- 1Department of Crop Genetics, John Innes Centre, Norwich, United Kingdom
- 2Department of Plant and Crop Sciences, School of Biosciences, Nottingham BBSRC Wheat Research Centre, University of Nottingham, Loughborough, United Kingdom
A diverse panel of wheat wild relative species was screened for resistance to Fusarium head blight (FHB) by spray inoculation. The great majority of species and accessions were susceptible or highly susceptible to FHB. Accessions of Triticum timopheevii (P95-99.1-1), Agropyron desertorum (9439957), and Elymus vaillantianus (531552) were highly resistant to FHB while additional accessions of T. timopheevii were found to be susceptible to FHB. A combination of spray and point inoculation assessments over two consecutive seasons indicated that the resistance in accession P95-99.1-1 was due to enhanced resistance to initial infection of the fungus (type 1 resistance), and not to reduction in spread (type 2 resistance). A panel of wheat-T. timopheevii (accession P95-99.1-1) introgression lines was screened for FHB resistance over two consecutive seasons using spray inoculation. Most introgression lines were similar in susceptibility to FHB as the wheat recipient (Paragon) but substitution of the terminal portion of chromosome 3BS of wheat with a similar-sized portion of 3G of T. timopheevii significantly enhanced FHB resistance in the wheat background.
Introduction
Fusarium head blight (FHB) is a highly damaging disease of bread wheat (Triticum aestivum) and durum wheat (T. durum) in many parts of the world. Infection generally occurs during flowering when susceptibility to FHB is greatest in the host (Franco et al., 2021) leading to yield loss and reduced grain quality (Spanic et al., 2021). The disease is mainly caused by Fusarium graminearum sensu stricto but other species including Fusarium culmorum and Fusarium asiaticum can be important in some regions (Valverde-Bogantes et al., 2020). These species can produce trichothecene mycotoxins such as deoxynivalenol (DON) and nivalenol (NIV) that contaminate grain and pose a risk to human and animal consumers (Amarasinghe et al., 2019). It is widely accepted that the use of FHB-resistant varieties is the most effective and sustainable means to mitigate against losses caused by FHB (Bai et al., 2018). Breeding for resistance to FHB is particularly challenging because of the generally polygenic nature of resistance, high level of genotype-by-environment interactions and the high cost of phenotyping.
Resistance to FHB was originally differentiated into two classes: resistance to initial infection (type 1) and resistance to spread within the spike (type 2) (Schroeder and Christensen, 1963). Additional classes of resistance have been proposed including degradation of DON (Miller et al., 1985) and DON tolerance being grouped and termed type 3 resistance (Mesterhazy et al., 1999) and resistance to kernel infection as type 4 (Mesterhazy et al., 1999). Type 1 resistance is determined by spraying spikes at mid-anthesis with a conidial suspension and measuring the percentage of diseased spikes whereas type 2 resistance is determined by inoculating single florets with conidial inoculum and measuring the number or percentage of diseased spikelets over time.
Resistance to FHB is quantitatively inherited and over 100 quantitative trait loci (QTL) have been reported to date distributed across all 21 chromosomes of bread wheat (Buerstmayr et al., 2020). Many potent QTL have been identified in Asian germplasm including Sumai 3, Wangshuibai, Nobeokabouzu, and Nyu Bai (Buerstmayr et al., 2020). The most highly studied source of resistance is the Chinese variety Sumai 3 in which three QTL were originally identified on the short arms of chromosomes 3B and 5A and the long arm of chromosome 6B (Buerstmayr et al., 2020).
Most studies have focused on assessing type 2 resistance because it is more stable than type 1 resistance and less prone to influence by environmental factors. Simple Mendelian inheritance has been demonstrated for a number of QTL when isolated into susceptible wheat variety backgrounds (Cuthbert et al., 2006, 2007). Seven such QTL have been formally recognized as genes Fhb1-Fhb7 (Guo et al., 2015). The identity of Fhb1 (type 2 resistance) was originally identified as a chimeric lectin with agglutinin domains and a pore forming toxin domain (Rawat et al., 2016) but later studies have cast doubt on this with a second gene being proposed to be responsible. A histidine-rich calcium-binding protein was demonstrated to provide resistance to FHB by two groups although one group (Su et al., 2019) concluded that the resistance is due to the loss of function while the second group (Li et al., 2019) concluded that resistance was the result of a gain of function.
The search for additional sources of potent FHB resistance continues and extends beyond the primary gene-pool into wheat relatives. Resistance has been identified in a number of chromosome segments introgressed into wheat from wild relatives. In some instances, these resistances have been considered as genes due to the lack, or extremely limited degree, of recombination between the introgressed segment and wheat chromosome. Introgression of a portion of chromosome 3St of Elymus repens into 3D of wheat confers high levels of type 2 resistance (Fedak et al., 2017; Gong et al., 2019). Substitution of the short arm of chromosome 7AS of wheat with the short arm of Leymus racemosus 7Lr#1 provides a high level of type 2 resistance and this has been designated as Fhb3 (Qi et al., 2008). Similarly, replacement of the short arm of chromosome 1A (1AS) of wheat with the short arm of chromosome 1Ets#1S of Elymus tsukushiensis also significantly enhances type 2 resistance in wheat and has been designated as Fhb6 (Cainong et al., 2015). The most extensively studied resistance in a wheat relative derives from Thinopyrum elongatum (Th. ponticum) (Guo et al., 2015; Ceoloni et al., 2017). Substitution of the long arm of wheat chromosome 7D (7DL) with the long arm of 7El2 of Th. elongatum confers very high levels of type 2 resistance (Shen and Ohm, 2007; Zhang et al., 2011; Wang et al., 2020). The gene responsible for this resistance, termed Fhb7 (formerly Fhblop), has recently been isolated and shown to encode a glutathione S-transferase (GST) that functions through de-epoxidation of trichothecenes (Wang et al., 2020).
The objectives of the present study were to: (1) screen accessions of wheat relatives to identify FHB resistance, (2) determine whether resistance is predominantly of type 1 (resistance to initial infection) or type 2 (resistance to spread in the spike), (3) determine whether segments of chromosomes from resistant wheat relatives conferred FHB resistance when introgressed into wheat.
Materials and Methods
Fungal Materials
All Fusarium isolates used in this study originated from the United Kingdom and are kept as part of the JIC facultative pathogen collection. Isolates were maintained as reported previously (Hales et al., 2020).
Wheat Wild Relative Species Fusarium Head Blight Screen
A diverse panel of wheat wild relative accessions from Nottingham/BBSRC Wheat Research Centre [originally obtained from the Germplasm Resource Unit (GRU) at the JIC and the United States Department of Agriculture (USDA)] was screened for FHB resistance. Material was sown in the winter of 2011 and given natural vernalization in an unheated, unlit glasshouse. In the spring of 2012 seedlings were transplanted into 1 liter pots of cereals mix and grown in a Keder Greenhouse until mid-anthesis. Due to the diverse growth habits and morphology of the material it was spray inoculated repeatedly around the time of mid-anthesis to ensure that all the material received inoculum at the period that highly favors infection. The inoculum consisted of conidia (1 × 105 conidia ml–1) of a DON producing isolate of F. culmorum, applied using a handheld mister.
The spikes of some species contain very few spikelets (1 or 2) making it inappropriate to use a conventional scoring system based solely upon the percentage of spikelets with symptoms. Disease levels were assessed between 3 and 4 weeks post inoculation using a 1–9 rating based upon an index of percentage of spikes affected and percentage of spikelets showing disease in infected spikes. A visual disease score of 1 indicating “no visible disease,” a visual disease score of 9 indicating very high disease levels of 90–100% infected spikelets on all spikes at or near mid-anthesis at the time of inoculation. The 1–9 index rating allowed comparison among the species within the screen but is not suitable for making comparison with published reports on relative resistance in wheat.
Fusarium Head Blight Disease Assessment of T. timopheevii Accessions by Spray Inoculation (2015 and 2016)
Seven T. timopheevii accessions (all obtained from the USDA) and a susceptible wheat variety (Highbury – obtained from the GRU) were assessed for FHB resistance in 2015 by spray inoculation with conidia (1 × 105 ml–1) of a DON producing isolate of F. graminearum. Between 11 and 39 individual spikes per line from multiple plants were spray inoculated at mid-anthesis, and disease was assessed at 21 days post inoculation (dpi) and the percentage of infected spikelets per spike calculated.
Three T. timopheevii accessions, a wheat/Tim_P95-99.1-1 amphidiploid and the wheat variety Highbury (parent to the amphidiploid) were assessed for FHB resistance in 2016 by spray inoculation with conidia (1 × 105 ml–1) of a DON producing isolate of F. graminearum. The amphidiploid was produced by crossing Chinese Spring as the female parent with Triticum timopheevii as the male parent to generate a F1 hybrid or amphihaploid. This F1 hybrid seedling was treated with colchicine in order to produce the amphidiploid (Nemeth et al., 2015). The fertility of the wheat/T. timopheevii amphidiploid is very low and therefore 2016 was the first year that sufficient seed was available to screen for Fusarium resistance. The amphidiploid was not screened for a second season again due to the problem of seed multiplication.
The trial had a randomized block design containing six replicate blocks with 5–8 plants per line. Multiple ears per plant were spray inoculated at mid-anthesis, and disease was assessed at 19 dpi and the percentage of infected spikelets per spike calculated.
Fusarium Head Blight Disease Assessment of T. timopheevii Accessions by Point Inoculation (2015 and 2016)
Seven T. timopheevii accessions and a susceptible wheat variety (Highbury) were assessed for FHB resistance following point inoculation in 2015. Between 10 and 27 individual spikes per line from multiple plants were point inoculated at mid-anthesis. Inoculum (10 μl) of a DON producing F. graminearum isolate (1 × 106 conidia ml–1) was introduced directly into a central spikelet for each spike. Disease was assessed at 21 dpi and the number of infected spikelets above and below the point of inoculation recorded.
Three T. timopheevii accessions, a wheat/Tim_P95-99.1-1 amphidiploid and the wheat variety Highbury (parent to the amphidiploid) were assessed for FHB resistance following point inoculation in 2016. The trial had a randomized block design containing 6 replicate blocks with 5–8 plants for each line with multiple spikes inoculated for each plant. Individual spikes were point inoculated into the central floret at mid-anthesis with F. graminearum at 1 × 10–6 spore per ml, using a 0.5 ml insulin syringe.
Disease was assessed at 14 dpi and the number of infected spikelets above and below the point of inoculation recorded.
Statistical Analysis
All statistical analysis used Genstat 18th. The trials in 2015 were unblocked, and GLM analysis used “Inoculation date” and “Line” in the model. GLM was used to calculate predicted means and standard errors for percentage of infected spikelets (spray inoculation) and the number of infected spikelets above and below the point of inoculation (point inoculation for each line.
The GLM analysis of trials in 2016 used “Inoculation date,” “Replicate,” and “Line” in the model. GLM was used to calculate predicted means and standard errors for percentage of infected spikelets (spray inoculation) and the number of infected spikelets above and below the point of inoculation (point inoculation) for each line.
