- 1Department of Agronomy, University of Agriculture, Faisalabad, Pakistan
- 2Department of Agricultural Engineering, Khwaja Fareed University of Engineering and Information Technology, Rahim Yar Khan, Pakistan
- 3College of Plant Science and Technology, Huazhong Agricultural University, Wuhan, China
- 4Division of Biological and Environmental Sciences and Engineering, Smart-Health Initiative and Red Sea Research Center, King Abdullah University of Science and Technology, Thuwal, Saudi Arabia
- 5Agricultural Botany Department, Faculty of Agriculture (Saba Basha), Alexandria University, Alexandria, Egypt
- 6Plant Protection and Biomolecular Diagnosis Department, Arid Lands Cultivation Research Institute, City of Scientific Research and Technological Applications, New Borg El Arab, Egypt
- 7Research Center Ecological Sciences, Jiangxi Agricultural University, Nanchang, China
Nitrogen (N) is an important macro-nutrient required for crop production and is considered an important commodity for agricultural systems. Urea is a vital source of N that is used widely across the globe to meet crop N requirements. However, N applied in the form of urea is mostly lost in soil, posing serious economic and environmental issues. Therefore, different approaches such as the application of urea coated with different substances are used worldwide to reduce N losses. Urea coating is considered an imperative approach to enhance crop production and reduce the corresponding nitrogen losses along with its impact on the environment. In addition, given the serious food security challenges in meeting the current and future demands for food, the best agricultural management strategy to enhance food production have led to methods that involve coating urea with different nutrients such as sulfur (S) and zinc (Zn). Coated urea has a slow-release mechanism and remains in the soil for a longer period to meet the demand of crop plants and increases nitrogen use efficiency, growth, yield, and grain quality. These nutrient-coated urea reduce nitrogen losses (volatilization, leaching, and N2O) and save the environment from degradation. Sulfur and zinc-coated urea also reduce nutrient deficiencies and have synergetic effects with other macro and micronutrients in the crop. This study discusses the dynamics of sulfur and zinc-coated urea in soil, their impact on crop production, nitrogen use efficiency (NUE), the residual and toxic effects of coated urea, and the constraints of adopting coated fertilizers. Additionally, we also shed light on agronomic and molecular approaches to enhance NUE for better crop productivity to meet food security challenges.
Introduction
The rate at which the global population is growing is posing a serious threat to food security (He et al., 2021). By 2050, the population is predicted to exceed 9.2 billion people (Silva, 2018), resulting in a 60–102% rise in food consumption (Elferink and Schierhorn, 2016). Currently, agricultural nitrogen utilization has already exceeded its “sustainable” level, calling for efficient nitrogen usage; however, that alone does not address the need for increasing agricultural production required by a growing population (He et al., 2021). On the other hand, continuous water shortage, labor scarcity, nutrient mining, and loss of soil fertility are significantly reducing agricultural production across the globe (Shivay et al., 2019; Mohammed et al., 2020).
Nitrogen plays a pivotal role in many physiological processes, grain quality, and biomass production (Anas et al., 2020). It plays a vital role in forming chlorophyll, proteides and proteins, and other essential compounds like plant hormones; however, plants are inefficient in the acquisition and utilization of applied nitrogen (Tiong et al., 2021). Additionally, the application of conventional urea is also not so efficient leading to excessive nitrogen loss from the soil. Therefore, farmers use enormous amounts of N to boost crop productivity which is not just undesirable but leads to many environmental problems (Elferink and Schierhorn, 2016). Excessive use of nitrogen (N) fertilizers causes soil acidification and groundwater pollution by water eutrophication, which also increases greenhouse gas emissions (Mohanty et al., 2020). Therefore, there is an urgent need to adopt an efficient N management approach such as nutrient-coated urea which increases nitrogen use efficiency (NUE), crop quality, and yield (Prasad and Hobbs, 2018).
The coating technology is designed for the slow release of the nutritional content of fertilizers and synchronization of their release rate with the nutritional demand of the plants. The characteristics of the gradual release of nitrogen contents can be physically imparted to the urea by coating its granules with various materials which delay its dissolution rate (Naz and Sulaiman, 2016; Ullah et al., 2020; Badshah et al., 2021; Al-Nemi et al., 2022). Coated fertilizer (Figure 1) is believed to be the best solution under the current scenario to deliver nutrients to crops for enhancing their productivity, reducing nutrient losses, and minimizing the subsequent impact on the environment (Shivay et al., 2019; Shah et al., 2022). Nutrient-coated urea behaves as a slow-release fertilizer as the thin nutrient layer that is applied over the urea granules hydrolyzes slowly and remains in the soil for a longer period, which in turn improves crop productivity and efficient nutrient use. Moreover, coated urea also improves nitrogen availability along with other macro and micronutrients that are vital for crop growth and development (Khan et al., 2015). Different coated fertilizers are currently available in local markets, but in this review, our main focus is on sulfur and zinc-coated urea.
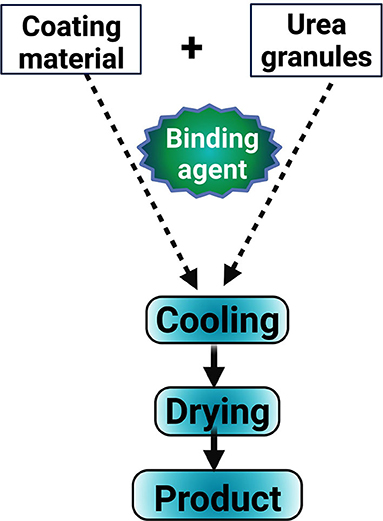
Figure 1. Graphical presentation of coated urea preparation. The urea is mixed with matter to be coated and allowed to cool and dry to obtain coated urea.
Sulfur is a fundamental element required for nitrogen metabolism and protein synthesis and is mandatory for many enzymatic and metabolic activities including plant defense mechanism systems against biotic or abiotic stress (Khan et al., 2014; Yu et al., 2018; Tavanti et al., 2021). The application of S-coated urea substantially improves crop growth, development, and yield compared to uncoated urea (Xin et al., 2017; Shivay et al., 2019). Sulfur-coated urea (SCU) boosts root growth by increasing its diameter and length which further helps in increasing nutrient uptake (Timilsena et al., 2015), and plays a part in dry matter assimilation (Haseeb-ur-Rehman et al., 2022). It also improved the availability of nitrogen (N), phosphorus (P), potassium (K), and zinc (Zn) (Najafian and Zahedifar, 2015), and improved chlorophyll content and photosynthetic efficiency (Awais et al., 2015). SCU augments the gradual release of nitrogen leading to a reduction in nitrogen leaching and volatilization, thereby increasing nitrogen use efficiency (Ke et al., 2018).
Zinc is an essential element for optimum plant growth and plays an important function in metabolism. It is necessary for the proper functioning of various physiological functions in plants, including photosynthesis and sugar generation, fertilization and seed production, growth regulation, and disease resistance (Hassan et al., 2020). Zinc stimulates enzymes involved in the creation of certain proteins. It aids the plant's ability to survive low temperatures by assisting in the creation of chlorophyll and certain carbohydrates, as well as the conversion of starch to sugar (Chattha et al., 2017; Hassan et al., 2019; Suganya et al., 2020; Hassan M. U. et al., 2021). Zinc-coated urea is a source of sufficient nutrients (Zn and N) that meet the initial requirements of growing plants (Qureshi et al., 2021; Beig et al., 2022). By boosting plant physiology and metabolism, zinc-coated urea (ZnCU) has the potential to provide a long-term enhancement in agricultural productivity (Figure 2) and quality (Ain et al., 2020; Hassan M. et al., 2021; Rehman et al., 2021). Zinc-coated urea increases zinc concentration in rice and wheat grains, resulting in higher crop quality (Irshad et al., 2021).
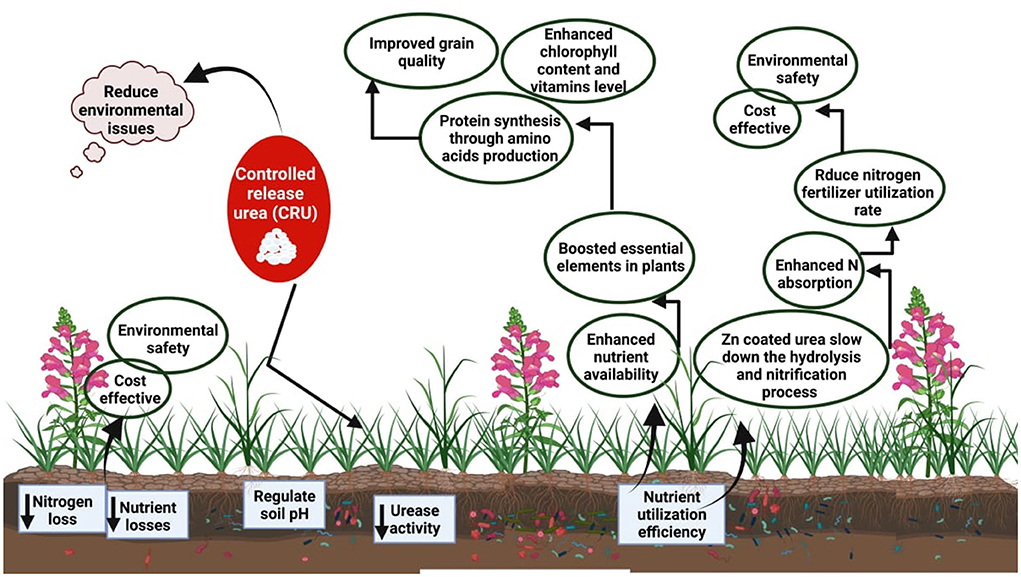
Figure 2. Benefits of coated urea. Urea coating reduces leaching and volatilization losses, improves grain yield, reduces the costs of fertilizer application, and increases nutrient absorption and nutrient availability in the soil.
The controlled release mechanism of nitrogenous fertilizers prevents N losses in plants, and the urea coated with compatible compounds is beneficial for the slow distribution of nitrogen and enhancement of NUE in plants. In this review, we focused on nutrient-coated urea, crop performance, their dynamics in soil, toxicity, and the deficiency of zinc and sulfur in plants, exploring agronomic and molecular approaches interlinked with sulfur and zinc-coated urea that enhance NUE and crop production.
Role of zinc and sulfur in plant biology
Sulfur and zinc are imperative nutrients required for plant growth and development. Sulfur contributes to the production of various metabolites, which in turn improve plant performance in terms of growth and productivity (Gigolashvili and Kopriva, 2014). Moreover, sulfur also plays a significant role in the electron transport chain and improves plant resistance to stressed conditions (Hell et al., 2010). Sulfur and nitrogen have the same assimilation pathway and S-containing metabolites are crucial to the photosynthetic process (Mazid et al., 2011). Zinc plays a significant role in physiological functions and it is necessary for the maintenance of protein structure functions, synthesis, enzyme structure, gene expression, energy production, carbohydrate metabolism, auxin metabolism, Krebs cycle, and photosynthesis (Nadeem et al., 2020). Moreover, Zn also protects against abiotic stresses and substantially improves crop productivity and quality (Nadeem et al., 2020).
Effect of zinc and sulfur-coated urea on photosynthesis
When sulfur absorption is reduced from 2,500 to around 500 (g g−1) dry weight; the rate of photosynthetic CO2 uptake from intact leaves, cyclic and non-cyclic photo-phosphorylation of isolated chloroplasts is significantly disrupted. The photosynthetic efficiency in plants is drastically reduced under S deficiency as sulfur is involved in the production of chlorophyll content (Figure 2) which has a direct impact on the rate of photosynthesis (Abadie and Tcherkez, 2019). Sulfur also protects the plant from oxidative stress and thereby improves the plant's performance under stressed conditions. Sulfur-containing proteins play a significant role in photosynthesis, carbon metabolism, and subsequent production of assimilates (Hooghe et al., 2013). The chlorophyll content can be reduced up to 39–65% in the event of S deficiency (Padhi et al., 2013) due to the accumulation of sugars in plant roots being increased by sulfur uptake (Abadie and Tcherkez, 2019). Sulfur inhibits several metabolic activities, including the bruising of chlorophyll membranes, the loss of pigments from cells, and the breakdown of chlorophyll, which results in diminished photosynthesis (Padhi et al., 2013).
Chlorophyll content, photosynthesis, and respiration rate along with carbonic anhydrase activity increase with zinc application. A small amount of zinc concentration of 13–14 g/g dry weight is required for optimum photosynthesis and chlorophyll production (Raza et al., 2018). Zinc is also involved in the production of chlorophyll content by regulating the amounts of specific nutrients in the cytoplasm (Aravind and Prasad, 2004, 2005). Zinc deficiency in plants results in disrupted photosynthesis due to changes in chloroplast structure and pigments (Kosesakal and Unal, 2009). It sustains the chlorophyll levels and is vital for maintaining chloroplast structure in plants (Chen et al., 2007).
Effect of zinc and sulfur-coated urea on protein synthesis
Methionine, homocysteine, cysteine, and taurine are the four main sulfur-containing amino acids. Amino acids regulate protein metabolism, and protein synthesis is significantly altered when there is a deficiency of sulfur-containing amino acids (David et al., 2016). They also regulate nutrient metabolism (Shigi et al., 2021). Methionine, a component of many proteins, is important in DNA and RNA synthesis and serves as a methyl group donor. Several sulfur-containing nucleosides consist of the derivatives of 2-thiocytidine (s2C), 2-thiouridine (s2U), 4-thiouridine (s4U), and 2-methylthioadenosine (ms2A), which ensure sulfur availability in each biosynthetic channel (Shigi, 2014). Intracellular antioxidants glutathione and N-acetyl cysteine are produced by sulfur amino acids which are involved in the antioxidant defense mechanism (Colovic et al., 2018). Cysteine also forms a disulfide bond which plays a pivotal role in protein-folding pathways and protein structure (Brosnan and Brosnan, 2006). Sulfur increases the uptake of P (phosphorus) and N (nitrogen) during seed formation; moreover, it is an integral part of nucleic acid and protein synthesis (Shigi et al., 2021).
