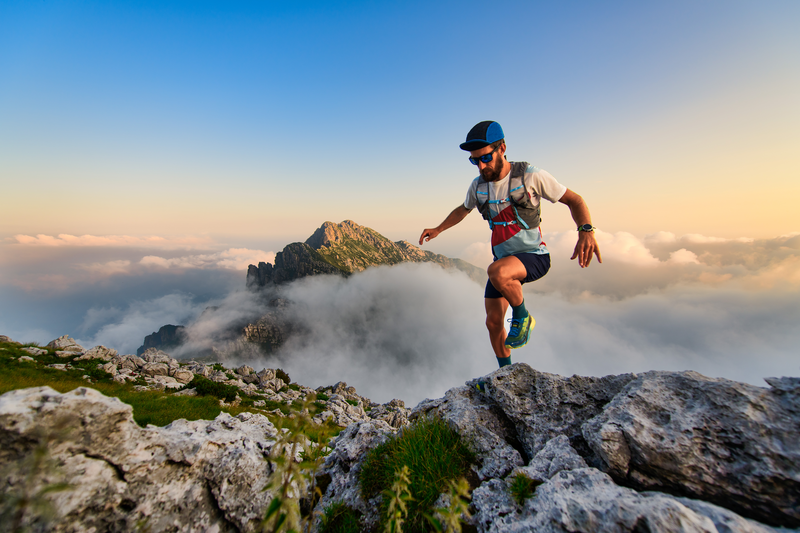
94% of researchers rate our articles as excellent or good
Learn more about the work of our research integrity team to safeguard the quality of each article we publish.
Find out more
REVIEW article
Front. Plant Sci. , 11 July 2022
Sec. Plant Abiotic Stress
Volume 13 - 2022 | https://doi.org/10.3389/fpls.2022.951752
This article is part of the Research Topic Nanofertilizers and Abiotic Stress Tolerance in Plants View all 9 articles
Abiotic stresses, such as heavy metals (HMs), drought, salinity and water logging, are the foremost limiting factors that adversely affect the plant growth and crop productivity worldwide. The plants respond to such stresses by activating a series of intricate mechanisms that subsequently alter the morpho-physiological and biochemical processes. Over the past few decades, abiotic stresses in plants have been managed through marker-assisted breeding, conventional breeding, and genetic engineering approaches. With technological advancement, efficient strategies are required to cope with the harmful effects of abiotic environmental constraints to develop sustainable agriculture systems of crop production. Recently, nanotechnology has emerged as an attractive area of study with potential applications in the agricultural science, including mitigating the impacts of climate change, increasing nutrient utilization efficiency and abiotic stress management. Nanoparticles (NPs), as nanofertilizers, have gained significant attention due to their high surface area to volume ratio, eco-friendly nature, low cost, unique physicochemical properties, and improved plant productivity. Several studies have revealed the potential role of NPs in abiotic stress management. This review aims to emphasize the role of NPs in managing abiotic stresses and growth promotion to develop a cost-effective and environment friendly strategy for the future agricultural sustainability.
The world’s population is expected to increase by 2 billion persons by 2050, inflating the food demands to feed the growing population (Mora et al., 2020; Zsögön et al., 2022). However, the global warming and climate change with an ever-increasing population, serve as the predominant limiting factors that hinder the efforts to meet the word’s food demand (Lowry et al., 2019). In the last few years, adverse climatic changes have induced abiotic stresses, such as heavy metal (HMs) toxicity, drought, heat, and high soil salinity, that lead to reduced crop productivity worldwide (Rizwan et al., 2012; Fahad et al., 2017). HMs in agricultural soils resulting from the large-scale application of chemical fertilizers, atmospheric deposition, sewage sludge, and rapid industrial growth. In recent decades, crop production has been continually effected by toxic HMs (Ahmed et al., 2021a; Chen et al., 2022). The high concentrations of HMs can reduce the plant growth by disrupting the nutrient uptake, antioxidant enzymes, photosynthetic machinery, and by increasing the reactive oxygen species (ROS) production (Adrees et al., 2021; Rizwan et al., 2021).
Soil salinity negatively affects at a physiological level, which disturbs ionic and water homeostasis. At the cellular level, salinity stress contributes to high accumulation of ROS that disturbs the cellular redox homeostasis (Abdel Latef et al., 2018). In addition, heat stress also causes ROS generation, which invariably impacts oxidative activities (Hasanuzzaman et al., 2012). Furthermore, prolonged drought has been reported to cause the reduction in stomatal opening, leaf size, leaf water potential, root growth, and seed number, size, and tolerance, inhibiting flowering, fruiting, and thus reduce crop production (Xu et al., 2016). Different approaches have been used to control abiotic stresses in plants, including conventional breeding, marker-assisted breeding, and transgenic crop engineering (Ashraf, 2010; Grover et al., 2013).
Among others, nano-enabled approaches have recently emerged as a promising tool to control nutrient deficiency, increase crop yields, transform biological systems, and management of plant stresses imposed by environment (Dimkpa et al., 2017; White and Gardea-Torresdey, 2018). Moreover, the use of nanoparticles (NPs) as nanofertilizers for targeted delivery of micronutrients is considered as efficient, cost-effective, eco-friendly, and best alternatives to chemical fertilizers (Irshad et al., 2021b; Shah et al., 2021). In the last few years, several methods, such as physical (electro-explosion, gamma radiations, pulse laser ablation, ion sputtering scattering, mechanical/ball milling), chemical (sol–gel, microemulsion, co-precipitation, hydrothermal) and biological approaches (using microorganisms/plants extracts), have been used for the production of metallic NPs (Ahmed et al., 2021d; Noman et al., 2022). Various studies have reported the application of NPs improved the abiotic stress tolerance in plants by modulating the biochemical, morpho-physiological and genetic mechanisms under salinity stress (Rossi et al., 2017), drought stress (Mozafari et al., 2018), and HMs stress (Bashir et al., 2020). Based on the revised literature, we conclude that the application of NPs against abiotic stress in crop production is considered as a reliable approach with long-term effectiveness compared with other traditional methods.
This review covers current opportunities for the potential application of NPs in agriculture and focuses on nano-enabled technologies for abiotic stress management. It further explores the possible NPs uptake mechanism and their positive effects on plant growth and soil fertility.
Climate change has been caused by global warming, anthropogenic activities, and other unavoidable factors, and results in multiple abiotic stresses, reducing agricultural productivity and degrading natural resources (Shahzad et al., 2018). Various studies have reported a substantial reduction in the yield of many crops under abiotic stress conditions, which is mostly caused by HMs, low temperature, drought and salinity stresses (Tripathi et al., 2014; Anjum et al., 2017; Singh et al., 2017; Hussain et al., 2018b; Khan et al., 2018). Most of these abiotic stress conditions encountered by plants at various growth stages resulted in developing various defense mechanisms in plants to deal with negative physiological changes induced by environmental stresses (Ahmad et al., 2015; Jiang et al., 2016; Dimkpa et al., 2017). Several studies have revealed that abiotic stresses cause many physiological, biochemical, and molecular responses that affect several cellular processes in plants (Figure 1).
Figure 1. The positive effect of nanoparticles (NPs) on plant growth and development under abiotic stress conditions. The figure created using BioRender (https://biorender.com/).
Among the abiotic stresses, soil salinity, drought and HMs contamination caused substantial reduction in crop productivity and considered as the most important threats to global crop production and food security (Godoy et al., 2021; Haider et al., 2021). Moreover, salinity and drought have been identified as damaging stressors that limit the production of many crops by inducing physiological and biochemical changes (Zia et al., 2021). These stresses restrict plant growth and productivity by imposing oxidative stress, osmotic stress, and nutritional imbalance (Shahid et al., 2020). Salinity stress causes several harmful effects on crop plants at molecular, physiological and biochemical level, which ultimately jeopardize the plant survival (Kumar et al., 2020). Accumulation of sodium (Na+) and chloride (Cl−) ions in the cytosol of the cell cause salt stress that ultimately causes considerable damage to the whole cell, resulting reduced plant growth (Rajput et al., 2019). Under drought stress, leaf stomata become closed, thus inhibiting photosynthesis in the plants, which reduces the total area of the leaf that causes a reduction in water potential and decreases plant growth by increasing osmolytes production and inducing ROS generation in plants (Ibrahim et al., 2019). The intensity and period of the drought stress are the two critical factors under drought stress, which might be correlated directly with the loss in crop productivity and economic yield (Farooq et al., 2009). However, drought combined with salinity stress causes a detrimental reduction in water potential, and decreases osmosis significantly (Hu and Schmidhalter, 2005). Abiotic stress activates agitation in plant metabolism, thus enabling reorganization of the metabolic networks to maintain important processes active (Minkina et al., 2019; Rajput et al., 2020).
