- Laboratory of Air Pollution and Global Climate Change, Department of Botany, Institute of Science, Banaras Hindu University, Varanasi, India
The ramifications of different concentrations of foliar particulate matter on the physiology, nutrient stoichiometry, allocation pattern, and their corresponding re-translocation rates were investigated for evergreen (Mangifera indica and Psidium guajava), semi-evergreen (Ficus religiosa and Azadirachta indica), and deciduous (Dalbergia sissoo) tree species in a simulation experiment over an exposure period of 2 years. Physiological parameters (Pn, gs, Ci, E, and WUE), nutrient stoichiometry (C: N) in different plant parts, and their allocation pattern for five macro- (C, N, K, Mg, Ca) and five (Zn, Ni, Mn, Cu, Fe) micro-elements at two different concentrations of particulate matter (ambient and elevated) with respect to control (no particulate load) were assessed. Significant differences in nutrient concentrations and their re-translocation rates were observed between the treatments in evergreen species compared to deciduous species. The photosynthetic rate significantly declined with an increase in foliar deposition of particulate matter. Higher variations in C, N, K, Mg, and Zn levels were found compared to other elements under particulate matter stress and the ratio of C/N showed a slight decline in mature leaves except in deciduous tree species. The nutrient stoichiometry revealed that the deciduous species were more tolerant whereas the re-translocation efficiency was maximum for the semi-evergreen tree species. The nutrient allocation was found greater in foliage compared to branch in evergreen and was opposite in semi-evergreen and deciduous tree species. The element re-translocation rate indicated an inconsistent behavior in nutrient recycling under the particulate matter load depending upon the tree species. The study entrenched a critical change in nutrient re-translocation and allocation pattern under the particulate stress in different parts of the tree, suggesting a novel approach for screening the tree species for sustainable plantation and planning of urban areas.
Introduction
The occurrence of particulate matter (PM) is ubiquitous in the tropospheric layer exerting an adverse impact on the climate and environment around the world. At the local level, PM can intensify the effects of greenhouse gases by scattering or absorbing incoming solar radiation that emitted back from the surface of the earth, thereby contributing to the global climate change (Buseck and Pósfai, 1999). Reduction in the concentration of PM particularly PM2.5 is of utmost importance as it has been reported to severely affect human health and the environment (Mukherjee and Agrawal, 2017). In urban areas, vehicles and industries are major anthropogenic sources of particulate emissions, despite their identification, halting these sources is neither economically feasible nor functionally possible. One of the best natural methods for the curtailment of PM is to increase urban greening which will provide a surface for the adherence of PM (Escobedo and Nowak, 2009). Adverse effects of PM have already been established on human health (Anderson et al., 2012; Patra et al., 2016; Hassan et al., 2021), but impacts on the plants especially trees, which are extensively used for the mitigation of PM, are still unexplored. Most of the studies relating PM with tree species either provide insight into their capture potential (Chen et al., 2017) depending upon their leaf characteristics (micromorphology, orientation, etc.) (Liu et al., 2018) or screen them as tolerant or sensitive based on their air pollution tolerance index (APTI) and related parameters (pH, relative water content, ascorbic acid, and total chlorophyll) (Sgrigna et al., 2020; Zhang et al., 2020).
PM deposition on the foliage of trees imposes its adverse impacts primarily through the clogging of the stomatal aperture thereby increasing leaf temperature which in turn affects the overall development of the plant (Popek et al., 2018). The layer of PM covering the foliage affects the foliar physiology by acting as a screen between the surface of the leaf and its ambiance. Thereby, blocking the environmental cues. In similar ways, it also hampers the amount of PAR received by the foliage (Mina et al., 2018). There are studies focusing on the nutrient status of plants subjected to various air pollutants (Cao et al., 2016; Shi et al., 2016b; Braun et al., 2020; Piao et al., 2020), but none of the studies have focused on the nutrient-related parameters of the trees under PM stress. For assessing the nutrient status of a plant, it is important to determine the nutrient allocation pattern, along with the determination of nutrient stoichiometry, and ultimately establish the nutrient re-translocation efficiency (NURE) under the applied stress. The allocation of nutrients in plant parts is indicative of plant resource uptake and utilization (Zhao et al., 2020). Leaf stoichiometry is an important determinant of plant composition and nutrient limitation over a period of time (Lanuza et al., 2019). One of the most essential processes to trace nutrient dynamics of tree species is analyzing the NURE. It refers to the quantity of nutrients reabsorbed from the organs of the plant which are showing signs/symptoms of senescence and utilized in the development of new plant parts (Shi et al., 2017).
The present study assesses the nutrient status and unravels the details of nutrient allocation of the dominant tree species employed for urban plantation in the Indo-Gangetic plains under a gradient of PM as a stress factor.
Materials and Methods
Experimental Details
The simulation experiment was performed for 2 consecutive years from November 2019 to November 2021 at the Botanical Garden of Banaras Hindu University, Varanasi, Uttar Pradesh, India. The 25° 81_N and 83° 1_E represent the coordinates of the experimental site situated on the eastern Gangetic plains of India. The details of the experimental setup show field dimensions along with the distance between individual tree species (Figure 1). The field design was a split plot with random plantation of tree species and treatment strategy.
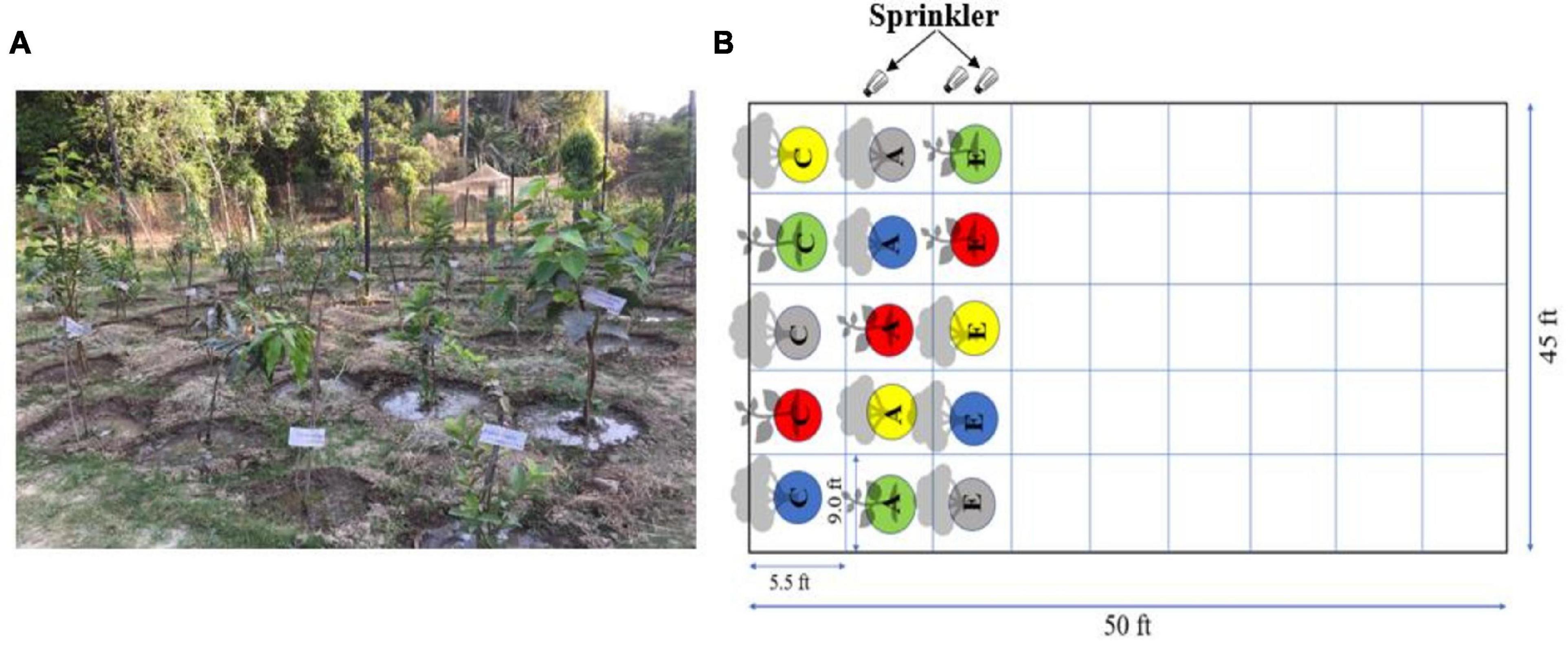
Figure 1. Representation of experimental setup: (A) the experimental field with test plant, (B) schematic representation of the field. The design is a split plot with a random plantation. The abbreviations for treatments are: C, control PM; A, ambient PM; and E, elevated PM.
Meteorological Parameters
The meteorological data including daily maximum and minimum temperature, total rainfall, and relative humidity, for the experimental period were obtained from the Indian Meteorological Division (IMD) of Banaras Hindu University, Varanasi, India. The mean maximum temperature recorded from November 2019 to November 2021 was 39.72°C in April 2021, while the mean minimum temperature was 9.66°C in January 2021 (Figure 2). The highest temperature was recorded to be 45.9°C on May 27, 2020, and the lowest to be 4.6°C on January 31, 2021. Mean relative humidity (RH) for the study period ranged from 51.10 to 91.03%. Maximum total rainfall was recorded to be 366.9 mm in June 2020.
