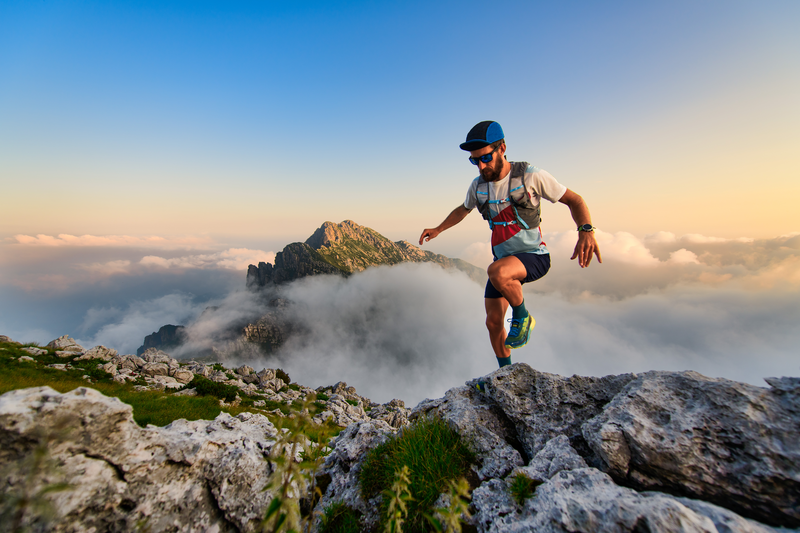
94% of researchers rate our articles as excellent or good
Learn more about the work of our research integrity team to safeguard the quality of each article we publish.
Find out more
ORIGINAL RESEARCH article
Front. Plant Sci. , 11 August 2022
Sec. Plant Metabolism and Chemodiversity
Volume 13 - 2022 | https://doi.org/10.3389/fpls.2022.944358
This article is part of the Research Topic Targeted and Untargeted Metabolomics for the Evaluation of Plant Metabolites in Response to the Environment View all 6 articles
Plants have developed diverse defense strategies to reduce the detrimental effects of a wide range of environmental stresses. The objectives of this study were to explore the function of spermine (Spm) on mediating growth and physiological changes in water homeostasis, photosynthetic performance, and oxidative damage and to further examine the regulatory mechanism of Spm on global metabolites reprogramming and associated metabolic pathways in horticultural creeping bentgrass (Agrostis stolonifera) under water and heat stresses. The 21-days-old plants were pretreated with or without 100 μM Spm for 3 days and then subjected to water stress (17% polyethylene glycol 6000), high-temperature stress (40/35°C, day/night), or normal condition (control without water stress and heat stress) for 18 days. Results demonstrated that exogenous application of Spm could significantly increase endogenous polyamine (PAs), putrescine (Put), spermidine (Spd), and Spm contents, followed by effective alleviation of growth retardant, water imbalance, photoinhibition, and oxidative damage induced by water and heat stress. Metabolites' profiling showed that a total of 61 metabolites were differentially or commonly regulated by Spm in leaves. Spm upregulated the accumulation of mannose, maltose, galactose, and urea in relation to enhanced osmotic adjustment (OA), antioxidant capacity, and nitrogen metabolism for growth maintenance under water and heat stress. Under water stress, Spm mainly induced the accumulation of sugars (glucose-1-phosphate, sucrose-6-phosphate, fructose, kestose, maltotriose, and xylose), amino acids (glutamic acid, methionine, serine, and threonine), and organic acids (pyruvic acid, aconitic acid, and ketoglutaric acid) involved in the respiratory pathway and myo-inositol associated with energy production, the ROS-scavenging system, and signal transduction. In response to heat stress, the accumulation of alanine, glycine, gallic acid, malic acid, or nicotinic acid was specifically enhanced by Spm contributing to improvements in antioxidant potency and metabolic homeostasis. This study provides novel evidence of Spm-induced,tolerance to water and heat stresses associated with global metabolites reprogramming in favor of growth maintenance and physiological responses in horticultural plants.
Abiotic stresses such as drought and high-temperature stress cause huge economic losses in horticulture. Predictably, the frequency and severity of drought and heat stress will further increase in the future due to global climate change (Cayan et al., 2010; Sun et al., 2019). Plants adapt to stressful environments by regulating physiological, biochemical, and phenotypic responses at molecular, enzymatic, and metabolic levels (Gong et al., 2020; Jing et al., 2021; Li et al., 2021). Although the analysis and identification of stress-responsive genes and proteins are still the mainstream research in plant species, the role of metabolites in stress tolerance is coming into notice in recent years (Ghatak et al., 2018). Increasing studies proved that global metabolites accumulation and transformation not only affected crop product and quality but also affected stress tolerance. Some of them are beneficial for enhanced stress tolerance, such as most of the amino acids and soluble sugars, but others like quinones and aldehydes overaccumulation are toxic to plants under stressful environments (Srivastava et al., 2017; Martínez-Noël and Tognetti, 2018; Batista-Silva et al., 2019). It has been demonstrated that the global reprogramming of metabolites was involved in adaptive responses to drought stress in different Lotus genus species by using comparative metabolomics (Sanchez et al., 2012). Non-protein amino acids, such as γ-aminobutyric acid (GABA), could mitigate heat and drought damage by altering primary and secondary metabolites in creeping bentgrass (Agrostis stolonifera) based on the analysis of non-targeted metabolomics (Li et al., 2016a, 2017a). Therefore, the study of the relations between changes in metabolites and stress tolerance is essential for a better understanding of the stress-adaptive mechanisms in different plant species.
Polyamines (PAs) including putrescine (Put), spermidine (Spd), and spermine (Spm) are known as low-molecular-weight plant growth regulators (PGRs) exhibiting strong biological activity in plants. Spm is a superlative form of PA which is synthesized from a lower form of PA, such as Put and Spd, and Spm can also be degraded into Spd and Put in plants (Chen et al., 2019). It has been widely reported about the function of PAs in regulating plant growth, development, senescence, and stress tolerance in various plant species (Chen et al., 2019). Put and Spd pretreatment mitigated cadmium damage to wheat (Triticum aestivum) by maintaining hormonal balance and antioxidant homeostasis (Tajti et al., 2018). Exogenously applied Spd enhanced endogenous PAs accumulation and tolerance to water stress in white clover (Trifolium repens) in relation to the improvement in antioxidant and proline metabolisms (Li et al., 2016b), whereas the inhibition of endogenous PAs biosynthesis could significantly decrease drought tolerance of white clover (Li et al., 2018). PAs could alleviate high-temperature stress by modulating starch and PAs accumulation and metabolism in rice (Oryza sativa) (Fu et al., 2019). Heat tolerance was established with the application of exogenous Spd in rice closely related to the enhancement of antioxidative and glyoxalase systems (Mostofa et al., 2014). However, the contribution of Spm to drought and heat tolerance associated with changes in global metabolites and relevant metabolic pathways is poorly documented in plants.