Zinc fingers (small protein structured motif) allow enzymes to act as a template for the production of a specific protein by transcribing a second genetic division from DNA to RNA. They have a wide range of functions, including DNA recognition, RNA packaging, transcriptional activation, apoptosis regulation, protein folding and assembly, and lipid binding. In addition, Zn catalyzes numerous enzymatic actions and helps keep protein sub-domains folded (McDonald et al., 2000). It is essential for the tetrahedral bonding of particular genes for the transcription of protein. As a result, a loop of 11-13 A.A. (amino acids) forms DNA-metalloproteins, which are then used as a DNA expression in the protein translation process with the help of Zn (McDonald et al., 2000). Zinc is a component of ribosomes and is responsible for protein synthesis. McDonald et al. (2000) found ribosomal disintegration in the absence of Zn. Moreover, Zn deficiency is the most common cause of protein synthesis inhibition, resulting in lower amounts of ribonucleic (RNA) acid and reduced plant growth (Krishna et al., 2020).
Effect of zinc and sulfur-coated urea on growth and development
Sulfur is an indispensable element for plant growth and development. It has been identified as a key nutrient that improves crop growth and quality (Table 1). Enough sulfur is required for plant functions and is critical for optimal crop performance (Marschner, 2012). It is taken up by the plant in the form of sulfate, which is absorbed by the roots or shoots. Sulfur deficiency lowers the concentration and activity of the ribulose-I,5-bisphosphate carboxylase/oxygenase (Rubisco) enzyme resulting in a substantial reduction in photosynthesis (Makino, 2003) which causes a significant decrease in plant growth, development and grain production (Chan et al., 2019). Sulfur protects plants from diseases in their reproductive stage—the period when plants are more vulnerable to sulfur deficiency. Sulfur deficiency reduces protein quality and many secondary metabolites involved in plant developmental mechanisms (Saleem et al., 2019).
Zinc is an essential component of several enzymes, which are responsible for a variety of metabolic reactions in all crops. It is also necessary to produce tryptophan, a precursor to IAA and a growth-promoting substance (Cabot et al., 2019). It is involved in cell division, cell proliferation, pollen production, and disease resistance, thus, influencing crop yield (Vadlamodi et al., 2020). Zinc deficiency can delay plant growth, shorten crop maturation time, produce spikelet sterility, and diminish harvest product quality (Suganya et al., 2020). Zinc in plants promotes leaf development, flowering, and production of gibberellin and trehalose 6-phosphate (signaling chemicals), as well as regulating the vegetative growth stage (Gonzalez et al., 2012). Pollen generating capacity, anther, and pollen grain size along with their viability are decreased under low Zn supply (Rudani et al., 2018).
Effect of zinc and sulfur-coated urea on assimilate partitioning
Accumulation of photosynthates in grains is the end product of photosynthetic activity. Carbon dioxide assimilation, sugar generation, nitrogen fixation (Table 2), and protein formation all require sulfur (Ali et al., 2013). Sulfur positively influences dry matter accumulation because it involves photosynthates partitioning from source to sink, resulting in a significant improvement in grain production (Rani et al., 2009). It also helps in glucose translocation to the reproductive segments of plants (Sahoo et al., 2018). Sulfur deficiency in oilseeds during early growth stages limits plant growth resulting in lowering biomass production. Ahmad et al. (2005) found that plants uptake and store sulfur and use it in their developmental and physiological processes; besides, sulfur has a synergistic effect with N, P, and K, which increase the biological yield in crops (Chen et al., 2010).
Zinc plays a chief role in assimilating portioning and its moderate applications (3.3–4.4 kg ha−1) can increase nutrient uptake and biomass production (Shrestha et al., 2020). Zinc deficiency reduces sucrose content in sugar, beet, and maize (Rengel and Pearson, 1997) and decreases assimilate partitioning toward reproductive parts, resulting in a decrease in crop output. Zinc insufficiency also affects carbohydrate transport and grain sucrose availability (Song et al., 2015), in addition to lowering carbonic anhydrase activity, which prevents CO2/HCO3 transport in the leaf mesophyll for photosynthetic CO2 fixation and in turn impairs photosynthetic rate and lowers biomass accumulation (Siddiqui et al., 2015). Toxic Zn levels hinder root sinking force and reduce assimilate translation by inhibiting root development and slightly expanding shoot growth (Gupta et al., 2016). The flow of photo assimilates, especially Zn, into grains (the sink) is governed by the source-sink connection throughout the grain-filling stage, and Zn-coated urea also stimulates Zn flow from source to sink (Chen et al., 2020). Sink capacity and strength are determined by the number and size of grains, as well as their ability to absorb Zn from source organs. The concentration of Zn in grains sinking presently varies between 20 and 35 mg/kg globally (Chen et al., 2020).
Dynamics of nutrient-coated urea in soil
To cope with the food security challenges, farmers have utilized extensive application of chemical fertilizers to increase crop yields in recent years (Qiao et al., 2016). Urea is a basic synthetic fertilizer that is regarded as a primary source of plant nutrition (Trenkel, 2010). Conventional urea has less NUE because most of the nitrogen is wasted in denitrification, volatilization, and leaching, which is harmful to the environment and has led to agro-ecological issues (Naz et al., 2014). Therefore, the best nitrogen management technique needs to reduce nitrogen losses in the agriculture sector and maximize the efficient use of nitrogen for sustainable agricultural development (Lu et al., 2010). Controlled release urea (CRU) or nutrient-coated urea is less soluble due to the coating on the urea granules that progressively release (Table 3) the fertilizer and makes it ideal for crop synchronization (Trenkel, 2010). It increases crop production while reducing nutrient losses to the environment. Controlled release fertilizers (CRFs) have been extensively explored to make them safer and more cost-effective. They keep nutrients in the soil for the longest possible time and increase NUE by 20–30% (Cong et al., 2010). Nutrient-coated fertilizers also improve water efficiency, soil water retention capacity, soil texture and structure, and ultimately, soil quality (Fashola et al., 2006). As urease inhibitors, the usage of environmentally acceptable coated urea and micronutrients can be advantageous as a two-in-one solution. They can temporarily reduce urease activity in the soil and function as a vital nutrient for plants and the soil. These materials are easily available, biodegradable, and inexpensive. The use of natural materials as an adhesive maintains nitrogen and micronutrients on the microsite together. As a result, the urease inhibitory and acidifying properties of natural materials control the rise in pH and urea concentration on the applied soil surface. To minimize nitrogen loss (Table 4) and improve urea efficiency, urea can be coated with sulfur, urease inhibitors, or other biodegradable compounds (Shaviv, 2001). Urease inhibitors (agrotain) are chemicals that limit the action of the urease enzyme in soil and slow down the urea hydrolysis process. The efficacy of these coated fertilizers varies significantly depending on land use, soil type, and soil moisture regime (Bolan et al., 2004). Sulfur-coated (SCU) has a projected release timing ranging between 45 and 180 d; however, the greatest impact on SCU's N release comes from temperature as the rate of diffusion doubles for about every 10°C increase (Hopkins, 2020). Fertilizer-related concerns such as leaf burning, water pollution, and eutrophication are minimized. Slow nutrient release reduces runoff and leaching losses by keeping available nutrient concentrations in soil solution at a lower level (Table 5). Alkaline soils with a lower pH provide improved nutrient absorption. Because both sulfur and urea contribute to raising soil acidity (dropping soil pH), applying sulfur-coated urea is likely to enhance soil acidity (Trenkel, 2010). Throughout all phases of plant growth, the nutrient release meets the crop's nutritional requirements. Using CRFs and SRFs at appropriate moments can meet the timely plant nutrient demand requirements, increase fertilizer usage efficiency, and reduce environmental issues.
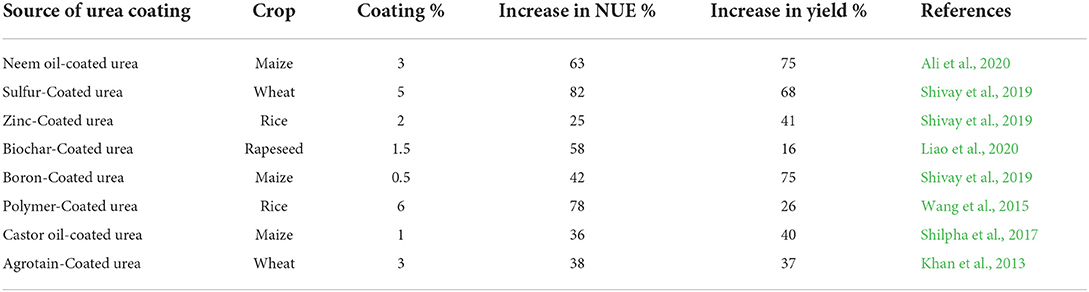
Table 5. Application of coated urea to improve nitrogen use efficiency and yield of particular crops with specific coating %.
Factors affecting nutrient-coated urea and their availability
Soil pH, alkalinity, poor soil organic matter (OM), water holding capacity of the soil, microbial activity, amount and forms of minerals in the soil, soil moisture content, and soil texture all influence the availability of nutrients to plants, particularly zinc and sulfur (Figure 3). Other parameters that impact the potential of zinc and sulfur-coated urea include temperature, humidity, and light intensity, as well as interactions among other nutrients.
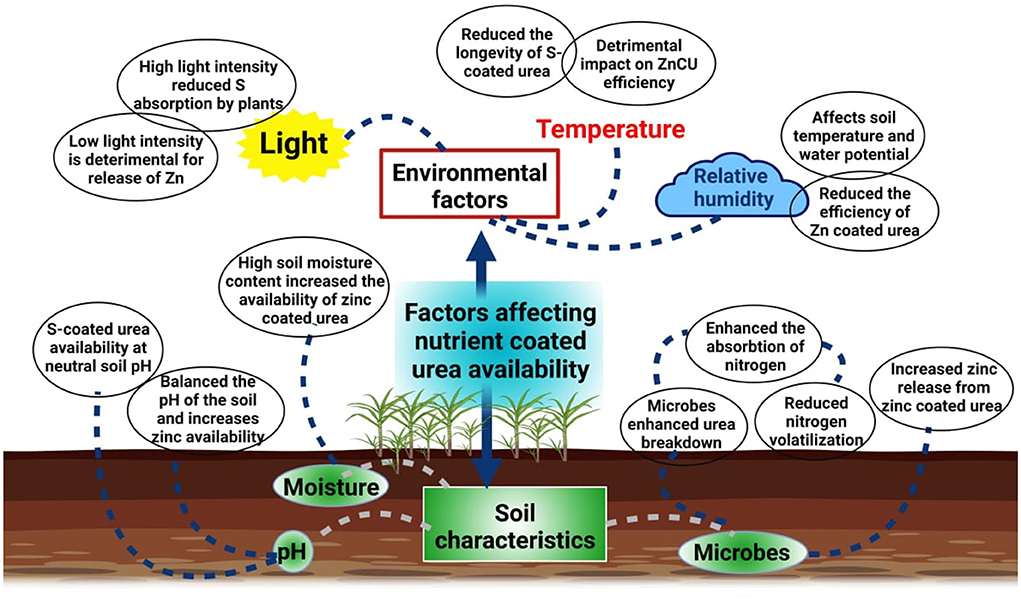
Figure 3. Factors affecting the availability of coated urea in soil. Low temperatures, moisture content, and low light intensity reduces the availability of coated urea in soil.
Effect of soil pH on coated urea
Soil pH greatly influences the availability of plant nutrients by changing their forms. Low pH alleviates the macro and secondary macronutrients, however high pH reduces the availability of some micronutrients. Microbial activity may be diminished or altered in varying soil pH (Neina, 2019) as soil pH triggers the abundance and movement of S oxidizers in the soil (Zhao et al., 2015). The potential of S-coated urea is more pronounced in neutral soil pH and plants cannot uptake sulfur at lower soil pH. When sulfur is applied to the soil with low pH, the acidulated fertilizer lowers the percentage of nitrified N, which slows down the nitrification process and eventually stops as the pH drops below six. Urease activity picks up as the pH of the soil rises, peaking at a pH of 6.0–6.5 (Beig et al., 2020). The only cost-effective way to lower pH in the rhizosphere is to apply sulfur-coated urea to the soil (Akay et al., 2019). Soil pH also controls zinc mobility in soil (Sauvé et al., 2000; Smolders et al., 2004). Zinc solubility in soil declines 100-fold for each pH unit rise in soil and Zn availability reduces in high soil pH (Zhao et al., 2016). Rengel (2015) stated that the soil pH (more than 6.5) significantly reduces Zn availability to plants. A high deficiency of Zn was found in sandy soils compared to clayey soils (Suganya et al., 2020), though clay particles reduce the Zn uptake (Moreno-Lora and Delgado, 2020). In addition, Ain et al. (2020) reported that calcium carbonate concentration due to high Ca2+ ions in elevated soil pH fixes Zn in soil colloids that make Zn unavailable to the plants. Thus, plants uptake less Zn in calcareous soils (Iratkar et al., 2014). However, Moosa et al. (2021) reported that the exchangeability of zinc isotopes increases with increasing pH in the soil. In another study, zinc uptake is more in soil having high OM (Iratkar et al., 2014). The breakdown of soil organic matter, nutrient cycling, and efficiency of micro-fauna is reducibly observed in acidic soil by Madigan et al. (2010). Low total Zn concentration, high pH and calcite concentration, and organic matter content are the key factors that affect Zn availability in plants (Kihara et al., 2020). The quantity of Zn adsorption and desorption is strongly linked to soil pH (Bereket, 2018). Low pH inhibits zinc adsorption more in sandy soils than in soils rich in colloidal-size components (Moosa et al., 2021). Zinc-coated urea decreases the pH of the soil, thus, it increases zinc availability in the rhizosphere. Babar et al. (2018) observed the positive effect of Zn-coated urea in controlling the fluctuation of pH ranging from 6.0 to 6.5 in submerged paddy soils. Hence, Zn-coated urea as a mineral-coated fertilizer significantly maintains the soil pH around the optimal plant value of 6.5.
Effect of soil moisture content on availability of coated urea
The effect of soil water potential on the degradation of coating components, urea absorption, and/or diffusion of dissolved urea from the granules may alter the rate of urea release from SCU (Zhang et al., 2011). The rate of nitrogen release from SCU depends on the type of coating, coating thickness, soil moisture, and soil temperature (Halvorson et al., 2014), whereas water permeability alters the nutrient release dynamic of the coated urea (Irfan et al., 2018). The coated material in SCU expands as it comes into contact with the soil water and gets converted into a hydrogel. Water diffuses into the cross-linked coated network, allowing the soluble half of the fertilizer to be gently released into the soil (Remya et al., 2021).