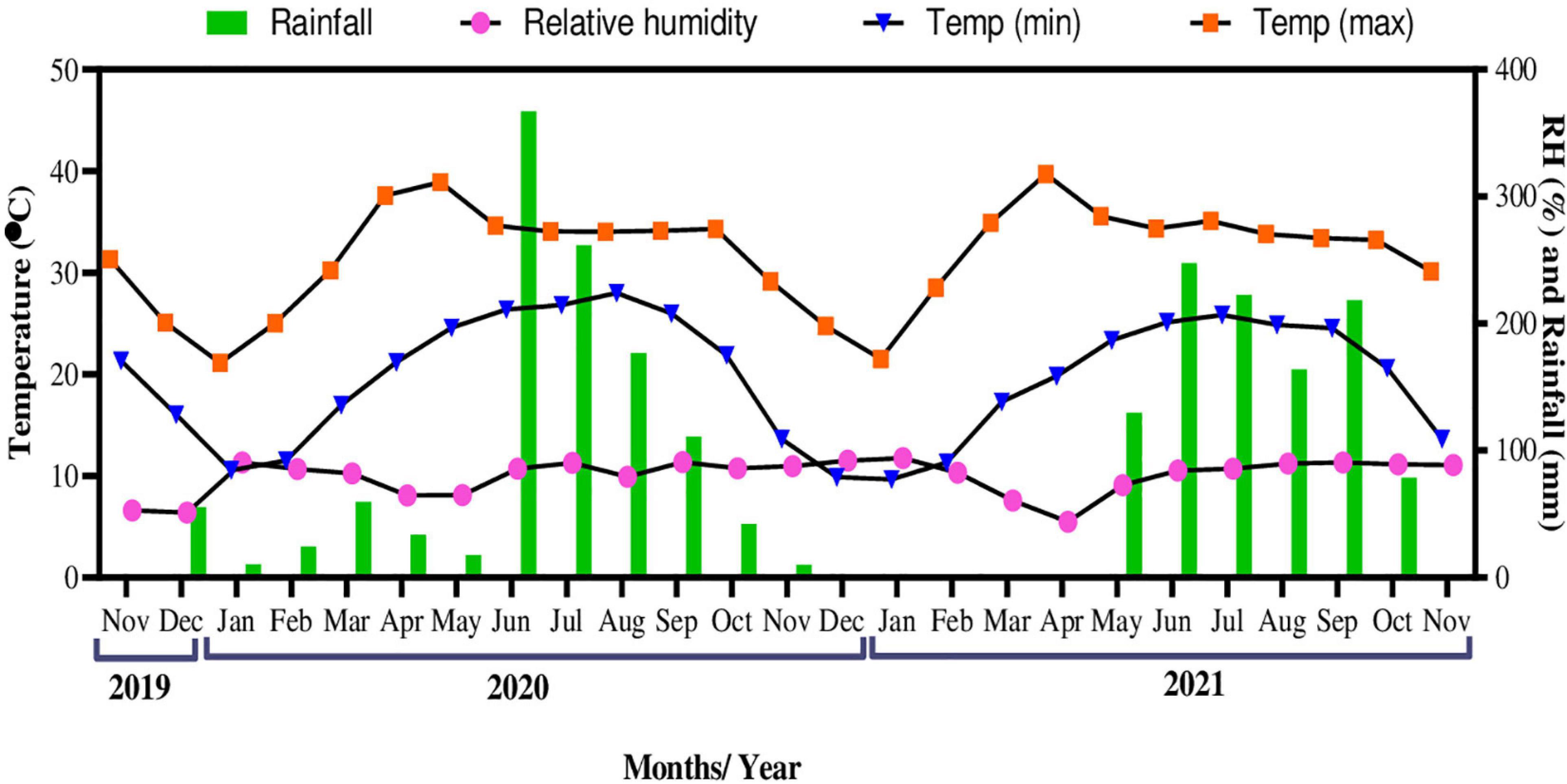
Figure 2. Variations in daily temperature (°C), relative humidity (%), and total rainfall (mm) during the experimental period.
Plant Material and Experimental Setup
Dominant trees of the Indo-Gangetic plains belonging to different functional types (evergreen and deciduous) with different leaf forms (simple and compound) (Table 1) were subjected to two different loads of PM viz. ambient (APM) and elevated (EPM) with a parallel control (CPM) setup according to the species-specific capture potential of the PM. The present study focused on the nutrient allocation pattern and stoichiometry of different plant parts and re-translocation efficiency of the studied elements in various tree functional types (two evergreen, two semi-evergreen, and one deciduous tree species). One-year-old saplings were purchased from the Horticulture Department of Banaras Hindu University, Varanasi, and were transplanted in the field of dimension 45 × 50 sq. ft. randomly optimizing the distance between the two saplings. A controlled release formulation was applied to the field soil for fertilization containing 13.4% organic carbon, 1.61% nitrogen, 1.02% phosphorus, 0.73% potassium, 7.61% calcium, 0.568% magnesium, 0.158% sodium, 0.110% zinc, and 0.0048% copper. Tap water was used for maintaining the optimum irrigation during the experimental period.
The PM for simulating the stress condition in the experiment was collected passively from the foliage of the trees in commercial area. The PM adhered on the leaves was washed with distilled water and the water was evaporated on a hot plate (80°C). The PM collected was used for the simulation experiment. The species-specific ambient load was determined using the methodology for deposition determination by Singh et al., 2021 mentioned in Table 2. Dust simulation was manually done with the help of a sprinkler (which was standardized according to its pore size, i.e., the PM expelled from it was quantified and the dose was given accordingly) using water as a surfactant for the adherence of dust on the leaf surface of each plant and the dusting was repeated after every 30 days interval. The EPM was double that of APM and was simulated similarly to APM.
Plant Sampling and Analysis
Nine plants of each species were assessed for physiological and nutrient analyses. Nine replicates of each species were considered for physiological measurement, that is, net photosynthesis (Pn), stomatal conductance (gs), internal CO2 concentration (Ci), and transpiration (E) using LICOR photosynthetic system (Model 6200, LICOR, Lincoln, United States). Water Use Efficiency (WUE) was calculated as the ratio of photosynthetic rate to the rate of transpiration. The instrument was calibrated with a known source of CO2 (509 ppm) before recording the measurements on a clear sky day. The data collection for the photosynthetic parameters was done between 8:00 and 10:00 h. This was collected from a fully expanded leaf, fourth from the top of the secondary branch of each treatment. The photosynthetically active radiation ranged between 1,100 and 1,200 μmol m–2 s–1.
The sampling for assessment of nutrient-related parameters was done at the end of the study period. The samples were collected in December 2021 after an exposure period of 2 years. Three mature leaves, senesced leaves, and branch samples from each treatment were randomly collected. The fresh leaves and branches were oven-dried at 70°C to constant weight. The dried samples were grounded in an automatic grinder and passed through a 0.15 mm sieve. The determination of C and N was done by an automatic CHNS analyzer (Euro EA Elemental Analyzer, EURO VECTOR Instruments). Apart from these, K, Mg, Ca, Zn, Ni, Mn, Cu, and Fe were quantified by atomic absorption spectrometer (Model Analyst 800, Perkin-Elmer and Norwalk, United States.) after digesting air-dried plant samples (0.1 g) in 10 mL di-acid (nitric acid:perchloric acid = 9:4) for total metal content (Gaidajis, 2003).
Repeated analyses of samples were conducted against the National Institute of Standards and Technology Standard Reference Material (NBS SRM-1570) for all the metals except C and N for which sulphanilamide OAS [Euro Vector S.r.l., Via F.lli Cuzio, Pavia (PV), Italy] was used to ensure the precision and accuracy of the analysis. To re-calibrate the instrument, blank and drift standards (Sisco Research Laboratories Pvt. Ltd., India) were run after every five sample runs. The results were found to be within 2% of the certified value. For different determinations and precision of analysis, the coefficients of variance of replicate analysis were determined. The variances were found to be fewer than 10%.
The relevant calculations for the determination of nutrient reabsorption efficiency (NURE) were done following the formula of Aerts (1996):
Statistical Analysis
The statistical analyses of the experimental data were performed by the IBM SPSS (Statistics 21.0) software. Data were first cohered for normality using the Shapiro–Wilk test and homogeneity of variance by Levene’s test. After meeting this analysis, the data were interpreted for analysis of variance (ANOVA), the difference among the means of different treatments with the same species and the variations among the means of different trees within the same treatments for each species were assessed via one-way ANOVA and least significant difference (LSD) post-hoc test, respectively. F-ratios were determined for various nutrients in the considered tree species, and treatment and parts by three-way ANOVA. The F-ratios for the other parameters apart from the nutrients were determined for tree species and treatment by two-way ANOVA. A significance level of 0.05 was the basis of statistical significance. The correlation coefficients were plotted using R 4.1.2 (R Core Development Team, 2021).
Results
Physiological Parameters
The applied PM load significantly reduced the assimilative rate in all the species under the study (Figure 3). The evergreen trees with simple leaves showed a greater reduction between treatments which was highest in M. indica, that is, 36.69% in APM and 49.55% in EPM, and lowest in P. guajava, which was 7.93% in APM and 22.40% in EPM. It was reduced by 10.35% in APM and 18.14% in EPM of A. indica which is semi-evergreen with compound leaves, while it was reduced by 23.86% in APM and 52.60% in EPM in D. sissoo, which is a deciduous tree with compound leaves. ANOVA test showed that both the treatments significantly affected all the physiological parameters of all the tree species individually and in combination (Table 3).
