- National Key Laboratory of Crop Biology, Agronomy College of Shandong Agricultural University, Tai'an, China
Clarifying factors that underpinning the variation in wheat yield components between high and middle soil fertility fields is critical to increase grain production and narrow yield gap for smallholder farming systems in the Huang–Huai–Hai Plain (3HP), which characterized by a large variation in soil fertility. Two-year field experiments were conducted to investigate wheat tillering, leaf photosynthesis, and grain filling characteristics in different soil fertility fields: high soil fertility field (HF) and middle soil fertility field (MF). Results showed that the spike formation rate in HF was 12.7%–13.0% higher than that in MF, leading to an 18.0%–19.8% increase in spike number. In addition, HF improved canopy light interception and leaf photosynthesis characteristics after anthesis and delayed leaf senescence, contributing to the increase in both the active grain filling period and grain filling rate. This resulted in a higher 1,000 grain weight in HF, which was 8.2%–8.3% higher than that in MF. Compared to MF, HF obtained higher yields at 9,840 kg ha−1 in 2017/18 and 11,462 kg ha−1 in 2018/19, respectively. In summary, higher spike number and 1,000-grain weight, which were mediated by spike-formation rate, maximization of light interception and improved leaf photosynthesis. These results would have important implications for narrowing yield gap between MF and HF in the 3HP.
Introduction
Food production is a great challenge in China for its limited farmland resources and large population of more than 1.40 billion citizens need to be fed (Lu and Fan, 2013; National Bureau of Statistics of China, 2020). The Huang–Huai–Hai Plain (3HP) is the primary winter wheat production region in China, producing approximately 61% of the wheat grown in China (Xu et al., 2018). In this region, the mean yield potential for winter wheat varied between 5,000 and 8,000 kg ha−1 from 1981 to 2008 (Chen et al., 2017), with the most recent attainable potential yield at 12,611 kg ha−1 between 2018 and 2019 (Farmer’s Daily, 2019).1 This yield gap can be attributed to irrigation, high-yield wheat cultivars, and different gradients of soil fertility in croplands arising from fertilization replenishment (Xiao and Tao, 2014; Chen et al., 2017; Wang et al., 2018). Smallholder fields in the 3HP have played a dominant role in crop production for decades (Feng et al., 2020), and these farming systems are characterized by a large variation in soil fertility (Tittonell et al., 2007). To increase wheat production and narrow the yield gap, studies on the physiological characteristics of wheat grown in a high soil fertility field (HF) with high potential yield are pivotal.
Soil fertility is defined as the quality of a soil to supply nutrients in adequate amounts and proper balance for the growth of crop plants (Fageria, 2007). Hag Husein et al. (2021) indicated that soil fertility increases as the soil organic matter (SOM) content increases. Soil nutrient supply and nutrient cycling in different soil fertility fields substantially affect crop growth and grain yield (Kautz et al., 2013; Zhang et al., 2018, 2019a). Wang et al. (2018) reported that nitrogen (N) contributes to the maintenance of SOM, and SOM at 10.0–14.9 g kg−1, total nitrogen (TN) at 1.0–1.5 g kg−1, available phosphorus (AP) at 10.0–19.8 mg kg−1, and available potassium (AK) at 100–200 mg kg−1 were the most effective soil fertility levels to improve wheat grain yield. Soil potassium levels had significant effect in tillering, spike number, and yield of wheat (Abbas et al., 2013). The application of maize straw mulch combined with no-tillage could increase plant-available soil N, thus promoting wheat tillering and grain yield (Yang et al., 2020). When water availability was not limited for wheat production, increased soil N arising from increased N application improved the tiller appearance rate, thereby leading to an increase in the maximum tiller number (Alzueta et al., 2012). Rational populations ensure superior canopy light interception and penetration and improve the light utilization efficiency of plants (Hussain et al., 2013). There have been many studies on the response of wheat tillering generation to different soil fertility fields, but most of these have focused on yield levels ranging from 4,500 to 9,000 kg ha−1 (Allard et al., 2013; Yang et al., 2020). However, there is a knowledge gap regarding the changes in soil nutrient content and tillering of winter wheat in fields with grain yield levels ranging from 9,000 to 11,000 kg ha−1.
Moreover, increased photosynthetic capacity and delayed leaf senescence are especially important for increasing crop yields (Wang et al., 2020). It is well known that flag-leaf photosynthesis contributes approximately 30%–50% of the assimilation for grain filling (Guo et al., 2015), whereas leaf photosynthetic performance would inevitably decline during grain filling because the initiation of grain filling coincides with the onset of senescence and the photosynthetic apparatus gradually breaks down (Man et al., 2017). Different soil nutrient status arising from tillage methods influenced the photosynthetic capacity and aging process of wheat, thereby grain filling (He et al., 2020). HF with grain-yield levels up to 11,000 kg ha−1 provides an excellent opportunity to identify physiological features associated with the attainable potential yield of wheat; therefore, it is necessary to study leaf photosynthesis and senescence and grain filling characteristics during the grain filling stage in HF.
This study aimed to investigate the differences in soil nutrient content, wheat tillering, light interception characteristics, photosynthesis and the senescence of flag leaves and grain filling characteristics of two different soil fertility fields with different grain-yield levels. This can be helpful to clarify the variation of yield components in different soil fertility fields and provide useful information for high-yielding wheat production.
Materials and methods
Experimental site description
Field experiments were conducted at Shijiawangzi village (116°41′E, 35°42’N), located in Yanzhou, Shandong Province, China. This area has a temperate, continental monsoon climate with an annual average temperature of 13.6°C, an annual cumulative sunshine hour of 2,460.9 h, an annual rainfall of 621.2 mm, of which 40% is received during the winter wheat-growing season (October–June). The rotation of winter wheat and summer maize is the main cropping system in this region. Daily precipitation during the wheat-growing season in 2017/18 and 2018/19 are shown in Figure 1.
Experimental design
High soil fertility wheat field (with grain-yield levels up to 11,000 kg ha−1) and middle soil fertility wheat field (with grain-yield levels up to 9,000 kg ha−1) were selected and represented by HF and MF, respectively. HF and MF are flat area and belong to two farmers. The straight-line distance between HF and MF is 100 m. The soil type of HF and MF is Haplic luvisol (FAO classification system). Before wheat sowing, additional 3,750 kg (0.65%N, 0.23% P2O5 and 0.78% K2O content) organic fertilizer was applied regularly in HF from 2001 to 2016, but the organic fertilizer was applied in MF only in 2016. The HF and MF with wheat yields of 11,015 and 9,336 kg ha−1, respectively, in 2016/17, and the yield gap between HF and MF was mainly caused by soil fertility (Yu et al., 2018). Annual precipitation were the same in HF and MF. The soil texture, bulk density, and field capacity at the experimental site before sowing are shown in Supplementary Tables S1 and S2. The soil particle size distribution was analyzed using the hydrometer method, and the triangle coordinates of the American system of soil texture classification was used to determine the soil texture. Based on the analysis of soil samples collected from the experimental site in 2017 and 2018 before sowing, the initial amount of soil nutrients (0–20 cm soil layer) in the two fields are shown in Table 1.
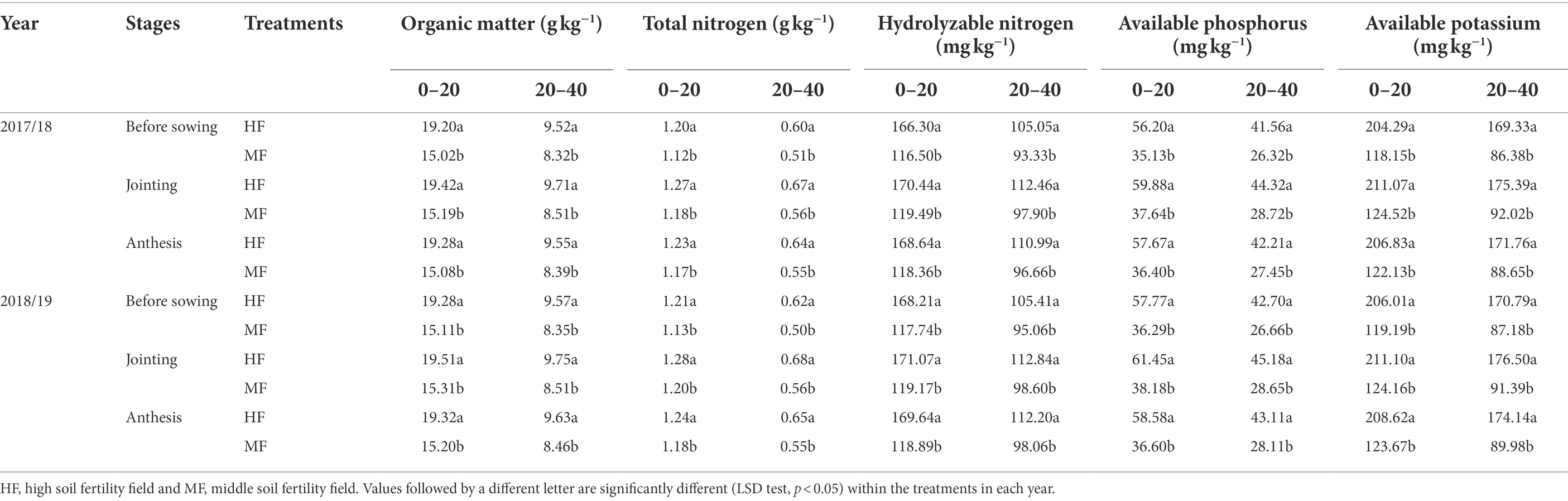
Table 1. Soil nutrient conditions in the 0–20 and 20–40 cm soil layers under different treatments before sowing, at jointing, and anthesis stages in 2017/18 and 2018/19 growing seasons.
