and a naturally saline field) and found that salt tolerance differences between genotypes were expressed when grown in the soil, but cannot be discerned when grown in the hydroponic system. As for plants grown in the potted soil or in the naturally saline field, they expressed similar degrees of salt tolerance. In the present study, we found that endophyte infection significantly increased plant height, leaf length and fibrous root biomass under both neutral and alkali salt stresses, indicating that Epichloë endophytes can improve the host resistance to both neutral salt as well as alkali salt grown in the potted soil, only their beneficial effect was more obvious under neutral salt stress than under alkali salt stress.
The mechanisms involved in endophyte-associated salt tolerance of the host have not been documented. According to reported studies and our research, the following possible reasons were proposed. Firstly, endophyte infection could improve root absorption that may alleviate drought and nutrients deficiency during salt stresses. Arbuscular mycorrhizal fungi has been reported to enhance plant growth under salt stress mainly through an extensive hyphal network which allowed enhancement of water and nutrient acquisition (Plenchette and Duponnois, 2005). Epichloë endophytes can also increase root absorption (Malinowski et al., 1999; Crush et al., 2004) in response to water stress. In the present study, L. chinensis produces two structurally and functionally different organs belowground: fibrous roots that play a central role for root absorption, and rhizomes that serve mainly as translocation conduits and overwinter storage. We found that endophyte infection significantly enhanced fibrous root biomass under both neutral and alkali salt stresses. Although we did not find that endophyte infection improved leaf water content of the host, we did find that endophyte infection increased LNC under neutral salt stress, which was usually negatively influenced by salt stress (Frechilla et al., 2001).
Another mechanism used by Epichloë endophytes to promote salt tolerance of the host may be the regulation of plant nutrition. The Na+ ion is the main toxic ion in salinized soil. Unlike Na+, K+ plays a key role in many physiological processes vital to plant growth. Under salt stress, a good balance of Na+/K+ ratio is important for maintaining ion balance and a number of enzymatic processes, and thus is a potential indicator of salt tolerance in the plants (Munns and Tester, 2008; Abdelhamid et al., 2010; Tomar and Agarwal, 2013). Arbuscular mycorrhizal fungi has been found to play a significant role in sustaining a high K+/Na+ ratio in plants that were exposed to salt stress (Selvakumar and Thamizhiniyan, 2011; Zhang et al., 2011; Kadian et al., 2013; Fariduddin et al., 2014). In the present study, we found that endophyte infection tended to decrease Na+/K+ ratio in leaf sheath under neutral salt stress, and also reduced Na+/K+ ratio in leaf blade in the 100 mmol/L alkali salt treatment.
The accumulations of Ca2+ and Mg2+ in plants are usually inhibited by salt stress (Khan et al., 1999; Aziz and Khan, 2001). Arbuscular mycorrhizal fungi has been documented to increase the uptake and concentration of Ca2+ in different plants (Elhindi et al., 2017; Cui et al., 2019). Rahman and Saiga (2005) reported that endophyte-infected tall fescue had a greater ability to take up Ca2+ and Mg2+, compared to uninfected plants under normal growth conditions. Bayat et al. (2009) found that endophytes increased Ca2+ content in tall fescue under drought stress. In the present study, endophyte infection increased Ca2+ and Mg2+ contents in the rhizome under neutral salt stress while increased Mg2+ content in the fibrous root in 100 mmol/L alkali salt treatment. It is well known that Mg2+ is the key component of chlorophyll. The Ca2+ ion can maintain membrane stability, help to form cell walls, and take part in signal transduction. Here, increased Mg2+ in response to endophyte infection may be related to alleviate chlorophyll breakdown, while increased Ca2+ may be related to membrane stability and signal transduction (Cui et al., 2019; Thor, 2019).
Conclusion
There are over 932 million hectares of land suffering salinization and alkalization around the world (Rengasamy, 2006). It is estimated that soil salinization caused a total loss of US$27.3 billion, with a direct impact on the global economy (Meena et al., 2017). This study demonstrated that endophyte infection could enhance host tolerance to both neutral salt and alkali salt stresses. The significant effect of endophyte infection was decreasing Na+/K+ ratio, increasing Ca2+and Mg2+ contents, and thus promoted leaf and fibrous root growth. It is estimated that at least 30% of approximately 3000 pooid grass species harbor systemic endophytes, and most can develop this mutualistic association (Leuchtmann, 1992). In this respect, the potential application of endophytes to mitigate negative salt stress impact would be a more favorable choice. Certainly, this experiment was conducted using soil in pots. Further comparisons of E+ and E− plants under natural field stress conditions will help to verify the potential use of grass-endophyte symbiota in phytoremediation of saline-alkaline soils.