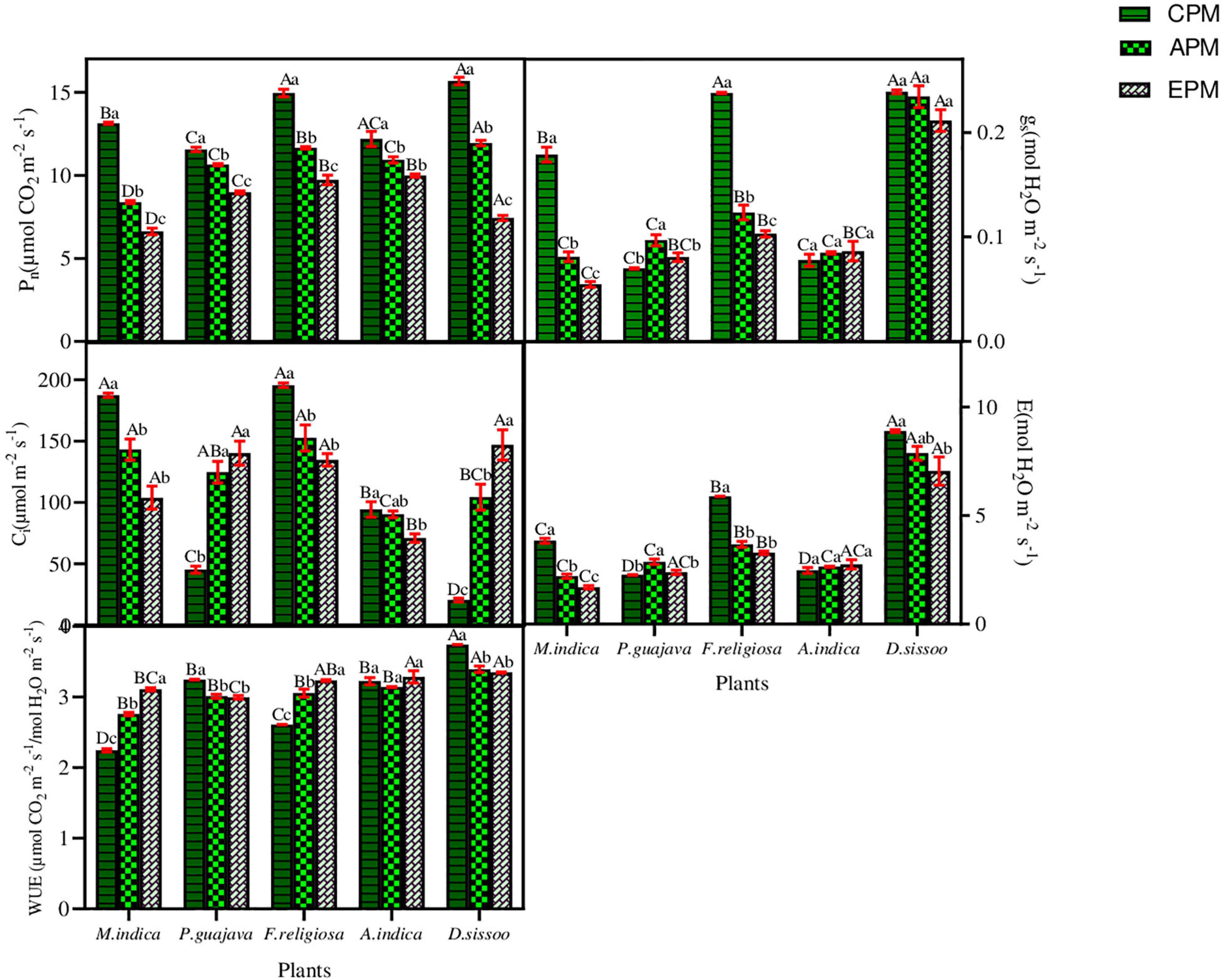
Figure 3. The photosynthetic parameters of different tree species under different PM treatments. Different letters in uppercase indicate a significant difference between PM treatments for different tree species (p < 0.05) and different lowercase letters indicate a significant difference between the treatments of a species (p < 0.05).
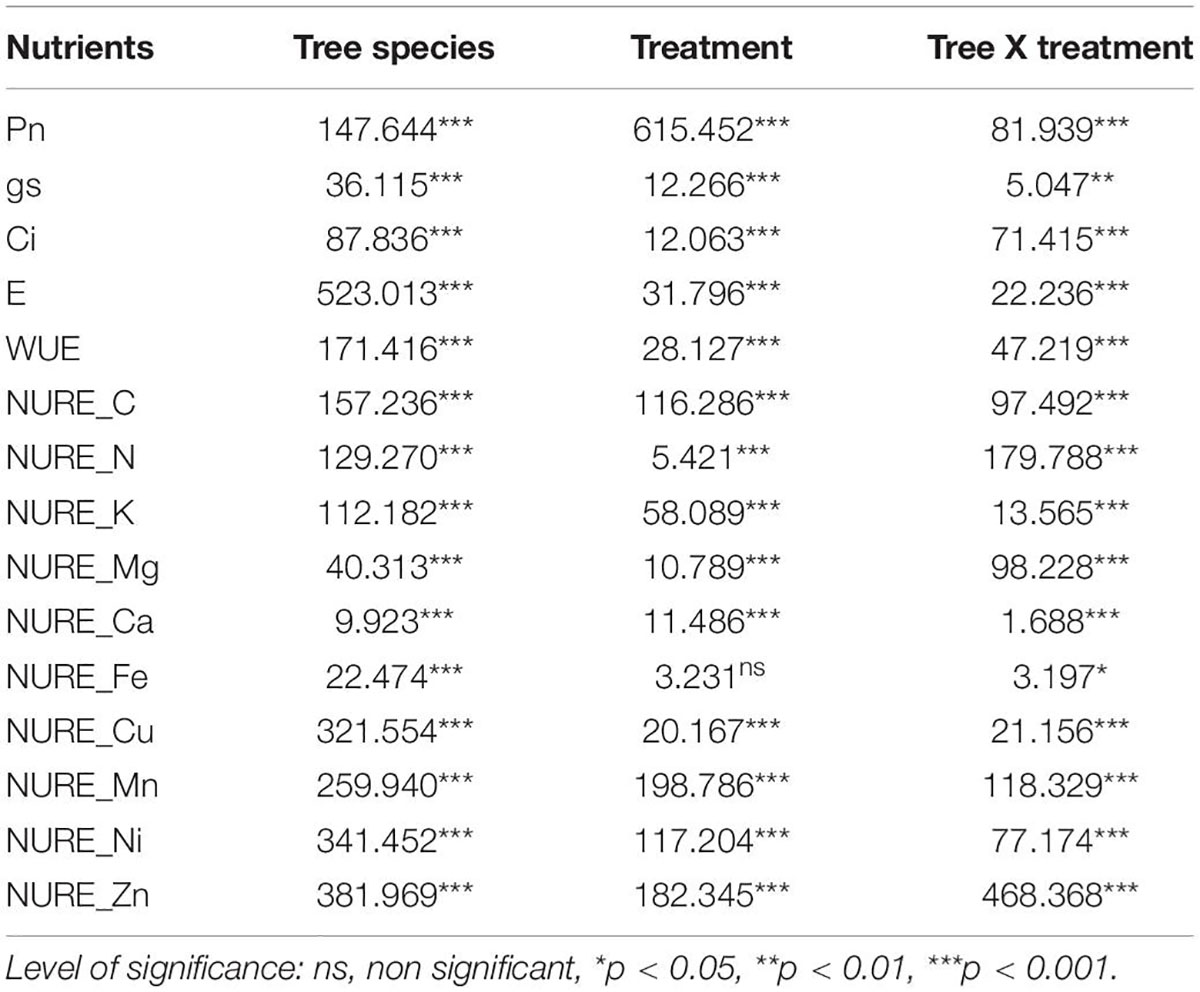
Table 3. F-ratios and level of significance of two-way ANOVA test for various parameters of the test tress species.
An inconsistency was observed in the gs of the studied species (Figure 3). A significant reduction in the gs was found in M. indica with 54.75% in APM and 69.52% in EPM. Similarly, F. religiosa showed a decline of 48.15% in APM and 56.77% in EPM. An exception was observed for P. guajava which showed a significant increase in gs of 38.48% in APM and 15.31% in EPM. A. indica followed a similar increase in gs as of P. guajava whereas D. sissoo showed an insignificant decline.
The Ci concentration was found to decline remarkably in simple leaf species with an exception in P. guajava where an increase in Ci of 176.36% in APM and 210.84% in EPM was found (Figure 3). A. indica with compound leaf comparably showed less reduction in Ci of 4.30% in APM and 24.79% in EPM. D. sissoo displayed the maximum increase in Ci of 412.66% in APM and 622.03% in EPM treatments.
EPM decreased maximally in M. indica by 42.60% in APM and 55.88% in EPM and least in D. sissoo which was 11.45% in APM and 20.69% in EPM (Figure 3). P. guajava and A. indica showed an increase in transpiration rate of 37.15% and 6.96% in APM and 44.15% and 11.32% in EPM, respectively.
The WUE increased in M. indica and F. religiosa whereas decreased in P. guajava, A. indica, and D. sissoo (Figure 3). The increase was maximum in M. indica (22.83% in APM and 38.40% in EPM) while the reduction was maximum in D. sissoo (9.35% in APM and 10.56% in EPM).
Allocation Pattern Under Particulate Matter Stress
It was observed that the nutrients considered follow two different patterns of allocation and re-translocation: (i) macroelements and (ii) microelements. Macroelements were further studied under two major divisions: phloem immobile elements (water in-soluble) and phloem mobile (water soluble) elements. Carbon is described separately as it follows both immobile and mobile behaviors. Phloem immobile elements are manganese (Mn) and calcium (Ca) and mobile elements are nitrogen (N), potassium (K), and magnesium (Mg). The micronutrients include copper (Cu), zinc (Zn), iron (Fe), and nickel (Ni).
