- 1Indian Council of Agricultural Research (ICAR)-National Bureau of Plant Genetic Resources Regional Station, Shimla, India
- 2International Centre for Agricultural Research in Dry Areas (ICARDA)-Food Legume Research Platform, Bhopal, India
- 3Indian Council of Agricultural Research (ICAR)-Central Potato Research Institute, Shimla, India
- 4Department of Plant Breeding and Genetics, Punjab Agricultural University, Ludhiana, India
- 5Department of Plant Breeding and Genetics, Sher-e-Kashmir University of Agricultural Sciences and Technology, Jammu, India
- 6Indian Council of Agricultural Research (ICAR)-National Bureau of Plant Genetic Resources, New Delhi, India
The wild Cicer species is well-known for having climate-resilient and productivity-enhancing traits of interest. Therefore, wide hybridization could be used as a realistic strategy for introgressing prospective traits from wild species into the cultivated gene pool. The present study was, thus, undertaken to evaluate F7 chickpea interspecific derivatives derived from Cicer reticulatum Ladiz. and C. echinospermum P. H. Davis wild annual Cicer species. As a result, a set of six interspecific crosses were advanced using the single seed descent (SSD) method of breeding. The F7 generation of these crosses was assessed in two diverse agro-ecological regions of India. The data revealed a wide range of variation with respect to seed yield and its important component traits, which resulted in the identification of the most promising derivatives carrying desirable characters as indicated by range, mean, and coefficient of variation. Further, fruitful heterosis was also estimated as promising selection criteria for identifying superior lines for earliness and high seed yield, including resistance against prevailing stresses (ascochyta blight, botrytis gray mold, dry root rot, and fusarium wilt). The superior derivatives carrying putative characters could be recommended for further breeding and selection of genetic materials for developing suitable genotypes.
Introduction
Chickpea (Cicer arietinum L.) is a true diploid (2n = 2x = 16) annual food legume species having a genome size of ~738 Mbp (Varshney et al., 2012). The domesticated species has evolved from its immediate wild progenitor C. reticulatum Ladiz. through the natural selection process. The genus Cicer consists of 9 annual and 35 perennial species (van der Maesen, 1987). Recently, Toker et al. (2021) introduced one more wild annual species C. turcicum Toker, Berger and Gokturk, thereby increasing the count to 10 annual species in the existing gene pool. It is a winter-season legume crop that thrives at temperatures between 20 and 25°C during the day and 15–20°C at night temperatures, thereby performing well in drier weather conditions (Saraf et al., 1998). In the last two decades (1995–2016), the global chickpea area has expanded by 8%, grain yield by 27%, and overall production by 40% (Food and Agriculture Organization, 2020). India, being the world's largest producer of chickpea, accounts for ~70% of total annual pulse production of ~12 million tons, which was harvested from ~11 million ha, which is far less than its true potential when compared to other major crops (Dixit, 2018). The main causes of such trivial gains are a variety of stresses that limit yield and stability (Siddique et al., 2000; Varshney et al., 2012). Furthermore, the non-availability of genetically improved crop varieties remains a serious concern in achieving desired production levels even in highly productive environments (Chaturvedi and Nadarajan, 2010). A narrow gene pool, due to its single domestication event and a high rate of self-pollination, is another constraint in breeding cultivated chickpea (Abbo et al., 2003, 2005). However, resistant sources to major biotic and abiotic stresses, including yield-related traits, are often not available within cultivated germplasm due to the domestication bottleneck, which has bifurcated the interest of chickpea researchers to use crop wild relatives (CWRs) for its genetic improvement (Croser et al., 2003; Mallikarjuna et al., 2007; Bakir et al., 2021; Vance et al., 2021; Sari et al., 2022). In the past, wild progenitors have been introgressed as potential donors of productivity-enhancement related characters in some other crop species, such as rice (Xiao et al., 1996) and tomato (Tanksley and McCouch, 1996). Therefore, to attain further breakthroughs for improving yield and stability in future crop varieties, new traits of interest must be incorporated into the cultivated background of chickpea. Moreover, it is also imperative to accumulate other complimentary genes and alleles from wild relatives to diversify the cultivated gene pool and maximize genetic gains from selection (Vega and Frey, 1980). The wild Cicer species consists of target characters for distinct morphological features and resistance against biotic and abiotic stresses (Robertson et al., 1997; Singh and Ocampo, 1997; Singh et al., 2005, 2014, 2021; Sandhu et al., 2006; Talip et al., 2018; Toker et al., 2021). As far as their crossability with cultivated chickpea is concerned, several cross-combination studies involving C. reticulatum and C. echinospermum have exhibited higher variability for important yield-related and stress-resistant traits (Koseoglu et al., 2017; Singh et al., 2018). So, an immediate thrust is required to broaden the genetic base of domesticated chickpea cultivars using the introgression of wild Cicer species. Consequently, the multi-location evaluation of interspecific derivatives (IDs) will aid in the identification of promising and stable recombinants across environments (Rakshit et al., 2012). It would also help in the identification of an optimal environment through which the stability of improved progenies could be assessed. Hence, the present study was carried out to evaluate and identify potential interspecific derivatives of chickpea for yield and its important component traits, including major biotic stress factors under two agro-ecological regions of India.
Materials and methods
Genetic materials, population development, and testing
The genetic materials included in the present study were three cultivated chickpea varieties Pusa372, PBG5, and BGD72 of C. arietinum, selected as recipients with two wild annual Cicer species, ILWC229 (EC720438) of C. reticulatum and ILWC246 (EC720481) of C. echinospermum, which were chosen as donor parents for interspecific hybridization. These wild annual Cicer species were selected on the basis of their resistance against ascochyta blight [Ascochyta rabiei (Pass.) Labr.], and botrytis gray mold (Botrytis cinerea Pers. ex. Fr.), including a high number of seeds plant−1 (Singh et al., 2014, 2018). These wild accessions have also been tested for their resistance against fusarium wilt [Fusarium oxysporum f. sp. ciceris (Padwick) Matuo and K. Sato] and dry root rot [Rhizoctonia bataticola (Taub.) E.J. Butler] (unpublished results). The wide hybridization experiments were undertaken at Indian Council of Agricultural Research (ICAR)-National Bureau of Plant Genetic Resources, Pusa New Delhi (28° 35′ N′, 70° 18′ E, 226 m amsl) and the Mountain Agricultural Research and Extension Center (MAREC) of Chaudhary Sarwan Kumar, Himachal Pradesh Krishi Vishvavidyalaya, Sangla (31° 55′ and 32° 20′ N and 77° 00′ and 79° 50′ E, 2758 m amsl), Himachal Pradesh, India during the winter season of 2012-13 and summer 2013. As a result, a total of six interspecific cross-combinations of Pusa372 × EC720438, PBG5 × EC720438, BGD72 × EC720438, BGD72 × EC720481, PBG5 × EC720481, and Pusa372 × EC720481 were successfully obtained as F1 hybrid seeds. Subsequently, the hybridity of true-to-type interspecific crosses were confirmed using distinct morphological and molecular markers (unpublished results). From F2 onward, value-added progenies of these interspecific derivatives (IDs) were advanced upto the F7 stage through the single seed descent (SSD) method of breeding (Goulden, 1939). Further, during the winter season of 2019–2020, the F7 IDs of Pusa372 × EC720438, PBG5 × EC720438, BGD72 × EC720438, BGD72 × EC720481, PBG5 × EC720481, and Pusa372 × EC720481 were planted in an experimental research farm of two different agro-ecological locations viz. International Center for Agricultural Research in Dry Areas (ICARDA) Pulse Research Platform, Bhopal (23° 10′N, 76° 88'E, 498 m amsl) and Punjab Agricultural University (PAU), Ludhiana (30° 54′N, 75° 48′E, 247 m amsl) of India. The agro-climatic description of each location is also given in Table 1. The experiments were undertaken in Augmented Block Design (Federer, 1956), and the performance was compared with regional and local checks of respective locations (susceptible JG62 and resistant PBG7 checks at Ludhiana, India, and resistant checks JG14, BG3043, and RVG202 at Bhopal, India). Seeds of each ID were sown in 3-m long rows spaced at 10 cm (plant to plant) and 40 cm (row to row) apart. One pre-sowing irrigation was also given to ensure satisfactory seed germination. Furthermore, recommended agronomic practices were followed for raising the chickpea genetic materials. During the whole cropping season, 2 natural rains were also experienced and hence the necessity of manual irrigation was not felt. The observations were recorded on five competitive plants from each regional and local check and 15 plants from each F7 IDs of all six crosses. The agro-morphological data were taken on days to 50% flowering (DF), days to 80% maturity (DM), plant height (cm) (PH), number of branches plant−1 (NBPP), number of seeds pod−1 (NSPP), 100-seed weight (g) (SW), seed yield plant−1 (g) (SYPP), and biological yield plant−1 (g) (BYPP). Fruitful heterosis was also estimated following Koseoglu et al. (2017) as HF (%) = [(F7-BP)/BP] × 100, where, F7 is 7th generation of IDs and BP is the mean of the better parent.

