- 1Institute of Wetland Ecology and Clone Ecology/Zhejiang Provincial Key Laboratory of Plant Evolutionary Ecology and Conservation, Taizhou University, Taizhou, China
- 2School of Ecology and Nature Conservation, Beijing Forestry University, Beijing, China
- 3Forest Dynamics, Swiss Federal Research Institute WSL, Birmensdorf, Switzerland
Parental effects can influence offspring fitness, which may further impact interspecific competition. However, few studies have tested the role of clonal parental effects in regulating interspecific interactions and examined the underlying mechanisms. We conducted two consecutive experiments with two clonal plants (Pistia stratiotes and Eichhornia crassipes). In the first experiment, the mother ramet of P. stratiotes and E. crassipes were grown in two nutrient levels and treated with a DNA demethylation reagent (5-azacytidine) or not. In the second experiment, the offspring ramets from each of the four treatments in the first experiment were grown alone (no competition) or with a heterospecific neighbor (with interspecific competition). We found no parental nutrient effect on the competitive ability of E. crassipes, but a significant parental nutrient effect of both E. crassipes and P. stratiotes on the competitive ability of P. stratiotes. Furthermore, the parental nutrient effect of P. stratiotes on the competitive ability of P. stratiotes varied depending on the DNA methylation status of both P. stratiotes and E. crassipes. These clonal parental effects were related to resource provisioning and/or DNA methylation. We conclude that clonal parental nutrient effects can regulate interspecific competition between P. stratiotes and E. crassipes by altering the competitive ability of P. stratiotes. Both resource provisioning and epigenetic mechanisms can be involved in these clonal parental effects. By regulating interspecific competition, clonal parental effects may further influence species coexistence, community structure, and ecosystem functioning.
Introduction
The environmental condition of a parent can influence the phenotype of their offspring (Wulff and Roach, 1987; Badyaev and Uller, 2009). Such a parental (environmental) effect can be transmitted to offspring generations via sexual propagules such as seeds (sexual parental effects) or clonal propagules such as offspring ramets (clonal parental effects) (Miao et al., 1991a; Agrawal, 2002; Dong et al., 2017; González et al., 2018; Baker et al., 2019; Luo et al., 2022). A large body of evidence shows that parental effects can influence fitness measures (e.g., growth and production) of offspring (Galloway, 2005; Latzel et al., 2014; Dong et al., 2018a,b), which may cascade to impact population- and community-level patterns and processes (Miao et al., 1991b; Bossdorf et al., 2009; Castro et al., 2013; Baker et al., 2019; Luo et al., 2022).
In nature, most plants do not grow alone, and interactions with heterospecific neighbors (i.e., interspecific interactions) are common (Grace and Tilman, 1990; Du et al., 2004). The significant role of parental effects in regulating fitness measures of offspring may further affect their interspecific interactions with heterospecific neighbors (Miao et al., 1991b; Bossdorf et al., 2009), thereby generating profound impacts on population dynamics, species coexistence, biodiversity maintenance, and ecosystem functions and services (Zou and Xu, 1998; Kraft et al., 2015; Valladares et al., 2015). If, for instance, parental effects can improve the growth of offspring (Latzel et al., 2014; Dong et al., 2017, 2018a,2019b), then they may enhance their competitive ability (Miao et al., 1991a; Bossdorf et al., 2009). On the contrary, if parental effects can reduce the growth of offspring, e.g., in some cases due to phenotypic changes (Galloway, 2005), then they may weaken their competitive ability (Bossdorf et al., 2009). However, studies on the roles of parental effects in regulating interspecific interactions are still limited (Bossdorf et al., 2009), and the few existing studies in this field focused mostly on the role of sexual parental effects in non-clonal plants (Miao et al., 1991a,b; Bossdorf et al., 2009). No studies have considered clonal parental effects on interspecific interactions (Luo et al., 2022).
Compared with non-clonal plants, clonal plants can avoid genetic variation due to meiosis, so that the epigenetic information of environmental interactions experienced by parents can be transmitted to offspring more effectively (Latzel and Klimešová, 2010; Verhoeven and Preite, 2014; Luo et al., 2022). As clonal propagules (e.g., ramets) are larger in size and mass than sexual propagules (e.g., seeds), the potential for resource provisioning might be relatively high in clonal than in non-clonal plants (Dong et al., 2019a). Therefore, parental effects may be very important for clonal plants (Verhoeven and Preite, 2014; Dodd and Douhovnikoff, 2016), particularly for those with a low ability of sexual reproduction. Thus, testing clonal parental effects on interspecific competition can deepen our understanding of the community-level roles of parental effects in clonal plants (Luo et al., 2022).
Epigenetic inheritance is an important mechanism underlying clonal parental effects (Jablonka and Raz, 2009; Latzel and Klimešová, 2010; Richards et al., 2017; González et al., 2018), and DNA methylation is one of the most important epigenetic modifications (Schulz et al., 2014). Even if the environment of offspring is different from their mother’s and the original stimulus of DNA methylation disappears, the offspring can still retain the previous methylation imprint after DNA replication (Rico et al., 2014). This epigenetic mechanism enables plants to remember past environmental experience, predict and overcome future environmental stress, and carry adaptive information through reliable transmission and selection over multiple generations (Schulz et al., 2014). By applying DNA demethylation reagent (e.g., 5-azacytidine) to plants, it was found that DNA methylation plays an important role in clonal parental effects on the morphology and growth of offspring (González et al., 2016, 2017; Portela et al., 2020). Consequently, DNA methylation may also regulate clonal parental effects on interspecific interactions. However, the epigenetic mechanism of parental effects on interspecific interactions has not been confirmed.
We conducted two consecutive experiments with two clonal floating plants (Pistia stratiotes and Eichhornia crassipes) to explore how clonal parental effects regulate interspecific interactions and the role of DNA methylation. We chose these two species because they frequently co-occur and compete with each other, and also because they can spread quickly by clonal growth. In the first experiment, the mother ramet of P. stratiotes and E. crassipes were grown in two nutrient levels and treated with a DNA demethylation reagent (5-azacytidine) or not. In the second experiment, the offspring ramets from each of the four treatments in the first experiment were grown alone (no competition) or with a heterospecific neighbor (with interspecific competition). Specifically, we tested two hypotheses: (1) clonal parental effects can alter interspecific interactions by influencing DNA methylation level; (2) the offspring produced by a mother ramet under the high nutrient condition were more competitive than those produced by a mother under the low nutrient condition, because parents under the high nutrient condition can produce offspring of higher quality.
Materials and methods
Study species
Pistia stratiotes L. (water lettuce, Araceae) is a stoloniferous floating rosette herb (Pettet and Pettet, 1970; Adomako et al., 2020, 2021). The main (i.e., vertical) stem is short with highly compressed internodes so that leaves are clustered (Odjegba and Fasidi, 2004; Adomako et al., 2021, 2022). Stolons come out from leaf axils, and new ramets are produced from stolon tips (Odjegba and Fasidi, 2004). This species is native to South America, and are now widely distributed in tropical and subtropical regions around the world, including China (Evans, 2013; Hussner et al., 2014). As one of the wetland weeds, a massive accumulation of P. stratiotes leads to the decline of biodiversity in local ecosystems; therefore, it has been included in the Global Invasive Species Database, and are also listed as one of the most dangerous invasive species in China (Wang et al., 2014; Galal et al., 2019).
Eichhornia crassipes (Mart.) Solms (water hyacinth, Pontederiaceae) is a stoloniferous floating rosette herb with a similar morphology and distribution of P. stratiotes (Wang et al., 2016). This species is also listed as an aggressive invasive species in many counties, including China (Wang et al., 2016), because it can quickly spread by clonal growth to form a dense mat on water surface and rapidly displace local species (Zhao, 2006). Eichhornia crassipes and P. stratiotes share similar niches and occur in lakes, rivers, ponds, and diches (Wang et al., 2016).
Sampling and cultivation
On July 5, 2020, a clone of ramets of both P. stratiotes and E. crassipes were collected from Yongning River (28°40′3″N, 121°23′4″E) in Taizhou, Zhejiang Province, China. They were brought to a greenhouse at Taizhou University, where they were vegetatively propagated in tanks (95 cm in diameter × 60 cm in height) filled with water. On July 26, 2020, 74 newly produced offspring ramets with similar size were selected for both P. stratiotes and E. crassipes (148 ramets in total), and the stolons attached to these ramets, if any, were removed. For each species, ten of the 74 ramets were randomly selected and dried for 48 h at 70°C and weighed to measure the initial dry mass (1.08 ± 0.04 g for P. stratiotes and 1.32 ± 0.03 g for E. crassipes; mean ± SE). The remaining 64 ramets of both P. stratiotes and E. crassipes were used in the experiments described below.