Seeds of wheat cultivar Yannong 1212 (broken the yield record of winter wheat twice in China since 2015 and showed high yields of over 12,000 kg ha−1) (Dazhong Daily, 2020)2 were planted in HF and MF. The plot size was 60 m2 (30 m long × 2 m wide) with three replicates and had a 2.0-m wide isolation area setting between each plot to minimize the effects of adjacent plots. The straw of the preceding maize crop was crushed and returned to the soil. Base fertilizer of 105 kg ha−1 N, 150 kg ha−1 P2O5, and 150 kg ha−1 K2O were surface-applied to the soil before sowing. At the jointing stage, each plot was fertilized with 165 kg ha−1 N by ditching. Fertilizer types were urea (46.4% N content), diammonium phosphate (46% P2O5 and 18% N content), and potassium sulfate (52% K2O content). Wheat seeds were sown on 23 October 2017, and 10 October 2018, with a population of 270 plants m−2 and 180 plants m−2, respectively. Wheat plants were harvested on 7 June 2018 and 11 June 2019. Other crop management was the same as used in local wheat production.
Sampling and measurements
Soil nutrient contents
Soil samples from 0 to 20 and 20 to 40 cm soil layers were randomly collected using a soil corer from each plot with three replicates before sowing, 1 day before irrigation at jointing and anthesis. SOM was determined using the K2Cr2O7-H2SO4 oxidation method, and TN was determined using the semimicro-Kjeldahl method (He et al., 2019). Soil hydrolyzable N, AP, and AK were measured using the routine soil sample laboratory analytical method described by Bao (2000).
Tillering production and survival
The number of maximum tillers at re-greening was determined by counting all the stems from three random sample areas of 5 m2 in each plot. The spike number at maturity was estimated by counting all spikes from the harvested area of 5 m2 in each plot. The tillering capacity (no. plant−1) and spike formation rate (%) were calculated using the equation described by Yang et al. (2020):
Photosynthetically active radiation measurements
Intercepted photosynthetically active radiation (PARi) was measured using the SS1 SunScan canopy analysis system (Delta-T Devices, Cambridge, United Kingdom) in three randomly non-destructive sampling areas from each plot between 10:00 am and 11:30 am at 7-day intervals from anthesis to 35 days after anthesis (DAA). With the reference photosynthetically active radiation (PAR) sunshine sensor, the incident PAR was measuring above and beneath wheat canopy by the 1-m probe. The fraction of PARi (PARF, %) was estimated according to the method described by Davidenco et al. (2020) and Liu et al. (2020):
where PAR0 (μmol m−1 s−1) is the incident PAR at the surface of the ground and PARt (μmol m−1 s−1) is the incident PAR at 50 cm above the wheat canopy.
Gas exchange parameters of flag leaves
Flag-leaf gas exchange parameters, including net photosynthetic rate (Pn) and transpiration rate (Tr), were measured using a LI-6400XT portable gas exchange measuring system (LI-COR, Lincoln, Nebraska, United States). Measurements were taken with three representative flag leaves from each plot between 9:00 am and 11:00 am at 7-day intervals from anthesis to 35 DAA, under the conditions of 25°C, 500 μmol s−1 flow rate, atmospheric CO2 concentration, and 1,300 mol m−2 s−1 photosynthetic active radiation in a 2 × 3 cm2 leaf chamber. The instantaneous water use efficiency (WUELeaf) of flag leaves was calculated using the equation (Fan et al., 2019):
where WUELeaf (μmol CO2/mmol H2O) is the instantaneous water use efficiency of flag leaves, Pn (μmol CO2 m−2 s−1) is the net photosynthetic rate of flag leaves, and Tr (mmol H2O m−2 s−1) is the transpiration rate of flag leaves.
Antioxidant enzyme activities of flag leaves
Twenty representative flag leaves from each plot were sampled at 7-day intervals from anthesis to 28 DAA. Fresh samples were immediately submerged in liquid N after collection and stored at −80°C for enzyme assays. Malondialdehyde (MDA) concentration, superoxide dismutase (SOD) activity, and soluble protein content were assayed following the method described by Guo et al. (2015).
Grain filling traits
Spikes emerging on the same day were chosen and tagged in each plot. Twenty spikes were collected from the tagged samples at 7-day intervals from anthesis to 35 DAA. After each sampling, spikes were heated at 105°C for 10 min, dried at 70°C to a constant weight, threshed, hand hulled, and counted before recording the grain weight. Grain filling was fitted using a logistic function (Li et al., 2020):
where W (g) is the 1,000-grain dry weight at time t, A (g) is the theoretical final dry weight of 1,000-grain, t (d) is the day after anthesis, and B and C are the coefficients determined by regression.
The following values, including the duration of grain filling (T), 1,000-grain weight at maximum grain-filling rate (Wmax), maximum grain-filling rate (Vmax), mean grain-filling rate (Vmean), and active grain-filling period (D) were calculated according to the method proposed by He et al. (2020):
Grain Yield and Yield Components
All plants were harvested in an area of 5 m2 from each plot at maturity, threshed, sun-dried to 12.5% moisture content, and weighed to calculate the grain yield. Total spikes of each sampled area were counted to determined the spike number. Twenty plants were selected randomly to recorded the number of grains per spike in each sampled area. 1,000-grain weight was determined by weighing 1,000 grains from the yield measurement samples in each sampled area with three replicates.
Statistical analysis
Data computation and statistical analyses were performed using Microsoft Excel 2016 and SPSS 22.0 (IBM Corp. Released 2013. IBM SPSS Statistics for Windows, Version 22.0. Armonk, NY: IBM Corp.). Treatment means were tested using the least significant difference (LSD) test at a 5% level of significance (p < 0.05). Pearson’s correlation analyses were used to reveal the relationships between soil nutrients, yield components and grain yield, and the relationships between PARF, photosynthesis and senescence characteristics of flag leaves after anthesis, grain filling characteristics and grain yield in a combined analysis of data over 2 years.
Results
Soil nutrient conditions
Table 1 shows the soil nutrient conditions under different treatments before sowing, at jointing and anthesis stages. In both years, HF showed higher SOM in the 0–20 cm soil layer than MF before sowing, at jointing and anthesis stages. In the 0–20 cm soil layer, TN in HF was 7.1%, 7.6%, and 5.1%, before sowing, at jointing and anthesis, higher than in MF in 2017/18, respectively. Compared to MF, HF showed higher hydrolyzable N, AP, and AK before sowing, at jointing and anthesis in 2017/18 and 2018/19. In the 20–40 cm soil layer, soil nutrient conditions in HF were higher than MF before sowing, at the jointing and anthesis stages in both years.
Tillering production and survival
The maximum tillers of wheat in HF were increased by 4.7% and 6.2% in 2017/18 and 2018/19, respectively, compared to those for MF (Table 2). HF showed greater spike number and tillering capacity than MF in both years. Compared to MF, HF significantly improved the spike formation rate with increases of 12.7% and 13.0% in 2017/18 and 2018/19, respectively. The comprehensive analysis of the indexes above showed that despite the difference in basic seedlings in 2017/18 and 2018/19, the order of spike number from high to low among the treatments and years was consistent with the spike formation rate (Figure 2).
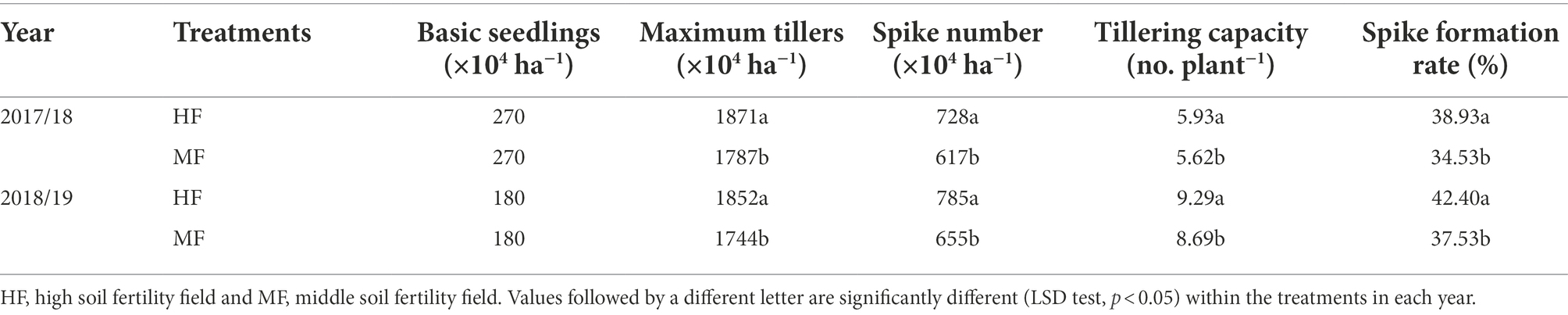
Table 2. Effects of soil fertility on tillering and spike-forming characteristics of wheat in 2017/18 and 2018/19 growing seasons.
