- 1Institute of Environmental Resources and Soil Fertilizers, Zhejiang Academy of Agricultural Sciences, Hangzhou, China
- 2Department of Biosystems and Technology, Swedish University of Agricultural Sciences, Alnarp, Sweden
- 3School of Biological Sciences, The University of Western Australia, Perth, WA, Australia
- 4Arable Soil Quality and Fertilizer Administration Station of Zhejiang Province, Hangzhou, China
- 5Centre of Excellence for Soil Biology, College of Resources and Environment, Southwest University, Chongqing, China
Soil acidification often occurs when the concentration of ammonium (NH4+) in soil rises, such as that observed in farmland. Both soil acidification and excess NH4+ have serious adverse effects on crop growth and food production. However, we still do not know which of these two inhibitors has a greater impact on the growth of crops, and the degree of their inhibitory effect on crop growth have not been accurately evaluated. 31 wheat cultivars originating in various areas of China were planted under 5 mM sole NH4+ (ammonium nitrogen, AN) or nitrate nitrogen in combined with two pH levels resembling acidified conditions (5.0 and 6.5). The results showed that the shoots and roots biomass were severely reduced by AN in both and these reduction effects were strengthened by a low medium pH. The concentration of free NH4+ and amino acids, the glutamine synthetase activity were significantly higher, but the total soluble sugar content was reduced under NH4+ conditions, and the glutamine synthetase activity was reduced by a low medium pH. Cultivar variance was responsible for the largest proportion of the total variance in plant dry weight, leaf area, nodal root number, total root length and root volume; the nitrogen (N) form explains most of the variation in N and C metabolism; the effects of pH were the greatest for plant height and root average diameter. So, soil acidification and excess NH4+ would cause different degrees of inhibition effects on different plant tissues. The findings are expected to be useful for applying effective strategies for reducing NH4+ stress in the field.
Introduction
The industrial nitrogen (N) fertilizers application has contributed tremendously to the increase in global food production (Gong et al., 2011; Zhang et al., 2013). However, less than half of the applied N (30–40%) is utilized by crops in China to date (Ding et al., 2020). The N lost included run-off, volatilization, leaching, and denitrification, which together lead to a range of major ecological challenges and environmental problems (Chen et al., 2014). Unlike nitrate nitrogen (NN), ammonium (NH4+) nitrogen (AN) can be assimilated into amino acid production by plants without further chemical reduction (Coskun et al., 2017). The NH4+-based stabilized fertilizers application was considered to be a promising future strategy for improving agronomic N use efficiency (Ren et al., 2021). Unfortunately, most dryland crops, including wheat, can be markedly inhibited when the concentration of ammonium in soil is too high, whereas the comparable inhibitory effect does not occur for NN fertilization (Miller and Cramer, 2005; Coskun et al., 2017). In southern China, especially in red-yellow soil areas, approach 20 mM NH4+ concentration in soil was recorded (Xu et al., 2012), much higher than the 0.1–0.5 mM optimal NH4+ concentration for upland crops (Liu et al., 2013).
The membrane potential would be depolarized during the NH4+ uptake and assimilation process, resulting in an increase in the net proton release, as well as external soil acidification (Zhu et al., 2012). Over the last few decades, the Ca2+, Mg2+, and K+ depletion and sulfur (S) and N atmospheric deposition have promoted soil acidification and reduced the soil’s buffering capacity, particularly in the red-yellow soil of southern China, which is located in tropical/subtropical regions; in this region, an average soil pH of 4.5–5.5 has been recorded (Guo et al., 2010). Soil acidification also produces adverse effects on cell metabolism (Van Den Berg et al., 2005). NH4+ was assimilated into organic N compounds in cells primarily through the GS/GOGAT pathway (glutamine synthetase, GS, EC 6.3.1.2; glutamate synthase, GOGAT, EC 1.4.1.14) (El Omari et al., 2010; Xu et al., 2012). Plant species maintaining a low intracellular with higher GS activity are thought to be more tolerant to NH4+ toxicity (Cruz et al., 2006). In addition, the increased carbon consumption caused by increased NH4+ assimilation in roots also partially explain NH4+-induced growth inhibition (Britto and Kronzucker, 2002). However, few studies have examined the relationship between NH4+ stress and soil acidification or whether the NH4+-induced growth inhibition is caused by an NH4+ form source or soil acidification caused by NH4+ absorption.
The understanding of plant growth in response to NH4+ tolerance is mainly based on studies of mutants and transformants or some model plants such as rice, Arabidopsis et al. (Guo et al., 2007; Zhu et al., 2009; Li et al., 2010). Wheat is one of the most widely cultivated crops in the world, which is sensitive to NH4+ toxicity (Wang et al., 2019). In many cases, wheat have to face excess NH4+ in China, which is often the case in the field with the use of slow-release fertilizers and nitrification inhibitors, especially during fertilization stag. It is a very important and necessary object to study genetic variability of wheat adaptation to NH4+ toxicity combined with soil acidification. Its ecotypes have been found show a wide range of habitats differing notably in soil richness. These natural variations indeed provide a framework to study the adaptation of different traits that define plant growth in relation to simultaneous genetic changes and environmental variations, while there is lack of studies on this topic In this study, we conducted hydroponic experiments using 31 wheat cultivars in media with different N forms (AN or NN) and pH values. The objectives of this study were to evaluate the inhibitory degree of NH4+ form source and soil acidification on crop growth. The results would provide useful insight into the selection and breeding of crop cultivars with greater potential to adapt to NH4+-form N source combined with soil acidification in the field.
Materials and methods
Plant materials and experimental design
Seeds of 31 wheat varieties of China selected from a collection that was shown to maximize the genetic diversity of a large set of genotypes of China (Supplementary Table S1) were used. 20% (v/v) H2O2 were used to surface-sterilized uniform seeds for 10 min, and then rinsed the seeds more than 5 times with distilled water. The seeds were germinated on filter paper covering with wet sterilized gauze, and then transplanted them to sterilized silica sand until the seedling bud length was about 1 cm. At two-leaf stage, uniform seedlings were transplanted into black plastic containers (length × width × height, 45 cm × 32 cm × 15 cm) in an artificial illumination incubator with a 16 h/8 h light (a 1,200 μmol m−2 s−1 photosynthetic photon flux density)/dark with temperatures of 18°C day/10°C night cycle. A modified Hoagland nutrient solution (Gao et al., 2018) was used with two N form sources: sole NO3− source solution (NN) or a sole NH4+ source solution (AN). The sole NO3− source solution composition was as follows: macronutrients (mmol L−1): 5.0 N as KNO3 and Ca(NO3)2 or (NH4)2SO4, 3.0 K as KH2PO4 and K2SO4 or KNO3, 1.5 Ca as CaSO4, or CaCl2 and Ca(NO3)2, 1.0 Mg as MgSO4, 1.0 P as KH2PO4, 0.5 Na as NaCl; and micronutrients (mmol L−1): 1.0 Fe as Fe-EDTA, 0.15 × 10−3 Zn as ZnSO4, 0.16 × 10−3 Cu as CuSO4, 9.10 × 10−3 Mn as MnSO4, 18.5 × 10−3 B as H3BO3, 0.52 × 10−3 Mo as MoO3. The sole NH4+ source solution composition was as follows, macronutrients (mmol L−1): 5.0 mM N as (NH4)2SO4, 3.0 mM K as K2SO4 and KH2PO4, 1.5 mM Ca as CaSO4 and CaCl2, 1.0 mM P as KH2PO4, 1.0 mM Mg as MgSO4, and 0.5 mM Na as NaCl. The micronutrients composition in the AN source solution were the same with those in the NN source solution.
The pH of the AN or NN source solution was adjusted to 5.0 (to imitate the acidified red-yellow soil region of southern China) or 6.5 (as the normal condition for wheat growth) by adding 0.1 mM H2SO4 or 0.1 mM NaOH to the solutions; thus, the solutions are denoted by the treatment solution-pH as NN-6.5, AN-6.5, NN-5.0, and AN-5.0. The pH of the Hoagland solution was adjusted daily to the pH 5.0 or 6.5 also using 0.1 mM NaOH or 0.1 mM H2SO4 during the whole experimental process. Dicyandiamide (A nitrification inhibitor, 1 mM) was added to prevent NH4+ microbial oxidation. The solutions were replenished every 3 days. All treatments had three replicates with a completely random design.