High soil moisture content improves the availability of zinc-coated urea in the soil (Angle et al., 2003). The amount of nutrients released is related to the water vapor pressure and is regulated by the ion concentration of the liquid medium. The moisture found in lower layers of the soil develops a moisture gradient, which augments the soil microbial activities responsible for nitrification and denitrification processes of urea in the soil (Kuang et al., 2019). In addition, the highest nitrification process was observed with 60% pore spaces of soil filled with water (Jinuntuya-Nortman et al., 2008). While soil moisture has a substantial impact on the rate of nitrogen release from Zn-coated urea, more nitrogen is released when soil humidity rises (Irfan et al., 2018). The rate of nutrient release is proportional to soil water pressure. The influence of soil moisture on nutrient release patterns may be described by the effects of vapor migration; however, this does not appear to be consistent with a diffusion process in the liquid phase (Moosa et al., 2021).
Effect of microbial activity on availability of coated urea
Microbial activity in the soil has been identified as a key component influencing nitrogen (N) release from slow-release fertilizers. The N released from S-coated urea is mainly governed by the activity of soil microbes (Nardi et al., 2018). In addition, nitrogen release can be delayed by 6–8 weeks by reducing soil microbial populations (Nardi et al., 2018). Sulfur-coated urea is hydrolyzed by microbial ureases into ammonia and carbon dioxide (Rutherford, 2014). Microbes cannot perform efficiently in low soil water as their potential is reduced; therefore, nitrogen release from SCU is reduced (Moosa et al., 2021). Moreover, when the concentration of urea around soil microsites and soil pH increases due to the application of urea fertilizer, urease enzyme activity starts to hydrolyze the applied urea which eventually causes N losses (Watson, 2000; Krajewska, 2009). The SCU acts as a urease inhibitor, a possible N loss remedy because it inhibits the activity of the urease enzyme in the soil and reduces the rate of hydrolysis of urea in soil (Shaviv, 2001; Bolan et al., 2004).
Microorganisms in the soil play a significant role in the release of nitrogen in the soil (Morgan et al., 2009). The release pattern of coated urea is directly proportional to the microbial activity in soil (Gil-Ortiz et al., 2020). Soil microbes cause complete urea breakdown, allowing plants to absorb nitrogen, reducing nitrogen volatilization, and increasing zinc release from zinc-coated urea (Giroto et al., 2021). Bacteria convert nitrogen in the compound to nitrate, lowering soil pH and diminishing soil microbial diversity, which has a detrimental influence on the coated urea release pattern with zinc nanoparticles (Sadiq et al., 2021). Soil bacteria infiltrate the coating through fractures in the material and break down the urea granules; the microbial population alters the nutrient release and duration of zinc-coated urea fertilizer (Santos et al., 2018). The rate of breakdown of coated urea fertilizers containing Zn is mostly determined by soil temperature and density of soil microflora, and the duration is also determined by the activity of soil microbes (Yang et al., 2018). Zinc-coated urea reduces the rate of urea hydrolysis and serves as a urease inhibitor which increases crop growth and yield (Babar et al., 2018).
Environmental factors
Nitrogen is found in the form of ammonia (NH3), nitrous oxide (N2O), and nitrate (NO) in the atmosphere. Nutrient-coated urea is one of the most effective ways to reduce environmental losses while also providing other nutrients that are coated on the urea to have a synergetic effect on the urea. Many environmental conditions, including light intensity, humidity, and temperature, have an impact on the dynamics of nutrient-coated urea. These environmental conditions affect nutrient requirements and availability in plants, and they are listed below:
Effect of light intensity on availability of coated urea
Sulfur concentration is badly affected by a high light intensity that reduces the plant biomass and accumulation of sulfur (Zenda et al., 2021). The ideal average light intensity is 30–40 micro Em−2, which does not deplete the concentration of sulfur. Higher light intensity boosts PSII activity, resulting in increased hydrogen production and a reduction in S concentration (Tatyana et al., 2004). Photo-degradation of SCU depends on the intensity and type of light (Gafoor et al., 2021). Light intensity has no direct effect on SCU efficiency, but it does reduce S absorption by plants, which has a negative impact on plant growth, quality, and NUE. Variations in light intensity may influence the rate of diffusion of SCU (Adams et al., 2013).
In contrast, zinc concentrations in plants are improved by high light intensity up to a certain point. As the light intensity rises from 200 to 3,000–4,000 foot-candle, zinc responses are enhanced; any further increase up to 11,000 foot-candle decreases zinc response (Baligar et al., 2006). When light intensity reaches near saturation point in photosynthesis, Zn reaction is at its peak. Due to Zn deficiency in crops, low light intensity limits root and shoot development (Edwards and Kamprath, 1974). Zinc-coated urea enhances pecan (a fruit having a single stone) growth and development by increasing photosynthesis and catching greater light intensity. Although low light intensity does not influence the release mechanism of Zn-coated urea, Zn absorption in plants is limited, which has a detrimental impact on plant development and production (Sadiq et al., 2021).
Effect of relative humidity on availability of coated urea
In general, the release of coated fertilizer increases with increasing soil humidity. Even if the soil moisture is below field capacity, the osmotic potential of high humidity in soil augments the release of dissolved nitrogen from coated material to soil media (Christianson, 1988). However, sulfur metabolite absorption is susceptible to high humidity that inhibits sulfate uptake and severely impairs photosynthesis in plants. Low vapor pressure deficit (VPD) affects the stomatal conductance, reduces S absorption, and thus, photosynthetic efficiency is lowered. Shivay et al. (2016) observed that the diffusion rate is maximum in low field capacity soil moisture. Humid conditions are required for the disintegration and transport of nitrogen from coated urea fertilizers outward as humidity lengthens the duration of SCU and alters the coating material's release process (Baboo and Manager, 2016).
Relative humidity reduces zinc concentrations in plants and limits the availability of other micronutrients (Cu, Fe) in green tea shoots (Sud et al., 1995). For instance, Muster et al. (2011) reported that Zn uptake is greatly affected by high temperatures in humid environments. High humidity contributes to accelerating the release of nitrogen from Zn-coated urea by increasing the amount of water retained at the soil surface (Giroto et al., 2021). Humidity affects soil temperature and water potential, reducing the efficiency of Zn-coated urea in soil and making it easier to solubilize the urea granules by adding water through coatings (Sadiq et al., 2021).
Effect of temperature on availability of coated urea
High temperature impairs photosynthesis, respiration, reproduction, and pollen development. Sulfur-coated urea is an emerging and efficient method to enhance plant defense mechanisms under heat stress conditions. Sulfur biomolecules ameliorate many negative impacts of environmental factors in plants (Ihsan et al., 2019). Nitrogen release from SCU fertilizers as diffusion rates is considerably boosted when sufficient moisture is available, moreover, its release is temperature dependent (Du et al., 2006; Morgan et al., 2009). For instance, high temperatures result in the early release of N from CRU fertilizer application in soil (Christianson, 1988). Similarly, Shivay et al. (2016) observed that temperature extremes reduced the longevity of all SCU products by altering the release mechanism of SCU along with soil characteristics such as soil moisture, temperature, and microbial activity.
Zinc intake and availability are restricted at high temperatures (Han et al., 2019), affecting chloroplast ultrastructure, its concentration, and chlorophyll fluorescence in zinc-deficient plants (Peck and McDonald, 2010). The use of nitrogen in combination with zinc, such as zinc-coated urea, minimizes the negative effects of high temperatures in plants to a certain extent (Raj et al., 2015). Heat stress in plants may be alleviated by providing Zn through Zn-coated urea, which boosts Zn levels in various plant sections; Zn increases ABA concentration, which in turn, strengthens the plant's defense system (Nazir et al., 2021). The kinetics of Zn-coated urea in the soil is altered by high temperatures, which has a detrimental impact on ZnCU efficiency (Nazir et al., 2021).
To minimize N loss in changing environmental conditions, controlled/slow-release fertilizers are specially designed that can sufficiently regulate the N requirement of crops during the growing season (Janke et al., 2022).
Interaction with other nutrients affects the availability of coated urea
The interaction between two or more nutrients could have a synergistic (positive), antagonistic (negative), or no influence on the availability and crop uptake of other nutrients but mostly interactions among the macronutrients represent synergistic results that enhance crop NUE (Aulakh and Malhi, 2004; Rietra et al., 2017). Likewise, studies found that the macronutrient K is an essential nutrient like S and Zn required for crop growth and development and could have a synergistic effect with S and Zn availability (Jones and Jacobsen, 2005).
Sulfur has an antagonistic relationship with potassium; hence potassium buildup depletes the sulfur content of plants. Sulfur should be used in conjunction with NPK, which is designed to provide agronomic bio-fortification (Klikocka and Marks, 2018). Sulfur and nitrogen have a synergetic effect, and because both nutrients are utilized in protein synthesis, a precise N:S ratio increases crop quality and production. At various levels, several interactions were discovered in the absorption of NO3 and SO4 in plants (Klikocka and Cybulska, 2014). Molybdenum molecules are comparable in size to sulfur and their concentration significantly increases in the presence of sulfur, while White et al. (2004) observed selenium replaces sulfur molecules in plant biology. Sulfur increases the content and availability of micronutrients such as Fe, Mn, Zn, and Cu. In high soil pH circumstances that limit sulfur concentrations or where sulfur contents are low, it is advisable to add sulfur-containing fertilizers or sulfur-coated urea (Klikocka and Marks, 2018). Zinc has a positive relationship with nitrogen and potassium, but antagonistic relationships with phosphorus, iron, calcium, and copper. They have a detrimental impact because these nutrients obstruct zinc absorption and transfer from roots to shoots in plants. For instance, Ca and Zn fight for adsorption sites on the soil surface and in the roots (Irfan et al., 2018). Mycorrhizal infection of roots was suppressed when P was increased by lowering zinc levels. Plants' zinc requirements are harmed by increased phosphorus deposition in their leaves (Shivay et al., 2008). Zinc-coated urea is a way to protect plants from boron toxicity (Irfan et al., 2018) and Zinc insufficiency boosts Fe and Mn concentrations in wet soils while zinc-copper interaction increases wheat production (Shivay et al., 2008).
Enhancement of yield through the application of nutrient-coated urea
Urea is a common fertilizer that is highly soluble in water and has volatilization as well as leaching losses severely impacting farmers' costs (Shivay et al., 2016). Urea granules should be encapsulated with less soluble compounds to avoid this problem. In addition, urea can be coated with sulfur, urease inhibitors, and other biodegradable environmentally safe compounds (Khan et al., 2015). Agricultural productivity is partly attributed to a continuous and long-lasting delivery of nutrients, which can only be achieved by using nutrient-coated urea. The use of SCU delays the nitrogen release while enhancing its utilization efficiency in rice fields. Rice production increased up to 55–68% with the use of sulfur-coated urea. The use of SCU in wheat and flooded rice fields minimizes nitrogen leaching losses (Wei et al., 2020). According to Shivay et al. (2019), the yield and seasonal distribution of growth achieved with a single application of sulfur-coated urea were similar to those obtained with repeated (3–5) applications of urea. Increased plant usage efficiency due to extended soil retention and fewer fertilizer applications, and resultant fertilizer and application cost savings are prospective benefits of using coated urea (Nasima et al., 2010). Sulfur-coated urea increased dry matter production in rice, nitrogen absorption was increased up to 39.4%, and grain protein percentage was boosted up to 5.8–14.9% with the use of SCU as compared to normal urea application in rice (Khan et al., 2015).
Zinc-coated urea increased grain yield by around 28% and is quite effective toward plant physiological attributes (Nazir et al., 2021). As compared to prilled urea, 29% of rice production was increased by the use of zinc-coated urea (Shivay et al., 2019). Zinc enhances several biochemical, physiological, quality, and yield-related factors in cereals by restoring plant vigor, oil content, sugars, and pigments. Using Zn-coated urea to slow down the hydrolysis and nitrification process resulted in enhanced N absorption and, as a result, a higher yield (Affendi et al., 2018). Zinc-coated urea also increased the yield by improving NUE while completing the Zn need of the sunflower crop (Sadiq et al., 2021). The application of zinc-coated urea to rice resulted in an increased transpiration rate and a higher percentage of normal kernels while lowering the percentage of opaque, abortive, and chalky kernels (Irshad et al., 2021). In comparison to the prilled urea (PU), the application of Zn-coated urea increased the yield by 29% and Zn concentration in aromatic rice grain (Shivay et al., 2019). As a result, the Zn concentration in zinc-coated urea has a significant impact on crop quality and output (Suganya et al., 2020). Zn content in maize grain was much greater than PU, and with 2.5 percent of ZnCU, total Zn intake (grain + stover) increased by 32.4 percent and improved crop yield (Pooniya et al., 2018). In maize, total zinc intake was 32.4% higher with the use of 2.5% Zn-coated urea compared to un-coated fertilizer, which increased the yield by about just 4.6% (Pooniya et al., 2018).
Enhancement of grain quality through the application of nutrient-coated urea
In comparison to prilled urea, sulfur-coated urea enhanced nitrogen absorption by 39.4% and increased grain protein percentage from 5.8 to 149%. Furthermore, S was active in the production of proteins, chlorophyll content, vitamins, and sulfur-containing amino acids like cysteine and methionine, which are essential components of protein (Naiknaware et al., 2015). Coating urea with sulfur resulted in more N remaining in the soil for longer periods. A higher proportion of absorbed N, and more N being translocated into the grain, improved urea N efficiency and grain quality (Khan et al., 2015). Sulfur-coated urea increased kernel size, density, oil content, protein content, and starch content while decreasing virtuousness, damaged kernels, and fungal infections, all of which contributed to grain quality (Haseeb-ur-Rehman et al., 2022).