Table 1. Agro-climatic description of the Indian locations where chickpea interspecific derivatives were evaluated during the study period of winter 2019–2020 and 2020–2021.
Screening against major biotic stresses
Ascochyta blight (A. rabiei)
Disease reaction of chickpea interspecific derivatives to ascochyta blight was organized at the Experimental Farm of Punjab Agricultural University, Ludhiana, India (30° 54'N, 75° 48′E, 247 m amsl) during the winter season of 2019–2020. All derivatives belonging to six interspecific crosses were planted in 3-m long rows spaced at 10 cm (plant to plant) and 40 cm (row to row) apart. All plant populations were artificially inoculated by frequently spraying with ascosporic suspension (1 × 106 spores ml−1) using local isolate of A. rabiei. The observations were recorded on terminal disease reaction at the vegetative and reproductive plant stages on a rating scale of 1–9, as suggested by Pande et al. (2010). Based on disease screening, the interspecific derivatives were categorized for their reaction to pathogen infection as: 1 = asymptomatic (Free), 3 = resistant (R), 5 = moderately resistant (MR), 7 = susceptible (S), and 9 = highly susceptible (HS).
Botrytis gray mold (B. cinerea)
Botrytis gray mold (BGM) is a devastating pathogen of chickpea, especially in those regions where warm, cloudy, and humid weather situation persists. Several epidemics of BGM causing whole crop loss in chickpea growing in the areas of northwestern India have been reported (Singh et al., 1991). All the interspecific derivatives were screened at Ludhiana, India using cut-twig screening technique, in which water was used as supportive media. Three to four twigs of a single line from each derivative were taken and placed in a test tube having fresh tap water. Subsequently, twigs were inoculated by spraying spore suspension of B. cinerea (10,000 spores ml−1) and covered with moist chambers for 144 h. An incubation period of 8 h dark and 16 h light was provided with fluorescent lamps [60 by 3.75 cm (24 × 1.5 in.); 20 W, 32 lumens W−1]. Further, observations on disease incidence against BGM were assessed using a 1–9 rating scale (Singh et al., 1991) after 6 days of inoculation where: 1 = asymptomatic (Free), 3 = resistant (R), 5 = moderately resistant (MR), 7 = susceptible (S), and 9 = highly susceptible (HS).
Dry root rot [R. bataticola (Taub.)]
Dry root rot is more dominant when the crop is exposed to drought stress (Rai et al., 2022). The symptoms of this pathogen are obvious during the post-flowering stage, leading to drooping and chlorosis of petioles and leaves as well. The leaves and stems of affected plants are generally straw-colored, and in some cases, the lower leaves and stems show brown discoloration and the tap root becomes dark. For screening of chickpea interspecific derivatives at Bhopal, India (23° 10′N, 76° 88′E, 498 m amsl), inoculated seedlings were observed and the data on disease severity was recorded using a 1–9 rating scale developed by Nene et al. (1991), where 1 = asymptomatic (Free), 3 = resistant (R), 5 = moderately resistant (MR), 7 = susceptible (S), and 9 = highly susceptible (HS).
Fusarium wilt (F. oxysporum)
Fusarium wilt is another devastating disease of chickpea, and the selection of highly resistant interspecific derivatives among enhanced progenies is the prime concern of this study. All F7 derivatives belonging to six wide crosses were screened under real field conditions at Bhopal, India. However, resistant and susceptible checks were also repeated after 20 rows of each replication. Observations on disease incidence were recorded using 1–9 rating scale (Nene and Haware, 1980) as: 1 = asymptomic (Free); 3 = resistant (R); 5 = moderately resistant (MR); 7 = susceptible (S); 9 = highly susceptible (HS). Wilt incidence percentage was also observed during the flowering and pod filling stages, as described by Biswas and Jubayer Ali (2017).
Statistical analyses
ANOVA for augmented design was carried out using R package “augmented RCBD” (Aravind et al., 2020). The linear mixed models were implemented in lmer from package lme4 of R using REML to calculate BLUEs and BLUPs and estimates of the variance components (R Core Team, 2018). The adjusted means of all the quantitative traits were used for the estimation of principal component analysis, cluster analysis, and correlations. PCA and correlation studies were done using ggplot and cor function in R, respectively. The R package “corrplot” was used for the depiction of correlation plots. The phenotypic and genotypic coefficients of variation (PCV and GCV) for different traits were calculated as PCV = √VP/ mean × 100, GCV = √VG/mean × 100 as per Burton (1952). Heritability (bs) was estimated as h2 (ns) = √A/VP × 100 as per Lush (1940). The expected genetic advance was calculated as EGA = k × VG/VP × √VP, as per the procedure of Johnson et al. (1955), where k = 2.06 (standard value assumed at 5% selection intensity), VG is the genotypic variance, and VP is the phenotypic variance. However, fruitful heterosis (HF) coined by Koseoglu et al. (2017) was also estimated over better parent (BP) for identifying superior derivatives in F7 generation as: HF (%) = [(F7-BP)/BP] × 100%, where, BP is the mean value of the better parent of a particular cross.
Results
Agro-morphological evaluation
Linear mixed model analysis showed significant differences among genotypes for all the traits, except days to maturity and number of seeds pod−1. The combined analysis of variance indicated significant variation for block, treatment, and interaction among test entries for days to flowering, days to maturity, the number of branches plant−1, plant height, and seed yield plant−1 at Bhopal, India (Table 2, Supplementary Table 1). Likewise, it was also significant for all the characters in Ludhiana, India (Table 2; Supplementary Table 1). However, the summary of descriptive statistical parameters revealed a wide range of variation with respect to important agro-morphological characters as also manifested by the range of variation. The distribution of genetic materials was highly skewed and significant for all the characters at both locations (Table 3). Further, the F7 interspecific derivatives of cross Pusa372 × EC720438, PBG5 × EC720438, BGD72 × EC720438, BGD72 × EC720481, PBG5 × EC720481, and Pusa372 × EC720481 exhibited a wide range of variation, as also reflected by the range, mean, and coefficient of variation (Table 4). The results showed sufficient variability among enhanced progenies of interspecific derivatives developed from C. reticulatum and C. echinospermum species. In general, days to flowering and maturity exhibited variation in two locations as the genetic materials flowered and matured early in Bhopal, India than Ludhiana, India. The maximum average plant height was observed in the cross of BGD72 × EC720481 followed by PBG5 × EC720481, PBG5 × EC720438, and minimum in cross Pusa372 × EC720438 and Pusa372 × EC720481 at both the locations (Table 4). As far as the number of seeds pod−1 is concerned, it was reported as an average of one to two seeds pod−1. The trait 100-seed weight revealed substantial variation in different interspecific derivatives and maximum 100-seed weight was reported in the cross of BGD72 × EC720438 and minimum in Pusa372 × EC720438 at both the locations (Table 4). The seed yield plant−1, being a very important economic trait of interest, also manifested variation ranging from the cross PBG5 × EC720438 (15.30 g) to Pusa372 × EC720438 (18.91 g) in Bhopal, India. Likewise, cross PBG5 × EC720481 and BGD72 × EC720481 exhibited maximum seed yield plant−1 in Ludhiana, India. In general, the extent of genetic parameters indicated that the magnitude of phenotypic variances and coefficient of variation was higher than genotypic variances for the majority of the traits (Table 5). Likewise, heritability was higher for all the agro-morphological characters, but comparatively genetic advance along with gain as a percentage of mean was low in magnitude. The summary of identifying promising interspecific derivatives for important agro-morphological traits has been depicted in Figure 1.
