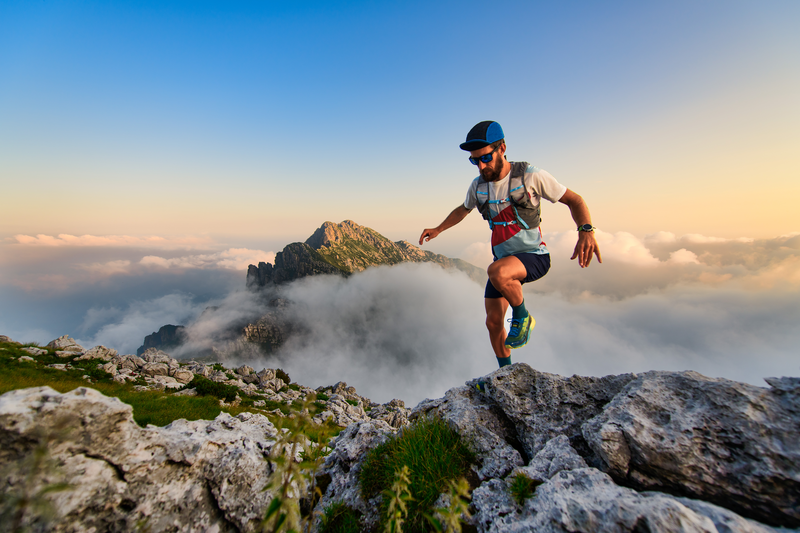
94% of researchers rate our articles as excellent or good
Learn more about the work of our research integrity team to safeguard the quality of each article we publish.
Find out more
ORIGINAL RESEARCH article
Front. Plant Sci. , 07 July 2022
Sec. Functional Plant Ecology
Volume 13 - 2022 | https://doi.org/10.3389/fpls.2022.955656
Natural ecosystems generally include litter decomposition as part of the natural cycle since the material properties and the environment greatly influence the decomposition rate. The invasion of exotic plants alters the species diversity and growth characteristics of plant communities, but its impact on litter decomposition is unknown in the riparian zone. This study examines how invasive plants affect the early stages of litter decomposition and how species richness impacts them. This experiment involved a random litter mixture of exotic (Alternanthera philoxeroides and Bidens pilosa) and native species in the riparian zone of the Three Gorges Dam Reservoir in China. There were 43 species mixture types, with various species richness ranging from 1 to 6. Litterbags were placed in the hydro-fluctuation zone and terrestrial zone, where they decomposed over the course of 55 days. Invasive plants decompose rapidly compared to native plants (35.71% of the remaining mass of the invasive plant). The invasive plant A. philoxeroides has the potential to accelerate native plant decomposition (0.29 of non-added synergetic effect), but Bidens pilosa cannot. Nonetheless, species richness had little effect on the decomposition rate. These effects are dependent upon differences in chemical functional characteristics among the species. The initial traits of the plants, specifically C, N, and C/N, were significantly and linearly correlated with the loss of mixed litter mass and mixing effect strength (P < 0.01). In addition, submergence decomposition conditions reduce the disturbance of invasive plants and predict decomposition rates based on litter characteristics. Invasive plants can therefore impact the material cycle of an ecosystem. There is a need to examine decomposition time, which may also involve considering other factors.
Litter decomposition plays a crucial role in determining ecosystem function (Chen et al., 2021; Zhang et al., 2021). It is an important link in the material cycle and nutrient flow that can continuously provide resources to the soil and plants (Scheffer and Aerts, 2000; Zhang et al., 2020). There is a rapid mass loss rate and nutrient release that increases the resource-use efficiency of the environment in the early decomposition stage (Zhou et al., 2021). The litter decomposition process is generally regulated by climatic patterns, soil nutrients, decomposer assemblages, and litter properties (Yelenik et al., 2007). Litter decomposition is considered the more important control factor. It has been shown that invasive plants decompose more rapidly due to their nitrogen-rich nature (Gao et al., 2016; Yang et al., 2022). While climate and environmental change caused the exotic species to invade, the variances in growth traits between exotic and native plants led to the invasive plant's entry into the plant community, altering the mean plant community traits, which may impact the litter decomposition rates (Ashton et al., 2005; Leffler et al., 2014). Invading plants typically have higher N content than native plants, and invertebrates and microorganisms favor a lower C/N proportion than native plants and the characteristic. Because of this, the plant litter from invasive plants is likely to break down more quickly. This quickly releases nitrogen and other nutrients into the soil, which changes the environment for the plants that have been taken over (Poulette and Arthur, 2012).
Due to the long-term coexistence of exotic plants and native plants, exotic plants will inevitably enter the native plant's community (Hamid et al., 2020). The effect of changing the plant community means accelerating or slowing down the decomposition rate of the litter mixture (Zhang et al., 2014). Many studies refer to the acceleration or slowing down of the litter mixture decomposition rate as a non-additive effect (Dai et al., 2020). The introduction of exotic plants into the native plant community has accelerated the mass loss and release of nitrogen from native plant litter (Liu et al., 2020). According to the nutrient transfer theory, material components and nutrient elements are transferred between different species during the litter mixture decomposition process. Due to the action of invertebrates and the formation of mycelium between the litter by fungal microorganisms, nutrients are transferred from high-quality to low-quality litter, thus increasing the nutrient content of low-quality litter and attracting more decomposing microorganisms to settle (Lopes et al., 2011; Purahong et al., 2016; Bani et al., 2018). Also, because invasive plants release secondary metabolites like polyphenols, the rate of litter decomposition can be slowed even if invasive plants break down faster than native plants (Hättenschwiler and Vitousek, 2000).
It is clear that invasive species change the average characteristics of a plant community, and scientists have found that invasive species fill niches in a plant community, which changes the number of species (Dawson et al., 2015). Species richness determines the structure and function of ecosystems, and it may predict the ecosystem material cycles (Wardle et al., 1997; Behzad et al., 2022). As species richness increases, the functional diversity of litter becomes complex, the decomposition microenvironment changes (Hector et al., 2000; Gul et al., 2022), and the decomposer community species diversity may subsequently change to affect decomposition rates (Lecerf et al., 2007). It has been shown that the reduction in plant litter and decomposer diversity slows down carbon and nitrogen cycling, and the main causes are litter quality and environmental (Wu et al., 2013; Handa et al., 2014). Therefore, we expected that the addition of invasive plants to the plant community, which have different traits than native plants, would impact litter decomposition.
Current studies on the relationship between exotic plant invasion and species diversity focus more on primary productivity and growth traits. A strong negative correlation between plant diversity and invasive plant performance indicates that high species diversity can resist exotic plant invasion (Naeem et al., 2000). As increased native species diversity alters the competitive environment in plants, it reduces the effect of light, decreases the effect of nutrients, and inhibits the growth of exotic species. However, when plants decay into litter after they die off, invasive species can rapidly decompose and release nitrogen nutrients, increasing soil resource use efficiency and causing more species invasion, achieving positive feedback regulation (Prescott and Zukswert, 2016). Moreover, mixing exotic plants with native plants accelerates the native plant's decomposition and nutrient release rate (Chen et al., 2013; Schuster and Dukes, 2014). Thus, the interconnection between native and invasive plants is more complicated. Most studies were based on exotic and native species mixtures to observe the results of mixed litter decomposition. There were few responses on the effect of the invasive plants on the native plant's litter decomposition in terms of biodiversity. Therefore, this research aimed to determine the effect of invasive species on litter decomposition at different species richness.