HMs are considered a major class of pollutants and are very hazardous to agricultural crop plants and human health (Guo et al., 2020; Xu et al., 2021). The rapid increase in HMs contamination during recent years corresponding to the rapid industrialization, combustion of fossil fuels, atmospheric deposition, spillage of petrochemicals, mining, agricultural practices, and disposal of waste material having high metal content directly to the agricultural lands and water bodies (Noman et al., 2020a). The most common HMs present in the environmental systems are cadmium (Cd), lead (Pb), arsenic (As), nickel (Ni), chromium (Cr), cobalt (Co) and zinc (Zn). The global economic impact of HMs pollution is projected to be more than $10 billion per year (Kumar et al., 2019). Previous studies have reported the increased HMs concentrations in crops and agricultural soils as a result of significant anthropogenic and industrial waste deposition (Rizwan et al., 2019b; Noman et al., 2020b). HMs disturb redox homeostasis by stimulating the free radical formation and enhancing ROS production, which cause cellular oxidative stress by altering the cell structure, damaging membrane permeability, and proteins functionality (Sharma et al., 2012). Furthermore, HMs accumulate in the human body through the food chain and cause health problems, such as diabetes, hypertension, cardiovascular diseases, and cancer (Ahmed et al., 2021b).
Nanotechnology has tremendous potential in agriculture, including mitigating climate change impacts, and improving abiotic stress management strategies (Mahakham et al., 2016). Nano-enabled technologies have been developed to promote plant growth, such as the application of nanofertilizers through different means (such as soil irrigation, foliar spray, seed coating), nano-sensors to monitor the real-time plant health condition, genetic engineering of plants to increase defense-related phytohormones and photosynthetic efficiency (Figure 2). The key benefits of using NPs compared to conventional fertilizers are the high surface-area-to-volume ratio, high contaminant removal efficiency, and efficient supply of essential nutrients for the soil health as nanofertilizers (Tripathi et al., 2015). Several studies have reported using NPs as nanofertilizers to enhance crop production under stress conditions (Iavicoli et al., 2017; Ahmed et al., 2020, 2021c).
Figure 2. Schematic representation of potential NPs applications in plant agriculture. The figure created using BioRender (https://biorender.com/).
The NPs mitigate the nutrient losses as they have more retention capacity (high surface area) for nutrients and provide potential benefits to plants (Wang et al., 2016; Ahmed et al., 2021b). The application of NPs as nanofertilizers showed efficient results in improving abiotic stress tolerance in plants by increasing the plant growth, nutrient content, phytohormones, antioxidant enzymes, and photosynthesis efficiency while reducing the cellular oxidative stress (Figure 1). Recently, iron oxide (Fe3O4) NPs have been used to enhance crop growth both under HMs contaminated soil as well as drought stress conditions (Hussain et al., 2019; Adrees et al., 2020). Furthermore, Konate et al. (2017) documented the enhanced wheat seedling growth with FeO NPs, which reduced oxidative stress induced by Cd and Pb contamination (López-Luna et al., 2015). NPs application efficiently ameliorates salt stress by decreasing salt concentration and associated toxic effects. Moreover, nano-silicon (Si) has been found to significantly ameliorate salt stress, increase seed germination, improve the antioxidant defense system, leaf turgor, and carbon-assimilation process (Haghighi and Pessarakli, 2013).
Recently, Wu et al. (2017) reported that the cerium oxide (CeO) NPs application maintained quantum yield of photosystem (PS) II and CO2 assimilation through ROS scavenging, particularly hydrogen peroxide, induced by abiotic stress (Horie et al., 2011). The application of titania (TiO2) NPs improved the activity of catalase (CAT), glutathione peroxidase (GPOX), and superoxide dismutase (SOD) and reduced oxidative stress in Duckweed (Lemna minor) plants (Song et al., 2012). Several beneficial and stress countering effects of NPs application in various crops have been reported, such as improved growth in Solanum lycopersicum L. (Shankramma et al., 2016) and Allium cepa L. (Anandaraj and Natarajan, 2017); S. lycopersicum L. (Juárez-Maldonado et al., 2016; Hernández-Hernández et al., 2018), Oryza sativa (Ahmed et al., 2021c), Capsicum annuum (Pinedo-Guerrero et al., 2017; Mozafari et al., 2018). Therefore, it can be concluded that the stress ameliorative potential of NPs can be exploited to combat various negative effects caused by abiotic stresses in crop plants.
Different application methods, such as seed coating, soil drenching, and foliar spray are the primary means to deliver NPs into the plants (Figure 3). Plants are the main component of soil and act as a likely path for the uptake, transport, and use of NPs in the food chain (Wang et al., 2013; Dang et al., 2019). Thus, it is important to have a mechanistic insights into the uptake mechanisms of NPs in plants. In a previous study, Zhu et al. (2008) reported the uptake, translocation and accumulation of FeO NPs in pumpkin (Cucurbita maxima) plants after adding in the growth medium without causing any harm to the plant. Moreover, from the total balance of Fe content (67.4%), 45.4% was accumulated in roots tissues (inside and outside the root surface) and 0.6% in leaf tissue. Similarly, copper oxide NPs translocated, and distributed in maize (Zea mays L.) plants via xylem and phloem vessels (Wang, 2012). Fullerene (C70) NPs were shown to be translocated through the vascular system of rice (O. sativa) and can be inherited to upcoming generations (Lin et al., 2009). NPs internalized by roots/leaves and translocated via two pathways, i.e., apoplastic and symplastic (Huang et al., 2022). In another study, Cui et al. (2020) observed the accumulation of SiO2 NPs in rice cells through fluorescence and transmission electron microscopy under As-spiked condition. NPs were dispersed between cell walls and plasma membrane after overcoming the initial barrier, i.e., cell walls, and their further movement could be affected by osmotic pressure and capillary forces (Lin et al., 2009). Besides ion channels and carrier or transport proteins, such as aquaporin, NPs may enter cells by endocytosis or breaching the cell membrane barrier (Rico et al., 2011). There is no such mechanistic approach that exploits the exact location and role of NPs in plant organelles. However, further studies should be focused to explore the translocation and uptake kinetics of NPs, and extended to study the biochemical, molecular and physiological patterns of NPs uptake and transport kinetics, which is of great significance for understanding their accumulation behavior in plants.
Figure 3. Schematic representation of NPs application approaches, uptake and translocation of NPs in plants. NPs can be delivered to plants by soil application, seed coating and foliar spray to protect plants against abiotic stresses. The figure created using BioRender (https://biorender.com/).
Abiotic stress triggers a wide range of plant responses, varying from growth and morphological changes to crop production and yield (Kazan, 2015; Lamaoui et al., 2018). Nanotechnology has great potential to combat different abiotic stress conditions, such as HMs stress, drought stress, salt stress and heat stress, in an eco-friendly manner (Table 1).
Currently, HMs contamination has been a major concern around the world (Ali et al., 2019). HMs contamination may arise due to the atmospheric deposition, mining, flourishing industrialization, waste incineration, spilling of petrochemicals, removal of high metal waste during different industrial processes (Mohammadi et al., 2018; Irshad et al., 2021a). Their long-term presence and potential toxicity in contaminated soils have been among the major environmental issues that negatively affect the sustainability of living organisms (plants, humans, and animals) (Mohammadi and Ghorbani, 2018; Rizwan et al., 2019b). Among them, Cd has greater significance in the environment due to being highly toxic to plants and humans (Khan et al., 2020; Noman et al., 2020a; Hussain et al., 2021). Accumulation of Cd in plants causes oxidative damage to the cells due to high accumulation of ROS; these negative changes in cells inhibit photosynthesis, lower ion regulation, and reduce nutrient absorption in plants, that result in reduced plant growth (Rizwan et al., 2019a; Li et al., 2020).
Numerous reports on the potential role of NPs in the reclamation of HMs-contaminated soil have been documented (Tripathi et al., 2015; Hussain et al., 2018a). In a recent study, Manzoor et al. (2021) demonstrated that FeO NPs ameliorated the Cd toxicity in wheat plants by improving the biomass, chlorophyll contents, and antioxidant enzymes. These NPs can decrease toxic ion accumulation in plant cells and protect from ionic stress. The effectiveness of NPs in alleviating HM toxicity in plants is due to their small size and large surface area (Figure 4). The mitigation of HM induced phytotoxicity by Si NPs has also been stated in rice (Gao et al., 2018), pea (Rehman et al., 2018), and wheat (Rehman et al., 2017). Furthermore, Konate et al. (2017) determined that FeO NPs improved the wheat plant growth by reducing the cellular oxidative stress induced by Pb and Cd toxicity. Correspondingly, magnetite NPs were also observed to increase the wheat seedlings growth by alleviating Cd and Cr toxicity (López-Luna et al., 2015). FeO NPs have recently been used effectively to enhance plant growth and to alleviate HM and drought stress simultaneously (Hussain et al., 2019; Adrees et al., 2020). However, it is necessary to develop novel nanoremediation approaches to ameliorate the negative impacts of HMs on plant growth and development.