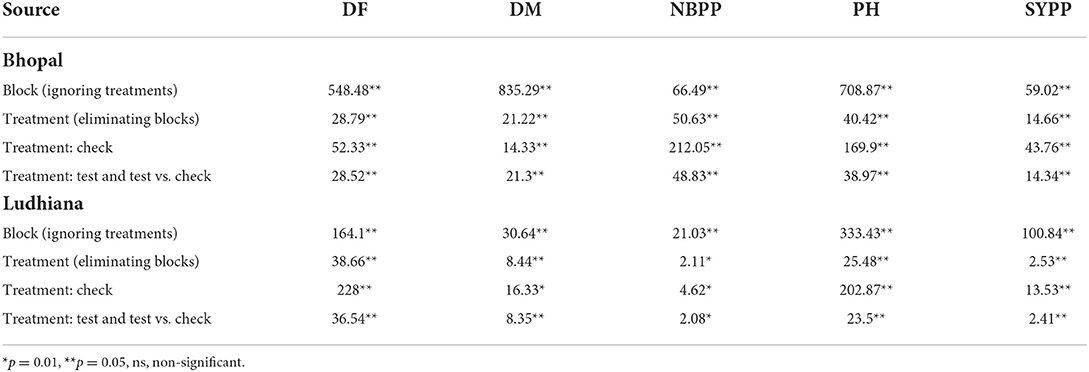
Table 2. Analysis of variance of chickpea interspecific derivatives for seed yield and its important component traits at Bhopal and Ludhiana, India.
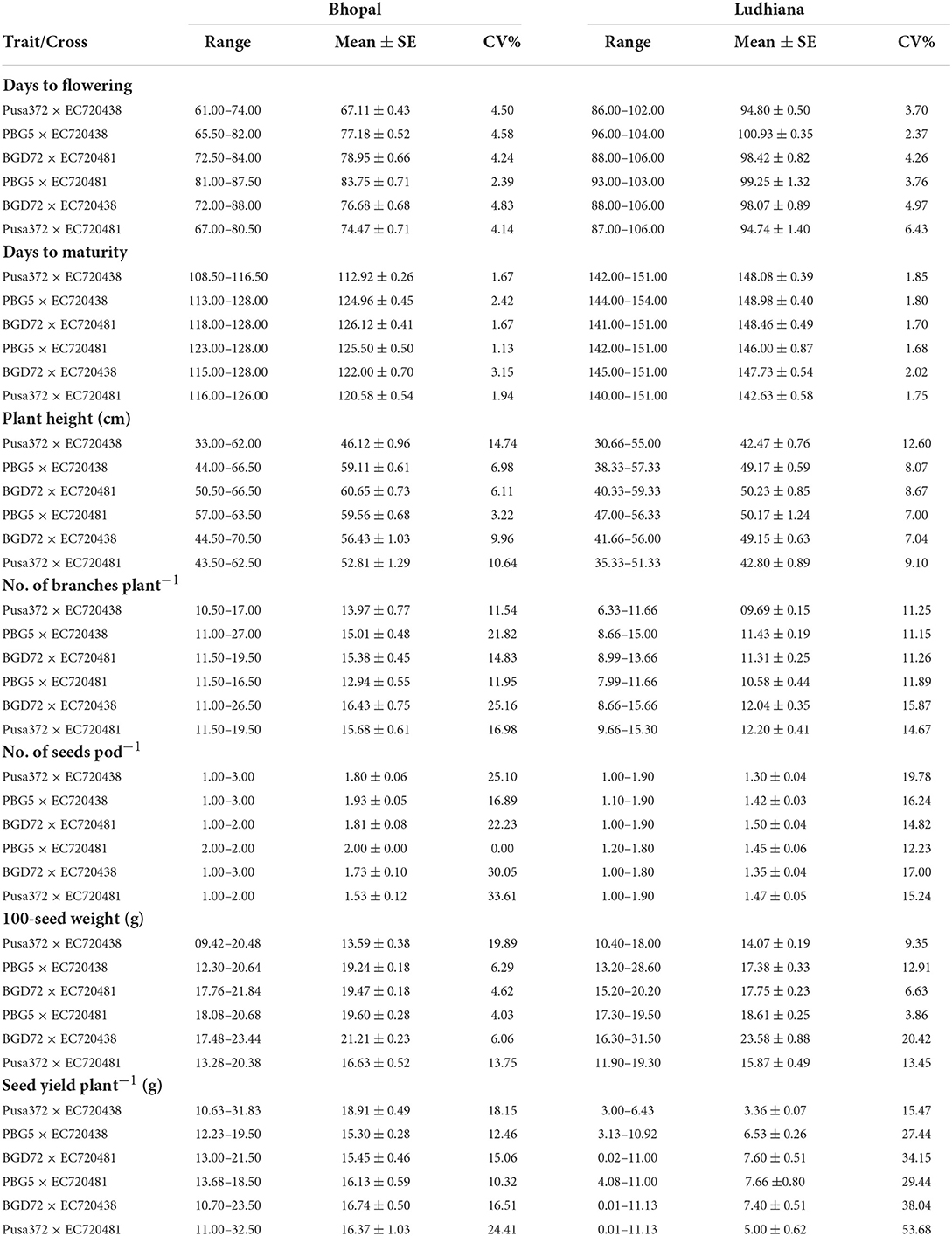
Table 4. Range, mean, standard error, and coefficient of variation of agro-morphological traits in F7 generation of chickpea interspecific derivatives at Bhopal and Ludhiana, India.
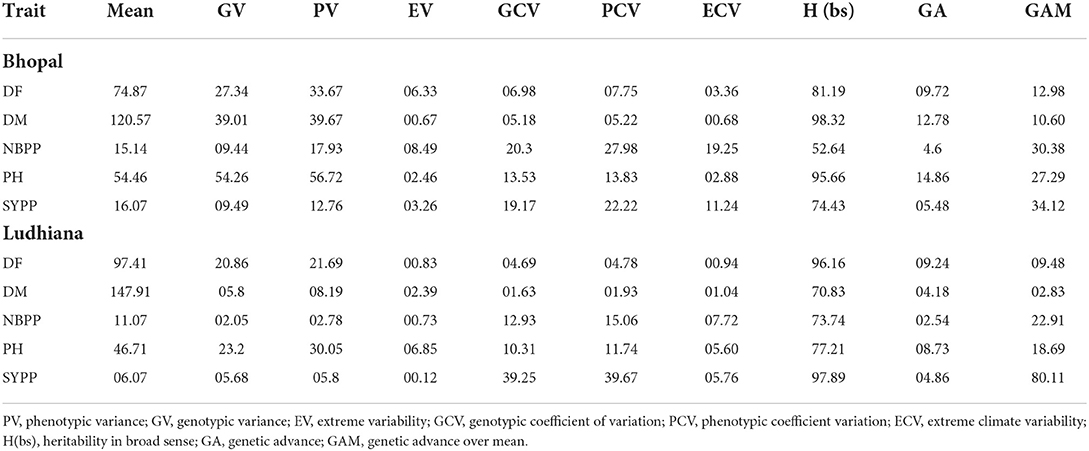
Table 5. Mean, variance, phenotypic, genotypic, and environmental coefficient of variance, heritability, genetic advance, and genetic advance as percent of mean for important traits.