Ecosystems on land and in water are connected by the riparian zone. Moreover, it connects habitats with diverse forms of life and provides important ecosystem services (Ding et al., 2021; Zheng et al., 2021a; Arif et al., 2022a). Three Gorges Dam Reservoir (TGDR) is in a fragile ecological ecotone due to the hydro-fluctuation, leading to exotic plant invasion, which may affect the native plant's decomposition (Xiao et al., 2017; Li et al., 2021). In the TGDR, Alternanthera philoxeroides and Bidens pilosa are the main invasive species, and their invasion changes the species composition and community structure (Lee et al., 2014). Due to differences in species growth traits, there may be deviations in the response of the abundant native plants to invasive plants in the TGDR zone. The role of invasive plants may deviate from that of different species' richness. The following scientific hypotheses are presented in this study: (i) invading plants can accelerate litter decomposition in the TGDR zone under submergence conditions; (ii) species diversity can mitigate the impact of invasive plants on litter decomposition; and (iii) there can be a relationship between litter traits and decomposition rates. We conducted this study to determine how invasive plants affect litter decomposition and how long it takes for mixed litter to decompose.
The experimental site was located in the Ruxi River basin, Shibao Town, Zhongxian County, Chongqing City, TGDR (30°36′ N, 108°06′ E). The TGDR is the largest reservoir in China, located between Jiangjin in Chongqing and Yichang in Hubei, with an area of 349 km2. The TGDR implements a hydrological dispatching model of winter storage and summer discharge, creating a hydro-fluctuation zone with a water level difference of up to 30 m during 1 year (Sang et al., 2019; Arif et al., 2022b). The site is part of the subtropical southeast monsoon region's mountainous climate, with an average annual accumulated temperature of 18.2°C, long sunshine hours, sufficient rainfall, 1,327.5 h of sunshine, 29% sunshine rate, rainfall mostly concentrated between June and August, with an annual rainfall of 1,200 mm and relative humidity of 80 %. Herbaceous plants dominate the reservoir area. This area's soil is designated as purple soil (Regosols in FAO Taxonomy or Entisols in USDA Taxonomy) (Chen et al., 2020; He et al., 2021; Hu et al., 2021).
There are abundant herbaceous plants in the TGDR. We selected 6 native and 2 invasive plants with high dominance, high cover, and wide distribution as experimental species based on previous studies on the vegetation distribution in the TGDR (Table 1). These species include both annual and perennial herbaceous plants with a predominance of graminoid herbs, which is consistent with the vegetation in the water-level-fluctuation zone of TGDR. The two invasive plants were chosen to analyze the importance of species traits because variations in species traits may have different effects on litter decomposition rates.
Table 1. An overview of selected species used in the riparian zone of the Three Gorges Reservoir, China.
The above-ground parts (stems and leaves) of eight herbaceous plants were collected from experimental site area in 2021. Experimental material was frozen and quickly brought back to the laboratory, chopped into 10 cm-sized pieces, and dried to a constant weight of 80°C.
Litterbags contained one of 43 mixture types (or combinations). This litter mixture type includes four species numbers. Species richness 1 is 8 monocultures, and 2, 4, and 6 species mixture litterbags include 3 classifications: (1) Combination of native and native species: native-group. We randomly selected five 2-species mixtures from all possible 2-native species combinations in the 6-native species pool. The selection method for the 4-species mixture is the same as above. The 6-species mixture was not selected because it includes all species. (2) Combination of native and invasive species A. philoxeroides: Ap-group. Six 2-species mixtures of Ap-group are all possible combinations of the 6-native species and the A. philoxeroides pool. To study the impact of invasive plants on litter mixture and the possibility of comparing native-group and AP-group without changing species richness, we substituted A. philoxeroides randomly from one native species of the selected native-group. (3) Combination of native and invasive species B. pilosa: Bp-group. To study the possible effects of different species litter mixtures, although they are both invasive plants, 2, 4, and 6-species mixtures of the Bp-group, we replaced B. pilosa with A. philoxeroides of the Ap-group. The number of species combinations is shown in Table 2. According to the combination design, the sample dried to constant weight was packed evenly weighted into nylon decomposition bags (15 × 20 cm, aperture 1 × 1 mm). Each decomposition bag was filled with a total of 6.0 g of sample (e. g., 2-species mixture litterbags contained 3.0 g of litter from each species and 4-species mixture litterbags contained 1.5 g of litter from each species). We set up 6 replicates of each mixture type, and 258 litterbags were prepared.
Table 2. The number of species combinations used in the riparian zone of the Three Gorges Reservoir in China.
We selected an experimental transect in the water-level-fluctuation zone of the TGDR, which is perpendicular to the river and has rich vegetation on the ground. We selected two sample points in the hydro-fluctuation zone (S) and terrestrial zone (CK) as experimental points in the transect. The highest level of water in the TGDR was 175 m. Our experimental points in the S and CK were, respectively, set at 170–175 m and 175–180 m. Although the water level fluctuated slightly during the experiment, it always flooded the litter bags at the experimental points. We cleared each experimental point's surface vegetation and debris and formed a 2 × 3 m experimental plot. Before the water level rose, the litterbags were carefully placed at the two experimental points to ensure that the arrangement between the decomposition bags was close and not overlapping. Start the experiment when the water level has raised and soaked the litterbags at S. The litterbags at S were flooded for a period of time. When the water level declined and the litterbags were exposed, all the litterbags at the two experimental plots were brought back to the laboratory (each litterbag was packed independently in a valve bag).
The decomposition experiment started in October 2021, and the decomposition bags were retrieved after the water level dropped, decomposing for 55 days. After bringing the decomposition bags back to the laboratory, the surface contaminants were carefully removed. The impurities were rinsed three times with ultrapure water after being progressively rinsed with tap water. The washed litter was placed in weighted and marked envelopes, dried to a constant weight in an oven at 80°C, and then weighed. The initial samples were pulverized in a ball mill, passed through a 0.25 mm sieve, and used for chemical composition determination.
The initial C and N contents were determined using an elemental analyzer (CHNS-O-Vario EL Cude; Heraeus Elementar, Hanau, Germany), and Ca, Mg, and P were first digested by microwave digestion (SpeedWave MWS-4) and then determined by inductively coupled plasma emission spectrometry (ICP-OES, Thermo Fisher Scientific iCAP 6300).
The formula for calculating the remaining rate based on mass is as follows (Voříšková and Baldrian, 2013):
Where M0 denotes the sample's initial mass; MT denotes the sample's weight after the time T.
The mixing effect intensity of litter decomposition is estimated by comparing the expected value with the actual value of litter mass loss. According to the mixing effect intensity, we can infer what kind of mixing effect (additive effect, synergistic effect, or antagonistic effect) exists in the litter mixture decomposition process. The expected decomposition rate of mixed litter is calculated from the actual decomposition rate of individual species under the summation effect and is calculated as follows (Lecerf et al., 2007):
where E is the remaining mass expectation value for litter decomposition for different litter mixtures. DMi is the initial mass of species i; Oi is the measured remaining mass rate of species i alone.