In Mature Leaves
The phloem immobile elements namely Mn and Ca showed a similar trend for the mature leaves (Figure 4). They displayed an increase in APM which ranged between 13.3 and 31.0% for Mn and 1.6% and 6.6% for Ca. The increase was maximum in A. indica for Mn and D. sissoo for Ca. A decrease was observed in the allocation under EPM which ranged between 8.3 and 64.8% for Mn and 9.2% and 51.8% for Ca. The decrease was maximum in M. indica for Mn and A. indica for Ca. For the phloem mobile elements, namely N, K, and Mg, an increase was observed in APM which ranged between 23.2 and 69.3%. The increase was minimum for N in A. indica and maximum for Mg in M. indica. EPM showed an inconsistent trend where a reduction (11.1–19.9%) was found in F. religiosa, A. indica, and D. sissoo for N, in F. religiosa for K, and in D. sissoo for Mg, whereas all others showed increases. The allocation of C displayed a marked decline with increasing PM load (Figure 4). It decreased between 0.5 and 15.5% under APM and 1.7% and 20.5% under EPM with an exception of P. guajava, where an increase of 26% was observed in both the treatments.
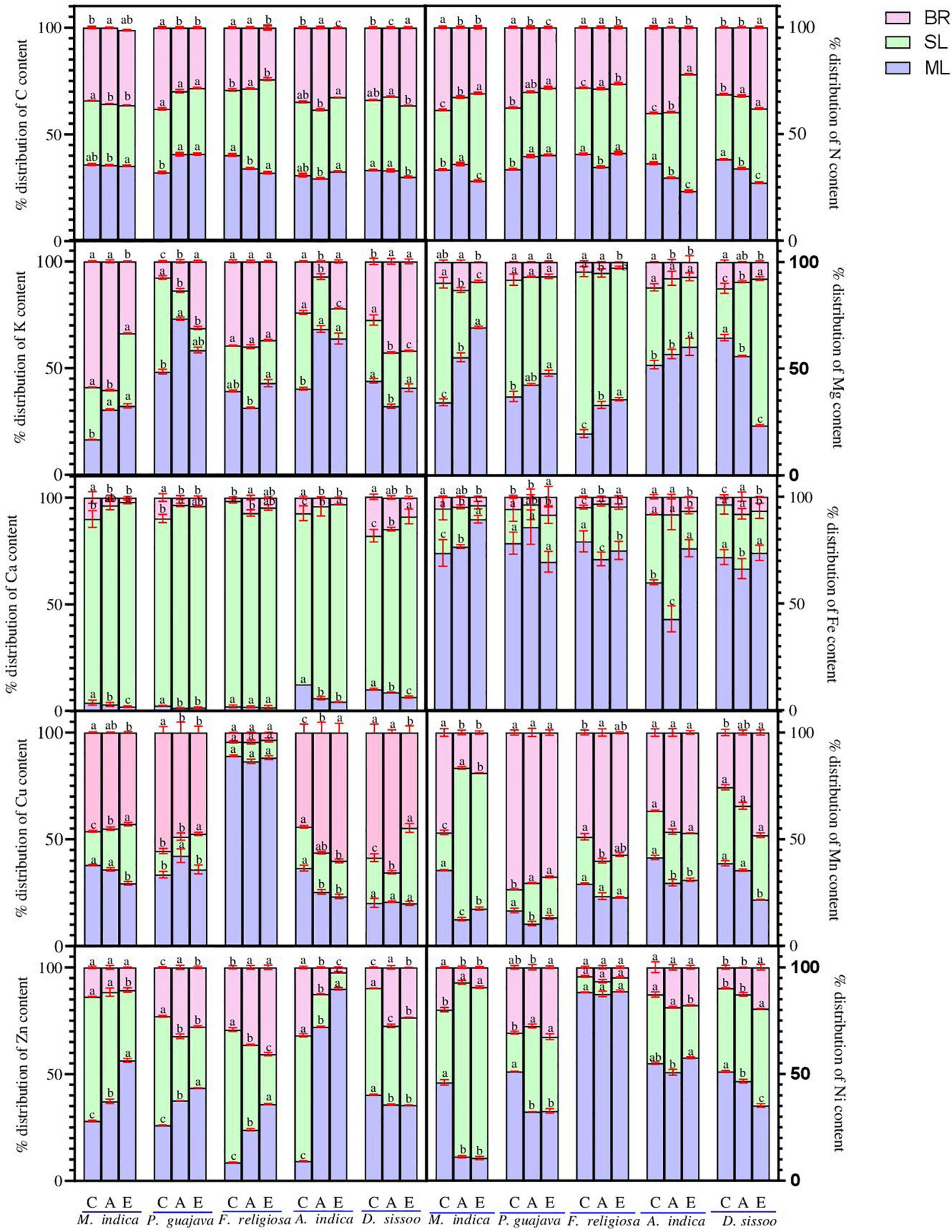
Figure 4. The nutrient allocation pattern of different tree species under different PM treatments. Different lowercase letters indicate a significant difference between the treatments within species (p < 0.05). BR, branch; SL, senesced leaf; ML, mature leaf.
The microelements Cu, Zn, Ni, and Fe, showed a consistent increase in the allocation pattern which ranged between 10.7 and 90% (Figure 4). This was highest in M. indica for Ni and lowest in A. indica for Zn. The EPM showed a marked decline in most of the microelements which ranged from 1 to 75.5% and was maximum in M. indica and minimum in F. religiosa for Ni.
In Senesced Leaves
The immobile elements showed an increase under APM ranging between 19 and 95.9% being minimum in P. guajava for Mn and M. indica for Ca (Figure 4). Under EPM, there was a marked increase in all except F. religiosa for both Mn and Ca and D. sissoo for Mn. The increase under EPM ranged between 6.3% in D. sissoo for Ca and 304.6% in M. indica for Mn. For the allocation of carbon, no definite trend was found under both the treatments. Carbon allocation increased in F. religiosa and D. sissoo under APM while decreased in all other tree species. Under EPM treatment, an increase was observed in C allocation from 1.5 to 43.6% except in M. indica where the allocation decreased by 5.3%.
The phloem mobile elements showed an increase in allocation pattern under APM which ranged between 10.2 and 68.8% (Figure 4). It was highest in D. sissoo for Mg and lowest in P. guajava for K. Under EPM, an increase was observed in all species for N whereas others showed a marked decline ranging between 1.9 and 70%, being maximum in A. indica for Mg and minimum for P. guajava for K. F. religiosa was an exception for K and D. sissoo for Mg which showed increment under EPM. For microelements, an increase was found under APM which ranged between 6.5 and 80% (Figure 4). It was found to be maximum in M. indica and minimum in F. religiosa for Ni. Under EPM, a reduction was observed in most microelements which ranged from 4.76 to 73.9% being highest in A. indica for Cu and lowest for Zn.
In Branches
The allocation pattern of the immobile elements consistently increased in all species under APM ranging from 18.9 to 96% and the increase was maximum in M. indica for Ca and minimum for Mn (Figure 4). Under EPM, most of the species showed an increase in allocation which ranged between 8.3 and 34% except Mn in M. indica and P. guajava and Ca in F. religiosa.
All phloem mobile elements under APM showed an increased allocation ranging between 2.7 and 38% (Figure 4). It was highest in D. sissoo for N and lowest in F. religiosa for Mg. Both increase and decrease in allocation were observed under EPM (Figure 4). The increase ranged between 1.4 and 80% which was maximum in P. guajava for K and minimum in F. religiosa for N. The decline ranged between 1.2 and 70.2% being highest for A. indica for K and lowest for N.
The microelements showed an increment in all the species under APM with a range of 2.3% to 60%, where A. indica for Cu showed a maximum increase, while the same showed a minimum for Zn. Most of the species under EPM showed an increase ranging between 1.3 and 182.4% and was maximum for Zn in D. sissoo and minimum for Fe in A. indica.
Nutrient Re-translocation Efficiency Under the Particulate Matter Stress
Among the 10 nutrients under consideration, 4 showed a consistent positive re-translocation (C, N, K, Mg) while 6 were negatively re-translocated (Ca, Fe, Cu, Mn, Ni, Zn) under the PM stress (Figure 5). The phloem immobile elements possessed negative NURE which decreased with the PM load for both Ca and Mn. Among the mobile elements, K showed an increase in NURE in all whereas Mg showed a marked decline with increasing PM load (Figure 5). A decrease in NURE with PM load was found for A. indica and D. sissoo while others showed a significant increase for N. For C, NURE showed a positive value which decreased with increasing PM load except in P. guajava and D. sissoo where the value increased in PM-treated plants.
