- 1Forest Ecology and Stable Isotope Center, College of Forestry, Fujian Agriculture and Forestry University, Fuzhou, China
- 2College of Natural Resources Management and Tourism, Mwalimu Julius K. Nyerere University of Agriculture and Technology, Musoma, Tanzania
- 3Department of Geography, Minjiang University, Fuzhou, China
Subtropical regions are currently experiencing a dramatic increase in nitrogen (N) deposition; however, the contributions of nitrification and denitrification processes to soil N2O emissions and the underlying mechanisms under increasing N deposition remain unclear. Therefore, a 15N-tracing laboratory experiment with four N application rates (0, 12.5, 25, and 50 μg 15N g–1 soil) was conducted to investigate the response of nitrification- and denitrification-derived N2O to N additions in an evergreen broad-leaved forest (BF) and a Pinus forest (PF) in the Wuyi Mountains in southeastern China. Moreover, the abundance of functional genes related to nitrification (amoA), denitrification (nirK, nirS, and nosZ), and soil properties were measured to clarify the underlying mechanisms. Results showed that nitrification-derived N2O emissions were generally decreased with increasing N input. However, denitrification-derived N2O emissions were a non-linear response to N additions, with maximum N2O emissions at the middle N application rate. Denitrification-derived N2O was the dominant pathway of N2O production, accounting for 64 to 100% of the total N2O fluxes. Soil NH4+-N content and pH were the predominant factors in regulating nitrification-derived N2O emissions in BF and PF, respectively. Soil pH and the nirS abundance contributed the most to the variations of denitrification-derived N2O emissions in BF and PF, respectively. Our results suggest that N application has the potential to increase the contribution of denitrification to N2O production in subtropical forest soils. Changes in soil chemical properties induced by N addition are more important than the abundance of nitrification and denitrification functional genes in regulating soil nitrification- and denitrification-derived N2O emissions.
Introduction
Nitrogen (N) deposition is continuously increasing primarily due to increased anthropogenic fossil fuel combustion, industrialization, and N fertilizer application. Elevated N deposition is expected to not only alter N cycling in forest ecosystems but also enhance N gas loss from the soils (Lu et al., 2011; Fang et al., 2015; Niu et al., 2016; Tang et al., 2018). As one of the primary forms of N gas loss from the soils, nitrous oxide (N2O) is more than 298 times as effective at trapping atmospheric heat as CO2 and is also one of the largest stratospheric ozone-depleting substances (IPCC, 2013). Terrestrial ecosystems contribute ∼60% of the global N2O emissions (IPCC, 2013), of which about 15 to 55% are from tropical and subtropical forests that are believed to be the largest N2O terrestrial source (Solomon, 2007; Zhang et al., 2019). Moreover, N deposition in tropical and subtropical areas has been steadily increasing in recent decades (ranging from 30 to 73 kg N ha–1 year–1), and this trend is projected to continue over the coming decades (Zhang et al., 2008; Liu et al., 2013; Zhu et al., 2015).
N2O is produced primarily during the soil microbial processes of nitrification and denitrification, the rates of which are influenced by the inputs of N. Recent studies in subtropical forests demonstrated that the contribution of nitrification-derived N2O to the total N2O emissions was ≤50% and that of denitrification were 53 to 100% under aerobic conditions (Zhang et al., 2011; Zhu et al., 2013a; Han et al., 2018; Tang et al., 2018). In addition, the rates of denitrification have generally increased following N application (Zhu et al., 2013b; Morse et al., 2015; Niu et al., 2016; Han et al., 2018). However, few studies have focused on the response of nitrification-derived N2O emissions to N application, especially in subtropical forests (Han et al., 2018). Therefore, whether the response of nitrification-derived N2O emissions to N application is more pronounced than that of denitrification-derived N2O emissions or whether the contribution of denitrification to N2O production under elevated N input is still dominant over that of nitrification remains unclear. Thus, quantifying the underlying nitrification and denitrification contributions to the N2O production will help us understand the changes in the N transformation process with increasing N input in forest ecosystems.
Soil nitrification and denitrification can be influenced by different soil microbial groups, soil properties, and climate conditions (Levy-Booth et al., 2014; Cheng et al., 2019). Ammonia oxidation is not only the first but also the rate-limiting step of nitrification, which is performed by both ammonia-oxidizing archaea (AOA) and bacteria (AOB) (Chen and Peng, 2020). On the other hand, denitrification is a four-step microbial facilitated reduction process, whereby NO3– is reduced to NO2–, NO, N2O, and eventually to N2. The first step for the gas production during denitrification is the reduction of NO2– to NO, which is catalyzed by nitrite reductases encoded by nirK and nirS genes (Levy-Booth et al., 2014; Hu et al., 2015). N2O can be further reduced to N2 by nitrous oxide reductases, which are encoded by nosZ genes (Levy-Booth et al., 2014). While the importance of nitrification and denitrification microorganisms in regulating soil N2O emission has been widely accepted (Chen and Peng, 2020), to what extent the abundance of nitrifier and denitrifier functional gene can be a good predictor of N2O emissions under elevated N inputs remain unclear. Moreover, several recent studies have reported that soil environmental factors (e.g., NH4+, NO3–, pH, TN/TP, and TC/TN) can explain better the changes in soil nitrification and denitrification activities than soil nitrification and denitrification functional genes in fertilized forest soils (Pett-Ridge et al., 2013; Shcherbak et al., 2014; Tang et al., 2018, 2019). Although tropical and subtropical forest soils are the largest N2O terrestrial sources (Solomon, 2007; Soper et al., 2018; Zhang et al., 2019), only a few studies so far in these regions have attempted to directly link the nitrification- and denitrification-derived N2O emissions in fertilized soils to N functional genes (Tang et al., 2018, 2019), and more importantly, to investigate the relative contribution of soil biotic and abiotic factors on the nitrification- and denitrification-derived N2O emissions. Greater insight into the regulation of these processes can help us understand the underlying mechanisms and adopt management practices to lower N2O emissions.
To evaluate the changes in soil N2O emissions resulting from nitrification and denitrification processes under N application in subtropical forests and clarify the underlying mechanisms, we carried out a 15N-NO3– and 15N-NH4+ labeling experiment under laboratory conditions. Mineral soils were collected from two subtropical forests, an evergreen broad-leaved forest (BF), and a Pinus forest (PF), which are located in the Wuyi Mountains in southeastern China. The evergreen BF was chosen because it is a product of the monsoon climate and it represents the climax vegetation type of subtropical regions (Zhou et al., 2013). Pinus forest was selected because of its strong contribution to afforestation purposes since the 1980s. It is worth highlighting that the selected pine species have a strong ability to adapt to poor soil conditions in the hilly red soil region of south China, which faced moderate to intense soil and water loss challenges in the 1980s. The forests have been widely planted since that time and account for about 30.5% of the subtropical forests in south China (Cao et al., 2015). Many previous studies have demonstrated that broadleaf/deciduous species produce more N2O than conifers due to a large difference in the production and reduction rates of N2O under the broadleaf/deciduous species (Zhang et al., 2008; Qin et al., 2019). However, some studies reported that coniferous stands emit more N2O than the adjacent deciduous stands that were affected by N deposition. Therefore, the role of different forest types (or tree species) in regulating N2O emissions remains unclear.
This study aimed to (1) investigate the responses of nitrification- and denitrification-derived N2O emissions to elevated N inputs, (2) clarify the dominant factors that control nitrification- and denitrification-derived N2O emissions, and (3) compare the differences in nitrification- and denitrification-derived N2O emissions between the BF and PF. We hypothesized that (1) nitrification- and denitrification-derived N2O emissions would be enhanced with the increased N application and that denitrification may contribute more to the increase in N2O emissions than nitrification, (2) the abundances of functional genes could be more important than soil environmental factors in regulating nitrification- and denitrification-derived N2O emissions, and (3) the nitrification- and denitrification-derived N2O emissions would be greater in the BF than the PF.
Materials and Methods
Study Site Descriptions and Soil Sampling Procedures
The experimental soil samples were collected at the Wuyishan National Nature Reserve (WNNR), located in Fujian Province (27°33′–27°54′N, 117°27′–117°51′E) in southern China. The climate of the WNNR (area: 56,527 ha) is humid monsoon, which is mainly characterized by the mean annual precipitation and temperature of 2,000 mm and 15°C, respectively, as well as 83.5% relative humidity and 100 fog days year–1 (Xu et al., 2010; Wang et al., 2013). The WNNR has two typical vegetation types, that is, evergreen BF and pine forest (PF). The BF and PF are located at about 550 m a.s.l. and 1,100 m a.s.l., respectively. While Castanopsis carlesii Hay and Castanopsis eyrie Tutch are the dominant species in the BF, Pinus taiwanensis Hayata is the dominant species in the PF (Xu et al., 2010; Lyu et al., 2019). Background atmospheric N deposition in the WNNR is about 8 kg N ha–1 year–1 (Xu et al., 2016; Gurmesa et al., 2022).
In April 2018, three 20 m × 20 m plots without N addition (plots were about 10 m away from each other) were randomly established in each vegetation type, and soil samples were collected with a soil core sampler (8 cm in diameter) from the surface soil layer (0–20 cm) after litter removal. Ten soil cores were randomly collected from each plot and there were a total of 30 soil cores collected in each forest stand. Soil samples from the same forest type were thoroughly mixed to form a composite sample. All soil samples were sieved through a 2-mm mesh sieve and were then divided into two sub-samples. The first sub-sample was maintained at 4°C for 1 week before being used for the incubation experiment. The second sub-sample was air-dried for the measurements of soil chemical properties. The soil at both sites is classified as yellow-red soil based on the Chinese Soil Classification System, equivalent to an Ultisol according to the United States Department of Agriculture Soil Taxonomy classification system (Lyu et al., 2019). Soil chemical properties were shown in Table 1. The concentrations of soil SOC, TN, NH4+-N, and NO3–-N in the PF were significantly higher than those in the BF, whereas soil pH and δ15N were lower in the PF and no significant difference was found in the soil C/N ratio in these two forest soils (Table 1).