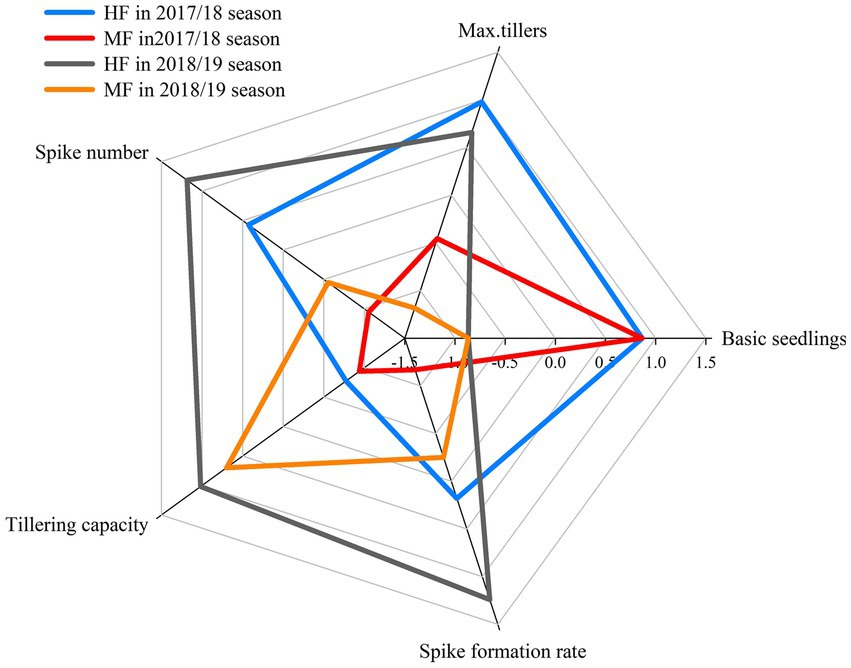
Figure 2. Comprehensive performance of basic seedlings, maximum tillers, spike number, tillering capacity, and spike formation rate of wheat in 2017/18 and 2018/19. HF, high soil fertility field and MF, middle soil fertility field. All data were standardized.
PAR capture
According to the results shown by Figure 3, soil fertility affected the PAR capture of wheat after anthesis. Compared to MF, HF significantly improved PARF after anthesis in both years. The effects were significantly enhanced from 21 DAA, and the PARF at 35 DAA in HF increased by 30.7% and 27.7% in 2017/18 and 2018/19, respectively, compared to MF.
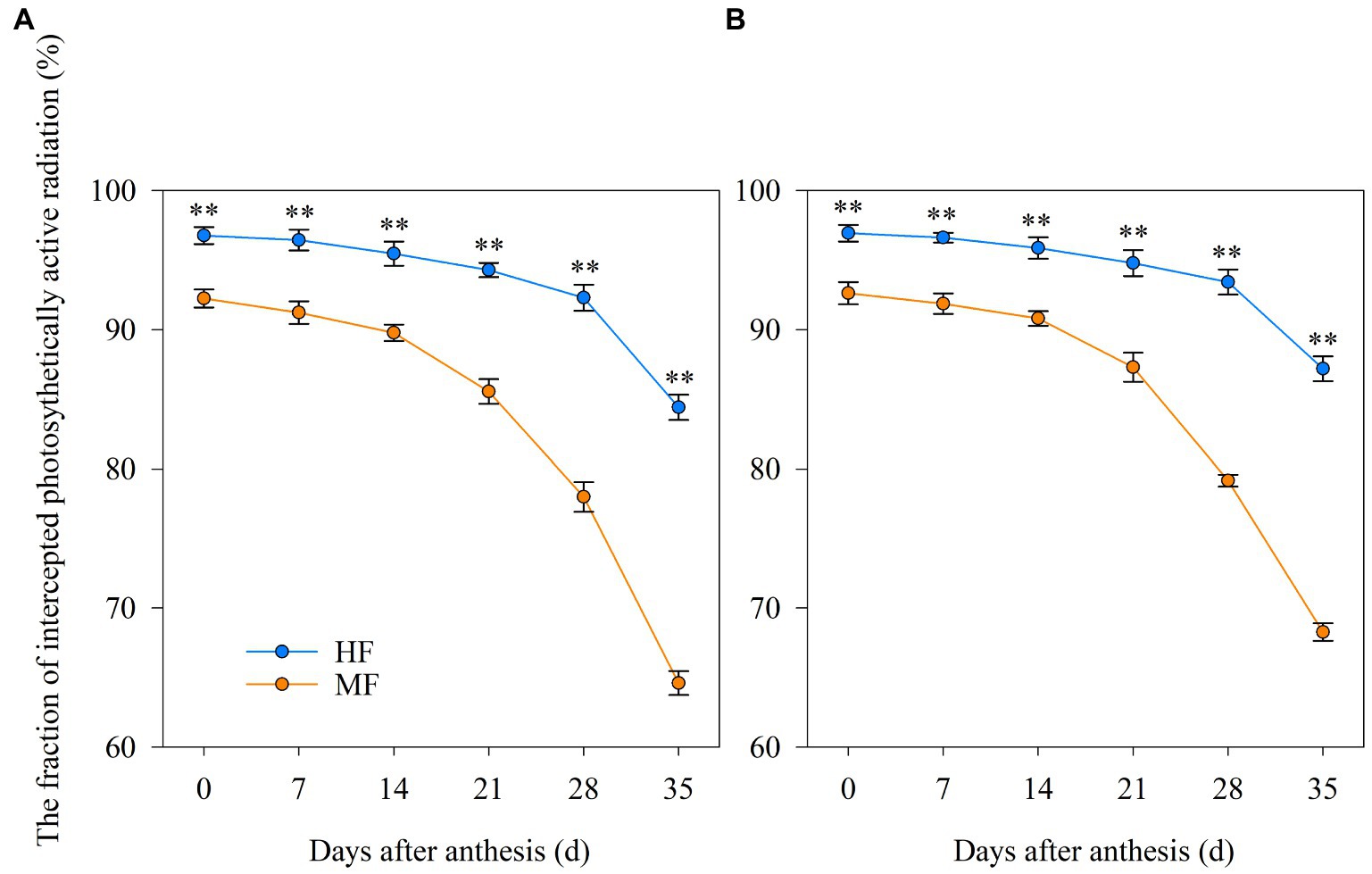
Figure 3. Dynamics of the intercepted photosynthetically active radiation fraction of wheat after anthesis in 2017/18 (A) and 2018/19 (B). HF, high soil fertility field and MF, middle soil fertility field. Vertical bars represent the SD of the means. **p < 0.01.
Photosynthesis characteristics after anthesis
Figure 4 shows the differences in photosynthesis characteristics after anthesis between HF and MF. In 2017/18, the Pn at 0, 7, 14, 21, 28, and 35 DAA in HF increased by 12.9%, 15.7%, 9.7%, 31.6%, 29.1%, and 100.8%, respectively, compared to MF. Moreover, MF significantly improved Tr from 0 to 35 DAA. Similar trends were observed in 2018/19. However, there was no considerable difference in WUELeaf at 0, 7, and 14 DAA between HF and MF. HF showed higher WUELeaf at 21, 28, and 35 DAA than those of MF in both years.
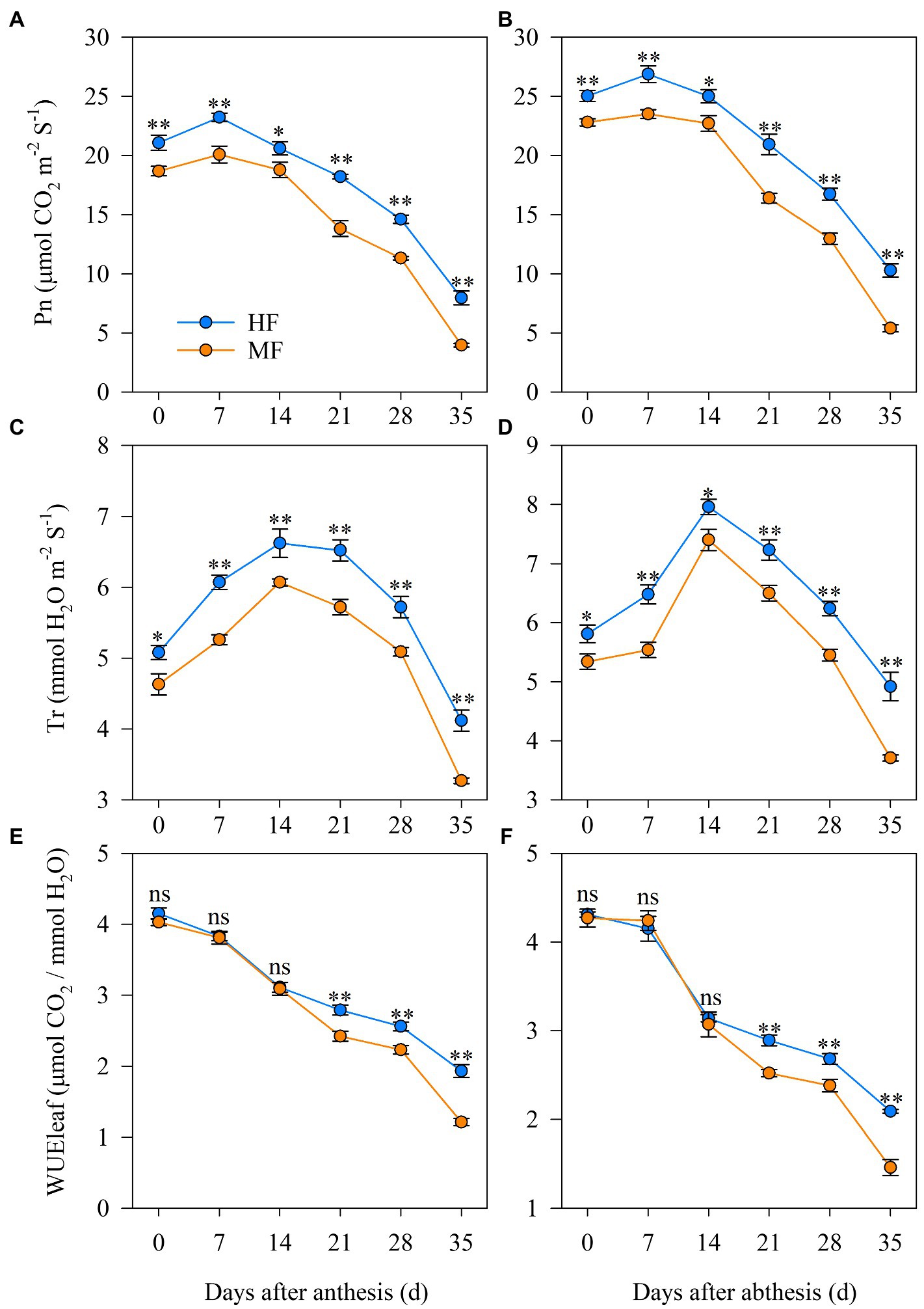
Figure 4. Dynamics of the photosynthesis characteristics of flag leaves after anthesis in 2017/18 (A,C,E) and 2018/19 (B,D,F) growing seasons. HF, high soil fertility field and MF, middle soil fertility field. Vertical bars represent the SD of the means. ns, p > 0.05; *p < 0.05 and **p < 0.01.