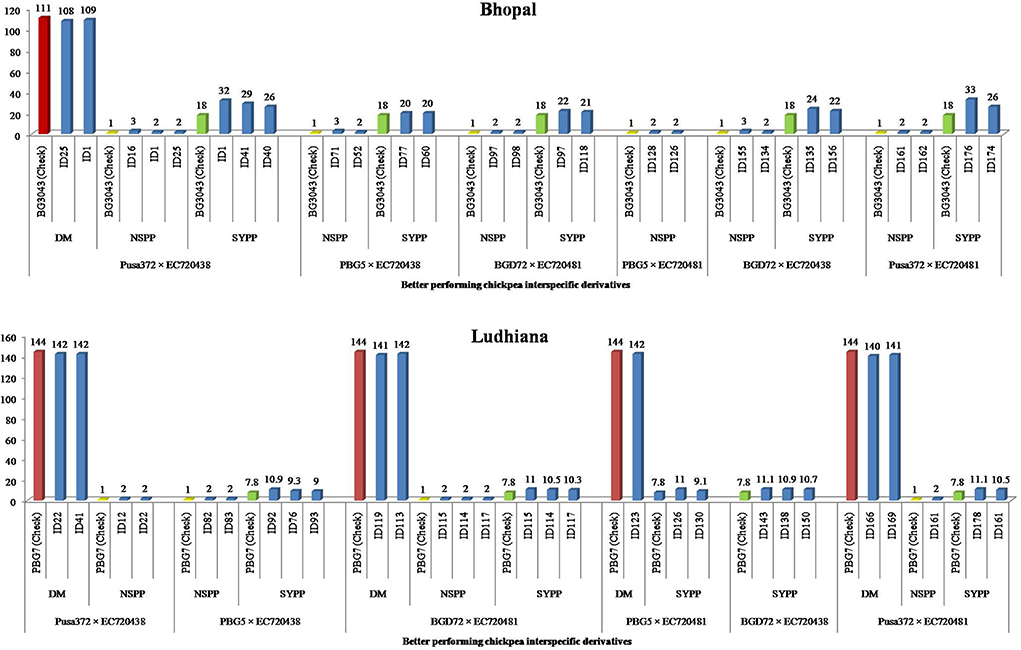
Figure 1. Performance of chickpea interspecific derivatives among different crosses and checks for major agro-morphological traits under two agro-ecological conditions of India. DM, days to maturity; NSPP, number of seeds pod−1; SYPP, seed yield plant−1.
Estimation of fruitful heterosis (%)
The nature and magnitude of fruitful heterosis (%) of F7 interspecific derivatives were also estimated for days to maturity, plant height, number of seeds pod−1, and seed yield plant−1 at both locations (Table 6). The extent of fruitful heterosis was calculated using the percentage of deviation of interspecific derivatives from a better parent (BP). At Bhopal, India, fruitful heterosis means performance ranged from −0.35% (Pusa372 × EC720438) to 12.33% (PBG5 × EC720481) for days to maturity. Likewise, it ranged from 0.31% (BGD72 × EC720481) to 2.04% (PBG5 × EC720438) for the same traits in Ludhiana, India. However, an average mean performance for plant height varied from −14.20% (Pusa372 × EC720438) to 16.32% (BGD72 × EC720481) in Bhopal, India, and it ranged from −6.95% (Pusa372 × EC720481) to 22.62% (PBG5 × EC720438) in Ludhiana, India. The estimation of fruitful heterosis for number of seeds pod−1 varied from 52.63% (Pusa372 × EC720481) to 100.00% (PBG5 × EC720481) in Bhopal, India and it varied from −35.56% (BGD72 × EC720438) to −11.99% (BGD72 × EC720481) in Ludhiana, India. There was a substantial variation for seed yield plant−1 that ranged from −46.75% (Pusa372 × EC720481) to −8.89% (Pusa372 × EC720438) in Bhopal, India, and it varied −55.50% (Pusa372 × EC720438) to 17.17% (BGD72 × EC720438) in Ludhiana, India.
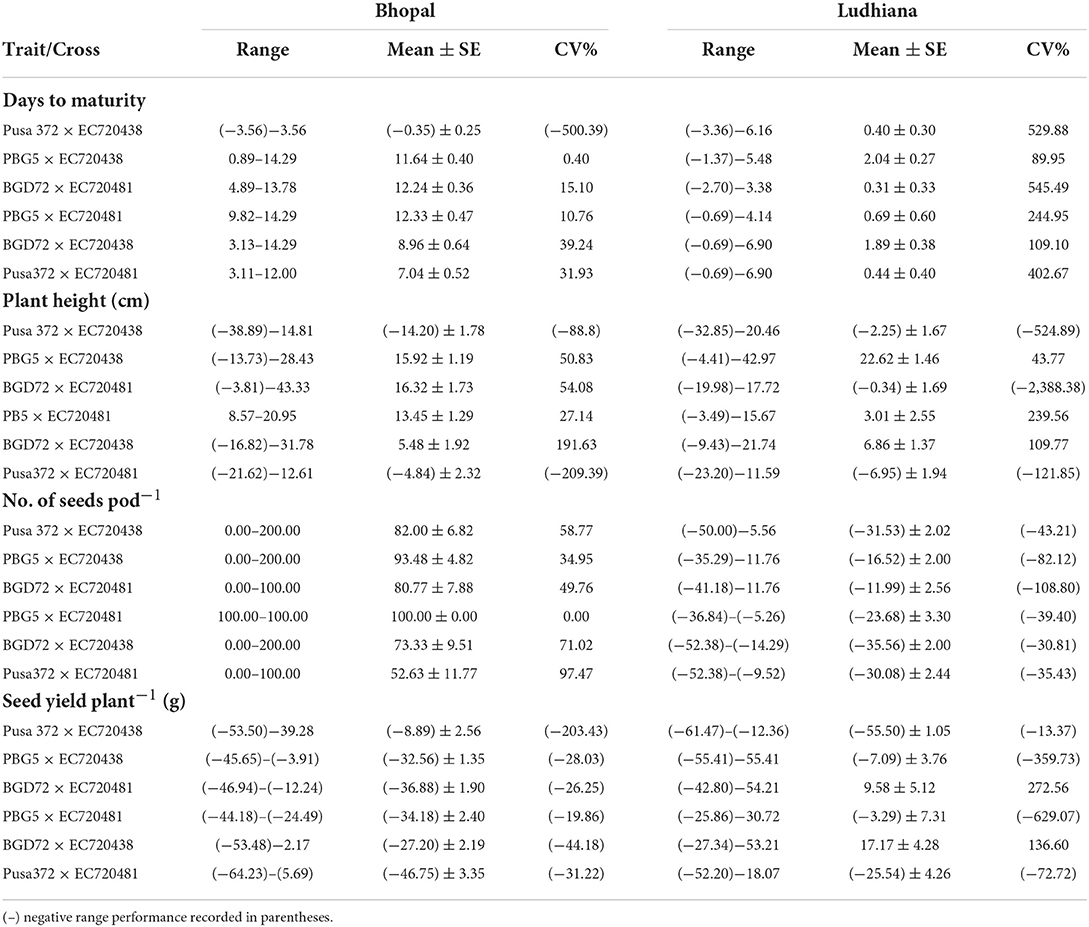
Table 6. Estimates of fruitful heterosis (%) in F7 wide cross populations for agro-morphological traits.
Adjusted mean box plots
The results on adjusted mean box plot performance exhibited that the chickpea interspecific derivatives exhibited a high degree of variation for days to flowering, days to maturity, and plant height, while the number of branches plant−1, 100-seed weight, and seed yield plant−1 showed lesser variations in Bhopal, India. The trend was similar in Ludhiana, India but the range of variation in data was tight, i.e., the variation in data was low in comparison to Bhopal, India (Figure 2).
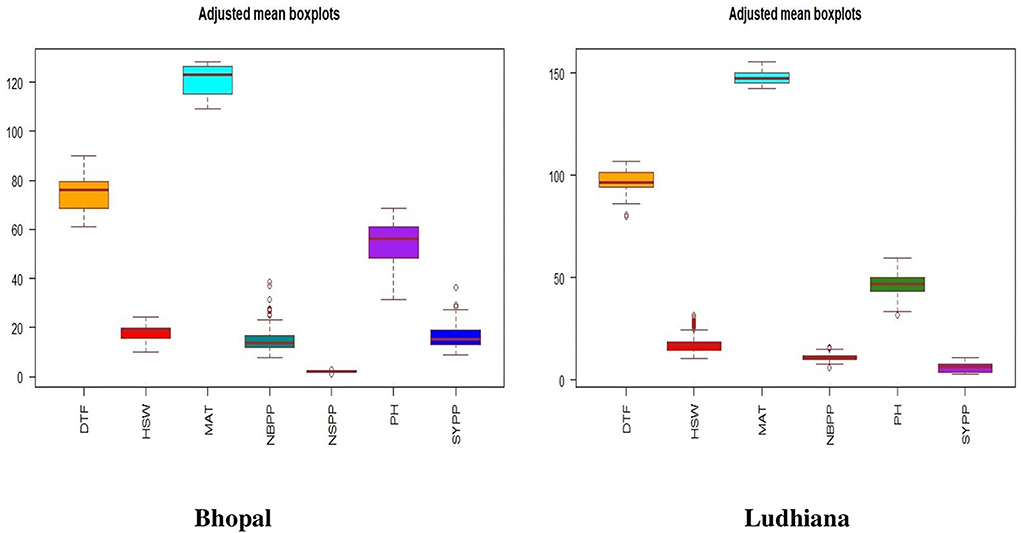
Figure 2. Adjusted mean boxplots of different agro-morphological traits for chickpea interspecific derivatives at Bhopal and Ludhiana, India. DTF, days to flowering; HSW, 100-seed weight; MAT, days to maturity, NBPP, number of branches plant−1; NSPP, number of seeds pod−1; PH, plant height; SYPP, seed yield plant−1.
