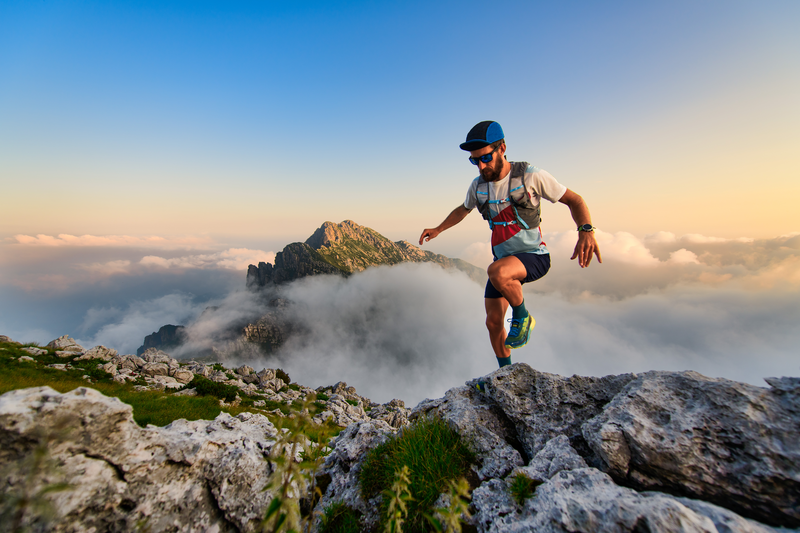
95% of researchers rate our articles as excellent or good
Learn more about the work of our research integrity team to safeguard the quality of each article we publish.
Find out more
ORIGINAL RESEARCH article
Front. Plant Sci. , 08 August 2022
Sec. Plant Pathogen Interactions
Volume 13 - 2022 | https://doi.org/10.3389/fpls.2022.966929
Extensive use of chemical control agents and fungicides typically leads to numerous risks to human health and the environment. Using plant extracts as natural substances represents a dual key for the environment and sustainable food production, as it reduces the input of synthetic pesticides into the environment and/or controls plant pathogens. For the first time, a Plantago lagopus ethanolic extract has been characterized and evaluated for its protective and curative effects against Rhizoctonia solani in tomato plants. The results showed that P. lagopus extract (10 μg/ml) completely inhibited R. solani mycelial growth in vitro. At 20 days of post fungal inoculation, the results demonstrated that using P. lagopus extract (100 μg/ml) in vivo enhanced tomato plant growth by significantly increasing shoot and root parameters in protective and curative treatments. Furthermore, the protective and curative treatments significantly reduced the disease index by 18.66 and 38.66%, respectively. Induction of systemic resistance with upregulation of PR-1 and PR-2 and a significant increase in the transcriptional levels of PR-3 and CHS in all P. lagopus extract-treated tomato plants were reported compared to untreated plants. HPLC analysis showed that the most common polyphenolic components detected in P. lagopus extract were rutin (74206.3 mg/kg), naringenin (2388.74 mg/kg), quercetin (1249.13 mg/kg), and p-hydroxybenzoic acid (1035.87 mg/kg). In addition, the ellagic acid (798.47 mg/kg), vanillic acid (752.55 mg/kg), catechol (648.89 mg/kg), cinnamic acid (332.51 mg/kg), ferulic acid (296.32 mg/kg), benzoic acid (295.95 mg/kg), and chlorogenic acid (116.63 mg/kg) were also reported. Our study is the first to show that P. lagopus extract can help plants fight off R. solani fungal infection. Furthermore, the findings imply that using the P. lagopus extract as a natural biocontrol agent could be a sustainable strategy to manage plant fungal diseases.
Tomatoes (Lycopersicon esculentum L.) are among the most remunerative and widely cultivated vegetables worldwide. It is in the family Solanaceae and has tryptophan and tomatin, which are very good for human health. Tomatin is a main component of the glycoalkaloid. Tomatoes are the first-ranked processed vegetable and rank second only to potatoes in the world growing area. According to FAOSTAT (2017), global tomato production is about 161.7 million metric tons with a value of $59 billion. Harmful microorganisms threaten crop production and environmental balance (Sabuquillo et al., 2006). Various pathogens (Rhizoctonia, Verticillium, Fusarium, and Pythium) are responsible for the soil-borne fungal diseases, damping-off, and wilt of tomato seedlings (Lucas et al., 1992; Kuprashvili, 1996). Rhizoctonia solani is a common soil-borne fungal pathogen that causes seedling damping-off and root rot in tomatoes (Gondal et al., 2019). Damping-off caused by Rhizoctonia solani is one of the most devastating tomato diseases managed by fungicides. Different symptoms are associated with damping-off, which causes the death of some seedlings in the population (Channa et al., 1995). Symptoms start with round spots on seedlings, then stem abrasions at the surface level of seedlings (Channa et al., 1995; Heflish et al., 2021). Because physiological pathogen races have become more resistant to fungicides and factors like fungicide residues and human health issues, it is hard to develop new ways to manage plant diseases.
The concept of biocontrol has sparked a significant technological, economic, and political discussion that seeks to promote environmentally sustainable agriculture at a lower cost to the environment. Accordingly, some nations have created a protection plan that minimizes pesticide use by approximately 50%. From this perspective, it appears vital to increase our understanding of biocontrol to improve its application and effectiveness. For all these reasons, research is making good progress toward a biological control point of view that could be combined with other methods to make a good plan for fighting plant diseases (Abo-Zaid et al., 2021). Many biocontrol agents are reported to fight R. solani pathogens such as Bacillus subtilis and Trichoderma species, which stop the pathogen’s growth or progress with different strategies like production of siderophores, cell lysis enzymes, or by direct intact (hyperparasitism; Wu et al., 2019; El-Benawy et al., 2020; Heflish et al., 2021). Also, various plant extracts are reported for their antifungal activity against R. solani, including a diverse array of bioactive chemicals that are well-known for their antimicrobial and antifungal activity without causing phytotoxic effects (Al-Askar and Rashad, 2010; Santas et al., 2010; Abd-El-Khair and El-Gamal Nadia, 2011; Hamza et al., 2016; Koka et al., 2017; Parveen et al., 2017). The main reasons for using plant extracts or essential oils as antifungal agents are that they come from nature, and pathogens are less likely to become resistant to them. Since plant products are easy to turn into common organic materials, they may have less of an effect on the physiological processes of plants and less of an impact on the environment than their synthetic alternatives (eco-friend; Nazzaro et al., 2017; Abdelkhalek et al., 2021).
Many plants and their extracts have been evaluated for their antimycotic activities. They are known to have good antifungal activities against plant pathogenic fungi (Shuping and Eloff, 2017), such as Plantago plants which have been used as anti-inflammatory and asthmatic medications in Asia and Europe (Nishibe et al., 1995). Plantago lanceolata L. extract was used as an antifungal against fungi such as Alternaria alternata, Mucor piriformis, and Penicillium expansum (Parveen et al., 2014). Also, Plantago extract was used as an inhibitor for spore germination of the Colletotrichum gloeosporioides Penz, which is the main causal agent of blister spot disease in coffee trees (Silva et al., 2008). Moreover, (Klironomos, 2003) postulated that P. lanceolata protects the treated plants against fungal infection. Four iridoid glucosides, i.e., plantamajoside, luteolin-7-O-monoglucoside, chlorogenic acid, and rosmarinic acid, were isolated from the aerial parts of P. lagopus (Velázquez-Fiz et al., 2000). Moreover, p-hydroxybenzoic, chlorogenic, gallic, and vanillic acids or apigenin, luteolin, and luteolin-7-O-glucoside were common compounds between P. altissima and P. lanceolata extracts (Beara et al., 2012).