The mixing effect intensity of litter decomposition was calculated from the actual observed and expected values of litter decomposition rate by the following equation (Chen et al., 2013):
For each of these chemical features, we estimated functional trait identity and diversity for all litter mixture elements. The abundance-weighted mean of the trait value across all constituent species in a community, known as the community-weighted mean (CWM), is used to demonstrate community-level trait identity for a given trait and community. Because all species were represented in equal abundance, CWM equaled the mean in this situation. For all features across all communities, we estimated functional dispersion, a marker of functional diversity. The functional dispersion for one trait is highest when species are maximally dissimilar for this trait (Laliberte and Legendre, 2010). The following equation calculated the community weighted mean (CWM) and functional dispersion (FDis) of the litter mixture (Grossman et al., 2020):
Where Pi is the relative richness of species i, ti is the trait value of species i, and S is the number of species in the community.
Where c is the weighted centroid in the j-dimensional space, ai is the abundance of species i, xij is the attribute of species i for trait j, and zi is the distance of species i to the weighted centroid c.
The data was analyzed using SPSS 22 (IBM, Chicago, USA) and Microsoft Excel. ANOVA was used to analyze the effect of species richness, environment, and invasive plants on litter mass loss (α = 0.05). Paired t-tests were used to test the variation between expected and observed values for litter decomposition and monoculture remaining mass difference in two environments (α = 0.05). Linear correlation was used to investigate the link between the CWM and FDis of litter mixture with the mass loss and the mean mixing effect intensity. R was estimated for CWM and FDis. OriginPro 2022 (Northampton, MA, USA) was used for mapping.
The litter's remaining mass rates were significantly affected by the species, submergence environment, and their interactions (P < 0.001). According to the individual species' litter remaining mass rate (Figure 1), the litter remaining mass at the CK and S were not significantly different, except for P. lapathifolium. X. sibiricum, C. dactylon, and P. lapathifolium decomposed more slowly, and the remaining mass rate at S was >60%, among which P. lapathifolium had the highest remaining mass. The remaining mass rate of P. alkekengi, A. philoxeroides, and B. pilosa was lower, at about 35% in both environments. According to the litter's initial elemental content (Table 3), the C content of all species was not very different, but the N element content of A. philoxeroides, and B. pilosa was high, up to 33.1 mg·g−1. Alternanthera philoxeroides had a lower C/N of 12.1 compared to other species. The N, P, Ca, and Mg element content of P. alkekengi was higher than that of the native species. The two-way ANOVA results for the litter remaining mass rates of the eight individual species are shown in Table 4.
Figure 1. Bar charts show individual species' mass remaining litter rates within the hydro-fluctuation zone (S) and the terrestrial zone (CK). The y-axis shows the mean value (%). The vertical whisker represents the standard deviation. Paired tests were used to determine the differences between S and CK. *Indicates significant differences at P < 0.05.
Table 3. The initial elemental content of litter of selected species in the riparian zone of the Three Gorges Reservoir in China.
Table 4. Two-way ANOVA results indicate the loss of litter mass of individual species in the riparian zone of the Three Gorges Reservoir in China.
According to the mean mixing effect intensity of litter decomposition (Figure 2), it can be seen that the litter decomposition mixing effect in submergence and terrestrial environments is similar, but the impact degree is different. The Ap-group's mean mixing effect intensity value is >0, indicating that A. philoxeroides has a non-additive effect on the native plants' litter decomposition. But the effect intensity value in the terrestrial zone is higher than in submergence, indicating that the non-additive effect of A. philoxeroides under terrestrial conditions is greater. The mean effect intensity values for the Bp-group were around 0, indicating that B. pilosa does not have a non-additive effect on the native plants' litter decomposition.
Figure 2. The mean mixing effect intensity of litter mixture mass remaining in the hydro-fluctuation zone (A) and terrestrial zone (B). The Ap-group indicates litter mixtures of A. philoxeroides and native species, and the Bp-group represents litter mixtures of B. pilosa and native species. All data are presented as mean ± standard deviation. In the mean mixing effect intensity values, values above 0 indicate synergistic effects, and values below 0 indicate inhibitory effects. Xs, X. sibiricum; Pl, P. lapathifolium; Pa, P. alkekengi; Ha, H. altissima; Cd, C. dactylon; Ds, D. sanguinalis; Ap, A. philoxeroides; Bp, B. pilosa.
On the basis of the three-way ANOVA results (Table 5) and the litter remaining mass rate at different species richness levels (Figure 3), it can be concluded that the submergence environment and invasive species greatly contributed to litter decomposition (P < 0.001). The interaction between species richness and invasive plants significantly affected litter decomposition (P < 0.05). As species richness increased, the difference in remaining mass rate between every litter combination gradually decreased. The extreme difference in individual species' remaining mass rates reached 54.66% in the hydro-fluctuation zone. The extreme difference in mass remaining rate for species richness 2 decreased to 34.24%, followed by 22.32 and 20.84% for species richness 4 and 6, respectively. A similar trend result was shown in the terrestrial zone, with the extreme difference in mass remaining rate from 31.97% for the species richness 1, decreasing gradually to 2.39% for the species richness 6.
Table 5. Three-way ANOVA results for litter mass remaining rates on different species richness in the riparian zone of the Three Gorges Reservoir in China.
Figure 3. The litter remaining mass at different rates for different levels of species richness in the hydro-fluctuation zone (A) and terrestrial zone (B). The Ap-group indicates litter mixtures of A. philoxeroides and native species, and the Bp-group represents litter mixtures of B. pilosa and native species. Data are expressed as mean values.
According to the mean mixing effect intensity for different litter species richness (Figure 4) and the paired t-test results of litter remaining mass rate observed and expected values (Figure 5), the mean mixing effect on different species richness has similar results, and the mixing effect intensity of the AP-group is higher than the 0 value. Still, the effect value gradually increases with the species richness. But the mixing effect intensity values of BP-group litter did not change significantly with the species richness. They were all around the 0 lines, indicating that the effect of the litter decomposition was relatively small. The decomposition environment difference significantly affected the mixing effect, with a significant difference (P < 0.001) between the observed and expected values for the Native-group litter on submergence conditions in the hydro-fluctuation zone but not for the combination mixed with B. pilosa. In contrast, the opposite result existed in the terrestrial zone. In addition, the AP-group litter observed values were significantly different from the expected values in both environments (P < 0.001), indicating that the effect of A. philoxeroides on the litter decomposition was highly significant and synergistic. Overall, the AP-group litter remaining mass rate was lower than the Native-group and BP-group.
Figure 4. The mean mixing effect intensity of litter mixture remaining mass on different species richness in the hydro-fluctuation zone (A) and terrestrial zone (B). The Ap-group indicates litter mixtures of A. philoxeroides and native species, and the Bp-group represents litter mixtures of B. pilosa and native species. Data are expressed as mean values. In the mean mixing effect intensity values, values above 0 indicate synergistic effects, and values below 0 indicate inhibitory effects.