Development of Wheat/T. timopheevii (P95-99.1-1) Introgression Lines
Development of wheat/T. timopheevii introgression lines was as outlined in Devi et al. (2019) and King et al. (2022). Briefly, Paragon ph1/ph1 (obtained from the GRU) was used as the female parent in a cross with T. timopheevii P95-99.1-1 to generate F1 interspecific hybrids. The F1 hybrids were then backcrossed to Paragon to generate BC1, BC2, BC3 and BC4 plants. Molecular characterization of the introgression lines was initially carried out using the Axiom® Wheat-Relative Genotyping Array (Devi et al., 2019). When genotyping of these plants showed the number of introgressions present to be three or less, the plants were then self-fertilized. Chromosome-specific KASP markers, polymorphic between wheat and T. timopheevii have been developed at the WRC and 480 of these KASP markers have been used to characterize a panel of homozygous wheat/T. timopheevii introgression lines including those investigated in this work (King et al., 2022).
Fluorescence in situ Hybridization
Fluorescence in situ hybridization analysis of wheat-T. timopheevii introgression lines was carried out as described in King et al. (2022). Root metaphase spreads of chromosomes were hybridized with probes pSc119.2 (McIntyre et al., 1990) and pAs.1 (Rayburn and Gill, 1986) that were nick-labeled (Rigby et al., 1977) with Alexa Fluor 488-5-dUTP (green) and Alexa Fluor 594-5-dUTP (red), respectively. Karyotyping of labeled chromosomes was done in accordance with the nomenclature reported by Badaeva et al. (2016).
Fusarium Head Blight Disease Assessment of Wheat-T. timopheevii Introgression Lines by Spray Inoculation (2020 and 2021)
Twenty-five wheat-T. timopheevii introgression lines and wheat variety Paragon were assessed for FHB resistance by spray inoculation in a Keder Greenhouse in 2020 as described above. The trial had a randomized block design with between 4 and 6 individual plants distributed within 3 replicate blocks. Multiple spikes per plant were spray inoculated at mid-anthesis with conidia (1 × 105 ml–1) of a DON producing isolate of F. culmorum using a handheld mister.
Twenty-nine wheat-T. timopheevii introgression lines and FHB susceptible wheat varieties Highbury and Paragon were assessed for FHB resistance by spray inoculation in a Keder Greenhouse in 2021 as described above. The trial had a randomized block design with between 5 and 10 individual plants distributed within 4 replicate blocks. Spikes were inoculated at mid-anthesis as described above.
Disease in both trials was assessed at 21 dpi and the percentage of infected spikelets per ear calculated. All statistical analysis used Genstat 18th, GLM analysis used “Inoculation date,” “Replicate,” and “Line” in the model. Using GLM, predicted means and standard errors were calculated for each line.
Results
Fusarium Head Blight Screen of Wheat Relatives
The initial screen of 113 wheat wild relative accessions revealed that most accessions of all species were susceptible or highly susceptible to FHB (Table 1). Where more than one accession of a species was tested most showed similar levels of FHB susceptibility but evidence of variation in FHB susceptibility within species was observed for some species. One accession of Agropyron desertorum (PI 439957) was highly resistant to FHB (FHB score 2) while a second accession (PI 439953) was highly susceptible (FHB score 8). Similarly, accession 2060002 of Aegilops biuncialis was moderately resistant (FHB score 3) while accession 2060003 was highly susceptible (FHB score 9). The majority of accessions of Aegilops sharonensis were highly susceptible to FHB (FHB score 8–9) but a few (AS_01512//8, AS_01850//17, and AS_01930//19) were moderately resistant (FHB score 4–5). The single accession of Elymus vaillantianus (PI 531552) was highly resistant to FHB (FHB score 2) and exhibited few symptoms. The single accession of Triticum timopheevii (Tim_P95-99.1-1) was notable as it exhibited a very high level of resistance to FHB (Table 1) with no symptoms being apparent even at later dates post inoculation.
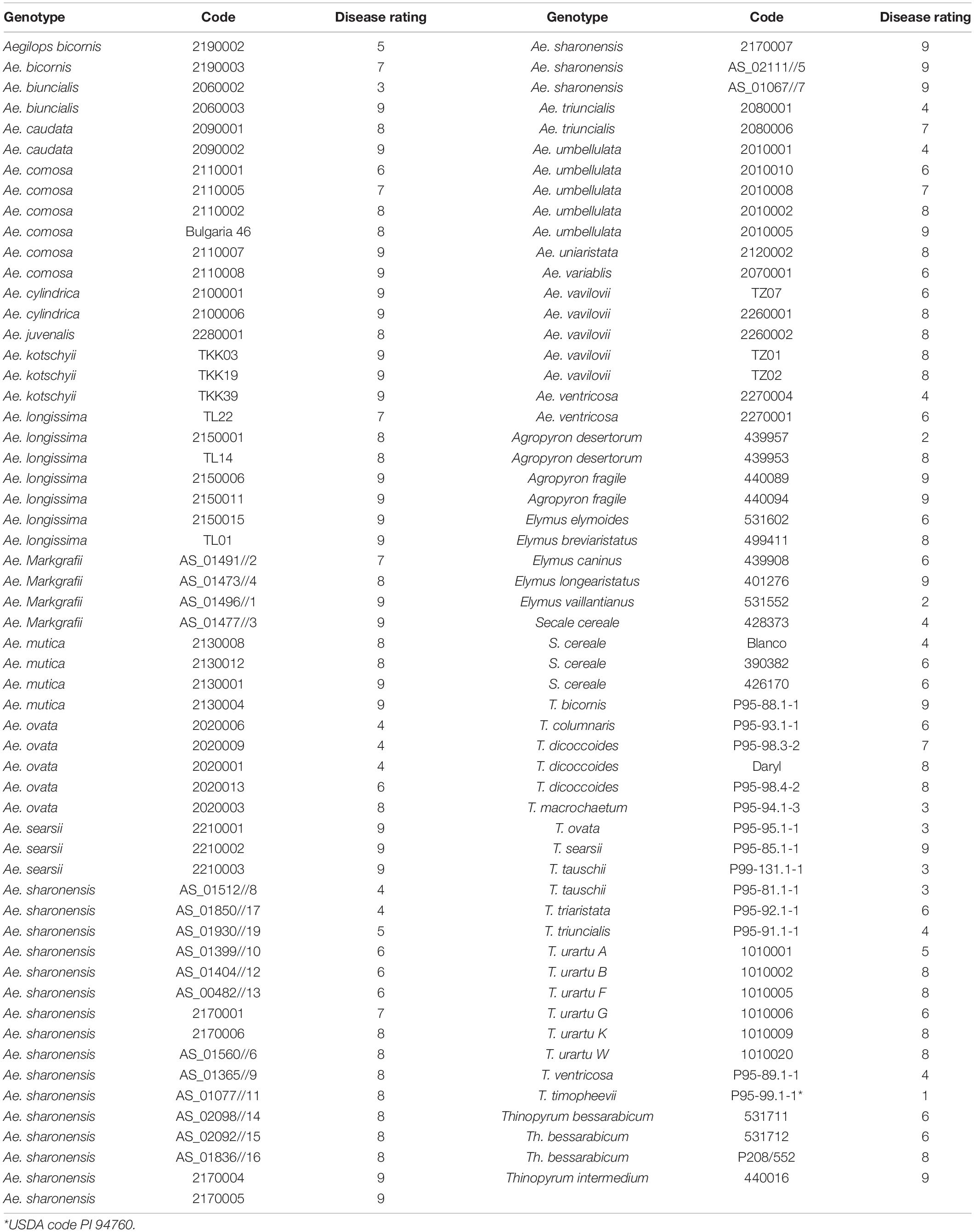
Table 1. Fusarium head blight disease rating of 113 wild grass species/accessions of wheat relatives following spray inoculation with conidia of F. graminearum.
Fusarium Head Blight Spray Inoculation Screening of Accessions of T. timopheevii
Given the variation in FHB resistance observed in many species in the initial screen, six additional accessions of T. timopheevii were obtained to compare their resistance with that of accession Tim_P95-99.1-1. Resistance to FHB is highly sensitive to environmental factors so Tim_P95-99.1-1 and selected additional accessions were tested across two seasons. Resistance derived from wheat relatives may not always be effective when introgressed into wheat (Innes and Kerber, 1994; Rines et al., 2007). An amphidiploid line (wheat/Tim-P95-99.1-1) was produced by crossing Tim_P95-99.1-1 to the FHB-susceptible wheat variety Highbury. The amphidiploid line was only available for FHB screening in 2016 due to restricted seed availability.
The FHB spray inoculation of six additional T. timopheevii accessions alongside Tim_P95-99.1-1 and the susceptible wheat variety Highbury was undertaken in 2015. At 21 dpi, over 84% of spikelets of Highbury were symptomatic for FHB. All accessions of T. timopheevii, with the exception of Tim_427998, were significantly more resistant (P < 0.001) than Highbury. Tim_P95-99.1-1 exhibited the greatest level of FHB resistance (29% spikelets infected) with a lower disease score than all the other T. timopheevii accessions tested. Indeed, the disease score for Tim_P95-99.1-1 was significantly lower (P < 0.001) than four of the T. timopheevii accessions and the wheat variety Highbury (Figure 1). Two other T. timopheevii accessions (Tim_PI_289752 and Tim_PI_427414) were also markedly less susceptible than Highbury with only 45 and 45.8% of spikelets infected, respectively.
