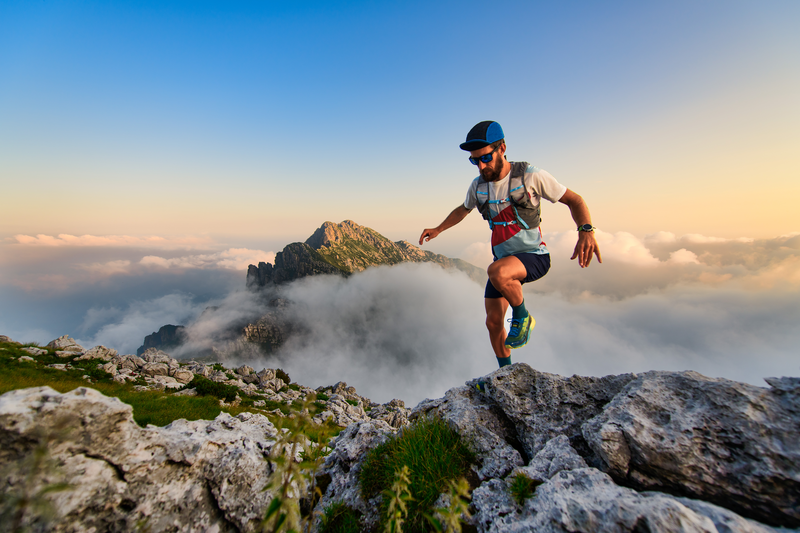
94% of researchers rate our articles as excellent or good
Learn more about the work of our research integrity team to safeguard the quality of each article we publish.
Find out more
ORIGINAL RESEARCH article
Front. Plant Sci. , 08 August 2022
Sec. Crop and Product Physiology
Volume 13 - 2022 | https://doi.org/10.3389/fpls.2022.934618
Adjusting the sowing date to optimize temperature conditions is a helpful strategy for mitigating the adverse impact of high temperature on summer maize growth in the North China Plain (NCP). However, the physiological processes of variation in summer maize yield with sowing date-associated changes in temperature conditions around flowering remain to be poorly understood. In this study, field experiments with two maize varieties and three sowing dates (early sowing date, SD1, 21 May; conventional sowing date, SD2, 10 June; delay sowing date, SD3, 30 June) were conducted at Xinxiang of Henan Province in 2019 and 2020. Early sowing markedly decreased the daily mean temperature (Tmean), maximum temperature (Tmax), and minimum temperature (Tmin) during pre-silking, while delay sowing markedly decreased those temperatures during post-silking. Under these temperature conditions, both varieties under SD1 at 12-leaf stage (V12) and silking stage (R1) while under SD3 at R1 and milking stage (R3) possessed significantly lower malondialdehyde (MDA) content in leaf due to higher activities of superoxide dismutase (SOD), peroxidase (POD), and catalase (CAT) compared to SD2. Therefore, SD1 at V12 and R1 stages and SD3 at R1 and R3 stages for both varieties showed significantly higher photosynthetic capacity, including higher SPAD, Fv/Fm, Pn, Tr, and Gs, which promoted greater pre-silking dry matter (DM) accumulation for SD1 to increase the kernel number, and promoted greater post-silking DM accumulation for SD3 to increase the kernel weight, eventually increased the grain yield of SD1 and SD3 compared to SD2. Results of regression analysis demonstrated that Tmean, Tmax, and Tmin values from V12 to R1 stages lower than 26.6, 32.5, and 20.3°C are necessary for improving the kernel number, while Tmean, Tmax, Tmin, and accumulated temperature (AT) values from R1 to R3 stages lower than 23.2, 28.9, 17.3, and 288.6°C are necessary for improving the kernel weight. Overall, optimal temperature conditions around flowering can be obtained by early (21 May) or delay (30 June) sowing to improve the kernel number or kernel weight due to improved photosynthetic capacity, eventually increasing the grain yield of summer maize in the NCP.
Maize (Zea mays L.) is one of the most important cereal crops in China and plays an important role in ensuring food security. The North China Plain (NCP) is one of the major maize production areas in China, accounting for 35% of the cultivated maize and 40% of the grain yield for maize production across the whole country (China Agricultural Yearbook, 2016). However, the climate has become warmer and warmer across the northern part of China in recent years (Tao et al., 2006; Liu et al., 2012), and the frequency of extreme high-temperature events has increased (Xiao et al., 2016), which could result in more than 15% decrease for maize yield or even no harvest (Chen et al., 2011; Liu et al., 2013).
In the NCP, summer maize is commonly planted immediately after winter wheat annually in the winter wheat-summer maize cropping system. From 2013 to 2018, the summer maize suffered from high-temperature stress yearly, with the duration extending from 10 to 30 days, and the temperature in most areas reached above 35°C, even reached over 40°C in some areas (Hua et al., 2020). Furthermore, the high-temperature stress commonly occurs in late July to early August, which is around the flowering stage of summer maize (Xu et al., 2021). Generally, the crop yield reduction promoted by the high-temperature stress around the flowering stage has been associated with a decrease in grain number and weight (Prasad et al., 2015). However, the damages to reproductive organs and losses of grain yield in maize vary with changing stages that high-temperature stress occurs (Lizaso et al., 2018). High-temperature stress (≥35°C) occurs during the maize flowering stage and results in a significant decrease of grain number by affecting pollen and silks activity, pollination, and fertilization, which is considered to be the main reason for maize yield reduction under high-temperature stress (Ehsan et al., 2015; Yan et al., 2018; Shao et al., 2021). Cicchino et al. (2010) and Rattalino et al. (2014) also found that the grain abortion caused by high-temperature stress (≥35°C) occurs after pollination and fertilization has a great effect on the grain number per ear of maize. The decrease of grains per ear could be over 88% under severe high-temperature stress (Suwa et al., 2010; Rattalino et al., 2014). Moreover, high-temperature stress during the grain filling stage could lower the grain filling rate and period, resulting in insufficient assimilate supply to grain, and then reduce maize grain weight and yield (Tao et al., 2016; Zhou et al., 2017). Therefore, for ensuring sustainable maize production in the NCP, it is essential to improve the understanding of summer maize grain yield responses to varying temperature conditions around flowering and explore suitable management practices to counteract the adverse effects of high-temperature stress on maize growth.
