- 1Department of Biology, University of Nyíregyháza, Nyíregyháza, Hungary
- 2Department of Tisza Research, Centre for Ecological Research, Debrecen, Hungary
- 3Doctoral School of Biological Sciences, Hungarian University of Agriculture and Life Sciences, Gödöllő, Hungary
- 4Institute of Water and Environmental Management, University of Debrecen, Debrecen, Hungary
- 5Isotope Climatology and Environmental Research Centre (ICER), Institute for Nuclear Research, Eötvös Loránd Research Network, Debrecen, Hungary
- 6Department of Community and Ecosystem Ecology, Leibniz Institute of Freshwater Ecology and Inland Fisheries (IGB), Berlin, Germany
Free-floating and rootless submerged macrophytes are typical, mutually exclusive vegetation types that can alternatively dominate in stagnant and slow flowing inland water bodies. A dominance of free-floating plants has been associated with a lower number of aquatic ecosystem services and can be explained by shading of rootless submerged macrophytes. Vice versa, high pH and competition for several nutrients have been proposed to explain the dominance of rootless submerged macrophytes. Here, we performed co-culture experiments to disentangle the influence of limitation by different nutrients, by pH effects and by allelopathy in sustaining the dominance of rootless submerged macrophytes. Specifically, we compared the effects of nitrogen (N), phosphorus (P), iron (Fe) and manganese (Mn) deficiencies and an increased pH from 7 to 10 in reducing the growth of free-floating Lemna gibba by the rootless Ceratophyllum demersum. These macrophyte species are among the most common in highly eutrophic, temperate water bodies and known to mutually exclude each other. After co-culture experiments, additions of nutrients and pH neutralisation removed the growth inhibition of free-floating plants. Among the experimentally tested factors significantly inhibiting the growth of L. gibba, an increase in pH had the strongest effect, followed by depletion of P, N and Fe. Additional field monitoring data revealed that in water bodies dominated by C. demersum, orthophosphate concentrations were usually sufficient for optimal growth of free-floating plants. However, pH was high and dissolved inorganic N concentrations far below levels required for optimal growth. Low N concentrations and alkaline pH generated by dense C. demersum stands are thus key factors sustaining the stable dominance of rootless submerged vegetation against free-floating plants. Consequently, N loading from e.g. agricultural runoff, groundwater or stormwater is assumed to trigger regime shifts to a dominance of free-floating plants and associated losses in ecosystem services.
Introduction
Competition for nutrients or light strongly influence plant-plant interactions which, together with other factors such as allelopathy or susceptibility to herbivores or pathogens, can induce significant changes in community structure (e.g., Bornette and Puijalon, 2011; Le Bagousse-Pinguet, et al., 2012; Szabó et al., 2020). In aquatic systems, there is a trade-off among primary producers regarding light availability and nutrient uptake (Szabó et al., 2010). In larger open water bodies with high nutrient concentrations, phytoplankton usually dominates the primary production (Scheffer et al., 1993; Scheffer and Van Nes, 2007). However, in small lentic water bodies and in slow-flowing channels, free-floating plant dominance is frequently observed (Scheffer et al., 2003; Smith, 2014; Kazanjian et al., 2018) that suppresses submerged macrophytes by shading (Morris et al., 2003; Van Gerven et al., 2015). Phytoplankton and free-floating plants retrieve their nutrients from the open water and can thus be outcompeted at lower nutrient concentrations by submerged vegetation with access to sediment nutrients (Van Zuidam and Peeters, 2013; Szabó et al., 2022). Such submerged macrophyte dominance is associated with the highest number of ecosystem services compared to other vegetation types (Janssen et al., 2021).
Interestingly, reviewing the database of European surface waters revealed that rootless submerged vegetation also often dominates (Birk and Willby, 2010) despite its inferiority in the competition for light. Coontail (Ceratophyllum demersum) is one the most abundant, rootless submerged species in temperate water bodies (Hilt et al., 2018; Szabó et al., 2022) and often forms thick mats below the surface in small ponds and slow-flowing ditches (Lombardo, 2003). Field observations revealed a negative correlation between the occurrence of C. demersum and free-floating plants such as the duckweed species Lemna gibba (Feuchtmayr et al., 2009; Van Zuidam and Peeters, 2013; Koleszár et al., 2022). This raised the question how rootless species without access to sediment nutrients ever win against free-floating competitors that can shade out submerged macrophytes (Scheffer et al., 2003; Larson, 2007; Lu et al., 2013). Experiments along with a field survey showed that rootless submerged vegetation can sustain stable dominance against free-floating plants below a threshold nutrient concentration (Szabó et al., 2022). It was observed that beyond extremely high pH levels, concentrations of several nutrients [nitrogen (N), phosphorus (P), iron (Fe), manganese (Mn)] dropped below optimal levels for growth of free-floating plants (Szabó et al., 2022). However, according to Liebig’s law of the minimum, it is likely that free-floating plant growth is dictated by the scarcest resource. Accordingly, we tested the hypothesis that high pH and different nutrient deficiencies are not equally important for sustaining the stable dominance of rootless submerged macrophytes competing with free-floating plants. We performed co-culture and nutrient deficiency experiments to compare the single influence of N, P, Fe and Mn limitation as well as increased pH levels as potential mechanisms of growth retardation of free-floating L. gibba by the rootless submerged C. demersum. To rule out a potential allelopathic impact of C. demersum (Hilt and Gross, 2008), additional treatments were tested. In addition, monitoring data on the abundance of C. demersum in Hungarian water bodies and respective hydrochemical conditions were studied to complement laboratory results with field observations.
Material and methods
Free-floating (L. gibba, subsequently termed Lemna) and rootless submerged plants (C. demersum, subsequently termed Ceratophyllum) plants were collected from canals near Nyíregyháza (NE Hungary, N 47.996376°, E 21.734152°). Plants were preincubated for two weeks under experimental conditions on a general purpose “BS” medium (Barko and Smart, 1985). This BS medium was supplemented by adding NH4NO3 to a final concentration of 5 mg N L-1. Phosphorus was added as K2HPO4 to a final concentration of 1.0 mg P L-1 and a supply of micronutrients was ensured by adding 0.1 mL L-1 TROPICA supplier micronutrient solution (final concentrations: 0.08 mg Fe L-1, 0.03 mg Mn L-1, 0.002 mg Zn L-1, 0.006 mg Cu L-1and 0.002 mg Mo L-1). The initial pH of the medium was adjusted to 7.3. Plant cultures were kept under 25°C, a photon flux density of 250 µmol m-2 s-1 and a 16 h light/8 h dark cycle.
Co-culture experiment
For the experiments, plastic tubes with a diameter of 5 cm were placed vertically in 2 L black plastic aquaria (Figure 1) serving as a duckweed enclosure (Szabó et al., 2003). For allowing nutrient transfer between the inner and outer medium, each tube had several holes. Portions of 100 ± 1 mg biomass (fresh weight) of Lemna (mean 83 ± 12 frond) were placed in the enclosures and co-cultured with 0 g (control) and 10 ± 0.1 g biomass of C. demersum plants (6-7 shoots, total length 122 - 135 cm) for 10 days. Co-cultures were grown in triplicate. In addition, 30 co-cultures were used for the subsequent nutrient deficiency experiment (Figure 1). Ceratophyllum shoots were grown outside of the Lemna enclosures. As soon as Lemna cover reached 100%, overcrowding was avoided by replacing the enclosure with a bigger one. In the control aquaria, the area outside the enclosures was completely covered by a grey plastic sheet to avoid algae growth. Biomass (fresh weight) of the Lemna fronds was measured on the 4th, 6th, 8th, and 10th days after blotting fronds on tissue to remove water. After 10 days, the Lemna plants were harvested, their dry weight (DW) was measured after drying to weight constancy at 80°C and relative growth rates (RGR) of the cultures were calculated: RGR = (lnDW10 – lnDW0)/t in which DW12 and DW0 are the dry weights after 10 days and at time zero, respectively, and t is the time in days. The RGR based on fresh weight (FW) was followed in time and was calculated for the 4th, 6th, 8th and 10th days of incubation: RGR10 = (lnFW10 – lnFW8)/2. Values of pH in the medium were recorded on every second day. Water samples were taken in triplicate at the beginning of the experiment and the 2nd, 4th, 6th and 10th day, filtered and analysed for concentrations of PO43-, NO3-, NH4+, Fe, Mn, Zn, Cu and Mo. At the 10th day, the culture medium was gently decanted from 30 aquaria treated with Ceratophyllum (in total 50 L, put into one big container). After 24 hours of particle sedimentation at 5 °C in the dark, this water was decanted again and filtered through 10 µm pore diameter filters and analysed for its nutrient content as detailed above. This filtrate was used for the subsequent nutrient deficiency experiment as nutrient-depleted medium (Table 1, Figure1).