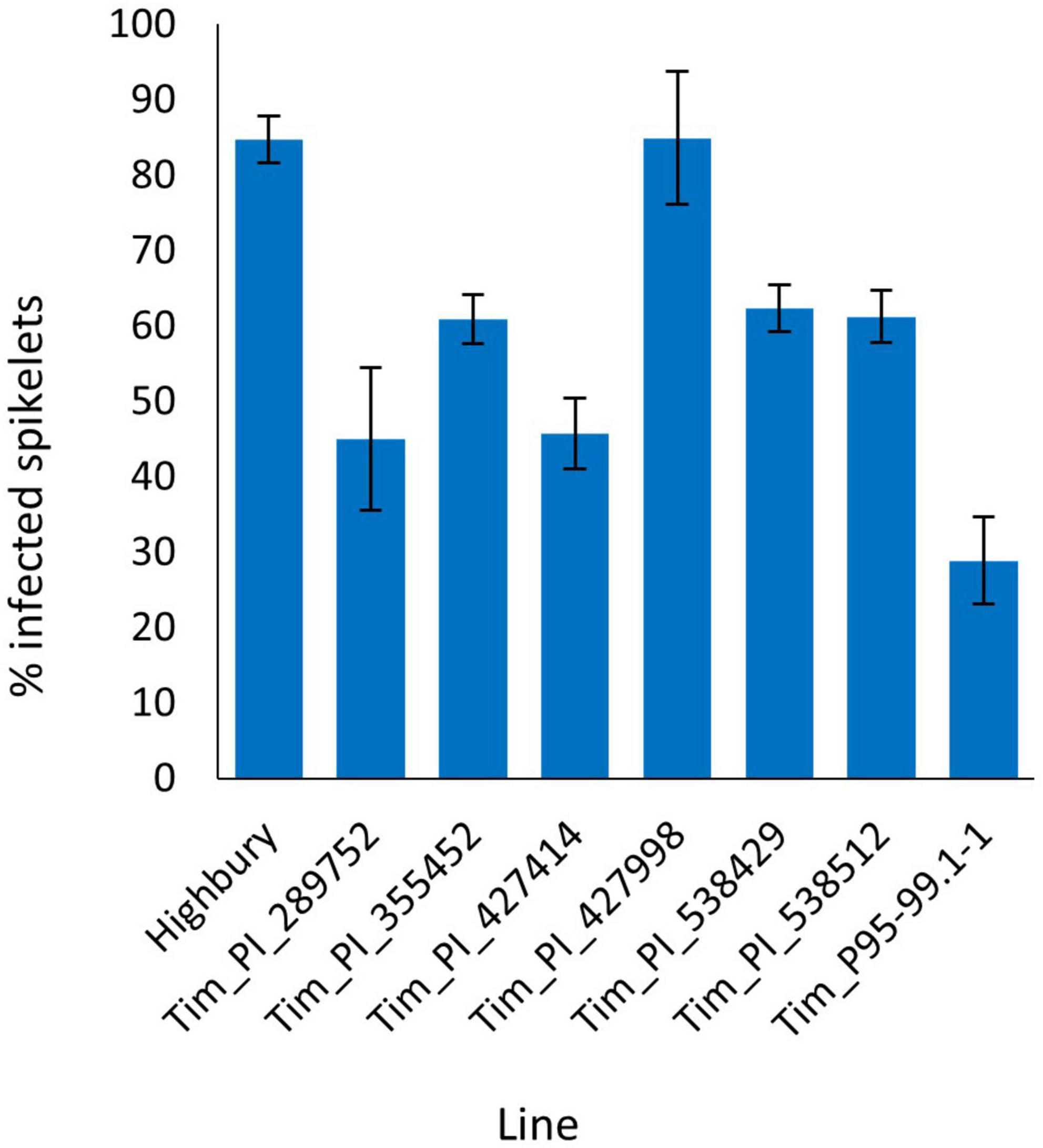
Figure 1. Visual Fusarium head blight (FHB) disease score from 2015 trial of seven T. timopheevii accessions and Highbury susceptible control expressed as percentage of infected spikelets 21 days post spray inoculation with F. graminearum (1 × 105 conidia per ml).
In the 2016 FHB spray screen disease levels were not as high as in 2015. Highbury had approximately 45% of spikelets exhibiting disease. In contrast, Tim_P95-99.1-1 had an extremely low level of disease with only 1.3% of spikelets exhibiting symptoms (Figure 2). As in 2015, accessions Tim_PI_538429 and Tim_PI_538512 were significantly less diseased (P < 0.001) than Highbury with 18.8 and 11% of spikelets infected. Disease levels in the amphidiploid (wheat/Tim-P95-99.1-1) were also very low with an average of 5% of spikelets infected and were not statistically different (P = 0.41) from those in the Tim_95-99.1-1 parent. These results reveal that the FHB resistance in Tim-P95-99.1-1 is stable and is expressed in the amphidiploid. Additional testing is required to confirm the latter.
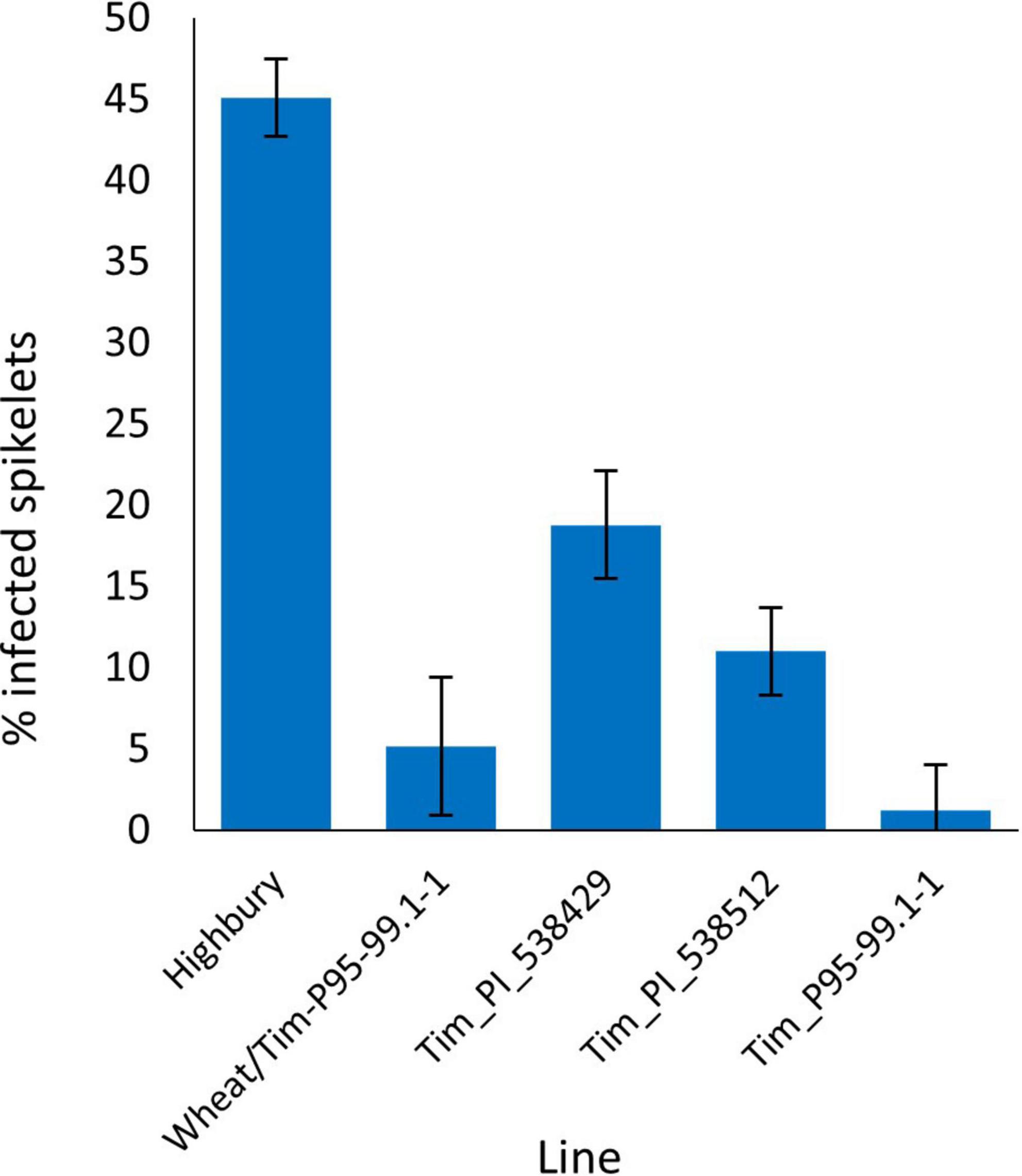
Figure 2. Visual FHB disease score from 2016 trial of three T. timopheevii accessions, T. timopheevii × Highbury amphidiploid (wheat/Tim-P95-99.1-1) and wheat variety Highbury expressed as percentage of infected spikelets 14 days post spray inoculation with F. graminearum (1 × 105 conidia per ml).
Fusarium Head Blight Point Inoculation Screening of Accessions of T. timopheevii
The preliminary FHB screen involved spray inoculation which reveals overall levels of FHB resistance. Point inoculation is used to determine whether the resistance is of type 2, resistance to spread. Tim_P95-99.1-1 along with six other T. timopheevii accessions and the wheat variety Highbury were point inoculated at mid-anthesis. Symptoms above the point of inoculation generally reflect susceptibility to the effects of DON while those below reflect colonization by the pathogen. For these reasons, disease above and below the point of inoculation was assessed separately. At 21 dpi disease levels in Highbury were 18 and 32.7% infected spikelets above and below the point of inoculation, respectively (Figure 3). Four accessions of T. timopheevii (Tim_PI_289752, Tim_PI_427414, Tim_PI_ 538429, and Tim_PI_538512) were significantly more susceptible to FHB than Highbury for symptoms both below and above the point of inoculation. None of the T. timopheevii accessions exhibited greater resistance to spread of symptoms either above or below the inoculation point than Highbury. Tim_538429 was extremely susceptible to spread of symptoms both above and below the inoculation point indicating an inability to restrict fungal colonization and a high level of susceptibility to the effects of DON (Figure 3). Accessions Tim_PI_289752 and Tim_PI_427414 exhibited high disease levels below the point of inoculation indicating that they lack the ability to restrict fungal colonization. Disease above and below the point of inoculation in the other accessions, including Tim_P95.99.1-1, were slightly, but not significantly, more susceptible than the wheat variety Highbury. Overall, no evidence was apparent to indicate that any of the T. timopheevii accessions possessed greater levels of type 2 resistance than Highbury.
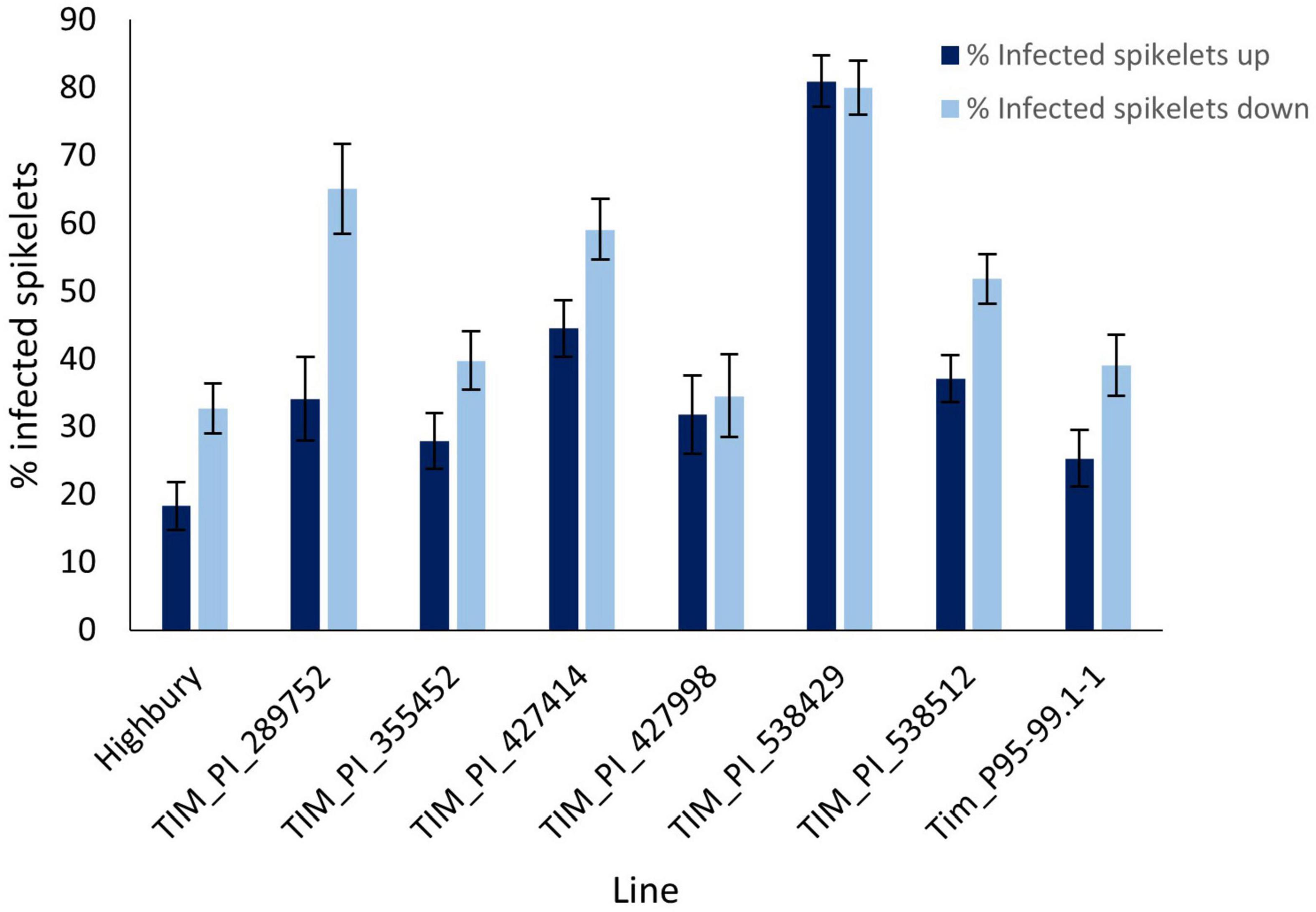
Figure 3. Visual FHB disease score from 2015 trial of seven T. timopheevii accessions and Highbury susceptible control expressed as number of infected spikelets above and below the point of inoculation, 21 days post inoculation with F. graminearum (1 × 106 conidia per ml).