Figure 4. Schematic representation of iron oxide nanoparticles (FeO NPs) to alleviate the Cadmium (Cd) and salinity stress by reducing the acropetal translocation of salt and metals ions. Reproduced with permission from Manzoor et al. (2021). Copyright 2021 Elsevier. The figure created using BioRender (https://biorender.com/).
Drought is considered an important environmental stress that has gained the considerable interest of both environmental and agricultural scientists. It is indeed a major agricultural issue in the world, which restricts plant growth and yield. Drought stress influences various plant growth factors, negatively impacting the economic sector (Kumar and Verma, 2018). Limited moisture content reduces cell size, disturbs membrane integrity, induce oxidative stress and leaf senescence, causing a reduction in crop productivity (Tiwari et al., 2016). Previous studies have revealed that Si NPs improved the drought stress tolerance in plants. For example, drought tolerance increased in hawthorns plants supplied with Si NPs, while the defense-related physiological parameters showed variations according different drought levels and Si NPs concentrations applied (Ashkavand et al., 2018). Correspondingly, Si NPs demonstrated a good potential for post-drought plant recovery by modulating morpho-physiological properties in barley plants (Ghorbanpour et al., 2020). Alsaeedi et al. (2019) reported that Si NPs enhanced cucumber growth and yield under water-deficient and saline conditions.
Chitosan NPs have increased relative water content, photosynthetic rate, CAT, SOD activities, yield, and biomass of wheat plants under drought stress (Behboudi et al., 2019). Foliar application of Fe NPs was reported to alleviate drought stress effects on safflower cultivars (Davar Zareii et al., 2014), while soil application of CeO NPs significantly improved plant growth at 100 mg/kg and increased the photosynthetic rate by regulating the water use efficiency in soybean (Glycine max) plants (Cao et al., 2017). The negative effects of drought stress on lentils (Lens culinaris Medic.) plants was reduced by the application of silver NPs (Das and Das, 2019). Sun et al. (2017) reported Si NPs-assisted delivery of abscisic acid as an effective management strategy to improve drought resistance in Arabidopsis thaliana.
Soil salinity substantially reduce crop production worldwide by inducing ionic toxicity and disturbs ionic homeostasis (Parihar et al., 2015; Etesami et al., 2021). Saline conditions lead to the deposition of Na+ and Cl− ions in plant cells, which cause ionic imbalance and toxicity (Shabala and Cuin, 2008; Khan et al., 2021). In plants, salt stress immensely induces K+ efflux from leaf mesophyll cells and enhances the deposition of Na+ in cytosols. Hence, CO2 assimilation is reduced in saline environments, thus reducing growth rate and production (Parida and Das, 2005). The application of different NPs is an alternative approach to combat the salt stress, which mitigates the accompanying toxicity impacts. In a previous study, Si NPs significantly improved the seed germination, carbon assimilation, leaf turgor, and antioxidant defense system in cherry tomatoes (S. lycopersicum L.) plants under salt stress conditions (Haghighi and Pessarakli, 2013).
Similarly, Manzoor et al. (2021) reported that FeO NPs alleviated the salt stress by improving the growth, chlorophyll contents, and antioxidant enzymes in wheat plants. These NPs can decrease salt ions accumulation in cells and protect plants from ionic stress (Figure 4). Under salt stress, improvement in seed germination and seedlings growth was reported in Si NPs-supplied lentils (L. culinaris Medic.) plants (Sabaghnia and Janmohammadi, 2015). In another study, Ye et al. (2020) revealed that seed priming of manganese NPs control salinity stress by modulating molecular responses in Pepper (C. annuum L.) plants. Zhao et al. (2019) observed that the multi-walled carbon nanotube amendments improved the salinity tolerance in rapeseed (Brassica napus L.) by decreasing the ROS production, thiobarbituric acid and Na+/K+ ratio. However, more research is required at physiochemical and molecular levels to explore the mode of actions of NPs to improve the salinity tolerance in plants.
The constantly rising temperature is considered as one of the most damaging stress among the ever-changing environmental factors (Ohama et al., 2017). Heat stress enhances the ROS production and induces oxidative stress, resulting in the membrane lipids degeneration, disturb cellular homeostasis and impairment of different metabolic processes, which finally cause cells death in crop plant (Savicka and Škute, 2010). Moreover, heat stress photosystem II disruption, electron flow disruption, carbon fixation diminution and induces chlorophyll degradation, which disrupt photosynthesis process and decreased the plant growth (Li et al., 2021). Recent advancements in nanotechnology have modernized agriculture system with promising application to improve the plant growth and development under stress condition (Rana et al., 2021). Several studies revealed the potential application of NPs to improve the heat stress tolerance in crop plants (Wu and Wang, 2020; Thakur et al., 2021).
In a previous study, Haghighi et al. (2014) observed the application of selenium (Se) NPs significantly reduced the heat stress by improving the chlorophyll content, hydration potential, and growth of tomato plants. Similarly, TiO2 NPs amendments significantly reduced the heat stress by stomatal opening in tomato plants (Qi et al., 2013). El-Saadony et al. (2021) reported that the application of biologically synthesized Se NPs (100 μg/ml) improved the wheat growth by increasing heat stress tolerance in plants. In another study, Iqbal et al. (2019) also revealed that silver NPs significantly increased the morphological attributes of wheat plants under heat stress condition. Overall, the application of metallic NPs as nanofertilizers can be used to improve the plant tolerance to heat stress for the sustainable agriculture.
In the last few years, a major concern of the research community is to overcome the negative effects of abiotic stresses on crop production. This review has revealed the potential of NPs to protect crop plants from different abiotic stresses and the mechanisms of NPs accumulation in plants. The application of NPs significantly improved the abiotic stress tolerance in plants by improving the cellular antioxidants, nutrient uptake, photosynthesis efficiency, and regulation of biochemical/molecular mechanisms. Although nanofertilizers can provide a cost-efficient approach for improving the abiotic stress tolerance in plants by providing essential nutrients, however, their extensive use has prompted potential concerns about their negative effects on the ecosystem. In the future, more research insights are required to explore the interactions between NPs and plants to study the adverse effects of residual NPs on the environment systems. The transition from the laboratory to the field is incredibly difficult without promising results from field trials. Further studies considered necessary before starting the field application, such as long-term effectiveness of NPs in field conditions, ecotoxicological risk factors, and the impact of NPs on the metabolome, proteome, metagenome, and transcriptome of the plant and soil systems. The industrial sectors should be established to scale up nanoproducts, train farmers on nanoformulation application, develop application procedures and manage the regulatory landscape. Moreover, future research should emphasize on the designing of cost efficient, nontoxic, self-degradable, and eco-friendly NPs by using green approaches. We anticipate that this review will be useful to develop effective nano-enabled techniques in agriculture sectors to manage the global problem of food security caused by different abiotic stresses.
NM and LA: conceptualization, visualization, and writing—original draft. TA: writing—review, validation, and editing. MN, MA, and MS: conceptualization, writing—review, and editing. SO and KR: writing—review and editing. GW and HZ: conceptualization, funding acquisition, supervision, writing—review, and editing. All authors contributed to the article and approved the submitted version.
This work is financially supported by the 2115 Talent Development Program of China Agricultural University (00109012) and the Scholarship of the “National Thousand (Young) Talents Program” of China (D1201040).
The authors declare that the research was conducted in the absence of any commercial or financial relationships that could be construed as a potential conflict of interest.
All claims expressed in this article are solely those of the authors and do not necessarily represent those of their affiliated organizations, or those of the publisher, the editors and the reviewers. Any product that may be evaluated in this article, or claim that may be made by its manufacturer, is not guaranteed or endorsed by the publisher.