Figure 5. The results of the paired t-test show the litter's remaining mass rate at observed and expected values for the hydro-fluctuation zone (A) and terrestrial zone (B). The Ap-group indicates litter mixtures of A. philoxeroides and native species, and the Bp-group represents litter mixtures of B. pilosa and native species. All data are presented as mean ± standard deviation. A paired t-test was used to test for significant differences between observed and expected values. NS, no significant difference; *P < 0.05; ***P < 0.001.
According to the linear regression relationship between the litter remaining mass rate (Figure 6) and the mixing effect intensity of litter decomposition (Figure 7) with litter CWM, the litter carbon-CWM and nutrient elements CWM have a significant linear relationship with the remaining mass rate at the litter decomposition early stage (P < 0.05). In the terrestrial environment, there was a significant negative correlation between the litter's P, Ca, Mg, and N-CWM and the remaining mass rate (P < 0.05). At the same time, C and C/N-CWM showed a significant positive relationship (P < 0.05). However, the relationship between litter P, Ca, Mg-CWM, and the remaining mass rate was not significant in the submergence environment (P > 0.05), but C, N, and C/N remained significant.
Figure 6. Linear regression relationships (A–F) between litter remaining mass rate and litter community weighted mean traits (CWM). S stands for litter that has been decomposed in the submergence environment, and CK stands for litter that has been decomposed in the terrestrial environment.
Figure 7. Linear regression relationships (A–F) between the mean mixing effect intensity of litter decomposition and community weighted mean traits (CWM) of litter mixture. S stands for litter that has been decomposed in the submergence environment, and CK stands for litter that has been decomposed in the terrestrial environment.
Regarding the mean mixing effect intensity of litter decomposition, there was a highly significant linear correlation between C, N, and C/N-CWM and the mixing effect intensity in the terrestrial environment. The N-CWM showed a positive correlation with the mixing effect intensity (Figure 7D, y = 0.0134–0.119, P < 0.001), and C and C/N-CWM showed a negative correlation with the mixing effect intensity (Figure 7E, y = −0.00432x + 1.862, P < 0.01; Figure 7F, y = −0.00859x + 0.325, P < 0.001). However, there was no such significant linear correlation in the submergence environment (P > 0.05). In addition, there was no significant linear regression between Ca, P, and Mg-CWM and the mixing effect intensity in both environments.
In addition, according to linear regression relationships between litter remaining mass rate (Figure 8) and the mean mixing effect intensity (Figure 9), and litter functional dispersion (FDis), N-FDis was significantly correlated with litter mass loss and litter mixing effect intensity (P < 0.05). This situation exists in both environmental conditions. Ca-FDis was significantly correlated with mass loss (P < 0.05), and C/N-FDis was extremely significantly correlated with decomposition in the terrestrial zone (P < 0.01).
Figure 8. Linear regression relationships between litter remaining mass rate and litter functional dispersion (FDis). S stands for litter that has been decomposed in the submergence environment, and CK stands for litter that has been decomposed in the terrestrial environment.
Figure 9. Linear regression relationships between mean mixing effect intensity of litter decomposition andfunctional dispersion (FDis) of litter mixture. S stands for litter that has been decomposed in the submergence environment, and CK stands for litter that has been decomposed in the terrestrial environment.
Litter decomposition simplifies and slowly releases complex compounds into chemical elements, regulated by the litter quality and environmental conditions (Gessner et al., 1999). Typically, invasive species in a plant community have different chemical functions and traits from native species and may interfere with litter decomposition (Table 3). In this experiment, A. philoxeroides and B. pilosa are both invasive species, but they have different effects on native plant decomposition due to their respective chemical traits. The mixture of A. philoxeroides with native species accelerated mixed litter mass loss, showing a synergistic decomposition effect in submergence and terrestrial environments, which is consistent with hypothesis 1. However, there was no significant difference in litter mass loss from the mixture of B. pilosa and native species compared with the native monoculture, which showed an additive effect and a smaller effect on the native species' decomposition (Figure 2). The reason for A. philoxeroides's accelerated litter decomposition may be the high N elemental content. Studies have shown that the rapid mass loss of invasive plants compared to native plants is related to the N element (Figure 1) (Gartner and Cardon, 2004; Incerti et al., 2018). Invertebrates and microorganisms feed on nutrients to meet growth needs, and decomposers prefer to feed on litter with high N content because N-containing components are a high-quality nutrient, resulting in a faster mass loss rate (Gessner et al., 2010). According to nutrient transfer theory, N and other nutrient elements can be transferred in litter, moving from high-quality to low-quality litter, thus accelerating the decomposition of low-quality litter and overall showing mixed litter rapid decomposition (Maisto et al., 2011). Enríquez showed a general positive linear relationship between litter N and P content and the decomposition rate during litter decomposition due to the high demand for these essential nutrients by decomposing organisms, which rapidly feed on the high-quality litter to meet their needs (Enríquez et al., 1993). Usually, the litter produced by different plant species differs in quality and attractiveness to decomposers, resulting in large differences in the mass and nutrient losses in different species and environments (Grossman et al., 2020).
In the early litter decomposition stage, a faster mass loss and nutrient release rate were strongly correlated with the nutrient content of the plant tissues (Debusk and Reddy, 2005; Zhang et al., 2019). The plant chemical functional traits related to the mixed litter decomposition are consistent with hypothesis 3, but this depends on the differences in material composition between litter species and the environment (Figures 6–9) (Hoorens et al., 2003). The growth traits and material composition of plants are the main characteristics that reflect the litter quality. Chemical elements exist in abundant forms of plant tissues, and the way of releasing chemical elements and the degree of attracting decomposing organisms are the litter decomposition control factors (Zhang et al., 2019). C is present in the plant in carbohydrates and tissues such as cellulose and lignin, which occupy most of the plant mass, so the litter C release rate is equal to the mass loss (Hu et al., 2021). Decomposing organisms prefer high-quality nutrient elements, and decomposing organisms prefer high-nitrogen substances to meet their growth (Wickings et al., 2012). Decomposing organisms have a higher demand for the N element. Therefore, litter with higher N content and lower C/N has a faster mass loss rate. And P is a turnover element in microbial growth and controls the normal growth of microorganisms (Webster and Benfield, 1986). Although the demand for trace elements is not as high as that for massive elements, they also play an indispensable role in regulating plant growth and metabolism, so discussing trace elements effectively predicts decomposition. Higher Ca content may accelerate litter mass loss because Ca is involved in some enzymatic activities and is more attractive to soil organisms (Grossman et al., 2020). Mg is an important component of chlorophyll and plays a key role in the fungi's growth (Cao et al., 2018).