Mesophytes including most of the horticultural plants, such as creeping bentgrass, are susceptible to stressful environments, leading to a decrease in quality and economic losses (Cramer et al., 2011). In this study, we focused on effects of exogenous application of Spm on changes in endogenous PAs content, physiological responses, including photosynthesis, osmotic adjustment (OA), and oxidative damage, and extensive organic metabolites based on metabolomics in leaves of creeping bentgrass under normal, drought, and heat conditions. Current findings will help to better understand critical roles of PAs and metabolites in cold-season creeping bentgrass in response to drought and high-temperature environments.
Seeds (cv. Penncross at a rate of 6 g·m−2) were germinated in rectangular plastic containers (25 cm in length, 15 cm in width, and 10 cm in height) filled with quartz sand in growth chambers (21/18°C (day/night), 65% relative humidity, and 700 μmol·m−2·s−1 PAR). After germinating in distilled water for 8 days, seedlings were irrigated with Hoagland's solution (Hoagland and Arnon, 1950) for 14 days. For hydroponic cultivation, seedlings were removed from quartz sand and suspended through small holes in Styrofoam boards that could float on the nutrient solution. After being transplanted in Hoagland's solution for 7 days for the acclimation to the hydroponic cultivation, seedlings were grown in the Hoagland's solution without (as control) or with 100 μmol/L Spm (as +Spm, purity ≥ 99.0%, Art. No. 85590, Sigma-Aldrich) for 3 days and then all plants were moved to a new Hoagland's solution without Spm. Three conditions were set up: (1) normal condition: Spm-pretreated and untreated plants grew in a normal Hoagland's solution and were placed in a normal growth chamber (mentioned above) for 18 days; (2) water stress: Spm-pretreated and untreated plants grew in a Hoagland's solution containing 17% polyethylene glycol 6000 (PEG-induced water stress) and were placed in a normal growth chamber for 18 days; (3) heat stress: Spm-pretreated and untreated plants grew in the normal Hoagland's solution and were placed in a high-temperature growth chamber [40/35°C (day/night), 65% relative humidity, and 700 μmol·m−2·s−1 PAR] for 18 days. All solutions were refreshed every day. Spm-pretreated and untreated plants were completely arranged in growth chambers. Four independent biologic replicates (four containers) for each treatment including 20 plants were used for the analysis of physiological parameters or metabolome.
The determination of chlorophyll (Chl) content, relative water content (RWC), hydrogen peroxide (H2O2), malondialdehyde (MDA) content, electrolyte leakage (EL), endogenous PAs, and relative growth rate (RGR) were performed based on the method of Amnon (1949); Barrs and Weatherley (1962); Blum and Ebercon (1981); Dhindsa et al. (1981); Velikova et al. (2000); Duan et al. (2008), and Ma et al. (2012), respectively. Specific measurement procedures have been clearly described in our previous study (Li et al., 2016b). For the determination of OA, fresh leaves were hydrated in deionized water for 12 h and fully turgid leaves were frozen in liquid nitrogen for 10 min. When frozen leaves were thawed in an ice bath, the sap in the leaves was pressed and then inserted (10 ml) into an osmometer (Wescor, Inc., Logan, UT) to get osmolality (mmol kg−1). The osmotic potential (OP) was calculated based on the formula OP = –([osmolality] × [0.001] × [2.58]). The OA was calculated based on the formula OA = OP (control leaves under the normal condition) – OP (stressed leaves) (Blum, 1989). A portable photosynthesis system (CIRAS-3, PP Systems, USA) was used for detecting net photosynthesis rate (Pn) and water use efficiency (WUE). The leaf chamber (400 μl L−1 CO2 and 800 μmol photon m−2 red and blue light) was overspread with a single layer of leaves and readings were recorded after the readings were stable. The protein carbonyl content was detected using the assay kit that was purchased from the Suzhou Comin Biotechnology Co., Ltd., Suzhou, China, according to the manufacturer's instructions.
The metabolome was detected using GC-TOFMS. The metabolite extraction, separation, and quantification were conducted according to the methods of Roessner et al. (2000) and Qiu et al. (2009) with some modifications that were clearly described in our previous study (Li et al., 2020a).
The analysis of variance (SAS 9.1, SAS Institute, Cary, NC, USA) was used to determine the significance of all measured parameters. Differences between treatment means were tested using Fisher's protected least significance (LSD) test at a 0.05 probability level.
Spm priming did not affect PA content in leaves under normal condition, but Spm-pretreated plants exhibited an 84 or 82% increase in PA content than non-treated control under water stress or heat stress, respectively (Figure 1A). The application of exogenous Spm significantly increased Put content under normal condition, water stress, and heat stress (Figure 1B). A 29 or 40% increase in Spd content was observed in +Spm treatment as compared to that in control under water stress or heat stress, respectively (Figure 1C). Spm-treated plants exhibited significantly higher endogenous Spm content than untreated plants under water stress and heat stress (Figure 1D). For untreated plants, Spm significantly accumulated under heat stress, but did not significantly increase under water stress as compared to the control. For Spm-treated plants, Spm significantly accumulated under water stress and heat stress (Figure 1D).
Figure 1. Changes in endogenous (A) polyamines (PAs), (B) putrescine (Put), (C) spermidine (Spd), and (D) spermine (Spm) in response to Spm application under different conditions. Vertical bars indicate ±SE of mean (n = 4). Different letters above striated or gray columns indicate significant differences for a particular treatment (control or +Spm) for comparison across the normal condition, water stress, and heat stress; the asterisk “*” indicates significant differences between the control and the +Spm treatments under one particular condition (normal condition, water stress, or heat stress) based on LSD (P < 0.05). Total PA content was calculated by the sum of Put, Spd, and Spm contents.
Water stress and heat stress significantly decreased the RGR of the control and +Spm treatments, but the +Spm treatment showed significantly higher RGR than the control under normal condition, water stress, and heat stress (Figure 2A). The application of exogenous Spm significantly alleviated the decline in leaf RWC induced by water stress and heat stress (Figure 2B). As compared to untreated control, Spm-pretreated plants had a 17 or 50% increase in OA under water stress or heat stress, respectively (Figure 2C). Chl content, Pn, and WUE also significantly declined in response to water stress and heat stress in both of Spm-pretreated and non-treated plants, whereas Spm-pretreated plants showed significantly higher Chl content, Pn, and WUE than non-treated plants under water stress and heat stress (Figures 3A–C).
Figure 2. Changes in (A) relative growth rate (RGR), (B) relative water content (RWC), and (C) osmotic adjustment (OA) in response to Spm application under different conditions. Vertical bars indicate ±SE of mean (n = 4). Different letters above striated or gray columns indicate significant differences for a particular treatment (Control or +Spm) for comparison across the normal condition, water stress, and heat stress; the asterisk “*” indicates significant differences between the control and the +Spm treatments under one particular condition (normal condition, water stress, or heat stress) based on LSD (P < 0.05).