In addition, the plant extracts contain various chemicals, including plant hormones, minerals, antioxidants, and osmoprotectants, which effectively boost the defense mechanisms (antioxidant enzymes) of plants against environmental challenges and play a crucial role in promoting plant growth (Desoky et al., 2019). These extracts possess protective enzymes and activate pathogenesis-related proteins, thereby inhibiting the progression of the disease. It was reported that these extracts might stop the spread of disease by activating the body’s defenses and causing systemic resistance (Prasannath, 2017). Recognizing the importance of screening and identifying new plant extracts with strong antifungal activities for agricultural applications, we hypothesized that P. lagopus extract can fight off the R. solani pathogen in vitro, improve tomato growth parameters in vivo, and could induce resistance against Rhizoctonia root rot disease. To test this hypothesis, we evaluated the growth inhibitory activity of P. lagopus ethanolic extract against R. solani, its effects on growth parameters, total chlorophyll, phenolic content, and defense-related gene expression levels, including PR-1, PR-2, PR-3, and CHS in tomato plants. Also, use HPLC to figure out the P. lagopus extract’s phenolic capacity and its main phytochemical components.
Phenolic standards, namely, catechin, catechol, syringic acid, chlorogenic acid, cinnamic acid, ellagic acid, kaempferol, ferulic acid, gallic acid, rutin, caffeic acid, naringenin, benzoic acid, o-coumaric acid, p-hydroxybenzoic acid, pyrogallol, p-coumaric acid, quercetin, quinol, rosmarinic acid, and vanillic acid were bought from Merck KGaA (Darmstadt, Germany). The purities of standards were up to 99%. The solvents used were of analytical grade, dimethylsulfoxide (DMSO; Alfa Aesar GmbH & Co KG, Massachusetts, United States), orthophosphoric acid (H3PO4), ethanol, methanol, and acetonitrile HPLC-grade (Fisher Scientific International, Inc., Hampton, New Hampshire, United States).
Tomato samples with root rot disease-like symptoms were gathered from El-Beheira Governorate, Egypt. The roots of the symptomatic plants were cut and washed with running tap water, then cleaned and sterilized several times before drying in laminar flow. PDA media were used in the isolation and cultivation process. The fungus purification was performed with the hyphal tip procedure (Dhingra and Sinclair, 2017). According to the previous descriptions (Alexopoulos et al., 1996), the fungus was identified based on morphological and microscopic characteristics. Moreover, molecular identification processes were performed using ITS1 and ITS4 primers (Table 1). PCR reaction conditions were done as previously described (Heflish et al., 2021). The PCR product was electrophoresed on agarose gel, purified, and subjected to sequencing. The obtained nucleotide sequences were aligned using MEGA X software and compared to other related organisms using NCBI-BLAST. After that, the fungus sequence was subjected to the NCBI-GenBank submission portal to obtain the accession number.
Plantago lagopus plants, gathered from the northwest of Egypt, were left to dry at room temperature (25°C) for a week and crushed to a fine powder using a grinder mill (Moulinex AR1044, France). Approximately 100 g of the dried powder was left in 200 ml of 95% ethanol for 4 days (Abdelkhalek et al., 2021). The alcoholic mixture was filtered through Whatman filter paper No. 1, and the obtained extract was evaporated and concentrated by a rotary evaporator until all the ethanol was removed. The P. lagopus extract was reserved in a brown bottle at 4°C until use. In the in vitro experiment, the extract was diluted with DMSO to obtain different concentrations (μg/mL). In the in vivo experiment, the extract was prepared in 0.1% Tween® 80 (w/v) to get a 100 μg/ml concentration.
The free radical scavenging activity was measured according to previously described methods (Shimada et al., 1992). A 3.94 mg of DPPH (1,1-diphenyl-2-picrylhydrazyl) was diluted to 0.1 ml in 100 ml of methanol. A 1 ml of diluted DPPH was mixed with 3 ml of each sample with different concentrations (250, 125, 62.5, 31.25, and 15.62 μg/ml). The mixture was well mixed by vigorous shaking and stored at 25°C for 30 min. Each combination’s absorbance value (AV) was spotted at 517 nm. , where As is the sample AV and Ao is the control AV reaction (contains all reagents except for the sample).
The polyphenolic components of ethanolic P. lagopus plant extract were determined using HPLC-Agilent. HPLC conditions and program properties are illustrated in Table 1.
Quantification was performed by the standard external method. The standard stock solutions (1–25 mg/l). A quantitative report is generated by combining known data from a calibration standard and unknown data from the sample. The UV detector can quantify a minimum concentration of 0.1 g/ml.
Using the food poisoning technique, P. lagopus extract was tested for its efficiency against the root rot pathogen (Kumar et al., 2008). Different concentrations of P. lagopus extract were prepared in final (2, 4, 6, 8, and 10) μg/mL by emerging in PDA plates (90 mm diameter) compared with fungicide (Fluconazole 2.5 μg/ml), and the negative control (PDA plates without any additives). Fungus discs were cut from the periphery of 6 days old cultures, inoculated in the center of the treated poured Petri dishes, and then incubated at 25°C for a week. Three replicates were used for each treatment. The efficacy of P. lagopus extract on the fungus linear growth was measured. The growth (Dissanayake, 2014), where C0 represents the length of the fungus growth on the control PDA plates and Tx represents the fungus growth in the plant extract treatment.
The ability of P. lagopus extract to reduce R. solani and promote tomato growth was tested in a pot trial under greenhouse climate conditions (28 ± 2°C; 75 ± 5%; 14 h light/10 h dark). Each pot (20 13.2 12.2 cm) was filled with sterilized soil and planted with 4-week-old tomato seedlings of the Peto 86 variety. Five days after transplanting, the plant extract of Plantago was used with a 100 μg/ml concentration and added to the potting soil (20 ml/pot). R. solani inoculum was prepared by inoculating pre-sterilized autoclaved moistened barley grains (500 g) with 2 plugs of R. solani (0.5 cm in diameter) and incubating for 1 week at 25 ± 2°C. By the end of incubation, the barley inoculum was air-dried, milled to a fine powder, and applied to the pots (5 g inoculum/kg) where the inoculum was introduced close to the root pan and crown of the plant was going to be placed (Montealegre et al., 2010). The treatments were distributed in five replicates. (1). a protective treatment in which plant extract was added 2 days before R. solani inoculation, (2). a curative treatment in which R. solani was inoculated 2 days before plant extract application, (3). a Rhizoctonia treatment in which tomato plants were only inoculated with R. solani, and (4). a plant extract treatment in which tomato plants were only treated with P. lagopus extract. The last treatment was the control treatment, in which tomato plants were inoculated with PDA-free microorganisms. The plants were carefully uprooted after 1 month of transplanting for root rot severity screening according to a 0–5 scale (Abdeljalil et al., 2016). The data were calculated as disease index percentage
The uprooted tomato plants were also used to measure the effectiveness of the P. lagopus extract on different tomato growth parameters, such as plant height (cm), root length (cm), shoot fresh and dry weight (g), root fresh and dry weight (g), and the total chlorophyll content (SPAD value). Furthermore, tomato leaf samples were collected from each treatment to study the activity of the defense-related genes and total phenolic components in response to different treatments under pot trials. Tomato leaves were collected 20 days of post-inoculation (dpi) with R. solani.
Folin–Ciocalteu (FC) method was used to measure the TPC values of tomato extracts. Tomato extract (100 μl, 1 mg/ml) was combined with 750 μl of FC (diluted to 1:10 in water). The mixture was left at 25°C for 5 min, then 750 μl of sodium carbonate was added to the mix and gently shaken. After 90 min, the absorbance value of the blend was measured at 725 nm. A calibration curve was created using a standard reference (a gallic acid concentration range of 0.01 to 0.05 mg/ml). As described previously (Velioglu et al., 1998), TPC was determined as gallic acid equivalents (μg GAE/100 g extract).
Plant total RNA was manually extracted from about 100 mg of tomato leaves collected at 20 dpi using a modified guanidium isothiocyanate (GITC) method (Abdelkhalek and Sanan-Mishra, 2019). SPECTROstar Nano was used to determine the purity and concentration of extracted RNA (BMG Labtech, Ortenberg, Germany). As previously described (AbdEl-Rahim et al., 2010; Abdelkhalek et al., 2019), the isolated RNA was utilized to generate cDNA using a reverse transcriptase (RT) enzyme. In an Eppendorf cycler (Hamburg, Germany), the RT reaction was conducted at 42°C for 1 h and then deactivated at 80°C for 5 min. The RT-PCR product was stored at −20°C until employed as a qRT-PCR template.