Disease progress in the point inoculation in 2016 was greater than in the previous year with disease levels in Highbury of 75.5 and 68.2% above and below the inoculation point, respectively (Figure 4). As in the 2015 screen, disease symptoms above and below the inoculation point in Tim_PI_538429 were significantly greater (P = 0.028 and P = 0.05, respectively) than those in Highbury although the differential was markedly less than that in the earlier screen where disease pressure was lower (compare Figures 3, 4). Unlike in the earlier trial, disease levels above and below the inoculation point in Tim_PI_538512 were not significantly greater than those in Highbury with disease level above the point of inoculation being significantly less (P < 0.001) than that of Highbury (Figure 4).
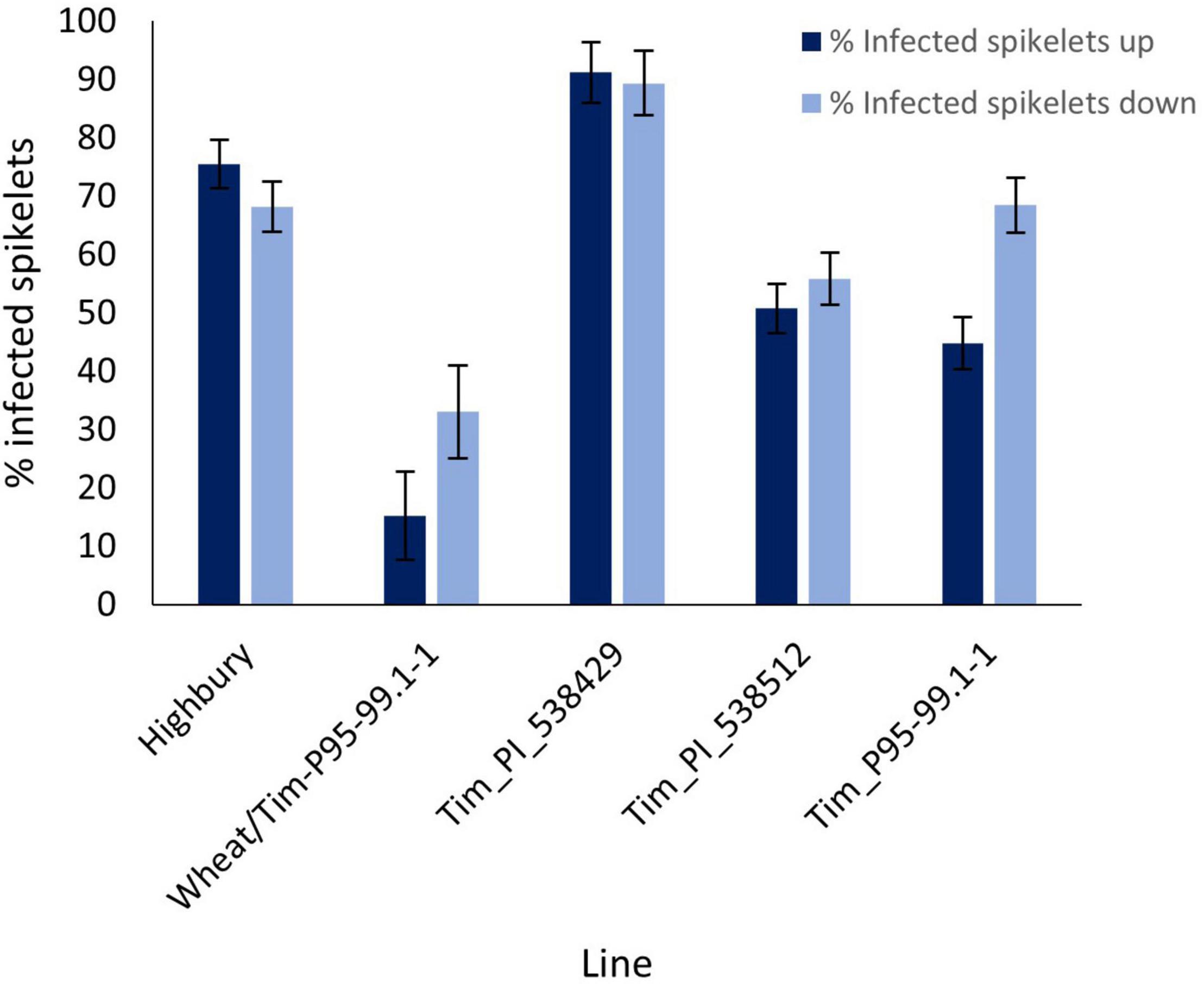
Figure 4. Visual FHB disease score from 2016 trial of three T. timopheevii accessions, T. timopheevii × Highbury amphidiploid (wheat/Tim-P95-99.1-1) and wheat variety Highbury expressed as number of infected spikelets above and below the point of inoculation, 14 days post inoculation with F. graminearum (1 × 106 conidia per ml).
Symptoms below the inoculation point in Tim_P95-99.1-1 were similar to those in Highbury (P = 0.96) while bleaching symptoms above the point of inoculation were significantly less than those in Highbury (P < 0.001). Despite this, the differential in disease levels between Tim_P95-99.1-1 and Highbury were very much less following point inoculation than after spray inoculation (compare Figures 2, 4). Unexpectedly, disease levels above (15.2%) and below (33.1%) the point of inoculation were significantly less (P < 0.001) in the amphidiploid line (wheat/Tim-P95-99.1-1) than either parent. It was noted that some spikes of the amphidiploid were partially sterile, and this may have reduced susceptibility in this line. The sterility issues with the amphidiploid make inference of resistance problematic as sterility can affect resistance to FHB (Wang et al., 2015).
Fusarium Head Blight Screening of Wheat Lines Carrying Introgressions From Tim_P95-99.1-1
The above studies revealed that accession Tim_P95-99.1-1 possesses a very high level of resistance to FHB and that this resistance is predominantly of type 1 rather than type 2. The studies also demonstrated that the FHB resistance in Tim_P95-99.1-1 was expressed in an amphidiploid line (wheat/Tim-P95-99.1-1) produced by crossing to the wheat variety Highbury. We next investigated whether introgression of Tim_P95-99.1-1 chromosome segments into wheat would confer any level of increased resistance to FHB. The development of a panel of introgressions of Tim_P95-99.1-1 into the wheat variety Paragon has been reported previously (Devi et al., 2019). These lines were advanced and a selection of 32 homozygous introgression lines from this panel (King et al., 2022; Table 2) were screened for FHB resistance by spray inoculation. Twenty-five of these introgression lines were tested in 2020 and 29 lines in 2021. Each line was subjected to genotyping using chromosome-specific KASP markers (King et al., 2022). These introgression lines contain a variety of segments from each of the two subgenomes present in T. timopheevii (At and G) (Table 2).
In 2020, disease levels on Paragon wheat were moderate with 47.5% spikelets exhibiting FHB symptoms (Figure 5). Significant differences were observed in the levels of FHB resistance among the introgression lines. Two introgression lines (Tim7 and Tim12) exhibited significantly higher disease levels than the Paragon recipient, 73.5 and 88.4%, respectively. Two introgression lines, Tim6 and Tim5 appeared highly resistant to FHB with disease levels of 4 and 10%, respectively and were significantly more resistant than Paragon (P < 0.001) (Figure 5). These two lines were of interest as although they both contained introgressed segments from linkage groups 2G and 3G of T. timopheevii, they were unique in the set tested as containing segments from 3G (Table 2). Three other introgression lines (Tim26, Tim28, and Tim11) were also significantly more resistant than Paragon, but to a lesser extent, with 22.9, 23.2, and 26.9% spikelets infected, respectively.
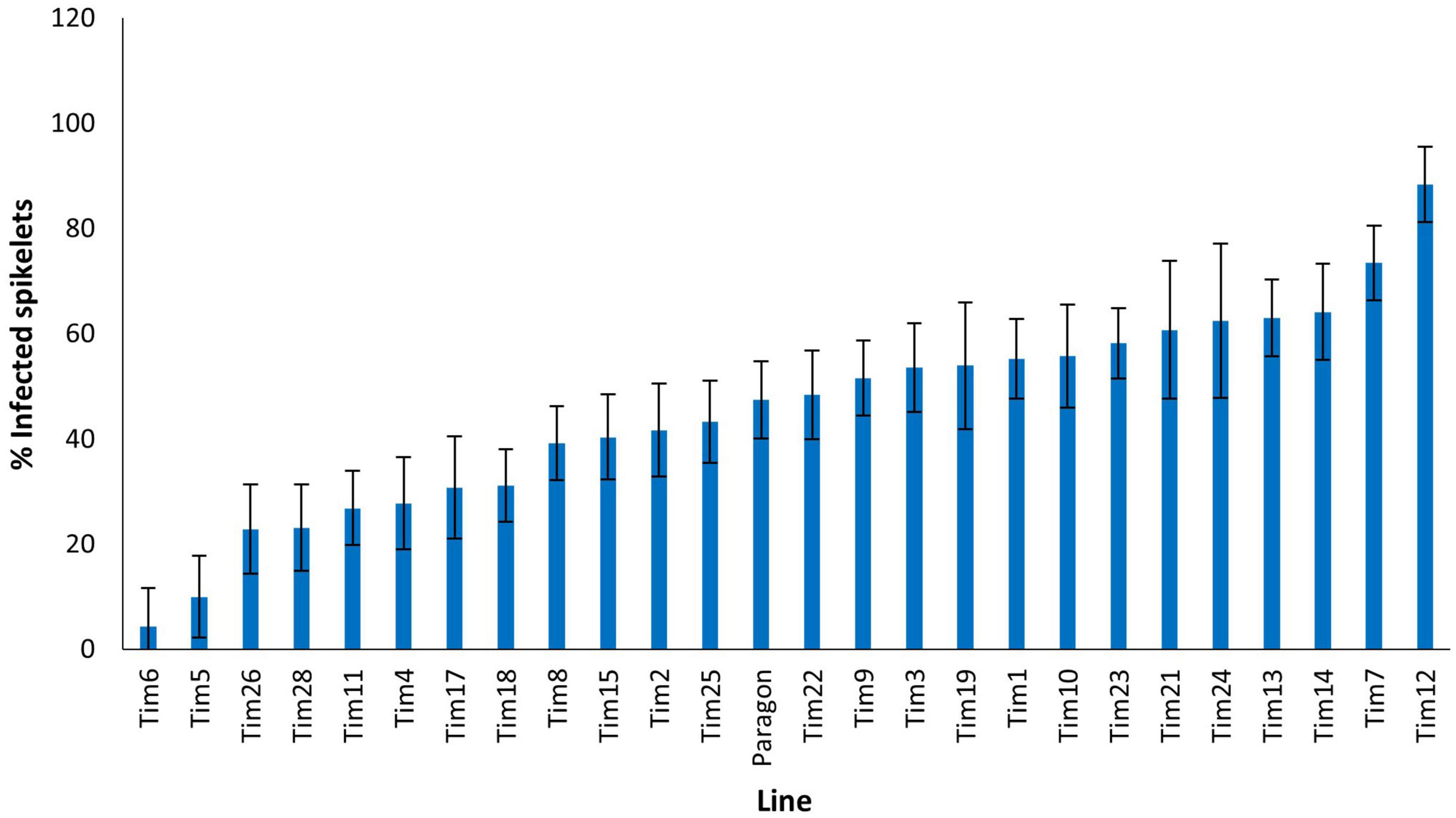
Figure 5. Visual FHB disease score from 2020 trial of 25 T. timopheevii accession P95-99.1-1/wheat introgression lines and Paragon susceptible control, expressed as percentage of infected spikelets 21 days post spray inoculation with F. culmorum (1 × 105 conidia per ml).