Plant sampling and traits analysis
Shoots and roots were harvested 21 days after the start of the different pH values and N forms treatment, frozen in liquid nitrogen, the stored at −80°C for physiological analysis, and dried partially at 105°C for 20 min and then 75°C for 24 h to obtain a constant weight. Root morphology was estimated from images of plants taken by a root scan machine (Epson 1,680, Indonesia), with WinRhizo Pro Vision 5.0 analysis program (Canada). A Li-3000 area meter (Li-Cor Inc., Lincoln, NE, United States) was used to measure leaf area. Free NH4+ concentration in plant shoot and root was measured according to the method of Balkos et al. (2010). Soluble sugars concentration in tissues were measured from the hydroalcoholic extracts using a colorimetric assay with an anthrone reagent (Wang et al., 2019). Free amino acids concentration in shoot and root was determined using the method of Moore and Stein (1954). GS activity and nitrate reductase (NR) activity were determined, as described previously (Wang et al., 2016).
Statistical analysis
The variance (ANOVA) analysis was carried out using the SPSS general linear model procedure (SPSS 19.0, United States). The different forms and pH levels of N nutrition and different varieties were considered the observed variation sources for the studied cultivars. The quantity of variation (%) for each factor was estimated using the total calculated sum of squares (Wang et al., 2016). The figures were created using SigmaPlot graphics software (ver. 14.0; Systat Inc., San Jose, CA, United States).
Results
Plant growth
Both the sole NH4+ source and rhizosphere acidification inhibited plant growth (Figure 1). Compared to normal growth conditions (NN-6.5, NN at a pH of 6.5), shoot, root, and plant dry weights, plant height; and leaf area were reduced by 14.7, 33.4, and 19.0%; 16.2%; and 13.0%, respectively, for AN-6.5; 23.7, 21.7, and 23.2%; 24.3%; and 23.3% for NN-5.0; and 33.3, 47.0, and 36.4%; 34.0%; and 31.7% for AN-5.0. All of these parameters significantly differed according to cultivar, N form, and pH level; also, there were N form × pH levels interaction effects (Table 1). In shoot dry weight, plant dry weight (41.4%), and leaf area (60.4%), cultivar variance explained the largest proportion of the total variance (48.1%). Differently, N forms and pH levels accounted for the largest proportion of the variance in root dry weight (40.3%) and plant height (38.2%), respectively.
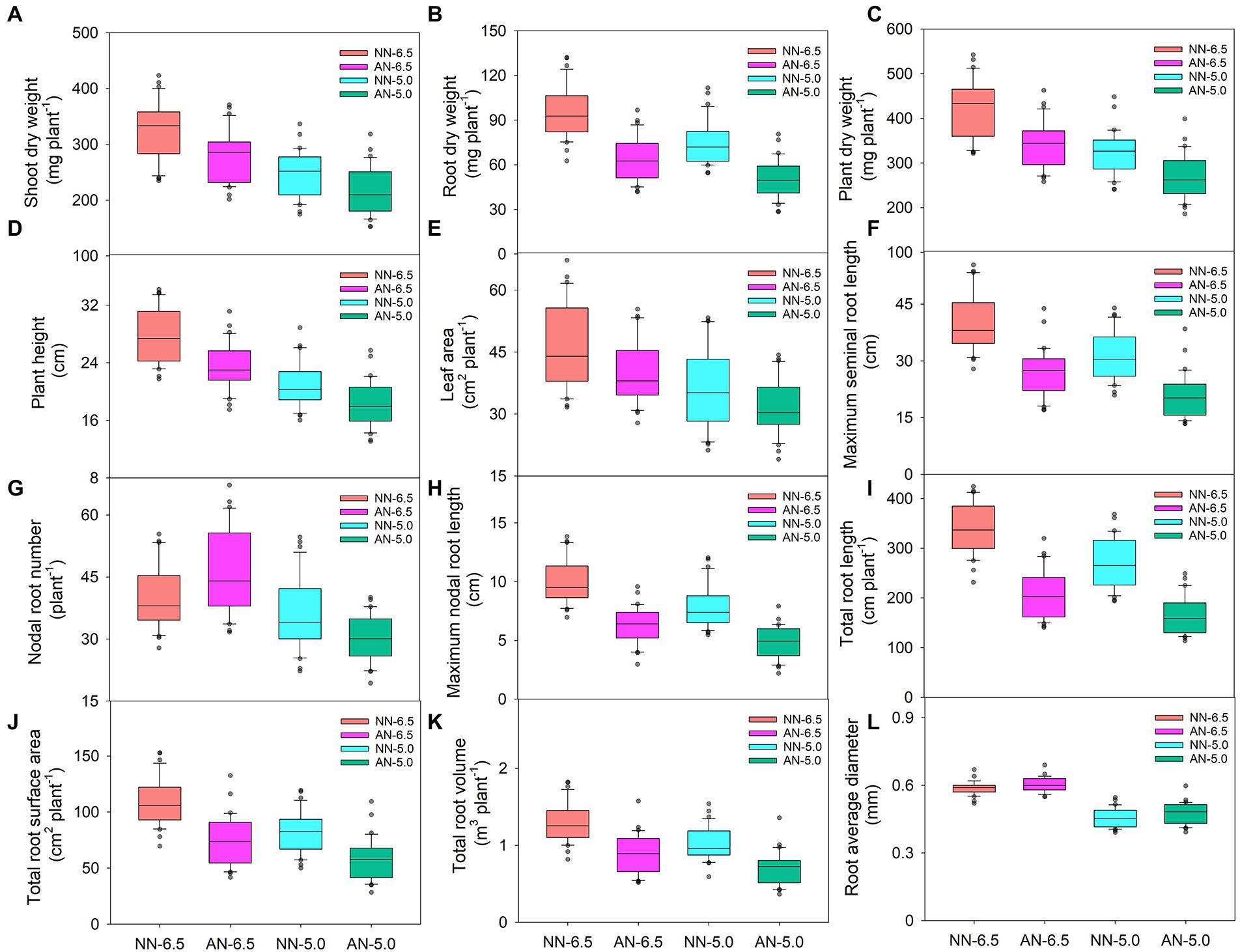
Figure 1. Biomass allocation, plant height, leaf area and root morphology of 31 wheat cultivars grown under different N-sources and pH levels. Data are means ± SE (n = 31). NN-6.5 refers to the nitrate nitrogen at a pH of 6.5; NN-4.5 refers to the nitrate nitrogen at a pH of 6.5; AN-6.5 refers to the ammonium nitrogen at a pH of 6.5; AN-5.0 refers to the ammonium nitrogen at a pH of 5.0.
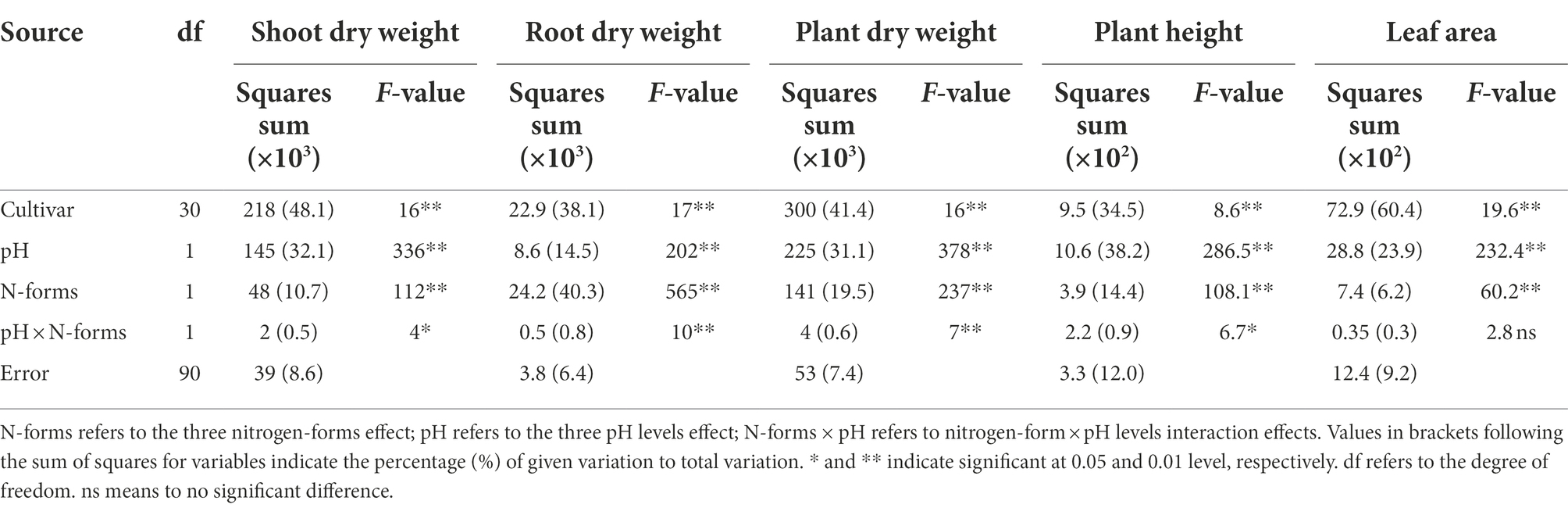
Table 1. F-values in two-way ANOVA analysis of shoot dry weight, root dry weight, plant dry weight, plant height and leaf area in 31 wheat cultivars seedlings under different N-sources and pH levels.