Figure 3. Changes in (A) chlorophyll (Chl) content, (B) net photosynthetic rate (Pn), and (C) water use efficiency (WUE) in response to Spm application under different conditions. Vertical bars indicate ±SE of mean (n = 4). Different letters above striated or gray columns indicate significant differences for a particular treatment (Control or +Spm) for comparison across the normal condition, water stress, and heat stress; the asterisk “*” indicates significant differences between the control and the +Spm treatments under one particular condition (normal condition, water stress, or heat stress) based on LSD (P < 0.05).
H2O2, protein carbonyl content, MDA content, and EL were not significantly affected by Spm priming under normal condition (Figures 4A–D). Water stress and heat stress significantly induced increases in these four parameters in both the control and +Spm treatments; however, Spm priming significantly decreased stress-induced increases in H2O2, carbonyl, MDA content, and EL in leaves (Figures 4A–D).
Figure 4. Changes in endogenous (A) hydrogen peroxide (H2O2), (B) carbonyl content, (C) malonaldehyde (MDA), and (D) electrolyte leakage (EL) in response to Spm application under different conditions. Vertical bars indicate ±SE of the mean (n = 4). Different letters above striated or gray columns indicate significant differences for a particular treatment (Control or +Spm) for comparison across the normal condition, water stress, and heat stress; the asterisk “*” indicates significant differences between the control and the +Spm treatments under one particular condition (normal condition, water stress, or heat stress) based on LSD (P < 0.05).
A total of 61 metabolites were identified in leaves of creeping bentgrass under normal condition, water stress, and heat stress (Figure 5A). These metabolites included 16 sugars, 16 amino acids, 15 organic acids, and 14 other metabolites. A heatmap of all metabolites showed downregulation and upregulation as Spm-treated plants compared to untreated plants under normal condition, water stress, or heat stress, respectively (Figure 5A). The ratio of no change, a significant increase, or a significant decline in metabolites was shown in Figure 5B, when Spm-pretreated plants were compared to untreated plants under normal condition, water stress, or heat stress. Water stress or heat stress significantly decreased total metabolites accumulation, and Spm-pretreated plants exhibited significantly higher total metabolites content than untreated plants under water stress (Figure 5C). Similarly, total sugars, amino acids, organic acids, or other metabolites also significantly declined in all plants under water stress and heat stress (Figures 6A–D). The Spm-pretreated plants accumulated significantly more total amino acids and organic acids under water stress (Figures 6B,C).
Figure 5. Changes in (A) the heatmap of all identified metabolites, (B) percentage of upregulated, downregulated, and unchanged metabolites, and (C) relative content of total metabolites in response to Spm application under different conditions. Vertical bars indicate ±SE of mean (n = 4). Different letters above striated or gray columns indicate significant differences for a particular treatment (Control or +Spm) for comparison across the normal condition, water stress, and heat stress; the asterisk “*” indicates significant differences between the control and the +Spm treatments under one particular condition (normal condition, water stress, or heat stress) based on LSD (P < 0.05).
Figure 6. Changes in the relative content of (A) sugars, (B) amino acids, (C) organic acids, and (D) other metabolites in response to Spm application under different conditions. Vertical bars indicate ±SE of mean (n = 4). Different letters above striated or gray columns indicate significant differences for a particular treatment (Control or +Spm) for comparison across the normal condition, water stress, and heat stress; the asterisk “*” indicates significant differences between the control and the +Spm treatments under one particular condition (normal condition, water stress, or heat stress) based on LSD (P < 0.05).
Supplementary Figures S1–S4 showed the relative content of 61 metabolites. Among these metabolites, Spm upregulated the accumulation of 10 sugars (kestose, maltotriose, maltose, galactose, fructose, xylose, tagatose, mannose, glucose-1-phosphate, and sucrose-6-phosphate), 5 amino acids (serine, threonine, methionine, histidine, and glutamic acid), 7 organic acids (pyruvic acid, ketoglutaric acid, aconitic acid, glyceric acid, itaconic acid, maleic acid, and quinic acid), and 5 other metabolites (ribitol, myo-inositol, urea, mannosylglycerate, and nicotinoylglycine) under water stress (Supplementary Figures S1–S4). Under heat stress, Spm application improved the accumulation of maltose, galactose, mannose, alanine, glycine, malic acid, ketobutyric acid, gallic acid, urea, nicotinic acid, and nicotinoylglycine (Supplementary Figures S1–S4). As seen in Figure 7, Spm regulated 21 metabolites under water stress. A total of 14 metabolites responded to Spm application under heat stress (Figure 7). Spm induced changes in 19 metabolites in leaves under water and heat stress (Figure 7). Pathway enrichment analysis showed 45 identified metabolites involved in the tricarboxylic acid (TCA) cycle, GABA shunt, and other metabolic pathways (Figure 8).
Figure 7. Common and differential metabolites regulated by spermine under water stress and heat stress in leaves of creeping bentgrass.
Figure 8. Pathway enrichment analysis of 45 identified metabolites in leaves of creeping bentgrass regulated by spermine under the normal condition, water stress, and heat stress.
Increases in endogenous PAs biosynthesis and catabolism were propitious to achieve tolerance to water stress in Arabidopsis thaliana (Sen et al., 2018; Sen and Mohapatra, 2022). However, enhanced PA metabolism could also improve the risk of oxidative damage, because PA catabolism was known to generate ROS (Mohapatra et al., 2009). Our current study showed that Spm pretreatment did not significantly affect the accumulation of total PAs, Spd, or Spm and physiological parameters, including RWC, Chl content, Pn, WUE, H2O2 content, carbonyl content, MDA content, and EL level in leaves of creeping bentgrass under the normal condition, but significantly improved RGR. These findings indicated that an appropriate dose of Spm pretreatment did not cause deleterious effects in creeping bentgrass under the normal condition, and Spm could be catabolized for maintaining growth during 18 days of normal cultivation. In addition, alterations of endogenous PA content under stress conditions are evident in many plant species in relation to drought and heat tolerance. For example, declines in endogenous Put, Spd, and Spm content by the application of a biosynthetic inhibitor of PAs significantly weakened the adaptive response of white clover to water stress (Li et al., 2015b). Significant increases in endogenous Spd and Spm levels induced by exogenous Spd enhanced the heat tolerance of rice (Zhou et al., 2020). Better maintenance of water balance and photosynthesis was associated with Spd-regulated tolerance to water stress in white clover (Li et al., 2015a). The study of Fu et al. (2014) also proved that Spm pretreatment conferred combined heat and drought tolerance in trifoliate orange (Poncirus trifoliata) by alleviating oxidative damage. Similar results were found in this study. Spm pretreatment induced significant increases in endogenous Put, Spd, and Spm under water or heat stress, followed by the mitigation of detrimental effects of water stress or heat stress, such as growth inhibition, photoinhibition, water loss, and oxidative damage in creeping bentgrass. Evidently, PA-regulated tolerance to water stress and heat stress were closely related to OA, photosynthetic maintenance, and oxidation-reduction equilibrium in plants. Some common or particular organic metabolites contributing to OA and redox homeostasis in creeping bentgrass in response to water and heat stress are discussed below.