15N-Tracing Experiment and Gas Sampling Procedures
This study used a completely randomized factorial design experiment that consisted of four N application rate treatments (0, 12.5, 25, and 50 μg 15N g–1 soil equivalent to 0, 16, 32, and 64 kg N ha–1 year–1). We applied N as 15NH4NO3 (99.10 atom %) and NH415NO3 (99.21 atom %). The rates of N application were in the range of those of N deposition (10 to 73 kg N ha–1 year–1) in subtropical forests (Zhang et al., 2011, 2019). For each forest soil type, 120 glass jars (500 mL) were prepared and divided into two groups: one group comprised of 60 glass jars containing 15NH4NO3 (4 N addition rates × 3 replications per N application rate × 5 destructive soil sampling) and the other group consisted of 60 glass jars containing NH415NO3. A total of 30 g (oven-dry basis) of fresh soil was weighed into each glass jar and pre-incubated for 7 days at 25°C to allow the recovery of soil microbial activities. Then, 15NH4NO3 or NH415NO3 was dissolved in 4 mL deionized water and added to the soil at a rate of 0, 12.5, 25, and 50 μg 15NH4+-N g–1 soil or 15NO3–-N g–1 soil. Soil without N addition was regarded as a control and was added with the same volume of deionized water to ensure its similarity in soil moisture content with the N-added soil. The soils were then incubated for 15 days at 25°C and their moisture content was maintained at 60% water-holding capacity throughout the entire experiment. Soils were corrected for water loss by weighing each glass jar plus soil every week and deionized water was added as required to maintain constant soil moisture. During gas sampling, three glass jars at each level of N treatment were randomly selected. The glass jars were closed with the gas-tight rubber lids, and gas sampling tubes were fixed into the middle of the rubber lids to enable the collection of the gas samples. Gas samples (20 mL) were collected from each glass jar with a 3-way stopcocks syringe on days 1, 2, 4, 9, and 15 after N application. Five millimeters were taken from each gas sample to determine the N2O concentration (Shimadzu, GC-2014C, Japan) and the remaining 15 mL were used to determine the N2O isotopic composition (IRMS, Isoprime 100, United Kingdom). Before each gas sampling, the glass jars were opened and flushed with ambient air for approximately 30 min and then resealed with stoppers for 30 min.
Quantification of the Contributions of Nitrification and Denitrification to N2O Emissions
The fractions of N2O derived from denitrification (FD) and nitrification (FN) processes were calculated using the following equations from Stevens et al. (1997):
where am is the 15N atom % of N2O, an is the 15N atom % of N2O from the nitrification process (assumed to be equivalent to 15N atom % of the soil NH4+), and ad is the 15N atom % of N2O from denitrification (assumed to be equivalent to 15N atom % of the soil NO3–).
Soil Inorganic N, Net Nitrification Rate, Dissolved Organic Carbon, Dissolved Organic Nitrogen, and pH Measurements
Soil samples were also collected after gas sampling. Five times destructive soil sampling was used for the analysis of the soil NH4+ and NO3– contents, as well as the δ15N values of soil NH4+ and NO3–. Dissolved organic carbon (DOC) and nitrogen (DON) contents and soil pH were also determined at the end of the experiment. Soil NH4+ and NO3– contents were extracted using 2 mol L–1 KCl for 1 h and then the extracted solutions were divided into two parts. One part was reacted with sodium salicylate (for NH4+ solution) and hydrazine sulfate solution (for NO3– solution) and measured, respectively, at 660 and 550 nm using a discrete wet chemistry analyzer (SmartChem 200, AMS Alliance, Italy) to determine the concentrations of soil NH4+-N and NO3–-N. The other part was used for 15N measurements by distillation using MgO and Devarda’s alloy (Zhang et al., 2011). The DOC in the soil was extracted with 2 mol L–1 KCl at 1:5 w/v and measured using a TOC-TN analyzer (Shimadzu TOC, Japan). The soil DON concentration was determined by calculating the difference between soil total dissolved nitrogen (2 mol L–1 KCl extracted at 1:5 w/v) and soil mineral nitrogen (Jones and Willett, 2006). Soil pH was measured at a 1:2.5 soil to solution ratio using a pH detector (PHS-3C, Sheng Ci, Shanghai, China).
Quantification of the amoA, nirK, nirS, and nosZ Gene Abundances
At the end of the incubation experiment, soil samples for molecular analyses were immediately stored at −80°C before the aforementioned analysis. DNA was extracted from 0.3 g of frozen soils using a Mo Bio PowerSoil DNA Isolation Kit (Mo Bio Laboratories, Carlsbad, CA, United States) according to the manufacturer’s protocol. The concentration and purity of the extracted DNA were determined with a spectrophotometer at 260 nm (NanoDrop Technologies, United States). All extracted soil DNA samples were stored at −80°C before analysis. Quantitative polymerase chain reaction (qPCR) was used to estimate the abundance of nitrification and denitrification functional genes (amoA, nirK, nirS, and nosZ) using a real-time PCR detection system. The PCR primers used in this study are listed in Supplementary Table 1. The 10-fold serially diluted plasmids carrying each target gene were subjected to real-time PCR assays in triplicate to generate a standard curve. The qPCR efficiencies for AOA-amoA, AOB-amoA, nirK, nirS, and nosZ were 0.85, 0.94, 0.96, 0.91, and 0.87, respectively. The corresponding determination coefficients of the standard curve for AOA-amoA, AOB-amoA, nirK, nirS, and nosZ were 0.998, 0.993, 0.990, 0.999, and 0.996, respectively.
Statistical Analyses
The gene copy numbers were log-transformed to meet the homogeneity of variance requirements. Three-way ANOVA was used to examine the effects of forest type, N application rate, sampling date, and their interactions on soil N2O flux, nitrification-, and denitrification-derived N2O emissions, as well as on soil NH4+-N, NO3–-N and their ratio (NH4+-N/NO3–-N). Two-way ANOVA with Duncan’s test was performed to determine the effects of forest type, N application rate, and their interactions on the soil properties (soil pH, DOC, and DON) and the abundances of soil nitrification and denitrification functional genes (amoA, nirK, nirS, and nosZ). All of the above statistical analyses were performed using SPSS 16.0 (SPSS Inc., Champaign, IL, United States).
To estimate the direct and indirect effects of soil biotic and abiotic factors on soil nitrification- and denitrification-derived N2O emissions in the BF and MF, structural equation models (SEM) were performed based on our knowledge of soil biotic and abiotic properties in relation to soil nitrification- and denitrification-derived N2O. Before the SEM analyses, the units of the predictor and dependent parameters were adjusted to obtain comparable parameter variances according to the standardized method. The quality of the SEM model was assessed by using a low χ2 value and P > 0.05, the root-mean-square error of approximation (RMSEA, the RMSEA ≤0.08 indicates a model fit), and the model fit index (GFI > 0.90). All SEM analyses were using Amos 17.0 (IBM, SPSS, NY, United States).
Results
Effects of N Addition on Total and Nitrification- and Denitrification-Derived N2O Emissions
Soil N2O fluxes decreased with increasing incubation period (Figure 1) and were significantly affected by forest type, N application rate, sampling date, and their interactions (Table 2). The N2O flux values in the BF ranged from 0.05 to 3.85 μg N kg–1 day–1 throughout the entire incubation period (Figure 1), with the average fluxes across all the treatments being about 2.4 times greater than those in the PF (Figure 1). Non-linear responses of the soil N2O fluxes to N addition were observed in both the BF and the PF. Specifically, the highest amount of soil N2O emissions was noted at low and moderate N applications in the BF and PF, respectively (Figure 2A).
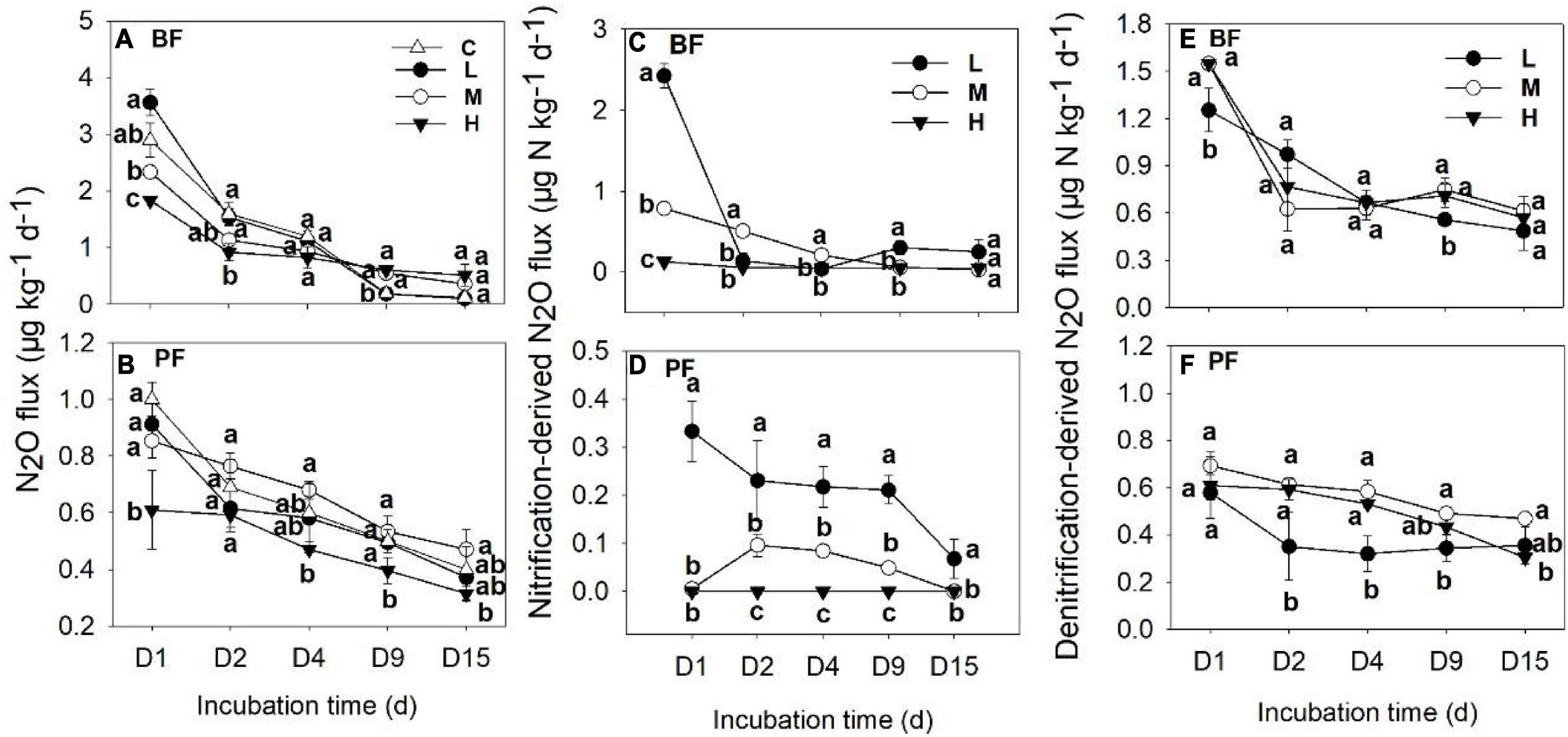
Figure 1. Dynamics of soil N2O fluxes (A,B), nitrification- (C,D), and denitrification-derived (E,F) soil N2O fluxes under different treatments. Values are means ± standard error (n = 3). Different lowercase letters for the same incubation time represent significant differences between different treatments. C, L, M, and H represent N application rates of 0, 12.5, 25, and 50 μg 15N g–1 soil, respectively. BF and PF indicate broad-leaved forest and Pinus forest, respectively.