Adjusting the sowing date is an effective strategy to mitigate the adverse effects of climatic factors by optimizing the climate conditions during the crop growth period (Santos et al., 2017; Coelho et al., 2021; Deng et al., 2022; Li et al., 2022). Our previous study has demonstrated that sowing date-associated variation in temperature was the primary factor that influenced maize grain yield in the NCP (Zhou et al., 2016, 2017). However, under the variation of temperature conditions around flowering stage associated with sowing dates, the physiological determinants of summer maize yield changes and the quantitative relationship between maize yield traits and temperatures around the flowering stage remain to be poorly understood. Therefore, a 2-year field experiment with two maize varieties and three sowing dates was conducted in NCP. The purpose of this study was to (a) determine the effect of early and delay sowing date on the temperature conditions around the maize flowering stage; (b) evaluate the effects of temperature conditions around the flowering stage on the pre- or post-silking dry matter (DM) accumulation, photosynthetic parameters, anti−oxidative properties, grain yield, and yield components of maize; and (c) quantify the relationship between maize yield traits and temperatures around the flowering stage.
Two field experiments were conducted in 2019 and 2020 at the Xinxiang Experimental Station of Chinese Academy of Agricultural Sciences (35°11inxiang Ex°48i08E), Xinxiang County, Henan Province, China. This area is a warm temperate continental monsoon climate, with annual average temperature of 14°, accumulated temperature (AT) above 10°C of 4,647°C, sunshine hours of 2,324 h, and precipitation of 573 mm. The average daily mean temperature and effective AT above 10°C during the maize growing season were 25 and 1,900°C, respectively. The International Soil Science Society (ISSS) Classification was used on the soil of this experimental field. In the 0–40 cm plow layer, the content of organic matter was 12.6 g kg–1, the content of available nitrogen was 62.2 mg kg–1, the content of available phosphorus was 16.7 mg kg–1, the content of available potassium was 109.8 mg kg–1, and the pH value was 8.1.
A completely randomized block design with three replications was used in this experiment. The treatments were done on three sowing dates, namely, 21 May (early sowing), 10 June (conventional sowing), and 30 June (delay sowing), which were called SD1, SD2, and SD3, respectively. Maize hybrids ZD958 and XY335, which were widely planted in this area, were used in this study. The previous crop winter wheat was planted on 31 October and harvested on 20 May. After wheat harvest, maize was planted at a density of 60,000 plants ha–1, with 0.6 m row spacing. Each plot was 15 m long and 4.8 m wide and consisted of 8 rows. Experimental plots were irrigated prior to sowing seeds, and basal fertilizer was applied at the rates of 130 kg N ha–1, 120 kg P2O5 ha–1, and 90 kg K2O ha–1. Additional nitrogen fertilizer (120 kg N ha–1) was top-dressed at the beginning of the jointing stage. The amount of fertilizer applied was based on the existing levels of N, P, and K (determined from soil tests) to ensure that there was no nutrient deficiency. All the experimental plots were well managed, and no obvious water stress, diseases, or insect pests were found during the growing period.
The meteorological data [daily mean temperature (Tmean), daily maximum temperature (Tmax), daily minimum temperature (Tmin), precipitation, and sunshine hours] during the experimental periods in 2019 and 2020 were provided by the Chinese Meteorological Administration (2020).
The effective AT was obtained by summing up the mean daily temperatures during the period in which the mean daily temperature was above a base temperature in each day (Yan et al., 2011).
where T0 is the base temperature (10°C for maize).
The solar radiation was calculated using the equation (Gao et al., 2018):
where Q is the total solar radiation, Q0 is the astronomical radiation, S is the actual sunshine hours, S0 is the possible sunshine hours, S/S0 is the proportion of sunshine, and a and b are correction coefficients.
The date was recorded when more than 50% of the maize plants in each plot reached the following stages: the emergency stage (VE), 6-leaf stage (V6), 12-leaf stage (V12), silking stage (R1), milking stage (R3), and physiological maturity (R6).
Green leaf areas of the sampled plants were measured at V6, V12, R1, R3, and R6 stages. For each leaf, the length and maximum width were recorded, and the leaf area was computed based on the following formula (Tian et al., 2018):
Leaf area index (LAI) was calculated using the following formula:
Plant samples were collected to determine DM content at V6, V12, R1, R3, and R6 stages. Three adjacent plants in each row were sampled randomly from each plot. The sampled plants were dried at 105°C for 30 min and then at 70°C to maintain constant moisture content before being weighed.
The SPAD-502 chlorophyll analyzer was used to determine the SPAD value of 15 marked ear leaves for each plot at V12, R1, and R3 stages, with the determination method referring to the literature (Earl and Tollen, 1997).
The photosynthetic parameters, such as photosynthetic rate (Pn), transpiration rate (Tr), and stomatal conductance (Gs), of 15 marked ear leaves for each plot were measured between 10:00 and 12:00 on sunny days at V12, R1, and R3 stages using a Li-6400 photosynthesis system (Li-Cor Inc., Lincoln, NB, United States). The photosynthetically active radiation, CO2 concentration in the leaf chamber, and leaf temperature were set at 1,800 μmol m–2 s–1, 400 μmol mol–1, and 30 ± 4°C, respectively, and the relative humidity was 50–60%.
Chlorophyll fluorescence parameters of the middle part of 15 ear leaves for each plot were determined with pocket PEA between 10:00 and 12:00 at V12, R1, and R3 stages on sunny days using a Li-6400 photosynthesis system. After a 20-min dark adaptation at the central region of a leaf using black leaf clips, the initial fluorescence (F0) and maximum fluorescence (Fm) were determined. Maximal photochemical efficiency of PSII is given as follows:
Five marked ear leaves for each plot were sampled at V12, R1, and R3 stages. The required enzyme solution for measuring the activity of superoxide dismutase (SOD), peroxidase (POD), and catalase (CAT) was extracted according to Bao et al. (2022). The SOD activity was recorded at 560 nm and defined as the amount of SOD required to produce a 50% inhibition of reduction of nitroblue tetrazolium (NBT); the POD activity was defined as the increase in absorbance of every 30 s at 470 nm; the CAT activity was assayed as a decrease in absorbance at 240 nm for 1 min, followed by the decomposition of hydrogen peroxide (H2O2). The content of malondialdehyde (MDA) was measured with thiobarbituric acid (TBA).