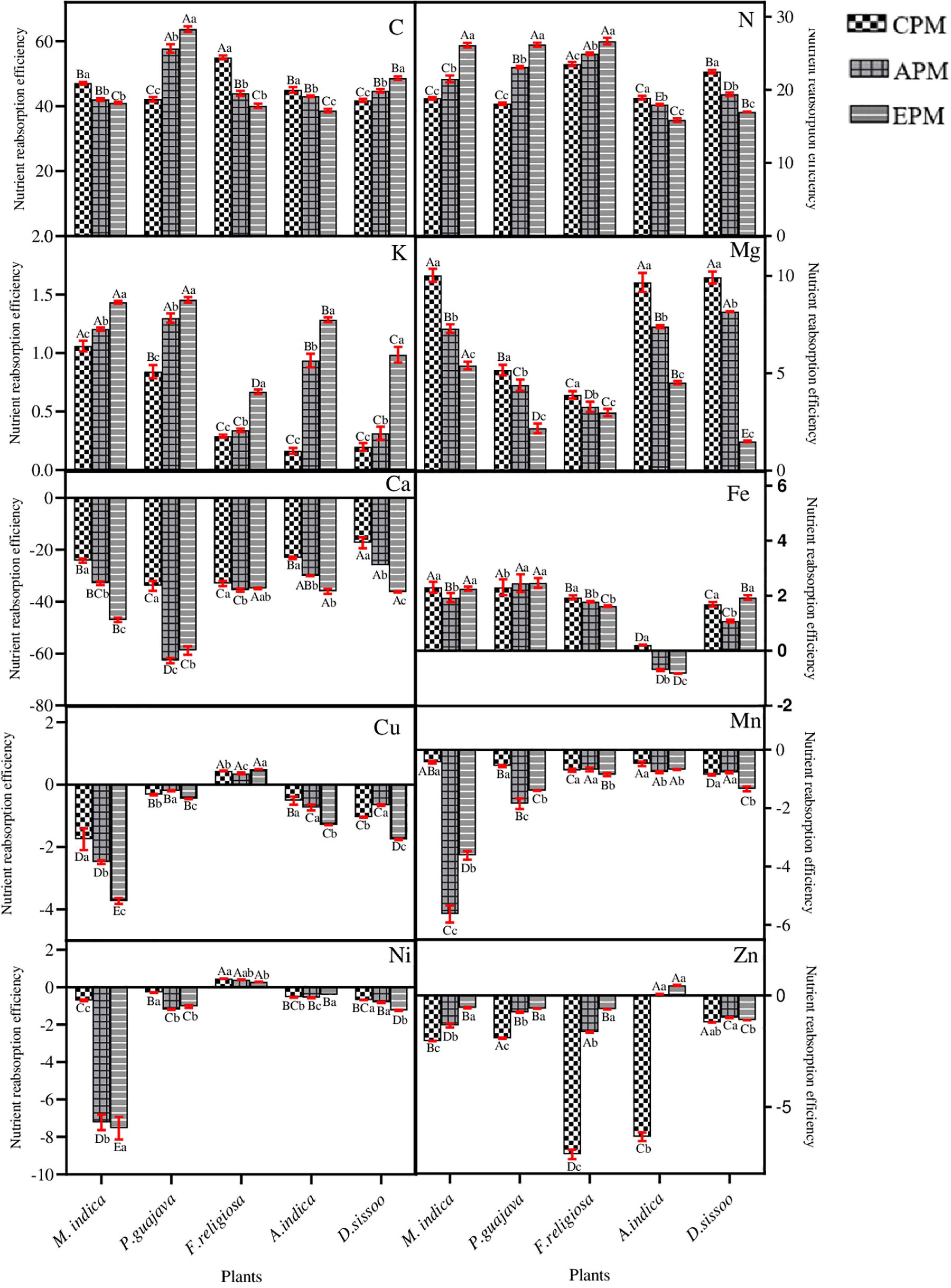
Figure 5. The nutrient re-translocation efficiency of different tree species under different PM treatments. Different letters in uppercase indicate a significant difference between PM treatments for different trees (p < 0.05) and different lowercase letters indicate a significant difference between the treatment with species (p < 0.05).
Among the microelements, Cu and Ni showed negative NURE which decreased with increasing PM for all except F. religiosa. Zn also showed negative NURE for all the species but the allocation was significantly increased with PM load. Fe showed positive NURE for all except A. indica, where the PM-treated species showed significantly negative NURE.
All the trees consistently showed positive NURE for C which decreased with PM load in M. indica, F. religiosa, and A. indica whereas increased in P. guajava and D. sissoo. ANOVA test showed that the treatments significantly affected the nutrients in all species except for Fe where the effect was non-significant (Table 4).
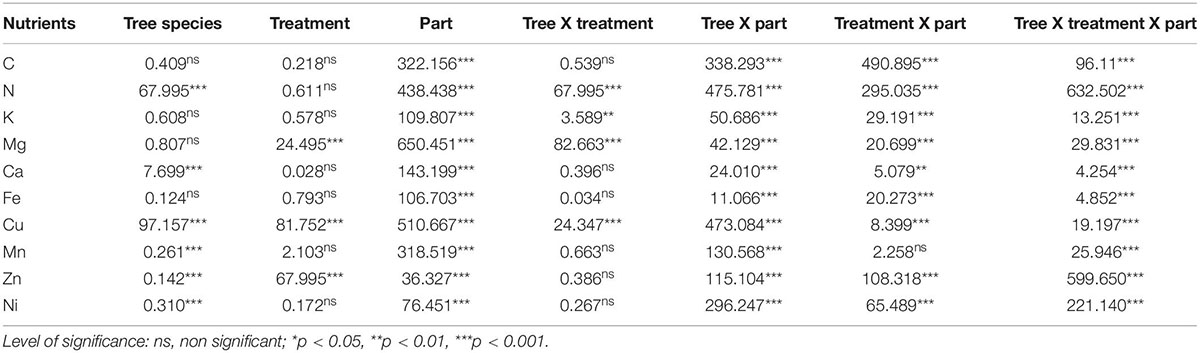
Table 4. F-ratios and level of significance of three-way ANOVA test for various nutrients of the test tree species.
Nutrient Stoichiometry Under the Particulate Matter Stress
Nutrient stoichiometry between C and N was analyzed between different parts of the species under study (Figure 6). In mature leaves, an inconsistency was observed among the trees for C:N ratio. It significantly increased in D. sissoo whereas a decrease was found in M. indica and A. indica. In the senesced leaves, a significant decrease was observed in M. indica and A. indica whereas all others remained unaffected. No differences were found between the C:N ratio of branches in all the tree species under PM treatments.
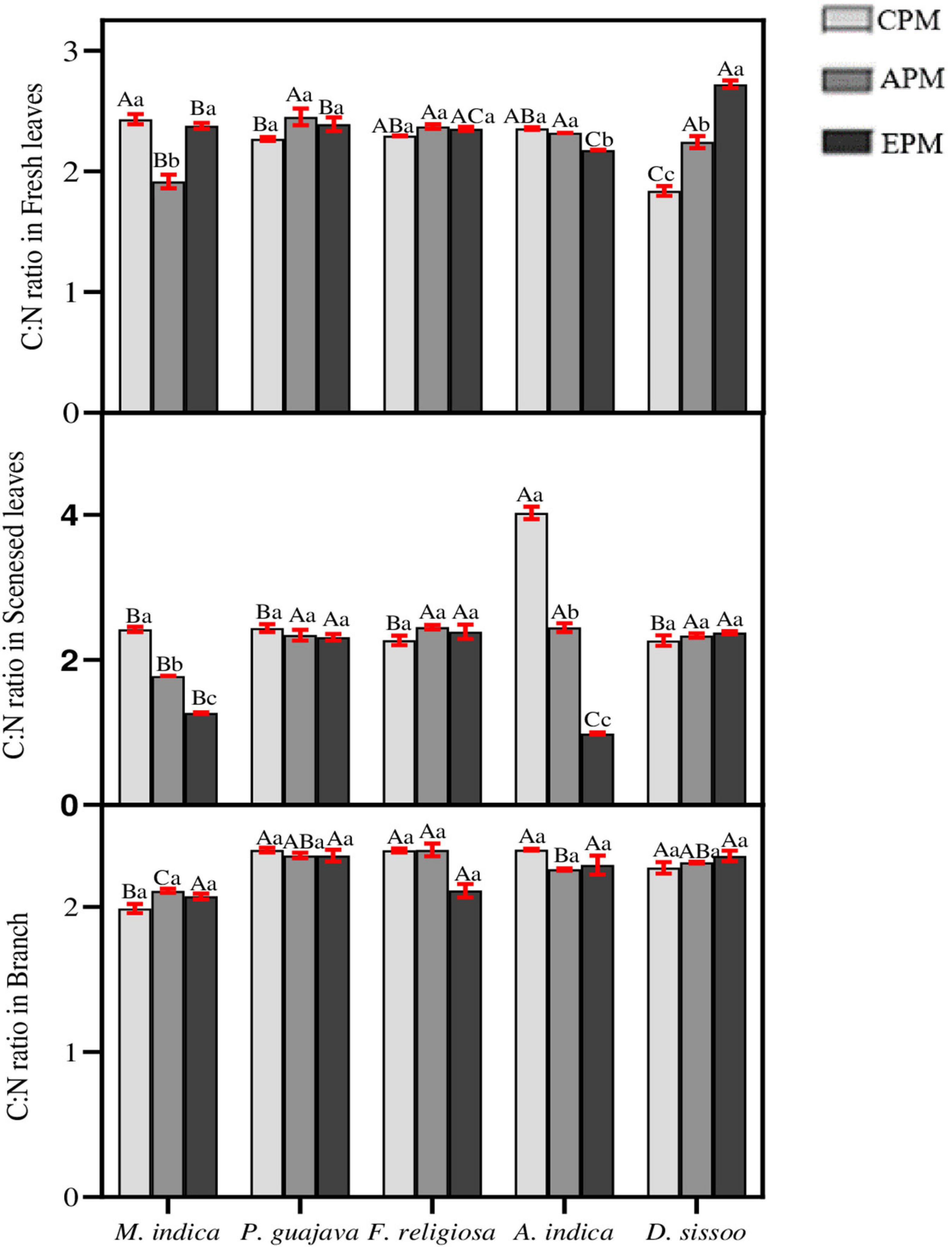
Figure 6. The nutrient stoichiometry of C and N for different tree species under different PM treatments. Different letters in uppercase indicate a significant difference between PM treatments for different trees (p < 0.05) and different lowercase letters indicate a significant difference between the treatment with species (p < 0.05).
Principal Component Analysis
The PCA conducted for parameters under study showed 86.17% of total variance, explained by six PCs (Figure 7). In the course of experiment, the primary component (PC1, eigen value = 6.350, variance = 25.40%) established C, N, NURE-C, and NURE-N as dominant variables. PC2 (eigen value = 5.543, variance = 22.17%) showed Ca, Pn, gs, and E with higher loading values whereas PC3 (eigen value = 4.220, variance = 16.88%) unveiled Ni, Cu, NURE-Cu, NURE-Mn, and NURE-Ni as the variables with higher loading. PC4 (eigen value = 2.520, variance = 10.08%) showed K, Ca, Zn, NURE-K, NURE-Ca, and NURE-Zn as the higher loading variables. PC5 (eigen value = 1.535, variance = 6.14%) demonstrated Mg, Ci, WUE, and NURE-Mg as the dominant variables, while Fe, NURE-Fe, and NURE-Mn found to be loaded maximally on PC6 (eigen value = 1.374, variance = 5.49%).