Leaf senescence characteristics after anthesis
According to the results shown in Figure 5, HF decreased the MDA concentration from 0 to 35 DAA compared to MF in both years contributing to the delayed leaf senescence. In addition, the SOD activity of flag leaves at 0, 7, 14, 21, and 28 DAA in HF were 4.1%, 4.9%, 7.5%, 10.9%, and 13.2%, respectively, higher than those of MF in 2017/18, respectively. Similar trends were observed in 2018/19. The SP concentration of flag leaves increased and then decreased after anthesis in both years. SP concentrations at 0, 7, 14, 21, and 28 DAA were higher for HF than MF.
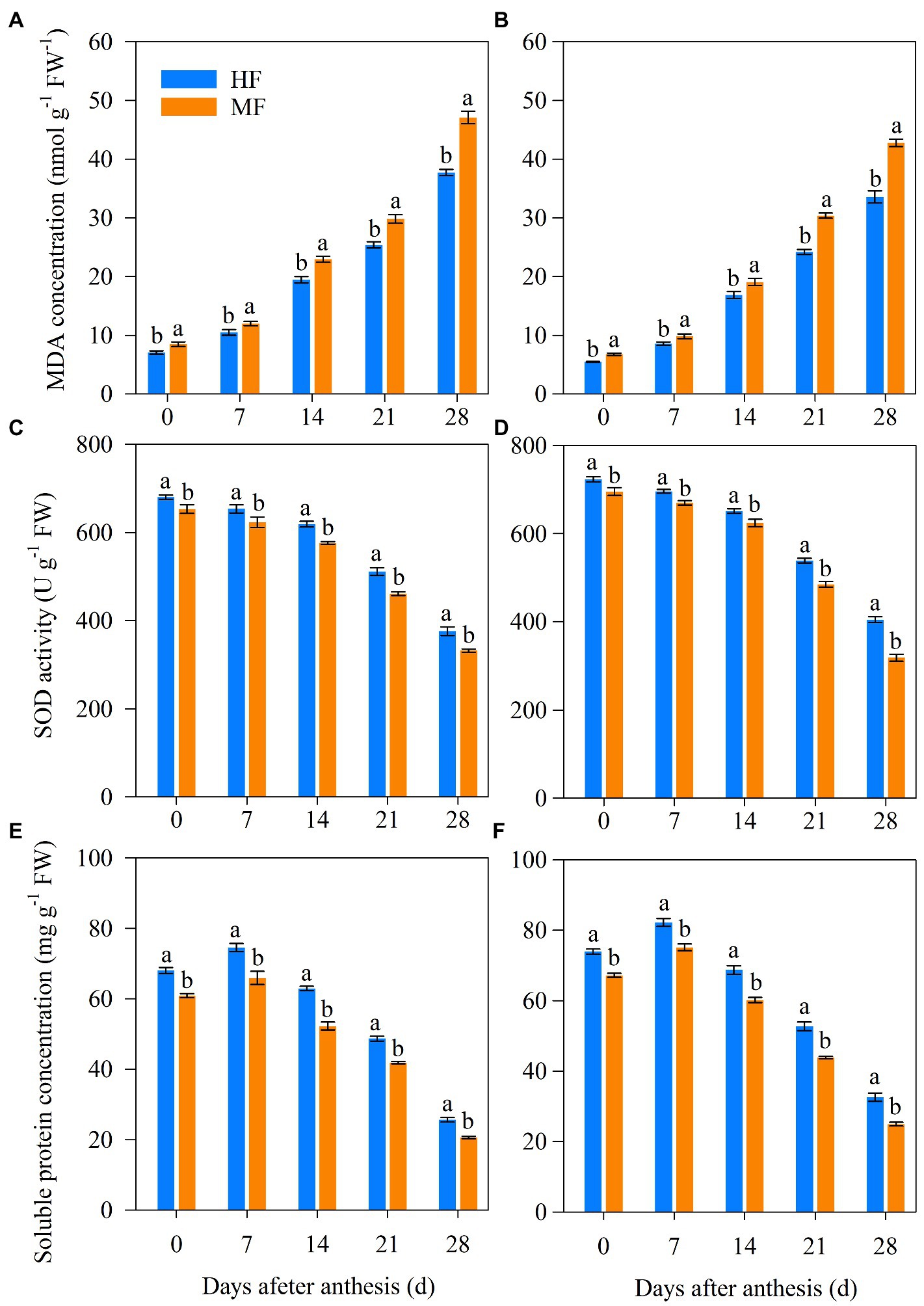
Figure 5. Malondialdehyde (MDA) concentration, superoxide dismutase (SOD) activity, and soluble protein (SP) concentration of flag leaves after anthesis in 2017/18 (A,C,E) and 2018/19 (B,D,F) growing seasons. HF, high soil fertility field and MF, middle soil fertility field. Vertical bars represent SD of the means. Different letters indicate statistical significance at p < 0.05 among treatments.
Grain filling characteristics
The 1,000-grain weight after anthesis is shown in Figure 6. The increase rate of the 1,000-grain weight was enhanced from 14 to 21 DAA and 21 to 28 DAA than from 0 to 7, 7 to 14, and 28 to 35 DAA. Moreover, HF significantly increased the 1,000-grain weight at 7, 14, 21, 28, and 35 DAA compared to MF. Table 3 shows that the grain filling processes in HF and MF were both fitted to the logistic growth curve equation in 2017/18 (correlation coefficient ≥ 0.9976) and 2018/19 (correlation coefficient ≥ 0.9989). Compared to MF, the Wmax in HF increased by 8.3% and 9.5% in 2017/18 and 2018/19, respectively. The Vmax and Vmean of HF were 4.7% and 5.5% higher than those of MF in 2017/2018 and 5.9% and 6.3% higher in 2018/19, respectively. D in HF was longer than that in MF in both years. Thus, compared to MF, the 1,000-grain weight superiority after anthesis in HF was due to its higher grain filling rate and longer active filling period.