At harvest, 36 m2 of the crop area was harvested manually from the four center rows in each plot. The ears were counted at harvest from the four center rows in each plot to determine the number of ears per hectare. The 1,000-kernel weight was calculated as the average of three random samples of 500 kernels. The kernel number was recorded as the mean kernel number of 10 ears from each replication. Grain yield was calculated at 14% moisture content.
Data preparation was performed using Microsoft Excel 2016. Grain yield, kernel number, kernel weight, photosynthetic parameters, MDA, SOD, POD, and CAT were subjected to a two-way ANOVA with sowing date and variety as fixed effects, using the general linear model of SPSS 20.0 (SPSS Institute, Inc.). DM was subjected to repeated measure analyses with sowing date and sampling stage (repeated measurement) as fixed effects. Residual normality was tested using quantile-quantile plots, while the variance homogeneity was obtained by Levene’s test. Means were compared using the least significant difference (LSD) test at a 5% level of probability. Nonlinear regression analysis was performed by using SPSS.
As shown in Table 1, the daily Tmean, Tmax, Tmin, effective AT, and Ra during the maize growth period varied by sowing date. Early sowing (SD1) markedly decreased the values of Tmean, Tmax, and Tmin during pre-silking, while delay sowing (SD3) markedly decreased the values of Tmean, Tmax, Tmin, and AT during post-silking for both varieties in 2 years. Compared to SD2, Tmean, Tmax, Tmin, and AT averagely decreased by 11.8, 10.5, 12.4, and 16.3% from V12 to R1 stages under SD1, while those values averagely decreased by 13.6, 15.6, 25.7, and 24.6% from R1 to R3 stages under SD3, respectively. Different from temperature trends, early sowing increased the accumulated radiation during sowing to V12, R1 to R3, and R3 to R6 stages, whereas delay sowing decreased them. Compared to SD2, SD1 increased the accumulated radiation averagely by 12.2, 10.9, and 8.5%, while SD3 decreased the accumulated radiation averagely by 7.9, 3.4, and 2.7% during sowing to V12, R1 to R3, and R3 to R6 stages, respectively.
Table 1. Daily mean temperature (Tmean), maximum temperature (Tmax), daily minimum temperature (Tmin), effective accumulated temperature (AT), and accumulated radiation (Ra), during the maize growth stage in 2019 and 2020.
The changes in temperature and radiation conditions contributed to the variation in phenological stages of maize (Table 2). Compared to SD2, SD1 increased the growth period before silking and after silking by 4.8 and 4.0 days, respectively, while SD3 decreased the growth period before silking by 3.3 days, and increased the growth period after silking by 9.5 days.
Both the sowing date and variety affected the MDA content and the SOD, POD, and CAT activities of the ear leaf (Figure 1). SD1 decreased the MDA content of ear leaf by 9.5 and 9.3% at V12, and 19.6 and 25.6% at R1, respectively, compared to SD2 across 2 years. Meanwhile, SD3 decreased the MDA content of ZD958 and XY335 by 9.7 and 21.6% at R1, and 12.5 and 12.1% at R3, respectively, compared to SD2 across 2 years.
Figure 1. MDA content (A–C) and activities of SOD (D–F), POD (G–I), and CAT (J–L) of maize ear leaf at V12, R1, and R3 stages under different sowing dates in 2019 and 2020. SD1, early sowing; SD2, conventional sowing; SD3, delay sowing; POD, peroxidase; CAT, catalase; MDA, malondialdehyde; V12, 12-leaf stage; R1, silking stage; R3, milking stage. Different letters above the error bars indicate significant difference at the 0.05 probability level.
Contrary to MDA content, SD1 increased the SOD, POD, and CAT activities of ZD958 by 14.8, 10.6, and 9.5% at V12, and 56.2, 59.7, and 55.5% at R1, while SD1 increased those of XY335 by 13.5, 10.4, and 11.9% at V12, and 73.7, 69.0, and 61.0% at R1, respectively, compared with SD2 across 2 years. Meanwhile, SD3 increased the SOD, POD, and CAT activities of ZD958 by 36.8, 41.7, and 42.8% at R1, and 20.7, 11.1, and 11.0% at R3, while SD3 increased those of XY335 by 48.8, 62.2, and 62.4% at R1, and 23.3, 10.9, and 11.2% at R3, respectively, compared with SD2 across 2 years.
Pn, Tr, and Gs varied by sowing dates and varieties (Figures 2A–I). Under SD1, Pn, Tr, and Gs of ZD958 increased by 9.3, 11.2, and 16.8% at V12, and 12.2, 14.2, and 20.3% at R3, while those of XY335 increased by 7.9, 10.6, and 8.7% at V12, and 24.8, 30.3, and 37.2% at R3, than those under SD2 across 2 years, respectively. Under SD3, Pn, Tr, and Gs of ZD958 increased by 5.9, 5.8, and 8.7% at R1, and 5.4, 7.2, and 9.7% at R3, while those of XY335 increased by 17.7, 21.3, and 22.5% at R1, and 5.7, 8.4, and 9.7% at R3, than those under SD2 across 2 years, respectively.
Figure 2. Pn (A–C), Tr (D–F), Gs (G–I), Fv/Fm (J–L), and SPAD (M–O) values of maize ear leaf at V12, R1, and R3 stages under different sowing dates in 2019 and 2020. SD1, early sowing; SD2, conventional sowing; SD3, delay sowing; Pn, photosynthetic rate; Tr, transpiration rate; Gs, stomatal conductance; Fv/Fm, chlorophyll fluorescence parameters; V12, 12-leaf stage; R1, silking stage; R3, milking stage. Different letters above the error bars indicate significant difference at the 0.05 probability level.