The expression levels of three tomato pathogenesis-related genes (PR-1, PR-2, and PR-3) and one polyphenolic gene (CHS) were analyzed using the qPCR and normalized to the reference β-actin gene. Primer nucleotide sequences used in this investigation are listed in Table 2. For each biological sample, the experiments were repeated three times. As previously described (Abdelkhalek et al., 2018), qRT-PCR was carried out using a QIAGEN Rotor-Gene 6,000 (ABI System, United States) with Thermo SYBR Green Mix (Foster, CA, USA). The relative expression level of the tested gene was calculated according to the 2−ΔΔCT method (Livak and Schmittgen, 2001).
The data were analyzed using CoStat software, and significant differences were estimated using Tukey’s honest significant differences technique (H.S.D.) at a p ≤ 0.05, with standard deviation (SD) presented as a column bar or values. Upregulation of a gene means that the relative expression levels are greater than 1, whereas downregulation means values less than 1.
Following accepted taxonomic phenotypic standards, the morphological study of the isolated Rhizoctonia isolate from tomato plant roots was compatible with Rhizoctonia genus characteristics. The amplified ITS fragment partial sequence was obtained, then submitted to GenBank, and was confirmed to be Rhizoctonia solani strain Rs34 and assigned the accession number MW664425. The alignment of the received sequence of R. solani Rs34 with the other sequences downloaded from the GenBank database indicated that the genetic homogeneity nearest to 99% of the ITS partial sequence was with R. solani (MT408040, MT108198, and MZ754369) and isolates of Rhizoctonia sp. (MK084681 and MN106353) Figure 1.
Figure 1. The phylogenetic cladogram shows the relationship of the Rhizoctonia solani Rs34 among closely related R. solani sequences. The GenBank alignment was based on partial inter transcripted spacer region (ITS) sequences.
The growth reductions of R. solani in response to the tested plant extracts are presented in Table 3. Radial growth of R. solani decreased significantly with an increase in the concentration of plant extracts from 2 to 10 μg/ml. It was found from the results that the extract of Plantago lagopus caused 100% inhibition of R. solani mycelial growth at 10 μg/ml. At the same time, there was no effect on R. solani mycelial growth at the dose of 2 μg/ml compared with antifungal fungicide (Fluconazole, 2.5 μg/ml).
Table 3. In vitro growth inhibition (%) of Rhizoctonia solani in response to Plantago lagopus extract.
The extract of P. lagopus was tested for its efficacy on R. solani under greenhouse growth conditions. The disease index (DI%) was estimated according to the root browning symptoms and their extent levels (using a 0–5 scale) based on each treatment (Figure 2). The plant extract substantially reduced the DI% compared to the untreated control. P. lagopus extract application before inoculation with R. solani (protective treatment) showed a significant reduction of the percentage of disease index (18.66%), followed by the curative treatment (38.66%; Figure 2). DI% was 86.66% in the Rhizoctonia treatment and 0.0% in the control and plant extract treatments.
Figure 2. Effect of Plantago lagopus extract on disease index (DI%) of tomato root rot caused by Rhizoctonia solani under greenhouse conditions. The different letters (a–d) represent significant differences.
In the greenhouse experiment, both protective and curative treatments of P. lagopus extract showed a significant enhancement (p ≤ 0.05) in the growth of tomato plants (Table 4). Moreover, the influence on the height was substantial due to the plant extract treatment. It was recorded that plant height was 36.60 cm in plant extract treatment, followed by protective treatment, which recorded 36.20 cm. Compared to Rhizoctonia treatment (5.2 cm), the plant extract and protective and curative treatments significantly increased root length by 21.30, 21.10, and 20.70 cm, respectively (Table 4). The plant extract, protective, and curative treatments increased shoot-fresh weight (14.12, 13.78, and 12.24 g, respectively) and root-fresh weight (5.96, 5.70, and 5.32 g, respectively) more than the Rhizoctonia or control treatments. In addition, non-significant results in all treatments were noticed for the dry shoot weights compared to control. The root dry weight of tomato plants changed after being treated with the plant extract, protective, and curative treatments compared with control (Table 4). The plant extract treatment was more effective in increasing chlorophyll content (38.88, SPAD value), followed by the protective and curative treatments, with significant SPAD values of 36.58 and 34.46, respectively, compared with the control (37.22) and Rhizoctonia treatment (27.64) Figure 3.
Figure 3. Effect of all treatments on total chlorophyll content (SPAD value) in tomato plants as affected by root rot disease under greenhouse conditions. The different letters (a–e) represent significant differences.
At 20 dpi, the inhibitory effects and relative transcriptional levels of four defense-related genes (PR-1, PR-2, PR-3, and CHS) were assessed. The antifungal activity of P. lagopus against fungal infection was validated by qPCR data, which showed a considerable elevation of defense genes inside the treated plants (Figure 4). Compared to the controls, a significant upregulation of PR-1 was observed in all plant extract treatments, while non-treated plants showed a downregulation. The highest relative expression level (1.81-fold) was reported in plant extract treatment, followed by protective and curative treatments with expression levels of 1.39- and 1.26-fold, respectively. The expression level of PR-2 showed upregulation in all plants treated with plant extract (Figure 4). The most outstanding transcriptional level (2.39-fold) was reported in curative treatment, followed by protective treatment with a relative transcriptional level of 2.12-fold compared to the control. On the other hand, plants in Rhizoctonia treatment showed a downregulation with a relative transcriptional level of 0.74-fold compared to the control (Figure 4).
Figure 4. A histogram shows the relative expression levels of the four genes PR-1, PR-2, PR-3, and CHS at 20 dpi of plant extract treatments (100 μg/ml) in different treatments compared with control. The different letters (a–e) represent significant differences.
Regarding PR-3 expression, a significant upregulation was shown with all treatments compared to untreated plants (Figure 4). The highest expression level (2.85-fold) was observed with plant extract-only treatment, followed by protective, curative, and non-treated with relative transcriptional levels of 2.43-, 2.17-, and 1.49-fold, respectively. The PR-3 gene produces the chitinase enzyme, which hydrolyzes the chitin and prevents plants from fungi invasions (Abdelkhalek et al., 2021). The PR-3 gene was upregulated in tomato plants infected with Rhizoctonia or treated with P. lagopus or the curative or protective treatments in the current investigation. P. lagopus-treated plants had the highest relative expression. The CHS gene showed an upregulated expression level in all treatments compared to untreated plants (control). The expression level of curative treatment exhibited the greatest level (2.77-fold), followed by protective, plant extract, and non-treated plants treatments with 1.66-, 1.62-, and 1.42-fold higher expression levels than control, respectively (Figure 4).
The phenolic concentration in the protective treatment was about 10-fold higher than in control, while in P. lagopus extract or R. solani treatments, it was 5.97- and 5.82-fold higher (Table 5). Additionally, in the curative treatment, the phenolic concentration was lower than those found in all treatments except the control (0.039 μg GAE/100 g) but still higher, by about 4.1-fold, than control plants.
The DPPH method was used to determine the antioxidant potential of P. lagopus extract to act as a free radical scavenger. The antioxidant activity value of the extract was 80.23 μg/ml compared with the control ascorbic acid (5.04 μg/ml).
HPLC was used to identify the P. lagopus extract compounds. The identified chemical compounds are shown in Figure 5 and Table 6. The main compounds were determined as mg/kg: rutin (74206.3), naringenin (2388.74), quercetin (1249.13), p-hydroxybenzoic acid (1035.87), ellagic acid (798.47), vanillic acid (752.55), catechol (648.89), cinnamic acid (332.51), ferulic acid (296.32), benzoic acid (295.95), and chlorogenic acid (116.63).
Rhizoctonia solani is a widespread fungus disease in agricultural soils and a major factor impacting the germination of many plant seedlings causing damping-off symptoms (El-Kazzaz et al., 2022). Chemical fungicides are routinely and effectively used to control Rhizoctonia root-rot. However, their application in the field might not always be desired. Considering the disadvantages of chemical control of plant diseases, using plant extracts to combat plant diseases is gaining prominence (Abdelkhalek et al., 2020b). Many plant materials, like plant extracts, essential oils, gum, resins, etc., have shown biological activity in vitro and in vivo and are used as biofungicides. In the current study, the antifungal activities of the ethanolic extract of P. lagopus against R. solani were evaluated on tomato plants under greenhouse conditions for the first time. Moreover, its effect on the tomato growth parameters, chlorophyll content, phenolic content, and defense-related gene expression was also assessed. Furthermore, the phytochemical components of the P. lagopus extract were identified using HPLC.