Root morphogenesis also depended on N form and rhizosphere acidification conditions (Figure 1). Compared with the NN-6.5 condition, maximum seminal and nodal root length, total root length, root surface area and root volume decreased by 33.4, 37.6, 38.3, 31.7 and 31.1% respectively, but nodal root number and root average diameter increased by 15.0 and 2.6%, respectively, at AN-6.5. Compared with NN-6.5, at NN-5.0, maximum seminal and nodal root length, nodal root number, total root length, root surface area, root volume, root average diameter decreased by 22.3, 21.7, 11.7, 20.8, 24.9, 20.3, 22.7% respectively, meanwhile, the decline was even greater at AN-5.0, which was decreased by 48.3, 37.6, 22.7, 51.2, 47.6, 45.5 and 19.7%, respectively. These traits also varied significantly according to cultivar, N form, pH level and N form × pH level interaction effects (Table 2). N forms accounted for the largest proportion of total variance in root length, and cultivar variance was the greatest for root number (59.4%), surface area (42.4%), and volume (47.0%). The average diameter of roots was significantly affected by pH level, but was not affected by the pH and N form interaction.
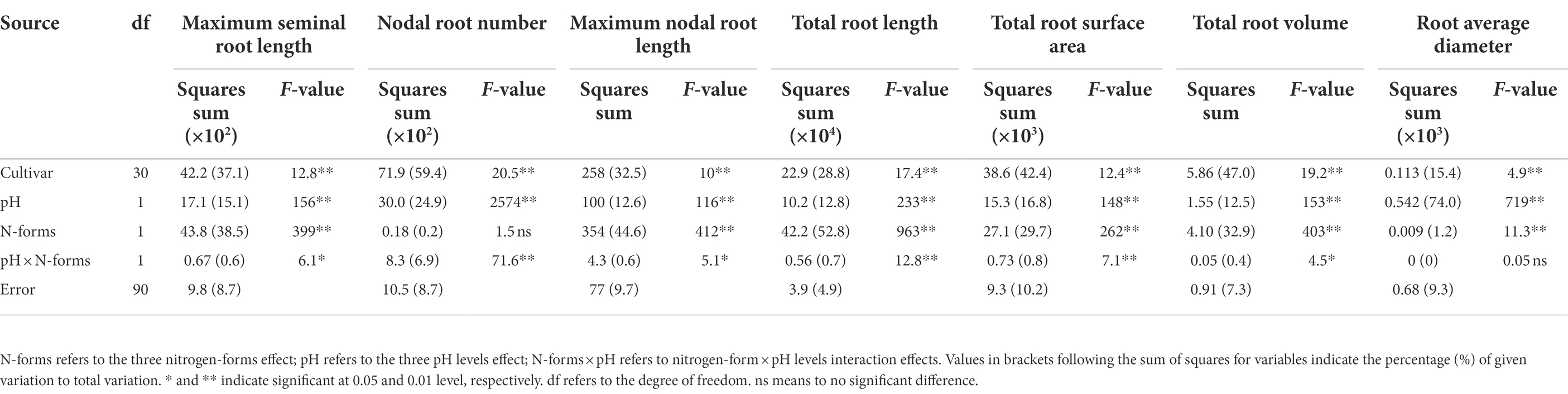
Table 2. F-values in two-way ANOVA analysis of root morphological traits in 31 wheat cultivar seedlings under different N-sources and pH levels.
Free NH4+ concentration
The concentration of free NH4+ in wheat under AN was apparently higher than that under NN nutrition condition at a uniform pH (Figure 2). Compared to NN-6.5, the concentration in shoots was 42.2 and 2.1% higher under AN-6.5 and AN-5.0 but 22.5% lower under NN-5.0 treatments. It changed more in roots than in aerial parts. Compared to NN-6.5, the concentration in roots increased by 365.3 and 214.4% under AN-6.5 and AN-5.0 but decreased by 21.5% under NN-5.0 treatments. In shoot and root, the free NH4+ concentrations significantly varied by cultivar, N form, and pH level, but changes in cultivars caused the highest proportion of the variance (47.1%) in shoot; but N forms explained the largest proportion of total variance (77.0%) in root.
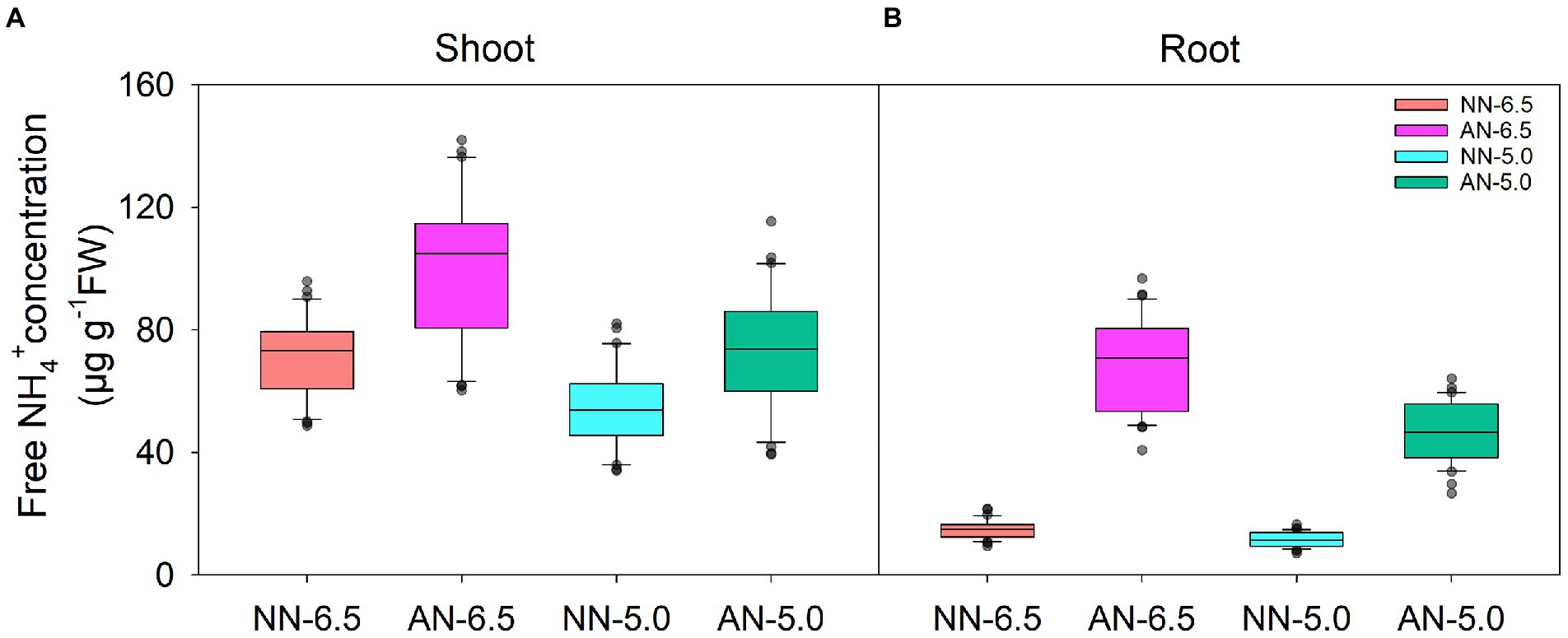
Figure 2. The levels of NH4+ in 31 wheat cultivars grown under different N-sources and pH levels. Data are means ± SE (n = 31). NN-6.5 refers to the nitrate nitrogen at a pH of 6.5; NN-4.5 refers to the nitrate nitrogen at a pH of 6.5; AN-6.5 refers to the ammonium nitrogen at a pH of 6.5; AN-5.0 refers to the ammonium nitrogen at a pH of 5.0.