Disease levels were higher in the 2021 screen with 58.6% of spikelets of Paragon exhibiting FHB symptoms (Figure 6). No introgression line appeared more susceptible to FHB than Paragon. Four introgression lines Tim11, (22.4%) BC2F4-40 (23.6%), Tim33 (24.6%), and Tim5 (25.5%) were significantly (P < 0.001) more resistant to FHB than Paragon. Both Tim11 and Tim5 had also shown significantly greater resistance to FHB in the screen in the previous year. Neither BC2F4-40 or Tim33 had been included in the screen in the previous year. Two additional introgression lines (Tim29 and Tim30) also exhibited significantly less disease than Paragon but significantly more than the above-mentioned four lines.
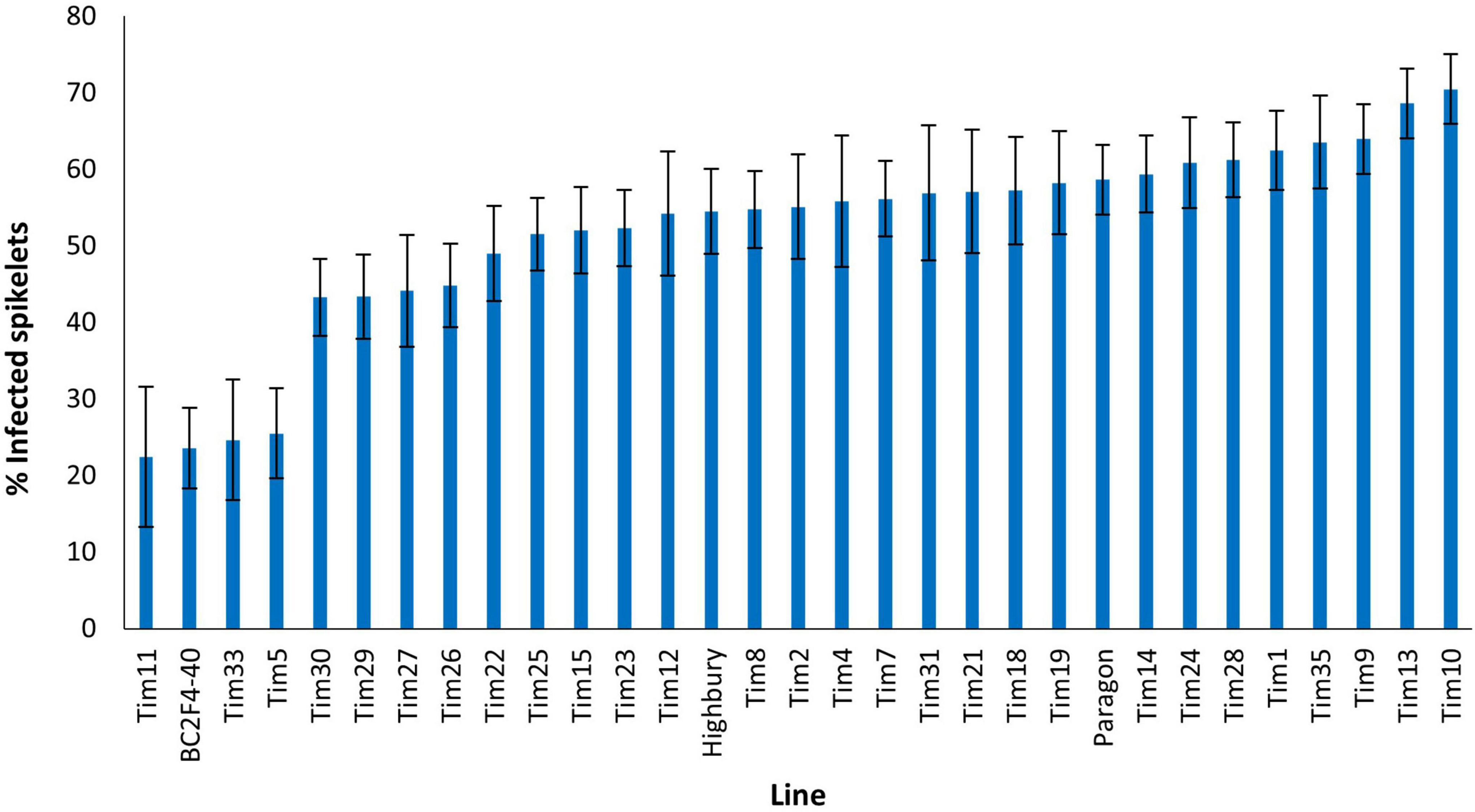
Figure 6. Visual FHB disease score from 2021 trial of 29 T. timopheevii accession P95-99.1-1/wheat introgression lines, Highbury and Paragon susceptible controls expressed as percentage of infected spikelets 21 days post spray inoculation with F. culmorum (1 × 105 conidia per ml).
Discussion
Several sources of potent FHB resistance have been identified in Triticum aestivum, particularly in lines from China and Japan (Buerstmayr et al., 2009). Subsequent study revealed that, in many cases, the genetic basis of resistance was similar and so research continues to expand the range of FHB resistance available to wheat breeders. Potentially useful resistance to FHB has been identified in both the secondary and tertiary gene pools (Steed et al., 2005; Ceoloni et al., 2017). In the present work, accessions of three species exhibited very high levels of FHB resistance. While resistance in Agropyron desertorum appears not to have been reported previously FHB resistance has been identified in both E. vaillantianus (syn. E. repens) (Fedak et al., 2017; Gong et al., 2019) and T. timopheevii (Malihipour et al., 2016). In the present study only one accession of T. timopheevii (P95-99.1-1) exhibited resistance to FHB while the other accessions were moderately to highly susceptible. Triticum timopheevii is an allopolyploid (2n = 4x = 28) comprising the At genome similar to that of the A genome progenitor of wheat (Triticum aestivum) and the G genome being more similar to the B genome of T. aestivum (Dvorak and Zhang, 1990; Dvorak et al., 1993; Devi et al., 2019). Recombination between the A and At genomes is more frequent than that between the B and G genomes reflecting their relative relatedness (Feldman, 1966; Devi et al., 2019).
Resistance identified in T. timopheevii to several pathogens has been introduced into wheat. Genes for resistance to stem rust include Sr36, Sr40, and Sr50 on chromosome 2B (Brown-Guedira et al., 2003) and Sr37 on 4B (Bai et al., 1998). Resistance to leaf rust has been introduced on 5B (Lr18) (Sadeghabad et al., 2017) and 5A (un-named resistance) (Bai et al., 1998). Resistance conditioned by both leaf rust genes was recessive in bread wheat while the 5A resistance was dominant in durum wheat indicating that the expression of resistance is dependent upon the background (Bai et al., 1998). Resistance to powdery mildew in T. timopheevii has also been introduced into wheat and used in breeding. The gene Pm6 derived from chromosome 2G and carried on chromosome 2B in wheat was reported in 1973 (Jorgensen and Jensen, 1973) and has proved durable to date (Wan et al., 2020). Resistance to diseases including spot blotch, tan spot, Stagonospora nodorum blotch, Septoria tritici blotch and loose smut has also been identified in T. timopheevii (Singh et al., 2006; Timonova et al., 2013) along with resistance to green bug (Rumyantsev et al., 2019) revealing it to be a rich source of resistance to a wide range of biotic stresses.
Resistance to FHB has been identified in a number of accessions of T. timopheevii. One of three accessions exhibited moderate type 2 resistance while all three lacked resistance to initial infection (type1) (Yong-Fang et al., 1997). FHB resistance derived from T. timopheevii has also been characterized in two separate studies. T. timopheevii accession PI 343447 was crossed and backcrossed to spring wheat Crocus and line TC 67 was selected on the basis of resistance to FHB and agronomic characteristics (Cao et al., 2009). TC 67 was crossed to the FHB susceptible variety Brio and a population of 230 F7 recombinant inbred lines produced and characterized for FHB resistance in glasshouse (type 2) and field trials [incidence (type 1) and severity (type 2)] (Malihipour et al., 2017). Two QTL were identified on the long arm of chromosome 5A with one near the centromere in the interval between markers cfd6.1 and barc48 and the second more distal between cfd39 and cfa2185. Both QTL contributed more than one FHB resistance trait. The QTL in the interval between cfd6.1 and barc48 was associated with reduced disease incidence and severity and reduced Fusarium damaged kernels (FDK) in field trials. The more potent QTL between cfd39 and cfa2185 was associated with reduced FDK in field trials and reduced severity in glasshouse trials following point inoculation indicating that it conferred type 2 resistance (Malihipour et al., 2017). Resistance to FHB derived from T. timopheevii was identified in a separate population developed between wheat line PI 277012 that contained T. timopheevii in its pedigree and wheat variety Grandin (PI 531005) (Chu et al., 2011). Two QTL contributing resistance to FHB in field trials and glasshouse point inoculation trials were identified on chromosome 5A with one on the short arm and one on the long arm. The QTL Qfhb.rwg-5A.1 on the short arm resides in a similar location to Qfhs.ifa-5A identified in Sumai 3 with both being in the region of marker XBarc180 (Chu et al., 2011). The QTL (Qfhb.rwg-5A.2) explaining most of the phenotypic variance flanks the QTL interval cfd39 and cfa2185 identified in TC 67 derived from T. timopheevii PI 343447 making it highly likely that these represent the same resistance (Chu et al., 2011). In both cases the QTL also flanked the Q locus with FHB resistance being associated with the q allele that prevents free-threshing reinforcing the view that the two QTL have a similar basis (Chu et al., 2011; Malihipour et al., 2016). It is unlikely that the differential FHB resistance is due to the Q locus itself because recombination between FHB resistance and non- free threshing was observed (Chu et al., 2011). The presence of the FHB resistance QTL on 5A indicates that they probably derive from chromosome 5At of T. timopheevii.