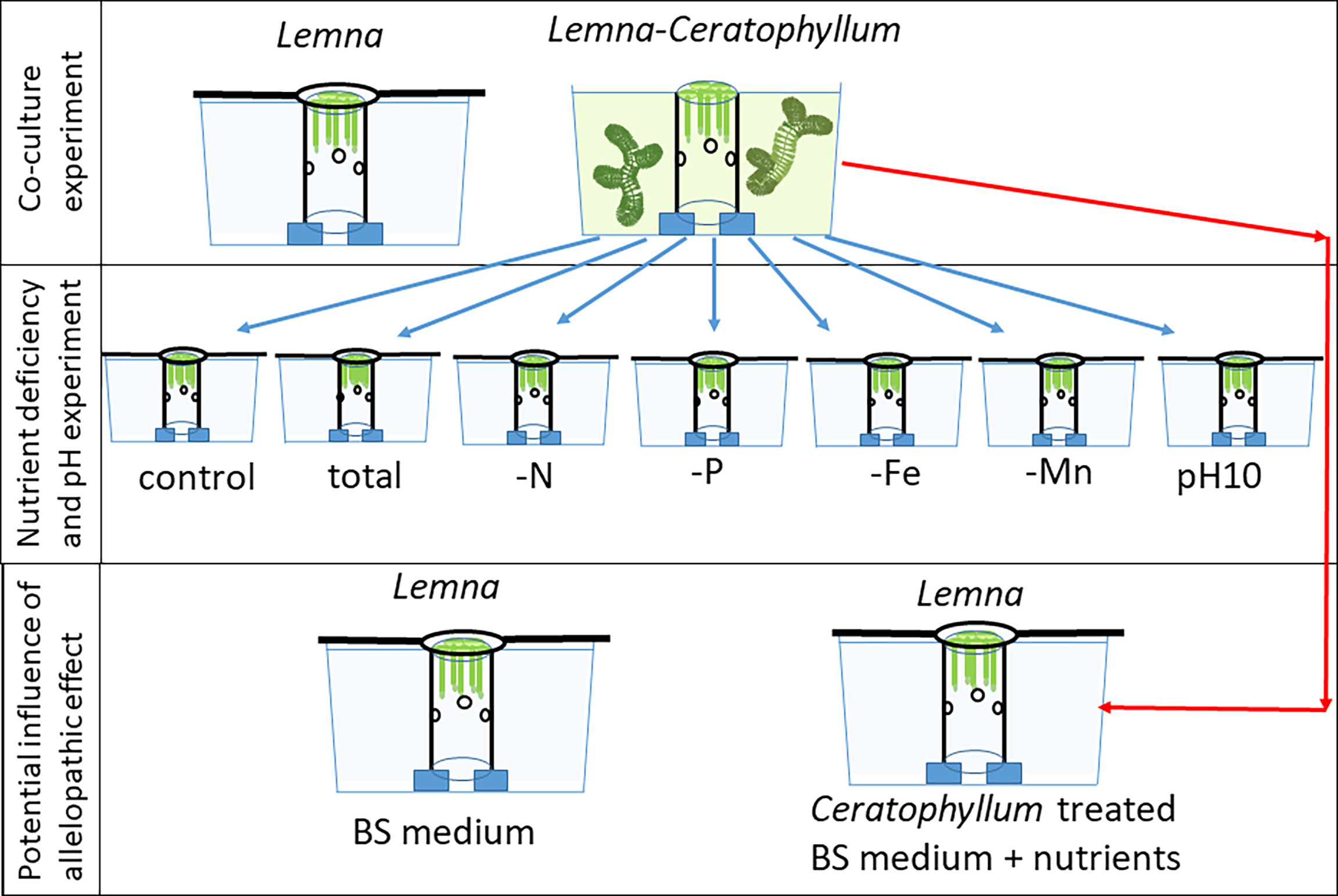
Figure 1 Setup of experiments testing the effect of the rootless submerged macrophyte Ceratophyllum demersum on the free-floating macrophyte Lemna gibba and disentangling the impact of different nutrient deficiencies, pH increases and allelopathic effects.
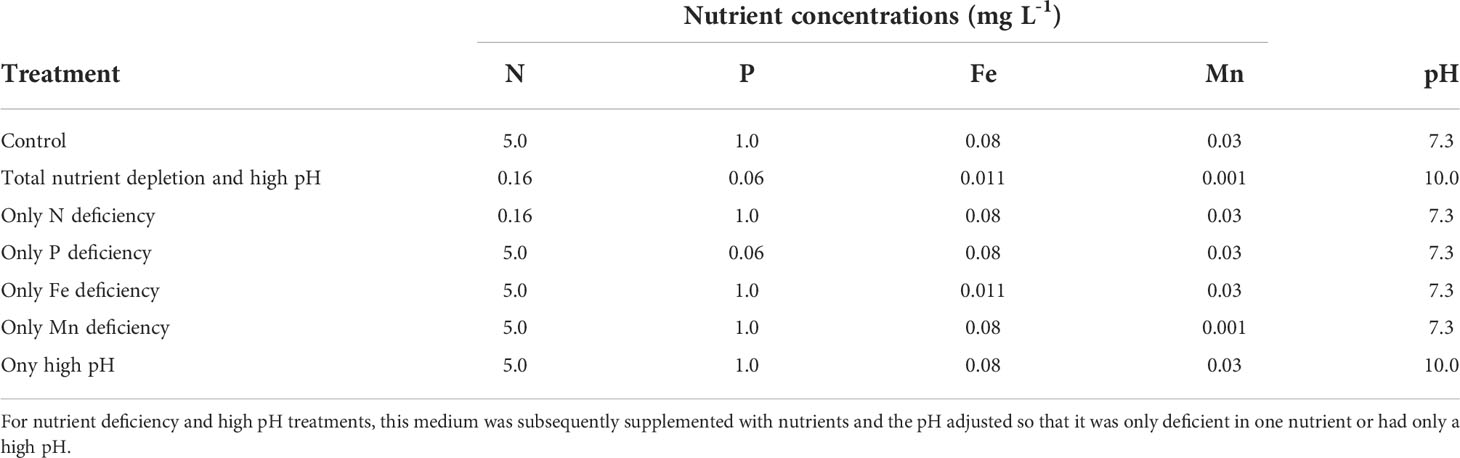
Table 1 Chemical composition of the culture medium in controls and in Ceratophyllum demersum treatments after 10 days (total nutrient depletion and high pH).
Nutrient deficiency and high pH experiment
Two litre aquaria containing Lemna enclosures were covered by black foil on the sides to avoid light penetration and filled with 2 L of nutrient depleted medium from the previous co-culture experiment after adjusting the pH to 7.3 by adding 0.1 M HCl to the medium (apart for the high pH treatment, see below and Table 1). Nutrients were added to arrive at treatments with a depletion of only one respective nutrient (N, P, Fe, Mn) (Table 1; Figure 1). In control cultures, nutrient concentrations (N, P, Fe, Mn) and pH were adjusted as in the non-treated “BS” medium (Table 1). Nutrients were supplemented as NH4NO3, K2HPO4, FeSO4 and MnCl2. In Lemna-Ceratophyllum co-cultures, pH rose to 10.02. To measure the potential pH effect on the growth of Lemna, nutrient depleted media were supplemented with all nutrients (N, P, Fe, Mn) and the pH was adjusted daily to 10.02 by 0.2 M KOH. Ceratophyllum treated L. gibba fronds in nutrient-depleted state from the previous experiment (100 mg fresh weight each) were placed in the enclosures (5 cm in diameter) in each of the aquaria (four replicates per treatment). Similar to controls in the first experiment, the surface of the aquaria outside the enclosures was covered by grey plastic sheets to avoid algal growth. Overcrowding was avoided by replacing the enclosures with bigger ones (up to 5 times, up to 10 cm diameter). Lemna cultures were incubated for 14 days and biomass (fresh weight after blotting) of the fronds was measured on the 6th, 10th and 14th day. Relative growth rates (RGR6-14) were calculated for the period between the 6 and 14th day (Szabó et al., 2022). This period was chosen to avoid an influence of the initial lag phase in Lemna growth due to initial nutrient deficiency in the fronds from the previous experiment. After 14 days the Lemna plants were harvested. One part of the samples (50-100 mg) was used for chlorophyll determination, while the rest was used for determining dry weight and dry matter content (DMC) and subsequent analyses of C, N, P, Fe and Mn content (see below).