Soluble sugar and free amino acid concentration
Soluble sugar, as the C skeleton for NH4+ assimilation, also varied with N form and rhizosphere acidification conditions (Figures 3A,B). Compared to NN-6.5, the soluble sugar concentrations in shoot and root were lower under AN-6.5, NN-5.0, and especially AN-5.0; also, the level of changed tended to be higher in roots than in shoots. In contrast, as the primary assimilation product of NH4+, free amino acid levels were significantly higher under AN than under NN nutrition. The concentration of free amino acids in shoots under AN-6.5 and AN-5.0 increased by 171.6 and 64.4%, respectively, compared to NN nutrition with pH 6.5, but decreased by 24.3% under NN-5.0 treatment, as shown in Figures 3C,D. Compared to NN-6.5, the concentration of free amino acids in roots under AN-6.5 and AN-5.0 increased by 161.9 and 38.0%, respectively, and was reduced by 22.8% in roots under NN-5.0. The ratio of soluble sugar to free amino acid reflects a balance status of C and N in the process of NH4+ assimilation. As shown in Figures 3E,F, compared to nitrate treatments, low pH and AN markedly reduced the ratio of soluble sugar of free amino acid in shoot and root. Compared to NN-6.5, NN-5.0, AN-6.5, and AN-5.0 showed reductions in the soluble sugar/free amino acid ratio of 72.3, 22.9, and 52.0% in shoots and by 76.1, 0.7, and 65.7% in roots, respectively. N form explained most of the variance in the soluble sugar and free amino acids concentrations in shoot and root (Table 3).
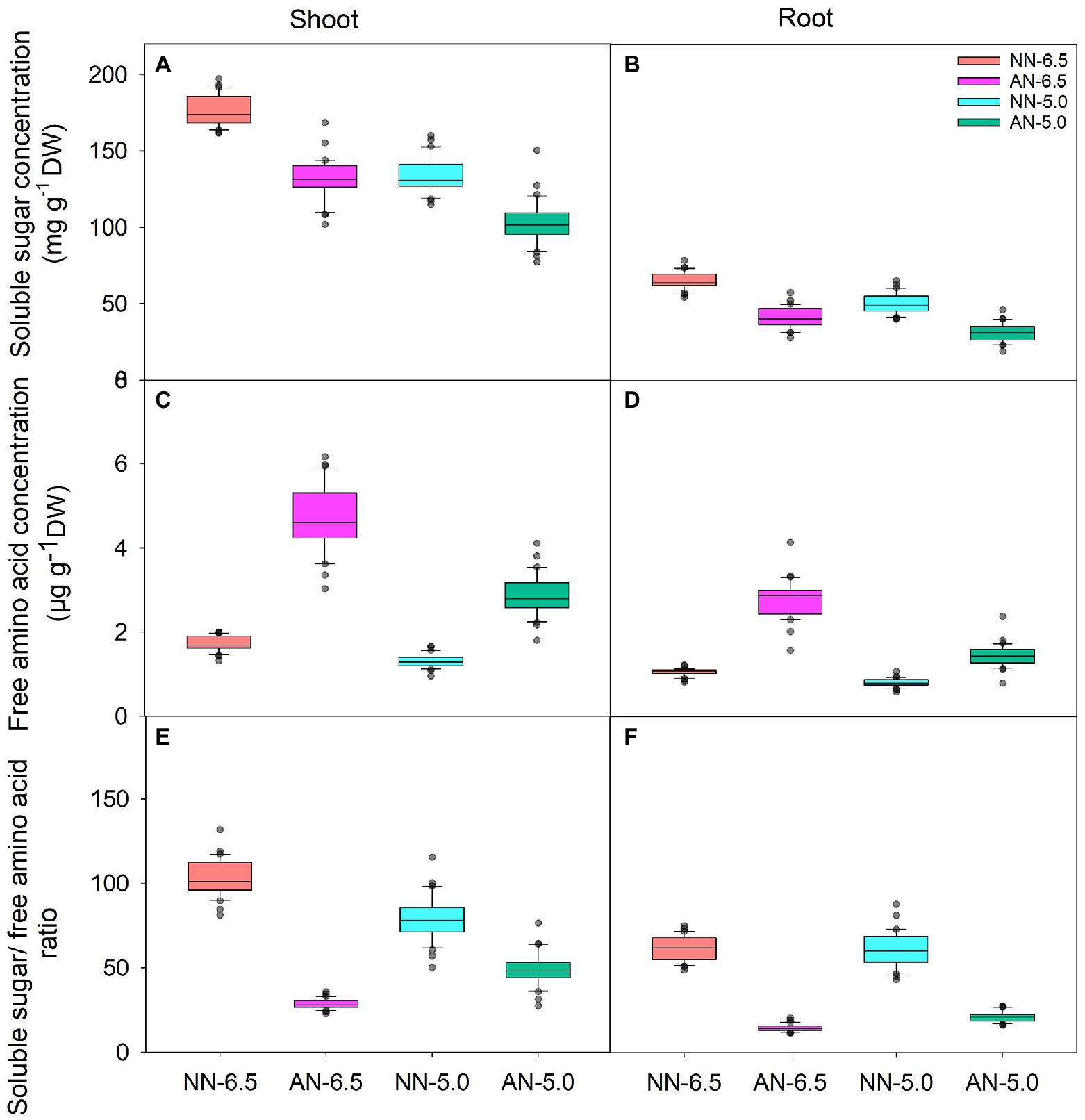
Figure 3. The levels of soluble sugar, free amino acids and soluble sugar/ free amino acid ratio in shoots and roots of 31 wheat cultivars grown under different N-sources and pH levels. Data are means ± SE (n = 31). NN-6.5 refers to the nitrate nitrogen at a pH of 6.5; NN-4.5 refers to the nitrate nitrogen at a pH of 6.5; AN-6.5 refers to the ammonium nitrogen at a pH of 6.5; AN-5.0 refers to the ammonium nitrogen at a pH of 5.0.
GS and NR activities
GS and NR activities represent the N assimilation ability of wheat and significantly differed by cultivar, N form, and pH level (Figure 4). Compared to NN-6.5, the GS activity increased (86.8%) in shoots under AN-6.5 and decreased (25.2 and 12.8%, respectively) under NN-5.0 and AN-5.0; in roots, it increased (133.7 and 83.2%, respectively) under AN-6.5 and AN-5.0 but decreased (83.2%) under NN-5.0. Compared to NN treatments, pH and AN markedly reduced the NR activity in both shoots and roots. Their activities also varied apparently among all the cultivar, N forms, pH levels and the interaction effects of nitrogen-form × pH levels (Table 4). Variance in pH levels had the strongest effect on shoot GS activity (42.0%); N form had the greatest effect on the GS activity in root, and the NR activity in both shoot and root.