Experiment design
The study consisted of two consecutive experiments. The first experiment was launched on July 26, 2020. We randomly subjected the 64 ramets (thereafter referred to as the mother ramets) of both P. stratiotes and E. crassipes to two nutrient levels (high and low) and two DNA demethylation treatments (ramets were treated with a DNA demethylation agent or not). Thus, for both species, each of the four treatments was replicated 16 times (with 16 mother ramets). Each mother ramet was grown in a bucket (65 cm in diameter × 44 cm in height) filled with 13 L of either a high or a low nutrient solution (30 and 6% Hoagland solution, respectively). The Hoagland solution contained 945 mg/L Ca (NO3)2⋅4H2O, 506 mg/L KNO3, 80 mg/L NH4NO3, 136 mg/L KH2PO4, 493 mg/L MgSO4, 13.9 mg/L FeSO4⋅7H2O, and 18.7 mg/L EDTA⋅2Na. We chose these two nutrient levels because the nitrogen and phosphorous concentrations are within the gradient of nutrient levels found in water body in China (Zhang et al., 2017) and also because their difference is likely to induce a significant effect on plant growth. For DNA demethylation, 10 ml of 50 μM 5-azacitidine (5-azaC) solution was sprayed to each plant once every 3 days. For the treatment without DNA demethylation, 10 ml distilled water was sprayed.
To supplement for evapotranspiration and nutrient loss due to plant uptake, 1 L of 30% or 6% Hoagland nutrient solution was added weekly to each bucket. The first experiment lasted for 3 weeks and ended on August 16, 2020. At the end of the experiment, 2–3 offspring ramets in each bucket (produced by each mother ramet) were selected as the materials for the second experiment, and the remaining parts in each bucket were harvested to measure growth traits.
The second experiment started on 16 August 2020. For each species, the offspring ramets produced by the mother ramets grown in each of the four conditions (i.e., high and low nutrients with and without DNA demethylation) in the first experiment were randomly assigned to one of five treatments: the target ramet was grown alone (no competition, one treatment), with a ramet of a different species whose mother ramet was grown in the same condition (interspecific competition from ramets with the same parental effects; one treatment) and with a ramet of a different species whose mother ramet was grown in a different condition (interspecific competition from ramets with different parental effects; three treatments). The 16 treatments with interspecific competition were shared by the two species, resulting in a total of 24 treatments (eight treatments without competition and 16 treatments with competition).
Ramets were grown in buckets (65 cm in diameter × 44 cm in height) filled with 30% Hoagland nutrient solution. Each treatment was replicated seven times, making a total of 168 buckets with a total of 280 ramets. To compensate for water loss and nutrient consumption, 1 L of 30% Hoagland nutrient solution was added weekly to each bucket. The second experiment lasted for 3 weeks and ended on September 6, 2020. The mean temperature was 29.2°C and the mean relative humidity was 79.8% (measured hourly with a Hygrochron temperature loggers; iButton DS1923; Maxim Integrated Products, United States). At noon, the photosynthetic photon flux density at water surface was 632–1806 μmol m–2 s–1 (Li-250A; LI–COR Biosciences, United States).
Harvest and measurements
At the end of the first experiment, we counted, for each species, the number of offspring ramets in each bucket, and measured biomass after oven-drying them at 70°C for 72 h. At the end of the second experiment, we measured biomass of each species in each bucket after drying them at 70°C for 72 h. Biomass per ramet was calculated as total biomass/number of ramets.
Data analysis
Two-way ANOVA was used to test the effects of the nutrient level (high and low) and DNA demethylation (treated with 5-azaC or not) on total biomass, number of ramets and biomass per ramet of P. stratiotes and E. crassipes separately for the first experiment. To analyze the data from the second experiment, we first quantified the competitive response of a target plant by calculating log response ratio of biomass (LnRR), i.e., LnRR = ln(total biomass of a target ramet grown with a competing ramet/average biomass of the target ramet grown alone across the seven replicates) (Goldberg et al., 1999; Hedges et al., 1999). This calculation was carried out for each type of ramets of each species. One-sample t-test was used to analyze whether the mean value of the competitive response of each treatment was significantly different from zero. Then we used four-way ANOVA to examine the effects of the nutrient level and DNA demethylation of the target’s mother ramet and the nutrient level and DNA demethylation of the competitor’s mother ramet on the competition response (LnRR) of the target plant.
Before analysis, data on biomass, number of ramet and biomass per ramet were checked for normality (by Kolmogorov–Smirnov test) and homogeneity of variance (by Levene’s test). During the first experiment, one replicate of P. stratiotes in the low nutrient level and not treated with 5-azaC, one replicate of E. crassipes in high nutrient level and treated with 5-azaC and one replicate of E. crassipes in low nutrient level and treated with 5-azaC were completely destroyed by herbivores. During the second experiment, plants in two buckets (one with a ramet of P. stratiotes whose mother was grown in the high nutrient level and treated with 5-azaC and a ramet of E. crassipes whose mother was grown in the low level and not treated with 5-azaC, and one with a ramet of P. stratiotes whose mother was grown in the high nutrients level and not treated with 5-azaC and a ramet of E. crassipes whose mother was grown in the low nutrient level and treated with 5-azaC) were also dead. Statistical analyses were carried out with SPSS 22.0 (IBM Corp., Armonk, NY, United States).
Results
Effects of nutrients and DNA demethylation on performance in the first experiment
Compared with the low nutrient level, the high nutrient level significantly increased both biomass (by 30 and 14%) and number of ramets (by 70 and 126%) of P. stratiotes and E. crassipes (Table 1 and Figures 1A,B,D,E). However, the final mean size of the ramets, as measured by biomass per ramet, was significantly higher in the low than in the high nutrient level for both species (Table 1): biomass per ramet of P. stratiotes was about 1.3 times larger under the low than under the high nutrient level (Figure 1C), and biomass per ramet of E. crassipes was about 2.0 times larger (Figure 1F). Compared to the control (no 5-azaC), the application of 5-azaC significantly reduced total biomass (by 32%) and biomass per ramet (by 37%) of P. stratiotes, but had no effect on ramet production (Table 1A and Figures 1A–C). The application of 5-azaC did not significantly affect total biomass, number of ramets and biomass per ramet of E. crassipes (Table 1B and Figures 1D–F).
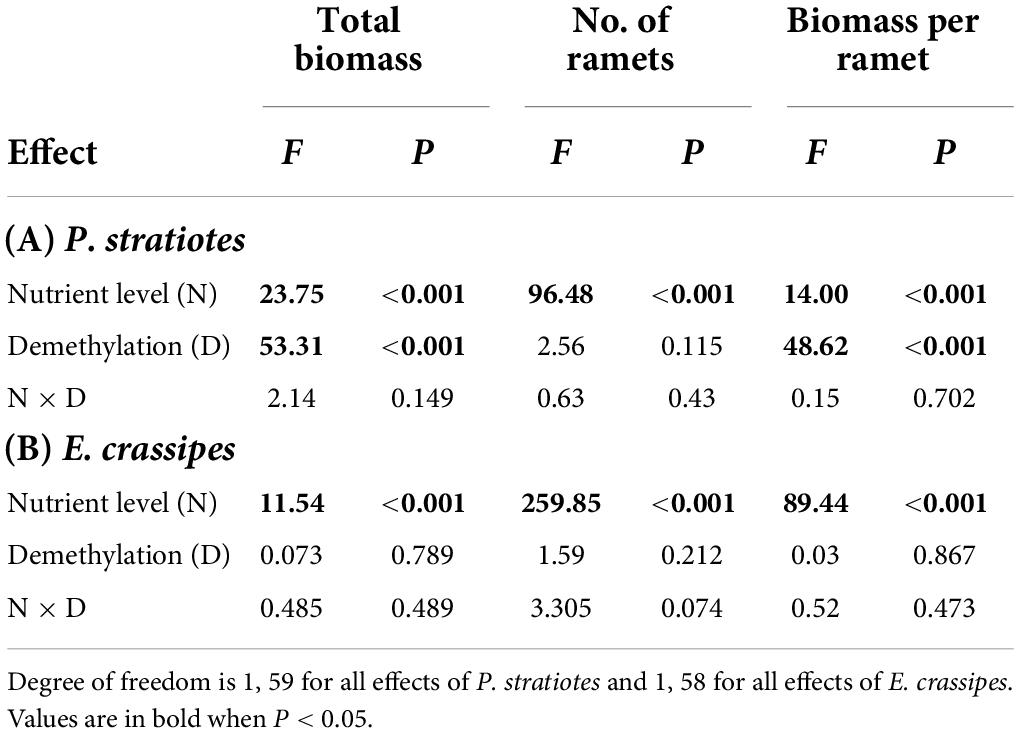
Table 1. ANOVA results for effects of nutrient level and DNA demethylation on the growth of (A) Pistia stratiotes and (B) Eichhornia crassipes in the first experiment.