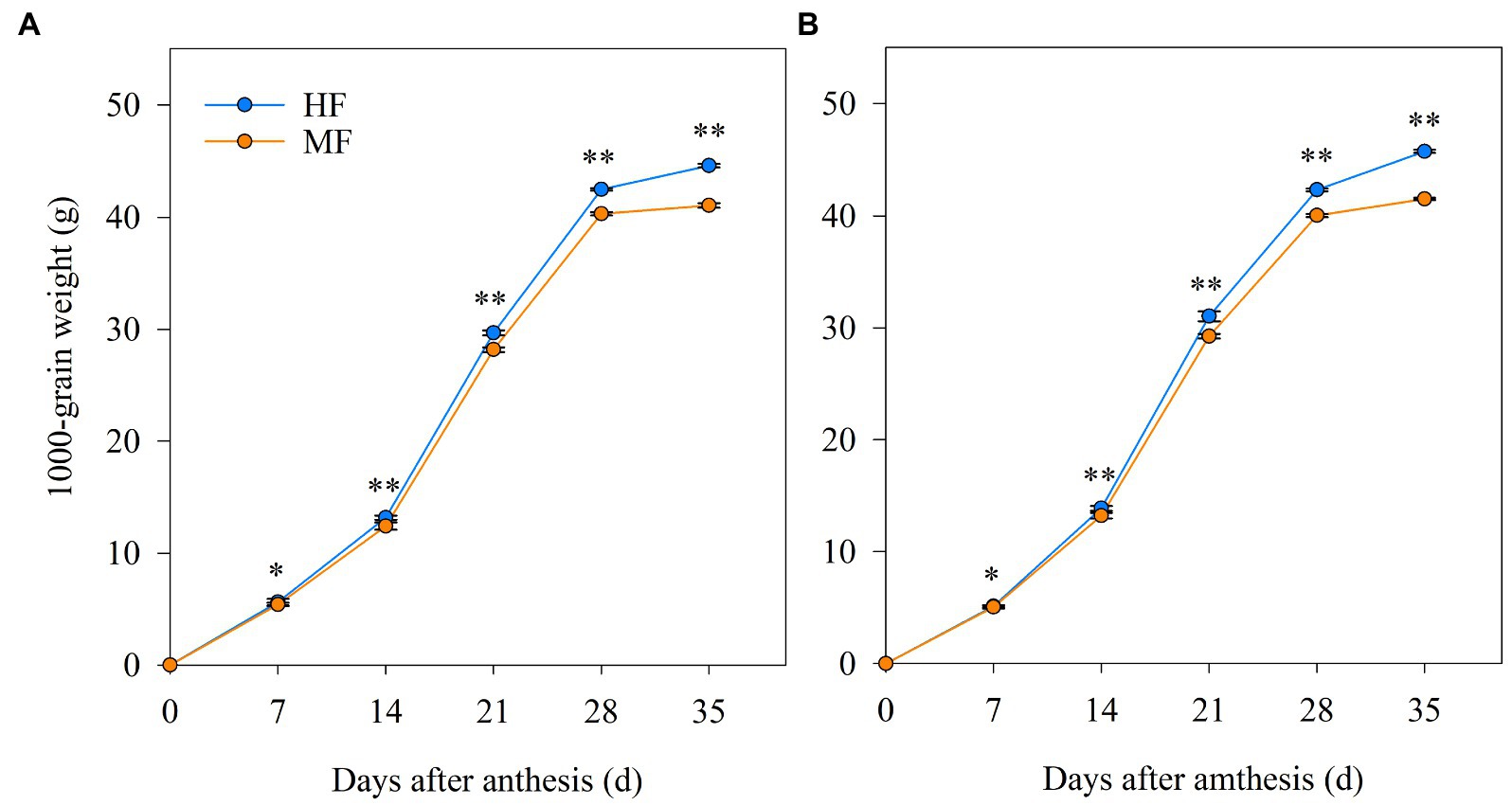
Figure 6. The 1,000-grain weight after anthesis in 2017/18 (A) and 2018/19 (B) growing seasons. HF, high soil fertility field and MF, middle soil fertility field. Vertical bars represent SD of the means. *p < 0.05 and **p < 0.01.
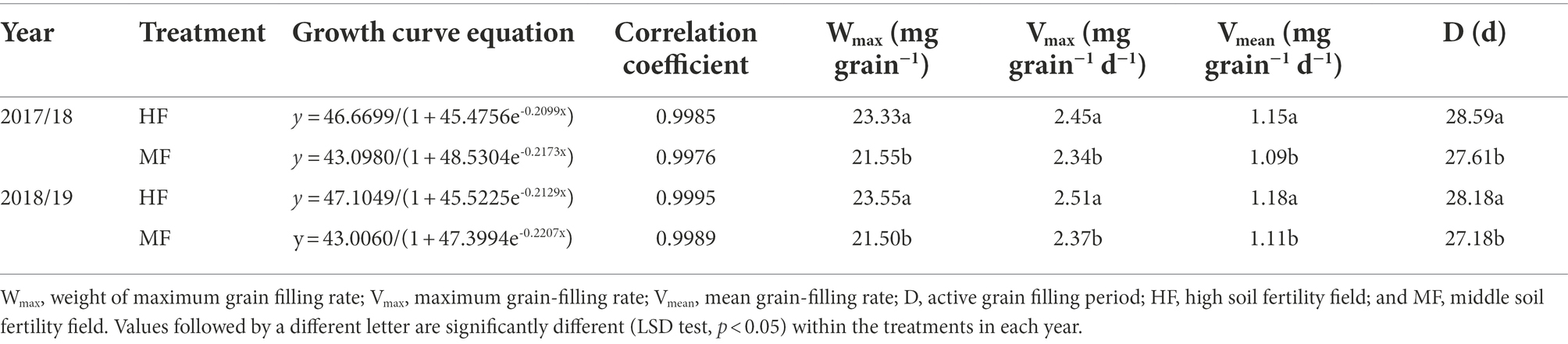
Table 3. The grain filling process model and grain filling parameters in 2017/18 and 2018/19 growing seasons.
Grain yield and yield components
Compared to MF, HF significantly improved the spike number and 1,000-grain weight, which resulted in an increase in grain yield and compensated for the decrease in grain number (Table 4). The grain yield was 20.4% and 24.1% greater in HF than in MF in 2017/18 and 2018/19, respectively.
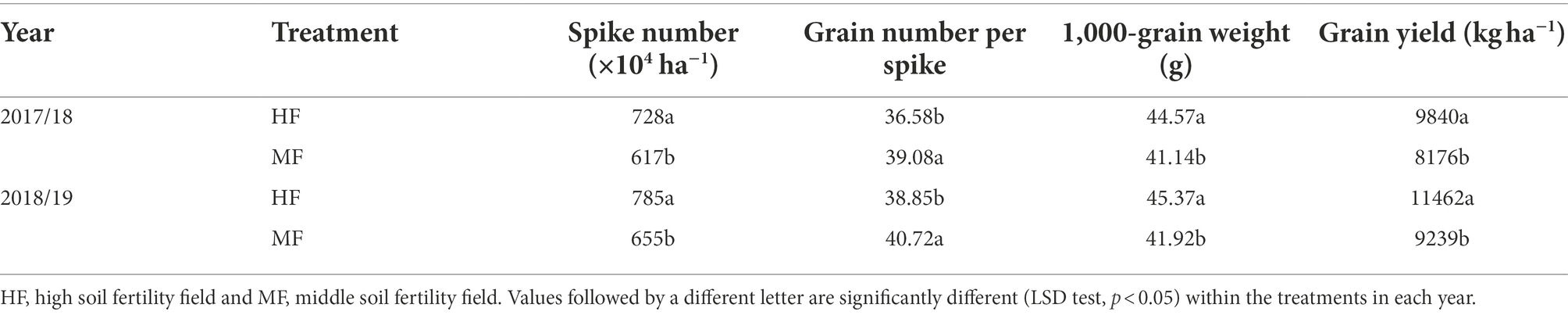
Table 4. Yield components and grain yield under different treatments in 2017/18 and 2018/19 growing seasons.
Correlation analysis
Correlation analysis showed that mean soil nutrients at 0–40 cm (SOM, TN, hydrolyzable N, AP and AK) during whole wheat growth stages were positively correlated with spike number, 1,000-grain weight, and grain yield (Table 5). Grain number had a negative relationship with mean soil nutrients at 0–40 cm during the whole wheat growth stages. Moreover, grain filling characteristic parameters (Wmax, Vmax, and Vmean) and grain yield were positively correlated with PARF, photosynthetic characteristics (Pn, Tr and WUELeaf) and senescence characteristics (SOD and SP), and were negatively correlated with MDA (Table 6). D was positively correlated to the PARF.
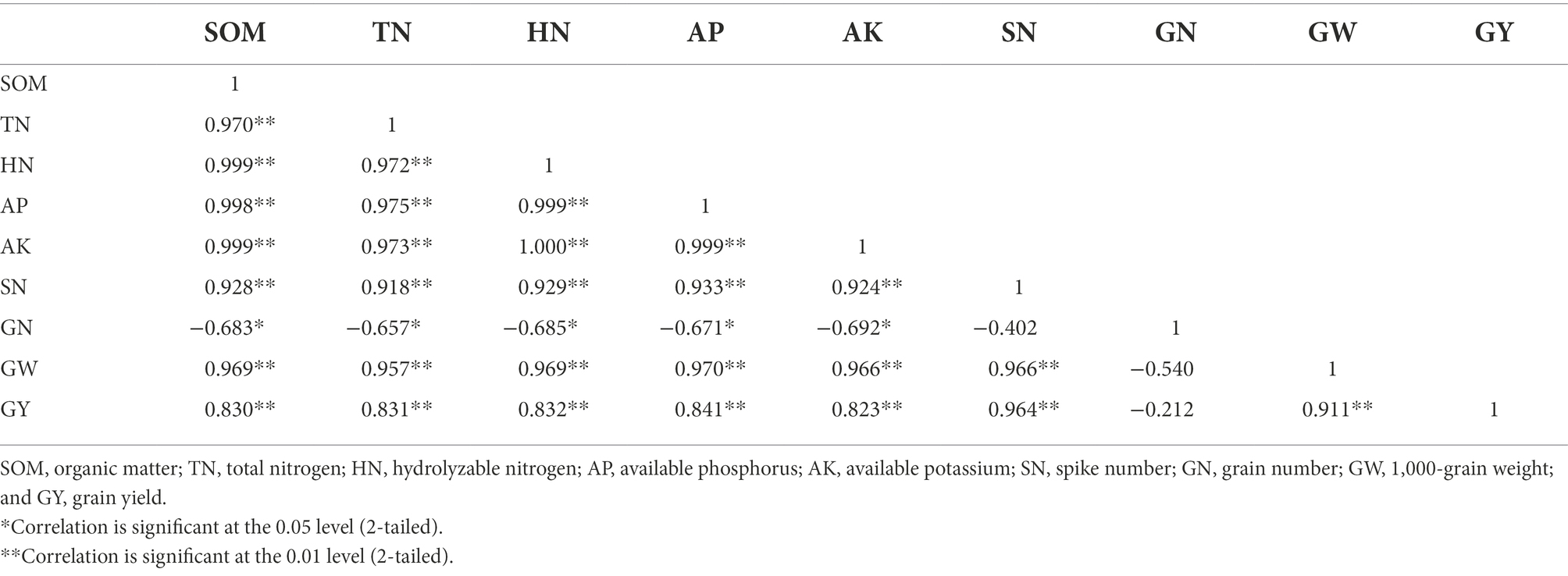
Table 5. Analysis of correlations among mean soil nutrients in 0–40 cm soil layer during whole wheat growth stages, yield components, and grain yield in 2017/18 and 2018/19 (n = 12).