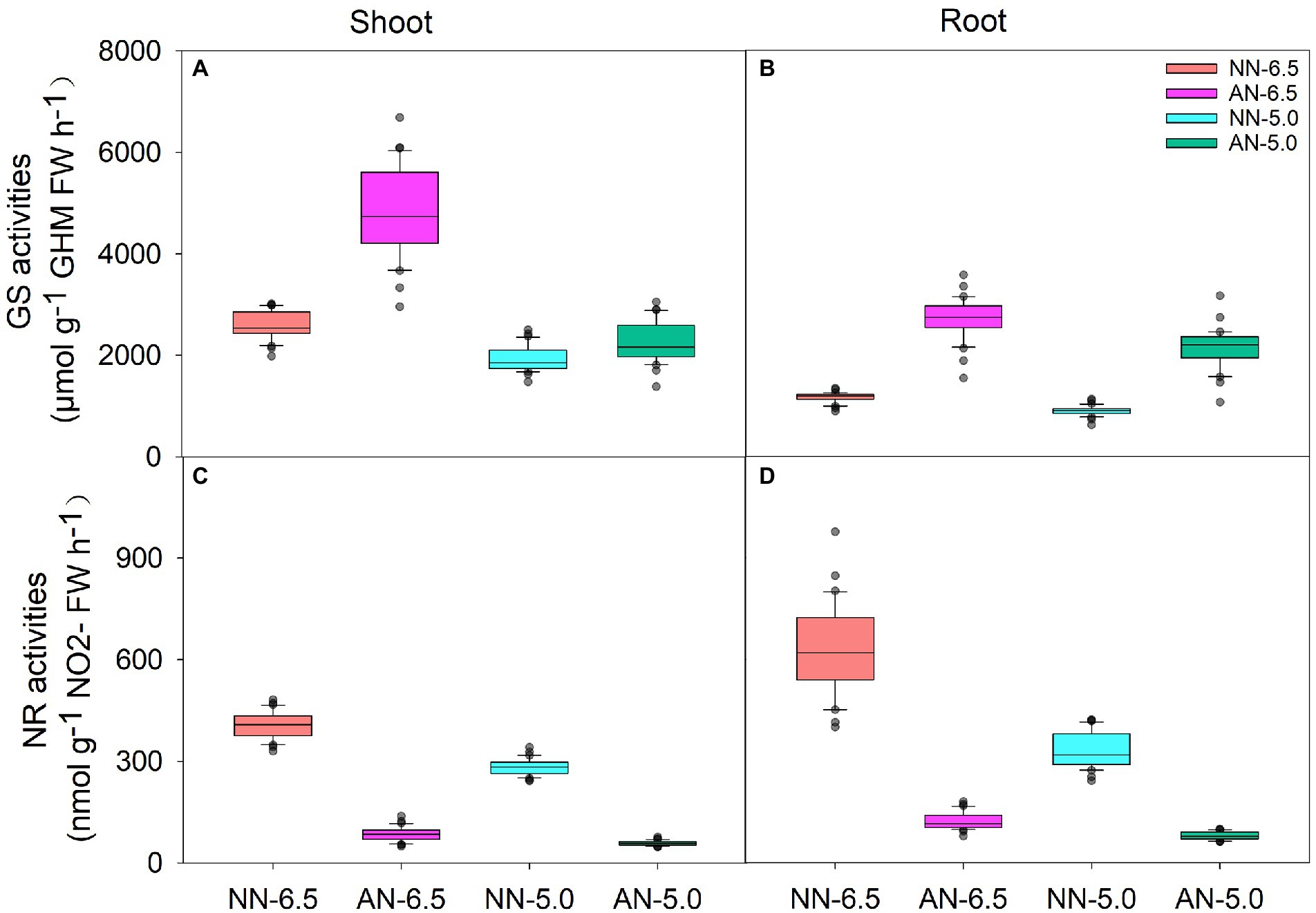
Figure 4. The enzymatic activities of GS and NR in shoots and roots of 31 wheat cultivars under different N-sources and pH levels. Data are means ± SE (n = 31). NN-6.5 refers to the nitrate nitrogen at a pH of 6.5; NN-4.5 refers to the nitrate nitrogen at a pH of 6.5; AN-6.5 refers to the ammonium nitrogen at a pH of 6.5; AN-5.0 refers to the ammonium nitrogen at a pH of 5.0.
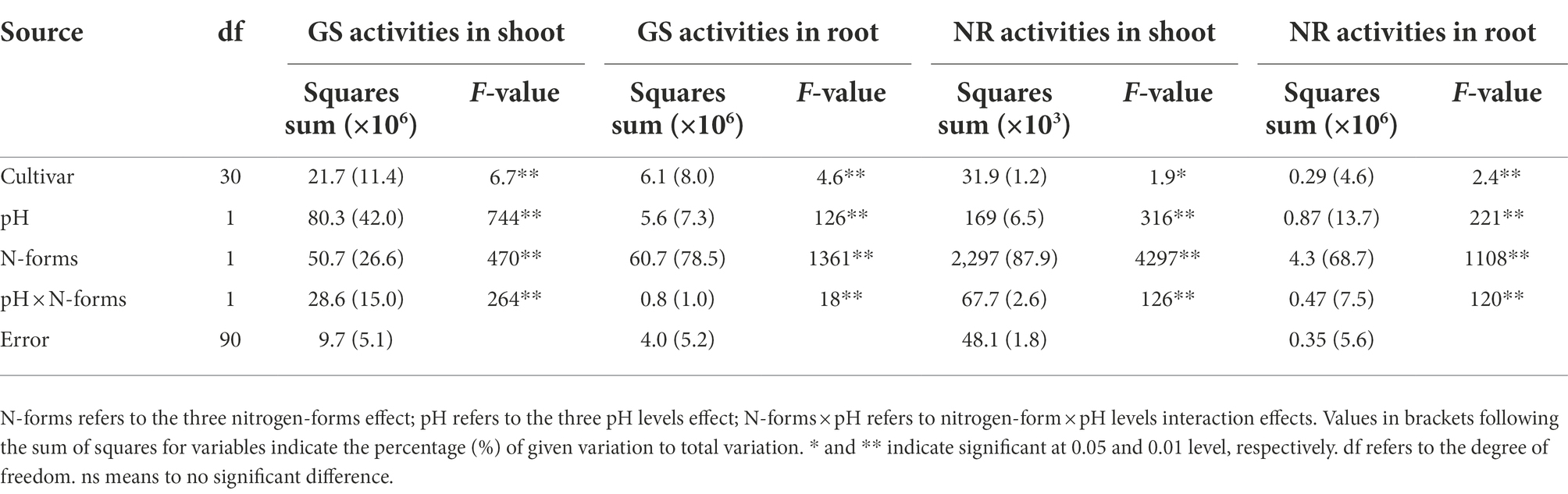
Table 3. F-values in two-way ANOVA analysis of GS and NR activities in shoot or root of 31 wheat cultivar seedlings under different N-sources and pH levels.
Discussion
Several studies have provided a general description of plant responses to changes in the AN environment (Britto and Kronzucker, 2013; Wang et al., 2019); however, most of them have considered only a small number of genotypes, in which the effects of soil acidification caused by NH4+ absorption is neglected, thus only a partial view of the phenomena given. Since wheat ecotypes have been found in a wide range of habitats differing notably in NH4+ conditions in our previous studies (Wang et al., 2016, 2019, 2020), few studies have reported that using this natural variation among ecotypes as an alternative way to search genes related to NH4+ tolerance. In the present studies, the responses of wheat to different N forms and pH values was studied by measuring morphological traits and metabolic traits in 31 wheat genotypes. Result showed that cultivar variance explained the largest proportion of total variance in shoot dry weight, plant dry weight, leaf area, nodal root number, total root surface area, total root volume and the free NH4+ concentration in shoot when wheat seedlings were planted in the solution as NN-6.5, AN-6.5, NN-5.0 or AN-5.0. Previous studies found wheat was sensitive to increased NH4+ concentration in soil (Cao et al., 2009; Ariz et al., 2010; Zhu et al., 2012; Setien et al., 2013), furtherly, the present results clearly indicated that NH4+ nutrition and soil acidification significantly reduced biomass accumulation and leaf area in wheat plants. The dry weight of roots was considerably reduced by AN treatment, not only due to limitations in root emergence but also because of a reduction in root length (reduced by 22.3 and 21.7% in maximum seminal root length and nodal root length, respectively). This NH4+-induced inhibition is related to root growth and development also has been observed by some previous studies (Cramer and Lewis, 1993; Britto and Kronzucker, 2002; Zhang and Wang, 2012). Notably, in the present study, the decline was even greater at lower pH levels under AN conditions, but N form still accounted for the largest proportion of variance in root length (Table 2). Li et al. (2010, 2013) found that nearly 70% of the NH4+-induced root elongation inhibition was accounted by the cell expansion reduction, which root elongation and development, was mediated by reduced auxin by NH4+ condition (Li et al., 2011). In the present study, the nodal root number was maintained or increased under AN but decreased at lower pH. The nodal root number was more strongly affected by variation in pH than by variation in N form (Table 2), which might be explanted by a higher accumulation of cytokinin in NH4+-fed roots (Wang et al., 2019) in our previous study.
As a typical symptom of “ammoniacal syndrome,” free NH4+ concentration in plant cells was significantly higher under AN conditions than under NN. This may be associated with the ability of root system to absorb NH4+ (Zhu et al., 2009), the rate of NH4+ efflux from root inside (Li et al., 2011), and also NH4+ assimilation in root cell (Setien et al., 2013). The free NH4+ concentration increased under AN condition but decreased with lower pH in roots, but N forms was the crucial factor as Table 4 shown. The uptake of NH4+ depolarizes the plasma membrane, increasing the net proton (H+) release from the cell inside to rhizosphere soil (Zhu et al., 2009), furtherly leading to rhizosphere acidification. The H+-ATPase in plasma membrane made a decisive contribution to maintain the balance of pH inside and outside cells, which hydrolyzed ATP to pump H+ out; and an electrochemical gradient generated which could drive the free NH4+ ions active transport crossing the plasma membrane (Zhu et al., 2012). At a low medium root pH, the activity of H+-ATPase must be smartly enhanced to pump H+ outside to adapt to an increased electrochemical gradient, to ensure NH4+ uptake (Zhu et al., 2009). Therefore, the low medium pH may inhibit NH4+ absorption while high pH may facilitate NH4+ uptake (Brix et al., 2002). Our results also showed that, in wheat root, the N form was the main reason for the changes in the free NH4+ concentration which was consistent with the previous studies; however, interestingly, free NH4+ in wheat shoot varied most by cultivar, which had not been reported before.