The morphological study of Rhizoctonia isolates from tomato plant roots was compatible with Rhizoctonia genus characteristics as it is an anamorphic mycelial septate fungus that did not produce asexual spores (Heflish et al., 2021). The ITS-PCR reaction confirmed the morphological identification to be R. solani strain Rs34. As stated before, pesticides, biological control, and azoles fungicides effectively reduce agricultural losses caused by fungal diseases (Hof, 2001; Singh et al., 2016). Our results revealed that the growth of R. solani was reduced significantly in response to the Plantago lagopus extract from 2 to 10 μg/ml compared to Fluconazole (positive control). While Fluconazole had the better results in our study, it is often used in commercial rapid antifungal susceptibility testing (Rex et al., 1993). It is one of the azole groups that had higher minimal inhibitory concentrations (MICs) against most Fusarium species (Al-Hatmi et al., 2015), and 90% of Candida albicans isolates had Fluconazole MICs of less than 1 μg/ml (Rex et al., 1995). In some conditions, Fluconazole has been proven ineffective against yeasts other than C. albicans (Rex et al., 1995).
The antifungal effect of the P. lagopus extract in vitro against R. solani in this study agreed with our recent research results. The ethanolic Coccoloba uvifera extract inhibited the development of R. solani, Botrytis cinerea, and Fusarium culmorum by 64.4, 100, and 38.5%, respectively (Ashmawy et al., 2020b). Also, the results are consistent with those documented by different authors (Deena and Thoppil, 2000; Valarini et al., 2003). They discovered that extracts of lantana, garlic, eucalyptus, and lemongrass have an antifungal effect on soil fungi mycelial growth. Besides, the n-hexane extract of Eucalyptus camaldulensis demonstrated the same high antifungal property against F. culmorum and R. solani, especially at 3% (Salem et al., 2019). Similarly, wood samples treated with Acacia saligna water extract inhibited the growth of F. culmorum and R. solani mycelium (Al-Huqail et al., 2019). Meanwhile, Plantago spp. such as P. major had antifungal action against all phytopathogenic fungi examined with 2000 μg/ml extract. The highest growth inhibition (32.2%) was observed against Phytophthora cinnamomi, followed by Colletotrichum gloeosporioides (25.7%), C. godetiae, and C. nymphaeae (21.1%; Ferreira and Oliveira, 2020). The ethyl acetate fraction of Plantago sp. was shown to be the most potent in vitro against Staphylococcus aureus and Pseudomonas aeruginosa bacteria (Karima et al., 2015), while Klebsiella pneumoniae, Proteus mirabilis, and Salmonella typhimurium had the lowest antibacterial activity (inhibition zone, 16.7 and 13.3 mm; Karima et al., 2015).
Under greenhouse growth conditions, the extract of P. lagopus was tested for its efficacy on R. solani and revealed a significant reduction in disease symptoms. The same results could be concluded from the study of Al-Askar and Rashad (Al-Askar and Rashad, 2010). They observed the efficacy of clove extract on pea root-rot disease incidence in a greenhouse experiment as the clove extract at 4% concentration, and a chemical fungicide showed a considerable improvement in the proportion of plants that survived (40 and 48%, respectively) and a significant decrease in disease incidence. Furthermore, our findings were consistent with a previous field experiment (Abd-El-Khair and El-Gamal Nadia, 2011). They reported that using chilli, lantana, and lemongrass extracts revealed an incidence reduction of R. solani damping-off disease. Even though some researchers who focused only on aqueous extracts found that these extracts had antifungal efficacy against specific fungi (Bhardwaj, 2012), other studies have compared the antimicrobial activities of alcoholic and aqueous extracts and noticed that the alcoholic is better than aqueous extracts (Ambikapathy et al., 2011; Ashraf et al., 2011; Behbahani et al., 2013; Jat and Agalave, 2013; Moorthy et al., 2013). In the greenhouse experiment, both protective and curative treatments of P. lagopus extract showed a significant enhancement (p ≤ 0.05) in the growth of tomato plants. Several studies had the same effective results that Moringa oleifera leaf extract increased tomato growth and yield (Culver et al., 2012), common bean growth and yield (Mvumi et al., 2013), and some Eruca vesicaria growth parameters and photosynthesis rate (Abdalla, 2014). Blueberry fruits, red grape, and hawthorn leaf extracts have improved maize growth, total chlorophyll content, and roots biomass (Ertani et al., 2016). Also, the secondary metabolites, including a wide range of compounds such as flavonoids, terpenoids, alkaloids, and phenolics, have bioactivity as stimulants or inhibitors of plant growth, as reported by many authors (James and Dubery, 2011; Singh et al., 2012; Biradar and Rachetti, 2013).
The relative transcriptional levels of four defense-related genes (PR-1, PR-2, PR-3, and CHS) were assessed at 20 dpi, which showed a considerable regulation of the four defense genes inside the treated plants. PR-1 is a crucial regulator of SAR and may serve as a marker for early plant defense responses (Hoegen et al., 2002), and its importance in plant immunity has been recognized for over two decades. The accumulation and expression of PR-1, a SA marker gene, are linked to the activation of SA in response to pathogens (D’Maris Amick Dempsey et al., 2011; Abo-Zaid et al., 2021). Compared to the controls, a significant upregulation of PR-1 was observed in all plant extract treatments, while non-treated plants showed a downregulation. This is consistent with previous findings of many authors (Su et al., 2013; Chandrasekaran and Chun, 2016), indicating that increased PR expression aids resistance against hemibiotrophic and necrotrophic pathogen infections. As a result, it is possible that the P. lagopus can modify the plant’s response, increase resistance, and prevent R. solani from suppressing defense genes. As a result of sterol extraction from fungal cell membranes, sterol-binding PR1 proteins limit fungal cell proliferation (Schneiter and Di Pietro, 2013), while PR2-1,3-glucanases, combined with PR-3-chitinases, lysis the fungus wall (Balasubramanian et al., 2012). The transcriptional levels of PR-2 were upregulated in the protective and curative treatments and downregulated in Rhizoctonia treatment compared to the control. Several reports have confirmed that PR-2 proteins are engaged in various physiological plant defense processes primarily induced by SAR inducers like SA (Abdelkhalek, 2019). The fungal secondary metabolites may have boosted PR-2 activity in P. lagopus extract-treated plants (Druzhinina et al., 2011). This conclusion aligns with recent research that shows microbial elicitors enhance PR-2 mRNA in plants (Roylawar et al., 2015). Increased PR-2 activity in the cell wall has been shown to enhance the number of oligosaccharides produced, which elicits plant defense systems.
The PR-3 gene was upregulated in tomato plants infected with Rhizoctonia or treated with P. lagopus or the curative or protective treatments in the current investigation. P. lagopus -treated plants had the highest relative expression. The PR-3 gene produces the chitinase enzyme, which hydrolyzes the chitin and prevents plants from fungi invasions (Abdelkhalek et al., 2021). The findings show that the PR-3 gene helps to strengthen plant resistance to fungal attacks. The application of P. lagopus increases the activation of multiple defensin genes, including PR-3, in leaf tissue, resulting in increased pathogen resistance (Heflish et al., 2021). The CHS gene showed an upregulated expression level in all treatments compared to control. CHS is a required precursor or first enzyme in plant flavonoids biosynthesis, converting p-coumaroyl CoA to naringenin chalcones (Abdelkhalek et al., 2020b). Surprisingly, P. lagopus extract, curative and protective treatments, resulted in the highest induction of CHS, which is necessary for flavonoid production (Marais et al., 2006; Abdelkhalek et al., 2021). In previous investigations, CHS overexpression was observed to produce large quantities of flavonoids with broad antifungal action against various plant pathogens (Martínez et al., 2017; Abdelkhalek et al., 2020a). As a result, treating tomato plants with P. lagopus as a protective or curative treatment may increase the quantity of flavonoid compounds. As a result, we believe that the P. lagopus extract contains elicitor metabolite chemicals that can activate SAR and boost plant resistance to fungal infection. Following pathogen exposure, the activation of defense-related genes has been described in numerous plant-pathogen interactions in the primed state (Ahn et al., 2007; Niu et al., 2011). This is also apparent in ISR against hemibiotrophic and necrotrophic diseases, as evidenced by Harpophora oryzae-primed defense genes in the rice-Magnaporthe oryzae interaction (Su et al., 2013) and B. subtilis-induced PR genes in tomato challenged with Pectobacterium carotovorum (Chandrasekaran and Chun, 2016). However, P. lagopus could be used to combat R. solani infections as a biocontrol agent. However, further research is needed for future field uses.