Plant traits are highly correlated with litter mass loss in the mixed litter decomposition of invasive and native plants, but the increase in species richness is less discussed. As species richness increases, the decomposition process also becomes complicated. A complex mixed litter decomposition environment with more microbial species and diversity, higher litter heterotrophic respiration, and more invertebrate fauna may accelerate litter mass loss (Liu et al., 2007; Lecerf et al., 2011). However, the results suggested that species richness did not significantly affect litter decomposition (Smith and Bradford, 2003). However, the interaction between species richness and invasive species still had a significant effect (Table 5). Species richness had an effect in the invasive-native mixed litter but was not found in the native single litter. Several reasons may be for this (Liu et al., 2016): (1) Chemical traits do not differ significantly among native species. The main driver of mixture decomposition is still the average decomposability of component species (Figures 6, 8) (Harguindeguy et al., 2008). The chemical traits of native plants selected for this experiment did not differ significantly (mainly for N elements), resulting in essentially the likely initial traits of the native-native mixed litter at different species richness, showing no significant effect. Invasive plants significantly changed the mean chemical traits of mixed litter, so invasive plants significantly affected litter decomposition in every species richness. (2) This study focused on the rapid litter decomposition stage. Litter releases nutrients rapidly and is more susceptible to regulation by litter nutrient elements such as C/N. As litter enters a slow decomposition stage, the predictive role of nutrients decreases, the relevance of structural substances increases, such as lignin, and the effects of species richness may change (Grossman et al., 2020). (3) As decomposition proceeds, microbial diversity changes, which may be regulated by species richness (Voříšková and Baldrian, 2013; Kennedy and El-Sabaawi, 2017). Bardgett and Shine reported that moderate species richness (2–4) reduced microbial biomass, which may neutralize the effect of material heterogeneity, showing no significant effect on species richness overall (Bardgett and Shine, 1999).
Although the influence of species on decomposition is stronger, the environmental role cannot be ignored. Rapid litter decomposition is mainly due to microbial feeding and leaching, and different decomposition environments significantly affect the litter decomposition (Table 5) (Makkonen et al., 2012). The scouring of the river body first leaches out many soluble substances from the litter, resulting in rapid mass loss and nutrient release. The rapid nutrient release caused by water body scouring affected the predictive effect of the initial litter traits, resulting in a non-significant linear correlation between the litter initial P, Ca, and Mg elemental content and mass loss in the submergence environment (Figures 6–9). In addition, the aquatic environment involves more fungi. Some studies have shown that the contribution of fungal organisms in the litter decomposition process is greater than bacteria (Hieber and Gessner, 2002; Allison et al., 2013). The different nutrient requirements of the microbiota lead to variable attachment of microorganisms to litter, so the predictive role of nutrient elements may be even less in the aqueous environment (Chen et al., 2020). Waterbody nutrients also reduced the interspecific differences between plant litter and affected the predicted decomposition rate of mixed litter (Rosemond et al., 2010). Although both species and the environment impact litter decomposition, many studies have shown that the relative importance of species is stronger.
There was a faster decomposition rate of invasive plants in the reservoir zone than native plants. There was a synergistic effect between A. philoxeroides and native plants on mixed litter decomposition, but the effect of B. pilosa was not significant. The litter decomposition rate did not differ significantly across species richness levels. However, the interaction between invasive plants and species richness greatly influenced litter mass loss. These results were associated with the initial chemical characteristics of the plants, and invasive plants altered the mean traits of plant communities. In particular, the carbon and nitrogen contents and the C/N ratio were linearly correlated with litter mass loss and mixing effect intensity. In addition, the submergence decomposition environment also mitigated the effect of invasive plants, particularly regarding water flushing and nutrients. In conclusion, invasive species accelerate the decomposition of native litter. However, this is influenced by the differences between the chemical functional traits of invasive and native plants. This experiment investigated the effects of overall plant trait differences and changes in species richness caused by invasive plants on litter mass loss during the rapid decomposition period. Furthermore, the decomposition time should be analyzed, which may involve several other factors in assessing the effects of invasive plants.
The raw data supporting the conclusions of this article will be made available by the authors, without undue reservation.
XHu, MA, and CL contributed to the conception and design of the study. XHu and MA organized the database. XHu, DD, JL, and XHe collected research samples and performed the statistical analysis. XHu, MA, and CL wrote and revised the manuscript. MA and CL supervised the research. All authors contributed to the article and approved the submitted version.
This research has been supported by the Chongqing Municipality Key Forestry Research Project (No. 2021-9), Chongqing Municipality Housing and Urban Construction Committee (No. Chengkezi 2019-1-4-2), Forestry Extension Project of China Central Finance (No. Yulinketui 2020-2), the Science Foundation of College of Life Sciences of Southwest University (No. 20212005406201), Ningxia Key Research and Development Project (No. 2020BFG03006), and Ningxia Natural Science Foundation Project (No. 2020AAC03107).
The authors declare that the research was conducted in the absence of any commercial or financial relationships that could be construed as a potential conflict of interest.
All claims expressed in this article are solely those of the authors and do not necessarily represent those of their affiliated organizations, or those of the publisher, the editors and the reviewers. Any product that may be evaluated in this article, or claim that may be made by its manufacturer, is not guaranteed or endorsed by the publisher.
We wish to thank all the partners in the research team and all the people who helped with the fieldwork throughout the experiments.