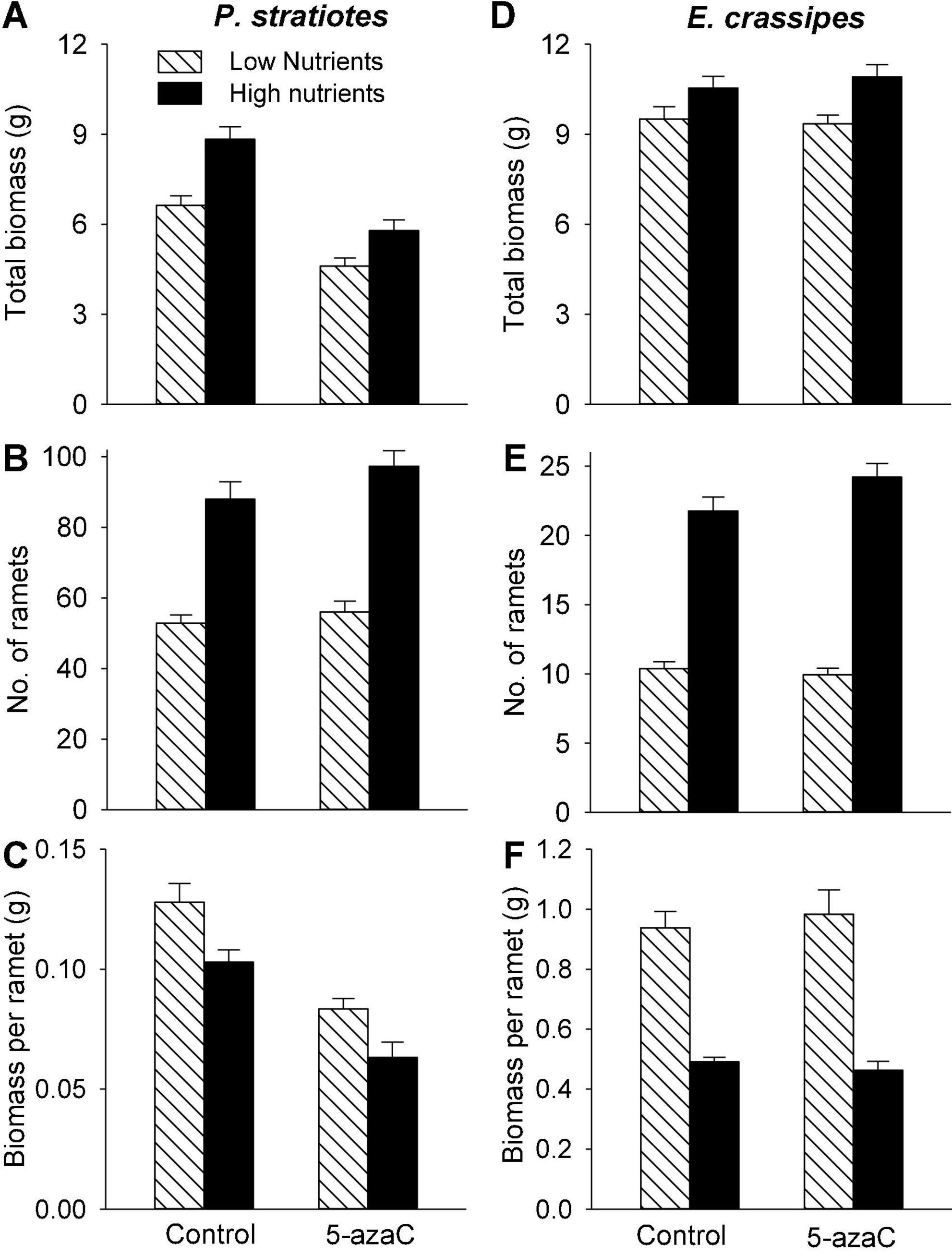
Figure 1. Effects of nutrient level (high and low) and DNA demethylation (control and 5-azaC) on the growth of offspring ramets of (A–C) Pistia stratiotes and (D–F) Eichhornia crassipes. Bars show means + SE.
Parental effects on interspecific interactions in the second experiment
The nutrient level of the target’s mother ramet, the nutrient level of competitor’s mother ramet and the demethylation of the competitor’s mother ramet had no significant effect on the competitive response of the target plant of P. stratiotes (Table 2B; Appendix Figure 1). For both E. crassipes and P. stratiotes, the application of the 5-azaC to the mother ramet of the target plant significantly decreased the competitive response of the target (Table 2 and Figures 2, 3A). The competitive response of P. stratiotes became less negative when the competitor’s mother had been grown under the high nutrient level than when it had been grown under the low nutrient level (Table 2A and Figure 3B). We observed a significant interactive effect between the nutrient level of the target’s mother ramet and DNA demethylation of the competitor’s mother ramet and between the nutrient level of the target’s mother ramet and DNA demethylation of the target’s mother ramet on the competitive response of the target plant of P. stratiote (Table 2A). When the competitor’ mother was not treated with 5-azaC, the competitive response of the target plant of P. stratiote was not significantly different from zero if the target’ mother had been grown under the low nutrient level, but was highly significantly negative when it had been grown under the high nutrient level; however, when the target’s mother was treated with 5-azaC, a reverse pattern was observed (Figure 4). When the target’ mother was not treated with 5-azaC, the competitive response of the target plant of P. stratiote was significantly negative if the target’ mother had been grown under the low nutrient level, but was close to zero when it had been grown under the high nutrient level; however, when the target’s mother was treated with 5-azaC, a reverse pattern was observed (Figure 5).
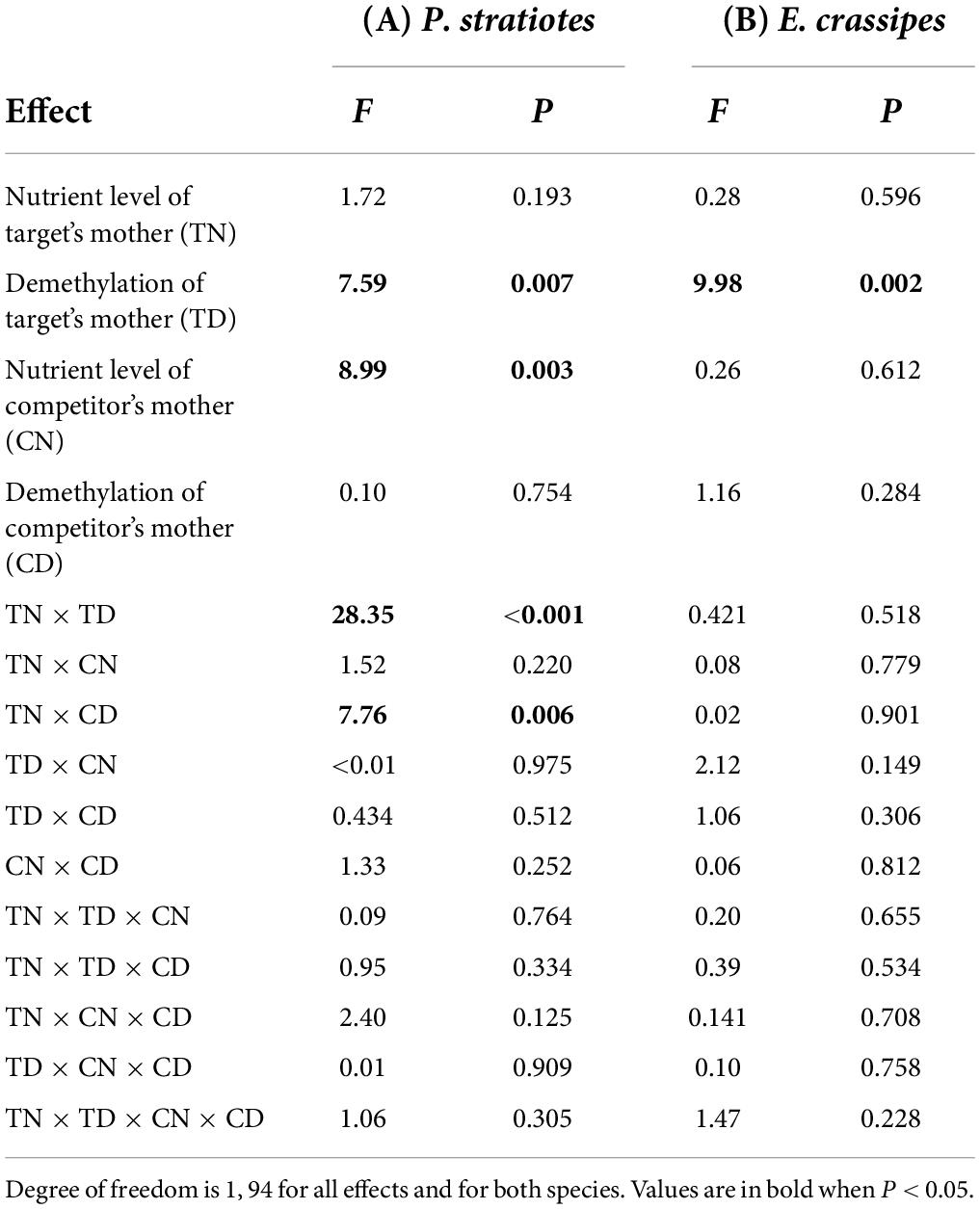
Table 2. Effects of nutrient level and DNA demethylation of the target‘s mother and the competitor’s mother on the interspecific competitive response (LnRR) of the target plant of (A) Pistia stratiotes and (B) Eichhornia crassipes in the second experiment.