Data availability statement
The raw data supporting the conclusions of this article will be made available by the authors, without undue reservation.
Author contributions
LY and MW did experimental work. GW analysed the data and drafted the manuscript. AR designed the experiments and wrote the manuscript. All authors contributed to the article and approved the submitted version.
Funding
This work was supported by the National Natural Science Foundation of China (31971425).
Acknowledgments
We greatly appreciate the support of Abaga banner grassland workstation for their invaluable assistance on this experiment.
Conflict of interest
The authors declare that the research was conducted in the absence of any commercial or financial relationships that could be construed as a potential conflict of interest.
Publisher’s note
All claims expressed in this article are solely those of the authors and do not necessarily represent those of their affiliated organizations, or those of the publisher, the editors and the reviewers. Any product that may be evaluated in this article, or claim that may be made by its manufacturer, is not guaranteed or endorsed by the publisher.
References
Abdelhamid, M. T., Shokr, M. M. B., Bekheta, M. A. (2010). Growth, root characteristics, and leaf nutrients accumulation of four faba bean (vicia fabal.) cultivars differing in their broomrape tolerance and the soil properties in relation to salinity. Commun. Soil Sci. Plan 41, 2713–2728. doi: 10.1080/00103624.2010.518263
Aziz, I., Khan, M. A. (2001). Experimental assessment of salinity tolerance of ceriops tagal seedlings and saplings from the indus delta, pakistan. Aquat Bot. 70, 259–268. doi: 10.1016/s0304-3770(01)00160-7
Bacon, C. W. (1993). Abiotic stress tolerances (moisture, nutrients) and photosynthesis in endophyte-infected tall fescue. Agric. Ecosyst. Environ. 44, 123–141. doi: 10.1016/0167-8809(93)90042-n
Bastias, D. A., Martínez-Ghersa, M. A., Ballaré, C. L., Gundel, P. E. (2017). Epichloë fungal endophytes and plant defenses: Not just alkaloids. Trends Plant Sci. 22, 939–948. doi: 10.1016/j.tplants.2017.08.005
Bayat, F., Mirlohi, A., Khodambashi, M. (2009). Effects of endophytic fungi on some drought tolerance mechanisms of tall fescue in a hydroponics culture. Rus J. Plant Physl 56, 510–516. doi: 10.1134/s1021443709040104
Chaves, M. M., Flexas, J., Pinheiro, C. (2009). Photosynthesis under drought and salt stress: Regulation mechanisms from whole plant to cell. Ann. Bot. 103, 551–560. doi: 10.1093/aob/mcn125
Chen, T., White, J. F., Li, C. (2021). Fungal endophyte epichloë bromicola infection regulates anatomical changes to account for salt stress tolerance in wild barley (hordeum brevisubulatum). Plant Soil 461, 533–546. doi: 10.1007/s11104-021-04828-w
Clay, K., Schardl, C. (2002). Evolutionary origins and ecological consequences of endophyte symbiosis with grasses. Am. Nat. 160 Suppl 4, S99–S127. doi: 10.1086/342161
Crush, J. R., Popay, A. J., Waller, J. (2004). Effect of different neotyphodium endophytes on root distribution of a perennial ryegrass (lolium perenne l.) cultivar. N. Z J. Agric. Res. 47, 345–349. doi: 10.1080/00288233.2004.9513603
Cui, L., Guo, F., Zhang, J., Yang, S., Meng, J., Geng, Y., et al. (2019). Synergy of arbuscular mycorrhizal symbiosis and exogenous ca(2+) benefits peanut (arachis hypogaea l.) growth through the shared hormone and flavonoid pathway. Sci. Rep. 9, 16281. doi: 10.1038/s41598-019-52630-7
Decunta, F. A., Perez, L. I., Malinowski, D. P., Molina-Montenegro, M. A., Gundel, P. E. (2021). A systematic review on the effects of epichloe fungal endophytes on drought tolerance in cool-season grasses. Front. Plant Sci. 12. doi: 10.3389/fpls.2021.644731
Elhindi, K. M., El-Din, A. S., Elgorban, A. M. (2017). The impact of arbuscular mycorrhizal fungi in mitigating salt-induced adverse effects in sweet basil (ocimum basilicum l.). Saudi J. Biol. Sci. 24, 170–179. doi: 10.1016/j.sjbs.2016.02.010