Potential influence of allelopathic effects
For testing the possible allelopathic effects of Ceratophyllum on Lemna growth, 100 mg FW of preincubated Lemna fronds were grown in BS medium (5 mg N L-1, 1.0 mg P L-1, plus micronutrients) as control and in Ceratophyllum treated nutrient depleted media with nutrient concentrations (N, P, Fe, Mn) and pH adjusted as in the non-treated BS medium (Table 1). Both treatments (3 replicates each) were cultivated as described above. After 10 days the Lemna plants were harvested and their dry weight was determined to calculate RGR.
Field surveys
Using the Database of Hungarian Surface Waters, we collected data from water bodies (246 locations) in which either C. demersum (n = 215) or L. gibba (n = 46) occurred between July and September in the period 2005 - 2019. We tested for correlations between the concentrations of dissolved inorganic nitrogen (DIN = NO3- + NH4+) and ortho-phosphate in the water and the cover (in percent) of Ceratophyllum. To avoid an influence of other submerged plants, we selected only sites with a total cover of other submerged plants below the cover of C. demersum or a lower than 15% of the same procedure was applied for L. gibba. In addition, we took water samples from the upper 5 cm layer in Ceratophyllum dominated water bodies (N = 39) from drainage ditches, oxbow lakes and ponds in the North-eastern part of the Great Hungarian Plain. Samples were taken between 9 am and 3 pm in July and August and were analysed for pH and filtered through a filter with a 0.45 µm pore diameter. After adding 0.1 ml 67% (m/m) nitric acid into 20 mL of filtrate, iron and manganese content of the samples were measured by ICP OES.
Analytical methods
Measurements of pH of the media were performed using a pH meter (Orion Start 2000). Nutrient (NH+4-N, NO3–N, PO4 3–P) concentrations of filtered (0.45 µm) water samples were determined photometrically according to Hungarian standards (MSZ ISO 7150-1, 1992; MSZ EN ISO 6878, 2004; MSZ 1484-13, 2009). Concentrations of Fe, Mn Zn, Mo and Cu in the medium were determined by Agilent 8800 inductively coupled plasma mass spectrometry (ICP MS, Agilent Technologies, Waldbronn, Germany). Chlorophyll (chl) of Lemna fronds was extracted in 6 mL 95% ethanol for 24 h at 4°C in the dark. Concentration of chl a and b were measured by spectrophotometry (T80+ Spectrometer, PG Instruments Limited, UK) and calculated according to Lichtentaler (1987). Total carbon and nitrogen content in the Lemna plants were determined by dry combustion using a Vario Max Cube elemental analyzer (Elementar GMBH, Germany). Phosphorus, iron and manganese content of Lemna was measured by MPAS after acidic digestion using 5 mL of 67% (m/m) nitric acid and 5 mL 30% (m/m) hydrogen peroxide at 90°C for one hour. In all but controls and manganese depleted cultures, Lemna plant samples of the replicates were merged to arrive at sufficient biomass for analyses.
Statistical methods
The impact of the different individual factors (nutrient deficiencies, high pH) on several dependent variables (biomass, RGR, total chl content, dry matter content) was tested by analysis of variance (ANOVA). Normal distribution of variables was checked by Kolmogorov-Smirnov tests. The homogeneity of the variances of the dependent variables was checked by Levene’s test. In Lemna-Ceratophyllum co-culture experiments, we used Pairwise Comparisons (PC) to test for significant differences between Lemna biomass and RGR6-14 in controls and Ceratophyllum treatments where mean differences (MD) ± SE were indicated. In nutrient deficiency experiments, we compared Lemna biomass (FW14), RGR6-14, and chl content among treatments using Tukey post-hoc tests. Field data were evaluated using a regression analysis with C. demersum density as independent and nutrient concentrations (DIN, PO43–P) as dependent variable. All statistical analyses were performed using SPSS 16.0.
Results
Co-culture experiment
The Control Lemna cultures co-cultivated without Ceratophyllum showed an exponential growth (RGR: 0.394 - 0.212 day-1). Ceratophyllum reduced the growth rate of Lemna significantly (MD 0.187 ± 0.042, P = 0.012). At the end of the experiment RGR of Lemna was reduced below 0.05 day-1 (Figure 2). The pH of treatments containing Ceratophyllum rose above 9.60 at the second day and reached 10.02 at the 10th day. The concentration of dissolved inorganic nitrogen (NH+4-N and NO3–N) was decreased to 0.2 mg L-1 and concentrations of PO43–P decreased below 0.02 mg L-1 within four days. Among the measured micronutrients (Fe, Mn, Zn, Cu, Mo) only the Fe and Mn concentrations showed a significant decline in treatments containing Ceratophyllum (Figure 3; Table 1).
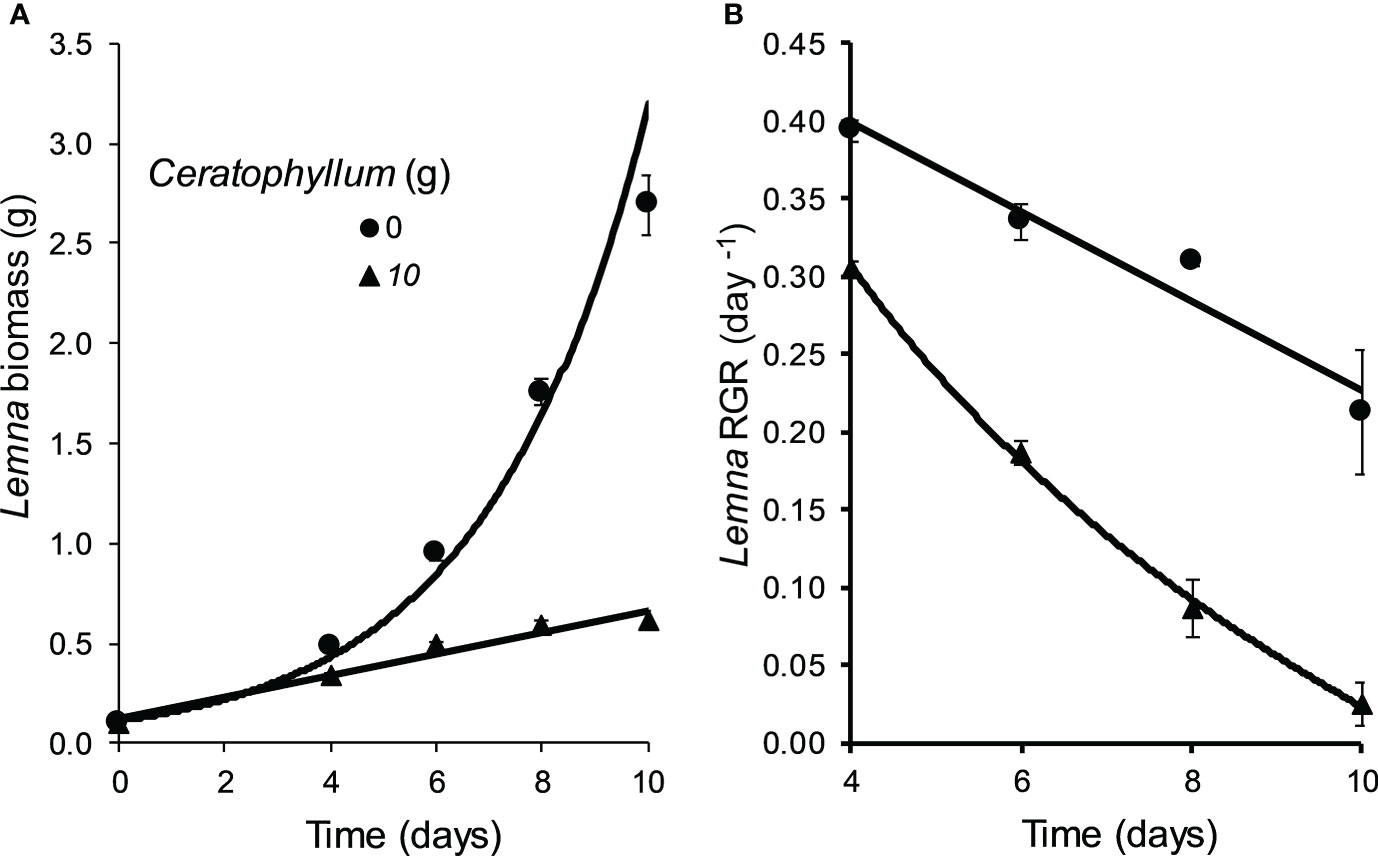
Figure 2 Effect of Ceratophyllum demersum on the biomass (fresh weight) (A) and the relative growth rate (RGR) (B) of Lemna gibba compared to treatments without C. demersum (means ± standard error, n = 3).