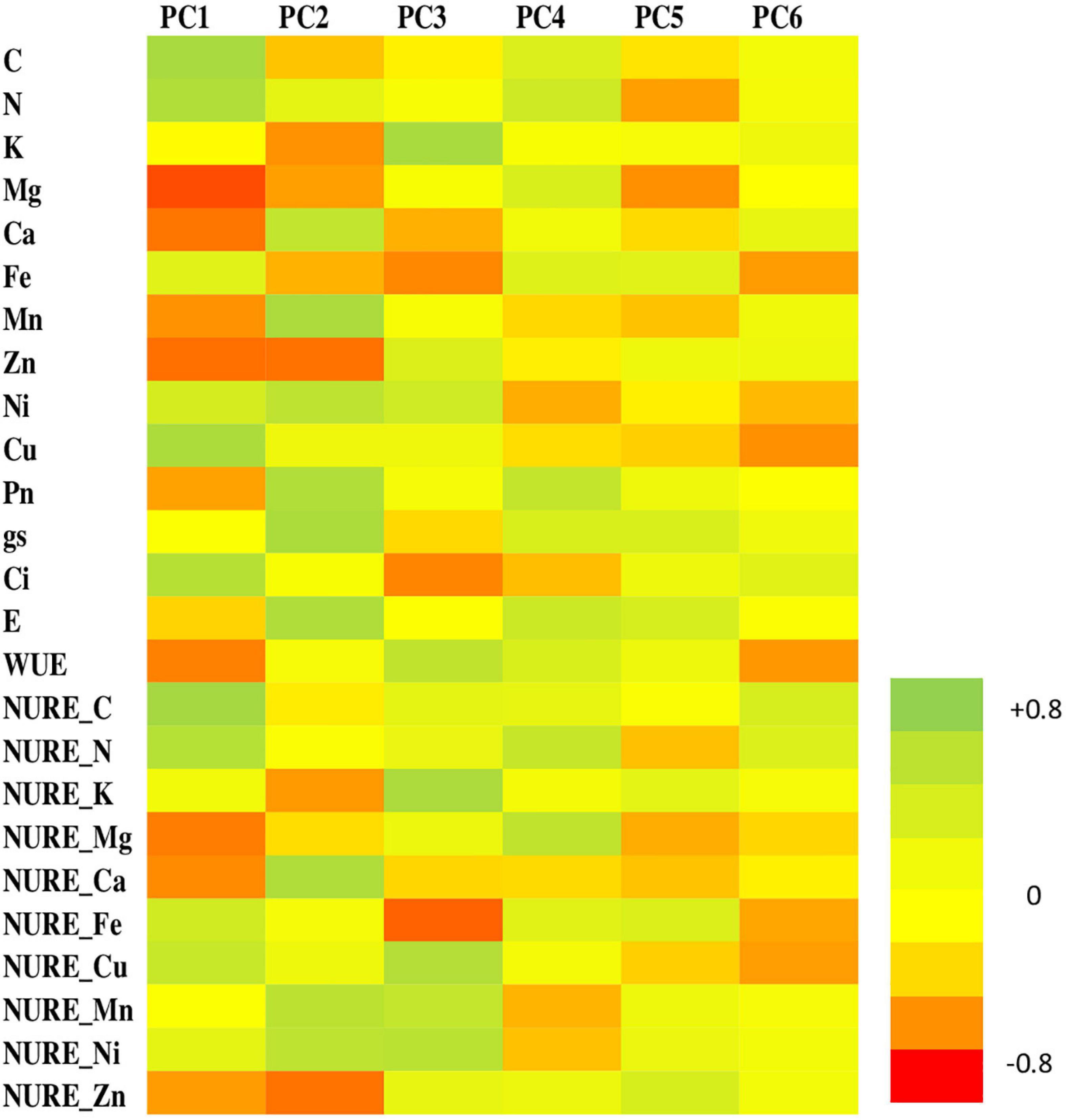
Figure 7. Heat map of six principal components (PCs) showing respective loadings of the parameters during the experimental period.
Discussion
The amount of nutrients required to be re-translocated and the rate of re-translocation is influenced by plant species, mobility of nutrients, and environmental stress conditions (Hagen-Thorn et al., 2004). PM deposition on the foliage of tree species varying in their functional types (evergreen or deciduous) and its consequences on nutrient status, nutrient allocation, and nutrient re-translocation efficiency were investigated in the present study. The physiological performance is assessed in terms of the plant’s photosynthetic capacity. In the present study, Pn showed a marked decline in all the tree species under both APM and EPM and the decrease was more pronounced in EPM. In terms of gs, a significant decrease was observed in M. indica and F. religiosa whereas an increase was observed in P. guajava which can be attributed to its hypostomatic condition as reported in the study of Shiva et al. (2017). A drop in Ci was observed for M. indica, F. religiosa, and A. indica while P. guajava and D. sissoo showed a significant increase which can be attributed to their corresponding stomatal behavior as previously reported by Jaiswal et al. (2021). A significant reduction was observed in EPM for all the tree species except P. guajava and D. sissoo which is also supported by the corresponding change in gs as previously reported in the study of Mahmood et al. (2016). WUE is in line with the E of the studied tree species where a marked increase was observed in WUE with a decrease in EPM.
In the plants, nutrients are required for maintaining the redox balance and changes caused in them due to stress conditions may lead to an unfavorable milieu in the plants (Tripathi et al., 2018). It is already established that the factors promoting plant growth will positively affect the re-translocation efficiency and vice versa (Nambiar and Fife, 1991). The results of this revealed distinct patterns of nutrient allocation and re-translocation in trees belonging to different functional types. The phloem-immobile elements, namely, Ca and Mn, primarily remain in senesced leaves leading to their deficiencies in mature leaves thereby anticipating negative values on reabsorption rates (Shi et al., 2016a). This is in line with our study where Ca displayed a continuous accumulation in senesced leaves and a negative NURE while the accumulation of Mn was found to be greater in the branches to prevent its phytotoxic impacts in mature leaves (Farahat and Linderholm, 2015). Due to the oxidative stress induced in tree species under PM treatment, it required Mn for the increased function of Superoxide Dismutase (SOD) (Alscher et al., 2002) which is the sole reason for its enhancement in treated plants.
The pattern of re-translocation observed for water-soluble elements N, K, and Mg that are phloem-mobile and should be re-translocated from old to younger leaves anticipates a positive value on reabsorption rate (Maillard et al., 2015). This is consistent with our study, where N, K, and Mg were found to be readily translocated from senesced to mature leaves and thereby anticipated positive values on the reabsorption rates of all the species. In spite of positive NURE, the efficiency was greater for PM-stressed plants in M. indica, P. guajava, and F. religiosa compared to CPM. The increase in NURE of N might be due to increased proteolysis under applied stress which would in turn increase the availability of N for re-translocation to actively growing parts of the trees (Temple and Riechers, 1995). In general, Pn is correlated with the N concentration which is displayed by A. indica and D. sissoo (Poorter and Evans, 1998). The major function of K in plants is its involvement in osmoregulation. It can be inferred from the study that PM-induced osmotic stress imposed an increase in K concentration as observed consistently in the PM-treated tree species compared to CPM, thereby favoring physiological processes important for the plants (Hsiao and Läuchli, 1986). It can also be correlated with the transpiration and WUE which decreased in the subsequent PM treatment where K concentration increased (Läuchli, 1984). Hence, the plants water economy depends on the K status of plants (Hsiao and Läuchli, 1986) and retards water loss through transpiration. Mg invariably decreases with an increase in PM load which can be attributed to the antagonistic effect of K over Mg (Ohno and Grunes, 1985). K and Mg exhibited functional synergism in various metabolic processes and Mg can partially replace K in some functions (Xie et al., 2021). Both are found to be negatively correlated in the present study (Figure 8).