Plants treated with plant extract result in the buildup of phenolic components, linked to reactive metabolic defense against infectious pathogens. Various studies reported that fungal or bacterial pathogen infections caused a potent increase in total phenolic content (Martin et al., 2009; Petkovsek et al., 2011; Rusjan et al., 2012). Consequently, polyphenolic compounds that accumulate in plant extract-treated plants potentially act as proton donors, reducing oxidative injury to root cells during pathogen infections (Singh et al., 2011). Therefore, the increase in TPC as a plant response to the plant extract application in protective treatment alone would be associated with protection against plant pathogens in tomato plants. These differences in TPC are caused by the phenylpropanoid pathway regulation, as cited (Mikulic-Petkovsek et al., 2014). Our results coincided with numerous studies showing that Plantago species had antioxidant activity. Few studies showed high antioxidant activity for Plantago spp. (Çoban et al., 2003; Gálvez et al., 2005; Beara et al., 2009), and P. major (Samuelsen, 2000; Chiang et al., 2002). In addition, a study on the antioxidant activity of P. lanceolate in different extracts showed that ethanolic extracts had the highest DPPH free radical scavenging capacity (Miser-Salihoglu et al., 2013).
The identified chemical compounds in HPLC analysis revealed the existence of many polyphenolic compounds such as rutin, naringenin, quercetin, p-hydroxybenzoic acid, ellagic acid, vanillic acid, catechol, cinnamic acid, ferulic acid, benzoic acid, and chlorogenic acid. Many polyphenols have antimicrobial, antiviral, and antioxidant properties, including ferulic acid, quercetin, ellagic acid, chlorogenic acid, catechins, gallic acid, caffeic acid, and myricetin (Shaygannia et al., 2016; Mani et al., 2020). According to our findings, the polyphenolic compounds may act as elicitor molecules and play essential roles in SAR. P. lanceolata plants are rich in polyphenolic compounds such as syringic acid, cinnamic acid, resveratrol, caffeic acid, ferulic acid, rutin, and quercetin. In contrast, in P. major plants, more flavonoids, iridoid glycosides, triterpene acids, caffeic acid, chlorogenic acid, vanillic acid, and p-coumaric acid were found in their HPLC-UV analysis (Samuelsen, 2000). Our earlier research has revealed a broad spectrum of phenolic chemicals responsible for various medicinal plants’ antioxidant and antibacterial activities (Mohamed et al., 2020). However, P. lagopus could be used to combat R. solani infections as a biocontrol agent. However, further research is needed for future field uses.
In this study, the application of the P. lagopus extract (100 μg/ml) boosted tomato plant growth, inducing systemic resistance and decreasing root rot disease caused by R. solani fungus. Based on our findings, the reduction in disease incidence was seen in P. lagopus-treated plants compared to control plants, along with upregulation increases of PR-1 and PR-2 and a significant increase in the transcriptional levels of PR-3 and CHS genes at 20 dpi. P. lagopus extract-HPLC analysis indicated that the main compounds were rutin, naringenin, quercetin, p-hydroxybenzoic acid, ellagic acid, vanillic acid, catechol, cinnamic acid, ferulic acid, benzoic acid, and chlorogenic acid. According to our results, the protective treatment best reduces root rot disease. Furthermore, our study provides the basis for additional investigation of plant extract antifungal properties to limit the usage of fungicides, which pose many risks to human health and the environment.
The original contributions presented in the study are included in the article/supplementary material, further inquiries can be directed to the corresponding author.
SB, AA, MS, and AH designed the research and wrote the manuscript. AA, SS, MS, ME, and SB performed the experiments. AA-A, AB, FA, ME, and EH helped with editing and provided suggestions for the experiments. AA, AH, MS, and SB analyzed the data. All authors contributed to the article and approved the submitted version.
This research was financially supported by the Researchers Supporting Project number (RSP2022R505), King Saud University, Riyadh, Saudi Arabia.
The authors would like to extend their appreciation to the Researchers Supporting Project number (RSP2022R505), King Saud University, Riyadh, Saudi Arabia.
The authors declare that the research was conducted in the absence of any commercial or financial relationships that could be construed as a potential conflict of interest.
All claims expressed in this article are solely those of the authors and do not necessarily represent those of their affiliated organizations, or those of the publisher, the editors and the reviewers. Any product that may be evaluated in this article, or claim that may be made by its manufacturer, is not guaranteed or endorsed by the publisher.
Abdalla, M. M. (2014). Boosting the growth of rocket plants in response to the application of Moringa oleifera extracts as a biostimulant. Life Sci. J. 11, 1113–1121.
Abdeljalil, N. O.-B., Vallance, J., Gerbore, J., Bruez, E., Martins, G., Rey, P., et al. (2016). Biocontrol of Rhizoctonia root rot in tomato and enhancement of plant growth using rhizobacteria naturally associated to tomato. J. Plant Pathol. Microbiol. 7, 1–8. doi: 10.4172/2157-7471.1000356
Abd-El-Khair, H., and El-Gamal Nadia, G. (2011). Effects of aqueous extracts of some plant species against Fusarium solani and Rhizoctonia solani in Phaseolus vulgaris plants. Arch. Phytopathol. Plant Prot. 44, 1–16. doi: 10.1080/03235400802678436
Abdelkhalek, A. (2019). Expression of tomato pathogenesis related genes in response to Tobacco mosaic virus. J. Anim. Plant Sci. 29, 1596–1602.
Abdelkhalek, A., Al-Askar, A. A., Alsubaie, M. M., and Behiry, S. I. (2021). First report of protective activity of Paronychia argentea extract against tobacco mosaic virus infection. Plan. Theory 10, 2435. doi: 10.3390/plants10112435
Abdelkhalek, A., Al-Askar, A. A., and Behiry, S. I. (2020a). Bacillus licheniformis strain POT1 mediated polyphenol biosynthetic pathways genes activation and systemic resistance in potato plants against Alfalfa mosaic virus. Sci. Rep. 10, 1–16. doi: 10.1038/s41598-020-72676-2
Abdelkhalek, A., Dessoky, E. S., and Hafez, E. (2018). Polyphenolic genes expression pattern and their role in viral resistance in tomato plant infected with Tobacco mosaic virus. Biosci. Res. 14, 3349–3356.
Abdelkhalek, A., Ismail, I. A. I. A., Dessoky, E. S. E. S., El-Hallous, E. I. E. I., and Hafez, E. (2019). A tomato kinesin-like protein is associated with Tobacco mosaic virus infection. Biotechnol. Biotechnol. Equip. 33, 1424–1433. doi: 10.1080/13102818.2019.1673207
Abdelkhalek, A., Salem, M. Z. M., Hafez, E., Behiry, S. I., and Qari, S. H. (2020b). The phytochemical, antifungal, and first report of the antiviral properties of Egyptian Haplophyllum tuberculatum extract. Biology 9, 248. doi: 10.3390/biology9090248
Abdelkhalek, A., and Sanan-Mishra, N. (2019). Differential expression profiles of tomato miRNAs induced by tobacco mosaic virus. J. Agric. Sci. Technol. 21, 475–485.