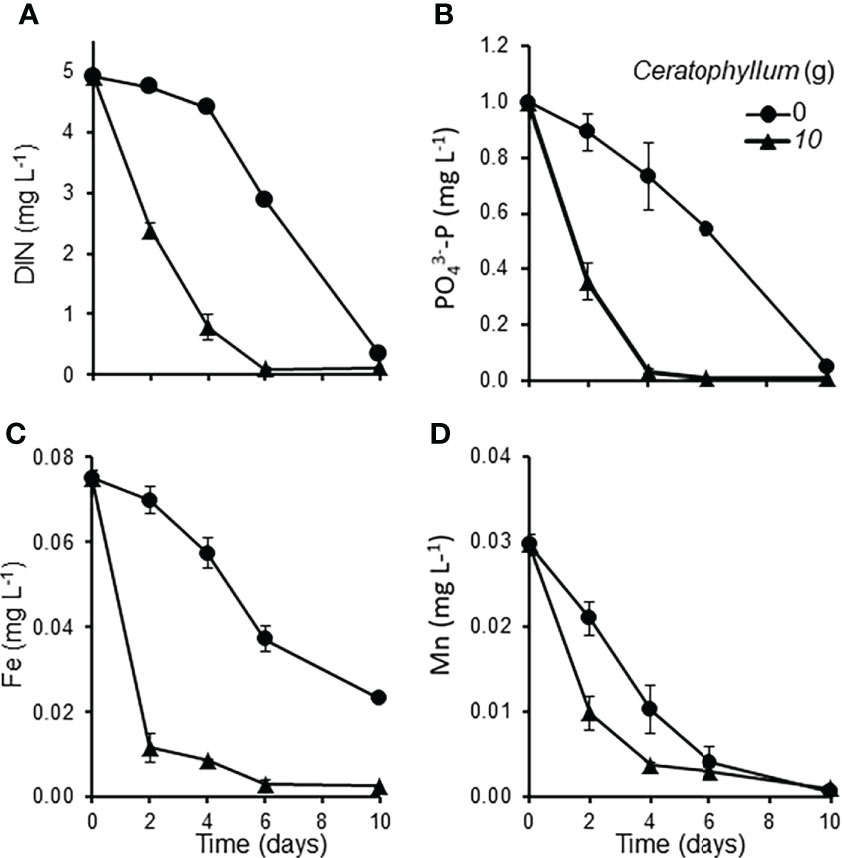
Figure 3 Nutrient concentrations in Lemna-Ceratophyllum co-cultures grown on static medium: (A) dissolved inorganic nitrogen (DIN: NH4+-N plus NO3- -N), (B) PO43–P, (C) total iron (Fe), (D) total manganese (Mn) (means ± standard error, n = 3).
Nutrient deficiency and high pH experiment
Manganese deficiency had a slight but significant (MD 0.018 ± 0.009, P < 0.048) negative effect on the relative growth rate (RGR6-14) of Lemna (Figures 4A, B). The same holds for iron deficiency (MD 0.069 ± 0.009, P < 0.001). The impact of N deficiency was even stronger (MD 0.108 ± 0.009, P < 0.001) lowering influence. Treatments with P deficiency (MD 0.152 ± 0.009, P < 0.001) also showed a significantly reduced growth of Lemna which was similar to the growth in N deficient treatments. In treatments with all nutrients replaced, but a high pH of 10.02, the growth of Lemna was significantly (MD 0.126 ± 0.009, P < 0.001) lower in treatments with C. demersum than in controls. Total nutrient depletion together with a high pH (10.02) resulted in the strongest growth reduction of Lemna (Figure 4, MD 0.176 ± 0.009, P < 0.001). Iron, N, and P deficiency decreased the RGR6-14 of Lemna by 30%, 47% and 66%, respectively, and the high pH by 55% (ESM1).
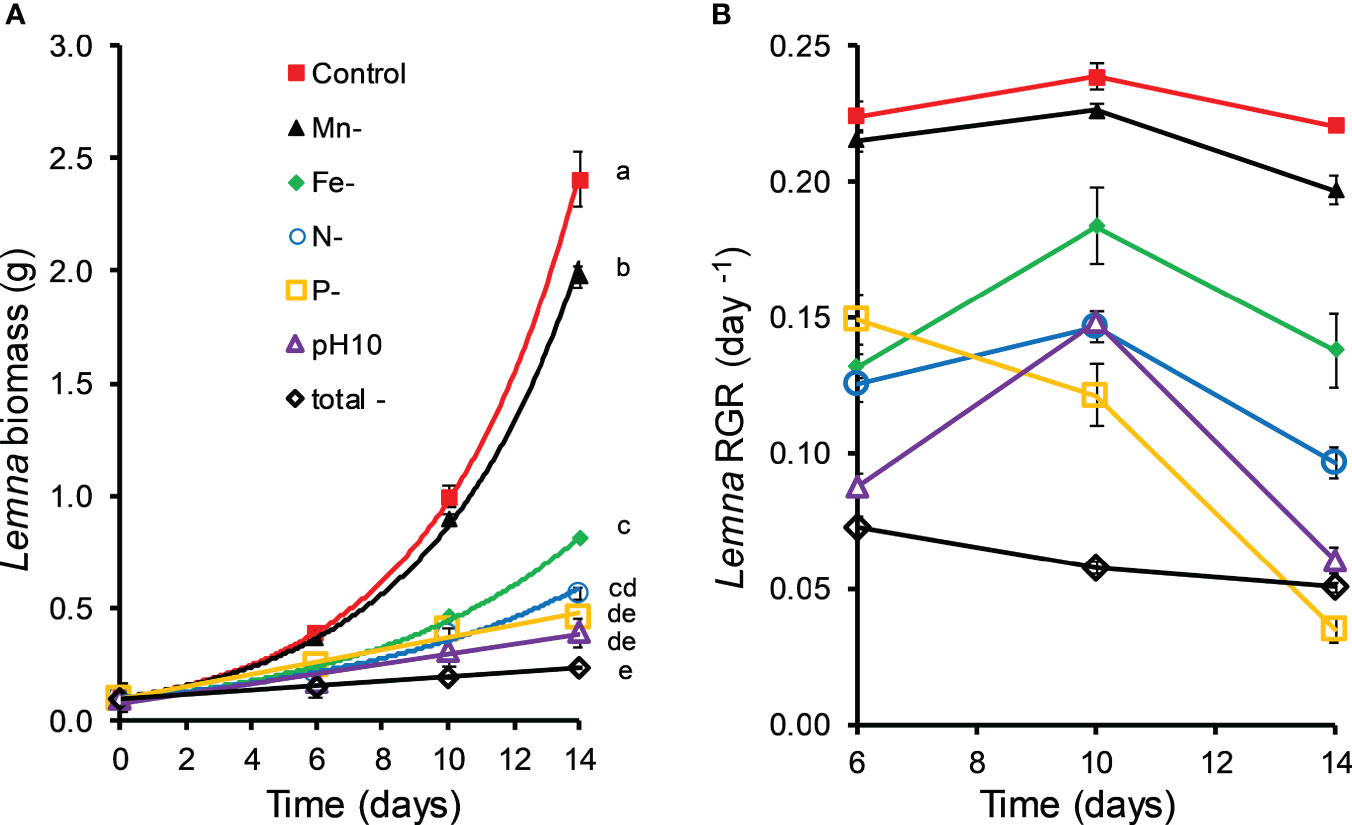
Figure 4 Biomass (A) and relative growth rates (RGR) (B) of Lemna gibba grown in nutrient deficient (N, P, Fe Mn) and high pH (10.0) media retrieved from co-culture experiments with Ceratophyllum demersum and supplemented with respective nutrients (see Table 2, means ± standard error, n = 4). Significant differences (oneway ANOVA, Tukey’s posthoc test, P < 0.05) among treatments are indicated with different lowercase letters.
All examined nutrient deficiencies (N, P, Fe, Mn) and high pH had significant effects on the chl content of Lemna. N deficiency of Lemna growth resulted in the strongest drop (MD 1.588 ± 0.066, P < 0.001) in chl content (Figure 5A). Mn, P, Fe and N deficiency decreased the chl content of L. gibba by 21%, 80%, 84% and 89%, respectively, and the alkaline pH by 83%.