Regulatory effects of Spm on sugar accumulation and metabolism have been reported in many plant species under drought since sugars exhibit the important function of OA, signal transduction, and energy supply (Hasan et al., 2021). For example, exogenous application of Spm improved total soluble sugars accumulation in favor of water relation in soybean (Glycine max) (Dawood and Abeed, 2020), white clover (Li et al., 2015a), or bermudagrass (Cynodon dactylon) (Shi et al., 2013) under water deficit condition. In addition, Spm-induced accumulation of total available carbohydrates improved OA and metabolic homeostasis, which alleviated heat-induced leaf senescence (Liang et al., 2021). However, Spm-regulated specific sugars, such as monosaccharides, disaccharides, and polysaccharides, have not been well reported in plants under drought or heat stress. In the current study, the Spm jointly regulated the accumulation of mannose, maltose, and galactose in leaves of creeping bentgrass under water and heat stress. Mannose, maltose, and galactose are not only important osmolytes and energy matters but also have other potential functions for stress adaptation in plants. It has been found that mannose was a negative regulator of senescence and also responsible for the improvement in redox homeostasis and growth under water deficit or high-temperature conditions (Wang et al., 2011; Zhao et al., 2020; Guo et al., 2022). The study of Ma et al. (2014) found that drought tolerance of transgenic Arabidopsis overexpressing the alfalfa (Medicago Sativa) GME gene that catalyzed the conversion of GDP-D-mannose to GDP-L-galactose was significantly improved and was associated with a significant increase in antioxidant capacity. Maltose is a compatible-solute stabilizing factor that has the ability to protect proteins, membranes, and photosynthetic electron transport chains under high-temperature stress (Kaplan and Guy, 2004). Maltose also exhibited a positive effect on alleviating drought-induced wheat growth inhibition (Ibrahim and Abdellatif, 2016). These studies together with our current findings indicated that Spm-regulated tolerance to water stress or heat stress could be related to enhanced OA, osmoprotection, and antioxidant capacity by improving the accumulation of mannose, maltose, and galactose in creeping bentgrass.
Interestingly, exogenous application of Spm significantly induced a 27, 100, 19, 77, 47, or 53% increase in glucose-1-phosphate, sucrose-6-phosphate, fructose, kestose, maltotriose, or xylose content when creeping bentgrass suffered from water stress. Sucrose-6-phosphate and glucose-1-phosphate play important roles in sugar metabolism, carbon flux, and the energy cycle in plants (Engels et al., 2008; Poonam et al., 2016). Nano-zinc oxide promoted the synthesis of glucose-1-phosphate that was a benefit for drought tolerance in maize (Zea mays) (Sun et al., 2021). It has been found that the accumulation of fructose as a common osmolyte for OA or precursor in cellular respiration helped drought-tolerant Thymus serpyllum and sensitive Thymus vulgaris plants to tolerate drought stress (Ashrafi et al., 2018). Exogenous application of chitosan and mannose enhanced drought tolerance of white clover and creeping bentgrass associated with the accumulation of fructose and xylose (Li et al., 2017b, 2019). In addition, the study by Liu et al. (2019) found that kestose accumulation likely functioned as a storage carbohydrate that provided energy for rhizosheath formation of switchgrass (Panicum virgatum) under drought stress. Maltotriose was a common trisaccharide among rice genotypes in response to drought stress (Da Costa et al., 2022). Spm-induced accumulation of these sugars could play positive roles in drought tolerance, but an individual function of these sugars deserved to be further investigated in our further study.
Amino acids are critical primary metabolites for plant growth and development, reproduction, and stress adaptation (Hildebrandt Tatjana et al., 2015). A previous study proved that upregulation of Put metabolism affected the accumulation of almost all amino acids in cells (Mohapatra et al., 2010). For the possible function of various amino acids, alanine and glycine could induce heat shock protein 70 (HSP70) expression in cells associated with the improvement in thermotolerance (Nissim et al., 1992). Significant increases in alanine and glycine were also related to improved heat tolerance of creeping bentgrass (Li et al., 2016a), which was consistent with our current finding in Spm-treated creeping bentgrass. It was noteworthy that Spm expressly induced the accumulation of glutamic acid, methionine, serine, and threonine in leaves of creeping bentgrass under water stress. Glutamic acid not only acts as a signal trigger and nitrogen resource for plant growth but also is involved in chlorophyll biosynthesis for photosynthetic performance (Forde and Walch-Liu, 2009; Toyota et al., 2018). Exogenous application of glutamic acid could suppress heat-induced leaf senescence and chlorophyll degradation by improving amino acid metabolism in creeping bentgrass (Stephanie et al., 2021). In addition, it was found that drought stress decreased the methionine biosynthesis pathways in plants (Larrainzar et al., 2014). However, exogenous application of methionine mitigated oxidative damage and growth retardation of bitter gourd (growth retardation) under drought stress (Akram et al., 2020). Enhanced accumulation of serine and threonine induced by exogenous application of chitosan improved stress adaptation of white clover to water deficit (Li et al., 2017b). In response to water stress, Spm-regulated accumulation of amino acids as mentioned above could maintain metabolic and growth homeostasis in creeping bentgrass.
In addition to sugars and amino acids, multiple organic acids were also significantly regulated by Spm in creeping bentgrass under water stress or heat stress. Pyruvic acid acts as an intermediate compound during the metabolism of carbohydrates and proteins and is also metabolized into the TCA cycle to produce energy in plants (Tovar-Méndez et al., 2003). Aconitic acid and ketoglutaric acid are two intermediates of the TCA cycle (Sweetlove et al., 2010). It was found that the maintenance of accumulation of TCA cycle intermediates was one of the important regulatory mechanisms for plant growth and stress tolerance under drought stress (Merewitz et al., 2011; Guo et al., 2018; Li et al., 2019). In the current study, water stress extremely decreased the accumulation of pyruvic acid, aconitic acid, and ketoglutaric acid in leaves of Spm-pretreated and untreated creeping bentgrass plants, but the application of Spm could significantly alleviate the deficit of these metabolites under water stress. This could indicate Spm-regulated growth and tolerance to water deficit associated with better maintenance of the TCA cycle for energy production in creeping bentgrass. However, Spm pretreatment did not enhance the accumulation of intermediates of the TCA cycle but increased the accumulation of other organic acids such as gallic acid and malic acid under heat stress. Previous studies proved that the antioxidant potency of gallic acid is associated with the amelioration of tolerance to high temperature, salt stress, and osmotic stress in plants (Ozfidan-Konakci et al., 2015; Farhoosh and Nyström, 2018). The study of Du et al. (2011) found that heat-tolerant hybrid bermudagrass (Cynodon transvaalensis × Cynodon dactylon) accumulated more malic acid than heat-sensitive Kentucky bluegrass (Poa pratensis) during long-term heat stress, suggesting that the malic acid could play an important role in hybrid bermudagrass adaptation to heat stress.