AbdEl-Rahim, W. M., Khalil, W. K. B., and Eshak, M. G. (2010). Evaluation of the gene expression changes in Nile tilapia (Oreochromis niloticus) as affected by the bio-removal of toxic textile dyes from aqueous solution in small-scale bioreactor. Environmentalist 30, 242–253. doi: 10.1007/s10669-010-9268-7
Abo-Zaid, G., Abdelkhalek, A., Matar, S., Darwish, M., and Abdel-Gayed, M. (2021). Application of bio-friendly formulations of Chitinase-producing Streptomyces cellulosae Actino 48 for controlling Peanut soil-borne diseases caused by Sclerotium rolfsii. J. Fungi 7, 167. doi: 10.3390/jof7030167
Ahn, I.-P., Lee, S.-W., and Suh, S.-C. (2007). Rhizobacteria-induced priming in Arabidopsis is dependent on ethylene, jasmonic acid, and NPR1. Mol. Plant-Microbe Interact. 20, 759–768. doi: 10.1094/MPMI-20-7-0759
Al-Askar, A. A., and Rashad, Y. M. (2010). Efficacy of some plant extracts against Rhizoctonia solani on pea. J. Plant Prot. Res. 50, 239–243. doi: 10.2478/v10045-010-0042-0
Alexopoulos, C. J., Mims, C. W., and Blackwell, M. (1996). Introductory Mycology. New York, NY: John Wiley and Sons.
Al-Hatmi, A. M. S., van Diepeningen, A. D., Curfs-Breuker, I., de Hoog, G. S., and Meis, J. F. (2015). Specific antifungal susceptibility profiles of opportunists in the Fusarium fujikuroi complex. J. Antimicrob. Chemother. 70, 1068–1071. doi: 10.1093/jac/dku505
Al-Huqail, A. A., Behiry, S. I., Salem, M. Z. M., Ali, H. M., Siddiqui, M. H., and Salem, A. Z. M. (2019). Antifungal, antibacterial, and antioxidant activities of Acacia saligna (Labill.) HL Wendl. flower extract: HPLC analysis of phenolic and flavonoid compounds. Molecules 24, 700. doi: 10.3390/molecules24040700
Ambikapathy, V., Gomathi, S., and Panneerselvam, A. (2011). Effect of antifungal activity of some medicinal plants against Pythium debaryanum (Hesse). Asian J Plant Sci Res 1, 131–134.
Ashmawy, N. A., Behiry, S. I., Al-Huqail, A. A., Ali, H. M., and Salem, M. Z. M. (2020a). Bioactivity of selected phenolic acids and hexane extracts from Bougainvilla spectabilis and Citharexylum spinosum on the growth of Pectobacterium carotovorum and Dickeya solani bacteria: an opportunity to save the environment. Processes 8, 482. doi: 10.3390/pr8040482
Ashmawy, N. A., Salem, M. Z. M., El Shanhorey, N., Al-Huqail, A., Ali, H. M., and Behiry, S. I. (2020b). Eco-friendly wood-biofungicidal and antibacterial activities of various Coccoloba uvifera L. leaf extracts: HPLC analysis of phenolic and flavonoid compounds. Bioresources 15, 4165–4187. doi: 10.15376/biores.15.2.4165-4187
Ashraf, Z., Muhammad, A., Imran, M., and Tareq, A. H. (2011). In vitro antibacterial and antifungal activity of methanol, chloroform and aqueous extracts of Origanum vulgare and their comparative analysis. Int. J. Org. Chem. 1, 257–261. doi: 10.4236/ijoc.2011.14037
Balasubramanian, V., Vashisht, D., Cletus, J., and Sakthivel, N. (2012). Plant β-1, 3-glucanases: their biological functions and transgenic expression against phytopathogenic fungi. Biotechnol. Lett. 34, 1983–1990. doi: 10.1007/s10529-012-1012-6
Beara, I. N., Lesjak, M. M., Jovin, E. Đ., Balog, K. J., Anackov, G. T., Orcic, D. Z., et al. (2009). Plantain (Plantago L.) species as novel sources of flavonoid antioxidants. J. Agric. Food Chem. 57, 9268–9273. doi: 10.1021/jf902205m
Beara, I. N., Lesjak, M. M., Orčić, D. Z., Simin, N. Đ., Četojević-Simin, D. D., Božin, B. N., et al. (2012). Comparative analysis of phenolic profile, antioxidant, anti-inflammatory and cytotoxic activity of two closely-related plantain species: Plantago altissima L. and Plantago lanceolata L. LWT-Food Sci. Technol. 47, 64–70. doi: 10.1016/j.lwt.2012.01.001
Behbahani, B. A., Shahidi, F., Yazdi, F. T., and Mohebbi, M. (2013). Antifungal effect of aqueous and ethanolic mangrove plant extract on pathogenic fungus “in vitro”. Int. J. Agron. Plant Prod. 4, 1652–1658. doi: 10.17795/zjrms-5992
Bhardwaj, S. K. (2012). Evaluation of plant extracts as antifungal agents against Fusarium solani (Mart.) Sacc. World J. Agric. Sci. 8, 385–388.
Biradar, S. R., and Rachetti, B. D. (2013). Extraction of some secondary metabolites & thin layer chromatography from different parts of Centella asiatica L. (URB). Am. J. Life Sci. 1, 243–247. doi: 10.11648/j.ajls.20130106.11
Chandrasekaran, M., and Chun, S. C. (2016). Expression of PR-protein genes and induction of defense-related enzymes by Bacillus subtilis CBR05 in tomato (Solanum lycopersicum) plants challenged with Erwinia carotovora subsp. carotovora. Biosci. Biotechnol. Biochem. 80, 2277–2283. doi: 10.1080/09168451.2016.1206811
Channa, M. Y., Pathan, M. A., Solangi, G. R., and Wondiar, M. (1995). Studies on Rhizoctonia solani (Kuhn) causing root rot of lentil. Sarhad J. Agric 11, 495–499.
Chiang, L. C., Chiang, W., Chang, M. Y., Ng, L. T., and Lin, C. C. (2002). Antiviral activity of Plantago major extracts and related compounds in vitro. Antivir. Res. 55, 53–62. doi: 10.1016/S0166-3542(02)00007-4
Çoban, T., Çitoǧlu, G. S., Sever, B., and İşcan, M. (2003). Antioxidant activities of plants used in traditional medicine in Turkey. Pharm. Biol. 41, 608–613. doi: 10.1080/13880200390501974
Culver, M., Fanuel, T., and Chiteka, A. Z. (2012). Effect of moringa extract on growth and yield of tomato. Greener J. Agric. Sci. 2, 207–211. doi: 10.5281/zenodo.3372890
D’Maris Amick Dempsey, A. C., Vlot, M. C. W., Daniel, F. K., Dempsey, D. A., Vlot, A. C., Wildermuth, M. C., et al. (2011). Salicylic acid biosynthesis and metabolism. Arab. Book 9:e0156. doi: 10.1199/tab.0156
Deena, M. J., and Thoppil, J. E. (2000). Antimicrobial activity of the essential oil of Lantana camara. Fitoterapia 71, 453–455. doi: 10.1016/S0367-326X(00)00140-4
Desoky, E.-S. M., ElSayed, A. I., Merwad, A.-R. M. A., and Rady, M. M. (2019). Stimulating antioxidant defenses, antioxidant gene expression, and salt tolerance in Pisum sativum seedling by pretreatment using licorice root extract (LRE) as an organic biostimulant. Plant Physiol. Biochem. 142, 292–302. doi: 10.1016/j.plaphy.2019.07.020
Dhingra, O. D., and Sinclair, J. B. (2017). Basic Plant Pathology Methods. Boca Raton, FL: CRC Press.