The Fv/Fm and SPAD value also varied by sowing dates and varieties (Figures 2J–O). Compared with SD2, the Fv/Fm and SPAD value of ZD958 under SD1 increased by 9.8 and 6.7% at V12, and 20.6 and 18.9% at R3, while those of XY335 increased by 8.1 and 6.8% at V12, and 236.4 and 36.8% at R3 across 2 years, respectively. Meanwhile, the Fv/Fm and SPAD value of ZD958 under SD3 increased by 10.6 and 11.6% at R1, and 8.1 and 7.3% at R3, while those of XY335 increased by 27.1 and 28.6% at V12, and 6.1 and 5.9% at R3 across 2 years, respectively.
The DM accumulation varied by sowing dates, varieties, and growth stages (Figure 3). Under SD1, the DM accumulation of ZD958 and XY335 averagely increased by 18.7 and 18.8% from V6 to V12 stages, and 43.4 and 36.2% from V12 to R1 stages, compared to SD2, respectively. Under SD3, the DM accumulation of ZD958 and XY335 averagely increased by 9.5 and 18.3% from R1 to R3 stages, and 5.6 and 13.2% from R3 to R6 stages, than that under SD2, respectively. Therefore, early sowing significantly increased maize DM accumulation pre-silking, while delay sowing significantly increased maize DM accumulation post-silking for both varieties in both years. Meanwhile, in both years, the DM of XY335 at V12, R1, R3, and R6 stages was greater than that of ZD958 under SD1 and SD3, while no significant variation was found between ZD958 and XY335 under SD2.
Figure 3. Dry matter accumulation of maize for each growth stage under different sowing dates in 2019 (A,B) and 2020 (C,D).
The kernel number, kernel weight, and grain yield were also affected by sowing date and variety (Figure 4). SD1 averagely increased the kernel number of ZD958 and XY335 by 13.7 and 22.1%, than that of SD2 in 2 years, respectively. However, SD3 averagely increased the kernel weight of ZD958 and XY335 by 7.5 and 14.7% in 2 years, respectively. Therefore, the grain yield of ZD958 and XY335 averagely increased by 11.2 and 17.8% under SD1, and 5.6 and 12.2% under SD3 in 2 years, compared to SD2, respectively. Meanwhile, XY335 revealed a greater kernel number under SD1 and greater kernel weight under SD3 than ZD958, and the grain yield of XY335 was greater than that of ZD958 under SD1 and SD3 in both years.
Figure 4. Kernel number (A,B), kernel weight (C,D), and grain yield (E,F) of maize under different sowing dates in 2019 and 2020. SD1, early sowing; SD2, conventional sowing; SD3, delay sowing. Different letters above the error bars indicate significant difference at the 0.05 probability level.
As shown in Table 3, kernel number was positively correlated with Pn, Tr, Gs, Fv/Fm, SPAD, MDA, SOD, POD, and CAT at V12 and R1 stages, kernel weight was correlated with those physiological parameters at the R3 stage, and grain yield was correlated with those physiological parameters at the R1 stage.
Correlation and regression analyses were performed between climatic factors and kernel number, kernel weight, and grain yield of maize (Table 4). Negative correlations were found between kernel number and Tmean, Tmax, and Tmin from V12 to R1 stages, between kernel weight and Tmean, Tmax, Tmin, and AT from R1 to R3 stages, and between grain yield and Tmean, Tmax, Tmin, and AT from V12 to R1 stages. There were no significant correlations between radiation and kernel number, kernel weight, and grain yield at each stage.
Table 4. Relationships between temperatures during maize growth stage and kernel number, kernel weight, and grain yield.
A significant linear relationship existed between kernel number and Tmean, Tmax, and Tmin from V12 to R1 stages (Figure 5), between kernel weight and Tmean, Tmax, Tmin, and AT from R1 to R3 stages (Figure 6), and between grain yield and Tmean, Tmax, Tmin, and AT from R1 to R3 stages (Figure 7). When Tmean, Tmax, and Tmin from V12 to R1 stages were higher than 26.6, 32.5, and 20.3°C, and Tmean, Tmax, Tmin, and AT from R1 to R3 stages were higher than 23.2, 28.9, 17.3, and 288.6°C, respectively, the kernel number, kernel weight, and grain yield decreased.
Figure 5. Relationships between kernel number and daily mean temperature (A), daily maximum temperature (B), and daily minimum temperature (C) from V12 to R1. **Significant at the 0.01 probability level.
Figure 6. Relationships between kernel weight and daily mean temperature (A), daily maximum temperature (B), daily minimum temperature (C), and effective accumulated temperature (D) from R1 to R3. **Significant at the 0.01 probability level.
Figure 7. Relationships between grain yield and daily mean temperature (A), daily maximum temperature (B), daily minimum temperature (C), and effective accumulated temperature (D) from V12 to R1. **Significant at the 0.01 probability level.
Previous studies have demonstrated that adjusting the sowing date is an effective way to avoid the adverse effects of climate conditions on maize growth by changing the climatic factors during the maize growth period (Zhang et al., 2020; Coelho et al., 2021; Tian et al., 2021). Our results showed that compared to conventional sowing (SD2), early sowing (SD1) markedly decreased Tmean, Tmax, and Tmin during pre-silking (especially from V12 to R1 stages), while delay sowing (SD3) markedly decreased those temperatures during post-silking (especially from R1 to R3 stages), which are closely related to the grain formation and yield of maize (Badu–Apraku et al., 1983). Therefore, SD1 and SD3 averagely increased the maize yield by 11.2 and 17.8% compared to SD2, respectively. Yield decrease for SD2 due to high temperature during flowering was associated with a reduction in kernel number and kernel weight. The kernel number of SD1 and kernel weight of SD3 were greater than that of SD2 for both varieties in both years, respectively. This finding could be confirmed by previous studies that high temperature could significantly affect the kernel number and kernel weight of maize (Borrás and Gambín, 2010; Zhang et al., 2019).