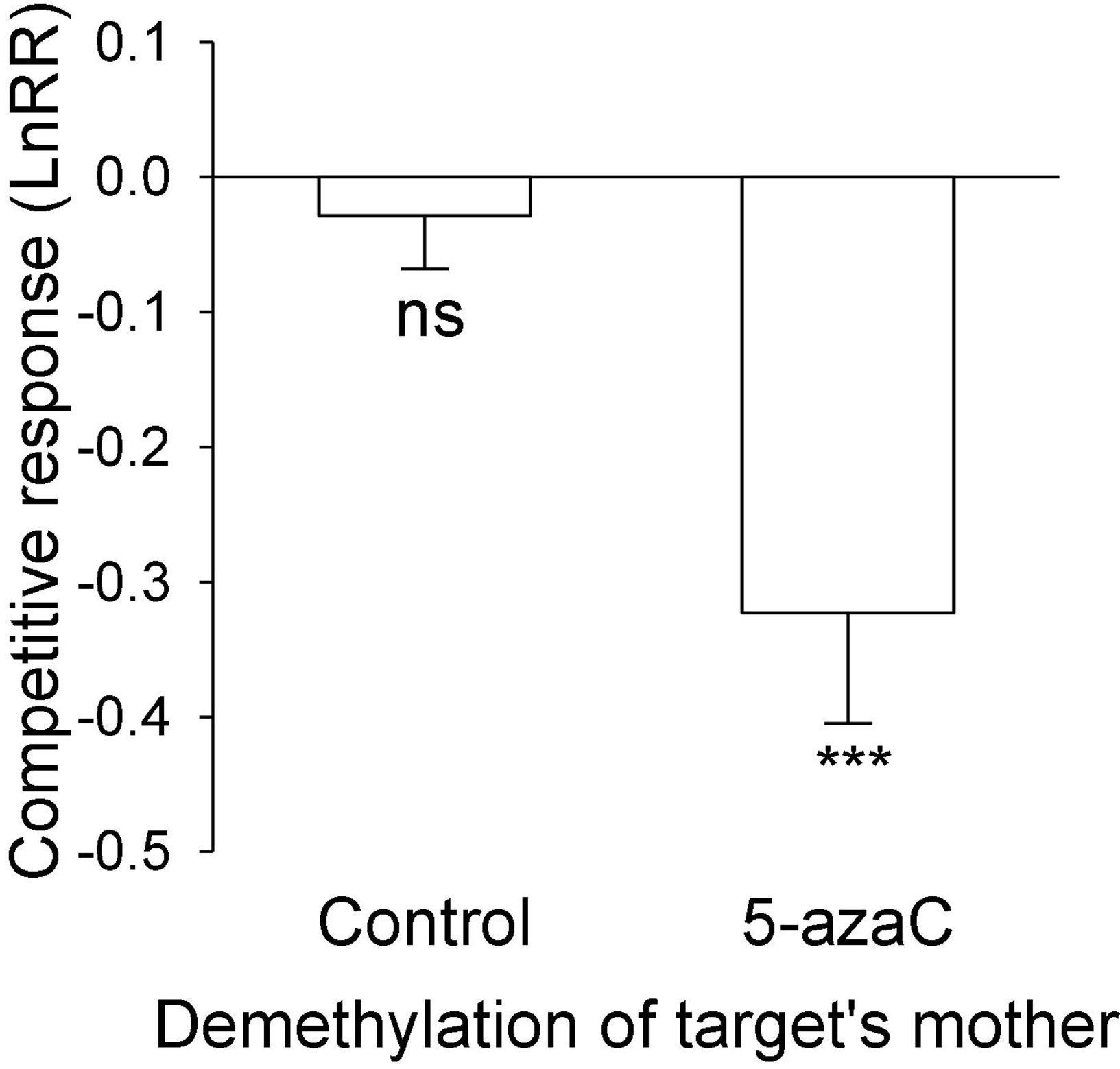
Figure 2. The effect of DNA demethylation of the target’s mother on the competitive response of the target plant of Eichhornia crassipes. Bars show means + SE. Symbols (***P < 0.001) indicate significant difference from zero (by one-sample t-test).
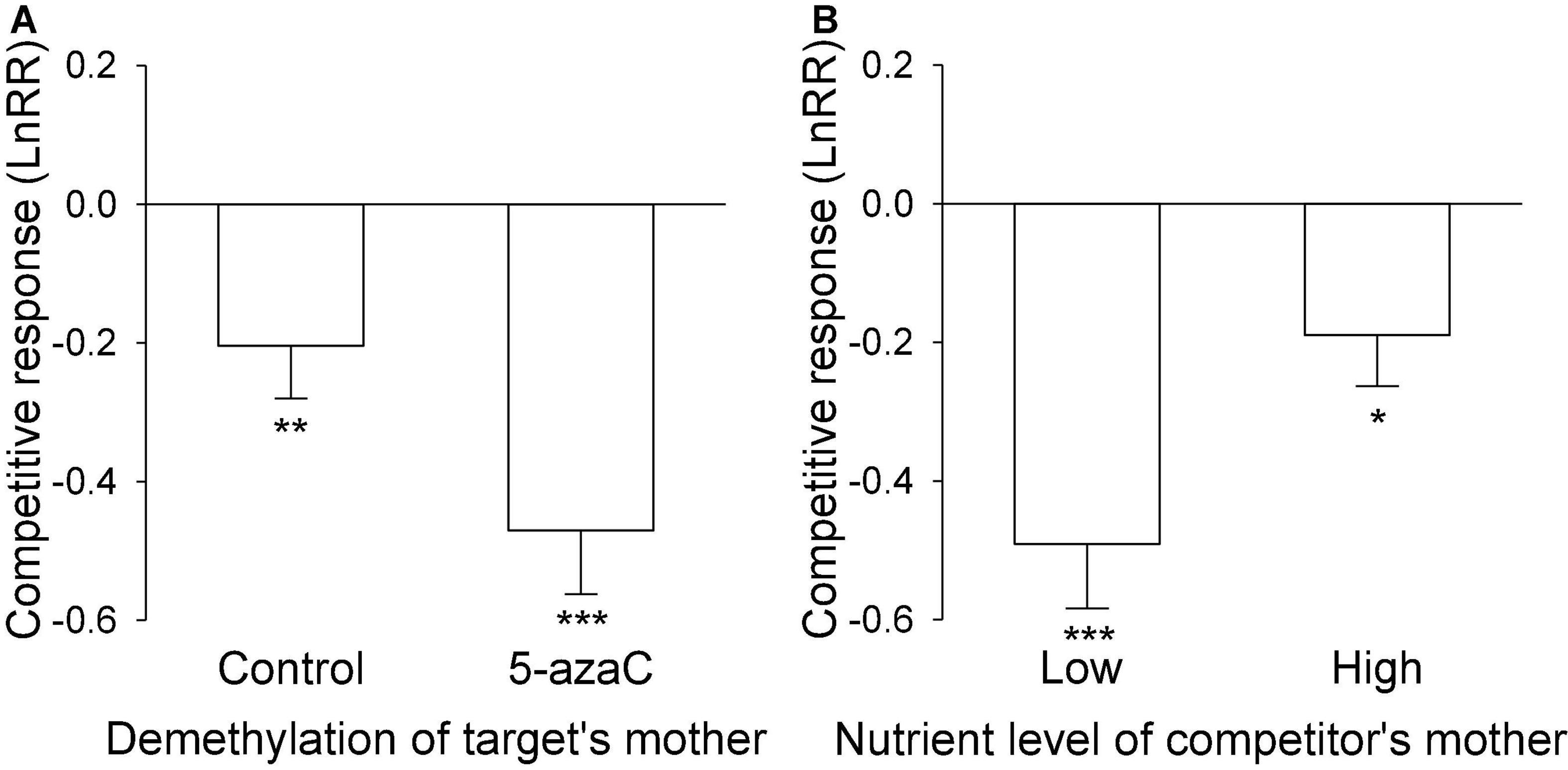
Figure 3. (A) The effect of DNA demethylation of the target’s mother and (B) the effect of the nutrient level of the competitor’s mother on the competitive response of the target plant of Pistia stratiotes. Bars show means + SE. Symbols (***P < 0.001; **P < 0.01; *P < 0.05) indicate significant difference from zero (by one-sample t-test).