Dissanayake, M. (2014). Inhibitory effect of selected medicinal plant extracts on phytopathogenic fungus Fusarium oxysporum (Nectriaceae) Schlecht. Emend. Snyder and Hansen. Annu. Res. Rev. Biol. 4, 133–142. doi: 10.9734/ARRB/2014/5777
Druzhinina, I. S., Seidl-Seiboth, V., Herrera-Estrella, A., Horwitz, B. A., Kenerley, C. M., Monte, E., et al. (2011). Trichoderma: the genomics of opportunistic success. Nat. Rev. Microbiol. 9, 749–759. doi: 10.1038/nrmicro2637
El-Benawy, N. M., Abdel-Fattah, G. M., Ghoneem, K. M., and Shabana, Y. M. (2020). Antimicrobial activities of Trichoderma atroviride against common bean seed-borne Macrophomina phaseolina and Rhizoctonia solani. Egypt. J. Basic Appl. Sci. 7, 267–280. doi: 10.1080/2314808X.2020.1809849
El-Kazzaz, M. K., Ghoneim, K. E., Agha, M. K. M., Helmy, A., Behiry, S. I., Abdelkhalek, A., et al. (2022). Suppression of pepper root rot and wilt diseases caused by Rhizoctonia solani and Fusarium oxysporum. Life 12, 587. doi: 10.3390/life12040587
Ertani, A., Pizzeghello, D., Francioso, O., Tinti, A., and Nardi, S. (2016). Biological activity of vegetal extracts containing phenols on plant metabolism. Molecules 21, 205. doi: 10.3390/molecules21020205
Ferreira, C., and Oliveira, R. (2020). Protective antifungal activity of Plantago major extract against the phytopathogenic fungi Phytophthora cinnamomi, Diplodia corticola and Colletotrichum species. Proceedings, 70:94. doi: 10.3390/foods_2020-07678
Gálvez, M., Martín-Cordero, C., Houghton, P. J., and Ayuso, M. J. (2005). Antioxidant activity of methanol extracts obtained from Plantago species. J. Agric. Food Chem. 53, 1927–1933. doi: 10.1021/jf048076s
Gondal, A. S., Rauf, A., and Naz, F. (2019). Anastomosis groups of Rhizoctonia solani associated with tomato foot rot in Pothohar Region of Pakistan. Sci. Rep. 9, 1–12. doi: 10.1038/s41598-019-40043-5
Hamza, A., Mohamed, A., and Derbalah, A. (2016). Unconventional alternatives for control of tomato root rot caused by Rhizoctonia solani under greenhouse conditions. J. plant Prot. Res. 56, 298–305. doi: 10.1515/jppr-2016-0046
Heflish, A. A., Abdelkhalek, A., Al-Askar, A. A., and Behiry, S. I. (2021). Protective and curative effects of Trichoderma asperelloides Ta41 on tomato root rot caused by Rhizoctonia solani Rs33. Agronomy 11, 1162. doi: 10.3390/agronomy11061162
Hoegen, E., Strömberg, A., Pihlgren, U., and Kombrink, E. (2002). Primary structure and tissue-specific expression of the pathogenesis-related protein PR-1b in potato. Mol. Plant Pathol. 3, 329–345. doi: 10.1046/j.1364-3703.2002.00126.x
Hof, H. (2001). Critical annotations to the use of azole antifungals for plant protection. Antimicrob. Agents Chemother. 45, 2987–2990. doi: 10.1128/AAC.45.11.2987-2990.2001
James, J., and Dubery, I. (2011). Identification and quantification of triterpenoid centelloids in Centella asiatica (L.) urban by densitometric TLC. J. Planar Chromatogr. 24, 82–87. doi: 10.1556/JPC.24.2011.1.16
Jat, J. G., and Agalave, H. R. (2013). Fungitoxic properties of some leaf extracts against oilseed-borne fungi. Sci. Res. Report 3, 210–215.
Karima, S., Farida, S., and Mihoub, Z. M. (2015). Antioxidant and antimicrobial activities of Plantago major. Int. J. Pharm. Pharm. Sci. 7, 58–64.
Klironomos, J. N. (2003). Variation in plant response to native and exotic arbuscular mycorrhizal fungi. Ecology 84, 2292–2301. doi: 10.1890/02-0413
Koka, J. A., Wani, A. H., Bhat, M. Y., and Parveen, S. (2017). Antagonistic activity of Trichoderma spp. against some fungi causing fungal rot disease of brinjal. Trends Biosci. 10, 2844–2846.
Kumar, A., Shukla, R., Singh, P., Prasad, C. S., and Dubey, N. K. (2008). Assessment of Thymus vulgaris L. essential oil as a safe botanical preservative against post harvest fungal infestation of food commodities. Innov. Food Sci. Emerg. Technol. 9, 575–580. doi: 10.1016/j.ifset.2007.12.005
Livak, K. J., and Schmittgen, T. D. (2001). Analysis of relative gene expression data using real- time quantitative PCR and the 2 Ϫ ⌬⌬ C T method. Methods 25, 402–408. doi: 10.1006/meth.2001.1262
Lucas, G. B., Campbell, C. L., and Lucas, L. T. (1992). Introduction to Plant Diseases: Identification and Management. New York, NY: Springer Science & Business Media
Mani, J. S., Johnson, J. B., Steel, J. C., Broszczak, D. A., Neilsen, P. M., Walsh, K. B., et al. (2020). Natural product-derived phytochemicals as potential agents against coronaviruses: a review. Virus Res. 284:197989. doi: 10.1016/j.virusres.2020.197989
Marais, J. P. J., Deavours, B., Dixon, R. A., and Ferreira, D. (2006). “The stereochemistry of flavonoids,” in The science of flavonoids, E. Grotewold (Berlin: Springer Press, 1–46.
Martin, N., Vesentini, D., Rego, C., Monteiro, S., Oliveira, H., and Ferreira, R. B. (2009). Phaeomoniella chlamydospora infection induces changes in phenolic compounds content in Vitis vinifera. Phytopathol. Mediterr. 48, 101–116. doi: 10.14601/Phytopathol_Mediterr-2879
Martínez, G., Regente, M., Jacobi, S., Del Rio, M., Pinedo, M., and de la Canal, L. (2017). Chlorogenic acid is a fungicide active against phytopathogenic fungi. Pestic. Biochem. Physiol. 140, 30–35. doi: 10.1016/j.pestbp.2017.05.012
Mikulic-Petkovsek, M., Schmitzer, V., Stampar, F., Veberic, R., and Koron, D. (2014). Changes in phenolic content induced by infection with Didymella applanata and Leptosphaeria coniothyrium, the causal agents of raspberry spur and cane blight. Plant Pathol. 63, 185–192. doi: 10.1111/ppa.12081
Miser-Salihoglu, E., Akaydin, G., Caliskan-Can, E., and Yardim-Akaydin, S. (2013). Evalution of antioxidant activity of various herbal folk medicines. J. Nutr. Food Sci, 3–222. doi: 10.4172/2155-9600.1000222
Mohamed, A. A., Behiry, S. I., Ali, H. M., EL-Hefny, M., Salem, M. Z. M., and Ashmawy, N. A. (2020). Phytochemical compounds of branches from P. halepensis oily liquid extract and S. terebinthifolius essential oil and their potential antifungal activity. Processes 8, 330. doi: 10.3390/pr8030330
Montealegre, J., Valderrama, L., Sánchez, S., Herrera, R., Besoain, X., and Pérez, L. M. (2010). Biological control of Rhizoctonia solani in tomatoes with Trichoderma harzianum mutants. Electron. J. Biotechnol. 13, 1–2. doi: 10.2225/vol13-issue2-fulltext-6
Moorthy, K. K., Subramaniam, P., and Senguttuvan, J. (2013). In vitro antifungal activity of various extracts of leaf and stem parts of Solena amplexicaulis (Lam.) Gandhi. Int. J. Pharm. Pharm. Sci. 5, 745–747.
Mvumi, C., Tagwira, F., and Chiteka, A. Z. (2013). Effect of moringa extract on growth and yield of maize and common beans. Greener J. Agric. Sci. 3, 55–62. doi: 10.15580/GJAS.2013.1.111512264
Nazzaro, F., Fratianni, F., Coppola, R., and De Feo, V. (2017). Essential oils and antifungal activity. Pharmaceuticals 10, 86. doi: 10.3390/ph10040086
Nishibe, S., Murai, M., and Tamayama, Y. (1995). Studies on constituents of Plantaginis Herba 7: flavonoids from Plantago asiatica and P. hostifolia. Nat. Med. 49, 340–342.
Niu, D.-D., Liu, H.-X., Jiang, C.-H., Wang, Y.-P., Wang, Q.-Y., Jin, H.-L., et al. (2011). The plant growth–promoting rhizobacterium Bacillus cereus AR156 induces systemic resistance in Arabidopsis thaliana by simultaneously activating salicylate-and jasmonate/ethylene-dependent signaling pathways. Mol. Plant-Microbe Interact. 24, 533–542. doi: 10.1094/MPMI-09-10-0213
Parveen, S., Wani, A. H., Bhat, M. Y., Malik, A. R., Koka, J. A., and Ashraf, N. (2017). Antimycotic potential of some phytoextracts on some pathogenic fungi. J. Biopest. 10, 60–65.