Allison, S. D., Lu, Y., Weihe, C., Goulden, M. L., Martiny, A. C., Treseder, K. K., et al. (2013). Microbial abundance and composition influence litter decomposition response to environmental change. Ecology 94, 714–725. doi: 10.1890/12-1243.1
Arif, M., Behzad, H. M., Tahir, M., and Changxiao, L. (2022a). Environmental literacy affects riparian clean production near major waterways and tributaries. Sci. Total Environ. 834, 155476. doi: 10.1016/j.scitotenv.2022.155476
Arif, M., Jie, Z., Tahir, M., Xin, H., and Changxiao, L. (2022b). The impact of stress factors on riparian zone degradation around dams and reservoirs. Land Degrad. Dev. 4310, 1–15. doi: 10.1002/ldr.4310
Arif, M., Tahir, M., Jie, Z., and Changxiao, L. (2021). Impacts of riparian width and stream channel width on ecological networks in main waterways and tributaries. Sci. Total Environ. 792, 148457. doi: 10.1016/j.scitotenv.2021.148457
Ashton, I. W., Hyatt, L. A., Howe, K. M., Gurevitch, J., and Lerdau, M. T. (2005). Invasive species accelerate decomposition and litter nitrogen loss in a mixed deciduous forest. Ecol. Appl. 15, 1263–1272. doi: 10.1890/04-0741
Bani, A., Pioli, S., Ventura, M., Panzacchi, P., Borruso, L., Tognetti, R., et al. (2018). The role of microbial community in the decomposition of leaf litter and deadwood. Appl. Soil Ecol. 126, 75–84. doi: 10.1016/j.apsoil.2018.02.017
Bardgett, R. D., and Shine, A. (1999). Linkages between plant litter diversity, soil microbial biomass and ecosystem function in temperate grasslands. Soil Biol. Biochem. 31, 317–321. doi: 10.1016/S0038-0717(98)00121-7
Behzad, H. M., Jiang, Y., Arif, M., Wu, C., He, Q., Zhao, H., et al. (2022). Tunneling-induced groundwater depletion limits long-term growth dynamics of forest trees. Sci. Total Environ. 811, 152375. doi: 10.1016/j.scitotenv.2021.152375
Cao, C., Liu, S. Q., Ma, Z. B., Lin, Y., Su, Q., Chen, H., et al. (2018). Dynamics of multiple elements in fast decomposing vegetable residues. Sci. Total Environ. 616–617, 614–621. doi: 10.1016/j.scitotenv.2017.10.287
Chen, B. M., Peng, S. L., D'antonio, C. M., Li, D. J., and Ren, W. T. (2013). Non-additive effects on decomposition from mixing litter of the invasive Mikania micrantha H.B.K. with native plants. PLoS ONE 8, e66289. doi: 10.1371/journal.pone.0066289
Chen, G., Li, X. L., Huang, J., Xiang, L., Sun, L., Yang, J., et al. (2022). Characteristics of plant communities and their relationships withenvironmental factors in the water level fluctuation zone of the Zigui region of the Three Gorges Reservoir. Acta Ecol. Sin. 42, 688–699. doi: 10.5846/stxb202008272233
Chen, Z., Arif, M., Wang, C., Chen, X., and Li, C. (2021). Effects of hydrological regime on foliar decomposition and nutrient release in the riparian zone of the Three Gorges Reservoir, China. Front. Plant Sci. 12, 661865. doi: 10.3389/fpls.2021.661865
Chen, Z., Wang, C., Chen, X., Yuan, Z., Song, H., and Li, C. (2020). Heterogeneous leaves of predominant trees species enhance decomposition and nutrient release in the riparian zone of the Three Gorges Reservoir. Sci. Rep. 10, 17382. doi: 10.1038/s41598-020-74062-4
Dai, X., Yu, Z., Yang, G., and Wan, R. (2020). Role of flooding patterns in the biomass production of vegetation in a typical herbaceous wetland, Poyang Lake Wetland, China. Front. Plant Sci. 11, 521358. doi: 10.3389/fpls.2020.521358
Dawson, W., Maurel, N., and Van Kleunen, M. (2015). A new perspective on trait differences between native and invasive exotic plants: comment. Ecology 96, 1150–1152. doi: 10.1890/14-1315.1
Debusk, W. F., and Reddy, K. R. (2005). Litter decomposition and nutrient dynamics in a phosphorus enriched everglades marsh. Biogeochemistry 75, 217–240. doi: 10.1007/s10533-004-7113-0
Deng, H., Zhang, X., Zhang, C., Wang, Y. M., and Wang, D. Y. (2017). Release characteristics of mercury from submersed typical herbaceous plants in the water-level fluctuation zone of the Three Gorges Reservoir area. Environ. Sci. 38, 987–992. doi: 10.13227/j.hjkx.201608187
Ding, D., Liu, M., Arif, M., Yuan, Z., Li, J., Hu, X., et al. (2021). Responses of ecological stoichiometric characteristics of carbon, nitrogen, and phosphorus to periodic submergence in mega-reservoir: growth of Taxodium distichum and Taxodium ascendens. Plants 10, 2040. doi: 10.3390/plants10102040
Enríquez, S., Duarte, C. M., and Sand-Jensen, K. (1993). Patterns in decomposition rates among photosynthetic organisms: the importance of detritus C:N:P content. Oecologia 94, 457–471. doi: 10.1007/BF00566960
Gao, J., Kang, F., and Han, H. (2016). Effect of litter quality on leaf-litter decomposition in the context of home-field advantage and non-additive effects in temperate forests in China. Pol. J. Environ. Stud. 25, 1911–1920. doi: 10.15244/pjoes/62822
Gartner, T. B., and Cardon, Z. G. (2004). Decomposition dynamics in mixed-species leaf litter. Oikos 104, 230–246. doi: 10.1111/j.0030-1299.2004.12738.x
Gessner, M. O., Chauvet, E., and Dobson, M. (1999). A perspective on leaf litter breakdown in streams. Oikos 85, 377–384. doi: 10.2307/3546505
Gessner, M. O., Swan, C. M., Dang, C. K., Mckie, B. G., Bardgett, R. D., Wall, D. H., et al. (2010). Diversity meets decomposition. Trends Ecol. Evol. 25, 372–380. doi: 10.1016/j.tree.2010.01.010
Grossman, J. J., Cavender-Bares, J., and Hobbie, S. E. (2020). Functional diversity of leaf litter mixtures slows decomposition of labile but not recalcitrant carbon over two years. Ecol. Monogr. 90, e01407. doi: 10.1002/ecm.1407
Gul, Z., Tang, Z.-H., Arif, M., and Ye, Z. (2022). An insight into abiotic stress and influx tolerance mechanisms in plants to cope in saline environments. Biology 11, 597. doi: 10.3390/biology11040597
Hamid, M., Khuroo, A. A., Malik, A. H., Ahmad, R., Singh, C. P., Dolezal, J., et al. (2020). Early evidence of shifts in Alpine summit vegetation: a case study from Kashmir Himalaya. Front. Plant Sci. 11, 421. doi: 10.3389/fpls.2020.00421
Handa, I. T., Aerts, R., Berendse, F., Berg, M. P., Bruder, A., Butenschoen, O., et al. (2014). Consequences of biodiversity loss for litter decomposition across biomes. Nature 509, 218–221. doi: 10.1038/nature13247
Harguindeguy, N. P., Blundo, C. M., Gurvich, D. E., Diaz, S., and Cuevas, E. (2008). More than the sum of its parts? Assessing litter heterogeneity effects on the decomposition of litter mixtures through leaf chemistry. Plant Soil 303, 151–159. doi: 10.1007/s11104-007-9495-y
Hättenschwiler, S., and Vitousek, P. M. (2000). The role of polyphenols in terrestrial ecosystem nutrient cycling. Trends Ecol. Evol. 15, 238–243. doi: 10.1016/S0169-5347(00)01861-9
He, X., Wang, T., Wu, K., Wang, P., Qi, Y., Arif, M., et al. (2021). Responses of swamp cypress (Taxodium distichum) and Chinese willow (Salix matsudana) roots to periodic submergence in mega-reservoir: changes in organic acid concentration. Forests 12, 203. doi: 10.3390/f12020203
Hector, A., Beale, A. J., Minns, A., Otway, S. J., and Lawton, J. H. (2000). Consequences of the reduction of plant diversity for litter decomposition: effects through litter quality and microenvironment. Oikos 90, 357–371. doi: 10.1034/j.1600-0706.2000.900217.x