Table 2. P-values from Repeated-measure ANOVA of the effects of forest type (FT), N application rates (N), sampling date (D), and their interaction on the N2O fluxes, nitrification-, and denitrification-derived N2O emissions, soil NO3-N, and NH4+-N, n = 3.
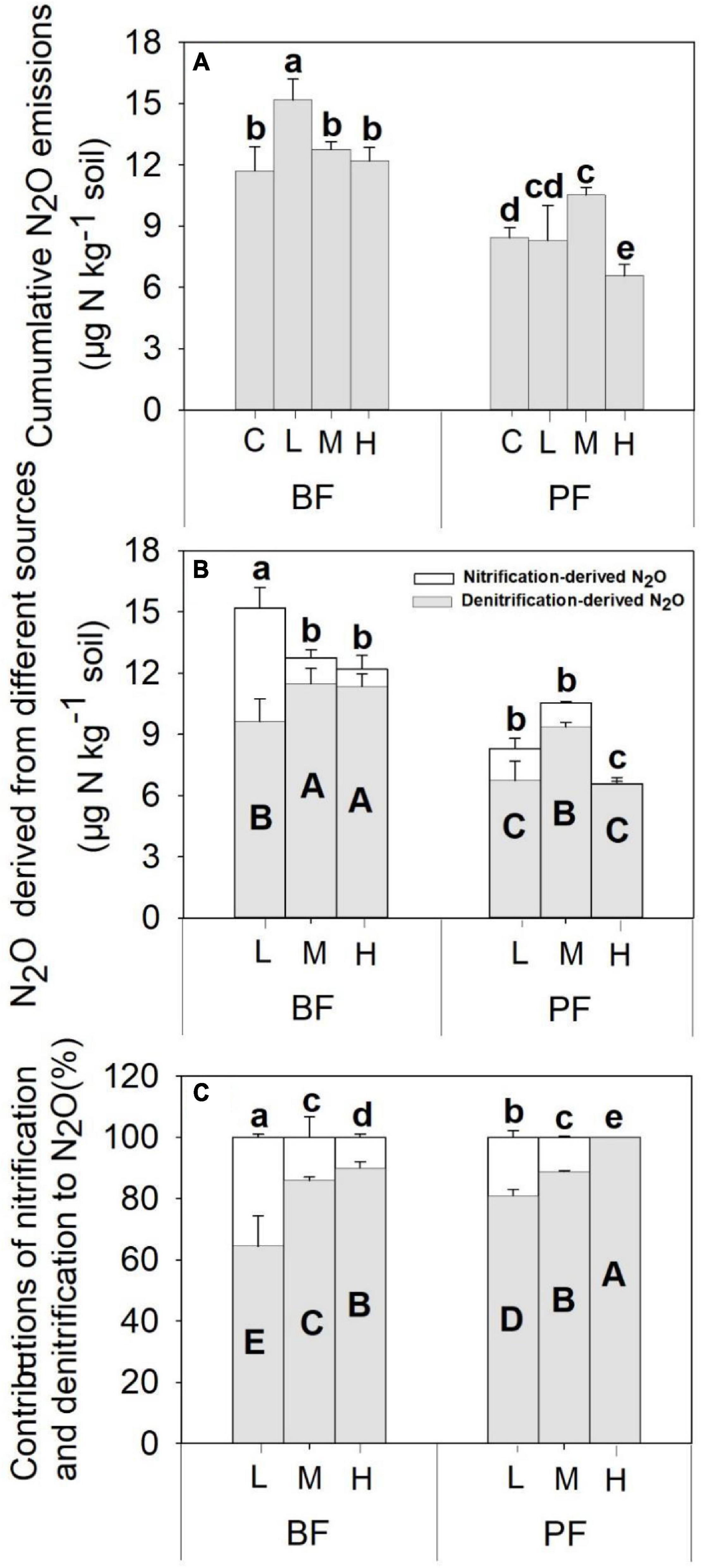
Figure 2. Nitrification-(A) and denitrification-(B) derived soil N2O emissions, as well as the contributions of nitrification and denitrification to total soil N2O emissions (C), under different treatments. Values are means ± standard error (n = 3). Different lowercase letters indicate that the nitrification-derived N2O differed significantly between treatments (P < 0.05). Different uppercase letters indicate that the denitrification-derived N2O differed significantly between treatments (P < 0.05). C, L, M, and H represent N application rates of 0, 12.5, 25, and 50 μg 15N g–1 soil, respectively. BF and PF represent the broad-leaved forest and Pinus forest, respectively.
The application rates of N fertilizer altered not only the total amount of soil N2O fluxes but also the nitrification- and denitrification-derived N2O fluxes (Figures 1, 2). The nitrification- and denitrification-derived N2O emissions were significantly higher in the BF than in the PF (Figure 2B). The nitrification-derived N2O fluxes were in the range of 0.03 to 2.66 μg N kg–1 day–1 and 0 to 0.45 μg N kg–1 day–1 in the BF and PF, respectively (Figures 1C,D), and these fluxes decreased significantly as N application rates increased in both forests. At high rates of N addition, the decrease in nitrification-derived N2O fluxes was approximately 84.6% in the BF and 100% in the PF relative to those observed at low N addition rates (Figure 2B). The denitrification-derived N2O fluxes were in the range of 0.4 to 1.7 μg N kg–1 day–1 and 0.2 to 0.8 μg N kg–1 day–1 in the BF and PF, respectively (Figures 1E,F) and maximum average of denitrification-derived N2O emissions was observed in middle N application rate in both BF and PF (Figure 2B). Moreover, denitrification was the dominant pathway of soil N2O production in the present study, accounting for 64 to 90% and 81 to 100% of the total N2O fluxes in the BF and PF, respectively (Figure 2C).
Effects of N Addition on Soil Inorganic N, pH, Dissolved Organic Carbon, and Dissolved Organic Nitrogen
Soil NO3–-N and NH4+-N contents were significantly affected by forest type and N application rate (Table 2). The soil NO3–-N and NH4+-N contents increased from day 1 to day 9 and then decreased significantly on the last day of the incubation experiment (Figure 3). These soil inorganic N contents increased with increasing N application rates, and they were significantly higher in the PF than in the BF (Figures 3A–D). Soil NH4+-N contents in the control treatments were, respectively, ∼9- and ∼7-fold greater in the BF and PF compared with soil NO3–-N contents (Figures 3E,F). Moreover, the ratio of soil NH4+-N to soil NO3–-N decreased with increasing N inputs. High N application significantly decreased soil pH in both forests. While high N addition significantly decreased soil DON content in PF, no significant effect of N addition on soil DOC was observed in this forest type (Figure 4).