Fariduddin, Q., Mir, B. A., Yusuf, M., Ahmad, A. (2014). 24-epibrassinolide and/or putrescine trigger physiological and biochemical responses for the salt stress mitigation in cucumis sativus l. Photosynthetica. 52, 464–474. doi: 10.1007/s11099-014-0052-7
Franzini, V. I., Azcon, R., Ruiz-Lozano, J. M., Aroca, R. (2019). Rhizobial symbiosis modifies root hydraulic properties in bean plants under non-stressed and salinity-stressed conditions. Planta. 249, 1207–1215. doi: 10.1007/s00425-018-03076-0
Frechilla, S., Lasa, B., Ibarretxe, L., Lamsfus, C., Aparicio-Tejo, P. (2001). Pea responses to saline stress is affected by the source of nitrogen nutrition (ammonium or nitrate). Plant Growth Regulation 35, 171–179. doi: 10.1023/a:1014487908495
Gong, B., Li, X., Bloszies, S., Wen, D., Sun, S., Wei, M., et al. (2014). Sodic alkaline stress mitigation by interaction of nitric oxide and polyamines involves antioxidants and physiological strategies in solanum lycopersicum. Free Radic. Biol. Med. 71, 36–48. doi: 10.1016/j.freeradbiomed.2014.02.018
Guo, R., Shi, L., Yang, Y. (2009). Germination, growth, osmotic adjustment and ionic balance of wheat in response to saline and alkaline stresses. Soil Sci. Plant Nutr. 55, 667–679. doi: 10.1111/j.1747-0765.2009.00406.x
Gutierrez-Gines, M. J., Hernandez, A. J., Pastor, J. (2016). Impacts of soil-soluble anions on wild and cultivated herbaceous species: Implications for soil phytoremediation. J. Soil Sci. Plant Nutr. 16, 423–437.
Kadian, N., Yadav, K., Badda, N., Aggarwal, A. (2013). Am fungi ameliorates growth, yield and nutrient uptake in cicer arietinum l. under salt stress. Russian Agric. Sci. 39, 321–329.
Kaiwen, G., Zisong, X., Yuze, H., Qi, S., Yue, W., Yanhui, C., et al. (2020). Effects of salt concentration, ph, and their interaction on plant growth, nutrient uptake, and photochemistry of alfalfa (medicago sativa) leaves. Plant Signal. Behav. 15, 1832373. doi: 10.1080/15592324.2020.1832373
Khan, M. A., Ungar, I. A., Showalter, A. M. (1999). Effects of salinity on growth, ion content, and osmotic relations inhalopyrum mucronatum(l.) stapf. J. Plant Nutr. 22, 191–204. doi: 10.1080/01904169909365617
Latch, G. C. M., Christensen, M. J., Samuels, G. J. (1984). Five endophytes of lolium and festuca in new zealand. Mycotaxon. 20, 535–550.
Leuchtmann, A. (1992). Systematics, distribution, and host specificity of grass endophytes. Nat. Toxins 1, 150–162. doi: 10.1002/nt.2620010303
Lin, F. P., Chen, Z. H., Chen, Z. P., Zhang, D. M. (1999). Physiological and biochemical responses of the seedlings of four legume tree species to high co2 concentration. Chin. J. Plant Ecol. 23, 220–222.
Lin, J., Wang, Y., Sun, S., Mu, C., Yan, X. (2017). Effects of arbuscular mycorrhizal fungi on the growth, photosynthesis and photosynthetic pigments of leymus chinensis seedlings under salt-alkali stress and nitrogen deposition. Sci. Total Environ. 576, 234–241. doi: 10.1016/j.scitotenv.2016.10.091
Li, R., Shi, F., Fukuda, K. (2010). Interactive effects of salt and alkali stresses on seed germination, germination recovery, and seedling growth of a halophyte spartina alterniflora (poaceae). S Afr J. Bot. 76, 380–387. doi: 10.1016/j.sajb.2010.01.004
Liu, Z., Liu, P., Qi, D., Peng, X., Liu, G. (2017). Enhancement of cold and salt tolerance of arabidopsis by transgenic expression of the s-adenosylmethionine decarboxylase gene from leymus chinensis. J. Plant Physiol. 211, 90–99. doi: 10.1016/j.jplph.2016.12.014
Liu, C., Mao, B., Yuan, D., Chu, C., Duan, M. (2022). Salt tolerance in rice: Physiological responses and molecular mechanisms. Crop J. 10, 13–25. doi: 10.1016/j.cj.2021.02.010
Malinowski, D. P., Alloush, G. A., Belesky, D. P. (1998). Evidence for chemical changes on the root surface of tall fescue in response to infection with the fungal endophyte neotyphodium coenophialum. Plant Soil 205, 1–12. doi: 10.1023/a:1004331932018