Abdel Latef, A. A. H., Srivastava, A. K., El-sadek, M. S. A., Kordrostami, M., and Tran, L.-S. P. (2018). Titanium dioxide nanoparticles improve growth and enhance tolerance of broad bean plants under saline soil conditions. L. Degrad. Dev. 29, 1065–1073. doi: 10.1002/ldr.2780
Adrees, M., Khan, Z. S., Ali, S., Hafeez, M., Khalid, S., ur Rehman, M. Z., et al. (2020). Simultaneous mitigation of cadmium and drought stress in wheat by soil application of iron nanoparticles. Chemosphere 238:124681. doi: 10.1016/j.chemosphere.2019.124681
Adrees, M., Khan, Z. S., Hafeez, M., Rizwan, M., Hussain, K., Asrar, M., et al. (2021). Foliar exposure of zinc oxide nanoparticles improved the growth of wheat (Triticum aestivum L.) and decreased cadmium concentration in grains under simultaneous cd and water deficient stress. Ecotoxicol. Environ. Saf. 208:111627. doi: 10.1016/j.ecoenv.2020.111627
Ahmad, P., Hashem, A., Abd-Allah, E. F., Alqarawi, A. A., John, R., Egamberdieva, D., et al. (2015). Role of Trichoderma harzianum in mitigating NaCl stress in Indian mustard (Brassica juncea L) through antioxidative defense system. Front. Plant Sci. 6:868. doi: 10.3389/fpls.2015.00868
Ahmed, T., Noman, M., Ijaz, M., Ali, S., Rizwan, M., Ijaz, U., et al. (2021a). Current trends and future prospective in nanoremediation of heavy metals contaminated soils: a way forward towards sustainable agriculture. Ecotoxicol. Environ. Saf. 227:112888. doi: 10.1016/j.ecoenv.2021.112888
Ahmed, T., Noman, M., Manzoor, N., Ali, S., Rizwan, M., Ijaz, M., et al. (2021d). Recent advances in nanoparticles associated ecological harms and their biodegradation: global environmental safety from nano-invaders. J. Environ. Chem. Eng. 9:106093. doi: 10.1016/j.jece.2021.106093
Ahmed, T., Noman, M., Manzoor, N., Shahid, M., Abdullah, M., Ali, L., et al. (2021c). Nanoparticle-based amelioration of drought stress and cadmium toxicity in rice via triggering the stress responsive genetic mechanisms and nutrient acquisition. Ecotoxicol. Environ. Saf. 209:111829. doi: 10.1016/j.ecoenv.2020.111829
Ahmed, T., Noman, M., Rizwan, M., Ali, S., Shahid, M. S., and Li, B. (2021b). Recent progress on the heavy metals ameliorating potential of engineered nanomaterials in rice paddy: a comprehensive outlook on global food safety with nanotoxicitiy issues. Crit. Rev. Food Sci. Nutr. 1–15. doi: 10.1080/10408398.2021.1979931 [Epub ahead of print].
Ahmed, T., Shahid, M., Noman, M., Niazi, M. B. K., Mahmood, F., Manzoor, I., et al. (2020). Silver nanoparticles synthesized by using Bacillus cereus SZT1 ameliorated the damage of bacterial leaf blight pathogen in rice. Pathogens 9:160. doi: 10.3390/pathogens9030160
Ali, S., Rizwan, M., Hussain, A., ur Rehman, M. Z., Ali, B., Yousaf, B., et al. (2019). Silicon nanoparticles enhanced the growth and reduced the cadmium accumulation in grains of wheat (Triticum aestivum L.). Plant Physiol. Biochem. 140, 1–8. doi: 10.1016/j.plaphy.2019.04.041
Alsaeedi, A., El-Ramady, H., Alshaal, T., El-Garawany, M., Elhawat, N., and Al-Otaibi, A. (2019). Silica nanoparticles boost growth and productivity of cucumber under water deficit and salinity stresses by balancing nutrients uptake. Plant Physiol. Biochem. 139, 1–10. doi: 10.1016/j.plaphy.2019.03.008
Anandaraj, K., and Natarajan, N. (2017). Effect of nanoparticles for seed quality enhancement in onion [Allium cepa (Linn) cv. CO (On)] 5. Int. J. Curr. Microbiol. App. Sci. 6, 3714–3724. doi: 10.20546/ijcmas.2017.611.435
Anjum, S. A., Ashraf, U., Zohaib, A., Tanveer, M., Naeem, M., Ali, I., et al. (2017). Growth and developmental responses of crop plants under drought stress: a review. Zemdirbyste-Agriculture 104, 267–276. doi: 10.13080/z-a.2017.104.034
Ashkavand, P., Tabari, M., Zarafshar, M., Tomášková, I., and Struve, D. (2018). Effect of SiO2 nanoparticles on drought resistance in hawthorn seedlings. For. Res. Pap. 76, 350–359. doi: 10.1515/frp-2015-0034
Ashraf, M. (2010). Inducing drought tolerance in plants: recent advances. Biotechnol. Adv. 28, 169–183. doi: 10.1016/j.biotechadv.2009.11.005
Bashir, A., Rizwan, M., Ali, S., Adrees, M., and Qayyum, M. F. (2020). Effect of composted organic amendments and zinc oxide nanoparticles on growth and cadmium accumulation by wheat; a life cycle study. Environ. Sci. Pollut. Res. 27, 23926–23936. doi: 10.1007/s11356-020-08739-8
Behboudi, F., Tahmasebi-Sarvestani, Z., Kassaee, M. Z., Modarres-Sanavy, S. A. M., Sorooshzadeh, A., and Mokhtassi-Bidgoli, A. (2019). Evaluation of chitosan nanoparticles effects with two application methods on wheat under drought stress. J. Plant Nutr. 42, 1439–1451. doi: 10.1080/01904167.2019.1617308
Cao, Z., Stowers, C., Rossi, L., Zhang, W., Lombardini, L., and Ma, X. (2017). Physiological effects of cerium oxide nanoparticles on the photosynthesis and water use efficiency of soybean (Glycine max (L.) Merr.). Environ. Sci. Nano 4, 1086–1094. doi: 10.1039/C7EN00015D
Chen, F., Bashir, A., ur Rehman, M. Z., Adrees, M., Qayyum, M. F., Ma, J., et al. (2022). Combined effects of green manure and zinc oxide nanoparticles on cadmium uptake by wheat (Triticum aestivum L.). Chemosphere 298:134348. doi: 10.1016/j.chemosphere.2022.134348
Cui, J., Li, Y., Jin, Q., and Li, F. (2020). Silica nanoparticles inhibit arsenic uptake into rice suspension cells via improving pectin synthesis and the mechanical force of the cell wall. Environ. Sci. Nano 7, 162–171. doi: 10.1039/C9EN01035A
Dang, F., Chen, Y. Z., Huang, Y. N., Hintelmann, H., Si, Y. B., and Zhou, D. M. (2019). Discerning the sources of silver nanoparticle in a terrestrial food chain by stable isotope tracer technique. Environ. Sci. Technol. 53, 3802–3810. doi: 10.1021/acs.est.8b06135
Das, A., and Das, B. (2019). “Nanotechnology a potential tool to mitigate abiotic stress in crop plants,” in Abiotic and Biotic Stress in Plants. ed. A. De Oliveira (London, UK: IntechOpen).
Davar Zareii, F., Roozbahani, A., and Hosnamidi, A. (2014). Evaluation the effect of water stress and foliar application of Fe nanoparticles on yield, yield components and oil percentage of safflower (Carthamus tinctorious L.). Int. J. Adv. Biol. Biomed. 16, 1150–1159.