Zinc-coated urea boosts Zn levels in a variety of plant components, including leaves, tubers, and fruit, as well as the whole grain and endosperm (Wakeel et al., 2018). To acquire good quality rice (increased Zn concentration in rice grains), special attention should be paid to balancing Zn levels in soil, which can only be achieved by using Zn-coated urea (Amanullah and Inamullah, 2016). Zn-coated urea improves Zn absorption and partitioning into different plant parts, potentially increasing rice grain output and nutritional quality (Wang et al., 2014). It also aids in the absorption of zinc in grains, which helps to improve grain quality by boosting protein and amino acid content (Li et al., 2015).
Enhancement of nitrogen use efficiency through the application of nutrient-coated urea
Coated urea fertilizers increase nitrogen supply while lowering nitrogen losses in the form of leaching, volatilization, and N2O emission (Jadon et al., 2018; Wei et al., 2020). Normal urea is less efficient as compared to nutrient-coated urea whose NUE (Figure 4) is 30–60% less than coated urea (Chen et al., 2020). With coating, 20–30% dose of urea can be saved than normal urea application while increasing its uptake and higher yield production. It increases nitrogen agronomy efficiency (NAE; 23.4%), reduces nitrogen fertilizer utilization rate (NUR; 34.65%), and enhances 25.83% nitrogen physiological efficiency (NPE; 25.83%) (Zhu et al., 2020).
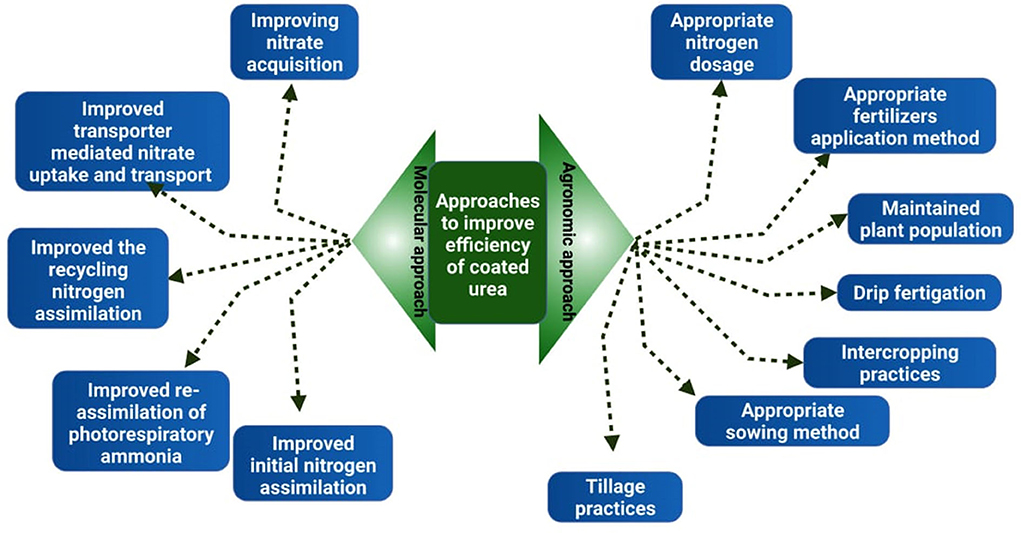
Figure 4. Approaches to improve the efficiency of coated urea. Appropriate dosage of nitrogen fertilizer, optimum plant population, drip fertigation, intercropping, appropriate sowing method, and improved genetic makeup of plants can enhance the utilization efficiency of coated urea.
The use of sulfur improves NUE by 50% and nitrogen recovery efficiency by 60% in wheat crops (Byatvarkeshi and Zareabbyaneh, 2016; Shivay et al., 2016). Additionally, volatilization losses of nitrogen are decreased in coated urea which improves nitrogen recovery in plants (Wang et al., 2016; Wei et al., 2020). For instance, Gafoor et al. (2021) found the least nitrate concentration (7.37 kg ha−1) with SCU owing to reduced nitrogen losses in groundwater table from wheat fields. With the application of zinc-coated urea, NO3-N leaching decreased to 35.1% (Jadon et al., 2018). Nitrogen use efficiency improved by up to 55% using Zn-coated urea, and ammonia volatilization losses were greatly reduced (Sadiq et al., 2021). Zn-coated urea enhanced NUE by up to 80%, increased rice yield by 50%, and grain Zn content by 126%. It provides nutrients to plants in a regulated and delayed way, reducing nutrient emissions, leaching, and runoff losses, while simultaneously improving crop NUE and yield (Sadiq et al., 2021). Nitrogen losses can be reduced by using Zn-coated urea, and N efficiency can be raised by slowing down the rate of urea hydrolysis in the soil (Babar et al., 2016).
Residual effects of nutrient-coated urea
Application of N fertilizer beyond its need generates undesirable consequences which result in the degradation of water, soil, and air quality. It also leads to soil acidification, decreased groundwater quality due to nitrate leaching, and increased nitrous oxide (N2O) emissions, which is a 300-fold more powerful greenhouse gas than CO2 contributing to global warming. When the concentration of nitrate exceeds 10 mg L−1 in drinking water, it becomes unfit for humans and animals (Jadon et al., 2018).
The accumulation of S in the soil is associated with reduced soil pH, inhibited root growth, and nutrient intake, as S is susceptible to leaching and the formation of sulfuric acid in soil media is common (Liu et al., 2010). Sulfur dioxide toxicity causes excessive water loss due to increased stomatal opening leading to water scarcity and thus disrupting photosynthetic efficiency and consequently reducing photosynthesis. In addition, S toxicity causes root damage, leaf senescence, and reduced growth of crop plants (Liu et al., 2010). Plant growth is impeded if soil S concentrations in the upper horizon (0–20 cm) reach 4% (Likus-Cieślik et al., 2017). The proficiency of Zn fertilizer rarely exceeds 10% because Zn is voluntarily fixed with soil colloids and is mostly available for succeeding crops due to its fixing nature (Li et al., 2013).
Zinc diffuses widely and reaches a phytotoxic level in many soils due to the intense application of excess fertilizers, pesticides, manures, sewage sludges, smelters, incinerators, mines, and galvanized products (Kaur and Garg, 2021). Zn toxicity causes curling and rolling of young leaves, stunting shoot growth, chlorosis, and death of leaf marginal cells. In high concentrations of Zn, cell organelles collapse leading to damage in the cytoplasmic structure in plants, and concentrations >10 mg/kg or 7.5 mM condenses the chromatic material, dilates the nuclear membrane, and disrupts the cortical cells (Moreira et al., 2018; Ning et al., 2020). Moreover, the intake of iron is inhibited by Zn toxicity in the soils, whereas Zn toxicity is caused by an overabundance of magnesium in the soil (Moreira et al., 2018).
Constraints in adopting coated fertilizers
There are several constraints to using nutrient-coated urea: (i) lack of farmer awareness about the need for nutrient-coated urea fertilizers, especially S and Zn-coated urea. Farmers are unaware of the effectiveness and significance of coated urea fertilizers, which is the task of extension workers to go door to door to advise farmers that coated urea not only reduces the amount of application as well as costs without reducing yield, but also adds additional nutrients to the soil, increases soil health, and crop quality; (ii) lack of monetary access for coated fertilizers; (iii) the source of information is not reliable; (iv) lack of knowledge on making nutrient-coated urea; (v) lack of capital; (vi) difficulty in calculating the optimal dose of nutrients to coat urea; and (vii) not available on time (Chouhan et al., 2018).
Agronomic approach to improving the efficiency of coated urea
The addition of organic manures to soils provides several micronutrients (Ca, Mg, S, Mn, Fe) that make it more fertile for better crop production by improving the soil's physical (sand, silt, and clay percentages), chemical (CEC, WHC, and pH), and biological (soil fauna population) properties (Wang et al., 2020). Crop rotation is the major agronomic practice that is adopted for sustainable nutrient balance in the soil. Nitrogen losses are too high, up to 70% of total available nitrogen, due to excess nitrogen, low plant population, inefficient application methods, or other factors. Improved agronomic practices such as appropriate nitrogen dosage, application of nitrogen through canopy sensors, maintaining plant population, drip fertigation, and legume-based intercropping can reduce these losses by up to 15–30% (Shivay et al., 2016). The amount of fertilizer applied is mostly dictated by soil properties and agroclimatic conditions. The improvement in nutrient utilization can be achieved using nutrient-coated fertilizer application in crop fields and that is the best agronomic approach to modern agriculture-farming (Luo et al., 2018). The coated material (sulfur and zinc) has a synergetic effect with urea that enhances nitrogen absorption and increases NUE (Shivay et al., 2016). Wang et al. (2020) suggested that the use of sulfur and zinc-coated urea is the best approach for farmers to alleviate sulfur and zinc deficiencies in crops. Bhuiyan (1994) found nutrient-coated urea better than poultry manure (PM) and it increased the efficiency of applied fertilizers. Sowing of crops on ridges and application of nutrient-coated urea fertilizer improves the water use efficiency of crops. Laser land leveling, conservation tillage and choosing good quality seeds, and sowing and irrigation methods along with coated urea fertilizers can change the whole scenario of agriculture and make crops vigorous and bio-fortified with specific nutrients whose availability is far less than their demand (Khan et al., 2006).
The use of nutrient-coated fertilizers (urea), therefore, is the straightforward and economically best approach that enhances food production to meet the current food security challenge. But it requires specific consideration in terms of nutrient source, application technique, and environmental consequences. Nutrient-coated urea must be administered as a single dose during each crop season, making them cost-effective in most situations.
Molecular approach to improving the efficiency of coated urea
Molecular techniques refer to the genetic modification that is done at DNA molecular level to enhance desired traits in plants, and these tools and techniques should be considered for crop improvement. The tools contain molecular marker techniques like PCR amplified DNA sequences, RAPD, and AFLP. Researchers should choose the appropriate technique that is best suited for their respective programs and they should be encouraged to integrate molecular tools based on gene maps, gene cloning, QTL mapping, marker-assisted selection, microsatellites, SNP, and molecular cytogenetics. Molecular approaches facilitate monitoring of plant health, detecting pathogens, reducing disease spread, and aiding in better crop management (Wan et al., 2017). In this context, nitrogen uptake, assimilation, and utilization by plants through DNA markers are new emerging molecular techniques that help improve fertilizer use efficiency. The dynamic character of nitrogen, as well as its tendency to leach into the soil and environment, provides a unique challenge for its better management. The Nitrate Transporter 1/Peptide Transporter (NPF) family (Léran et al., 2014), the Nitrate Transporter 2 (NRT2) family, the Chloride Channel (CLC) family, the Slow Anion Associated Channel Homolog (SLC/SLAH) family, and aluminum-activated malate transporters (ALMT) family mediate nitrate uptake and transport in plants (Li H. et al., 2017). Many other metabolic processes and gene expression levels regulate nitrogen in plants. The reduction of nitrate to nitrite by the nitrate reductase enzyme happens in the cytosol. Nitrite is carried into chloroplasts in leaves and transformed into ammonium ions by the enzyme nitrite reductase. During the production of nucleic acid, chlorophyll, and amino acids, the products of ammonia, glutamine, and glutamate, serve as nitrogen donors. Three major ammonium assimilation processes have been proposed: initial nitrogen assimilation, reassimilation of photorespiratory ammonia, and “recycled” nitrogen assimilation. Organic nitrogen is transported from source to sink organs in the form of amino acids. Due to ammonium remobilization, ammonium absorbing enzymes are required during the grain filling stage (Wan et al., 2017). The GS and GOGAT pathways are involved in the production of amino acids from ammonia. Two types of genes, GLN1 and GLN2, are regulated by GS with a decameric structure. GLN2 (single nuclear gene) encodes chloroplastic GS2, which is involved in ammonium absorption or re-assimilation in C3 and C4 plants, or from the photorespiratory product of C3 plants. The GLN1 gene family, on the other hand, encodes the GS1 isoform, which recycles ammonium during leaf senescence and transport in phloem sap. Overexpression of HATS-like NRT2.1 enhances nitrate influx, while NUE and its phenotypic utilization remain unaltered. The transgenic rice plant's grain production improved by overexpressing NADH-GOGAT. To increase yield by overexpressing GS or GOGAT genes, it is necessary to understand the alleles of genes and their promoters (Zhou et al., 2017). In Arabidopsis, overexpression of ASN1 boosted soluble protein content in seed, total protein, and the plant's ability to grow on a nitrogen-limited diet. These findings indicate that modifying downstream N-remobilization stages could improve NUE (Li W. et al., 2017).
The inconsistency of overexpressed important enzymes (NR, NiR, GS, and GOGAT) for improving NUE or phenotypic change is also a problem. Nitrogen utilization efficiency is linked to nitrate acquisition, which can be improved further by modifying nitrate assimilation enzymes and proteins using various biotechnological techniques. To improve NUE, it is critical to target several mechanisms, enzymes, and variables rather than focusing on a single rate-limiting mechanism. As a result of these factors, new molecular techniques such as microarray and transcriptome are being considered emerging tools for studying the response of the entire genome of plants. In all plants, whole genome RNA sequencing is a current way of understanding changes at the genomic level, gene expression level, and individual genes associated with desirable features. In the future, the combination of DEGs (differentially expressed genes) with QTL databases will be critical in developing new nitrogen use-efficient genotypes (Gelli et al., 2014). It is concluded that a combination of agronomic and molecular techniques has the potential to improve nitrogen use efficiency.
Conclusion and future thrusts
Nutrient-coated urea is an efficient N management approach for ensuring better productivity in crops, reducing nitrogen losses (leaching, volatilization, and denitrification), and the subsequent impact on the environment. The application of S and Zn-coated urea improved chloroplast structure, chlorophyll contents, stomatal conductance, photosynthetic and transpiration rate, assimilate partitioning, protein synthesis, and antioxidant activity, therefore improving plant growth, biomass, and grain yield. Moreover, S and Zn-coated urea substantially improved the NUE and reduced N losses as compared to conventional urea. Controlled release urea (CRU) or nutrient-coated urea is less soluble due to the coating on the urea granules that progressively releases the fertilizer making it ideal for crop synchronization. It retains the nutrients in the soil for the longest possible time and improves water use efficiency, soil water retention capacity, soil microbial activity, soil texture and structure, and ultimately soil quality. Environmental factors such as light intensity, humidity, and temperature also play an important role in the release mechanism of coated urea and its availability in soil for plants.