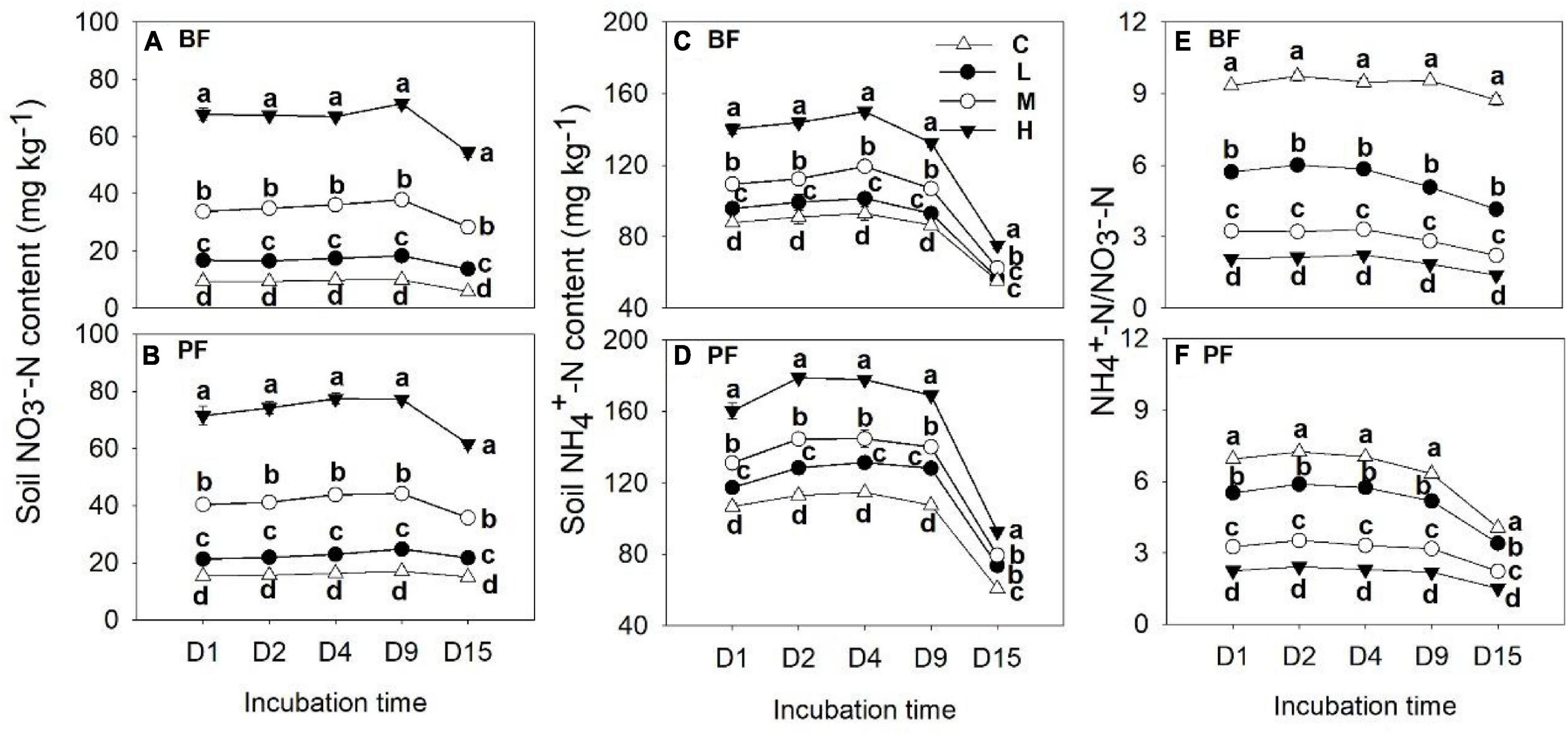
Figure 3. Dynamics of soil NO3–-N (A,B) and NH4+-N contents (C,D) and soil NH4+-N to NO3–-N ratio (E,F) under different treatments. Values are means ± standard error (n = 3). Different lowercase letters indicate that the soil NO3–-N and NH4+-N contents and their ratios differed significantly among treatments at each sampling time (P < 0.05). C, L, M, and H represent N application rates of 0, 12.5, 25, and 50 μg 15N g–1 soil, respectively. BF and PF represent the broad-leaved forest and Pinus forest, respectively.
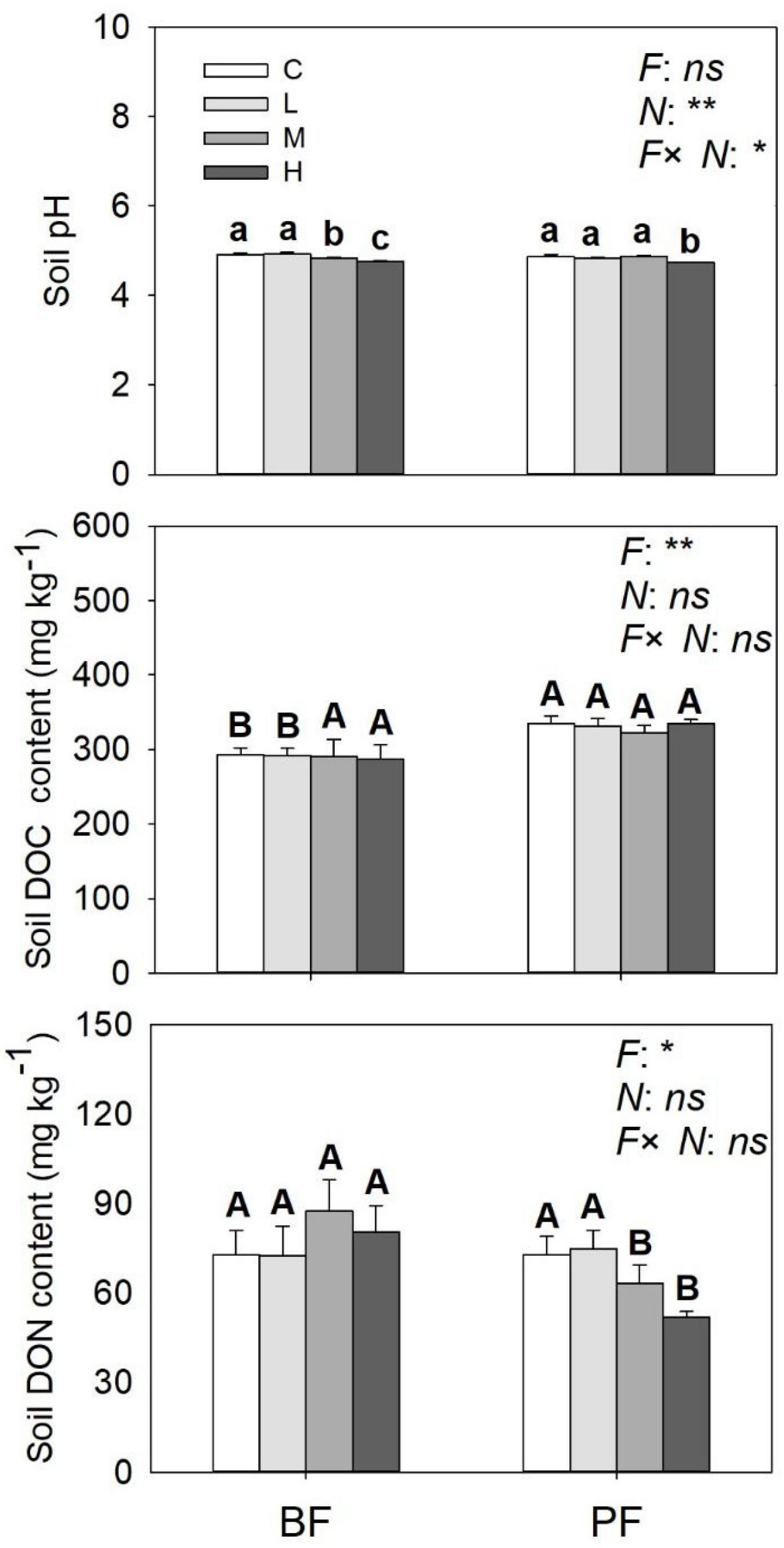
Figure 4. Soil pH, soil DOC, and DON contents under different treatments. Values are means ± standard error (n = 3). Different lowercase letters indicate that soil pH, soil DOC, and DON contents differed significantly between treatments (P < 0.05). Different uppercase letters indicate that soil pH, soil DOC, and DON were significantly different between the two forest soils (P < 0.05). C, L, M, and H represent N application rates of 0, 12.5, 25, and 50 μg 15N g–1 soil, respectively. BF and PF represent the broad-leaved forest and Pinus forest, respectively. F, N, and F × N represent the effects of forest type, N application, and their interactions on these indicators. * and ** represent the effects of forest type or N application or their interactions on these indicators were significant at P < 0.05 and P < 0.01, respectively. ns indicate their effects were not significant at P < 0.05.
Effects of N Addition on the Abundances of Nitrification and Denitrification Functional Genes
While the abundances of AOA- and AOB-amoA genes in the BF and PF were not significantly affected by N inputs (Figures 5A–D), those of nirK and nirS genes were increased substantially by N inputs (Figures 5E,F). The increase in abundance of nirK genes in the PF (12%) was greater than that in the BF (5%), whereas the increase in abundance of nirS genes in the BF (15%) was slightly higher compared with that in the PF (14%). Inputs of N had no significant effect on nosZ gene abundance (Figure 5G).