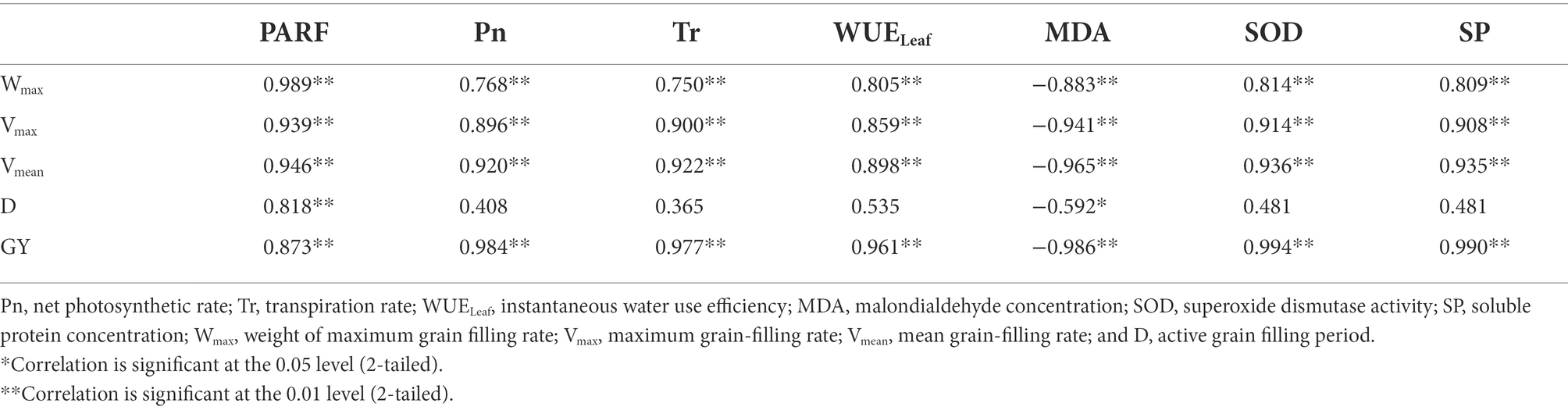
Table 6. Analysis of correlations among intercepted photosynthetically active radiation fraction (PARF), photosynthesis and senescence characteristics of flag leaves after anthesis, grain filling characteristic parameters, grain yield (GY) in 2017/18 and 2018/19 (n = 12).
Discussion
The composition of tillers directly affects the structure and quality of the wheat population, which is highly related to grain yield (Cai et al., 2014). Shang et al. (2021) indicated that N regulates tiller bud growth by regulating N metabolism and can be redistributed from the senescent and aborted tillers to the rest of plant to improve tiller survival. When yield levels ranging from 4,500 to 6,000 kg ha−1, low N rates (210 kg ha−1 vs. 240 and 270 kg ha−1) had a prominent decrease in spike formation rate, resulting in a decreased spike number at maturity (Ding et al., 2021). However, Wang et al. (2021) found that N application alone had less effect on wheat tiller number, while N and phosphorus combination can significantly increase the tiller number. In our results, soil nutrients in the 0–40 cm soil layers were higher for HF (with grain yield levels up to 11,000 kg ha−1) than for MF (with grain yield levels up to 9,000 kg ha−1) during the wheat growth period from 2017 to 2019 (Table 1). This ensured the soil N and phosphorus requirements for the wheat tillering in HF. In this study, the significantly increases of maximum tiller number, tillering capacity and spike formation rate shown in HF compared to MF (Table 2) can be attributed to the improved soil nutrients, since soil nutrients can regulate wheat tillering (Otteson et al., 2008; Wang et al., 2021). Although the basic seedlings in 2017/18 and 2018/19 were different, the increase of spike number was consistent with the increase of spike formation rate among the treatments and years in the comprehensive analysis (Figure 2). These results suggested that the spike formation rate of wheat contributed most to the spike number rather than the maximum tiller number or tillering capacity. This result agrees with that of Hu et al. (2017), who reported that increasing the spike formation rate reduced growth competition and wastage of photosynthates from ineffective tillers, which is an effective way to increase productive tiller number.
Canopy light interception plays a crucial role in elevating crop productivity (Plénet et al., 2000; Zhang et al., 2019b). Bingham et al. (2019) indicated that the protection of canopy PAR interception was required to maximize grain yield until approximately 80% of the grain filling was completed in spring barley. With yield levels ranging from 3,300 to 4,800 kg ha−1, PARF in the middle and lower layers of wheat canopy can be increased by applying N fertilizer ranging from 90 to 180 kg ha−1, but excessive N application at 270 kg ha−1 led to the decreased canopy PARF (Luo et al., 2021). Inferior soil N availability due to the decreased N application rates resulted in a decrease in the PARF of crops, which could be attributed to the decreased leaf area index (Hamzei and Soltani, 2012). In this study, different soil fertility fields affected the PARF, and HF increased PARF after anthesis by 4.7%–30.7% as compared to MF (Figure 3), which ensured energy availability for photosynthesis during the grain filling stage. This result could be attributed to the higher soil nutrients for HF during the wheat growth period (Table 1), as different N fertilizer amounts has a great impact on PARF (Kandel et al., 2019). Moreover, positive relationships were observed among PARF and grain filling characteristic parameters (Table 6), suggesting that improved canopy light interception was beneficial for the increasing of the grain filling rate and for the extension of grain filling duration.
Photosynthesis is the main driver of biomass accumulation and yield (Parry et al., 2011) and is highly sensitive to soil N deficit (Zhang et al., 2017). The decrease in Pn is due to the start of leaf senescence, and the photosynthetic machinery rapidly disassembles, reducing the photosynthetic capacity of flag leaves (Brouwer et al., 2012; Man et al., 2017). The disturbance of soil nutrients affected by tillage influenced leaf senescence in wheat (He et al., 2020). Comparable results were found in our study where MF with grain yield levels up to 9,000 kg ha−1 had lower soil nutrients leading to earlier leaf senescence after anthesis as compared to HF (Table 1; Figure 5). In HF, the higher SOD activity and the SP concentration of flag leaves relieved the damage of the leaf protective enzyme system (Figure 5) and increased the photosynthetic characteristics (Figure 4), lengthening the duration of leaf photosynthesis during grain filling. Moreover, positive relationships between deferred senescence of flag leaves and grain yield were found in this study (Table 6) and are in line with the results of He et al. (2020).
It is well known that N application and SOM significantly influence 1,000-grain weight and grain yield of wheat (Wang et al., 2018). Yan et al. (2019) showed that an increased fertilization rate of N, phosphorus, and potassium contributed to an increase in the duration of grain filling in winter wheat and obtained the yields ranging from 6,700 to 9,400 kg ha−1. Lv et al. (2017) reported that with yield levels ranging from 7,000 to 8,500 kg ha−1, potassium foliage application significantly increased grain weight by increasing the grain filling rate, whereas phosphorus foliage application relieved wheat senescence and increased D, leading to an increase in grain weight. In this study, mean soil nutrients in 0–40 cm soil layer during whole wheat growth stages were positively related to the 1,000-grain weight (Table 5). Positive correlations among grain filling characteristic parameters (i.e., Wmax, Vmax, and Vmean), SOD and SP, and negative relationships between D and MDA (Table 6) indicated that alleviating flag-leaf senescence contributed to the improvement in the rate and duration of grain filling, and delayed leaf senescence positively contributes to prolonging source activity to grain filling and increased crop yield (Gelang et al., 2000; Derkx et al., 2012). Moreover, leaf photosynthesis is another contributor to the improvement of grain filling. Positive relationships were observed among photosynthetic characteristics and grain filling characteristic parameters (Table 6), suggesting that elevated leaf photosynthesis characteristics in HF contributed to the increase in grain filling rate.
Potassium fertilization and/or straw return alleviated soil potassium depletion and increased soil potassium fertility, thus leading to the increases in crop yields (Zhao et al., 2014). Increased plant-available soil N and phosphorus by maize straw mulch combined with no-tillage obtained the highest yields of 7,477 kg ha−1, leading to 16.7% increase in grain yield as compared to conventional tillage (Yang et al., 2020). Compared to the inorganic fertilizer treatment, long-term manure application (with grain yield levels up to 1,700 kg ha−1) increased the stock of soil organic carbon and TN, leading to the increase in crop yields (Qaswar et al., 2022). Schmitz and Ransom (2021) indicated that spike number, grain number and 1,000-grain weight are closely related to wheat yield in both high (>5,000 kg ha−1) and low (>5,000 kg ha−1) yield environments. In most of the high-yield fields, SOM, hydrolyzable N, AP, and AK were higher than in the mid-low-yield field, and effective spike number was the main yield component in the high-yield wheat field, followed by 1,000-grain weight (Peng et al., 2016). Comparable results were observed in the present study, and mean soil nutrients in 0–40 cm soil layer during whole wheat growth stages had positive relationships with spike number, 1,000-grain weight, and grain yield (Table 5). Yield advantage in HF came from the increased spike number and 1,000-grain weight advantage, leading to the yield gaps of 1,664 and 2,223 kg ha−1 compared to those seen for MF (Table 4). The decrease in spike number per m2 and grain number in 2017/18 resulted in a lower grain yield compared to 2018/19 (Table 4). This was due to the monthly precipitation fluctuations, and more rain occurred from jointing to maturity the following year (Figure 1). The guaranteed more water supply during the reproductive and grain filling stages contributed to the improvements of the yield formation (Mu et al., 2021).