Hickman, J. E., Ashton, I. W., Howe, K. M., and Lerdau, M. T. (2013). The native-invasive balance: implications for nutrient cycling in ecosystems. Oecologia 173, 319–328. doi: 10.1007/s00442-013-2607-x
Hieber, M., and Gessner, M. O. (2002). Contribution of stream detrivores, fungi, and bacteria to leaf breakdown based on biomass estimates. Ecology 83, 1026–1038. doi: 10.1890/0012-9658(2002)083[1026:COSDFA]2.0.CO;2
Hoorens, B., Aerts, R., and Stroetenga, M. (2003). Does initial litter chemistry explain litter mixture effects on decomposition? Oecologia 137, 578–586. doi: 10.1007/s00442-003-1365-6
Hu, X., Xie, T., Arif, M., Ding, D., Li, J., Yuan, Z., et al. (2021). Response of annual herbaceous plant leaching and decomposition to periodic submergence in mega-reservoirs: changes in litter nutrients and soil properties for restoration. Biology 10, 1141. doi: 10.3390/biology10111141
Incerti, G., Cartenì, F., Cesarano, G., Sarker, T. C., Abd El-Gawad, A. M., D'ascoli, R., et al. (2018). Faster N release, but not C loss, from leaf litter of invasives compared to native species in mediterranean ecosystems. Front. Plant Sci. 9, 534. doi: 10.3389/fpls.2018.00534
Kennedy, K. T. M., and El-Sabaawi, R. W. (2017). A global meta-analysis of exotic versus native leaf decay in stream ecosystems. Freshw. Biol. 62, 977–989. doi: 10.1111/fwb.12918
Laliberte, E., and Legendre, P. (2010). A distance-based framework for measuring functional diversity from multiple traits. Ecology 91, 299–305. doi: 10.1890/08-2244.1
Lecerf, A., Marie, G., Kominoski, J. S., Leroy, C. J., Bernadet, C., and Swan, C. M. (2011). Incubation time, functional litter diversity, and habitat characteristics predict litter-mixing effects on decomposition. Ecology 92, 160–169. doi: 10.1890/10-0315.1
Lecerf, A., Risnoveanu, G., Popescu, C., Gessner, M. O., and Chauvet, E. (2007). Decomposition of diverse litter mixtures in streams. Ecology 88, 219–227. doi: 10.1890/0012-9658(2007)88[219:DODLMI]2.0.CO;2
Lee, H., Fitzgerald, J., Hewins, D. B., Mcculley, R. L., Archer, S. R., Rahn, T., et al. (2014). Soil moisture and soil-litter mixing effects on surface litter decomposition: a controlled environment assessment. Soil Biol. Biochem. 72, 123–132. doi: 10.1016/j.soilbio.2014.01.027
Leffler, A. J., James, J. J., Monaco, T. A., and Sheley, R. L. (2014). A new perspective on trait differences between native and invasive exotic plants. Ecology 95, 298–305. doi: 10.1890/13-0102.1
Li, J., Li, L., Arif, M., Ding, D., Hu, X., Zheng, J., et al. (2021). Artificial plantation responses to periodic submergence in massive dam and reservoir riparian zones: changes in soil properties and bacterial community characteristics. Biology 10, 819. doi: 10.3390/biology10080819
Liu, C., Liu, Y., Guo, K., Zhao, H., Qiao, X., Wang, S., et al. (2016). Mixing litter from deciduous and evergreen trees enhances decomposition in a subtropical karst forest in southwestern China. Soil Biol. Biochem. 101, 44–54. doi: 10.1016/j.soilbio.2016.07.004
Liu, J., Liu, X., Song, Q., Compson, Z. G., Leroy, C. J., Luan, F., et al. (2020). Synergistic effects: a common theme in mixed-species litter decomposition. New Phytol. 227, 757–765. doi: 10.1111/nph.16556
Liu, P., Sun, O. J., Huang, J. H., Li, L. H., and Han, X. G. (2007). Nonadditive effects of litter mixtures on decomposition and correlation with initial litter N and P concentrations in grassland plant species of northern China. Biol. Fertil. Soils 44, 211–216. doi: 10.1007/s00374-007-0195-9
Liu, Z. M., Ma, M. H., Wu, S. J., Liang, Z., Wang, Y., Ye, F., et al. (2019). Effects of single and multiple plant species in balancing in two key ecosystem functions. Bangladesh J. Bot. 48, 809–815.
Lopes, M. L., Martins, P., Ricardo, F., Rodrigues, A. M., and Quintino, V. (2011). In situ experimental decomposition studies in estuaries: a comparison of Phragmites australis and Fucus vesiculosus. Estuarine Coastal Shelf Sci. 92, 573–580. doi: 10.1016/j.ecss.2011.02.014
Maisto, G., Marco, A. D., Meola, A., Sessa, L., and Santo, A. V. D. (2011). Nutrient dynamics in litter mixtures of four Mediterranean maquis species decomposing in situ. Soil Biol. Biochem. 43, 520–530. doi: 10.1016/j.soilbio.2010.11.017
Makkonen, M., Berg, M. P., Logtestijn, R. S. P. V., Hal, J. R. V., and Aerts, R. (2012). Do physical plant litter traits explain non-additivity in litter mixtures? A test of the improved microenvironmental conditions theory. Oikos 122, 987–997. doi: 10.1111/j.1600-0706.2012.20750.x
Mi, W., Zou, Y., Li, M., Chen, M., and Dong, F. (2016). Nitrogen and phosphorus stoichiometry characteristics of typical herb plants in the water-fluctuation-zone of Three Gorges Reservoir. J. Lake Sci. 28, 802–811. doi: 10.18307/2016.0413
Naeem, S., Knops, J. M. H., Tilman, D., Howe, K. M., Kennedy, T., and Gale, S. (2000). Plant diversity increases resistance to invasion in the absence of covarying extrinsic factors. Oikos 91, 97–108. doi: 10.1034/j.1600-0706.2000.910108.x
Poulette, M. M., and Arthur, M. A. (2012). The impact of the invasive shrub Lonicera maackii on the decomposition dynamics of a native plant community. Ecol. Appl. 22, 412–424. doi: 10.1890/11-1105.1
Prescott, C. E., and Zukswert, J. M. (2016). Invasive plant species and litter decomposition: time to challenge assumptions. New Phytol. 209, 5–7. doi: 10.1111/nph.13741
Purahong, W., Wubet, T., Lentendu, G., Schloter, M., Pecyna, M. J., Kapturska, D., et al. (2016). Life in leaf litter: novel insights into community dynamics of bacteria and fungi during litter decomposition. Mol. Ecol. 25, 4059–4074. doi: 10.1111/mec.13739
Rosemond, A. D., Swan, C. M., Kominoski, J. S., and Dye, S. E. (2010). Non-additive effects of litter mixing are suppressed in a nutrient-enriched stream. Oikos 119, 326–336. doi: 10.1111/j.1600-0706.2009.17904.x
Sang, C., Zheng, Y., Zhou, Q., Li, D., Liang, G., and Gao, Y. (2019). Effects of water impoundment and water-level manipulation on the bioaccumulation pattern, trophic transfer and health risk of heavy metals in the food web of Three Gorges Reservoir (China). Chemosphere 232, 403–414. doi: 10.1016/j.chemosphere.2019.04.216
Scheffer, R. A., and Aerts, R. (2000). Root decomposition and soil nutrient and carbon cycling in two temperate fen ecosystems. Oikos 91, 541–549. doi: 10.1034/j.1600-0706.2000.910316.x
Schuster, M. J., and Dukes, J. S. (2014). Non-additive effects of invasive tree litter shift seasonal N release: a potential invasion feedback. Oikos 123, 1101–1111. doi: 10.1111/oik.01078
Smith, V. C., and Bradford, M. A. (2003). Do non-additive effects on decomposition in litter-mix experiments result from differences in resource quality between litters? Oikos 102, 235–242. doi: 10.1034/j.1600-0706.2003.12503.x
Sun, R., Liu, H., Ding, J.-J., and Yuan, X.-Z. (2011). Quantitative analyses of plant communities in the hydro-fluctuation area in Kaixian county after impoundment of the Three-Gorge Reservoir. J. Ecol. Rural Environ. 27, 23–28.