Correlations and principal component analysis
The association between days to flowering, days to maturity, and plant height showed positive correlations with each other for chickpea interspecific derivatives in Bhopal, India. Similarly, the number of branches plant−1 and seed yield plant−1 showed positive correlations in Ludhiana, India (Figure 3). Further, PCA biplots were used to ascertain this relationship among studied characters at both locations, but they gave contrasting results. It was observed that days to flowering, days to maturity, and plant height were highly correlated to each other while traits like the number of branches plant−1 and seed yield plant−1 showed negative correlations in Bhopal, India. However, in Ludhiana, India, days to flowering, days to maturity, and plant height exhibited negative correlations against the number of branches plant−1 and seed yield plant−1 which showed positive relations (Figure 4).
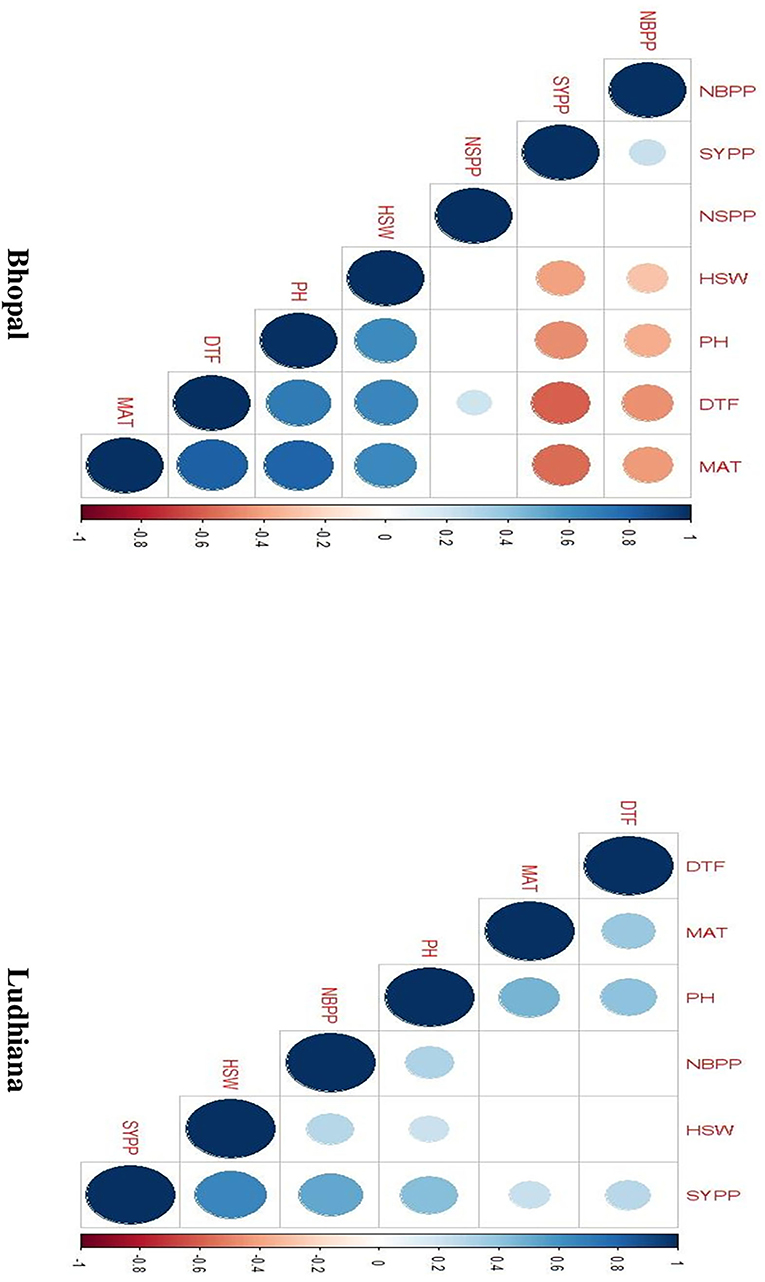
Figure 3. Correlation plots for agro-morphological traits among chickpea interspecific derivatives at Bhopal and Ludhiana, India. DTF, days to flowering; HSW, 100-seed weight; MAT, days to maturity, NBPP, number of branches plant−1; NSPP, number of seeds pod−1; PH, plant height; SYPP, seed yield plant−1.
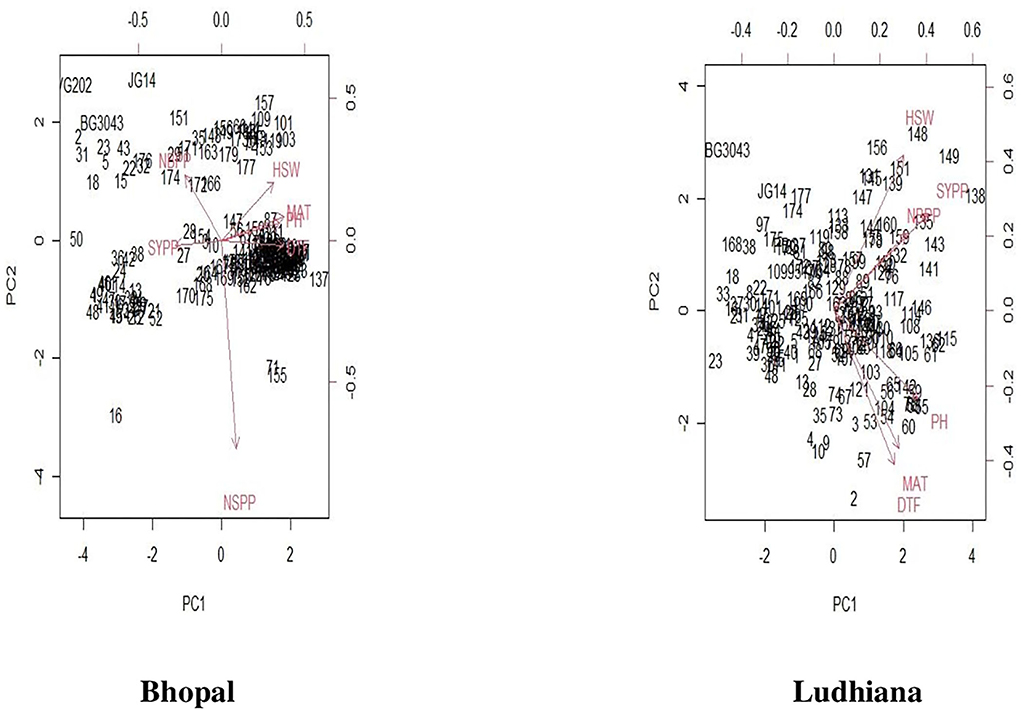
Figure 4. PCA biplots for chickpea interspecific derivatives at Bhopal and Ludhiana, India. DTF, days to flowering; HSW, 100-seed weight; MAT, days to maturity, NBPP, number of branches plant−1; NSPP, number of seeds pod−1; PH, plant height; SYPP, seed yield plant−1.