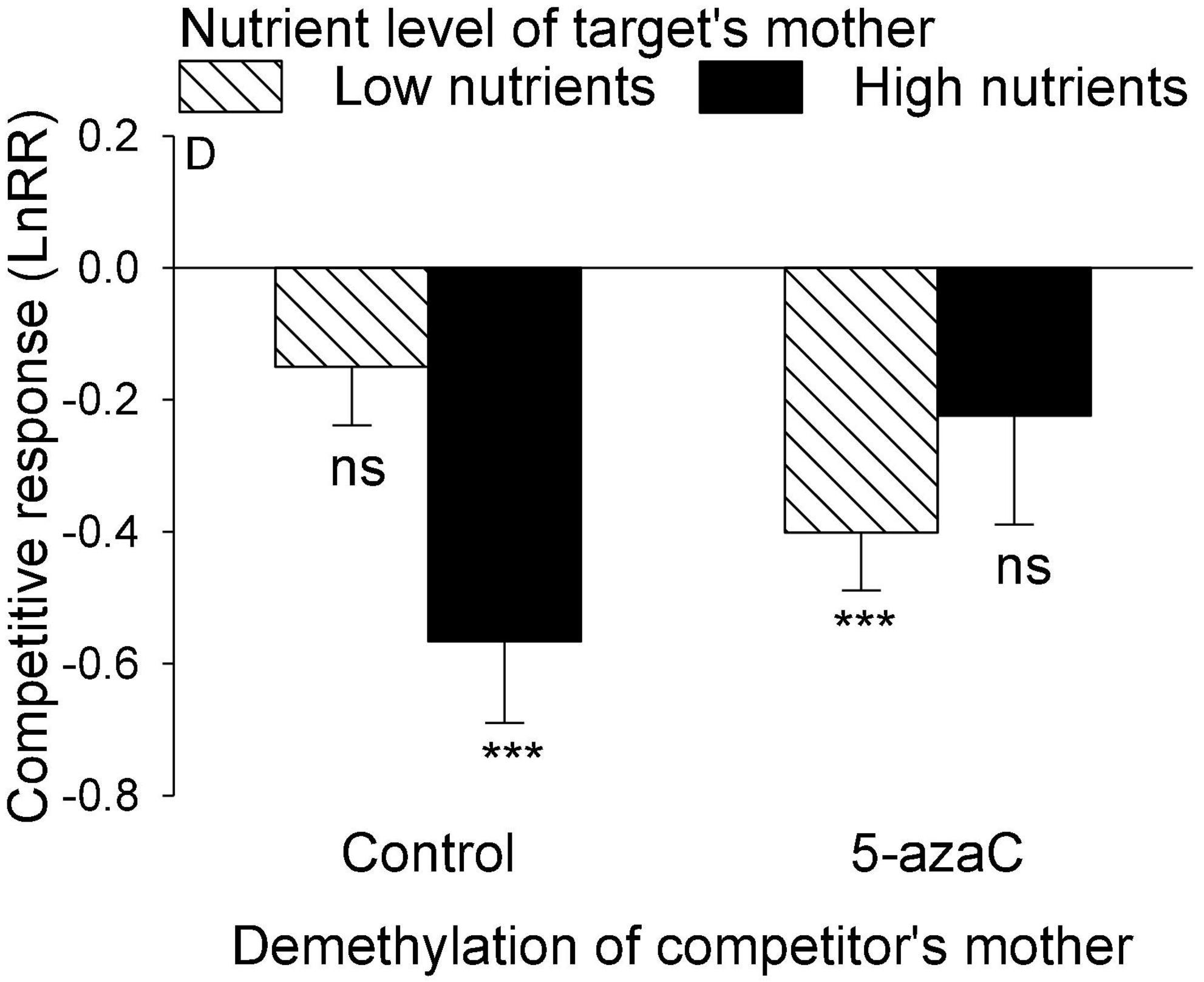
Figure 4. The interactive effect between DNA demethylation of the competitor’s mother and the nutrient level of the target’s mother on the competitive response of the target plant of Pistia stratiotes. Bars show means + SE. Symbols (***P < 0.001) indicate significant difference from zero (by one-sample t-test).
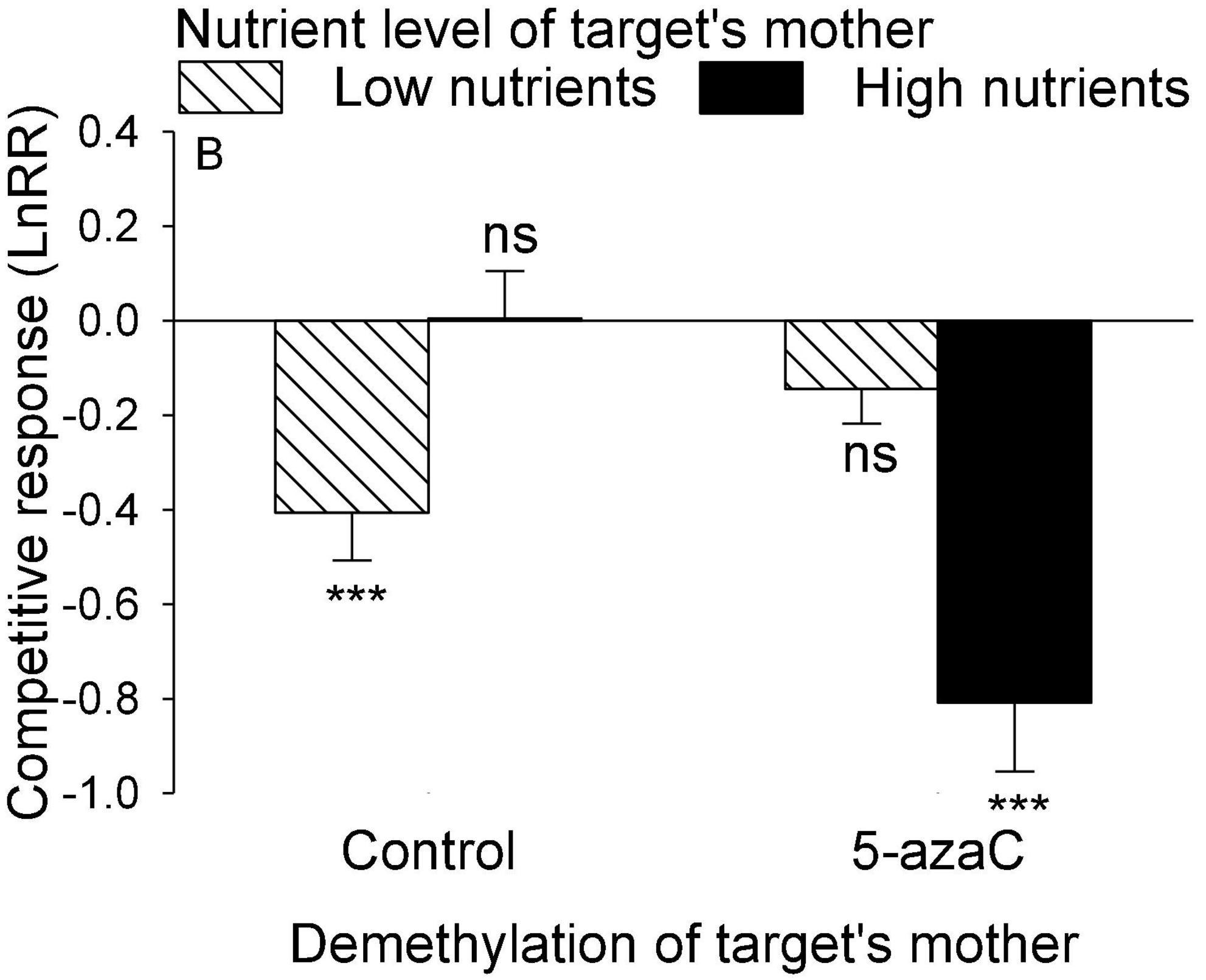
Figure 5. The interactive effect between nutrient level and DNA demethylation of the target’s mother on the competitive response of the target plant of Pistia stratiotes. Bars show means + SE. Symbols (***P < 0.001) indicate significant difference from zero (by one-sample t-test).