The FHB resistance of accession P95-99.1-1 appears to be predominantly of type 1 (resistance to initial infection) rather than type 2 (resistance to spread) as the greater resistance in this line compared to the other accessions tested was only evident following spray inoculation. This characteristic differentiates the resistance from that reported previously from T. timopheevii (Chu et al., 2011; Malihipour et al., 2016). Resistance to FHB in wheat has been shown to be effective toward both F. graminearum and F. culmorum (Van Eeuwijk et al., 1995). The resistance of T. timopheevii accession P95-99.1-1 also appears to be effective against both species.
A large panel of interspecific hybrid lines has been developed of introgressions from T. timopheevii accession P95-99.1-1 in spring wheat Paragon. Advanced back-cross lines were further back-crossed with Paragon and self-fertilized to produce lines containing a relatively small number of introgressions (King et al., 2022). The number of introgressions retained within each line reduced with each back-cross with the exception of part of chromosome 2G (Devi et al., 2019). It has been demonstrated that chromosome 2G of T. timopheevii is preferentially transmitted accounting for its retention in lines over numerous back-crossings (Brown-Guedira et al., 1996). Assessment of FHB resistance requires relatively large numbers of plants and these need to be fixed for the presence of the introgression(s). Sufficient grain was available for only a proportion of the introgression panel and 32 of these carrying 57 unique introgressions from T. timopheevii (Figures 5, 6) were assessed for resistance to FHB following spray inoculation.
Four introgression lines (Tim3, Tim4, Tim10, and Tim27) carry segments from 5At of T. timopheevii that are believed to cover the region associated with the potent FHB resistance QTL reported previously (Chu et al., 2011; Malihipour et al., 2017). In addition, the introgression carried by line Tim4 appears to cover the region containing the less potent resistance. None of these lines exhibited significantly greater FHB resistance than the wheat donor and it is concluded that accession P95-99.1-1 does not contain either of these FHB QTL.
Two lines (Tim5 and Tim6) were highly FHB resistant in the first year of testing. Both lines contain the preferentially transmitted segment of 2G and a segment of 3G equivalent to 3BS in wheat as revealed by KASP (Figure 7) and FISH (Figure 8) analysis. As many lines also contained the 2G segment but did not exhibit increased FHB resistance it was assumed that the resistance was conferred by the 3G segment. This was confirmed in the second year of trials. Line BC2F4-40 contains a 3G segment similar in size to that in Tim5 but lacks the preferentially transmitted segment of 2G. This line exhibited a similar high level of FHB resistance to Tim5 in the second year of trials. The size of the 3G segment in Tim5 and line BC2F4-40 is much smaller than that in Tim6, 48.8 and 762.2 Mb, respectively. Tim2, Tim24, and Tim35 all contain small segments (up to 10.75 Mb) of 3G introgressed onto the distal end of 3B but none of these lines showed enhanced FHB resistance in either trial (Figures 5, 6). It is assumed, therefore, that the region associated with FHB resistance on the distal portion of 3BS is contributed by the equivalent region of 3G in the interstitial 38.05 Mb region between 10.75 and 48.8 Mb.
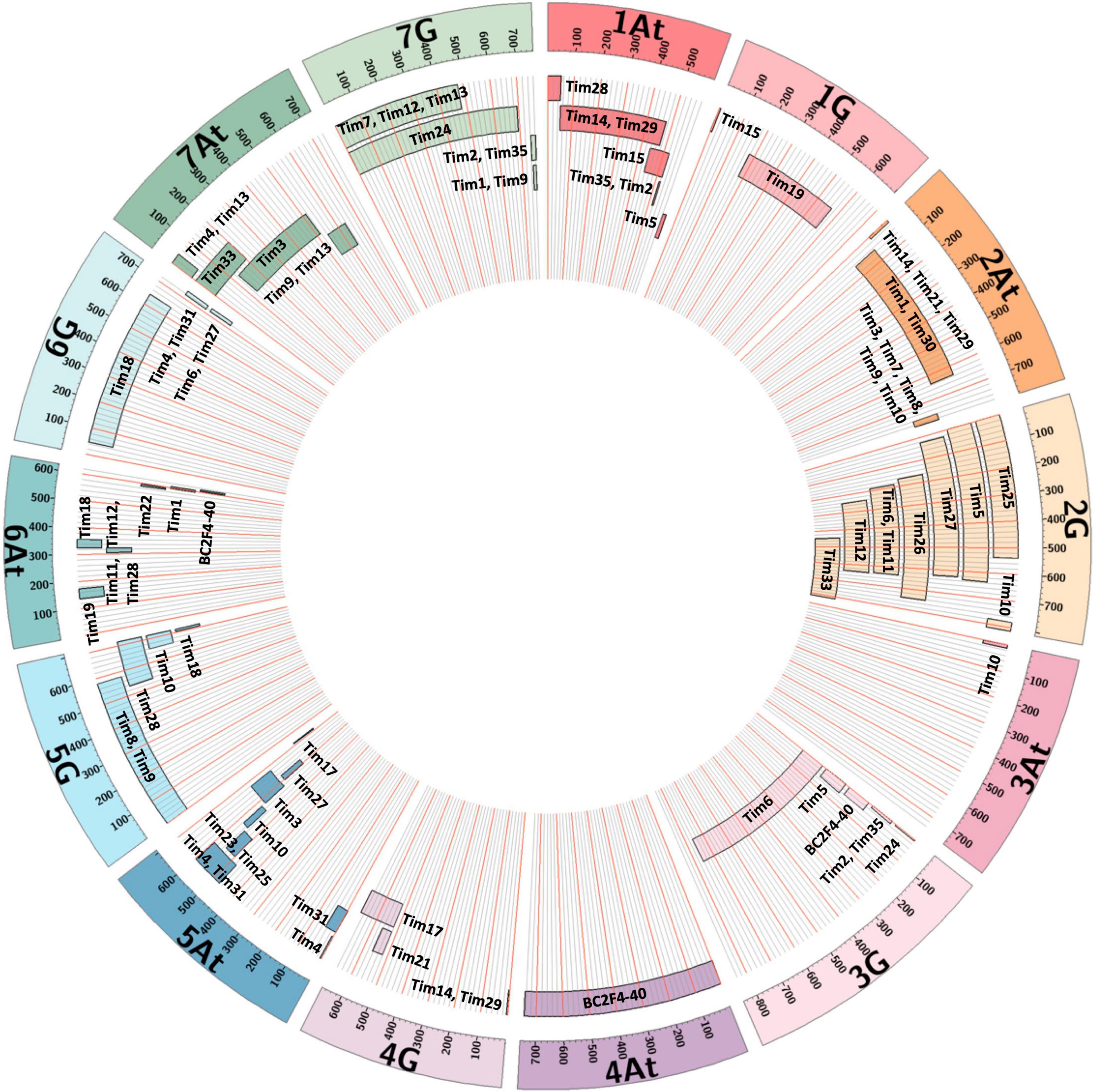
Figure 7. Representation of T. timopheevii chromosome segments contained within each Tim introgression line showing approximate position and size of each segment relative to each T. timopheevii chromosome.
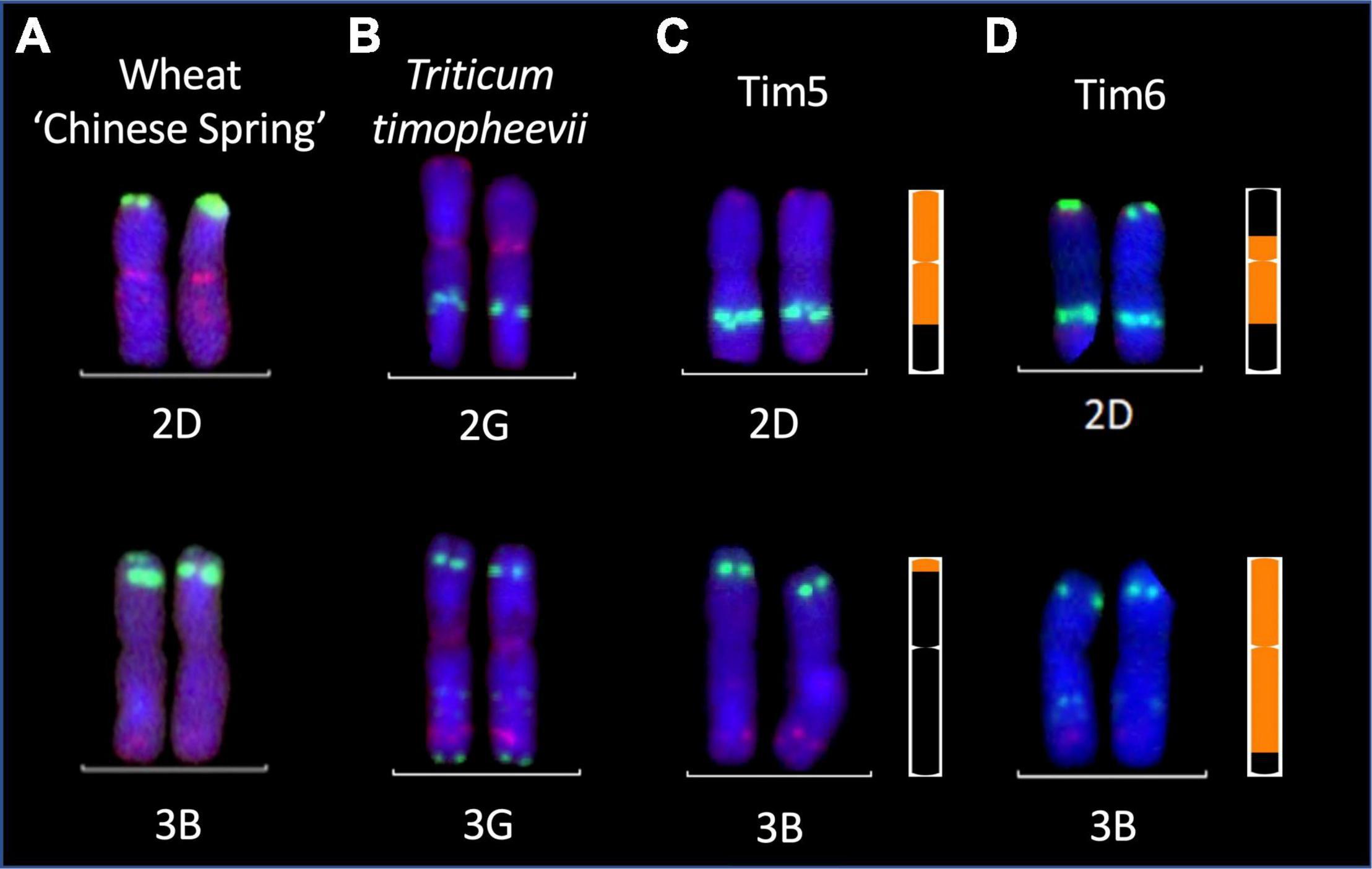
Figure 8. Multi-color fluorescence in situ hybridization analysis of root metaphase spreads of 2D and 3B chromosomes from (A) wheat cv. Chinese Spring (B) chromosomes 2G and 3G of T. timopheevii and chromosomes 2D and 3B of FHB resistant wheat-T. timopheevii introgression lines (C) Tim 5 and (D) Tim6. Green and red signals show pSc119.2 and pAs.1 binding sites, respectively. KASP marker-derived ideograms of the introgressions in Tim 5 and Tim 6 are shown to the right. Size of introgressions from At and G subgenomes is indicated in orange in a wheat chromosome shown in black.