However, there are many unanswered questions. Future research should concentrate on molecular approaches such as marker-assisted selection and inclusive hybridization (integration of desirable genes), as well as biotechnological approaches, such as next-generation DNA and RNA sequencing, and genetic alterations to create genotypes with improved zinc and sulfur contents and aptitudes to acquire more sulfur and zinc from the soil. A GIS-based mapping can be used to discover nutrient-deficient zones/regions to regulate the most appropriate basis of nutrient-coated fertilizer and application methods to boost nutrient efficiency. Broad and long-term research should be done on the release kinetics of nutrient-coated urea, particularly on how their release synchronizes with the requirement of the plant. The role of coated urea should be explored under modern sowing methods including bed and ridge sowing methods and new irrigation methods including drip and sprinkler irrigations. Its role in acid and highly alkaline soils should be studied as the soil pH is a major factor that affects nutrient uptake by plants. The effect of Zn and sulfur-coated urea on soil microbial activities and their compositions are poorly studied, and it is imperative to bridge this research gap. Similarly, the role of sulfur and Zn-coated urea in plant functioning is also poorly studied. For instance, the role of coated urea in germination mechanisms has not been explored and it would be pertinent to explore the role coated urea plays in seed germination. While the role of sulfur and Zn-coated urea on nutrient uptake has been well-researched, their role in nutrient signaling and their impact on nutrient and ionic transporters needs attention. Likewise, their influence on stomata movement, electron transport, and efficiency of PS-II is poorly studied, therefore it is important to explore their roles in these aspects. Additionally, their impact on seed quality compositions and genes involved in anti-oxidant activities needs further study. The effect of S and Zn-coated urea on different hormones and osmolytes have not been adequately explored, particularly under normal and diverse stress conditions. Lastly, their impact on plant growth, yield, and various physiological functions under different abiotic stress (cold, drought, heat, heavy metals, and salinity stress) must also be studied given that zinc, sulfur, and nitrogen play an appreciable role in plant tolerance against different stresses.
Author contributions
AMu, IK, and MC: conceptualization. AMu, IK, MC, and MH: writing original draft. MN, AS, AMd, MB, MJ, NA, and RG: writing-review and editing. All authors have read and agreed to the published version of the manuscript.
Acknowledgments
Authors would like to acknowledge Alexandria University, Alexandria, Egypt and King Abdullah University of Science and Technology (KAUST), Thuwal, 23955-6900, Saudi Arabia for supporting the research.
Conflict of interest
The authors declare that the research was conducted in the absence of any commercial or financial relationships that could be construed as a potential conflict of interest.
Publisher's note
All claims expressed in this article are solely those of the authors and do not necessarily represent those of their affiliated organizations, or those of the publisher, the editors and the reviewers. Any product that may be evaluated in this article, or claim that may be made by its manufacturer, is not guaranteed or endorsed by the publisher.
References
Abadie, C., and Tcherkez, G. (2019). Plant sulfur metabolism is stimulated by photorespiration. Commun. Biol. 2, 379. doi: 10.1038/s42003-019-0616-y
Adams, W. W., Muller, I. I. I., Cohu, O., and Demmig-Adams, C. M. B. (2013). May photoinhibition be a consequence, rather than a cause, of limited plant productivity? Photosynth. Res. 117, 31–44. doi: 10.1007/s11120-013-9849-7
Adil, A., Zhu, X., Zhu, M., Quan, M., Irshad, S., Xu, D., et al. (2021). Effects of environmental stresses (Heat, Salt, Waterlogging) on grain yield and associated traits of wheat under application of sulfur-coated urea. Agronomy 11, 2340. doi: 10.3390/agronomy11112340
Affendi, N. M. N., Yusop, M. K., and Othman, R. (2018). Efficiency of coated urea on nutrient uptake and maize production. Commun. Soil Sci. Plant Anal. 49, 1–7. doi: 10.1080/00103624.2018.1464182
Ahmad, A., Khan, I., Anjum, N. A. M., Diva, I., Abdin, M. Z., and Iqbal, M. (2005). Effect of timing of sulfur fertilizer application on growth and yield of rapeseed. J. Plant Nutr. 28, 1049–1059. doi: 10.1081/PLN-200058905
Ain, U. N., Naveed, M., Hussain, A., Mumtaz, M. Z., Rafique, M., Bashir, M. A., et al. (2020). Impact of coating of urea with Bacillus-Augmented zinc oxide on wheat grown under salinity stress. Plants 9, 1375. doi: 10.3390/plants9101375
Akay, A., Serek, C., and Negis, H. (2019). Effect of enhanced elemental sulfur doses on pH value of a calcareous oil. J. Agric. Sci. 29, 34–40. doi: 10.29133/yyutbd.467632
Ali, A., Iqbal, Z., Hassan, S. W., Yasin, M., Khaliq, T., and Ahmed, S. (2013). Effect of nitrogen and sulfur on phenology, growth and yield parameters of maize crop. Sci. Int. 25, 363–366.
Ali, M., Maqsood, M. A., Aziz, T., and Awan, M. I. (2020). Neem (Azadirachta indica) oil coated urea improves nitrogen use efficiency and maize growth in an alkaline calcareous soil. Pak. J. Agri. Sci. 57, 675–684. doi: 10.21162/PAKJAS/20.9850
Al-Nemi, R., Makki, A. A., Sawalha, K., Hajjar, D., and Jaremko, M. (2022). Untargeted metabolomic profiling and antioxidant capacities of different solvent crude extracts of Ephedra foeminea. Metabolites 12, 451. doi: 10.3390/metabo12050451
Amanullah and Inamullah. (2016). Residual phosphorus and zinc influence wheat productivity under rice–wheat cropping system. Springerplus 255, 5. doi: 10.1186/s40064-016-1907-0
Anas, M., Liao, F., Verma, K. K., Sarwar, M. A., Mahmood, A., Chen, Z. L., et al. (2020). Fate of nitrogen in agriculture and environment: agronomic, eco-physiological and molecular approaches to improve nitrogen use efficiency. Biol. Res. 53, 1–20. doi: 10.1186/s40659-020-00312-4
Angle, J. S., Baker, A. J. M., Whiting, S. N., and Chaney, R. L. (2003). Soil moisture effects on uptake of metals by Thlaspi, Alyssum, and Berkheya. Plant Soil 256, 325–332. doi: 10.1023/A:1026137624250
Aravind, P., and Prasad, M. N. V. (2004). Carbonic anhydrase impairment in cadmium treated Ceratophyllum demersum L. (free floating freshwater macrophyte): toxicity reversal by zinc. J. Anal. Atomic Spectrom. 19, 52–57. doi: 10.1039/b307282g
Aravind, P., and Prasad, M. N. V. (2005). Modulation of cadmium-induced oxidative stress in Ceratophyllum demersum by zinc involves ascorbate-glutathione cycle and glutathione metabolism. J. Plant Physiol. Biochem. 43, 107–116. doi: 10.1016/j.plaphy.2005.01.002
Aulakh, M. S., and Malhi, S. S. (2004). “Fertilizer nitrogen use efficiency as influenced by interactions with other nutrients,” in Agriculture and the Nitrogen Cycle: Assessing the Impacts of Fertilizer Use on Food Production and the Environment (Washington, DC: Island Press), 181–192.
Awais, M., Wajid, A., Ahmad, A., Saleem, M. F., Bashir, M. U., Saeed, U., et al. (2015). Nitrogen fertilization and narrow plant spacing stimulates sunflower productivity. Turk. J. Field Crop. 20, 99–108. doi: 10.17557/.17374
Babar, S. K., Naheed, A. T., and Aijaz, A. K. (2018). Effects of Cu and Zn coated urea on Eh, pH and solubility of Cu and Zn in rice soils. Mehran Univ. Res. J. Eng. Technol. 37, 615–622. doi: 10.22581/muet1982.1804.14
Babar, S. K., YuSop, M. K., Babar, S. A., and Khooharo, A. (2016). Consequences of Cu and Zn coated urea to minimize ammonia volatilization. J. Technol. 78, 6–12. doi: 10.11113/jt.v78.9209
Badshah, S. L., Faisal, S., Muhammad, A., Poulson, B. G., Emwas, A. H., and Jaremko, M. (2021). Antiviral activities of flavonoids. Biomed. Pharm. 140, 111596. doi: 10.1016/j.biopha.2021.111596
Baligar, V. C., Fageria, N. K., Paiva, A. Q., Silveira, A., Pomella, A. W. V., and Machado, R. C. R. (2006). Light intensity effects on growth and micronutrient uptake by tropical legume cover crops. J. Plant Nutr. 29, 1959–1974. doi: 10.1080/01904160600927633
Beig, B., Niazi, M. B. K., Jahan, Z., Kakar, S. J., Shah, G. A., Shahid, M., et al. (2020). Biodegradable polymer coated granular urea slows down N release kinetics and improves spinach productivity. Polymers 12, 2623. doi: 10.3390/polym12112623
Beig, B., Niazi, M. B. K., Jahan, Z., Zia, M., Shah, G. A., Iqbal, Z., et al. (2022). Facile coating of micronutrient zinc for slow release urea and its agronomic effects on field grown wheat (Triticum aestivum L.). Sci. Total Environ. 838, 155965. doi: 10.1016/j.scitotenv.2022.155965
Bereket, H. (2018). Ph.D. Thesis. Haramaya University; Dire Dawa, Ethiopia. Assessment of Zn and Iron, Zn and adsorption, and effects of Zn and iron on yields and grain nutrient concentration of teff and bread wheat in some soils of tigray, Northern Ethiopia. Agr. Ecosyst. Environ. 265, 402–412.
Bhuiyan, N. J. (1994). “Crop production trends and need of sustainability in agriculture,” in Integrated Nutrient Management for Sustainable Agriculture, Held at Soil Resource Development Institute (Dhaka).
Bolan, N., Saggar, S., and Singh, J. (2004). The role of inhibitors in mitigating nitrogen losses in grazed pasture. N. Zeal. Soil News 52, 52–58.
Brosnan, J. T., and Brosnan, M. E. (2006). The sulfur-containing amino acids: an overview. J. Nutr. 136, 1636S–1640S. doi: 10.1093/jn/136.6.1636S
Byatvarkeshi, M., and Zareabbyaneh, H. (2016). Effects of slow release fertilizers on nitrate leaching, its distribution in soil profile, nitrogen use efficiency and yield in potato crop. Environ. Earth Sci. 74, 3385–3393. doi: 10.1007/s12665-015-4374-y
Cabot, C., Martos, S., Llugany, M., Gallego, B., Tolra, R., and Charlotte, P. (2019). A role for zinc in plant defense against pathogens and herbivores. Front. Plant Sci. 10, 1171. doi: 10.3389/fpls.2019.01171
Chan, K. X., Phua, S. Y., and Van-Breusegem, F. (2019). Secondary sulfur metabolism in cellular signalling and oxidative stress responses. J. Exp. Bot. 70, 4237–4250. doi: 10.1093/jxb/erz119
Chattha, M. U., Hassan, M. U., Khan, I., Chattha, M. B., Mahmood, A., Chattha, M. U., et al. (2017). Biofortification of wheat cultivars to combat zinc deficiency. Front. Plant Sci. 8, 281. doi: 10.3389/fpls.2017.00281
Chen, S., Zhang, X., Sun, H., Ren, T., and Wang, Y. (2010). Effects of winter wheat row spacing on evapotranspiration, grain yield and water use efficiency. Agric. Water Manag. 97, 1126–1132. doi: 10.1016/j.agwat.2009.09.005
Chen, W., Yang, X., He, Z. Z., Feng, Y., and Hu, F. (2007). Differential changes in photosynthetic capacity, 77K chlorophyll fluorescence and chloroplast ultrastructure between Zn-efficienct and Zn-inefficient rice genotypes (Oryza sativa L.) under low Zn stress. J. Plant Physiol. 132, 89–101. doi: 10.1111/j.1399-3054.2007.00992.x
Chen, Z., Wang, Q., Zou, J. M. P., and Jiang, L. (2020). Impact of controlled-release urea on rice yield, nitrogen use efficiency and soil fertility in a single rice cropping system. Sci. Rep. 10, 10432. doi: 10.1038/s41598-020-67110-6