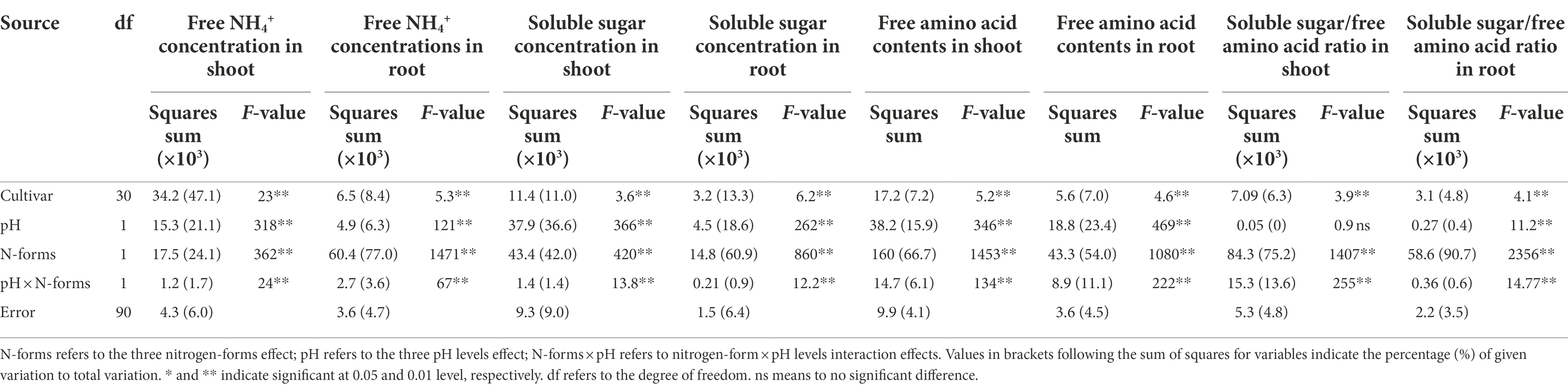
Table 4. F-values in two-way ANOVA analysis of free NH4+ concentration, soluble sugar, free amino acid concentration and soluble sugar/ free amino acid ratio in shoot or root of 31 wheat cultivars seedlings under different N-sources and pH levels.
Apart from the ability of plant root to absorb NH4+, the allocation of NH4+ also depends on the N metabolism process. NH4+ absorbed by roots is mostly assimilated into amino acids in root cells (Masclaux-Daubresse et al., 2010). In the present study, AN resulted in a remarkedly increase in the free amino acid concentration in shoots and roots, confirming a metabolic adaptation to AN condition, as previously discussed by Lothier et al. (2011). The enhanced N compound pool was probably due to both high NH4+ absorption and increased N assimilation to cope with having solely AN to maintain plant growth (Wang et al., 2020). The negative correlation between free amino acid concentration and soluble sugar concentration in roots and shoots was detected, and AN markedly reduced the soluble sugar/free amino acid ratio, indicating that more N-assimilation occurred in roots. Thus, a sufficient carbon skeleton supply for NH4+ assimilation is required under AN condition. A higher photosynthetic capacity increases plant NH4+ assimilation and tolerance, providing C skeletons to fuel the cell respiratory action and translocate more carbohydrates downward to maintain root growth (Ariz et al., 2011). In the present study, a distinct decrease in soluble sugar concentration accrued under AN condition (as the carbon skeleton for NH4+ assimilation) in 31 wheat cultivars, particularly in the roots (Figure 3); however, this decrease was lower at pH 6.5 than at pH 5.0, possibly due to the higher photosynthetic capacity at pH 6.5 (Ariz et al., 2011, 2013), and also the inhibited capacity of NH4+ assimilation. If the capacity of NH4+ assimilation is depressed by low pH, more NH4+ may accumulate in root and then be transported upward to the shoot, which is proven to be more sensitive to NH4+ stress (Britto and Kronzucker, 2002; Cruz et al., 2006); this leads to inhibition of photosynthesis and CO2 fixation (Guo et al., 2007), resulting in a further reduction in C assimilation.
Some studies have found that the relative assimilation enzyme activity in the GS/GOGAT cycle plays an important role for crops in adaptation to AN condition (El Omari et al., 2010). In the present study, GS activity was much increased, but NR activity was decreased by AN (Figure 4); pH level had the strongest effect on shoot GS activity and N form had the greatest effect on root GS activity. In different tissues of cells, GS exists as isoforms GS1 (found in the cell cytosol) and GS2 (in plastids) (Singh and Ghosh, 2013). GS1 is the predominant enzyme in non-photosynthetic tissues or root, but slightly presents in green tissues like young stem or leaf (Simon and Sengupta-Gopalan, 2010), which metabolic role includes assimilating NH4+ into amino acids (glutamine) for N transport and distribution (Bernard et al., 2008). AN treatment increased the GS activity, especially the root GS activity (the predominant isoform GS1). The increased capacity of NH4+assimilation by GS1 could prevent NH4+ accumulation in the roots and transport to the shoots (Setien et al., 2013), but this higher GS activity was not inhibited by the low medium pH significantly, indicating GS1 was insensitive to low pH. GS2 played important roles in NH4+ re-assimilation during photorespiration and the part of NH4+, which was derived from nitrate reduction (Singh and Ghosh, 2013), mostly located in the chloroplasts stroma, and its physiological importance has been demonstrated in transgenic plants overexpressing GS2 in leaves (Bernard et al., 2008). Increased GS2 activity had an enhanced capacity for photorespiration, also retained more than 90% of their photosystem II activity to fight against osmotic stress (Bernard and Habash, 2009). In the present study, a relative increase in shoot GS activity (GS2) may maintain photorespiratory capacity while improving the cultivar’s tolerance to NH4+ stress, but unlike GS1, its activity decreased sharply under low pH, and pH level had the strongest effect on it, indicating GS2 was more susceptible to soil acidification stress.
Conclusion
In conclusion, we studied the variation in N assimilation capacity of 31 wheat seedlings with respect to plant resistance to NH4+ stress and rhizosphere acidification (the model as shown in Figure 5). The growth of wheat seedling was seriously inhibited by AN, and this inhibitory effect was enhanced by soil acidification stress. Under AN, crops accumulated more NH4+ in tissues and more efficiently converted NH4+ into amino acids, and the N form accounted for the largest proportion of the variance in this process compared to the cultivar and pH level in root, but pH level had the strongest effect in shoot. To select and breed crop cultivars with a greater capacity to adapt to NH4+-N nutrition combined with soil acidification stress in the future, a higher capacity for N assimilation in both shoot and root and the ability to maintain high levels of C, must be a key focus.