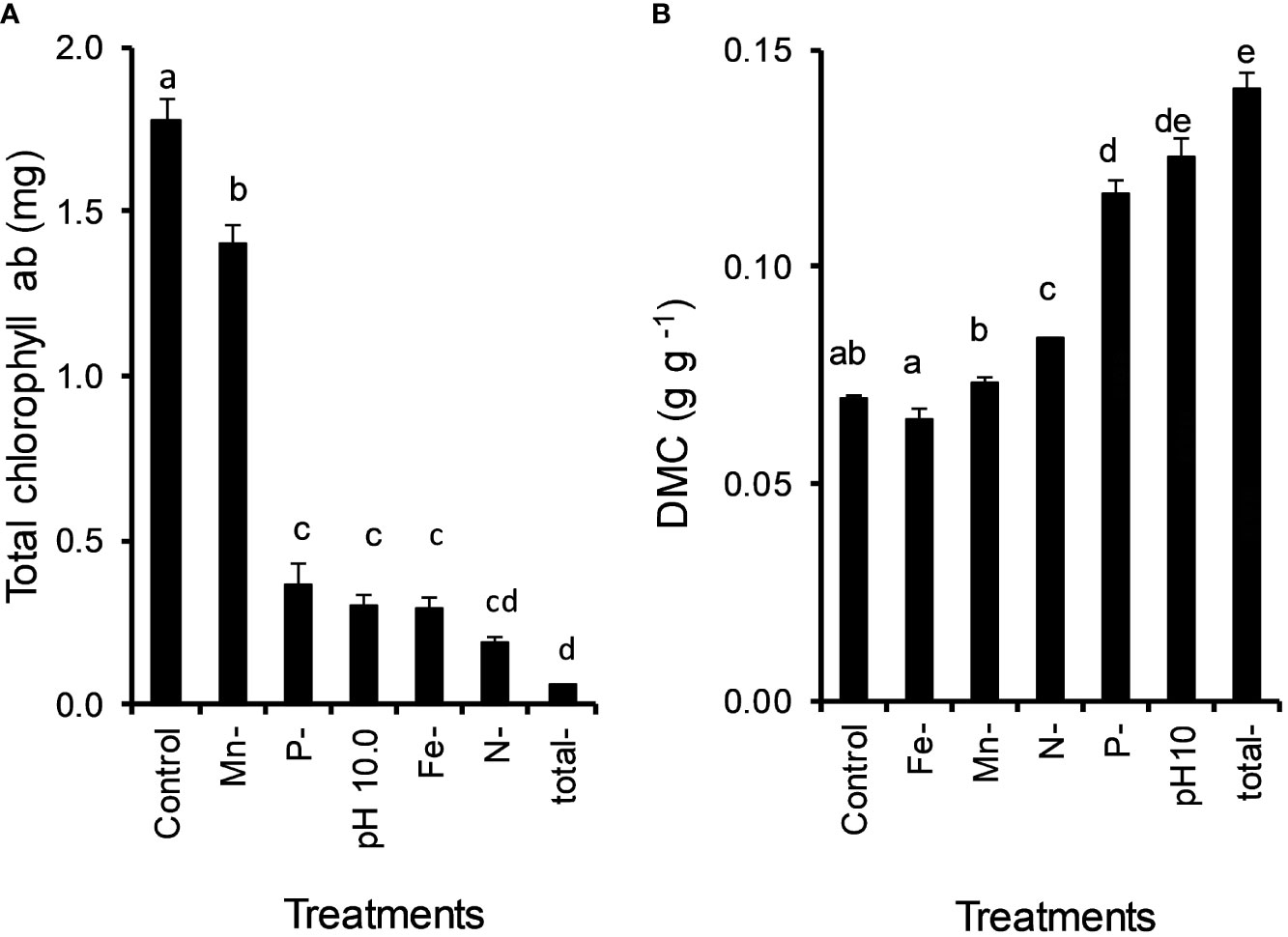
Figure 5 Effect of nutrient deficiencies (N, P, Fe, Mn) and high pH (10) caused by Ceratophyllum demersum on the total chlorophyll content (A) and dry matter content (DMC) (B) of Lemna gibba (means ± standard error, n = 4). Significant differences (oneway ANOVA, Tukey’s test, P < 0.05) among treatments are indicated with different lowercase letters.
Nutrient deficiency and high pH resulted 49-66% drop in elemental concentrations in Lemna (Table 2). The nitrogen concentration in N depleted Lemna fronds was reduced to a low level (1.3% DW). High pH resulted intermediate reduction in elemental concentrations of Lemna compared with nutrient deficiency (N, P, Fe, or Mn) or with total depleted fronds (Table 2).
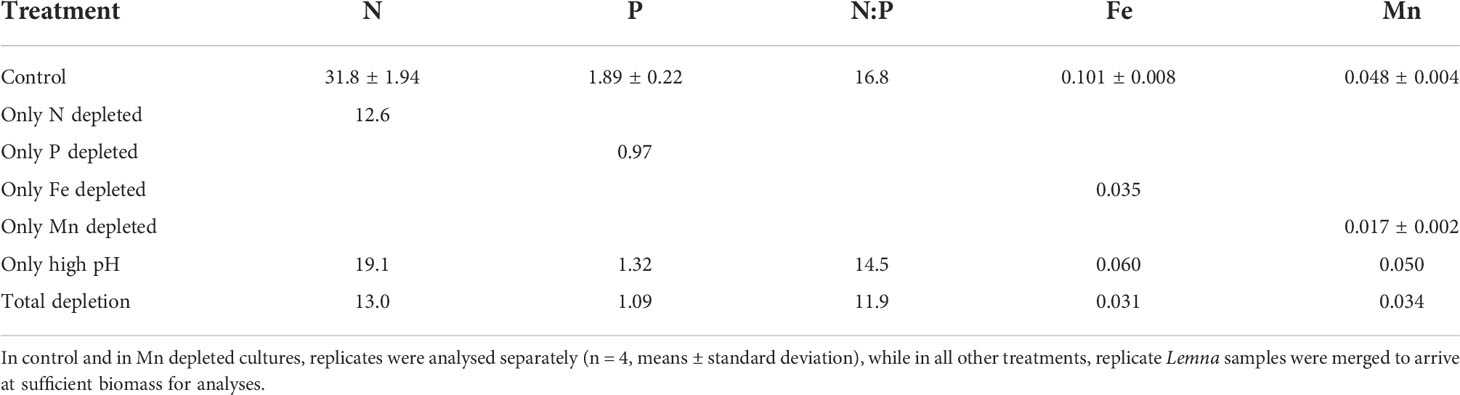
Table 2 Elemental composition of Lemna fronds in nutrient deficiency and high pH experiment (data are in mg g-1 dry weight).
Nutrient deficiency and high pH significantly increased the dry matter content (DMC) of Lemna with the following order: N and P deficiency, high pH (by 20%, 68%, 81%). Total nutrient deficiency with high pH showed the highest DMC of Lemna (103%) (Figure 5B).
Potential allelopathic effects
Ceratophyllum treated media with total nutrient supplement and adjusted pH had no significant (MD 0.013 ± 0.006, P = 0.087) effect on the RGR (calculated based on the dry weight) of Lemna.
Field surveys
Based on the Database of Hungarian Surface Waters (n = 245) the concentrations of DIN and ortho-phosphate were 0.78 ± 0.22 mg L-1 (standard error) and 0.25 ± 0.09 mg L-1, respectively, in water bodies dominated by Ceratophyllum (coverage > 87.5%) (Figure 6). In 60% of the data, DIN concentrations were lower than 0.5 mg L -1. DIN was negatively correlated with the cover of Ceratophyllum and this relationship was significant (ANOVA F = 8.141, P = 0.005). However, there was no significant relationship between the cover of Ceratophyllum and ortophosphate concentration (ANOVA, F = 0.285, P = 0.594).