Parveen, S., Wani, A. H., Ganie, A. A., Pala, S. A., and Mir, R. A. (2014). Antifungal activity of some plant extracts on some pathogenic fungi. Arch. Phytopathol. Plant Prot. 47, 279–284. doi: 10.1080/03235408.2013.808857
Petkovsek, M. M., Slatnar, A., Stampar, F., and Veberic, R. (2011). Phenolic compounds in apple leaves after infection with apple scab. Biol. Plant. 55, 725–730. doi: 10.1007/s10535-011-0176-6
Prasannath, K. (2017). Plant defense-related enzymes against pathogens: a review. AGRIEAST J. Agric. Sci. 11, 38. doi: 10.4038/agrieast.v11i1.33
Rex, J. H., Pfaller, M. A., Rinaldi, M. G., Polak, A., and Galgiani, J. N. (1993). Antifungal susceptibility testing. Clin. Microbiol. Rev. 6, 367–381. doi: 10.1128/CMR.6.4.367
Rex, J. H., Rinaldi, M. G., and Pfaller, M. A. (1995). Resistance of Candida species to fluconazole. Antimicrob. Agents Chemother. 39, 1–8. doi: 10.1128/AAC.39.1.1
Roylawar, P., Panda, S., and Kamble, A. (2015). Comparative analysis of BABA and Piriformospora indica mediated priming of defence-related genes in tomato against early blight. Physiol. Mol. Plant Pathol. 91, 88–95. doi: 10.1016/j.pmpp.2015.06.004
Rusjan, D., Veberič, R., and Mikulič-Petkovšek, M. (2012). The response of phenolic compounds in grapes of the variety ‘Chardonnay’ (Vitis vinifera L.) to the infection by phytoplasma Bois noir. Eur. J. Plant Pathol. 133, 965–974. doi: 10.1007/s10658-012-9967-7
Sabuquillo, P., De Cal, A., and Melgarejo, P. (2006). Biocontrol of tomato wilt by Penicillium oxalicum formulations in different crop conditions. Biol. Control 37, 256–265. doi: 10.1016/j.biocontrol.2006.02.009
Salem, M. Z. M., Behiry, S. I., and EL-Hefny, M. (2019). Inhibition of Fusarium culmorum, Penicillium chrysogenum and Rhizoctonia solani by n-hexane extracts of three plant species as a wood-treated oil fungicide. J. Appl. Microbiol. 126, 1683–1699. doi: 10.1111/jam.14256
Samuelsen, A. B. (2000). The traditional uses, chemical constituents and biological activities of Plantago major L. A review. J. Ethnopharmacol. 71, 1–21. doi: 10.1016/S0378-8741(00)00212-9
Santas, J., Almajano, M. P., and Carbó, R. (2010). Antimicrobial and antioxidant activity of crude onion (Allium cepa, L.) extracts. Int. J. Food Sci. Technol. 45, 403–409. doi: 10.1111/j.1365-2621.2009.02169.x
Schneiter, R., and Di Pietro, A. (2013). The CAP protein superfamily: function in sterol export and fungal virulence. Biomol. Concepts 4, 519–525. doi: 10.1515/bmc-2013-0021
Shaygannia, E., Bahmani, M., Zamanzad, B., and Rafieian-Kopaei, M. (2016). A review study on Punica granatum L. J. Evid. Based. Complement. Altern. Med. 21, 221–227. doi: 10.1177/2156587215598039
Shimada, K., Fujikawa, K., Yahara, K., and Nakamura, T. (1992). Antioxidative properties of xanthan on the autoxidation of soybean oil in cyclodextrin emulsion. J. Agric. Food Chem. 40, 945–948. doi: 10.1021/jf00018a005
Shuping, D. S. S., and Eloff, J. N. (2017). The use of plants to protect plants and food against fungal pathogens: a review. African J. Tradit. Complement. Altern. Med. 14, 120–127. doi: 10.21010/ajtcam.v14i4.14
Silva, P. A., Oliveira, D. F., do Prado, N. R. T., de Carvalho, D. A., and de Carvalho, G. A. (2008). Evaluation of the antifungal activity by plant extracts against Colletotrichum gloeosporioides PENZ. Ciênc. Agrotecnol. 32, 420–428. doi: 10.1590/S1413-70542008000200012
Singh, D., Singh, P., Gupta, A., Solanki, S., Sharma, E., and Nema, R. (2012). Qualitative estimation of the presence of bioactive compound in Centella asiatica: an important medicinal plant. Int. J. Life Sci. Med. Sci. 2, 4–7.
Singh, R. P., Singh, P. K., Rutkoski, J., Hodson, D. P., He, X., Jørgensen, L. N., et al. (2016). Disease impact on wheat yield potential and prospects of genetic control. Annu. Rev. Phytopathol. 54, 303–322. doi: 10.1146/annurev-phyto-080615-095835
Singh, B. N., Singh, A., Singh, S. P., and Singh, H. B. (2011). Trichoderma harzianum-mediated reprogramming of oxidative stress response in root apoplast of sunflower enhances defence against Rhizoctonia solani. Eur. J. Plant Pathol. 131, 121–134. doi: 10.1007/s10658-011-9792-4
Su, Z.-Z., Mao, L.-J., Li, N., Feng, X.-X., Yuan, Z.-L., Wang, L.-W., et al. (2013). Evidence for biotrophic lifestyle and biocontrol potential of dark septate endophyte Harpophora oryzae to rice blast disease. PLoS One 8:e61332. doi: 10.1371/journal.pone.0061332
Valarini, P. J., de Melo, I. S., and Morsoletto, R. V. (2003). Alternative control of root rot of common beans (Phaseolus vulgaris L.). Summa Phytopathol. 29, 334–339.
Velázquez-Fiz, M. P., Díaz-Lanza, A. M., and Fernández-Matellano, L. (2000). Iridoids from Plantago lagopus. Pharm. Biol. 38, 268–270. doi: 10.1076/1388-0209(200009)3841-AFT268
Velioglu, Y., Mazza, G., Gao, L., and Oomah, B. D. (1998). Antioxidant activity and total phenolics in selected fruits, vegetables, and grain products. J. Agric. Food Chem. 46, 4113–4117. doi: 10.1021/jf9801973
Keywords: Plantago lagopus, Rhizoctonia solani, tomato, defense-related genes, gene expression, HPLC
Citation: Behiry SI, Al-Askar AA, Soliman SA, Alotibi FO, Basile A, Abdelkhalek A, Elsharkawy MM, Salem MZM, Hafez EE and Heflish AA (2022) Plantago lagopus extract as a green fungicide induces systemic resistance against Rhizoctonia root rot disease in tomato plants. Front. Plant Sci. 13:966929. doi: 10.3389/fpls.2022.966929
Received: 11 June 2022; Accepted: 19 July 2022;
Published: 08 August 2022.
Edited by:
Rachid Lahlali, Ecole Nationale d'Agriculture de Meknès, MoroccoReviewed by:
Umakanta Sarker, Bangabandhu Sheikh Mujibur Rahman Agricultural University, BangladeshCopyright © 2022 Behiry, Al-Askar, Soliman, Alotibi, Basile, Abdelkhalek, Elsharkawy, Salem, Hafez and Heflish. This is an open-access article distributed under the terms of the Creative Commons Attribution License (CC BY). The use, distribution or reproduction in other forums is permitted, provided the original author(s) and the copyright owner(s) are credited and that the original publication in this journal is cited, in accordance with accepted academic practice. No use, distribution or reproduction is permitted which does not comply with these terms.
*Correspondence: Said I. Behiry, c2FpZC5iZWhpcnlAYWxleHUuZWR1LmVn
Disclaimer: All claims expressed in this article are solely those of the authors and do not necessarily represent those of their affiliated organizations, or those of the publisher, the editors and the reviewers. Any product that may be evaluated in this article or claim that may be made by its manufacturer is not guaranteed or endorsed by the publisher.
Research integrity at Frontiers
Learn more about the work of our research integrity team to safeguard the quality of each article we publish.