Screening against major biotic stresses
Ascochyta blight
The selected agronomically promising F7 interspecific derivatives of Pusa372 × EC720438, PBG5 × EC720438, BGD72 × EC720438, BGD72 × EC720481, PBG5 × EC720481, and Pusa372 × EC720481 were screened against ascochyta blight resistance (Figure 5). The following interspecific derivatives of cross Pusa372 × EC720438 (2 IDs), PBG 5 × EC720438 (18 IDs), BGD72 × EC720438 (3 IDs), BGD 72 × EC720481 (14 IDs), and PBG5 × EC720481 (5 IDs) showed resistant disease reaction against the pathogen. Likewise, cross Pusa372 × EC720438 (5 IDs), PBG5 × EC720438 (26 IDs), BGD72 × EC720438 (16 IDs), BGD72 × EC720481 (11 IDs), PBG5 × EC720481 (3 IDs), and Pusa372 × EC720481 (9 IDs) were reported as moderately resistant against ascochyta blight (Figure 5). The remaining interspecific derivatives exhibited either susceptible or highly susceptible disease reaction. Further, the mean disease incidence score ranged in 1 to 9 scales with an overall mean of 6.18 and a coefficient of variation of 29.49%. However, susceptible (JG62) and resistant (PBG7) checks revealed disease reaction with a rating of 9 and 3 scores, respectively. The overall latent semantic indexing percentage (LSI %) against the disease infestation was 5.02 %.
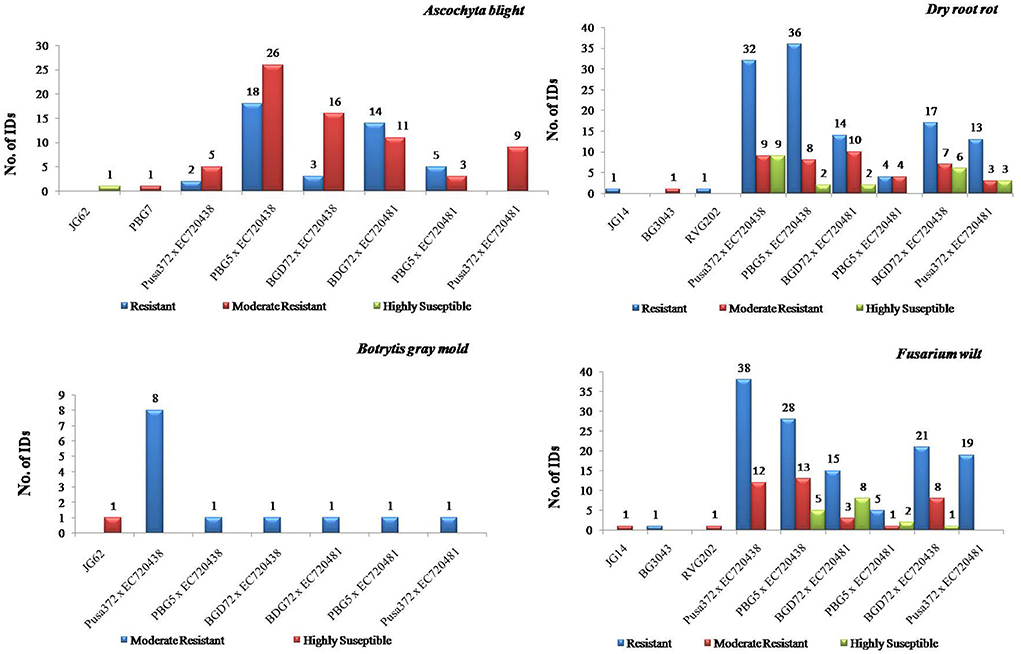
Figure 5. Performance of chickpea interspecific derivatives among different crosses and checks for ascochyta blight, botrytis gray mold, dry root rot, and fusarium wilt, respectively.
Botrytis gray mold
The results of the screening of chickpea interspecific derivatives against botrytis gray mold revealed that none of the interspecific derivatives showed resistant disease reaction in real field conditions. But a moderate level of resistance has been reported in the following interspecific derivatives of Pusa372 × EC720438 (8 IDs), one each of PBG5 × EC720438, BGD72 × EC720438, BGD72 × EC720481, PBG5 × EC720481, and Pusa372 × EC720481. The overall latent semantic indexing percentage (LSI %) against the pathogen infestation was 7.02 % (Figure 5).
Dry root rot
The results of the screening of chickpea interspecific derivatives against dry root rot showed resistant disease reaction in the crosses of Pusa372 × EC720438 (32 IDs), PBG5 × EC720438 (36 IDs), BGD72 × EC720481 (14 IDs), PBG5 × EC720481 (4 IDs), BGD72 × EC720438 (17 IDs), and Pusa372 × EC720481 (13 IDs). Likewise, maximum moderate resistance was reported in the cross of Pusa372 x EC720438, followed by BGD72 × EC720481, PBG5 × EC720438, BGD72 × EC720438, and Pusa372 × EC720481. The remaining interspecific derivatives were either susceptible or highly susceptible to the disease reaction (Figure 5).
Fusarium wilt
The results of chickpea interspecific derivatives against fusarium wilt revealed that the crosses of Pusa372 × EC720438 (38 IDs), PBG5 × EC720438 (28 IDs), BGD72 × EC720481 (15 IDs), BGD72 × EC720438 (21 IDs), and Pusa372 × EC720481 (19 IDs) exhibited resistant disease reaction against the pathogen. Likewise, a moderate level of resistance has also been reported in the crosses of Pusa372 × EC720438 (12 IDs), PBG5 × EC720438 (13 IDs), BGD72 × EC720481 (3 IDs), and BGD72 × EC720438 (8 IDs). The remaining derivatives belonging to different crosses were either susceptible or highly susceptible to disease reaction (Figure 5).
Discussion
Crop improvement programs are increasingly relying on pre-breeding and genetic enhancement employing CWRs to identify novel genes and alleles to broaden the genetic base of released cultivars (Singh et al., 2015). In chickpea, 41% of the crop varieties developed through hybridization had Pb7 as one of the ancestors in their pedigree (Kumar et al., 2003). The genetic base revealed by the pedigree records of released varieties appears to be narrow in major pulse crops, including chickpea, due to the frequent use of the same parents and their derivatives in breeding programs. To overcome these constraints, an attempt was, therefore, undertaken using wide hybridization of cultivated varieties (Pusa372, PBG5, and BGD72) taken as female and ILWC229 (EC720438) of C. reticulatum and ILWC246 (EC720481) of C. echinospermum taken as the male parents. True to type hybridity of F1 crosses was tested using Inter Simple Sequence Repeats (ISSR) and Random Amplified Polymorphic DNA (RAPD) markers (Singh et al., 2015). The experimental results revealed sufficient variation among genetic materials as indicated by mixed model analysis and analysis of variance (ANOVA) for the target traits (significant at p = 0.01, 0.05) assessed, and subsequently, the same was also reflected by range, mean, and coefficient of variation for important agro-morphological characters. However, descriptive statistics like skewness and kurtosis exhibited the normal distribution of expression performance for characters like the number of branches plant−1 and seed yield plant−1. Further, the interspecific derivatives provide a better opportunity for selecting promising transgressive segregants carrying potential traits of interest (Lewontin and Birch, 1966) along with classical genetic studies that have given a very compelling approach to the hypotheses that transgression can result from the expression of rare recessive alleles (Rick and Smith, 1953) and/or due to complementary gene action (Vega and Frey, 1980). Our results also exhibited the presence of transgressive segregations derived from C. reticulatum and C. echinospermum. All six interspecific derivatives were also assessed for their agronomic performance at two locations, including prevailing biotic stresses viz. ascochyta blight, botrytis gray mold, dry root rot, and fusarium wilt resistance under real field conditions. The genetic materials matured earlier in Bhopal, India than Ludhiana, India, suggesting certain physical factors and role of genotypic × environmental interactions, which could be responsible for the same. Erskine (1997) also reported the independent role of temperature and day length in determining the onset of ontogenesis in lentil. There were substantial variations with respect to plant height, the number of branches, seed number, and seed yield suggesting a good opportunity for selecting desirable ideotypes carrying important trait of interest (Eker et al., 2022). Further, hybrid vigor has also opened an era of genetic amelioration of crop plants and is often described as heterosis breeding. The fruitful heterosis also showed a wide range of variations among all interspecific derivatives for days to maturity, plant height, number of seeds pod−1, and seed yield plant−1. Similar results were also obtained by Singh and Ocampo (1997), Singh et al. (2005), and Singh et al. (2015) for yield-related traits in chickpea. An expression of the heterotic potential for certain interesting characters in advanced interspecific derivatives might be primarily due to the accumulation of favorable additive gene effects. Such derivatives may be advanced as suggested by Redden and Jensen (1974) for developing suitable genotypes. It was further indicated using estimation of other genetic parameters which revealed that high heritability and low genetic advance were predominantly assessed for the majority of characters indicating that non-additive gene effect and selection would be useful in later segregating generations when non-additive gene effect would have diminished. The genetic materials included in the study are interspecific cross populations belonging to different backgrounds carrying buffer hereditary information that lead to more transgression. Therefore, the estimate of heritability acts as a predictive approach in exercising reliability of phenotypic value, helping breeders to make a selection for a particular trait of interest when the heritability is high. Likewise, genetic advance is a useful indicator of progress, which can be expected as a result of exercising selection on population. Heritability in conjunction with genetic advance is more useful than heritability alone in predicting effects for selecting the best individual genotype because additive gene effects are likely to be present (Singh et al., 2014).