Other metabolites including myo-inositol, nicotinic acid, and urea were also related to stress adaptation in plants. Beneficial effects of myo-inositol on ameliorating drought damage have been widely studied in plants. For example, foliar application of myo-inositol effectively alleviated drought damage to creeping bentgrass through detoxifying reactive oxygen species (ROS) and improving OA (Li et al., 2020b). Transgenic sweet potato (Ipomoea batatas) plants overexpressing a myo-inositol-1-phosphate synthase (MIPS) gene encoding myo-inositol biosynthesis exhibited better drought tolerance than wild type associated with stronger ROS-scavenging system and sensitive signal transduction pathways in response to drought stress (Zhai et al., 2016). Nicotinic acid known as vitamin B3 has also been reported to be propitious to plants' survival and growth under abiotic stress. Overexpression of a nicotinamidase 3 (NIC3) gene involved in nicotinic acid biosynthesis exhibited similar effects with exogenous application of nicotinic acid on improving stress tolerance and biomass of Arabidopsis thaliana under drought stress (Ahmad et al., 2021). Exogenous application of nicotinic acid could also effectively alleviate salinity-induced damage and biomass loss of onion (Allium cepa) plants (Hussein et al., 2014). In addition, urea as the main nitrogen source has dual functions of growth promotion and stress tolerance in plants (Witte, 2011). Urea application could delay the senescence of creeping bentgrass by stabilizing proteins and cell membranes under heat stress (Jespersen and Huang, 2015). These studies together with our current findings indicated that Spm-induced a 59% increase in myo-inositol under water stress, a 321% increase in nicotinic acid under heat stress, and a 184 or a 29% increase in urea under water stress or heat stress could contribute to growth maintenance and better stress tolerance in creeping bentgrass.
Exogenous application of Spm could effectively alleviate growth retardant and damage effects induced by water and heat stress by increasing endogenous PAs, Put, Spd, and Spm contents. The physiological analysis found that Spm regulated water homeostasis, photosynthetic performance, oxidative damage, and cell membrane stability when creeping bentgrass responded to water or heat stress. Metabolites profiling showed that a total of 61 metabolites were differentially or commonly regulated by Spm in leaves of creeping bentgrass under water stress and heat stress. Under water stress, Spm mainly induced the accumulation of glucose-1-phosphate, sucrose-6-phosphate, fructose, kestose, maltotriose, xylose, glutamic acid, methionine, serine, threonine pyruvic acid, aconitic acid, ketoglutaric acid, and myo-inositol. In response to heat stress, the accumulation of alanine, glycine, gallic acid, malic acid, and nicotinic acid was specifically regulated by Spm. Spm also commonly enhanced the accumulation of mannose, maltose, galactose, and urea under water and heat stress. The current study provides novel evidence of global metabolites reprogramming associated with Spm-induced tolerance to water stress and heat stress in horticultural plants.
The original contributions presented in the study are included in the article/Supplementary material, further inquiries can be directed to the corresponding author.
ZL: methodology, investigation, conceptualization, writing—original draft, and funding acquisition. BC and XW: methodology and investigation. YZ and GF: writing—review and editing. YP: supervision, conceptualization, writing—review and editing, and funding acquisition. All authors contributed to the article and approved the submitted version.
This research was supported by the China Postdoctoral Science Foundation (2021T140058) and the Sichuan Forage Innovation Team Project of the Industrial System Construction of Modern Agriculture of China (sccxtd-2020-16).
The authors declare that the research was conducted in the absence of any commercial or financial relationships that could be construed as a potential conflict of interest.
All claims expressed in this article are solely those of the authors and do not necessarily represent those of their affiliated organizations, or those of the publisher, the editors and the reviewers. Any product that may be evaluated in this article, or claim that may be made by its manufacturer, is not guaranteed or endorsed by the publisher.
The Supplementary Material for this article can be found online at: https://www.frontiersin.org/articles/10.3389/fpls.2022.944358/full#supplementary-material
Ahmad, Z., Bashir, K., Matsui, A., Tanaka, M., Sasaki, R., Oikawa, A., et al. (2021). Overexpression of nicotinamidase 3 (NIC3) gene and the exogenous application of nicotinic acid (NA) enhance drought tolerance and increase biomass in Arabidopsis. Plant Mol. Biol. 107, 63–84. doi: 10.1007/s11103-021-01179-z
Akram, N. A., Umm, H., Ashraf, M., Ashraf, M., and Sadiq, M. (2020). Exogenous application of L-methionine mitigates the drought-induced oddities in biochemical and anatomical responses of bitter gourd (Momordica charantia L.). Sci. Hortic. 267, 109333. doi: 10.1016/j.scienta.2020.109333
Amnon, D. (1949). Copper enzymes in isolated chloroplasts. Polyphenoloxidase in Beta vulgaris. Plant Physiol. 24, 1–15. doi: 10.1104/pp.24.1.1
Ashrafi, M., Azimi-Moqadam, M. R., Moradi, P., MohseniFard, E., Shekari, F., and Kompany-Zareh, M. (2018). Effect of drought stress on metabolite adjustments in drought tolerant and sensitive thyme. Plant Physiol. Biochem. 132, 391–399. doi: 10.1016/j.plaphy.2018.09.009
Barrs, H., and Weatherley, P. (1962). A re-examination of the relative turgidity technique for estimating water deficits in leaves. Aust. J. Biol. Sci. 15, 413–428. doi: 10.1071/BI9620413
Batista-Silva, W., Heinemann, B., Rugen, N., Nunes-Nesi, A., Araújo, W. L., Braun, H., et al. (2019). The role of amino acid metabolism during abiotic stress release. Plant Cell Environ. 42, 1630–1644. doi: 10.1111/pce.13518
Blum, A. (1989). Osmotic adjustment and growth of barley genotypes under drought stress. Crop Sci. 29, 230–233. doi: 10.2135/cropsci1989.0011183X002900010052x