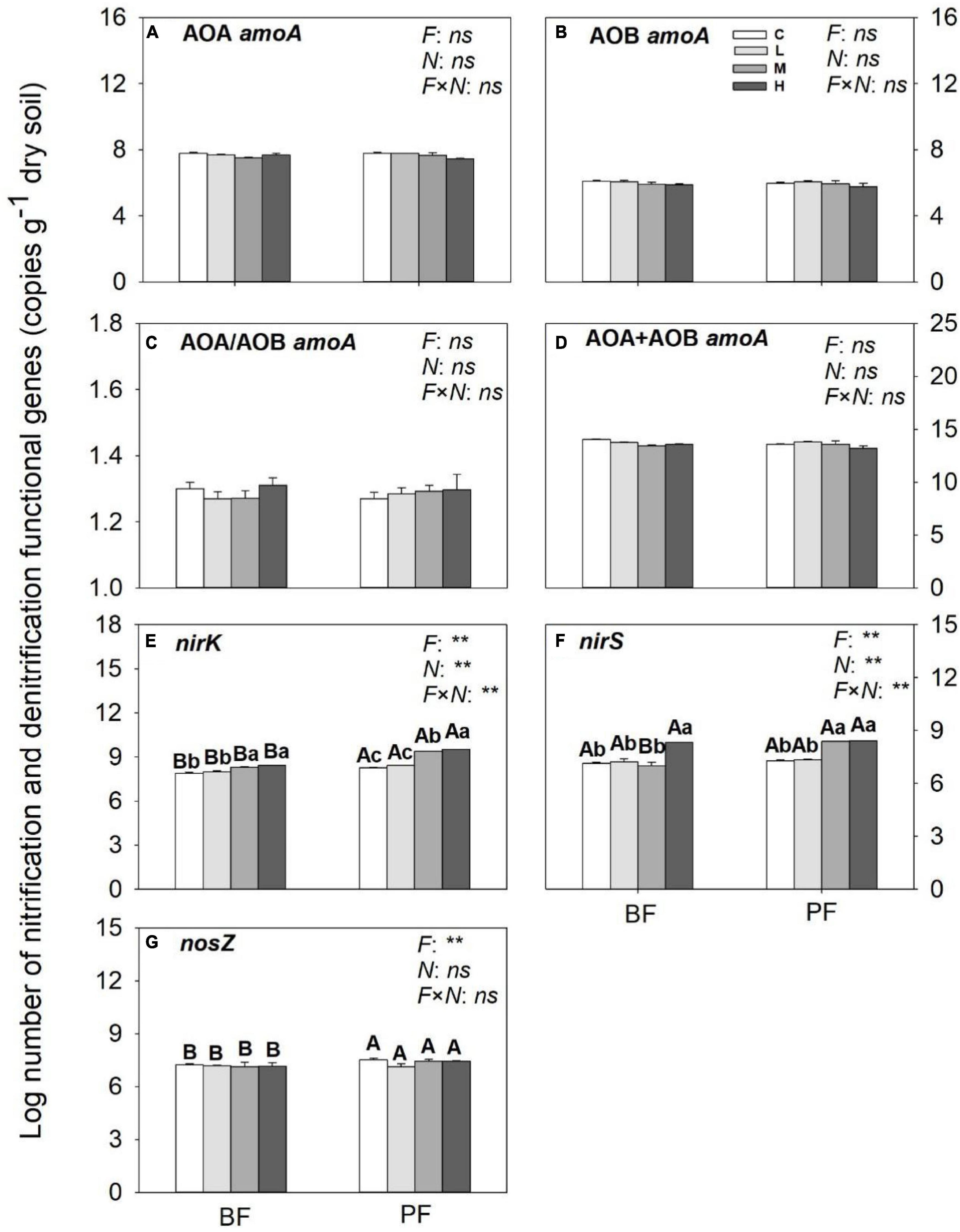
Figure 5. Abundances of nitrification (A–D) and denitrification (E,F) functional genes under different treatments. Values are means ± standard error (n = 3). Different lowercase letters indicate that the functional gene abundance differed significantly among treatments (P < 0.05). C, L, M, and H represent N application rates of 0, 12.5, 25, and 50 μg 15N g–1 soil, respectively. BF and PF represent the broad-leaved forest and Pinus forest, respectively. F, N, and F × N represent the effects of forest type, N application, and their interactions on these indicators. ** represent the effects of forest type or N application or their interactions on these indicators were significant at P < 0.05 and P < 0.01, respectively. ns indicate their effects were not significant at P < 0.05.
Controlling Factors of Nitrification- and Denitrification-Derived N2O Emissions
According to the SEM, soil properties (soil NH4+-N, NO3–-N, DON, and pH) and nitrification and denitrification functional genes (AOA-amoA, AOB-amoA, nirK, nirS, and nosZ) explained 99 and 95% of the variance observed in nitrification-derived N2O emissions in the BF and PF, respectively (Figures 6A,B). These variables also could explain 55 and 88% of the variance of denitrification-derived N2O emissions in the BF and PF, respectively (Figures 6C,D). The dominant factor in regulating soil nitrification-derived N2O emission in the BF and PF was soil NH4+-N (standardized total coefficient = –0.93, P < 0.001) and soil pH (standardized total coefficient = −0.74, P < 0.001), respectively, (Table 3). The dominant factors in regulating soil denitrification-derived N2O in BF and PF were significantly differently (Figures 6C,D and Table 3). In the BF, it was soil pH. However, in the PF, the dominant regulatory factor was nirS (Table 3).
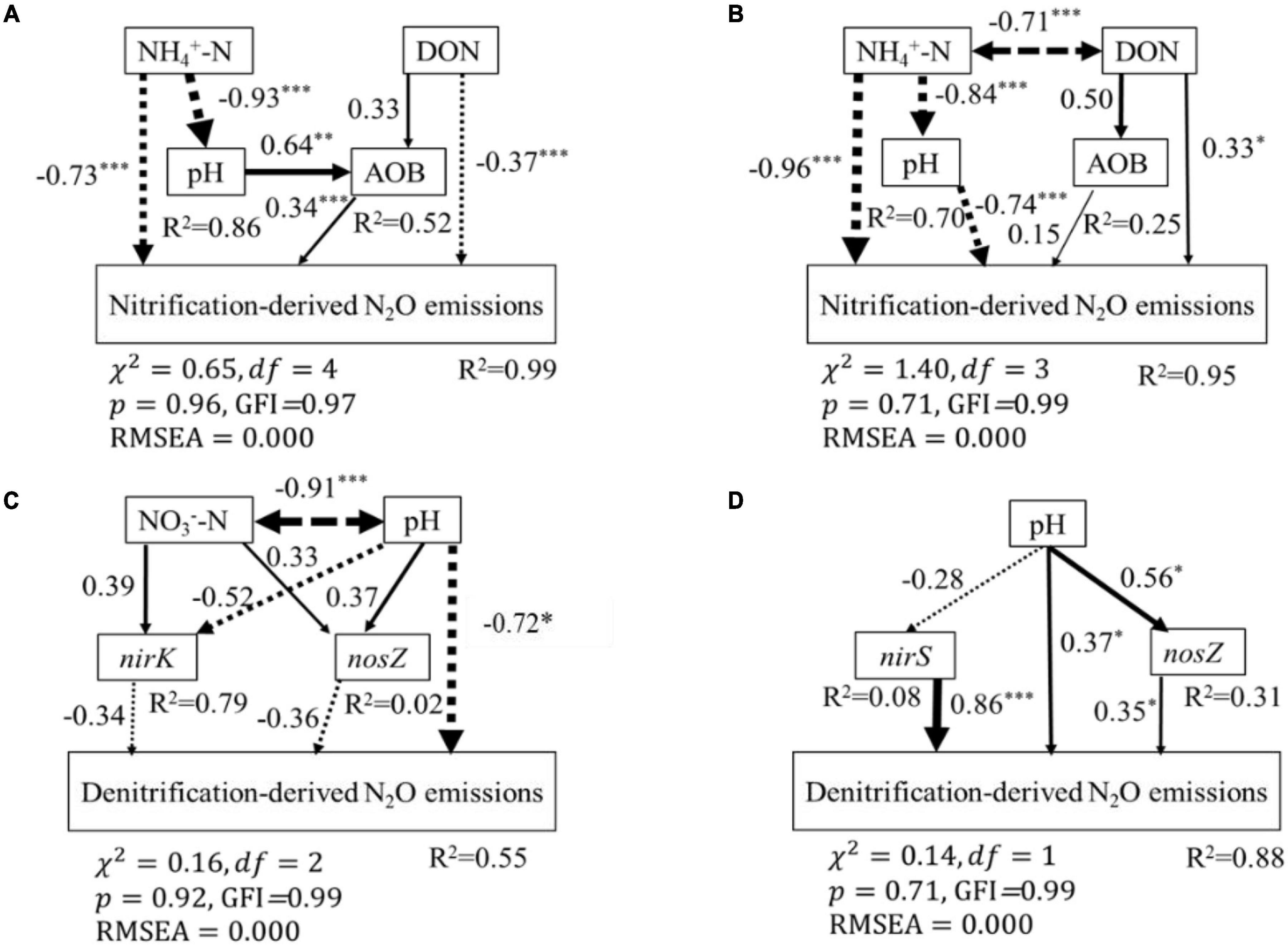
Figure 6. Structural equation modeling (SEM) analysis of the effects of soil biotic and abiotic properties on soil nitrification-derived N2O emissions in BF (A), PF (B), and on soil denitrification-derived N2O emissions in BF (C) and PF (D), respectively. Continued and dashed arrows indicated positive and negative relationships in a fitted SEM, respectively. Arrow line thickness indicates the strength of the causal relationship. Numbers adjacent to arrows represented standardized path coefficients of the relationships (*P < 0.05, **P < 0.01, ***P < 0.01). Double-headed arrows represented covariance between variables. The total variation explained by the model is indicated by R2.
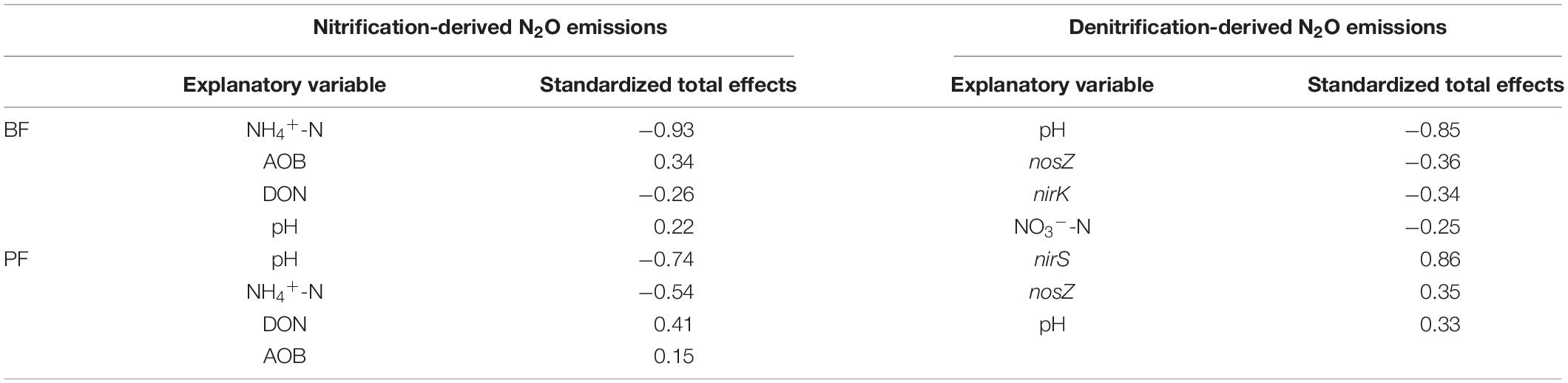
Table 3. Standardized total effects of each explanatory variable on nitrification- and denitrification-derived N2O emissions.