Malinowski, D. P., Belesky, D. P., Fedders, J. M. (1999). Endophyte infection may affect the competitive ability of tall fescue grown with red clover. J. Agron. Crop Science 183, 91–101. doi: 10.1046/j.1439-037x.1999.00322.x
Manzur, M. E., Garello, F. A., Omacini, M., Schnyder, H., Sutka, M. R., Garcia-Parisi, P. A. (2022). Endophytic fungi and drought tolerance: Ecophysiological adjustment in shoot and root of an annual mesophytic host grass. Funct. Plant Biol. 49, 272–282. doi: 10.1071/FP21238
Ma, P., Shi, Z., Diao, F., Hao, L., Zhang, J., Xu, J., et al. (2021). Effects of arbuscular mycorrhizal fungi on growth and na+ accumulation of suaeda glauca (bunge) grown in salinized wetland soils. Appl. Soil Ecol. 166, 104065. doi: 10.1016/j.apsoil.2021.104065
Meena, B. L., Rattan, R. K., Datta, S. P. (2017). Solubility relationships of iron and evaluation of its fertility status in degraded soils. Commun. Soil Sci. Plan 48, 1059–1067. doi: 10.1080/00103624.2017.1323098
Munns, R., Tester, M. (2008). Mechanisms of salinity tolerance. Annu. Rev. Plant Biol. 59, 651–681. doi: 10.1146/annurev.arplant.59.032607.092911
Parihar, P., Singh, S., Singh, R., Singh, V. P., Prasad, S. M. (2015). Effect of salinity stress on plants and its tolerance strategies: A review. Environ. Sci. pollut. Res. Int. 22, 4056–4075. doi: 10.1007/s11356-014-3739-1
Plenchette, C., Duponnois, R. (2005). Growth response of the saltbush atriplex nummularia l. to inoculation with the arbuscular mycorrhizal fungus glomus intraradices. J. Arid Environ. 61, 535–540. doi: 10.1016/j.jaridenv.2004.10.003
Porcel, R., Aroca, R., Ruiz-Lozano, J. M. (2011). Salinity stress alleviation using arbuscular mycorrhizal fungi. a review. Agron. Sustain. Dev. 32, 181–200. doi: 10.1007/s13593-011-0029-x
Rahman, M. H., Saiga, S. (2005). Endophytic fungi (neotyphodium coenophialum) affect the growth and mineral uptake, transport and efficiency ratios in tall fescue (festuca arundinacea). Plant Soil 272, 163–171. doi: 10.1007/s11104-004-4682-6
Ren, A., Clay, K. (2009). Impact of a horizontally transmitted endophyte,balansia henningsiana, on growth and drought tolerance ofpanicum rigidulum. Int. J. Plant Sci. 170, 599–608. doi: 10.1086/597786
Rengasamy, P. (2006). World salinization with emphasis on australia. J. Exp. Bot. 57, 1017–1023. doi: 10.1093/jxb/erj108
Ren, A. Z., Li, X., Han, R., Yin, L. J., Wei, M. Y., Gao, Y. B. (2011). Benefits of a symbiotic association with endophytic fungi are subject to water and nutrient availability in achnatherum sibiricum. Plant Soil 346, 363–373. doi: 10.1007/s11104-011-0824-9
Reza Sabzalian, M., Mirlohi, A. (2010). Neotyphodium endophytes trigger salt resistance in tall and meadow fescues. J. Plant Nutr. Soil Sci. 173, 952–957. doi: 10.1002/jpln.200900345
Richardson, M., Chapman, G., Jr., Hoveland, C., Bacon, C. (1992). Sugar alcohols in endophyte-infected tall fescue under drought. Crop Sci. 32, 1060–1061.
Rodriguez, C., Mayo, J. C., Sainz, R. M., Antolin, I., Herrera, F., Martin, V., et al. (2004). Regulation of antioxidant enzymes: A significant role for melatonin. J. Pineal Res. 36, 1–9. doi: 10.1046/j.1600-079x.2003.00092.x
Rodriguez, R., Redman, R. (2008). More than 400 million years of evolution and some plants still can't make it on their own: Plant stress tolerance via fungal symbiosis. J. Exp. Bot. 59, 1109–1114. doi: 10.1093/jxb/erm342
Saikkonen, K., Lehtonen, P., Helander, M., Koricheva, J., Faeth, S. H. (2006). Model systems in ecology: Dissecting the endophyte-grass literature. Trends Plant Sci. 11, 428–433. doi: 10.1016/j.tplants.2006.07.001
Selvakumar, G., Thamizhiniyan, P. (2011). The effect of the arbuscular mycorrhizal (am) fungus glomus intraradices on the growth and yield of chilli (capsicum annuum l.) under salinity stress. World Appl. Sci. J. 14, 1209–1214.