Dimkpa, C. O., White, J. C., Elmer, W. H., and Gardea-Torresdey, J. (2017). Nanoparticle and ionic Zn promote nutrient loading of sorghum grain under low NPK fertilization. J. Agric. Food Chem. 65, 8552–8559. doi: 10.1021/acs.jafc.7b02961
El-Saadony, M. T., Saad, A. M., Najjar, A. A., Alzahrani, S. O., Alkhatib, F. M., Shafi, M. E., et al. (2021). The use of biological selenium nanoparticles to suppress Triticum aestivum L. crown and root rot diseases induced by Fusarium species and improve yield under drought and heat stress. Saudi J. Biol. Sci. 28, 4461–4471. doi: 10.1016/j.sjbs.2021.04.043
Etesami, H., Fatemi, H., and Rizwan, M. (2021). Interactions of nanoparticles and salinity stress at physiological, biochemical and molecular levels in plants: a review. Ecotoxicol. Environ. Saf. 225:112769. doi: 10.1016/j.ecoenv.2021.112769
Fahad, S., Bajwa, A. A., Nazir, U., Anjum, S. A., Farooq, A., Zohaib, A., et al. (2017). Crop production under drought and heat stress: plant responses and management options. Front. Plant Sci. 8:1147. doi: 10.3389/fpls.2017.01147
Farooq, M., Wahid, A., Kobayashi, N., Fujita, D., and Basra, S. M. A. (2009). Plant drought stress: effects, mechanisms and management. Sustain. Agric. 24, 153–188. doi: 10.1007/978-90-481-2666-8_12
Gao, M., Zhou, J., Liu, H., Zhang, W., Hu, Y., Liang, J., et al. (2018). Foliar spraying with silicon and selenium reduces cadmium uptake and mitigates cadmium toxicity in rice. Sci. Total Environ. 631-632, 1100–1108. doi: 10.1016/j.scitotenv.2018.03.047
Ghorbanpour, M., Mohammadi, H., and Kariman, K. (2020). Nanosilicon-based recovery of barley (Hordeum vulgare) plants subjected to drought stress. Environ. Sci. Nano 7, 443–461. doi: 10.1039/C9EN00973F
Godoy, F., Olivos-Hernández, K., Stange, C., and Handford, M. (2021). Abiotic stress in crop species: improving tolerance by applying plant metabolites. Plan. Theory 10:186. doi: 10.3390/plants10020186
Gohari, G., Mohammadi, A., Akbari, A., Panahirad, S., Dadpour, M. R., Fotopoulos, V., et al. (2020). Titanium dioxide nanoparticles (TiO2 NPs) promote growth and ameliorate salinity stress effects on essential oil profile and biochemical attributes of Dracocephalum moldavica. Sci. Rep. 10, 912–914. doi: 10.1038/s41598-020-57794-1
Grover, A., Mittal, D., Negi, M., and Lavania, D. (2013). Generating high temperature tolerant transgenic plants: achievements and challenges. Plant Sci. 205-206, 38–47. doi: 10.1016/j.plantsci.2013.01.005
Guo, B., Hong, C., Tong, W., Xu, M., Huang, C., Yin, H., et al. (2020). Health risk assessment of heavy metal pollution in a soil-rice system: a case study in the Jin-Qu Basin of China. Sci. Rep. 10:11490. doi: 10.1038/s41598-020-68295-6
Haghighi, M., Abolghasemi, R., and da Silva, J. A. T. (2014). Low and high temperature stress affect the growth characteristics of tomato in hydroponic culture with se and nano-se amendment. Sci. Hortic. 178, 231–240. doi: 10.1016/j.scienta.2014.09.006
Haghighi, M., and Pessarakli, M. (2013). Influence of silicon and nano-silicon on salinity tolerance of cherry tomatoes (Solanum lycopersicum L.) at early growth stage. Sci. Hortic. 161, 111–117. doi: 10.1016/j.scienta.2013.06.034
Haider, F. U., Liqun, C., Coulter, J. A., Cheema, S. A., Wu, J., Zhang, R., et al. (2021). Cadmium toxicity in plants: impacts and remediation strategies. Ecotoxicol. Environ. Saf. 211:111887. doi: 10.1016/j.ecoenv.2020.111887
Hasanpour, H., Maali-Amir, R., and Zeinali, H. (2015). Effect of TiO2 nanoparticles on metabolic limitations to photosynthesis under cold in chickpea. Russ. J. Plant Physiol. 62, 779–787. doi: 10.1134/S1021443715060096
Hasanuzzaman, M., Hossain, M. A., Da Silva, J. A. T., and Fujita, M. (2012). “Plant response and tolerance to abiotic oxidative stress: antioxidant defense is a key factor,” in Crop Stress and Its Management: Perspectives and Strategies. eds. P. Ahmad, M. M. Azooz, and M. N. V. Prasad (Springer: Netherlands), 261–315.
Hernández-Hernández, H., González-Morales, S., Benavides-Mendoza, A., Ortega-Ortiz, H., Cadenas-Pliego, G., and Juárez-Maldonado, A. (2018). Effects of chitosan–PVA and cu nanoparticles on the growth and antioxidant capacity of tomato under saline stress. Molecules 23:178. doi: 10.3390/molecules23010178
Horie, M., Nishio, K., Kato, H., Fujita, K., Endoh, S., Nakamura, A., et al. (2011). Cellular responses induced by cerium oxide nanoparticles: induction of intracellular calcium level and oxidative stress on culture cells. J. Biochem. 150, 461–471. doi: 10.1093/jb/mvr081
Hu, Y., and Schmidhalter, U. (2005). Drought and salinity: a comparison of their effects on mineral nutrition of plants. J. Plant Nutr. Soil Sci. 168, 541–549. doi: 10.1002/jpln.200420516
Huang, D., Dang, F., Huang, Y., Chen, N., and Zhou, D. (2022). Uptake, translocation, and transformation of silver nanoparticles in plants. Environ. Sci. Nano 9, 12–39. doi: 10.1039/D1EN00870F
Hussain, A., Ali, S., Rizwan, M., Rehman, M. Z., ur Qayyum, M. F., Wang, H., et al. (2019). Responses of wheat (Triticum aestivum) plants grown in a cd contaminated soil to the application of iron oxide nanoparticles. Ecotoxicol. Environ. Saf. 173, 156–164. doi: 10.1016/j.ecoenv.2019.01.118
Hussain, A., Ali, S., Rizwan, M., Zia ur Rehman, M., Javed, M. R., Imran, M., et al. (2018a). Zinc oxide nanoparticles alter the wheat physiological response and reduce the cadmium uptake by plants. Environ. Pollut. 242, 1518–1526. doi: 10.1016/j.envpol.2018.08.036
Hussain, B., Ashraf, M. N., Shafeeq-ur-Rahman,, Abbas, A., Li, J., and Farooq, M. (2021). Cadmium stress in paddy fields: effects of soil conditions and remediation strategies. Sci. Total Environ. 754:142188. doi: 10.1016/j.scitotenv.2020.142188
Hussain, M., Farooq, S., Hasan, W., Ul-Allah, S., Tanveer, M., Farooq, M., et al. (2018b). Drought stress in sunflower: physiological effects and its management through breeding and agronomic alternatives. Agric Water Manag 201, 152–166. doi: 10.1016/j.agwat.2018.01.028
Iavicoli, I., Leso, V., Beezhold, D. H., and Shvedova, A. A. (2017). Nanotechnology in agriculture: opportunities, toxicological implications, and occupational risks. Toxicol. Appl. Pharmacol. 329, 96–111. doi: 10.1016/j.taap.2017.05.025
Ibrahim, W., Zhu, Y. M., Chen, Y., Qiu, C. W., Zhu, S., and Wu, F. (2019). Genotypic differences in leaf secondary metabolism, plant hormones and yield under alone and combined stress of drought and salinity in cotton genotypes. Physiol. Plant. 165, 343–355. doi: 10.1111/ppl.12862
Ikram, M., Raja, N. I., Javed, B., Mashwani, Z. u. R., Hussain, M., Hussain, M., et al. (2020). Foliar applications of bio-fabricated selenium nanoparticles to improve the growth of wheat plants under drought stress. Green Process. Synth. 9, 706–714. doi: 10.1515/gps-2020-0067
Iqbal, M., Raja, N. I., Mashwani, Z. U. R., Hussain, M., Ejaz, M., and Yasmeen, F. (2019). Effect of silver nanoparticles on growth of wheat under heat stress. Iran. J. Sci. Technol. Trans. A: Sci. 43, 387–395. doi: 10.1007/s40995-017-0417-4
Irshad, M. A., Nawaz, R., ur Rehman, M. Z., Adrees, M., Rizwan, M., Ali, S., et al. (2021b). Synthesis, characterization and advanced sustainable applications of titanium dioxide nanoparticles: a review. Ecotoxicol. Environ. Saf. 212:111978. doi: 10.1016/j.ecoenv.2021.111978
Irshad, M. A., ur Rehman, M. Z., Anwar-ul-Haq, M., Rizwan, M., Nawaz, R., Shakoor, M. B., et al. (2021a). Effect of green and chemically synthesized titanium dioxide nanoparticles on cadmium accumulation in wheat grains and potential dietary health risk: a field investigation. J. Hazard. Mater. 415:125585. doi: 10.1016/j.jhazmat.2021.125585
Jiang, Q. Y., Zhuo, F., Long, S. H., Zhao, H. Di, Yang, D. J., Ye, Z. H., et al. (2016). Can arbuscular mycorrhizal fungi reduce cd uptake and alleviate cd toxicity of Lonicera japonica grown in cd-added soils? Sci. Rep. 6, 1–9. doi: 10.1038/srep21805
Juárez-Maldonado, A., Ortega-Ortíz, H., Pérez-Labrada, F., Cadenas-Pliego, G., and Benavides-Mendoza, A. (2016). Cu nanoparticles absorbed on chitosan hydrogels positively alter morphological, production, and quality characteristics of tomato. J. Appl. Bot. Food Qual. 89, 183–189. doi: 10.5073/JABFQ.2016.089.023