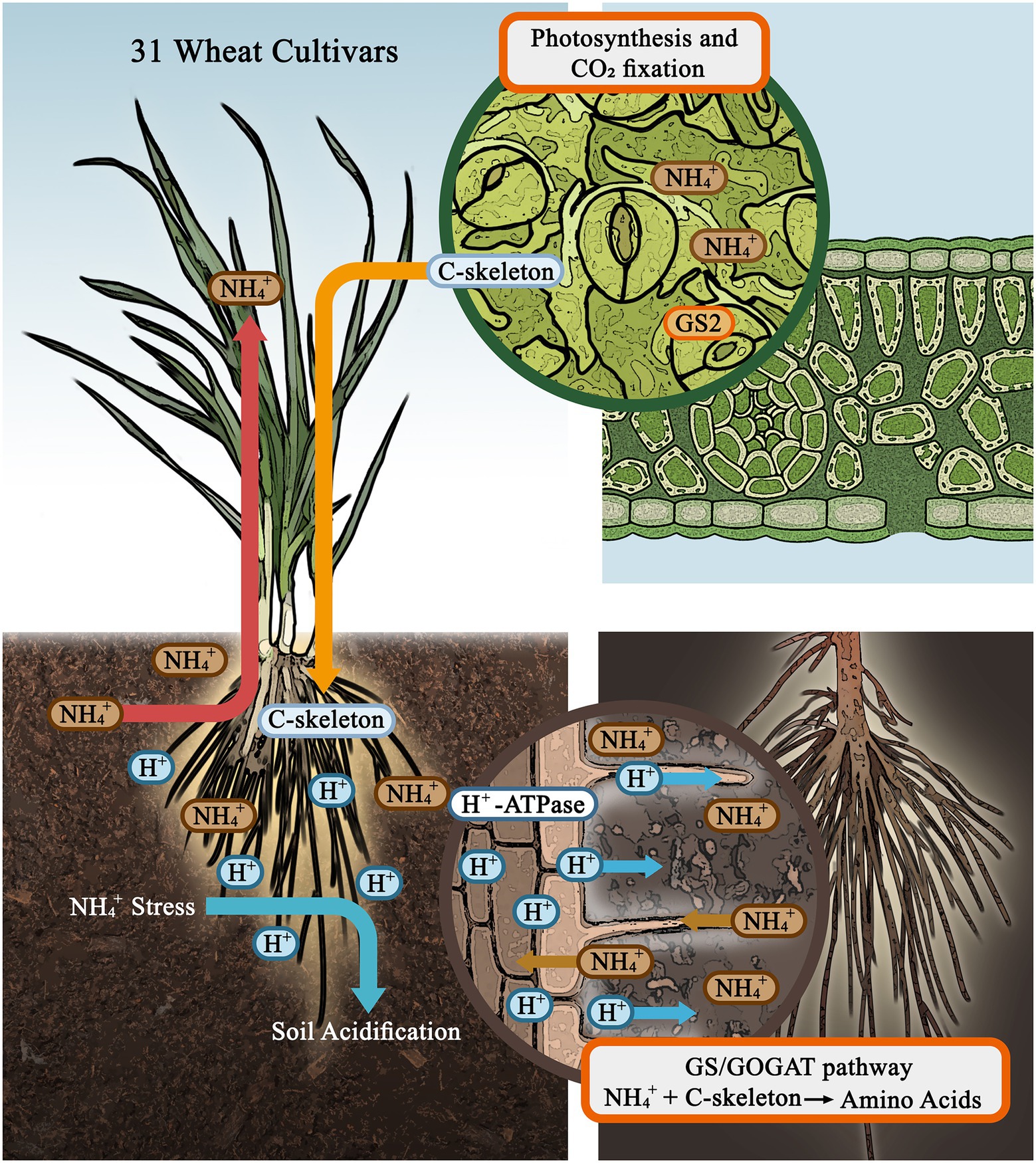
Figure 5. A proposed model of wheat physiological and biochemical responses to ammonium stress and soil acidification.
Data availability statement
The raw data supporting the conclusions of this article will be made available by the authors, without undue reservation.
Author contributions
FW: experiments implementation, writing–original draft, resources, data curation, methodology, formal analysis, and visualization. JM: supervision and funding acquisition. QW, QY, JYe, JG, and HL: writing–original draft, data curation, methodology, formal analysis, and visualization. JYo and XH: conceptualization and writing–review and editing. YY, XL, and HK: investigation. All authors contributed to the article and approved the submitted version.
Funding
We acknowledge generous financial support from the “Zhejiang Provincial Key Research and Development Program” (2020C02030 and 2021C02035); Project of leading talents of scientific and technological innovation in Zhejiang Province (2021R52045); Construction Project of Agricultural Green Development Pilot Support System of Huangyan District (HY202001); and Project of Unification of Water and Soil Science for One Healthy funded by the Zhejiang Academy of Agricultural Sciences.
Conflict of interest
The authors declare that the research was conducted in the absence of any commercial or financial relationships that could be construed as a potential conflict of interest.
Publisher’s note
All claims expressed in this article are solely those of the authors and do not necessarily represent those of their affiliated organizations, or those of the publisher, the editors and the reviewers. Any product that may be evaluated in this article, or claim that may be made by its manufacturer, is not guaranteed or endorsed by the publisher.
Supplementary material
The Supplementary material for this article can be found online at: https://www.frontiersin.org/articles/10.3389/fpls.2022.968707/full#supplementary-material
References
Ariz, I., Artola, E., Cabrera, A. A., Cruchaga, S., Maria, A. P., and Fernando, M. J. (2011). High irradiance increases NH4+ tolerance in Pisum sativum: higher carbon and energy availability improve ion balance but not N assimilation. J. Plant Physiol. 168, 1009–1015. doi: 10.1016/j.jplph.2010.11.022
Ariz, I., Asensio, A. C., Zamarreno, A. M., Garcia-Mina, J. M., Aparicio-Tejo, P. M., and Moran, J. F. (2013). Changes in the C/N balance caused by increasing external ammonium concentrations are driven by carbon and energy availabilities during ammonium nutrition in pea plants: the key roles of asparagine synthetase and anaplerotic enzymes. Physiol. Plant. 148, 522–537. doi: 10.1111/j.1399-3054.2012.01712.x
Ariz, I., Esteban, R., Ignacio, G. J., Maria, B. J., Maria, A. P., and Fernando, M. J. (2010). High irradiance induces photoprotective mechanisms and a positive effect on NH4+ stress in Pisum sativum L. J. Plant Physiol. 167, 1038–1045. doi: 10.1016/j.jplph.2010.02.014
Balkos, K. D., Britto, D. T., and Kronzucker, H. J. (2010). Optimization of ammonium acquisition and metabolism by potassium in rice (Oryza sativa L. cv. IR-72). Plant Cell Environ. 33, 23–34. doi: 10.1111/j.1365-3040.2009.02046.x
Bernard, S. M., and Habash, D. Z. (2009). The importance of cytosolic glutamine synthetase in nitrogen assimilation and recycling. New Phytol. 182, 608–620. doi: 10.1111/j.1469-8137.2009.02823.x
Bernard, S. M., Moller, A. L. B., Dionisio, G., Kichey, T., Jahn, T. P., Dubois, F., et al. (2008). Gene expression, cellular localisation and function of glutamine synthetase isozymes in wheat (Triticum aestivum L.). Plant Mol. Biol. 67, 89–105. doi: 10.1007/s11103-008-9303-y
Britto, D. T., and Kronzucker, H. J. (2002). NH4+ toxicity in higher plants: a critical review. J. Plant Physiol. 159, 567–584. doi: 10.1078/0176-1617-0774
Britto, D. T., and Kronzucker, H. J. (2013). Ecological significance and complexity of N-source preference in plants. Ann. Bot. 112, 957–963. doi: 10.1093/aob/mct157
Brix, H., Dyhr-Jensen, K., and Lorenzen, B. (2002). Root-zone acidity and nitrogen source affects Typha latifolia L. growth and uptake kinetics of ammonium and nitrate. J. Exp. Bot. 53, 2441–2450. doi: 10.1093/jxb/erf106
Cao, T., Xie, P., Ni, L., Zhang, M., and Xu, J. (2009). Carbon and nitrogen metabolism of an eutrophication tolerative macrophyte, Potamogeton crispus, under NH4+ stress and low light availability. Environ. Exp. Bot. 66, 74–78. doi: 10.1016/j.envexpbot.2008.10.004
Chen, X., Cui, Z., Fan, M., Vitousek, P., Zhao, M., Ma, W., et al. (2014). Producing more grain with lower environmental costs. Nature 514, 486–489. doi: 10.1038/nature13609
Coskun, D., Britto, D. T., Shi, W., and Kronzucker, H. J. (2017). Nitrogen transformations in modern agriculture and the role of biological nitrification inhibition. Nat. Plants 3:17074. doi: 10.1038/nplants.2017.74
Cramer, M., and Lewis, O. (1993). The influence of nitrate and ammonium nutrition on the growth of wheat (Triticum aestivum) and maize (Zea mays) plants. Ann. Bot. 72, 359–365. doi: 10.1006/anbo.1993.1119
Cruz, C., Bio, A. F. M., Dominguez-Valdivia, M. D., Aparicio-Tejo, P. M., Lamsfus, C., and Martins-Loucao, M. A. (2006). How does glutamine synthetase activity determine plant tolerance to ammonium? Planta 223, 1068–1080. doi: 10.1007/s00425-005-0155-2
Ding, W., He, P., Zhang, J. J., Liu, Y., Xu, X., Ullah, S., et al. (2020). Optimizing rates and sources of nutrient input to mitigate nitrogen, phosphorus, and carbon losses from rice paddies. J. Clean. Prod. 256:120603. doi: 10.1016/j.jclepro.2020.120603
El Omari, R., Rueda-López, M., Avila, C., Crespillo, R., Nhiri, M., and Cánovas, F. M. (2010). Ammonium tolerance and the regulation of two cytosolic glutamine synthetases in the roots of sorghum. Funct. Plant Biol. 37, 55–63. doi: 10.1071/FP09162
Gao, J., Wang, F., Sun, J., Tian, Z., Hu, H., Jiang, S., et al. (2018). Enhanced Rubisco activation associated with maintenance of electron transport alleviates inhibition of photosynthesis under low nitrogen conditions in winter wheat seedlings. J. Exp. Bot. 69, 5477–5488. doi: 10.1093/jxb/ery315
Gong, P., Liang, L., and Zhang, Q. (2011). China must reduce fertilizer use too. Nature 473, 284–285. doi: 10.1038/473284e