Shi, X., Qin, T., Liu, H., Wu, M., Li, J., Shi, Y., et al. (2020). Endophytic fungi activated similar defense strategies of achnatherum sibiricum host to different trophic types of pathogens. Front. Microbiol. 11, 1607 doi: 10.3389/fmicb.2020.01607
Tavakkoli, E., Fatehi, F., Rengasamy, P., McDonald, G. K. (2012). A comparison of hydroponic and soil-based screening methods to identify salt tolerance in the field in barley. J. Exp. Bot. 63, 3853–3867. doi: 10.1093/jxb/ers085
Tavakkoli, E., Rengasamy, P., McDonald, G. (2010). The response of barley to salinity stress differs between hydroponic and soil systems. Funct. Plant Bio 37, 621–633. doi: 10.1071/FP09202
Tomar, N. S., Agarwal, R. M. (2013). Influence of treatment of jatropha curcas l. leachates and potassium on growth and phytochemical constituents of wheat (triticum aestivum l.). Amer J. Bot. 04, 1134–1150. doi: 10.4236/ajps.2013.45140
Wang, X., Qin, J., Chen, W., Zhou, Y., Ren, A., Gao, Y. (2015). Pathogen resistant advantage of endophyte-infected over endophyte-free leymus chinensis is strengthened by pre-drought treatment. Eur. J. Plant Pathol. 144, 477–486. doi: 10.1007/s10658-015-0788-3
Wei, Y. K., Gao, Y. B., Xu, H., Su, D., Zhang, X., Wang, Y. H., et al. (2006). Occurrence of endophytes in grasses native to northern china. Grass Forage Sci. 61, 422–429. doi: 10.1111/j.1365-2494.2006.00551.x
White, J. F. (1993). Endophyte-host associations in grasses. xix. a systematic study of some sympatric species of epichloe in england. Mycologia. 85, 444. doi: 10.1080/00275514.1993.12026295
Xia, C., Zhang, X., Christensen, M. J., Nan, Z., Li, C. (2015). Epichloë endophyte affects the ability of powdery mildew (blumeria graminis) to colonise drunken horse grass (achnatherum inebrians). Fungal Eco 16, 26–33. doi: 10.1016/j.funeco.2015.02.003
Yang, C. W., Xu, H. H., Wang, L. L., Liu, J., Shi, D. C., Wang, D. L. (2009). Comparative effects of salt-stress and alkali-stress on the growth, photosynthesis, solute accumulation, and ion balance of barley plants. Photosynthetica. 47, 79–86. doi: 10.1007/s11099-009-0013-8
Yin, L., Ren, A., Wei, M., Wu, L., Zhou, Y., Li, X., et al. (2014). Neotyphodium coenophialum-infected tall fescue and its potential application in the phytoremediation of saline soils. Int. J. Phytoremediation 16, 235–246. doi: 10.1080/15226514.2013.773275
Zhang, J., Zhang, Y., Du, Y., Chen, S., Tang, H. (2011). Dynamic metabonomic responses of tobacco (nicotiana tabacum) plants to salt stress. J. Proteome Res. 10, 1904–1914. doi: 10.1021/pr101140n
Keywords: endophyte, Epichloë bromicola, Leymus chinensis, neutral salt stress, alkali salt stress
Citation: Yin L, Wei M, Wu G and Ren A (2022) Epichloë endophytes improved Leymus chinensis tolerance to both neutral and alkali salt stresses. Front. Plant Sci. 13:968774. doi: 10.3389/fpls.2022.968774
Received: 14 June 2022; Accepted: 04 October 2022;
Published: 18 October 2022.
Edited by:
Janusz Zwiazek, University of Alberta, CanadaReviewed by:
Barbara Wiewióra, Plant Breeding and Acclimatization Institute, PolandÁgnes Szepesi, University of Szeged, Hungary
Copyright © 2022 Yin, Wei, Wu and Ren. This is an open-access article distributed under the terms of the Creative Commons Attribution License (CC BY). The use, distribution or reproduction in other forums is permitted, provided the original author(s) and the copyright owner(s) are credited and that the original publication in this journal is cited, in accordance with accepted academic practice. No use, distribution or reproduction is permitted which does not comply with these terms.
*Correspondence: Guanghong Wu, d3VndWFuZ2hvbmdAZ21haWwuY29t; Anzhi Ren, cmVuYW56aGlAbmFua2FpLmVkdS5jbg==