Kazan, K. (2015). Diverse roles of jasmonates and ethylene in abiotic stress tolerance. Trends Plant Sci. 20, 219–229. doi: 10.1016/j.tplants.2015.02.001
Khan, I., Awan, S. A., Raza, M. A., Rizwan, M., Tariq, R., Ali, S., et al. (2021). Silver nanoparticles improved the plant growth and reduced the sodium and chlorine accumulation in pearl millet: a life cycle study. Environ. Sci. Pollut. Res. 28, 13712–13724. doi: 10.1007/s11356-020-11612-3
Khan, F., Hussain, S., Tanveer, M., Khan, S., Hussain, H. A., Iqbal, B., et al. (2018). Coordinated effects of lead toxicity and nutrient deprivation on growth, oxidative status, and elemental composition of primed and non-primed rice seedlings. Environ. Sci. Pollut. Res. 25, 21185–21194. doi: 10.1007/s11356-018-2262-1
Khan, Z. S., Rizwan, M., Hafeez, M., Ali, S., Adrees, M., Qayyum, M. F., et al. (2019a). Effects of silicon nanoparticles on growth and physiology of wheat in cadmium contaminated soil under different soil moisture levels. Environ. Sci. Pollut. Res. 27, 4958–4968. doi: 10.1007/S11356-019-06673-Y
Khan, Z. S., Rizwan, M., Hafeez, M., Ali, S., Adrees, M., Qayyum, M. F., et al. (2020). Effects of silicon nanoparticles on growth and physiology of wheat in cadmium contaminated soil under different soil moisture levels. Environ. Sci. Pollut. Res. 27, 4958–4968. doi: 10.1007/s11356-019-06673-y
Khan, Z. S., Rizwan, M., Hafeez, M., Ali, S., Javed, M. R., and Adrees, M. (2019b). The accumulation of cadmium in wheat (Triticum aestivum) as influenced by zinc oxide nanoparticles and soil moisture conditions. Environ. Sci. Pollut. Res. 26, 19859–19870. doi: 10.1007/s11356-019-05333-5
Kohan-Baghkheirati, E., and Geisler-Lee, J. (2015). Gene expression, protein function and pathways of Arabidopsis thaliana responding to silver nanoparticles in comparison to silver ions, cold, salt, drought, and heat. Nanomaterials 5, 436–467. doi: 10.3390/nano5020436
Konate, A., He, X., Zhang, Z., Ma, Y., Zhang, P., Alugongo, G., et al. (2017). Magnetic (Fe3O4) nanoparticles reduce heavy metals uptake and mitigate their toxicity in wheat seedling. Sustainability 9:790. doi: 10.3390/su9050790
Kumar, S., Prasad, S., Yadav, K. K., Shrivastava, M., Gupta, N., Nagar, S., et al. (2019). Hazardous heavy metals contamination of vegetables and food chain: role of sustainable remediation approaches—a review. Environ. Res. 179:108792. doi: 10.1016/j.envres.2019.108792
Kumar, A., Singh, S., Gaurav, A. K., Srivastava, S., and Verma, J. P. (2020). Plant growth-promoting bacteria: biological tools for the mitigation of salinity stress in plants. Front. Microbiol. 11:1216. doi: 10.3389/fmicb.2020.01216
Kumar, A., and Verma, J. P. (2018). Does plant—microbe interaction confer stress tolerance in plants: a review? Microbiol. Res. 207, 41–52. doi: 10.1016/j.micres.2017.11.004
Lamaoui, M., Jemo, M., Datla, R., and Bekkaoui, F. (2018). Heat and drought stresses in crops and approaches for their mitigation. Front. Chem. 6:26. doi: 10.3389/fchem.2018.00026
Li, Y., Guo, P., Liu, Y., Su, H., Zhang, Y., Deng, J., et al. (2020). Effects of sulfur on the toxicity of cadmium to Folsomia candida in red earth and paddy soil in southern Fujian. J. Hazard. Mater. 387:121683. doi: 10.1016/j.jhazmat.2019.121683
Li, D., Wang, M., Zhang, T., Chen, X., Li, C., Liu, Y., et al. (2021). Glycinebetaine mitigated the photoinhibition of photosystem II at high temperature in transgenic tomato plants. Photosynth. Res. 147, 301–315. doi: 10.1007/s11120-020-00810-2
Lin, S., Reppert, J., Hu, Q., Hudson, J. S., Reid, M. L., Ratnikova, T. A., et al. (2009). Uptake, translocation, and transmission of carbon nanomaterials in rice plants. Small 5, 1128–1132. doi: 10.1002/smll.200801556
Liu, J., Li, G., Chen, L., Gu, J., Wu, H., and Li, Z. (2021). Cerium oxide nanoparticles improve cotton salt tolerance by enabling better ability to maintain cytosolic K+/na+ ratio. J. Nanobiotechnol. 19:153. doi: 10.1186/s12951-021-00892-7
López-Luna, J., Silva-Silva, M. J., Martinez-Vargas, S., Mijangos-Ricardez, O. F., González-Chávez, M. C., Solís-Domínguez, F. A., et al. (2015). Magnetite nanoparticle (NP) uptake by wheat plants and its effect on cadmium and chromium toxicological behavior. Sci. Total Environ. 565, 941–950. doi: 10.1016/j.scitotenv.2016.01.029
Lowry, G. V., Avellan, A., and Gilbertson, L. M. (2019). Opportunities and challenges for nanotechnology in the Agri-tech revolution. Nat. Nanotechnol. 14, 517–522. doi: 10.1038/s41565-019-0461-7
Mahakham, W., Theerakulpisut, P., Maensiri, S., Phumying, S., and Sarmah, A. K. (2016). Environmentally benign synthesis of phytochemicals-capped gold nanoparticles as nanopriming agent for promoting maize seed germination. Sci. Total Environ. 573, 1089–1102. doi: 10.1016/j.scitotenv.2016.08.120
Mahmoud, L. M., Shalan, A. M., El-Boray, M. S., Vincent, C. I., El-Kady, M. E., Grosser, J. W., et al. (2022). Application of silicon nanoparticles enhances oxidative stress tolerance in salt stressed ‘Valencia’ sweet orange plants. Sci. Hortic. 295:110856. doi: 10.1016/j.scienta.2021.110856
Manzoor, N., Ahmed, T., Noman, M., Shahid, M., Nazir, M. M., Ali, L., et al. (2021). Iron oxide nanoparticles ameliorated the cadmium and salinity stresses in wheat plants, facilitating photosynthetic pigments and restricting cadmium uptake. Sci. Total Environ. 769:145221. doi: 10.1016/j.scitotenv.2021.145221
Martínez-Fernández, D., Vítková, M., Bernal, M. P., and Komárek, M. (2015). Effects of nano-maghemite on trace element accumulation and drought response of Helianthus annuus L. in a contaminated mine soil. Water Air Soil Pollut. 226, 1–9. doi: 10.1007/s11270-015-2365-y
Minkina, T., Rajput, V., Fedorenko, G., Fedorenko, A., Mandzhieva, S., Sushkova, S., et al. (2019). Anatomical and ultrastructural responses of Hordeum sativum to the soil spiked by copper. Environ. Geochemistry Heal. 42, 45–58. doi: 10.1007/S10653-019-00269-8
Mohammadi, H., and Ghorbani, M. (2018). Synthesis photocatalytic TiO2/ZnO nanocomposite and investigation through anatase, wurtzite and ZnTiO3 phases antibacterial behaviors. J. Nanopart. Res. 51, 69–77. doi: 10.4028/www.scientific.net/JNanoR.51.69
Mohammadi, H., Hatami, M., Feghezadeh, K., and Ghorbanpour, M. (2018). Mitigating effect of nano-zerovalent iron, iron sulfate and EDTA against oxidative stress induced by chromium in Helianthus annuus L. Acta Physiol. Plant. 40, 1–15. doi: 10.1007/s11738-018-2647-2
Mora, O., Le Mouël, C., de Lattre-Gasquet, M., Donnars, C., Dumas, P., Réchauchère, O., et al. (2020). Exploring the future of land use and food security: a new set of global scenarios. PLoS One 15:e0235597. doi: 10.1371/journal.pone.0235597
Mozafari, A. A., Havas, F., and Ghaderi, N. (2018). Application of iron nanoparticles and salicylic acid in in vitro culture of strawberries (Fragaria × ananassa Duch.) to cope with drought stress. Plant Cell Tiss. Org. Cult. 132, 511–523. doi: 10.1007/s11240-017-1347-8
Nazir, M. M., Li, Q., Noman, M., Ulhassan, Z., Ali, S., Ahmed, T., et al. (2022). Calcium oxide nanoparticles have the role of alleviating arsenic toxicity of barley. Front. Plant Sci. 13:843795. doi: 10.3389/fpls.2022.843795
Nejatzadeh, F. (2021). Effect of silver nanoparticles on salt tolerance of Satureja hortensis l. during in vitro and in vivo germination tests. Heliyon 7:e05981. doi: 10.1016/j.heliyon.2021.e05981
Noman, M., Ahmed, T., Hussain, S., Niazi, M. B. K., Shahid, M., and Song, F. (2020a). Biogenic copper nanoparticles synthesized by using a copper-resistant strain Shigella flexneri SNT22 reduced the translocation of cadmium from soil to wheat plants. J. Hazard. Mater. 398:123175. doi: 10.1016/j.jhazmat.2020.123175
Noman, M., Ahmed, T., Ijaz, U., Hameed, A., Shahid, M., Azizullah, L., et al. (2022). Microbe-oriented nanoparticles as phytomedicines for plant health management: an emerging paradigm to achieve global food security. Crit. Rev. Food Sci. Nutr. 1–21. doi: 10.1080/10408398.2022.2046543 [Epub ahead of print].