Line Tim2 used in the FHB trial in 2020 contained segments of chromosomes 1At and 7G from T. timopheevii and segregated for loss of the short arm of chromosome 3B (3BS). The loss of 3BS was fixed in this line in the FHB trial in 2021. It has been postulated that the resistance conferred by Fhb1 on chromosome 3BS is due to a loss of function (Su et al., 2019) of the histidine rich calcium binding protein. No significant increase in FHB resistance in Tim2 was observed in either year indicating that loss of 3BS does not result in increased FHB resistance. This observation is in agreement with the finding that loss of 3BS (Ma et al., 2006) or replacement of 3BS with 3HS (Hales et al., 2020) does not result in an increase in FHB resistance.
Two other lines showed enhanced FHB resistance in the 2021 trial. Tim11 was moderately resistant in the first year of trials and was one of the most resistant in the second trial when disease pressure was higher (Figures 5, 6). This line contains segments from 2G and 6At. The 6A segment is similar to that in a number of other lines and the 2G segment is present in Tim5. None of the lines containing either the 2G and 6At segments showed enhanced resistance and so the origin of this resistance is unclear. It is possible that this line contains additional segments of T. timopheevii chromosome that are too small to detect with either the SNP markers or with FISH. This is particularly relevant for introgressions from the At genome. Recombination between the A genome of wheat and the At genome of T. timopheevii is much more prevalent than that between the B or D genome of wheat and the G genome of T. timopheevii (Timonova et al., 2013). The size of At introgressions may be reduced during the process of backcrossing to reduce and stabilize the number of introgressions and so their presence may not be detected using the current SNP marker set.
Insufficient grain of Tim 33 was available for FHB resistance assessment in 2020 but this line exhibited a high level of resistance in the 2021 trial (Figure 6). Tim33 contains the preferentially transmitted segment of 2G and a segment on 7AS presumed to originate from 7At of T. timopheevii. The segment on 7AS of Tim33 is in the region between 0 to ∼200 Mb. Two other lines (Tim4 and Tim13) also possess segments introduced to 7AS but the size of the introgression (0–∼42 Mb) is considerably less than that in Tim33. In addition, Tim3 contains a segment introduced in the region 128–515 Mb on 7A. None of these lines exhibited high levels of FHB resistance and so it is concluded that the resistance exhibited by Tim33 is due to genes present in the region between ∼42 and 128 Mb. Additional testing of these lines is required to confirm the presence and location of the FHB resistance conferred by this interval of 7At.
Triticum timopheevii is a rich source of diversity for the introduction of beneficial traits from the secondary gene pool of wheat. We have identified an accession of T. timopheevii with very high levels of type 1 FHB resistance. The resistance appears to be novel and is expressed when introduced into wheat. Introgression of individual chromosomal segments (e.g., 38.05 Mb region between 10.75 and 48.8 Mb of 3G) significantly increase FHB resistance in wheat. The material generated within this study provides a new source of FHB resistance for evaluation in wheat breeding programs.
Data Availability Statement
The raw data supporting the conclusions of this article will be made available by the authors, without undue reservation, to any qualified researcher.
Author Contributions
IK, JK, and PN conceived this work. JK, C-yY, and SG developed the introgression lines reported here. SG carried out the genotyping. C-yY carried out the FISH analysis of introgression lines. AS, MC, UD, and PN carried out the disease screening of plants. AS analyzed the data produced. PN, AS, IK, JK, C-yY, and SG contributed to writing this manuscript. All authors contributed to the article and approved the submitted version.
Funding
This work was supported by the United Kingdom Biotechnology and Biological Sciences Research Council (BBSRC) through the Designing Future Wheat [BB/P016855/1] Institute Strategic Programme.
Conflict of Interest
The authors declare that the research was conducted in the absence of any commercial or financial relationships that could be construed as a potential conflict of interest.
Publisher’s Note
All claims expressed in this article are solely those of the authors and do not necessarily represent those of their affiliated organizations, or those of the publisher, the editors and the reviewers. Any product that may be evaluated in this article, or claim that may be made by its manufacturer, is not guaranteed or endorsed by the publisher.
Acknowledgments
AS and PN thank the Horticultural Services team of the John Innes Centre for their input into care of the plants used in these experiments.
References
Amarasinghe, C., Sharanowski, B., and Fernando, W. G. D. (2019). Molecular Phylogenetic Relationships, Trichothecene Chemotype Diversity and Aggressiveness of Strains in a Global Collection of Fusarium graminearum Species. Toxins 11:263. doi: 10.3390/toxins11050263
Badaeva, E. D., Ruban, A. S., Zoshchuk, S. A., Surzhikov, S. A., Knuepffer, H., and Kilian, B. (2016). Molecular cytogenetic characterization of Triticum timopheevii chromosomes provides new insight on genome evolution of T. zhukovskyi. Plant Syst. Evol. 302, 943–956. doi: 10.1007/s00606-016-1309-3
Bai, D. P., Knott, D. R., and Zale, J. (1998). The transfer of leaf rust resistance from Triticum timopheevii to durum and bread wheat and the location of one gene ore chromosome 1A. Can. J. Plant Sci. 78, 683–687.
Bai, G., Su, Z., and Cai, J. (2018). Wheat resistance to Fusarium head blight. Can. J. Plant Sci. 40, 336–346. doi: 10.1080/07060661.2018.1476411
Brown-Guedira, G. L., Badaeva, E. D., Gill, B. S., and Cox, T. S. (1996). Chromosome substitutions of Triticum timopheevii in common wheat and some observations on the evolution of polyploid wheat species. Theor. Appl. Genet. 93, 1291–1298.
Brown-Guedira, G. L., Singh, S., and Fritz, A. K. (2003). Performance and mapping of leaf rust resistance transferred to wheat from Triticum timopheevii subsp armeniacum. Phytopathology 93, 784–789. doi: 10.1094/phyto.2003.93.7.784
Buerstmayr, H., Ban, T., and Anderson, J. A. (2009). QTL mapping and marker-assisted selection for Fusarium head blight resistance in wheat: a review. Plant Breed. 128, 1–26. doi: 10.1111/j.1439-0523.2008.01550.x
Buerstmayr, M., Steiner, B., and Buerstmayr, H. (2020). Breeding for Fusarium head blight resistance in wheat-Progress and challenges. Plant Breed. 139, 429–454. doi: 10.1111/pbr.12797
Cainong, J. C., Bockus, W. W., Feng, Y., Chen, P., Qi, L., Sehgal, S. K., et al. (2015). Chromosome engineering, mapping, and transferring of resistance to Fusarium head blight disease from Elymus tsukushiensis into wheat. Theor. Appl. Genet. 128, 1019–1027. doi: 10.1007/s00122-015-2485-1
Cao, W. G., Fedak, G., Armstrong, K., Xue, A., and Savard, M. E. (2009). Registration of Spring Wheat Germplasm TC 67 Resistant to Fusarium Head Blight. J. Plant Regist. 3, 104–106. doi: 10.3198/jpr2008.08.0465crg
Ceoloni, C., Forte, P., Kuzmanovic, L., Tundo, S., Moscetti, I., De Vita, P., et al. (2017). Cytogenetic mapping of a major locus for resistance to Fusarium head blight and crown rot of wheat on Thinopyrum elongatum 7EL and its pyramiding with valuable genes from a Th. ponticum homoeologous arm onto bread wheat 7DL. Theor. Appl. Genet. 130, 2005–2024. doi: 10.1007/s00122-017-2939-8
Chu, C., Niu, Z., Zhong, S., Chao, S., Friesen, T. L., Halley, S., et al. (2011). Identification and molecular mapping of two QTLs with major effects for resistance to Fusarium head blight in wheat. Theor. Appl. Genet. 123, 1107–1119. doi: 10.1007/s00122-011-1652-2
Cuthbert, P. A., Somers, D. J., and Brule-Babel, A. (2007). Mapping of Fhb2 on chromosome 6BS: a gene controlling Fusarium head blight field resistance in bread wheat (Triticum aestivum L.). Theor. Appl. Genet. 114, 429–437. doi: 10.1007/s00122-006-0439-3
Cuthbert, P. A., Somers, D. J., Thomas, J., Cloutier, S., and Brule-Babel, A. (2006). Fine mapping Fhb1, a major gene controlling fusarium head blight resistance in bread wheat (Triticum aestivum L.). Theor. Appl. Genet. 112, 1465–1472. doi: 10.1007/s00122-006-0249-7
Devi, U., Grewal, S., Yang, C.-, Hubbart-Edwards, S., Scholefield, D., Ashling, S., et al. (2019). Development and characterisation of interspecific hybrid lines with genome-wide introgressions from Triticum timopheevii in a hexaploid wheat background. BMC Plant Biol. 19:183. doi: 10.1186/s12870-019-1785-z
Dvorak, J., Diterlizzi, P., Zhang, H. B., and Resta, P. (1993). The evolution of polyploid wheats-identification of the A-genome donor species. Genome 36, 21–31. doi: 10.1139/g93-004
Dvorak, J., and Zhang, H. B. (1990). Variation on repeated nucleotide-sequences hseds light on the phylogeny of the wheat B-genomes and G-genomes. Proc. Natl. Acad. Sci. U.S.A. 87, 9640–9644. doi: 10.1073/pnas.87.24.9640
Fedak, G., Cao, W., Wolfe, D., Chi, D., and Xue, A. (2017). Molecular Characterization of Fusarium Resistance from Elymus repens Introgressed into Bread Wheat. Cytol. Genet. 51, 130–133. doi: 10.3103/s0095452717020025
Feldman, M. (1966). Identification of unpaired chromosomes in F1 hybrids involving Triticum aestivum and T. timopheevii. Can. J. Genet. Cytol. 8, 144–151. doi: 10.1139/g66-019
Franco, M. F., Lori, G. A., Cendoya, M. G., Panelo, J. S., Alonso, M. P., Mirabella, N. E., et al. (2021). Using anthesis date as a covariate to accurately assessing type II resistance to Fusarium head blight in field-grown bread wheat. Crop Prot. 142:105504. doi: 10.1016/j.cropro.2020.105504
Gong, B., Zhu, W., Li, S., Wang, Y., Xu, L., Wang, Y., et al. (2019). Molecular cytogenetic characterization of wheat-Elymus repens chromosomal translocation lines with resistance to Fusarium head blight and stripe rust. BMC Plant Biol. 19:590. doi: 10.1186/s12870-019-2208-x
Guo, J., Zhang, X., Hou, Y., Cai, J., Shen, X., Zhou, T., et al. (2015). High-density mapping of the major FHB resistance gene Fhb7 derived from Thinopyrum ponticum and its pyramiding with Fhb1 by marker-assisted selection. Theor. Appl. Genet. 128, 2301–2316. doi: 10.1007/s00122-015-2586-x
Hales, B., Steed, A., Giovannelli, V., Burt, C., Lemmens, M., Molnar-Lang, M., et al. (2020). Type II Fusarium head blight susceptibility conferred by a region on wheat chromosome 4D. J. Exp. Bot. 71, 4703–4714. doi: 10.1093/jxb/eraa226
Innes, R. L., and Kerber, E. R. (1994). Resistance to wheat leaf rust and stem rust in Triticum tauschii and inheritance in hexaploid wheat of resistance transferred from T. tauschii. Genome 37, 813–822. doi: 10.1139/g94-116
Jorgensen, J. H., and Jensen, C. J. (1973). Gene PM6 for resistance to powdery mildew in wheat. Euphytica 22, 423–423.