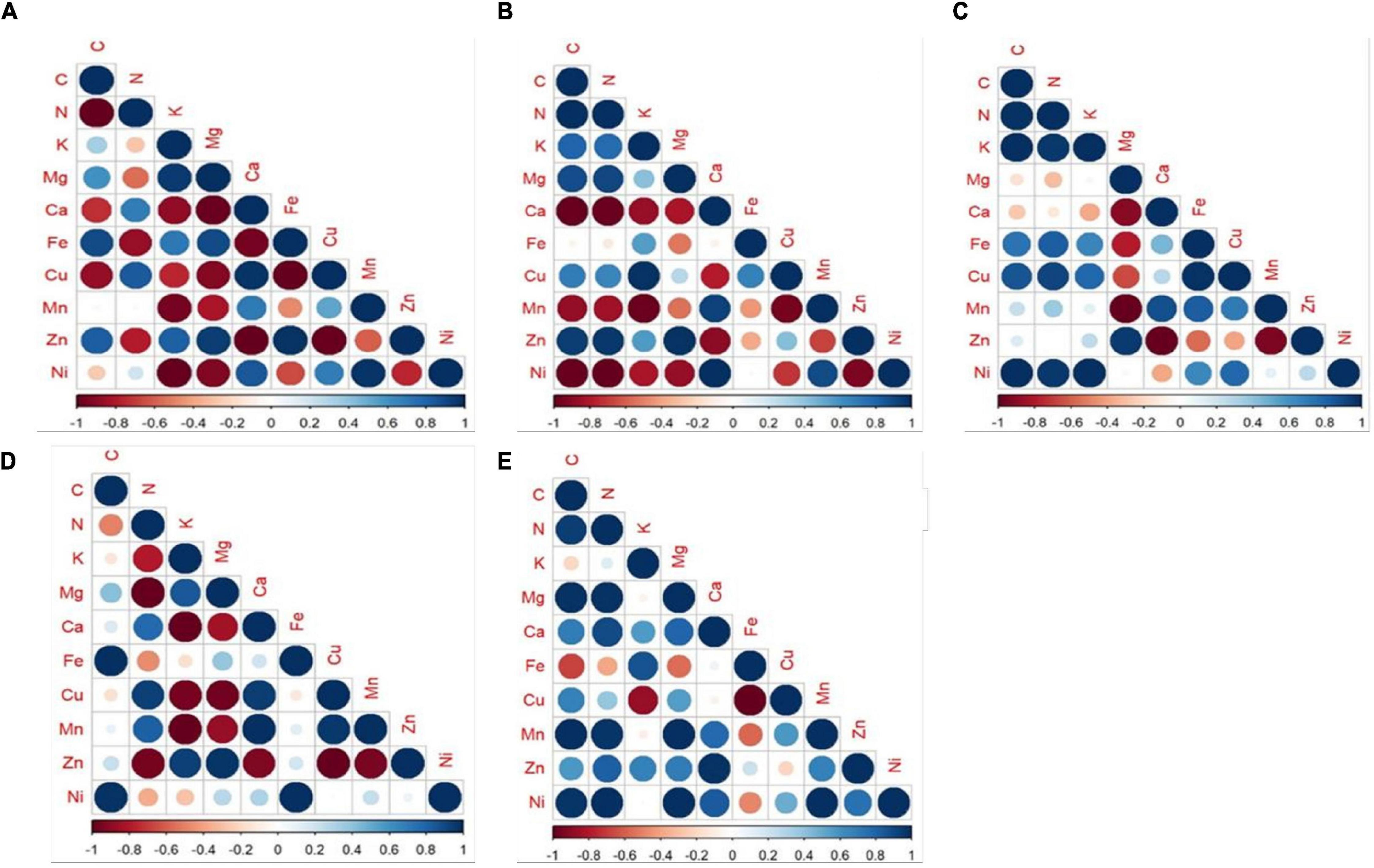
Figure 8. Correlation between the nutrients under study in mature leaves subjected to different treatments of PM- (A) M. indica, (B) P. guajava, (C) F. religiosa, (D) A. indica, (E) D. sissoo. Blue-large dots showed maximum positive correlation and red-large dots showed maximum negative correlation with a gradient of color and size showing in between correlation values.
The micronutrients considered in this study showed negative NURE because of their low mobility and phytotoxic behavior (Farahat and Linderholm, 2015) except Fe. In spite of their negative NURE, Cu and Zn along with Fe (showed positive NURE) showed lesser accumulation in senesced leaves under increasing PM load which might be due to the oxidative stress induced by PM which in turn increased the activity of different groups of SOD, namely, Fe SOD and Cu/Zn SOD (Alscher et al., 2002), which are common elements required for the functioning of SOD. Fe SOD is mainly located in chloroplast and Cu/Zn SOD in the cytosol, chloroplasts, and peroxisomes. The concentrations of Fe, Cu, and Zn were found to decrease in senesced leaves as they are required for the enhanced activity of SOD under PM-induced oxidative stress. Another micronutrient Ni showed negative NURE which might be an effort to protect the tree species from the phytotoxic effect of Ni (Farahat and Linderholm, 2015).
The carbon showed differential allocation and NURE patterns. In M. indica, F. religiosa, and A. indica, the NURE decreased with an increase in PM load which can be correlated with a decrease in gs, which in turn reduces the atmospheric uptake of carbon (Sulman et al., 2016). In P. guajava and D. sissoo, an increase in NURE was observed with increasing PM concentration which might be due to their hypostomatic leaf, thereby facilitating the uptake of carbon which is reflected in the insignificant changes in gs.
The re-translocation efficiency was found to be affected more in evergreen trees with increasing PM load compared to semi-evergreen or deciduous tree species under study. The evergreen trees have a simple leaf type which provided greater surface area for the adherence of PM. Thus, the higher the loading of PM, the greater the impact observed. To meet their higher demand for assimilating translocation, evergreen trees with longer leaf lifespan need to invest more in phloem loading and export apparatus (Bazzaz and Grace, 1997; Givnish, 2002). Thereby increasing the allocation of nutrients more to branches than in leaves as seen in our study. On the contrary, deciduous trees evolved with a short leaf lifespan allocate more nutrients to the leaf as they have a requirement to maximize their photosynthetic activity to utilize the light accessibility during the shorter growing period (Yan et al., 2016). This is in line with the results obtained in our study. Based on the correlation between different elements, it is inferred that the evergreen trees showed more correlation among studied elements compared to the deciduous trees, whereas most of the elements displayed a non-correlating relationship in semi-evergreen tree species (Figure 8). The uptake of nutrients depends on the specific transporter present on the plasma membrane of the cells (Barzana et al., 2021). The differences in the correlation pattern of nutrients observed in the present study might be due to the change in nutrient transporters (number or nature) under the applied stress of PM.
Our results suggest that both APM and EPM imposed significant impacts on the nutrient status of the tree species. About the evergreen tree species, Psidium guajava is relatively more tolerant under the applied stress. In the semi-evergreen tree species, F. religiosa showed a better response and can be considered efficient in enduring the PM stress. Among all the studied species, F. religiosa is marked as an efficient remobilizer due to its ability to remobilize 7 nutrients efficiently out of the studied 10 nutrients with decreasing NURE for most of the nutrients under increasing PM load. Thus, P. guajava and F. religiosa, owing to their better tolerance, can be used extensively for plantation and green belt development in and around urban areas.
Data Availability Statement
The original contributions presented in this study are included in the article/supplementary material, further inquiries can be directed to the corresponding author.
Author Contributions
HS planned and conducted the experiment, did statistical analysis, and data curation. HS and PS wrote the manuscript. MA and SA visualized, investigated, and edited the manuscript. MA supervised the work. All authors contributed to the article and approved the submitted version.
Funding
This work was supported by the CSIR project [Sanction no. 38(1500)/21/EMR-II]. The financial assistance in the form of Junior Research Fellow (IF190187) by DST-INSPIRE, Government of India, to HS is gratefully acknowledged.
Conflict of Interest
The authors declare that the research was conducted in the absence of any commercial or financial relationships that could be construed as a potential conflict of interest.
Publisher’s Note
All claims expressed in this article are solely those of the authors and do not necessarily represent those of their affiliated organizations, or those of the publisher, the editors and the reviewers. Any product that may be evaluated in this article, or claim that may be made by its manufacturer, is not guaranteed or endorsed by the publisher.
Acknowledgments
We express our gratitude to the Head, Department of Botany, and the Coordinator, Institute of Eminence (IOE), Interdisciplinary School of Life Sciences (ISLS) and the Head, Department of Geology, Banaras Hindu University for providing the necessary lab facilities and instrumental support.
References
Aerts, R. (1996). Nutrient resorption from senescing leaves of perennials: are there general patterns? J. Ecol. 84, 597–608. doi: 10.2307/2261481
Alscher, R. G., Erturk, N., and Heath, L. S. (2002). Role of superoxide dismutases (SODs) in controlling oxidative stress in plants. J. Exp. Bot. 53, 1331–1341. doi: 10.1093/jexbot/53.372.1331
Anderson, J. O., Thundiyil, J. G., and Stolbach, A. (2012). Clearing the air: a review of the effects of particulate matter air pollution on human health. J. Med. Toxicol. 8, 166–175. doi: 10.1007/s13181-011-0203-1
Barzana, G., Rios, J. J., Lopez-Zaplana, A., Nicolas-Espinosa, J., Yepes-Molina, L., Garcia-Ibañez, P., et al. (2021). Interrelations of nutrient and water transporters in plants under abiotic stress. Physiol. Plant. 171, 595–619. doi: 10.1111/l.13206
Braun, S., Schindler, C., and Rihm, B. (2020). Foliar nutrient concentrations of European beech in Switzerland: relations with nitrogen deposition, ozone, climate and soil chemistry. Front. For. Glob. Change 3:33. doi: 10.3389/ffgc.2020.00033
Buseck, P. R., and Pósfai, M. (1999). Airborne minerals and related aerosol particles: effects on climate and the environment. Proc. Natl. Acad. Sci. 96, 3372–3379. doi: 10.1073/pnas.96.7.3372
Cao, J., Shang, H., Chen, Z., Tian, Y., and Yu, H. (2016). Effects of elevated ozone on stoichiometry and nutrient pools of Phoebe Bournei (Hemsl.) Yang and Phoebe Zhennan S. Lee et FN Wei seedlings in subtropical China. Forests 7:78. doi: 10.3390/f7040078
Chen, L., Liu, C., Zhang, L., Zou, R., and Zhang, Z. (2017). Variation in tree species ability to capture and retain airborne fine particulate matter (PM2.5). Sci. Rep. 7:3206. doi: 10.1038/s41598-017-03360-1
Escobedo, F. J., and Nowak, D. J. (2009). Spatial heterogeneity and air pollution removal by an urban forest. Landsc. Urban Plan. 90, 102–110. doi: 10.1016/j.landurbplan.2008.10.021
Farahat, E., and Linderholm, H. W. (2015). Nutrient resorption efficiency and proficiency in economic wood trees irrigated by treated wastewater in desert planted forests. Agric. Water Manag. 155, 67–75. doi: 10.1016/j.agwat.2015.03.008
Gaidajis, G. (2003). Ambient concentrations of total suspended particulate matter and its elemental constituents at the wider area of the mining facilities of TVX Hellas in Chalkidiki, Greece. J. Environ. Sci. Health A Tox. Hazard. Subst. Environ. Eng. 38, 2509–2520. doi: 10.1081/ESE-120024443
Givnish, T. J. (2002). Adaptive significance of evergreen vs. deciduous leaves: solving the triple paradox. Silva Fenn. 36, 703–743.