Furthermore, screening of chickpea enhanced progenies against major biotic stresses, which revealed that large numbers of interspecific derivatives were reported as resistant against ascochyta blight, indicating a substantial source of interspecific genetic resistance against the pathogen. Likewise, we were unable to find complete resistance against botrytis gray mold, but moderate resistance has been reported in 13 interspecific derivatives belonging to six cross-combinations of chickpea. However, for dry root rot and fusarium wilt, the interspecific derivatives exhibited resistance in various cross combinations of chickpea suggesting the potential of genetic materials to be taken further for developing disease-resistant cultivars. Overall, the wild Cicer species are a potential resource of useful untapped variations, including agro-morphological characters and major biotic stresses, as demonstrated by our findings. In F7 interspecific derivatives of chickpea, we found a wide range of variability for agro-morphological characters and major biotic traits, including considerable fruitful heterosis. It was also observed that derivatives derived from wild species showed more stability and yield levels, including resistance against major stresses. Thus, more emphasis should be given to the base-broadening program for tailoring usable germplasm with wider adaptations in future chickpea improvement programs. The potential lines carrying target traits could be a valuable genetic material for generation advancement to develop suitable genotypes. Lastly, useful genetic materials are being advanced for further breeding and desirable selection.
Data availability statement
The original contributions presented in the study are included in the article/Supplementary material, further inquiries can be directed to the corresponding author.
Author contributions
MS conceptualized the study and prepared the original draft. TK, UR, IS, and SB recorded data and performed field experimentation. SSo, NM, SSi, and SanjK helped in data analysis and manuscript editing. SandK helped in final editing of the manuscript. All authors contributed to the article and approved the submitted version.
Acknowledgments
The authors are thankful to the Department of Agriculture and Farmer's Welfare, Government of India for providing research grant under DAC-ICAR-ICARDA collaborative programs under NFSM to fund this study.
Conflict of interest
The authors declare that the research was conducted in the absence of any commercial or financial relationships that could be construed as a potential conflict of interest.
Publisher's note
All claims expressed in this article are solely those of the authors and do not necessarily represent those of their affiliated organizations, or those of the publisher, the editors and the reviewers. Any product that may be evaluated in this article, or claim that may be made by its manufacturer, is not guaranteed or endorsed by the publisher.
Supplementary material
The Supplementary Material for this article can be found online at: https://www.frontiersin.org/articles/10.3389/fpls.2022.941372/full#supplementary-material
Abbreviations
CWRs, crop wild relatives; IDs, interspecific derivatives; ANOVA, analysis of variance; ILWC, international legume wild Cicer; EC, exotic collection; ICAR, Indian Council of Agricultural Research; NBPGR, National Bureau of Plant Genetic Resources; MAREC, Mountain Agriculture Research and Extension Center; ICARDA, International Center for Agricultural Research in Dry Areas; PAU, Punjab Agricultural University; BP, better parent; PBG, Punjab gram; BGD, Bangalore gram; PCA, principal component analysis; PCV, phenotypic coefficient of variation; GCV, genotypic coefficient of variation; SSD, single seed descent; ABD, augmented block design; FAO, Food and Agriculture Organization.
References
Abbo, S., Berger, J., and Turner, N. C. (2003). Evolution of cultivated chickpea: four bottlenecks limit diversity and constrain adaptation. Func. Plant Biol. 30, 1081–1087 doi: 10.1071/FP03084
Abbo, S., Molina, C., Jungmann, R., Grusak, M. A., Berkovitch, Z., and Reifen, R. (2005). Quantitative trait loci governing carotenoid concentration and weight in seeds of chickpea. Theor. Appl. Genet. 111:185–195. doi: 10.1007/s00122-005-1930-y
Aravind, J., Mukesh Sankar, S., Wankhede, D. P., and Kaur, V. (2020). Augmented RCBD: Analysis of Augmented Randomised Complete Block Designs. R package version 0.1.2. Available online at: https://aravindj.github.io/augmentedRCBD/; https://cran.rproject.org/package=augmentedRCBD
Bakir, M., Sari, D., Waqas, M., and Atif, R. M. (2021). “Chickpea wild relatives: potential hidden source for the development of climate resilient chickpea varieties,” in Wild Germplasm for Genetic Improvement in Crop Plants, eds M. T. Azhar., and S. H. Wani (Academic Press, Elsevier), 269–297. doi: 10.1016/B978-0-12-822137-2.00015-1
Biswas, M. K., and Jubayer Ali, S. K. (2017). Management of fusarium wilt of chickpea (Cicer arietinum L.) under the undulating red and lateritic belt of West Bengal. J. Mycopathol. Res. 54, 461–468.
Burton, G. W. (1952). “Quantitative inheritance in grasses,” in Proceedings of the 454 International Grassland Congress, Vol. 1 (State College, PA: Pennsylvania State College), 277–283.
Chaturvedi, S. K., and Nadarajan, N. (2010). Genetic enhancement for grain yield in chickpea–accomplishments and resetting research agenda. Electron. J. Plant Breed. 1, 611–615.
Croser, J. S., Ahmad, C. F., Clarke, H. J., and Siddique, K. H. M. (2003). Utilization of wild Cicer in chickpea improvement- progress, constraints, and prospects. Austr. J. Agric. Res. 54, 429–444. doi: 10.1071/AR02157
Dixit, G. P. (2018). Project Coordinator's Report of All India Coordinated Research Project on Chickpea. Indian Institute of Pulses Research, Kanpur.
Eker, T., Sari, D., Sari, H., Tosun, H. S., and Toker, C. (2022). A kabuli chickpea ideotype. Sci. Rep. 12, 1611. doi: 10.1038/s41598-022-05559-3
Food Agriculture Organization (2020). FAOSTAT Statistical database of the United Nations Food and Agriculture Organization. Rome: FAO. Available online at: http://faostat.fao.org (accessed September 14, 2021).
Goulden, C. H. (1939). “Problems in plant selection,” in Proceedings of the 7th International Genetics Congress (Cambridge, MA: Cambridge University Press), 132–133.
Johnson, H. W., Robinson, H. F., and Comstock, R. E. (1955). Estimates of genetic and 484 environmental variability in Soybean. Agron. J. 47, 314–318. doi: 10.2134/agronj1955.00021962004700070009x
Koseoglu, K., Adak, A., Sari, D., Sari, H., Ceylan, F. O., and Toker, C. (2017). Transgressive segregation for yield criteria in reciprocal interspecific crosses between C. arietinum L. and C. reticulatum ladiz. Euphytica 213, 116. doi: 10.1007/s10681-017-1903-7
Kumar, S., Gupta, S., Chandra, S., and Singh, B. B. (2003). “How wide is the genetic base of pulse crops,” in Pulse in New Perspective, eds M. Ali., B. B. Singh., S. Kumar., and V. Dhar (Kanpur: Indian Society of Pulse Research and Development), 34–45.
Lewontin, R. C., and Birch, L. C. (1966). Hybridization as a source of variation for adaptation to new environments. Evolution 20, 315–336. doi: 10.1111/j.1558-5646.1966.tb03369.x
Lush, J. L. (1940). Intrusive collection of regression of offspring on dams asa method of estimating heritability of characters. Proc. Am. Soc. Anim. Prod. 33, 293–301.
Mallikarjuna, N., Sharma, H. C., and Upadhyaya, H. D. (2007). Exploitation of wild relatives of pigeonpea and chickpea for resistance to Helicoverpa armigera. J. SAT Agric. Res. 3, 1–4.