Photo-assimilated carbon as the primary factor in determining maize yield generally decreases with increasing temperature (Roger et al., 1983; Yan et al., 2017; Zheng et al., 2018). In this study, high temperatures (Tmean, Tmax, and Tmin) occurred from V12 to R3 stages under the SD2 condition, which was greater than that for SD1 from V12 to R1 stages, and for SD3 from R1 to R3 stages. High-temperature stress could increase the number of reactive oxygen species (ROS) such as superoxide anion radical (O2–), H2O2, and hydroxyl radical (OH–) (Liu and Huang, 2000; Barnabas et al., 2008) and then result in the membrane peroxidation and accumulation of MDA (Xu et al., 2006). Moreover, although high-temperature stress could increase the activities of SOD, POD, and CAT to remove sufficient ROS (Blokhina et al., 2003; Obata et al., 2015), the activities of these enzymes decreased with an increase in the frequency of extreme high-temperature events (Dat et al., 1998; Doru, 2021). Therefore, maize under SD2 had greater MDA content with lower activity of SOD, POD, and CAT in maize ear leaf compared to SD1 at V12 and R1 stages, and SD3 at R1 and R3 stages. This could damage the structure of a photosynthetic system and reduce the electron transport efficiency and chlorophyll content (Prasad et al., 2011), thus decreasing the photosynthetic rate (Veerasamy et al., 2007) and the assimilate capacity of a leaf (Sinsawat et al., 2004; Rattalino et al., 2014). However, the lower temperatures for SD1 from V12 to R1 stages, while for SD3 from R1 to R3 stages compared to SD2, promoted maize leaf to maintain higher antioxidant capacity (e.g., lower MDA and higher activities of SOD, POD, and CAT) and photosynthetic activity (e.g., higher SPAD, Fv/Fm, Pn, Tr, and Gs). As a result, the pre-silking DM accumulation for SD1 and the post-silking DM accumulation for SD3 averagely increased by 17.9 and 8.4% compared to SD2, respectively. Greater pre-silking DM accumulation of maize had an effect on developing kernels, with greater translocation of DM to help establish viable embryos (He et al., 2005; Yan et al., 2017; Yu et al., 2021), while greater post-silking DM accumulation improved the capacity of maize to provide assimilates during grain filling (Echarte et al., 2008; Ning et al., 2013; Paponov et al., 2020). Moreover, optimal temperature condition could increase the activities of key enzymes in carbon metabolism of grain, and promote the transport, transformation, and accumulation of assimilates into grains (Suwa et al., 2010). Consequently, kernel number of SD1 and kernel weight of SD3 for both varieties significantly increased in both years, which contributed to increase the yield of SD1 and SD3 compared to SD2.
Previous studies have suggested that Tmean of 24–26°C is required for maize flowering to achieve a high yield of maize (Xu et al., 2021). Tmax of more than 32°C during the pre-silking stage reduces the kernel number, while Tmax of more than 25°C during the grain-filling stage decreases the kernel weight (Wilhelm et al., 1999). Our results showed that the kernel number was negatively correlated with Tmean, Tmax, and Tmin from V12 to R1 stages, while the kernel weight was negatively correlated with Tmean, Tmax, Tmin, and AT from R1 to R3 stages. The results of regression analysis indicated that high temperatures (Tmean > 26.6°C, Tmax > 32.5°C, and Tmin > 20.3°C) from V12 to R1 stages could decrease the kernel number, while high temperatures (Tmean > 23.2°C, Tmax > 28.9°C, Tmin > 17.3°C, and AT > 288.6°C) from R1 to R3 stages could decrease the kernel weight, eventually decreasing the grain yield. These findings were consistent with earlier studies that high-temperature stress during the 9th leaf stage to the tasseling stage of maize could decrease the grain number at the ear tip (Shao et al., 2021), while high-temperature stress during the grain filling stage could lower the filling rate and reduce the kernel weight (Tao et al., 2016). Therefore, in our study, SD1 had greater kernel number and SD3 had greater kernel weight compared to SD2 due to optimized temperature condition around flowering. However, Tian et al. (2018) found that late sowing of maize could lead to the late grain filling phase coincided with decreasing temperature (average minimum temperature was 11.3 and 12.9°C 5 and 10 days before maturity, respectively), thus decreasing the grain filling rate. As we know, DM accumulation in kernels depends on both kernel growth rate and duration of grain filling (Borrás and Gambín, 2010), and lower temperature decreases grain filling rate while extending grain filling duration (Jones et al., 1981). In our study, the average minimum temperature in SD3 was lower than 12°C from R3 to R6 stages, which may decrease the grain filling rate, but the duration of grain filling (after silking) in SD3 increased by 9.5 days compared with SD2. Several studies also demonstrate that the solar radiation and rainfall are also limiting factors for kernel weight of late sowing maize (Gao et al., 2018; Li et al., 2022). In our study, the maize was fully irrigated in the field experiment, and no obvious water stress was observed during the growing season. Meanwhile, SD3 decreased the radiation from R1 to R3 and R3 to R6 only by 3.4 and 2.7% compared to SD2, and by 12.8 and 10.4% compared to SD1 across years and varieties, respectively, which might decrease the production of photosynthate post-silking (Gao et al., 2018). However, a previous study has demonstrated that 19–34% of the grain yield maize was from remobilization of the reserved carbon pre-silking (He et al., 2005), while the increased duration of grain filling of SD3 could provide more time for remobilization of DM pre-silking to increase the kernel weight. We concluded that temperature conditions around flowering of summer maize with early sowing (21 May) or delay sowing (30 June) in the NCP are suitable for kernel growth and a relatively high maize yield can be obtained.
Obviously, under global climate change conditions, adjusting the sowing date could be considered an effective way to avoid the adverse effects of high temperature on maize growth by changing the temperature conditions during the growing period of maize. However, maize hybrid with different maturations also could be chosen to make the crop growth match well with the changing climatic conditions. This study is an important future research priority for us.