Conclusion
HF, with grain yield levels up to 11,000 kg ha−1, had higher soil nutrients than those for MF during wheat growth stages, providing sufficient nutrient supply during the wheat tillering period. Compared to MF, HF improved tiller production, including spike formation rate, resulting in an increased spike number. Furthermore, improvements in grain filling duration and grain filling rate in HF led to the higher 1,000-grain weight. These improvements were also due to the increased canopy light interception and leaf photosynthesis characteristics after anthesis, and alleviated leaf senescence in HF. These results suggest that increasing the spike formation rate, maximizing light interception, and increasing and extending the leaf photosynthesis contributed to narrowing the yield gap between high and middle soil nutrients fields.
Data availability statement
The raw data supporting the conclusions of this article will be made available by the authors, without undue reservation.
Author contributions
YS and ZY conceived and design the study and revised the manuscript. XZ and FY performed the experiments. XZ analyzed the data and wrote the manuscript. All authors contributed to the article and approved the submitted version.
Funding
This study was supported by the National Natural Science Foundation of China (nos. 32172114 and 31771715) and the China Agriculture Research System of MOF and MARA (no. CARS-03).
Conflict of interest
The authors declare that they have no known competing financial interests or personal relationships that could have appeared to influence the work reported in this paper.
Publisher’s note
All claims expressed in this article are solely those of the authors and do not necessarily represent those of their affiliated organizations, or those of the publisher, the editors and the reviewers. Any product that may be evaluated in this article, or claim that may be made by its manufacturer, is not guaranteed or endorsed by the publisher.
Supplementary material
The Supplementary materials for this article can be found online at: https://www.frontiersin.org/articles/10.3389/fpls.2022.932821/full#supplementary-material
Footnotes
References
Abbas, G., Khattak, J. Z. K., Ishaque, M., Aslam, M., Abbas, Z., Amer, M., et al. (2013). Profit maximizing level of potassium fertilizer in wheat production under arid environment. Pak. J. Bot. 45, 961–965.
Allard, V., Martre, P., and Gouis, J. L. (2013). Genetic variability in biomass allocation to roots in wheat is mainly related to crop tillering dynamics and nitrogen status. Eur. J. Agron. 46, 68–76. doi: 10.1016/j.eja.2012.12.004
Alzueta, L., Abeledo, L. G., Mignone, C. M., and Miralles, D. J. (2012). Differences between wheat and barley in leaf and tillering coordination under contrasting nitrogen and sulfur conditions. Eur. J. Agron. 41, 92–102. doi: 10.1016/j.eja.2012.04.002
Bao, S. D. (2000). Analytical Methods for Soil and Agro-Chemistry (3rd Edn.). China Agricultural Press, Beijing.
Bingham, I. J., Young, C., Bounds, P., and Paveley, N. D. (2019). In sink-limited spring barley crops, light interception by green canopy does not need protection against foliar disease for the entire duration of grain filling. Field Crop Res 239, 124–134. doi: 10.1016/j.fcr.2019.04.020
Brouwer, B., Ziolkowska, A., Bagard, M., Keech, O., and Gardeström, P. (2012). The impact of light intensity on shade-induced leaf senescence. Plant Cell Environ. 35, 1084–1098. doi: 10.1111/j.1365-3040.2011.02474.x
Cai, T., Xu, H. C., Peng, D. L., Yin, Y. P., Yang, W. B., Ni, Y. L., et al. (2014). Exogenous hormonal application improves grain yield of wheat by optimizing tiller productivity. Field Crop Res. 155, 172–183. doi: 10.1016/j.cj.2020.04.001
Chen, Y., Zhang, Z., Tao, F. L., Wang, P., and Wei, X. (2017). Spatio-temporal patterns of winter wheat yield potential and yield gap during the past three decades in North China. Field Crop Res. 206, 11–20. doi: 10.1016/j.fcr.2017.02.012
Davidenco, V., Pelissero, P. J., Argüello, J. A., and Vega, C. R. C. (2020). Ecophysiological determinants of oregano productivity: effects of plant’s canopy architecture on radiation capture and use, biomass partitioning and essential oil yield. Sci. Hortic. 272:109553. doi: 10.1016/j.scienta.2020.109553
Dazhong Daily (2020). With yields of over 800 kg per mu in successive years, Yannong 1212 has achieved a new breakthrough in high-yield breeding technology. Available at: https://3g.163.com/dy/article/FGEV87NM0530WJTO.html (Accessed April 23, 2022).
Derkx, A. P., Orford, S., Griffiths, S., Foulkes, M. J., and Hawkesford, M. J. (2012). Identification of differentially senescing mutants of wheat and impacts on yield, biomass and nitrogen partitioning. J. Integr. Plant Biol. 54, 555–566. doi: 10.1111/j.1744-7909.2012.01144.x
Ding, J. F., Li, F. J., Xu, D. Y., Wu, P., Zhu, M., Li, C. Y., et al. (2021). Tillage and nitrogen managements increased wheat yield through promoting vigor growth and production of tillers. Agron. J. 113, 1640–1652. doi: 10.1002/agj2.20562
Fageria, N. (2007). Soil fertility and plant nutrition research under field conditions: basic principles and methodology. J. Plant Nutr. 30, 203–223. doi: 10.1080/01904160601117887
Fan, Y. L., Liu, J. M., Zhao, J. T., Ma, Y. Z., and Li, Q. Q. (2019). Effects of delayed irrigation during the jointing stage on the photosynthetic characteristics and yield of winter wheat under different planting patterns. Agric Water Manag 221, 371–376. doi: 10.1016/j.agwat.2019.05.004
Farmer’s Daily (2019). The background of incessantly new record in high-yielding winter wheat. Available at: https://szb.farmer.com.cn/2019/20190807/20190807_001/20190807_001_5.htm (Accessed April 23, 2022).
Feng, Y. P., Zhang, Y. Y., Li, S., Wang, C., Yin, X. G., Chu, Q. Q., et al. (2020). Sustainable options for reducing carbon inputs and improving the eco-efficiency of smallholder wheat-maize cropping systems in the Huanghuaihai farming region of China. J. Clean. Prod. 244:118887. doi: 10.1016/j.jclepro.2019.118887
Gelang, J., Pleijel, H., Sild, E., Danielsson, H., Younis, S., and Selldén, G. (2000). Rate and duration of grain filling in relation to flag leaf senescence and grain yield in spring wheat (Triticum aestivum) exposed to different concentrations of ozone. Physiol. Plant. 110, 366–375. doi: 10.1111/j.1399-3054.2000.1100311.x
Guo, Z. J., Shi, Y., Yu, Z. W., and Zhang, Y. L. (2015). Supplemental irrigation affected flag leaves senescence post-anthesis and grain yield of winter wheat in the Huang-Huai-Hai plain of China. Field Crop Res. 180, 100–109. doi: 10.1016/j.fcr.2015.05.015
Hag Husein, H., Lucke, B., Bäumler, R., and Sahwan, W. (2021). A contribution to soil fertility assessment for arid and semi-arid lands. Soil Syst. 5, 42. doi: 10.3390/soilsystems5030042
Hamzei, J., and Soltani, J. (2012). Deficit irrigation of rapeseed for water-saving: effects on biomass accumulation, light interception and radiation use efficiency under different N rates. Agric. Ecosyst. Environ. 155, 153–160. doi: 10.1016/j.agee.2012.04.003
He, J. N., Shi, Y., and Yu, Z. W. (2019). Subsoiling improves soil physical and microbial properties, and increases yield of winter wheat in the Huang-Huai-Hai plain of China. Soil Tillage Res. 187, 182–193. doi: 10.1016/j.still.2018.12.011
He, J. N., Shi, Y., Zhao, J. Y., and Yu, Z. W. (2020). Strip rotary tillage with subsoiling increases winter wheat yield by alleviating leaf senescence and increasing grain filling. Crop J. 8, 327–340. doi: 10.1016/j.cj.2019.08.0072214-5141
Hu, Y. S., Ren, T. H., Li, Z., Tang, Y. Z., Ren, Z. L., and Yan, B. J. (2017). Molecular mapping and genetic analysis of a QTL controlling spike formation rate and tiller number in wheat. Gene 634, 15–21. doi: 10.1016/j.gene.2017.08.039
Hussain, M., Khan, M. B., Mehmood, Z., Zia, A. B., Jabran, K., and Farooq, M. (2013). Optimizing row spacing in wheat cultivars differing in tillering and stature for higher productivity. Arch. Agron. Soil Sci. 59, 1457–1470. doi: 10.1080/03650340.2012.725937
Kandel, T. P., Gowda, P. H., Northup, B. K., and Rocateli, A. C. (2019). Impacts of tillage systems, nitrogen fertilizer rates and a legume green manure on light interception and yield of winter wheat. Cogent Food Agr. 5:1580176. doi: 10.1080/23311932.2019.1580176
Kautz, T., Amelung, W., Ewert, F., Gaiser, T., Horn, R., Jahn, R., et al. (2013). Nutrient acquisition from arable subsoils in temperate climates: A review. Soil Biol. Biochem. 57, 1003–1022. doi: 10.1016/j.soilbio.2012.09.014
Li, Q., Du, L. J., Feng, D. J., Ren, Y., Li, Z. X., Kong, F. L., et al. (2020). Grain-filling characteristics and yield differences of maize cultivars with contrasting nitrogen efficiencies. Crop J. 8, 990–1001. doi: 10.1016/j.cj.2020.04.001
Liu, K., Yang, R., Deng, J., Huang, L. Y., Wei, Z. W., Ma, G. B., et al. (2020). High radiation use efficiency improves yield in the recently developed elite hybrid rice Y-liangyou 900. Field Crop Res. 253:107804. doi: 10.1016/j.fcr.2020.107804
Lu, C. H., and Fan, L. (2013). Winter wheat yield potentials and yield gaps in the North China plain. Field Crop Res. 143, 98–105. doi: 10.1016/j.fcr.2012.09.015
Luo, C. S., Guo, Z. P., Xiao, J. X., Dong, K., and Dong, Y. (2021). Effects of applied ratio of nitrogen on the light environment in the canopy and growth, development and yield of wheat when intercropped. Front. Plant Sci. 12:719850. doi: 10.3389/fpls.2021.719850
Lv, X. K., Han, J., Liao, Y. C., and Liu, Y. (2017). Effect of phosphorus and potassium foliage application post-anthesis on grain filling and hormonal changes of wheat. Field Crop Res. 214, 83–93. doi: 10.1016/j.fcr.2017.09.001
Man, J. G., Yu, Z. W., and Shi, Y. (2017). Radiation interception, chlorophyll fluorescence and senescence of flag leaves in winter wheat under supplemental irrigation. Sci. Rep. 7:7767. doi: 10.1038/s41598-017-07414-2
Mu, Q., Cai, H. J., Sun, S. K., Wen, S. S., Xu, J. T., Dong, M. Q., et al. (2021). The physiological response of winter wheat under short-term drought conditions and the sensitivity of different indices to soil water changes. Agric. Water Manag. 243:106475. doi: 10.1016/j.agwat.2020.106475
National Bureau of Statistics of China (2020). China Statistical Yearbook. China Statistics Press, Beijing.