Blum, A., and Ebercon, A. (1981). Cell membrane stability as a measure of drought and heat tolerance in wheat. Crop Sci. 21, 43–47. doi: 10.2135/cropsci1981.0011183X002100010013x
Cayan, D. R., Das, T., Pierce, D. W., Barnett, T. P., Tyree, M., Gershunov, A., et al. (2010). Future dryness in the southwest US and the hydrology of the early 21st century drought. Proc. Natl. Acad. Sci. USA. 107, 21271–21276. doi: 10.1073/pnas.0912391107
Chen, D., Shao, Q., Yin, L., Younis, A., and Zheng, B. (2019). Polyamine function in plants: metabolism, regulation on development, and roles in abiotic stress responses. Front. Plant Sci. 9, 1945. doi: 10.3389/fpls.2018.01945
Cramer, G. R., Kaoru, U., Serge, D., Mario, P., and Kazuo, S. (2011). Effects of abiotic stress on plants: a systems biology perspective. BMC Plant Biol. 11, 163. doi: 10.1186/1471-2229-11-163
Da Costa, M. V. J., Ramegowda, V., Ramakrishnan, P., Karaba, N. N., and Sheshshayee, M. S. (2022). Comparative metabolite profiling of rice contrasts reveal combined drought and heat stress signatures in flag leaf and spikelets. Plant Sci. 320, 111262. doi: 10.1016/j.plantsci.2022.111262
Dawood, M. F., and Abeed, A. H. (2020). Spermine-priming restrained water relations and biochemical deteriorations prompted by water deficit on two soybean cultivars. Heliyon 6, e04038. doi: 10.1016/j.heliyon.2020.e04038
Dhindsa, R., Plumb-Dhindsa, P., and Thorpe, T. (1981). Leaf senescence: correlated with increased levels of membrane permeability and lipid peroxidation, and decreased levels of superoxide dismutase and catalase. J. Exp. Bot. 32, 93–101. doi: 10.1093/jxb/32.1.93
Du, H., Wang, Z., Yu, W., Liu, Y., and Huang, B. (2011). Differential metabolic responses of perennial grass Cynodon transvaalensis × Cynodon dactylon (C4) and Poa Pratensis (C3) to heat stress. Physiol. Plant. 141, 251–264. doi: 10.1111/j.1399-3054.2010.01432.x
Duan, J., Li, J., Guo, S., and Kang, Y. (2008). Exogenous spermidine affects polyamine metabolism in salinity-stressed Cucumis sativus roots and enhances short-term salinity tolerance. J. Plant Physiol. 165, 0–1635. doi: 10.1016/j.jplph.2007.11.006
Engels, V., Georgi, T., and Wendisch, V. F. (2008). ScrB (Cg2927) is a sucrose-6-phosphate hydrolase essential for sucrose utilization by Corynebacterium glutamicum. FEMS Microbiol. Lett. 289, 80–89. doi: 10.1111/j.1574-6968.2008.01370.x
Farhoosh, R., and Nyström, L. (2018). Antioxidant potency of gallic acid, methyl gallate and their combinations in sunflower oil triacylglycerols at high temperature. Food Chem. 244, 29–35. doi: 10.1016/j.foodchem.2017.10.025
Forde, B. G., and Walch-Liu, P. (2009). Nitrate and glutamate as environmental cues for behavioural responses in plant roots. Plant Cell Environ. 32, 682–693. doi: 10.1111/j.1365-3040.2008.01927.x
Fu, X. Z., Xing, F., Wang, N. Q., Peng, L. Z., Chun, C. P., Cao, L., et al. (2014). Exogenous spermine pretreatment confers tolerance to combined high-temperature and drought stress in vitro in trifoliate orange seedlings via modulation of antioxidative capacity and expression of stress-related genes. Biotechnol. Biotechnol. Equip. 28, 192–198. doi: 10.1080/13102818.2014.909152
Fu, Y., Gu, Q., Dong, Q., Zhang, Z., Lin, C., Hu, W., et al. (2019). Spermidine enhances heat tolerance of rice seeds by modulating endogenous starch and polyamine metabolism. Molecules 24, 1395. doi: 10.3390/molecules24071395
Ghatak, A., Chaturvedi, P., and Weckwerth, W. (2018). Metabolomics in plant stress physiology. Adv. Biochem. Eng. Biotechnol. 164, 187–236. doi: 10.1007/10_2017_55
Gong, Z., Xiong, L., Shi, H., Yang, S., Herrera-Estrella, L. R., Xu, G., et al. (2020). Plant abiotic stress response and nutrient use efficiency. Sci. China Life Sci. 63, 39. doi: 10.1007/s11427-020-1683-x
Guo, F., Han, A., Gao, H., Liang, J., Zhao, K., Cao, S., et al. (2022). Mannose alleviates yellowing process of broccoli florets by regulating chlorophyll catabolism and delaying programmed cell death. Sci. Hortic. 295, 110888. doi: 10.1016/j.scienta.2022.110888
Guo, R., Shi, L., Jiao, Y., Li, M., Zhong, X., Gu, F., et al. (2018). Metabolic responses to drought stress in the tissues of drought-tolerant and drought-sensitive wheat genotype seedlings. AoB Plants 10, ply016. doi: 10.1093/aobpla/ply016
Hasan, M., Skalicky, M., Jahan, M. S., Hossain, M., Anwar, Z., Nie, Z., et al. (2021). Spermine: its emerging role in regulating drought stress responses in plants. Cells 10, 261. doi: 10.3390/cells10020261
Hildebrandt Tatjana, M., Nunes Nesi, A., Araújo Wagner, L., and Braun, H.-P. (2015). Amino acid catabolism in plants. Mol. Plant 8, 1563–1579. doi: 10.1016/j.molp.2015.09.005
Hoagland, D. R., and Arnon, D. I. (1950). The water culture method for growing plants without soil. California Agric. Exp. Station Circ. 347, 32.
Hussein, M., Faham, S., and Alva, A. (2014). Role of foliar application of nicotinic acid and tryptophan on onion plants response to salinity stress. J. Agric. Sci. 6, 41–51. doi: 10.5539/jas.v6n8p41
Ibrahim, H. A., and Abdellatif, Y. M. R. (2016). Effect of maltose and trehalose on growth, yield and some biochemical components of wheat plant under water stress. Ann. Agric. Sci. 61, 267–274. doi: 10.1016/j.aoas.2016.05.002
Jespersen, D., and Huang, B. (2015). Proteins associated with heat-induced leaf senescence in creeping bentgrass as affected by foliar application of nitrogen, cytokinins, and an ethylene inhibitor. Proteomics 15, 798–812. doi: 10.1002/pmic.201400393
Jing, Z., Qing, Z., Jing, X., Huibin, L., Jiamin, M., Bin, X., et al. (2021). Acetic acid mitigated salt stress by alleviating ionic and oxidative damages and regulating hormone metabolism in perennial ryegrass (Lolium perenne L.). Grass Res. 1, 1–10. doi: 10.48130/GR-2021-0003
Kaplan, F., and Guy, C. L. (2004). β-amylase induction and the protective role of maltose during temperature shock. Plant Physiol. 135, 1674–1684. doi: 10.1104/pp.104.040808