Early sowing markedly decreased the daily mean temperature (Tmean), maximum temperature (Tmax), and minimum temperature (Tmin) during pre-silking, while delay sowing markedly decreased those temperatures during post-silking. Thus, maize of SD1 at V12 and R1 stages while SD3 at R1 and R3 stages showed lower MDA content and higher activities of CAT and POD, and higher SPAD, Fv/Fm, Pn, Tr, and Gs. As a result, SD1 accumulated greater pre-silking DM to increase the kernel number, while SD3 accumulated greater post-silking DM to increase the kernel weight, eventually increasing the grain yield of SD1 and SD3 compared to SD2. We concluded that early sowing (21 May) or delay sowing (30 June) could optimize the temperature conditions around the flowering of summer maize to improve kernel growth and obtain a relatively higher maize yield in the NCP.
The original contributions presented in the study are included in the article/supplementary material, further inquiries can be directed to the corresponding author/s.
BZ, ML, and MZ conceived and designed the experiment. DG, CC, XL, and RW carried out the experiments and performed the statistical analyses. DG, CC, and BZ drafted the manuscript. ZD, WM, XW, and CL reviewed and edited the manuscript. All authors contributed to the article and approved the submitted version.
This study was supported by the Central Public-Interest Scientific Institution Basal Research Fund (S2022ZD05), the China Agriculture Research System of MOF and MARA (CARS-02), and the CAAS Science and Technology Innovation Program (2060302-2).
The authors declare that the research was conducted in the absence of any commercial or financial relationships that could be construed as a potential conflict of interest.
All claims expressed in this article are solely those of the authors and do not necessarily represent those of their affiliated organizations, or those of the publisher, the editors and the reviewers. Any product that may be evaluated in this article, or claim that may be made by its manufacturer, is not guaranteed or endorsed by the publisher.
Badu–Apraku, B., Hunter, R. B., and Tollenaar, M. (1983). Effect of temperature during grain filling on whole plant and grain–yield in maize (Zea mays L.). Can. J. Plant Sci. 63, 357–363. doi: 10.4141/cjps83-040
Bao, Y. Z., Xing, J. P., Liang, Y., Ren, Z. P., Fu, L. H., Yu, J., et al. (2022). Analysis of overwintering indexes of winter wheat in alpine regions and establishment of a cold resistance model. Field Crops Res. 275:108347. doi: 10.1016/j.fcr.2021.108347
Barnabas, B., Jager, K., and Feher, A. (2008). The effect of drought and heat stress on reproductive processes in cereals. Plant Cell Environ. 31, 11–38.
Blokhina, O., Eija, V., and Kurt, V. (2003). Antioxidants oxidative damage and oxygen deprivation stress: A review. Ann. Bot. 91, 179–194. doi: 10.1093/aob/mcf118
Borrás, L., and Gambín, B. L. (2010). Trait dissection of maize kernel weight: Towards integrating hierarchical scales using a plant growth approach. Field Crops Res. 118, 1–12. doi: 10.1016/j.fcr.2010.04.010
Chen, C. Q., Lei, C. X., Deng, A. X., Qian, C. R., and Zhang, W. J. (2011). Will higher minimum temperatures increase corn production in Northeast China. An analysis of historical data over 1965–2008. Agr. Forest. Meteorol. 151, 1580–1588. doi: 10.1016/j.agrformet.2011.06.013
China Agricultural Yearbook (2016). China agricultural yearbook. Beijing: China Agricultural Publishing House.
Chinese Meteorological Administration (2020). Chinese meteorological administration archives. Beijing: Chinese Meteorological Administration.
Cicchino, M., Edreira, J., Uribelarrea, M., and Otegui, M. E. (2010). Heat stress in field–grown maize: Response of physiological determinants of grain yield. Crop Sci. 50, 1438–1448. doi: 10.2135/cropsci2009.10.0574
Coelho, A. E., Sangoi, L., Junior, A., Fioreze, S. L., and Júnior, M. (2021). Growth patterns and yield of maize (Zea mays L.) hybrids as affected by nitrogen rate and sowing date in southern Brazil. Crop Pasture Sci. 71, 976–986. doi: 10.1071/CP20077
Dat, J. F., Lopez, D. H., Foyer, C. H., and Scott, I. M. (1998). Parallel changes in H2O2 and catalase during thermotolerance induced by salicylic acid or heat acclimation in mustard seedlings. Plant Physiol. 116, 1351–1357. doi: 10.1104/pp.116.4.1351
Deng, F., Zhang, C., He, L. H., Liao, S., Li, Q. P., Li, B., et al. (2022). Delayed sowing date improves the quality of mechanically transplanted rice by optimizing temperature conditions during growth season. Field Crops Res. 281:108493. doi: 10.1016/j.fcr.2022.108493
Doru, A. (2021). Effects of heat stress on photosystem II activity and antioxidant enzymes in two maize cultivars. Planta 253, 1–15. doi: 10.1007/s00425-021-03611-6
Earl, H. J., and Tollen, M. (1997). Maize leaf absorptance of photosynthetically active radiation and its estimation using a chlorophyll meter. Crop Sci. 37, 436–440. doi: 10.2135/cropsci1997.0011183X003700020022x
Echarte, L., Rothstein, S., and Tollenaar, M. (2008). The response of leaf photosynthesis and dry matter accumulation to nitrogen supply in an older and a newer maize hybrid. Crop Sci. 48, 656–665. doi: 10.2135/cropsci2007.06.0366
Ehsan, E. R., Heidi, W., Thomas, G., and Jesse, B. N. (2015). Heat stress in cereals: Mechanisms and modelling. Eur. J. Agron. 64, 98–113. doi: 10.1016/j.eja.2014.10.003
Gao, Z., Feng, H. Y., Liang, X. G., Zhang, L., Lin, S., Zhao, X., et al. (2018). Limits to maize productivity in the North China Plain: A comparison analysis for spring and summer maize. Field Crops Res. 2018, 39–47. doi: 10.1016/j.fcr.2018.08.022
He, P., Zhou, W., and Jin, J. Y. (2005). Carbon and nitrogen metabolism related to grain formation in two different senescent types of maize. J. Plant Nutr. 27, 295–311. doi: 10.1081/PLN-120027655
Hua, Y., Hu, Q., Pan, X. B., Ma, X. Q., Hu, L. T., Wang, X. C., et al. (2020). Characteristics of high temperature heat damage and suitable sowing date of summer maize at flowering stage under climate change in North China Plain. Chin. J. Agrometeorol. 41, 1–15.