Nene, Y. L., and Haware, M. P. (1980). Screening chickpea for resistance to wilt. Plant Dis. 64, 379–380. doi: 10.1094/PD-64-379
Nene, Y. L., Haware, M. P., and Reddy, M. V. (1991). Chickpea disease resistance-screening technique. ICRISAT Info. Bull. 28, 28–29.
Pande, S., Sharma, M., Gaur, P. M., and Gowda, C. L. L. (2010). Host Plant Resistance to Ascochyta Blight of Chickpea. Andhra Pradesh: Information Bulleting of ICRISAT-Patancheru.
R Core Team (2018). R: A Language and Environment for Statistical Computing. Vienna: R Foundation for Statistical Computing. Available online at: http://www.R-project.org/
Rai, A., Irulappan, V., and Senthil-Kumar, M. (2022). Dry root rot of chickpea: a disease favored by drought. Plant Dis. 106, 346–356 doi: 10.1094/PDIS-07-21-1410-FE
Rakshit, S., Ganapathy, K. N., Gomashe, S. S., Rathore, A., Ghorade, R. B., Nagesh, K., et al. (2012). GGE biplot analysis to evaluate genotype, environment and their interactions in sorghum multi-location data. Euphytica 185, 465–479. doi: 10.1007/s10681-012-0648-6
Redden, R. J., and Jensen, N. F. (1974). Mass selection and mating systems in cereals. Crop Sci. 14, 345–350 doi: 10.2135/cropsci1974.0011183X001400030001x
Rick, C. M., and Smith, P. G. (1953). Novel variation in tomato species hybrids. Am. Nat. 88, 359–373. doi: 10.1086/281796
Robertson, L. D., Ocampo, B., and Singh, K. B. (1997). Morphological variation in wild annual Cicer species in comparison to the cultigen. Euphytica 95, 309–319. doi: 10.1023/A:1003004516921
Sandhu, J. S., Gupta, S. K., Singh, G., Sharma, Y. R., and Bains, T. S. (2006). “Inter-specific hybridization between Cicer arietinum and Cicer pinnatifidum for improvement of yield and other traits,” in 4th International Food Legumes Research Conference (New Delhi), 18–22.
Saraf, C. S., Rupela, O. P., Hegde, D. M., Yadav, R. L., Shivkumar, B. G., Bhattarai, S., et al. (1998). “Biological nitrogen fixation and residual effects of winter grain legumes in rice and wheat cropping systems of the Indo-Gangetic plain,” in Residual Effects of Legumes in Rice and Wheat Cropping Systems of the Indo-Gangetic Plain, eds J. V. D. K. Kumar., C. Johansen., and T. J. Rego (New Delhi: Oxford/IBH Publishing), 14–30.
Sari, D., Sari, H., Eker, T., Ikten, C., Uzun, B., and Toker, C. (2022). Intraspecific versus interspecific crosses for superior progeny in Cicer species. Crop Sci. doi: 10.1002/csc2.20765
Siddique, K. H. M., Brinsmead, R. B., Knight, R., Knights, E. J., Paull, J. G., and Rose, I. A. (2000). “Adaptation of chickpea (Cicer arietinum L.) and faba bean (Vicia faba L.) to Australia,” in Linking Research and Marketing Opportunities for Pulses in the 21st Century, eds R. Knight (Springer), 289–303. doi: 10.1007/978-94-011-4385-1_26
Singh, G., Kaur, L., and Sharma, Y. R. (1991). Ascochyta blight and botrytis gray mold resistance in wild species of Cicer. Crop Improve. 18, 150–151.
Singh, K. B., and Ocampo, B. (1997). Exploitation of wild Cicer species for yield improvement in chickpea. Theor. Appl. Genet. 95, 418–423. doi: 10.1007/s001220050578
Singh, M., Bisht, I. S., Dutta, M., Kumar, K., Basandrai, A. K., Kaur, L., et al. (2014). Characterization and evaluation of wild annual Cicer species for agro-morphological traits and major biotic stresses under North-western Indian conditions. Crop Sci. 54, 229–239. doi: 10.2135/cropsci2013.04.0225
Singh, M., Kumar, K., Bisht, I. S., Dutta, M., Rana, M. K., Rana, J. C., et al. (2015). Exploitation of wild annual Cicer species for widening the gene pool of chickpea cultivars. Plant Breed. 134, 186–192. doi: 10.1111/pbr.12254
Singh, M., Malhotra, N., and Singh, K. (2021). Broadening the genetic base of cultivated chickpea following introgression of wild Cicer species-progress, constraints and prospects. Genet Resour. Crop Evol. 68, 2181–2205. doi: 10.1007/s10722-021-01173-w
Singh, M., Rani, S., Malhotra, N., Katna, G., and Sarker, A. (2018). Transgressive segregations for agronomic improvement using interspecific crosses between C. arietinum L. × C. reticulatum Ladiz. and C. arietinum L. × C. echinospermum Davis species. PLoS ONE 13, e0203082. doi: 10.1371/journal.pone.0203082
Singh, S., Gumber, R. K., Joshi, N., and Singh, K. (2005). Introgression from wild Cicer reticulatum to cultivated chickpea for productivity and disease resistance. Plant Breed. 124, 477–480 doi: 10.1111/j.1439-0523.2005.01146.x
Talip, M., Adak, A., Kahraman, A., Berger, J., Sari, D., Sari, H., et al. (2018). Agro-morphological traits of Cicer reticulatum Ladizinsky in comparison to C. echinospermum P.H. Davis in terms of potential to improve cultivated chickpea (C. arietinum L.). Genet. Resour. Crop Evol. 65, 951–962 doi: 10.1007/s10722-017-0587-0
Tanksley, S. D., and McCouch, S. R. (1996). Seed banks and molecular maps: unlocking genetic potential from the wild. Science 277, 1063–1066 doi: 10.1126/science.277.5329.1063
Toker, C., Berger, J., Eker, T., Sari, D., Sari, H., Gokturk, R. S., et al. (2021). Cicer turcicum: a new Cicer species and its potential to improve chickpea. Front. Plant Sci. 12, 662891. doi: 10.3389/fpls.2021.662891
van der Maesen, L. J. G. (1987). “Origin, history and taxonomy of chickpea,” in The Chickpea, eds M. C. Saxena., and K. B. Singh (Cambridge: CAB International), 11–34.
Vance, W., Pradeep, K., Strachan, S. R., Diffey, S., and Bell, R. W. (2021). Novel sources of tolerance to aluminium toxicity in wild cicer (Cicer reticulatum and Cicer echinospermum) collections. Front. Plant Sci. 12, 678211. doi: 10.3389/fpls.2021.678211
Varshney, R. K., Song, C., Saxena, R. K., Azam, S., Yu, S., Sharpe, A. G., et al. (2012). Draft genome sequence of chickpea (Cicer arietinum) provides a resource for trait improvement. Nat. Biotechnol. 31, 240–246 doi: 10.1038/nbt.2491
Vega, U., and Frey, K. J. (1980). Transgressive segregation in inter and intraspecific crosses of barley. Euphytica 29, 585–694. doi: 10.1007/BF00023206
Keywords: transgressive segregation, fruitful heterosis, wild Cicer, genetic improvement, biotic stress
Citation: Singh M, Kumar T, Sood S, Malhotra N, Rani U, Singh S, Singh I, Bindra S, Kumar S and Kumar S (2022) Identification of promising chickpea interspecific derivatives for agro-morphological and major biotic traits. Front. Plant Sci. 13:941372. doi: 10.3389/fpls.2022.941372
Received: 11 May 2022; Accepted: 08 July 2022;
Published: 04 August 2022.
Edited by:
Jaime Prohens, Universitat Politècnica de València, SpainCopyright © 2022 Singh, Kumar, Sood, Malhotra, Rani, Singh, Singh, Bindra, Kumar and Kumar. This is an open-access article distributed under the terms of the Creative Commons Attribution License (CC BY). The use, distribution or reproduction in other forums is permitted, provided the original author(s) and the copyright owner(s) are credited and that the original publication in this journal is cited, in accordance with accepted academic practice. No use, distribution or reproduction is permitted which does not comply with these terms.
*Correspondence: Mohar Singh, TW9oYXIuc2luZ2gyQGljYXIuZ292Lmlu