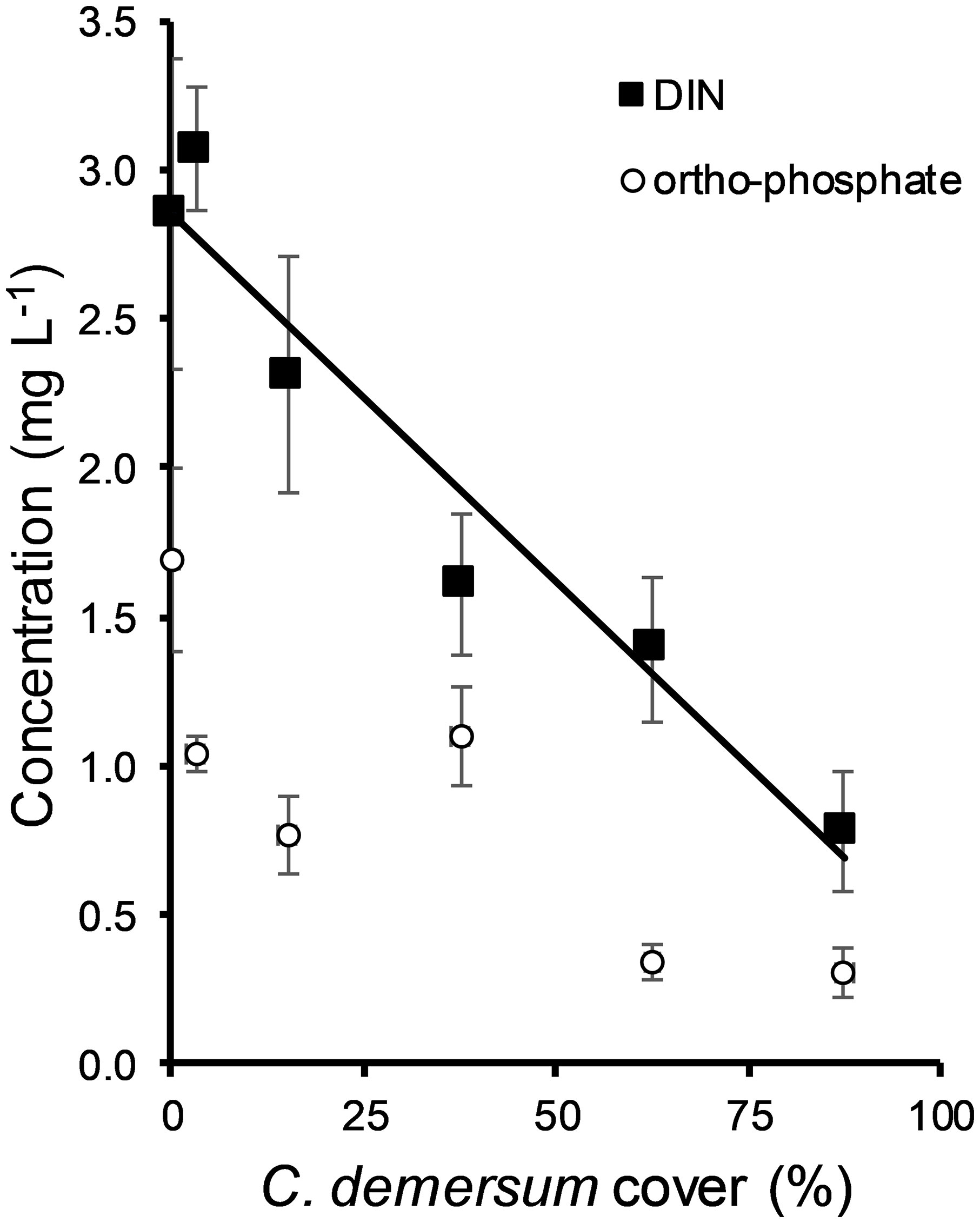
Figure 6 Correlation between the coverage of Ceratophyllum demersum and concentrations of dissolved inorganic nitrogen (DIN) and ortho-phosphate (means ± standard error) in Hungarian water bodies. Data were collected from the database of Hungarian surface waters (n = 173).
In Ceratophyllum dominated (coverage > 87.5%) water bodies sampled in the northestern Great Hungarian Plain (N = 39), the pH of the water was high (10.18 ± 0.06), while Fe concentrations were moderately low (41.4 ± 6.7 µg L-1) and Mn concentrations were close to the detection limit (1.4 ± 0.2 µg L-1).
Discussion
Our study disentangled the role of several potential factors that can limit the growth of free-floating plants when co-occurring with rootless submerged vegetation. While laboratory experiments pointed to a potentially strong influence of high pH and P, N or Fe deficiencies, field data revealed that P and Fe deficiencies were unlikely due to high concentrations in the water among dense stands of the rootless submerged plant Ceratophyllum demersum. Consequently, a high pH (10) and low N concentrations in water containing the rootless submerged Ceratophyllum demersum were indicated as key factors in their growth-inhibiting effect on Lemna gibba. Deficiencies in Mn played a lower role and allelopathy could be ruled out.
Nutrient deficiency inhibiting the growth of free-floating plants
Ceratophyllum plants did reduce the concentrations of several nutrients (N, P, Fe, Mn) in the growth medium, but also alkalized the pH to high values (10.2 - 10.5). Low nutrient concentrations were probably not only caused by the uptake of macrophytes but also by their epiphytes (Koleszár et al., 2022). The reduced growth of floating plants in nutrient deficient media with high pH was relaxed after replacing nutrients and neutralizing the pH. Nutrient removal and high pH are thus explaining the inhibitory effect of rootless submerged plants on free-floating plants. Ceratophyllum and its epiphyton showed dissolved inorganic P- and N-uptake rates of 50 and 860 µg g-1 fresh weight day-1, respectively, during the first 4 days. These values were higher than those measured for rooted submerged macrophyte species such as Myriophyllum spicatum (Hilt and Lombardo, 2010). Even higher P uptake rates of up to 1 mg g-1 fresh weight day-1 have been measured for C. demersum during the first day, but declined exponentially with time (Lombardo and Cooke, 2003). Fast nutrient uptake by rootless submerged Ceratophyllum decreased inorganic N and P concentrations in the water below optimal levels for duckweed (Oscarson et al., 1989; Giblin et al., 2014; Petersen et al., 2021). Accordingly, the tissue N concentration in Lemna was reduced far below the range (22 - 38 mg N g–1) considered optimal for duckweed (Oscarson et al., 1989; Vermaat and Hanif, 1998). Low tissue N concentrations in the nutrient deficient Lemna cultures support the notion that N limitation was responsible for low growth rates (Oscarson et al., 1989; Demars and Edwards, 2007). In total nutrient depleted cultures, altough the tissue P concentration of Lemna was also quite low (13.0 mg P g-1), yet the plants were rather N than P limited, since their N:P ratio was still below the threshold indicating P limitation (25; Giblin et al., 2014). Iron concentrations in the Fe-deficient medium were lower than the minimal amount (0.028 mg L-1) reported for duckweed growth (Eyster, 1966 in Landolt and Kandeler, 1987). An allelopathic influence on Lemna was ruled out because Ceratophyllum treated media with total nutrient supplement did not significantly influence Lemna growth.
High pH limiting the growth of Lemna
Under intensive underwater photosynthesis, hydrogen carbonate is taken up and Ceratophyllum plants alkalize the water by a release of OH- ions (Pedersen et al., 2013). The resulting pH in our experiment was much higher than the range (pH 5 - 9) for optimal growth of Lemna species (McLay, 1976). This inhibitory mechanism may not only play a role in aquarium experiments (Szabó et al., 2005; Szabó et al., 2010; Szabó et al., 2022), but also under field conditions, because elevated pH values (>10) have frequently been found above dense stands of submerged vegetation (Frodge et al., 1990; Spencer et al., 1994; Stiers et al., 2011) and in Ceratophyllum stands in our field survey. Laboratory experiments suggest that alkaline pH (9.8 - 10.78) above dense stands of Ceratophyllum may be sufficient to completely inhibit the growth of floating plants. It is well known that alkaline pH does not only reduce the bioavailability of anions (ie. NO3-, PO43-) (Loeppert et al., 1977; Ullrich-Eberius et al., 1981), but also leads to precipitation of phosphate (Otsuki and Wetzel, 1972), Fe and Mn (Stumm et al., 1960; Stumm and Morgan, 1995). In our experiments, high pH treatments resulted in reduced growth and lowered tissue N, P and Mn concentration and higher DMC in Lemna. Consequently, the higher pH treatment included multiple, potentially interactive factors and disentangling the impact of alkalinity alone was not possible.
Differences among the impact of factors
Among potentially limiting factors, alkaline pH (84%) was the strongest, followed by P deficiency (81%), N deficiency (77%), and Fe deficiency (66%) based on biomass data of Lemna. Total chl content of Lemna was an even more sensitive indicator of limitation than growth rate implying that dense Ceratophyllum vegetation may eventually hinder Lemna growth completely. Each of these factors may intensify the negative effect of submerged plants on floating plants, increasing the chance for the occurrence of a hysteresis and thus alternative stable states (Scheffer et al., 2003; Scheffer and Van Nes, 2007).