Guo, S., Chen, G., Zhou, Y., and Shen, Q. (2007). Ammonium nutrition increases photosynthesis rate under water stress at early development stage of rice (Oryza sativa L.). Plant Soil 296, 115–124. doi: 10.1007/s11104-007-9302-9
Guo, J. H., Liu, X. J., Zhang, Y., Shen, J. L., Han, W. X., Zhang, W. F., et al. (2010). Significant acidification in major Chinese croplands. Science 327, 1008–1010. doi: 10.1126/science.1182570
Li, B., Li, G., Kronzucker, H. J., Baluška, F., and Shi, W. (2013). Ammonium stress in Arabidopsis: signaling, genetic loci, and physiological targets. Trends Plant Sci. 19, 107–114. doi: 10.1016/j.tplants.2013.09.004
Li, Q., Li, B.-H., Kronzucker, H. J., and Shi, W.-M. (2010). Root growth inhibition by NH4+ in Arabidopsis is mediated by the root tip and is linked to NH4+ efflux and GMPase activity. Plant Cell Environ. 33, 1529–1542. doi: 10.1111/j.1365-3040.2010.02162.x
Li, B., Li, Q., Su, Y., Chen, H., Xiong, L., Mi, G., et al. (2011). Shoot-supplied ammonium targets the root auxin influx carrier AUX1 and inhibits lateral root emergence in Arabidopsis. Plant Cell Environ. 34, 933–946. doi: 10.1111/j.1365-3040.2011.02295.x
Liu, Y., Lai, N., Gao, K., Chen, F., Yuan, L., Mi, G., et al. (2013). Ammonium inhibits primary root growth by reducing the length of meristem and elongation zone and decreasing elemental expansion rate in the root apex in arabidopsis thaliana. PLOS ONE 8:e61031. doi: 10.1371/journal.pone.0061031
Lothier, J., Gaufichon, L., Sormani, R., Lemaître, T., Azzopardi, M., Morin, H., et al. (2011). The cytosolic glutamine synthetase GLN1; 2 plays a role in the control of plant growth and ammonium homeostasis in Arabidopsis rosettes when nitrate supply is not limiting. J. Exp. Bot. 62, 1375–1390. doi: 10.1093/jxb/erq299
Masclaux-Daubresse, C., Daniel-Vedele, F., Dechorgnat, J., Chardon, F., Gaufichon, L., and Suzuki, A. (2010). Nitrogen uptake, assimilation and remobilization in plants: challenges for sustainable and productive agriculture. Ann. Bot. 105, 1141–1157. doi: 10.1093/aob/mcq028
Miller, A. J., and Cramer, M. D. (2005). Root nitrogen acquisition and assimilation. Plant Soil 274, 1–36. doi: 10.1007/s11104-004-0965-1
Moore, S., and Stein, W. H. (1954). A modified ninhydrin reagent for the photometric determination of amino acids and related compounds. J. Biol. Chem. 211:907e913. doi: 10.1016/S0021-9258(18)71178-2
Ren, K., Xu, M., Li, R., Zheng, L., Liu, S., Reis, S., et al. (2021). Optimizing nitrogen fertilizer use for more grain and less pollution. J. Clean. Prod. 360:132180. doi: 10.21203/rs.3.rs-361734/v1
Setien, I., Fuertes-Mendizabal, T., Gonzalez, A., Ma Aparicio-Tejo, P., Gonzalez-Murua, C., Begona Gonzalez-Moro, M., et al. (2013). High irradiance improves ammonium tolerance in wheat plants by increasing N assimilation. J. Plant Physiol. 170, 758–771. doi: 10.1016/j.jplph.2012.12.015
Simon, B., and Sengupta-Gopalan, C. (2010). The 3’untranslated region of the two cytosolic glutamine synthetase (GS1) genes in alfalfa (Medicago sativa) regulates transcript stability in response to glutamine. Planta 232, 1151–1162. doi: 10.1007/s00425-010-1247-1
Singh, K. K., and Ghosh, S. (2013). Regulation of glutamine synthetase isoforms in two differentially drought-tolerant rice (Oryza sativa L.) cultivars under water deficit conditions. Plant Cell Rep. 32, 183–193. doi: 10.1007/s00299-012-1353-6
Van Den Berg, L. J., Dorland, E., Vergeer, P., Hart, M. A., Bobbink, R., and Roelofs, J. G. (2005). Decline of acid-sensitive plant species in heathland can be attributed to ammonium toxicity in combination with low pH. New Phytol. 166, 551–564. doi: 10.1111/j.1469-8137.2005.01338.x
Wang, F., Gao, J., Shi, S., He, X., and Dai, T. (2019). Impaired electron transfer accounts for the photosynthesis inhibition in wheat seedlings (Triticum aestivum L.) subjected to ammonium stress. Physiol. Plant. 167, 159–172. doi: 10.1111/ppl.12878
Wang, F., Gao, J., Tian, Z., Liu, Y., Abid, M., Jiang, D., et al. (2016). Adaptation to rhizosphere acidification is a necessary prerequisite for wheat (Triticum aestivum L.) seedling resistance to ammonium stress. Plant Physiol. Biochem. 108, 447–455. doi: 10.1016/j.plaphy.2016.08.011
Wang, F., Gao, J., Yong, J., Liu, Y., Cao, D., and He, X. (2020). Glutamate over-accumulation may serve as an endogenous indicator of tricarboxylic acid (TCA) cycle suppression under NH4+ nutrition in wheat (Triticum aestivum L.) seedlings. Environ. Exp. Bot. 177:104130. doi: 10.1016/j.envexpbot.2020.104130
Xu, G., Fan, X., and Miller, A. J. (2012). Plant nitrogen assimilation and use efficiency. Annu. Rev. Plant Biol. 63, 153–182. doi: 10.1146/annurev-arplant-042811-105532
Zhang, F. S., Chen, X. P., and Vitousek, P. (2013). Chinese agriculture: an experiment for the world. Nature 497, 33–35. doi: 10.1038/497033a
Zhang, W., and Wang, S. (2012). Effects of NH4+ and NO3− on litter and soil organic carbon decomposition in a Chinese fir plantation forest in South China. Soil Biol. Biochem. 47, 116–122. doi: 10.1016/j.soilbio.2011.12.004
Zhu, Y., Di, T., Xu, G., Chen, X., Zeng, H., Yan, F., et al. (2009). Adaptation of plasma membrane H+-ATPase of rice roots to low pH as related to ammonium nutrition. Plant Cell Environ. 32, 1428–1440. doi: 10.1111/j.1365-3040.2009.02009.x
Keywords: soil acidification, ammonium stress, nitrogen assimilation, wheat growth, natural variation
Citation: Wang F, Wang Q, Yu Q, Ye J, Gao J, Liu H, Yong JWH, Yu Y, Liu X, Kong H, He X and Ma J (2022) Is the NH4+-induced growth inhibition caused by the NH4+ form of the nitrogen source or by soil acidification? Front. Plant Sci. 13:968707. doi: 10.3389/fpls.2022.968707
Edited by:
Mohsin Tanveer, University of Tasmania, AustraliaReviewed by:
Peng Yan, Institute of Crop Sciences (CAAS), ChinaYefeng Jiang, Zhejiang University, China
Copyright © 2022 Wang, Wang, Yu, Ye, Gao, Liu, Yong, Yu, Liu, Kong, He and Ma. This is an open-access article distributed under the terms of the Creative Commons Attribution License (CC BY). The use, distribution or reproduction in other forums is permitted, provided the original author(s) and the copyright owner(s) are credited and that the original publication in this journal is cited, in accordance with accepted academic practice. No use, distribution or reproduction is permitted which does not comply with these terms.
*Correspondence: Junwei Ma, bWFqd0B6YWFzLmFjLmNu