Jones, R. J., Gengenback, B. G., and Cardwell, V. B. (1981). Temperature effects on In vitro kernel development of maize. Agron. J. 21, 761–766. doi: 10.2135/cropsci1981.0011183X002100050033x
Li, T., Zhang, X. P., Liu, Q., Yan, P., Liu, J., Chen, Y. Q., et al. (2022). Yield and yield stability of single cropping maize under different sowing dates and the corresponding changing trends of climatic variables. Field Crops Res. 285, 108589. doi: 10.1016/j.fcr.2022.108589
Liu, X. Z., and Huang, B. R. (2000). Heat stress injury in relation to membrane lipid peroxidation in creeping bent grass. Crop Sci. 40, 503–510. doi: 10.2135/cropsci2000.402503x
Liu, Y. E., Xie, R. Z., Hou, P., Li, S. K., Zhang, H. B., Ming, B., et al. (2013). Phenological responses of maize to changes in environment when grown at different latitudes in China. Field Crops Res. 144, 192–199. doi: 10.1016/j.fcr.2013.01.003
Liu, Z. J., Yang, X. G., Hubbard, K. G., and Lin, X. M. (2012). Maize potential yields and yield gaps in the changing climate of northeast China. Glob. Change Biol. 18, 3441–3454. doi: 10.1111/j.1365-2486.2012.02774.x
Lizaso, J. I., Ruiz, R. M., Rodríguez, L., Gabaldon, L. C., Oliveira, J. A., Lorite, I. J., et al. (2018). Impact of high temperatures in maize: Phenology and yield components. Field Crops Res. 216, 129–140. doi: 10.1016/j.fcr.2017.11.013
Ning, P., Li, S., Yu, P., Zhang, Y., and Li, C. J. (2013). Post–silking accumulation and partitioning of dry matter, nitrogen, phosphorus and potassium in maize varieties differing in leaf longevity. Field Crops Res. 44, 19–27. doi: 10.1016/j.fcr.2013.01.020
Obata, T., Witt, S., Lisec, J., Palacios, R. N., Florez, S. I., Yousfi, S., et al. (2015). Metabolite profiles of maize leaves in drought, heat, and combined stress field trials reveal the relationship between metabolism and grain yield. Plant Physiol. 169, 2665–2683. doi: 10.1104/pp.15.01164
Paponov, I. A., Paponov, M., Sambo, P., and Engels, C. (2020). Differential regulation of kernel set and potential kernel weight by nitrogen supply and carbohydrate availability in maize genotypes contrasting in nitrogen use efficiency. Front. Plant Sci. 11:586. doi: 10.3389/fpls.2020.00586
Prasad, P. V. V., Djanaguiraman, M., Perumal, R., and Ciampitti, I. A. (2015). Impact of high temperature stress on floret fertility and individual grain weight of grain sorghum: Sensitive stages and thresholds for temperature and duration. Front. Plant Sci. 6:820. doi: 10.3389/fpls.2015.00820
Prasad, P. V. V., Pisipati, S. R., Momcilovic, I., and Ristic, Z. (2011). Independent and combined effects of high temperature and drought stress during grain filling on plant yield and chloroplast EF-Tu expression in spring wheat. J. Agron. Crop Sci. 197, 430–441. doi: 10.1111/j.1439-037X.2011.00477.x
Rattalino, E. J. I., Mayer, L. I., and Otegui, M. E. (2014). Heat stress in temperate and tropical maize hybrids: Kernel growth, water relations and assimilate availability for grain filling. Field Crops Res. 166, 162–172. doi: 10.1016/j.fcr.2014.06.018
Roger, M. W., Keys, A. J., Lea, P. J., and Miflin, B. J. (1983). Photosynthesis, photorespiration and nitrogen metabolism. Plant Cell Environ. 6, 301–309. doi: 10.1111/1365-3040.ep11612102
Santos, R. D., Boote, K. J., Sollenberger, L. E., Neves, A. L. A., Pereira, L. G. R., Scherer, C. B., et al. (2017). Simulated optimum sowing date for forage pearl millet cultivars in multilocation trials in Brazilian Semi-Arid region. Front. Plant Sci. 8:2074. doi: 10.3389/fpls.2017.02074
Shao, R. X., Yu, K. K., Li, H. W., Jia, S. J., Yang, Q. H., Zhao, X., et al. (2021). The effect of elevating temperature on the growth and development of reproductive organs and yield of summer maize. J. Integr. Agr. 20, 1783–1795. doi: 10.1016/S2095-3119(20)63304-4
Sinsawat, V., Leipner, J., Stamp, P., and Fracheboud, Y. (2004). Effect of heat stress on the photosynthetic apparatus in maize (Zea mays L.) grown at control or high temperature. Environ. Exp. Bot. 52, 123–129. doi: 10.1016/j.envexpbot.2004.01.010
Suwa, R., Hakata, H., Hara, H., and Hany, E. S. (2010). High temperature effects on photosynthate partitioning and sugar metabolism during ear expansion in maize (Zea mays L.) genotypes. Plant Physiol. Bioch. 48, 124–130. doi: 10.1016/j.plaphy.2009.12.010
Tao, F. L., Yokozawa, M., Xu, Y. L., Yousay, H., and Zhang, Z. (2006). Climate changes and trends in phenology and yields of field crops in China, 1981–2000. Agr. Forest Meteorol. 138, 82–92. doi: 10.1016/j.agrformet.2006.03.014
Tao, Z. Q., Chen, Y. Q., Li, C., Zou, J. X., Yan, P., Yuan, S. F., et al. (2016). The causes and impacts for heat stress in spring maize during grain filling in the North China Plain–A review. J. Integr. Agr. 15, 2677–2687. doi: 10.1016/S2095-3119(16)61409-0
Tian, B. J., Zhu, J. C., Liu, X. W., Huang, S. B., and Wang, P. (2021). Interacting leaf dynamics and environment to optimize maize sowing date in North China Plain. J. Integr. Agr. 19, 1227–1240. doi: 10.1016/S2095-3119(19)62831-5