Hagen-Thorn, A., Armolaitis, K., Callesen, I., and Stjernquist, I. (2004). Macronutrients in tree stems and foliage: a comparative study of six temperate forest species planted at the same sites. Ann. For. Sci. 61, 489–498. doi: 10.1051/forest:2004043
Hassan, A., Ilyas, S. Z., Agathopoulos, S., Hussain, S. M., Jalil, A., Ahmed, S., et al. (2021). Evaluation of adverse effects of particulate matter on human life. Heliyon 7:e05968. doi: 10.1016/j.heliyon.2021.e05968
Hsiao, T. C and Läuchli, A. (1986). “Role of potassium in plant-water relations,” in Advances in Plant Nutrition, eds B. Tinker and A. Läuchli (New York: Praeger). 2, 281–312.
Jaiswal, B., Singh, S., Agrawal, S. B., and Agrawal, M. (2021). Assessment of physiological, biochemical and yield responses of wheat plants under natural saline and non-saline field conditions. Physiol. Mol. Biol. Plants 27, 2315–2331. doi: 10.1007/s12298-021-01070-7
Lanuza, O., Casanoves, F., Delgado, D., and Van den Meersche, K. (2019). Leaf litter stoichiometry affects decomposition rates and nutrient dynamics in tropical forests under restoration in Costa Rica. Restor. Ecol. 27, 549–558. doi: 10.1111/rec.12893
Läuchli, A. (1984). “Mechanisms of nutrient fluxes at membranes of the root surface and their regulation in the whole plant,” in Roots, Nutrient and Water Influx, and Plant Growth, eds S.A. Barber, D.R. Bouldin (Hoboken: Wiley). 49, 1–25. doi: 10.2134/asaspecpub49.c1
Liu, J., Cao, Z., Zou, S., Liu, H., Hai, X., Wang, S., et al. (2018). An investigation of the leaf retention capacity, efficiency and mechanism for atmospheric particulate matter of five greening tree species in Beijing. China. Sci. Total Environ. 616, 417–426. doi: 10.1016/j.scitotenv.2017.10.314
Mahmood, S., Daur, I., Al-Solaimani, S. G., Ahmad, S., Madkour, M. H., Yasir, M., et al. (2016). Plant growth promoting rhizobacteria and silicon synergistically enhance salinity tolerance of mung bean. Front. Plant Sci. 7:876. doi: 10.3389/fpls.2016.00876
Maillard, A., Diquélou, S., Billard, V., Laîné, P., Garnica, M., Prudent, M., et al. (2015). Leaf mineral nutrient remobilization during leaf senescence and modulation by nutrient deficiency. Front. Plant Sci. 6:317. doi: 10.3389/fpls.2015.00317
Mina, U., Chandrashekara, T. K., Kumar, S. N., Meena, M. C., Yadav, S., Tiwari, S., et al. (2018). Impact of particulate matter on basmati rice varieties grown in Indo-Gangetic Plains of India: growth, biochemical, physiological and yield attributes. Atmos. Environ. 188, 174–184. doi: 10.1016/j.atmosenv.2018.06.015
Mukherjee, A., and Agrawal, M. (2017). World air particulate matter: sources, distribution and health effects. Environ. Chem. Lett. 15, 283–309. doi: 10.1007/s10311-017-0611-9
Nambiar, E. S., and Fife, D. N. (1991). Nutrient retranslocation in temperate conifers. Tree Physiol. 9, 185–207. doi: 10.1093/treephys/9.1-2.185
Ohno, T., and Grunes, D. L. (1985). Potassium-magnesium interactions affecting nutrient uptake by wheat forage. Soil Sci. Soc. Am. J. 49, 685–690. doi: 10.2136/sssaj1985.03615995004900030032x
Patra, A. K., Gautam, S., and Kumar, P. (2016). Emissions and human health impact of particulate matter from surface mining operation—A review. Environ. Technol. Innov. 5, 233–249. doi: 10.1016/j.eti.2016.04.002
Piao, H. C., Li, S. L., Yan, Z., and Li, C. (2020). Understanding nutrient allocation based on leaf nitrogen isotopes and elemental ratios in the karst region of Southwest China. Agric. Ecosyst. Environ. 294:106864. doi: 10.1016/j.agee.2020.106864
Poorter, H., and Evans, J. R. (1998). Photosynthetic nitrogen-use efficiency of species that differ inherently in specific leaf area. Oecologia 116, 26–37. doi: 10.1007/s004420050560
Popek, R., Przybysz, A., Gawrońska, H., Klamkowski, K., and Gawroński, S. W. (2018). Impact of particulate matter accumulation on the photosynthetic aaratus of roadside woody plants growing in the urban conditions. Ecotoxicol. Environ. Saf. 163, 56–62. doi: 10.1016/j.ecoenv.2018.07.051
Sgrigna, G., Baldacchini, C., Dreveck, S., Cheng, Z., and Calfapietra, C. (2020). Relationships between air particulate matter capture efficiency and leaf traits in twelve tree species from an Italian urban-industrial environment. Sci. Total Environ. 718:137310. doi: 10.1016/j.scitotenv.2020.137310
Shi, C., Eguchi, N., Meng, F., Watanabe, T., Satoh, F., and Koike, T. (2016b). Retranslocation of foliar nutrients of deciduous tree seedlings in different soil condition under free-air O3 enrichment. iForest 9:835–841. doi: 10.3832/ifor1889-009
Shi, C., Kitao, M., Agathokleous, E., Watanabe, M., Tobita, H., Yazaki, K. I., et al. (2016a). Foliar chemical composition of two oak species grown in a free-air enrichment system with elevated O3 and CO2. J. Agric. Meteorol. 72, 50–58. doi: 10.2480/agrmet.D-14-00018
Shi, C., Watanabe, T., and Koike, T. (2017). Leaf stoichiometry of deciduous tree species in different soils exposed to free-air O3 enrichment over two growing seasons. Environ. Exp. Bot. 138, 148–163. doi: 10.1016/j.envexpbot.2017.03.012
Shiva, B., Nagaraja, A., Srivastava, M., and Goswami, A. K. (2017). Determination of Correlation between Stomatal Density and Gas Exchange Traits in Guava. Int. J. Curr. Microbiol. App. Sci 6, 1328–1334. doi: 10.20546/ijcmas.2017.609.160
Singh, S., Pandey, B., Roy, L. B., Shekhar, S., and Singh, R. K. (2021). Tree responses to foliar dust deposition and gradient of air pollution around opencast coal mines of Jharia coalfield, India: gas exchange, antioxidative potential and tolerance level. Environ. Sci. Pollut. Res. 28, 8637–8651. doi: 10.1007/s11356-020-11088-1
Sulman, B. N., Roman, D. T., Yi, K., Wang, L., Phillips, R. P., and Novick, K. A. (2016). High atmospheric demand for water can limit forest carbon uptake and transpiration as severely as dry soil. Geophys. Res. Lett. 43, 9686–9695. doi: 10.1002/2016GL069416
Temple, P. J., and Riechers, G. H. (1995). Nitrogen allocation in ponderosa pine seedlings exposed to interacting ozone and drought stresses. New Phytol. 130, 97–104. doi: 10.1111/j.1469-8137.1995.tb01819.x
Tripathi, A. M., Klem, K., Fischer, M., Orság, M., Trnka, M., and Marek, M. V. (2018). Water availability influences accumulation and allocation of nutrients and metals in short-rotation poplar plantation. Biomass Bioenergy 116, 151–160. doi: 10.1016/j.biombioe.2018.06.010
Xie, K., Cakmak, I., Wang, S., Zhang, F., and Guo, S. (2021). Synergistic and antagonistic interactions between potassium and magnesium in higher plants. Crop J. 9, 249–256. doi: 10.1016/j.cj.2020.10.005
Yan, Z., Li, P., Chen, Y., Han, W., and Fang, J. (2016). Nutrient allocation strategies of woody plants: an aroach from the scaling of nitrogen and phosphorus between twig stems and leaves. Sci Rep. 6:20099. doi: 10.1038/srep20099
Zhang, W., Zhang, Y., Gong, J., Yang, B., Zhang, Z., Wang, B., et al. (2020). Comparison of the suitability of plant species for greenbelt construction based on particulate matter capture capacity, air pollution tolerance index, and antioxidant system. Environ. Pollut. 263:114615. doi: 10.1016/j.envpol.2020.114615
Keywords: particulate matter, tree species, nutrients, stoichiometry, allocation, re-translocation efficiency
Citation: Singh H, Singh P, Agrawal SB and Agrawal M (2022) Implications of Foliar Particulate Matter Deposition on the Physiology and Nutrient Allocation of Dominant Perennial Species of the Indo-Gangetic Plains. Front. Plant Sci. 13:939950. doi: 10.3389/fpls.2022.939950
Received: 09 May 2022; Accepted: 15 June 2022;
Published: 19 July 2022.
Edited by:
Yanbo Hu, Northeast Forestry University, ChinaReviewed by:
Puja Khare, Central Institute of Medicinal and Aromatic Plants (CSIR), IndiaRavindra Khaiwal, Post Graduate Institute of Medical Education and Research (PGIMER), India
Dheeraj Rathore, Central University of Gujarat, India
Huiliang Liu, Xinjiang Institute of Ecology and Geography (CAS), China
Indra Jeet Chaudhary, Central University of Gujarat, India
Copyright © 2022 Singh, Singh, Agrawal and Agrawal. This is an open-access article distributed under the terms of the Creative Commons Attribution License (CC BY). The use, distribution or reproduction in other forums is permitted, provided the original author(s) and the copyright owner(s) are credited and that the original publication in this journal is cited, in accordance with accepted academic practice. No use, distribution or reproduction is permitted which does not comply with these terms.
*Correspondence: Madhoolika Agrawal, bWFkaG9vLmFncmF3YWxAZ21haWwuY29t