Discussion
Effects of nutrient availability and 5-azaC on offspring growth
As expected, high nutrient availability of the mother ramets promoted total biomass and number of offspring ramets of both P. stratiotes and E. crassipes (Figure 1), which is consistent with previous findings on other aquatic plants (Zhao, 2006; Jampeetong and Brix, 2008; Zhang et al., 2020). This effect is related to physiological integration as mother ramets growing under higher nutrient availability can transfer more resources (photo-assimilates and nutrients) to newly produced offspring ramets that remain connected to them (Slade and Hutchings, 1987a,b; Wang et al., 2017, 2021; Portela et al., 2021). However, the magnitude of this growth promotion was stronger for number of ramets than for total biomass so that biomass per offspring ramet was significantly smaller under high than under low nutrient availability (Figures 1C,F). This result suggests that the favorable nutrient environment experienced by the mother ramets can increase the fitness of the whole offspring population, but may not necessarily promote the competitive ability of individual offspring in their subsequent growth and development.
We observed that application DNA demethylation agent 5-azaC decreased biomass per offspring ramet of P. stratiotes as it decreased total biomass of all offspring ramets but had no effect on their number. This finding indicates that, in addition to DNA demethylation, 5-azaC was toxic to the growth of P. stratiotes. A detrimental effect of 5-azaC on plant growth and development has also been reported in other species (Fieldes, 1994; Finnegan et al., 1996; Bossdorf et al., 2010), which should be considered when parental effects are subsequently considered. However, 5-azaC application had no effect on the growth of offspring ramets of E. crassipes, suggesting no side effect of 5-azaC on the growth of E. crassipes.
Parental nutrient effects on interspecific interactions
Parental effects were found to frequently influence fitness measures of offspring (Latzel and Klimešová, 2010; González et al., 2016; Dong et al., 2019a), and thus may further influence their competitive ability when they grow with heterospecific neighbors (Miao et al., 1991a; Baker et al., 2019). For examples, parental effects improved the growth and thus competitive ability of Polygonum persicaria offspring in shade conditions (Baker et al., 2019). If two co-existing species both benefit from parental effects but the degree of the benefit is different (Li et al., 2021), or if parental effects can influence fitness measures of one species but had no impact on the other (Miao et al., 1991a), then parental effects can modify the competitive interactions of these co-occurring species. We found no clonal parental nutrient effects on the competitive ability of E. crassipes (Table 2B), but significant clonal parental nutrient effects on that of P. stratiotes (Table 2A and Figures 3B, 4, 5). Consequently, clonal parental nutrient effects significantly influenced the competitive interaction between E. crassipes and P. stratiotes. In a previous study, Miao et al. (1991a) reported signifiant sexual parental nutrient effects on the competitive ability of two Plantago species, showing the competitive ability of Plantago major depended on the period of parental nutrient pulse, while that of Plantago rugelii depended on maternal background nutrient levels. If plant communities are highly niche-differentiated, then parental effects may facilitate species coexistence (Li et al., 2021).
We observed significant parental nutrient effects of the competitor E. crassipes on the competitive ability of the target P. stratiotes (Figure 3B). The competitive ability of the offspring ramet of P. stratiotes became much lower when it competed with the offspring ramet of E. crassipes produced by the mother ramet growing under low than under high nutrient availability. It is well-known that the size of a plant is commonly positively related to its competitive ability (Goldberg and Fleetwood, 1987; Gaudet and Keddy, 1988). Because the mother ramet of E. crassipes produced much larger offspring ramets under low than under high nutrient availability (Figure 1F), the competitive ability of the offspring ramets originated from the mother ramet growing under low nutrients would show a much greater competitive ability when competing with P. stratiotes. Consequently, the parental low nutrient effect of E. crassipes greatly reduced the competitive ability of the offspring ramet of P. stratiotes. As the size of the offspring (biomass per offspring ramet) is closely related to their ability of resource provisioning (Dong et al., 2019a), the parental nutrient effect of E. crassipes on the competitive ability of P. stratiotes was likely due to resource provisioning, as reported before (Wulff and Roach, 1987; Herman and Sultan, 2011; Zas et al., 2013). However, this parental nutrient effect of E. crassipes was not affected by the application of 5-azaC, suggesting that DNA methylation played little role during this process (Griffin et al., 2016).
We also observed a parental nutrient effect of P. stratiotes on the competitive ability of its offspring ramet, but such an effect varied depending on the DNA methylation status of the mother ramet of the competitor E. crassipes (Figure 4). Without application of 5-azaC to the mother ramet of E. crassipes, the competitive ability of the offspring ramet of P. stratiotes was much smaller when its mother ramet had been grown under the high than under the low nutrient level. This parental nutrient effect can also be explained by resource provisioning (Wulff and Roach, 1987; Herman and Sultan, 2011; Germain et al., 2013; Zas et al., 2013) as the size of the offspring ramet of P. stratiotes was significantly smaller when its mother ramet had been grown under the high than under the low nutrient level (Figure 1C). However, with application of 5-azaC to the mother ramet of E. crassipes, the nutrient level of the mother ramet of P. stratiotes had no significant effect on the competitive ability of its offspring ramet, suggesting that DNA demethylation of the competitor’s mother can alter the parental nutrient effect of the target plant. The underlying mechanism for this observation is not clear and deserves further studies.
The parental nutrient effect of P. stratiotes on the competitive ability of its offspring ramet also varied depending on DNA demethylation status of mother ramet (Figure 5). Without application of 5-azaC to the mother ramet of P. stratiotes, competition from E. crassipes resulted in a significantly negative competitive response of the offspring ramet of P. stratiotes when its mother had been grown under the low nutrient level, but had no significant negative effect on the offspring ramet of P. stratiotes when its mother ramet had been grown under the high nutrient level. This result cannot be explained by resource provisioning (Cheplick, 1997) as the size of the offspring ramet of P. stratiotes was significantly smaller when its mother ramet had been grown under the high than under the low nutrient level (Figure 1C). When the mother ramet of P. stratiotes was treated with 5-azaC, an opposite pattern was observed (Figure 5). These results suggest that epigenetic mechanisms such as DNA methylation must have played a role in mediating the parental nutrient effect, as reported also in other studies (Latzel and Klimešová, 2010; Verhoeven and Preite, 2014; González et al., 2016).
Parental 5-azaC effects on interspecific interactions
Average across all other treatments, application of 5-azaC to the mother ramet of P. stratiotes markedly decreased the competitive ability of its offspring ramets when competing with E. crassipes (Figure 3A), suggesting a parental effect of 5-azaC application. This parental effect was likely caused by resource provisioning as the size of the offspring ramet was significantly reduced when the mother ramet was treated with 5-azaC (Figure 1C) so that the offspring contained less energy for their subsequent growth and competition (Goldberg and Fleetwood, 1987; Gaudet and Keddy, 1988).
Surprisingly, application of 5-azaC to the mother ramet of E. crassipes greatly decreased the competitive ability of its offspring ramet (Figure 2), despite the fact that it did not influence the size of the offspring (Figure 1F). Thus, resource provisioning cannot explain this clonal parental effect and DNA methylation might have played a role.
Conclusions
This appears the first study testing the role of clonal parental effects in shaping interspecific competition between plants. Our findings suggest that clonal parental nutrient effects can regulate interspecific competition between P. stratiotes and E. crassipes by altering the competitive ability of P. stratiotes in different ways. Both resource provisioning and epigenetic mechanisms can be involved in these clonal parental effects. By regulating interspecific competition, clonal parental effects may further influence species coexistence, community structure, and ecosystem functioning.
Data availability statement
The raw data supporting the conclusions of this article will be made available by the authors, without undue reservation.
Author contributions
W-HY conducted the experiment, analyzed the data, and drafted the manuscript. L-MZ assisted with data analysis and contributed substantially to manuscript revision. F-LL and M-HL contributed substantially to manuscript revision. F-HY designed the experiment and contributed substantially to manuscript revision. All authors contributed to the article and approved the submitted version.
Funding
This study was supported by the National Natural Science Foundation of China (grants: 32071527, 3210126, and 32071525) and National College Students’ Innovation and Entrepreneurship Training Program (202010350031).
Conflict of interest
The authors declare that the research was conducted in the absence of any commercial or financial relationships that could be construed as a potential conflict of interest.
Publisher’s note
All claims expressed in this article are solely those of the authors and do not necessarily represent those of their affiliated organizations, or those of the publisher, the editors and the reviewers. Any product that may be evaluated in this article, or claim that may be made by its manufacturer, is not guaranteed or endorsed by the publisher.