Tian, B. J., Zhu, J. C., Nie, Y. S., Xu, C. L., Meng, Q. F., and Wang, P. (2018). Mitigating heat and chilling stress by adjusting the sowing date of maize in the North China Plain. J Agro Crop Sci. 205, 77–87. doi: 10.1111/jac.12299
Veerasamy, M., He, Y., and Huang, B. (2007). Leaf senescence and protein metabolism in creeping bent grass exposed to heat stress and treated with cytokinin. J. Am. Soc. Hortic. Sci. 132, 467–472. doi: 10.21273/JASHS.132.4.467
Wilhelm, E. P., Mullen, R. E., Keeling, P. L., and Singletary, G. W. (1999). Heat stress during grain filling in maize: Effects on kernel growth and metabolism. Crop Sci. 39, 1733–1740. doi: 10.2135/cropsci1999.3961733x
Xiao, D. P., Qi, Y. Q., Shen, Y. J., Tao, F. L., Moiwo, J. P., Liu, J. F., et al. (2016). Impact of warming climate and cultivar change on maize phenology in the last three decades in North China Plain. Theor. Appl. Climatol. 124, 653–661. doi: 10.1007/s00704-015-1450-x
Xu, S., Li, J. L., Zhang, X. Q., and Wei, H. (2006). Effects of heat acclimation pretreatment on changes of membrane lipid peroxidation, antioxidant metabolites, and ultrastructure of chloroplasts in two cool–season turfgrass species under heat stress. Environ. Exp. Bot. 56, 274–285. doi: 10.1016/j.envexpbot.2005.03.002
Xu, Y. H., Liu, T. X., Fang, W. S., and Li, S. Y. (2021). Risk analysis of high temperature heat injury of summer maize at flowering stage in Henan Province. Chin. J. Agrometeorol. 42, 879–888.
Yan, M. H., Liu, X. T., Zhang, W., Li, X. J., and Liu, S. (2011). Spatio–temporal changes of =10°C accumulated temperature in northeastern China since 1961. Chinese Geogr. Sci. 21, 17–26. doi: 10.1007/s11769-011-0438-4
Yan, P., Chen, Y. Q., Sui, P., Alison, V., and Zhang, X. P. (2018). Effect of maize plant morphology on the formation of apical kernels at different sowing dates and under different plant densities. Field Crops Res. 223, 83–92. doi: 10.1016/j.fcr.2018.04.008
Yan, P., Tao, Z. Q., Chen, Y. Q., Zhang, X. P., and Sui, P. (2017). Spring maize kernel number and assimilate supply responses to high-temperature stress under field conditions. Agron. J. 109, 1433–1441. doi: 10.2134/agronj2016.11.0662
Yu, Y., Qian, C. R., Gu, W. R., and Li, C. F. (2021). Responses of root characteristic parameters and plant dry matter accumulation, distribution and transportation to nitrogen levels for spring maize in Northeast China. Agriculture 11:308. doi: 10.3390/agriculture11040308
Zhang, L. L., Zhang, Z., Luo, Y. C., Cao, J., and Li, Z. Y. (2020). Optimizing genotype–environment–management interactions for maize farmers to adapt to climate change in different agro–ecological zones across China. Sci. Total Environ. 728:138614. doi: 10.1016/j.scitotenv.2020.138614
Zhang, X. P., Cheng, J. L., Wang, B., Yan, P., Dai, H. C., Chen, Y. Q., et al. (2019). Optimum sowing dates for high-yield maize when grown as sole crop in the North China Plain. Agronomy 9:198. doi: 10.3390/agronomy9040198
Zheng, Y. P., Guo, L. L., Hou, R. X., Zhou, H. R., Hao, L. H., Li, F., et al. (2018). Experimental warming enhances the carbon gain but does not affect the yield of maize (Zea mays L.) in the North China Plain. Flora 240, 152–163. doi: 10.1016/j.flora.2018.02.001
Zhou, B. Y., Yue, Y., Sun, X. F., Ding, Z. S., Ma, W., and Zhao, M. (2017). Maize kernel weight responses to sowing date–associated variation in weather conditions. Crop J. 5, 43–51. doi: 10.1016/j.cj.2016.07.002
Keywords: summer maize, sowing date, temperature conditions, photosynthetic capacity, grain yield
Citation: Guo D, Chen C, Li X, Wang R, Ding Z, Ma W, Wang X, Li C, Zhao M, Li M and Zhou B (2022) Adjusting sowing date improves the photosynthetic capacity and grain yield by optimizing temperature condition around flowering of summer maize in the North China Plain. Front. Plant Sci. 13:934618. doi: 10.3389/fpls.2022.934618
Received: 02 May 2022; Accepted: 18 July 2022;
Published: 08 August 2022.
Edited by:
Ep Heuvelink, Wageningen University and Research, NetherlandsReviewed by:
Anuj Kumar, University of Arkansas, United StatesCopyright © 2022 Guo, Chen, Li, Wang, Ding, Ma, Wang, Li, Zhao, Li and Zhou. This is an open-access article distributed under the terms of the Creative Commons Attribution License (CC BY). The use, distribution or reproduction in other forums is permitted, provided the original author(s) and the copyright owner(s) are credited and that the original publication in this journal is cited, in accordance with accepted academic practice. No use, distribution or reproduction is permitted which does not comply with these terms.
*Correspondence: Ming Li, bGltaW5nQG5lYXUuZWR1LmNu; Baoyuan Zhou, emhvdWJhb3l1YW5AY2Fhcy5jbg==
†These authors have contributed equally to this work
Disclaimer: All claims expressed in this article are solely those of the authors and do not necessarily represent those of their affiliated organizations, or those of the publisher, the editors and the reviewers. Any product that may be evaluated in this article or claim that may be made by its manufacturer is not guaranteed or endorsed by the publisher.
Research integrity at Frontiers
Learn more about the work of our research integrity team to safeguard the quality of each article we publish.