Discussion
Effects of N Addition on Nitrification- and Denitrification-Derived N2O Emissions
In the present study, soil N2O fluxes responded non-linearly to N addition. Low- and middle-level N application rates increased soil N2O emissions which might be due to N application and increased N availability (Figure 3) for nitrifying and denitrifying microorganisms. However, high N input had no significant effect on soil N2O emission from BF or even decreased soil N2O emission from PF (Figure 2B). Similarly, previous studies carried out in a subtropical montane forest also reported that short-term low and medium N addition favored N2O production but this effect became weaker or even decreased N2O emissions at high N addition (Han et al., 2018). A possible reason may be that the positive effect of high N application on denitrification-derived N2O in both forests was partially offset by its negative effect on nitrification-derived N2O (Figure 2B). Another explanation may be that the consumption of N2O may be greater than the production of N2O at high N treatment (Senbayram et al., 2012).
The application of N not only affects total soil N2O emissions but also the contribution of nitrification and denitrification processes to soil N2O production. In the present study, the average nitrification-derived N2O emissions under different N treatments ranged from 0 to 0.37 μg N kg–1 day–1 (Figures 1C,D), which is similar to a previous study in subtropical forests [0.06–0.40 μg N kg–1 day–1, Zhang et al. (2011)]. Over 15 days of incubation, nitrification-derived N2O contributed 0 to 36% of the total N2O emissions (Figure 2). This range is within the range (<50%) that has been documented in previous studies carried out in the subtropical forest with similar N application rates (Zhang et al., 2011; Han et al., 2018). In contrast to our first hypothesis, our result showed a decrease in the contribution of the nitrification process to the total N2O emissions following N application (Figure 3), and the magnitude of decrease was about 80 to 100% across the two forests. Deppe et al. (2017) also found that N addition can significantly decrease the contribution of nitrification to N2O production in a Haplic Luvisol soil, but the magnitude of decrease (7 to 21%) was not as pronounced as the one observed in the present study. One possible explanation for this phenomenon may be that N application decreased soil pH (Figure 4A), and soil nitrification rates have been demonstrated to be relatively low in acidic soils and decrease with decreasing soil pH (Cheng et al., 2013; Wang et al., 2020).
In contrast to the response of nitrification-derived N2O emissions to N input as explained above, the highest denitrification-derived N2O emissions occurred at the middle N application rate in both BF and PF, and the denitrification process is the dominant pathway for the production of soil N2O emission, accounting for 64 to 100% of the total N2O fluxes (Figure 3C). Previous studies carried out across subtropical forests reported that 53 to 100% of the total N2O emissions were derived from denitrification (Zhang et al., 2011; Zhu et al., 2013a; Han et al., 2018). Moreover, the relative contributions of denitrification to N2O emissions in forest soils were found to increase significantly with increasing N application (Zhu et al., 2013a; Morse et al., 2015; Niu et al., 2016), with the magnitude of increase ranging from 51 to 130% in a subtropical forest (Zhu et al., 2013a). The significantly higher relative contribution of denitrification to total N2O production in the PF compared with the BF in the present study can be partly attributed to the greater decrease in nitrification-derived N2O emissions in the PF (100%) than in the BF (80%).
Moreover, both nitrification- and denitrification-derived N2O emissions were significantly higher in the BF relative to the PF, which indicated that the BF was a more important N2O emission source than the PF. This is consistent with our third hypothesis. A study by Zhang et al. (2008) in southern China also reported that the emissions of N2O from a mature monsoon evergreen BF were significantly higher compared to those from a PF. The authors attributed their observation to the higher soil N availability in the monsoon evergreen BF. However, their explanation cannot be extended to our findings because the BF had significantly lower soil available N content than the PF (Figure 3). Considerable differences in the amount of N2O emissions among the investigated forest soils might be attributed to the differences in tree species, or more specifically, their influences on the soil’s abiotic and/or biotic properties (Butterbach-Bahl et al., 1997; Qin et al., 2019). It has been reported that trees in BF are associated with arbuscular mycorrhizal fungi, which can promote soil microbial communities with higher N cycling potential and activity relative to microbial communities in soils dominated by the trees associated with ectomycorrhizal fungi in the PF (Mushinski et al., 2021).
Overall, our results supported part of our first hypothesis as the denitrification process was the dominant pathway of soil N2O production. However, the denitrification-derived N2O emissions were observed as non-linear responses to N application in both forests. In addition, the nitrification-derived N2O emissions decreased with increasing N application in both BF and PF, which is in contrast with our first hypothesis. An explanation for this phenomenon is presented in section “Controlling Factors Related to Nitrification-Derived N2O Emissions in Forest Soils.”
Controlling Factors Related to Nitrification-Derived N2O Emissions in Forest Soils
Soil NH4+-N content and soil pH contributed the most to the variations of nitrification-derived N2O emissions in the BF and PF, respectively (Figure 6 and Table 3). Their effect was more pronounced than the effects of AOB-amoA on nitrification-derived N2O emission. Therefore, in contrast to our second hypothesis, our results suggest that N-induced changes in soil environmental factors were more important than the abundance of amoA genes in regulating nitrification-derived N2O emissions. Similarly, Tang et al. (2019) found that soil environmental factors (especially soil NH4+ content) accounted for 50 to 77% of the variation in potential nitrification activities, which was better explained than nitrification functional genes in fertilized soils. In the BF, high soil NH4+-N concentrations not only had a direct negative effect on nitrification-derived N2O emission but also had an indirect negative effect via influencing soil pH and the abundance of AOB (Figure 6A). The reasons for high soil NH4+-N contents reducing nitrification-derived N2O emission may be as follows: ammonia oxidizers are unable to tolerate high NH4+-N contents (>100 mg N kg–1 soil) in acidic soils (Gubry-Rangin et al., 2010; Carey et al., 2016; Farquharson, 2016; Breuillin-Sessoms et al., 2017; Li et al., 2018; Liu et al., 2018). In the current study, the average soil NH4+-N content in N-amended soils was much higher than not only the aforementioned 100 mg N kg–1 soil but also other soil NH4+-N content averages that have been reported in previous studies in various subtropical fertilized forest soils (ranging from 1 to 50 mg N kg–1 soil) (Han et al., 2018; Tang et al., 2018, 2019; Wang et al., 2018). This indicates the lack of efficient oxidation of NH4+ to NO3– during nitrification that has led to lower nitrification-derived N2O emissions in N-amended soils (Farquharson, 2016; Wang et al., 2020).
Soil NH4+-N content also had a direct significant effect on nitrification-derived N2O emission in the BF, but its total effect was offset by its indirect effect on soil pH (Figure 6B). This resulted in soil pH playing a more important role in regulating nitrification-derived N2O emissions in the PF (Table 3). Previous studies have reported that soil with a lower pH support less microbial diversity and lower N-cycle potential (Cheng et al., 2019; Mushinski et al., 2021). A meta-analysis reports that N addition dramatically decreases the ratios of fungi to bacteria and microbial C to N on an average by 10 and 8%, respectively (Zhou et al., 2017). In addition, the nitrification rate is generally decreased with decreasing soil pH, and its rate can be inhibited in soil with a pH lower than 5 (Zhao et al., 2018; Cheng et al., 2019). This could explain why the nitrification-derived N2O emission at high N addition in the PF is close to zero (Figure 2B). Overall, in terms of nitrification-derived N2O emissions, changes in soil environmental factors (e.g., soil NH4+-N and pH) induced by N application play more important roles than changes in the abundance of AOA and AOB in controlling its emission.
Controlling Factors Related to Denitrification-Derived N2O Emissions in Forest Soils
Soil pH is the most important controller of denitrification-derived N2O emission in the BF (Figure 6C and Table 3), and high N application significantly decreased soil pH (Figure 4). The reasons for soil pH regulating denitrification-derived N2O emissions in BF may be as follows. Soil pH can exert a direct and an indirect effect on biological properties (e.g., reductase activities, the abundance, and expression of functional genes, etc.) (Liu et al., 2010; Hu et al., 2015; Han et al., 2019). The reductases for nitrate, nitrite, and nitric oxide are more active at pH <7 (Gillam et al., 2008), while the N2O reductase activities are inhibited in soil with low pH (Levy-Booth et al., 2014). A review covering 50 years of research on the effects of soil pH on denitrification also reports that there is a consistent negative relationship between denitrification-derived N2O production and soil pH (ŠImek and Cooper, 2002). A close relationship between soil pH and denitrification-derived N2O in the BF suggested that soil pH is a strong predictor of denitrifier activity in the BF. In the PF, the abundance of nirS is the dominant factor controlling denitrification-derived N2O emissions. Previous studies have also reported that the abundance of denitrification genes correlated with nirS gene abundance (Morales et al., 2010).
The dominant factors in regulating soil denitrification-derived N2O emission were different in these two forests. This may be because differences in the tree species lead to variations in soil environment and the community composition of functional microbes, and changes in these variations induced the differences in the nitrification- and denitrification-derived N2O emissions in these acidic forest soils (Barberán et al., 2015; Soper et al., 2018). Moreover, regardless of nitrification- or denitrification-derived N2O emissions, soil environmental factors are more important than nitrifier and denitrifier abundance in regulating soil N2O emissions in both forests (except denitrification-derived N2O emission in PF). Previous studies also demonstrate that tree species-induced changes in community composition and environmental factors play more important roles in regulating soil N2O emissions than the abundance of nitrifier/denitrifier functional genes (Qin et al., 2019). In the present study, we still do not know how the species diversity or the nature of individual species affect nitrification- and denitrification-derived N2O production in the field, even though several studies have investigated N2O emission from plantation forest soils (Arai et al., 2008; Wang et al., 2014; Kou-Giesbrecht and Menge, 2021). Further study needs to focus on the role of the identities of specific species in the production processes of N2O under different N inputs.