Larrainzar, E., Molenaar, J. A., Wienkoop, S., Gil-Quintana, E., Alibert, B., Limami, A. M., et al. (2014). Drought stress provokes the down-regulation of methionine and ethylene biosynthesis pathways in M .edicago truncatula roots and nodules. Plant Cell Environ. 37, 2051–2063. doi: 10.1111/pce.12285
Li, Z., Cheng, B., Zeng, W., Zhang, X., and Peng, Y. (2020a). Proteomic and metabolomic profilings reveal crucial functions of γ-aminobutyric acid in regulating Ionic, water, and metabolic homeostasis in creeping bentgrass under salt stress. J. Proteome Res. 19, 769–780. doi: 10.1021/acs.jproteome.9b00627
Li, Z., Fu, J., Shi, D., and Peng, Y. (2020b). Myo-inositol enhances drought tolerance in creeping bentgrass through alteration of osmotic adjustment, photosynthesis, and antioxidant defense. Crop Sci. 60, 2149–2158. doi: 10.1002/csc2.20186
Li, Z., Jing, W., Peng, Y., Zhang, X. Q., Ma, X., Huang, L. K., et al. (2015a). Spermine alleviates drought stress in white clover with different resistance by influencing carbohydrate metabolism and dehydrins synthesis. PLoS ONE 10, e0120708. doi: 10.1371/journal.pone.0120708
Li, Z., Yong, B., Cheng, B., Wu, X., Zhang, Y., Zhang, X., et al. (2019). Nitric oxide, γ-aminobutyric acid, and mannose pretreatment influence metabolic profiles in white clover under water stress. J. Integr. Plant Biol. 61, 1255–1273. doi: 10.1111/jipb.12770
Li, Z., Yu, J., Peng, Y., and Huang, B. (2016a). Metabolic pathways regulated by γ-aminobutyric acid (GABA) contributing to heat tolerance in creeping bentgrass (Agrostis stolonifera). Sci. Rep. 6, 30338. doi: 10.1038/srep30338
Li, Z., Yu, J., Peng, Y., and Huang, B. (2017a). Metabolic pathways regulated by abscisic acid, salicylic acid and -aminobutyric acid in association with improved drought tolerance in creeping bentgrass (Agrostis stolonifera). Physiol. Plant. 159, 42–58. doi: 10.1111/ppl.12483
Li, Z., Zhang, Y., Peng, D., Peng, Y., Zhang, X., Ma, X., et al. (2018). The inhibition of polyamine biosynthesis weakens the drought tolerance in white clover (Trifolium repens) associated with the alteration of extensive proteins. Protoplasma 255, 803–817. doi: 10.1007/s00709-017-1186-9
Li, Z., Zhang, Y., Peng, D., Wang, X., Peng, Y., He, X., et al. (2015b). Polyamine regulates tolerance to water stress in leaves of white clover associated with antioxidant defense and dehydrin genes via involvement in calcium messenger system and hydrogen peroxide signaling. Front. Physiol. 6, 280. doi: 10.3389/fphys.2015.00280
Li, Z., Zhang, Y., Zhang, X., Merewitz, E., Peng, Y., Ma, X., et al. (2017b). Metabolic pathways regulated by chitosan contributing to drought resistance in white clover. J. Proteome Res. 16, 3039–3052. doi: 10.1021/acs.jproteome.7b00334
Li, Z., Zhang, Y., Zhang, X., Peng, Y., Merewitz, E., Ma, X., et al. (2016b). The alterations of endogenous polyamines and phytohormones induced by exogenous application of spermidine regulate antioxidant metabolism, metallothionein and relevant genes conferring drought tolerance in white clover. Environ. Exp. Bot. 124, 22–38. doi: 10.1016/j.envexpbot.2015.12.004
Li, Z., Zou, Y., Li, Y., Sun, C., Liu, Y., Cui, L., et al. (2021). Exploring the salt- and drought-tolerant genes of alfalfa through expression library screening strategy. Grass Res. 1, 1–14. doi: 10.48130/GR-2021-0011
Liang, L. L., Cao, Y. Q., Wang, D., Peng, Y., Zhang, Y., Li, Z., et al. (2021). Spermine alleviates heat-induced senescence in creeping bentgrass by regulating water and oxidative balance, photosynthesis, and heat shock proteins. Biol. Plant. 65, 184–192. doi: 10.32615/bp.2021.008
Liu, T. Y., Chen, M. X., Zhang, Y., Zhu, F. Y., Liu, Y. G., Tian, Y., et al. (2019). Comparative metabolite profiling of two switchgrass ecotypes reveals differences in drought stress responses and rhizosheath weight. Planta 250, 1355–1369. doi: 10.1007/s00425-019-03228-w
Ma, L., Wang, Y., Liu, W., and Liu, Z. (2014). Overexpression of an alfalfa GDP-mannose 3, 5.-epimerase gene enhances acid, drought and salt tolerance in transgenic Arabidopsis by increasing ascorbate accumulation. Biotechnol. Lett. 36, 2331–2341. doi: 10.1007/s10529-014-1598-y
Ma, Q., Yue, L. J., Zhang, J. L., Wu, G. Q., and Bao, A. K. (2012). Sodium chloride improves photosynthesis and water status in the succulent xerophyte Zygophyllum xanthoxylum. Tree Physiol. 32, 4–13. doi: 10.1093/treephys/tpr098
Martínez-Noël, G. M. A., and Tognetti, J. A. (2018). Sugar signaling under abiotic stress in plants. Plant Metab. Regul. Under Environ. Stress 2018, 397–406. doi: 10.1016/B978-0-12-812689-9.00022-4
Merewitz, E. B., Du, H., Yu, W., Liu, Y., Gianfagna, T., Huang, B., et al. (2011). Elevated cytokinin content in ipt transgenic creeping bentgrass promotes drought tolerance through regulating metabolite accumulation. J. Exp. Bot. 63, 1315–1328. doi: 10.1093/jxb/err372
Mohapatra, S., Minocha, R., Long, S., and Minocha, S. C. (2009). Putrescine overproduction negatively impacts the oxidative state of poplar cells in culture. Plant Physiol. Biochem. 47, 262–271. doi: 10.1016/j.plaphy.2008.12.007
Mohapatra, S., Minocha, R., Long, S., and Minocha, S. C. (2010). Transgenic manipulation of a single polyamine in poplar cells affects the accumulation of all amino acids. Amino Acids 38, 1117–1129. doi: 10.1007/s00726-009-0322-z
Mostofa, M. G., Yoshida, N., and Fujita, M. (2014). Spermidine pretreatment enhances heat tolerance in rice seedlings through modulating antioxidative and glyoxalase systems. Plant Growth Regul. 73, 31–44. doi: 10.1007/s10725-013-9865-9
Nissim, I., Hardy, M., Pleasure, J., Nissim, I., and States, B. (1992). A mechanism of glycine and alanine cytoprotective action: stimulation of stress-induced HSP70 mRNA. Kidney Int. 42, 775–782. doi: 10.1038/ki.1992.347
Ozfidan-Konakci, C., Yildiztugay, E., and Kucukoduk, M. (2015). Upregulation of antioxidant enzymes by exogenous gallic acid contributes to the amelioration in Oryza sativa roots exposed to salt and osmotic stress. Environ. Sci. Pollut. Res. 22, 1487–1498. doi: 10.1007/s11356-014-3472-9
Poonam, R. B., Handa, N., Kaur, H., Rattan, A., Bali, S., Gautam, V., et al. (2016). Sugar signalling in plants: a novel mechanism for drought stress management. Water Stress Crop Plants 2016, 287–302. doi: 10.1002/9781119054450.ch19