Noman, M., Shahid, M., Ahmed, T., Tahir, M., Naqqash, T., Muhammad, S., et al. (2020b). Green copper nanoparticles from a native Klebsiella pneumoniae strain alleviated oxidative stress impairment of wheat plants by reducing the chromium bioavailability and increasing the growth. Ecotoxicol. Environ. Saf. 192:110303. doi: 10.1016/j.ecoenv.2020.110303
Ohama, N., Sato, H., Shinozaki, K., and Yamaguchi-Shinozaki, K. (2017). Transcriptional regulatory network of plant heat stress response. Trends Plant Sci. 22, 53–65. doi: 10.1016/j.tplants.2016.08.015
Oliveira, H. C., Gomes, B. C. R., Pelegrino, M. T., and Seabra, A. B. (2016). Nitric oxide-releasing chitosan nanoparticles alleviate the effects of salt stress in maize plants. Nitric Oxide 61, 10–19. doi: 10.1016/j.niox.2016.09.010
Palmqvist, N. G. M., Seisenbaeva, G. A., Svedlindh, P., and Kessler, V. G. (2017). Maghemite nanoparticles acts as nanozymes, improving growth and abiotic stress tolerance in Brassica napus. Nanoscale Res. Lett. 12:631. doi: 10.1186/s11671-017-2404-2
Parida, A. K., and Das, A. B. (2005). Salt tolerance and salinity effects on plants: a review. Ecotoxicol. Environ. Saf. 60, 324–349. doi: 10.1016/j.ecoenv.2004.06.010
Parihar, P., Singh, S., Singh, R., Singh, V. P., and Prasad, S. M. (2015). Effect of salinity stress on plants and its tolerance strategies: a review. Environ. Sci. Pollut. Res. 22, 4056–4075. doi: 10.1007/s11356-014-3739-1
Pinedo-Guerrero, Z. H., Hernández-Fuentes, A. D., Ortega-Ortiz, H., Benavides-Mendoza, A., Cadenas-Pliego, G., and Juárez-Maldonado, A. (2017). Cu nanoparticles in hydrogels of chitosan-PVA affects the characteristics of post-harvest and bioactive compounds of Jalapeño pepper. Molecules 22:926. doi: 10.3390/molecules22060926
Qi, M., Liu, Y., and Li, T. (2013). Nano-TiO2 improve the photosynthesis of tomato leaves under mild heat stress. Biol. Trace Elem. Res. 156, 323–328. doi: 10.1007/s12011-013-9833-2
Rady, M. M., Desoky, E. S. M., Ahmed, S. M., Majrashi, A., Ali, E. F., Arnaout, S. M. A. I., et al. (2021). Foliar nourishment with nano-selenium dioxide promotes physiology, biochemistry, antioxidant defenses, and salt tolerance in Phaseolus vulgaris. Plan. Theory 10:1189. doi: 10.3390/plants10061189
Rajput, V., Minkina, T., Semenkov, I., Klink, G., Tarigholizadeh, S., and Sushkova, S. (2020). Phylogenetic analysis of hyperaccumulator plant species for heavy metals and polycyclic aromatic hydrocarbons. Environ. Geochemistry Heal. 43, 1629–1654. doi: 10.1007/S10653-020-00527-0
Rajput, V., Minkina, T., Sushkova, S., Behal, A., Maksimov, A., Blicharska, E., et al. (2019). ZnO and CuO nanoparticles: a threat to soil organisms, plants, and human health. Environ. Geochemistry Heal. 42, 147–158. doi: 10.1007/S10653-019-00317-3
Rana, R. A., Siddiqui, M. N., Skalicky, M., Brestic, M., Hossain, A., Kayesh, E., et al. (2021). Prospects of nanotechnology in improving the productivity and quality of horticultural crops. Horticulturae 7, 1–19. doi: 10.3390/horticulturae7100332
Rehman, M. Z. u., Khalid, H., Akmal, F., Ali, S., Rizwan, M., Qayyum, M. F., et al. (2017). Effect of limestone, lignite and biochar applied alone and combined on cadmium uptake in wheat and rice under rotation in an effluent irrigated field. Environ. Pollut. 227, 560–568. doi: 10.1016/j.envpol.2017.05.003
Rehman, M. Z. u., Rizwan, M., Hussain, A., Saqib, M., Ali, S., Sohail, M. I., et al. (2018). Alleviation of cadmium (cd) toxicity and minimizing its uptake in wheat (Triticum aestivum) by using organic carbon sources in cd-spiked soil. Environ. Pollut. 241, 557–565. doi: 10.1016/j.envpol.2018.06.005
Rico, C. M., Majumdar, S., Duarte-Gardea, M., Peralta-Videa, J. R., and Gardea-Torresdey, J. L. (2011). Interaction of nanoparticles with edible plants and their possible implications in the food chain. J. Agric. Food Chem. 59, 3485–3498. doi: 10.1021/jf104517j
Rizwan, M., Ali, S., Ali, B., Adrees, M., Arshad, M., Hussain, A., et al. (2019b). Zinc and iron oxide nanoparticles improved the plant growth and reduced the oxidative stress and cadmium concentration in wheat. Chemosphere 214, 269–277. doi: 10.1016/j.chemosphere.2018.09.120
Rizwan, M., Ali, S., ur Rehman, M. Z., Adrees, M., Arshad, M., Qayyum, M. F., et al. (2019a). Alleviation of cadmium accumulation in maize (Zea mays L.) by foliar spray of zinc oxide nanoparticles and biochar to contaminated soil. Environ. Pollut. 248, 358–367. doi: 10.1016/j.envpol.2019.02.031
Rizwan, M., Ali, S., ur Rehman, M. Z., Riaz, M., Adrees, M., Hussain, A., et al. (2021). Effects of nanoparticles on trace element uptake and toxicity in plants: A review. Ecotoxicol. Environ. Saf. 221:112437. doi: 10.1016/j.ecoenv.2021.112437
Rizwan, M., Meunier, J. D., Miche, H., and Keller, C. (2012). Effect of silicon on reducing cadmium toxicity in durum wheat (Triticum turgidum L. cv. Claudio W.) grown in a soil with aged contamination. J. Hazard. Mater. 209–210, 326–334. doi: 10.1016/j.jhazmat.2012.01.033
Rossi, L., Zhang, W., and Ma, X. (2017). Cerium oxide nanoparticles alter the salt stress tolerance of Brassica napus L. by modifying the formation of root apoplastic barriers. Environ. Pollut. 229, 132–138. doi: 10.1016/j.envpol.2017.05.083
Sabaghnia, N., and Janmohammadi, M. (2015). Effect of nano-silicon particles application on salinity tolerance in early growth of some lentil genotypes. Ann. Univ. Mariae Curie-Sklodowska, Sect. C – Biol. 69:39. doi: 10.17951/c.2014.69.2.39
Savicka, M., and Škute, N. (2010). Effects of high temperature on malondialdehyde content, superoxide production and growth changes in wheat seedlings (Triticum aestivum L.)Aukštos temperatūros poveikis kviečių (Triticum aestivum L.) daigų malondialdehido koncentracijai, superoksido gamybai ir augimui. Ekologija 56, 26–33. doi: 10.2478/v10055-010-0004-x
Shabala, S., and Cuin, T. A. (2008). “Potassium transport and plant salt tolerance,” in Physiologia Plantarum. eds. I. Cakmak and J. K. Schjoerring (Hoboken, New Jersey, U.S.: John Wiley & Sons, Ltd), 651–669.