Conclusion
Our results demonstrated clearly that nitrification-derived N2O emissions decreased with N application rates, while denitrification-derived N2O emissions were non-linear responses to N addition. The total soil N2O emissions, nitrification-, and denitrification-derived N2O emissions were significantly higher in the broadleaf forest than in the conifers forest. Changes in soil environmental factors (i.e., pH and soil NH4+-N content) induced by N addition are more important than the abundance of nitrification and denitrification functional genes in regulating the production process of soil N2O. The incorporation of soil nitrogen substrates, soil pH, and forest type into the nitrogen cycling model will more precisely predict the global N2O from soil under elevated N deposition.
Data Availability Statement
The raw data supporting the conclusions of this article will be made available by the authors, without undue reservation.
Author Contributions
QQ: conceptualization, methodology, software, investigation, supervision, validation, formal analysis, writing–original draft, writing–reviewing and editing, visualization, data curation, and resources. AM: investigation and writing–reviewing and editing. SJ: writing–original draft, writing–reviewing and editing, visualization, and supervision. YH: conceptualization, methodology, software, validation, formal analysis, writing–original draft, writing–reviewing and editing, visualization, and supervision. All authors contributed to the article and approved the submitted version.
Funding
This work was funded by the National Natural Science Foundation of China (Grant Number 41703066), the Key Laboratory of Vegetation Restoration and Management of Degraded Ecosystems, Chinese Academy of Sciences (Grant Number Y821161001-DE2018025), the Forestry Peak Discipline Construction Project, and the star-up founding of Minjiang University (32304307).
Conflict of Interest
The authors declare that the research was conducted in the absence of any commercial or financial relationships that could be construed as a potential conflict of interest.
Publisher’s Note
All claims expressed in this article are solely those of the authors and do not necessarily represent those of their affiliated organizations, or those of the publisher, the editors and the reviewers. Any product that may be evaluated in this article, or claim that may be made by its manufacturer, is not guaranteed or endorsed by the publisher.
Supplementary Material
The Supplementary Material for this article can be found online at: https://www.frontiersin.org/articles/10.3389/fpls.2022.950367/full#supplementary-material
References
Arai, S., Ishizuka, S., Ohta, S., Ansori, S., Tokuchi, N., Tanaka, N., et al. (2008). Potential N2O emissions from leguminous tree plantation soils in the humid tropics. Glob. Biogeochem. Cycles 22:GB2028. doi: 10.1029/2007GB002965
Barberán, A., McGuire, K. L., Wolf, J. A., Jones, F. A., Wright, S. J., Turner, B. L., et al. (2015). Relating belowground microbial composition to the taxonomic, phylogenetic, and functional trait distributions of trees in a tropical forest. Ecol. Lett. 18, 1397–1405. doi: 10.1111/ele.12536
Breuillin-Sessoms, F., Venterea, R. T., Sadowsky, M. J., Coulter, J. A., Clough, T. J., and Wang, P. (2017). Nitrification gene ratio and free ammonia explain nitrite and nitrous oxide production in urea-amended soils. Soil Biol. Biochem. 111, 143–153. doi: 10.1016/j.soilbio.2017.04.007
Butterbach-Bahl, K., Gasche, R., Breuer, L., and Papen, H. (1997). Fluxes of NO and N2O from temperate forest soils: impact of forest type, N deposition and of liming on the NO and N2O emissions. Nutr. Cycl. Agroecosysyst. 48, 79–90. doi: 10.1023/A:1009785521107
Cao, L., Liang, Y., Wang, Y., and Lu, H. (2015). Runoff and soil loss from Pinus massoniana forest in southern China after simulated rainfall. Catena 129, 1–8. doi: 10.1016/j.catena.2015.02.009
Carey, C. J., Dove, N. C., Beman, J. M., Hart, S. C., and Aronson, E. L. (2016). Meta-analysis reveals ammonia-oxidizing bacteria respond more strongly to nitrogen addition than ammonia-oxidizing archaea. Soil Biol. Biochem. 99, 158–166. doi: 10.1016/j.soilbio.2016.05.014
Chen, W. B., and Peng, S. L. (2020). Land-use legacy effects shape microbial contribution to N2O production in three tropical forests. Geoderma 358, 113979. doi: 10.1016/j.geoderma.2019.113979
Cheng, Y., Wang, J., Chang, S. X., Cai, Z., Müller, C., and Zhang, J. (2019). Nitrogen deposition affects both net and gross soil nitrogen transformations in forest ecosystems: a review. Environ. Pollut. 244, 608–616. doi: 10.1016/j.envpol.2018.10.054
Cheng, Y., Wang, J., Mary, B., Zhang, J. B., Cai, Z. C., and Chang, S. X. (2013). Soil pH has contrasting effects on gross and net nitrogen mineralizations in adjacent forest and grassland soils in central Alberta, Canada. Soil Biol. Biochem. 57, 848–857. doi: 10.1016/j.soilbio.2012.08.021
Deppe, M., Well, R., Giesemann, A., Spott, O., and Flessa, H. (2017). Soil N2O fluxes and related processes in laboratory incubations simulating ammonium fertilizer depots. Soil Biol. Biochem. 104, 68–80. doi: 10.1016/j.soilbio.2016.10.005
Fang, Y., Koba, K., Makabe, A., Takahashi, C., Zhu, W., Hayashi, T., et al. (2015). Microbial denitrification dominates nitrate losses from forest ecosystems. Proc. Natl. Acad. Sci. U.S.A. 112, 1470. doi: 10.1073/pnas.1416776112
Farquharson, R. (2016). Nitrification rates and associated nitrous oxide emissions from agricultural soils – a synopsis. Soil Res. 54, 469–480. doi: 10.1071/SR15304
Gillam, K., Zebarth, B., and Burton, D. (2008). Nitrous oxide emissions from denitrification and the partitioning of gaseous losses as affected by nitrate and carbon addition and soil aeration. Can. J. Soil Sci. 88, 133–143. doi: 10.4141/CJSS06005
Gubry-Rangin, C., Nicol, G. W., and Prosser, J. I. (2010). Archaea rather than bacteria control nitrification in two agricultural acidic soils. FEMS Microbiol. Ecol. 74, 566–574. doi: 10.1111/j.1574-6941.2010.00971.x
Gurmesa, G. A., Wang, A., Li, S., Peng, S., de Vries, W., Gundersen, P., et al. (2022). Retention of deposited ammonium and nitrate and its impact on the global forest carbon sink. Nat. Commun. 13, 1–9. doi: 10.1038/s41467-022-28345-1
Han, X., Shen, W., Zhang, J., and Müller, C. (2018). Microbial adaptation to long-term N supply prevents large responses in N dynamics and N losses of a subtropical forest. Sci. Total Environ. 626, 1175–1187. doi: 10.1016/j.scitotenv.2018.01.132
Han, X., Xu, C., Nie, Y., He, J., Wang, W., Deng, Q., et al. (2019). Seasonal variations in N2O emissions in a subtropical forest with exogenous nitrogen enrichment are predominately influenced by the abundances of soil nitrifiers and denitrifiers. J. Geophys. Res. Biogeosci. 124, 3635–3651. doi: 10.1029/2019JG005477
Hu, H. W., Chen, D., and He, J. Z. (2015). Microbial regulation of terrestrial nitrous oxide formation: understanding the biological pathways for prediction of emission rates. FEMS Microbiol. Rev. 39, 729–749. doi: 10.1093/femsre/fuv021
IPCC (2013). “Climate change 2013: the physical science basis,” in Contribution of Working Group I to the Fifth Assessment Report of the Intergovernmental Panel on Climate Change, eds T. F. Stocker, D. Qin, G. K. Plattner, M. Tignor, S. K. Allen, J. Boschung, et al. (Cambridge: Cambridge University Press), 1535.