Our experiments may overestimate the strength of the inhibitory effects, since many naturally occurring buffer mechanisms were excluded. Under field conditions, nutrient deficiency may be weakened by nutrient release from sediments, water movements or plant decomposition at the end of the growing season. Furthermore, top-down control of epiphytic algae by grazing snails may also affect the inhibitory effects (Yang et al., 2020; Koleszár et al., 2022). However, water chemistry data of the field survey revealed that above dense Ceratophyllum vegetation, P and Fe concentrations were sufficiently high, but dissolved inorganic N and Mn concentrations and pH were not in the range for optimal growth of Lemna. Experimental results nonetheless did not support that Mn could be a potential limiting factor. Consequently, low N levels and alkaline pH values generated by dense Ceratophyllum stands are suggested as key factors sustaining the stable dominance of rootless submerged vegetation against floating plants.
Implications
A recent meta-analysis indicated that more than two thirds of aquatic freshwater ecosystems suffer from high nutrient loading (Nõges et al., 2016). Annual N flow to rivers is expected to increase rapidly from 64 Tg N yr−1 in 2000 to 84 Tg in 2050 (Bouwman et al., 2013), and N loading to freshwaters has increased relative to P (Peñuelas et al., 2013). Our finding that low N concentrations are a key factor for sustaining the stable dominance of rootless submerged vegetation against free-floating plants imply that higher N loading in the future increases the chance of regime shifts to a dominance of free-floating plants. This stable vegetation state is associated with significantly fewer ecosystem services (Janssen et al., 2021). Lowering N loading, which has been suggested to induce summer N limitation and control cyanobacterial blooms in polymictic lakes (Shatwell and Köhler, 2019), may thus also be an appropriate restoration measure for water bodies suffering from a dominance of free-floating macrophytes.
Data availability statement
The raw data supporting the conclusions of this article will be made available by the authors, without undue reservation.
Author contributions
SS, GK and GZ designed and performed the experiments. GK, GZ, PN and MB were responsible for chemical analytics. SS, GK and SH analyzed the data and wrote the manuscript. All authors contributed to the article and approved the submitted version.
Funding
This study was financed by the Scientific Board of University of Nyíregyháza. GZ and GK received funding from the Doctoral School of Biological Sciences of the Hungarian University of Agriculture and Life Sciences.
Conflict of interest
The authors declare that the research was conducted in the absence of any commercial or financial relationships that could be construed as a potential conflict of interest.
Publisher’s note
All claims expressed in this article are solely those of the authors and do not necessarily represent those of their affiliated organizations, or those of the publisher, the editors and the reviewers. Any product that may be evaluated in this article, or claim that may be made by its manufacturer, is not guaranteed or endorsed by the publisher.
References
Barko, J. W., Smart, R. M. (1985). Laboratory culture of submerged freshwater macrophytes on natural sediments. Aquat. Bot. 21, 251–263. doi: 10.1016/0304-3770(85)90053-1
Birk, S., Willby, N. (2010). Towards harmonization of ecological quality classification: establishing common grounds in European macrophyte assessment for rivers. Hydrobiologia 652, 149–163. doi: 10.1007/s10750-010-0327-3
Bornette, G., Puijalon, S. (2011). Response of aquatic plants to abiotic factors: a review. Aquat. Sci. 73, 1–14. doi: 10.1007/s00027-010-0162-7
Bouwman, A. F., Beusen, A. H. W., Griffioen, J., Van Groenigen, J. W., Hefting, M. M., Oenema, O., et al. (2013). Global trends and uncertainties in terrestrial denitrification and N2O emissions. Phil. Trans. R. Soc B 368, 20130112. doi: 10.1098/rstb.2013.0112
Demars, B. O. L., Edwards, A. C. (2007). Tissue nutrient concentrations in freshwater aquatic macrophytes: High inter-taxon differences and low phenotypic response to nutrient supply. Freshw. Biol. 52, 2073–2086. doi: 10.1111/j.1365-2427.2007.01817.x
Feuchtmayr, H., Moran, R., Hatton, K., Connor, L., Heyes, T., Moss, B., et al. (2009). Global warming and eutrophication: Effects on water chemistry and autotrophic communities in experimental hypertrophic shallow lake mesocosms. J. Appl. Ecol. 46, 713–723. doi: 10.1111/j.1365-2664.2009.01644.x
Frodge, J. D., Thomas, G. L., Pauley, G. B. (1990). Effects of canopy formation by floating and submergent aquatic macrophytes on the water quality of two shallow pacific Northwest lakes. Aquat. Bot. 38, 231–248. doi: 10.1016/0304-3770(90)90008-9
Giblin, S. M., Houser, J. N., Sullivan, J. F., Langrehr, H. A., Rogala, J. T., Campbell, B. D. (2014). Thresholds in the response of free-floating plant abundance to variation in hydraulic connectivity, nutrients, and macrophyte abundance in a large floodplain river. Wetlands 34, 413–425. doi: 10.1007/s13157-013-0508-8
Hilt, S., Alirangues Nunez, M. M., Bakker, E. S., Blindow, I., Davidson, T., Gillefalk, M., et al. (2018). Response of submerged macrophytes to external and internal restoration measures of temperate shallow lakes. Front. Plant Sci. 9, 194. doi: 10.3389/fpls.2018.00194
Hilt, S., Gross, E. M. (2008). Can allelopathically active submerged macrophytes stabilize clear-water states in shallow lakes? Bas. Appl. Ecol. 9, 422–432. doi: 10.1016/j.baae.2007.04.003
Hilt, S., Lombardo, P. (2010). Effects of macrophytes on phytoplankton: nutrient uptake versus allelopathy. Verh. Internat. Verein. Limnol. 30, 1317–1320. doi: 10.1080/03680770.2009.11902323
Janssen, A. B. G., Hilt, S., Kosten, S., de Klein, J. J. M., Paerl, H., Van de Waal, D. B. (2021). Shifting states, shifting services: Impacts of eutrophication on water quality and ecosystem services. Freshw. Biol. 66, 1–12. doi: 10.1111/fwb.13582
Kazanjian, G., Flury, S., Attermeyer, K., Kalettka, T., Kleeberg, A., Premke, K., et al. (2018). Primary production in nutrient-rich kettle holes and consequences for sediment deposition and carbon mineralization. Hydrobiologia 806, 77–93. doi: 10.1007/s10750-017-3337-6
Koleszár, G., Nagy, Z., Peeters, E. T. H. M., Borics, G., Várbíró, G., Birk, S., et al. (2022). The role of epiphytic algae and grazing snails in stable states of submerged and of free-floating plants. Ecosystems 25, 1371–1383. doi: 10.1007/s10021-021-00721-w
Landolt, E., Kandeler, R. (1987). The family of Lemnaceae - a monographic study (vol. 2). Veröff Geobot. Inst. ETH Stiftung Rübel Zürich 95, 638.
Larson, D. (2007). Growth of three submerged plants below different densities of nymphoides peltata (S. g. gmel.) kuntze. Aquat. Bot. 86, 280–284. doi: 10.1016/j.aquabot.2006.10.007
Le Bagousse-Pinguet, Y., Gross, E. M., Straile, D. (2012). Release from competition and protection determine the outcome of plant interactions along a grazing gradient. Oikos 121, 95–101. doi: 10.1111/j.1600-0706.2011.19778.x
Loeppert, H., Kronberger, W., Kandeler, R. (1977). “Correlation between nitrate uptake and alkalinisation by lemna paucicostata,” in Transmembrane ionic exchanges in plants. Eds. Thellier, M., Monnier, A., Demarty, M. (Paris et Mount Saint Aignan: CNRS et Université Rouen), pp 283–pp 288.
Lombardo, P. (2003). Ceratophyllum demersum – phosphorus interactions in nutrient enriched aquaria. Hydrobiologia 497, 79–90. doi: 10.1023/A:1025461604808
Lu, J., Wang, Z., Xing, W., Liu, G. (2013). Effects of substrate and shading on the growth of two submerged macrophytes. Hydrobiologia 700, 157–167. doi: 10.1007/s10750-012-1227-5
McLay, C. L. (1976). The effect of pH on the population growth of three species of duckweed: Spirodela oligorrhiza, Lemna minor and Wolffia arrhiza. freshw. Biol 6, 125–136. doi: 10.1111/j.1365-2427.1976.tb01596.x
Morris, K., Bailey, P. C., Boon, P. I., Hughes, L. (2003). Alternative stable states in the aquatic vegetation of shallow urban lakes. II. catastrophic loss of aquatic plants consequent to nutrient enrichment. Mar. Freshw. Res. 54, 201–215. doi: 10.1071/MF02003
MSZ 1484-13 (2009). Hungarian Standard. spectrophotometric determination of nitrate ion (Budapest, Hungary: Hungarian Standard Institution).