Qiu, Y., Cai, G., Su, M., Chen, T., Zheng, X., Xu, Y., et al. (2009). Serum metabolite profiling of human colorectal cancer using GC–TOFMS and UPLC–QTOFMS. J. Proteome Res. 8, 4844–4850. doi: 10.1021/pr9004162
Roessner, U., Wagner, C., Kopka, J., Trethewey, R. N., and Willmitzer, L. (2000). Simultaneous analysis of metabolites in potato tuber by gas chromatography-mass spectrometry. Plant J. 23, 131–142. doi: 10.1046/j.1365-313x.2000.00774.x
Sanchez, D. H., Schwabe, F., Erban, A., Udvardi, M. K., and Kopka, J. (2012). Comparative metabolomics of drought acclimation in model and forage legumes. Plant Cell Environ. 35, 136–149. doi: 10.1111/j.1365-3040.2011.02423.x
Sen, S., Ghosh, D., and Mohapatra, S. (2018). Modulation of polyamine biosynthesis in Arabidopsis thaliana by a drought mitigating Pseudomonas putida strain. Plant Physiol. Biochem. 129, 180–188. doi: 10.1016/j.plaphy.2018.05.034
Sen, S., and Mohapatra, S. (2022). Drought-mitigating Pseudomonas putida strain modulates polyamine catabolism in Arabidopsis thaliana. J. Plant Growth Regul. 41, 300–313. doi: 10.1007/s00344-021-10297-3
Shi, H., Ye, T., and Chan, Z. (2013). Comparative proteomic and physiological analyses reveal the protective effect of exogenous polyamines in the bermudagrass (Cynodon dactylon) response to salt and drought stresses. J. Proteome Res. 12, 4951–4964. doi: 10.1021/pr400479k
Srivastava, S., Brychkova, G., Yarmolinsky, D., Soltabayeva, A., Samani, T., and Sagi, M. (2017). Aldehyde oxidase 4 plays a critical role in delaying silique senescence by catalyzing aldehyde detoxification. Plant Physiol. 173, 1977–1997. doi: 10.1104/pp.16.01939
Stephanie, R., Cathryn, C., Bo, Y., and Bingru, H. (2021). Glutamate acts as a repressor for heat-induced leaf senescence involving chlorophyll degradation and amino acid metabolism in creeping bentgrass. Grass Research 1, 1–10. doi: 10.48130/GR-2021-0004
Sun, L., Song, F., Zhu, X., Liu, S., Liu, F., Wang, Y., et al. (2021). Nano-ZnO alleviates drought stress via modulating the plant water use and carbohydrate metabolism in maize. Archives of Agronomy and Soil Science 67, 245–259. doi: 10.1080/03650340.2020.1723003
Sun, Q., Miao, C., Hanel, M., Borthwick, A. G. L., Duan, Q., Ji, D., et al. (2019). Global heat stress on health, wildfires, and agricultural crops under different levels of climate warming. Environ. Int. 128, 125–136. doi: 10.1016/j.envint.2019.04.025
Sweetlove, L. J., Beard, K. F., Nunes-Nesi, A., Fernie, A. R., and Ratcliffe, R. G. (2010). Not just a circle: flux modes in the plant TCA cycle. Trends Plant Sci. 15, 462–470. doi: 10.1016/j.tplants.2010.05.006
Tajti, J., Janda, T., Majláth, I., Szalai, G., and Pál, M. (2018). Comparative study on the effects of putrescine and spermidine pre-treatment on cadmium stress in wheat. Ecotoxicol. Environ. Saf. 148, 546–554. doi: 10.1016/j.ecoenv.2017.10.068
Tovar-Méndez, A., Miernyk, J. A., and Randall, D. D. (2003). Regulation of pyruvate dehydrogenase complex activity in plant cells. Eur. J. Biochem. 270, 1043–1049. doi: 10.1046/j.1432-1033.2003.03469.x
Toyota, M., Spencer, D., Sawai-Toyota, S., Jiaqi, W., Zhang, T., Koo, A. J., et al. (2018). Glutamate triggers long-distance, calcium-based plant defense signaling. Science. 361, 1112–1115. doi: 10.1126/science.aat7744
Velikova, V., Yordanov, I., and Edreva, A. (2000). Oxidative stress and some antioxidant systems in acid rain-treated bean plants: protective role of exogenous polyamines. Plant Sci. 151, 59–66. doi: 10.1016/S0168-9452(99)00197-1
Wang, H. S., Chao, Y., Zhu, Z. J., and Yu, X. C. (2011). Overexpression in tobacco of a tomato GMPase gene improves tolerance to both low and high temperature stress by enhancing antioxidation capacity. Plant Cell Rep. 30, 1029–1040. doi: 10.1007/s00299-011-1009-y
Witte, C.-P. (2011). Urea metabolism in plants. Plant Sci. 180, 431–438. doi: 10.1016/j.plantsci.2010.11.010
Zhai, H., Wang, F., Si, Z., Huo, J., Xing, L., An, Y., et al. (2016). A myo-inositol-1-phosphate synthase gene, IbMIPS1, enhances salt and drought tolerance and stem nematode resistance in transgenic sweet potato. Plant Biotechnol. J. 14, 592–602. doi: 10.1111/pbi.12402
Zhao, S. Y., Zeng, W. H., Li, Z., and Peng, Y. (2020). Mannose regulates water balance, leaf senescence, and genes related to stress tolerance in white clover under osmotic stress. Biol. Plant. 64, 406–416. doi: 10.32615/bp.2020.017
Keywords: abiotic stress, metabolites, metabolic pathways, osmotic adjustment (OA), polyamine (PA), signal transduction
Citation: Li Z, Cheng B, Wu X, Zhang Y, Feng G and Peng Y (2022) Spermine-mediated metabolic homeostasis improves growth and stress tolerance in creeping bentgrass (Agrostis stolonifera) under water or high-temperature stress. Front. Plant Sci. 13:944358. doi: 10.3389/fpls.2022.944358
Received: 15 May 2022; Accepted: 13 July 2022;
Published: 11 August 2022.
Edited by:
Jane Ward, Rothamsted Research, United KingdomReviewed by:
Hafeez Ur Rehman, University of Agriculture, PakistanCopyright © 2022 Li, Cheng, Wu, Zhang, Feng and Peng. This is an open-access article distributed under the terms of the Creative Commons Attribution License (CC BY). The use, distribution or reproduction in other forums is permitted, provided the original author(s) and the copyright owner(s) are credited and that the original publication in this journal is cited, in accordance with accepted academic practice. No use, distribution or reproduction is permitted which does not comply with these terms.
*Correspondence: Yan Peng, cGVuZ3lhbmxlZUAxNjMuY29t
†These authors have contributed equally to this work
Disclaimer: All claims expressed in this article are solely those of the authors and do not necessarily represent those of their affiliated organizations, or those of the publisher, the editors and the reviewers. Any product that may be evaluated in this article or claim that may be made by its manufacturer is not guaranteed or endorsed by the publisher.
Research integrity at Frontiers
Learn more about the work of our research integrity team to safeguard the quality of each article we publish.