King, J., Grewal, S., Othmeni, M., Coombes, B., Yang, C.-Y., Walter, N., et al. (2022). Introgression of the Triticum timopheevii Genome Into Wheat Detected by Chromosome-Specific Kompetitive Allele Specific PCR Markers. Front. Plant Sci. 13:919519. doi: 10.3389/fpls.2022.919519
Li, G., Zhou, J., Jia, H., Gao, Z., Fan, M., Luo, Y., et al. (2019). Mutation of a histidine-rich calcium-binding-protein gene in wheat confers resistance to Fusarium head blight. Nat. Genet. 51, 1106–1112. doi: 10.1038/s41588-019-0426-7
Ma, H.-X., Bai, G.-H., Gill, B. S., and Hart, L. P. (2006). Deletion of a chromosome arm altered wheat resistance to Fusarium head blight and deoxynivalenol accumulation in Chinese Spring. Plant Dis. 90, 1545–1549. doi: 10.1094/pd-90-1545
Malihipour, A., Gilbert, J., Fedak, G., Brule-Babel, A., and Cao, W. (2016). Characterization of agronomic traits in a population of wheat derived from Triticum timopheevii and their association with Fusarium head blight. Eur. J. Plant Pathol. 144, 31–43. doi: 10.1007/s10658-015-0744-2
Malihipour, A., Gilbert, J., Fedak, G., Brule-Babel, A., and Cao, W. (2017). Mapping the A Genome for QTL Conditioning Resistance to Fusarium Head Blight in a Wheat Population with Triticum timopheevii Background. Plant Dis. 101, 11–19. doi: 10.1094/pdis-02-16-0144-re
McIntyre, C. L., Pereira, S., Moran, L. B., and Appels, R. (1990). New Secale cereale (rye) DNA derivatives for the detection of rye chromosome segments in wheat. Genome 33, 635–640. doi: 10.1139/g90-094
Mesterhazy, A., Bartok, T., Mirocha, C. G., and Komoroczy, R. (1999). Nature of wheat resistance to Fusarium head blight and the role of deoxynivalenol for breeding. Plant Breed. 118, 97–110. doi: 10.1046/j.1439-0523.1999.118002097.x
Miller, J. D., Young, J. C., and Sampson, D. R. (1985). Deoxynivalenol and Fusarium head Blight reisstane in spring cereals. J. Phytopathol. 113, 359–367.
Nemeth, C., Yang, C. Y., Kasprzak, P., Hubbart, S., Scholefield, D., Mehra, S., et al. (2015). Generation of amphidiploids from hybrids of wheat and related species from the genera Aegilops, Secale, Thinopyrum, and Triticum as a source of genetic variation for wheat improvement. Genome 58, 71–79. doi: 10.1139/gen-2015-0002
Qi, L. L., Pumphrey, M. O., Friebe, B., Chen, P. D., and Gill, B. S. (2008). Molecular cytogenetic characterization of alien introgressions with gene Fhb3 for resistance to Fusarium head blight disease of wheat. Theor. Appl. Genet. 117, 1155–1166. doi: 10.1007/s00122-008-0853-9
Rawat, N., Pumphrey, M. O., Liu, S., Zhang, X., Tiwari, V. K., Ando, K., et al. (2016). Wheat Fhb1 encodes a chimeric lectin with agglutinin domains and a pore-forming toxin-like domain conferring resistance to Fusarium head blight. Nat. Genet. 48, 1576–1580. doi: 10.1038/ng.3706
Rayburn, A. L., and Gill, B. S. (1986). Molecular identification of the D-genome chromosomes of wheat. J. Hered. 77, 253–255.
Rigby, P. W., Dieckmann, M., Rhodes, C., and Berg, P. (1977). Labeling deoxyribonucleic acid to high specific activity in vitro by nick translation with DNA polymerase I. J. Mol. Biol. 113, 237–251. doi: 10.1016/0022-2836(77)90052-3
Rines, H. W., Porter, H. L., Carson, M. L., and Ochocki, G. E. (2007). Introgression of crown rust resistance from diploid oat Avena strigosa into hexaploid cultivated oat A sativa by two methods: direct crosses and through an initial 2x center dot 4x synthetic hexaploid. Euphytica 158, 67–79. doi: 10.1007/s10681-007-9426-2
Rumyantsev, S. D., Veselova, S. V., Burkhanova, G. F., and Maksimov, I. V. (2019). Induced resistance to the greenbug aphid Schizaphis graminum Rond. in species of the genus Triticum. Vavilovskii Zh. Genet. Sel. 23, 865–872. doi: 10.18699/vj19.560
Sadeghabad, A. A., Dadkhodaie, A., Heidari, B., Razi, H., and Mostowfizadeh-Ghalamfarsa, R. (2017). Microsatellite markers for the Triticurn timopheevi-derived leaf rust resistance gene Lr18 on wheat 5BL chromosome. Breed. Sci. 67, 129–134. doi: 10.1270/jsbbs.16148
Schroeder, H. W., and Christensen, J. J. (1963). Factors affecting resistance of wheat to scab caused by Gibberella zeae. Phytopathology 53, 831–838.
Shen, X., and Ohm, H. (2007). Molecular mapping of Thinopyrum-derived Fusarium head blight resistance in common wheat. Mol. Breed. 20, 131–140. doi: 10.1007/s11032-007-9079-9
Singh, P. K., Mergoum, M., Ali, S., Adhikari, T. B., Elias, E. M., and Hughes, G. R. (2006). Identification of new sources of resistance to tan spot, Stagonospora nodorum blotch, and Septoria tritici blotch of wheat. Crop Sci. 46, 2047–2053. doi: 10.2135/cropsci2005.12.0469
Spanic, V., Dvojkovic, K., Babic, J., Drezner, G., and Zdunic, Z. (2021). Fusarium Head Blight Infestation in Relation to Winter Wheat End-Use Quality-A Three-Year Study. Agronomy 11:1648. doi: 10.3390/agronomy11081648
Steed, A., Chandler, E., Thomsett, M., Gosman, N., Faure, S., and Nicholson, P. (2005). Identification of type I resistance to Fusarium head blight controlled by a major gene located on chromosome 4A of Triticum macha. Theor. Appl. Genet. 111, 521–529. doi: 10.1007/s00122-005-2043-3
Su, Z., Bernardo, A., Tian, B., Chen, H., Wang, S., Ma, H., et al. (2019). A deletion mutation in TaHRC confers Fhb1 resistance to Fusarium head blight in wheat. Nat. Genet. 51, 1099–1105. doi: 10.1038/s41588-019-0425-8
Timonova, E. M., Leonova, I. N., Roder, M. S., and Salina, E. (2013). Marker-assisted development and characterization of a set of Triticum aestivum lines carrying different introgressions from the T. timopheevii genome. Mol. Breed. 31, 123–136. doi: 10.1007/s11032-012-9776-x
Valverde-Bogantes, E., Bianchini, A., Herr, J. R., Rose, D. J., Wegulo, S. N., and Hallen-Adams, H. E. (2020). Recent population changes of Fusarium head blight pathogens: drivers and implications. Can. J. Plant Pathol. 42, 315–329. doi: 10.1080/07060661.2019.1680442
Van Eeuwijk, F. A., Mesterhazy, A., Kling, C. I., Ruckenbauer, P., Saur, L., Burstmayr, H., et al. (1995). Assessing nonspecificity of resistance in wheat to head blight caused by Fusarium culmorum, F. graminearum and F. nivale using a multiplicative model for interaction. Theor. Appl. Genet. 90, 221–228. doi: 10.1007/bf00222205
Wan, W. T., Xiao, J., Li, M. L., Tang, X., Wen, M. X., Cheruiyot, A. K., et al. (2020). Fine mapping of wheat powdery mildew resistance gene Pm6 using 2B/2G homoeologous recombinants induced by the ph1b mutant. Theor. Appl. Genet. 133, 1265–1275. doi: 10.1007/s00122-020-03546-8
Wang, H., Sun, S., Ge, W., Zhao, L., Hou, B., Wang, K., et al. (2020). Horizontal gene transfer of Fhb7 from fungus underlies Fusarium head blight resistance in wheat. Science 368:eaba5435. doi: 10.1126/science.aba5435
Wang, M. Y., Baenziger, P. S., El-Basyoni, I. S., and Wegulo, S. N. (2015). Comparison of Fusarium Head Blight Resistance in Cytoplasmic Male Sterile, Maintainer and Restorer Lines in Winter Wheat. Cereal Res. Commun. 43, 374–383. doi: 10.1556/0806.43.2015.006
Yong-Fang, W., Chi, Y., and Jun-Liang, Y. (1997). Sources of resistance to head scab in Triticum. Euphytica 94, 31–36. doi: 10.1023/a:1002982005541
Keywords: wheat, disease, cytogenetics, plant pathology, fungi, breeding
Citation: Steed A, King J, Grewal S, Yang C-y, Clarke M, Devi U, King IP and Nicholson P (2022) Identification of Fusarium Head Blight Resistance in Triticum timopheevii Accessions and Characterization of Wheat-T. timopheevii Introgression Lines for Enhanced Resistance. Front. Plant Sci. 13:943211. doi: 10.3389/fpls.2022.943211
Received: 13 May 2022; Accepted: 15 June 2022;
Published: 06 July 2022.
Edited by:
István Molnár, Hungarian Academy of Sciences (MTA), HungaryReviewed by:
Mahmoud Said, Academy of Sciences of the Czech Republic, CzechiaOfere Francis Emeriewen, Julius Kühn Institute (JKI), Germany
Copyright © 2022 Steed, King, Grewal, Yang, Clarke, Devi, King and Nicholson. This is an open-access article distributed under the terms of the Creative Commons Attribution License (CC BY). The use, distribution or reproduction in other forums is permitted, provided the original author(s) and the copyright owner(s) are credited and that the original publication in this journal is cited, in accordance with accepted academic practice. No use, distribution or reproduction is permitted which does not comply with these terms.
*Correspondence: Paul Nicholson, cGF1bC5uaWNob2xzb25AamljLmFjLnVr