Otteson, B. N., Mergoum, M., Ransom, J. K., and Schatz, B. (2008). Tiller contribution to spring wheat yield under varying seeding and nitrogen management. Agron. J. 100, 406–413. doi: 10.2134/agrojnl2007.0109
Parry, M. A. J., Reynolds, M., Salvucci, M. E., Raines, C., Andralojc, P. J., Zhu, X. G., et al. (2011). Raising yield potential of wheat II increasing photosynthetic capacity and efficiency. J. Exp. Bot. 62, 453–467. doi: 10.1093/jxb/erq304
Peng, Z. P., Ma, S. Y., Wang, Y. Q., Men, M. X., Liu, Y. N., Yahya, A., et al. (2016). Plant and soil fertility characteristics of different winter wheat fields in the Huang-huai-hai plain of China. Acta Agric. Scand. B Soil Plant Sci. 66, 630–639. doi: 10.1080/09064710.2016.1206616
Plénet, D., Mollier, A., and Pellerin, S. (2000). Growth analysis of maize field crops under phosphorus deficiency. II. Radiation-use efficiency, biomass accumulation and yield components. Plant and Soil 224, 259–272. doi: 10.1023/A:1004835621371
Qaswar, M., Li, D. C., Huang, J., Han, T. F., Ahmed, W., Ali, S., et al. (2022). Dynamics of organic carbon and nitrogen in deep soil profile and crop yields under long-term fertilization in wheat-maize cropping system. J. Integr. Agric. 21, 826–839. doi: 10.1016/S2095-3119(20)63501-8
Schmitz, P. K., and Ransom, J. K. (2021). Seeding rate effects on hybrid spring wheat yield, yield components, and quality. Agronomy 11:1240. doi: 10.3390/agronomy11061240
Shang, Q. S., Wang, Y. P., Tang, H., Sui, N., Zhang, X. S., and Wang, F. (2021). Genetic, hormonal, and environmental control of tillering in wheat. Crop J. 9, 986–991. doi: 10.1016/j.cj.2021.03.002
Tittonell, P., Vanlauwe, B., De Ridder, N. D., and Giller, K. E. (2007). Heterogeneity of crop productivity and resource use efficiency within smallholder Kenyan farms: soil fertility gradients or management intensity gradients? Agr. Syst. 94, 376–390. doi: 10.1016/j.agsy.2006.10.012
Wang, L. F., Sun, J. T., Zhang, Z. B., Xu, P., and Shangguan, Z. P. (2018). Winter wheat grain yield in response to different production practices and soil fertility in northern China. Soil Tillage Res. 176, 10–17. doi: 10.1016/j.still.2017.10.001
Wang, R., Wang, Y., Hu, Y. X., Dang, T. H., and Guo, S. L. (2021). Divergent responses of tiller and grain yield to fertilization and fallow precipitation: insights from a 28-year long-term experiment in a semiarid winter wheat system. J. Integr. Agric. 20, 3003–3011. doi: 10.1016/S2095-3119(20)63296-8
Wang, H. G., Yu, Z. W., Shi, Y., and Zhang, Y. L. (2020). Effects of tillage practices on grain yield formation of wheat and the physiological mechanism in rainfed areas. Soil Tillage Res. 202:104675. doi: 10.1016/j.still.2020.104675
Xiao, D. P., and Tao, F. L. (2014). Contributions of cultivars, management and climate change to winter wheat yield in the North China plain in the past three decades. Eur. J. Agron. 52, 112–122. doi: 10.1016/j.eja.2013.09.020
Xu, X. X., Zhang, M., Li, J. P., Liu, Z. Q., Zhao, Z. G., Zhang, Y. H., et al. (2018). Improving water use efficiency and grain yield of winter wheat by optimizing irrigations in the North China plain. Field Crop Res. 221, 219–227. doi: 10.1016/j.fcr.2018.02.011
Yan, S. C., Wu, Y., Fan, J. L., Zhang, F. C., Qiang, S. C., Zheng, J., et al. (2019). Effects of water and fertilizer management on grain filling characteristics, grain weight and productivity of drip-fertigated winter wheat. Agric. Water Manag. 213, 983–995. doi: 10.1016/j.agwat.2018.12.019
Yang, H. K., Wu, G., Mo, P., Chen, S. H., Wang, S. Y., Xiao, Y., et al. (2020). The combined effects of maize straw mulch and no-tillage on grain yield and water and nitrogen use efficiency of dry-land winter wheat (Triticum aestivum L.). Soil Tillage Res. 197:104485. doi: 10.1016/j.still.2019.104485
Yu, F. X., Shi, Y., Zhao, J. Y., Wang, X. Z., and Yu, Z. W. (2018). Effect of different soil fertility on chlorophyll fluorescence characteristics and yield in high-yielding wheat variety Yannong 1212. J. Triticeae Crops. 38, 1222–1228. doi: 10.7606/j.issn.1009-1041.2018.10.11
Zhang, X. D., Kamran, M., Xue, X. K., Zhao, J., Cai, T., Jia, Z. K., et al. (2019b). Ridge-furrow mulching system drives the efficient utilization of key production resources and the improvement of maize productivity in the loess plateau of China. Soil Tillage Res. 190, 10–21. doi: 10.1016/j.still.2019.02.015
Zhang, Y. Q., Wang, J. D., Gong, S. H., Xu, D., and Sui, J. (2017). Nitrogen fertigation effect on photosynthesis, grain yield and water use efficiency of winter wheat. Agric. Water Manag. 179, 277–287. doi: 10.1016/j.agwat.2016.08.007
Zhang, Y. J., Wang, R., Wang, H., Wang, S. L., Wang, X. L., and Li, J. (2019a). Soil water use and crop yield increase under different long-term fertilization practices incorporated with two-year tillage rotations. Agric. Water Manag. 221, 362–370. doi: 10.1016/j.agwat.2019.04.018
Zhang, X. F., Zhu, A. N., Xin, X. L., Yang, W. L., Zhang, J. B., and Ding, S. J. (2018). Tillage and residue management for long-term wheat-maize cropping in the North China plain: I. crop yield and integrated soil fertility index. Field Crop Res. 221, 157–165. doi: 10.1016/j.fcr.2018.02.025
Keywords: soil nutrients, yield gap, spike formation, photosynthesis characteristics, grain filling
Citation: Zheng X, Yu Z, Yu F and Shi Y (2022) Grain-filling characteristics and yield formation of wheat in two different soil fertility fields in the Huang–Huai–Hai Plain. Front. Plant Sci. 13:932821. doi: 10.3389/fpls.2022.932821
Edited by:
Min Huang, Hunan Agricultural University, ChinaReviewed by:
Guanglong Hu, Beijing Academy of Agricultural and Forestry Sciences, ChinaPeng Yan, Institute of Crop Sciences (CAAS), China
Ke Liu, University of Tasmania, Australia
Copyright © 2022 Zheng, Yu, Yu and Shi. This is an open-access article distributed under the terms of the Creative Commons Attribution License (CC BY). The use, distribution or reproduction in other forums is permitted, provided the original author(s) and the copyright owner(s) are credited and that the original publication in this journal is cited, in accordance with accepted academic practice. No use, distribution or reproduction is permitted which does not comply with these terms.
*Correspondence: Yu Shi, c2hpeXVAc2RhdS5lZHUuY24=