Chouhan, R. S., Niranja, H. K., Sharma, H. O., Rath, D., and Kurmi, H. S. (2018). Constraint in adoption of neem coated urea (NCU) in Madhya Pradesh. Int. J. Bio Resour. Stress Manag. 9, 173–177. doi: 10.23910/IJBSM/2018.9.1.3C0530
Christianson, C. B. (1988). Factors affecting N release of urea from reactive layer coated urea. Fertilizer Res. 16, 273–284. doi: 10.1007/BF01051376
Colovic, M. B., Vasic, V. M., Dragon, M., Djuric, M., and Krstic, D. Z. (2018). Sulfur-containing amino acids: protective role against free radicals and heavy metals. Front. Genet. 25, 324–335. doi: 10.2174/0929867324666170609075434
Cong, Z., Yazhen, S., Changwen, D., Jianmin, Z., Huoyan, W., and Xiaoqin, C. (2010). Evaluation of waterborne coating for controlled-release fertilizer using wurster fluidized bed. Ind. Eng. Chem. Res. 49, 9644–9647. doi: 10.1021/ie101239m
David, M. B., Gentry, L. E., and Mitchell, C. A. (2016). Riverine response of sulfate to declining atmospheric sulfur deposition in agricultural watersheds. J. Environ. Qual. 45, 1313–1319. doi: 10.2134/jeq2015.12.0613
Dawar, K., Shah, F. J., Munir, M. M. R., Alam, I., Khan, S. S., Alam, S., et al. (2021). Biochar and urease inhibitor mitigate NH3 and N2O emissions and improve wheat yield in a urea fertilized alkaline soil. Sci. Rep. 11, 17413. doi: 10.1038/s41598-021-96771-0
Du, C., Zhou, J., and Shaviv, A. (2006). Release characteristics of nutrients from polymer-coated compound controlled release fertilizers. J. Polym. Environ. 14, 223–230. doi: 10.1007/s10924-006-0025-4
Edwards, J. H., and Kamprath, E. J. (1974). Zinc accumulation by corn seedlings as influenced by phosphorus, temperature, and light intensity. Agron. J. 66, 479–482. doi: 10.2134/agronj1974.00021962006600040003x
Elferink, M., and Schierhorn, F. (2016). Global Demand for Food Is Rising. Can We Meet It? Available online at: https://hbr.org/2016/04/global-demand-for-food-is-rising-can-wemeet-it
Emerson, F. C., Rosen, C. J., and Venterea, R. T. (2021). Co-application of DMPSA and NBPT with urea mitigates both nitrous oxide emissions and nitrate leaching during irrigated potato production. J. Environ. Pollut. 284, 117–124. doi: 10.1016/j.envpol.2021.117124
Essa, R., Afifi, A., and Ashry, S. (2021). Influence of sulfur coated urea and algae fertilization on productivity of some leguminous crops in sandy soils. Bull. Natl. Res. Cent. 45, 60. doi: 10.1186/s42269-021-00521-1
Fashola, O., Hayashi, K., and Toshiyuki, W. (2006). Effect of water management and polyolefin-coated urea on growth and nitrogen uptake of indicia rice. J. Plant Nutr. Available online at: https://www.tandfonline.com
Gafoor, I., Rahman, M. H. U., Ali, M., Afzal, M., Ahmad, W., Gaiser, T., et al. (2021). Slow-release nitrogen fertilizers enhance growth, yield, NUE in wheat crop and reduce nitrogen losses under an arid environment. Environ. Sci. Pollut. Res. 28, 43528–43543. doi: 10.1007/s11356-021-13700-4
Gelli, M., Duo, Y., Konda, A. R., Zhang, C., Holding, D., and Dweikat, I. (2014). Identification of differentially expressed genes between sorghum genotypes with contrasting nitrogen stress tolerance by genome-wide transcriptional profiling. BMC Genom. 15, 179. doi: 10.1186/1471-2164-15-179
Gigolashvili, T. S., and Kopriva, S. (2014). Transporters in plant sulfur metabolism. Front. Plant Sci. 5, 442. doi: 10.3389/fpls.2014.00442
Gil-Ortiz, R., Naranjo, M. Á., Ruiz-Navarro, A., Caballero-Molada, M., Atares, S., García, C., et al. (2020). New eco-friendly polymeric-coated urea fertilizers enhanced crop yield in wheat. Agronomy 10, 438. doi: 10.3390/agronomy10030438
Giroto, A. S., Valle, S. F. D., Guimaraes, G. G. F., Jablonowski, N. D., Ribeiro, C., and Mattoso, L. H. C. (2021). Different Zn loading in urea–formaldehyde influences the N controlled release by structure modification. Sci. Rep. 11, 7621. doi: 10.1038/s41598-021-87112-2
Gonzalez, N., Vanhaeren, H., and Inzé, D. (2012). Leaf size control: complex coordination of cell division and expansion. Trends Plant Sci. 17, 332–340. doi: 10.1016/j.tplants.2012.02.003
Gupta, N., Ram, H., and Kumar, B. (2016). Mechanism of zinc absorption in plants: uptake, transport, translocation and accumulation. Rev. Environ. Sci. Bio Technol. 15, 89–109. doi: 10.1007/s11157-016-9390-1
Hai-yan, W., Zhi-feng, C., Zhi-peng, X., Lei, Z., Qiu-yuan, L., Zhen-zhen, Z., et al. (2018). Effects of slow or controlled release fertilizer types and fertilization modes on yield and quality of rice. J. Integ. Agric. 17, 2222–2234. doi: 10.1016/S2095-3119(18)62052-0
Halvorson, A. D., Snyder, C. S., Blaylock, A. D., and Grosso, S. J. (2014). Enhanced-efficiency nitrogen fertilizers: potential role in nitrous oxide emission mitigation. Agron. J. 106, 715–722. doi: 10.2134/agronj2013.0081
Hamadallah, G. M., Prasad, J. D., and Al-Hendi, A. A. (1988). Crop response to sulfur-coated urea (SCU) and other N-carriers in sandy soils of Saudi Arabia. Fertilizer Res. 15, 47–54. doi: 10.1007/BF01049186
Han, W., Huomg, L., and Owojori, O. J. (2019). Foliar application of zinc alleviates the heat stress of pakchoi (Brassica chinensis L.). J. Plant Nutr. 43, 194–213. doi: 10.1080/01904167.2019.1659350
Haseeb-ur-Rehman, Asghar, M. G., Ikram, R. M., Hashim, S., Hussain, S., Irfan, M., et al. (2022). Sulphur coated urea improves morphological and yield characteristics of transplanted rice (Oryza sativa L.) through enhanced nitrogen uptake. J. King Saud Univ. Sci. 34, 101664. doi: 10.1016/j.jksus.2021.101664
Hassan, M., Aamer, M., Umer Chattha, M., Haiying, T., Khan, I., Seleiman, M. F., et al. (2021). Sugarcane distillery spent wash (DSW) as a bio-nutrient supplement: a win-win option for sustainable crop production. Agronomy 11, 183. doi: 10.3390/agronomy11010183
Hassan, M. U., Aamer, M., Nawaz, M., Rehman, A., Aslam, T., Afzal, U., et al. (2021). Agronomic bio-fortification of wheat to combat zinc deficiency in developing countries. Pak. J. Agric. Res. 34, 201–217. doi: 10.17582/journal.pjar/2021/34.1.201.217
Hassan, M. U., Aamer, M., Umer Chattha, M., Haiying, T., Shahzad, B., Barbanti, L., et al. (2020). The critical role of zinc in plants facing the drought stress. Agriculture 10, 396. doi: 10.3390/agriculture10090396
Hassan, M. U., Chattha, M. U., Ullah, A., Khan, I., Qadeer, A., Aamer, M., et al. (2019). Agronomic biofortification to improve productivity and grain Zn concentration of bread wheat. Int. J. Agric. Biol. 21, 615–620. doi: 10.17957/IJAB/15.0936
He, G., Liu, X., and Cui, Z. (2021). Achieving global food security by focusing on nitrogen efficiency potentials and local production. Glob. Food Sec. 29, 100536. doi: 10.1016/j.gfs.2021.100536
Hell, R., Khan, M. S., and Writz, M. (2010). Cellular Biology of Sulfur and Its Functions in Plants. Singapore: Springer, 243–279. doi: 10.1007/978-3-642-10613-2_11
Hooghe, D. P., Escamez, S., and Avice, J. C. (2013). Sulfur limitations provokes physiological and leaf proteome changes in oilseed rape that lead to perturbation sulfur, carbon and oxidative metabolism. BMC Plant Biol. 13, 23. doi: 10.1186/1471-2229-13-23
Hopkins, B. G. (2020). “Developments in the use of fertilizers,” in Achieving Sustainable Crop Nutrition, Vol. 1, Chapter. 26, ed Z. Rengel (Cambridge: Burleigh Dodds Science Publishing). doi: 10.19103/AS.2019.0062.26
Ihsan, M. Z., Duar, I., Alghabari, F., Alzamanan, S., Rizwan, S., Ahmad, M., et al. (2019). Heat stress and plant development: role of sulfur metabolites and management strategies. J. Soil Plant Sci. 69, 332–342. doi: 10.1080/09064710.2019.1569715
Iratkar, A. G., Giri, J. D., Kadam, M. M., Giri, J. N., and Dabhade, M. B. (2014). Distribution of DTPA extractable micronutrients and their relationship with soil properties in soil of parsori watershed of Nagpur district of Maharashtra. Asian J. Soil Sci. 9, 297–299.
Irfan, M., Niazi, M. B. K., Hussain, A., Farooq, W., and Zia, M. H. (2018). Synthesis and characterization of zinc-coated urea fertilizer. J. Plant Nutr. 41, 1625–1635. doi: 10.1080/01904167.2018.1454957
Irshad, M., Wahid, M. A., Saleem, M. F., Khan, S., Irshad, S., Matloob, A., et al. (2021). Zinc coated urea enhanced the growth and quality of rice cultivated under aerobic and anaerobic culture. J. Plant Nutr. 45, 1198–1213. doi: 10.1080/01904167.2021.2005803
Jadon, P., Selladurai, R., Yadav, S. S., Coumar, M. Y., Dotaniya, M. L., Singh, A. K., et al. (2018). Volatilization and leaching losses of nitrogen from different coated urea fertilizers. J. Soil Sci. Plant Nutr. 18, 462–474. doi: 10.4067/S0718-95162018005002903
Janke, C., Moody, P., and Fujinuma, R. (2022). The impact of banding polymer-coated urea on nitrogen availability and distribution in contrasting soils. J. Soil Sci. Plant. Nutr. 1–15. doi: 10.1007/s42729-022-00869-x
Jia, Y., Hu, Z., Ba, Y., and Qi, W. (2021). Application of biochar-coated urea controlled loss of fertilizer nitrogen and increased nitrogen use efficiency. Chem. Biol. Tech. Agric. 8, 3. doi: 10.1186/s40538-020-00205-4
Jinuntuya-Nortman, M., Sutka, R. L., Ostrom, P. H., Gandhi, H., and Ostrom, N. E. (2008). Isotopologue fractionation during microbial reduction of N2O within soil mesocosms as a function of water-filled pore space. Soil Biol. Biochem. 40, 2273–2280. doi: 10.1016/j.soilbio.2008.05.016
Jones, C., and Jacobsen, J. (2005). “Plant nutrition and soil fertility,” in Nutrient Management Module. eds Tracy M. Sterling, Bozeman: Montana State University, 1–11.
Junejo, N., Khanif, M., Dharejo, K., Abdul, A., and Abdul-Hamid, H. (2011). A field evaluation of coated urea with biodegradable. Afr. J. Biotechnol. 10, 19729–19736.
Kaur, H., and Garg, N. (2021). Zinc toxicity in plants: a review. Planta 253, 129. doi: 10.1007/s00425-021-03642-z
Ke, J., He, R., Hou, P., Ding, C., Ding, Y., Wang, S., et al. (2018). Combined controlled-released nitrogen fertilizers and deep placement effects of N leaching, rice yield and N recovery in machine-transplanted rice. Agric. Ecosyst. Environ. 265, 402–412. doi: 10.1016/j.agee.2018.06.023
Khan, A. Z., Ali, B., Afzal, M., Wahab, S., Khalil, S. K., Amin, N., et al. (2015). Effects of sulfur and urease coated controlled release urea on dry matter yield, N uptake and grain quality of rice. J. Anim. Plant Sci. 25, 679–685. Available online at: http://www.thejaps.org.pk/docs/v-25-03/12.pdf
Khan, M. A., Shah, Z., Rab, A., Arif, M., and Shah, T. (2013). Effect of urease and nitrification inhibitors on wheat yield. Sarhad J. Agric. 29, 371–378.
Khan, N. A., Khan, M. I. R., Asgher, M., Fatma, M., Masood, A., and Syeed, S. (2014). Salinity tolerance in plants: revisiting the role of sulfur metabolites. J. Plant Biochem. Physiol. 2, 2. doi: 10.4172/2329-9029.1000120
Khan, R., Gurmani, A. H., Gurmani, A. R., and Zia, M. S. (2006). Effect of boron application on rice yield under wheat–rice system. Int. J. Agric. Biol. 8, 805–808.
Kihara, J., Bolo, P., Kinyua, M., Rurinda, J., and Piikki, K. (2020). Micronutrient deficiencies in African soils and the human nutritional nexus: opportunities with staple crops. Environ. Geochem. Health 42, 3015–3033. doi: 10.1007/s10653-019-00499-w
Klikocka, H., and Cybulska, M. (2014). “Sulfur and nitrogen fertilization of spring wheat,” in Mineral Fertilization of Spring Wheat (Saarbrücken: Saarbrucken LAP Lambert Academic Publishing).