MSZ EN ISO 6878 (2004). Hungarian Standard. water quality. determination of phosphorus. ammonium molybdate spectrometric method.
MSZ ISO 7150-1 (1992). Hungarian Standard. determination of ammonium in water. manual spectrophotometric method. (Budapest, Hungary: Hungarian Standard Institution)
Nõges, P., Argillier, C., Borja, Á., Garmendia, J. M., Hanganu, J., Kodeš, V., et al. (2016). Quantified biotic and abiotic responses to multiple stress in freshwater, marine and ground waters. Sci. Total Environ. 540, 43–52. doi: 10.1016/j.scitotenv.2015.06.045
Oscarson, P., Ingemarsson, B., Larsson, C. M. (1989). Growth and nitrate uptake properties of plants grown at different relative rates of nitrogen supply. i. growth of Pisum and Lemna in relation to nitrogen. Plant Cell Environ. 12, 779–785. doi: 10.1111/j.1365-3040.1989.tb01639.x
Otsuki, A., Wetzel, R. G. (1972). Coprecipitation of phosphates with carbonates in marl lake. Limnol. Oceanogr. 17, 763–767. doi: 10.4319/lo.1972.17.5.0763
Pedersen, O., Colmer, T. D., Sand-Jensen, K. (2013). Underwater photosynthesis of submerged plants – recent advances and methods. Front. Plant Sci. 4, 140. doi: 10.3389/fpls.2013.00140
Peñuelas, J., Poulter, B., Sardans, J., Ciais, P., van der Velde, M., Bopp, L., et al. (2013). Human-induced nitrogen–phosphorus imbalances alter natural and managed ecosystems across the globe. Nat. Commun. 4, 2934. doi: 10.1038/ncomms3934
Petersen, F., Demann, J., Restemeyer, D., Ulbrich, A., Olfs, H. W., Westendarp, H., et al. (2021). Influence of the nitrate-n to ammonium-n ratio on relative growth rate and crude protein content in the duckweeds Lemna minor and Wolffiella hyalina. Plants 10, 1741. doi: 10.3390/plants10081741
Scheffer, M., Hosper, S. H., Meijer, M. L., Moss, B., Jeppesen, E. (1993). Alternative equilibria in shallow lakes. TREE 8, 275–279. doi: 10.1016/0169-5347(93)90254-M
Scheffer, M., Szabó, S., Gragnani, A., Van Nes, E. H., Rinaldi, S., Kautsky, N., et al. (2003). Floating plant dominance as a stable state. PNAS 100, 4040–4045. doi: 10.1073/pnas.0737918100
Scheffer, M., Van Nes, E. H. (2007). Shallow lakes theory revisited: various alternative regimes driven by climate, nutrients, depth and lake size. Hydrobiologia 584, 455–466. doi: 10.1007/s10750-007-0616-7
Shatwell, T., Köhler, J. (2019). Decreased nitrogen loading controls summer cyanobacterial blooms without promoting nitrogen-fixing taxa: Long-term response of a shallow lake. Limnol .Oceanogr. 64, S166–S178. doi: 10.1002/lno.11002
Smith, S. D. D. (2014). The role of nitrogen and phosphorus in regulating the dominance of floating and submerged aquatic plants in field mesocosm experiment. Aquat Bot. 112, 1–9. doi: 10.1016/j.aquabot.2013.07.001
Spencer, W. E., Terri, J., Wetzel, R. G. (1994). Acclimation of photosynthetic phenotype to environmental heterogeneity. Ecology 75, 301–314. doi: 10.2307/1939536
Stiers, I., Njambuya, J., Triest, L. (2011). Competitive abilities of invasive Lagarosiphon major and native Ceratophyllum demersum in monocultures and mixed cultures in relation to experimental sediment dredging. Aquat. Bot. 95, 161–166. doi: 10.1016/j.aquabot.2011.05.011
Stumm, W., Morgan, J. J. (1995). Aquatic chemistry: chemical equilibria and rates in natural waters. 3rd edition (New York: J. Wiley & Sons), 1040 pp.
Szabó, S., Koleszár, G., Braun, M., Nagy, Z., Vicei, T. T., Peeters, E. T. H. M. (2022). Submerged rootless macrophytes sustain stable state against free-floating plants. Ecosystems 25, 17–29. doi: 10.1007/s10021-021-00637-5
Szabó, S., Peeters, E, T. H. M., Borics, G., Veres, S., Nagy, P. T., Lukacs, B. A. (2020). The ecophysiological response of two invasive submerged plants to light and nitrogen. Front. Plant Sci. 10, 1747. doi: 10.3389/fpls.2019.01747
Szabó, S., Roijackers, R. M. M., Scheffer, M. (2003). A simple method for analysing the effects of algae on the growth of Lemna and preventing algal growth in duckweed bioassays. Arch. Hydrobiol. 157, 567–575. doi: 10.1127/0003-9136/2003/0157-0567
Szabó, S., Roijackers, R. M. M., Scheffer, M., Borics, G. (2005). The strength of limiting factors for duckweed during algal competition. Arch. Hydrobiol 164, 127–140. doi: 10.1127/0003-9136/2005/0164-0127
Szabó, S., Scheffer, M., Roijackers, R., Waluto, B., Braun, M., Nagy, P., et al. (2010). Strong growth limitation of a floating plant (Lemna gibba) by submerged macrophyte (Elodea nuttallii) under laboratory conditions. Freshw. Biol. 55, 681–690. doi: 10.1111/j.1365-2427.2009.02308.x
Ullrich-Eberius, C. I., Novacky, A., Fisher, E., Luettge, U. (1981). Relationship between energy-dependent phosphate uptake and the electrical membrane potential in Lemna gibba G1. Plant Physiol. 67, 797–801. doi: 10.1104/pp.67.4.797
Van Gerven, L. P. A., De Klein, J. J. M., Gerla, D. J., Kooi, B. W., Kuiper, J. J., Mooij, W. M. (2015). Competition for light and nutrients in layered communities of aquatic plants. Am. Nat. 186, 72–83. doi: 10.1086/681620
Van Zuidam, J. P., Peeters, E. T. H. M. (2013). Occurrence of macrophyte monocultures in drainage ditches relates to phosphorus in both sediment and water. SpringerPlus 2, 564. doi: 10.1186/2193-1801-2-564
Vermaat, J. E., Hanif, K. M. (1998). Performance of common duckweed species (Lemnaceae) and waterfern Azolla filiculoides on different types of waste water. Wat. Res. 32, 2569–2576. doi: 10.1016/S0043-1354(98)00037-2
Yang, L., He, H., Guan, B., Yu, J., Yao, Z., Zhen, W., et al. (2020). Mesocosm experiment reveals a strong positive effect of snail presence on macrophyte growth, resulting from control of epiphyton and nuisance filamentous algae: Implications for shallow lake management. Sci. Total Environ. 705, 135958. doi: 10.1016/j.scitotenv.2019.135958
Keywords: alternative stable states, Lemna, multiple stressors, nitrogen, nutrients, pH
Citation: Szabó S, Koleszár G, Zavanyi G, Nagy PT, Braun M and Hilt S (2022) Disentangling the mechanisms sustaining a stable state of submerged macrophyte dominance against free-floating competitors. Front. Plant Sci. 13:963579. doi: 10.3389/fpls.2022.963579
Received: 20 August 2022; Accepted: 17 October 2022;
Published: 31 October 2022.
Edited by:
Xinsheng Chen, Anhui University, ChinaReviewed by:
Yao-Bin Song, Hangzhou Normal University, ChinaBeibei Hao, Guangdong Academy of Sciences, China
Copyright © 2022 Szabó, Koleszár, Zavanyi, Nagy, Braun and Hilt. This is an open-access article distributed under the terms of the Creative Commons Attribution License (CC BY). The use, distribution or reproduction in other forums is permitted, provided the original author(s) and the copyright owner(s) are credited and that the original publication in this journal is cited, in accordance with accepted academic practice. No use, distribution or reproduction is permitted which does not comply with these terms.
*Correspondence: Sabine Hilt, c2FiaW5lLmhpbHRAaWdiLWJlcmxpbi5kZQ==; Sándor Szabó, ZHJzemFib3M4QGdtYWlsLmNvbQ==