Shah, A. A., Aslam, S., Akbar, M., Ahmad, A., Khan, W. U., Yasin, N. A., et al. (2021). Combined effect of Bacillus fortis IAGS 223 and zinc oxide nanoparticles to alleviate cadmium phytotoxicity in Cucumis melo. Plant Physiol. Biochem. 158, 1–12. doi: 10.1016/j.plaphy.2020.11.011
Shahid, M. A., Sarkhosh, A., Khan, N., Balal, R. M., Ali, S., Rossi, L., et al. (2020). Insights into the physiological and biochemical impacts of salt stress on plant growth and development. Agron 10:938. doi: 10.3390/AGRONOMY10070938
Shahzad, B., Tanveer, M., Che, Z., Rehman, A., Cheema, S. A., Sharma, A., et al. (2018). Role of 24-epibrassinolide (EBL) in mediating heavy metal and pesticide induced oxidative stress in plants: a review. Ecotoxicol. Environ. Saf. 147, 935–944. doi: 10.1016/j.ecoenv.2017.09.066
Shankramma, K., Yallappa, S., Shivanna, M. B., and Manjanna, J. (2016). Fe2O3 magnetic nanoparticles to enhance S. lycopersicum (tomato) plant growth and their biomineralization. Appl. Nanosci. 6, 983–990. doi: 10.1007/s13204-015-0510-y
Sharma, P., Jha, A. B., Dubey, R. S., and Pessarakli, M. (2012). Reactive oxygen species, oxidative damage, and antioxidative defense mechanism in plants under stressful conditions. J. Bot. 2012, 1–26. doi: 10.1155/2012/217037
Singh, S., Tripathi, D. K., Singh, S., Sharma, S., Dubey, N. K., Chauhan, D. K., et al. (2017). Toxicity of aluminium on various levels of plant cells and organism: a review. Environ. Exp. Bot. 137, 177–193. doi: 10.1016/j.envexpbot.2017.01.005
Song, G., Gao, Y., Wu, H., Hou, W., Zhang, C., and Ma, H. (2012). Physiological effect of anatase TiO2 nanoparticles on Lemna minor. Environ. Toxicol. Chem. 31, 2147–2152. doi: 10.1002/etc.1933
Sun, D., Hussain, H. I., Yi, Z., Rookes, J. E., Kong, L., and Cahill, D. M. (2017). Delivery of abscisic acid to plants using glutathione responsive mesoporous silica nanoparticles. J. Nanosci. Nanotechnol. 18, 1615–1625. doi: 10.1166/jnn.2018.14262
Thakur, S., Asthir, B., Kaur, G., Kalia, A., and Sharma, A. (2021). Zinc oxide and titanium dioxide nanoparticles influence heat stress tolerance mediated by antioxidant defense system in wheat. Cereal Res. Commun. 49, 1–12. doi: 10.1007/s42976-021-00190-w
Tiwari, S., Lata, C., Chauhan, P. S., and Nautiyal, C. S. (2016). Pseudomonas putida attunes morphophysiological, biochemical and molecular responses in Cicer arietinum L. during drought stress and recovery. Plant Physiol. Biochem. 99, 108–117. doi: 10.1016/j.plaphy.2015.11.001
Tripathi, D. K., Singh, V. P., Gangwar, S., Prasad, S. M., Maurya, J. N., and Chauhan, D. K. (2014). “Role of silicon in enrichment of plant nutrients and protection from biotic and abiotic stresses,” in Improvement of Crops in the Era of Climatic Changes. Vol. 1. eds. P. Ahmad, M. R. Wani, M. M. Azooz, and L.-S. P. Tran (New York: Springer), 39–56.
Tripathi, D. K., Singh, V. P., Prasad, S. M., Chauhan, D. K., and Dubey, N. K. (2015). Silicon nanoparticles (SiNp) alleviate chromium (VI) phytotoxicity in Pisum sativum (L.) seedlings. Plant Physiol. Biochem. 96, 189–198. doi: 10.1016/j.plaphy.2015.07.026
Wahid, I., Rani, P., Kumari, S., Ahmad, R., Hussain, S. J., Alamri, S., et al. (2022). Biosynthesized gold nanoparticles maintained nitrogen metabolism, nitric oxide synthesis, ions balance, and stabilizes the defense systems to improve salt stress tolerance in wheat. Chemosphere 287:132142. doi: 10.1016/j.chemosphere.2021.132142
Wang, X. (2012). Nanomaterials as sorbents to remove heavy metal ions in wastewater treatment. J. Environ. Anal. Toxicol. 2:7. doi: 10.4172/2161-0525.1000154
Wang, P., Lombi, E., Zhao, F. J., and Kopittke, P. M. (2016). Nanotechnology: a new opportunity in plant sciences. Trends Plant Sci. 21, 699–712. doi: 10.1016/j.tplants.2016.04.005
Wang, P., Menzies, N. W., Lombi, E., McKenna, B. A., Johannessen, B., Glover, C. J., et al. (2013). Fate of ZnO nanoparticles in soils and cowpea (Vigna unguiculata). Environ. Sci. Technol. 47, 13822–13830. doi: 10.1021/es403466p
White, J. C., and Gardea-Torresdey, J. (2018). Achieving food security through the very small. Nat. Nanotechnol. 13, 627–629. doi: 10.1038/s41565-018-0223-y
Wu, H., Tito, N., and Giraldo, J. P. (2017). Anionic cerium oxide nanoparticles protect plant photosynthesis from abiotic stress by scavenging reactive oxygen species. ACS Nano 11, 11283–11297. doi: 10.1021/acsnano.7b05723
Wu, J., and Wang, T. (2020). Synergistic effect of zinc oxide nanoparticles and heat stress on the alleviation of transcriptional gene silencing in Arabidopsis thaliana. Bull. Environ. Contam. Toxicol. 104, 49–56. doi: 10.1007/s00128-019-02749-0
Xu, D. M., Fu, R. B., Liu, H. Q., and Guo, X. P. (2021). Current knowledge from heavy metal pollution in Chinese smelter contaminated soils, health risk implications and associated remediation progress in recent decades: a critical review. J. Clean. Prod. 286:124989. doi: 10.1016/j.jclepro.2020.124989
Xu, Z., Jiang, Y., Jia, B., and Zhou, G. (2016). Elevated-CO2 response of stomata and its dependence on environmental factors. Front. Plant Sci. 7:657. doi: 10.3389/fpls.2016.00657
Yasmin, H., Mazher, J., Azmat, A., Nosheen, A., Naz, R., Hassan, M. N., et al. (2021). Combined application of zinc oxide nanoparticles and biofertilizer to induce salt resistance in safflower by regulating ion homeostasis and antioxidant defence responses. Ecotoxicol. Environ. Saf. 218:112262. doi: 10.1016/j.ecoenv.2021.112262
Ye, Y., Cota-Ruiz, K., Hernández-Viezcas, J. A., Valdés, C., Medina-Velo, I. A., Turley, R. S., et al. (2020). Manganese nanoparticles control salinity-modulated molecular responses in Capsicum annuum L. through priming: a sustainable approach for agriculture. ACS Sustain. Chem. Eng. 8, 1427–1436. doi: 10.1021/acssuschemeng.9b05615
Zhao, G., Zhao, Y., Lou, W., Su, J., Wei, S., Yang, X., et al. (2019). Nitrate reductase-dependent nitric oxide is crucial for multi-walled carbon nanotube-induced plant tolerance against salinity. Nanoscale 11, 10511–10523. doi: 10.1039/C8NR10514F
Zhou, H., Wu, H., Zhang, F., Su, Y., Guan, W., Xie, Y., et al. (2021). Molecular basis of cerium oxide nanoparticle enhancement of rice salt tolerance and yield. Environ. Sci. Nano 8, 3294–3311. doi: 10.1039/D1EN00390A
Zhu, H., Han, J., Xiao, J. Q., and Jin, Y. (2008). Uptake, translocation, and accumulation of manufactured iron oxide nanoparticles by pumpkin plants. J. Environ. Monit. 10, 713–717. doi: 10.1039/b805998e
Zia, R., Nawaz, M. S., Siddique, M. J., Hakim, S., and Imran, A. (2021). Plant survival under drought stress: implications, adaptive responses, and integrated rhizosphere management strategy for stress mitigation. Microbiol. Res. 242:126626. doi: 10.1016/j.micres.2020.126626
Keywords: abiotic stresses, drought, heavy metals, salinity, nanofertilizers
Citation: Manzoor N, Ali L, Ahmed T, Noman M, Adrees M, Shahid MS, Ogunyemi SO, Radwan KSA, Wang G and Zaki HEM (2022) Recent Advancements and Development in Nano-Enabled Agriculture for Improving Abiotic Stress Tolerance in Plants. Front. Plant Sci. 13:951752. doi: 10.3389/fpls.2022.951752
Received: 24 May 2022; Accepted: 20 June 2022;
Published: 11 July 2022.
Edited by:
Adalberto Benavides-Mendoza, Universidad Autónoma Agraria Antonio Narro, MexicoReviewed by:
Balal Yousaf, University of Science and Technology of China, ChinaCopyright © 2022 Manzoor, Ali, Ahmed, Noman, Adrees, Shahid, Ogunyemi, Radwan, Wang and Zaki. This is an open-access article distributed under the terms of the Creative Commons Attribution License (CC BY). The use, distribution or reproduction in other forums is permitted, provided the original author(s) and the copyright owner(s) are credited and that the original publication in this journal is cited, in accordance with accepted academic practice. No use, distribution or reproduction is permitted which does not comply with these terms.
*Correspondence: Gang Wang, Z2FuZ3dhbmdAY2F1LmVkdS5jbg==; Haitham E. M. Zaki, aGFpdGhhbS56YWtpQG11LmVkdS5lZw==
†These authors have contributed equally to this work
Disclaimer: All claims expressed in this article are solely those of the authors and do not necessarily represent those of their affiliated organizations, or those of the publisher, the editors and the reviewers. Any product that may be evaluated in this article or claim that may be made by its manufacturer is not guaranteed or endorsed by the publisher.
Research integrity at Frontiers
Learn more about the work of our research integrity team to safeguard the quality of each article we publish.