Klikocka, H., and Marks, M. (2018). Sulfur and nitrogen fertilization as a potential means of agronomic biofortification to improve the content and uptake of microelements in spring wheat grain. J. Chem. 2018, 9326820. doi: 10.1155/2018/9326820
Kosesakal, T., and Unal, M. (2009). Role of zinc deficiency in photosynthetic pigments and peroxidase activity of tomato seedlings. J. Biol. 68, 113–120. Available online at: https://www.researchgate.net/publication/236893150
Krajewska, B. (2009). Urease I. Functional, catalytic and kinetic properties: a review. J. Mol. Catal. B Enzym. 59, 9–21. doi: 10.1016/j.molcatb.2009.01.003
Krishna, T. P. A., Maharajan, T., Roch, G. V., Ignacimuthu, S., and Ceasar, S. A. (2020). Structure, function, regulation and phylogenetic relationship of ZIP family transporters of plants. Front. Plant Sci. 11, 662. doi: 10.3389/fpls.2020.00662
Kuang, W., Gao, X., Tenuta, M., Gui, D., and Zeng, F. (2019). Relationship between soil profile accumulation and surface emission of N2O: effects of soil moisture and fertilizer nitrogen. Biol. Fertil. Soils 55, 97–107. doi: 10.1007/s00374-018-01337-4
Kundu, S., Adhikari, T., Coumar, M. V., Rajendiran, S., and Saha, J. K. (2016). Enhancing Nitrogen Use Efficiency and Reducing N2O Emissions by Coating Urea With Newly Identified Bio-Molecule (C20H30O2) Nano-Zinc Oxide and Nano Rock Phosphate. New Delhi: Springer, 89–101. doi: 10.1007/978-981-10-5798-4_9
Léran, S., Varala, K., Boyer, J. C., Chiurazzi, M., Crawford, N., and Daniel-Vedele, F. (2014). A unified nomenclature of nitrate transporter 1/peptide transporter family members in plants. Trends Plant Sci. 19, 5–9. doi: 10.1016/j.tplants.2013.08.008
Li, H., Hu, B., and Chu, C. (2017). Nitrogen use efficiency in crops: Lessons from Arabidopsis and rice. J. Exp. Bot. 68, 2477–2488. doi: 10.1093/jxb/erx101
Li, M. H., Wang, Z. H., Mao, Y., Dai, J., Li, Q., and Zou, C. Q. (2013). Effects of Zn application methods on wheat grain yield and Zn utilization in Zn-deficient soils of dryland. J. Plant Nutr. Fert. 19, 1346–1355. doi: 10.1080/01904169709365267
Li, P., Lin, C., Cheng, H., Duan, X., and Lei, K. (2015). Contamination and health risks of soil heavy metals around a lead/zinc smelter in southwestern China. Ecotoxicol. Environ. Saf. 113, 391–399. doi: 10.1016/j.ecoenv.2014.12.025
Li, W., Xiang, F., Zhong, M., Zhou, L., Liu, H., Li, S., et al. (2017). Transcriptome and metabolite analysis identifies nitrogen utilization genes in tea plant (Camellia sinensis). Sci. Rep. 7, 1693. doi: 10.1038/s41598-017-01949-0
Liao, J., Liu, X., Hu, A., Song, H., Chen, X., and Zhang, Z. (2020). Effects of biochar-based controlled release nitrogen fertilizer on nitrogen-use efficiency of oilseed rape (Brassica napus L.). Nature 10, 11063. doi: 10.1038/s41598-020-67528-y
Likus-Cieślik, J., Pietrzykowski, M., Szostak, M., and Szulczewski, M. (2017). Spatial distribution and concentration of sulfur in relation to vegetation cover and soil properties on a reclaimed sulfur mine site (southern Poland). Environ. Monit. Assess 189, 87. doi: 10.1007/s10661-017-5803-z
Liu, K. H., Fang, Y. T., Yu, F. M., Liu, Q., Li, R. F., and Peng, S. L. (2010). Soil acidification in response to acid deposition in three subtropical forests of subtropical China. Pedosphere 20, 399–408. doi: 10.1016/S1002-0160(10)60029-X
Lu, C., Zhang, X., Chen, X., Shi, Y., Ma, J., Zhao, M., et al. (2010). Fixation of labeled (15NH4)2 SO4 and its subsequent release in black soil of Northeast China over consecutive crop cultivation. Soil Tillage Res. 106, 329–334. doi: 10.1016/j.still.2009.11.009
Luo, Z., Liu, H., Li, W., Zhao, Q., Dai, J., Tian, L., et al. (2018). Effects of reduced nitrogen rate on cotton yield and nitrogen use efficiency as mediated by application mode or plant density. Field Crop Res. San Francisco, 218,150–157. doi: 10.1016/j.fcr.2018.01.003
Madigan, M. T., Martino, J. M., Stahl, D. A., and Clark, D. P. (2010). Brock Biology of Microorganisms. Global 13th Edition. San Francisco, CA: Benjamin Cummings, 670–711
Makino, A. (2003). Rubisco and nitrogen relationships in rice: leaf photosynthesis and plant growth. Soil Sci. Plant Nutr. 49, 319–327. doi: 10.1080/00380768.2003.10410016
Malakouti, M., Bourdi, B., Elah, L., Shahabi, K., Siavashi, R., Vakil, J., et al. (2008). Comparison of complete and sulfur coated urea fertilizers with pre-plant urea in increasing grain yield and nitrogen use efficiency in wheat. J. Agric. Sci. Technol. 10, 173–183. Available online at: https://www.sid.ir/en/journal/viewpaper.aspx?Id=104331
Mathialagan, R. M., Khateeb, N. A., Beshr, M., Shamsuddin, M., and Rashid, M. (2017). Evaluation of allicin as soil urease inhibitor. Proc. Eng. 184, 449-459. doi: 10.1016/j.proeng.2017.04.116
Mazid, M., Khan, Z. H., Qudussi, S., Khan, T. A., and Mohammad, F. (2011). Significance of sulfur nutrition against metal induced oxidative stress in plants. J. Stress Physiol. Biochem. 7, 165–184.
McDonald, G. K., Graham, R. D., Lloyd, J., Lewis, L., Lonergan, P., and Khabaz-Saberi, H. (2000). “Breeding for improved zinc and manganese efficiency in wheat and barley,” in Proceeding of the 10th Australian Agronomy Conference (Glen Osmond, SA: Department of Plant Science, Waite Institute; Hobart).
Mohammed, S. A., Khan, R. A., El-Readi, M. Z., Emwas, A. H., Sioud, S., Poulson, B. G., et al. (2020). Suaeda vermiculata aqueous-ethanolic extract-based mitigation of ccl4-induced hepatotoxicity in rats, and hepg-2 and hepg-2/adr cell-lines-based cytotoxicity evaluations. Plants 9, 1291. doi: 10.3390/plants9101291
Mohanty, S., Swain, C. K., Kumar, A., and Nayak, A. K. (2020). “Nitrogen footprint: a useful indicator of agricultural sustainability,” in Nutrient Dynamics for Sustainable Crop Production (Singapore: Springer), 135–156. doi: 10.1007/978-981-13-8660-2_5
Moosa, A. W., Gashu, D., Broadley, M. R., Dunham, S. J., McGrath, S. P., Bailey, E. H., et al. (2021). The effect of soil properties on zinc lability and solubility in soils of Ethiopia – an isotopic dilution study. Soil 7, 255–268. doi: 10.5194/soil-7-255-2021
Moreira, A., Moraes, L. A., and Reis, A. R. (2018). “The molecular genetics of zinc uptake and utilization efficiency in crop plants,” in Plant Micronutrient Use Efficiency (Amsterdam: Elsevier), 87–108. doi: 10.1016/B978-0-12-812104-7.00006-X
Moreno-Lora, A., and Delgado, A. (2020). Factors determining Zn availability and uptake by plants in soils developed under Mediterranean climate. Geoderma 376, 114509. doi: 10.1016/j.geoderma.2020.114509
Morgan, K. T., Cushman, K. E., and Sato, S. (2009). Release mechanisms for slow-and controlled-release fertilizers and strategies for their use in vegetable production. HortTechnology 19, 10–12. doi: 10.21273/HORTSCI.19.1.10
Muster, T. H., Bradbury, A., Trinchi, A., Cole, I. S., Markley, T., Lau, D., et al. (2011). Effect of weather conditions on concentration of calcium, manganese, zinc, copper and iron in green tea [Camellia sinensis (L) O kuntze] leaves of North-Western India. J. Sci. Food Agric. 67, 341–346.
Nadeem, F., Farooq, M., Ullah, A., Rehman, A., Nawaz, A., and Naveed, M. (2020). Influence of Zn nutrition? Grain quality and grain biofortification of wheat under conventional and conservation rice–wheat cropping systems. Arch. Agron. Soil Sci. 17, 1–6. doi: 10.1080/03650340.2019.1652273
Naiknaware, M. D., Pawar, G. R., and Murumkar, S. B. (2015). Effect of varying levels of boron and sulfur on growth, yield and quality of summer groundnut (Arachis hypogea L.). Int. J. Trop. Agric. 33, 471–474. doi: 10.13140/RG.2.2.31245.67044
Najafian, S., and Zahedifar, M. (2015). Antioxidant activity and essential oil composition of Saturejahortensis L. as influenced by sulfur fertilizer. J. Sci. Food Agric. 95, 2404–2408. doi: 10.1002/jsfa.6959
Nardi, P., Neri, U., Matteo, G. T., Trinchera, A., Napoli, R., Farina, R., et al. (2018). Nitrogen release from slow-release fertilizers in soils with different microbial activities. Pedosphere 28, 332–340. doi: 10.1016/S1002-0160(17)60429-6
Nasima, J., Khanif, M. Y., Wan, W. Y., and Dharejo, K. A. (2010). Maize response to biodegradable polymer and urease inhibitor coated urea. Int. J. Agric. Biol. 12, 773–776.
Naz, M. Y., and Sulaiman, S. A. (2016). Slow release coating remedy for nitrogen loss from conventional urea: a review. J. Control. Release 225, 109–120. doi: 10.1016/j.jconrel.2016.01.037
Naz, M. Y., Sulaiman, S. A., Ariwahjoedi, B., and Shaari, K. Z. K. (2014). Characterization of modified tapioca starch solutions and their sprays for high temperature coating applications. Sci. World J. 2014, 372506. doi: 10.1155/2014/375206
Nazir, Q., Wang, X., Hussain, A., Ditta, A., Aimen, A., Saleem, I., et al. (2021). Variation in growth, physiology, yield, and quality of wheat under the application of different zinc coated formulations. Appl. Sci. 11, 4797. doi: 10.3390/app11114797
Neina, D. (2019). The role of Soil pH in plant nutrition and soil remediation. Appl. Environ. Soil Sci. 2019, 5794869. doi: 10.1155/2019/5794869
Ning, Q., Meiting, S., Lixiang, Z., Chunmei, G., and Jie, W. (2020). Residual effects of soil Zn fertilization on soil characteristics, yield and quality of Platycodon grandiflorum. J. Soil Sci. Plant Nutr. 66, 1715831. doi: 10.1080/00380768.2020.1715831
Osman, M. A. (2017). Effect of polymer-coated urea and ammonium nitrate application on nitrogen behavior in sandy soil and yield productivity. J. Soil Sci. Agric. Eng. 8, 101–113 doi: 10.21608/jssae.2017.37228
Padhi, S. K., Dash, M., and Swain, S. C. (2013). Effect of sulfur dioxide on growth, chlorophyll and sulfur content of tomato. Europ. Sci. J. 9. doi: 10.4038/tare.v16i1.5269
Peck, A. W., and McDonald, G. K. (2010). Adequate zinc nutrition alleviates the adverse effects of heat stress in bread wheat. Plant Soil 337, 355–374. doi: 10.1007/s11104-010-0532-x
Pooniya, V., Shivay, Y., Pal, M., and Bansal, R. (2018). Relative performance of boron, sulfur and zinc coatings onto prilled urea for increasing productivity and nitrogen use efficiency in maize. Exp. Agric. 54, 577–591. doi: 10.1017/S0014479717000254
Prasad, R., and Hobbs, P. R. (2018). “Efficient nitrogen management in the tropics and subtropics,” in Soil Nitrogen Uses and Environmental Impacts, eds R. Prasad and P. R. Hobbs (CRC Press), 191–232. doi: 10.1201/b22044-8
Qiao, D., Liu, H., Yu, L., Bao, X., Simon, G. P., Petinakis, E., et al. (2016). Preparation and characterization of slow-release fertilizer encapsulated by starch-based superabsorbent polymer. Carbohydr. Polym. 147, 146–154. doi: 10.1016/j.carbpol.2016.04.010
Qureshi, K. A., Bholay, A. D., Rai, P. K., Mohammed, H. A., Khan, R. A., Azam, F., et al. (2021). Isolation, characterization, anti-MRSA evaluation, and in-silico multi-target anti-microbial validations of actinomycin X2 and actinomycin D produced by novel Streptomyces smyrnaeus UKAQ_23. Sci. Rep. 11, 14539. doi: 10.1038/s41598-021-93285-7
Raj, A., Chakrabarti, B., Pathak, H., Singh, S. D., and Pratap, D. (2015). Impact of elevated temperature on iron and zinc uptake in rice crop. Int. J. Agric. Environ. Biotechnol. 8, 691–697. doi: 10.5958/2230-732X.2015.00077.7
Rani, K., Sharma, K., Nagasri, K., Srinivas, K., Vishnu-Murthy, T., Shankar, G. M., et al. (2009). Response of sunflower to sources and levels of sulfur under rainfed semi-arid tropical conditions. Commun. Soil Sci Plant Anal. 40, 2926–2944. doi: 10.1080/00103620903175389
Raza, M. A., Feng, L. Y., Manaf, A., Wasaya, A., Ansar, M., Hussain, A., et al. (2018). Sulfur application increases seed yield and oil content in sesame seeds under rainfed conditions. Field Crop. Res. 218, 51–58. doi: 10.1016/j.fcr.2017.12.024
Rehman, A., Nawaz, M., Chattha, M. U., Khan, I., Chattha, M. B., Hussain, F., et al. (2021). Neem coated urea improves the productivity, nitrogen use efficiency and economic return of wheat crop. Int. J. Agric. Biol. 26, 450–460. doi: 10.17957/IJAB/15.1856
Remya, V. R., George, J. S., and Thomas, S. (2021). Controlled Released Fertilizers for Sustainable for Agriculture. Academic Press. 183–194. doi: 10.1016/B978-0-12-819555-0.00011-X
Rengel, Z. (2015). Availability of Mn, Zn and Fe in the rhizosphere. J. Soil Sci. Plant Nutr. 15, 397–409. doi: 10.4067/S0718-95162015005000036
Rengel, Z., and Pearson, J. N. (1997). Genotypic differences in the production and partitioning of carbohydrates in between roots and shoots of wheat grown under zinc and manganese deficiency. Ann. Bot. 80, 803–808. doi: 10.1006/anbo.1997.0523
Rietra, R. P. J. J., Heinen, M., Dimkpa, C. O., and Bindraban, P. S. (2017). Effects of nutrient antagonism and synergism on yield and fertilizer use efficiency. Commun. Soil Sci. Plant Anal. 48, 1895–1920. doi: 10.1080/00103624.2017.1407429
Rudani, K., Patel, V., and Prajapati, V. (2018). The importance of zinc in plant growth-a review. Int. Res. J. Nat. Appl. Sci. 5, 2349–4077.
Rutherford, J. C. (2014). The emerging role of urease as a general microbial virulence factor. PLoS Pathog. 10, e1004062. doi: 10.1371/journal.ppat.1004062
Sadiq, M., Mazhar, U., Shah, G. A., Hassan, Z., Iqbal, Z., Mahmood, I., et al. (2021). Zinc plus biopolymer coating slows nitrogen release, decreases ammonia volatilization from urea and improves sunflower productivity. Polymers 13, 3170. doi: 10.3390/polym13183170
Sahoo, P., Brar, A., and Sharma, S. (2018). Effect of methods of irrigation and sulfur nutrition on seed yield, economic and bio-physical water productivity of two sunflower (Helianthus annuus L.) hybrids. Agric. Water Manag. 206, 158–164. doi: 10.1016/j.agwat.2018.05.009
Saleem, M., Elahi, E., Gandahi, A. W., Bhatti, S. M., Ibrahim, H., and Ali, M. (2019). Effect of sulfur application on growth, oil content and yield of sunflower. Sarhad J. Agric. 35, 1198–1203. doi: 10.17582/journal.sja/2019/35.4.1198.1203
Santos, G. A., Korndorfer, G. H., Pereira, H. S., and Paye, W. (2018). Addition of micronutrients to NPK formulation and initial development of maize plants. Bio. Sci. J. 34, 927–936. doi: 10.14393/BJ-v34n1a2018-36690
Sauvé, S., Hendershot, W., and Allen, H. E. (2000). Solid-solution partitioning of metals in contaminated soils: dependence on pH, total metal burden, and organic matter. Environ. Sci. Technol. 34, 1125–1131. doi: 10.1021/es9907764
Shah, Z., Badshah, S. L., Iqbal, A., Shah, Z., Emwas, A. H., and Jaremko, M. (2022). Investigation of important biochemical compounds from selected freshwater macroalgae and their role in agriculture. Chem. Biol. Technol. Agric. 9, 1–11. doi: 10.1186/s40538-021-00273-0
Shaviv, A. (2001). Improvement of fertilizer efficiency product processing, positioning and application methods. Proc. Int. Fert. Soc. 469, 1–23.