Acknowledgments
We thank Jia-Fan Zhang, Chen-Yan Qiu, Dan-Hao Wang, Si-Mei Yao, and Yu Jin for assistance with harvesting.
Supplementary material
The Supplementary Material for this article can be found online at: https://www.frontiersin.org/articles/10.3389/fpls.2022.924001/full#supplementary-material
References
Adomako, M. O., Alpert, P., Du, D. -L., and Yu, F.-H. (2020). Effects of clonal integration, nutrients and cadmium on growth of the aquatic macrophyte Pistia stratiotes. J. Plant Ecol. 13, 765–772. doi: 10.1093/jpe/rtaa068
Adomako, M. O., Alpert, P., Du, D.-L., and Yu, F.-H. (2021). Effects of fragmentation of clones compound over vegetative generations in the floating plant Pistia stratiotes. Ann. Bot. 127, 123–133. doi: 10.1093/aob/mcaa150
Adomako, M. O., Zhang, Q., and Yu, F.-H. (2022). Genotypic differences in response to different patterns of clonal fragmentation in the aquatic macrophyte Pistia stratiotes. J. Plant Ecol. doi: 10.1093/jpe/rtac035
Agrawal, A. A. (2002). Herbivory and maternal effects: mechanisms and consequences of transgenerational induced plant resistance. Ecology 83, 3408–3415. doi: 10.2307/3072089
Badyaev, A. V., and Uller, T. (2009). Parental effects in ecology and evolution: mechanisms, processes and implications. Philos. Trans. R. Soc. Lond. B Biol. Sci. 364, 1169–1177. doi: 10.1098/rstb.2008.0302
Baker, B. H., Sultan, S. E., Lopez-Ichikawa, M., and Waterman, R. (2019). Transgenerational effects of parental light environment on progeny competitive performance and lifetime fitness. Philos. Trans. R. Soc. Lond. B Biol. Sci. 374:20180182. doi: 10.1098/rstb.2018.0182
Bossdorf, O., Arcuri, D., Richards, C. L., and Pigliucci, M. (2010). Experimental alteration of DNA methylation affects the phenotypic plasticity of ecologically relevant traits in Arabidopsis thaliana. Evol. Ecol. 24, 541–553. doi: 10.1007/s10682-010-9372-7
Bossdorf, O., Shuja, Z., and Banta, J. A. (2009). Genotype and maternal environment affect belowground interactions between Arabidopsis thaliana and its competitors. Oikos 118, 1541–1551. doi: 10.1111/j.1600-0706.2009.17559.x
Castro, B. M., Moriuchi, K. S., Friesen, M. L., Badri, M., Nuzhdin, S. V., Strauss, S. Y., et al. (2013). Parental environments and interactions with conspecifics alter salinity tolerance of offspring in the annual Medicago truncatula. J. Ecol. 101, 1281–1287. doi: 10.1111/1365-2745.12125
Cheplick, G. P. (1997). Responses to severe competitive stress in a clonal plant: differences between genotypes. Oikos 79, 581–591. doi: 10.2307/3546902
Dodd, R. S., and Douhovnikoff, V. (2016). Adjusting to global change through clonal growth and epigenetic variation. Front. Ecol. Evol. 4:86. doi: 10.3389/fevo.2016.00086
Dong, B.-C., Alpert, P., and Yu, F.-H. (2019a). Transgenerational effects of herbivory and soil nutrients transmitted via vegetative reproduction in the clonal plant Alternanthera philoxeroides. Perspect. Plant Ecol. Evol. Syst. 41:125498. doi: 10.1016/j.ppees.2019.125498
Dong, B.-C., Meng, J., and Yu, F.-H. (2019b). Effects of parental light environment on growth and morphological responses of clonal offspring. Plant Biol. 21, 1083–1089. doi: 10.1111/plb.13001
Dong, B.-C., Fu, T., Luo, F.-L., and Yu, F.-H. (2017). Herbivory-induced maternal effects on growth and defense traits in the clonal species Alternanthera philoxeroides. Sci. Total Environ. 605, 114–123. doi: 10.1016/j.scitotenv.2017.06.141
Dong, B.-C., van Kleunen, M., and Yu, F.-H. (2018a). Context-dependent parental effects on clonal offspring performance. Front. Plant Sci. 9:1824. doi: 10.3389/fpls.2018.01824
Dong, B.-C., Wang, M.-Z., Liu, R.-H., Luo, F.-L., Li, H.-L., and Yu, F.-H. (2018b). Direct and legacy effects of herbivory on growth and physiology of a clonal plant. Biol. Invasions 20, 3631–3645. doi: 10.1007/s10530-018-1801-5
Du, F., Liang, Z.-S., and Hu, L.-J. (2004). A review on plant competition. Chin. J. Ecol. 23, 157–163. doi: 10.3321/j.issn:1000-4890.2004.04.032
Evans, J. (2013). Pistia stratiotes L. in the Florida Peninsula: biogeographic evidence and conservation implications of native tenure for an ‘invasive’ aquatic plant. Conserv. Soc. 11, 233–246. doi: 10.4103/0972-4923.121026
Fieldes, M. A. (1994). Heritable effects of 5-azacytidine treatments on the growth and development of flax (Linum usitatissimum) genotrophs and genotypes. Genome 37, 1–11. doi: 10.1139/g94-001
Finnegan, E. J., Peacock, W. J., and Dennis, E. S. (1996). Reduced DNA methylation in Arabidopsis thaliana results in abnormal plant development. Proc. Natl. Acad. Sci. U. S. A. 93, 8449–8454. doi: 10.1073/pnas.93.16.8449
Galal, T. M., Dakhil, M. A., Hassan, L. M., and Eid, E. M. (2019). Population dynamics of Pistia stratiotes L. Rend. Lincei Sci. Fis. Nat. 30, 367–378. doi: 10.1007/s12210-019-00800-0
Galloway, L. F. (2005). Maternal effects provide phenotypic adaptation to local environmental conditions. New Phytol. 166, 93–99. doi: 10.1111/j.1469-8137.2004.01314.x
Gaudet, C. L., and Keddy, P. A. (1988). A comparative approach to predicting competitive ability from plant traits. Nature 334:242. doi: 10.1038/334242a0
Germain, R. M., Caruso, C. M., and Maherali, H. (2013). Mechanisms and consequences of water stress–induced parental effects in an invasive annual grass. Int. J. Plant Sci. 174, 886–895. doi: 10.1086/670691
Goldberg, D. E., and Fleetwood, L. (1987). Competitive effect and response in four annual plants. J. Ecol. 75, 1131–1143. doi: 10.2307/2260318
Goldberg, D. E., Rajaniemi, T., Gurevitch, J., and Stewart-Oaten, A. (1999). Empirical approaches to quantifying interaction intensity: competition and facilitation along productivity gradients. Ecology 80, 1118–1131. doi: 10.2307/177059
González, A. P. R., Chrtek, J., Dobrev, P. I., Dumalasová, V., Fehrer, J., Mráz, P., et al. (2016). Stress-induced memory alters growth of clonal offspring of white clover (Trifolium repens). Am. J. Bot. 103, 1567–1574. doi: 10.3732/ajb.1500526
González, A. P. R., Dumalasová, V., Rosenthal, J., Skuhrovec, J., and Latzel, V. (2017). The role of transgenerational effects in adaptation of clonal offspring of white clover (Trifolium repens) to drought and herbivory. Evol. Ecol. 31, 345–361. doi: 10.1007/s10682-016-9844-5
González, A. P. R., Preite, V., Verhoeven, K. J., and Latzel, V. (2018). Transgenerational effects and epigenetic memory in the clonal plant Trifolium repens. Front. Plant Sci. 9:1677. doi: 10.3389/fpls.2018.01677
Grace, J. B., and Tilman, D. (1990). Perspectives on plant competition. Science 249:1054. doi: 10.1126/science.249.4972.1054
Griffin, P. T., Niederhuth, C. E., and Schmitz, R. J. (2016). A comparative analysis of 5-azacytidine and zebularine-induced DNA demethylation. G3 6, 2773–2780. doi: 10.1534/g3.116.030262
Hedges, L. V., Gurevitch, J., and Curtis, P. S. (1999). The meta-analysis of response ratios in experimental ecology. Ecology 80, 1150–1156. doi: 10.2307/177062
Herman, J. J., and Sultan, S. E. (2011). Adaptive transgenerational plasticity in plants: case studies, mechanisms, and implications for natural populations. Front. Plant Sci. 2:102. doi: 10.3389/fpls.2011.00102
Hussner, A., Heidbuechel, P., and Heiligtag, S. (2014). Vegetative overwintering and viable seed production explain the establishment of invasive Pistia stratiotes in the thermally abnormal Erft River (North Rhine-Westphalia, Germany). Aquat. Bot. 119, 28–32. doi: 10.1016/j.aquabot.2014.06.011