Voříšková, J., and Baldrian, P. (2013). Fungal community on decomposing leaf litter undergoes rapid successional changes. ISME J. 7, 477–486. doi: 10.1038/ismej.2012.116
Wardle, D. A., Bonner, K. I., and Nicholson, K. S. (1997). Biodiversity and plant litter: experimental evidence which does not support the view that enhanced species richness improves ecosystem function. Oikos 79, 247–258. doi: 10.2307/3546010
Webster, J. R., and Benfield, E. F. (1986). Vascular plant breakdown in freshwater ecosystems. Annu. Rev. Ecol. Syst. 17, 567–594. doi: 10.1146/annurev.es.17.110186.003031
Wickings, K., Grandy, A. S., Reed, S. C., and Cleveland, C. C. (2012). The origin of litter chemical complexity during decomposition. Ecol. Lett. 15, 1180–1188. doi: 10.1111/j.1461-0248.2012.01837.x
Wu, D., Li, T., and Wan, S. (2013). Time and litter species composition affect litter-mixing effects on decomposition rates. Plant Soil 371, 355–366. doi: 10.1007/s11104-013-1697-x
Xiao, L., Zhu, B., Kumwimba, M. N., and Jiang, S. (2017). Plant soaking decomposition as well as nitrogen and phosphorous release in the water-level fluctuation zone of the Three Gorges Reservoir. Sci. Total Environ. 592, 527–534. doi: 10.1016/j.scitotenv.2017.03.104
Xiong, G. M., Zhang, A. Y., Fan, D. Y., Ge, J. L., Yang, D., Xie, Z. Q., et al. (2018). Functional coordination between leaf traits and biomass allocation and growth of four herbaceous species in a newly established reservoir riparian ecosystem in China. Ecol. Evol. 8, 11372–11384. doi: 10.1002/ece3.4494
Yang, J., Li, E.-H., Yang, C., Xia, Y., and Zhou, R. (2022). Effects of South-to-North water diversion project cascade dams on riparian vegetation along the middle and lower reaches of the Hanjiang River, China. Front. Plant Sci. 13, 849010. doi: 10.3389/fpls.2022.849010
Yelenik, S. G., Stock, W. D., and Richardson, D. M. (2007). Functional group identity does not predict invader impacts: differential effects of nitrogen-fixing exotic plants on ecosystem function. Biol. Invasions 9, 117–125. doi: 10.1007/s10530-006-0008-3
Yuan, Z. X., Ni, X. L., Arif, M., Dong, Z., Zhang, L. M., Tan, X., et al. (2021). Transcriptomic analysis of the photosynthetic, respiration, and aerenchyma adaptation strategies in bermudagrass (Cynodon dactylon) under different submergence stress. Int. J. Mol. Sci. 22, 7905. doi: 10.3390/ijms22157905
Zhang, J., Li, H., Zhang, H., Zhang, H., and Tang, Z. (2021). Responses of litter decomposition and nutrient dynamics to nitrogen addition in temperate shrublands of North China. Front. Plant Sci. 11, 849010. doi: 10.3389/fpls.2020.618675
Zhang, L., Zhang, Y. J., Zou, J. W., and Siemann, E. (2014). Decomposition of Phragmites australis litter retarded by invasive Solidago canadensis in mixtures: an antagonistic non-additive effect. Sci. Rep. 4, 5488. doi: 10.1038/srep05488
Zhang, M., Cheng, X., Geng, Q., Shi, Z., Luo, Y., and Xu, X. (2019). Leaf litter traits predominantly control litter decomposition in streams worldwide. Glob. Ecol. Biogeogr. 28, 1469–1486. doi: 10.1111/geb.12966
Zhang, P., Scheu, S., Li, B., Lin, G., Zhao, J., and Wu, J. (2020). Litter C transformations of invasive Spartina alterniflora affected by litter type and soil source. Biol. Fertil. Soils 56, 369–379. doi: 10.1007/s00374-019-01429-9
Zhang, A. Y., Xiong, G. M., Fan, D. Y., and Xie, Z. Q. (2016). Effects of damming on plant diversity in the inundated and riparian zones of the Three Gorges Reservoir Area, China. Chin. J. Ecol. 35, 2505–2518. doi: 10.13292/j.1000-4890.201609.019
Zheng, J., Arif, M., Zhang, S., Yuan, Z., Zhang, L., Dong, Z., et al. (2021a). The convergence of species composition along the drawdown zone of the Three Gorges Dam Reservoir, China: implications for restoration. Environ. Sci. Pollut. Res. 28, 42609–42621. doi: 10.1007/s11356-021-13774-0
Zheng, J., Arif, M., Zhang, S. L., Yuan, Z. X., Zhang, L. M., Li, J. J., et al. (2021b). Dam inundation simplifies the plant community composition. Sci. Total Environ. 801, 149827. doi: 10.1016/j.scitotenv.2021.149827
Zhong, R. H., He, X. B., Bao, Y. H., Tang, Q., Gao, J. Z., Yan, D. D., et al. (2016). Estimation of soil reinforcement by the roots of four post-dam prevailing grass species in the riparian zone of Three Gorges Reservoir, China. J. Mt. Sci. 13, 508–521. doi: 10.1007/s11629-014-3397-2
Zhou, L., Yu, H., Yang, K., Chen, L., Yin, W., and Ding, J. (2021). Latitudinal and longitudinal trends of seed traits indicate adaptive strategies of an invasive plant. Front Plant Sci. 12, 657813. doi: 10.3389/fpls.2021.657813
Keywords: Three Gorges Reservoir, hydro-fluctuation zone, exotic plants, species diversity, submergence environment, plant traits
Citation: Hu X, Arif M, Ding D, Li J, He X and Li C (2022) Invasive Plants and Species Richness Impact Litter Decomposition in Riparian Zones. Front. Plant Sci. 13:955656. doi: 10.3389/fpls.2022.955656
Received: 29 May 2022; Accepted: 14 June 2022;
Published: 07 July 2022.
Edited by:
Runguo Zang, Chinese Academy of Forestry, ChinaCopyright © 2022 Hu, Arif, Ding, Li, He and Li. This is an open-access article distributed under the terms of the Creative Commons Attribution License (CC BY). The use, distribution or reproduction in other forums is permitted, provided the original author(s) and the copyright owner(s) are credited and that the original publication in this journal is cited, in accordance with accepted academic practice. No use, distribution or reproduction is permitted which does not comply with these terms.
*Correspondence: Changxiao Li, bGljaGFuZ3hAc3d1LmVkdS5jbg==
Disclaimer: All claims expressed in this article are solely those of the authors and do not necessarily represent those of their affiliated organizations, or those of the publisher, the editors and the reviewers. Any product that may be evaluated in this article or claim that may be made by its manufacturer is not guaranteed or endorsed by the publisher.
Research integrity at Frontiers
Learn more about the work of our research integrity team to safeguard the quality of each article we publish.