Jones, D. L., and Willett, V. B. (2006). Experimental evaluation of methods to quantify dissolved organic nitrogen (DON) and dissolved organic carbon (DOC) in soil. Soil Biol. Biochem. 38, 991–999. doi: 10.1016/j.soilbio.2005.08.012
Kou-Giesbrecht, S., and Menge, D. N. (2021). Nitrogen-fixing trees increase soil nitrous oxide emissions: a meta-analysis. Ecology 102:e03415. doi: 10.1002/ecy.3415
Levy-Booth, D. J., Prescott, C. E., and Grayston, S. J. (2014). Microbial functional genes involved in nitrogen fixation, nitrification and denitrification in forest ecosystems. Soil Biol. Biochem. 75, 11–25. doi: 10.1016/j.soilbio.2014.03.021
Li, D., Zhang, X., Green, S. M., Dungait, J. A. J., Wen, X., Tang, Y., et al. (2018). Nitrogen functional gene activity in soil profiles under progressive vegetative recovery after abandonment of agriculture at the Puding Karst Critical Zone Observatory, SW China. Soil Biol. Biochem. 125, 93–102. doi: 10.1016/j.soilbio.2018.07.004
Liu, B., Mørkved, P. T., Frostegård, Å, and Bakken, L. R. (2010). Denitrification gene pools, transcription and kinetics of NO, N2O and N2 production as affected by soil pH. FEMS Microbiol. Ecol. 72, 407–417. doi: 10.1111/j.1574-6941.2010.00856.x
Liu, H., Ding, Y., Zhang, Q., Liu, X., Xu, J., Li, Y., et al. (2018). Heterotrophic nitrification and denitrification are the main sources of nitrous oxide in two paddy soils. Plant Soil 445, 39–53. doi: 10.1007/s11104-018-3860-x
Liu, X., Zhang, Y., Han, W., Tang, A., Shen, J., Cui, Z., et al. (2013). Enhanced nitrogen deposition over China. Nature 494:459. doi: 10.1038/nature11917
Lu, M., Yang, Y., Luo, Y., Fang, C., Zhou, X., Chen, J., et al. (2011). Responses of ecosystem nitrogen cycle to nitrogen addition: a meta-analysis. New Phytol. 189, 1040–1050. doi: 10.1111/j.1469-8137.2010.03563.x
Lyu, M., Nie, Y., Giardina, C. P., Vadeboncoeur, M. A., Ren, Y., Fu, Z., et al. (2019). Litter quality and site characteristics interact to affect the response of priming effect to temperature in subtropical forests. Funct. Ecol. 33, 2226–2238. doi: 10.1111/1365-2435.13428
Morales, S. E., Cosart, T., and Holben, W. E. (2010). Bacterial gene abundances as indicators of greenhouse gas emission in soils. ISME J. 4, 799–808. doi: 10.1038/ismej.2010.8
Morse, J. L., Durán, J., Beall, F., Enanga, E. M., Creed, I. F., Fernandez, I., et al. (2015). Soil denitrification fluxes from three northeastern North American forests across a range of nitrogen deposition. Oecologia 177, 17–27. doi: 10.1007/s00442-014-3117-1
Mushinski, R. M., Payne, Z. C., Raff, J. D., Craig, M. E., Pusede, S. E., Rusch, D. B., et al. (2021). Nitrogen cycling microbiomes are structured by plant mycorrhizal associations with consequences for nitrogen oxide fluxes in forests. Glob. Change Biol. 27, 1068–1082. doi: 10.1111/gcb.15439
Niu, S., Classen, A. T., Dukes, J. S., Kardol, P., Liu, L., Luo, Y., et al. (2016). Global patterns and substrate-based mechanisms of the terrestrial nitrogen cycle. Ecol. Lett. 19, 697–709. doi: 10.1111/ele.12591
Pett-Ridge, J., Petersen, D. G., Nuccio, E., and Firestone, M. K. (2013). Influence of oxic/anoxic fluctuations on ammonia oxidizers and nitrification potential in a wet tropical soil. FEMS Microbiol. Ecol. 85, 179–194. doi: 10.1111/1574-6941.12111
Qin, H. L., Xing, X. Y., Tang, Y. F., Hou, H. J., Yang, J., Shen, R., et al. (2019). Linking soil N2O emissions with soil microbial community abundance and structure related to nitrogen cycle in two acid forest soils. Plant Soil 435, 95–109. doi: 10.1007/s11104-018-3863-7
Senbayram, M., Chen, R., Budai, A., Bakken, L., and Dittert, K. (2012). N2O emission and the N2O/(N2O+ N2) product ratio of denitrification as controlled by available carbon substrates and nitrate concentrations. Agric. Ecosyst. Environ. 147, 4–12. doi: 10.1016/j.agee.2011.06.022
Shcherbak, I., Millar, N., and Robertson, G. P. (2014). Global metaanalysis of the nonlinear response of soil nitrous oxide (N2O) emissions to fertilizer nitrogen. Proc. Natl. Acad. Sci. U.S.A. 111, 9199. doi: 10.1073/pnas.1322434111
ŠImek, M., and Cooper, J. E. (2002). The influence of soil pH on denitrification: progress towards the understanding of this interaction over the last 50 years. Eur. J. Soil Sci. 53, 345–354. doi: 10.1046/j.1365-2389.2002.00461.x
Solomon, S. (2007). Climate Change 2007 – The Physical Science Basis: Working Group I Contribution to the Fourth Assessment Report of the IPCC. Cambridge: Cambridge University Press.
Soper, F. M., Sullivan, B. W., Nasto, M. K., Osborne, B. B., Bru, D., Balzotti, C. S., et al. (2018). Remotely sensed canopy nitrogen correlates with nitrous oxide emissions in a lowland tropical rainforest. Ecology 99, 2080–2089. doi: 10.1002/ecy.2434
Stevens, R., Laughlin, R., Burns, L., Arah, J., and Hood, R. (1997). Measuring the contributions of nitrification and denitrification to the flux of nitrous oxide from soil. Soil Biol. Biochem. 29, 139–151. doi: 10.1016/S0038-0717(96)00303-3
Tang, W., Chen, D., Phillips, O. L., Liu, X., Zhou, Z., Li, Y., et al. (2018). Effects of long-term increased N deposition on tropical montane forest soil N2 and N2O emissions. Soil Biol. Biochem. 126, 194–203. doi: 10.1016/j.soilbio.2018.08.027
Tang, Y., Yu, G., Zhang, X., Wang, Q., Tian, D., Tian, J., et al. (2019). Environmental variables better explain changes in potential nitrification and denitrification activities than microbial properties in fertilized forest soils. Sci. Total Environ. 647, 653–662. doi: 10.1016/j.scitotenv.2018.07.437
Wang, G., Zhou, Y., Xu, X., Ruan, H., and Wang, J. (2013). Temperature sensitivity of soil organic carbon mineralization along an elevation gradient in the Wuyi Mountains, China. PLoS One 8:e53914. doi: 10.1371/journal.pone.0053914
Wang, H., Liu, S., Zhang, X., Mao, Q., Li, X., You, Y., et al. (2018). Nitrogen addition reduces soil bacterial richness, while phosphorus addition alters community composition in an old-growth N-rich tropical forest in southern China. Soil Biol. Biochem. 127, 22–30. doi: 10.1016/j.soilbio.2018.08.022
Wang, J., Tu, X. S., Zhang, H. M., Cui, J. Y., Ni, K., Chen, J. L., et al. (2020). Effects of ammonium-based nitrogen addition on soil nitrification and nitrogen gas emissions depend on fertilizer-induced changes in pH in a tea plantation soil. Sci. Total Environ. 747:8. doi: 10.1016/j.scitotenv.2020.141340
Wang, Y., Cheng, S., Fang, H., Yu, G., Xu, M., Dang, X., et al. (2014). Simulated nitrogen deposition reduces CH4 uptake and increases N2O emission from a subtropical plantation forest soil in southern China. PloS One 9:e93571. doi: 10.1371/journal.pone.0093571
Xu, W., Li, W., Chen, S., Yang, D., Zhang, Y., Xue, J., et al. (2016). Quantification of nitrogen dry and wet deposition in Fujian tobacco planting area. Acta Ecol. Sin. 36, 424–432. doi: 10.1016/j.chnaes.2016.09.007
Xu, X., Zhou, Y., Ruan, H., Luo, Y., and Wang, J. (2010). Temperature sensitivity increases with soil organic carbon recalcitrane along an elevational gradient in the Wuyi Mountains, China. Soil Biol. Biochem. 42, 1811–1815. doi: 10.1016/j.soilbio.2010.06.021
Zhang, J., Cai, Z., and Zhu, T. (2011). N2O production pathways in the subtropical acid forest soils in China. Environ. Res. 111, 643–649. doi: 10.1016/j.envres.2011.04.005
Zhang, K., Zhu, Q., Liu, J., Wang, M., Zhou, X., Li, M., et al. (2019). Spatial and temporal variations of N2O emissions from global forest and grassland ecosystems. Agric. For. Meteorol. 266-267, 129–139. doi: 10.1016/j.agrformet.2018.12.011
Zhang, W., Mo, J., Yu, G., Fang, Y., Li, D., Lu, X., et al. (2008). Emissions of nitrous oxide from three tropical forests in Southern China in response to simulated nitrogen deposition. Plant Soil 306, 221–236. doi: 10.1007/s11104-008-9575-7
Zhao, W., Zhang, J., Müller, C., and Cai, Z. (2018). Effects of pH and mineralisation on nitrification in a subtropical acid forest soil. Soil Res. 56, 275–283. doi: 10.1071/SR17087
Zhou, G., Peng, C., Li, Y., Liu, S., Zhang, Q., Tang, X., et al. (2013). A climate change-induced threat to the ecological resilience of a subtropical monsoon evergreen broad-leaved forest in Southern China. Glob. Change Biol. 19, 1197–1210. doi: 10.1111/gcb.12128
Zhou, Z., Wang, C., Zheng, M., Jiang, L., and Luo, Y. (2017). Patterns and mechanisms of responses by soil microbial communities to nitrogen addition. Soil Biol. Biochem. 115, 433–441. doi: 10.1016/j.soilbio.2017.09.015
Zhu, J., He, N., Wang, Q., Yuan, G., Wen, D., Yu, G., et al. (2015). The composition, spatial patterns, and influencing factors of atmospheric wet nitrogen deposition in Chinese terrestrial ecosystems. Sci. Total Environ. 511, 777–785. doi: 10.1016/j.scitotenv.2014.12.038
Zhu, J., Mulder, J., Bakken, L., and Dörsch, P. (2013a). The importance of denitrification for N2O emissions from an N-saturated forest in SW China: results from in situ 15N labeling experiments. Biogeochemistry 116, 103–117. doi: 10.1007/s10533-013-9883-8
Keywords: nitrification, denitrification, microbial functional genes, N2O emissions, N application
Citation: Qiu Q, Mgelwa AS, Jin S and Hu Y (2022) Nitrogen-Induced Changes in Soil Environmental Factors Are More Important Than Nitrification and Denitrification Gene Abundance in Regulating N2O Emissions in Subtropical Forest Soils. Front. Plant Sci. 13:950367. doi: 10.3389/fpls.2022.950367
Received: 22 May 2022; Accepted: 14 June 2022;
Published: 12 July 2022.
Edited by:
Hui Wang, Shandong University, ChinaReviewed by:
Xinhou Zhang, Nanjing Normal University, ChinaZhi Quan, Institute of Applied Ecology (CAS), China
Copyright © 2022 Qiu, Mgelwa, Jin and Hu. This is an open-access article distributed under the terms of the Creative Commons Attribution License (CC BY). The use, distribution or reproduction in other forums is permitted, provided the original author(s) and the copyright owner(s) are credited and that the original publication in this journal is cited, in accordance with accepted academic practice. No use, distribution or reproduction is permitted which does not comply with these terms.
*Correspondence: Shaofei Jin, jinsf@tea.ac.cn; Yalin Hu, huyl@iae.ac.cn