Shigi, N. (2014). Biosynthesis and functions of sulfur modifications in tRNA. Front. Genet. 5, 67. doi: 10.3389/fgene.2014.00067
Shigi, N., Horitant, M., Miyauchi, K., Suzuki, T., and Kurokii, M. (2021). An ancient type of MnmA protein is an iron–sulfur cluster-dependent sulfur transferase for tRNA anticodons. Cold Spring Harbor Lab. Press 26, 240–250. doi: 10.1261/rna.072066.119
Shilpha, S. M., Soumya, T. M., Girijesh, G. K., and Dhananjaya, B. C. (2017). Effect of different natural oil coated urea fertilizers on productivity and nutrient uptake of maize. Int. J. Pure App. Bio. Sci. 5, 807–812. doi: 10.18782/2320-7051.2755
Shivay, S. Y., Pooniya, V., Pal, M., Chand, P., Ghasal, D., Bana, R., et al. (2019). Coated urea materials for improving yields, profitability, and nutrient use efficiencies of aromatic rice. Global Chall. 3, 1900013. doi: 10.1002/gch2.201900013
Shivay, S. Y., Pooniya, V., Prasad, R., Pal, M., and Bansal, R. (2016). Sulfur-coated urea as a source of sulfur and an enhanced efficiency of nitrogen fertilizer for spring wheat. J. Cereal. Res. Commun. 44, 513–523. doi: 10.1556/0806.44.2016.002
Shivay, Y. S., Kumar, D., Prasad, R., and Ahlawat, I. (2008). Relative yield and zinc uptake by rice from zinc sulphate and zinc oxide coatings onto urea. Nutr. Cycl. Agroecosyst. 80, 181–188. doi: 10.1007/s10705-007-9131-5
Shivay, Y. S., and Prasad, R. (2012). Zinc-coated urea improves productivity and quality of basmati rice (Oryza sativa L.) under zinc stress condition. J. Plant Nutr. 35, 928–951. doi: 10.1080/01904167.2012.663444
Shrestha, S., Becker, M., Lamersand, J., and Wimmer, R. (2020). Boron and zinc fertilizer applications are essential in emerging vegetable-based crop rotations in Nepal. J. Plant Nutr. Soil Sci. 183, 439–456. doi: 10.1002/jpln.202000151
Siddiqui, S. N., Umar, S., and Iqbal, M. (2015). Zinc-induced modulation of some biochemical parameters in a high- and a low-zinc-accumulating genotype of Cicer arietinum L. grown under Zn-deficient condition. Protoplasma 252, 1335–1345. doi: 10.1007/s00709-015-0767-8
Silva, G. (2018). Feeding the World in 2050 and Beyond–Part 1: Productivity Challenges. Michigan State University Extension. Available online at: https://www.canr.msu.edu/news/feeding-the-world-in-2050-~and-beyond-part-1
Smolders, E., Buekers, J., Oliver, I., and McLaughlin, M. J. (2004). Soil properties affecting toxicity of zinc to soil microbial properties in laboratory-spiked and field-contaminated soils. Environ. Toxicol. Chem. 23, 2633–2640. doi: 10.1897/04-27
Song, C. Z., Liu, M. Y., Meng, J. F., Chi, M., Xi, Z. M., and Zhang, Z. W. (2015). Promoting effect of foliage sprayed zinc sulfate on accumulation of sugar and phenolic in berries of Vitis vinifera cv. Merlotsingh et al., 201 growing zinc deficient soil. Molecules 20, 2536–2554. doi: 10.3390/molecules20022536
Sud, R. G., Prasad, R., and Bhargava, M. (1995). Effect of weather conditions on concentration of calcium, manganese, zinc, copper and iron in green tea [Camellia sinensis (L) O kuntze] leaves of North-Western India. J. Sci. Food Agric. 67, 341–346. doi: 10.1002/jsfa.2740670311
Suganya, A., Saravanan, A., and Manivannan, N. (2020). Role of zinc nutrition for increasing zinc availability, uptake, yield, and quality of maize (Zea mays L.) grains: an overview. Commun. Soil Sci. Plant Anal. 51, 2001–2021. doi: 10.1080/00103624.2020.1820030
Tatyana, C., Vichene, L. T., and Cauthor, I. (2004). The effect of light intensity on hydrogen production by sulfur-deprived Chlamydomonas reinhardtii. J. Biotecchnol. 114, 143–151. doi: 10.1016/j.jbiotec.2004.05.012
Tavanti, T. R., de Melo, A. A. R., Moreira, L. D. K., Sanchez, D. E. J., dos Santos Silva, R., da Silva, R. M., et al. (2021). Micronutrient fertilization enhances ROS scavenging system for alleviation of abiotic stresses in plants. Plant Physiol. Biochem. 160, 386–396. doi: 10.1016/j.plaphy.2021.01.040
Timilsena, Y. P., Adhikari, R., Casey, P., Muster, T., Gill, H., and Adhikari, B. (2015). Enhanced efficiency fertilisers: a review of formulation and nutrient release patterns. J. Sci. Food Agric. 95, 1131–1142. doi: 10.1002/jsfa.6812
Tiong, J., Sharma, N., Sampath, R., MacKenzie, N., Watanabe, S., Metot, C., et al. (2021). Improving nitrogen use efficiency through overexpression of alanine aminotransferase in rice, wheat, and barley. Front. Plant Sci. 12, 628521. doi: 10.3389/fpls.2021.628521
Trenkel, M. E. (2010). Slow-and Controlled-Release and Stabilized Fertilizers: An Option for Enhancing Nutrient Use Efficiency in Agriculture. Paris: International Fertilizer Industry Association.
Ullah, A., Farooq, M., and Hussain, M. (2019). Improving the productivity, profitability and grain quality of kabuli chickpea with co-application of zinc and endophyte bacteria Enterobacter sp. MN17. Arch. Agron. Soil. Sci. 25, 1–6. doi: 10.1080/03650340.2019.1644501
Ullah, A., Munir, S., Badshah, S. L., Khan, N., Ghani, L., Poulson, B. G., et al. (2020). Important flavonoids and their role as a therapeutic agent. Molecules 25, 5243. doi: 10.3390/molecules25225243
Vadlamodi, K., Upadhyay, H., Singh, A., and Reddy, M. (2020). Influence of zinc application in plant growth: an overview. Eur. J. Mol. Clin. Med. 7, 2515–8260.
Wakeel, A., Farooq, M., Bashir, K., and Ozturk, L. (2018). “Micronutrient malnutrition and biofortification: recent advances and future perspectives,” in Plant Micronutrient Use Efficiency: Molecular and Genomic Perspectives in Crop Plants, eds M. A. Hossain, T. Kamiya, D. J. Burritt, L. -S. P. Tran, and T. Fujiwara (New York, NY; London: Elsevier), 225–243. doi: 10.1016/B978-0-12-812104-7.00017-4
Wan, Y., King, R., Mitchell, R. A. C., HASSANi-Pak, K., and Hawkesford, M. J. (2017). Spatiotemporal expression patterns of wheat amino acid transporters reveal their putative roles in nitrogen transport and responses to abiotic stress. Sci. Rep. 7, 5461–5474. doi: 10.1038/s41598-017-04473-3
Wang, C., Zhang, L., Li, Y., Buttar, Z. A., Wang, N., Xie, Y., et al. (2020). Single nucleotide mutagenesis of the TaCHLI gene suppressed chlorophyll and fatty acid biosynthesis in common wheat seedlings. Front. Plant Sci. 11, 97. doi: 10.3389/fpls.2020.00097
Wang, H., Hegazy, A. M., Jiang, X., Hu, Z., Lu, J., Mu, J., et al. (2016). Suppression of ammonia volatilization from rice–wheat rotation fields amended with controlled-release urea and urea. Agron. J. 108, 1214–1224. doi: 10.2134/agronj2015.0295
Wang, S., Zhao, X., Xing, G., Yang, Y., and Zhang, M. (2015). Improving grain yield and reducing N loss using polymer-coated urea in southeast China. Springer 35, 1103–1115. doi: 10.1007/s13593-015-0300-7
Wang, Y. Y., Wei, Y. Y., Dong, X. L., Lu, Y., Feng, L. L., Zhang, J., et al. (2014). Improved yield and Zn accumulation for rice grain by Zn fertilization and optimized water management. J. Zhejiang Uni. Sci. B. 15, 365–374. doi: 10.1631/jzus.B1300263
Wei, X., Chen, J., Gao, B., and Wang, Z. (2020). “Role of controlled and slow release fertilizers in fruit crop nutrition,” in Diagnosis and Management of Nutrient Constraints, eds A. K. Srivastava and C. Hu (Amsterdam: Elsevier), 555–566. doi: 10.1016/B978-0-12-818732-6.00039-3
White, P. J., Bowen, H. C., Parmaguru, P., Fritz, M., Spracklen, W. P., and Spiby, R. E. (2004). Interactions between selenium and sulfur nutrition in Arabidopsis thaliana. J. Exp. Bot. 55, 1927–1937. doi: 10.1093/jxb/erh192
Xin, Y., Wenhai, M., Shaofu, W., Lianghuan, W., and Jianqiu, C. (2017). Rice response to single application of coated urea on yield, dry matter accumulation and nitrogen uptake in Southern China. J. Plant Nutr. 40, 2181–2191. doi: 10.1080/01904167.2017.1346675
Yang, K. Y., Doxey, S., McLean, J. E., Britt, D., Watson, A., and Al Qassy, D. (2018). Remodeling of root morphology by CuO and ZnO nanoparticles: effects on drought tolerance for plants colonized by a beneficial pseudomonad. Botany 96, 175–186. doi: 10.1139/cjb-2017-0124
Yang, M., Fang, Y., Sun, D., and Shi, Y. (2016). Efficiency of two nitrification inhibitors (dicyandiamide and 3, 4-dimethypyrazole phosphate) on soil nitrogen transformations and plant productivity: a meta-analysis. Sci. Rep. 6, 22075. doi: 10.1038/srep22075
Yu, Z., Juhasz, A., Islam, S., Diepeveen, D., Zhang, J., Wang, P., et al. (2018). Impact of mid-season sulphur deficiency on wheat nitrogen metabolism and biosynthesis of grain protein. Sci. Rep. 8, 2499. doi: 10.1038/s41598-018-20935-8
Zenda, T., Songtao, L., Anyi, D., and Huijun, D. (2021). Revisiting sulphur—the once neglected nutrient: it's roles in plant growth, metabolism. Stress Toler. Crop Prod. Agric. 11, 626. doi: 10.3390/agriculture11070626
Zhang, Y., Luan, S., Chen, L., and Shao, M. (2011). Estimating the volatilization of ammonia from synthetic nitrogenous fertilizers used in China. J. Environ. Manage. 92, 480–493. doi: 10.1016/j.jenvman.2010.09.018
Zhao, C., Degryse, F., Gupta, V., and McLaughlin, M. J. (2016). Low effective surface area explains slow oxidation of co-granulated elemental sulfur. Soil Sci Soc. Am. J. 80, 911–918. doi: 10.2136/sssaj2015.09.0337
Zhao, C., Degryse, F., Gupta, V. V. S. R., and McLaughlin, M. J. (2015). Elemental sulfur oxidation in Australian cropping soils. Soil Sci. Soc. Am. J. 79, 89–96. doi: 10.2136/sssaj2014.08.0314
Zhao, C. H., Tian, Q., Zhang, V. V. S. R., and Liu, Z. (2021). Preparation of urea-containing starch-castor oil superabsorbent polyurethane coated urea and investigation of controlled nitrogen release. Carbohydr. Polym. 253, 117240. doi: 10.1016/j.carbpol.2020.117240
Zhou, Y., Tao, Y., Tang, D., Wang, J., Zhong, J., Wang, Y., et al. (2017). Identification of QTLs associated with nitrogen uptake and nitrogen use efficiency using high throughput genotyped CSSLs in rice (Oryza sativa L.). Front Plant Sci. 8, 1166. doi: 10.3389/fpls.2017.01166
Keywords: coated urea, growth, photosynthesis, nitrogen use effiency, nitrogen loss
Citation: Mustafa A, Athar F, Khan I, Chattha MU, Nawaz M, Shah AN, Mahmood A, Batool M, Aslam MT, Jaremko M, Abdelsalam NR, Ghareeb RY and Hassan MU (2022) Improving crop productivity and nitrogen use efficiency using sulfur and zinc-coated urea: A review. Front. Plant Sci. 13:942384. doi: 10.3389/fpls.2022.942384
Received: 12 May 2022; Accepted: 27 July 2022;
Published: 14 October 2022.
Edited by:
Hamada AbdElgawad, Beni-Suef University, EgyptReviewed by:
Jayanta Kumar Patra, Dongguk University Seoul, South KoreaEssam Esmail Kandil, Alexandria University, Egypt
Copyright © 2022 Mustafa, Athar, Khan, Chattha, Nawaz, Shah, Mahmood, Batool, Aslam, Jaremko, Abdelsalam, Ghareeb and Hassan. This is an open-access article distributed under the terms of the Creative Commons Attribution License (CC BY). The use, distribution or reproduction in other forums is permitted, provided the original author(s) and the copyright owner(s) are credited and that the original publication in this journal is cited, in accordance with accepted academic practice. No use, distribution or reproduction is permitted which does not comply with these terms.
*Correspondence: Adnan Noor Shah, ans.786@yahoo.com; Muhammad Umair Hassan, muhassanuaf@gmail.com