Jablonka, E., and Raz, G. (2009). Transgenerational epigenetic inheritance: prevalence, mechanisms, and implications for the study of heredity and evolution. Q. Rev. Biol. 84, 131–176. doi: 10.1086/598822
Jampeetong, A., and Brix, H. (2008). Nitrogen nutrition of Salvinia natans : effects of inorganic nitrogen form on growth, morphology, nitrate reductase activity and uptake kinetics of ammonium and nitrate. Aquat. Bot. 90, 67–73. doi: 10.1016/j.aquabot.2008.06.005
Kraft, N. J., Godoy, O., and Levine, J. M. (2015). Plant functional traits and the multidimensional nature of species coexistence. Proc. Natl. Acad. Sci. U. S. A. 112, 797–802. doi: 10.1073/pnas.1413650112
Latzel, V., Janeèek, Š, Doležal, J., Klimešová, J., and Bossdorf, O. (2014). Adaptive transgenerational plasticity in the perennial Plantago lanceolata. Oikos 123, 41–46. doi: 10.1111/j.1600-0706.2013.00537.x
Latzel, V., and Klimešová, J. (2010). Transgenerational plasticity in clonal plants. Evol. Ecol. 24, 1537–1543. doi: 10.1007/s10682-010-9385-2
Li, Y., Hou, L.-Y., Yang, L.-Y., and Yue, M. (2021). Transgenerational effect alters the interspecific competition between two dominant species in a temperate steppe. Ecol. Evol. 11, 1175–1186. doi: 10.1002/ECE3.7066
Luo, F.-L., Wang, M.-Z., Dong, B.-C., Chen, Y.-H., and Yu, F.-H. (2022). Clonal and sexual parental effects and their mechanisms. Acta Ecol. Sin. 42, 4313–4323. doi: 10.5846/stxb202107211968
Miao, S. L., Bazzaz, F. A., and Primack, R. B. (1991a). Effects of maternal nutrient pulse on reproduction of two Colonizing plantago species. Ecology 72, 586–596. doi: 10.2307/2937198
Miao, S. L., Bazzaz, F. A., and Primack, R. B. (1991b). Persistence of maternal nutrient effects in plantago major: the third generation. Ecology 72, 1634–1642. doi: 10.2307/1940963
Odjegba, V. J., and Fasidi, I. O. (2004). Accumulation of trace elements by Pistia stratiotes : implications for phytoremediation. Ecotoxicology 13, 637–646. doi: 10.1007/s10646-003-4424-1
Pettet, A., and Pettet, S. J. (1970). Biological control of Pistia stratiotes L. in Western State. Nigeria. Nature 226, 282. doi: 10.1038/226282a0
Portela, R., Barreiro, R., and Roiloa, S. R. (2021). Effects of resource sharing directionality on physiologically integrated clones of the invasive Carpobrotus edulis. J. Plant Ecol. 14, 884–895. doi: 10.1093/jpe/rtab040
Portela, R., Dong, B.-C., Yu, F.-H., Barreiro, R., Roiloa, S. R., and Silva Matos, D. M. (2020). Trans-generational effects in the clonal invader Alternanthera philoxeroides. J. Plant Ecol. 13, 122–129. doi: 10.1093/jpe/rtz043
Richards, C. L., Alonso, C., Becker, C., Bossdorf, O., Bucher, E., Colomé-Tatché, M., et al. (2017). Ecological plant epigenetics: evidence from model and non-model species, and the way forward. Ecol. Lett. 20, 1576–1590. doi: 10.1111/ele.12858
Rico, L., Ogaya, R., Barbeta, A., and Peñuelas, J. (2014). Changes in DNA methylation fingerprint of Quercus ilex trees in response to experimental field drought simulating projected climate change. Plant Biol. 16, 419–427. doi: 10.1111/plb.12049
Schulz, B., Eckstein, R. L., and Durka, W. (2014). Epigenetic variation reflects dynamic habitat conditions in a rare floodplain herb. Mol. Ecol. 23, 3523–3537. doi: 10.1111/mec.12835
Slade, A. J., and Hutchings, M. J. (1987a). An analysis of the costs and benefits of physiological integration between ramets in the clonal perennial herb Glechoma hederacea. Oecologia 73, 425–431. doi: 10.1007/BF00385260
Slade, A. J., and Hutchings, M. J. (1987b). Clonal integration and plasticity in foraging behaviour in Glechoma hederacea. J. Ecol. 75, 1023–1036. doi: 10.2307/2260311
Valladares, F., Bastias, C. C., Godoy, O., Granda, E., and Escudero, A. (2015). Species coexistence in a changing world. Front. Plant Sci. 6:866. doi: 10.3389/fpls.2015.00866
Verhoeven, K. J., and Preite, V. (2014). Epigenetic variation in asexually reproducing organisms. Evolution 68, 644–655. doi: 10.1111/evo.12320
Wang, J.-Y., Xu, T.-T., Wang, Y., Li, G.-Y., Abdullah, I., Zhong, Z.-W., et al. (2021). A meta-analysis of effects of physiological integration in clonal plants under homogeneous vs. heterogeneous environments. Funct. Ecol. 35, 578–589. doi: 10.1111/1365-2435.13732
Wang, P., Alpert, P., and Yu, F.-H. (2016). Clonal integration increases relative competitive ability in an invasive aquatic plant. Am. J. Bot. 103, 2079–2086. doi: 10.3732/ajb.1600160
Wang, P., Xu, Y.-S., Dong, B.-C., Xue, W., and Yu, F.-H. (2014). Effects of clonal fragmentation on intraspecific competition of a stoloniferous floating plant. Plant Biol. 16, 1121–1126. doi: 10.1111/plb.12170
Wang, Y.-J., Müller-Schärer, H., van Kleunen, M., Cai, A.-M., Zhang, P., Yan, R., et al. (2017). Invasive alien plants benefit more from clonal integration in heterogeneous environments than natives. New Phytol. 216, 1072–1078. doi: 10.1111/nph.14820
Wulff, R. D., and Roach, D. A. (1987). Maternal effects in plants. Annu. Rev. Ecol. Syst. 18, 209–235. doi: 10.1146/annurev.es.18.110187.001233
Zas, R., Cendán, C., and Sampedro, L. (2013). Mediation of seed provisioning in the transmission of environmental maternal effects in Maritime pine (Pinus pinaster Aiton). Heredity 111, 248–255. doi: 10.1038/hdy.2013.44
Zhang, L.-M., Jin, Y., Yao, S.-M., Lei, N.-F., Chen, J.-S., Zhang, Q., et al. (2020). Growth and morphological responses of duckweed to clonal fragmentation, nutrient availability, and population density. Front. Plant Sci. 11:618. doi: 10.3389/fpls.2020.00618
Zhang, W., Jin, X., Liu, D., Lang, C., and Shan, B. (2017). Temporal and spatial variation of nitrogen and phosphorus and eutrophication assessment for a typical arid river — Fuyang River in northern China. J. Environ. Sci. 55, 41–48. doi: 10.1016/j.jes.2016.07.004
Zhao, Y.-Q. (2006). Effects of nutrient levels on growth characteristics and competitive ability of water hyacinth (Eichhornia crassipes), an aquatic invasive plant. Biodiversity Sci. 14, 159–159. doi: 10.1360/biodiv.050243
Keywords: clonal plants, DNA demethylation, epigenetic inheritance, maternal effect, nutrients, transgenerational plasticity
Citation: Yu W-H, Zhang L-M, Luo F-L, Yu F-H and Li M-H (2022) Roles of clonal parental effects in regulating interspecific competition between two floating plants. Front. Plant Sci. 13:924001. doi: 10.3389/fpls.2022.924001
Received: 20 April 2022; Accepted: 30 June 2022;
Published: 22 July 2022.
Edited by:
Xiaohua Jin, Institute of Botany (CAS), ChinaReviewed by:
Antonio Pompeiano, Mendel University in Brno, CzechiaChengcang Ma, Tianjin Normal University, China
Copyright © 2022 Yu, Zhang, Luo, Yu and Li. This is an open-access article distributed under the terms of the Creative Commons Attribution License (CC BY). The use, distribution or reproduction in other forums is permitted, provided the original author(s) and the copyright owner(s) are credited and that the original publication in this journal is cited, in accordance with accepted academic practice. No use, distribution or reproduction is permitted which does not comply with these terms.
*Correspondence: Fei-Hai Yu, feihaiyu@126.com
†These authors have contributed equally to this work